- 1Department of Pharmacy and Yonsei Institute of Pharmaceutical Sciences, College of Pharmacy, Yonsei University, Incheon, South Korea
- 2College of Pharmacy and Research Institute of Pharmaceutical Sciences, Seoul National University, Seoul, South Korea
- 3Transdisciplinary Department of Medicine and Advanced Technology, Seoul National University Hospital, Seoul, South Korea
- 4Department of Neurology, Yonsei University College of Medicine, Seoul, South Korea
- 5Department of Pharmaceutical Medicine and Regulatory Sciences, Colleges of Medicine and Pharmacy, Yonsei University, Incheon, South Korea
Background: Renin-angiotensin system (RAS) inhibitors have been suggested as protective agents in Parkinson’s disease (PD). However, epidemiological evidence on the association between RAS inhibitors and the development of PD is inconsistent.
Objectives: To investigate the effect of RAS inhibitors on PD risk in patients with ischemic heart disease (IHD) by type and cumulative duration of RAS inhibitors and their degree of blood-brain barrier (BBB) penetration ability.
Methods: This was a propensity score-matched retrospective cohort study using 2008–2019 healthcare claims data from the Korean Health Insurance Review and Assessment database. The association between RAS inhibitor use and PD in patients with IHD was evaluated using multivariate Cox proportional hazard regression analysis. The risks are presented as adjusted hazard ratios (aHRs) and 95% confidence intervals (CIs).
Results: Over a 10-year follow-up, 1,086 of 62,228 IHD patients developed PD. The Cox regression model showed that the use of RAS inhibitors was significantly associated with a lower risk of PD (aHR = 0.75; 95% CI 0.66–0.85) than the non-use of RAS inhibitors. Specifically, this reduced risk of PD only remained with the use of BBB-crossing angiotensin II receptor blockers (ARBs) (aHR = 0.62; 95% CI = 0.53–0.74), and this association was more definite with an increasing cumulative duration. A significantly reduced risk of PD was not observed with the use of BBB-crossing angiotensin-converting enzyme inhibitors.
Conclusions: The use of ARBs with BBB-penetrating properties and a high cumulative duration significantly reduces the risk of PD in IHD patients. This protective effect could provide insight into disease-modifying drug candidates for PD.
Introduction
Parkinson’s disease (PD) is the second most common neurodegenerative disease, and the worldwide prevalence was reported as 1% in people aged ≥60 years (Tysnes and Storstein, 2017). PD is pathogenetically characterized by a gradual loss of dopaminergic neurons from the substantia nigra pars to the striatum, resulting in reduction of dopamine to the striatum (Samii et al., 2004; Connolly and Lang, 2014). Despite the effective symptomatic treatment for PD by restoring the dopamine level or activating the receptors, multiple trials to discover disease-modifying therapy have failed in the clinical phase (Kalia et al., 2015). As PD has traditionally been regarded irreversible, there is a growing number of unmet therapeutic need to delay disease progression or prevent premature mortality. Research on novel therapies to slow the neurodegenerative progression or to prevent PD in the early stages has been conducted (Kalia et al., 2015). Specifically, a number of studies have suggested various medications including statins (Kumar et al., 2012; Kang et al., 2017), phosphodiesterase inhibitors (Nthenge-Ngumbau and Mohanakumar, 2018; Erro et al., 2021), and renin-angiotensin system (RAS) inhibitors (Ana Flavia et al., 2017; Victor Teatini Ribeiro, 2020) as drug repurposing candidates with demonstrated neuroprotective effects.
The RAS is a circulating hormonal system responsible for the regulation of systemic blood pressure, electrolyte homeostasis, and volume status, which exerts its function via two main receptors: angiotensin (Ang) II type 1 (AT1) and type 2 (AT2) (Oro et al., 2007). These two receptors are present in various tissues, including the brain (Rivas-Santisteban et al., 2020). Recent evidence from animal studies showed that inhibition of brain RAS is associated with decreased dopaminergic neuronal loss and reduced oxidative stress in PD via the via alleviation of mitochondrial function (Perez-Lloret et al., 2017; Ray et al., 2021). Brain RAS blockade may play a protective role in neuroinflammation and accumulation of phosphorylated alpha-synuclein. This is accomplished by blocking the Ang II-related pro-inflammatory oxidative effects and reducing the production of reactive oxygen species associated with AT1 receptor overexpression in the substantia nigra (Wright and Harding, 2012; Perez-Lloret et al., 2017; Ray et al., 2021).
Although the role of RAS inhibition in preventing PD is well documented in animal and in vitro/in vivo studies, only a few human studies have focused on the association between RAS inhibitors and PD, and the results have been inconsistent. While some clinical studies have found evidence of the neuroprotective effect of RAS inhibitors (Y.-C. Lee et al., 2014; Reardon et al., 2000), others have shown no association between RAS inhibitor use and PD (Becker et al., 2008; Ritz et al., 2010; Warda et al., 2019). Moreover, considering the potential influence of the duration of RAS inhibitors and differences in central nervous system penetration among these agents (Wu et al., 2016; Ouk et al., 2021), additional clinical evidence based on a valid study design and taking into account these factors is required to support the effect of RAS inhibitors on the incidence of PD.
Herein, we aimed to investigate the effect of RAS inhibitors on PD occurrence using a longitudinal national claims database in Korea. Specifically, we analyzed the cumulative duration-response relationship and compared the effect on PD based on RAS inhibitor type and blood-brain barrier (BBB) penetration.
Materials and Methods
Study Design and Data Source
This retrospective cohort study was conducted using national claims data from the Health Insurance Review and Assessment Service (HIRA) database between 1 January 2008 and 31 December 2019. The Republic of Korea has a single-payer health insurance system that provides compulsory universal health insurance that covers the entire population of Korea (J. A. Kim et al., 2017). Healthcare providers must submit medical claims to the HIRA to receive an evaluation of the appropriateness of the reimbursement. The HIRA generates a research-ready database containing sociodemographic characteristics and clinical information. The database includes diagnoses, procedures, and prescription records from submitted claims after anonymizing all participants according to strict confidentiality guidelines from the medical care claims of all patients.
This study was approved by the Institutional Review Board (IRB) of Yonsei University (IRB number: 7001988-202004-HR-846-01E). The requirement for informed consent was waived because the data were publicly available and de-identified.
Study Population
To evaluate the effect of RAS inhibitors on the development of PD in patients with ischemic heart disease (IHD), we enrolled patients aged ≥60 years who were diagnosed with angina or myocardial infarction during the identification period (1 January 2009, to 30 June 2009). Patients diagnosed with angina or myocardial infarction were identified based on the International Classification of Diseases 10th Revision (ICD-10) diagnostic codes I20.0-I22.0. We designated a specific time point (1 July 2009) as the index date for all the study participants. All study participants were followed-up on the index date.
To identify new users of RAS inhibitors during the identification period, patients who had prescription records of RAS inhibitors prior to the identification period or who had been prescribed their first RAS inhibitors after the identification period were excluded. Patients who had died, had last claims records, or were previously diagnosed with parkinsonism (G20-G26), including PD before the index date, were also excluded. Given that PD may have a prodromal period (Postuma and Berg, 2016), a 1-year lag time was included to minimize the reverse causality of PD. The new users of RAS inhibitors during the identification period were classified into user groups. Patients who had never been prescribed RAS inhibitors during the study period were classified into a non-user group.
Exposure Assessment and Data Collection
The RAS inhibitors used in this study included angiotensin-converting enzyme inhibitors (ACEIs) and AT1 receptor blockers (ARBs), based on the Anatomic Therapeutic Chemical classification system of drugs by the World Health Organization (WHO) Collaborating Centre for Drug Statistic Methodology (World Health Organization, 2021). A detailed list of the RAS inhibitors is provided in Supplementary Table S1. To evaluate the cumulative effect of RAS inhibitors on the incidence of PD, we accumulated the doses and duration of RAS inhibitors during the follow-up period by collecting prescription information, including dosage, frequency, and days of supply.
The accumulated doses were calculated by multiplying the dosage, frequency, and number of supply days. These values were converted into the cumulative defined daily dose (cDDD) developed by the WHO (Methodology, 2021). The cumulative duration of RAS inhibitor use was calculated as the sum of the prescription days. The daily equivalent dosage, defined as the ratio of the cumulative dose to the cumulative exposure period, was calculated. To compare the preventive effect on PD, the patients were categorized into four groups according to their prescriptions: (1) use of non-BBB-crossing ACEIs, (2) use of BBB-crossing ACEIs, (3) use of non-BBB-crossing ARBs, and (4) use of BBB-crossing ARBs (Supplementary Table S1) (Alzahrani et al., 2020; C. K. ; Kim et al., 2015; Oka et al., 1988; Sink et al., 2009; Takai et al., 2004; Wharton et al., 2012; Yagi et al., 2013). These groups were based on the degree of BBB penetration and the type of RAS inhibitor prescribed.
Data on sociodemographic characteristics, including the patient’s age, sex, and insurance type (i.e., health insurance or medical aid), were collected during the identification period. Potential confounding factors affecting the development of PD, including comorbid diseases and concurrent medications, were recorded until the date of outcome occurrence or the end of the study period. These confounding factors were confirmed when patients had continuous claim records on diagnosis and prescription at least once per year until the last follow-up (J. Lee et al., 2018).
Comorbid diseases known as potential risk or protective factors for PD included hypertension, dyslipidemia, diabetes mellitus, end-stage renal disease, stroke, brain injury, dementia, chronic obstructive pulmonary disease (COPD), depression, gout, osteoarthritis, osteoporosis, and severe liver disease (Hu et al., 2020; Jafari et al., 2013; C. H. ; Li et al., 2015; Rugbjerg et al., 2010; Torsney et al., 2014; Tryc et al., 2013; Vale et al., 2018; Wang et al., 2018). Concurrent medications known to be potential risk or protective factors for PD include antipsychotics, antiemetics, calcium channel antagonists, antiepileptics, trimetazidine, dopamine depleters, mood stabilizers, beta-blockers, dihydropyridine calcium channel blockers, and statins (Ascherio and Schwarzschild, 2016; Jeong et al., 2019; S. ; Kim et al., 2020; López-Sendón et al., 2013; Mullapudi et al., 2016; Shin and Chung, 2012). The ICD-10 codes for comorbid diseases and details of the concurrent medications are listed in Supplementary Tables S2, 3.
Outcome Measures
The primary outcome measure was new-onset Parkinson’s disease during the follow-up period. New-onset PD was defined as an ICD-10 diagnostic code for PD (G20), accompanied by the PD registration code and prescription records for any PD treatment drug. These include L-dopa, carbidopa/benserazide, pramipexole, ropinirole, bromocriptine, selegiline, rasagiline, amantadine, entacapone, trihexyphenidyl, and benztropine. In Korea, the government established a registry called the Rare and Intractable Diseases Program, which registered patients with neurologist-confirmed PD (Park et al., 2019). To enhance the validity of PD diagnosis in this study, we used the PD registration code (V124) for the outcome definition. The date of study outcome was defined as the date of the first appearance of the PD diagnostic code for G20.
Statistical Analysis
To adjust for selection bias, we used a propensity score (PS)-matched design. PS was estimated using multivariate logistic regression. The predictors of PS included sex, age, insurance type, follow-up duration, comorbid diseases, and concurrent medications. Patients who did and did not use RAS inhibitors were matched in a 1:1 ratio without replacement, using the greedy method. This was within a caliper that was 0.2 times the standard deviation of the logit PS. After PS matching, the balance diagnostics between RAS inhibitor users and non-users was assessed by evaluating the standardized difference between them. When the standardized difference was >0.1, it was regarded as a sign of imbalance (Austin, 2009). Furthermore, the similar distribution of PS between RAS inhibitor users and non-users after matching is confirmed graphically in Supplementary Figure S1.
Descriptive statistics were used to summarize the characteristics of the matched population. Between-group comparisons of the distribution of sociodemographic data, comorbid diseases, and concurrent medications between RAS inhibitor users and non-users were performed using Pearson’s chi-squared test and Student’s t-test. The incidence rate of PD per 1,000 person-years was calculated by dividing the number of outcome events by the total number of person-years at risk and multiplying the result by 1,000. The person-years at risk were accumulated for each patient from the index date to the outcome date, death, or 31 December 2019, whichever occurred first.
Multivariable Cox proportional hazard regression analyses adjusted for demographic characteristics, comorbidities, and concurrent medications were used to estimate the hazard ratio (HR) and 95% confidence interval (CI) for the risk of PD in relation to RAS inhibitor use. The proportional hazard assumption was confirmed by a statistical test of the correlation between Schoenfeld residuals and survival time, using a cumulative log(minus) log curve (Supplementary Figure S2). To investigate the cumulative dose-response and duration-response relationships, the risk of PD was investigated according to the cumulative dose, total duration, and daily equivalent dosage of RAS inhibitors, using a multivariate Cox regression model. Additionally, the adjusted hazard ratios (aHRs) of PD were estimated according to the type of RAS inhibitor (i.e., ACEIs and ARBs) used and the degree of BBB penetration. Subgroup analyses were performed to evaluate the effect of RAS inhibitors on PD risk according to patient sex and age. Age was divided into two groups based on the mean age of the study population.
Sensitivity analyses were conducted using three steps. First, the index date from 1 July 2009 was shifted to 1 July 2010 and 2011. As the identification period changed accordingly, drug exposure, outcomes, and confounding factors were re-assessed. Second, the lag time was extended for 3 and 5 years, resulting in a decrease in the follow-up period and the number of study participants. As the lag time was extended, captured PD events as outcomes during the lag time were excluded from the analysis. Finally, the operational definition of PD was changed, and the study outcome was re-evaluated according to the following new definitions. First, patients had a diagnostic code for PD accompanied by a PD registration code. Second, patients had a diagnostic code of PD and at least 60 days of prescription records for any PD treatment medications after the first diagnosis. All statistical analyses were performed using SAS statistical software (version 9.4; SAS Institute, Cary, NC, United States). p < 0.05 was considered significant.
Results
Baseline Patient Characteristics
Among the 537,116 patients diagnosed with IHD between January 2009 and June 2009, 31,114 RAS inhibitor users and 31,114 RAS inhibitor non-users were included in the analysis (Figure 1). The baseline characteristics of the study population are shown in Table 1. The mean age (±standard deviation) was 70.3 (±7.1) years, and males accounted for 44.4% of the cohort. The mean duration of follow-up was 7.6 and 7.7 years for RAS inhibitor users and non-users, respectively. After PS matching, the distribution between the two groups became similar for all variables, with standardized differences <0.1. This indicates that the differences between covariates were negligible.
RAS Inhibitor Use and the Risk of PD
A total of 1,086 new PD cases were identified from 477,255 person-years of observation in the main analysis. The overall incidence rate was 2.28 per 1,000 person-years. The median time to censor was 9.4 years (interquartile range, 6.1–9.5 years) in those censored with PD over 10 years of follow-up. The incidence rate of PD was significantly lower in RAS inhibitor users than in non-users (2.04 per 1,000 person-years vs. 2.51 per 1,000 person-years, p < 0.0001).
RAS inhibitor use was significantly associated with a reduced risk of PD after adjusting for confounders (aHR = 0.75; 95% CI = 0.66–0.85). Male sex and aged ≥65 years were associated with an increased risk of PD. Comorbidities of hypertension, dyslipidemia, diabetes mellitus, stroke, brain injury, dementia, COPD, depression, osteoarthritis, and osteoporosis were significantly associated with an increased risk of PD. Consistent use of medications including antipsychotics, antiemetics, calcium channel antagonists, antiepileptics, trimetazidine, and beta-blockers was significantly associated with an increased risk of PD. Statins were significantly associated with a decreased risk of PD (Table 2).
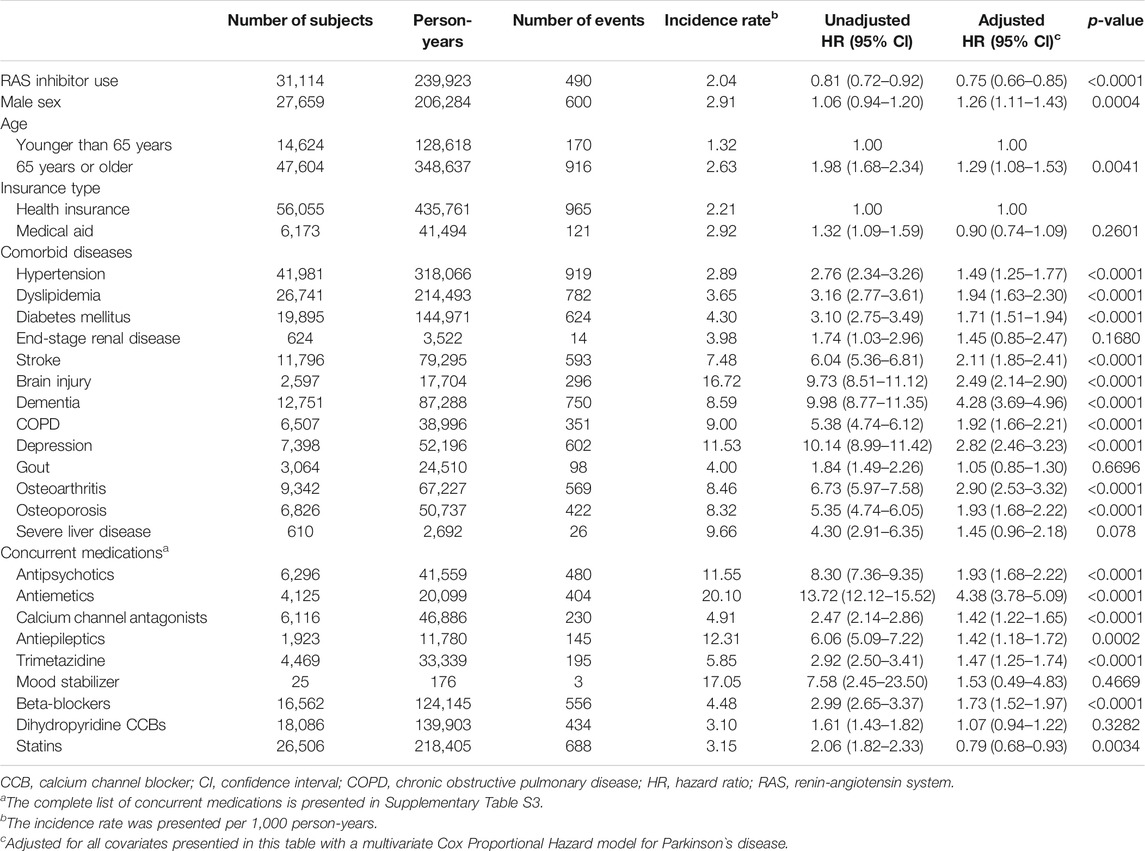
TABLE 2. Cox regression analysis on the association of Parkinson’s disease with renin-angiotensin system inhibitor use and confounding factors (N = 62,228).
A significantly reduced risk of PD was observed with the use of ARBs (aHR = 0.74; 95% CI = 0.65–0.85). However, ACEI use was not significantly associated with the risk of PD (aHR = 0.91; 95% CI = 0.77–1.08). The risk of PD was significantly reduced with the use of BBB-crossing RAS inhibitors (aHR = 0.67; 95% CI = 0.57–0.78), but not with the use of non-BBB-crossing RAS inhibitors. Regarding the risk of PD by drug type in relation to the degree of BBB penetrability, only the use of BBB-crossing ARBs showed significant decreases in PD incidence (aHR = 0.62; 95% CI = 0.53–0.74) (Table 3).
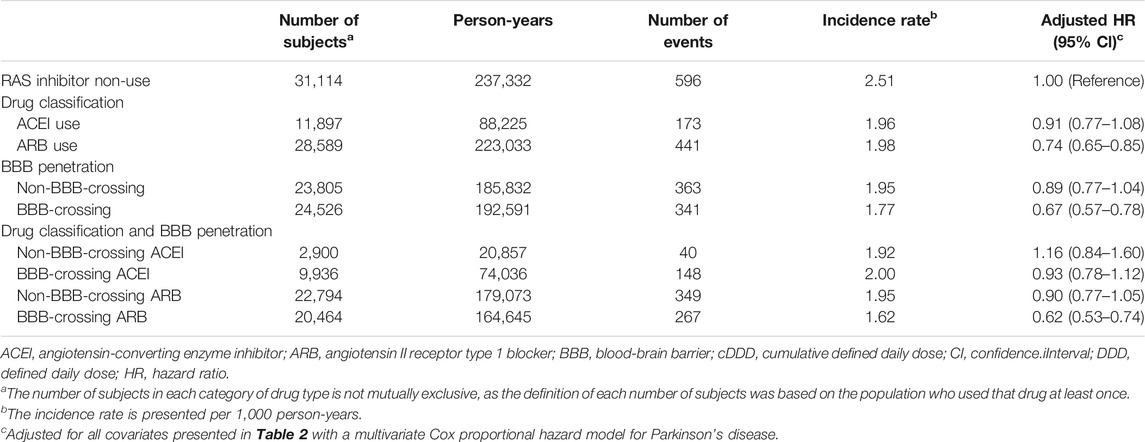
TABLE 3. Risk of Parkinson’s disease by type of renin-angiotensin system inhibitors and central nervous system penetration (N = 62,228).
Table 4 presents the PD risk breakdown by cumulative dose, duration, and daily equivalent dosage of BBB-crossing ARBs. In all cDDD groups, the use of BBB-crossing ARBs was significantly associated with a reduced risk of PD. Similar reduced risks of PD were observed in all groups at the daily equivalent dosage. Regarding cumulative duration, RAS inhibitor users who were exposed to ≥2 years of BBB-crossing ARBs showed a lower risk of PD than non-users, whereas those who were exposed to <2 years of BBB-crossing ARBs did not. The risk of developing PD tended to decrease with the increase in the cumulative dose and duration (P for trend = 0.03 and <0.01, respectively). Figure 2 shows the Kaplan-Meier curves of the cumulative hazard (1- survival rate) according to the cumulative duration of BBB-crossing ARBs. In the subgroup analyses, the aHRs of BBB-crossing ARBs were comparable in the populations aged<70 years (aHR = 0.65; 95% CI = 0.51–0.83) and ≥70 years (aHR = 0.58; 95% CI = 0.46–0.73). In the sex subgroup analysis, the aHR of BBB-crossing ARBs in the female population (aHR = 0.53; 95% CI = 0.43–0.66) was lower than that in the male population (aHR = 0.75; 95% CI = 0.58–0.96).
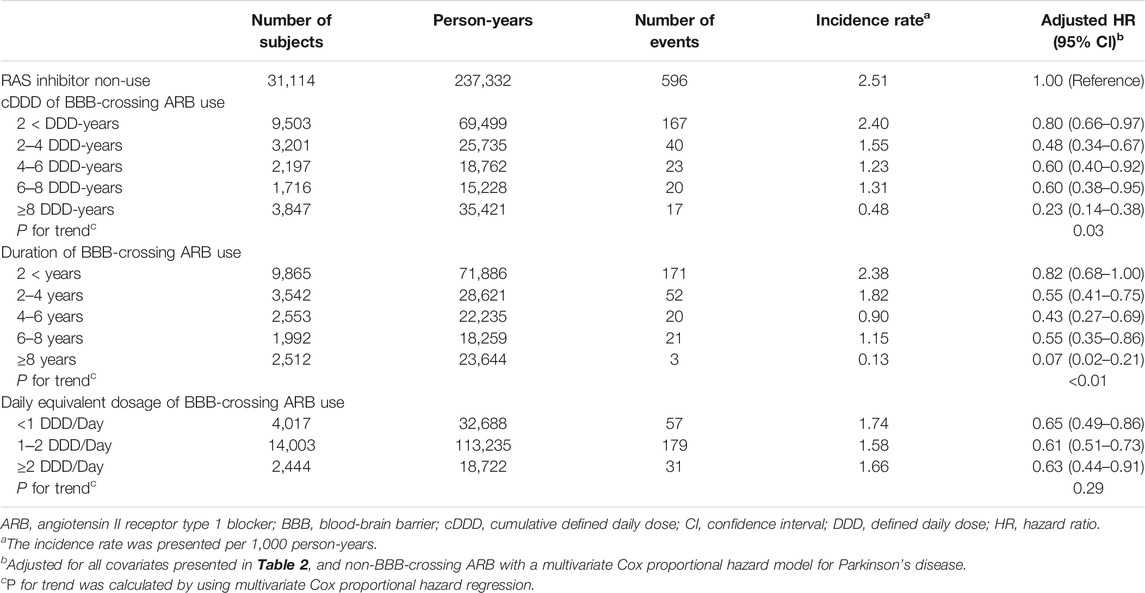
TABLE 4. Risk of Parkinson’s disease associated with cumulative doses and durations of BBB-crossing ARB use (N = 62,228).
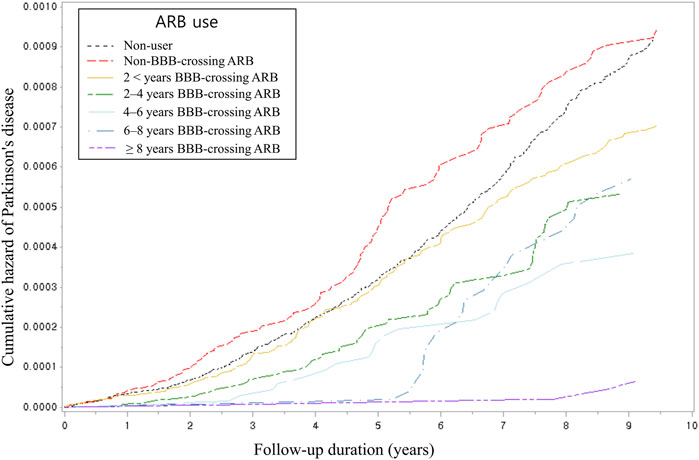
FIGURE 2. Kaplan-Meier cumulative hazard using Cox proportional hazard analysis by categorized cumulative duration. ARB, angiotensin II type 1 receptor blocker; BBB, blood-brain barrier.
Sensitivity Analyses
Sensitivity analyses for aHRs of RAS inhibitor use for PD are shown in Table 5. The PD risk associated with RAS inhibitor use remained lower than that the risk associated with RAS inhibitor non-use in all sensitivity analyses according to index date shifting, lengthening of the lagged period, and changing the outcome definitions.
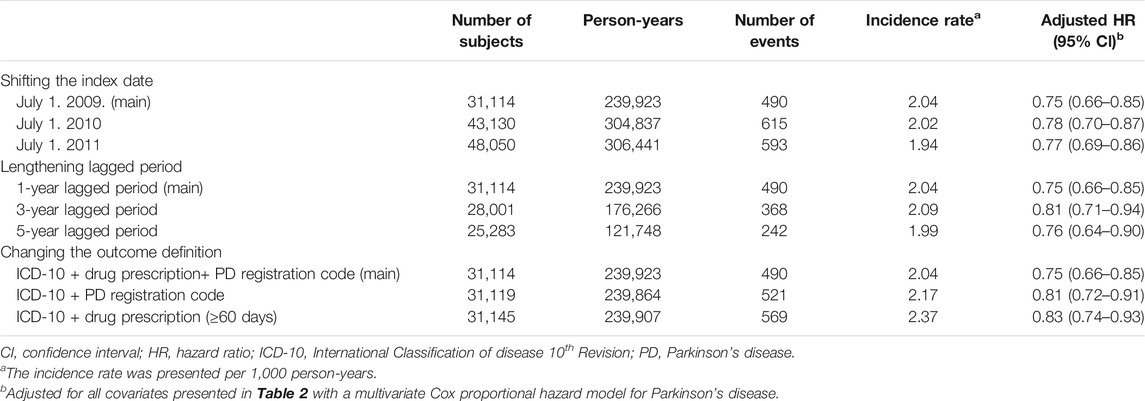
TABLE 5. Sensitivity analyses of adjusted hazard ratios of renin-angiotensin inhibitor use for Parkinson’s disease.
Discussion
In this nationwide population-based longitudinal cohort study, IHD patients treated with RAS inhibitors, particularly ARBs, had a lower risk of developing PD than did those who did not use RAS inhibitors. To date, few clinical studies have investigated the effect of RAS inhibitors on PD, and most of them have reported insignificant associations (Becker et al., 2008; Ritz et al., 2010; Warda et al., 2019). Compared to previous studies, our study focused on the effect of RAS inhibitors in the older IHD population. Furthermore, we adopted a robust study design, including analysis of a nationwide cohort with PS matching and landmark analysis, application of the lag-time to avoid reverse causation, adjustment of all possible confounders (Ascherio and Schwarzschild, 2016; Jafari et al., 2013; S. ; Kim et al., 2020; C. H. ; Li et al., 2015; Mullapudi et al., 2016; Rugbjerg et al., 2010; Torsney et al., 2014; Tryc et al., 2013; Vale et al., 2018; Wang et al., 2018), sufficient follow-up, and various sensitivity analyses. Importantly, to our best knowledge, this is the first study to evaluate the effect of BBB-penetration properties of RAS inhibitors on PD occurrence. The results showed that the risk of PD was only significantly reduced in patients who used BBB-crossing ARBs. This association was robust in patients exposed to a larger cumulative dose or longer duration of BBB-crossing ARBs. This result supports that BBB-crossing ARBs should be considered as one of the clinical options for preventing PD in patients with IHD primarily using RAS inhibitors. Moreover, our findings, which were derived from real-world data with 10 years of follow-up and included an evaluation of the impact of cumulative duration and BBB penetration, could add evidence to elucidate the conflicting results reported by previous studies regarding the preventive effect of RAS inhibitors on PD.
Our assessment of the risk of PD according to the type of RAS inhibitors (ACEIs and ARBs) revealed that only ARBs, but not ACEIs, were associated with a lower risk of PD compared with RAS inhibitor non-use. Although, similar to ARBs, the neuroprotective effect of ACEIs was supported by theoretical and experimental evidence, clinical evidence for an association between ACEIs and PD is lacking (Levi Marpillat et al., 2013; N. C. ; Li et al., 2010). Given that ACEIs and ARBs exert RAS inhibition at different sites, the discrepancy in PD risk might be attributable in part to the mechanism of action exerted by each drug class. ACEIs act upstream of the RAS pathway by inhibiting the synthesis of Ang II, which blocks both AT1 and AT2 receptors. Meanwhile, ARBs bind to the AT1 receptor directly to inhibit activation and may relatively increase the activity of endogenous Ang II at the AT2 receptor. This counteracts the neurodegenerative effect by AT1 activation (Mertens et al., 2010; Gebre et al., 2018). Moreover, blocking the AT1 receptor can maintain the elevated Ang-(1-7) by the ACE-sparing effect and indirectly upregulate the conversion of Ang II into Ang-(1-7). The Ang-(1-7)/Mas receptor axis could alleviate the pro-oxidative effects of the Ang II/AT1 axis in dopaminergic neurons (Wright et al., 2015; Gironacci et al., 2018). The conversion of excess Ang II to Ang IV also stimulates an increase in dopaminergic neurotransmission in the brain (Braszko, 2004). Additionally, ARBs may exert neuroprotective effects via RAS-independent mechanisms, such as proliferator-activated receptor gamma-associated peroxisome induction inhibition (Garrido-Gil et al., 2012). Further research is warranted to elucidate the specific mechanisms involved in the differences in neuroprotective activity between ACEIs and ARBs.
To the best of our knowledge, this is the first comprehensive study to evaluate PD risk based on the level of BBB penetration of RAS inhibitors. The results of this study indicated that only the use of BBB-crossing ARBs was associated with a reduced risk of PD. Our finding is noteworthy, as the preventive effect of RAS inhibitors on PD was based on a central-acting mechanism. A recent animal study showed that AT1 and AT2 form AT1/2 heterodimers, which are expressed in both striatal neurons and microglia in the central nervous system (Rivas-Santisteban et al., 2020). Moreover, some experimental studies on the effects of telmisartan and candesartan, which are typical ARBs known to penetrate the BBB, showed an improvement in dopaminergic and mitochondrial functions in an animal PD model (Sekar et al., 2018; Ray et al., 2021). Previous clinical studies on dementia also showed that BBB-crossing RAS inhibitors have better neuroprotective effects than non-BBB-crossing agents (Ho et al., 2021; Ouk et al., 2021). Thus, our real-world evidence suggests the potential value of BBB-crossing ARBs as a prophylactic or disease-modifying therapy for PD. Further studies evaluating the effects of individual ARBs on PD are required based on their BBB penetration ability measured using in vitro models and their pharmacodynamic properties such as receptor affinity.
Significantly reduced PD risks were observed in individuals with a greater cumulative dose and longer duration of BBB-crossing ARB use. Similar reduced risks of PD were observed regardless of the daily equivalent dosage. These results indicate that the exposure duration, rather than the dose of BBB-crossing ARBs, might be an influencing factor for this trend. Long-term blockade of the AT1 receptor by ARB administration may result in neurological improvement in cerebral ischemia and attenuation of neuronal damage (Culman et al., 2002; Ozacmak et al., 2007). Previous clinical studies have also investigated the duration-response relationship of RAS inhibitors in other neurodegenerative diseases. These studies showed that a longer duration of RAS inhibitor use is associated with a lower risk of neurodegenerative diseases (Davies et al., 2011; Lin et al., 2015). Consistent with these findings, our results identified the long-term effects of RAS inhibitors in the prevention of PD in humans. However, a more robust study to evaluate the long-term effects is required. Interestingly, the protective effect of BBB-crossing ARBs on PD was more remarkable in female than in male. Sex-specific differences in PD risk have been attributed to various potential mechanisms, including hormonal effects and brain RAS expression (Sullivan, 2008; Rodriguez-Perez et al., 2011; Labandeira-Garcia et al., 2016; Sumien et al., 2021). Moreover, enhanced effectiveness of ARBs in females has been shown in both clinical and experimental studies, according to sex differences in the AT1/AT2 receptor expression ratio (Okumura et al., 2005; Sullivan, 2008). Therefore, our results might be attributed to sex differences in response to brain RAS stimulation and inhibition, as well as to the estrogen-induced dopaminergic neuroprotective effect.
This study had some limitations. First, the study population selection protocol was vulnerable to selection bias. Although the PS matching method may minimize the treatment assignment bias by assembling a sample in which confounders are balanced between RAS users and non-users, some eligible patients, whose characteristics can be related to both the treatment and outcome, were excluded from the study. We also considered the control group as non-users and not active comparators such as those using beta-blockers and calcium channel blockers. Using an active comparator design could reduce selection bias (Yoshida et al., 2015). To address the potential selection bias, we adjusted for various confounders including medications by precise definition. Second, the study findings cannot be generalized to the entire population. The effect of RAS inhibitor use on PD was evaluated in IHD patients, which could affect the incidence of parkinsonism, including PD (Q. Li et al., 2018). Thus, the association between RAS inhibitor use and PD in the general population should be investigated in further studies. Third, our findings could not be extrapolated to young-onset PD occurring in populations aged <50 years because we included a cohort of patients aged ≥60 years. Given that genetics may play a role in young-onset PD (Laperle et al., 2020), additional research on genetic and environmental factors is required. Fourth, as all data used in this study were based on secondary claims data of HIRA, we could not confirm clinical information such as symptoms, laboratory measurements, and the Unified Parkinson’s disease Rating Scale. Given that an accurate diagnosis of PD should be based on clinical and imaging biomarker correlations, the PD cases in our study may have been underestimated or overestimated. Further studies with complete clinical information are warranted to confirm the association of PD progression or motor symptom improvement with RAS inhibitor use (Louis et al., 2009; Kim et al., 2017). However, we used a PD registration code that excludes secondary parkinsonism, which is considered to have a high accuracy for copayment reduction, along with the ICD-10 codes for PD, into the outcome. Additionally, we adopted several approaches to perform sensitivity tests to confirm the robustness and validity of our findings.
In conclusion, our nationwide cohort study highlights that the use of RAS inhibitors, especially BBB-crossing ARBs, was associated with a reduction in the risk of PD in IHD patients. The risk of PD showed a decreasing trend with a higher cumulative duration of BBB-crossing ARBs. These findings could provide insight into the development of disease-modifying drug candidates for PD, adding to existing evidence.
Data Availability Statement
The data analyzed in this study was obtained from the Health Insurance Review and Assessment Service, the following licenses/restrictions apply: data used in this study are handled and stored by the Health Insurance Review and Assessment Service. Requests to access these datasets should be directed to https://www.hira.or.kr.
Ethics Statement
The studies involving human participants were reviewed and approved by Institutional Review Board of Yonsei University. Written informed consent for participation was not required for this study in accordance with the national legislation and the institutional requirements.
Author Contributions
YJ and SK contributed for study design, analysis and interpretation of data, and writing of the manuscript. YMY and EL contributed for study conceptualization, interpretation of data, critical revision of the manuscript, and supervision of the study. BSY contributed to clinical interpretation of data for the work and critical revision of the manuscript. All authors reviewed, amended, and approved the final manuscript submitted.
Funding
This work was supported by the National Research Foundation of Korea (NRF) grant funded by the Korea government (MSIT) (No. 2020R1G1A1101205) ※ MSIT: Ministry of Science and ICT.
Conflict of Interest
The authors declare that the research was conducted in the absence of any commercial or financial relationships that could be construed as a potential conflict of interest.
Publisher’s Note
All claims expressed in this article are solely those of the authors and do not necessarily represent those of their affiliated organizations, or those of the publisher, the editors and the reviewers. Any product that may be evaluated in this article, or claim that may be made by its manufacturer, is not guaranteed or endorsed by the publisher.
Acknowledgments
The authors are grateful to Prof. YoungSoo Kim for his helpful comments and discussion. This study was performed using the database from the Health Insurance Review and Assessment Service (M20200414461).
Supplementary Material
The Supplementary Material for this article can be found online at: https://www.frontiersin.org/articles/10.3389/fphar.2022.837890/full#supplementary-material
References
Alzahrani, Y. M., Alim A Sattar, M. A., Kamel, F. O., Ramadan, W. S., and Alzahrani, Y. A. (2020). Possible Combined Effect of Perindopril and Azilsartan in an Experimental Model of Dementia in Rats. Saudi Pharm. J. 28, 574–581. doi:10.1016/j.jsps.2020.03.009
Ana Flavia, A.-S., Lucas, M. K., and Jose, M. (2017). The Renin-Angiotensin System and the Neurodegenerative Diseases: A Brief Review. Protein Pept. Lett. 24, 841–853. doi:10.2174/0929866524666170822120258
Ascherio, A., and Schwarzschild, M. A. (2016). The Epidemiology of Parkinson's Disease: Risk Factors and Prevention. Lancet Neurol. 15, 1257–1272. doi:10.1016/S1474-4422(16)30230-7
Austin, P. C. (2009). Balance Diagnostics for Comparing the Distribution of Baseline Covariates between Treatment Groups in Propensity-Score Matched Samples. Stat. Med. 28, 3083–3107. doi:10.1002/sim.3697
Becker, C., Jick, S. S., and Meier, C. R. (2008). Use of Antihypertensives and the Risk of Parkinson Disease. Neurology 70, 1438–1444. doi:10.1212/01.wnl.0000303818.38960.44
Braszko, J. J. (2004). Involvement of D1 Dopamine Receptors in the Cognitive Effects of Angiotensin IV and Des-Phe6 Angiotensin IV. Peptides 25, 1195–1203. doi:10.1016/j.peptides.2004.04.014
Connolly, B. S., and Lang, A. E. (2014). Pharmacological Treatment of Parkinson Disease: A Review. JAMA 311, 1670–1683. doi:10.1001/jama.2014.3654
Culman, J., Blume, A., Gohlke, P., and Unger, T. (2002). The Renin-Angiotensin System in the Brain: Possible Therapeutic Implications for AT(1)-receptor Blockers. J. Hum. Hypertens. 16 (16 Suppl. l), S64–S70. doi:10.1038/sj.jhh.1001442
Davies, N. M., Kehoe, P. G., Ben-Shlomo, Y., and Martin, R. M. (2011). Associations of Anti-hypertensive Treatments with Alzheimer's Disease, Vascular Dementia, and Other Dementias. J. Alzheimers Dis. 26, 699–708. doi:10.3233/jad-2011-110347
Erro, R., Mencacci, N. E., and Bhatia, K. P. (2021). The Emerging Role of Phosphodiesterases in Movement Disorders. Mov Disord. 36, 2225–2243. doi:10.1002/mds.28686
Garrido-Gil, P., Joglar, B., Rodriguez-Perez, A. I., Guerra, M. J., and Labandeira-Garcia, J. L. (2012). Involvement of PPAR-γ in the Neuroprotective and Anti-inflammatory Effects of Angiotensin Type 1 Receptor Inhibition: Effects of the Receptor Antagonist Telmisartan and Receptor Deletion in a Mouse MPTP Model of Parkinson's Disease. J. Neuroinflammation 9, 38. doi:10.1186/1742-2094-9-38
Gebre, A. K., Altaye, B. M., Atey, T. M., Tuem, K. B., and Berhe, D. F. (2018). Targeting Renin-Angiotensin System against Alzheimer's Disease. Front. Pharmacol. 9, 440. doi:10.3389/fphar.2018.00440
Gironacci, M. M., Vicario, A., Cerezo, G., and Silva, M. G. (2018). The Depressor axis of the Renin-Angiotensin System and Brain Disorders: a Translational Approach. Clin. Sci. (Lond) 132, 1021–1038. doi:10.1042/cs20180189
Ho, J. K., Moriarty, F., Manly, J. J., Larson, E. B., Evans, D. A., and Rajan, K. B. Blood-Brain Barrier Crossing Renin-Angiotensin Drugs and Cognition in the Elderly: A Meta-Analysis. Hypertension (Dallas, Tex. : 1979) (2021) 78, 629–643. doi:10.1161/hypertensionaha.121.17049
Hu, L. Y., Yang, A. C., Lee, S. C., You, Z. H., Tsai, S. J., Hu, C. K., et al. (2020). Risk of Parkinson's Disease Following Gout: a Population-Based Retrospective Cohort Study in Taiwan. BMC Neurol. 20, 338. doi:10.1186/s12883-020-01916-9
Jafari, S., Etminan, M., Aminzadeh, F., and Samii, A. (2013). Head Injury and Risk of Parkinson Disease: a Systematic Review and Meta-Analysis. Movement Disorders. official J. Move. Disord. Soc. 28, 1222–1229. doi:10.1002/mds.25458
Jeong, S. M., Jang, W., and Shin, D. W. (2019). Association of Statin Use with Parkinson's Disease: Dose-Response Relationship. Movement Disorders. official J. Move. Disord. Soc. 34, 1014–1021. doi:10.1002/mds.27681
Kalia, L. V., Kalia, S. K., and Lang, A. E. (2015). Disease-modifying Strategies for Parkinson's Disease. Movement Disorders. official J. Move. Disord. Soc. 30, 1442–1450. doi:10.1002/mds.26354
Kang, S. Y., Lee, S. B., Kim, H. J., Kim, H. T., Yang, H. O., and Jang, W. (2017). Autophagic Modulation by Rosuvastatin Prevents Rotenone-Induced Neurotoxicity in an In Vitro Model of Parkinson's Disease. Neurosci. Lett. 642, 20–26. doi:10.1016/j.neulet.2017.01.063
Kim, C. K., Yang, X.-L., Kim, Y.-J., Choi, I.-Y., Jeong, H.-G., Park, H.-K., et al. (2015). Effect of Long-Term Treatment with Fimasartan on Transient Focal Ischemia in Rat Brain. Biomed. Res. Int. 2015, 295925. doi:10.1155/2015/295925
Kim, J. A., Yoon, S., Kim, L. Y., and Kim, D. S. (2017). Towards Actualizing the Value Potential of Korea Health Insurance Review and Assessment (HIRA) Data as a Resource for Health Research: Strengths, Limitations, Applications, and Strategies for Optimal Use of HIRA Data. J. Korean Med. Sci. 32, 718–728. doi:10.3346/jkms.2017.32.5.718
Kim, S., Yu, Y. M., Kwon, J., Jeong, K. H., Lee, J. S., and Lee, E. (2020). Trimetazidine Use and the Risk of Parkinsonism: A Nationwide Population-Based Study. Int. J. Environ. Res. Public Health 17, 17197256. doi:10.3390/ijerph17197256
Kumar, A., Sharma, N., Gupta, A., Kalonia, H., and Mishra, J. (2012). Neuroprotective Potential of Atorvastatin and Simvastatin (HMG-CoA Reductase Inhibitors) against 6-hydroxydopamine (6-OHDA) Induced Parkinson-like Symptoms. Brain Res. 1471, 13–22. doi:10.1016/j.brainres.2012.06.050
Labandeira-Garcia, J. L., Rodriguez-Perez, A. I., Valenzuela, R., Costa-Besada, M. A., and Guerra, M. J. (2016). Menopause and Parkinson's Disease. Interaction between Estrogens and Brain Renin-Angiotensin System in Dopaminergic Degeneration. Front. Neuroendocrinol 43, 44–59. doi:10.1016/j.yfrne.2016.09.003
Laperle, A. H., Sances, S., Yucer, N., Dardov, V. J., Garcia, V. J., Ho, R., et al. (2020). iPSC Modeling of Young-Onset Parkinson’s Disease Reveals a Molecular Signature of Disease and Novel Therapeutic Candidates. Nat. Med. 26, 289–299. doi:10.1038/s41591-019-0739-1
Lee, J., Jung, S. J., Choi, J. W., Shin, A., and Lee, Y. J. (2018). Use of Sedative-Hypnotics and the Risk of Alzheimer's Dementia: A Retrospective Cohort Study. PLoS One 13, e0204413. doi:10.1371/journal.pone.0204413
Lee, Y.-C., Lin, C.-H., Wu, R.-M., Lin, J.-W., Chang, C.-H., and Lai, M.-S. (2014). Antihypertensive Agents and Risk of Parkinson's Disease: A Nationwide Cohort Study. PLOS ONE 9, e98961. doi:10.1371/journal.pone.0098961
Levi Marpillat, N., Macquin-Mavier, I., Tropeano, A.-I., Bachoud-Levi, A.-C., and Maison, P. (2013). Antihypertensive Classes, Cognitive Decline and Incidence of Dementia: a Network Meta-Analysis. J. Hypertens. 31, 1073–1082. doi:10.1097/HJH.0b013e3283603f53
Li, C. H., Chen, W. C., Liao, W. C., Tu, C. Y., Lin, C. L., Sung, F. C., et al. (2015). The Association between Chronic Obstructive Pulmonary Disease and Parkinson's Disease: a Nationwide Population-Based Retrospective Cohort Study. QJM : monthly J. Assoc. Physicians 108, 39–45. doi:10.1093/qjmed/hcu136
Li, N. C., Lee, A., Whitmer, R. A., Kivipelto, M., Lawler, E., Kazis, L. E., et al. (2010). Use of Angiotensin Receptor Blockers and Risk of Dementia in a Predominantly Male Population: Prospective Cohort Analysis. Bmj 340, b5465. doi:10.1136/bmj.b5465
Li, Q., Wang, C., Tang, H., Chen, S., and Ma, J. (2018). Stroke and Coronary Artery Disease Are Associated with Parkinson’s Disease. Can. J. Neurol. Sci. /J. Canadien des Sci. Neurologiques 45, 559–565. doi:10.1017/cjn.2018.56
Lin, F. C., Tsai, C. P., Kuang-Wu Lee, J., Wu, M. T., and Tzu-Chi Lee, C. (2015). Angiotensin-converting Enzyme Inhibitors and Amyotrophic Lateral Sclerosis Risk: a Total Population-Based Case-Control Study. JAMA Neurol. 72, 40–48. doi:10.1001/jamaneurol.2014.3367
López-Sendón, J., Mena, M. A., and de Yébenes, J. G. (2013). Drug-induced Parkinsonism. Expert Opin. Drug Saf. 12, 487–496. doi:10.1517/14740338.2013.787065
Louis, E. D., Benito-León, J., and Bermejo-Pareja, F. (2009). Antihypertensive Agents and Risk of Parkinson's Disease, Essential Tremor and Dementia: a Population-Based Prospective Study (NEDICES). Neuroepidemiology 33, 286–292. doi:10.1159/000235641
Mertens, B., Vanderheyden, P., Michotte, Y., and Sarre, S. (2010). The Role of the central Renin-Angiotensin System in Parkinson's Disease. J. Renin Angiotensin Aldosterone Syst. 11, 49–56. doi:10.1177/1470320309347789
Mullapudi, A., Gudala, K., Boya, C., and Bansal, D. (2016). Use of Antihypertensive Agents and Risk of Parkinson's Disease: A Meta-Analysis of Observational Studies. Value in Health 19, A100. doi:10.1016/j.jval.2016.03.1719
Nthenge-Ngumbau, D. N., and Mohanakumar, K. P. (2018). Can Cyclic Nucleotide Phosphodiesterase Inhibitors Be Drugs for Parkinson’s Disease? Mol. Neurobiol. 55, 822–834. doi:10.1007/s12035-016-0355-8
Oka, Y., Nishikawa, K., Kito, G., Mayahara, H., Tanayama, S., Muto, H., et al. (1988). Delapril 6, 192–205. doi:10.1111/j.1527-3466.1988.tb00376.x
Okumura, M., Iwai, M., Ide, A., Mogi, M., Ito, M., and Horiuchi, M. (2005). Sex Difference in Vascular Injury and the Vasoprotective Effect of Valsartan Are Related to Differential AT2 Receptor Expression. Hypertension 46, 577–583. doi:10.1161/01.HYP.0000178564.14464.80
Oro, C., Qian, H., and Thomas, W. G. (2007). Type 1 Angiotensin Receptor Pharmacology: Signaling beyond G Proteins. Pharmacol. Ther. 113, 210–226. doi:10.1016/j.pharmthera.2006.10.001
Ouk, M., Wu, C.-Y., Rabin, J. S., Jackson, A., Edwards, J. D., Ramirez, J., et al. (2021). The Use of Angiotensin-Converting Enzyme Inhibitors vs. Angiotensin Receptor Blockers and Cognitive Decline in Alzheimer’s Disease: the Importance of Blood-Brain Barrier Penetration and APOE ε4 Carrier Status. Alzheimer's Res. Ther. 13, 43. doi:10.1186/s13195-021-00778-8
Ozacmak, V. H., Sayan, H., Cetin, A., and Akyıldız-Igdem, A. (2007). AT1 Receptor Blocker Candesartan-Induced Attenuation of Brain Injury of Rats Subjected to Chronic Cerebral Hypoperfusion. Neurochem. Res. 32, 1314–1321. doi:10.1007/s11064-007-9305-1
Park, J.-H., Kim, D.-H., Kwon, D.-Y., Choi, M., Kim, S., Jung, J.-H., et al. (2019). Trends in the Incidence and Prevalence of Parkinson’s Disease in Korea: a Nationwide, Population-Based Study. BMC Geriatr. 19, 320. doi:10.1186/s12877-019-1332-7
Perez-Lloret, S., Otero-Losada, M., Toblli, J. E., and Capani, F. (2017). Renin-angiotensin System as a Potential Target for New Therapeutic Approaches in Parkinson's Disease. Expert Opin. Investig. Drugs 26, 1163–1173. doi:10.1080/13543784.2017.1371133
Postuma, R. B., and Berg, D. (2016). Advances in Markers of Prodromal Parkinson Disease. Nat. Rev. Neurol. 12, 622–634. doi:10.1038/nrneurol.2016.152
Ray, B., Ramesh, G., Verma, S. R., Ramamurthy, S., Tuladhar, S., Mahalakshmi, A. M., et al. (2021). Effects of Telmisartan, an AT1 Receptor Antagonist, on Mitochondria-specific Genes Expression in a Mouse MPTP Model of Parkinsonism. Front. Biosci. (Landmark Ed. 26, 262–271. doi:10.52586/4942
Reardon, K. A., Mendelsohn, F. A., Chai, S. Y., and Horne, M. K. (2000). The Angiotensin Converting Enzyme (ACE) Inhibitor, Perindopril, Modifies the Clinical Features of Parkinson's Disease. Aust. New Zealand J. Med. 30, 48–53. doi:10.1111/j.1445-5994.2000.tb01054.x
Ritz, B., Rhodes, S. L., Qian, L., Schernhammer, E., Olsen, J. H., and Friis, S. (2010). L-Type Calcium Channel Blockers and Parkinson Disease in Denmark. Ann. Neurol. 67, 600–606. doi:10.1002/ana.21937
Rivas-Santisteban, R., Rodriguez-Perez, A. I., Muñoz, A., Reyes-Resina, I., Labandeira-García, J. L., and Navarro, G. (2020). Angiotensin AT1 and AT2 Receptor Heteromer Expression in the Hemilesioned Rat Model of Parkinson's Disease that Increases with Levodopa-Induced Dyskinesia. J. Neuroinflammation 17, 243. doi:10.1186/s12974-020-01908-z
Rodriguez-Perez, A. I., Valenzuela, R., Joglar, B., Garrido-Gil, P., Guerra, M. J., and Labandeira-Garcia, J. L. (2011). Renin Angiotensin System and Gender Differences in Dopaminergic Degeneration. Mol. Neurodegener 6, 58. doi:10.1186/1750-1326-6-58
Rugbjerg, K., Friis, S., Jørgensen, T. L., Ritz, B., Korbo, L., and Olsen, J. H. (2010). Risk for Parkinson's Disease Among Patients with Osteoarthritis: a Danish Cohort Study. Movement Disorders. official J. Move. Disord. Soc. 25, 2355–2360. doi:10.1002/mds.23274
Samii, A., Nutt, J. G., and Ransom, B. R. (2004). Parkinson's Disease. The Lancet 363, 1783–1793. doi:10.1016/S0140-6736(04)16305-8
Sekar, S., Mani, S., Rajamani, B., Manivasagam, T., Thenmozhi, A. J., Bhat, A., et al. (2018). Telmisartan Ameliorates Astroglial and Dopaminergic Functions in a Mouse Model of Chronic Parkinsonism. Neurotox Res. 34, 597–612. doi:10.1007/s12640-018-9921-3
Shin, H. W., and Chung, S. J. (2012). Drug-induced Parkinsonism. J. Clin. Neurol. (Seoul, Korea) 8, 15–21. doi:10.3988/jcn.2012.8.1.15
Sink, K. M., Leng, X., Williamson, J., Kritchevsky, S. B., Yaffe, K., Kuller, L., et al. (2009). Angiotensin-converting Enzyme Inhibitors and Cognitive Decline in Older Adults with Hypertension: Results from the Cardiovascular Health Study. Arch. Intern. Med. 169, 1195–1202. doi:10.1001/archinternmed.2009.175
Sullivan, J. C. (2008). Sex and the Renin-Angiotensin System: Inequality between the Sexes in Response to RAS Stimulation and Inhibition. Am. J. Physiol. Regul. Integr. Comp. Physiol. 294, R1220–R1226. doi:10.1152/ajpregu.00864.2007
Sumien, N., Cunningham, J. T., Davis, D. L., Engelland, R., Fadeyibi, O., Farmer, G. E., et al. (2021). Neurodegenerative Disease: Roles for Sex, Hormones, and Oxidative Stress. Endocrinology 162, bqab185. doi:10.1210/endocr/bqab185,
Takai, S., Jin, D., Sakaguchi, M., and Miyazaki, M. (2004). Significant Target Organs for Hypertension and Cardiac Hypertrophy by Angiotensin-Converting Enzyme Inhibitors. Hypertension Research. official J. Jpn. Soc. Hypertens. 27, 213–219. doi:10.1291/hypres.27.213
Torsney, K. M., Noyce, A. J., Doherty, K. M., Bestwick, J. P., Dobson, R., and Lees, A. J. (2014). Bone Health in Parkinson's Disease: a Systematic Review and Meta-Analysis. J. Neurol. Neurosurg. Psychiatry 85, 1159–1166. doi:10.1136/jnnp-2013-307307
Tryc, A. B., Goldbecker, A., Berding, G., Rümke, S., Afshar, K., Shahrezaei, G. H., et al. (2013). Cirrhosis-related Parkinsonism: Prevalence, Mechanisms and Response to Treatments. J. Hepatol. 58, 698–705. doi:10.1016/j.jhep.2012.11.043
Tysnes, O.-B., and Storstein, A. (2017). Epidemiology of Parkinson’s Disease. J. Neural Transm. 124, 901–905. doi:10.1007/s00702-017-1686-y
Vale, T. C., Barbosa, M. T., Resende, E. P. F., Maia, D. P., Cunningham, M. C. Q., Guimarães, H. C., et al. (2018). Parkinsonism in a Population-Based Study of Individuals Aged 75+ Years: The Pietà Study. Parkinsonism Relat. Disord. 56, 76–81. doi:10.1016/j.parkreldis.2018.06.030
Victor Teatini Ribeiro (2020). Leonardo Cruz de, S., and Ana Cristina Simões e, S. Renin-Angiotensin System and Alzheimer’s Disease Pathophysiology: From the Potential Interactions to Therapeutic Perspectives. Protein Pept. Lett. 27, 484–511. doi:10.2174/0929866527666191230103739
Wang, S., Mao, S., Xiang, D., and Fang, C. (2018). Association between Depression and the Subsequent Risk of Parkinson's Disease: A Meta-Analysis. Prog. neuro-psychopharmacology Biol. Psychiatry 86, 186–192. doi:10.1016/j.pnpbp.2018.05.025
Warda, A., Reese, J. P., Tanislav, C., and Kostev, K. (2019). The Association between Antihypertensive Therapy and the Incidence of Parkinson's Disease in Patients Followed in General Practices in Germany. Int. J. Clin. Pharmacol. Ther. 57, 483–488. doi:10.5414/cp203559
Wharton, W., Stein, J. H., Korcarz, C., Sachs, J., Olson, S. R., Zetterberg, H., et al. (2012). The Effects of Ramipril in Individuals at Risk for Alzheimer's Disease: Results of a Pilot Clinical Trial. J. Alzheimers Dis. 32, 147–156. doi:10.3233/jad-2012-120763
World Health Organization. (2021). ATC Classification index with DDDs. Available at: https://www.whocc.no/atc_ddd_index_and_guidelines/atc_ddd_index/[Accessed December 15, 2021].
Wright, J. W., and Harding, J. W. (2012). Importance of the Brain Angiotensin System in Parkinson's Disease. Parkinsons Dis. 2012, 860923. doi:10.1155/2012/860923
Wright, J. W., Kawas, L. H., and Harding, J. W. (2015). The Development of Small Molecule Angiotensin IV Analogs to Treat Alzheimer's and Parkinson's Diseases. Prog. Neurobiol. 125, 26–46. doi:10.1016/j.pneurobio.2014.11.004
Wu, J.-Y., Lee, M.-T. G., Lee, S.-H., Lee, S.-H., Tsai, Y.-W., Hsu, S.-C., et al. (2016). Angiotensin-Converting Enzyme Inhibitors and Active Tuberculosis: A Population-Based Study. Medicine 95, e3579. doi:10.1097/MD.0000000000003579
Yagi, S., Akaike, M., Ise, T., Ueda, Y., Iwase, T., and Sata, M. (2013). Renin-angiotensin-aldosterone System Has a Pivotal Role in Cognitive Impairment. Hypertension Research. official J. Jpn. Soc. Hypertens. 36, 753–758. doi:10.1038/hr.2013.51
Keywords: Parkinson’s disease, renin-angiotensin system, myocardial ischemia, neuroprotective agents, pharmacoepidemiology
Citation: Jo Y, Kim S, Ye BS, Lee E and Yu YM (2022) Protective Effect of Renin-Angiotensin System Inhibitors on Parkinson’s Disease: A Nationwide Cohort Study. Front. Pharmacol. 13:837890. doi: 10.3389/fphar.2022.837890
Received: 17 December 2021; Accepted: 14 February 2022;
Published: 03 March 2022.
Edited by:
Elisabetta Poluzzi, University of Bologna, ItalyReviewed by:
Luisa Sambati, University of Bologna, ItalyJose L. Labandeira-Garcia, University of Santiago de Compostela, Spain
Copyright © 2022 Jo, Kim, Ye, Lee and Yu. This is an open-access article distributed under the terms of the Creative Commons Attribution License (CC BY). The use, distribution or reproduction in other forums is permitted, provided the original author(s) and the copyright owner(s) are credited and that the original publication in this journal is cited, in accordance with accepted academic practice. No use, distribution or reproduction is permitted which does not comply with these terms.
*Correspondence: Yun Mi Yu, eXVubWl5dUB5b25zZWkuYWMua3I=; Euni Lee, ZXVuaWxlZUBzbnUuYWMua3I=
†ORCID: Yun Mi Yu, orcid.org/ 0000-0002-8267-9453; Euni Lee, orcid.org/ 0000-0002-4487-4450
‡These authors have contributed equally to this work and share first authorship