- Department of Breast Surgical Oncology, National Cancer Center/National Clinical Research Center for Cancer/Cancer Hospital, Chinese Academy of Medical Sciences and Peking Union Medical College, Beijing, 100021, China
Immune checkpoint inhibitors (ICIs) induce T-cell activation against cancer cells, and due to their anti-tumor function in multiple cancers, ICIs have been considered an important option for oncotherapy. PD-1/PD-L1 inhibitors are now widely used as ICIs for many types of cancers in clinical practices. Myocarditis induced by anti-PD-1/PD-L1 agents is uncommon but shows potentially fatal toxicity. In this review, we attempted to conclude the incidence, characteristics, diagnosis, and treatments, as well as illustrate the potential pathogenesis from the perspectives of T-lymphocyte infiltration, disturbance of regulatory T cells, cytokines, macrophage-mediated inflammatory response, and synergistic effect of PD-1/PD-L1 and CTLA4.
1 Introduction
PD-1/PD-L1, the immune checkpoints, produce a negative regulation of T-cell function that maintains the balance between T-cell activation, tolerance, and immune-mediated tissue damage and also control the induction and maintenance of immune tolerance in the tumor microenvironment (Li et al., 2018; Han et al., 2020). PD-1, also called CD279 and homologous to the CD28, is a 288-amino acid type I transmembrane protein receptor (95 amino acids composing intracellular domain), which is encoded by the PDCD 1 gene that is located on chromosome 2. PD-L1, also known as CD 274, is a 290-amino acid protein receptor (with cytoplasmic 30 residues), which is encoded by the CD274 gene that is located on chromosome 9 (Ishida et al., 1992; Freeman et al., 2000; Keir et al., 2008). PD-1 is expressed on so many functional cells, like macrophages, activated T lymphocytes, natural killer lymphocytes, dendritic cells (DCs), B lymphocytes, and monocytes, whereas the ligand of PD-1 is usually located on activated B cells and T cells, DCs, and some epithelial cells. In the tumor microenvironment, PD-1 can be highly expressed on tumor-specific T cells, and PD-L1 can also be expressed on tumor cells (Ahmadzadeh et al., 2009). The combination of PD-1/PD-L1 can lead to attenuation of activated tumor-specific T cells, causing adaptive immunity and maintaining immune tolerance in the tumor microenvironment (Bivona et al., 2003; Patsoukis et al., 2013; Wang and Wu, 2020). Tumor cells can utilize this immune homeostasis to escape the immune surveillance, promoting malignant tumor dilation (Li et al., 2018; Salmaninejad et al., 2018). With the understanding of the immune-related mechanism in the process of tumor genesis and development, immunotherapy has become an important part of comprehensive tumor therapy. Recently, the immune checkpoint inhibitors have been widely used in tumor immunotherapy. Monoclonal antibody treatments of programmed cell death-1 (PD-1) and programmed death-ligand 1 (PD-L1) have shown promising therapeutic effects in many cancers, including melanoma, non-small cell lung cancer (NSCLC), renal cell carcinoma, head and neck squamous cell carcinoma, urothelial cancer, refractory Hodgkin lymphoma, breast cancer, and malignancies with microsatellite instability (Motzer et al., 2015; Ferris et al., 2016; Bellmunt et al., 2017; Lee et al., 2017; Hu J. R. et al., 2019). However, T cells may simultaneously cause damage to normal tissues, which subsequently result in immune-related adverse events (irAEs) (Cousin and Italiano, 2016; Hu J. R. et al., 2019; Su et al., 2020). As PD-1/PD-L1 inhibitors induce the immune cells to attack normal tissues, many organs can be damaged, such as skin, lungs, heart, muscles, bowels, liver, endocrine tissues, eyes, kidneys, as well as central nervous system (Naidoo et al., 2015; Ferris et al., 2016; Bellmunt et al., 2017; Hahn et al., 2017; Qin et al., 2019; Su et al., 2020). According to meta-analyses, myocarditis is one of the less common but with more fatal types in immune checkpoint inhibitor treatment. About 1% of patients were reported with myocarditis, but deaths due to immune myocarditis accounted for a large proportion of deaths induced by irAEs, ranging from 27 to 46% (Wang et al., 2018; Rubio-Infante et al., 2021). Myocarditis refers to the alterations in the number and function of lymphocyte subsets and macrophages and antibody-mediated injury in cardiomyocytes (Sagar et al., 2012). Also, T lymphocyte infiltration can be found in most cases with PD-1/PD-L1 inhibitor-associated myocarditis. Like normal myocarditis, it can also manifest diverse symptoms, like cardio-specific or non-specific symptoms (Sagar et al., 2012; Martín et al., 2021; Thompson et al., 2021). But the incidence, diagnosis, and other detailed information of it have been still unclear (Norwood et al., 2017). There is a need to better understand the clinical characteristics of myocarditis with high lethality, particularly, in an environment where more and more cancers can be treated by PD-1/PD-L1 inhibitors. In this article, we aimed to review the incidence, pathogenesis, clinical characteristics, diagnosis, and treatment of cardiotoxicity of PD-1/PD-L1 inhibitors. PD-1/PD-L1 inhibitor-associated myocarditis is a kind of immune checkpoint inhibitor-related myocarditis. Under the circumstances that there is no study specialized for PD-1/PD-L1-related myocarditis, this review has summarized the current cohort studies, case reports, or case series that focus on PD-1/PD-L1 inhibitors or have a large proportion of PD-1/PD-L1 inhibitors in immune checkpoint inhibitor treatment, with the proportion ranging from 63 to 86% (Escudier et al., 2017; Mahmood et al., 2018; Salem et al., 2018; Wang et al., 2018; Awadalla et al., 2020). The structure of this review is shown in Figure 1.
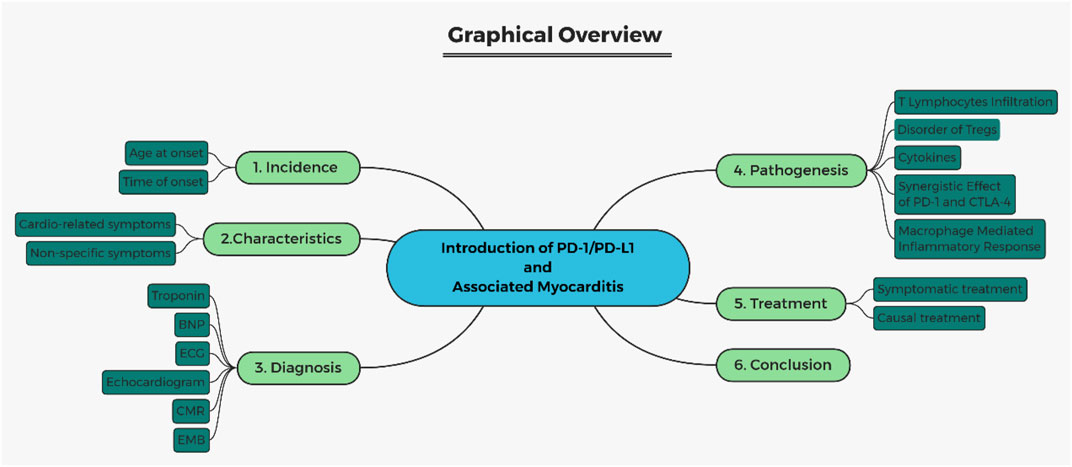
FIGURE 1. Graphical structure overview. BNP, brain natriuretic peptide; ECG, electrocardiogram; CMR, cardiovascular magnetic resonance; EMB, endomyocardial biopsy.
2 Incidence
Nestor et al. conducted an immune checkpoint inhibitor pharmacovigilance study and reported a number of 4,401 cardiac disorder records (4.2% of the total 104,276 records concerning adverse drug reactions) based on VigiBase (the WHO’s pharmacovigilance database). In their study, PD-1 inhibitors (nivolumab® and pembrolizumab®) have induced the majority of cardiac disorders. Numerically, at least 80% (676/839 patients) of the total cardiac disorders had a relationship with the treatment of anti-PD-1/PD-L1 axis treatment. Eight hundred thirty-nine patients were confirmed with PD-1/PD-L1 inhibitor-related myocarditis, accounting for 15.4% of all cardiac disorders; 0.65% of all irAEs are caused by immune checkpoint inhibitor treatment (Rubio-Infante et al., 2021). Furthermore, using the same database, Salem et al. concluded similar outcomes that the incidence of anti-PD-1/PD-L1 treatment-related myocarditis was only 0.41% (84/28,909 patients). But with the combination of CTLA4 inhibitors, the myocarditis has tripled to 1.33% (32/2,412 patients) (94.6% cases in this study received PD-1/PD-L1 antibodies) (Salem et al., 2018).
Moslehi et al. reported that patients with severe myocarditis treated with immune checkpoint inhibitors showed a wide range of age at the onset, with a median of 69 years (from 20 to 90 years old), most commonly happened in melanoma and lung cancer (partially due to the selection bias in this study). The main onset is 27 days (widely from 5–155 days), and 76% occurred in the first 6 weeks of treatment (Moslehi et al., 2018). Mahmood found that the mean onset age of patients (n = 35) with immune myocarditis was 65 years. The mean time to onset in this study was 34 days, with 81% of cases happening within 90 days of starting the treatment (Mahmood et al., 2018). Salem et al. concluded that median onset day of myocarditis was 30 days, and most cases happened early after immune checkpoint inhibitor administration. But there was a dramatic decrease in the proportion of immune checkpoint inhibitors associated with significant cardiovascular irAEs after 3 months, reducing from about 70% to nearly 20% (Salem et al., 2018). This result was similar to what Moslehi and Mahmood have found, manifesting that the age of onset was about 65–70 years, usually owning an acute course within 1–3 months. Moslehi and Salem both confirmed that mortality was higher with a combination of anti-PD-1/PD-L1 plus anti-CTLA4 than with monotherapy (67 vs 36%, p = 0.008; 65.6 vs 44.4%, p = 0.04). In general, the results of multiple clinical studies indicate that PD-1/PD-L1 inhibitors related myocarditis is relatively rare. The age of onset is mostly 65–70 years old, and the time of onset is mostly in the early stage of administration. The age of onset and time of onset of immune myocarditis caused PD-1/PD-L1 inhibitors are summarized in Table 1.

TABLE 1. Summary of onset time and age of myocarditis caused by PD-1/PD-L1 inhibitors in retrospective studies.
In addition, we have investigated some studies to find the incidence of other cardiac diseases (shown in Table 2). Based on the fact that those studies were not all specifically designed for PD-1/PD-L1 inhibitors, we have tried to extract PD-1/PD-L1 inhibitors associated data from some reviews (Mir et al., 2018), meta-analysis (Rubio-Infante et al., 2021), RCTs, and pharmacovigilance studies (Robert et al., 2015; Antonia et al., 2017; Barlesi et al., 2018; Horn et al., 2018; Salem et al., 2018; Jain et al., 2021).
3 Clinical Characteristics
Since PD-1/PD-L1 inhibitors associated with cardiotoxicity may progress rapidly and cause hemodynamic instability, clinicians should maintain a high degree of alertness to patients receiving immunotherapy and showing symptoms of heart failure. Early detection of cardio-related symptoms is particularly important to improve the prognosis of patients. The symptoms of immune myocarditis are diverse. It can also be manifested as chest pain, palpitations, myalgia, hypotension, heart failure, complete heart block, and lower extremity edema (Frigeri et al., 2018; Yamaguchi et al., 2018; Charles et al., 2019), while some cases only manifested as non-specific symptoms such as fatigue, general malaise, ptosis, diplopia, paresis, asthenia, dyspnea, stiffness, rash, vomiting, and nausea (Gibson et al., 2016; Chen et al., 2018; Monge et al., 2018; Salem et al., 2018; Agrawal et al., 2019; Martin Huertas et al., 2019; Salem et al., 2019). Severe cases manifest major adverse cardiovascular events (MACE) as hemodynamically unstable and/or electrophysiologically unstable fulminant myocarditis, cardiogenic shock and death, cardiac arrest, and heart block, while mild cases may only have elevated cardiac markers without symptoms (Johnson et al., 2016; Escudier et al., 2017; Ganatra and Neilan, 2018; Finke et al., 2021). Mahmood has concluded in their published study that nearly half of myocarditis cases (16/35 cases) have experienced MACE (Mahmood et al., 2018). Because of the high fatality rate of myocarditis, early detection and diagnosis of myocarditis is the key to the treatment. The clinical characteristics of 29 previously reported myocarditis cases caused by PD-1/PD-L1 inhibitors are summarized in Table 3.
4 Diagnosis
The incidence of immune myocarditis is still unclear, which has ranged from 0.39 to 0.8% (Johnson et al., 2016; Salem et al., 2018). Ganatra et al. assumed that the incidence of true immune myocarditis may be underestimated due to multiple factors, including lack of specific cardio-related symptoms, possibility of overlap with other cardiovascular diseases, non-standard, and non-specific diagnostic measurements (Ganatra and Neilan, 2018). The common clinical diagnostic methods for myocarditis include symptoms, electrocardiogram, biomarkers (creatine kinase isoenzyme, troponin I, brain natriuretic peptide, etc.), and cardiac imaging (echocardiography and cardiac magnetic resonance). Endomyocardial biopsy has been regarded as the diagnostic gold standard for PD-1/PD-L1 inhibitor-associated myocarditis.
4.1 Troponin
According to the characteristics of troponins, the type of cardiac troponin I and T are the new isoforms that are present in adult cardiac tissues (Yu et al., 2021). About 46–97% of patients with immune myocarditis have increased serum troponin levels (Escudier et al., 2017; Mahmood et al., 2018; Mir et al., 2018; Awadalla et al., 2020; Finke et al., 2021). In Awadalla’s study, the troponin levels of patients with immune checkpoint inhibitor treatment were elevated, with a median value of 0.85 ng/dl. But the troponin levels were <0.01 ng/dl in the control group (p < 0.001). Mahmood advocated that troponin T of ≥1.5 ng/ml may be related to a fourfold increased risk of MACE caused by immune myocarditis. Cardiac troponin T (cTnT) is also increased in patients with myositis but cardiac troponin I (cardiac troponin I, cTnI) is more specific to myocardial injury (Lilleker et al., 2018). Cardiac tissue damage, like cell death or necrosis with myofilament injuries, will inevitably cause cTn elevation. Meanwhile, the severity of cardiac damage can be reflected by the level of increased cTn (Bass et al., 2010). Mir and Heinzerling have reported that some cases with PD-1/PD-L1 inhibitor immunotherapy-related heart failure, cardiomyopathy, myocarditis, and myocardial fibrosis may manifest normal troponin levels (Heinzerling et al., 2016; Mir et al., 2018). Unfortunately, there was no study that can tell us that the specific troponin level can play a role in predicting PD-1/PD-L1 inhibitor-associated myocarditis.
4.2 Brain Natriuretic Peptide
Based on the present study, serum brain natriuretic peptide (BNP) or N-terminal-pro brain natriuretic peptide (NT-pro BNP) levels increased in 65.71–88% of patients with immune checkpoint inhibitor-related myocarditis (Mahmood et al., 2018; Awadalla et al., 2020). Escudier reported 100% patients with elevated BNP or NT-pro BNP in their study with a limited sample size (n = 14, the BNP or NT-pro BNP information can be obtained from 14 out of 30 patients) (Escudier et al., 2017). The ventricular expansion and volume overload can activate the natriuretic peptide system to promote the secretion of BNP. BNP is a specific biomarker reflecting the pressure or overload. BNP can indirectly tell us the function of the left ventricular (LV) strain in patients with heart failure or myocarditis (Yu et al., 2021). All the pathogenesis which can lead to dysfunction of LV can also result in elevated BNP levels. By the way, elevated serum BNP in cancer patients can also be caused by cancer-related chronic inflammation (Bando et al., 2017). In many different cardiovascular conditions, like myocarditis, BNP and NT-proBNP are sensitive biomarkers in predicting heart failure (Elamm et al., 2012). In view of the fact that BNP is a biological marker of left ventricular systolic function, we propose a hypothesis whether BNP can be used to predict the existence of subclinical functional and morphological damage of the left ventricle in cancer patients with PD-1/PD-L1 inhibitors.
4.3 Electrocardiogram
Based on the universality of the electrocardiogram (ECG) examination, Ganatra suggested that the ECG should be routinely performed for suspected immune myocarditis (Ganatra and Neilan, 2018). ECG performances in PD-1/PD-L1 inhibitor-associated myocarditis cases were neither specific nor sensitive, such as sinus tachycardia, QRS/QT prolongation, conduction abnormalities, diffuse T-wave inversion, Q waves, ventricular arrhythmias, non-specific ST-segment changes, and local or diffuse ST-T elevation like the manifestation of acute coronary syndrome and conduction disorders (Johnson et al., 2016; Ammirati et al., 2017). It is difficult to make a diagnosis of myocarditis only relying on ECG. But given its availability and simplicity, it should be the first-line test for cancer patients with cardiac symptoms.
4.4 Echocardiogram
Echocardiogram has been considered as a useful tool for assessing the cardiac function and ruling out other cardiovascular diseases, with the limitation that cannot manifest tissue characteristics and detect small myocardial abnormalities. Decreased left ventricular ejection fraction (LVEF), abnormal left ventricular internal dimensions in diastole, and abnormal motion of the ventricular wall can be manifested by echocardiogram in PD-1/PD-L1 inhibitor-related myocarditis cases. About 40–49% of patients have decreased left ventricular systolic function (Escudier et al., 2017; Mahmood et al., 2018; Awadalla et al., 2020). Escudier reported that nearly half of the patients had a significant decrease in LVEF (≤35%) (Escudier et al., 2017). Awadalla et al. showed that cases with decreased LVEF (median LVEF was 45%) had more possibility to develop MACE compared to the normal myocarditis patients with normal LVEF (median LVEF was 55%) (Awadalla et al., 2020). Different from the traditional fulminant myocarditis with a significant decrease in LVEF (Ammirati et al., 2017; Zhang et al., 2020), 38% of patients with fulminant myocarditis caused by immune checkpoint inhibitors had normal LVEF (Mahmood et al., 2018). Therefore, it may be not safe to rule out severe PD-1/PD-L1 inhibitor fulminant myocarditis according to the normal LVEF in echocardiogram.
4.5 Cardiovascular Magnetic Resonance
Cardiovascular magnetic resonance (CMR) can provide better spatial resolution and tissue specificity than echocardiogram; show myocardial edema, necrosis, and scar formation caused by myocarditis; and can manifest left ventricular systolic function. Myocardial necrosis and fibrosis are manifested by late gadolinium enhancement (LGE) (Frigeri et al., 2018; Zhang et al., 2020; Finke et al., 2021). Although CMR has a sensitivity of 89% and a specificity of 96% in the diagnosis of other forms of myocarditis (Yang and Asnani, 2018), the incidence of LGE happened in immune checkpoint inhibitor-related myocarditis ranging from 23 to 48% (Escudier et al., 2017; Zhang et al., 2020). Escudier found only 3 cases with LGE out of 13 patients, making the incidence less convinced. Zhang et al. concluded that LGE increased from 21.6 to 72.0% with prolonged hospitalization. We wonder whether LGE has relationship with the time when CMR was completed, so the incidence of LGE need to be confirmed by randomized control trials with large sample size.
4.6 Endomyocardial Biopsy
Endomyocardial biopsy (EMB) is the gold standard for diagnosing myocarditis (Guglin and Nallamshetty, 2012; Sagar et al., 2012). The pathological feature of PD-1/PD-L1 inhibitors related was T-cell infiltration. Mostly, the infiltrating cells were CD8+ T and CD4+ T cells. Macrophages were also common. Some cases may have eosinophil, giant cells, and neutrophils infiltration (Heinzerling et al., 2016; Johnson et al., 2016; Mahmood et al., 2018; Champion and Stone, 2020). T-lymphocyte infiltration can be found in the atria, ventricles, cardiac conduction system, and interventricular septum. In addition, necrosis, edema, or fibrosis of the myocardium can also be found in the cardiac tissues with immune myocarditis (Heinzerling et al., 2016; Johnson et al., 2016; Norwood et al., 2017; Matson et al., 2018; Champion and Stone, 2020; Sobol et al., 2020). Although EMB is the most accurate diagnostic method for PD-1/PD-L1 inhibitor-related myocarditis, the operation was really accompanied by certain risks, such as transient arrhythmia, pericardial tamponade, and myocardial perforation (Yang and Asnani, 2018). Champion and Stone have proclaimed that the myocyte injury caused by myocarditis was in discrete foci, with no case with diffuse myocarditis throughout the whole specimen (Champion and Stone, 2020). So under this setting, EMB may provide us the false-negative results (Agrawal et al., 2019).
5 Pathogenesis
5.1 Autoimmunity-Related T-Lymphocyte Infiltration
As mentioned above, activated T cells can express one kind of immune-inhibitory checkpoint on their surface named PD-1. When PD-1 binds to its ligands, T cells lose their functional abilities. This interaction is a kind of significantly important immunologic tolerance mechanism in the peripheral tissues and at sites of ongoing inflammation (Fife and Bluestone, 2008). Under this circumstance, even auto-activated T cells flow into the myocardium; PD-1 signaling can restrict T cells, making immune homeostasis (Yi et al., 2021). PD-L1 has been already confirmed to express on the cardiac tissue (Dong et al., 1999; Freeman et al., 2000; Yamaguchi et al., 2018). Douglas and others have carried out T-cell receptor next-generation sequencing (CDR3 region, the antigen-binding portion of the T-cell receptor beta chain), indicating that patients with PD-1/PD-L1 inhibitors related myocarditis have their own high-frequency T-cell receptor sequences among cardiac, skeletal muscle, and tumor tissue (Johnson et al., 2016). PD-1/PD-L1 monoclonal antibodies block PD-1 or its ligand, resulting in invigoration of these quiescent T cells again. That was helpful for the T cells to eliminate the tumor cells, at the same time providing activated T cells a possibility to non-selectively attack the normal cells. The autoimmune phenomenon may account for the immune-related adverse events (irAEs) (Cousin and Italiano, 2016). Carolyn and Douglas have previously reported some cases of myocarditis induced by PD-1/PD-L1 inhibitor treatment, with strong evidence of lymphocytic infiltration when the PD-1\PD-L1 axis was cut (Johnson et al., 2016; Glass and Mitchell, 2017), which was consistent with the histological examination in myocarditis cases (Tarrio et al., 2012; Heinzerling et al., 2016; Chen et al., 2018; Ganatra and Neilan, 2018; Mir et al., 2018; Yamaguchi et al., 2018; Martin Huertas et al., 2019). Ji et al. (2019) found mononuclear cell infiltration in myocardium with myocarditis in monkeys, with a large proportion of T cells (CD3+, CD4+, and CD8+). Heinzerling have found the same pathological manifestation in their cases (Heinzerling et al., 2016). Hyperproliferation of T cells happened in the monkey model with PD-1 inhibition treatment. Wang et al. have found that MRL-PD-1−/− mice had more possibility to develop fatal myocarditis with antibodies against cardiac myosin. They have also investigated T cells in the hearts of MRL-PD-1−/− mice but not in the spleen and lymph nodes were activated, which suggested that antigen-specific autoimmune response may mediate the myocarditis (Wang et al., 2010). Muath and his colleagues have investigated PD-1/PD-L1 expression in human transplant hearts. In the rejecting hearts caused by vasculopathy, PD-L1 expression was nearly absent in the myocardium, instead PD-1 expression was high. Meanwhile, PD-1 and PD-L1 owned similar and moderate expression levels in non-rejection allografts. By the way, PD-1/PD-L1 inhibitor agents blocking the specific immune checkpoint will achieve the disproportionate expression level between PD-1 and PD-L1, making the combination of PD-1/PD-L1 impossible with the unmatched numbers. Muath provided us a new perspective that the different expression levels between PD-1/PD-L1 (increased PD-1, decreased PD-L1) may be the trigger to cause the autoimmune responses in the myocardium (Bishawi et al., 2021).
5.2 Disorder of Regulatory T-Cell Homeostasis
Regulatory T cells (Tregs) suppress autoimmunity mainly through inhibitory receptors, like CTLA4 and PD-1. And the deficiency of these inhibitory receptors can result in the dysfunction of Tregs. PD-L1 has been testified to induce and maintain Tregs to inhibit T-cell function (Francisco et al., 2009; Zhang et al., 2016). PD-1 absence with forkhead box P3 (FoxP3) insufficiency can cause generation of ex-FoxP3 (FoxP3 expression) T cells with pro-inflammatory properties, accompany the expansion of effector T cells (Teffs) and memory T cells (Tregs), thereby promoting the autoimmune destruction of target tissues (Zhang et al., 2016; Tajmir-Riahi et al., 2018). Genetic or environmental disturbances that influence both PD-1 and Foxp3 will lead to the disruption of T-cell homeostasis, causing lethal autoimmune disease (Zhang et al., 2016). Alissafi et al. have reported that Foxp3+ Tregs may suppress the autoimmune via inhabitation of autophagic machinery of dendritic cells in the CTLA4-mediated pathway. And anti-CTLA4 treatment can disrupt the interaction of DCs and Tregs, making autoimmune aggravation (Alissafi et al., 2017). Foxp3+ Tregs are the fundamental stone of immune homeostasis. PD-1/PD-L1 inhibitors disturb Tregs, resulting in imbalance between Teffs and Tregs in normal tissues, which may be the origin of irAEs, consistent with the myocardial biopsy result found by Laubli which stated that only a few Foxp3+ Tregs were found in myocardial tissue with signs of apoptosis (Läubli et al., 2015; Kumar et al., 2020).
5.3 Cytokines
Rodig et al. have found that tumor necrosis factor-α (TNF-α) and interferon-γ (IFN-γ) can stimulate PD-L1 expressed on endothelial cells, with the inhibition of T cells (Rodig et al., 2003). Grabie et al. have also testified that myocarditis induced by cytotoxic T-lymphocyte was accompanied by the upregulation of PD-L1 expressed on cardio-endothelia. And the PD-L1 was upregulated by the stimulation of interferon-γ. So anti-IFN-γ treatment can lead to high expression of PD-L1, finally causing aggravation of myocarditis (Grabie et al., 2007). Meanwhile, the PD-L1/2−/− mice were more susceptible to severe myocarditis. And the same outcomes can be obtained via PD-L1 blocking treatment (Rodig et al., 2003). Seko et al. have also found that IFN-γ secreted from infiltrating lymphocytes can induce PD-L1 expression in myocarditis. They also testified that anti-PD-1 monoclonal antibodies can increase the level of IFN-γ, Fas ligand, pore-forming protein (PFP), which have been confirmed to promote the inflammatory process. IFN-γ, Fas ligand, and PFP potentially become the activator for the infiltrating lymphocytes in myocarditis (Seko et al., 2007). Zheng et al. (2018) have confirmed that the overexpression of fibrinogen-like protein-2 (FGL2) can significantly reduce PD-1 and aggravated cardiac function in mice with immune-related myocarditis. And they also found that forkhead box P3 (Foxp3) significantly increased in those mouse models, which was considered to collaborate with PD-1 to maintain and regulate the function of Tregs (Zhang et al., 2016). Finke has found that upregulation of the IFN-γ pathway can assemble the NLRP3 inflammasome (Finke et al., 2021), which has been testified to increase the number of infiltrating CD8+ T cells (Theivanthiran et al., 2020). Cytokines are reservoirs for mechanism research, and the levels of various cytokines can regulate the expression of PD-1 or PD-L1, thereby affecting the PD-1/PD-L1 axis.
5.4 Synergistic Effect of PD-1/PD-L1 and CTLA4
APCs will capture the neoantigens that are released by tumor cells. Through the major histocompatibility complex I (MHC I) molecule/TCR signal pathway, APCs transfer the peptides to CD8+ cytotoxic T cells. APCs can also present peptides through the MHC II pathway to CD4+ T helper cells. T cells activation relying on TCR signaling needs the help of CD28, which is costimulatory signals expressed on the surface of T cells. Upregulated T-lymphocyte-associated antigen 4 (CTLA4) on the surface of activated T cells, which may compete with CD28 for binding to CD80 and/or CD86. The conjunction of CTLA4 with CD80 or CD86 results in attenuation of T cells. So the CTLA4 checkpoint inhibitor can enhance immune responses against tumor cells that can express neoantigen, leading to tumor cells being killed (Tarrio et al., 2012; Grabie et al., 2007; Varricchi et al., 2017). Spencer has testified that there was a dosage-dependent genetic and functional interaction between the T cell with genes Ctla4 and Pdcd1. Compared to Ctla4+/+ Pdcd1−/− mice, the Ctla4+/− Pdcd1−/− mice have increased immune infiltration and cardiomegaly, confirming the gene dosage of Ctla4 and Pdcd1 together defining the functional up-limit of T cells (Wei et al., 2021). It has been testified that CTLA4 and PD-1 can cooperate to attenuate T-cell activation, with cellular and molecular regulation (Chen and Flies, 2013; Wei et al., 2018; Chemnitz et al., 2004). Therefore, T-cell hyperfunction caused by monoclonal antibody treatment of CTLA4 and PD-1 is an indisputable fact. And the blockages of PD-1/PD-L1 and CTLA4 can synergistically enhance each other. The attenuation and activation of T lymphocytes via CTLA4 and PD-1/PD-L1 axes are demonstrated in Figure 2. In clinical studies, it has been proved that mortality was higher with a combination of anti-PD-1/PD-L1 plus anti-CTLA4 than with monotherapy (67 vs 36%, p = 0.008) (Moslehi et al., 2018; Wei et al., 2021).
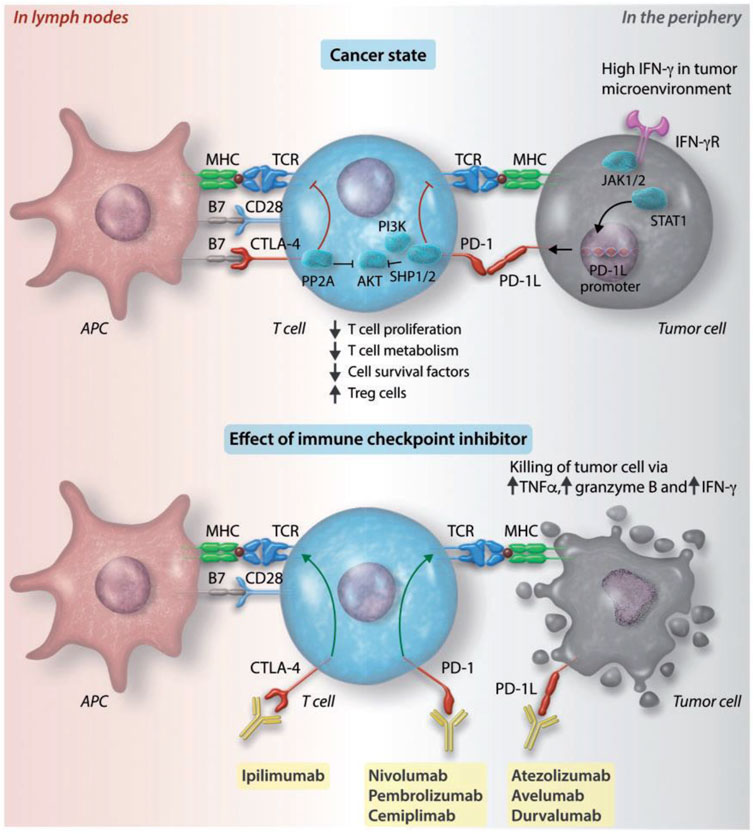
FIGURE 2. Attenuation and activation of T lymphocytes via CTLA4 and PD-1/PD-L1 axes. APCs can capture neoantigens and present to T lymphocytes on MHC molecules. The activated T cells, receiving the neoantigens, will migrate to the tumor bed from lymph nodes. The activation of T cells can be accelerated by costimulatory signals via the combination of B7 with CD28. The attenuation of T cells is managed by inhibitory axes, which are CTLA4/B7 and PD-1/PD-L1. The tumor cells can recognize the IFN-γ via the IFN-γ receptor, which promotes the expression of PD-L1 on their surfaces. PD-L1 can help tumor cells to escape the attack of T cells. The monoclonal antibodies of those immune checkpoints, like CTLA4 (ipilimumab®), PD-L1 (atezolizumab®, avelumab®, and durvalumab®), and PD-1 (nivolumab®, pembrolizumab®, and cemiplimab®), can stop the attenuation of T cells, making activated T cells to attack tumor cells. Modified and reprinted from Han J. R. et al. (2019). Copyright © The Author(s). 2019. Reproduction with permission from The Author(s). 2019 Open Access.
5.5 Macrophage-Mediated Inflammatory Response
Macrophage has been proved to play a vital role in controlling inflammation. Its subtypes M1 macrophages can secrete cytokines to accelerate inflammatory responses, while M2 macrophages suppress inflammation and promote scar formation (Shivshankar et al., 2014). Hu et al. manifested that human kallikrein can reduce cardiac fibrosis related to cardiac damage or dysfunction not only by reducing, gathering, or trapping the activated M2 macrophages but also through decreased shift of macrophages to M2 (Hu D. et al., 2019). The CD68+ macrophages have been found in the PD-1/PD-L1 inhibitors related case (Johnson et al., 2016; Norwood et al., 2017; Mir et al., 2018; Liu et al., 2019). Xia et al. have testified that cardio-injury caused by PD-1/PD-L1 inhibitors may be explained via exosomes that derived from PD-1 inhibitor-treated macrophages (ExosomePD−1 inhibitor), which can lead to cardiomyocyte senescence (damage or dysfunction). In their murine models, Xia found a significant decrease in the left ventricular ejection fraction and fractional shortening in the PD-1 inhibitor group by echocardiography. More myocardial cells stuck in the G0/G1-phase were found in the PD-1 inhibitor group and a higher percentage of senescence-associated β-galactosidase (SA-β-gal) staining positive cells. On the contrary, telomere length and telomerase activity-β decreased. When miR-34a-5p inhibition was adopted in PD-1 inhibitor-treated macrophages, the percentage of G0/G1-phase cells and the number of SA-β-gal-positive cells decreased, while accompanying the recovery of the telomere length and telomerase activity. Finally, they hypothesized that ExosomePD−1 inhibitor may exert a pro-senescent effect through modulating the miR-34a-5p/PNUTS signaling pathway (Xia et al., 2020a). Xia et al. (2020b) have also found that the PD-1 inhibitor may promote M1 macrophage polarization and cardiac injury by modulating the miR-34a/KLF4 (Krüppel-like factor 4) signaling pathway.
Champion and Stone (2020) have also demonstrated that more CD68+ macrophages were found in high-grade myocarditis cases (>50 CD3+ cells/high power field).
Ji et al. (2019) found mononuclear cell infiltration in myocardium with myocarditis in monkeys, with a large proportion of T cells (CD3+, CD4+, and CD8+) and a lower number of CD68+ macrophages.
6 Treatment
The treatment of PD-1/PD-L1 inhibitors related to adverse cardiac events mainly includes symptomatic and causal treatment. If the patient has symptoms of chronic heart failure, β-blockers and angiotensin-converting enzyme inhibitor/angiotensin II receptor antagonists are used for treatment. The main causal treatment is to suppress the over-activated T lymphocytes. The first-line immunosuppressant is the first choice for high-dose corticosteroids. The National Comprehensive Cancer Network (NCCN) has summarized the grades of cardiovascular adverse events that may be related to immune checkpoint inhibitor treatment. For grade 2, 3, and 4 cardiovascular irAEs, immunotherapy should be permanently discontinued. The experts have recommended a pulsed dose of methylprednisolone (1 g/day for 3–5 days) in severe or life-threatening situations (Thompson et al., 2021). As for the re-administration of PD-1/PD-L1 inhibitors, the society for immunotherapy of cancer (SITC) recommended that patients with grade 4 irAEs (moderate to severe decompensation, life-threatening conditions) should permanently discontinue the ICIs treatment. But SITC also suggested high-dose corticosteroids for severe conditions (Puzanov et al., 2017). The European Society for Medical Oncology (ESMO) recommended that when myocarditis was suspected, the patient should be admitted to the hospital and immediately start using high-dose prednisone (methylprednisolone 1,000 mg/day). If the condition worsened, consider adding another immunosuppressive drug (mycophenolate mofetil or tacrolimus) (Curigliano et al., 2020). In a multi-center registration report, Mahmood found that initial high-dose steroids were seemly more effective for patients, with a lower rate of MACE (Mahmood et al., 2018). Mycophenolate mofetil, tacrolimus, infliximab, and anti-thymocyte globulin have also been reported to be combined with steroids to alleviate the symptoms (Brahmer et al., 2018; Agrawal et al., 2019; Curigliano et al., 2020; Guo et al., 2020; Thompson et al., 2021).
7 Conclusion
PD-1/PD-L1 inhibitors associated with myocarditis are a rare and fatal complication. Given this, it is difficult to carry out high-quality large-scale prospective or retrospective clinical trials. Case reports or case series reports are effective and convenient ways for us to know the disease, but learning from the literature usually has poor timeliness. Patient follow-up information cannot be instantly tracked. Databases such as VigiBase allow us to know the incidence of immune-related myocarditis, but there is no information on the clinical manifestations, onset time, and therapy of the disease detailed mentioned in case reports, which are the keys to the diagnosis and treatment of myocarditis (Salem et al., 2018; Rubio-Infante et al., 2021). It is urgent to establish a public database of rare diseases, which records the primary disease, complications, onset time, treatment and survival status, etc., and can also real-time update the follow-up information, so that the public can dynamically evaluate the treatment of the disease.
Until now there are no specific clinical manifestations or laboratory biomarkers for PD-1/PD-L1 inhibitors associated myocarditis (Puzanov et al., 2017; Brahmer et al., 2018; Curigliano et al., 2020; Thompson et al., 2021). According to the location of myocarditis, the electrocardiogram may have nonspecific manifestations such as conduction block or ST-T changes. Echocardiography can accurately reflect the motion state of the ventricular wall, but in the early stage of the disease, when the left ventricular systolic function is normal, the manifestation that ultrasonography can provide us is unpredictive for myocarditis. CMR can find the structural abnormality of myocardial tissue and measure the ejection function of the heart. Compared with ultrasound, CMR has better accuracy and sensitivity. EMB is the gold standard for the diagnosis of immune-related myocarditis, which is characterized by a large number of immune T-cell infiltration. Some patients with deteriorated cardiac conditions cannot afford to suffer the EMB that may result in some fatal complications. Myocarditis is usually an acute course with severe symptoms. Early diagnosis and treatment are important measures to improve patient prognosis and reduce patient mortality. We proposed two strategies to help diagnose and treat PD-1/PD-L1 inhibitors associated myocarditis. First, an assessment of patients’ cardiovascular risk factors and history of cardiac adverse events should be completed before the administration of PD-1/PD-L1 inhibitors. Under this circumstance, we can get patients’ baseline index that can help evaluate the deteriorated extent of cardiac function. Second, for patients who cannot tolerate EMB, we can score the examinations or biomarkers related to the heart, such as electrocardiogram, echocardiogram, BNP, troponin, and CMP. When the score reaches a certain level, we can try to use steroids, with the hypothesis that the patients are likely to have myocarditis.
PD-1/PD-L1 inhibitors leading to T-lymphocyte infiltration is the histological foundation of immune myocarditis. It has been testified that PD-L1 can be expressed on myocardial tissue, so that PD-1/PD-L1 inhibitors may disturb the homeostasis that already existed. Considering the extremely low incidence of PD-1/PD-L1 inhibitors associated myocarditis, histological susceptibility may not be reasonable for the happening. Myocardial immune microenvironment changes, like, the unbalance of Tregs and Teffs, unnormal levels of cytokines, and macrophages polarization, will lead to T-lymphocyte infiltration, causing the fundamental pathogenesis of myocarditis. Francisco et al. have found PD-L1 can regulate the induction and function of Tregs by many signal pathways, like the down-regulation of phospho-Akt, mTOR, S6, and ERK2 and the upregulation of PTEN (Francisco et al., 2009). Zhang thought that PD-1 may play a key role in maintaining the balance and activation threshold of Tregs and Teffs, with FoxP3 maintaining the function of Tregs (Zhang et al., 2016). TNF-α, IFN-γ, and other cytokines can differently influence the expression of PD-1 or PD-L1 leading to the final phenotype of T-lymphocyte infiltration (Rodig et al., 2003; Seko et al., 2007; Zheng et al., 2018; Theivanthiran et al., 2020; Finke et al., 2021). Lv et al., have found that protein α-isoform of myosin heavy chain (α-MyHC, a kind of cardiac antigen) encoded by Myb6 let mice and humans receive the immune tolerance with the help of medullary thymic epithelial cells (mTECs) and peripheral lymphoid stromal cells, preventing from T-lymphocyte infiltration myocarditis (Lv et al., 2011). Tarrio has described that a αMyHC peptide can successfully induce the susceptibility of autoimmune myocarditis (with CD4+ and CD8+ T-lymphocyte infiltration) in PD-1 deficient mice (Tarrio et al., 2012). Tsuruoka et al. (2020) have found that the concurrent and subsequent administration of mPD1ab with MyHC-α fragment can manifest opposite effects on immune cell infiltration. Given that, whether thymic related auto-immune tolerance is the key point for immune myocarditis triggered by PD-1/PD-L1 inhibitors? Future consideration: 1) More studies should focus on the genetic level to investigate the genetic predisposition in myocarditis case; 2) exosome and cytokines are the hoping territories for the mechanism investigation because there are the messengers of information in different pathways; 3) immune microenvironment is the core for understanding and investigating the myocarditis. In addition, we have tried to summarize the potential mechanism of PD-1/PD-L1 inhibitors related in Table 4 from five different aspects based on the current published studies.
For patients diagnosed with PD-1/PD-L1 inhibitors related, corticosteroid treatment is a recommended principle in the guidelines of the NCCN, SITC, ESMO, and American Society of Clinical Oncology. In the American Society of Clinical Oncology, NCCN, and ESMO guidelines, prompt steroids should be used initially. NCCN guideline-recommended high-dose steroids (1 g/day, IV) used for 3–5 days, while ESMO failed to detail the period of high-dose steroids usage, but suggested that steroids should be used until the symptoms improved and biomarker normalized. The American Society of Clinical Oncology recommended that the ways of administration of high-dose steroids (1–2 mg/kg) mainly depended on the severity of symptoms (Brahmer et al., 2018; Curigliano et al., 2020; Thompson et al., 2021). The consensus of SITC suggested that steroids treatment (2 mg/kg/day, IV) were used under the condition that patients with moderately abnormal testing or symptoms with mild activity (Puzanov et al., 2017). As for the PD-1/PD-L1 inhibitor rechallenge, those guidelines or consensuses have different opinions. NCCN and the American society of clinical oncology guidelines suggested the permanent discontinuation of PD-1/PD-L1 inhibitors in the setting of grade 2–4 (Brahmer et al., 2018; Thompson et al., 2021). While the consensus of SITC considered that it was reasonable to stop immune checkpoint inhibitors forever for patients in grade 4 (Puzanov et al., 2017). In the ESMO guideline, the PD-1/PD-L1 inhibitor rechallenge should balance the benefits and risk for patients, and if necessary very close surveillance of anti-PD-1 agents should be carried out (Curigliano et al., 2020). Given the diagnostic difficulty of PD-1/PD-L1 inhibitor-associated myocarditis, patients with suspected heart failure or severe cardiac symptoms are highly recommended to receive steroids, because the EMB may be a dangerous intervention in that setting. And in some situations, doctors will make the diagnosis of PD-1/PD-L1 associated myocarditis, according to the cardiac imaging performance, clinical symptoms and the usage of PD-1/PD-L1 inhibitors (Gibson et al., 2016; Mehta et al., 2016; Semper et al., 2016; Frigeri et al., 2018; Monge et al., 2018; Thibault et al., 2018; Agrawal et al., 2019; Charles et al., 2019; Esfahani et al., 2019; Khoury et al., 2019; Salem et al., 2019). Steroid therapy is not only a treatment method but also a diagnostic method for myocarditis if the patients’ symptoms improved. If there are no obvious improvements in clinical symptoms under steroid usage, immunosuppressive agents should be used as second-line treatment. There are two dilemmas we have to find the answers to. First, the anti-tumor efficacy of PD-1/PD-L1 inhibitors will be compromised by the usage of steroids or immunosuppressive agents? Second, when and how to judge the rechallenge under the setting that PD-1/PD-L1 inhibitors achieve promising anti-tumor effect? For the first question, we think that it is wise to use steroid or immunosuppressive agents to treat myocarditis under the characteristics that are acute and more lethal. Unfortunately, there is no consensus on the second question.
In this article, we reviewed the epidemiological manifestations, clinical characteristics, diagnosis, pathogenesis, and treatment of PD-1/PD-L1 inhibitor-related myocarditis and summarized the reported myocarditis cases in order to outline the clinical symptoms. Immuno-myocarditis is a rare but severe adverse event of PD-1/PD-L1 inhibitirs related treatment, usually with an early onset. Biomarkers, like BNP or troponin, ECG, and MCR can help diagnose immuno-myocarditis. Exploring the possible mechanisms of pathogenesis from the histological level, cellular level, and molecular transcription level found that T-lymphocyte infiltration was the basis of myocarditis phenotype. Macrophages mediated immune-response; synergistic effect of PD-1/CTLA4 may be the catalysts in this process. The core treatment of PD-1/PD-L1 inhibitor-related myocarditis is to suppress the over-activated T lymphocytes and corticosteroids are the first-line immunosuppressant.
Author Contributions
HD conceptualized and designed this study, HD and YQ carried out the primary literature search, and drafted the manuscript; XK performed the literature search and revised the manuscript; HD, YQ, XK, ZW, YF and JW discussed, revised, and edited the manuscript. All authors read and approved the final manuscript.
Funding
This work was supported by the Natural Science Foundation of China (No. 81872160), the Natural Science Foundation of China (No. 82072940), the Natural Science Foundation of China (No. 82103047), the Natural Science Foundation of China (No. 82102887), the China National Key R&D (or Research and Development) Program (No. 2020AAA0105000 and 2020AAA0105004), the Beijing Municipal Natural Science Foundation (Key Project) (No. 7191009), the Beijing Municipal Natural Science Foundation (No. 7204293), the Special Research Fund for Central Universities, Peking Union Medical College (No. 3332019053), the Beijing Hope Run Special Fund of Cancer Foundation of China (No. LC2019B03), the Beijing Hope Run Special Fund of Cancer Foundation of China (No. LC2019L07), the Beijing Hope Run Special Fund of Cancer Foundation of China (No. LC2020L01), the Golden Bridge Project Seed Fund of Beijing Association for Science and Technology (No. ZZ20004), the 2021 Chaoyang District Social Development Science and Technology Plan Project (Medical and Health Field) (No. CYSF2115), the Chinese Young Breast Experts Research project (No. CYBER-2021-005), the XianSheng Clinical Research Special Fund of China International Medical Foundation (No. Z-2014-06-2103), the Beijing Xisike Clinical Oncology Research Foundation (No. Y-Young2021-0017), the Chinese Academy of Medical Sciences Clinical and Translational Medicine Research Fund Project (No. 2021-I2M-C&T-A-012), and the Chinese Academy of Medical Sciences Clinical and Translational Medicine Research Fund Project (No. 2021-I2M-C&T-B-044).
Conflict of Interest
The authors declare that the research was conducted in the absence of any commercial or financial relationships that could be construed as a potential conflict of interest.
Publisher’s Note
All claims expressed in this article are solely those of the authors and do not necessarily represent those of their affiliated organizations, or those of the publisher, the editors, and the reviewers. Any product that may be evaluated in this article, or claim that may be made by its manufacturer, is not guaranteed or endorsed by the publisher.
Acknowledgments
We would like to thank our colleagues at the Department of Breast and Abdominal Surgical Oncology, National Cancer Center/National Clinical Research Center for Cancer/Cancer Hospital, Chinese Academy of Medical and Peking Union Medical College.
References
Agrawal, N., Khunger, A., Vachhani, P., Colvin, T. A., Hattoum, A., Spangenthal, E., et al. (2019). Cardiac Toxicity Associated with Immune Checkpoint Inhibitors: Case Series and Review of the Literature. Case Rep. Oncol. 12 (1), 260–276. doi:10.1159/000498985
Ahmadzadeh, M., Johnson, L. A., Heemskerk, B., Wunderlich, J. R., Dudley, M. E., White, D. E., et al. (2009). Tumor Antigen-specific CD8 T Cells Infiltrating the Tumor Express High Levels of PD-1 and Are Functionally Impaired. Blood 114 (8), 1537–1544. doi:10.1182/blood-2008-12-195792
Alissafi, T., Banos, A., Boon, L., Sparwasser, T., Ghigo, A., Wing, K., et al. (2017). Tregs Restrain Dendritic Cell Autophagy to Ameliorate Autoimmunity. J. Clin. Invest. 127 (7), 2789–2804. doi:10.1172/JCI92079
Ammirati, E., Cipriani, M., Lilliu, M., Sormani, P., Varrenti, M., Raineri, C., et al. (2017). Survival and Left Ventricular Function Changes in Fulminant versus Nonfulminant Acute Myocarditis. Circulation 136 (6), 529–545. doi:10.1161/CIRCULATIONAHA.117.026386
Antonia, S. J., Villegas, A., Daniel, D., Vicente, D., Murakami, S., Hui, R., et al. (2017). Durvalumab after Chemoradiotherapy in Stage III Non-small-cell Lung Cancer. N. Engl. J. Med. 377 (20), 1919–1929. doi:10.1056/NEJMoa1709937
Awadalla, M., Mahmood, S. S., Groarke, J. D., Hassan, M. Z. O., Nohria, A., Rokicki, A., et al. (2020). Global Longitudinal Strain and Cardiac Events in Patients with Immune Checkpoint Inhibitor-Related Myocarditis. J. Am. Coll. Cardiol. 75 (5), 467–478. doi:10.1016/j.jacc.2019.11.049
Bando, S., Soeki, T., Matsuura, T., Tobiume, T., Ise, T., Kusunose, K., et al. (2017). Plasma Brain Natriuretic Peptide Levels Are Elevated in Patients with Cancer. Plos One 12 (6), e0178607. doi:10.1371/journal.pone.0178607
Barlesi, F., Vansteenkiste, J., Spigel, D., Ishii, H., Garassino, M., de Marinis, F., et al. (2018). Avelumab versus Docetaxel in Patients with Platinum-Treated Advanced Non-small-cell Lung Cancer (JAVELIN Lung 200): an Open-Label, Randomised, Phase 3 Study. Lancet Oncol. 19 (11), 1468–1479. doi:10.1016/S1470-2045(18)30673-9
Bass, A., Patterson, J. H., and Adams, K. F. (2010). Perspective on the Clinical Application of Troponin in Heart Failure and States of Cardiac Injury. Heart Fail. Rev. 15 (4), 305–317. doi:10.1007/s10741-008-9124-8
Bellmunt, J., de Wit, R., Vaughn, D. J., Fradet, Y., Lee, J. L., Fong, L., et al. (2017). Pembrolizumab as Second-Line Therapy for Advanced Urothelial Carcinoma. N. Engl. J. Med. 376 (11), 1015–1026. doi:10.1056/NEJMoa1613683
Bishawi, M., Bowles, D., Pla, M. M., Oakes, F., Chiang, Y., Schroder, J., et al. (2021). PD-1 and PD-L1 Expression in Cardiac Transplantation. Cardiovasc. Pathol. 54, 107331. doi:10.1016/j.carpath.2021.107331
Bivona, T. G., Pérez De Castro, I., Ahearn, I. M., Grana, T. M., Chiu, V. K., Lockyer, P. J., et al. (2003). Phospholipase Cgamma Activates Ras on the Golgi Apparatus by Means of RasGRP1. Nature 424 (6949), 694–698. doi:10.1038/nature01806
Bockstahler, M., Fischer, A., Goetzke, C. C., Neumaier, H. L., Sauter, M., Kespohl, M., et al. (2020). Heart-Specific Immune Responses in an Animal Model of Autoimmune-Related Myocarditis Mitigated by an Immunoproteasome Inhibitor and Genetic Ablation. Circulation 141 (23), 1885–1902. doi:10.1161/CIRCULATIONAHA.119.043171
Brahmer, J. R., Lacchetti, C., Schneider, B. J., Atkins, M. B., Brassil, K. J., Caterino, J. M., et al. (2018). Management of Immune-Related Adverse Events in Patients Treated with Immune Checkpoint Inhibitor Therapy: American Society of Clinical Oncology Clinical Practice Guideline. J. Clin. Oncol. 36 (17), 1714–1768. doi:10.1200/JCO.2017.77.6385
Champion, S. N., and Stone, J. R. (2020). Immune Checkpoint Inhibitor Associated Myocarditis Occurs in Both High-Grade and Low-Grade Forms. Mod. Pathol. 33 (1), 99–108. doi:10.1038/s41379-019-0363-0
Charles, J., Giovannini, D., Terzi, N., Schwebel, C., Sturm, N., Masson, D., et al. (2019). Multi-organ Failure Induced by Nivolumab in the Context of Allo-Stem Cell Transplantation. Exp. Hematol. Oncol. 8, 8. doi:10.1186/s40164-019-0132-2
Chemnitz, J. M., Parry, R. V., Frauwirth, K. A., Lanfranco, A. R., Braunstein, I., Kobayashi, S. V., et al. (2004). CTLA-4 and PD-1 Receptors Inhibit T-Cell Activation by Distinct Mechanisms. Blood 104 (11), 726A. doi:10.1182/blood.v104.11.2657.2657
Chen, L., and Flies, D. B. (2013). Molecular Mechanisms of T Cell Co-stimulation and Co-inhibition. Nat. Rev. Immunol. 13 (4), 227–242. doi:10.1038/nri3405
Chen, Q., Huang, D. S., Zhang, L. W., Li, Y. Q., Wang, H. W., and Liu, H. B. (2018). Fatal Myocarditis and Rhabdomyolysis Induced by Nivolumab during the Treatment of Type B3 Thymoma. Clin. Toxicol. (Phila) 56 (7), 667–671. doi:10.1080/15563650.2017.1401079
Cousin, S., and Italiano, A. (2016). Molecular Pathways: Immune Checkpoint Antibodies and Their Toxicities. Clin. Cancer Res. 22 (18), 4550–4555. doi:10.1158/1078-0432.CCR-15-2569
Curigliano, G., Lenihan, D., Fradley, M., Ganatra, S., Barac, A., Blaes, A., et al. (2020). Management of Cardiac Disease in Cancer Patients throughout Oncological Treatment: ESMO Consensus Recommendations. Ann. Oncol. 31 (2), 171–190. doi:10.1016/j.annonc.2019.10.023
Dong, H., Zhu, G., Tamada, K., and Chen, L. (1999). B7-H1, a Third Member of the B7 Family, Co-stimulates T-Cell Proliferation and Interleukin-10 Secretion. Nat. Med. 5 (12), 1365–1369. doi:10.1038/70932
Elamm, C., Fairweather, D., and Cooper, L. T. (2012). Pathogenesis and Diagnosis of Myocarditis. Heart 98 (11), 835–840. doi:10.1136/heartjnl-2012-301686
Escudier, M., Cautela, J., Malissen, N., Ancedy, Y., Orabona, M., Pinto, J., et al. (2017). Clinical Features, Management, and Outcomes of Immune Checkpoint Inhibitor-Related Cardiotoxicity. Circulation 136 (21), 2085–2087. doi:10.1161/CIRCULATIONAHA.117.030571
Esfahani, K., Buhlaiga, N., Thébault, P., Lapointe, R., Johnson, N. A., and Miller, W. H. (2019). Alemtuzumab for Immune-Related Myocarditis Due to PD-1 Therapy. N. Engl. J. Med. 380 (24), 2375–2376. doi:10.1056/NEJMc1903064
Ferris, R. L., Blumenschein, G., Fayette, J., Guigay, J., Colevas, A. D., Licitra, L., et al. (2016). Nivolumab for Recurrent Squamous-Cell Carcinoma of the Head and Neck. N. Engl. J. Med. 375 (19), 1856–1867. doi:10.1056/NEJMoa1602252
Fife, B. T., and Bluestone, J. A. (2008). Control of Peripheral T-Cell Tolerance and Autoimmunity via the CTLA-4 and PD-1 Pathways. Immunol. Rev. 224, 166–182. doi:10.1111/j.1600-065X.2008.00662.x
Finke, D., Heckmann, M. B., Salatzki, J., Riffel, J., Herpel, E., Heinzerling, L. M., et al. (2021). Comparative Transcriptomics of Immune Checkpoint Inhibitor Myocarditis Identifies Guanylate Binding Protein 5 and 6 Dysregulation. Cancers 13 (10), 15. doi:10.3390/cancers13102498
Francisco, L., Salinas, V., Brown, K., Vanguri, V., Freeman, G., Kuchroo, V., et al. (2009). OR.100. PD-L1 Regulates the Development, Maintenance and Function of Induced-Regulatory T Cells. Clin. Immunol. 131, S41. doi:10.1016/j.clim.2009.03.115
Freeman, G. J., Long, A. J., Iwai, Y., Bourque, K., Chernova, T., Nishimura, H., et al. (2000). Engagement of the PD-1 Immunoinhibitory Receptor by a Novel B7 Family Member Leads to Negative Regulation of Lymphocyte Activation. J. Exp. Med. 192 (11), 1027–1034. doi:10.1084/jem.192.7.1027
Friedrich, M. G., Sechtem, U., Schulz-Menger, J., Holmvang, G., Alakija, P., Cooper, L. T., et al. (2009). Cardiovascular Magnetic Resonance in Myocarditis: A JACC White Paper. J. Am. Coll. Cardiol. 53 (17), 1475–1487. doi:10.1016/j.jacc.2009.02.007
Frigeri, M., Meyer, P., Banfi, C., Giraud, R., Hachulla, A. L., Spoerl, D., et al. (2018). Immune Checkpoint Inhibitor-Associated Myocarditis: A New Challenge for Cardiologists. Can. J. Cardiol. 34 (1), 92–e3. doi:10.1016/j.cjca.2017.09.025
Ganatra, S., and Neilan, T. G. (2018). Immune Checkpoint Inhibitor-Associated Myocarditis. Oncologist 23 (8), 879–886. doi:10.1634/theoncologist.2018-0130
Gibson, R., Delaune, J., Szady, A., and Markham, M. (2016). Suspected Autoimmune Myocarditis and Cardiac Conduction Abnormalities with Nivolumab Therapy for Non-small Cell Lung Cancer. BMJ Case Rep. 2016, 1. doi:10.1136/bcr-2016-216228
Glass, C. K., and Mitchell, R. N. (2017). Winning the Battle, but Losing the War: Mechanisms and Morphology of Cancer-Therapy-Associated Cardiovascular Toxicity. Cardiovasc. Pathol. 30, 55–63. doi:10.1016/j.carpath.2017.06.009
Grabie, N., Gotsman, I., DaCosta, R., Pang, H., Stavrakis, G., Butte, M. J., et al. (2007). Endothelial Programmed Death-1 Ligand 1 (PD-L1) Regulates CD8+ T-Cell Mediated Injury in the Heart. Circulation 116 (18), 2062–2071. doi:10.1161/CIRCULATIONAHA.107.709360
Guglin, M., and Nallamshetty, L. (2012). Myocarditis: Diagnosis and Treatment. Curr. Treat. Options. Cardiovasc. Med. 14 (6), 637–651. doi:10.1007/s11936-012-0204-7
Guo, C. W., Alexander, M., Dib, Y., Lau, P. K. H., Weppler, A. M., Au-Yeung, G., et al. (2020). A Closer Look at Immune-Mediated Myocarditis in the Era of Combined Checkpoint Blockade and Targeted Therapies. Eur. J. Cancer 124, 15–24. doi:10.1016/j.ejca.2019.09.009
Hahn, A. W., Gill, D. M., Agarwal, N., and Maughan, B. L. (2017). PD-1 Checkpoint Inhibition: Toxicities and Management. Urol. Oncol. 35 (12), 701–707. doi:10.1016/j.urolonc.2017.08.005
Han, Y., Liu, D., and Li, L. (2020). PD-1/PD-L1 Pathway: Current Researches in Cancer. Am. J. Cancer Res. 10 (3), 727–742.
Heinzerling, L., Ott, P. A., Hodi, F. S., Husain, A. N., Tajmir-Riahi, A., Tawbi, H., et al. (2016). Cardiotoxicity Associated with CTLA4 and PD1 Blocking Immunotherapy. J. Immunother. Cancer 4, 50. doi:10.1186/s40425-016-0152-y
Horn, L., Mansfield, A. S., Szczęsna, A., Havel, L., Krzakowski, M., Hochmair, M. J., et al. (2018). First-Line Atezolizumab Plus Chemotherapy in Extensive-Stage Small-Cell Lung Cancer. N. Engl. J. Med. 379 (23), 2220–2229. doi:10.1056/NEJMoa1809064
Hu, D., Dong, R., Yang, Y., Chen, Z., Tang, Y., Fu, M., et al. (2019). Human Kallikrein Overexpression Alleviates Cardiac Aging by Alternatively Regulating Macrophage Polarization in Aged Rats. FASEB J. 33 (7), 8436–8452. doi:10.1096/fj.201802371RR
Hu, J. R., Florido, R., Lipson, E. J., Naidoo, J., Ardehali, R., Tocchetti, C. G., et al. (2019). Cardiovascular Toxicities Associated with Immune Checkpoint Inhibitors. Cardiovasc. Res. 115 (5), 854–868. doi:10.1093/cvr/cvz026
Ishida, Y., Agata, Y., Shibahara, K., and Honjo, T. (1992). Induced Expression of PD-1, a Novel Member of the Immunoglobulin Gene Superfamily, upon Programmed Cell Death. EMBO J. 11 (11), 3887–3895. doi:10.1002/j.1460-2075.1992.tb05481.x
Jain, P., Gutierrez Bugarin, J., Guha, A., Jain, C., Patil, N., Shen, T., et al. (2021). Cardiovascular Adverse Events Are Associated with Usage of Immune Checkpoint Inhibitors in Real-World Clinical Data across the United States. ESMO Open 6 (5), 100252. doi:10.1016/j.esmoop.2021.100252
Ji, C., Roy, M. D., Golas, J., Vitsky, A., Ram, S., Kumpf, S. W., et al. (2019). Myocarditis in Cynomolgus Monkeys Following Treatment with Immune Checkpoint Inhibitors. Clin. Cancer Res. 25 (15), 4735–4748. doi:10.1158/1078-0432.CCR-18-4083
Johnson, D. B., Balko, J. M., Compton, M. L., Chalkias, S., Gorham, J., Xu, Y., et al. (2016). Fulminant Myocarditis with Combination Immune Checkpoint Blockade. N. Engl. J. Med. 375 (18), 1749–1755. doi:10.1056/NEJMoa1609214
Keir, M. E., Butte, M. J., Freeman, G. J., and Sharpe, A. H. (2008). PD-1 and its Ligands in Tolerance and Immunity. Annu. Rev. Immunol. 26, 677–704. doi:10.1146/annurev.immunol.26.021607.090331
Khoury, Z. H., Hausner, P. F., Idzik-Starr, C. L., Frykenberg, M. R. A., Brooks, J. K., Dyalram, D., et al. (2019). Combination Nivolumab/Ipilimumab Immunotherapy for Melanoma with Subsequent Unexpected Cardiac Arrest: A Case Report and Review of Literature. J. Immunother. 42 (8), 313–317. doi:10.1097/CJI.0000000000000282
Kumar, P., Saini, S., and Prabhakar, B. S. (2020). Cancer Immunotherapy with Check point Inhibitor Can Cause Autoimmune Adverse Events Due to Loss of Treg Homeostasis. Semin. Cancer Biol. 64, 29–35. doi:10.1016/j.semcancer.2019.01.006
Läubli, H., Balmelli, C., Bossard, M., Pfister, O., Glatz, K., and Zippelius, A. (2015). Acute Heart Failure Due to Autoimmune Myocarditis under Pembrolizumab Treatment for Metastatic Melanoma. J. Immunother. Cancer 3, 11. doi:10.1186/s40425-015-0057-1
Lee, C. K., Man, J., Lord, S., Links, M., Gebski, V., Mok, T., et al. (2017). Checkpoint Inhibitors in Metastatic EGFR-Mutated Non-small Cell Lung Cancer-A Meta-Analysis. J. Thorac. Oncol. 12 (2), 403–407. doi:10.1016/j.jtho.2016.10.007
Li, X., Shao, C., Shi, Y., and Han, W. (2018). Lessons Learned from the Blockade of Immune Checkpoints in Cancer Immunotherapy. J. Hematol. Oncol. 11 (1), 31. doi:10.1186/s13045-018-0578-4
Lilleker, J. B., Diederichsen, A. C. P., Jacobsen, S., Guy, M., Roberts, M. E., Sergeant, J. C., et al. (2018). Using Serum Troponins to Screen for Cardiac Involvement and Assess Disease Activity in the Idiopathic Inflammatory Myopathies. Rheumatology (Oxford) 57 (6), 1041–1046. doi:10.1093/rheumatology/key031
Liu, S. Y., Huang, W. C., Yeh, H. I., Ko, C. C., Shieh, H. R., Hung, C. L., et al. (2019). Sequential Blockade of PD-1 and PD-L1 Causes Fulminant Cardiotoxicity-From Case Report to Mouse Model Validation. Cancers (Basel) 11 (4), 17. doi:10.3390/cancers11040580
Lv, H., Havari, E., Pinto, S., Gottumukkala, R. V., Cornivelli, L., Raddassi, K., et al. (2011). Impaired Thymic Tolerance to α-myosin Directs Autoimmunity to the Heart in Mice and Humans. J. Clin. Invest. 121 (4), 1561–1573. doi:10.1172/JCI44583
Mahmood, S., Fradley, M. G., Cohen, J. V., Nohria, A., Reynolds, K. L., Heinzerling, L. M., et al. (2018). Myocarditis in Patients Treated with Immune Checkpoint Inhibitors. J. Am. Coll. Cardiol. 71 (11), 699. doi:10.1016/s0735-1097(18)31240-3
Martin Huertas, R., Saavedra Serrano, C., Perna, C., Ferrer Gómez, A., and Alonso Gordoa, T. (2019). Cardiac Toxicity of Immune-Checkpoint Inhibitors: a Clinical Case of Nivolumab-Induced Myocarditis and Review of the Evidence and New Challenges. Cancer Manag. Res. 11, 4541–4548. doi:10.2147/CMAR.S185202
Martín, P., Blanco-Domínguez, R., and Sánchez-Díaz, R. (2021). Novel Human Immunomodulatory T Cell Receptors and Their Double-Edged Potential in Autoimmunity, Cardiovascular Disease and Cancer. Cell Mol Immunol 18 (4), 919–935. doi:10.1038/s41423-020-00586-4
Matson, D. R., Accola, M. A., Rehrauer, W. M., and Corliss, R. F. (2018). Fatal Myocarditis Following Treatment with the PD-1 Inhibitor Nivolumab. J. Forensic Sci. 63 (3), 954–957. doi:10.1111/1556-4029.13633
Mehta, A., Gupta, A., Hannallah, F., Koshy, T., and Reimold, S. (2016). Myocarditis as an Immune-Related Adverse Event with Ipilimumab/nivolumab Combination Therapy for Metastatic Melanoma. Melanoma Res. 26 (3), 319–320. doi:10.1097/CMR.0000000000000251
Mir, H., Alhussein, M., Alrashidi, S., Alzayer, H., Alshatti, A., Valettas, N., et al. (2018). Cardiac Complications Associated with Checkpoint Inhibition: A Systematic Review of the Literature in an Important Emerging Area. Can. J. Cardiol. 34 (8), 1059–1068. doi:10.1016/j.cjca.2018.03.012
Monge, C., Maeng, H., Brofferio, A., Apolo, A. B., Sathya, B., Arai, A. E., et al. (2018). Myocarditis in a Patient Treated with Nivolumab and PROSTVAC: a Case Report. J. Immunother. Cancer 6, 150. doi:10.1186/s40425-018-0473-0
Moslehi, J. J., Salem, J. E., Sosman, J. A., Lebrun-Vignes, B., and Johnson, D. B. (2018). Increased Reporting of Fatal Immune Checkpoint Inhibitor-Associated Myocarditis. Lancet 391 (10124), 933. doi:10.1016/S0140-6736(18)30533-6
Motzer, R. J., Escudier, B., McDermott, D. F., George, S., Hammers, H. J., Srinivas, S., et al. (2015). Nivolumab versus Everolimus in Advanced Renal-Cell Carcinoma. N. Engl. J. Med. 373 (19), 1803–1813. doi:10.1056/NEJMoa1510665
Naidoo, J., Page, D. B., Li, B. T., Connell, L. C., Schindler, K., Lacouture, M. E., et al. (2015). Toxicities of the Anti-PD-1 and Anti-PD-L1 Immune Checkpoint Antibodies. Ann. Oncol. 26 (12), 2375–2391. doi:10.1093/annonc/mdv383
Norwood, T. G., Westbrook, B. C., Johnson, D. B., Litovsky, S. H., Terry, N. L., McKee, S. B., et al. (2017). Smoldering Myocarditis Following Immune Checkpoint Blockade. J. Immunother. Cancer 5, 91. doi:10.1186/s40425-017-0296-4
Patsoukis, N., Li, L., Sari, D., Petkova, V., and Boussiotis, V. A. (2013). PD-1 Increases PTEN Phosphatase Activity while Decreasing PTEN Protein Stability by Inhibiting Casein Kinase 2. Mol. Cel Biol 33 (16), 3091–3098. doi:10.1128/MCB.00319-13
Puzanov, I., Diab, A., Abdallah, K., Bingham, C. O., Brogdon, C., Dadu, R., et al. (2017). Managing Toxicities Associated with Immune Checkpoint Inhibitors: Consensus Recommendations from the Society for Immunotherapy of Cancer (SITC) Toxicity Management Working Group. J. Immunother. Cancer 5, 95. doi:10.1186/s40425-017-0300-z
Qin, W., Hu, L., Zhang, X., Jiang, S., Li, J., Zhang, Z., et al. (2019). The Diverse Function of PD-1/PD-L Pathway beyond Cancer. Front. Immunol. 10, 2298. doi:10.3389/fimmu.2019.02298
Robert, C., Schachter, J., Long, G. V., Arance, A., Grob, J. J., Mortier, L., et al. (2015). Pembrolizumab versus Ipilimumab in Advanced Melanoma. N. Engl. J. Med. 372 (26), 2521–2532. doi:10.1056/NEJMoa1503093
Rodig, N., Ryan, T., Allen, J. A., Pang, H., Grabie, N., Chernova, T., et al. (2003). Endothelial Expression of PD-L1 and PD-L2 Down-Regulates CD8+ T Cell Activation and Cytolysis. Eur. J. Immunol. 33 (11), 3117–3126. doi:10.1002/eji.200324270
Rubio-Infante, N., Ramírez-Flores, Y. A., Castillo, E. C., Lozano, O., García-Rivas, G., and Torre-Amione, G. (2021). Cardiotoxicity Associated with Immune Checkpoint Inhibitor Therapy: a Meta-Analysis. Eur. J. Heart Fail. 23 (10), 1739–1747. doi:10.1002/ejhf.2289
Sagar, S., Liu, P. P., and Cooper, L. T. (2012). Myocarditis. The Lancet 379 (9817), 738–747. doi:10.1016/s0140-6736(11)60648-x
Salem, J. E., Allenbach, Y., Vozy, A., Brechot, N., Johnson, D. B., Moslehi, J. J., et al. (2019). Abatacept for Severe Immune Checkpoint Inhibitor-Associated Myocarditis. N. Engl. J. Med. 380 (24), 2377–2379. doi:10.1056/NEJMc1901677
Salem, J. E., Manouchehri, A., Moey, M., Lebrun-Vignes, B., Bastarache, L., Pariente, A., et al. (2018). Cardiovascular Toxicities Associated with Immune Checkpoint Inhibitors: an Observational, Retrospective, Pharmacovigilance Study. Lancet Oncol. 19 (12), 1579–1589. doi:10.1016/S1470-2045(18)30608-9
Salmaninejad, A., Khoramshahi, V., Azani, A., Soltaninejad, E., Aslani, S., Zamani, M. R., et al. (2018). PD-1 and Cancer: Molecular Mechanisms and Polymorphisms. Immunogenetics 70 (2), 73–86. doi:10.1007/s00251-017-1015-5
Seko, Y., Yagita, H., Okumura, K., Azuma, M., and Nagai, R. (2007). Roles of Programmed Death-1 (PD-1)/PD-1 Ligands Pathway in the Development of Murine Acute Myocarditis Caused by Coxsackievirus B3. Cardiovasc. Res. 75 (1), 158–167. doi:10.1016/j.cardiores.2007.03.012
Semper, H., Muehlberg, F., Schulz-Menger, J., Allewelt, M., and Grohé, C. (2016). Drug-induced Myocarditis after Nivolumab Treatment in a Patient with PDL1- Negative Squamous Cell Carcinoma of the Lung. Lung Cancer 99, 117–119. doi:10.1016/j.lungcan.2016.06.025
Shivshankar, P., Halade, G. V., Calhoun, C., Escobar, G. P., Mehr, A. J., Jimenez, F., et al. (2014). Caveolin-1 Deletion Exacerbates Cardiac Interstitial Fibrosis by Promoting M2 Macrophage Activation in Mice after Myocardial Infarction. J. Mol. Cel Cardiol 76, 84–93. doi:10.1016/j.yjmcc.2014.07.020
Sobol, I., Chen, C. L., Mahmood, S. S., and Borczuk, A. C. (2020). Histopathologic Characterization of Myocarditis Associated with Immune Checkpoint Inhibitor Therapy. Arch. Pathol. Lab. Med. 144 (11), 1392–1396. doi:10.5858/arpa.2019-0447-OA
Socinski, M. A., Jotte, R. M., Cappuzzo, F., Orlandi, F., Stroyakovskiy, D., Nogami, N., et al. (2018). Atezolizumab for First-Line Treatment of Metastatic Nonsquamous NSCLC. N. Engl. J. Med. 378 (24), 2288–2301. doi:10.1056/NEJMoa1716948
Su, C., Wang, H., Liu, Y., Guo, Q., Zhang, L., Li, J., et al. (2020). Adverse Effects of Anti-PD-1/pd-L1 Therapy in Non-small Cell Lung Cancer. Front. Oncol. 10, 554313. doi:10.3389/fonc.2020.554313
Tadokoro, T., Keshino, E., Makiyama, A., Sasaguri, T., Ohshima, K., Katano, H., et al. (2016). Acute Lymphocytic Myocarditis with Anti-PD-1 Antibody Nivolumab. Circ. Heart Fail. 9 (10), 3. doi:10.1161/CIRCHEARTFAILURE.116.003514
Tajmir-Riahi, A., Bergmann, T., Schmid, M., Agaimy, A., Schuler, G., and Heinzerling, L. (2018). Life-threatening Autoimmune Cardiomyopathy Reproducibly Induced in a Patient by Checkpoint Inhibitor Therapy. J. Immunother. 41 (1), 35–38. doi:10.1097/CJI.0000000000000190
Tarrio, M. L., Grabie, N., Bu, D. X., Sharpe, A. H., and Lichtman, A. H. (2012). PD-1 Protects against Inflammation and Myocyte Damage in T Cell-Mediated Myocarditis. J. Immunol. 188 (10), 4876–4884. doi:10.4049/jimmunol.1200389
Theivanthiran, B., Evans, K. S., DeVito, N. C., Plebanek, M., Sturdivant, M., Wachsmuth, L. P., et al. (2020). A Tumor-Intrinsic PD-L1/nlrp3 Inflammasome Signaling Pathway Drives Resistance to Anti-PD-1 Immunotherapy. J. Clin. Invest. 130 (5), 2570–2586. doi:10.1172/JCI133055
Thibault, C., Vano, Y., Soulat, G., and Mirabel, M. (2018). Immune Checkpoint Inhibitors Myocarditis: Not All Cases Are Clinically Patent. Eur. Heart J. 39 (38), 3553. doi:10.1093/eurheartj/ehy485
Thompson, J. A., Schneider, B. J., Brahmer, J., Andrews, S., Armand, P., Bhatia, S., et al. (2021). NCCN Guidelines: Management of Immunotherapy-Related Toxicities. National Comprehensive Cancer Network. Version 1.2021.
Tsuruoka, K., Wakabayashi, S., Morihara, H., Matsunaga, N., Fujisaka, Y., Goto, I., et al. (2020). Exacerbation of Autoimmune Myocarditis by an Immune Checkpoint Inhibitor Is Dependent on its Time of Administration in Mice. Int. J. Cardiol. 313, 67–75. doi:10.1016/j.ijcard.2020.04.033
Varricchi, G., Galdiero, M. R., Marone, G., Criscuolo, G., Triassi, M., Bonaduce, D., et al. (2017). Cardiotoxicity of Immune Checkpoint Inhibitors. Esmo Open 2 (4), e000247. doi:10.1136/esmoopen-2017-000247
Wang, D. Y., Salem, J. E., Cohen, J. V., Chandra, S., Menzer, C., Ye, F., et al. (2018). Fatal Toxic Effects Associated with Immune Checkpoint Inhibitors: A Systematic Review and Meta-Analysis. JAMA Oncol. 4 (12), 1721–1728. doi:10.1001/jamaoncol.2018.3923
Wang, J., Okazaki, I. M., Yoshida, T., Chikuma, S., Kato, Y., Nakaki, F., et al. (2010). PD-1 Deficiency Results in the Development of Fatal Myocarditis in MRL Mice. Int. Immunol. 22 (6), 443–452. doi:10.1093/intimm/dxq026
Wang, Z., and Wu, X. (2020). Study and Analysis of Antitumor Resistance Mechanism of PD1/PD-L1 Immune Checkpoint Blocker. Cancer Med. 9 (21), 8086–8121. doi:10.1002/cam4.3410
Wei, S. C., Duffy, C. R., and Allison, J. P. (2018). Fundamental Mechanisms of Immune Checkpoint Blockade Therapy. Cancer Discov. 8 (9), 1069–1086. doi:10.1158/2159-8290.CD-18-0367
Wei, S. C., Meijers, W. C., Axelrod, M. L., Anang, N. A. S., Screever, E. M., Wescott, E. C., et al. (2021). A Genetic Mouse Model Recapitulates Immune Checkpoint Inhibitor-Associated Myocarditis and Supports a Mechanism-Based Therapeutic Intervention. Cancer Discov. 11 (3), 614–625. doi:10.1158/2159-8290.CD-20-0856
Xia, W., Chen, H., Chen, D., Ye, Y., Xie, C., and Hou, M. (2020). PD-1 Inhibitor Inducing Exosomal miR-34a-5p Expression Mediates the Cross Talk between Cardiomyocyte and Macrophage in Immune Checkpoint Inhibitor-Related Cardiac Dysfunction. J. Immunother. Cancer 8 (2), 13. doi:10.1136/jitc-2020-001293
Xia, W., Zou, C., Chen, H., Xie, C., and Hou, M. (2020). Immune Checkpoint Inhibitor Induces Cardiac Injury through Polarizing Macrophages via Modulating microRNA-34a/Kruppel-like Factor 4 Signaling. Cell Death Dis 11 (7), 575. doi:10.1038/s41419-020-02778-2
Yamaguchi, S., Morimoto, R., Okumura, T., Yamashita, Y., Haga, T., Kuwayama, T., et al. (2018). Late-Onset Fulminant Myocarditis with Immune Checkpoint Inhibitor Nivolumab. Can. J. Cardiol. 34 (6), 812–e3. doi:10.1016/j.cjca.2018.03.007
Yang, S., and Asnani, A. (2018). Cardiotoxicities Associated with Immune Checkpoint Inhibitors. Curr. Probl. Cancer 42 (4), 422–432. doi:10.1016/j.currproblcancer.2018.07.002
Yi, M., Niu, M., Xu, L., Luo, S., and Wu, K. (2021). Regulation of PD-L1 Expression in the Tumor Microenvironment. J. Hematol. Oncol. 14 (1), 13. doi:10.1186/s13045-020-01027-5
Yu, S. R., Zhang, C. Y., Xiong, W. J., Chen, J. T., Song, J. X., and Chen, H. (2021). An Hypothesis: Disproportion between Cardiac Troponin and B-type Natriuretic Peptide Levels-A High Risk and Poor Prognostic Biomarker in Patients with Fulminant Myocarditis? Heart Lung Circ. 30 (6), 837–842. doi:10.1016/j.hlc.2020.12.012
Zhang, B., Chikuma, S., Hori, S., Fagarasan, S., and Honjo, T. (2016). Nonoverlapping Roles of PD-1 and FoxP3 in Maintaining Immune Tolerance in a Novel Autoimmune Pancreatitis Mouse Model. Proc. Natl. Acad. Sci. U S A. 113 (30), 8490–8495. doi:10.1073/pnas.1608873113
Zhang, L., Awadalla, M., Mahmood, S. S., Nohria, A., Hassan, M. Z. O., Thuny, F., et al. (2020). Cardiovascular Magnetic Resonance in Immune Checkpoint Inhibitor-Associated Myocarditis. Eur. Heart J. 41 (18), 1733–1743. doi:10.1093/eurheartj/ehaa051
Keywords: immunotherapy, myocarditis, epidemiology, mechanism, PD-1/PD-L1 inhibitors
Citation: Dong H, Qi Y, Kong X, Wang Z, Fang Y and Wang J (2022) PD-1/PD-L1 Inhibitor-Associated Myocarditis: Epidemiology, Characteristics, Diagnosis, Treatment, and Potential Mechanism. Front. Pharmacol. 13:835510. doi: 10.3389/fphar.2022.835510
Received: 14 December 2021; Accepted: 14 March 2022;
Published: 19 April 2022.
Edited by:
Olavi R. Pelkonen, University of Oulu, FinlandCopyright © 2022 Dong, Qi, Kong, Wang, Fang and Wang. This is an open-access article distributed under the terms of the Creative Commons Attribution License (CC BY). The use, distribution or reproduction in other forums is permitted, provided the original author(s) and the copyright owner(s) are credited and that the original publication in this journal is cited, in accordance with accepted academic practice. No use, distribution or reproduction is permitted which does not comply with these terms.
*Correspondence: Jing Wang, wangjing@cicams.ac.cn; Yi Fang, fangyi@cicams.ac.cn; Zhongzhao Wang, wangzhongzhao206@sina.com
†These authors have contributed equally to this work