- 1School of Life Science, Anhui Agricultural University, Hefei, China
- 2Mabwell (Shanghai) Bioscience Co., Ltd., Shanghai, China
Globally, gastric cancer (GC) is one of the three most deadly cancers. Dendrobium officinale (D. officinale) is a traditional Chinese medicine (TCM), and its extract can significantly inhibit the proliferation of gastric cancer cells. However, there are no unified conclusions on its potential active components and possible mechanisms of action. This paper aims at exploring the potential active components, targets, and cell pathways of D. officinale extract in inhibiting the proliferation of gastric cancer cells by using network pharmacology and cytology experiments. In this paper, UPLC-MS/MS was used to identify the main chemical components in the extracts of D. officinale, and the an ADME model was used to screen the potential active components. Network pharmacology methods such as target prediction, pathway identification, and network construction were used to determine the mechanism through which the D. officinale extract inhibited gastric cancer cell proliferation. MTT assays, fluorescence confocal microscopy, clone formation, and flow cytometry were used to verify the inhibitory activity of the D. officinale extract on gastric cancer cell proliferation in vitro. The UPLC-MS/MS analysis identified 178 chemical components from the D. officinale extract. Network pharmacology analysis showed that 13 chemical components had the potential to inhibit the proliferation of gastric cancer cells, with the possible involvement of 119 targets and 20 potential signaling pathways. In vitro experiments confirmed that the D. officinale extract could significantly inhibit the proliferation of gastric cancer cells. Therefore, we believe that the D. officinale extract can inhibit the proliferation of gastric cancer cells through effects on multiple components, multiple targets, and multiple pathways.
Introduction
Gastric cancer is a malignant tumor with high morbidity and mortality rates (Joshi and Badgwell, 2021). Although the incidence of gastric cancer has decreased in recent years, it is still the third leading cause of cancer-related death in the world. The 5-year relative survival rate of patients with gastric cancer in most countries is still less than 30% (Chen et al., 2016). Global cancer statistics for 2018 indicate more than 1 million new cases of gastric cancer and an estimated 783,000 deaths (Bray et al., 2018). Although surgical resection and chemotherapy are used to improve the therapeutic effect of gastric cancer, their prognosis is not optimistic. Therefore, the development of new anti-gastric cancer drugs will be of great significance.
In contrast to the approach of Western medicine, Chinese medicine researchers generally believe that the multiple compounds in a Chinese herbal medicine can act on multiple various cell targets through different means, which achieves the therapeutic effects with fewer side effects and less drug resistance. According to preliminary studies, Chinese herbal medicine treatments can be helpful to maintain a reduced tumor size and alleviate tumor-related symptoms (Ling et al., 2014). In addition, studies have shown that Chinese herbal medicine alone or in combination with conventional chemotherapy or radiotherapy can improve the immunity and quality of life of patients with tumors (Liu et al., 2017; Xiao and Tao, 2017; Yang et al., 2019). However, the presence of multiple compounds in Chinese herbal medicine also leads to the complexity of its molecular mechanism. Consequently, revealing the mechanism of the multiple components of Chinese herbal medicines is of great significance in promoting the clinical application of Chinese herbal medicine in the field of antitumor research.
D. officinale is a perennial herb belonging to the genus Dendrobium of the family Orchidaceae. As a traditional Chinese herbal medicine and health food, it has been used in China for thousands of years and offers great value for medicine development value and excellent application prospects. Existing studies have shown that D. officinale contains an abundance of diverse secondary metabolites, including polysaccharides, alkaloids, stilbenes (phenanthrenes, bibenzyls), sesquiterpenes, and flavonoids; these compounds, have broad-spectrum biological effects, enhance the immune function of the body (Li et al., 2020), and exert antitumor and adjuvant antitumor effects (Zhang et al., 2020). They are commonly used in the clinical adjuvant treatment of malignant tumors and provide a potential resources for the research and development of antitumor drugs.
In this study, we identified the main bioactive substances of the D. officinale extract. Through a series of network pharmacological analyses including target prediction and enrichment, pathway analysis, and network construction were carried out to determine the potential mechanism of GC-related targets and the D. officinale extract. In addition, to confirm the inhibitory effect of the D. officinale extract on the proliferation of gastric cancer cells, a series of cytological experiments including cell proliferation, monoclonal formation, and apoptosis were performed in three gastric cancer cell lines SGC-7901, MGC-803, and MKN-45. A schematic diagram of the network pharmacological analysis and overall experimental flow for the D. officinale extract in this study is shown in Figure 1. The results of our study not only proved the synergistic anticancer properties of the compounds in D. officinale extract but also provided further insight into the molecular mechanism through which the D. officinale extract inhibits the proliferation of GC cell.
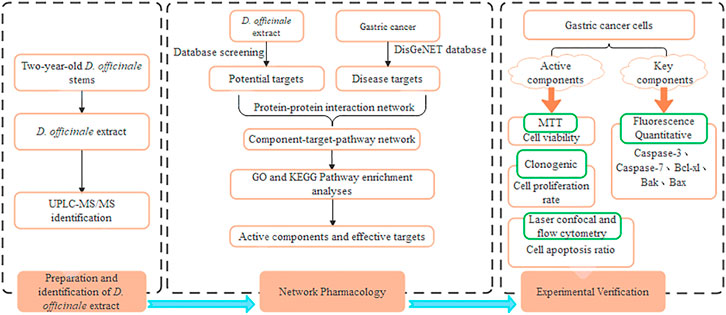
FIGURE 1. Flowchart of the network pharmacological and experimental studies of the D. officinale extract in human gastric cancer cells.
Methods
Preparation of the Dendrobium officinale extract
D. officinale 2-year fresh stems were taken, placed at 105°C for 30 min, dried in a 60°C oven, and ground into powder, through a 40-mesh sieve. An appropriate amount of D. officinale powder was weighed, added with anhydrous ethanol at the ratio of 1:20, soaked at room temperature for 48 h, and ultrasonically extracted at 55°C for 45 min (120 W, 60 HZ). The obtained extract was centrifuged at 6,000 r/min for 10 min. The supernatant was taken and filtered with a .22-μm nylon membrane, then blown to constant weight with nitrogen. An appropriate amount of pure water was added to the above extract to form a suspension, then extracted with an equal volume of petroleum ether, the lower phase was collected, an equal volume of n-butanol was added to continue extraction, and the lower phase was collected and blown to constant weight with nitrogen, which is the D. officinale extract.
Ultra-Performance Liquid Chromatography-Tandem Mass Spectrometry
The 20-mg D. officinale extract was mixed with 300 μl pre-cooled methanol–water solution (v/v = 7:1), ultrasonically extracted for 30 min, centrifuged at 14,000 r/min for 10 min, and filtered by a .22-μm filter membrane. The samples were analyzed by UPLC-MS/MS (Thermo Finnigan, San Jose, CA, USA). The specific chromatographic conditions were as follows: column temperature was 40°C, flow rate was 0.35 ml/min, and sample volume was 5 μl.
Under positive ion mode, the chromatographic column was ACQUITY™ BEHC8 (100 mm × 2.1 mm, 1.7 μm). Mobile phase A was .1% formic acid–water, and mobile phase B was .1% formic acid–acetonitrile. The capillary temperature is 350°C, and the standard pressure of sheath and auxiliary gas is 45 and 10 MPa, respectively.
Under negative ion mode, the chromatographic column was ACQUITY™ HSS T3 (100 mm × 2.1 mm, 1.8 μm), the mobile phase A was 0.1% formic acid–water, and the mobile phase B was .1% formic acid–acetonitrile. The capillary temperature is 360°C, and the standard pressure of sheath and auxiliary gas is 50 and 13 MPa, respectively.
In the positive and negative ion modes, the liquid chromatography-double partial pressure linear trap-electrostatic field orbital trap tandem mass spectrometer (Thermo Finnigan, San Jose, CA, USA) was used for analysis (scanning range m/z: 50–1,000), and the spray voltage was +3.5 kV/−3.0 kV.
Network Pharmacology-Based Analysis
Identification of Associated MolecularTargets of Dendrobium officinale Extract
The potential molecular targets of the D. officinale extract were predicted using the Traditional Chinese Medicines for Systems Pharmacology Database and Analysis Platform (TCMSP) (Huang et al., 2020), the SwissADME (Ge et al., 2019), and the Comparative Toxicogenomics Database (CTD) (Davis et al., 2021).
The GC-associated human genes were comprehensively retrieved from DisGeNET (Pinero et al., 2020).
Protein-Protein Interaction Network
The online software STRING was used to obtain PPI data (Ge et al., 2018). The online software generates a score for each protein interaction information. The higher the score, the greater the credibility of the interaction between the target proteins. Therefore, in the parameter setting, we have selected the human species, and the confidence value is set to high confidence (.700).
Gene Ontology and KEGG Pathway Enrichment Analyses
The GO functional annotation and KEGG pathway analysis were performed on the target proteins in the PPI network using the Metascape database (p ≤ .01), so as to explain the role of these target proteins in molecular function, cell localization, biological processes, and signaling pathways involved.
Network Construction and Analysis
The network was constructed using Cytoscape 3.8.2 software. The constructed component, target, and signaling pathway network was constructed based on the active components obtained from the analysis, the predicted target proteins, and signaling pathways related to human gastric cancer. The nodes in the network diagram represent the chemical components analyzed or the predicted target proteins and signaling pathways, and the edges represent the compound–target or target–pathway interactions.
Experimental Verification
Chemicals and Reagents
D. officinale (2-year-old) was purchased from the Dendrobium base in Huoshan County, Anhui Province, China (simulated field growing environment). All chemical reagents and solutions were of analytical grade or LC-MS mass spectrometry grade, and methanol, acetonitrile, formic acid, and ammonium bicarbonate were purchased from CNW (Dusseldorf, Germany). Fetal bovine serum (FBS) was purchased from Sangon Biotech (Shanghai, China). RPMI Medium Modified 1640 medium and phosphate-buffered saline (PBS) were purchased from HyClone, Thermo Scientific (MA). Thiazolyl Blue Tetrazolium Blue (MTT) was purchased from Sigma (St. Louis, MO, USA). Annexin V-FITC/PI Double Staining Kit was purchased from BD (Franklin Lakes, NJ, USA). RNeasy™ Animal RNA Isolation Kit with Spin Column was purchased from Beyotime (Shanghai, China). TransStart® Green qPCR SuperMix and TransScript® Reverse Transcriptase [M-MLV,R-NaseH-] were purchased from TransGen Biotech (Beijing, China).
Cell Lines and Cell Culture
The Human GC cell lines SGC-7901, MGC-803, and MKN-45 were donated by Dr. Guodong Shen’s research group of the First Affiliated Hospital of University of Science and Technology of China. The cells were placed in RPMI-1640 medium containing 10% FBS and cultured in a carbon dioxide incubator (CO2 concentration of 5%, culture temperature of 37°C).
MTT Assay
The cell viability was determined by MTT assay. Cells were seeded in 96-well plates and treated with PBS and different concentrations of D. officinale extract (.0625, .125, .25,.5, 1, and 2 mg/ml). After incubation in the carbon dioxide incubator for 24 h, 20 μl MTT (5 mg/ml) was added to each well, and the culture was continued for 4 h. Then, MTT was discarded and 150 μl DMSO was added per well. The absorbance was measured at 495 nm by Molecular Devices (San Jose, CA, USA).
Clonogenic Assay
The cells were seeded onto 6-well plates (104 cells/well). SGC-7901 cells were treated with 0, .015, .031, .062, .125, and .250 mg/ml D. officinale extract for 24 h, respectively. The medium was replaced by fresh 1,640 medium without the D. officinale extract and then cultured for 2 weeks. The plate was washed with a PBS buffer solution, and Giemsa staining was used to observe cell colony formation.
Laser Confocal Assay
A total of 1 ml of cells growing in the logarithmic phase with a concentration of 104 cells/l was placed in a laser confocal culture dish. After the cells adhered to the wall and grew, the supernatant was discarded. The cells were treated with RPMI 1640 medium containing different concentrations of the D. officinale extract, and the same volume of RPMI 1640 medium was used as the control. After 24 h of extraction, the cells were rinsed twice with precooled PBS. Then, 100 μl 1× binding buffer was added, and 5 μl Annexin V-FITC and 5 μl PI were added. The cells were gently mixed and incubated in the dark at room temperature for 15 min, washed with precooled PBS three times, and observed by laser confocal microscopy.
Flow Cytometry
The cells in the logarithmic growth phase were placed in a disposable sterile plate, and the supernatant was discarded after adherent growth. The RPMI 1640 medium containing different concentrations of D. officinale extract was added, and the RPMI 1640 medium containing equal volumes was used as control. Each treatment group was set up in three parallel groups. After 24 h, the cells were digested with .25% trypsin and washed twice with precooled PBS. After passing through a 200-mesh sieve, 100 μl 1× binding buffer was added, and then 5 μl Annexin V-FITC and 5 μl PI were added. The cells were gently mixed and incubated in the dark at room temperature for 15 min.
Fluorescence Quantitative Detection
The cells in the logarithmic growth phase were taken, and the group of control groups without drug treatment was set. The experimental group was added with 0.5 mg/ml D. officinale extract. After treatment for 30 min and 2 and 4 h, the relative expression levels of each gene were detected.
Results
Identification of Bioactive Components in Dendrobium officinale Extract
The extracts of D. officinale were determined by UHPLC-LTQ Orbitrap: Ultimate 3000 Velos Pro (Thermo Finnigan, San Jose, CA, USA) and OSI/SMMS (Dalian Dashuo, Dalian, China), and 178 compounds were identified using MS2 and RT/MS1. The 178 identified chemical components include secondary metabolites such as alkaloids, terpenes, and stilbene. The obtained chemical components were analyzed using the TCMSP database and the SwissADME web tool, and the ADME model was used for evaluation. ADME stands for “absorption (A), distribution (D), metabolism (M), and efflux (E),” and represents the processes that drugs are subjected to after entering the body. The screening rules for the TCMSP were OB ≥ 30% and DL ≥ .18. The screening rules for SwissADME were high gastrointestinal absorption (GI absorption) properties in the Pharmacokinetics column and at least two YESs for the first five conditions in the Druglikeness column. Through this screening, 13 qualifying compounds were obtained. The details are shown in Figure 2 and Table 1.
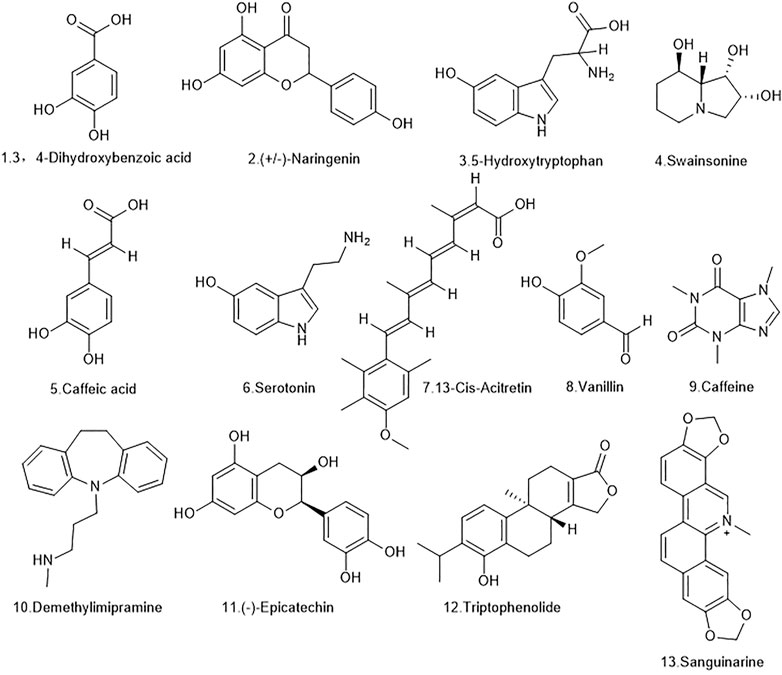
FIGURE 2. The molecular formulas of the 13 bioactive components identified from D. officinale extract.
Identification of GC-Related Targets of Dendrobium officinale Extract
Based on the 13 active substances screened, 119 targets were obtained in the Swiss Target Prediction and TCMSP databases (Supplementay Table S1), and 343 genes related to human gastric cancer were identified from the DisGeNET database (Supplementay Table S2). 94 overlapping genes between D. officinale extract-associated genes and human gastric cancer-associated genes were obtained by the online tool Venny 2.1.0 software (Table 2).
Component-Target Network Analysis
In order to better understand the potential mechanism of the D. officinale extract on gastric cancer, we used software Cytoscape 3.8.2 to construct the component–target network and component–target–pathway network. As shown in Figure 3, the component–target interaction network has 13 composite nodes and 94 target nodes with 191 edges. The network indicated the potential relationship between active substances and targets, thus revealing the potential mechanism of the D. officinale extract in the treatment of gastric cancer. The active substance with the highest degree of connection to the target is likely to be the substance that plays a major role in the treatment of gastric cancer. The active substance with the highest degree value was Caffeine (degree = 57), the active substances (−)-Epicatechin and (±)-Naringenin also had high degree values, which were 40 and 23, respectively. This indicates that a single compound can affect multiple targets, which may be related to the role of the D. officinale extract in the treatment of gastric cancer.
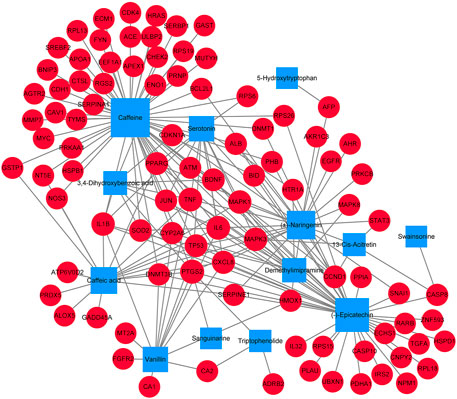
FIGURE 3. Component-target network of D. officinale extract and gastric cancer. The blue nodes represent candidate active components, and the red nodes represent potential protein targets. The edges represent the interactions between them, and the node sizes are proportional to the node degrees.
From the analysis of the target nodes, PTGS2, IL6, and TNF were associated with 9, 8, and 8 active substances, respectively. IL1B, MAPK1, and MAPK3 were associated with seven active substances, respectively; cXCL8 was associated with six active substances. These results showed that a variety of compounds could act on a single target in an interactive manner, indicating that they play a therapeutic role in gastric cancer through multiple components and multiple targets.
PPI Network of Dendrobium officinale Extract-Related GC Target
The PPI network was constructed based on the protein interaction screened from the related targets of the D. officinale extract on gastric cancer. The PPI network in Figure 4 contains 91 nodes and 135 boundaries. In the PPI network, nodes with larger degree values often play a more important role (Figure 5; Supplementay Table S3). It can be seen that the degree values of TP53, STAT3, JUN, MAPK3, EGFR, HRAS, MAPK8, MAPK1, and other eight target proteins are not less than 20, which are likely to be the key targets of the D. officinale extract for gastric cancer.
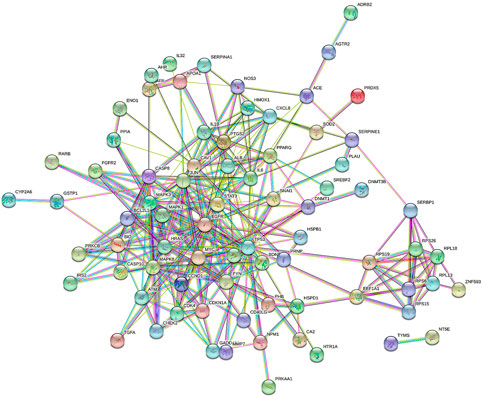
FIGURE 4. Protein–protein interaction (PPI) network of D. officinale extract-related targets in gastric cancer.
GO and KEGG Pathway Enrichment Analysis
In order to verify the biological characteristics of target genes related to gastric cancer treatment by the D. officinale extract, the GO enrichment analysis of the obtained proteins was performed using the Metascape database (p < .01, Figure 6A). The results showed that these proteins were related to 20 molecular functions, among which kinase binding, protein homodimerization activity, and protein kinase activity accounted for a higher proportion. Correlated with 16 cell types, the perinuclear region of the cytoplasm is the first. These proteins were related to 20 biological processes, with the largest association with apoptotic signaling pathway, positive regulation of cell death, cellular response to nitrogen compound, and response to drug (Supplementay Table S4).
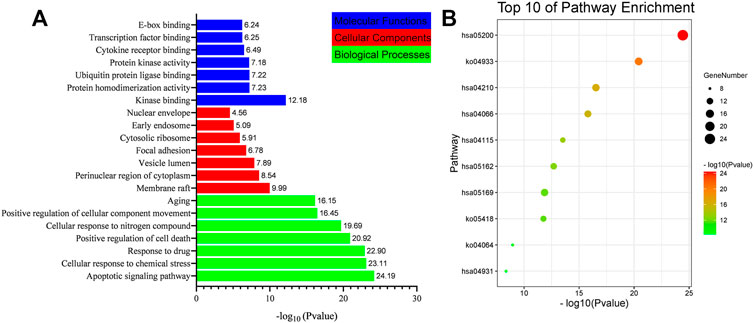
FIGURE 6. List of Gene Ontology (GO) and Kyoto Encyclopedia of Genes and Genomes (KEGG) pathway enrichment results in relation to the potential targets of D. officinale extract. (A) The first 21 GO terms were identified based on p < .01. (B) The top 10 pathways were identified based on p < .01.
In order to verify the correlation between the biological process involved in the target protein and the occurrence of gastric cancer, KEGG pathway analysis was performed for the target proteins (p < .01), and 20 signaling pathways were identified (Figure 6B). These signaling pathways included Pathway in cancer, the AGE-RAGE signaling pathway in diabetic complications, apoptosis, Epstein–Barr virus infection, HIF-1 signaling pathway, fluid shear stress and atherosclerosis, and the p53 signaling pathway (Supplementay Table S5). To better elucidate the relationship between target proteins and signaling pathways, we used Cytoscape 3.8.2 to construct a target-signaling pathway network (Figure 7) consisting of 94 nodes (20 signaling pathways and 74 target proteins) and 249 edges.
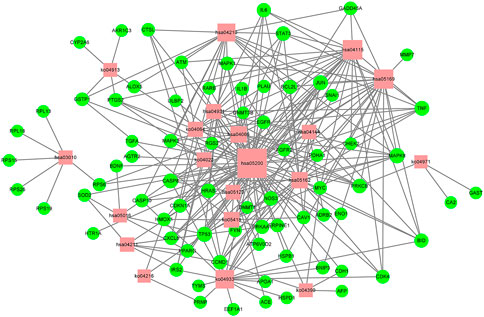
FIGURE 7. Target-pathway network of the D. officinale extract. The red nodes represent the pathways, and the green nodes represent the targets. The size of the nodes is proportional to the degree of the node.
In order to provide further illustration of the regulatory effect of active components of D. officinale’s extract on signaling pathways through target proteins, we constructed a component–target–signaling pathway network diagram (Figure 8), which included 127 nodes (including 13 active substances, 94 target proteins, and 20 signaling pathways) and 439 edges. Among the 94 target proteins, TNF, PTGS2, IL6, TP53, MAPK3, MAPK1, MAPK8, IL1B, JUN, and CASP8 were identified as relatively high-participation molecules, indicating that these molecules may play an important role in the development of GC.
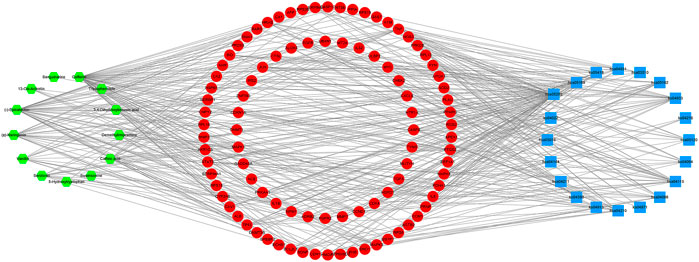
FIGURE 8. Component–target–pathway network of the D. officinale extract. The green nodes represent the component, and the red nodes represent the targets and the blue nodes represent the pathways.
Dendrobium officinale Extract Inhibited GC Proliferation
In order to verify the effect of the D. officinale extract on the proliferation of gastric cancer cells, a series of cytological experiments was performed. The MTT assay was conducted to identify the effects of the D. officinale extract on the viability of SGC-7901, MGC-803, and MKN-45 cells. After exposure to 0–2 mg/ml of the D. officinale extract for 24 h, a dose-dependent decrease in cell viability was observed (Figure 9A). The IC50 (half-maximal inhibitory concentration) values of the D. officinale extract in SGC-7901, MGC-803 and MKN-45 cells were 0.160, 0.250 and 0.285 mg/ml, respectively.
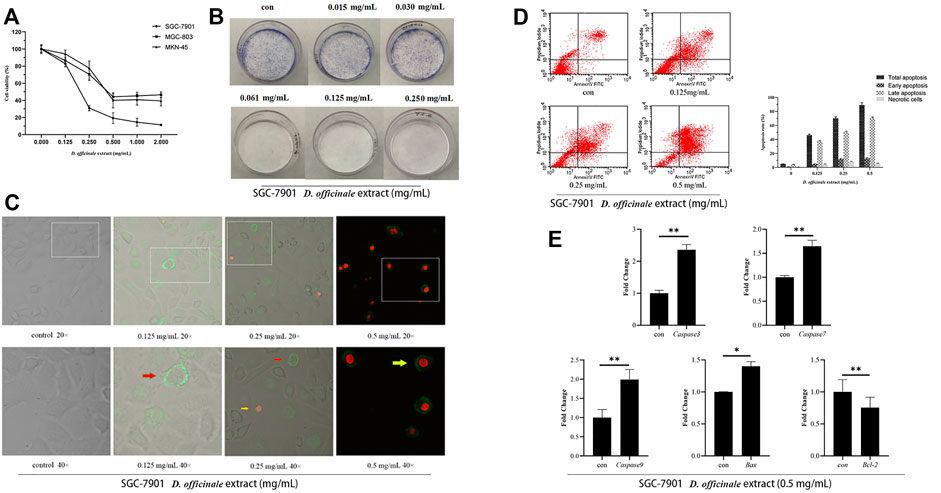
FIGURE 9. D. officinale extract exhibited anti-cancer effects in SGC-7901, MGC-803, and MKN-45 cells. (A) The cell viability was assessed by MTT assay after D. officinale extract administration for 24 h. The cells were incubated with 0–2 mg/ml of D. officinale extract. n = 3. (B) Colony formation in the presence of D. officinale extract. n = 3. (C) Confocal laser analysis of GC cells treated with D. officinale extract. n = 3. (D) Apoptosis analysis of D. officinale extract-treated GC. n = 3. (E) Fluorescence quantitative detection of Caspase-3, Caspase-7, Caspase-9, Bax, and Bcl-2 after treating GC cells with .5 mg/ml D. officinale extract for 4 h *p < .05, **p < .01 vs. con.
Here, we examined the anticancer effect of the D. officinale extract in SGC-7901 cells. The cells were exposed to the D. officinale extract (.015–.250 mg/ml) for 24 h. The colony formation assay was used to detect the ability of SGC-7901 cells to form colonies in the presence of the D. officinale extract. The results showed that the D. officinale extract could significantly reduce the formation of SGC-7901 cell colonies (Figure 9B). In addition, the results of laser confocal microscopy and flow cytometry showed that the D. officinale extract could induce SGC-7901 cell apoptosis (Figures 9C,D).
Discussion
We explored the molecular mechanism through which the D. officinale extract inhibited the proliferation of gastric cancer cells by network pharmacology. In this paper, a series of databases were used to screen the potential active substances of the D. officinale extract and identify their related targets. The protein interaction network identified the key proteins associated with the inhibition of gastric cancer proliferation by the D. officinale extract. The results showed that the D. officinale extract directly or indirectly interacted with eight key targets such as TP53, STAT3, JUN, MAPK3, EGFR, HRAS, MAPK8, and MAPK1, thus exerting anticancer effects.
TP53 connects 35 nodes in the PPI network. Its main functions are to control cell-cycle progression, cell senescence, DNA repair, apoptosis, and the inhibition of angiogenesis. The inhibition of TP53 promotes the development of gastric cancer (Feng et al., 2019), which means that TP53 acts as a tumor suppressor in human gastric cancer. EGFR is a transmembrane glycoprotein that connects 22 nodes in the PPI network. The activation of EGFR may lead to the activation of multiple signaling pathways, thereby regulating cell proliferation and differentiation (Komposch and Sibilia, 2015). MAPK8, MAPK1, and MAPK3 are members of the MAPK family, and they are involved in cell proliferation, differentiation, migration, transformation, and apoptosis. JUN, MAPK8, MAPK1 and MAPK3 are signal proteins in the MAPK signaling pathway, and they are involved in the process of apoptosis (Li et al., 2021). Therefore, these proteins are the key targets of the D. officinale extract inhibiting gastric cancer cell proliferation.
The main feature of cancerous cells is that they are immortalized without apoptosis. Screening assays for antitumor compounds in natural plants have revealed that the target substances have cytotoxic effects, that can directly kill cancer cells rather than through the induction of apoptosis (Hengartner, 2000). Screening for compounds that can induce apoptosis in tumor cells is conducive to reducing the toxicity of drugs and lead to better development prospect. In this paper, GO enrichment analysis and KEGG pathway analysis were performed, and it was found that the potential active components of the D. officinale extract could act on signaling pathways such as axon guidance and the MAPK signaling pathway. The activation of the caspase signal transduction pathway can play an anticancer role by inducing SGC-7901 cell apoptosis.
Members of the caspase family of proteins play an important role in the regulation of apoptosis (McIlwain et al., 2013; Wnek et al., 2017; Van Opdenbosch and Lamkanfi, 2019). Bcl-2 family members are important regulators of the endogenous pathway of mitochondrial initiation. Based on their regulatory function, proteins in the Bcl-2 family are divided into two categories, antiapoptotic and apoptotic. The anti-apoptotic members include Bcl-2, Bcl-xl, and Mcl-1 and apoptotic members include Bak and Bax. Bcl-2 family members form dimers, which interact with each other, affecting the permeability of mitochondrial membrane, releasing apoptosis-related factors and regulating apoptosis (Nagata, 2018). Quantitative fluorescence experiments showed that the expression levels of Caspase-3, Caspase-7, Caspase-9, Bax, and other genes in SGC-7901 cells treated with the D. officinale extract at different times were significantly upregulated, while the expression level of Bcl-2 decreased (Figure 9E), this was consistent with the results of the network pharmacology analysis.
Conclusion
In this study, 13 potential active components were identified by screening the extract of D. officinale. Through a network pharmacology analysis, it was clarified that the D. officinale extract inhibited the proliferation of gastric cancer cells through various signalling pathways in cancer, including PI3K signaling pathway, WNT signaling pathway, and HIF-1 signaling pathway. The expression of Bcl-2 was inhibited and the expression of Bax was upregulated. Moreover, the expression levels of Caspase-3, Caspase-7, and Caspase-9 were upregulated, and the activation of the caspase signaling pathway led to the development of apoptosis.
Data Availability Statement
The original contributions presented in the study are included in the article/Supplementary Material; further inquiries can be directed to the corresponding author.
Author Contributions
Conceptualization, LW and YC; data curation, LW, WD, and ZH; formal analysis, LW, WD, and CC; methodology, LW and WD; project administration, LW, QJ, JH, and YC; resources, LW, JH, and YC; writing—original draft, WD, LW, and CC.
Funding
This work was supported by the Natural Science Research Project of Provincial Natural Science Foundation of Anhui (1908085MC82 and 2108085MC80), the Natural Science Research Project of Anhui Higher Education Institution (YJS20210229) and Anhui Provincial Engineering Technology Research Center for Development & Utilization of Regional Characteristic Plants which were not involved in the study design or the collection, analysis, and interpretation of data.
Conflicts of Interest
Author ZH was employed by the company Mabwell (Shanghai) Biotechnology Co., Ltd.
The remaining authors declare that the research was conducted in the absence of any commercial or financial relationships that could be construed as a potential conflict of interest.
Publisher’s Note
All claims expressed in this article are solely those of the authors and do not necessarily represent those of their affiliated organizations, or those of the publisher, the editors, and the reviewers. Any product that may be evaluated in this article, or claim that may be made by its manufacturer, is not guaranteed or endorsed by the publisher.
Acknowledgments
We thank Dr. Guodong Shen and our colleagues in our laboratory for their technical assistance and helpful comments.
Supplementary Material
The Supplementary Material for this article can be found online at: https://www.frontiersin.org/articles/10.3389/fphar.2022.832134/full#supplementary-material
References
Bray, F., Ferlay, J., Soerjomataram, I., Siegel, R. L., Torre, L. A., and Jemal, A. (2018). Global Cancer Statistics 2018: GLOBOCAN Estimates of Incidence and Mortality Worldwide for 36 Cancers in 185 Countries. CA Cancer J. Clin. 68 (6), 394–424. doi:10.3322/caac.21492
Chen, W., Zheng, R., Baade, P. D., Zhang, S., Zeng, H., Bray, F., et al. (2016). Cancer Statistics in China, 2015. CA Cancer J. Clin. 66 (2), 115–132. doi:10.3322/caac.21338
Davis, A. P., Grondin, C. J., Johnson, R. J., Sciaky, D., Wiegers, J., Wiegers, T. C., et al. (2021). Comparative Toxicogenomics Database (CTD): Update 2021. Nucleic Acids Res. 49 (D1), D1138–D1143. doi:10.1093/nar/gkaa891
Feng, L., Li, J., Li, F., Li, H., Bei, S., Zhang, X., et al. (2019). Long Noncoding RNA VCAN-AS1 Contributes to the Progression of Gastric Cancer via Regulating P53 Expression. J. Cel. Physiol. 235 (5), 4388–4398. doi:10.1002/jcp.29315
Ge, Q., Chen, L., Tang, M., Zhang, S., Liu, L., Gao, L., et al. (2018). Analysis of Mulberry Leaf Components in the Treatment of Diabetes Using Network Pharmacology. Eur. J. Pharmacol. 833, 50–62. doi:10.1016/j.ejphar.2018.05.021
Ge, B., Guo, C., Liang, Y., Liu, M., and Wu, K. (2019). Network Analysis, and Human and Animal Studies Disclose the Anticystitis Glandularis Effects of Vitamin C. Biofactors 45 (6), 912–919. doi:10.1002/biof.1558
Hengartner, M. O. (2000). The Biochemistry of Apoptosis. Nature 407 (6805), 770–776. doi:10.1038/35037710
Huang, J., Chen, F., Zhong, Z., Tan, H. Y., Wang, N., Liu, Y., et al. (2020). Interpreting the Pharmacological Mechanisms of Huachansu Capsules on Hepatocellular Carcinoma through Combining Network Pharmacology and Experimental Evaluation. Front. Pharmacol. 11, 414. doi:10.3389/fphar.2020.00414
Joshi, S. S., and Badgwell, B. D. (2021). Current Treatment and Recent Progress in Gastric Cancer. CA Cancer J. Clin. 71 (3), 264–279. doi:10.3322/caac.21657
Komposch, K., and Sibilia, M. (2015). EGFR Signaling in Liver Diseases. Int. J. Mol. Sci. 17 (1), 30. doi:10.3390/ijms17010030
Li, M., Yue, H., Wang, Y., Guo, C., Du, Z., Jin, C., et al. (2020). Intestinal Microbes Derived Butyrate Is Related to the Immunomodulatory Activities of Dendrobium Officinale Polysaccharide. Int. J. Biol. Macromol 149, 717–723. doi:10.1016/j.ijbiomac.2020.01.305
Li, Y. L., Zhou, D. J., Cui, Z. G., Sun, L., Feng, Q. W., Zakki, S. A., et al. (2021). The Molecular Mechanism of a Novel Derivative of BTO-956 Induced Apoptosis in Human Myelomonocytic Lymphoma Cells. Apoptosis 26 (3-4), 219–231. doi:10.1007/s10495-021-01664-2
Ling, C. Q., Yue, X. Q., and Ling, C. (2014). Three Advantages of Using Traditional Chinese Medicine to Prevent and Treat Tumor. J. Integr. Med. 12 (4), 331–335. doi:10.1016/s2095-4964(14)60038-8
Liu, X., Xiu, L. J., Jiao, J. P., Zhao, J., Zhao, Y., Lu, Y., et al. (2017). Traditional Chinese Medicine Integrated with Chemotherapy for Stage IV Non-surgical Gastric Cancer: A Retrospective Clinical Analysis. J. Integr. Med. 15 (6), 469–475. doi:10.1016/s2095-4964(17)60377-7
McIlwain, D. R., Berger, T., and Mak, T. W. (2013). Caspase Functions in Cell Death and Disease. Cold Spring Harb. Perspect. Biol. 5 (4), a008656. doi:10.1101/cshperspect.a008656
Nagata, S. (2018). Apoptosis and Clearance of Apoptotic Cells. Annu. Rev. Immunol. 36, 489–517. doi:10.1146/annurev-immunol-042617-053010
Piñero, J., Ramírez-Anguita, J. M., Saüch-Pitarch, J., Ronzano, F., Centeno, E., Sanz, F., et al. (2020). The DisGeNET Knowledge Platform for Disease Genomics: 2019 Update. Nucleic Acids Res. 48 (D1), D845–D855. doi:10.1093/nar/gkz1021
Van Opdenbosch, N., and Lamkanfi, M. (2019). Caspases in Cell Death, Inflammation, and Disease. Immunity 50 (6), 1352–1364. doi:10.1016/j.immuni.2019.05.020
Wnęk, A., Andrzejewska, E., Kobos, J., Taran, K., and Przewratil, P. (2017). Molecular and Immunohistochemical Expression of Apoptotic Proteins Bax, Bcl-2 and Caspase 3 in Infantile Hemangioma Tissues as an Effect of Propranolol Treatment. Immunol. Lett. 185, 27–31. doi:10.1016/j.imlet.2017.03.005
Xiao, L.-J., and Tao, R. (2017). “Traditional Chinese Medicine (TCM) Therapy,” in Substance and Non-Substance Addiction. Editors X. Zhang, J. Shi, and R. Tao (Singapore: Springer Singapore), 261–280. doi:10.1007/978-981-10-5562-1_13
Yang, L., Zhao, L., Zeng, T., Chen, H., Shao, J., Yang, S., et al. (2019). Mechanisms Underlying Therapeutic Effects of Traditional Chinese Medicine on Gastric Cancer. Cancer Manag. Res. 11, 8407–8418. doi:10.2147/CMAR.S218214
Keywords: D. officinale, gastric cancer, network pharmacology, protein-protein interaction, MTT
Citation: Wei L, Dong W, Han Z, Chen C, Jin Q, He J and Cai Y (2022) Network Pharmacologic Analysis of Dendrobium officinale Extract Inhibiting the Proliferation of Gastric Cancer Cells. Front. Pharmacol. 13:832134. doi: 10.3389/fphar.2022.832134
Received: 09 December 2021; Accepted: 17 January 2022;
Published: 25 March 2022.
Edited by:
Jiang-Jiang Qin, Institute of Cancer and Basic Medicine (CAS), ChinaReviewed by:
Bang xing Han, West Anhui University, ChinaJing Sun, Institute of Food Science and Technology (CAAS), China
Copyright © 2022 Wei, Dong, Han, Chen, Jin, He and Cai. This is an open-access article distributed under the terms of the Creative Commons Attribution License (CC BY). The use, distribution or reproduction in other forums is permitted, provided the original author(s) and the copyright owner(s) are credited and that the original publication in this journal is cited, in accordance with accepted academic practice. No use, distribution or reproduction is permitted which does not comply with these terms.
*Correspondence: Yongping Cai, eXBjYWlhaEAxNjMuY29t
†These authors have contributed equally to this work and share first authorship