- 1Pharmacological Research Center of Medicinal Plants, Mashhad University of Medical Sciences, Mashhad, Iran
- 2Department of Pharmacodynamics and Toxicology, School of Pharmacy, Mashhad University of Medical Sciences, Mashhad, Iran
- 3Department of Clinical Biochemistry, School of Medicine, Shahid Beheshti University of Medical Sciences, Tehran, Iran
- 4Department of Clinical Biochemistry, School of Medicine, Kermanshah University of Medical Sciences, Kermanshah, Iran
- 5Department of Pharmacology, School of Medicine, Shahid Beheshti University of Medical Sciences, Tehran, Iran
- 6Neurobiology Research Center, Shahid Beheshti University of Medical Sciences, Tehran, Iran
- 7Pharmaceutical Research Center, Pharmaceutical Technology Institute, Mashhad University of Medical Sciences, Mashhad, Iran
Potassium ion (K+) channels are pore-forming transmembrane proteins that control the transport of K+ ions. Medicinal plants are widely used as complementary therapies for several disorders. Studies have shown that the modulation of K+ channels is most likely involved in various pharmacological effects of medicinal plants. This review aimed to evaluate the modulatory effects of medicinal plants and their active constituents on K+ channels under pathological conditions. This systematic review was prepared according to the Preferred Reporting Items for the Systematic Reviews and Meta-analyses (PRISMA) 2020 guideline. Four databases, including PubMed, Web of Science, embase, and Scopus, were searched. We identified 687 studies from these databases, from which we selected 13 in vivo studies for the review by using the Population, Intervention, Comparison, Outcomes, Study (PICOS) tool. The results of the 13 selected studies showed a modulatory effect of medicinal plants or their active constituents on ATP-sensitive potassium channels (KATP), and small (SKCa) and large (BKCa) conductance calcium-activated K+ channels in several pathological conditions such as nociception, brain ischemia, seizure, diabetes, gastric ulcer, myocardial ischemia-reperfusion, and hypertension via possible involvement of the nitric oxide/cyclic GMP pathway and protein kinase. K+ channels should be considered as significant therapeutic milestones in the treatment of several diseases. We believe that understanding the mechanism behind the interaction of medicinal plants with K+ channels can facilitate drug development for the treatment of various K+ channel-related disorders.
Introduction
Potassium-selective ion channels are pore-forming proteins that allow the flow of potassium ions (K+) across the plasma membrane. K+ channels regulate a cell’s excitability and resting membrane potential and determine the shape of the action potential waveform in cells such as neurons and myocytes (Mathie et al., 2021). K+ channel families are classified into four groups: voltage-gated K (Kv), calcium-activated (KCa), inwardly rectifying K (Kir), and two-pore domain potassium (K2P) channels (Taura et al., 2021).
Kv channels, the largest subset of K+ channels, assemble as homo- or hetero-tetramers, and the monomers form the central pore domain. Each monomer comprises six transmembrane segments (S1-S6) (Tian et al., 2014). They are activated by membrane depolarization and involved in many important physiological functions, including nervous and cardiac cellular excitability, regulation of hormone secretion such as the insulin release pathway, and immune response. Kv channels are mutated in some cardiac and nervous diseases, such as cardiac arrhythmias, epilepsy, episodic ataxia, and congenital deafness (Blunck and Batulan, 2012).
Calcium-activated K+ channels (KCa) are formed by α-subunit tetramers (Kshatri et al., 2018). KCa channels have been categorized into three classes based on single-channel conductance: small conductance (SKCa), intermediate conductance (IKCa), and large conductance (BKCa) calcium-activated K+ channels. There are eight members in this family of ion channels (Tian et al., 2014). KCa channels are expressed in a wide range of cells, including central nervous system cells, epithelial cells, blood cells, and arterial smooth muscle cells (Kshatri et al., 2018). These channels control the vascular tone, maintain K+ homeostasis, and regulate cellular excitability (Tano and Gollasch, 2014; Kshatri et al., 2018).
The Kir family consists of 15 members categorized into four functional groups. The most important subfamilies include the classical Kir channels (strong inward-rectifier K+ channel/Kir2.x), G-protein-activated Kir channels (GIRK, Kir3.x), ATP-sensitive K+ channels (KATP, Kir6.x), and K+ transport channels (Kir1.x, Kir4.x, Kir5.x, and Kir7.x) (Tian et al., 2014). Kir channels are critical in the control of cellular excitability and K+ ion homeostasis (Mathie et al., 2021).
K2P channels have two pore domains per α-subunit, and each α-subunit contains four transmembrane (TM) segments (TM1-TM4) (Tian et al., 2014). K2P channels are considered “leak channels” for maintaining a negative membrane potential in various cells, including skeletal and heart myocytes, neurons, glia, and different types of epithelial cells (Enyedi and Czirják, 2010; Tian et al., 2014).
Several studies have documented the pathophysiological role of the K+ channel in cardiac arrhythmia, hypertension, epilepsy, Alzheimer’s disease, type 2 diabetes mellitus, and age-related hearing loss (Tian et al., 2014; Burg and Attali, 2021; Singh et al., 2021). Figure 1 illustrates some disorders associated with K+ channel dysfunction.
Moreover, mitochondrial KATP channels are located in the intracellular membrane of mitochondria and composed of pore-forming (MITOK) and ATP-binding (MITOSUR) subunits. These channels play a vital role in mitochondrial physiology and are involved in the homeostatic control of cellular metabolism during stress conditions (Paggio et al., 2019).
For many years, studying K+ channels has been challenging due to the nonspecificity and other problems associated with classical pharmacological tools. However, several methods are currently available for studying K+ channels. Among them, manual voltage-clamp electrophysiology is considered the best method for measuring individual K+ channel activity. Other techniques include radioligand displacement, fluorescent sensors, and thallium flux assays (Weaver and Denton, 2021).
Given that clinically used drugs target only 7% of ligand-ion and 5% of voltage-gated channels, ion channels seem to be underrepresented in the drug discovery program (Djokic and Novakovic, 2020). This is because the chemical diversity information of ion channels available in databases is low, making drug designing against them all the more challenging (Bedoya et al., 2019). Moreover, another limitation is the lack of in vivo studies using specific ligands to determine the exact mechanism of interaction of herbal medicine or its active constituents with K+ channels. Although there are several in vitro studies on the interaction of herbal medicines with other channels or receptors, the lack of in vivo studies makes it difficult to differentiate the effects of herbal medicines on K+ channels from the other channels or receptors.
Several in vitro and in vivo studies have shown that herbal medicines and their active constituents have a variety of pharmacological properties, including antinociceptive, anxiolytic, antidepressant, antidiabetic, antiarrhythmic, antiischemic, gastroprotective, and vasorelaxant effects due to their selective targeting of K+ channels (Joseph et al., 2018; Zakaria et al., 2014; Zambrana et al., 2018; da Rosa et al., 2019; Imtiaz et al., 2019; Li et al., 2008; Li et al., 2019). Since medicinal plants and their active components are critical for modulating the K+ channels, in this review we focus on the interaction of medicinal plants and their constituents with the K+ channels. We believe this review will help identify the possible mechanisms of medicinal plants in various K+ channel-related disorders, which may further accelerate the drug design against these disorders.
Methods
This systematic review was conducted as a guide for the Preferred Reporting Items for Systematic Reviews and Meta-Analyses (PRISMA) 2020 statement consisting of a 27-item checklist, the PRISMA abstract checklist, and a flow diagram (Page et al., 2021).
Search Strategy
Search terms used in the four databases—Scopus, PubMed, Embase, and Web of Science—on April 27, 2021, were “Medicinal plants,” “Herbal medicine,” “Botany,” “Herb,” “Phytotherapy,” “Chinese Herbal Medicine,” “Herbal Preparations,” “Phytochemical,” “Ethnomedicine,’’ and “K+ channel’’ or “potassium channel.” The complete search strategy for all databases, including filters, is shown in Table 1. All titles and abstracts from each database were imported to the reference management software EndNote™ X9, in which duplicate references were excluded. Subsequently, all remaining studies were screened.
Inclusion/Exclusion Criteria
We designed a systematic search strategy by using the Population, Intervention, Comparison, Outcomes, and Study (PICOS) search tool (Methley et al., 2014; Bramer et al., 2018) and selected the articles that follow the PICOS design. The population included all animal models (male and female, of all ages). The intervention included the effect of medicinal plants or their active constituents on K+ channels under pathological conditions, including nociceptive, cerebral ischemia/reperfusion, seizure, diabetes, gastric ulcer, myocardial ischemia/reperfusion models, intestinal motility, and blood pressure. The comparison was between the control group and the group which was treated with the plant extract or its active components. The outcome was the effect of medicinal plants or their active constituents on nociception and writhing, cerebral ischemia, seizure, diabetes, gastric ulcer, intestinal motility, myocardial ischemia-reperfusion, and blood pressure. The study included all in vivo studies related to the effect of medicinal plants or their active constituents specifically on K+ channels and their possible mechanisms, except one study having both in vivo and in vitro experiments, from which only the in vivo experimental data was considered.
The exclusion criteria included review articles, books, editorials, conference abstracts, and letters; studies with no abstract or free full-text access; studies not written in English; studies that did not focus on the interaction of medicinal plants or their active constituents on K+ channels, in vivo; and studies on the role of K+ channels in plant physiology. Further, studies on mixed herbal components with a brand name or in combination with other drugs such as non-steroidal anti-inflammatory drugs, studies with unknown extraction methods, and in silico, ex vivo, and molecular docking studies were removed.
Data Collection and Management
One author (MNA) evaluated the titles and abstracts of the electronic databases with the inclusion criteria. If a title and abstract met the inclusion criteria, the full text of that article was retrieved for further investigation. Two authors (MNA and FB) independently collected data from each full-text paper using the PICOS design and analyzed them. A third researcher (HH) confirmed the data from the study investigators. Data were stored in a file.
Assessment Risk of Bias
The risk of bias (RoB) was assessed independently by two authors (MNA and FB), and disagreements were resolved by a third author (HH). The RoB tool was provided by the SYstematic Review Center for Laboratory animal Experimentation (SYRCLE) for animal intervention studies to assess the risk of bias, which contains 10 criteria (Hooijmans et al., 2014). This tool was adapted from the Cochrane Collaboration RoB tool used in clinical studies (Higgins et al., 2011). The RoB contains 1) sequence generation, 2) baseline characteristics, 3) allocation concealment, 4) random housing, 5) blinding caregivers and/or investigators, 6) random outcome assessment, 7) blinding outcome, 8) incomplete outcome data, 9) selective reporting of outcomes, and (10) other sources of bias (Hooijmans et al., 2014). These items were scored with ‘+’ low risk of bias, ‘−’ high risk of bias, and ‘?’ unclear risk of bias (Su et al., 2021).
Results
Selection of Articles
A total of 687 articles were identified from all the databases—PubMed (72), Scopus (491), Embase (55), and Web of Science (69). Duplicate records were removed using EndNote (n = 173). Subsequently, 514 articles were left. Following the inclusion criteria, another 102 records were excluded, as described in Figure 2. From the remaining 412 studies, 406 were assessed for eligibility, and 13 studies were included in the review. More details are shown in the PRISMA flowchart diagram in Figure 2.
Characteristics of the Included Studies
In all the selected studies, the effects of medicinal plants and their active constituents on K+ channels have been described. A summary of the selected studies is shown in Table 2. There were six studies on the effect of medicinal plants or their active constituents on KATP channels in the peripheral nervous system. Adeyemi et al. (2018) reported that the hydroethanolic leaf extract of Tetracera alnifolia (HeTA; 50, 100, 200, and 400 mg/kg, p.o.) might have antinociceptive effects on acetic acid-induced writhing in mice. Pretreatment of animals with naloxone, l-arginine (L-Arg; precursor of nitric oxide (NO) synthase), or glibenclamide (a KATP channel inhibitor) prevented the antinociceptive effects; however, L-nitro-arginine could not reverse this effect. Hence, the antinociceptive effect of T. alnifolia may occur through the opioid/L-Arg-NO/KATP pathways (Adeyemi et al., 2018).
Brandão et al. (2013) investigated the antinociceptive effect of the ethereal fraction of Lecythis pisonis leaves (LPEF; 50 and 100 mg/kg, p.o.) on the glutamate-evoked nociceptive response in mice. LPEF reduced nociception, and pretreatment with naloxone, L-Arg, or glibenclamide antagonized this effect. Hence, it seems that LPEF exerts its antinociception effect via the opioid/KATP/L-Arg-NO pathways (Brandão et al., 2013).
Ferdous et al. (2020) investigated the antinociceptive effects of the methanol extract of Bougainvillea spectabilis leaves (MEBS; 50, 100, and 200 mg/kg, p.o.) on acetic acid-induced writhing in mice. MEBS reduced the number of writhing episodes and pain. Pretreatment of animals with methylene blue (as an inhibitor of the cGMP pathway) synergized the antinociceptive effect of MEBS; in contrast, pretreatment with glibenclamide reversed the antinociceptive effect. These results suggest that MEBS have antinociceptive effects, possibly through the modulation of KATP channels and cGMP (Ferdous et al., 2020).
Islam et al. (2016) found that the methanol extract of Celosia cristata (MECC; 50, 100, 200, and 400 mg/kg, p.o.) has antinociceptive effects on the acetic acid-induced writhing in mice. For the mechanistic evaluation of the antinociceptive activity of MECC, they pretreated the animals with various compounds. Pretreatment with glibenclamide reversed the antinociceptive effect of MECC, whereas co-administration of methylene blue with MECC (400 mg/kg) amplified the antinociceptive activity. These results indicate that the antinociceptive effect of MECC may be partly related to the cGMP and KATP channels. However, the antinociceptive effects of MECC on central and peripheral nervous systems have been shown in several nociception tests including formalin and glutamate-induced paw licking and edema, immersion test, and hot plate test. From these tests, the possible role of the opioid system was indicated. However, in this review, we considered only the part of the study that evaluated the role of K+ channels in antinociception (Islam et al., 2016).
Khalid et al. (2011) found that the essential oil of Zingiber zerumbet (EOZZ; 50, 100, 200, and 300 mg/kg, i.p.) has an antinociceptive effect in acetic acid-induced writhing in mice. The initial results showed that the i.p. route of EOZZ administration was more potent than the p.o. route. Pretreatment of animals with L-Arg and glibenclamide reversed the antinociceptive effect of EOZZ (200 mg/kg), while pretreatment with methylene blue enhanced the antinociceptive activity. It seems that EOZZ acts via the KATP channels and modulates the L-Arg/NO/cGMP pathway, apart from its possible involvement in the inhibition of the glutamatergic system and transient receptor potential vanilloid 1 (TRPV4) receptors (Khalid et al., 2011).
Shajib et al. (2018) reported that polymethoxyflavones (PMFs; four compounds), the active constituents of the methanol extract of Nicotiana. plumbaginifolia leaves (12.5 and 25 mg/kg, p.o.), have antinociceptive effects in writhing tests in mice. However, pretreatment of animals using glibenclamide decreased the protective effects of these PMFs. For more details, see Table 2. Moreover, the antinociceptive effect of 6,7,4′,5′-dimethylenedioxy-3,5,3′-trimethoxyflavone was greater than that of the other PMFs. Opioid receptors have also been implied in the antinociceptive effect of these PMFs. Hence, these results suggest that the antinociceptive effect of PMFs may be related to the ATP-sensitive K+ channel opening and the opioid system, apart from the possible suppression of inflammatory mediators such as prostaglandins (PGs), cyclooxygenase, lipoxygenase (Shajib et al., 2018).
Han et al. (2018) investigated the role of the total flavon of Rhododendron (TFR) on K+ channels in cerebral brain ischemia/reperfusion in rats. TFR (100 mg/kg, i.v.) improved the pathological injury of the cerebral cortex. Moreover, it increased the protein expression of TRPV4, IKCa, and SKCa channels in the endothelial cells from the cerebral basal artery (CBA), as well as reduced the mean fluorescence intensity of Ca2+ in the smooth muscle cells of CBA. These results suggest that the activation of the TRPV4-dependent pathway has two consequences. First, it opens the endothelial IKCa/SKCa channels, which in turn leads to the hyperpolarization of the endothelium and smooth muscle cell membranes. Second, it activates the BKCa channel and reduces Ca2+ in smooth muscle cells of CBA (Han et al., 2018).
The study by Adongo et al. (2017) investigated the effects of Pseudospondias microcarpa hydroethanolic leaf extract (PME) on the activation of K+ channels in the 4-aminopyridine (4-AP)-induced seizures in mice. They reported that PME (30, 100, 300 mg/kg, p.o.) delayed the latency of both clonic and tonic seizures and protected against 4-AP-induced seizures. It seems that PME acts via the direct activation of the K+ channel and membrane hyperpolarization or through the inhibition of the glutamate signaling pathway. However, this study also found the possible involvement of other systems in different seizure models in animals (Adongo et al., 2017).
Wu et al. (2011) investigated the role of Belamcanda chinensis leaf extract (BCL) on the KATP channel in normal and streptozotocin (STZ)-induced diabetic rats. BCL (400, 800, 1,600 mg/kg, p.o.) lowered the fasting blood glucose levels and oral glucose tolerance in both normal and diabetic animals. Moreover, it increased serum insulin levels in normal rats. However, this effect was reversed in the presence of nicorandil (an ATP-sensitive K+ ion channel opener) and nifedipine (a Ca+2 ion channel blocker). These results indicate that BCL lowers glucose levels and stimulates insulin secretion by closing K+ATP and opening Ca2+ channels (Wu et al., 2011).
Arunachalam et al. (2019) investigated the effect of the hydroethanolic extract of Cochlospermum regium xylopodium (HECr) on the K+ATP channel in an acute gastric ulcer mouse model. They demonstrated the gastroprotective effect of HECr (25, 100, 400 mg/kg, p.o.) in acidified ethanol-induced gastric ulcers in mice. Pretreatment of animals with glibenclamide reduced the antiulcer activity of HECr (100 mg/kg). Furthermore, pretreatment with indomethacin (an inhibitor of PGs), N-nitro-l-arginine methyl ester (l-NAME, a non-selective nitric oxide synthase inhibitor), or yohimbine (α2-adrenoreceptor antagonist) reversed the gastroprotective effect of HECr. Hence, HECr seems to have a gastroprotective effect non-specifically through the activation of K+ATP channels and α2-adrenergic receptors, and the stimulation of PGs and NO (Arunachalam et al., 2019).
Formiga et al. (2017) investigated the role of Maytenus erythroxylon (Me) extract on intestinal motility and the possible involvement of the K+ATP channel in mice. Me (62.5, 125, 250, and 500 mg/kg, p.o.) reduced the percentage of intestinal transit in mice, which was reversed by the pretreatment of animals with glibenclamide, l-NAME, or propranolol. These results indicate that the effect of ME extract on intestinal motility may involve the NO/cGMP/KATP pathways, apart from the modulation of adrenergic receptors (Formiga et al., 2017).
We also found two studies related to the effects of medicinal plants and phytochemicals on the cardiovascular system. Miao et al. (2011) showed that polydatin, a stilbene compound, has a cardioprotective effect in a rat model of myocardial ischemia/reperfusion. Polydatin reduced the heart rate and infarct size, whereas this effect was reversed by 5-hydroxydecanoate (5-HD), a selective blocker of mitochondrial KATP channels, and two potent protein kinase C (PKC) inhibitors, chelerythrine or GF109203X (GF). In addition, the decrease in the release of creatine phosphokinase (CPK) and lactate dehydrogenase (LDH) by polydatin was abolished in the presence of 5-HD, chelerythrine, or GF. These results suggest that the cardioprotective effects of polydatin may be related to the activation of PKC-K+ATP signaling, apart from its free radical scavenging activity that was indicated as a different mechanism for the cardioprotective effect (Miao et al., 2011).
Tirloni et al. (2017) showed that ethanol-soluble fractions from Acanthospermum hispidum (ESAH; 30, 100, and 300 mg/kg, intraduodenal) has a hypotensive effect in normotensive rats, and pretreatment of animals with l-NAME, methylene blue, or tetraethylammonium (TEA; a nonspecific K+ channel blocker) prevented this effect. These results suggest the involvement of the NO/cGMP/K+ channels in the hypotensive response of ESAH (Tirloni et al., 2017).
Discussion
Of the 13 studies discussed in this systematic review, six examined the antinociceptive effects of medicinal plants and their possible involvement with the K+ATP channel. Two studies investigated the neuroprotective effect of medicinal plants and their modulation of the SKCa and IKCa channels. One study examined the anti-diabetic effect of a medicinal plant that acts by closing the K+ATP channel. Two studies evaluated the therapeutic effects of medicinal plants on gastric ulcers and intestinal motility, possibly via modulating the K+ATP channels. Finally, two studies examined the cardioprotective effect of medicinal plants through the possible involvement of K+ channels.
In all 13 studies, K+ channels were demonstrated as a possible pharmacological target of medicinal plants or their active constituents. Hence, in this systematic review, we will focus on the molecular mechanism of the interaction between medicinal plants and their active constituents with K+ channels to evaluate if it can be a novel drug target in the treatment of various diseases.
The Modulatory Effects of Medicinal Plants or Their Active Constituents on K+ Channels in the Nervous System
The Involvement of NO/cGMP/KATP Pathway for Antinociceptive Effects
The antinociceptive effects of medicinal plants HeTA, LPEF, MEBS, MECC, EOZZ, and polymethoxyflavones (active constituents of the methanol extract of N. plumbaginifolia leaves) are linked to the modulation of KATP channels in six studies (Khalid et al., 2011; Brandão et al., 2013; Islam et al., 2016; Adeyemi et al., 2018; Shajib et al., 2018; Ferdous et al., 2020). KATP channels are widely distributed in both the central and peripheral nervous systems. These channels have several physiological functions, including the regulation of neuronal excitability and suppression of hyperalgesia (Li et al., 2021).
Kir6.2, SUR1, and SUR2 are expressed in the dorsal root of the ganglia (DRG) and are important for inhibiting hyperalgesia during severing neurons (Zoga et al., 2010; Tinker et al., 2018). It has been shown that intracellular calcium [Ca2+]i 57 or 610 nM activates DRG neuronal KATP channels via the Ca2+/Ca2+-calmodulin/CaM-dependent kinase II (Ca2+/CaM/CaMKII) signaling pathway. This in turn opens K+ channels, reduces excitability, and exerts possible antihyperalgesic effects. It is suggested that the opening of KATP channels can serve as a novel analgesic target in the treatment of neuropathic pain (Kawano et al., 2009b). NO activated KATP channels in large DRG neurons in the SUR1 subunit through direct S-nitrosylation of cysteine residues (Kawano et al., 2009a).
Furthermore, the effect of medicinal plants or phytochemicals on NO/cGMP signaling was demonstrated in five studies (Khalid et al., 2011; Brandão et al., 2013; Islam et al., 2016; Adeyemi et al., 2018; Ferdous et al., 2020). The studies indicated that the antinociceptive effects of medicinal plants or phytochemicals depend on the activation of ATP-dependent K+ channels due to the modulation of the NO/cGMP signaling pathway.
However, NO has diverse roles in the modulation of analgesia. It may have a nociceptive or antinociceptive effect depending on the animal model, time, dose, and route of administration (Sousa and Prado, 2001; Cury et al., 2011; Staurengo-Ferrari et al., 2014). Several studies have shown the involvement of the l-arginine/NO/cGMP/KATP channel pathway in antinociceptive action (Ghorbanzadeh et al., 2019; Alizamani et al., 2021).
In this review, based on inclusion criteria, we only checked whether cGMP or NO inhibitors could boost the antinociceptive effect of medicinal plants or their active constituents; these medicinal plants or phytochemicals act via modulating the NO/cGMP/KATP channel pathway. Further studies on their role in pain treatment are needed to evaluate their therapeutic potential by targeting the NO/cGMP/KATP channel pathway. A possible mechanism of the interaction of medicinal plants or phytochemicals with K+ channels in nociception is illustrated in Figure 3.
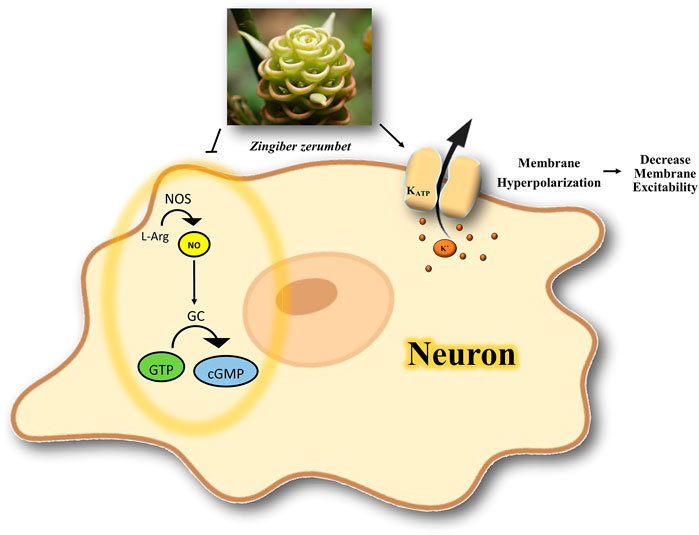
FIGURE 3. Possible mechanism of the interaction of medicinal plants or phytochemicals with K+ channels in nociception. The activation of ATP-dependent K+ channels and modulation of NO/cGMP signaling pathway is involved in antinociception. NOS, nitric oxide synthase; NO, nitric oxide; L-Arg, l-Arginine; GC, guanylate cyclase; GTP, guanosine Triphosphate; cGMP, cyclic guanosine monophosphate; K+, potassium; KATP, ATP-sensitive potassium channel.
The Involvement of BKCa Channels for Anticerebral Ischemia-Reperfusion Injury
Han et al. (2018) demonstrated the protective effect of TFR on ischemic brain injury and determined the functions of TRPV4, SKCa, IKCa, and BKCa channels in cerebral ischemia-reperfusion (Han et al., 2018). There is a link between the activation of TRPV4 and the opening of BKCa channels in the smooth muscle cells that cause dilation in the rat cerebral arteries, resulting in the improvement of hypoperfusion in the infarcted area (Liu et al., 2020b). This vasodilation occurs by the entry of Ca2+ through TRPV4 channels that stimulate Ca2+ release from ryanodine receptors in the sarcoplasmic reticulum. Subsequently, Ca2+ sparks are induced, activating the BKCa channels, thereby leading to hyperpolarization and vasodilation (Liu et al., 2020a). The possible mechanism of the interaction of medicinal plants or phytochemicals with K+ channels in ischemia is summarized in Figure 4. The neuroprotective effects of BKCa channels in cerebral ischemic stroke have been reported (Liu et al., 2020b). Thus, studying the role of TFR or a similar compound on BKCa channels could lead to the development of a potential therapeutic target in ischemia-reperfusion injury.
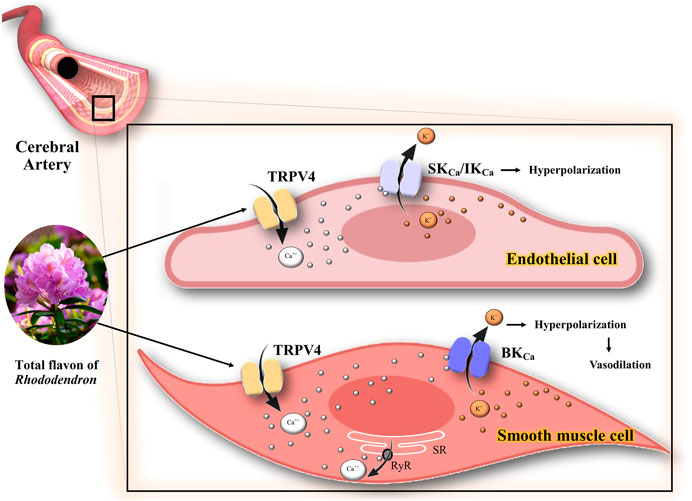
FIGURE 4. Possible mechanism of the interaction of TFR with K+ channels in ischemia. The activation of TRPV4 channels has two consequences: 1) Opening of the endothelial IKCa IKCa/SKCa channels, which in turn leads to the hyperpolarization of the endothelium and smooth muscle cell membranes. 2) Stimulation of Ca++ release from ryanodine receptors in the sarcoplasmic reticulum and opening the BKCa channels, thereby leading to hyperpolarization and vasodilation in smooth muscle cells of CBA. TRPV4, transient receptor potential vanilloid 4; Ca++, calcium; K+, potassium; IKCa, intermediate conductance calcium-activated K+ channels; SKCa, small conductance calcium-activated K+ channels; BKCa, large conductance calcium-activated K+ channels; RyR, ryanodine receptors; SR, sarcoplasmic reticulum.
The Involvement of K+ Channel in Anticonvulsant Effects
PME could have an anticonvulsant effect in the 4-AP-induced seizure. 4-AP is an antagonist of Kv channels (Adongo et al., 2017). It can be administered systemically or intracerebrally to animals to study the anticonvulsant activity of drugs. 4-AP is also a stimulator of voltage-gated Ca+2 channels and contributes to the release of excitatory neurotransmitters such as glutamate (Brito et al., 2009). Recently, the 4-AP model has been used to detect antiepileptic effects in new-generation drugs and induce seizure-like events in in vitro studies (Heuzeroth et al., 2019). Therefore, the activation of K+ channels by medicinal plants like PME can be an important drug target in the treatment of seizures. Further studies are needed on the anticonvulsant activity of PME to investigate the type of Kv channel involved in the protection against 4-AP.
The Involvement of KATP Channels for Antidiabetic Effect
The study by Wu et al. (2011) indicates that BCL lowers glucose levels and increases insulin secretion by closing KATP channels and opening Ca2+ channels. Isoflavone glycosides may be involved in the antidiabetic effect of BCL (Wu et al., 2011). KATP channels are critical in the release of insulin from pancreatic β cells (Tinker et al., 2018). The combination of the subunits of Kir6.2/SUR1 of the KATP channel in pancreatic β-cells regulates the release of insulin (Li et al., 2021). KATP channels provide a link between adenine nucleotides and electrical activity following changes in blood glucose levels in β cells (Rustenbeck et al., 2021). High glucose levels block KATP channels in the cell membrane, leading to depolarization of the membrane and an increase in Ca2+ influx, resulting in exocytosis of insulin granules and vice versa (Wei et al., 2019). Therefore, it seems that the closure of KATP channels by BCL can be a therapeutic target in the treatment of diabetes, and further studies should be conducted to evaluate the hypoglycemic effects of herbal medicine on KATP channels in the pancreas. A possible mechanism of the interaction of BCL with K+ channels in diabetes is shown in Figure 5.
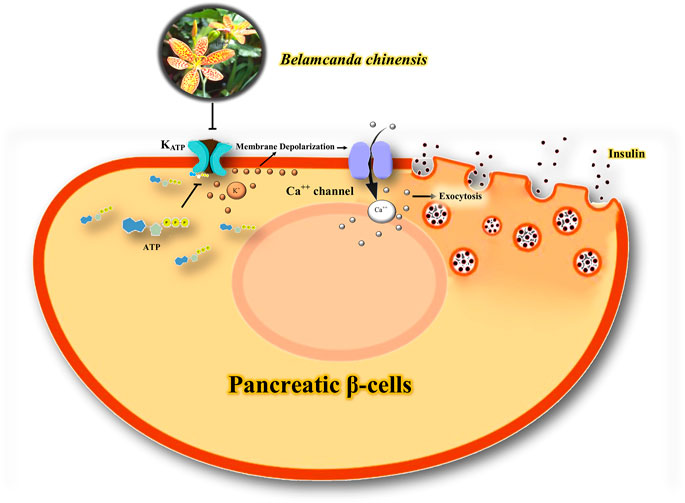
FIGURE 5. Possible mechanism of the interaction of BCL with K+ channels in diabetes. Blockage of KATP channels in the cell membrane leads to depolarization of the membrane and an increase in Ca2+ influx, resulting in exocytosis of insulin granules. ATP, adenosine Triphosphate; KATP, ATP-sensitive potassium channel; Ca++, calcium; K+, potassium.
The Modulatory Effects of Medicinal Plants on NO/cGMP/KATP Cascade in the Gastrointestinal Tract
We found two studies related to the gastroprotective and antimotility effects of medicinal plants in the gastrointestinal tract, which summarize their possible molecular mechanisms.
The Involvement of NO/cGMP/KATP Pathway in Gastroprotective Effects
HECr shows gastroprotective effects through non-specific complexes, including activation of KATP channels, α2-adrenergic receptors, and stimulation of PGs and NO (Arunachalam et al., 2019). Recently, the efficacy of several medicinal plants and possible mechanisms for the treatment of peptic ulcer disease has been discussed (Ardalani et al., 2020). One of the possible gastroprotective effects of medicinal plants and their active constituents is via the NO/cGMP/KATP pathway (Serafim et al., 2020). Endothelial nitric oxide synthase releases NO. NO stimulates soluble guanylyl cyclase (sGC) and increases cGMP in smooth muscle cells, opening the KATP channels (da Silva Monteiro et al., 2019). The efflux of K+ blocks the voltage-sensitive calcium channels, which relaxes the smooth muscle, improves blood flow, and facilitates the healing process (Serafim et al., 2020). PGs are another mediator that could activate the KATP channels (Peskar et al., 2002).
Drugs that could open KATP channels can protect against gastric and small intestine injury induced by ethanol or indomethacin in animals (Akar et al., 1999; Peskar et al., 2002; Menozzi et al., 2011). Since the activation of NO, KATP, and PGs, apart from α2-adrenergic receptors, have gastroprotective effects (Figure 6), it seems that the modulation of the NO/cGMP/KATP signaling by medicinal plants should be considered as a novel therapeutic target for the treatment of gastrointestinal ulcers.
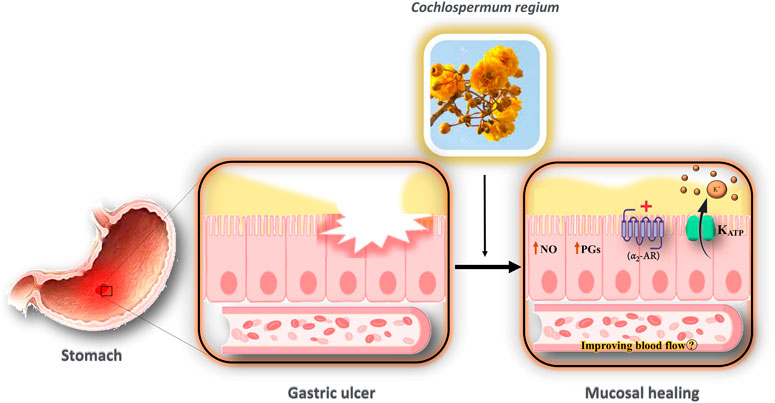
FIGURE 6. Possible mechanism of the interaction of HECr with K+ channels in the gastrointestinal tract. The gastroprotective effect of HECr occurs non-specifically through the activation of K+ATP channels and α2-adrenergic receptors, and the stimulation of PGs and NO. The efflux of K+ blocks the voltage-sensitive calcium channels, which relaxes the smooth muscle, improves blood flow, and facilitates the healing process. NO, nitric oxide; PGs, prostaglandins; K+, potassium; KATP, ATP-sensitive potassium channel; α2-AR, alpha-2 adrenergic receptor.
The Involvement of the NO/cGMP/KATP Pathway for Antimotility
Formiga et al. (2017) indicated that the NO/cGMP/KATP pathway is involved in the inhibitory effect of ME on intestinal motility in mice. However, the modulation of the adrenergic system by ME should not be overlooked (Formiga et al., 2017). NO is an inhibitory co-transmitter released from the enteric nervous system. NO activates sGC, leading to the production of cGMP, which in turn activates K+ channels (Matsuda and Miller, 2010; Modzelewska et al., 2021). The expression of subunits Kir6.2/SUR2B in KATP channels has been reported in the murine colon (Koh et al., 1998; Currò, 2016). It has been shown that the activation of KATP channels leads to resting membrane potential in the colonic smooth muscle of some species (Currò, 2016). The important functional roles of the inwardly rectifying type 6 K+ (Kir6) and KV1.2, 1.5, 2.2, 4.3, 7.4, and 11.1, and KCa1.1 and 2.3 channels were determined in the gastrointestinal smooth muscle. Theoretically, activators of these channels may relax these muscles, thereby promising a new therapeutic target for functional gastrointestinal disorders (Currò, 2016). Hence, the activation of the NO/cGMP/KATP pathway by medicinal plants could be considered as a novel target for the treatment of motility disorders in the gastrointestinal tract.
The Modulatory Effects of Medicinal Plants or Their Active Constituents on K+ Channels in the Cardiovascular System
We found two studies related to the cardioprotective and hypotensive effects of polydatin and ESAH.
The Involvement of PKC-K+ATP Signaling for Antiischemic/Reperfusion Injury
The role of PKC-K+ATP signaling was demonstrated by the finding that 5-HD and two PK inhibitors reversed the cardioprotective effect of polydatin in ischemia/reperfusion injury (Miao et al., 2011). The cardioprotective role of PKC has been reported in ischemic preconditioning (Liu et al., 1996; Murata et al., 2001; Perricone and Vander Heide, 2014; Foster and Coetzee, 2016). PKC could activate mitochondrial KATP channels, possibly leading to an increase in the resistance of mitochondria to mitochondrial permeability transition (Perricone and Vander Heide, 2014). It has been suggested that opening mitochondrial KATP channels partially depolarizes the mitochondrial potential, leading to attenuation of mitochondrial calcium accumulation in ischemia by decreasing the overload of Ca2+ (Murata et al., 2001). A possible mechanism for the interaction of polydatin with K+ channels in the cardiovascular system is shown in Figure 7.
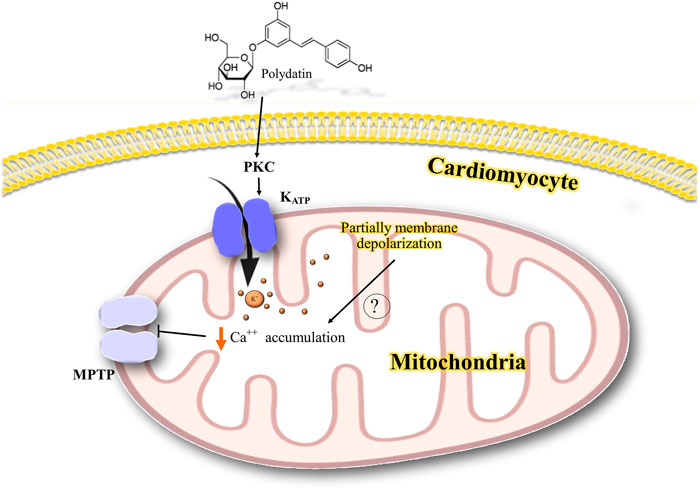
FIGURE 7. Possible mechanism of the interaction of polydatin with K+ channels in the cardiovascular system. PKC activates mitochondrial KATP channels possibly leading to a partial depolarization of mitochondrial potential and reducing the mitochondrial calcium accumulation and inhibiting mitochondrial permeability transition. PKC, protein kinase C; K+, potassium; Ca++, calcium; KATP, ATP-sensitive potassium channel; MPTP, mitochondrial permeability transition pore.
Involvement of NO/cGMP/K+ Channels for Hypotensive Effects
Tirloni et al. (2017) showed that the hypotensive effect of ESAH is linked to the involvement of NO/cGMP and K+ channels in normotensive rats. The hypotensive effect of ESAH was completely inhibited by l-NAME or methylene blue. Thus, it seems that the NO/cGMP signaling also contributes to this effect. However, it is not known how this extract increased endothelial NO activity, which increased cGMP and, in turn, opened K+ channels. Therefore, the trigger for NO release needs to be investigated. However, it seems that the NO/cGMP/K channels could be a new target in the treatment of hypertension (Tirloni et al., 2017).
Methodological Quality/Risk of Bias
The assessment of risk bias is shown in Table 3. Most studies scored 4–6 in our validation. All papers have a low risk of bias for the three domains of “incomplete outcome data,” “selective outcome reporting,” and “other sources of bias.” “Baseline characteristic” is observed with a low risk of bias in 11 publications (84.61%). “Random housing” was reported by six publications (46.15%). However, we found that one study (7.69%) had a low risk of bias in “blinding caregivers and/or investigators”. Furthermore, RoB was unclear for “random sequence generation,” “allocation concealment,” “random outcome assessment,” and “blinding outcome” for 13 papers.
Limitations
This study represents the first systematic evaluation of preclinical in vivo studies related to the effects of medicinal plants or phytochemicals on different K+ channels. Among the screened databases, only 13 studies met the inclusion criteria. We excluded all in vitro or both in vivo and in vitro studies to measure the RoB as the guideline of SYRCLE for animal intervention studies. Another limitation is the lack of clinical studies on the therapeutic role of herbal medicines in K+ channel-related diseases. Furthermore, the interaction of herbal medicines and their active constituents with several K+ channels was determined in various organs with different functions. Hence, a meta-analysis is not feasible because of the heterogeneity among these studies.
Conclusion
Dysregulation of K+ channels has been implicated in the pathophysiology of cardiovascular, gastrointestinal, neurological, and metabolic disorders. This is the first systematic review to show the various biological effects of medicinal plants and their constituents on hypotensive, antiischemic, antidiarrheal, antispasmodic, anti-inflammatory, antinociceptive, and hypoglycemic effects. These effects have been linked to the modulation of the activity of KATP, SKCa, BKCa, and K+ channels via possible involvement of the NO/cGMP pathway and PKC. Hence, K+ channels should be considered as significant therapeutic milestones in the treatment of several diseases. Future studies should focus on new technologies to study phytochemicals or their active constituents that interact with K+ channels to develop novel antinociceptive, anticonvulsant, cardioprotective, gastroprotective, and anti-ischemic therapeutics. We believe that this review will be a reliable guide for the target development and drug design for K+ channel-related disorders.
Data Availability Statement
The raw data supporting the conclusions of this article will be made available by the authors, without undue reservation.
Author Contributions
AR and FR: Writing- Original draft preparation. FB: Methodology, Formal analysis, Investigation, Writing—review & editing. MM: Writing—original draft, Visualization. HH and MN-A: Conceptualization, Methodology, Validation, Formal analysis, Investigation, Writing—review & editing, Project administration, Supervision.
Conflict of Interest
The authors declare that the research was conducted in the absence of any commercial or financial relationships that could be construed as a potential conflict of interest.
Publisher’s Note
All claims expressed in this article are solely those of the authors and do not necessarily represent those of their affiliated organizations, or those of the publisher, the editors and the reviewers. Any product that may be evaluated in this article, or claim that may be made by its manufacturer, is not guaranteed or endorsed by the publisher.
References
Adeyemi, O. O., Ishola, I. O., Adesanya, E. T., and Alohan, D. O. (2018). Antinociceptive and Anti-inflammatory Properties of Tetracera Alnifolia Willd. (Dilleniaceae) Hydroethanolic Leaf Extract. J. Basic Clin. Physiol. Pharmacol. 30 (2), 173–184. doi:10.1515/jbcpp-2016-0190
Adongo, D. W., Mante, P. K., Kukuia, K. K. E., Biney, R. P., Boakye-Gyasi, E., Benneh, C. K., et al. (2017). Anticonvulsant Activity of Pseudospondias Microcarpa (A. Rich) Engl. Hydroethanolic Leaf Extract in Mice: The Role of Excitatory/inhibitory Neurotransmission and Nitric Oxide Pathway. J. Ethnopharmacol 206, 78–91. doi:10.1016/j.jep.2017.05.017
Akar, F., Uydeş-Doğan, B. S., Buharalioğlu, C. K., Abban, G., Heinemann, A., Holzer, P., et al. (1999). Protective Effect of Cromakalim and Diazoxide, and Proulcerogenic Effect of Glibenclamide on Indomethacin-Induced Gastric Injury. Eur. J. Pharmacol. 374 (3), 461–470. doi:10.1016/s0014-2999(99)00277-0
Alizamani, E., Ghorbanzadeh, B., Naserzadeh, R., and Mansouri, M. T. (2021). Montelukast, a Cysteinyl Leukotriene Receptor Antagonist, Exerts Local Antinociception in Animal Model of Pain through the L-Arginine/nitric Oxide/cyclic GMP/KATP Channel Pathway and PPARγ Receptors. Int. J. Neurosci. 131 (10), 1004–1011. doi:10.1080/00207454.2020.1769618
Ardalani, H., Hadipanah, A., and Sahebkar, A. (2020). Medicinal Plants in the Treatment of Peptic Ulcer Disease: A Review. Mini Rev. Med. Chem. 20 (8), 662–702. doi:10.2174/1389557520666191227151939
Arunachalam, K., Damazo, A. S., Pavan, E., Oliveira, D. M., Figueiredo, F. F., Machado, M. T. M., et al. (2019). Cochlospermum Regium (Mart. Ex Schrank) Pilg: Evaluation of Chemical Profile, Gastroprotective Activity and Mechanism of Action of Hydroethanolic Extract of its Xylopodium in Acute and Chronic Experimental Models. J. Ethnopharmacol 233, 101–114. doi:10.1016/j.jep.2019.01.002
Bedoya, M., Rinné, S., Kiper, A. K., Decher, N., González, W., and Ramírez, D. (2019). TASK Channels Pharmacology: New Challenges in Drug Design. J. Med. Chem. 62 (22), 10044–10058. doi:10.1021/acs.jmedchem.9b00248
Blunck, R., and Batulan, Z. (2012). Mechanism of Electromechanical Coupling in Voltage-Gated Potassium Channels. Front. Pharmacol. 3, 166. doi:10.3389/fphar.2012.00166
Bramer, W. M., de Jonge, G. B., Rethlefsen, M. L., Mast, F., and Kleijnen, J. (2018). A Systematic Approach to Searching: an Efficient and Complete Method to Develop Literature Searches. J. Med. Libr. Assoc. 106 (4), 531–541. doi:10.5195/jmla.2018.283
Brandão, M. S., Pereira, S. S., Lima, D. F., Oliveira, J. P., Ferreira, E. L., Chaves, M. H., et al. (2013). Antinociceptive Effect of Lecythis Pisonis Camb. (Lecythidaceae) in Models of Acute Pain in Mice. J. Ethnopharmacol 146 (1), 180–186. doi:10.1016/j.jep.2012.12.028
Brito, V. B., Rocha, J. B., Folmer, V., and Erthal, F. (2009). Diphenyl Diselenide and Diphenyl Ditelluride Increase the Latency for 4-Aminopyridine-Induced Chemical Seizure and Prevent Death in Mice. Acta Biochim. Pol. 56 (1), 125–134. doi:10.18388/abp.2009_2524
Burg, S., and Attali, B. (2021). Targeting of Potassium Channels in Cardiac Arrhythmias. Trends Pharmacol. Sci. 42 (6), 491–506. doi:10.1016/j.tips.2021.03.005
Currò, D. (2016). The Modulation of Potassium Channels in the Smooth Muscle as a Therapeutic Strategy for Disorders of the Gastrointestinal Tract. Adv. Protein Chem. Struct. Biol. 104, 263–305. doi:10.1016/bs.apcsb.2015.12.002
Cury, Y., Picolo, G., Gutierrez, V. P., and Ferreira, S. H. (2011). Pain and Analgesia: The Dual Effect of Nitric Oxide in the Nociceptive System. Nitric Oxide 25 (3), 243–254. doi:10.1016/j.niox.2011.06.004
da Rosa, R. L., de Almeida, C. L., Somensi, L. B., Boeing, T., Mariano, L. N. B., de Medeiros Amorim Krueger, C., et al. (2019). Chrysophyllum Cainito (apple-star): a Fruit with Gastroprotective Activity in Experimental Ulcer Models. Inflammopharmacology 27 (5), 985–996. doi:10.1007/s10787-017-0427-z
da Silva Monteiro, C. E., Franco, Á. X., Sousa, J. A. O., Matos, V. E. A., de Souza, E. P., Fraga, C. A. M., et al. (2019). Gastroprotective Effects of N-Acylarylhydrazone Derivatives on Ethanol-Induced Gastric Lesions in Mice Are Dependent on the NO/cGMP/KATP Pathway. Biochem. Pharmacol. 169, 113629. doi:10.1016/j.bcp.2019.113629
Djokic, V., and Novakovic, R. (2020). Potassium Channels as a Potential Target Spot for Drugs. doi:10.5772/intechopen.92176
Enyedi, P., and Czirják, G. (2010). Molecular Background of Leak K+ Currents: Two-Pore Domain Potassium Channels. Physiol. Rev. 90 (2), 559–605. doi:10.1152/physrev.00029.2009
Ferdous, A., Janta, R. A., Arpa, R. N., Afroze, M., Khan, M., and Moniruzzaman, M. (2020). The Leaves of Bougainvillea Spectabilis Suppressed Inflammation and Nociception In Vivo through the Modulation of Glutamatergic, cGMP, and ATP-Sensitive K+ Channel Pathways. J. Ethnopharmacol 261, 113148. doi:10.1016/j.jep.2020.113148
Formiga, R. O., Quirino, Z. G. M., Diniz, M. F. F. M., Marinho, A. F., Tavares, J. F., and Batista, L. M. (2017). Maytenus Erythroxylon Reissek (Celastraceae) Ethanol Extract Presents Antidiarrheal Activity via Antimotility and Antisecretory Mechanisms. World J. Gastroenterol. 23 (24), 4381–4389. doi:10.3748/wjg.v23.i24.4381
Foster, M. N., and Coetzee, W. A. (2016). KATP Channels in the Cardiovascular System. Physiol. Rev. 96 (1), 177–252. doi:10.1152/physrev.00003.2015
Ghorbanzadeh, B., Kheirandish, V., and Mansouri, M. T. (2019). Involvement of the L-arginine/Nitric Oxide/Cyclic GMP/KATP Channel Pathway and PPARγ Receptors in the Peripheral Antinociceptive Effect of Carbamazepine. Drug Res. (Stuttg) 69 (12), 650–657. doi:10.1055/a-0959-5896
Han, J., Xu, H. H., Chen, X. L., Hu, H. R., Hu, K. M., Chen, Z. W., et al. (2018). Total Flavone of Rhododendron Improves Cerebral Ischemia Injury by Activating Vascular TRPV4 to Induce Endothelium-Derived Hyperpolarizing Factor-Mediated Responses. Evid. Based Complement. Alternat Med. 2018, 8919867. doi:10.1155/2018/8919867
Heuzeroth, H., Wawra, M., Fidzinski, P., Dag, R., and Holtkamp, M. (2019). The 4-Aminopyridine Model of Acute Seizures In Vitro Elucidates Efficacy of New Antiepileptic Drugs. Front. Neurosci. 13, 677. doi:10.3389/fnins.2019.00677
Higgins, J. P., Altman, D. G., Gøtzsche, P. C., Jüni, P., Moher, D., Oxman, A. D., et al. (2011). The Cochrane Collaboration's Tool for Assessing Risk of Bias in Randomised Trials. BMJ 343, d5928. doi:10.1136/bmj.d5928
Hooijmans, C. R., Rovers, M. M., de Vries, R. B., Leenaars, M., Ritskes-Hoitinga, M., and Langendam, M. W. (2014). SYRCLE's Risk of Bias Tool for Animal Studies. BMC Med. Res. Methodol. 14, 43. doi:10.1186/1471-2288-14-43
Imtiaz, S. M., Aleem, A., Saqib, F., Ormenisan, A. N., Neculau, A. E., and Anastasiu, C. V. (2019). The Potential Involvement of an ATP-dependent Potassium Channel-Opening Mechanism in the Smooth Muscle Relaxant Properties of Tamarix Dioica Roxb. Biomolecules 9 (11). doi:10.3390/biom9110722
Islam, S., Shajib, M. S., and Ahmed, T. (2016). Antinociceptive Effect of Methanol Extract of Celosia Cristata Linn. In Mice. BMC Complement. Altern. Med. 16 (1), 400. doi:10.1186/s12906-016-1393-5
Joseph, A., Thuy, T. T. T., Thanh, L. T., and Okada, M. (2018). Antidepressive and Anxiolytic Effects of Ostruthin, a TREK-1 Channel Activator. PLoS One 13 (8), e0201092. doi:10.1371/journal.pone.0201092
Kawano, T., Zoga, V., Gemes, G., McCallum, J. B., Wu, H. E., Pravdic, D., et al. (2009a). Suppressed Ca2+/CaM/CaMKII-dependent K(ATP) Channel Activity in Primary Afferent Neurons Mediates Hyperalgesia after Axotomy. Proc. Natl. Acad. Sci. U S A. 106 (21), 8725–8730. doi:10.1073/pnas.0901815106
Kawano, T., Zoga, V., Kimura, M., Liang, M.-Y., Wu, H.-E., Gemes, G., et al. (2009b). Nitric Oxide Activates ATP-Sensitive Potassium Channels in Mammalian Sensory Neurons: Action by Direct S-Nitrosylation. Mol. Pain 5, 1744–8069. doi:10.1186/1744-8069-5-12
Khalid, M. H., Akhtar, M. N., Mohamad, A. S., Perimal, E. K., Akira, A., Israf, D. A., et al. (2011). Antinociceptive Effect of the Essential Oil of Zingiber Zerumbet in Mice: Possible Mechanisms. J. Ethnopharmacol 137 (1), 345–351. doi:10.1016/j.jep.2011.05.043
Koh, S. D., Bradley, K. K., Rae, M. G., Keef, K. D., Horowitz, B., and Sanders, K. M. (1998). Basal Activation of ATP-Sensitive Potassium Channels in Murine Colonic Smooth Muscle Cell. Biophys. J. 75 (4), 1793–1800. doi:10.1016/S0006-3495(98)77621-0
Kshatri, A. S., Gonzalez-Hernandez, A., and Giraldez, T. (2018). Physiological Roles and Therapeutic Potential of Ca2+ Activated Potassium Channels in the Nervous System. Front. Mol. Neurosci. 11, 258. doi:10.3389/fnmol.2018.00258
Li, G. R., Wang, H. B., Qin, G. W., Jin, M. W., Tang, Q., Sun, H. Y., et al. (2008). Acacetin, a Natural Flavone, Selectively Inhibits Human Atrial Repolarization Potassium Currents and Prevents Atrial Fibrillation in Dogs. Circulation 117 (19), 2449–2457. doi:10.1161/CIRCULATIONAHA.108.769554
Li, T., Zhang, Y., Tian, J., Yang, L., and Wang, J. (2019). Ginkgo Biloba Pretreatment Attenuates Myocardial Ischemia-Reperfusion Injury via mitoBKCa. Am. J. Chin. Med. 47 (5), 1057–1073. doi:10.1142/S0192415X1950054X
Li, Y., Aziz, Q., and Tinker, A. (2021). The Pharmacology of ATP-Sensitive K+ Channels (KATP). Handb Exp. Pharmacol. 267, 357–378. doi:10.1007/164_2021_466
Liu, N., Wu, J., Chen, Y., and Zhao, J. (2020b). Channels that Cooperate with TRPV4 in the Brain. J. Mol. Neurosci. 70 (11), 1812–1820. doi:10.1007/s12031-020-01574-z
Liu, N., Yan, F., Ma, Q., and Zhao, J. (2020a). Modulation of TRPV4 and BKCa for Treatment of Brain Diseases. Bioorg. Med. Chem. 28 (16), 115609. doi:10.1016/j.bmc.2020.115609
Liu, Y., Gao, W. D., O'Rourke, B., and Marban, E. (1996). Synergistic Modulation of ATP-Sensitive K+ Currents by Protein Kinase C and Adenosine. Implications for Ischemic Preconditioning. Circ. Res. 78 (3), 443–454. doi:10.1161/01.res.78.3.443
Mathie, A., Veale, E. L., Golluscio, A., Holden, R. G., and Walsh, Y. (2021). Pharmacological Approaches to Studying Potassium Channels. Handb Exp. Pharmacol. 267, 83–111. doi:10.1007/164_2021_502
Matsuda, N. M., and Miller, S. M. (2010). Non-adrenergic Non-cholinergic Inhibition of Gastrointestinal Smooth Muscle and its Intracellular Mechanism(s). Fundam. Clin. Pharmacol. 24 (3), 261–268. doi:10.1111/j.1472-8206.2009.00761.x
Menozzi, A., Pozzoli, C., Poli, E., Passeri, B., Gianelli, P., and Bertini, S. (2011). Diazoxide Attenuates Indomethacin-Induced Small Intestinal Damage in the Rat. Eur. J. Pharmacol. 650 (1), 378–383. doi:10.1016/j.ejphar.2010.09.078
Methley, A. M., Campbell, S., Chew-Graham, C., McNally, R., and Cheraghi-Sohi, S. (2014). PICO, PICOS and SPIDER: a Comparison Study of Specificity and Sensitivity in Three Search Tools for Qualitative Systematic Reviews. BMC Health Serv. Res. 14, 579. doi:10.1186/s12913-014-0579-0
Miao, Q., Wang, S., Miao, S., Wang, J., Xie, Y., and Yang, Q. (2011). Cardioprotective Effect of Polydatin against Ischemia/reperfusion Injury: Roles of Protein Kinase C and Mito K(ATP) Activation. Phytomedicine 19 (1), 8–12. doi:10.1016/j.phymed.2011.06.023
Modzelewska, B., Drygalski, K., Kleszczewski, T., Chomentowski, A., Koryciński, K., Kiełczewska, A., et al. (2021). Quercetin Relaxes Human Gastric Smooth Muscles Directly through ATP-Sensitive Potassium Channels and Not Depending on the Nitric Oxide Pathway. Neurogastroenterol Motil. 33 (7), e14093. doi:10.1111/nmo.14093
Murata, M., Akao, M., O'Rourke, B., and Marbán, E. (2001). Mitochondrial ATP-Sensitive Potassium Channels Attenuate Matrix Ca(2+) Overload during Simulated Ischemia and Reperfusion: Possible Mechanism of Cardioprotection. Circ. Res. 89 (10), 891–898. doi:10.1161/hh2201.100205
Page, M. J., McKenzie, J. E., Bossuyt, P. M., Boutron, I., Hoffmann, T. C., Mulrow, C. D., et al. (2021). The PRISMA 2020 Statement: an Updated Guideline for Reporting Systematic Reviews. BMJ 372, n71. doi:10.1136/bmj.n71
Paggio, A., Checchetto, V., Campo, A., Menabò, R., Di Marco, G., Di Lisa, F., et al. (2019). Identification of an ATP-Sensitive Potassium Channel in Mitochondria. Nature 572 (7771), 609–613. doi:10.1038/s41586-019-1498-3
Perricone, A. J., and Vander Heide, R. S. (2014). Novel Therapeutic Strategies for Ischemic Heart Disease. Pharmacol. Res. 89, 36–45. doi:10.1016/j.phrs.2014.08.004
Peskar, B. M., Ehrlich, K., and Peskar, B. A. (2002). Role of ATP-Sensitive Potassium Channels in Prostaglandin-Mediated Gastroprotection in the Rat. J. Pharmacol. Exp. Ther. 301 (3), 969–974. doi:10.1124/jpet.301.3.969
Rustenbeck, I., Schulze, T., Morsi, M., Alshafei, M., and Panten, U. (2021). What Is the Metabolic Amplification of Insulin Secretion and Is it (Still) Relevant? Metabolites 11 (6). doi:10.3390/metabo11060355
Serafim, C., Araruna, M. E., Júnior, E. A., Diniz, M., Hiruma-Lima, C., and Batista, L. (2020). A Review of the Role of Flavonoids in Peptic Ulcer (2010-2020). Molecules 25 (22). doi:10.3390/molecules25225431
Shajib, M. S., Rashid, R. B., Ming, L. C., Islam, S., Sarker, M. M. R., Nahar, L., et al. (2018). Polymethoxyflavones from Nicotiana Plumbaginifolia (Solanaceae) Exert Antinociceptive and Neuropharmacological Effects in Mice. Front. Pharmacol. 9, 85. doi:10.3389/fphar.2018.00085
Singh, S., Agarwal, P., and Ravichandiran, V. (2021). Two-Pore Domain Potassium Channel in Neurological Disorders. J. Membr. Biol. 254 (4), 367–380. doi:10.1007/s00232-021-00189-8
Sousa, A. M., and Prado, W. A. (2001). The Dual Effect of a Nitric Oxide Donor in Nociception. Brain Res. 897 (1-2), 9–19. doi:10.1016/s0006-8993(01)01995-3
Staurengo-Ferrari, L., Zarpelon, A. C., Longhi-Balbinot, D. T., Marchesi, M., Cunha, T. M., Alves-Filho, J. C., et al. (2014). Nitroxyl Inhibits Overt Pain-like Behavior in Mice: Role of cGMP/PKG/ATP-sensitive Potassium Channel Signaling Pathway. Pharmacol. Rep. 66 (4), 691–698. doi:10.1016/j.pharep.2014.04.003
Su, C. F., Jiang, L., Zhang, X. W., Iyaswamy, A., and Li, M. (2021). Resveratrol in Rodent Models of Parkinson's Disease: A Systematic Review of Experimental Studies. Front. Pharmacol. 12, 644219. doi:10.3389/fphar.2021.644219
Tano, J. Y., and Gollasch, M. (2014). Calcium-activated Potassium Channels in Ischemia Reperfusion: a Brief Update. Front. Physiol. 5, 381. doi:10.3389/fphys.2014.00381
Taura, J., Kircher, D. M., Gameiro-Ros, I., and Slesinger, P. A. (2021). Comparison of K+ Channel Families. Handb Exp. Pharmacol. 267, 1–49. doi:10.1007/164_2021_460
Tian, C., Zhu, R., Zhu, L., Qiu, T., Cao, Z., and Kang, T. (2014). Potassium Channels: Structures, Diseases, and Modulators. Chem. Biol. Drug Des. 83 (1), 1–26. doi:10.1111/cbdd.12237
Tinker, A., Aziz, Q., Li, Y., and Specterman, M. (2018). ATP-sensitive Potassium Channels and Their Physiological and Pathophysiological Roles. Compr. Physiol. 8 (4), 1463–1511. doi:10.1002/cphy.c170048
Tirloni, C. A. S., Lívero, F. A. D. R., Palozi, R. A. C., Silveira, R. C. A., Vasconcelos, P. C. P., Souza, R. I. C., et al. (2017). Ethnopharmacological Investigations of the Cardio-Renal Properties of a Native Species from the Region of Pantanal, State of Mato Grosso Do Sul, Brazil. J. Ethnopharmacol 206, 125–134. doi:10.1016/j.jep.2017.05.027
Weaver, C. D., and Denton, J. S. (2021). Next-generation Inward Rectifier Potassium Channel Modulators: Discovery and Molecular Pharmacology. Am. J. Physiol. Cel Physiol 320 (6), C1125–C1140. doi:10.1152/ajpcell.00548.2020
Wei, W., Ehlerding, E. B., Lan, X., Luo, Q. Y., and Cai, W. (2019). Molecular Imaging of β-cells: Diabetes and beyond. Adv. Drug Deliv. Rev. 139, 16–31. doi:10.1016/j.addr.2018.06.022
Wu, C., Li, Y., Chen, Y., Lao, X., Sheng, L., Dai, R., et al. (2011). Hypoglycemic Effect of Belamcanda Chinensis Leaf Extract in normal and STZ-Induced Diabetic Rats and its Potential Active Faction. Phytomedicine 18 (4), 292–297. doi:10.1016/j.phymed.2010.07.005
Zakaria, Z. A., Mohd Sani, M. H., Cheema, M. S., Kader, A. A., Kek, T. L., and Salleh, M. Z. (2014). Antinociceptive Activity of Methanolic Extract of Muntingia calabura Leaves: Further Elucidation of the Possible Mechanisms. BMC Complement. Altern. Med. 14, 63. doi:10.1186/1472-6882-14-63
Zambrana, S., Lundqvist, L. C. E., Mamani, O., Catrina, S. B., Gonzales, E., and Östenson, C. G. (2018). Lupinus Mutabilis Extract Exerts an Anti-diabetic Effect by Improving Insulin Release in Type 2 Diabetic Goto-Kakizaki Rats. Nutrients 10 (7). doi:10.3390/nu10070933
Keywords: medicinal plants, phytochemicals, potassium channels, nociception, ischemia, diabetes
Citation: Rajabian A, Rajabian F, Babaei F, Mirzababaei M, Nassiri-Asl M and Hosseinzadeh H (2022) Interaction of Medicinal Plants and Their Active Constituents With Potassium Ion Channels: A Systematic Review. Front. Pharmacol. 13:831963. doi: 10.3389/fphar.2022.831963
Received: 09 December 2021; Accepted: 31 January 2022;
Published: 22 February 2022.
Edited by:
Murat Oz, Health Science Center, KuwaitReviewed by:
Devasena Ponnalagu, The Ohio State University, United StatesElena Grossini, University of Eastern Piedmont, Italy
Copyright © 2022 Rajabian, Rajabian, Babaei, Mirzababaei, Nassiri-Asl and Hosseinzadeh. This is an open-access article distributed under the terms of the Creative Commons Attribution License (CC BY). The use, distribution or reproduction in other forums is permitted, provided the original author(s) and the copyright owner(s) are credited and that the original publication in this journal is cited, in accordance with accepted academic practice. No use, distribution or reproduction is permitted which does not comply with these terms.
*Correspondence: Hossein Hosseinzadeh, aG9zc2VpbnphZGVoaEBtdW1zLmFjLmly; Marjan Nassiri-Asl, bWFyamFubmFzc2lyaWFzbEBzYm11LmFjLmly