- 1Center of Molecular Biology and Pharmacogenetics, Scientific and Technological Bioresource Nucleus, Universidad de La Frontera, Temuco, Chile
- 2School of Medical Sciences, University of Campinas, Campinas, Brazil
- 3Faculty of Pharmaceutical Sciences, University of Campinas, Campinas, Brazil
Cisplatin (DDP) is a well-known anticancer drug used for the treatment of numerous human cancers in solid organs, including bladder, breast, cervical, head and neck squamous cell, ovarian, among others. Its most important mode of action is the DNA-platinum adducts formation, inducing DNA damage response, silencing or activating several genes to induce apoptosis; these mechanisms result in genetics and epigenetics modifications. The ability of DDP to induce tumor cell death is often challenged by the presence of anti-apoptotic regulators, leading to chemoresistance, wherein many patients who have or will develop DDP-resistance. Cancer cells resist the apoptotic effect of chemotherapy, being a problem that severely restricts the successful results of treatment for many human cancers. In the last 30 years, researchers have discovered there are several types of RNAs, and among the most important are non-coding RNAs (ncRNAs), a class of RNAs that are not involved in protein production, but they are implicated in gene expression regulation, and representing the 98% of the human genome non-translated. Some ncRNAs of great interest are long ncRNAs, circular RNAs, and microRNAs (miRs). Accumulating studies reveal that aberrant miRs expression can affect the development of chemotherapy drug resistance, by modulating the expression of relevant target proteins. Thus, identifying molecular mechanisms underlying chemoresistance development is fundamental for setting strategies to improve the prognosis of patients with different types of cancer. Therefore, this review aimed to identify and summarize miRs that modulate chemoresistance in DDP-resistant in the top five deadliest cancer, both in vitro and in vivo human models.
1 Introduction
Globally, cancer is the first leading cause of death. In 2020, 19.3 million new cases of cancer and almost 10 million deaths from cancer (Ferlay et al., 2021; Sung et al., 2021). Cisplatin [cis-diamminedichloroplatinum (II), DDP], discovered by Rosenberg and his colleagues in 1965 (Rosenberg et al., 1969), was the first platinum compound approved by FDA for cancer treatment in the United States in 1978 (FDA, 1978). It is a well-known chemotherapeutic drug used for the treatment of numerous human cancer in solid organs, including head and neck, testis, small cells and non-small cells lung cancer, ovarian, cervical, and bladder. Once DDP crosses the cytosol, the low concentration of chloride present triggers two hydrolyses of the DDP, forming positively charged DDP derivative, which binds to negatively charged DNA bases, inducing DNA damage by forming DNA-platinum adducts, and simultaneously initiating self-defense mechanisms to activate or silence multiple genes, resulting in DNA damage response and repair pathways (Hu et al., 2016), cell cycle arrest (Velma et al., 2016) and DDP-induced apoptosis (Tanida et al., 2012). However, treatment response to DDP differs, and the main problem to its effectiveness is the development of drug resistance (Amable, 2016; Ko and Li, 2019). Cisplatin-resistance is inferred mainly when the usual clinical dose of DDP is magnified in drug-intensive therapy protocols and may require cytotoxic concentrations as much as 50–100-fold in addition to those needed for sensitive cells (Siddik, 1999). In fact, any factor that influences those processes can lead to the development of resistance to DDP. Moreover, drug resistance is responsible for over 90% of deaths in cancer patients receiving traditional chemotherapeutic drugs (Bukowski et al., 2020). Besides, the epithelial-mesenchymal transition (EMT) process contributes to chemoresistance by transforming epithelial cells into mesenchymal cells and altering cell-cell adhesion as well as the cellular extracellular matrix, leading to invasion of tumor cells (Ashrafizadeh et al., 2020). Autophagy, a process which degrades and recycles cellular proteins and organelles in response to cellular stresses, has been shown to attenuate the sensitivity of therapeutic drugs, protecting cancerous cells from death (Li W. et al., 2019). Thus, there is a crucial necessity to comprehend the underlying molecular mechanisms and recognize strategies to counteract DDP and facilitate predictions of the clinical response to therapy.
Non-coding RNAs are molecules that regulate gene expression under physiological and pathological conditions (Virginie et al., 2019) and are further divided into two principal groups, small ncRNAs (shorter than 200 bp) and long ncRNA (longer than 200 bp). MicroRNAs a class of small ncRNAs, are a kind of short-chain, linear, approximately 21–25 nucleotides long that negatively regulate gene targets the post-transcriptional level by perfect complementarity of their “seed” region to 3′-UTR of its target mRNA, inducing their degradation. If there is a mismatch or imperfect complementarity, it results in translational repression (Guo et al., 2019). The latest release of the miRbase database (v22) contains 2654 human mature miRs sequences (Kozomara et al., 2019), which confirms their importance on gene expression regulation. Not surprisingly, atypical expression and/or activity of ncRNAs can affect the outcome of cancer treatment and allow tumors to acquire drug-resistant phenotypes (Yo et al., 2012; Tang et al., 2020). An increasing number of studies have shown that ncRNAs play an essential role in several types of cancer and miRs have been associated with DDP resistance, making them important potential therapeutic targets. So, in this narrative review, we summarize the current literature on the contribution of miRs that modulate chemoresistance to DDP in the top five deadliest cancer reported in 2020, some strategies to sensitize DDP-cells and reduce their malignant capacities, both in vitro and in vivo human models.
2 The Top Five Deadliest Cancer
The most common cause of cancer death for about 13% of total cancer diagnoses remains by far lung cancer (Ferlay et al., 2021; Sung et al., 2021). The global incidence of lung cancer estimated in 2020 was approximately 2206800 new cases and 1796100 cancer deaths (Figure 1). In terms of clinical and tumor genetics, lung cancer can be divided into small and non-small cell lung cancer. Non-small cell lung cancer (NSCLC) represents about 80%–95% of all diagnosed lung cancer cases, and NSCLC remains the leading cause of cancer death worldwide. The efficacy of DDP-based chemotherapy in cancer is limited by the occurrence of innate and acquired drug resistance and acquired resistance of NSCLC cells against cisplatin is the consequence of altered signaling leading to reduced G2/M cell cycle arrest and apoptosis (Sarin et al., 2017). On the other hand, small-cell lung cancer (SCLC) is a distinct form of lung cancer with unique clinical and histological characteristics, representing 10%–15% of all new cases of lung cancer, and SCLC cancer tends to grow and spread faster than NSCLC (Kitamura et al., 2009). SCLC is highly sensitive to the initial cycle of chemotherapy and, in many cases chemotherapy-resistant SCLC emerges, leading to rapid patient mortality. DDP-resistance in lung cancer can be induced by alterations to a huge number of intracellular pathways, where miRNAs play a vital role (Table 1), even though very few studies have demonstrated the role of miR on DDP-resistance in SCLC.
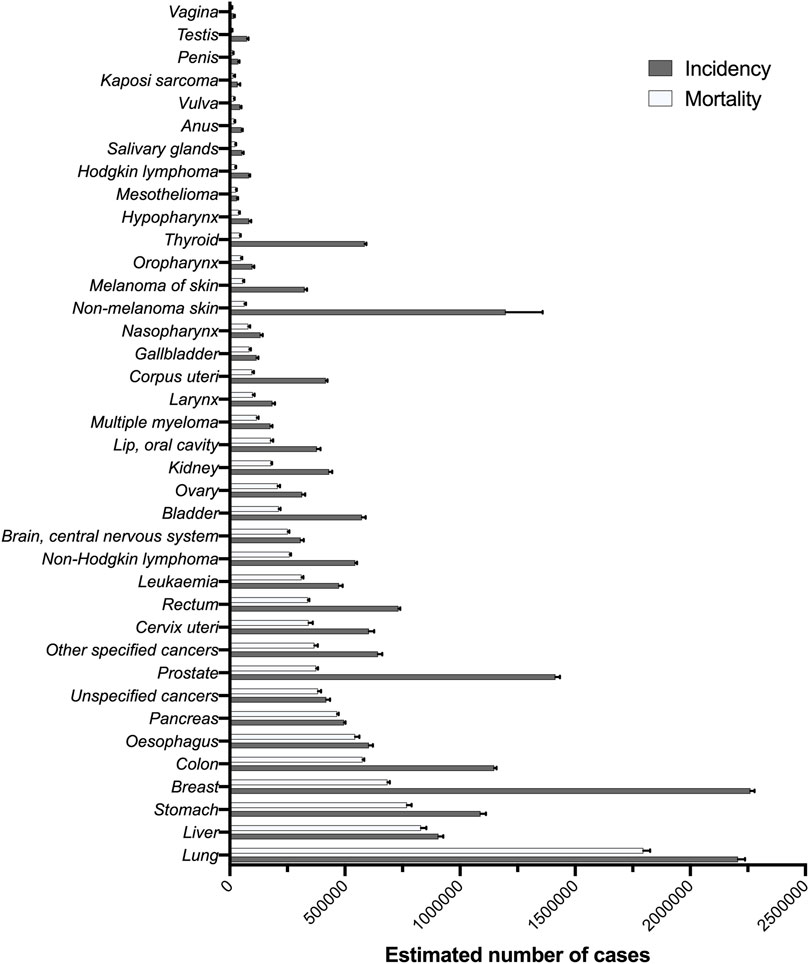
FIGURE 1. Estimated number of new cancer cases and cancer deaths worldwide for 2020 (Ferlay et al., 2021; Sung et al., 2021).
Followed by lung cancer, the second cause of cancer death is due to liver cancer (Ferlay et al., 2021; Sung et al., 2021). Liver cancer comprises a heterogeneous group of malignant liver tumors with different histological features and an unfavorable prognosis (Anwanwan et al., 2020). The global incidence of liver cancer estimated in 2020 was approximately 905700 new cases and 830200 cancer deaths (Figure 1). The prognosis for liver cancer is poor, due to merely 5%–15% of patients are eligible for surgical removal, because of diminished hepatic regenerative capacity (Anwanwan et al., 2020). Treatment options for more advanced stages include chemotherapy, however, fewer than one-third of patients benefit from the treatment, and drug resistance is evident within 6 months of initiating the regimen (El-Serag et al., 2008). On this basis, miRs have been involved in DDP-resistance in lung cancer and will be listed in Table 2.
Followed by liver cancer, the third cause of cancer death is due to stomach cancer (Ferlay et al., 2021; Sung et al., 2021). Gastric cancer is one of the most commonly diagnosed malignancies. The global incidence of stomach cancer estimated in 2020 was approximately 1089100 new cases and 768800 cancer deaths (Figure 1). In recent years, a rising body of evidence has revealed that miRs are dysregulated in almost all types of tumors, including gastric, modulating the proliferation, stemness, tumor immune escape, invasion, angiogenesis, and drug resistance of tumor cells (Chen et al., 2021). Some studies in which miRs play a major role in mediating DDP-resistance in stomach cancer will be detailed in Table 3.
After stomach cancer, the fourth cause of cancer mortality is due to breast cancer (Ferlay et al., 2021; Sung et al., 2021). Breast cancer is the most commonly diagnosed cancer worldwide and female breast cancer is the most commonly diagnosed cancer (Sung et al., 2021). The global incidence estimated in 2020 was approximately 2261400 new cases and 685000 cancer deaths due to breast cancer (Figure 1). Cisplatin is currently the most effective drug used to treat breast cancer; however, DDP-resistance presents a major challenge in the successful treatment of breast cancer. Breast cancer can be invasive or non-invasive. Invasive breast cancer is cancer that spreads into adjacent tissues and/or distant organs, while non-invasive breast cancer does not go beyond the milk ducts or lobules in the breast (Beikman et al., 2013). Some studies in which miRs play a major role in mediating DDP-resistance in breast cancer will be detailed on Table 4.
The last cause of cancer mortality is due to colorectal cancer. Colorectal cancer starts when normal cells in the lining of the colon or rectum change and grow out of control, forming a mass called a tumor (Weitz et al., 2005). The global incidence of colon and rectum cancer estimated in 2020 was approximately 1880700 new cases and 915900 cancer deaths (Figure 1). The relative survival rate for colorectal cancer is 64% at 5-year following diagnosis and 58% at 10 years (Siegel et al., 2020). This can be determined by resistance to DDP, which may compromise the efficacy of chemotherapy, and some miRs related are described in Table 5.
3 MicroRNAs Involved in DDP-Chemoresistance
3.1 MicroRNAs Involved in Cell Cycle
The cell cycle is a highly and complex mechanism that ensures complete and accurate cell division, and it is driven by several proteins named CDKs, which in turn are positively regulated by cyclins (A-E) and negatively regulated by CDKIs (1-9) (Sherr and Roberts, 1999); and also by MDMs (Flores and Sobrevia, 2000). Cells can acquire drug resistance due to a relative insensitivity to a chemotherapeutic agent because of the position of the cells in the cell cycle (Shah and Schwartz, 2001). Mechanistically, some miRs can target multiple proteins involved in cell cycle progression. Cisplatin-resistant colorectal cancer cell lines, HCT 116/DDP and LoVo/DDP, have been shown to express low levels of miR-526-3p, compared with parental cells, and silencing of miR-526b-3p increases cyclin D1 expression and reduced cell cycle arrest, promoting thus DDP-resistance in colorectal cancer cells enhances (Zhang et al., 2021). Mechanistically, miR-526-3p targeted KLF12. This is important, due to KLF12 is able to regulate cell death, by promoting the cell cycle transition through S phase and therefore cell proliferation and reduced expression levels of KLF12 results in increased ability of lung cancer cells to form tumors in vivo (Godin-Heymann et al., 2016). Another miRs involved in cell cycle is miR-25. High levels of miR-25 are found in several lung cancer cells (Zhao et al., 2014). Also, tumor-derived cells express elevated cyclin E2 levels, which accelerated cell cycle in G1 stage (Gudas et al., 1999). However, downregulation of miR-25 is able to induce cell cycle arrest by reducing CDK2 and cyclin E2 expression in lung cancer cells, thus sensitizing cells to DDP (Zhao et al., 2014). Roundabout guidance receptor 1 (ROBO1), a cancer-promoting oncogene, has been negatively correlated with the prognosis of patients, due to ROBO1 promotes the genesis and progression of cancer metastasis (Li et al., 2013). On this basis, miR-32 and miR-548a have proved to target the 3′-UTR sequence of ROBO1, promoting ROBO1 expression and activating the Wnt/β-catenin pathway. In addition, lung cancer cells exhibit low miR-32 and miR-548a levels, leading to an enhanced ROBO1 expression and displaying a DDP-resistant phenotype in A-549 cells (Zheng J. et al., 2021). In the same way, tankyrase 1 and 2 (TNKS1/2) are regulators of Wnt signaling by controlling the activity of the β-catenin destruction complex by reducing G1 cell cycle arrest and senescence (Foronda et al., 2019). Mechanistically, TNKS2 is targeted by miR-490-3p, and its increased expression promoted the chemoresistance of colorectal cancer cells (Li J. et al., 2021).
Likewise, levels of miR-103 are upregulated hepatocellular carcinoma cells (Luo et al., 2019), while miR-200a is reduced in DDP-resistant lung cancer cells (Tang et al., 2020). Also, NOR1 was targeted by miR-103 (Luo et al., 2019). It has been demonstrated that NOR1, a tumor suppressor gene, is downregulated in NPC cells and NOR1 that enhances cancer stem-like cell properties in tumor cells by enhancing the Akt and Wnt/β-catenin pathways (Wang et al., 2017). Additionally, miR-200a targeted β-catenin, regulating negatively its expression and its downstream molecules cyclin D1 and vimentin (Tang et al., 2020). Furthermore, cyclin D1 is also directly targeted by miR-593-5p in colorectal cancer cells (Qu et al., 2020) and by miR-1296 in breast cancer cells (Albakr et al., 2021). Cyclin D1 levels must be high during G1 phase for a cell to begin DNA synthesis, but then must be reduced to low levels during S phase to allow for efficient DNA synthesis, however, an aberrant cyclin D1 activity is observed in tumor cells (Montalto and De Amicis, 2020). Additionally, enhanced cyclin D1 and surviving expression enhance resistance by reducing G1 phase arrest and apoptosis, downregulating REV3L expression and leading to enhanced cell proliferation and invasive capacity (Zhu et al., 2016). Moreover, REV3L was targeted by miR-29a and miR-4458 and high expression was observed in tumoral tissues due to a decreased expression in lung cancer cells (Chen X. et al., 2019; Pang et al., 2020). However, overexpression of miR-29a could reduce viability and proliferation and enhance DDP-induced apoptosis of A-549/DDP cells treated with 5 μg/ml DDP (Chen X. et al., 2019).
Expression of miR-203 is enhanced in breast cancer cells, and, mechanistically, miR-203 targeted SOCS3, enhancing DDP-resistance (Ru et al., 2011). However, silencing of miR-203 sensitized breast cancer cells, and it was observed that those cells displayed a higher level of p21, associating these changes with decreased chemoresistance (Ru et al., 2011). This is important, due to p21 being a type of cell cycle regulator that plays a dual role in tumor cells, regulating the cell cycle, inducing apoptosis, and inhibiting cell proliferation (Wang L. et al., 2021). MDMs are nuclear factors that regulate the cell cycle at the G1/S phase transition, whose function and expression are altered in various types of human neoplasms (Momand et al., 1998). Degradation of p21 could be mediated by MDM4, in cooperation with MDM2, leading to abrogation of G1 cell cycle arrest (Jin et al., 2008). It has been reported that miR-1307 and miR-127-3p are downregulated in DDP-resistant breast cancer and lung cancer cell, respectively, and, mechanistically, they directly targeted MDM4 and MDM2, promoting DDP-resistance (Wang and Zhu, 2018; Zeng et al., 2020).
3.2 MicroRNAs involved in Autophagy
Autophagy is an intracellular self-digesting process for the regulation of cell homeostasis, that occurs under several stressful conditions, including organelle damage, the presence of abnormal proteins, and nutrient deprivation (Yun and Lee, 2018). In addition, autophagy regulates the properties of cancer stem-cells by contributing to the maintenance of stemness and the development of resistance to anticancer reagents (Yun and Lee, 2018). MiRs are involved in DDP response of tumor cells by regulation of autophagy.
A key initial event in autophagy is the formation of the autophagosome, and this step is mediated by the serine/threonine protein kinase ULK1 (Zachari and Ganley, 2017). Mechanistically, ULK1 is targeted by miR-514a-3p in NSCLC cells (Shen Q. et al., 2020). Moreover, miR-514a-3p was markedly downregulated in lung tissues and cells, and autophagy was found to be promoted (Shen Q. et al., 2020).
Autophagy-related (ATG) genes are indispensable for autophagosome formation, and enhanced autophagy and proliferation, and reduced apoptosis have been related to enhanced ATGs expression in cancer cells (Wang Q. et al., 2021). In this context, miR-17, miR-138-5p and miR-1236-3p enhances autophagy activity in lung cancer cells via ATG7 targeting (Huang FX. et al., 2019; Pan et al., 2019; Wang et al., 2020c). In addition, miR-4486 also enhances autophagy by targeting ATG7 in colorectal carcinoma cells (Wang W. et al., 2021). ATG3 is another key gene involved in autophagy and it is targeted by miR-1 in NSCLC cells (Hua et al., 2018). It has been observed that there was significant miR-1, miR-17, miR-138-5p and miR-1236-3p downregulation in NSCLC cells (Hua et al., 2018; Huang FX. et al., 2019; Pan et al., 2019; Wang et al., 2020c). Likewise, miR-4486 was also decreased in colorectal cancer cells (Wang W. et al., 2021). Moreover, miR-4443 is also upregulated in lung cancer cells. Besides, METTL3 was confirmed as a direct target gene of miR-4443 (Song et al., 2021). METTL3, a m6A methyltransferase, is able to regulate autophagy by increasing the critical genes, such as ATG5 and ATG7 (Liu S. et al., 2020). In this way, enhanced ATG7 levels promote the conversion of LC3-I into LC3-II and improve Beclin-1 expression, supporting autophagy and chemoresistance of lung cancer (Huang FX. et al., 2019; Wang et al., 2020c) and colorectal cancer cells (Wang W. et al., 2021). Beclin-1 also plays an important role in autophagy-induced tumorigenesis and drug resistance, altering cell growth, cellular microenvironment and cell division (Usman et al., 2021). Beclin-1 has been reported to be targeted by miR-30a in liver cancer cells (Zou et al., 2012) and by miR-216b in lung cancer cells (Chen L. et al., 2019), and both miRs are downregulated in both cancer types, suggesting their role in autophagy activity.
Moreover, miR-99a-5p was found to be upregulated in DDP-resistant gastric cancer cells (Sun G. et al., 2020). Mechanistically, miR-99a-5p targeted MTMR3 and enhanced MTMR was confirmed to induce autophagic activity (Taguchi-Atarashi et al., 2010), promoting resistance to chemotherapy in tumors.
3.3 MicroRNAs Involved in Epithelial-to-Mesenchymal Transition
The initiation of metastasis involves an increase in cell motility mediated by loss of cell-cell adhesion, caused by E-cadherin repression and augmented N-cadherin expression, in a process commonly known as epithelial-to-mesenchymal transition (EMT) (Taylor et al., 2010). In this way, high invasive potential, decreased E-cadherin expression and increased DDP-resistance has been founded in lung NCI-H1299, H596 and NCI-H522 cancer cells, due to a reduced miR-200c expression (Ceppi et al., 2010), and in liver Hep-G2 and Huh-7 cancer cells, also due to a decreased miR-31-5p expression (Chen et al., 2020).
A molecule implicated in the EMT process is polycomb ring finger (BMI1). Enhanced BMI1 expression, a known proto-oncogene, promoted EMT, augmented stemness and rendered cell drug resistance (Paranjape et al., 2014). On this basis, it has been reported that miR-802 expression is downregulated in DDP-resistant gastric cancer tissues and cells. Mechanistically, miR-802 directly targeted BMI1 and their boosted levels in gastric cancer cells promote EMT process (Liu et al., 2020d).
Zinc finger E-box binding homeobox 1 and 2 (ZEB1 and ZEB2) are transcription factors that promote tumor invasion and metastasis by inducing EMT in carcinoma cells (Zhang P. et al., 2015; DaSilva-Arnold et al., 2019). Also, ZEB1 has been founded to be targeted by miR-574-3p (Wang M. et al., 2019), while ZEB2 is targeted by miR-200c in gastric cancer cells (Jiang T. et al., 2017) and their upregulation contributed to DDP-resistance. Both miRs were founded to be downregulated in SGC-7901/DDP cells (Jiang T. et al., 2017; Wang M. et al., 2019). Even more, miR-223, miR-363 and miR-500a-3p directly targeted F-box and WD repeat domain containing 7 (FBXW7) and promote DDP-resistance in gastric cancer cells (Zhang et al., 2016; Wang et al., 2020b; Lin et al., 2020). FBXW7 (also known as FBW7) directly binds and degrades the EMT-inducing transcription factor ZEB2 in a phosphorylation-dependent manner and its loss can induce an EMT phenotype (Li N. et al., 2019). However, since miR-363 and miR-500a-3p are upregulated in gastric cancer MGC-803 and HGC-27 cells, and miR-223 in lung cancer A-549, NCI-H358 and NCI-H1299 cells, those cell lines display an EMT phenotype (Zhang et al., 2016; Wang et al., 2020b; Lin et al., 2020).
Another molecule that participated in the EMT process is doublecortin-like kinase 1 (DCLK1), a cancer stem cell marker. DCLK1 is functionally involved in maintaining cancer stemness and the process of EMT (Chandrakesan et al., 2016). Also, DCLK1 has been found to be targeted by miR-330-5p and its upregulation contributes to DDP-resistance. Likewise, miR-330-5p was found to be downregulated in lung cancer A-549 and NCI-H1299 resistant cells, promoting DDP-resistance in lung cancer cells (Ge et al., 2021).
Melanoma-associated antigen A3 (MAGEA3) enhances migration, invasion and proliferation by activation of EMT and Wnt signaling pathway in HeLa cells (Gao et al., 2020). In the same way, enhanced expression of MAGEA3 was found in drug-resistant cells (Bertram et al., 1998) and knockdown of MAGEA3 expression caused a reduction in proliferation and colony formation ability (Xie et al., 2016). Mechanistically, MAGEA3 is targeted by miR-31-5p, and its upregulation is associated with DDP-resistance. Likewise, miR-31-5p was founded to be downregulated in liver Hep-G2 and Huh-7 cancer cells, thus promoting DDP-resistance (Chen et al., 2020).
Collagen 1A1 (COL1A1) has been highly expressed and associated with poor prognosis in multiple cancers and positively correlated with the abundance of CAFs, macrophages, and tumor-infiltrating lymphocytes, and activation of EMT process (Liu et al., 2021). Also, miR-29b-3p directly target COL1A1 to promote DDP-resistance. Parallel that, it has been reported miR-29a-3p expression was reduced in lung A-549 cancer resistant cells, and augmented COL1A1 levels are associated with DDP-resistance (Jia and Wang, 2020).
Six homeobox 1 (SIX1) and Notch receptor 2 (NOTCH2) protein expressions have been associated with invasive lung cancer, by inducing EMT and thus promoting advanced malignant phenotypes (Mimae et al., 2012). Expression of miR-186-5p was downregulated lung A-549/DDP and NCI-H1299 resistant cancer cells. In addition, miR-186-5p negatively regulated SIX1 and SIX1 was upregulated in DDP resistant cancer cells (Liu X. et al., 2020).
SRY-related high mobility group-box 9 (SOX9) is a transcription factor, which acts as a proto-oncogene, implicated with the Wnt/β-catenin pathway activation and in the expression of EMT-associated proteins (Huang JQ. et al., 2019; Panda et al., 2021). Mechanistically, SOX9 is targeted by miR-487a-3p in colorectal cancer cells. Additionally, colorectal cancer cells displayed low miR-487a-3p levels, promoting SOX9 expression in colorectal HT29 and SW480 cells, exhibiting the DDP-resistant phenotype (Sun Y. et al., 2020).
Paxillin (PXN) is a cytoplasmatic protein which regulates focal adhesion. Also, PXN has been shown to promote the activation of ERK and enhance the EMT process (Wen et al., 2020). Bioinformatic analysis has proved that PXN is a direct target of miR-199b-5p. Also, decreased miR-199b-5p levels are observed in breast cancer cells, promoting the EMT process by reducing E-cadherin levels (Du et al., 2020). Loss of E-cadherin has been shown to promote the growth, invasion, and enhance drug resistance of CrC cells and, contribute to the progression and metastatic dissemination (Chen et al., 2012).
EIF5A2 plays an important role in many biological processes, including tumor formation, cancer cell growth, maintenance of cancer stem cells and EMT process (Meng et al., 2019). Bao et al. (2020) demonstrated EIF5A2 was targeted by miR-9 and was upregulated in lung tumor cells, thus promoting chemoresistance to DDP by increasing EMT process. Also, Wnt10b has been involved in enhanced tumor cell stemness by upregulation of OCT4 and NANOG expression. In colorectal cancer, WNT10b is directly targeted by miR-148a and the reduced miR-148a expression enhances Wnt10b levels to allow drug resistance in cancer therapy (Shi et al., 2019).
3.4 MicroRNAs Involved in Apoptosis
Apoptosis is a form of programmed cell death. In this pathway, molecular mechanisms which trigger inhibition of apoptosis responsible for DDP-resistance includes MAPK dysregulation, enhanced Bcl-2 or Bcl-XL expression, suppression of caspase-3 activity, enhanced PI3K/Akt activity, and so on (Siddik, 2003). A number of miRs have been described to be involved in the regulation of apoptosis.
Mitogen-activating protein kinases (MAPK) are molecules involved in apoptosis. There are three major MAPK pathways that involve the extracellular signal-regulated kinases: ERK1/2, JNK and p38 kinase. Chen et al., proved that MAPK3 was directly targeted by miR-206 in gastric cancer cells (Chen Z. et al., 2019). Also, Sun T. et al. (2020) demonstrated that miR-325-3p interacted with GITR, and upregulated expression contributes to DDP-resistance in gastric cancer cells. On this basis, GITR is able to enhance ERK phosphorylation, suggesting that GITR is associated whit MAPK-pathway activation (Ronchetti et al., 2004). Also, Rao et al. (2019) showed that miR-219a-5p directly targeted FGF9, and its enhanced expression leads to DDP-resistance in lung cancer cells. In this way, the low miR-325-3p and miR-219a-5p expression observed in gastric and lung cancer cells activate MAPK pathway, contributing to DDP-resistance (Ronchetti et al., 2004; Rao et al., 2019). Also, Zhou and Chen demonstrated that miR-135b interacted with MST1, and upregulated expression activates MAPK pathway, contributing to DDP-resistant phenotype (Zhou and Chen, 2019).
The intrinsic-mediated apoptotic pathway causes mitochondrial membrane potential loss, cytochrome c release and cleaved caspase-3. Bcl-2 is located in the mitochondrion membrane, and is related to the mitochondrial membrane potential loss and the cytochrome c release (Chen et al., 2015). MiR-7, miR-145, miR-146a, miR-152, miR-181b, miR-200b, miR-200c, miR-429, miR-451, miR-497 and miR-630 are reported to target Bcl-2 in lung cancer tissues and/or cells, and negatively regulate its expression (Zhu et al., 2010; Bian et al., 2011; Zhu et al., 2012a; Zhu et al., 2012b; Cheng et al., 2017; Pang et al., 2017; Chen et al., 2018; Zhang et al., 2019; Zhao et al., 2019). Likewise, miR-497 also interacts with Bcl-2 in colorectal cancer cells (Zheng ZH. et al., 2021). In this way, the low miR-7, miR-152, miR-181b, miR-200b, miR-200c, miR-429, miR-497 and miR-630 expression shown leads to decreased apoptosis incidence, resulting in a DDP-resistant phenotype in lung and colorectal cancer cells. Also, increased Bcl-2 levels have been associated with decreased cleaved-caspase 3 and E-cadherin levels, triggering EMT process and promoting DDP-phenotype (Du et al., 2020). The E2F family consists of 8 genes and 10 protein products encoded by these genes, which are crucial for regulating apoptosis, and they have been classified as transcriptional activators (E2F1-3), predicted to be oncogenic, or transcriptional repressors (E2F4-8), predicted to have tumor suppressor functions (Xie et al., 2021). E2Fs have been associated with the upregulation of Bcl-2, which contributes to uncontrolled tumor growth (Donzelli et al., 2012; Zheng et al., 2020; Zhou, 2020; Wu et al., 2021). In this context, miR-432 and miR-503 suppress E2F3 (Chen L. et al., 2016; Zhou, 2020), miR-34c targets E2F1 (Zheng et al., 2020), and miR-128-2 interacts with E2F5 (Donzelli et al., 2012), by targeting their 3′UTR mRNA. The reduced miR-432 and miR-34c expression observed in lung and gastric cancer cells were associated with advanced tumor stage and mortality and allowed E2F1 and E2F3 to be overexpressed in DDP-resistant phenotype (Chen L. et al., 2016; Zheng et al., 2020). Another molecule implicated in apoptosis is ID1. ID1 regulates p53 and NF-κB pathways, regulating Bax and Bcl-2 genes, thus providing a survival advantage under drug treatment (Kim et al., 2008). In this sense, miR-381 directly targeted ID1 and the reduced miR-381 levels observed in lung cancer cells allows an enhanced ID1 expression, reducing apoptosis and triggering a DDP-resistant phenotype (Huang et al., 2018). Finally, JNK2 negatively regulates the activity of genes related to tumor suppression and the induction of cell apoptosis (Chen et al., 2002). Regarding that, JNK2 was identified as a direct target of miR-146a and the low miR-146a levels reduced the apoptosis rate and enhanced the relative invasion rate of lung cancer cells (Pang et al., 2017).
The PI3K-Akt pathway is a major survival pathway activated in cancer. In this sense, phosphatase and tensin homolog (PTEN) is a molecule capable of inactivate the Akt signaling pathway and acts as a negative regulator of PI3K/Akt signaling (Georgescu, 2010). Also, PTEN/PI3K/Akt pathway regulates the signaling of multiple biological processes, such as apoptosis, and also enhances PI3K/Akt/mTOR pathway, conferring drug resistance and further cancer progression in breast cancer cells (Dong et al., 2021). MiR-18a, miR-19a, miR-21, miR-25-3p, miR-130b and miR-1269b in lung cancer cells (Xiao et al., 2018; Zhang et al., 2018; Xing et al., 2019; Yang W. et al., 2020; Gu et al., 2020; Sun B. et al., 2021; Liang et al., 2021), and miR-21 and miR-106a in gastric cancer cells (Fang et al., 2013; Yang et al., 2013; Zheng et al., 2017) directly regulate PTEN, and reduced miRs levels expression in cancer cells promote PTEN expression, triggering apoptosis and DDP-resistance. Additionally, HMGA2 and KLF4 regulation are able to promote PI3K/Akt phosphorylation, resulting in increased drug resistance (Deng et al., 2021), and miR-26a interacts with HMGA2 (Yang et al., 2016) and miR-145 with KLF4 (Cui et al., 2018) to promote DDP-resistance in lung cancer cells. Moreover, MET, a proto-oncogene, also activates PI3K/Akt pathway via promoting PTEN and CDKN1A expression and reducing apoptosis (Ohta et al., 2015). In this way, miR-206 regulates MET protein in A-549 lung cancer cells by directly targeting MET 3′-UTR and activated MET/PI3K/Akt/mTOR signaling pathway to induce DDP resistance (Chen QY. et al., 2016). To contribute to Akt activation and DDP-resistance, PI3K also is targeted by miR-10a (Huang T. et al., 2020). In the same way, two downstream effectors of the PI3K/Akt pathway are also regulated by miRs. Mammalian target of rapamycin (mTOR) acts as a target gene of miR-100-5p in lung cancer (Qin et al., 2017) controlling cell growth, proliferation and survival (Populo et al., 2012). Besides, FOXO3 also is regulated by miR-155 in colorectal cancer cells (Gao et al., 2018) and by miR-372 in gastric cancer cells (Wang C. et al., 2020), and enhanced FOXO3 expression by Akt promotes cell survival and resistance (Populo et al., 2012). Even more, miR-155-5p also targets PDK1 in liver cancer (Li et al., 2021c). It has been shown that PDK1 and PDK2 cause phosphorylation and activation of Akt after its translocation to inner membrane, modulating the function of numerous substrates involved in the regulation of cell survival, cell cycle progression and cellular growth (Fresno Vara et al., 2004). So, enhanced expression of miR-155-5p increases cell proliferation and reduces apoptosis of Hep3B2.1-7 liver cancer cells (Li et al., 2021c).
Other signal transductions involved in DDP-resistance are the nuclear factor (NF)-κB and apoptosis-related signaling pathways. NF-κB is known to play an important role in cell survival and inflammation. Several miRs have been reported to regulate NF-κB, such as miR-146a, miR-152 and miR-381 in lung cancer cells (Jiang P. et al., 2017; Huang et al., 2018; Zhao et al., 2019). Reduced expression of miR-146a (Jiang P. et al., 2017), miR-152 (Zhao et al., 2019) and miR-381 (Huang et al., 2018) is observed in DDP-resistant A-549 cells, which gives rise to a heightened NF-κB expression and promotes DDP-resistant phenotype. Moreover, another study demonstrated that GSTP1 was able to interact with IKKβ to activate NF-κB and induced the expression and release of IL-6, thus mediating drug resistance in breast cancer cells (Dong et al., 2020). Furthermore, miR-133b was diminished in DDP-resistant lung cells (Lin et al., 2018). Finally, miR-362 and miR-505 overexpression were observed in gastric cancer cells (Xia et al., 2014; Wang Z. et al., 2020), and their enhanced expression promoted nuclear accumulation of NF-κB/p65, due to both miRs targeted CYLD directly and its downregulation mediated NF-κB activation. Besides, Zhang and Luo found that miR-29c was downregulated in HepG2/DDP cells, and demonstrated that miR-29c targeted SIRT1 (Zhang and Luo, 2018). SIRT1 may have enhanced activity in tumor cell growth by promoting NF-κB expression (Yeung et al., 2004).
The Wnt/β-catenin signaling pathway participates in various physiological processes such as proliferation, differentiation, apoptosis, migration and invasion; on the other hand, dysregulation of the Wnt/β-catenin contributes to the development and progression of some solid tumors (Ge and Wang, 2010). Mir-130b, miR-140-3p, miR-326, and miR-1249 directly enhance the noncanonical Wnt pathway in liver and lung cancer cells (Zhang et al., 2018; Wu S. et al., 2020; Carotenuto et al., 2020; Wu Y. et al., 2020). Also, SOX30, a tumor suppressor, acts as a transcription factor by binding directly to the p53 promoter and reduces SOX30 expression, resulting in enhanced β-catenin expression and Wnt/β-catenin pathway activation (Liu et al., 2020c). Guo et al. (2017) demonstrated miR-645 directly targeted SOX30 in colorectal cancer cells, enhancing DDP-resistant phenotype.
Other molecules also have been reported to confer DDP-resistance by inhibiting apoptosis. CYP1B1, a cytochrome P450 enzyme, is overexpressed in malignant ovarian cancer (Zhu et al., 2015). MiR-513a-3p had the same binding site to CYP1B1, low miR-513a-3p levels enhance CYP1B1 expression, conferring DDP-resistance by reducing DDP-induced apoptosis in gastric cancer cells (Cheng et al., 2021). ROCK1 and ROCK2 proteins are narrowly associated with tumor progress and lymph node metastasis (Zhang J. et al., 2015). Moreover, ROCK2 was regulated by miR-142-3p, and its reduced levels enhance ROCK2 expression, resulting in a DDP-resistant phenotype by reducing DDP-induced apoptosis in gastric cancer cells (Peng et al., 2020).
3.5 MicroRNAs Involved in Drug Efflux
The reduced uptake of water-soluble drugs and augmented drug efflux from cancer cells are the biochemical and cytological mechanisms of drug resistance in cancer cells (Chen et al., 2015). P-glycoprotein (P-gp, also known as MDR1) is encoded by the multidrug resistance gene (ABCB1). P-gp acts as a drug pump and it can bind to several drugs and pump them out of the cells, thereby decreasing their intracellular concentration and the sensitivity of cancer cells to the drug (Breier et al., 2005). P-gp is influenced by miR-30 and miR-129 in gastric cancer cells (Lu et al., 2017; Du et al., 2018), and by miR-144-3p, miR-145, and miR-202-5p in lung cancer cells (Tian et al., 2019; Zhang et al., 2019; Shen JG. et al., 2020).
Also, two additional ABC transporters, the multidrug resistance-associated protein 1 (MRP1; encoded by ABCC1), and ABCG2 are also implicated in multidrug resistance (Robey et al., 2018). Mechanistically, ABCC1 was targeted by miR-185-5p and negatively regulated its expression in lung cancer cells (Pei et al., 2016). Additionally, miR-144-3p and miR-145 also influenced the expression of MRP1 in lung cancer cells (Tian et al., 2019; Zhang et al., 2019), and by miR-381 in breast cancer cells (Yi et al., 2019) thus contributing to DDP-resistant phenotype.
4 Conclusion and Future Perspectives
Resistance to DDP is a major challenge that hampers the success of cancer treatment. According to current knowledge, multiple factors such as DNA damage and repair, transport process, autophagy, and apoptosis are involved in resistance to platinum-based drugs. Some dysregulated miRs functioned as an oncogenic molecules and others acted as a tumor repressor, and we tried to provide a general vision about this effect. Understanding the underlying molecular mechanisms of DDP-resistance is fundamental to reverse chemoresistance. In this way, it is possible to develop strategies to identify biomarkers of drug response and resistance, being useful in future clinical trials and rational management of cancer patients (Figure 2). Vast evidence shows that specific miRs can be regulated and then targets downstream genes to re-sensitize cancer cells to the effects of DDP. For example, lidocaine alleviates DDP-resistance of MGC-803/DDP gastric cancer cells, inhibiting their migration through decreasing miR-10b expression (Zhang X. et al., 2020). Besides, the use nanoliposomes loaded with miR-1296 sensitizes breast cancer cells to DDP, by reducing CCND1, and thus, EMT process (Albakr et al., 2021). Finally, curcumin treatment is able to restrain the proliferation and facilitated apoptosis in HCT8/DDP cells, by promoting miR-497/Bcl-2 axis (Zheng ZH. et al., 2021). Consequently, it is just a matter of time until miR-based therapies be proved to restore the sensitivity of tumor cells to some anticancer drugs including DDP.
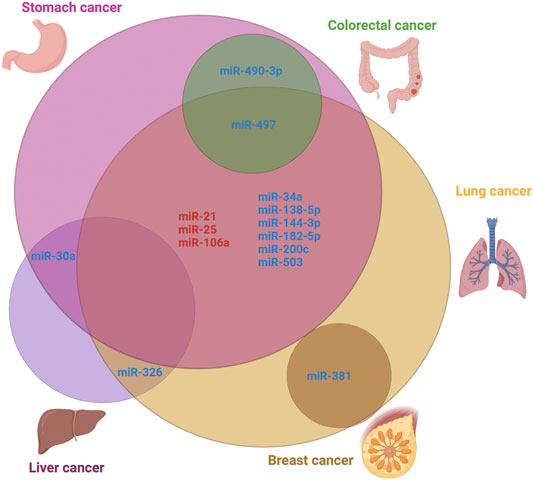
FIGURE 2. Venn diagram showing the common microRNAs expressed in the top five deadliest cancer. Upregulated microRNAs are highlighted in red color, whereas downregulated ones are in blue color.
In this review, we have summarized some of our current understanding of microRNAs that affect DDP-resistance and some strategies that have been employed to sensitize cancer cells to DDP chemotherapy. These studies have improved our understanding of the involvement of miRs in drug resistance and provide a starting point for the development of ncRNA-based therapy to accelerate the resolution of DDP-resistance in many cancers, to improve the quality of life and prognosis of patients.
Author Contributions
PL, NS, and KS contributed to the conception of the summarize, performed the data analyses and wrote the manuscript. NT and MV performed figures and tables. NT, PM, and LS reviewed and edited the manuscript. All authors read and approved the manuscript.
Funding
This research was funded by ANID-FAPESP (Grant No. 19/13250-1), FONDECYT (Grant No. 1171765), Programa de Formación de Investigadores Postdoctorales, VRIP, Universidad de La Frontera, Temuco, Chile (Code VRIP 21001) and FONDECYT Postdoctoral Grant No. 3220404 awarded to PL.
Conflict of Interest
The authors declare that the research was conducted in the absence of any commercial or financial relationships that could be construed as a potential conflict of interest.
Publisher’s Note
All claims expressed in this article are solely those of the authors and do not necessarily represent those of their affiliated organizations, or those of the publisher, the editors and the reviewers. Any product that may be evaluated in this article, or claim that may be made by its manufacturer, is not guaranteed or endorsed by the publisher.
References
Albakr, L., Alqahtani, F. Y., Aleanizy, F. S., Alomrani, A., Badran, M., Alhindas, H., et al. (2021). Improved Delivery of miR-1296 Loaded Cationic Nanoliposomes for Effective Suppression of Triple Negative Breast Cancer. Saudi Pharm. J. 29 (5), 446–455. doi:10.1016/j.jsps.2021.04.007
Amable, L. (2016). Cisplatin Resistance and Opportunities for Precision Medicine. Pharmacol. Res. 106, 27–36. doi:10.1016/j.phrs.2016.01.001
Anwanwan, D., Singh, S. K., Singh, S., Saikam, V., and Singh, R. (2020). Challenges in Liver Cancer and Possible Treatment Approaches. Biochim. Biophys. Acta Rev. Cancer 1873 (1), 188314. doi:10.1016/j.bbcan.2019.188314
Ashrafizadeh, M., Zarrabi, A., Hushmandi, K., Kalantari, M., Mohammadinejad, R., Javaheri, T., et al. (2020). Association of the Epithelial-Mesenchymal Transition (EMT) with Cisplatin Resistance. Int. J. Mol. Sci. 21 (11), 4002. doi:10.3390/ijms21114002
Bao, Y., Zhang, Y., Lu, Y., Guo, H., Dong, Z., Chen, Q., et al. (2020). Overexpression of microRNA-9 Enhances Cisplatin Sensitivity in Hepatocellular Carcinoma by Regulating EIF5A2-Mediated Epithelial-Mesenchymal Transition. Int. J. Biol. Sci. 16 (5), 827–837. doi:10.7150/ijbs.32460
Bar, J., Gorn-Hondermann, I., Moretto, P., Perkins, T. J., Niknejad, N., Stewart, D. J., et al. (2015). miR Profiling Identifies Cyclin-dependent Kinase 6 Downregulation as a Potential Mechanism of Acquired Cisplatin Resistance in Non-small-cell Lung Carcinoma. Clin. Lung Cancer 16 (6), e121–9. doi:10.1016/j.cllc.2015.01.008
Beikman, S., Gordon, P., Ferrari, S., Siegel, M., Zalewski, M. A., and Rosenzweig, M. Q. (2013). Understanding the Implications of the Breast Cancer Pathology Report: a Case Study. J. Adv. Pract. Oncol. 4 (3), 176–181. doi:10.6004/jadpro.2013.4.3.6
Bertram, J., Palfner, K., Hiddemann, W., and Kneba, M. (1998). Elevated Expression of S100P, CAPL and MAGE 3 in Doxorubicin-Resistant Cell Lines: Comparison of mRNA Differential Display Reverse Transcription-Polymerase Chain Reaction and Subtractive Suppressive Hybridization for the Analysis of Differential Gene Expression. Anticancer Drugs 9 (4), 311–317. doi:10.1097/00001813-199804000-00004
Bian, H. B., Pan, X., Yang, J. S., Wang, Z. X., and De, W. (2011). Upregulation of microRNA-451 Increases Cisplatin Sensitivity of Non-small Cell Lung Cancer Cell Line (A549). J. Exp. Clin. Cancer Res. 30, 20. doi:10.1186/1756-9966-30-20
Breier, A., Barancík, M., Sulová, Z., and Uhrík, B. (2005). P-glycoprotein--implications of Metabolism of Neoplastic Cells and Cancer Therapy. Curr. Cancer Drug Targets 5 (6), 457–468. doi:10.2174/1568009054863636
Bukowski, K., Kciuk, M., and Kontek, R. (2020). Mechanisms of Multidrug Resistance in Cancer Chemotherapy. Int. J. Mol. Sci. 21 (9), 3233. doi:10.3390/ijms21093233
Carotenuto, P., Hedayat, S., Fassan, M., Cardinale, V., Lampis, A., Guzzardo, V., et al. (2020). Modulation of Biliary Cancer Chemo-Resistance through MicroRNA-Mediated Rewiring of the Expansion of CD133+ Cells. Hepatology 72 (3), 982–996. doi:10.1002/hep.31094
Ceppi, P., Mudduluru, G., Kumarswamy, R., Rapa, I., Scagliotti, G. V., Papotti, M., et al. (2010). Loss of miR-200c Expression Induces an Aggressive, Invasive, and Chemoresistant Phenotype in Non-small Cell Lung Cancer. Mol. Cancer Res. 8 (9), 1207–1216. doi:10.1158/1541-7786.MCR-10-0052
Chandrakesan, P., Panneerselvam, J., Qu, D., Weygant, N., May, R., Bronze, M. S., et al. (2016). Regulatory Roles of Dclk1 in Epithelial Mesenchymal Transition and Cancer Stem Cells. J. Carcinog Mutagen 7 (2). doi:10.4172/2157-2518.1000257
Chekhun, V. F., Lukyanova, N. Y., Burlaka, C. A., Bezdenezhnykh, N. A., Shpyleva, S. I., Tryndyak, V. P., et al. (2013). Iron Metabolism Disturbances in the MCF-7 Human Breast Cancer Cells with Acquired Resistance to Doxorubicin and Cisplatin. Int. J. Oncol. 43 (5), 1481–1486. doi:10.3892/ijo.2013.2063
Chen, D., Ping, S., Xu, Y., Wang, M., Jiang, X., Xiong, L., et al. (2021). Non-Coding RNAs in Gastric Cancer: From Malignant Hallmarks to Clinical Applications. Front Cel Dev Biol 9, 732036. doi:10.3389/fcell.2021.732036
Chen, L., Han, X., Hu, Z., and Chen, L. (2019). The PVT1/miR-216b/Beclin-1 Regulates Cisplatin Sensitivity of NSCLC Cells via Modulating Autophagy and Apoptosis. Cancer Chemother. Pharmacol. 83 (5), 921–931. doi:10.1007/s00280-019-03808-3
Chen, L., Kong, G., Zhang, C., Dong, H., Yang, C., Song, G., et al. (2016a). MicroRNA-432 Functions as a Tumor Suppressor Gene through Targeting E2F3 and AXL in Lung Adenocarcinoma. Oncotarget 7 (15), 20041–20053. doi:10.18632/oncotarget.7884
Chen, M. J., Wu, D. W., Wang, G. C., Wang, Y. C., Chen, C. Y., and Lee, H. (2018). MicroRNA-630 May Confer Favorable Cisplatin-Based Chemotherapy and Clinical Outcomes in Non-small Cell Lung Cancer by Targeting Bcl-2. Oncotarget 9 (17), 13758–13767. doi:10.18632/oncotarget.24474
Chen, N., She, Q. B., Bode, A. M., and Dong, Z. (2002). Differential Gene Expression Profiles of Jnk1- and Jnk2-Deficient Murine Fibroblast Cells. Cancer Res. 62 (5), 1300–1304.
Chen, Q. Y., Jiao, D. M., Wang, J., Hu, H., Tang, X., Chen, J., et al. (2016). miR-206 Regulates Cisplatin Resistance and EMT in Human Lung Adenocarcinoma Cells Partly by Targeting MET. Oncotarget 7 (17), 24510–24526. doi:10.18632/oncotarget.8229
Chen, X., Wang, Y., Xia, H., Wang, Q., Jiang, X., Lin, Z., et al. (2012). Loss of E-Cadherin Promotes the Growth, Invasion and Drug Resistance of Colorectal Cancer Cells and Is Associated with Liver Metastasis. Mol. Biol. Rep. 39 (6), 6707–6714. doi:10.1007/s11033-012-1494-2
Chen, X., Zhu, H., Ye, W., Cui, Y., and Chen, M. (2019). MicroRNA-29a E-nhances C-isplatin S-ensitivity in N-on-small C-ell L-ung C-ancer through the R-egulation of REV3L. Mol. Med. Rep. 19 (2), 831–840. doi:10.3892/mmr.2018.9723
Chen, Y., Gao, Y., Zhang, K., Li, C., Pan, Y., Chen, J., et al. (2015). MicroRNAs as Regulators of Cisplatin Resistance in Lung Cancer. Cell Physiol Biochem 37 (5), 1869–1880. doi:10.1159/000438548
Chen, Y., Zhao, H., Li, H., Feng, X., Tang, H., Qiu, C., et al. (2020). LINC01234/MicroRNA-31-5p/MAGEA3 Axis Mediates the Proliferation and Chemoresistance of Hepatocellular Carcinoma Cells. Mol. Ther. Nucleic Acids 19, 168–178. doi:10.1016/j.omtn.2019.10.035
Chen, Z., Gao, Y. J., Hou, R. Z., Ding, D. Y., Song, D. F., Wang, D. Y., et al. (2019). MicroRNA-206 Facilitates Gastric Cancer Cell Apoptosis and Suppresses Cisplatin Resistance by Targeting MAPK2 Signaling Pathway. Eur. Rev. Med. Pharmacol. Sci. 23 (1), 171–180. doi:10.26355/eurrev_201901_16761
Cheng, C., Qin, Y., Zhi, Q., Wang, J., and Qin, C. (2018). Knockdown of Long Non-coding RNA HOTAIR Inhibits Cisplatin Resistance of Gastric Cancer Cells through Inhibiting the PI3K/Akt and Wnt/β-Catenin Signaling Pathways by Up-Regulating miR-34a. Int. J. Biol. Macromol 107 (Pt B), 2620–2629. doi:10.1016/j.ijbiomac.2017.10.154
Cheng, H., Sharen, G., Wang, Z., and Zhou, J. (2021). LncRNA UCA1 Enhances Cisplatin Resistance by Regulating CYP1B1-Mediated Apoptosis via miR-513a-3p in Human Gastric Cancer. Cmar 13, 367–377. doi:10.2147/CMAR.S277399
Cheng, M. W., Shen, Z. T., Hu, G. Y., and Luo, L. G. (2017). Prognostic Significance of microRNA-7 and its Roles in the Regulation of Cisplatin Resistance in Lung Adenocarcinoma. Cel Physiol Biochem 42 (2), 660–672. doi:10.1159/000477884
Cui, Y., Li, G., Zhang, X., Dai, F., and Zhang, R. (2018). Increased MALAT1 Expression Contributes to Cisplatin Resistance in Non-small Cell Lung Cancer. Oncol. Lett. 16 (4), 4821–4828. doi:10.3892/ol.2018.9293
DaSilva-Arnold, S. C., Kuo, C. Y., Davra, V., Remache, Y., Kim, P. C. W., Fisher, J. P., et al. (2019). ZEB2, a Master Regulator of the Epithelial-Mesenchymal Transition, Mediates Trophoblast Differentiation. Mol. Hum. Reprod. 25 (2), 61–75. doi:10.1093/molehr/gay053
Deng, X., Kong, F., Li, S., Jiang, H., Dong, L., Xu, X., et al. (2021). A KLF4/PiHL/EZH2/HMGA2 Regulatory axis and its Function in Promoting Oxaliplatin-Resistance of Colorectal Cancer. Cell Death Dis 12 (5), 485. doi:10.1038/s41419-021-03753-1
Dong, C., Wu, J., Chen, Y., Nie, J., and Chen, C. (2021). Activation of PI3K/AKT/mTOR Pathway Causes Drug Resistance in Breast Cancer. Front. Pharmacol. 12, 628690. doi:10.3389/fphar.2021.628690
Dong, X., Sun, R., Wang, J., Yu, S., Cui, J., Guo, Z., et al. (2020). Glutathione S-Transferases P1-Mediated Interleukin-6 in Tumor-Associated Macrophages Augments Drug-Resistance in MCF-7 Breast Cancer. Biochem. Pharmacol. 182, 114289. doi:10.1016/j.bcp.2020.114289
Dong, Z., Zhong, Z., Yang, L., Wang, S., and Gong, Z. (2014). MicroRNA-31 Inhibits Cisplatin-Induced Apoptosis in Non-small Cell Lung Cancer Cells by Regulating the Drug Transporter ABCB9. Cancer Lett. 343 (2), 249–257. doi:10.1016/j.canlet.2013.09.034
Donzelli, S., Fontemaggi, G., Fazi, F., Di Agostino, S., Padula, F., Biagioni, F., et al. (2012). MicroRNA-128-2 Targets the Transcriptional Repressor E2F5 Enhancing Mutant P53 Gain of Function. Cell Death Differ 19 (6), 1038–1048. doi:10.1038/cdd.2011.190
Du, C., Wang, Y., Zhang, Y., Zhang, J., Zhang, L., and Li, J. (2020). LncRNA DLX6-AS1 Contributes to Epithelial-Mesenchymal Transition and Cisplatin Resistance in Triple-Negative Breast Cancer via Modulating Mir-199b-5p/Paxillin Axis. Cel Transpl. 29, 963689720929983. doi:10.1177/0963689720929983
Du, X., Liu, B., Luan, X., Cui, Q., and Li, L. (2018). miR-30 Decreases Multidrug Resistance in Human Gastric Cancer Cells by Modulating Cell Autophagy. Exp. Ther. Med. 15 (1), 599–605. doi:10.3892/etm.2017.5354
El-Serag, H. B., Marrero, J. A., Rudolph, L., and Reddy, K. R. (2008). Diagnosis and Treatment of Hepatocellular Carcinoma. Gastroenterology 134 (6), 1752–1763. doi:10.1053/j.gastro.2008.02.090
Fang, Y., Shen, H., Li, H., Cao, Y., Qin, R., Long, L., et al. (2013). miR-106a Confers Cisplatin Resistance by Regulating PTEN/Akt Pathway in Gastric Cancer Cells. Acta Biochim. Biophys. Sin (Shanghai) 45 (11), 963–972. doi:10.1093/abbs/gmt106
FDA (1978). Approval Summary for Cisplatin for Metastatic Ovarian Tumors. Silver Spring: FDA Oncology Tools.
Ferlay, J., Colombet, M., Soerjomataram, I., Parkin, D. M., Piñeros, M., Znaor, A., et al. (2021). Cancer Statistics for the Year 2020: An Overview. Int. J. Cancer 149, 778–789. doi:10.1002/ijc.33588
Flores, C., and Sobrevia, L. (2000). Tumorogenesis and Mdm2 Protein. Rev. Med. Chil 128 (5), 539–546. doi:10.4067/S0034-98872000000500014
Foronda, M., Tarumoto, Y., Schatoff, E. M., Leach, B. I., Diaz, B. J., Zimmerman, J., et al. (2019). Tankyrase Inhibition Sensitizes Cells to CDK4 Blockade. PLoS One 14 (12), e0226645. doi:10.1371/journal.pone.0226645
Fresno Vara, J. A., Casado, E., de Castro, J., Cejas, P., Belda-Iniesta, C., and González-Barón, M. (2004). PI3K/Akt Signalling Pathway and Cancer. Cancer Treat. Rev. 30 (2), 193–204. doi:10.1016/j.ctrv.2003.07.007
Gao, X., Chen, G., Cai, H., Wang, X., Song, K., Liu, L., et al. (2020). Aberrantly Enhanced Melanoma-Associated Antigen (MAGE)-A3 Expression Facilitates Cervical Cancer Cell Proliferation and Metastasis via Actuating Wnt Signaling Pathway. Biomed. Pharmacother. 122, 109710. doi:10.1016/j.biopha.2019.109710
Gao, Y., Liu, Z., Ding, Z., Hou, S., Li, J., and Jiang, K. (2018). MicroRNA-155 Increases colon Cancer Chemoresistance to Cisplatin by Targeting Forkhead Box O3. Oncol. Lett. 15 (4), 4781–4788. doi:10.3892/ol.2018.7976
Ge, P., Cao, L., Zheng, M., Yao, Y., Wang, W., and Chen, X. (2021). LncRNA SNHG1 Contributes to the Cisplatin Resistance and Progression of NSCLC via miR-330-5p/DCLK1 axis. Exp. Mol. Pathol. 120, 104633. doi:10.1016/j.yexmp.2021.104633
Ge, X., Liu, X., Lin, F., Li, P., Liu, K., Geng, R., et al. (2016). MicroRNA-421 Regulated by HIF-1α Promotes Metastasis, Inhibits Apoptosis, and Induces Cisplatin Resistance by Targeting E-Cadherin and Caspase-3 in Gastric Cancer. Oncotarget 7 (17), 24466–24482. doi:10.18632/oncotarget.8228
Ge, X., and Wang, X. (2010). Role of Wnt Canonical Pathway in Hematological Malignancies. J. Hematol. Oncol. 3, 33. doi:10.1186/1756-8722-3-33
Georgescu, M. M. (2010). PTEN Tumor Suppressor Network in PI3K-Akt Pathway Control. Genes Cancer 1 (12), 1170–1177. doi:10.1177/1947601911407325
Godin-Heymann, N., Brabetz, S., Murillo, M. M., Saponaro, M., Santos, C. R., Lobley, A., et al. (2016). Tumour-suppression Function of KLF12 through Regulation of Anoikis. Oncogene 35 (25), 3324–3334. doi:10.1038/onc.2015.394
Gu, Y., Fei, Z., and Zhu, R. (2020). miR-21 Modulates Cisplatin Resistance of Gastric Cancer Cells by Inhibiting Autophagy via the PI3K/Akt/mTOR Pathway. Anticancer Drugs 31 (4), 385–393. doi:10.1097/CAD.0000000000000886
Gu, Y., Zhang, Z., Yin, J., Ye, J., Song, Y., Liu, H., et al. (2017). Epigenetic Silencing of miR-493 Increases the Resistance to Cisplatin in Lung Cancer by Targeting Tongue Cancer Resistance-Related Protein 1(TCRP1). J. Exp. Clin. Cancer Res. 36 (1), 114. doi:10.1186/s13046-017-0582-5
Guan, Y., Zhang, Y., Hao, L., and Nie, Z. (2020). CircRNA_102272 Promotes Cisplatin-Resistance in Hepatocellular Carcinoma by Decreasing MiR-326 Targeting of RUNX2. Cancer Manag. Res. 12, 12527–12534. doi:10.2147/CMAR.S258230
Gudas, J. M., Payton, M., Thukral, S., Chen, E., Bass, M., Robinson, M. O., et al. (1999). Cyclin E2, a Novel G1 Cyclin that Binds Cdk2 and Is Aberrantly Expressed in Human Cancers. Mol. Cel Biol 19 (1), 612–622. doi:10.1128/MCB.19.1.612
Guo, C., Dong, G., Liang, X., and Dong, Z. (2019). Epigenetic Regulation in AKI and Kidney Repair: Mechanisms and Therapeutic Implications. Nat. Rev. Nephrol. 15 (4), 220–239. doi:10.1038/s41581-018-0103-6
Guo, S. T., Guo, X. Y., Wang, J., Wang, C. Y., Yang, R. H., Wang, F. H., et al. (2017). MicroRNA-645 Is an Oncogenic Regulator in colon Cancer. Oncogenesis 6 (5), e335. doi:10.1038/oncsis.2017.37
Guo, S. T., Jiang, C. C., Wang, G. P., Li, Y. P., Wang, C. Y., Guo, X. Y., et al. (2013). MicroRNA-497 Targets Insulin-like Growth Factor 1 Receptor and Has a Tumour Suppressive Role in Human Colorectal Cancer. Oncogene 32 (15), 1910–1920. doi:10.1038/onc.2012.214
He, B., Wu, C., Sun, W., Qiu, Y., Li, J., Liu, Z., et al. (2021). miR-383 I-ncreases the C-isplatin S-ensitivity of L-ung A-denocarcinoma C-ells through I-nhibition of the RBM24-mediated NF-κB S-ignaling P-athway. Int. J. Oncol. 59 (5), 87. doi:10.3892/ijo.2021.5267
He, J., Qi, H., Chen, F., and Cao, C. (2017). MicroRNA-25 Contributes to Cisplatin Resistance in Gastric Cancer Cells by Inhibiting Forkhead Box O3a. Oncol. Lett. 14 (5), 6097–6102. doi:10.3892/ol.2017.6982
He, X., Xiao, X., Dong, L., Wan, N., Zhou, Z., Deng, H., et al. (2015). MiR-218 Regulates Cisplatin Chemosensitivity in Breast Cancer by Targeting BRCA1. Tumour Biol. 36 (3), 2065–2075. doi:10.1007/s13277-014-2814-z
Hu, J., Lieb, J. D., Sancar, A., and Adar, S. (2016). Cisplatin DNA Damage and Repair Maps of the Human Genome at Single-Nucleotide Resolution. Proc. Natl. Acad. Sci. U S A. 113 (41), 11507–11512. doi:10.1073/pnas.1614430113
Hua, G., Zeng, Z. L., Shi, Y. T., Chen, W., He, L. F., and Zhao, G. F. (2021). LncRNA XIST Contributes to Cisplatin Resistance of Lung Cancer Cells by Promoting Cellular Glycolysis through Sponging miR-101-3p. Pharmacology 106 (9-10), 498–508. doi:10.1159/000512621
Hua, L., Zhu, G., and Wei, J. (2018). MicroRNA-1 Overexpression Increases Chemosensitivity of Non-small Cell Lung Cancer Cells by Inhibiting Autophagy Related 3-mediated Autophagy. Cell Biol Int 42 (9), 1240–1249. doi:10.1002/cbin.10995
Huang, F. X., Chen, H. J., Zheng, F. X., Gao, Z. Y., Sun, P. F., Peng, Q., et al. (2019). LncRNA BLACAT1 Is Involved in Chemoresistance of Non-small C-ell L-ung C-ancer C-ells by R-egulating A-utophagy. Int. J. Oncol. 54 (1), 339–347. doi:10.3892/ijo.2018.4614
Huang, J. Q., Wei, F. K., Xu, X. L., Ye, S. X., Song, J. W., Ding, P. K., et al. (2019). SOX9 Drives the Epithelial-Mesenchymal Transition in Non-small-cell Lung Cancer through the Wnt/β-Catenin Pathway. J. Transl Med. 17 (1), 143. doi:10.1186/s12967-019-1895-2
Huang, R. S., Zheng, Y. L., Zhao, J., and Chun, X. (2018). microRNA-381 Suppresses the Growth and Increases Cisplatin Sensitivity in Non-small Cell Lung Cancer Cells through Inhibition of Nuclear Factor-Κb Signaling. Biomed. Pharmacother. 98, 538–544. doi:10.1016/j.biopha.2017.12.092
Huang, T., Ren, K., Ding, G., Yang, L., Wen, Y., Peng, B., et al. (2020). miR-10a I-ncreases the C-isplatin R-esistance of L-ung A-denocarcinoma C-irculating T-umor C-ells via T-argeting PIK3CA in the PI3K/Akt P-athway. Oncol. Rep. 43 (6), 1906–1914. doi:10.3892/or.2020.7547
Huang, X., Li, Z., Zhang, Q., Wang, W., Li, B., Wang, L., et al. (2019). Circular RNA AKT3 Upregulates PIK3R1 to Enhance Cisplatin Resistance in Gastric Cancer via miR-198 Suppression. Mol. Cancer 18 (1), 71. doi:10.1186/s12943-019-0969-3
Huang, X. X., Zhang, Q., Hu, H., Jin, Y., Zeng, A. L., Xia, Y. B., et al. (2020). A Novel Circular RNA circFN1 Enhances Cisplatin Resistance in Gastric Cancer via Sponging miR-182-5p. J. Cel Biochem 122, 1009–1020. doi:10.1002/jcb.29641
Jia, R., and Wang, C. (2020). MiR-29b-3p Reverses Cisplatin Resistance by Targeting COL1A1 in Non-small-cell Lung Cancer A549/DDP Cells. Cancer Manag. Res. 12, 2559–2566. doi:10.2147/CMAR.S246625
Jiang, J., Fang, H., Sun, W., Feng, Y., and Zhou, L. (2020). Reversal of Drug Resistance in Gastric Cancer Cells by miR-503 and its Mechanism. Acta Med. Mediterranea 36, 1841–1848. doi:10.19193/0393-6384_2020_3_289
Jiang, P., Jia, W., Wei, X., Zhang, X., Wang, C., Li, B., et al. (2017). MicroRNA-146a Regulates Cisplatin-Resistance of Non-small Cell Lung Cancer Cells by Targeting NF-Κb Pathway. Int. J. Clin. Exp. Pathol. 10 (12), 11545–11553.
Jiang, P., Wu, X., Wang, X., Huang, W., and Feng, Q. (2016). NEAT1 Upregulates EGCG-Induced CTR1 to Enhance Cisplatin Sensitivity in Lung Cancer Cells. Oncotarget 7 (28), 43337–43351. doi:10.18632/oncotarget.9712
Jiang, T., Dong, P., Li, L., Ma, X., Xu, P., Zhu, H., et al. (2017). MicroRNA-200c Regulates Cisplatin Resistance by Targeting ZEB2 in Human Gastric Cancer Cells. Oncol. Rep. 38 (1), 151–158. doi:10.3892/or.2017.5659
Jin, X., Pang, W., Zhang, Q., and Huang, H. (2019). MicroRNA-486-5p Improves Nonsmall-Cell Lung Cancer Chemotherapy Sensitivity and Inhibits Epithelial-Mesenchymal Transition by Targeting Twinfilin Actin Binding Protein 1. J. Int. Med. Res. 47 (8), 3745–3756. doi:10.1177/0300060519850739
Jin, Y., Zeng, S. X., Sun, X. X., Lee, H., Blattner, C., Xiao, Z., et al. (2008). MDMX Promotes Proteasomal Turnover of P21 at G1 and Early S Phases Independently of, but in Cooperation with, MDM2. Mol. Cel Biol 28 (4), 1218–1229. doi:10.1128/MCB.01198-07
Kim, H., Chung, H., Kim, H. J., Lee, J. Y., Oh, M. Y., Kim, Y., et al. (2008). Id-1 Regulates Bcl-2 and Bax Expression through P53 and NF-kappaB in MCF-7 Breast Cancer Cells. Breast Cancer Res. Treat. 112 (2), 287–296. doi:10.1007/s10549-007-9871-6
Kitamura, H., Yazawa, T., Sato, H., Okudela, K., and Shimoyamada, H. (2009). Small Cell Lung Cancer: Significance of RB Alterations and TTF-1 Expression in its Carcinogenesis, Phenotype, and Biology. Endocr. Pathol. 20 (2), 101–107. doi:10.1007/s12022-009-9072-4
Ko, T., and Li, S. (2019). Genome-wide Screening Identifies Novel Genes and Biological Processes Implicated in Cisplatin Resistance. FASEB J. 33 (6), 7143–7154. doi:10.1096/fj.201801534RR
Kozomara, A., Birgaoanu, M., and Griffiths-Jones, S. (2019). miRBase: from microRNA Sequences to Function. Nucleic Acids Res. 47 (D1), D155–D162. doi:10.1093/nar/gky1141
Li, J., Chen, Y., Jin, M., Wang, J., Li, S., Chen, Z., et al. (2017). MicroRNA-134 Reverses Multidrug Resistance in Human Lung Adenocarcinoma Cells by Targeting FOXM1. Oncol. Lett. 13 (3), 1451–1455. doi:10.3892/ol.2017.5574
Li, J. H., Luo, N., Zhong, M. Z., Xiao, Z. Q., Wang, J. X., Yao, X. Y., et al. (2016a). Inhibition of microRNA-196a Might Reverse Cisplatin Resistance of A549/DDP Non-small-cell Lung Cancer Cell Line. Tumour Biol. 37 (2), 2387–2394. doi:10.1007/s13277-015-4017-7
Li, J., Mo, R., and Zheng, L. (2021a). MicroRNA-490-3p Inhibits Migration and Chemoresistance of Colorectal Cancer Cells via Targeting TNKS2. World J. Surg. Onc 19 (1), 117. doi:10.1186/s12957-021-02226-1
Li, M., Gao, M., Xie, X., Zhang, Y., Ning, J., Liu, P., et al. (2019a). MicroRNA-200c Reverses Drug Resistance of Human Gastric Cancer Cells by Targeting Regulation of the NER-Ercc3/4 Pathway. Oncol. Lett. 18 (1), 145–152. doi:10.3892/ol.2019.10304
Li, N., Babaei-Jadidi, R., Lorenzi, F., Spencer-Dene, B., Clarke, P., Domingo, E., et al. (2019b). An FBXW7-ZEB2 axis Links EMT and Tumour Microenvironment to Promote Colorectal Cancer Stem Cells and Chemoresistance. Oncogenesis 8 (3), 13. doi:10.1038/s41389-019-0125-3
Li, N., Zhang, F., Li, S., and Zhou, S. (2014). Epigenetic Silencing of MicroRNA-503 Regulates FANCA Expression in Non-small Cell Lung Cancer Cell. Biochem. Biophys. Res. Commun. 444 (4), 611–616. doi:10.1016/j.bbrc.2014.01.103
Li, W., Dong, X., He, C., Tan, G., Li, Z., Zhai, B., et al. (2019c). LncRNA SNHG1 Contributes to Sorafenib Resistance by Activating the Akt Pathway and Is Positively Regulated by miR-21 in Hepatocellular Carcinoma Cells. J. Exp. Clin. Cancer Res. 38 (1), 183. doi:10.1186/s13046-019-1177-0
Li, W., Wang, W., Ding, M., Zheng, X., Ma, S., and Wang, X. (2016b). MiR-1244 Sensitizes the Resistance of Non-small Cell Lung Cancer A549 Cell to Cisplatin. Cancer Cel Int 16, 30. doi:10.1186/s12935-016-0305-6
Li, X. X., Jin, L., Sun, Z. F., Gu, F., Li, W. L., and Ma, Y. J. (2013). Robo1 Expression in Non-small Cell Lung Cancer and its Brain Metastasis. Zhonghua Zhong Liu Za Zhi 35 (3), 198–201. doi:10.3760/cma.j.issn.0253-3766.2013.03.008
Li, Y., Wang, L., Xu, X., Sun, H., and Wu, L. (2021b). LncRNA HLA Complex Group 11 Knockdown Alleviates Cisplatin Resistance in Gastric Cancer by Targeting the miR-144-3p/UBE2D1 Axis. Cancer Manag. Res. 13, 7543–7557. doi:10.2147/CMAR.S329846
Li, Y., Zhang, Y., Zhang, S., Huang, D., Li, B., Liang, G., et al. (2021c). circRNA circARNT2 Suppressed the Sensitivity of Hepatocellular Carcinoma Cells to Cisplatin by Targeting the miR-155-5p/PDK1 Axis. Mol. Ther. Nucleic Acids 23, 244–254. doi:10.1016/j.omtn.2020.08.037
Liang, L., Jia, J., Kang, H., and Song, B. (2021). Exosomes Mediate Mir-21 to Promote Cisplatin Resistance in Lung Cancer by Regulating Pten. Acta Med. Mediterranea 37, 971–977. doi:10.19193/0393-6384_2021_2_148
Lin, C., Xie, L., Lu, Y., Hu, Z., and Chang, J. (2018). miR-133b Reverses Cisplatin Resistance by Targeting GSTP1 in Cisplatin-Resistant Lung Cancer Cells. Int. J. Mol. Med. 41 (4), 2050–2058. doi:10.3892/ijmm.2018.3382
Lin, H., Zhang, L., Zhang, C., and Liu, P. (2020). Exosomal MiR-500a-3p Promotes Cisplatin Resistance and Stemness via Negatively Regulating FBXW7 in Gastric Cancer. J. Cell Mol Med 24 (16), 8930–8941. doi:10.1111/jcmm.15524
Liu, S., Li, Q., Li, G., Zhang, Q., Zhuo, L., Han, X., et al. (2020). The Mechanism of m6A Methyltransferase METTL3-Mediated Autophagy in Reversing Gefitinib Resistance in NSCLC Cells by β-elemene. Cell Death Dis 11 (11), 969. doi:10.1038/s41419-020-03148-8
Liu, X., Zhou, X., Chen, Y., Huang, Y., He, J., and Luo, H. (2020). miR-186-5p Targeting SIX1 Inhibits Cisplatin Resistance in Non-small-cell Lung Cancer Cells (NSCLCs). Neoplasma 67 (1), 147–157. doi:10.4149/neo_2019_190511N420
Liu, Y., Wang, W., Li, Y., and Huang, Y. (2020). SOX30 Confers a Tumor Suppressive Effect in Acute Myeloid Leukemia through Inactivation of Wnt/β-Catenin Signaling. Mol. Cell Probes 52, 101578. doi:10.1016/j.mcp.2020.101578
Liu, Y., Xu, J., Jiang, M., Ni, L., and Ling, Y. (2020). CircRNA DONSON Contributes to Cisplatin Resistance in Gastric Cancer Cells by Regulating miR-802/BMI1 axis. Cancer Cell Int 20, 261. doi:10.1186/s12935-020-01358-w
Liu, Y., Xue, J., Zhong, M., Wang, Z., Li, J., and Zhu, Y. (2021). Prognostic Prediction, Immune Microenvironment, and Drug Resistance Value of Collagen Type I Alpha 1 Chain: From Gastrointestinal Cancers to Pan-Cancer Analysis. Front. Mol. Biosci. 8, 692120. doi:10.3389/fmolb.2021.692120
Lu, C., Shan, Z., Li, C., and Yang, L. (2017). MiR-129 Regulates Cisplatin-Resistance in Human Gastric Cancer Cells by Targeting P-Gp. Biomed. Pharmacother. 86, 450–456. doi:10.1016/j.biopha.2016.11.139
Luo, S., Shen, M., Chen, H., Li, W., and Chen, C. (2020). Long Non-coding RNA TP73-AS1 A-ccelerates the P-rogression and C-isplatin R-esistance of N-on-small C-ell L-ung C-ancer by U-pregulating the E-xpression of TRIM29 via C-ompetitively T-argeting microRNA-34a-5p. Mol. Med. Rep. 22 (5), 3822–3832. doi:10.3892/mmr.2020.11473
Luo, Y., Fu, Y., Huang, R., Gao, M., Liu, F., Gui, R., et al. (2019). CircRNA_101505 Sensitizes Hepatocellular Carcinoma Cells to Cisplatin by Sponging miR-103 and Promotes Oxidored-nitro Domain-Containing Protein 1 Expression. Cell Death Discov 5, 121. doi:10.1038/s41420-019-0202-6
Ma, Y., Li, X., Cheng, S., Wei, W., and Li, Y. (2015). MicroRNA-106a Confers Cisplatin Resistance in Non-small Cell Lung Cancer A549 Cells by Targeting Adenosine Triphosphatase-Binding Cassette A1. Mol. Med. Rep. 11 (1), 625–632. doi:10.3892/mmr.2014.2688
Meng, Q. B., Peng, J. J., Qu, Z. W., Zhu, X. M., Wen, Z., and Kang, W. M. (2019). Eukaryotic Initiation Factor 5A2 and Human Digestive System Neoplasms. World J. Gastrointest. Oncol. 11 (6), 449–458. doi:10.4251/wjgo.v11.i6.449
Meng, W., Tai, Y., Zhao, H., Fu, B., Zhang, T., Liu, W., et al. (2017). Downregulation of miR-33a-5p in Hepatocellular Carcinoma: A Possible Mechanism for Chemotherapy Resistance. Med. Sci. Monit. 23, 1295–1304. doi:10.12659/msm.902692
Mi, H., Wang, X., Wang, F., Li, L., Zhu, M., Wang, N., et al. (2020). SNHG15 Contributes to Cisplatin Resistance in Breast Cancer through Sponging miR-381. Onco Targets Ther. 13, 657–666. doi:10.2147/OTT.S223321
Mimae, T., Okada, M., Hagiyama, M., Miyata, Y., Tsutani, Y., Inoue, T., et al. (2012). Upregulation of Notch2 and Six1 Is Associated with Progression of Early-Stage Lung Adenocarcinoma and a More Aggressive Phenotype at Advanced Stages. Clin. Cancer Res. 18 (4), 945–955. doi:10.1158/1078-0432.CCR-11-1946
Momand, J., Jung, D., Wilczynski, S., and Niland, J. (1998). The MDM2 Gene Amplification Database. Nucleic Acids Res. 26 (15), 3453–3459. doi:10.1093/nar/26.15.3453
Montalto, F. I., and De Amicis, F. (2020). Cyclin D1 in Cancer: A Molecular Connection for Cell Cycle Control, Adhesion and Invasion in Tumor and Stroma. Cells 9 (12). doi:10.3390/cells9122648
Ni, Q., Zhang, Y., Tao, R., Li, X., and Zhu, J. (2021). MicroRNA-95-3p Serves as a Contributor to Cisplatin Resistance in Human Gastric Cancer Cells by Targeting EMP1/PI3K/AKT Signaling. Aging (Albany NY) 13 (6), 8665–8687. doi:10.18632/aging.202679
Ning, J., Jiao, Y., Xie, X., Deng, X., Zhang, Y., Yang, Y., et al. (2019). miR-138-5p M-odulates the E-xpression of E-xcision R-epair C-ross-complementing P-roteins ERCC1 and ERCC4, and R-egulates the S-ensitivity of G-astric C-ancer C-ells to C-isplatin. Oncol. Rep. 41 (2), 1131–1139. doi:10.3892/or.2018.6907
Ning, M. Y., Cheng, Z. L., and Zhao, J. (2020). MicroRNA-448 Targets SATB1 to Reverse the Cisplatin Resistance in Lung Cancer via Mediating Wnt/β-Catenin Signalling Pathway. J. Biochem. 168 (1), 41–51. doi:10.1093/jb/mvaa024
Ohta, K., Hoshino, H., Wang, J., Ono, S., Iida, Y., Hata, K., et al. (2015). MicroRNA-93 Activates C-Met/PI3K/Akt Pathway Activity in Hepatocellular Carcinoma by Directly Inhibiting PTEN and CDKN1A. Oncotarget 6 (5), 3211–3224. doi:10.18632/oncotarget.3085
Pan, X., Chen, Y., Shen, Y., and Tantai, J. (2019). Knockdown of TRIM65 Inhibits Autophagy and Cisplatin Resistance in A549/DDP Cells by Regulating miR-138-5p/ATG7. Cell Death Dis 10 (6), 429. doi:10.1038/s41419-019-1660-8
Panda, M., Tripathi, S. K., and Biswal, B. K. (2021). SOX9: An Emerging Driving Factor from Cancer Progression to Drug Resistance. Biochim. Biophys. Acta Rev. Cancer 1875 (2), 188517. doi:10.1016/j.bbcan.2021.188517
Pang, J., Ye, L., Zhao, D., Zhao, D., and Chen, Q. (2020). Circular RNA PRMT5 Confers Cisplatin-Resistance via miR-4458/REV3L axis in Non-small-cell Lung Cancer. Cell Biol Int 44 (12), 2416–2426. doi:10.1002/cbin.11449
Pang, L., Lu, J., Huang, J., Xu, C., Li, H., Yuan, G., et al. (2017). Upregulation of miR-146a Increases Cisplatin Sensitivity of the Non-small Cell Lung Cancer A549 Cell Line by Targeting JNK-2. Oncol. Lett. 14 (6), 7745–7752. doi:10.3892/ol.2017.7242
Paranjape, A. N., Balaji, S. A., Mandal, T., Krushik, E. V., Nagaraj, P., Mukherjee, G., et al. (2014). Bmi1 Regulates Self-Renewal and Epithelial to Mesenchymal Transition in Breast Cancer Cells through Nanog. BMC Cancer 14, 785. doi:10.1186/1471-2407-14-785
Pei, K., Zhu, J. J., Wang, C. E., Xie, Q. L., and Guo, J. Y. (2016). MicroRNA-185-5p Modulates Chemosensitivity of Human Non-small Cell Lung Cancer to Cisplatin via Targeting ABCC1. Eur. Rev. Med. Pharmacol. Sci. 20 (22), 4697–4704.
Peng, C., Huang, K., Liu, G., Li, Y., and Yu, C. (2019). MiR-876-3p Regulates Cisplatin Resistance and Stem Cell-like Properties of Gastric Cancer Cells by Targeting TMED3. J. Gastroenterol. Hepatol. 34 (10), 1711–1719. doi:10.1111/jgh.14649
Peng, L., Sang, H., Wei, S., Li, Y., Jin, D., Zhu, X., et al. (2020). circCUL2 Regulates Gastric Cancer Malignant Transformation and Cisplatin Resistance by Modulating Autophagy Activation via miR-142-3p/ROCK2. Mol. Cancer 19 (1), 156. doi:10.1186/s12943-020-01270-x
Pópulo, H., Lopes, J. M., and Soares, P. (2012). The mTOR Signalling Pathway in Human Cancer. Int. J. Mol. Sci. 13 (2), 1886–1918. doi:10.3390/ijms13021886
Qi, M., Liu, D., and Zhang, S. (2017). MicroRNA-21 Contributes to the Discrimination of Chemoresistance in Metastatic Gastric Cancer. Cancer Biomark 18 (4), 451–458. doi:10.3233/CBM-161732
Qin, X., Yu, S., Zhou, L., Shi, M., Hu, Y., Xu, X., et al. (2017). Cisplatin-resistant Lung Cancer Cell-Derived Exosomes Increase Cisplatin Resistance of Recipient Cells in Exosomal miR-100-5p-dependent Manner. Int. J. Nanomedicine 12, 3721–3733. doi:10.2147/IJN.S131516
Qu, W., Huang, W., Yang, F., Ju, H., and Zhu, G. (2020). Long Noncoding RNA LINC00461 Mediates Cisplatin Resistance of Rectal Cancer via miR-593-5p/CCND1 axis. Biomed. Pharmacother. 124, 109740. doi:10.1016/j.biopha.2019.109740
Rao, C., Miao, X., Zhao, G., Zhang, C., Shen, H., Dong, C., et al. (2019). MiR-219a-5p Enhances Cisplatin Sensitivity of Human Non-small Cell Lung Cancer by Targeting FGF9. Biomed. Pharmacother. 114, 108662. doi:10.1016/j.biopha.2019.108662
Robey, R. W., Pluchino, K. M., Hall, M. D., Fojo, A. T., Bates, S. E., and Gottesman, M. M. (2018). Revisiting the Role of ABC Transporters in Multidrug-Resistant Cancer. Nat. Rev. Cancer 18 (7), 452–464. doi:10.1038/s41568-018-0005-8
Ronchetti, S., Zollo, O., Bruscoli, S., Agostini, M., Bianchini, R., Nocentini, G., et al. (2004). GITR, a Member of the TNF Receptor Superfamily, Is Costimulatory to Mouse T Lymphocyte Subpopulations. Eur. J. Immunol. 34 (3), 613–622. doi:10.1002/eji.200324804
Rosenberg, B., VanCamp, L., Trosko, J. E., and Mansour, V. H. (1969). Platinum Compounds: a New Class of Potent Antitumour Agents. Nature 222 (5191), 385–386. doi:10.1038/222385a0
Ru, P., Steele, R., Hsueh, E. C., and Ray, R. B. (2011). Anti-miR-203 Upregulates SOCS3 Expression in Breast Cancer Cells and Enhances Cisplatin Chemosensitivity. Genes Cancer 2 (7), 720–727. doi:10.1177/1947601911425832
Sarin, N., Engel, F., Kalayda, G. V., Mannewitz, M., Cinatl, J., Rothweiler, F., et al. (2017). Cisplatin Resistance in Non-small Cell Lung Cancer Cells Is Associated with an Abrogation of Cisplatin-Induced G2/M Cell Cycle Arrest. PLoS One 12 (7), e0181081. doi:10.1371/journal.pone.0181081
Seidl, C., Panzitt, K., Bertsch, A., Brcic, L., Schein, S., Mack, M., et al. (2020). MicroRNA-182-5p Regulates Hedgehog Signaling Pathway and Chemosensitivity of Cisplatin-Resistant Lung Adenocarcinoma Cells via Targeting GLI2. Cancer Lett. 469, 266–276. doi:10.1016/j.canlet.2019.10.044
Shah, M. A., and Schwartz, G. K. (2001). Cell Cycle-Mediated Drug Resistance: an Emerging Concept in Cancer Therapy. Clin. Cancer Res. 7 (8), 2168–2181.
Shen, J. G., Xu, S. N., and Yin, L. G. (2020). LncRNA NORAD/miR-202-5p Regulates the Drug Resistance of A549/DDP to Cisplatin by Targeting P-Gp. Gen. Physiol. Biophys. 39 (5), 481–489. doi:10.4149/gpb_2020027
Shen, Q., Xu, Z., and Xu, S. (2020). Long Non-coding RNA LUCAT1 C-ontributes to C-isplatin R-esistance by R-egulating the miR-514a-3p/ULK1 axis in H-uman N-on-small C-ell L-ung C-ancer. Int. J. Oncol. 57 (4), 967–979. doi:10.3892/ijo.2020.5106
Sherr, C. J., and Roberts, J. M. (1999). CDK Inhibitors: Positive and Negative Regulators of G1-phase Progression. Genes Dev. 13 (12), 1501–1512. doi:10.1101/gad.13.12.1501
Shi, F., Zhang, L., Liu, X., and Wang, Y. (2021). Knock-down of microRNA miR-556-5p Increases Cisplatin-Sensitivity in Non-small Cell Lung Cancer (NSCLC) via Activating NLR Family Pyrin Domain Containing 3 (NLRP3)-Mediated Pyroptotic Cell Death. Bioengineered 12 (1), 6332–6342. doi:10.1080/21655979.2021.1971502
Shi, H., Li, K., Feng, J., Liu, G., Feng, Y., and Zhang, X. (2020). LncRNA-DANCR Interferes with miR-125b-5p/HK2 Axis to Desensitize Colon Cancer Cells to Cisplatin Vis Activating Anaerobic Glycolysis. Front. Oncol. 10, 1034. doi:10.3389/fonc.2020.01034
Shi, L., Xi, J., Xu, X., Peng, B., and Zhang, B. (2019). MiR-148a Suppressed Cell Invasion and Migration via Targeting WNT10b and Modulating β-catenin Signaling in Cisplatin-Resistant Colorectal Cancer Cells. Biomed. Pharmacother. 109, 902–909. doi:10.1016/j.biopha.2018.10.080
Siddik, Z. H. (2003). Cisplatin: Mode of Cytotoxic Action and Molecular Basis of Resistance. Oncogene 22 (47), 7265–7279. doi:10.1038/sj.onc.1206933
Siddik, Z. H. (1999). Expression of P53 in Cisplatin-Resistant Ovarian Cancer Cell Lines: Modulation with the Novel Platinum Analogue (1R, 2R-diaminocyclohexane)(trans-diacetato)(dichloro)-Platinum(IV). Clin. Cancer Res. 5, 655–663. pmid = 10100719.
Siegel, R. L., Miller, K. D., Goding Sauer, A., Fedewa, S. A., Butterly, L. F., Anderson, J. C., et al. (2020). Colorectal Cancer Statistics, 2020. CA Cancer J. Clin. 70 (3), 145–164. doi:10.3322/caac.21601
Song, A. L., Zhao, L., Wang, Y. W., He, D. Q., and Li, Y. M. (2019). Chemoresistance in Gastric Cancer Is Attributed to the Overexpression of Excision Repair Cross-Complementing 1 (ERCC1) Caused by microRNA-122 Dysregulation. J. Cell Physiol 234 (12), 22485–22492. doi:10.1002/jcp.28812
Song, Z., Jia, G., Ma, P., and Cang, S. (2021). Exosomal miR-4443 Promotes Cisplatin Resistance in Non-small Cell Lung Carcinoma by Regulating FSP1 m6A Modification-Mediated Ferroptosis. Life Sci. 276, 119399. doi:10.1016/j.lfs.2021.119399
Song, Z., Jia, N., Li, W., and Zhang, X. Y. (2020). LINC01572 Regulates Cisplatin Resistance in Gastric Cancer Cells by Mediating miR-497-5p. Onco Targets Ther. 13, 10877–10887. doi:10.2147/OTT.S267915
Sui, C., Meng, F., Li, Y., and Jiang, Y. (2015). miR-148b Reverses Cisplatin-Resistance in Non-small Cell Cancer Cells via Negatively Regulating DNA (Cytosine-5)-methyltransferase 1(DNMT1) Expression. J. Transl Med. 13, 132. doi:10.1186/s12967-015-0488-y
Sun, B., Hu, N., Cong, D., Chen, K., and Li, J. (2021). MicroRNA-25-3p Promotes Cisplatin Resistance in Non-small-cell Lung Carcinoma (NSCLC) through Adjusting PTEN/PI3K/AKT Route. Bioengineered 12 (1), 3219–3228. doi:10.1080/21655979.2021.1939577
Sun, G., Li, Z., He, Z., Wang, W., Wang, S., Zhang, X., et al. (2020). Circular RNA MCTP2 Inhibits Cisplatin Resistance in Gastric Cancer by miR-99a-5p-Mediated Induction of MTMR3 Expression. J. Exp. Clin. Cancer Res. 39 (1), 246. doi:10.1186/s13046-020-01758-w
Sun, H., Wu, P., Zhang, B., Wu, X., and Chen, W. (2021). MCM3AP-AS1 Promotes Cisplatin Resistance in Gastric Cancer Cells via the miR-138/FOXC1 axis. Oncol. Lett. 21 (3), 211. doi:10.3892/ol.2021.12472
Sun, J., Pan, L. M., Chen, L. B., and Wang, Y. (2017). LncRNA XIST Promotes Human Lung Adenocarcinoma Cells to Cisplatin Resistance via let-7i/BAG-1 axis. Cell Cycle 16 (21), 2100–2107. doi:10.1080/15384101.2017.1361071
Sun, L., Yao, Y., Liu, B., Lin, Z., Lin, L., Yang, M., et al. (2012). MiR-200b and miR-15b Regulate Chemotherapy-Induced Epithelial-Mesenchymal Transition in Human Tongue Cancer Cells by Targeting BMI1. Oncogene 31 (4), 432–445. doi:10.1038/onc.2011.263
Sun, T., Li, K., Zhu, K., Yan, R., Dang, C., and Yuan, D. (2020). SNHG6 Interacted with miR-325-3p to Regulate Cisplatin Resistance of Gastric Cancer by Targeting GITR. Onco Targets Ther. 13, 12181–12193. doi:10.2147/OTT.S262896
Sun, W., Ma, Y., Chen, P., and Wang, D. (2015). MicroRNA-10a Silencing Reverses Cisplatin Resistance in the A549/cisplatin Human Lung Cancer Cell Line via the Transforming Growth Factor-β/Smad2/STAT3/STAT5 Pathway. Mol. Med. Rep. 11 (5), 3854–3859. doi:10.3892/mmr.2015.3181
Sun, W., Ping, W., Tian, Y., Zou, W., Liu, J., and Zu, Y. (2018). miR-202 Enhances the Anti-tumor Effect of Cisplatin on Non-small Cell Lung Cancer by Targeting the Ras/MAPK Pathway. Cell Physiol Biochem 51 (5), 2160–2171. doi:10.1159/000495835
Sun, Y., Cao, Z., Shan, J., Gao, Y., Liu, X., Ma, D., et al. (2020). Hsa_circ_0020095 Promotes Oncogenesis and Cisplatin Resistance in Colon Cancer by Sponging miR-487a-3p and Modulating SOX9. Front. Cell Dev Biol 8, 604869. doi:10.3389/fcell.2020.604869
Sung, H., Ferlay, J., Siegel, R. L., Laversanne, M., Soerjomataram, I., Jemal, A., et al. (2021). Global Cancer Statistics 2020: GLOBOCAN Estimates of Incidence and Mortality Worldwide for 36 Cancers in 185 Countries. CA Cancer J. Clin. 71 (3), 209–249. doi:10.3322/caac.21660
Taguchi-Atarashi, N., Hamasaki, M., Matsunaga, K., Omori, H., Ktistakis, N. T., Yoshimori, T., et al. (2010). Modulation of Local PtdIns3P Levels by the PI Phosphatase MTMR3 Regulates Constitutive Autophagy. Traffic 11 (4), 468–478. doi:10.1111/j.1600-0854.2010.01034.x
Tang, H., Han, X., Li, M., Li, T., and Hao, Y. (2019). Linc00221 Modulates Cisplatin Resistance in Non-small-cell Lung Cancer via Sponging miR-519a. Biochimie 162, 134–143. doi:10.1016/j.biochi.2019.04.019
Tang, W., Yu, X., Zeng, R., and Chen, L. (2020). LncRNA-ATB Promotes Cisplatin Resistance in Lung Adenocarcinoma Cells by Targeting the miR-200a/β-Catenin Pathway. Cancer Manag. Res. 12, 2001–2014. doi:10.2147/CMAR.S240695
Tanida, S., Mizoshita, T., Ozeki, K., Tsukamoto, H., Kamiya, T., Kataoka, H., et al. (2012). Mechanisms of Cisplatin-Induced Apoptosis and of Cisplatin Sensitivity: Potential of BIN1 to Act as a Potent Predictor of Cisplatin Sensitivity in Gastric Cancer Treatment. Int. J. Surg. Oncol. 2012, 862879. doi:10.1155/2012/862879
Taylor, M. A., Parvani, J. G., and Schiemann, W. P. (2010). The Pathophysiology of Epithelial-Mesenchymal Transition Induced by Transforming Growth Factor-Beta in normal and Malignant Mammary Epithelial Cells. J. Mammary Gland Biol. Neoplasia 15 (2), 169–190. doi:10.1007/s10911-010-9181-1
Tian, L. J., Wu, Y. P., Wang, D., Zhou, Z. H., Xue, S. B., Zhang, D. Y., et al. (2019). Upregulation of Long Noncoding RNA (lncRNA) X-Inactive Specific Transcript (XIST) Is Associated with Cisplatin Resistance in Non-small Cell Lung Cancer (NSCLC) by Downregulating MicroRNA-144-3p. Med. Sci. Monit. 25, 8095–8104. doi:10.12659/MSM.916075
Usman, R. M., Razzaq, F., Akbar, A., Farooqui, A. A., Iftikhar, A., Latif, A., et al. (2021). Role and Mechanism of Autophagy-Regulating Factors in Tumorigenesis and Drug Resistance. Asia Pac. J. Clin. Oncol. 17 (3), 193–208. doi:10.1111/ajco.13449
Velma, V., Dasari, S. R., and Tchounwou, P. B. (2016). Low Doses of Cisplatin Induce Gene Alterations, Cell Cycle Arrest, and Apoptosis in Human Promyelocytic Leukemia Cells. Biomark Insights 11, 113–121. doi:10.4137/BMI.S39445
Virginie, B. L., Legault, L.-M., and McGraw, S. (2019). “Endocrine Epigenetics, Epigenetic Profiling and Biomarker Identification,” in Encyclopedia of Endocrine Diseases (Academic Press), 31–35. doi:10.1016/B978-0-12-801238-3.65830-0
Wang, C., Wang, C., Qiu, C., and Su, Z. (2020). Targeted Regulation of FoxO3a by miR-372 to Mediate Gastric Carcinoma Cell Apoptosis and DDP Drug Resistance. Cancer Biother. Radiopharm. 35 (10), 753–759. doi:10.1089/cbr.2019.3299
Wang, H., Chen, J., Zhang, S., Zheng, X., Xie, S., Mao, J., et al. (2020). MiR-223 Regulates Autophagy Associated with Cisplatin Resistance by Targeting FBXW7 in Human Non-small Cell Lung Cancer. Cancer Cell Int 20 (1), 258. doi:10.1186/s12935-020-01284-x
Wang, H., Guo, M., Ding, D., Yang, F., and Chen, Z. (2020). Long Non-coding RNA NNT-AS1 Contributes to Cisplatin Resistance via miR-1236-3p/ATG7 Axis in Lung Cancer Cells. Onco Targets Ther. 13, 3641–3652. doi:10.2147/OTT.S237576
Wang, H., Wang, L., Zhang, G., Lu, C., Chu, H., Yang, R., et al. (2018). MALAT1/miR-101-3p/MCL1 axis Mediates Cisplatin Resistance in Lung Cancer. Oncotarget 9 (7), 7501–7512. doi:10.18632/oncotarget.23483
Wang, L., Han, H., Dong, L., Wang, Z., and Qin, Y. (2021). Function of P21 and its Therapeutic Effects in Esophageal Cancer. Oncol. Lett. 21 (2), 136. doi:10.3892/ol.2020.12397
Wang, L., Shang, X., and Feng, Q. (2019). LncRNA TATDN1 Contributes to the Cisplatin Resistance of Non-small Cell Lung Cancer through TATDN1/miR-451/TRIM66 axis. Cancer Biol. Ther. 20 (3), 261–271. doi:10.1080/15384047.2018.1529091
Wang, M., Zhang, R., Zhang, S., Xu, R., and Yang, Q. (2019). MicroRNA-574-3p Regulates Epithelial Mesenchymal Transition and Cisplatin Resistance via Targeting ZEB1 in Human Gastric Carcinoma Cells. Gene 700, 110–119. doi:10.1016/j.gene.2019.03.043
Wang, Q., Liang, D., Shen, P., Yu, Y., Yan, Y., and You, W. (2021). Hsa_circ_0092276 Promotes Doxorubicin Resistance in Breast Cancer Cells by Regulating Autophagy via miR-348/ATG7 axis. Transl Oncol. 14 (8), 101045. doi:10.1016/j.tranon.2021.101045
Wang, W., Chen, L., Zhu, W., Huang, X., Lin, L., Quan, Z., et al. (2021). miR-4486 Reverses Cisplatin-Resistance of colon Cancer Cells via Targeting ATG7 to Inhibiting Autophagy. Exp. Ther. Med. 22 (6), 1465. doi:10.3892/etm.2021.10900
Wang, W., Yi, M., Chen, S., Li, J., Zhang, H., Xiong, W., et al. (2017). NOR1 Suppresses Cancer Stem-like Cells Properties of Tumor Cells via the Inhibition of the AKT-GSK-3β-Wnt/β-Catenin-Aldh1a1 Signal Circuit. J. Cell Physiol 232 (10), 2829–2840. doi:10.1002/jcp.25706
Wang, X., and Zhu, J. (2018). Mir-1307 Regulates Cisplatin Resistance by Targeting Mdm4 in Breast Cancer Expressing Wild Type P53. Thorac. Cancer 9 (6), 676–683. doi:10.1111/1759-7714.12607
Wang, Z., Wang, Q., Xu, G., Meng, N., Huang, X., Jiang, Z., et al. (2020). The Long Noncoding RNA CRAL Reverses Cisplatin Resistance via the miR-505/CYLD/AKT axis in Human Gastric Cancer Cells. RNA Biol. 17 (11), 1576–1589. doi:10.1080/15476286.2019.1709296
Wei, X., Gao, M., Ahmed, Y., Gao, M., Liu, W., Zhang, Y., et al. (2019). MicroRNA-362-5p Enhances the Cisplatin Sensitivity of Gastric Cancer Cells by Targeting Suppressor of Zeste 12 Protein. Oncol. Lett. 18 (2), 1607–1616. doi:10.3892/ol.2019.10496
Wei, Y., Wei, L., Li, J., Ma, Z., Zhang, Q., Han, Z., et al. (2020). SLCO4A1-AS1 Promotes Cell Growth and Induces Resistance in Lung Adenocarcinoma by Modulating miR-4701-5p/NFE2L1 axis to Activate WNT Pathway. Cancer Med. 9 (19), 7205–7217. doi:10.1002/cam4.3270
Weitz, J., Koch, M., Debus, J., Höhler, T., Galle, P. R., and Büchler, M. W. (2005). Colorectal Cancer. Lancet 365 (9454), 153–165. doi:10.1016/S0140-6736(05)17706-X
Wen, L., Zhang, X., Zhang, J., Chen, S., Ma, Y., Hu, J., et al. (2020). Paxillin Knockdown Suppresses Metastasis and Epithelial-mesenchymal T-ransition in C-olorectal C-ancer via the ERK S-ignalling P-athway. Oncol. Rep. 44 (3), 1105–1115. doi:10.3892/or.2020.7687
Wu, C., Hu, Y., Ning, Y., Zhao, A., Zhang, G., and Yan, L. (2020a). Long Noncoding RNA Plasmacytoma Variant Translocation 1 Regulates Cisplatin Resistance via miR-3619-5p/TBL1XR1 Axis in Gastric Cancer. Cancer Biother. Radiopharm. 35 (10), 741–752. doi:10.1089/cbr.2019.3342
Wu, H., Zhou, J., Mei, S., Wu, D., Mu, Z., Chen, B., et al. (2017). Circulating Exosomal microRNA-96 Promotes Cell Proliferation, Migration and Drug Resistance by Targeting LMO7. J. Cell Mol Med 21 (6), 1228–1236. doi:10.1111/jcmm.13056
Wu, L., Wan, S., Li, J., Xu, Y., Lou, X., Sun, M., et al. (2021). Expression and Prognostic Value of E2F3 Transcription Factor in Non-small Cell Lung Cancer. Oncol. Lett. 21 (5), 411. doi:10.3892/ol.2021.12672
Wu, L. L., Cai, W. P., Lei, X., Shi, K. Q., Lin, X. Y., and Shi, L. (2019). NRAL Mediates Cisplatin Resistance in Hepatocellular Carcinoma via miR-340-5p/Nrf2 axis. J. Cell Commun Signal 13 (1), 99–112. doi:10.1007/s12079-018-0479-x
Wu, S., Wang, H., Pan, Y., Yang, X., and Wu, D. (2020b). miR-140-3p Enhances Cisplatin Sensitivity and Attenuates Stem Cell-like Properties through Repressing Wnt/β-Catenin Signaling in Lung Adenocarcinoma Cells. Exp. Ther. Med. 20 (2), 1664–1674. doi:10.3892/etm.2020.8847
Wu, Y., Cheng, K., Liang, W., and Wang, X. (2020c). lncRNA RPPH1 Promotes Non-small Cell Lung Cancer Progression through the miR-326/WNT2B axis. Oncol. Lett. 20 (4), 105. doi:10.3892/ol.2020.11966
Xia, J. T., Chen, L. Z., Jian, W. H., Wang, K. B., Yang, Y. Z., He, W. L., et al. (2014). MicroRNA-362 Induces Cell Proliferation and Apoptosis Resistance in Gastric Cancer by Activation of NF-Κb Signaling. J. Transl Med. 12, 33. doi:10.1186/1479-5876-12-33
Xia, Y., Lv, J., Jiang, T., Li, B., Li, Y., He, Z., et al. (2021). CircFAM73A Promotes the Cancer Stem Cell-like Properties of Gastric Cancer through the miR-490-3p/HMGA2 Positive Feedback Loop and HNRNPK-Mediated β-catenin Stabilization. J. Exp. Clin. Cancer Res. 40 (1), 103. doi:10.1186/s13046-021-01896-9
Xiao, F., Ouyang, B., Zou, J., Yang, Y., Yi, L., and Yan, H. (2020). Trim14 Promotes Autophagy and Chemotherapy Resistance of Gastric Cancer Cells by Regulating AMPK/mTOR Pathway. Drug Dev. Res. 81 (5), 544–550. doi:10.1002/ddr.21650
Xiao, H., Liu, Y., Liang, P., Wang, B., Tan, H., Zhang, Y., et al. (2018). TP53TG1 Enhances Cisplatin Sensitivity of Non-small Cell Lung Cancer Cells through Regulating miR-18a/PTEN axis. Cell Biosci 8, 23. doi:10.1186/s13578-018-0221-7
Xiao, X. H., and He, S. Y. (2020). ELF1 Activated Long Non-coding RNA CASC2 Inhibits Cisplatin Resistance of Non-small Cell Lung Cancer via the miR-18a/IRF-2 Signaling Pathway. Eur. Rev. Med. Pharmacol. Sci. 24 (6), 3130–3142. doi:10.26355/eurrev_202003_20680
Xie, C., Subhash, V. V., Datta, A., Liem, N., Tan, S. H., Yeo, M. S., et al. (2016). Melanoma Associated Antigen (MAGE)-A3 Promotes Cell Proliferation and Chemotherapeutic Drug Resistance in Gastric Cancer. Cell Oncol (Dordr) 39 (2), 175–186. doi:10.1007/s13402-015-0261-5
Xie, D., Pei, Q., Li, J., Wan, X., and Ye, T. (2021). Emerging Role of E2F Family in Cancer Stem Cells. Front. Oncol. 11, 723137. doi:10.3389/fonc.2021.723137
Xie, X., Huang, N., Zhang, Y., Wei, X., Gao, M., Li, M., et al. (2019). MiR-192-5p Reverses Cisplatin Resistance by Targeting ERCC3 and ERCC4 in SGC7901/DDP Cells. J. Cancer 10 (4), 1039–1051. doi:10.7150/jca.25814
Xing, S., Qu, Y., Li, C., Huang, A., Tong, S., Wu, C., et al. (2019). Deregulation of lncRNA-AC078883.3 and microRNA-19a Is Involved in the Development of Chemoresistance to Cisplatin via Modulating Signaling Pathway of PTEN/AKT. J. Cell Physiol 234 (12), 22657–22665. doi:10.1002/jcp.28832
Yan, J., Dang, Y., Liu, S., Zhang, Y., and Zhang, G. (2016). LncRNA HOTAIR Promotes Cisplatin Resistance in Gastric Cancer by Targeting miR-126 to Activate the PI3K/AKT/MRP1 Genes. Tumour Biol. 37, 16345–16355. doi:10.1007/s13277-016-5448-5
Yang, S. M., Huang, C., Li, X. F., Yu, M. Z., He, Y., and Li, J. (2013). miR-21 Confers Cisplatin Resistance in Gastric Cancer Cells by Regulating PTEN. Toxicology 306, 162–168. doi:10.1016/j.tox.2013.02.014
Yang, W., Xiao, W., Cai, Z., Jin, S., and Li, T. (2020a). miR-1269b Drives Cisplatin Resistance of Human Non-small Cell Lung Cancer via Modulating the PTEN/PI3K/AKT Signaling Pathway. Onco Targets Ther. 13, 109–118. doi:10.2147/OTT.S225010
Yang, X., Meng, L., Zhong, Y., Hu, F., Wang, L., and Wang, M. (2021). The Long Intergenic Noncoding RNA GAS5 Reduces Cisplatin-Resistance in Non-small Cell Lung Cancer through the miR-217/LHPP axis. Aging (Albany NY) 13 (2), 2864–2884. doi:10.18632/aging.202352
Yang, X., Zhang, Q., and Guan, B. (2020b). Circ_0110805 Knockdown Enhances Cisplatin Sensitivity and Inhibits Gastric Cancer Progression by miR-299-3p/ENDOPDI Axis. Onco Targets Ther. 13, 11445–11457. doi:10.2147/OTT.S279563
Yang, Y., Zhang, P., Zhao, Y., Yang, J., Jiang, G., and Fan, J. (2016). Decreased MicroRNA-26a Expression Causes Cisplatin Resistance in Human Non-small Cell Lung Cancer. Cancer Biol. Ther. 17 (5), 515–525. doi:10.1080/15384047.2015.1095405
Yeung, F., Hoberg, J. E., Ramsey, C. S., Keller, M. D., Jones, D. R., Frye, R. A., et al. (2004). Modulation of NF-kappaB-dependent Transcription and Cell Survival by the SIRT1 Deacetylase. EMBO J. 23 (12), 2369–2380. doi:10.1038/sj.emboj.7600244
Yi, D., Xu, L., Wang, R., Lu, X., and Sang, J. (2019). miR-381 Overcomes Cisplatin Resistance in Breast Cancer by Targeting MDR1. Cell Biol Int 43 (1), 12–21. doi:10.1002/cbin.11071
Yo, Y. T., Lin, Y. W., Wang, Y. C., Balch, C., Huang, R. L., Chan, M. W., et al. (2012). Growth Inhibition of Ovarian Tumor-Initiating Cells by Niclosamide. Mol. Cancer Ther. 11 (8), 1703–1712. doi:10.1158/1535-7163.MCT-12-0002
Yu, S., Qin, X., Chen, T., Zhou, L., Xu, X., and Feng, J. (2017). MicroRNA-106b-5p Regulates Cisplatin Chemosensitivity by Targeting Polycystic Kidney Disease-2 in Non-small-cell Lung Cancer. Anticancer Drugs 28 (8), 852–860. doi:10.1097/CAD.0000000000000524
Yun, C. W., and Lee, S. H. (2018). The Roles of Autophagy in Cancer. Int. J. Mol. Sci. 19 (11). doi:10.3390/ijms19113466
Zachari, M., and Ganley, I. G. (2017). The Mammalian ULK1 Complex and Autophagy Initiation. Essays Biochem. 61 (6), 585–596. doi:10.1042/EBC20170021
Zeng, Z., Zhao, G., Zhu, H., Nie, L., He, L., Liu, J., et al. (2020). LncRNA FOXD3-AS1 Promoted Chemo-Resistance of NSCLC Cells via Directly Acting on miR-127-3p/MDM2 axis. Cancer Cell Int 20, 350. doi:10.1186/s12935-020-01402-9
Zhan, Y., Abuduwaili, K., Wang, X., Shen, Y., Nuerlan, S., and Liu, C. (2020). Knockdown of Long Non-coding RNA HOTAIR Suppresses Cisplatin Resistance, Cell Proliferation, Migration and Invasion of DDP-Resistant NSCLC Cells by Targeting miR-149-5p/Doublecortin-like Kinase 1 Axis. Cancer Manag. Res. 12, 7725–7737. doi:10.2147/CMAR.S246299
Zhang, H., Luo, Y., Xu, W., Li, K., and Liao, C. (2019). Silencing Long Intergenic Non-coding RNA 00707 Enhances Cisplatin Sensitivity in Cisplatin-Resistant Non-small-cell Lung Cancer Cells by Sponging miR-145. Oncol. Lett. 18 (6), 6261–6268. doi:10.3892/ol.2019.10959
Zhang, J., He, X., Ma, Y., Liu, Y., Shi, H., Guo, W., et al. (2015a). Overexpression of ROCK1 and ROCK2 Inhibits Human Laryngeal Squamous Cell Carcinoma. Int. J. Clin. Exp. Pathol. 8 (1), 244–251.
Zhang, P., Sun, Y., and Ma, L. (2015b). ZEB1: at the Crossroads of Epithelial-Mesenchymal Transition, Metastasis and Therapy Resistance. Cell Cycle 14 (4), 481–487. doi:10.1080/15384101.2015.1006048
Zhang, P. F., Sheng, L. L., Wang, G., Tian, M., Zhu, L. Y., Zhang, R., et al. (2016). miR-363 Promotes Proliferation and Chemo-Resistance of Human Gastric Cancer via Targeting of FBW7 Ubiquitin Ligase Expression. Oncotarget 7 (23), 35284–35292. doi:10.18632/oncotarget.9169
Zhang, Q., Miao, Y., Fu, Q., Hu, H., Chen, H., Zeng, A., et al. (2020a). CircRNACCDC66 Regulates Cisplatin Resistance in Gastric Cancer via the miR-618/BCL2 axis. Biochem. Biophys. Res. Commun. 526 (3), 713–720. doi:10.1016/j.bbrc.2020.03.156
Zhang, Q., Zhang, B., Sun, L., Yan, Q., Zhang, Y., Zhang, Z., et al. (2018). MicroRNA-130b Targets PTEN to Induce Resistance to Cisplatin in Lung Cancer Cells by Activating Wnt/β-Catenin Pathway. Cell Biochem Funct 36 (4), 194–202. doi:10.1002/cbf.3331
Zhang, W., and Luo, P. (2018). MicroRNA-29c Restores Cisplatin Sensitivity in Liver Cancer through Direct Inhibition of Sirtuin 1 Expression. Oncol. Lett. 16 (2), 1543–1550. doi:10.3892/ol.2018.8801
Zhang, W., Wang, Z., Cai, G., and Huang, P. (2021). Downregulation of Circ_0071589 Suppresses Cisplatin Resistance in Colorectal Cancer by Regulating the MiR-526b-3p/KLF12 Axis. Cancer Manag. Res. 13, 2717–2731. doi:10.2147/CMAR.S294880
Zhang, X., Gu, G., Li, X., and Zhang, C. (2020b). Lidocaine Alleviates Cisplatin Resistance and Inhibits Migration of MGC-803/DDP Cells through Decreasing miR-10b. Cell Cycle 19 (19), 2530–2537. doi:10.1080/15384101.2020.1809914
Zhao, R., Zhang, X., Zhang, Y., Zhang, Y., Yang, Y., Sun, Y., et al. (2020a). HOTTIP Predicts Poor Survival in Gastric Cancer Patients and Contributes to Cisplatin Resistance by Sponging miR-216a-5p. Front. Cell Dev Biol 8, 348. doi:10.3389/fcell.2020.00348
Zhao, W., Li, H., Yang, S., Guo, D., Chen, J., Miao, S., et al. (2019). MicroRNA-152 Suppresses Cisplatin Resistance in A549 Cells. Oncol. Lett. 18 (5), 4613–4620. doi:10.3892/ol.2019.10834
Zhao, X., Li, X., Zhou, L., Ni, J., Yan, W., Ma, R., et al. (2018). LncRNA HOXA11-AS Drives Cisplatin Resistance of Human LUAD Cells via Modulating miR-454-3p/Stat3. Cancer Sci. 109 (10), 3068–3079. doi:10.1111/cas.13764
Zhao, Y., Zheng, R., Chen, J., and Ning, D. (2020b). CircRNA CDR1as/miR-641/HOXA9 Pathway Regulated Stemness Contributes to Cisplatin Resistance in Non-small Cell Lung Cancer (NSCLC). Cancer Cell Int 20, 289. doi:10.1186/s12935-020-01390-w
Zhao, Z., Liu, J., Wang, C., Wang, Y., Jiang, Y., and Guo, M. (2014). MicroRNA-25 Regulates Small Cell Lung Cancer Cell Development and Cell Cycle through Cyclin E2. Int. J. Clin. Exp. Pathol. 7 (11), 7726–7734.
Zhao, Z., Zhang, L., Yao, Q., and Tao, Z. (2015). miR-15b Regulates Cisplatin Resistance and Metastasis by Targeting PEBP4 in Human Lung Adenocarcinoma Cells. Cancer Gene Ther. 22 (3), 108–114. doi:10.1038/cgt.2014.73
Zheng, H., Wang, J. J., Yang, X. R., and Yu, Y. L. (2020). Upregulation of miR-34c after Silencing E2F Transcription Factor 1 Inhibits Paclitaxel Combined with Cisplatin Resistance in Gastric Cancer Cells. World J. Gastroenterol. 26 (5), 499–513. doi:10.3748/wjg.v26.i5.499
Zheng, H., Zhang, M., Ke, X., Deng, X., Li, D., Wang, Q., et al. (2021). LncRNA XIST/miR-137 axis Strengthens Chemo-Resistance and Glycolysis of Colorectal Cancer Cells by Hindering Transformation from PKM2 to PKM1. Cancer Biomark 30 (4), 395–406. doi:10.3233/CBM-201740
Zheng, J., Li, X., Cai, C., Hong, C., and Zhang, B. (2021). MicroRNA-32 and MicroRNA-548a Promote the Drug Sensitivity of Non-small Cell Lung Cancer Cells to Cisplatin by Targeting ROBO1 and Inhibiting the Activation of Wnt/β-Catenin Axis. Cancer Manag. Res. 13, 3005–3016. doi:10.2147/CMAR.S295003
Zheng, P., Chen, L., Yuan, X., Luo, Q., Liu, Y., Xie, G., et al. (2017). Exosomal Transfer of Tumor-Associated Macrophage-Derived miR-21 Confers Cisplatin Resistance in Gastric Cancer Cells. J. Exp. Clin. Cancer Res. 36 (1), 53. doi:10.1186/s13046-017-0528-y
Zheng, Z. H., You, H. Y., Feng, Y. J., and Zhang, Z. T. (2021). LncRNA KCNQ1OT1 Is a Key Factor in the Reversal Effect of Curcumin on Cisplatin Resistance in the Colorectal Cancer Cells. Mol. Cell Biochem 476 (7), 2575–2585. doi:10.1007/s11010-020-03856-x
Zhou, D., Gu, J., Wang, Y., Wu, H., Cheng, W., Wang, Q., et al. (2021). Long Non-coding RNA NEAT1 Transported by Extracellular Vesicles Contributes to Breast Cancer Development by Sponging microRNA-141-3p and Regulating KLF12. Cell Biosci 11 (1), 68. doi:10.1186/s13578-021-00556-x
Zhou, J., and Chen, Q. (2019). Poor Expression of microRNA-135b Results in the Inhibition of Cisplatin Resistance and Proliferation and Induces the Apoptosis of Gastric Cancer Cells through MST1-Mediated MAPK Signaling Pathway. FASEB J. 33 (3), 3420–3436. doi:10.1096/fj.201800618RRR
Zhou, J. J. H. F. W. S. Y. F. L. (2020). Reversal of Drug Resistance in Gastric Cancer Cells by miR-503 and its Mechanism. Acta Med. Mediterranea 36, 1841–1848. doi:10.19193/0393-6384_2020_3_289
Zhu, F., Niu, R., Shao, X., and Shao, X. (2021). FGD5-AS1 P-romotes C-isplatin R-esistance of H-uman L-ung A-denocarcinoma C-ell via the miR-142-5p/PD-L1 axis. Int. J. Mol. Med. 47 (2), 523–532. doi:10.3892/ijmm.2020.4816
Zhu, H., Yang, J., and Yang, S. (2020a). MicroRNA-103a-3p Potentiates Chemoresistance to Cisplatin in Non-small Cell Lung Carcinoma by Targeting Neurofibromatosis 1. Exp. Ther. Med. 19 (3), 1797–1805. doi:10.3892/etm.2020.8418
Zhu, Q. L., Li, Z., Lv, C. M., and Wang, W. (2019). MiR-187 Influences Cisplatin-Resistance of Gastric Cancer Cells through Regulating the TGF-β/Smad Signaling Pathway. Eur. Rev. Med. Pharmacol. Sci. 23 (22), 9907–9914. doi:10.26355/eurrev_201911_19556
Zhu, W., Shan, X., Wang, T., Shu, Y., and Liu, P. (2010). miR-181b Modulates Multidrug Resistance by Targeting BCL2 in Human Cancer Cell Lines. Int. J. Cancer 127 (11), 2520–2529. doi:10.1002/ijc.25260
Zhu, W., Xu, H., Zhu, D., Zhi, H., Wang, T., Wang, J., et al. (2012a). miR-200bc/429 Cluster Modulates Multidrug Resistance of Human Cancer Cell Lines by Targeting BCL2 and XIAP. Cancer Chemother. Pharmacol. 69 (3), 723–731. doi:10.1007/s00280-011-1752-3
Zhu, W., Zhu, D., Lu, S., Wang, T., Wang, J., Jiang, B., et al. (2012b). miR-497 Modulates Multidrug Resistance of Human Cancer Cell Lines by Targeting BCL2. Med. Oncol. 29 (1), 384–391. doi:10.1007/s12032-010-9797-4
Zhu, X., Han, J., Lan, H., Lin, Q., Wang, Y., and Sun, X. (2020b). A Novel Circular RNA hsa_circRNA_103809/miR-377-3p/GOT1 Pathway Regulates Cisplatin-Resistance in Non-small Cell Lung Cancer (NSCLC). BMC Cancer 20 (1), 1190. doi:10.1186/s12885-020-07680-w
Zhu, X., Zou, S., Zhou, J., Zhu, H., Zhang, S., Shang, Z., et al. (2016). REV3L, the Catalytic Subunit of DNA Polymerase ζ, Is Involved in the Progression and Chemoresistance of Esophageal Squamous Cell Carcinoma. Oncol. Rep. 35 (3), 1664–1670. doi:10.3892/or.2016.4549
Zhu, Z., Mu, Y., Qi, C., Wang, J., Xi, G., Guo, J., et al. (2015). CYP1B1 Enhances the Resistance of Epithelial Ovarian Cancer Cells to Paclitaxel In Vivo and In Vitro. Int. J. Mol. Med. 35 (2), 340–348. doi:10.3892/ijmm.2014.2041
Zou, Z., Wu, L., Ding, H., Wang, Y., Zhang, Y., Chen, X., et al. (2012). MicroRNA-30a Sensitizes Tumor Cells to Cis-Platinum via Suppressing Beclin 1-mediated Autophagy. J. Biol. Chem. 287 (6), 4148–4156. doi:10.1074/jbc.M111.307405
Keywords: microRNA, drug-resistance, cisplatin, sensitivity, cancer
Citation: Loren P, Saavedra N, Saavedra K, De Godoy Torso N, Visacri MB, Moriel P and Salazar LA (2022) Contribution of MicroRNAs in Chemoresistance to Cisplatin in the Top Five Deadliest Cancer: An Updated Review. Front. Pharmacol. 13:831099. doi: 10.3389/fphar.2022.831099
Received: 08 December 2021; Accepted: 16 March 2022;
Published: 04 April 2022.
Edited by:
Mohamed I. Husseiny Elsayed, Beckman Research Institute of City of Hope, United StatesReviewed by:
Juan Du, The Chinese University of Hong Kong, Shenzhen, ChinaMagdi Elsallab, University of Southern California, United States
Copyright © 2022 Loren, Saavedra, Saavedra, De Godoy Torso, Visacri, Moriel and Salazar. This is an open-access article distributed under the terms of the Creative Commons Attribution License (CC BY). The use, distribution or reproduction in other forums is permitted, provided the original author(s) and the copyright owner(s) are credited and that the original publication in this journal is cited, in accordance with accepted academic practice. No use, distribution or reproduction is permitted which does not comply with these terms.
*Correspondence: Luis A. Salazar, bHVpcy5zYWxhemFyQHVmcm9udGVyYS5jbA==