- 1Department of Oncology, Renmin Hospital of Wuhan University, Wuhan, China
- 2Department of Radiation Oncology, Shandong Cancer Hospital and Institute, Shandong First Medical University and Shandong Academy of Medical Sciences, Jinan, China
- 3Department of Radiation Oncology, Shandong Cancer Hospital and Institute Affiliated to Shandong University, Jinan, China
Brain metastases are more and more common among patients with non-small cell lung cancer (NSCLC). TKI therapy could provide ideal outcomes for patients harboring epidermal growth factor receptor or ALK mutations. For wild-type patients, however, survival is poor because there are few effective treatments other than radiotherapy. Immune checkpoint inhibitors (ICIs) have changed the management of advanced NSCLC. However, the exclusion of patients with active brain metastasis (BM) from most ICI trials precludes the generalization of results. Accordingly, a variety of appropriate real-world studies and clinical trials are being developed to evaluate tumor response. Increasingly encouraging results have suggested that ICIs could be active in the central nervous system (CNS) in select patients with high PD-L1 expression and low CNS disease burden. With the extensive use of ICIs in NSCLC patients with BM, many important questions have emerged concerning issues such as the clinical response to a single ICI, use of ICIs combined with chemotherapy or radiation, the biological mechanism and appropriate sequencing of local and systemic therapy combinations, and safety and toxicity. The present review summarizes the advances in systemic ICIs for the treatment of NSCLC patients with BM, discusses factors associated with efficacy and toxicity, and explores future directions.
1 Introduction
Brain metastases (BMs) are common among patients with lung cancer, and the occurrence of BMs has steadily increased with the prolonged survival time. Central nervous system (CNS) metastases, including BMs and leptomeningeal metastases, are present in approximately 10%–20% of non-small cell lung cancer (NSCLC) patients at initial diagnosis. It has been postulated that approximately 25%–40% of NSCLC patients will develop a BM during the course of the disease (Metro et al., 2015). Emerging evidence suggests that patients with epidermal growth factor receptor (EGFR) gene mutation–positive NSCLC are particularly prone to BMs, with the frequency of patients with BM ranging from 44% to 63% (Bhatt et al., 2013). For patients harboring EGFR or ALK mutations, TKIs have a powerful ability to penetrate the blood–brain barrier and a high potency regarding controlling BM.
For patients without driver mutations, radiation therapy, including stereotactic radiosurgery or stereotactic fractionated radiotherapy (RT), usually combined with systemic chemotherapy, is the main treatment modality. The median survival of NSCLC patients with BM is 6 months with current treatments, but the results vary depending on tumor histology, disease control, patient age, and initial therapy response.
Immune checkpoint inhibitors (ICIs) have changed the management of advanced NSCLC. However, the exclusion of patients with active BMs from most ICI trials precludes the generalization of results. Accordingly, a variety of appropriate real-world studies and clinical trials are being developed to evaluate tumor response.
ICI-induced neurotoxicity is a highly relevant issue because these compounds can enhance immune responses, not only against the tumor but also against self-antigens (McFaline-Figueroa and Lee, 2021). The most common mechanism of neurotoxicity is autoimmunity, and hypophysitis is the most common complication following ipilimumab treatment (up to 17% of patients) (McFaline-Figueroa and Lee, 2021). Conversely, neurologic complications associated with antiPD1 or anti–programmed death ligand 1 (PD-L1) antibody treatment are rare but include acute or chronic inflammatory demyelinating polyneuropathy, myasthenia gravis, and polymyositis. Dual blockade using antiCTLA4 and antiPD1 or anti–PD-L1 antibodies appears to increase the risk of brain edema and headache, but whether it also increases the risk of major immune neurological complications is unclear (Tran et al., 2019; McFaline-Figueroa and Lee, 2021).
In this review, we provide an overview of recent studies using ICIs to treat BMs and discuss how these results are challenging previous paradigms and current clinical practice. Our purpose is to critically summarize advances to date regarding the role and acceptable toxicity of systemic ICIs for the treatment of NSCLC patients with BMs. We also discuss the biological mechanism of systemic therapy combinations. The advances we describe are expected to change the management of NSCLC patients, but further research is needed to address concerns regarding the appropriate sequencing of local and systemic therapy combinations.
2 Clinical Outcomes of ICBs in NSCLC With BMs
2.1 Immunotherapy Monotherapy
Advanced NSCLC patients with PD-L1–positive (PD-L1 TPS ≥1%) were enrolled in KEYNOTE-001 (NCT01295827), KEYNOTE-010 (NCT01905657), KEYNOTE-024 (NCT02142738), and KEYNOTE-042 (NCT02220894) and then assigned to single pembrolizumab or docetaxel groups. Hazard ratios (HRs) for overall survival (OS) and progression-free survival (PFS) were <1.0 for pembrolizumab vs. chemotherapy, irrespective of baseline BM status. These results showed that pembrolizumab could provide clinical benefit for patients with BMs, such that it has become the standard treatment in patients with advanced PD-L1–positive NSCLC, irrespective of BM status at baseline (Reck et al., 2016; Mansfield et al., 2019).
One prospective phase II trial with pembrolizumab specifically addressed the efficacy of ICIs in patients with BMs, reporting a 29.4% intracranial objective response rate (ORR) in the PD-L1–positive cohort, which was similar to the extracranial ORR (Goldberg et al., 2016). To date, the most robust evidence on the activity of pembrolizumab for the treatment of NSCLC BMs comes from a phase II trial (NCT02085070) that included melanoma and NSCLC patients (18 NSCLC patients and 18 melanoma patients). The diameter of BMs was restricted to between 5 and 20 mm, and patients had to be steroid free and neurologically asymptomatic. In the NSCLC arm, the status of PD-L1 in tumor tissue had to be positive (≥1%). Eight of the 18 NSCLC patients had received no prior local therapy for BMs. Six of 18 patients (33%) achieved an intracranial response. Systemic RR was 33%, with only one patient suffering progression in the CNS while responding systemically. All other responses were concordant (Goldberg et al., 2020). The above data showed that pembrolizumab monotherapy could provide excellent intracranial response for patients with PD-L1 ≥1%.
Nivolumab, another anti-PD1 agent, has been affirmed as second-line standard treatment basing on the CheckMate017 and 057 studies. In order to evaluate the efficacy of nivolumab in patients with BMs, a pooled analysis including patients with NSCLC and pretreated stable BMs who were enrolled in three nivolumab clinical trials (CheckMate 063, 017, and 057) was conducted. A total of 46 patients with BMs in the nivolumab group and 42 patients receiving docetaxel as second-line treatment were analyzed. Most patients had been previously treated with brain RT (74% in the nivolumab group and 83% in the docetaxel group). The median time to new BM occurrence and median survival time were 8 and 8.4 months, respectively, in the nivolumab group and 9 and 6.2 months, respectively, in the docetaxel group (Antonia et al., 2019).
Another retrospective study enrolled 5 patients with asymptomatic and corticosteroid-free BMs before nivolumab initiation. Two intracranial responses were observed, which were maintained for up to 24 and 28 weeks. Notably, intracranial and systemic responses were largely concordant, except in one patient in whom stable CNS disease was associated with rapid systemic progression (Dudnik et al., 2016). Real-world data from 38 patients with squamous NSCLC and asymptomatic BM were also published. One patient obtained CR, 6 patients achieved partial response (PR), and 11 achieved stable disease after nivolumab treatment (Bidoli et al., 2016). In an Italian clinical trial, 409 NSCLC BM patients were treated with nivolumab. The Kaplan-Meier method was used to estimate PFS and OS. Overall RR was 17% in patients with BMs and 18% in the entire cohort. Median PFS and OS were 3 and 8.6 months in patients with BMs and 3 and 11.3 months in the entire cohort, respectively (Crinò et al., 2019). These above trials showed that nivolumab also provides clinical benefit for NSCLC with BMs.
With regard to the activity of anti–PD-L1 ICI therapy in patients with BMs, the OAK trial evaluated the efficacy in the BM subgroup. The results showed that atezolizumab was superior to docetaxel in terms of delaying the appearance of new symptomatic BMs (HR 0.38, 95% confidence interval [CI]: 0.16–0.91) (Rittmeyer et al., 2017; Fehrenbacher et al., 2018; Gadgeel et al., 2019).
Some reports, however, have suggested that the RR of BMs in real-world settings might be lower than in more-selective clinical trial populations (Kim et al., 2019). Hendriks showed that the ORRs were similar: 20.6% in the BM group and 22.7% in non-BM group. However, the disease control rate (DCR) was significantly lower in patients with BMs, 43.9% vs. 52.0%. The median PFS was 1.7 and 2.1 months in the BM and non-BM groups, respectively. The median OS of patients with BMs was 8.6 months, compared with 11.4 months for patients with no BMs (p = 0.035). In the BM subgroup multivariate analysis, stable BMs and higher diagnosis-specific graded prognostic assessment (DS-GPA) classification were associated with improved OS (Hendriks et al., 2019).
The safety of ICIs alone in patients with BMs has been reported in several pooled analyses including data from five clinical trials. These studies showed that among 1,452 patients, serious neurological AE incidence was higher in patients with BMs compared to those without BMs (6% vs. 3%), but none reached grade 4. Thus, the abovementioned results suggest that PD-L1–positive NSCLC patients with BMs could benefit more from treatment with ICIs, with no relevant serious safety differences reported (Lukas et al., 2017). Clinical trials of immunotherapy monotherapy for NSCLC with BMs are detailed in Table 1.
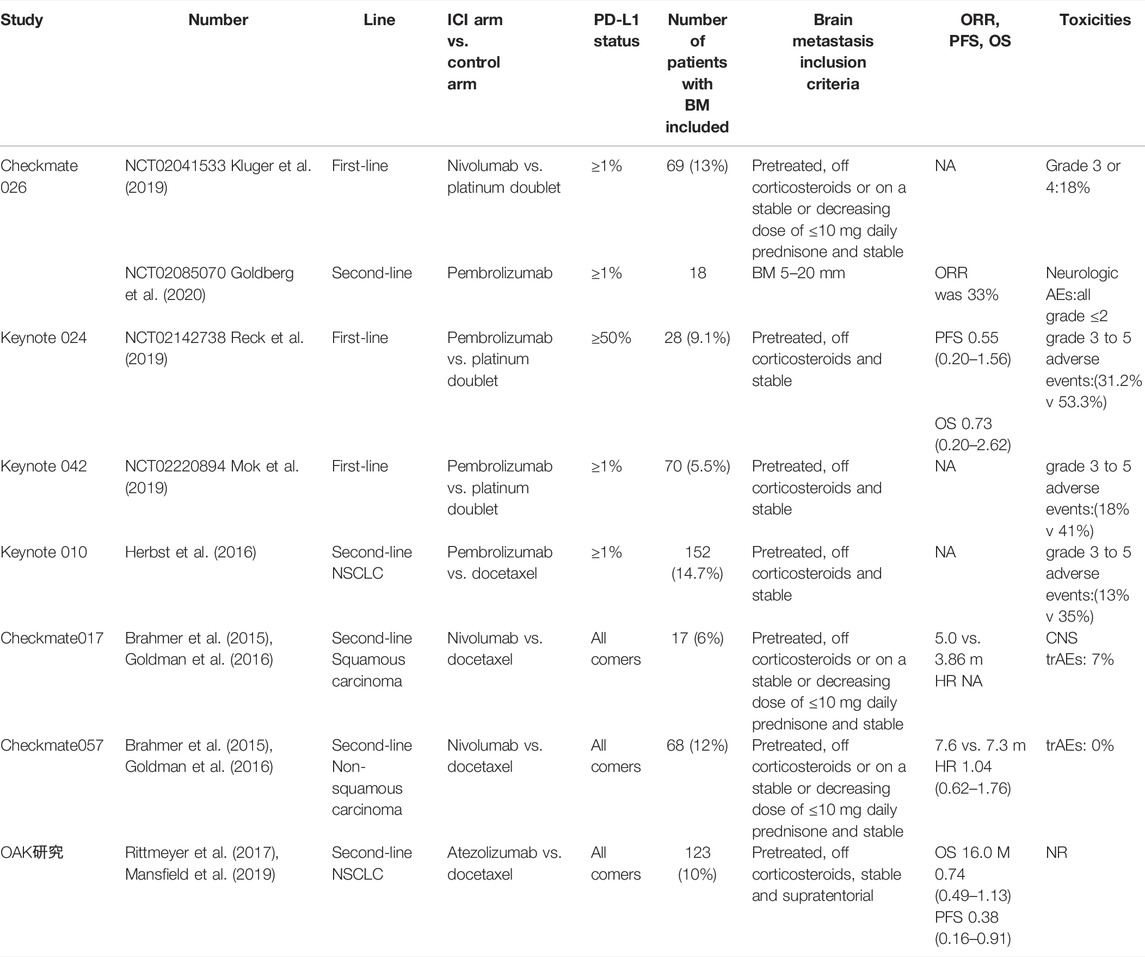
TABLE 1. Clinical investigations of Immunotherapy monotherapy in the treatment of NSCLC patients with brain metastases.
These data demonstrate that the efficacy of ICIs alone in patients with NSCLC with BMs is limited. ICI therapy combined with other treatments such as chemotherapy, radiation therapy, and anti-angiogenic therapy may be more clinically advantageous for this special subgroup. The possible mechanism and pathway of ICI combined modalities are summarized in Figure 1. The mechanism of combined modalities and their clinical outcomes are discussed below.
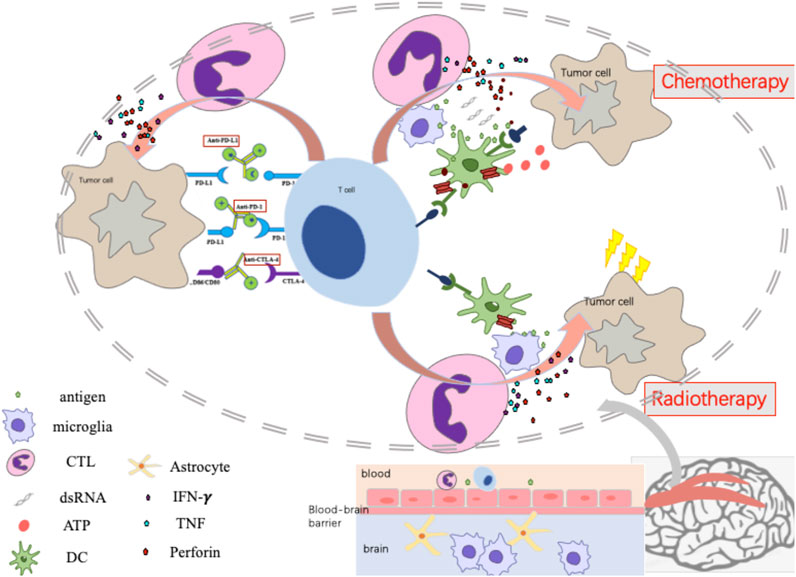
FIGURE 1. The mechanism of Immunotherapy monotherapy, Immune-checkpoint inhibitor combined with Chemotherapy or Radiotherapy.
2.2 ICI Therapy Combined With Chemotherapy
Chemotherapy is known to promote tumor immunity in two major ways, via inducing immunogenic cell death and disrupting the ability of tumors to evade the immune response. This process involves the concomitant release of tumor antigens during cell death. Anthracyclines activate expression of the pattern recognition receptor Toll-like receptor-3, the rapid secretion of type I interferons (IFNs), and release of the chemokine CXCL10. The type I IFN gene signature predicts the response to anthracycline therapy in breast cancer patients (Sistigu et al., 2014). Phylogenetically conserved chemokine signaling via CXCL8 increases the exposure of calreticulin on the tumor cell surface, which is critical for dendritic cells (DCs) to recognize and engulf dying tumor cells (Sukkurwala et al., 2014). Immunogenic chemotherapy–induced cell death also induces autophagy (Michaud et al., 2011) and necroptosis (Inoue and Tani, 2014). In addition to inducing immunogenic cell death and type I IFN secretion, anthracyclines promote the recruitment of CCL2/CCR2–dependent functional antigen-presenting cells (APCs) into tumor sites but not into tumor-draining lymph nodes (Ma et al., 2014). Chemotherapy can enhance tumor antigen presentation by either upregulating the expression of tumor antigens themselves or antigen-bound MHC class I molecules. Alternatively, chemotherapy can upregulate the expression of costimulatory molecules (B7-1) or downregulate the expression of co-inhibitory molecules (PD-L1/B7-H1 or B7-H4) expressed on the tumor cell surface, enhancing the activity of effector T cells (Wargo et al., 2015). Therefore, treatment strategies combining and ICI with chemotherapy may have certain application value in the treatment of NSCLC BMs, but the choice of specific regimen needs further study.
The KEYNOTE series clinical trials (021, 189, and 407) showed that pembrolizumab combined with chemotherapy provides longer survival than chemotherapy alone, irrespective of the presence of BMs at baseline (Powell et al., 2019). In the whole study group, 13% (171/1298) had baseline BMs. Median (range) follow-up was 10.9 (0.1–35.1) months and 11.0 (0.1–34.9) months in patients with and without BMs, respectively. Among patients with BMs, pembrolizumab plus chemotherapy had a median OS of 18.8 months, chemotherapy had a median OS of 7.6 months, and median PFS was 6.9 and 4.1 months, respectively. In the patients with BMs, grade 3–5 AEs were observed in 81.4% of patients in the pembrolizumab plus chemotherapy group and 70.3% in the chemotherapy alone group. In patients without BMs, the rate of AEs was lower than in patients with BMs: 68.3% and 65.6% in pembrolizumab plus chemotherapy group and chemotherapy alone group, respectively. Thus, pembrolizumab plus chemotherapy provides clinical benefit for patients with NSCLC, regardless of the presence/absence of stable BMs (Powell et al., 2019). The KEYNOTE-189 trial included some patients with previously treated stable and untreated asymptomatic BMs (with no lesions larger than 1.5 cm). It was recently reported that the benefit of the combination in terms of PFS and OS was confirmed in the subgroup of patients with BMs: HR (95% CI) of 0.42 (0.27–0.67) and 0.41 (0.24–0.67), respectively. Notably, the magnitude of benefit attributable to the addition of pembrolizumab was greater in patients with BMs than in those without CNS involvement (Gandhi et al., 2018). A real-world retrospective cohort experience with the same combination of drugs reported similar results (Afzal et al., 2018). The results of clinical trials of ICI combined with chemotherapy for NSCLC with BMs are detailed in Table 2.
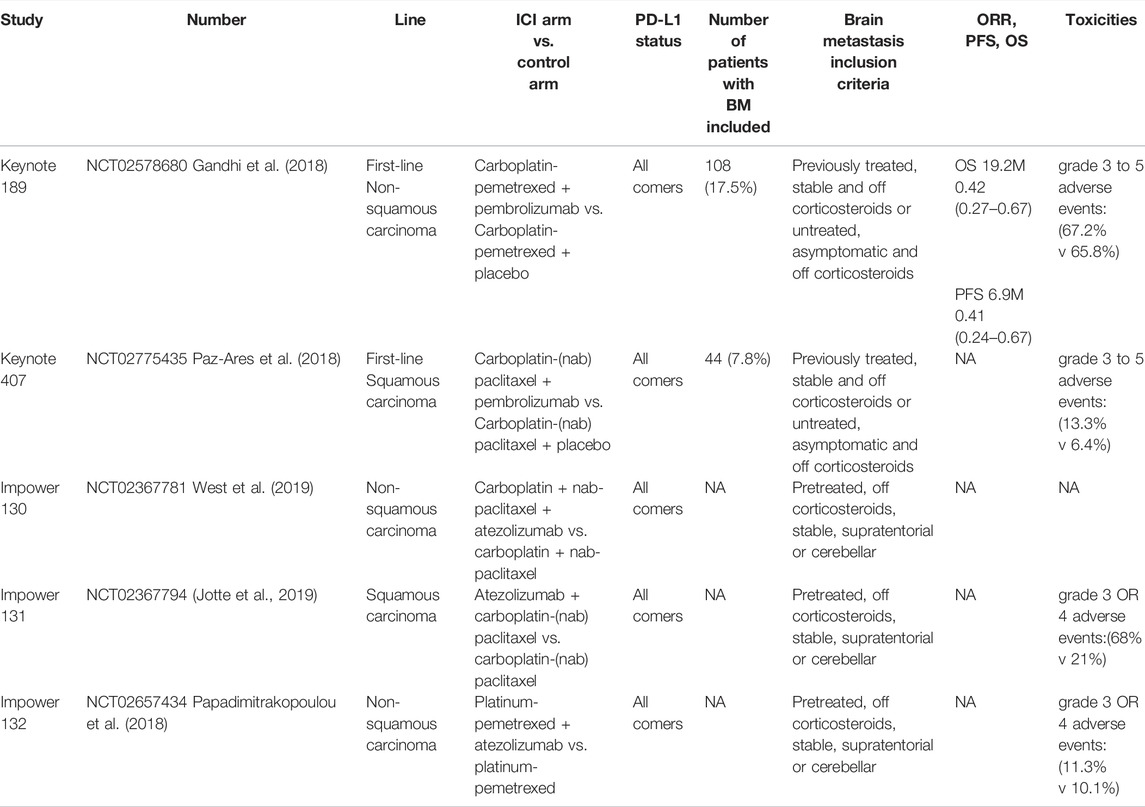
TABLE 2. Clinical investigations of ICI combined with chemotherapy in the treatment of NSCLC patients with brain metastases.
2.3 Combination ICI and RT
RT induces the immunogenic death of cancer cells, which is characterized by the transfer of calreticulin to the surface of dying tumor cells together with high-mobility group box-1 and adenosine triphosphate release. The dying cells release danger-associated molecular patterns (DAMPs) and promote the transfer of tumour-associated antigens to dendritic cells (DCs), and thus activate DC maturation (Sistigu et al., 2014). RT synergistically promotes tumor antigen uptake and cross-presentation by DCs to T cells in the draining lymph nodes. Radiation can prompt a rapid increase in the number of tumor-infiltrating CD8+ T cells. Tumor-derived DNA activates DNA-sensing pathways to induce IFNβ production by DCs, which is required for DC activation. Simultaneously, activation of transforming growth factor β (TGFβ) and colony-stimulating factor 1 increases infiltration of T regulatory cells (Tregs) and myeloid-derived suppressor cells (MDSCs) (DemariaGolden and Formenti, 2015). From the perspective of the abscopal effect, the migration of T cells primed against TAA could enhance the anti-tumor immune response to other lesions with similar antigens, and multi-focal RT may promote this effect more effectively (Brooks and Chang, 2019). In addition, one study suggested that for NSCLC patients with negative PD-L1 expression in the primary origin, RT may lead to positive changes in BMs (Takamori et al., 2017). In summary, RT can affect immunotherapy through a variety of pathways.
There is a strong rationale behind the combination of RT and ICI, both preclinical and clinical. The rationale behind the combination originally stemmed from the observation of the abscopal effect. The abscopal effect is a phenomenon in which radiation at one site leads to the regression of metastatic cancer at a distant site that has not been exposed to any radiation. Before the era of clinical cancer immunotherapy, anecdotal reports of the abscopal effect suggested that the response of tumors distant from the irradiation field could be immune-mediated (Abuodeh et al., 2016). This hypothesis was supported by loss- and gain-of-function experiments using T-cell–deficient athymic nude mice and DC enhancers, respectively (Demaria et al., 2004). Some preliminary reports suggested that immune enhancers could significantly contribute to abscopal responses in patients with a variety of solid cancers (Golden et al., 2015), but confirmatory results for combining modern ICI and RT on BM are still awaited. Moreover, additional complexity is suggested by trials in which preclinical and clinical data suggest that appropriate timing and dosing of irradiation might be critical for the induction of an effective anti-tumor immune response (Vanpouille-Box et al., 2017; Minniti et al., 2019).
Synergism or additivity of ICI combined with RT can be assessed in the CNS. Increased PD-L1 expression in resected BM specimens after radiation supports this potential interplay (Takamori et al., 2018). Interestingly, a variety of retrospective analyses from observational studies have suggested that the combination of RT and ICI therapy could have a positive impact on patient survival over exclusively systemic treatment (Shaverdian et al., 2017; Chen et al., 2018; Karivedu et al., 2018).
One retrospective study evaluated concurrent ipilimumab therapy and stereotactic radiosurgery (SRS) in 46 patients with 113 BMs. The results showed that SRS during or before treatment with ipilimumab provided better OS and less regional recurrence compared with patients treated with SRS lately (Kiess et al., 2015). Another group analyzed 25 patients with 58 BMs and found increased time to regional brain control and CNS progression when SRS was delivered within 30 days of immunotherapy, compared with patients not treated simultaneously (Skrepnik et al., 2017). A study that analyzed over 560 intracranial lesions in 75 patients with melanoma BMs, provided perhaps the best evidence for the benefit of concurrent therapy. The authors of that study found that concurrent therapy involving SRS and immunotherapy significantly decreased the lesion volume compared with non-concurrent treatment (Qian et al., 2016). Although most of the literature suggests that concurrent therapy is beneficial, it should be noted that no benefit was found in another study involving 35 patients (Liniker et al., 2016). The above data demonstrate that SRS combined with an ICI is a useful modality for patients with BMs.
Some studies have explored which NSCLC patients with BMs could obtain the best benefit from SRS combined with an ICI. Singh et al. reported that Karnofsky performance status (KPS) score <80 (p = 0.001) and lung-specific molecular marker graded prognostic assessment (lung molGPA) score <1.5 (p = 0.02) were the inferior predictors of OS. There was no significant benefit in terms of either OS or total degree of lesional response in patients with both KPS score <80 and lung molGPA score <1.5 when anti–PD-1 was combined with SRS. However, in lesions with a volume >500 mm3, combining SRS with an ICI resulted in faster and better volumetric response, which was particularly beneficial for patients with mass effects (Singh et al., 2019).
Recently, the fraction of radiation was evaluated to elucidate the relationship of them and AEs in a retrospective single-center analysis. Of 163 patients, 50 (31%) patients received an ICI, while 113 (69%) were ICI-naïve. Overall, 94 (58%), 28 (17%), and 101 (62%) patients received SRS, partial brain irradiation, and/or whole-brain RT (WBRT), respectively. Fifty percent of patient received more than one radiation course. No significant differences in rates of all-grade AEs or grade ≥3 AEs between ICI-naïve and ICI-treated patients were observed across different cranial RT types (grade ≥3 AEs: 8% ICI− vs. 9% ICI + for SRS [p = 1.00]; 8% ICI− vs. 10% ICI + for WBRT [p = 0.71]). Additionally, there were no differences in AE rates based on the timing of ICI administration with respect to RT (Ahmed et al., 2017). However, a small-sample study found an association between receipt of immunotherapy and symptomatic radiation necrosis (RN) in patients with BMs undergoing stereotactic radiation (HR: 2.56; 95% CI: 1.35–4.86; p = 0.004) (Nimmerjahn et al., 2005a).
Another retrospective study from a small series supported the hypothesis that the neurological toxicity of an ICI combined with brain RT is manageable, with no patients undergoing surgical resection for symptomatic RN among 17 patients who received SRT and nivolumab or durvalumab (Martin et al., 2018a). However, a recently published retrospective evaluation showed that, treatment with an ICI was associated with a significantly increased risk of symptomatic RN, regardless of tumor histology. Notably, a tendency toward increased symptomatic RN remained, but statistical significance was not reached if patients who received ipilimumab were excluded from the analysis (Kluger et al., 2019). Of note, the median time to RN after SRT in patients treated with immunotherapy was about 10 months. Therefore, the patients with prolonged survival could have biased the increased RN incidence. An innovative clinical trial (NCT02681549) that is currently recruiting patients with melanoma and NSCLC BMs intends to evaluate whether bevacizumab in combination with pembrolizumab is capable of reducing brain edema and RN incidence while potentially synergizing with immune cell trafficking (Colaco et al., 2016; Kluger et al., 2019).
2.4 Combination of ICI and Anti-Angiogenic Drugs
The growth and metastasis of a tumor depends on the formation of blood vessels for nutrition, and anti-angiogenic therapy can reduce the oxygen content in tumors and thereby delay tumor growth. Normalization of blood vessels is beneficial for sensitization to chemotherapy and RT. It can also reduce cerebral edema in patients with BMs. In addition, anti-angiogenic therapy is performed by inhibiting endothelial cell proliferation, thus eliminating the need to cross the blood-brain barrier (BBB) (Carbone et al., 2017).
Vascular endothelial growth factor (VEGF) exerts a systemic effect on immune-regulatory cell function via multiple mechanisms. VEGF induces the proliferation of inhibitory immune-cell subsets, such as Tregs and MDSCs, inhibits DC maturation, and inhibits T-cell development from hematopoietic progenitor cells (Gabrilovich et al., 1998; Shojaei et al., 2007). MDSCs also secret angiogenic factors such as Bombina variegata peptide 8 and VEGF, upregulating STAT3 to promote tumor metastasis (Feng et al., 2018). Therefore, anti-VEGF antibodies may promote the normalization of tumor blood vessels, reshaping the tumor microenvironment and enhancing the effect of ICIs (Chongsathidkiet et al., 2019). Immunotherapy combined with anti-angiogenic therapy has complementary effects: anti-angiogenic therapy plays a role in antigen recognition, immune cell recruitment, and remodeling of the immune microenvironment, whereas immunotherapy can restore immune function (Khan and Kerbel, 2018).
In recent years, the safety and efficacy of bevacizumab as an anti-angiogenic agent in the treatment of NSCLC with BMs have been recognized (Socinski et al., 2018). The results of a phase III clinical trial, IMPOWER 150, were presented at ASCO 2020. This study demonstrate that, compared with ACP (atezolizumab plus carboplatin plus paclitaxel) group, the incidence of new BMs was lower in the atezolizumab plus bevacizumab plus carboplatin plus paclitaxel (ABCP) and bevacizumab plus carboplatin plus paclitaxel (BCP) groups that received bevacizumab, and the results were similar between the two groups (7% and 6%). A tendency toward delayed onset of BM was observed in the ABCP group. The study’s data suggested that the addition of atezolizumab to BCP may not reduce the incidence of new BMs, but may delay TTD (time to development). In conclusion, bevacizumab, especially when combined with an ICI, plays a definite role in delaying the occurrence of BMs in patients with metastatic non-squamous NSCLC (Federico et al., 2020). The international, randomized, double-blind phase III study, ONO-4538-52/TASUKI-52, evaluated nivolumab with bevacizumab and cytotoxic chemotherapy as first-line treatment for nonsquamous non-small-cell lung cancer (NSCLC). Among patients with BMs subgroup, nivolumab arm had a median PFS of 10.6 months, placebo arm had a median PFS of 7.1 months. Potential benefits have been observed in patients with BMs, but these results need to be confirmed by prospective studies (D’après Lee, 2020).
2.5 Combination ICIs
Combination ICI therapy, such as the combination of anti–CTLA-4 and anti–PD-1, aims to recruit T cells into tumors and then prevent them from being “turned off.” CTLA4 blockade induces frequent increases in intratumoral T cell infiltration beyond its clinical response rate (Zhou et al., 2018a), and concurrent ipilimumab plus nivolumab in melanoma has shown promise (Long et al., 2017; Martin et al., 2018b).
CheckMate 012 was a phase I multi-cohort study evaluating the safety and tolerability of nivolumab alone or in combination with other therapies in patients with advanced NSCLC. The study analyzed 12 patients with at least one asymptomatic and untreated BM. Intracranial responses occurred in 2 patients (iCR: 16.7%; 95% CI: 2.1–48.4); the median PFS was 1.6 months (95% CI: 0.92–2.50), and the median OS was 8.0 months (95% CI: 1.38–15.50). No treatment-related neurological adverse events were reported. The median OS was longer than chemotherapy. Therefore, combined ICI treatment might also result in favorable outcomes for patients with NSCLC and BMs (Goldman et al., 2016; Hellmann et al., 2019; Reck et al., 2020). However, a large-sample clinical trial is needed, and the irAEs need more attention.
3 Factors Related to the Efficacy of Immunotherapy for BMs
3.1 BBB
Because the immune system in the brain differs from that of the rest of the body, access to the tumors is limited by the BBB, and the host is subject to substantial endogenous and treatment-induced immunosuppression (Figure 2). The BBB is a dynamic interface between the systemic blood circulation and the CNS that selectively blocks the passage of harmful substances to keep them out of the brain tissue. The neurovascular unit is the basic unit of the BBB and includes endothelial cells, pericytes, and astrocytic end-feet (Kim et al., 2016; Iadecola, 2017). Multiple signaling pathways determine the role of the BBB, such as oligodendrocyte precursor cells that reduce BBB leakage via the Wnt/β-catenin pathway (Van Steenwinckel et al., 2019; Wang et al., 2020). The sonic hedgehog protein secreted by CNS astrocytes, increases the protein level of netrin-1 to regulate features of the vascular architecture and function (Alvarez et al., 2011; Arvanitis et al., 2020). TGF-β/Smad signaling plays a unique role in maintaining cerebrovascular integrity (Li et al., 2011). In addition, lipopolysaccharide can increase the permeability of endothelial cells (Haileselassie et al., 2020). New research has overturned the idea that the BBB prevents immune cells from entering the brain and demonstrated the entry of T cells and immune monitoring within the brain. Similarly, in the case of gliomas and other tumors, damage to the BBB limits the protection that it normally provides (Owens et al., 2008).
The brain is considered an immune-privileged site due to the presence of the BBB, and this may inhibit the therapeutic efficacy of ICIs. Because ICIs block the inhibitory signals of T-cell activation, T cells are able to respond against tumors. However, due to poor prognosis and risk of pseudoprogression of brain tumors, patients with untreated or active BMs are systematically excluded from clinical trials of ICIs.
3.2 PD-L1 Expression in BMs
Activation of the PD-1 pathway is a common mechanism that blunts the killing function of effector T cells by tumor cells and immune cells in their microenvironment, and this is an important pathway for tumor immune escape (termed adaptive immune resistance) (Tumeh et al., 2014). PD-L1 (B7-H1) emerged early as a marker associated with PD-1 inhibition and is widely expressed in different tumor types (Taube et al., 2014). Similar to other metastatic tumors, there is often spatio-temporal heterogeneity in tumor immunity between BMs and their lung primary tumors. Mansfield analyzed 146 paired primary lung cancers and BMs from 73 cases. There was a difference in tumor cell PD-L1 expression in 10 cases (14%) and a difference in TIL PD-L1 expression in 19 cases (26%). These data suggest that heterogeneity between intracranial and extracranial lesions should be considered (Mansfield et al., 2016). Zhou found that in patients with BMs, in addition to 32% of patients expressing PD-L1 on TCs and ICs, patients simultaneously expressing PD-L1 only on TCs or ICs also had different groups (for example, 8% of patients expressed PD-L1 only on ICs). Further analysis of PD-L1 overexpression patterns showed that only 4% of patients with primary lesions exhibited high expression of PD-L1 on both TCs and ICs, whereas 16% of patients with BMs had strong positive expression of PD-L1 on both TCs and ICs (Zhou et al., 2018b).
One pooled analysis from seven European cancer centers included NSCLC patients who were treated with ICIs in a variety of settings, including daily standard practice. Among patients with active BMs that were evaluable in the CNS (n = 73), the intracranial RR was 27.3%. Among the 23 patients with active BMs and available PD-L1 expression status, positive expression of PD-L1 (≥1%) was associated with a higher intracranial RR, 35.7% vs. 11.1% in PD-L1–negative patients (Federico et al., 2020). Recently, updated data from 34 patients with PD-L1–positive tumors and 5 patients with PD-L1–negative disease showed that CNS RR was 10/34 (29.4%) in PD-L1–positive patients, with a median duration of response of 10.7 months. Discordance was observed between intracranial and systemic responses in 7 patients. Among these, 4 individuals experienced PD in the brain and PR systemically, whereas the remaining 3 patients exhibited the opposite findings. Interestingly, no intracranial responses were observed among patients with PD-L1–negative tumors (Goldberg et al., 2018).
3.3 BM Microenvironment Immunobiology
The CNS is armed with resident myeloid cells such as microglia, which are innate immune cells in the CNS, the brain’s equivalent of tissue-resident macrophages, and perivascular macrophages that colonize the CNS in early development and maintain homeostasis in the brain parenchyma and at brain–blood vessel interfaces (Owens et al., 2008). Microglia and macrophages are different from tumor cells in that they are involved in multiple stages of disease metastasis and are genetically stable and predictable. Under physiological conditions, microglia participate in regulation of the nervous system by phagocytosis and removal of apoptosis debris and do not cause inflammation, thereby contributing to CNS homeostasis (Nimmerjahn et al., 2005b; Glass et al., 2010; Ginhoux et al., 2013). CD49D is considered a good flow cytometry marker for the identification of microglia and peripheral macrophages in human brain tumors (Bowman et al., 2016). Cancer cells increase their proliferation and survival by polarizing microglia and infiltrating surrounding macrophages through a variety of mechanisms (Raza et al., 2018).
CNS tumors exhibit a low number of tumor-infiltrating lymphocytes (TILs) and other immune effector cell types that are different from other tumor types. Although both primary and metastatic brain malignancies can subvert and manipulate immune response, their immune landscapes are of great significance. In general, metastatic brain tumor has higher level of T cells and neutrophils infiltration compared with primary brain tumors including glioblastoma and gliomas, which were considered as “immune-cold” tumors (Klemm et al., 2020; Sampson et al., 2020). Primary brain tumors also have lower TMB compared to brain metastatic tumors including NSCLC and melanomas (Alexandrov et al., 2013). As result, patients with primary brain tumor shows inferior response to ICI treatment compared to NSCLC or melanoma patients with brain metastasis (Tawbi et al., 2018; Cloughesy et al., 2019). This “cold tumor” phenotype is associated with adverse reactions to immune-stimulating therapies, such as immune checkpoint blockade (Gajewski et al., 2017). For decades, brain has been regarded as one of the “immune privileged” organs in body, yet, the discovery of functional lymphatic vessels in meninges in 2015 suggested that the CNS immune privilege paradigm is overstated (Aspelund et al., 2015). However, as a number of mechanisms have been evolved by brain to restrict immune response, which may be harmful, many have suggested that brain should be considered as a “immune distinct” organ (Kipnis, 2016). Even when T-cell responses are induced by means such as vaccination, the number of antigen-specific TILs is still very low, and those that are present often exhibit a depleted phenotype (Keskin et al., 2019). Due to the unique immune microenvironment of the brain, the decrease in the number and activity of T cells in CNS tumors is largely limited. Uncontrolled inflammation in the brain poses a threat that inflammation in peripheral organs does not because of the brain’s rigid outer shell and the potential for damage from elevated intracranial pressure. Thus, the CNS may have evolved into a special immune microenvironment in which both inflammation and adaptive immune responses are tightly regulated. This regulation involves a variety of mechanisms at the molecular and cellular levels (Quail and Joyce, 2017).
Tumor-associated macrophages (TAMs) and microglia communicate significantly with tumor cells in the brain. Brain tumor cells release cytokines and chemokines to recruit TAMs into the microenvironment, and TAMs in turn provide tumor-promoting and survival factors. DCs can present tumor antigens to T cells to induce an anti-tumor immune response. Increased neutrophils in brain tumor tissue are associated with bevacizumab resistance and the development of high-grade gliomas. Tregs can inhibit cytotoxic T cells, leading to an immunosuppressive microenvironment that allows tumor growth. Astrocytes are unique to the CNS and act as physical channels for signaling molecules in a heterogeneous manner (Quail and Joyce, 2017). The mechanism of immuno-resistance was showen in Figure 3.
4 Future Perspectives for NSCLC With BMs
Drug delivery faces major challenges due to the presence of the BBB (Tang et al., 2019). Extracellular vesicles (EVs), including all membrane-derived vesicles located outside the cell, have developed into important intercellular communication agents (Tkach and Théry, 2016). The in vivo efficacy of exosome delivery of anticancer drug into the brain was studied in zebrafish embryos (Yang et al., 2015). Moreover, exosomes were capable of delivering siRNA to the brain, across the BBB in mice (Alvarez-Erviti et al., 2011). Many studies have indicated that exosomes can function in innate and adaptive immune responses (Kurywchak et al., 2018). Tumor-derived exosomes and immune-cell-derived exosomes enhance anti-tumor responses by activating antigen-specific T-cell responses by transferring antigens to APCs. Our recent studies have shown that EVs contribute to the progression of a variety of brain diseases, including BM, and they are thus considered promising therapeutic and drug delivery vectors. Many studies have determined that tumor-derived EVs can disrupt the intact BBB in vivo. Further clinical studies are needed to investigate the optimal chemotherapy regimens, appropriate doses, and fraction of RT when combined with immunotherapy to treat BMs (Table 3).
5 Conclusion
ICIs are challenging the traditional idea that monoclonal antibodies are marginally active against BMs. There is encouraging preliminary evidence to support the efficiency of ICIs alone or combined with other treatments. Based on current evidence, ICIs play a relevant role in acquisition of long-term disease control in the CNS of patients with advanced NSCLC. Despite the encouraging efficacy of ICIs in treating BMs, other modalities that can enhance the activity of anti–PD-1/PD-L1 monotherapy are urgently needed, and prospective trials in NSCLC BM patients are warranted. Additionally, improved imaging modalities are needed to differentiate RN, pseudoprogression, and tumor re-growth in previously irradiated lesions in order to identify patients who will ultimately obtain clinical benefit from the systemic delivery of ICIs.
Author Contributions
HZ and JY designed the study. JL drafted the manuscript. JL, MW, SX, YL, and JTL coordinated, edited, and finalized the drafting of the manuscript. All authors read and approved the final manuscript.
Funding
This study was supported by Innovation Project of Shandong Academy of Medical Sciences (2019-04) and the Academic Promotion Program of Shandong First Medical University (grant number: 2019ZL002); the National Natural Science Foundation of China (grant number: 81972862).
Conflict of Interest
The authors declare that the research was conducted in the absence of any commercial or financial relationships that could be construed as a potential conflict of interest.
Publisher’s Note
All claims expressed in this article are solely those of the authors and do not necessarily represent those of their affiliated organizations, or those of the publisher, the editors and the reviewers. Any product that may be evaluated in this article, or claim that may be made by its manufacturer, is not guaranteed or endorsed by the publisher.
Acknowledgments
The authors would such as to express their great thanks to the Innovation Project of the Shandong Academy of Medical Science.
Abbreviations
APCs, antigen-presenting cells; ATP, adenosine triphosphate; BBB, blood brain barrier; CTLA-4, cytotoxic T lymphocyte-associated antigen 4; CXCL10, CXC-motif chemokine ligand 10; DCs, dendritic cells; EVs, extracellular vesicles; IEXs, immune cell-derived exosomes; MDSCs, myeloid-derived suppressor cells; NSCLC, non-small cell lung cancer; OS, overall survival; PD-1, programmed cell death 1; PD-L1, programmed death-ligand 1; PFS, progression-free survival; PR, partial response; SD, stable disease; TAMs, tumor-associated macrophages; TEXs, tumor-derived exosomes; TGFβ, transforming growth factor beta; treg cells, Regulatory T cells
References
Abuodeh, Y., Venkat, P., and Kim, S. Systematic Review of Case Reports on the Abscopal Effect. Curr. Probl. Cancer. 2016;40(1):25–37. doi:10.1016/j.currproblcancer.2015.10.001
Afzal, M. Z., Dragnev, K., and Shirai, K. A Tertiary Care Cancer center Experience with Carboplatin and Pemetrexed in Combination with Pembrolizumab in Comparison with Carboplatin and Pemetrexed Alone in Non-squamous Non-small Cell Lung Cancer. J. Thorac. Dis. 2018;10(6):3575–3584. doi:10.21037/jtd.2018.06.08
Ahmed, K. A., Kim, S., Arrington, J., Naghavi, A. O., Dilling, T. J., Creelan, B. C., et al. Outcomes Targeting the PD-1/pd-L1 axis in Conjunction with Stereotactic Radiation for Patients with Non-small Cell Lung Cancer Brain Metastases. J. Neurooncol. 2017;133(2):331–338. doi:10.1007/s11060-017-2437-5
Alexandrov, L. B., Nik-Zainal, S., Wedge, D. C., Aparicio, S. A., Behjati, S., Biankin, A. V., et al. Signatures of Mutational Processes in Human Cancer. Nature. 2013;500(7463):415–421. doi:10.1038/nature12477
Alvarez, J. I., Dodelet-Devillers, A., Kebir, H., Ifergan, I., Fabre, P. J., Terouz, S., et al. The Hedgehog Pathway Promotes Blood-Brain Barrier Integrity and CNS Immune Quiescence. Science 2011;334:1727–1731. doi:10.1126/science.1206936
Alvarez-Erviti, L., Seow, Y., Yin, H., Betts, C., Lakhal, S., and Wood, M. J. Delivery of siRNA to the Mouse Brain by Systemic Injection of Targeted Exosomes. Nat. Biotechnol. 2011;29:341–345. doi:10.1038/nbt.1807
Antonia, S. J., Borghaei, H., Ramalingam, S. S., Horn, L., De Castro Carpeño, J., Pluzanski, A., et al. Four-year Survival with Nivolumab in Patients with Previously Treated Advanced Non-small-cell Lung Cancer: a Pooled Analysis. Lancet Oncol.,2019;20(10):1395–1408. doi:10.1016/S1470-2045(19)30407-3
Arvanitis, C. D., Ferraro, G. B., and Jain, R. K. The Blood-Brain Barrier and Blood-Tumour Barrier in Brain Tumours and Metastases. Nat. Rev. Cancer 2020;20:26–41. doi:10.1038/s41568-019-0205-x
Aspelund, A., Antila, S., Proulx, S. T., Karlsen, T. V., Karaman, S., Detmar, M., et al. A Dural Lymphatic Vascular System that Drains Brain Interstitial Fluid and Macromolecules. J. Exp. Med. 212, 991–999. (2015)doi:10.1084/jem.20142290
Bhatt, V. R., Kedia, S., Kessinger, A., and Ganti, A. K. Brain Metastasis in Patients with Non-small-cell Lung Cancer and Epidermal Growth Factor Receptor Mutations. J. Clin. Oncol. 2013;31(25):3162–3164. doi:10.1200/JCO.2013.49.8915
Bidoli, P., Chiari, R., Catino, A., Grossi, F., Noberasco, C., Gelsomino, F., et al. Efficacy and Safety Data from Patients with Advanced Squamous NSCLC and Brain Metastases Participating in the Nivolumab Expanded Access Programme (EAP) in Italy. Ann. Oncol. 2016;27(Suppl. l_6).doi:10.1093/annonc/mdw383.28
Bowman, R. L., Klemm, F., Akkari, L., Pyonteck, S. M., Sevenich, L., Quail, D. F., et al. Macrophage Ontogeny Underlies Differences in Tumor-specific Education in Brain Malignancies. Cell Rep. (2016) 17:2445–2459. doi:10.1016/j.celrep.2016.10.052
Brahmer, J., Reckamp, K. L., Baas, P., Crinò, L., Eberhardt, W. E., Poddubskaya, E., et al. Nivolumab versus Docetaxel in Advanced Squamous-Cell Non-small-cell Lung Cancer. N. Engl. J. Med. 2015;373:123–135. doi:10.1056/NEJMoa1504627
Brooks, E. D., and Chang, J. Y. Time to Abandon Single-Site Irradiation for Inducing Abscopal Effects. Nat. Rev. Clin. Oncol., 2019, 16(2): 123–135. doi:10.1038/s41571-018-0119-7
Carbone, D. P., Reck, M., Paz-Ares, L., Creelan, B., Horn, L., Steins, M., et al. First-Line Nivolumab in Stage IV or Recurrent Non-small-cell Lung Cancer. N. Engl. J. Med. 2017;376(25):2415–2426. doi:10.1056/NEJMoa1613493
Chen, L., Douglass, J., Kleinberg, L., Ye, X., Marciscano, A. E., Forde, P. M., et al. Concurrent Immune Checkpoint Inhibitors and Stereotactic Radiosurgery for Brain Metastases in Non-small Cell Lung Cancer, Melanoma, and Renal Cell Carcinoma. Int. J. Radiat. Oncol. Biol. Phys. 2018;100(4):916–925. doi:10.1016/j.ijrobp.2017.11.041
Chongsathidkiet, P., Jackson, C., Koyama, S., Loebel, F., Cui, X., Farber, S. H., et al. Author Correction: Sequestration of T Cells in Bone Marrow in the Setting of Glioblastoma and Other Intracranial Tumors. Nat. Med., 2019, 25(3): 529. doi:10.1038/s41591-019-0355-0
Cloughesy, T. F., Mochizuki, A. Y., Orpilla, J. R., Hugo, W., Lee, A. H., Davidson, T. B., et al. Neoadjuvant Anti-PD-1 Immunotherapy Promotes a Survival Benefit with Intratumoral and Systemic Immune Responses in Recurrent Glioblastoma. Nat. Med. 25, 477–486. (2019).doi:10.1038/s41591-018-0337-7
Colaco, R. J., Martin, P., Kluger, H. M., Yu, J. B., and Chiang, V. L.. Does Immunotherapy Increase the Rate of Radiation Necrosis after Radiosurgical Treatment of Brain Metastases? J. Neurosurg. 2016;125(1):17–23. doi:10.3171/2015.6.JNS142763
Crinò, L., Bronte, G., Bidoli, P., Cravero, P., Minenza, E., Cortesi, E., et al. Nivolumab and Brain Metastases in Patients with Advanced Non-squamous Non-small Cell Lung Cancer. Lung Cancer 2019;129:35–40. doi:10.1016/j.lungcan.2018.12.025
D’après Lee, J. S. Étude ONO-4538-52/TASUKI 52 : phase III comparant carboplatine paclitaxel bévacizumab associé au nivolumab ou à un placebo, ESMO 2020,abstr. LBA 54, actualisé.
Demaria, S., Ng, B., Devitt, M. L., Babb, J. S., Kawashima, N., Liebes, L., et al. Ionizing Radiation Inhibition of Distant Untreated Tumors (Abscopal Effect) Is Immune Mediated. Int. J. Radiat. Oncol. Biol. Phys. 2004;58(3):862–870. doi:10.1016/j.ijrobp.2003.09.012
Demaria, S., Golden, E. B., and Formenti, S. C. Role of Local Radiation Therapy in Cancer Immunotherapy. JAMA Oncol.,2015;1(9):1325–1332. doi:10.1001/jamaoncol.2015.2756
Dudnik, E., Yust-Katz, S., Nechushtan, H., Goldstein, D. A., Zer, A., Flex, D., et al. Intracranial Response to Nivolumab in NSCLC Patients with Untreated or Progressing CNS Metastases. Lung Cancer. 2016;98:114–117. doi:10.1016/j.lungcan.2016.05.031
Federico, C., Martin, R., Mark, A. S., Tony, S. K. M., and Fabrice, B. IMpower150: Exploratory Analysis of Brain Metastases Development. J. Clin. Oncol. 38, no.15_suppl (May 20, 2020) 9587–9587. doi:10.1200/JCO.2020.38.15_suppl.9587
Fehrenbacher, L., von Pawel, J., Park, K., Rittmeyer, A., Gandara, D. R., Ponce Aix, S., et al. Updated Efficacy Analysis Including Secondary Population Results for OAK: a Randomized Phase III Study of Atezolizumab versus Docetaxel in Patients with Previously Treated Advanced Non-small Cell Lung Cancer. J. Thorac. Oncol. 2018;13(8):1156–1170. doi:10.1016/j.jtho.2018.04.039
Feng, P. H., Chen, K. Y., Huang, Y. C., Luo, C. S., Wu, S. M., Chen, T. T., et al. Bevacizumab Reduces S100A9-Positive MDSCs Linked to Intracranial Control in Patients with EGFR-Mutant Lung Adenocarcinoma. J. Thorac. Oncol., 2018, 13(7): 958–967. doi:10.1016/j.jtho.2018.03.032
Gabrilovich, D., Ishida, T., Oyama, T., Ran, S., Kravtsov, V., Nadaf, S., et al. Vascular Endothelial Growth Factor Inhibits the Development of Dendritic Cells and Dramatically Affects the Differentiation of Multiple Hematopoietic Lineages In Vivo. Blood. 1998;92(11):4150–4166. doi:10.1182/blood.v92.11.4150.423k45_4150_4166
Gajewski, T. F., Corrales, L., Williams, J., Horton, B., Sivan, A., and Spranger, S. Cancer Immunotherapy Targets Based on Understanding the T Cell-Inflamed versus Non-T Cell-Inflamed Tumor Microenvironment. Adv. Exp. Med. Biol. 2017;1036:19–31. doi:10.1007/978-3-319-67577-0_2
Gadgeel, S. M., Lukas, R. V., Goldschmidt, J., Conkling, P., Park, K., Cortinovis, D., et al. Atezolizumab in Patients with Advanced Non-small Cell Lung Cancer and History of Asymptomatic, Treated Brain Metastases: Exploratory Analyses of the Phase III OAK Study. Lung Cancer. 2019;128:105–112. doi:10.1016/j.lungcan.2018.12.017
Gandhi, L., Rodríguez-Abreu, D., Gadgeel, S., Esteban, E., Felip, E., De Angelis, F., et al. Pembrolizumab Plus Chemotherapy in Metastatic Non-small-cell Lung Cancer. N. Engl. J. Med. 2018;378(22):2078–2092. doi:10.1056/NEJMoa1801005
Ginhoux, F., Lim, S., Hoeffel, G., Low, D., and Huber, T. Origin and Differentiation of Microglia. Front Cel Neurosci. (2013) 7:45. doi:10.3389/fncel.2013.00045
Glass, C. K., Saijo, K., Winner, B., Marchetto, M. C., and Gage, F. H. Mechanisms Underlying Inflammation in Neurodegeneration. Cell. (2010) 140:918–934. doi:10.1016/j.cell.2010.02.016
Goldberg, S. B., Schalper, K. A., Gettinger, S. N., Mahajan, A., Herbst, R. S., Chiang, A. C., et al. Pembrolizumab for Management of Patients with NSCLC and Brain Metastases: Long-Term Results and Biomarker Analysis from a Non-randomised, Open-Label, Phase 2 Trial. Lancet Oncol. 2020;21(5):655–663. doi:10.1016/S1470-2045(20)30111-X
Goldberg, S. B., Gettinger, S. N., Mahajan, A., Chiang, A. C., Herbst, R. S., Sznol, M., et al. Pembrolizumab for Patients with Melanoma or Non-small-cell Lung Cancer and Untreated Brain Metastases: Early Analysis of a Non-randomised, Open-Label, Phase 2 Trial. Lancet Oncol.,2016;17(7):976–983. doi:10.1016/S1470-2045(16)30053-5
Goldberg, S. B., Gettinger, S. N., Mahajan, A., Herbst, R. S., Chiang, A. C., Lilenbaum, R., et al. Durability of Brain Metastasis Response and Overall Survival in Patients with Non-small Cell Lung Cancer (NSCLC) Treated with Pembrolizumab. Jco. 2018;36(15_Suppl. l):2009, .doi:10.1200/jco.2018.36.15_suppl.2009
Golden, E. B., Chhabra, A., Chachoua, A., Adams, S., Donach, M., Fenton-Kerimian, M., et al. Local Radiotherapy and Granulocyte-Macrophage colony-stimulating Factor to Generate Abscopal Responses in Patients with Metastatic Solid Tumours: a Proof-Of-Principle Trial. Lancet Oncol. 2015;16(7):795–803. doi:10.1016/S1470-2045(15)00054-6
Goldman, J. W., Crino, L., Vokes, E. E., Holgado, E., Reckamp, K., Pluzanski, A., et al. P2.36: Nivolumab (Nivo) in Patients (Pts) with Advanced (Adv) NSCLC and Central Nervous System (CNS) Metastases (Mets). J. Thorac. Oncol. 2016;11:S238–S239. doi:10.1016/j.jtho.2016.08.107
Haileselassie, B., Joshi, A. U., Minhas, P. S., Mukherjee, R., Andreasson, K. I., and Mochly-Rosen, D. Mitochondrial Dysfunction Mediated through Dynamin-Related Protein 1 (Drp1) Propagates Impairment in Blood Brain Barrier in Septic Encephalopathy. J. Neuroinflammation 2020;17:36. doi:10.1186/s12974-019-1689-8
Hellmann, M. D., Paz-Ares, L., Bernabe Caro, R., Zurawski, B., Kim, S. W., Carcereny Costa, E., et al. Nivolumab Plus Ipilimumab in Advanced Non-small-cell Lung Cancer. N. Engl. J. Med. 2019;381:2020–2031. doi:10.1056/NEJMoa1910231
Hendriks, L. E. L., Henon, C., Auclin, E., Mezquita, L., Ferrara, R., Audigier-Valette, C., et al. Outcome of Patients with Non-small Cell Lung Cancer and Brain Metastases Treated with Checkpoint Inhibitors. J. Thorac. Oncol. 2019;14:1244–1254. doi:10.1016/j.jtho.2019.02.009
Herbst, R. S., Baas, P., Kim, D. W., Felip, E., Pérez-Gracia, J. L., Han, J. Y., et al. Pembrolizumab versus Docetaxel for Previously Treated, PD-L1-Positive, Advanced Non-small-cell Lung Cancer (KEYNOTE-010): a Randomised Controlled Trial. Lancet 2016;387:1540–1550. doi:10.1016/S0140-6736(15)01281-7
Iadecola, C. The Neurovascular Unit Coming of Age: A Journey through Neurovascular Coupling in Health and Disease. Neuron. 2017;96(1):17–42. doi:10.1016/j.neuron.2017.07.030
Inoue, H., and Tani, K. Multimodal Immunogenic Cancer Cell Death as a Consequence of Anticancer Cytotoxic Treatments. Cell Death Differ. 2014;21(1):39–49. doi:10.1038/cdd.2013.84
Jotte, R., Cappuzzo, F., Vynnychenko, I., Stroyakovskiy, D., Abreu, D. R., Hussein, M., et al. OA14.02 IMpower131: Final OS Results of Carboplatin + Nab-Paclitaxel ± Atezolizumab in Advanced Squamous NSCLC. J. Thorac. Oncol. 2019;14:S243–S244. doi:10.1016/j.jtho.2019.08.484
Karivedu, V., Jandarov, R., and Wise-Draper, T. M.. Brain Metastases Treated with Immune Checkpoint Inhibitors: A Single center Experience Jco. 2018;36(15_Suppl. l, e14012.doi:10.1200/jco.2018.36.15_suppl.e14012
Keskin, D. B., Anandappa, A. J., Sun, J., Tirosh, I., Mathewson, N. D., Li, S., et al. Neoantigen Vaccine Generates Intratumoral T Cell Responses in Phase Ib Glioblastoma Trial. Nature. 2019;565(7738):234–239. doi:10.1038/s41586-018-0792-9
Khan, K. A., and Kerbel, R. S. Improving Immunotherapy Outcomes with Anti-angiogenic Treatments and Vice Versa. Nat. Rev. Clin. Oncol. 2018;15(5):310–324. doi:doi:10.1038/nrclinonc.2018.9
Kiess, A. P., Wolchok, J. D., Barker, C. A., Postow, M. A., Tabar, V., Huse, J. T., et al. Stereotactic Radiosurgery for Melanoma Brain Metastases in Patients Receiving Ipilimumab: Safety Profile and Efficacy of Combined Treatment. Int. J. Radiat. Oncol. Biol. Phys. 2015;92(2):368–375. doi:10.1016/j.ijrobp.2015.01.004
Kim, K. J., Ramiro Diaz, J., Iddings, J. A., and Filosa, J. A. Vasculo-Neuronal Coupling: Retrograde Vascular Communication to BrainNeurons. J. Neurosci. 2016;36(50):12624–12639. doi:10.1523/JNEUROSCI.1300-16.2016
Kim, R., Keam, B., Kim, S., Kim, M., Kim, S. H., Kim, J. W., et al. Differences in Tumor Microenvironments between Primary Lung Tumors and Brain Metastases in Lung Cancer Patients: Therapeutic Implications for Immune Checkpoint Inhibitors. BMC Cancer. 2019;19(1):19. doi:10.1186/s12885-018-5214-8
Kipnis, J. Multifaceted Interactions between Adaptive Immunity and the central Nervous System. Science 353, 766–771. (2016).doi:10.1126/science.aag2638
Klemm, F., Maas, R. R., Bowman, R. L., Kornete, M., Soukup, K., Nassiri, S., et al. Interrogation of the Microenvironmental Landscape in Brain Tumors Reveals Disease-specific Alterations of Immune Cells. Cell. 2020;181(7):1643-e17. doi:10.1016/j.cell.2020.05.007
Kluger, H. M., Chiang, V., Mahajan, A., Zito, C. R., Sznol, M., Tran, T., et al. Long-Term Survival of Patients with Melanoma with Active Brain Metastases Treated with Pembrolizumab on a Phase II Trial. J. Clin. Oncol. 2019;37(1):52–60. doi:10.1200/JCO.18.00204
Kurywchak, P., Tavormina, J., and Kalluri, R. The Emerging Roles of Exosomes in the Modulation of Immune Responses in Cancer. Genome Med. 2018;10(1):23. doi:10.1186/s13073-018-0535-4
Li, F., Lan, Y., Wang, Y., Wang, J., Yang, G., Meng, F., et al. Endothelial Smad4 Maintains Cerebrovascular Integrity by Activating N-Cadherin through Cooperation with Notch. Dev. Cel 2011;20:291–302. doi:10.1016/j.devcel.2011.01.011
Liniker, E., Menzies, A. M., Kong, B. Y., Cooper, A., Ramanujam, S., Lo, S., et al. Activity and Safety of Radiotherapy with Anti-PD-1 Drug Therapy in Patients with Metastatic Melanoma. Oncoimmunology. 2016;5:e1214788.doi:10.1080/2162402X.2016.1214788
Long, G. V., Atkinson, V., Menzies, A. M., Lo, S., and McArthur, G. A. A Randomized Phase II Study of Nivolumab or Nivolumab Combined with Ipilimumab in Patients (Pts) with Melanoma Brain Metastases (Mets): The Anti-PD1 Brain Collaboration (ABC). J. Clin. Oncol. 2017;35(Suppl. 15):9508–9508doi:10.1200/jco.2017.35.15_suppl.9508
Lukas, R. V., Gandhi, M., O’Hear, C., Hu, S., Lai, C., and Patel, J. D. Safety and Efficacy Analyses of Atezolizumab in Advanced Non-small Cell Lung Cancer (NSCLC) Patients with or without Baseline Brain Metastases. Ann. Oncol. 2017;28(Suppl. l_2).doi:10.1093/annonc/mdx091.001
Ma, Y., Mattarollo, S. R., Adjemian, S., Yang, H., Aymeric, L., Hannani, D., et al. CCL2/CCR2-dependent Recruitment of Functional Antigen-Presenting Cells into Tumors upon Chemotherapy. Cancer Res. 2014;74(2):436–445. doi:10.1158/0008-5472.CAN-13-1265
Mansfield, A. S., Aubry, M. C., Moser, J. C., Harrington, S. M., Dronca, R. S., Park, S. S., et al. Temporal and Spatial Discordance of Programmed Cell Death-Ligand 1 Expression and Lymphocyte Tumor Infiltration between Paired Primary Lesions and Brain Metastases in Lung Cancer. Ann. Oncol., 2016, 27(10): 1953–1958. doi:10.1093/annonc/mdw289
Mansfield, A. S., Herbst, R. S., Castro, G., Hui, R., Peled, N., Kim, D.-W., et al. Outcomes with Pembrolizumab (Pembro) Monotherapy in Patients (Pts) with PD-L1-Positive NSCLC with Brain Metastases: Pooled Analysis of KEYNOTE-001, -010, -024, and -042. Ann. Oncol. 2019;30:v604–v606. doi:10.1093/annonc/mdz260.004
Martin, A. M., Cagney, D. N., Catalano, P. J., Alexander, B. M., Redig, A. J., Schoenfeld, J. D., et al. Immunotherapy and Symptomatic Radiation Necrosis in Patients with Brain Metastases Treated with Stereotactic Radiation. JAMA Oncol.. 2018;4(8):1123–1124. doi:10.1001/jamaoncol.2017.3993
Martin, A. M., Daniel, N., Cagney, D. N., Catalano, P. J., Alexander, B. M., Redig, A. J., Schoenfeld, J. D., et al. Immunotherapy and Symptomatic Radiation Necrosis in Patients with Brain Metastases Treated with Stereotactic Radiation. JAMA Oncol. 2018;4(8):1123–1124. doi:10.1001/jamaoncol.2017.3993
McFaline-Figueroa, J. R., and Lee, E. Q. Neurological Complications of Targeted Therapies and Immunotherapies for Cancer. Curr. Treat. Options. Neurol. 23, 9 (2021). doi:10.1007/s11940-021-00663-7
Metro, G., Chiari, R., Ricciuti, B., Rebonato, A., Lupattelli, M., Gori, S., et al. Pharmacotherapeutic Options for Treating Brain Metastases in Non-small Cell Lung Cancer. Expert Opin. Pharmacother. 2015;16(17):2601–2613. doi:10.1517/14656566.2015.1094056
Michaud, M., Martins, I., Sukkurwala, A. Q., Adjemian, S., Ma, Y., Pellegatti, P., et al. Autophagy-dependent Anticancer Immune Responses Induced by Chemotherapeutic Agents in Mice. Science. 2011;334(6062):1573–1577. doi:10.1126/science.1208347
Minniti, G., Anzellini, D., Reverberi, C., Cappellini, G. C. A., Marchetti, L., Bianciardi, F., et al. Stereotactic Radiosurgery Combined with Nivolumab or Ipilimumab for Patients with Melanoma Brain Metastases: Evaluation of Brain Control and Toxicity. J. Immunother. Cancer. 2019;7(1):102. doi:10.1186/s40425-019-0588-y
Mok, T. S. K., Wu, Y. L., Kudaba, I., Kowalski, D. M., Cho, B. C., Turna, H. Z., et al. Pembrolizumab versus Chemotherapy for Previously Untreated, PD-L1-Expressing, Locally Advanced or Metastatic Non-small-cell Lung Cancer (KEYNOTE-042): a Randomised, Open-Label, Controlled, Phase 3 Trial. Lancet 2019;393:1819–1830. doi:10.1016/S0140-6736(18)32409-7
Nimmerjahn, A., Kirchhoff, F., and Helmchen, F. Resting Microglial Cells Are Highly Dynamic Surveillants of Brain Parenchyma In Vivo. Science. (2005) 308:1314– 8. doi:10.1126/science.1110647
Nimmerjahn, A., Kirchhoff, F., and Helmchen, F. Resting Microglial Cells Are Highly Dynamic Surveillants of Brain Parenchyma In Vivo. Science. (2005) 308:1314– 8. doi:10.1126/science.1110647
Owens, T., Bechmann, I., and Engelhardt, B. Perivascular Spaces and the Two Steps to Neuroinflammation. J. Neuropathol. Exp. Neurol. 2008;67(12):1113–1121. doi:10.1097/NEN.0b013e31818f9ca8
Papadimitrakopoulou, V., Cobo, M., Bordoni, R., Dubray-Longeras, P., Szalai, Z., Ursol, G., et al. OA05.07 IMpower132: PFS and Safety Results with 1L Atezolizumab + Carboplatin/Cisplatin + Pemetrexed in Stage IV Non-squamous NSCLC. J. Thorac. Oncol. 2018;13:S332, S333. (abstr OA05.07). doi:10.1016/j.jtho.2018.08.262
Paz-Ares, L., Luft, A., Vicente, D., Tafreshi, A., Gümüş, M., Mazières, J., et al. Pembrolizumab Plus Chemotherapy for Squamous Non-small-cell Lung Cancer. N. Engl. J. Med. 2018;379:2040–2051. doi:10.1056/NEJMoa1810865
Powell, S. F., Abreu, D. R., Langer, C. J., Tafreshi, A., Paz-Ares, L., Kopp, H.-G., et al. Pembrolizumab (Pembro) Plus Platinum-Based Chemotherapy (Chemo) in NSCLC with Brain Metastases: Pooled Analysis of KEYNOTE-021, 189, and 407. Ann. Oncol. 2019;30:v606–v607. doi:10.1093/annonc/mdz260.005
Qian, J. M., Yu, J. B., Kluger, H. M., and Chiang, V. L. Timing and Type of Immune Checkpoint Therapy Affect the Early Radiographic Response of Melanoma Brain Metastases to Stereotactic Radiosurgery. Cancer. 2016;122(19):3051–3058. doi:10.1002/cncr.30138
Quail, D. F., and Joyce, J. A. The Microenvironmental Landscape of Brain Tumors. Cancer Cell. 2017;31(3):326–341. doi:10.1016/j.ccell.2017.02.009
Raza, M., Prasad, P., Gupta, P., Kumar, N., Sharma, T., Rana, M., et al. Perspectives on the Role of Brain Cellular Players in Cancer-Associated Brain Metastasis: Translational Approach to Understand Molecular Mechanism of Tumor Progression. Cancer Metastasis Rev. (2018) 37:791–804. doi:10.1007/s10555-018-9766-5
Reck, M., Ciuleanu, T-E., Dols, M. C., Schenker, M., Zurawski, B., Menezes, J., et al. Nivolumab (NIVO) + Ipilimumab (IPI) + 2 Cycles of Platinum-Doublet Chemotherapy (Chemo) vs 4 Cycles Chemo as First-Line (1L) Treatment (Tx) for Stage IV/recurrent Non-small Cell Lung Cancer (NSCLC): CheckMate 9LA. 9501–9501 JCO 2020;38(15_Suppl. l). doi:10.1200/jco.2020.38.15_suppl.9501
Reck, M., Rodríguez-Abreu, D., Robinson, A. G., Hui, R., Csőszi, T., Fülöp, A., et al. Pembrolizumab versus Chemotherapy for PD-L1-Positive Non-small-cell Lung Cancer. N. Engl. J. Med. 2016;375(19):1823–1833. doi:10.1056/NEJMoa1606774
Reck, M., Rodríguez-Abreu, D., Robinson, A. G., Hui, R., Csőszi, T., Fülöp, A., et al. Updated Analysis of KEYNOTE-024: Pembrolizumab versus Platinum-Based Chemotherapy for Advanced Non-small-cell Lung Cancer with PD-L1 Tumor Proportion Score of 50% or Greater. J. Clin. Oncol. 2019;37:537–546. doi:10.1200/JCO.18.00149
Rittmeyer, A., Barlesi, F., Waterkamp, D., Park, K., Ciardiello, F., von Pawel, J., et al. Atezolizumab versus Docetaxel in Patients with Previously Treated Non-small-cell Lung Cancer (OAK): a Phase 3, Open-Label, Multicentre Randomised Controlled Trial. Lancet. 2017;389(10066):255–265. doi:10.1016/S0140-6736(16)32517-X
Sampson, J. H., Gunn, M. D., Fecci, P. E., and Ashley, D. M. Brain Immunology and Immunotherapy in Brain Tumours. Nat. Rev. Cancer. 2020;20(1):12–25. doi:10.1038/s41568-019-0224-7
Shaverdian, N., Lisberg, A. E., Bornazyan, K., Veruttipong, D., Goldman, J. W., Formenti, S. C., et al. Previous Radiotherapy and the Clinical Activity and Toxicity of Pembrolizumab in the Treatment of Non-small-cell Lung Cancer: a Secondary Analysis of the KEYNOTE-001 Phase 1 Trial. Lancet Oncol. 2017;18(7):895–903. doi:10.1016/S1470-2045(17)30380-7
Shojaei, F., Wu, X., Zhong, C., Yu, L., Liang, X.-H., Yao, J., et al. Nature. 2007, Bv8 Regulates Myeloid-cell-dependent Tumour Angiogenesis;450(7171):825–831. doi:10.1038/nature06348
Sistigu, A., Yamazaki, T., Vacchelli, E., Chaba, K., Enot, D. P., Adam, J., et al. Cancer Cell-Autonomous Contribution of Type I Interferon Signaling to the Efficacy of Chemotherapy. Nat. Med. 2014;20(11):1301–1309. doi:10.1038/nm.3708
Singh, C., Qian, J. M., Yu, J. B., and Chiang, V. L. Local Tumor Response and Survival Outcomes after Combined Stereotactic Radiosurgery and Immunotherapy in Non-small Cell Lung Cancer with Brain Metastases. J. Neurosurg., 132 2019;:512–517. doi:10.3171/2018.10.JNS181371
Skrepnik, T., Sundararajan, S., Cui, H., and Stea, B. Improved Time to Disease Progression in the Brain in Patients with Melanoma Brain Metastases Treated with Concurrent Delivery of Radiosurgery and Ipilimumab. Oncoimmunology. 2017;6(3):e1283461. doi:10.1080/2162402X.2017.1283461
Socinski, M. A., Jotte, R. M., Cappuzzo, F., Orlandi, F., Stroyakovskiy, D., Nogami, N., et al. Atezolizumab for First-Line Treatment of Metastatic Nonsquamous NSCLC. N. Engl. J. Med. 2018;378:2288–2301. doi:10.1056/NEJMoa1716948
Sukkurwala, A. Q., Martins, I., Wang, Y., Schlemmer, F., Ruckenstuhl, C., Durchschlag, M., et al. Immunogenic Calreticulin Exposure Occurs through a Phylogenetically Conserved Stress Pathway Involving the Chemokine CXCL8. Cel Death Differ. 2014;21(1):59–68. doi:10.1038/cdd.2013.73
Tang, W., Fan, W., Lau, J., Deng, L., Shen, Z., and Chen, X. Emerging Blood-Brain-Barrier-Crossing Nanotechnology for Brain Cancer Theranostics. Chem. Soc. Rev. 2019;48(11):2967–3014. doi:10.1039/c8cs00805a
Takamori, S., Toyokawa, G., Okamoto, I., Takada, K., Kinoshita, F., Kozuma, Y., et al. Clinical Significance of PD-L1 Expression in Brain Metastases from Non-small Cell Lung Cancer. Anticancer Res. 2018;38(1):553–557. doi:10.21873/anticanres.12258
Takamori, S., Toyokawa, G., Okamoto, I., Takada, K., Kozuma, Y., Matsubara, T., et al. Discrepancy in Programmed Cell Death-Ligand 1 between Primary and Metastatic Non-small Cell Lung Cancer. Anticancer Res., 2017, 37: 4223–4228. doi:10.21873/anticanres.11813
Taube, J. M., Klein, A., Brahmer, J. R., Xu, H., Pan, X., Kim, J. H., et al. Association of PD-1, PD-1 Ligands, and Other Features of the Tumor Immune Microenvironment with Response to Anti-PD-1 Therapy. Clin. Cancer Res. 2014;20(19):5064–5074. doi:10.1158/1078-0432.CCR-13-3271
Tawbi, H. A., Forsyth, P. A., Algazi, A., Hamid, O., Hodi, F. S., Moschos, S. J., et al. Combined Nivolumab and Ipilimumab in Melanoma Metastatic to the Brain. N. Engl. J. Med. 379, 722–730. (2018).doi:10.1056/NEJMoa1805453
Tkach, M., and Théry, C. Communication by Extracellular Vesicles: where We Are and where We Need to Go. Cell. 2016;164(6):1226–1232. doi:10.1016/j.cell.2016.01.043
Tran, T. T., Jilaveanu, L. B., Omuro, A., Chiang, V. L., Huttner, A., and Kluger, H. M. Complications Associated with Immunotherapy for Brain Metastases. Curr. Opin. Neurol. 2019;32(6):907–916. doi:10.1097/WCO.0000000000000756
Tumeh, P. C., Harview, C. L., Yearley, J. H., Shintaku, I. P., Taylor, E. J., Robert, L., et al. PD-1 Blockade Induces Responses by Inhibiting Adaptive Immune Resistance. Nature, 2014, 515(7528): 568–571. doi:10.1038/nature13954
Van Steenwinckel, J., Schang, A. L., Krishnan, M. L., Degos, V., Delahaye-Duriez, A., Bokobza, C., et al. Decreased Microglial Wnt/β-Catenin Signalling Drives Microglial Pro-inflammatory Activation in the Developing Brain. Brain 2019;142:3806–3833. doi:10.1093/brain/awz319
Vanpouille-Box, C., Alard, A., Aryankalayil, M. J., Sarfraz, Y., Diamond, J. M., Schneider, R. J., et al. DNA Exonuclease Trex1 Regulates Radiotherapy-Induced Tumour Immunogenicity. Nat. Commun. 2017;8:15618. doi:10.1038/ncomms15618
Wang, L., Geng, J., Qu, M., Yuan, F., Wang, Y., Pan, J., et al. Oligodendrocyte Precursor Cells Transplantation Protects Blood-Brain Barrier in a Mouse Model of Brain Ischemia via Wnt/β-Catenin Signaling. Cell Death Dis 2020;11:9. doi:10.1038/s41419-019-2206-9
Wargo, J. A., Reuben, A., Cooper, Z. A., Oh, K. S., and Sullivan, R. J. Immune Effects of Chemotherapy, Radiation, and Targeted Therapy and Opportunities for Combination with Immunotherapy. Semin. Oncol. 2015;42(4):601–616. doi:doi:10.1053/j.seminoncol.2015.05.007
West, H., McCleod, M., Hussein, M., Morabito, A., Rittmeyer, A., Conter, H. J., et al. Atezolizumab in Combination with Carboplatin Plus Nab-Paclitaxel Chemotherapy Compared with Chemotherapy Alone as First-Line Treatment for Metastatic Non-squamous Non-small-cell Lung Cancer (IMpower130): a Multicentre, Randomised, Open-Label, Phase 3 Trial. Lancet Oncol. 2019;20:924–937. doi:10.1016/S1470-2045(19)30167-6
Yang, T., Martin, P., Fogarty, B., Brown, A., Schurman, K., Phipps, R., et al. Exosome Delivered Anticancer Drugs across the Blood-Brain Barrier for Brain Cancer Therapy in Danio rerio. Pharm. Res. 2015;32(6):2003–2014. doi:doi:10.1007/s11095-014-1593-y
Zhou, J., Gong, Z., Jia, Q., Wu, Y., Yang, Z. Z., and Zhu, B. Programmed Death Ligand 1 Expression and CD8+ Tumor-Infiltrating Lymphocyte Density Differences between Paired Primary and Brain Metastatic Lesions in Non-small Cell Lung Cancer. Biochem. Biophys. Res. Commun., 2018, 498(4): 751–757. doi:10.1016/j.bbrc.2018.03.053
Zhou, J., Gong, Z., Jia, Q., Wu, Y., Yang, Z. Z., and Zhu, B. Programmed Death Ligand 1 Expression and CD8+ Tumor-Infiltrating Lymphocyte Density Differences between Paired Primary and Brain Metastatic Lesions in Non-small Cell Lung Cancer. Biochem. Biophys. Res. Commun., 2018, 498(4): 751–757. doi:10.1016/j.bbrc.2018.03.053
Keywords: NSCLC, brain metastasis, immune checkpoint inhibitors, irAEs, mechanisms
Citation: Li J, Wang M, Xu S, Li Y, Li J, Yu J and Zhu H (2022) The Strategies and Mechanisms of Immune Checkpoint Inhibitors for Brain Metastases in NSCLC. Front. Pharmacol. 13:841623. doi: 10.3389/fphar.2022.841623
Received: 22 December 2021; Accepted: 08 March 2022;
Published: 17 May 2022.
Edited by:
Kwong Tsang, Precision Biologics, Inc., United StatesReviewed by:
Masaki Terabe, National Cancer Institute (NIH), United StatesPierpaolo Correale, Azienda Ospedaliera “Bianchi-Melacrino-Morelli”, Italy
Copyright © 2022 Li, Wang, Xu, Li, Li, Yu and Zhu. This is an open-access article distributed under the terms of the Creative Commons Attribution License (CC BY). The use, distribution or reproduction in other forums is permitted, provided the original author(s) and the copyright owner(s) are credited and that the original publication in this journal is cited, in accordance with accepted academic practice. No use, distribution or reproduction is permitted which does not comply with these terms.
*Correspondence: Jinming Yu, c2R5dWppbm1pbmdAMTYzLmNvbQ==; Hui Zhu, ZHJ6aHVoQDEyNi5jb20=