- 1State Key Laboratory of Southwestern Chinese Medicine Resources, Chengdu University of Traditional Chinese Medicine, Chengdu, China
- 2School of Ethnic Medicine, Chengdu University of Traditional Chinese Medicine, Chengdu, China
- 3Waters Technology (Beijing) Co., Ltd., Beijing, China
- 4Innovative Institute of Chinese Medicine and Pharmacy, Chengdu University of Traditional Chinese Medicine, Chengdu, China
Fuzi is a famous toxic traditional herbal medicine, which has long been used for the treatment of various diseases in China and many other Asian countries because of its extraordinary pharmacological activities and high toxicity. Different processing methods to attenuate the toxicity of Fuzi are important for its safe clinical use. In this study, desorption electrospray ionization mass spectrometry imaging (DESI-MSI) with a metabolomics-combined multivariate statistical analysis approach was applied to investigate a series of Aconitum alkaloids and explore potential metabolic markers to understand the differences between raw and processed Fuzi with different steaming time points. Moreover, the selected metabolic markers were visualized by DESI-MSI, and six index alkaloids’ contents were determined through HPLC. The results indicated visible differences among raw and processed Fuzi with different steaming times, and 4.0 h is the proper time for toxicity attenuation and efficacy reservation. A total of 42 metabolic markers were identified to discriminate raw Fuzi and those steamed for 4.0 and 8.0 h, which were clearly visualized in DESI-MSI. The transformation from diester-diterpenoid alkaloids to monoester-diterpenoid alkaloids and then to non-esterified diterpene alkaloids through hydrolysis is the major toxicity attenuation process during steaming. DESI-MSI combined with metabolomics provides an efficient method to visualize the changeable rules and screen the metabolic markers of Aconitum alkaloids during steaming. The wide application of this technique could help identify markers and reveal the possible chemical transition mechanism in the “Paozhi” processes of Fuzi. It also provides an efficient and easy way to quality control and ensures the safety of Fuzi and other toxic traditional Chinese medicine.
1 Introduction
Fuzi, the lateral root of Aconitum carmichaelii Debeaux (Fam. Ranunculaceae), primarily cultivated in Jiangyou and Butuo in Sichuan, China, has been an indispensable herb in traditional Chinese medicine (TCM) for thousands of years (Chinese Pharmacopoeia Commission, 2020). Jiangyou Fuzi and Butuo Fuzi are not only well-known genuine medicinal materials but also important agricultural products of Sichuan Province. The initial record of Fuzi can be dated back to the Han Dynasty in the earliest Chinese material medical classic work “Shennong Bencao Jing,” wherein it is listed in the inferior category because of its highly toxic properties (Singhuber et al., 2009; Zhou et al., 2015). However, Fuzi also has potent efficacy to restore from collapse, reinforce fire and Yang, and dispel cold to relieve pain (Luo et al., 2019; Chinese Pharmacopoeia Commission, 2020). It is usually combined with other herbs in formulations such as Si Ni Tang, Shen Fu Tang, and Fuzi Lizhong Wan to treat cold-damp-type illness (Singhuber et al., 2009). Given its outstanding clinical effects, Fuzi is one of “The Four Pillars” of TCM, together with Ginseng Radix et Rhizoma, Rehmanniae Radix, and Rhei Radix et Rhizoma (Dong et al., 2020). Modern studies have indicated that Fuzi has cardiotonic, anti-inflammatory, analgesic, and anti-tumor activities, which can be utilized to heal heart failure, shock, and hypotension subsequent to acute myocardial infarction, coronary heart disease, rheumatic heart disease, rheumatoid arthritis, tumors, skin wounds, depression, and diarrhea (Li et al., 2012; Zhao et al., 2012; Wu et al., 2018; Chinese Pharmacopoeia Commission, 2020). However, the toxicity of Fuzi coexists with its pharmaceutical activities, and thousands of Fuzi poisoning cases have been reported worldwide (Yang et al., 2018; Lei et al., 2021). Therefore, Fuzi is only used internally after processing, and various processing methods have been established by which the highly toxic alkaloids were transformed to lowly toxic and non-toxic forms through processing. The studies of toxic components and their mechanisms and the proper methods to attenuate the toxicity and reserve efficacy are the primary concern of scholars.
A literature survey has revealed that over 100 alkaloids have been isolated and identified in this herb, including C20-, C19-, and C18-diterpenoid alkaloids and double diterpenoid alkaloids, which are the main source of biological activities and toxicities. Among these alkaloids, the aconitine type, which belongs to C19-diterpenoid alkaloids, can be further divided into four categories: diester-diterpenoid alkaloids (DDAs), monoester-diterpenoid alkaloids (MDAs), non-esterified diterpene alkaloids (NDAs) (Figure 1), and lipo-diterpenoid alkaloids (LDAs). In recent years, numerous studies have demonstrated that the high toxicity of Fuzi is primarily attributed to DDAs (Tong et al., 2013). Based on previous studies, the main toxic effect of Fuzi is that it can affect the central nervous system, heart, and muscle tissues because of the interaction with voltage-dependent sodium channels, modulation of neurotransmitter release and related receptors, promotion of lipid peroxidation, and induction of cell apoptosis in the heart, liver, or other organs (Fu et al., 2006; Zhou et al., 2015; Liu et al., 2017). Therefore, the pretreatment method to reduce such toxic components is highly essential for the safe use of Fuzi.
The appropriate processing methods, which are known as “Paozhi” in Chinese, for TCM are usually necessary to reduce the toxicity while retaining or enhancing its pharmacological activities. Various methods can be used to process Fuzi, such as soaking, saturating, steaming, and decocting. According to the Chinese Pharmacopeia (CP) (2020 Edition), soaking in Danba (halogen solution), then continuous soaking with salt, steaming or decocting, long rinsing, and oven drying are the major processing methods for Fuzi (Chinese Pharmacopoeia Commission, 2020). During these heating procedures, the highly toxic DDAs were hydrolyzed to low toxic MDAs and non-toxic NDAs (Wang et al., 2003; Zhao et al., 2010; Tan et al., 2016). However, toxic Aconitum alkaloids are also responsible for the pharmacological activity and therapeutic efficacy of Fuzi, and efficacy preservation should be observed during toxicity attenuation. Recent research has indicated that DDAs, MDAs, and water-soluble active constituents have a massive loss after soaking in Danba and subsequent rinsing. Moreover, inorganic impurities are easily introduced to Fuzi (Ye et al., 2019). Thus, a Danba-free process becomes a more recognized processing approach for Fuzi. Nevertheless, the visual changes and distribution of DDAs and MDAs during steaming are rarely investigated. Therefore, a suitable methodology to explore these variation characteristics is highly necessary.
At present, the high-performance liquid chromatography (HPLC) and liquid chromatography coupled with mass spectrometry (LC-MS) are commonly used methods for alkaloid analysis of Fuzi (Yue et al., 2009; Xu et al., 2014; Yang et al., 2014; Miao et al., 2019; Sun et al., 2020). However, these techniques require complex preparation procedures, especially extraction from crushed samples. The ambient ionization MS, such as direct analysis in real-time mass spectrometry (DART-MS), was also utilized for direct analysis of Fuzi and other processed herbal medicine. Nevertheless, this method still needs sample pretreatment (Zhu et al., 2012). Therefore, analyzing and recognizing the distribution of specific alkaloids in original Fuzi has become challenging.
Mass spectrometry imaging (MSI) integrates sensitivities and high-throughput screening mass spectrometry with spatial and temporal chemical information and enables the visualized distribution of specific metabolites within non-destructive tissues (Stoeckli et al., 2001; Garza et al., 2018; Parrot et al., 2018). Matrix-assisted laser desorption/ionization (MALDI) and desorption electrospray ionization (DESI) are the commonly used ionization techniques for MSI (Parrot et al., 2018; Xu et al., 2019). Compared with MALDI-MSI, DESI-MSI allows 2D polar biomolecular distribution analysis with minimal sample pretreatment. Furthermore, no matrix is needed at ambient conditions (Takáts et al., 2004).
The extensive application of DESI-MSI to determine natural products (primary and secondary metabolites) demonstrated its power and good practicability (Dill et al., 2009; Li et al., 2012; Hemalatha and Pradeep, 2013; Hemalatha et al., 2015; Liao et al., 2019; Mohana Kumara et al., 2019).
In this study, an easy-handling and comprehensive method combining DESI-MSI with metabolomics was applied to investigate the variation of key ester-diterpenoid alkaloids and identify the metabolic markers in raw and processed Fuzi with different steaming time points. In addition, the contents of six ester-type alkaloids were determined through HPLC.
2 Materials and Methods
2.1 Materials and Reagents
The reference standards (>98%, quantification grade) of aconitine (40), mesaconitine (22), hypaconitine (36), benzoylaconine (34), benzoylmesaconine (32), and benzoylhypaconine (29) were obtained from Chengdu Herbpurify Co., Ltd. (Chengdu, China). LC-MS-grade methanol (MeOH), formic acid (FA), acetonitrile, and isopropanol were purchased from Sigma-Aldrich (Sigma-Aldrich, United States). Ammonium acetate of HPLC grade was obtained from Chengdu Chron Chemical Co., Ltd. (Chengdu, China). The Elga Labwater Purelab system (Elga-Veolia, High Wycombe, United Kingdom) was used to obtain purified water for UPLC analyses. All other reagents were of analytical grade.
A batch of crude Fuzi was collected from Jiangyou (Sichuan, China) and authenticated by Professor Yi Zhang from Chengdu University of TCM (Figure 2). The voucher specimens were deposited in the School of Ethnic Medicine, Chengdu University of TCM, China.
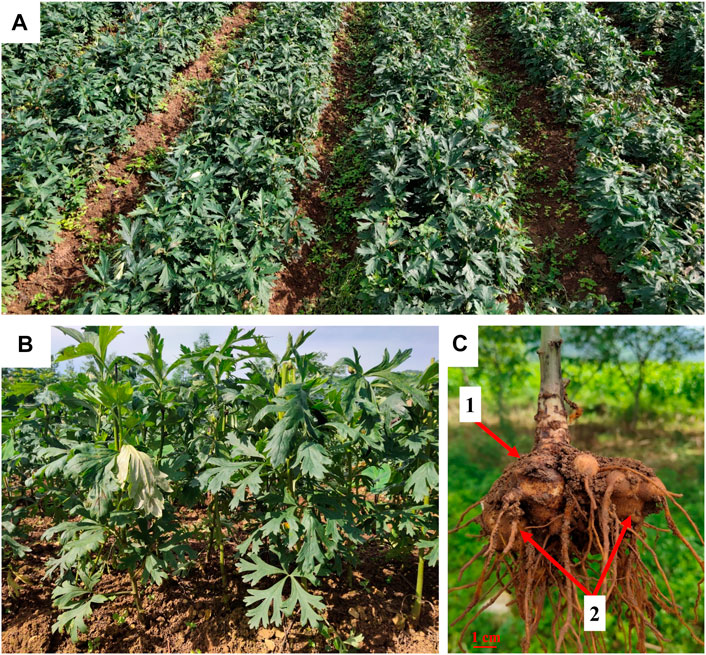
FIGURE 2. Fuzi planting base in Jiangyou, Sichuan (A,B). The mother root (1) and lateral root (2) of A. carmichaelii (C).
2.2 Sample Preparation and DESI-MSI Analysis
Cleaned raw Fuzi were randomly divided into 11 groups, one of which was selected as raw Fuzi (without steaming), and the rest were placed into a suitable container for steaming for 1.0, 2.0, 3.0, 4.0, 5.0, 6.0, 7.0, 8.0, 9.0, and 10.0 h. All the samples were directly sliced, and the thickness of cross-sectional slices was approximately 5 mm. Subsequently, the sample was glued onto glass slides using double-sided tape and then subjected to DESI-MSI analysis.
Imaging experiments were conducted using a Waters Xevo G2-XS QTof mass spectrometer equipped with a DESI source (Waters Corporation, Milford, MA, United States). The following DESI parameters were optimized to obtain good signal intensity: nebulizing gas (dry nitrogen) pressure: 0.45 MPa; spray solvent 70% MeOH, 30% H2O, 0.2% FA, and 0.1 mM leucine enkephalin (LE) delivered at 2 μl/min; capillary voltage: 4.5 kV; and ionization mode: positive. The pixel size (X and Y pixel size of 200 μm) was determined on the basis of the total scan time of the mass spectra and the x–y scanner speed. A mass range of m/z 100–1,000 and the scan rate of 400 μm/s were used for imaging, and the images were viewed using high-definition image (HDI) v1.5 software (Waters Corporation, Milford, MA, United States). The MS images were created from Raw MS files through HDI with LE as the lockmass (m/z 556.2766) for resolution MS.
2.3 Desorption Electrospray Ionization Mass Spectrometry Imaging Data Processing and Statistical Analysis
MS raw data files were imported into HDI for imaging. The regions of interest (ROIs) were selected from root areas. Each sample had six ROIs that were converted into a data matrix of mass m/z and signal intensity. The data matrix was imported into SIMCA-P 13.0 (Umetrics, Umea, Sweden) to establish PAC, PLS-DA, and OPLS-DA models for distinguishing classification analysis and selecting crucial variable constituents for Fuzi steaming for different time points. The data were standardized using a Pareto-scaling technique.
2.4 Sample Preparation and HPLC Analysis
According to the CP (2020 Edition) (Chinese Pharmacopoeia Commission, 2020), all the processed or raw samples were oven-dried at 50°C for 24 h. Then, the dried samples were finely powered and passed through a 50-mesh sieve. Each sample powder (2.0 g) was weighed and then extracted by ultrasonication with 3 ml of ammonia TS and 50 ml of isopropanol–ethyl acetate (1/1, v/v) for 30 min (300 w, 40 kHz). Following extraction, isopropanol–ethyl acetate (1/1, v/v) was added to compensate for the lost weight during extraction and then filtered. 25 ml of filtrate was accurately measured and evaporated under reduced pressure at 40°C to dryness. Three milliliters of 0.05 M hydrochloric acid–methanol solution was precisely added to dissolve the residue. The supernatants were used as the sample solution after being centrifuged at 15,000 rpm for 30 min. The concentration of aconitine (AC, 40), mesaconitine (MAC, 22), hypaconitine (HAC, 36), benzoylaconine (BAC, 34), benzoylmesaconine (BMAC, 32), and benzoylhypaconine (BHAC, 29) in every sample was calculated on the basis of established calibration curves.
The filtered sample was analyzed using Agilent Technology 1260 Infinity (Agilent Technologies, Santa Clara, CA, United States) equipped with a binary pump, mobile phase degasser, temperature-controlled autosampler, column thermostat, and diode array detector (1260-DAD). HPLC separation was performed on an Agilent Eclipse XDB C18 column (4.6 mm × 250 mm, 5 μm) and maintained at 30°C. The mobile phase consisted of 0.04 M of ammonium acetate solution (solvent A, adjusting pH to 8.5 with an ammonia solution) and acetonitrile (solvent B) using the following gradient: 20%–28% B for 0–10 min, 28%–30% B for 10–20 min, 30%–35% B for 20–50 min, and 35%–38% B for 50–60 min. The flow rate was kept at 1 ml/min, and the injection volume was 10 μl. The UV detection wavelength was set at 235 nm.
HPLC-DAD was validated with regard to linearity, stability, recovery, repeatability, and precision. The storage solutions of six compounds were used in the regression equations between the peak areas and concentrations of six compounds. The limit of detection (LOD) and quantification (LOQ) were determined at signal-to-noise ratios of 3 and 10, respectively.
3 Results
3.1 Optimization of Spray Solvent for Desorption Electrospray Ionization Mass Spectrometry Imaging Analysis
In DESI analysis, the ratio selection of solvent and acid is an important step to achieve sensitive and high MS signal response to target compounds. The solvent compositions are selected based on the analyte of interest and the tissue surface, which play a major role in the elution of each category of the compound (Claude et al., 2017). A large number of literature studies indicate that Fuzi primarily contains alkaloids. Therefore, a mixture of MeOH and water (with optional FA for positive ion mode analysis) is used as the DESI spray solvent for alkaloid analysis (Kumara et al., 2016). In the present experiment, the volume ratio of water to MeOH and FA concentration were evaluated (Supplementary Figure S1). As shown in Figures 3A,B, using 70% MeOH with 0.2% FA provided high response and uniform detection of six ester-type alkaloids across the Fuzi sample. Therefore, this solvent condition was used in the following MSI experiments.
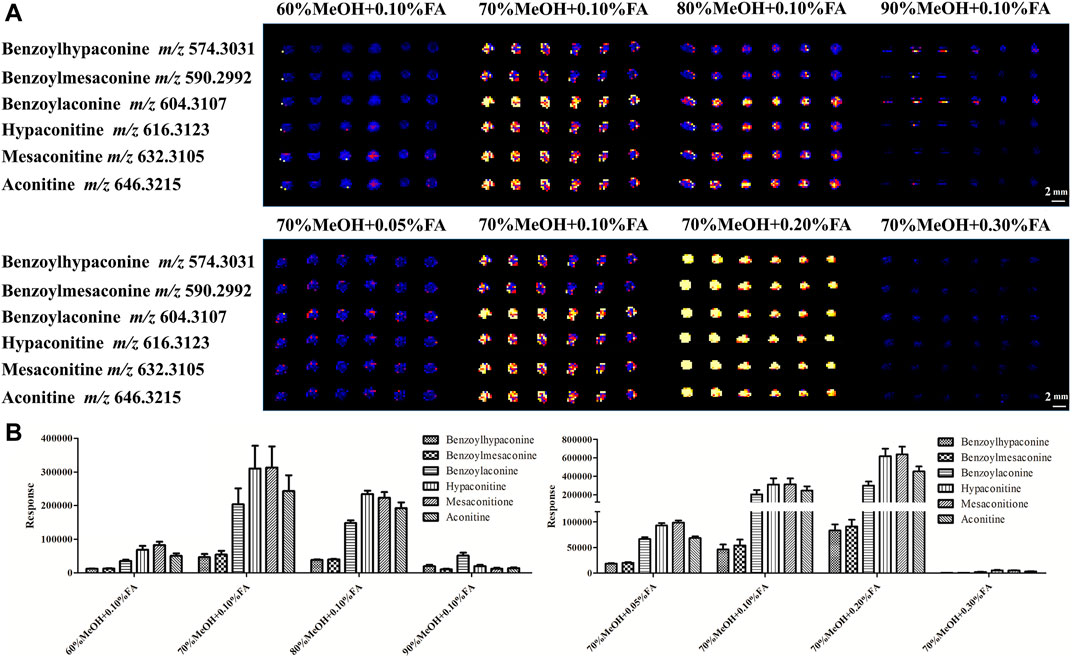
FIGURE 3. DESI-MS images (A) and responses (B) of six alkaloids using different concentrations of MeOH with different proportions of FA.
3.2 Effects of Steaming on Toxicity and Active Components of Fuzi
As a highly toxic medicinal material, improper use of Fuzi will seriously affect the safety of people. According to the CP (2020 edition), DDAs shall not exceed 0.020% with regard to the total amount of mesaconitine (MAC, 22), hypaconitine (HAC, 36), and aconitine (AC, 40). In addition, the total amount of MDAs, including benzoylmesaconine (BMAC, 32), benzoylaconine (BAC, 34), and benzoylhypaconine (BHAC, 29), should not be less than 0.010%.
The changes of the six aforementioned alkaloids in the samples of Fuzi steamed for different time points were analyzed by HPLC and DESI-MSI. The HPLC data (Table 1) showed that the content of three DDAs decreased under 0.020%, and MDAs increased above 0.010% in the investigated samples after 3.0 h of steaming. However, the MDA levels slightly declined to 0.096% after 4.0 h of steaming and then stabilized over 0.100% until 9.0 h of steaming. At 10.0 h of steaming, the total content of three MDAs reduced again. Therefore, the requirements stipulated by the CP can be met by steaming Fuzi for over 3.0 h. In addition, three DDAs of Fuzi could not be detected by HPLC (below the detection limit) after 5.0 h. The results of the methodological study are shown in Supplementary Tables S1, S2.
In situ molecular detection was conducted in simulated industrial sections of Fuzi using DESI-MS with a mass range of m/z 100–1,000 in the positive ion mode to explore the change process of six alkaloids specified by the CP during different steaming time points of Fuzi. As shown in the MS images (Figure 4), six alkaloids, namely, mesaconitine (22, m/z 632.3105), aconitine (40, m/z 646.3215), hypaconitine (36, m/z 616.3123), benzoylmesaconine (32, m/z 590.2992), benzoylaconine (34, m/z 604.3107), and benzoylhypaconine (29, m/z 574.3012), were visually presented and compared. With the extension of steaming time, the concentrations of three DDAs decreased sharply, whereas the three MDAs increased significantly, among which mesaconitine (22) and hypaconitine (36) decreased faster than aconitine (40) and could not be visualized after 1.0 h, and the intensity of benzoylmesaconine (32) and benzoylhypaconine (29) increased faster than that of benzoylaconine (34). The DESI-MSI results are consistent with those of HPLC experiments, which indicated its reliability.
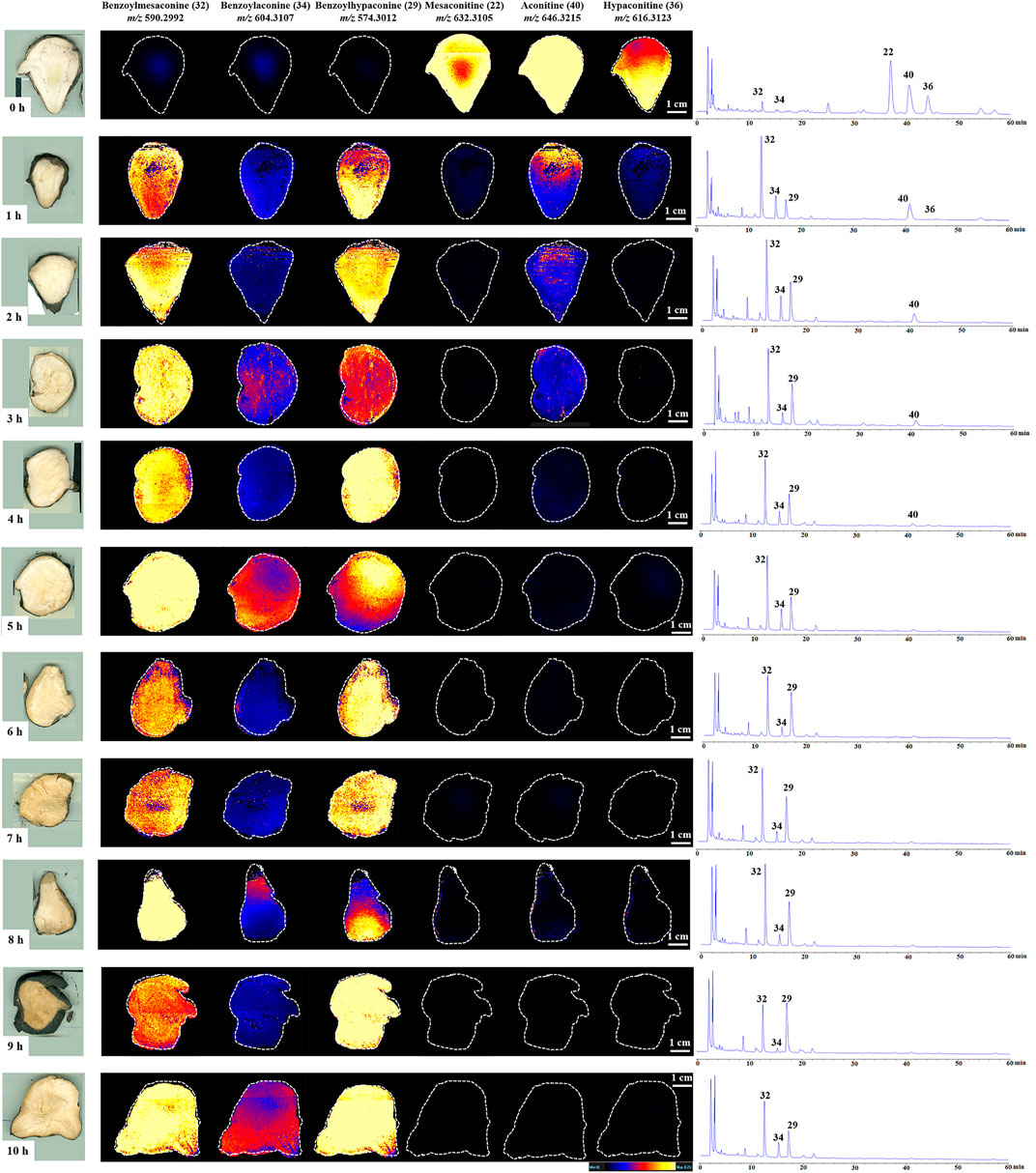
FIGURE 4. DESI-MS images and the corresponding HPLC chromatograms of six ester-type alkaloids in raw and processed Fuzi steamed for different time points.
3.3 PCA and PLS-DA of Raw and Processed Fuzi With Different Steaming Hours
A data matrix of mass m/z and signal intensity from DESI-MSI was submitted to PCA and PLS-DA to discriminate the variations of Fuzi with different steaming time points, visualize the underlying trend, and understand the metabolic differentiation among them. The PCA score plot (R2X = 0.884 and Q2 = 0.798) showed that all the ingredients could be classified into three major groups (Figure 5A). Raw Fuzi and processed Fuzi steamed only for 1.0 h were highly different from Fuzi steamed for more than 2.0 h. The ingredients of Fuzi steamed for 2.0–5.0 h were grouped together, but those steamed for 3.0 h were located relatively far away from the other samples. This may be due to the insufficient hydrolysis reaction from DDAs to MDAs. Fuzi steamed for 6.0–9.0 h were in a separate cluster. However, samples steamed for 10.0 h were back into the 2.0–5.0 h steaming group. The classification results also indicated that, after steaming for 5.0 h, these ingredients were located closer compared with those steamed for a short time. PLS-DA discrimination (R2X = 0.916, R2Y = 0.775, and Q2 = 0.701) (Figure 5B) was in good agreement with the PCA model, and all samples were separated into three groups. In addition, the samples steamed for a longer time were distributed closer. The close points indicated that these samples share similar chemical components. Combined with the results of HPLC, DESI-MSI, and multivariate statistical analysis, 4.0 h is demonstrated to be the appropriate steaming time for toxicity attenuation and efficacy reservation of Fuzi.
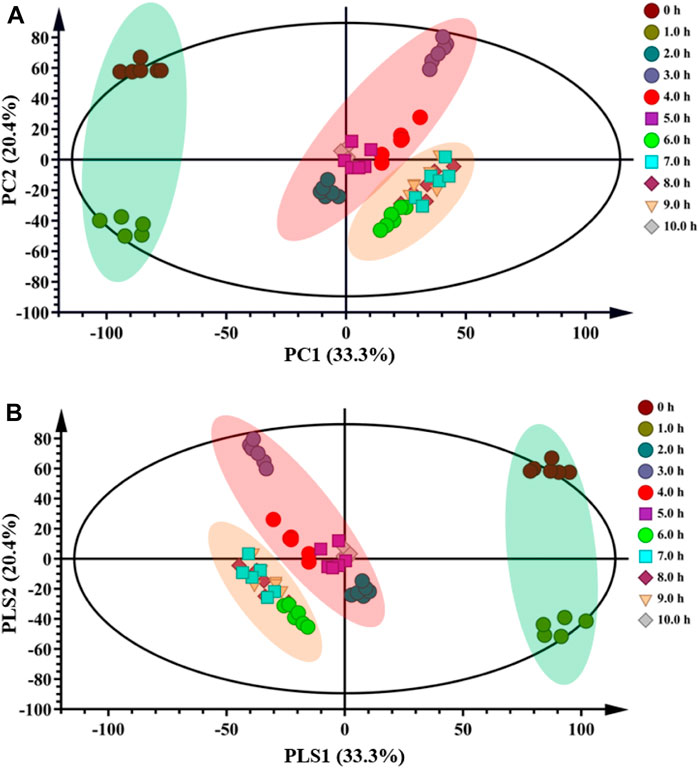
FIGURE 5. PCA (A) and PLS-DA (B) score plots of raw and processed Fuzi steamed for different time points.
3.4 Metabolic Markers for the Identification of Raw and Processed Fuzi With Different Steaming Hours
Based on the HPLC, DESI-MSI, PCA, and PLS-DA results, 4.0 and 8.0 h were selected as the key steaming time points to uncover the metabolic markers. OPLS-DA was applied to compare the metabolic changes between the 0 and 4.0 h groups and the 4.0 and 8.0 h groups. As shown in Figures 6A,C, the Fuzi samples with different steaming time points were separated from one another, indicating that the metabolic perturbation significantly occurred in raw Fuzi and processed Fuzi steamed at different time points. As shown in the corresponding S-plot (Figures 6B,D), the ions away from the origin contributed significantly to the separation between the 0 and 4.0 h groups and the 4.0 and 8.0 h groups. Therefore, the ions may be regarded as the discrimination metabolites for raw and processed Fuzi steamed for different hours. After combining the results of the S-plots, the VIP value (VIP >1) was obtained from OPLS-DA analysis, and the corresponding metabolites could be selected. The chemical structures of the metabolites were identified using online databases such as SciFinder (https://scifinder.cas.org/), and further confirmation was obtained through comparisons with authentic standards and published literature (Wang et al., 2002; Yue et al., 2007; Hu et al., 2009; Yan et al., 2010; Sun et al., 2012; Zhang et al., 2012; Sun et al., 2013; Xu et al., 2014; Yang et al., 2014; Luo et al., 2016; Zhang et al., 2017; Lei et al., 2021).
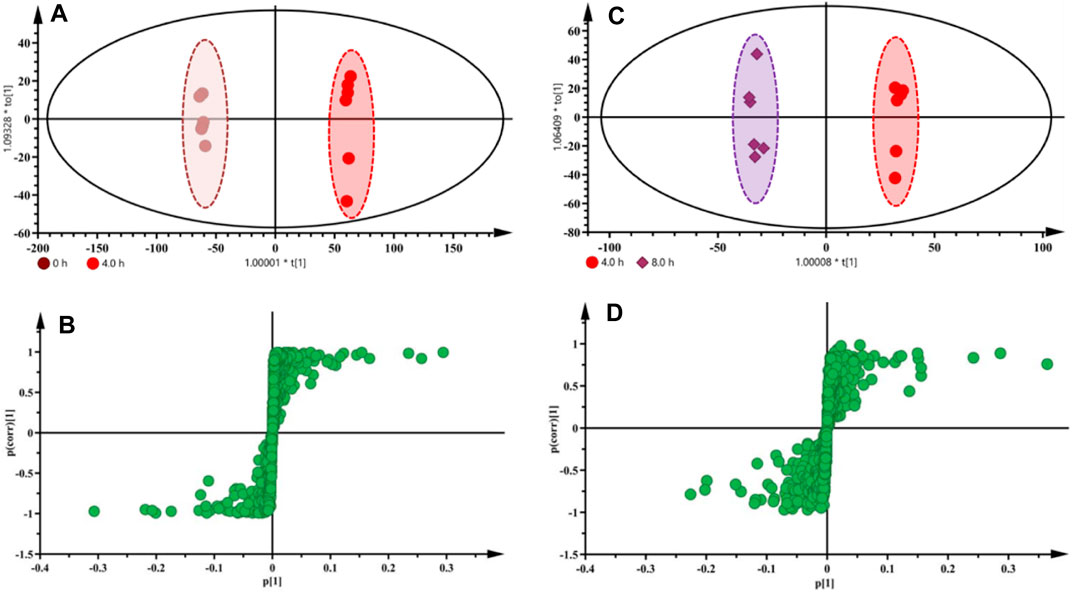
FIGURE 6. OPLS-DA score plots and S-plots based on raw and processed Fuzi steamed for 4.0 h (A,B) and Fuzi steamed for 4.0 and 8.0 h (C,D) from DESI-MSI data.
A total of 42 discriminating metabolites were tentatively identified, and the detailed information is given in Table 2 and Supplementary Figure S2. All 42 metabolites were visualized using DESI-MSI (Supplementary Figure S3). The intensities of 37 metabolites, including hypaconitine (36, m/z 616.3123), benzoylhypaconine (29, m/z 574.3012), neoline (17, m/z 438.2841), benzoylmesaconine (32, m/z 590.2992), mesaconitine (39, m/z 632.3105), aconitine (40, m/z 646.3215), and indaconitine (38, m/z 630.3278), changed after steaming for 4.0 h. Thus, these metabolites were considered as chemical markers to distinguish raw Fuzi and processed Fuzi steamed for 4.0 h. In addition, a total of 20 metabolites, such as neoline (17, m/z 438.2841), karacoline (10, m/z 378.2618), isotalatizidine/talatizidine (13, m/z 408.2755), benzoylhypaconine (29, m/z 574.3012), songorine (6, m/z 358.2386), fuzitine (2, m/z 342.1706), and talatisamine (14, m/z 422.2891), were set as the markers to distinguish Fuzi steamed for 4.0 and 8.0 h.
A heat map was used to visualize the changes in the content of the 42 metabolites in raw Fuzi and processed Fuzi for different steaming hours. As shown in Figure 7, the content of 15 metabolites increased, whereas that of 27 metabolites decreased. Metabolites with increased content are primarily MDAs. However, the content of most DDAs, including three highly toxic ones, mesaconitine (22), aconitine (40), hypaconitine (36), and some of the MDAs decreased dramatically within two steaming hours. The content of napelline (7), denudatine/Guanfu base H (3), karakolidine/Chuanfumine (11), karakanine (9), episcopalisine (24), and aconicarchamine B (25) declined to low levels after steaming for 4.0–5.0 h, but such content increased again when steaming was continued for 10.0 h. Nine metabolites, including dehydrated benzoylhypaconine (26), fuziline (19), senbusine A/B (15), guiwuline (16), karacoline (10), talatisamine (14), dictysine (5), 16-hydroxycardiopetaline (8), and nevadenine (12), showed volatility decreases, and most of them belong to NDAs. As an isoquinoline alkaloid, fuzitine (2) generally exists in the form of salt, which showed a particular changing rule during steaming. The content reached the highest on steaming after 4.0 h and decreased or transformed to other metabolites with the extension of steaming time. The heat map also indicated that some of the metabolites were unstable when steamed for 3.0 h and then tended to be stable. When steamed for 10.0 h, the content of metabolites became similar to samples steamed for 4.0–5.0 h. This phenomenon corresponds to the aforementioned PCA and PLS-DA results (Figure 6).
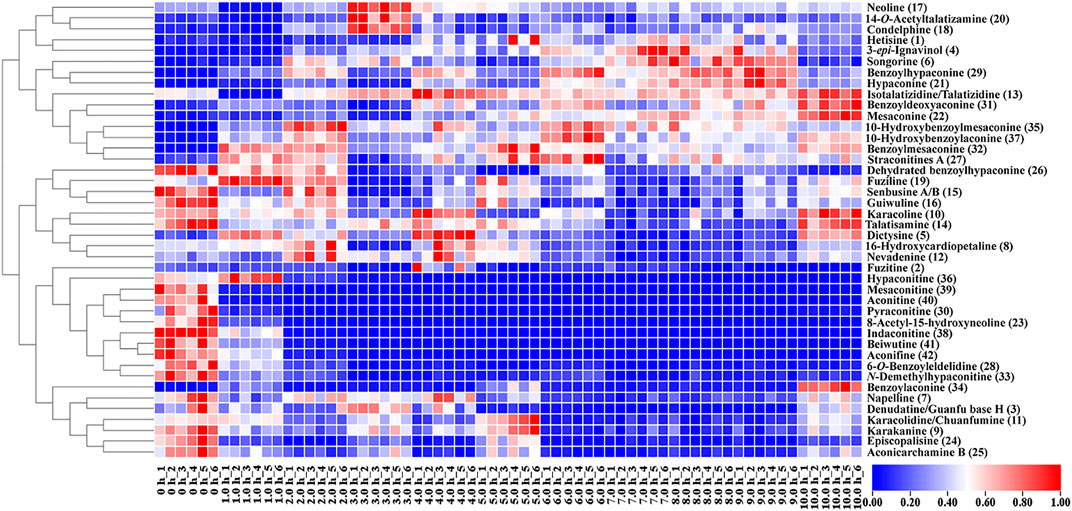
FIGURE 7. Heat map of metabolic markers of raw and processed Fuzi steamed for different time points.
4 Discussion
As a typical toxic traditional herbal medicine that is known for its extraordinary pharmacological activities and toxicity, Fuzi has long been used as an irreplaceable traditional herbal medicine in TCM, Kampo medicine, and homeopathy for thousands of years (Zhou et al., 2015; Wu et al., 2018; Qiu et al., 2021).
As toxicity and efficacy are interdependent in Fuzi, the toxicities of processed products such as Yanfuzi, Heishunpian, Baifupian, Danfupian, and Paofupian dramatically decreased compared with raw Fuzi (Zhou et al., 2012; Zhao et al., 2014), but the efficacy also decreased. Considering that Danba is needed during the aforementioned procedures, which would induce halide ions and inorganic impurities into Fuzi, the alkaloids will be lost with a long rinsing time (Ye et al., 2019). Recent studies indicated that the total alkaloids and various ester-diterpenoid alkaloids’ levels were high in directly steamed, stir-fried, or baked Fuzi, which showed better detoxification and efficacy reservation effects than Danba-used procedures (Liu et al., 2019; Ye et al., 2019). Thus, this study focused on direct steaming, discussed changeable rules of the key ester-diterpenoid alkaloids, and identified the metabolic markers in raw and processed Fuzi steamed at different time points through DESI-MSI combined with metabolomics.
Investigations of basal metabolism are important to improve the quality control of TCMs (Jiang et al., 2022). DDAs, the major pharmaceutical and toxic secondary metabolites, are important to determine the quality, safety, and efficacy of Fuzi, which were hydrolyzed during heating processing procedures, such as steaming. However, the changeable patterns during steaming are rarely reported. Here, DESI-MSI provides an easy and effective way to explore the change characteristics of the key ester-diterpenoid Aconitum alkaloids. Combining metabolism and multivariate statistical analysis, the metabolites and important markers would be identified, which are essential to assess the quality of steamed Fuzi.
HPLC was initially applied to determine variation in the content of three MDAs and three DDAs. A total of 10 steaming time points were set, and HPLC data indicated that the content limit of steamed Fuzi met the requirements of the CP after 3.0 h of steaming and became steady after 4.0 h. DESI-MSI was performed to visualize the HPLC results with high resolutions in mass and space: three MDAs were difficult to detect, and three DDAs were highly visible in raw Fuzi. The images showed an increase in MDAs and a decrease in DDAs during steaming. After 4.0 h of steaming, DDAs and MDAs met the standards of CP, and parts of the DDAs still remain. PCA and PLS-DA analyses also illustrated that the samples after steaming for over 4.0 h were grouped closer and located in the coordinate origin region. Consequently, 4.0 h of steaming was recommended for the toxicity attenuation and efficacy reservation of Fuzi.
Chemometric analysis was combined with a heat map to identify the significant metabolic markers, and 4.0 h was set as the key time node. The DESI-MSI comparison of the 42 metabolites of raw Fuzi and processed Fuzi steamed for 4.0 and 8.0 h showed that the content of highly toxic DDAs decreased dramatically, and a large part of the content of MDAs and NDAs fluctuated during steaming, which indicated a reversible process (Figure 8). DDAs and MDAs were the major metabolic markers between raw and processed Fuzi steamed for 4.0 h, whereas NDAs were identified as the predominant markers of Fuzi steamed for 4.0 and 8.0 h. During 0–4.0 h of steaming, hydrolysis was conducted to convert DDAs to MDAs and then to NDAs, which are considered as the major chemical transformations based on the present results and published literature (Liu et al., 2008; Chen et al., 2013; Qiu et al., 2021), and three main paths for the transformation of C19-ester-diterpenoid alkaloids were proposed (Figure 9). Except for conventional hydrolysis and dehydration processes (Figures 9A,B), esterification reactions 1 and 2 in path C also indicated two important reversible processes, which explained why some of the alkaloids’ content fluctuated during steaming. However, NDAs were the important hydrolysis products after long and continuous steaming, and the pharmaceutical activities will then decrease.
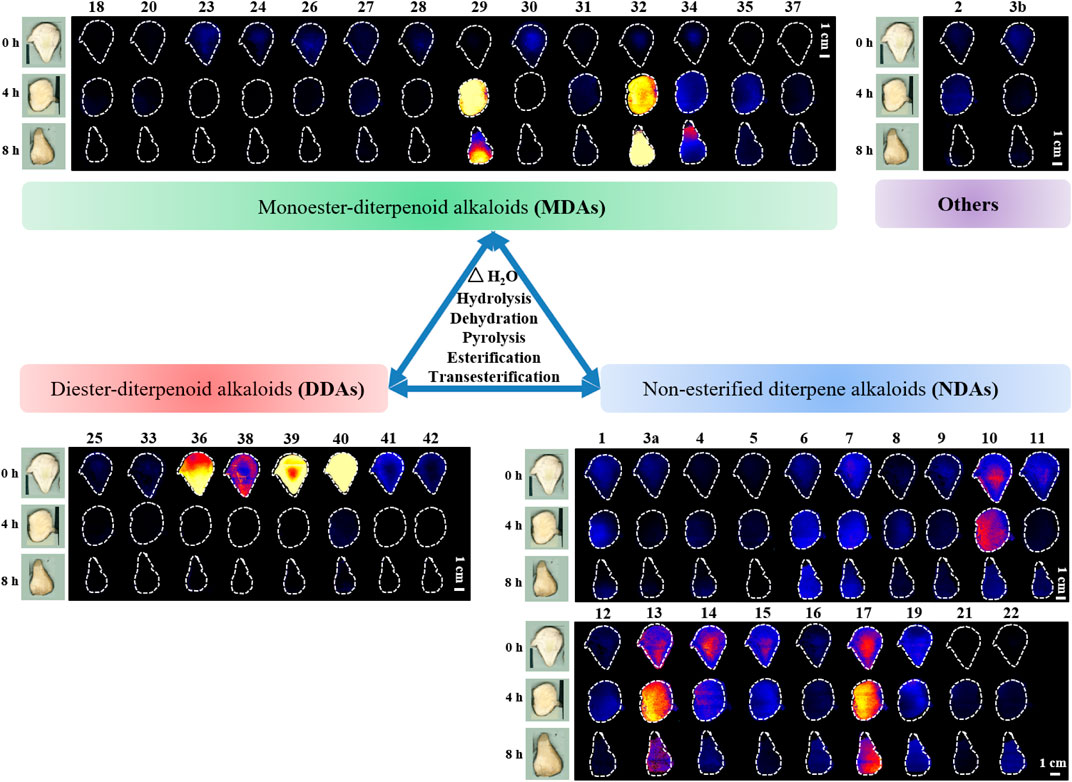
FIGURE 8. DESI-MS images of 42 metabolic markers in raw and processed Fuzi steamed for 4.0 and 8.0 h.
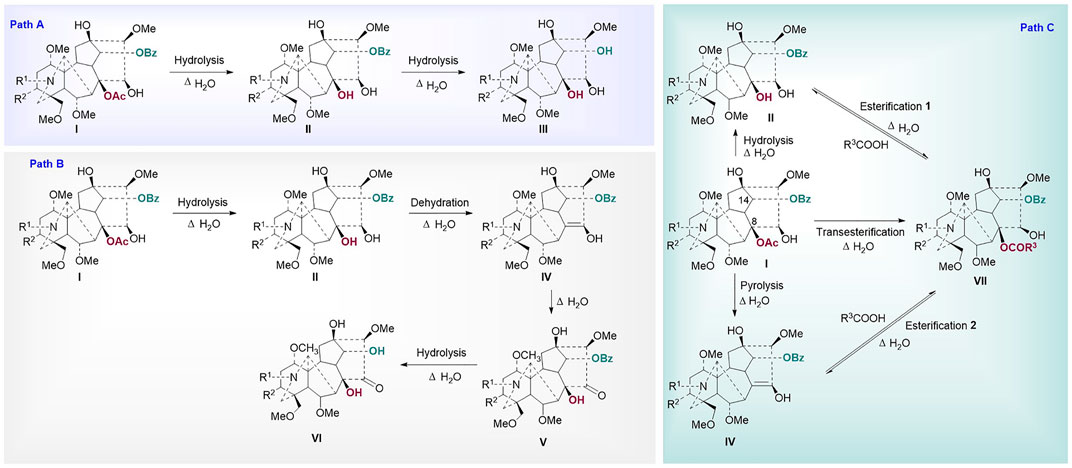
FIGURE 9. Proposed mechanism for the transformation of ester type alkaloids (Path (A): hydrolysis reactions; Path (B): hydrolysis and dehydration reactions; Path (C): esterification and transesterification reactions I: diester-diterpenoid alkaloids, II: monoester-diterpenoid alkaloids; III: non-esterified diterpene alkaloids; IV: enol-type monoester-diterpenoid alkaloids; V: ketone-type monoester-diterpenoid alkaloids; VI: ketone-type non-esterified diterpene alkaloids; VII: lipo-diterpenoid alkaloids).
5 Conclusion
In this study, the combination of DESI-MSI and metabolomics for rapid and high-resolution characterization of ester alkaloids in raw and steamed Fuzi was developed. The changes in alkaloids of raw and processed Fuzi with different steaming time periods were observed, and the results of HPLC, DESI-MS coupled with PCA, PLS-DA, and OPLS-DA all indicated that 4.0 h of steaming was appropriate for toxicity attenuation and efficacy reservation of Fuzi. In addition, a total of 37 metabolic markers to distinguish raw and processed Fuzi steamed for 4.0 h and 22 metabolites to distinguish Fuzi steamed for 4.0 and 8.0 h were identified through DESI-MSI-based metabolomics. Moreover, three major chemical transformation pathways of C19-ester alkaloids were summarized. Therefore, the novel method provides an efficient approach to visualize the changeable rules and screen the metabolic markers of alkaloids during steaming. The wide application of the method could help to identify biomarkers and reveal the possible chemical transition mechanism in the “Paozhi” processes of TCM.
Data Availability Statement
The raw data supporting the conclusion of this article will be made available by the authors, without undue reservation.
Author Contributions
YL wrote the main manuscript, drew the figures, and conducted part of the experiments. TK, ZW, XY, CZ, JS, and BX conducted the experiments. XM and YZ reviewed and edited the manuscript. CT supervised the experiments and prepared the manuscript and directed the final version of the manuscript. All authors reviewed and approved this manuscript.
Funding
This study was supported by the National Natural Science Foundation of China (Nos. 81903922 and 82130113), the China Postdoctoral Science Foundation (No. 2021MD703800), the Applied Basic Research Program of Sichuan Province (No. 2020YJ0375), the Key Research and Development Projects of Sichuan Province (No. 2021YFS0042), and the “Xinglin Scholars” Research Promotion Program of Chengdu University of TCM (Nos. BSH2019002 and BSH2021009).
Conflict of Interest
Authors XY and CZ were employed by the company Waters Technology (Beijing) Co., Ltd.
The remaining authors declare that the research was conducted in the absence of any commercial or financial relationships that could be construed as a potential conflict of interest.
Publisher’s Note
All claims expressed in this article are solely those of the authors and do not necessarily represent those of their affiliated organizations, or those of the publisher, the editors and the reviewers. Any product that may be evaluated in this article, or claim that may be made by its manufacturer, is not guaranteed or endorsed by the publisher.
Acknowledgments
The authors thank XY and CZ of Waters Technology (Beijing) Co., Ltd., for their great help in mass spectrometry imaging.
Supplementary Material
The Supplementary Material for this article can be found online at: https://www.frontiersin.org/articles/10.3389/fphar.2022.842890/full#supplementary-material
References
Chen, X., Tan, P., He, R., and Liu, Y.-g. (2013). Study on the Fragmentation Pathway of the Aconitine-type Alkaloids under Electrospray Ionization Tandem Mass Spectrometry Utilizing Quantum Chemistry. J. Pharm. Innov. 8, 83–89. doi:10.1007/s12247-013-9148-z
Chinese Pharmacopoeia Commission (2020). The Pharmacopoeia of the People's Republic of China. 2020 edition. Beijing: China Medical Science Press, 200.
Claude, E., Jones, E. A., and Pringle, S. D. (2017). “DESI Mass Spectrometry Imaging (MSI),” in Imaging Mass Spectrometry: Methods and Protocols. Editor L.M. Cole (New York, NY: Springer), 65–75. doi:10.1007/978-1-4939-7051-3_7
Dill, A. L., Ifa, D. R., Manicke, N. E., Ouyang, Z., and Cooks, R. G. (2009). Mass Spectrometric Imaging of Lipids Using Desorption Electrospray Ionization. J. Chromatogr. B Analyt Technol. Biomed. Life Sci. 877 (26), 2883–2889. doi:10.1016/j.jchromb.2008.12.058
Dong, S.-H., Meng, J., Wu, M.-H., Ma, Z.-G., Cao, H., and Zhang, Y. (2020). Herbalogical Study of Aconiti Lateralis Radix Praeparata (Fuzi). Zhongguo Zhong Yao Za Zhi 45 (22), 5567–5575. doi:10.19540/j.cnki.cjcmm.20200802.102
Fu, M., Wu, M., Qiao, Y., and Wang, Z. (2006). Toxicological Mechanisms of Aconitum Alkaloids. Pharmazie 61 (9), 735–741. PMID: 17020146.
Garza, K. Y., Feider, C. L., Klein, D. R., Rosenberg, J. A., Brodbelt, J. S., and Eberlin, L. S. (2018). Desorption Electrospray Ionization Mass Spectrometry Imaging of Proteins Directly from Biological Tissue Sections. Anal. Chem. 90 (13), 7785–7789. doi:10.1021/acs.analchem.8b00967
Hemalatha, R. G., and Pradeep, T. (2013). Understanding the Molecular Signatures in Leaves and Flowers by Desorption Electrospray Ionization Mass Spectrometry (DESI MS) Imaging. J. Agric. Food Chem. 61 (31), 7477–7487. doi:10.1021/jf4011998
Hemalatha, R. G., Naik, H. R., Mariappa, V., and Pradeep, T. (2015). Rapid Detection of Fusarium Wilt in Basil (Ocimum sp.) Leaves by Desorption Electrospray Ionization Mass Spectrometry (DESI MS) Imaging. RSC Adv. 5 (62), 50512–50522. doi:10.1039/C4RA16706F
Hu, R., Zhao, J., Qi, L.-W., Li, P., Jing, S.-L., and Li, H.-J. (2009). Structural Characterization and Identification of C19-And C20-Diterpenoid Alkaloids in Roots of Aconitum Carmichaeli by Rapid-Resolution Liquid Chromatography Coupled with Time-Of-Flight Mass Spectrometry. Rapid Commun. Mass. Spectrom. 23, 1619–1635. doi:10.1002/rcm.4038
Jiang, H., Zhang, Y., Liu, Z., Wang, X., He, J., and Jin, H. (2022). Advanced Applications of Mass Spectrometry Imaging Technology in Quality Control and Safety Assessments of Traditional Chinese Medicines. J. Ethnopharmacol. 284, 114760. doi:10.1016/j.jep.2021.114760
Kumara, P. M., Srimany, A., Arunan, S., Ravikanth, G., Shaanker, R. U., and Pradeep, T. (2016). Desorption Electrospray Ionization (DESI) Mass Spectrometric Imaging of the Distribution of Rohitukine in the Seedling of Dysoxylum Binectariferum Hook. F. PLoS ONE 11 (6), e0269099. doi:10.1371/journal.pone.0158099
Lei, H., Zhang, Y., Ye, J., Cheng, T., Liang, Y., Zu, X., et al. (2021). A Comprehensive Quality Evaluation of Fuzi and its Processed Product through Integration of UPLC-QTOF/MS Combined MS/MS-based Mass Spectral Molecular Networking with Multivariate Statistical Analysis and HPLC-MS/MS. J. Ethnopharmacol. 266, 113455. doi:10.1016/j.jep.2020.113455
Li, S. X., Zheng, F. Y., Li, Y. C., Cai, T. S., and Zheng, J. Z. (2012). Determination of Zinc and Copper in Edible Plants by Nanometer Silica Coated, Slotted Quartz Tube, Flame Atomic Absorption Spectrometry. J. Agric. Food Chem. 60 (47), 11691–11695. doi:10.1021/jf304485q
Liao, Y., Fu, X., Zhou, H., Rao, W., Zeng, L., and Yang, Z. (2019). Visualized Analysis of Within-Tissue Spatial Distribution of Specialized Metabolites in tea (Camellia Sinensis) Using Desorption Electrospray Ionization Imaging Mass Spectrometry. Food Chem. 292, 204–210. doi:10.1016/j.foodchem.2019.04.055
Liu, Y.-G., Yu, D.-L., Chen, Y.-J., Zhang, H.-G., and Qiao, Y.-J. (2008). Study on Chemical Reaction of Mesaconitine in Water by HPLC-ESI-MSn. Chin. J. New Drugs 17 (2), 153–156.
Liu, S., Li, F., Li, Y., Li, W., Xu, J., and Du, H. (2017). A Review of Traditional and Current Methods Used to Potentially Reduce Toxicity of Aconitum Roots in Traditional Chinese Medicine. J. Ethnopharmacol. 207, 237–250. doi:10.1016/j.jep.2017.06.038
Liu, Y., Liu, H., Ye, Q., Fang, C., Liu, X., Liu, R., et al. (2019). Study on the Effect of Brine Processing on Contents of Alkaloids in Aconiti Lateralis Radix Praeparata. Trad. Chin. Drug Res. Clin. Pharm. 30 (4), 472–477. doi:10.19378/j.issn.1003-9783.2019.04.013
Luo, H., Huang, Z., Tang, X., Yi, J., Chen, S., Yang, A., et al. (2016). Dynamic Variation Patterns of Aconitum Alkaloids in Daughter Root of Aconitum Carmichaelii (Fuzi) in the Decoction Process Based on the Content Changes of Nine Aconitum Alkaloids by HPLC- MS- MS. Iran. J. Pharm. Res. 15 (1), 263–273. doi:10.22037/ijpr.2016.1780
Luo, C., Yi, F., Xia, Y., Huang, Z., Zhou, X., Jin, X., et al. (2019). Comprehensive Quality Evaluation of the Lateral Root of Aconitum Carmichaelii Debx. (Fuzi): Simultaneous Determination of Nine Alkaloids and Chemical Fingerprinting Coupled with Chemometric Analysis. J. Sep. Sci. 42 (5), 980–990. doi:10.1002/jssc.201800937
Miao, L. L., Zhou, Q. M., Peng, C., Meng, C. W., Wang, X. Y., and Xiong, L. (2019). Discrimination of the Geographical Origin of the Lateral Roots of Aconitum Carmichaelii Using the Fingerprint, Multicomponent Quantification, and Chemometric Methods. Molecules 24 (22), 4124. doi:10.3390/molecules24224124
Mohana Kumara, P., Uma Shaanker, R., and Pradeep, T. (2019). UPLC and ESI-MS Analysis of Metabolites of Rauvolfia Tetraphylla L. And Their Spatial Localization Using Desorption Electrospray Ionization (DESI) Mass Spectrometric Imaging. Phytochemistry 159, 20–29. doi:10.1016/j.phytochem.2018.11.009
Parrot, D., Papazian, S., Foil, D., and Tasdemir, D. (2018). Imaging the Unimaginable: Desorption Electrospray Ionization - Imaging Mass Spectrometry (DESI-IMS) in Natural Product Research. Planta Med. 84, 584–593. doi:10.1055/s-0044-100188
Qiu, Z. D., Zhang, X., Wei, X. Y., Chingin, K., Xu, J. Q., Gao, W., et al. (2021). Online Discovery of the Molecular Mechanism for Directionally Detoxification of Fuzi Using Real-Time Extractive Electrospray Ionization Mass Spectrometry. J. Ethnopharmacol. 277, 114216. doi:10.1016/j.jep.2021.114216
Singhuber, J., Zhu, M., Prinz, S., and Kopp, B. (2009). Aconitum in Traditional Chinese Medicine: a Valuable Drug or an Unpredictable Risk? J. Ethnopharmacol. 126, 18–30. doi:10.1016/j.jep.2009.07.031
Stoeckli, M., Chaurand, P., Hallahan, D. E., and Caprioli, R. M. (2001). Imaging Mass Spectrometry: a New Technology for the Analysis of Protein Expression in Mammalian Tissues. Nat. Med. 7 (4), 493–496. doi:10.1038/86573
Sun, H., Ni, B., Zhang, A., Wang, M., Dong, H., and Wang, X. (2012). Metabolomics Study on Fuzi and its Processed Products Using Ultra-Performance Liquid-Chromatography/electrospray-Ionization Synapt High-Definition Mass Spectrometry Coupled with Pattern Recognition Analysis. Analyst 137, 170–185. doi:10.1039/c1an15833c
Sun, H., Wang, M., Zhang, A., Ni, B., Dong, H., and Wang, X. (2013). UPLC-Q-TOF-HDMS Analysis of Constituents in the Root of Two Kinds of Aconitum Using a Metabolomics Approach. Phytochem. Anal. 24, 263–276. doi:10.1002/pca.2407
Sun, L., Liu, F., You, G., Feng, T., Wang, M., Liu, Y., et al. (2020). A Comparative Analysis of Aconiti Lateralis Radix and Processed Products Using UHPLC-Q-TOF-MS Combined with Multivariate Chemometrics Strategies. J. Liq. Chromatogr. Relat. Technol. 43 (1-2), 37–44. doi:10.1080/10826076.2019.1659150
Takáts, Z., Wiseman, J. M., Gologan, B., and Cooks, R. G. (2004). Mass Spectrometry Sampling under Ambient Conditions with Desorption Electrospray Ionization. Science 306 (5695), 471–473. doi:10.1126/science.1104404
Tan, M.-L., Huang, Q.-W., Xiao, F., Fan, R.-Y., Wang, Z.-L., and Yi, J.-J. (2016). Selection of Suitable Herbs for Steaming Fupian Based on the Change of Contents of Ester-Type Alkaloids. Chin. Trad. Pat. Med. 38 (2), 366–372. doi:10.3969/j.issn.1001-1528.2016.02.029
Tong, P., Wu, C., Wang, X., Hu, H., Jin, H., Li, C., et al. (2013). Development and Assessment of a Complete-Detoxication Strategy for Fuzi (Lateral Root of Aconitum Carmichaeli) and its Application in Rheumatoid Arthritis Therapy. J. Ethnopharmacol. 146 (2), 562–571. doi:10.1016/j.jep.2013.01.025
Wang, Y., Liu, Z., Song, F., and Liu, S. (2002). Electrospray Ionization Tandem Mass Spectrometric Study of the Aconitines in the Roots of Aconite. Rapid Commun. Mass. Spectrom. 16 (22), 2075–2082. doi:10.1002/rcm.828
Wang, Y., Shi, L., Song, F., Liu, Z., and Liu, S. (2003). Exploring the Ester-Exchange Reactions of Diester-Diterpenoid Alkaloids in the Aconite Decoction Process by Electrospray Ionization Tandem Mass Spectrometry. Rapid Commun. Mass. Spectrom. 17 (4), 279–284. doi:10.1002/rcm.914
Wu, J. J., Guo, Z. Z., Zhu, Y. F., Huang, Z. J., Gong, X., Li, Y. H., et al. (2018). A Systematic Review of Pharmacokinetic Studies on Herbal Drug Fuzi: Implications for Fuzi as Personalized Medicine. Phytomedicine 44, 187–203. doi:10.1016/j.phymed.2018.03.001
Xu, W., Zhang, J., Zhu, D., Huang, J., Huang, Z., Bai, J., et al. (2014). Rapid Separation and Characterization of Diterpenoid Alkaloids in Processed Roots of Aconitum Carmichaeli Using Ultra High Performance Liquid Chromatography Coupled with Hybrid Linear Ion Trap-Orbitrap Tandem Mass Spectrometry. J. Sep. Sci. 37 (20), 2864–2873. doi:10.1002/jssc.201400365
Xu, J., Li, X., Zhang, F., Tang, L., Wei, J., Lei, X., et al. (2019). Integrated UPLC-Q/TOF-MS Technique and MALDI-MS to Study of the Efficacy of YiXinshu Capsules against Heart Failure in a Rat Model. Front. Pharmacol. 10, 1474. doi:10.3389/fphar.2019.01474
Yan, G., Sun, H., Sun, W., Zhao, L., Meng, X., and Wang, X. (2010). Rapid and Global Detection and Characterization of Aconitum Alkaloids in Yin Chen Si Ni Tang, a Traditional Chinese Medical Formula, by Ultra Performance Liquid Chromatography-High Resolution Mass Spectrometry and Automated Data Analysis. J. Pharm. Biomed. Anal. 53 (3), 421–431. doi:10.1016/j.jpba.2010.05.004
Yang, Y., Yin, X. J., Guo, H. M., Wang, R. L., Song, R., Tian, Y., et al. (2014). Identification and Comparative Analysis of the Major Chemical Constituents in the Extracts of Single Fuzi Herb and Fuzi-Gancao Herb-Pair by UFLC-IT-TOF/MS. Chin. J. Nat. Med. 12 (7), 542–553. doi:10.1016/S1875-5364(14)60084-4
Yang, M., Ji, X., and Zuo, Z. (2018). Relationships between the Toxicities of Radix Aconiti Lateralis Preparata (Fuzi) and the Toxicokinetics of its Main Diester-Diterpenoid Alkaloids. Toxins (Basel) 10 (10), 391. doi:10.3390/toxins10100391
Ye, Q., Liu, Y.-S., Liu, H.-M., Liu, R.-J., Tang, X.-M., and Guo, L. (2019). Effects of Different Processing Technology on Contents of Alkaloids in Aconiti Lateralis Radix Praeparata. Chin. Trad. Pat. Med 41 (3), 601–607. doi:10.3969/j.issn.1001-1528.2019.03.024
Yue, H., Pi, Z. F., Song, F. R., Liu, Z. Q., and Liu, S. Y. (2007). Analysis of Aconite Alkaloids in the Combination of Radix Aconiti Lateralis Preparata with Different Herbs by ESI-MS Spectrometry. Yao Xue Xue Bao 42 (2), 201–205. PMID: 17518052. doi:10.16438/j.0513-4870.2007.02.017
Yue, H., Pi, Z., Song, F., Liu, Z., Cai, Z., and Liu, S. (2009). Studies on the Aconitine-type Alkaloids in the Roots of Aconitum Carmichaeli Debx. By HPLC/ESIMS/MSn. Talanta 77, 1800–1807. doi:10.1016/j.talanta.2008.10.022
Zhang, J., Huang, Z. H., Qiu, X. H., Yang, Y. M., Zhu, D. Y., and Xu, W. (2012). Neutral Fragment Filtering for Rapid Identification of New Diester-Diterpenoid Alkaloids in Roots of Aconitum Carmichaeli by Ultra-high-pressure Liquid Chromatography Coupled with Linear Ion Trap-Orbitrap Mass Spectrometry. PLOS ONE 7 (12), e52352. doi:10.1371/journal.pone.0052352
Zhang, M., Wang, M., Liang, J., Wen, Y., and Xiong, Z. (2017). Chemical UPLC-ESI-MS/MS Profiling of Aconitum Alkaloids and Their Metabolites in Rat Plasma and Urine after Oral Administration of Aconitum Carmichaelii Debx. Root Extract. Biomed. Chromatogr. 32, e4049. doi:10.1002/bmc.4049
Zhao, Z., Liang, Z., Chan, K., Lu, G., Lee, E. L., Chen, H., et al. (2010). A Unique Issue in the Standardization of Chinese Materia Medica: Processing. Planta Med. 76 (17), 1975–1986. doi:10.1055/s-0030-1250522
Zhao, D., Wang, J., Cui, Y., and Wu, X. (2012). Pharmacological Effects of Chinese Herb Aconite (Fuzi) on Cardiovascular System. J. Tradit. Chin. Med. 32 (3), 308–313. doi:10.1016/S0254-6272(13)60030-8
Zhao, Y., Wang, J., Sun, X., Jia, L., Li, J., Shan, L., et al. (2014). Microcalorimetry Coupled with Chemometric Techniques for Toxicity Evaluation of Radix Aconiti Lateralis Preparata (Fuzi) and its Processed Products on Escherichia coli. Appl. Microbiol. Biotechnol. 98 (1), 437–444. doi:10.1007/s00253-013-5385-9
Zhou, Z.-Y., Xiong, Y.-A., Huang, Q.-W., and Yang, M. (2012). Acute Toxicity of Different Processed Products and its Parts of Aconitum Carmichalii Debx. J. Chengdu Univ. Trad. Chin. Med 35 (3), 63–65. doi:10.13593/j.cnki.51-1501/r.2012.03.026
Zhou, G., Tang, L., Zhou, X., Wang, T., Kou, Z., and Wang, Z. (2015). A Review on Phytochemistry and Pharmacological Activities of the Processed Lateral Root of Aconitum Carmichaelii Debeaux. J. Ethnopharmacol. 160, 173–193. doi:10.1016/j.jep.2014.11.043
Keywords: Fuzi, DESI-MSI, diterpenoid alkaloids, toxicity attenuation, processing
Citation: Liu Y, Yang X, Zhou C, Wang Z, Kuang T, Sun J, Xu B, Meng X, Zhang Y and Tang C (2022) Unveiling Dynamic Changes of Chemical Constituents in Raw and Processed Fuzi With Different Steaming Time Points Using Desorption Electrospray Ionization Mass Spectrometry Imaging Combined With Metabolomics. Front. Pharmacol. 13:842890. doi: 10.3389/fphar.2022.842890
Received: 24 December 2021; Accepted: 16 February 2022;
Published: 10 March 2022.
Edited by:
Abdul Rohman, Gadjah Mada University, IndonesiaCopyright © 2022 Liu, Yang, Zhou, Wang, Kuang, Sun, Xu, Meng, Zhang and Tang. This is an open-access article distributed under the terms of the Creative Commons Attribution License (CC BY). The use, distribution or reproduction in other forums is permitted, provided the original author(s) and the copyright owner(s) are credited and that the original publication in this journal is cited, in accordance with accepted academic practice. No use, distribution or reproduction is permitted which does not comply with these terms.
*Correspondence: Ce Tang, dGFuZ2NlQGNkdXRjbS5lZHUuY24=