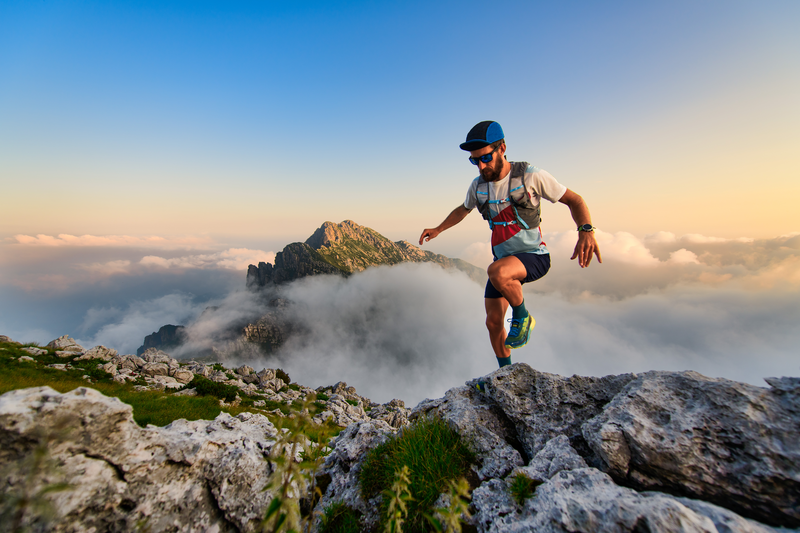
94% of researchers rate our articles as excellent or good
Learn more about the work of our research integrity team to safeguard the quality of each article we publish.
Find out more
ORIGINAL RESEARCH article
Front. Pharmacol. , 22 February 2022
Sec. Ethnopharmacology
Volume 13 - 2022 | https://doi.org/10.3389/fphar.2022.827733
This article is part of the Research Topic Therapeutic Effects of Herbal Medicines: How Can We Best Investigate Bioactive Metabolites? View all 19 articles
Si-Miao-Yong-An-Tang (SMYAT) is a classic prescription for the treatment of thromboangiitis obliterans (TAO). However, the effect and mechanism are still unclear. This experiment aims to evaluate the therapeutic effect and mechanism of SMYAT on sodium laurate solution induced thromboangiitis obliterans model rats using urine metabolomics. The therapeutic effect of SMYAT was evaluated by histopathology, hemorheology and other indexes. The urine metabolomic method, principal component analysis (PCA) and orthogonal partial least squares discriminant analysis (OPLS-DA) were used for clustering group and discriminant analysis to screen urine differential metabolic biomarkers, and explore new insight into pathophysiological mechanisms of SMYAT in the treatment of TAO. SMYAT has significant antithrombotic and anti-inflammatory effects, according to the results of urine metabolomic analysis, and regulate the metabolic profile of TAO rats, and its return profile is close to the state of control group. Through metabolomics technology, a total of 35 urine biomarkers of TAO model were characterized. Among them, SMYAT treatment can regulate 22 core biomarkers, such as normetanephrine and 4-pyridoxic acid. It is found that the therapeutic effect of SMYAT is closely related to the tyrosine metabolism, vitamin B6 metabolism and cysteine and methionine metabolism. It preliminarily explored the therapeutic mechanism of SMYAT, and provided a scientific basis for the application of SMYAT.
Traditional Chinese medicine (TCM) is widely used in China, and its curative effect is remarkable (Wang et al., 2011; Zhang et al., 2013; Guo et al., 2020). However, due to the lack of modern scientific evidence to prove the use of TCM, the mechanism of action is unclear, leading to the therapeutic effect of TCM did not play an original potential. Metabolomics is an innovative method in modern research and it can find biomarkers and clarify biochemical pathways, thereby improving diagnosis and treatment (Zhang et al., 2012a; Liang et al., 2015a; Liang et al., 2016b). The analysis of key biomarkers and the monitoring of metabolic pathways are, in analytical biology, an important means of physical state (Zhang et al., 2012b). Its purpose is to measure a wide range of small molecules under physiological stimulation or disease state (Roberts et al., 2012; Filla and Edwards, 2016). Due to the high sensitivity of metabolomics, it can detect subtle changes in metabolic pathways to explore the underlying mechanism of disease (Johnson et al., 2016). At present, metabolomics has been increasingly used to identify biomarkers in diseases (Liang et al., 2014; Liang et al., 2015b), and is considered a very powerful tool.
Metabolomics analysis based on mass spectrometry provides information on the metabolic pathways in the development of thromboangiitis obliterans disease, which has a certain reference value for studying the potential mechanisms of treatment strategies. Metabolomics can provide metabolic fingerprints of organisms, which can be used to study the mechanism of disease (Zhang et al., 2015; Zhang et al., 2019 A.-H.). It can explore metabolites and body pathology through high-throughput analysis of metabolites in organisms and explore the correlation between metabolites and the pathophysiological changes of the body (Li et al., 2018; Zhang et al., 2016; Zhang et al., 2019 AH.), and clarifies the interaction of complex systems in the body (Liang et al., 2016a; Liang et al., 2017; Zhang et al., 2018). It can btain corresponding biomarkers through metabolomics technology to characterize or reveal the functional state of a specific time or environment (Zhang et al., 2017; 2020). For example, by establishing a powerful metabolomics strategy to explain the pathological changes of Yanghuang syndrome and the therapeutic effect of TCM, and the Yin-Chen-Hao-Tang was used to achieve significant results (Liu X.-y. et al., 2018). Through metabolomics, the mechanism of Gancao Fuzi decoction achieved good results in the treatment of rheumatoid arthritis in rats by regulating various metabolic pathways (Liu Y. et al., 2018). Using the metabolomics method, the effect of Angelica sinensis on urinary metabolites in blood deficiency in mice was studied and the mechanism of blood tonifying was explored. It was found that Angelica sinensis could regulate a variety of metabolic pathways and exert a curative effect (Wang et al., 2016). In our research, the potential mechanism of SMYAT in the treatment of thromboangiitis obliterans through multi-component, multi-target, and multi-channel regulation of body function is explored by metabolomics. The study of metabolomics and related pathways will help us to increase our understanding of pathophysiology and mechanisms of disease (Laíns et al., 2019). Moreover, urine is one of the important samples for metabolome research (Zhang et al., 2012c; Harpole et al., 2016), which contains a variety of endogenous small molecule metabolites and can well reflect the changes of the body. As a very attractive sample in metabolomics research, it has several advantages, such as low protein content and simple pretreatment (Khamis et al., 2017).
Thromboangiitis obliterans (TAO) is a staged inflammatory disease and mainly occurs in the arteries, veins, and nerve parts of the limbs (Quintas and Albuquerque, 2008; Piazza and Creager, 2010; Highlander et al., 2011). The clinical symptoms are mainly manifested in the following conditions: limb ischemia; cold; severe pain limp, weakening or disappearance of arterial pulsation; gangrene, etc. Serious cases can endanger limbs and life and . the exact pathogenesis and etiology of the disease are not clear (Fazeli and Ravari, 2015; Li et al., 2020). In this experiment, a rat model of thromboangiitis obliterans was induced by an injection of sodium laurate solution. Sodium laurate has a strong endothelial damage effect and can cause the vascular endothelium to fall off. It is the most commonly used method of modeling (Munnix et al., 2009; Asada et al., 2018; Liu et al., 2019; Wang et al., 2021). Existing models mostly evaluate the success of model preparation by observing changes in apparent behavior and the degree of gangrene, hemorheology, and histopathology are the most commonly used detection indicators. In our research, hemorheology and histopathology test results support the successful model building. The SMYAT is included in《YAN-FANG-XIN-BIAN》 (Li et al., 2013; Yang et al., 2020), and is a famous classic prescription in ancient China, which is composed of four kinds of traditional Chinese medicines, namely Flos Lonicerae Japonicae [Lonicera japonica Thunb. (Jinyinhua), Caprifoliaceae], Radix Scrophulariae Ningpoensis [Scrophularia ningpoensis Hemsl. (Xuanshen) Scrophulariaceae], Radix Scrophulariae Ningpoensis [Angelica sinensis (Oliv.) Diels (Danggui) Apiaceae], and Radix Glycyrrhizae Uralensis [Glycyrrhiza uralensis Fisch. (Gancao) Leguminosae]. (Peng et al., 2012; Liu et al., 2017; Zhong et al., 2017), which contain terpenoids, flavonoids, phenylpropanoid, and organic acids, which can achieve anti-inflammatory, anti-oxidation stress, regulating blood lipid, inhibiting thrombosis and improving hemorheology, etc. (Zheng et al., 2019). It has the function of clearing heat and detoxifying poison. It is commonly used in the treatment of thromboangitis obliterans (Zhao et al., 2020). In our experiment, we analyzed the chemical components in the sample of Si-Miao-Yong-An-Tang, and a total of 79 chemical components were characterized. The relevant chromatograms and specific information tables of the compounds are shown in Supplementary Material S1. This study determined urine biomarkers of TAO model rats, which were used to distinguish between the control group and the TAO model groupThe biomarkers are expected to be used as therapeutic targets and to regulate relevant monitoring and treatment biomarkers when using SMYAT.
Acquity™ UPLC liquid chromatography (Waters, USA); Synapt™ G2-Si mass spectrometry (Waters, USA); Masslynx v4.1 workstation (Waters, USA); Ultrasonic cleaner (KQ-250 DB, Kunshan Ultrasonic Instrument Co., Ltd., China); Table centrifuge (sorvall ST 16R, Thermo Scientific, USA); Thermo Scientific 995 ultra low temperature refrigerator (Thermo Scientific, USA).
All herbs were obtained from Harbin No.4 Chinese Medicine Factory Co., Ltd. Flos Lonicerae Japonicae (Lot number: JL030-180401), Radix Scrophulariae Ningpoensis (Lot number: JL031-180601), Radix Angelicae Sinensis (Lot number: JL048-180601) and Radix Glycyrrhizae Uralensis (Lot number: JL054-180501) were weighed out according to the ratio 3:3:2:1 and soaked in distilled water for 30 min and boiled for 1 h. The solution was filtered and freeze-dried under vacuum to obtain a loose powder and freeze-dried powder was dissolved in water and prepared in 8.2 g/kg solution for oral gavage administration to rats. In addition, we carried out quality control on the sample of Si-Miao-Yong-An-Tang. For details, please refer to the Supplementary Material S2.
Mai-Luo-Ning Granules (MLN) are provided by Jiangxi Yintao Pharmaceutical Co., Ltd. (Lot number:1808025). Sodium Laurate are provided by Sinopharm Chemical Reagent Co., Ltd. (Lot number:20171224). Methanol and acetonitrile (HPLC grade) were purchased from Fisher Scientific Corporation (Fisher, United States); leucine enkephalin was purchased from Sigma-Aldrich (SIGMA, United States); formic acid (HPLC grade) was purchased from Aladdin Industrial Corporation (Shanghai, China); sodium chloride injection was purchased from Kelun Pharmaceutical Co., Ltd., (Sichuan, China); all other reagents were HPLC grade.
Sixty male SD rats with the weight of (280–320) g were purchased from the Liaoning Changsheng Biotechnology Co., Ltd. [Permit number: SCXK (Liao) 2015-0001]. Those rats were exposed to a temperature of 24 ± 2°C and the humidity of 40 ± 5%, the animals were reared in separate cages, all rats were adaptive fed for 7 days and then randomly divided into four groups: Control group (n = 20), Model group (n = 20), SMYAT group (n = 10), and MLN group (n = 10). Rats in model group, SMYAT group and MLN group were injected with a sodium laurate solution to induce thromboangiitis obliterans model, while rats in control group were injected with the same amount of normal saline. After 10 days, the rats in SMYAT group (rats were fed with SMYAT 8.2 g/kg per day for 15 days) and MLN group (rats were fed with MLN 2.7 g/kg per day for 15 days) were given medicine treatment. The rats in control group and thromboangiitis obliterans model group were given pure water. This research was approved by the Ethical Committee of Heilongjiang University of Chinese Medicine and was conducted according to the principles expressed in the Declaration of Helsinki.
Control group and model group of urine samples were collected at 10th day of the experiment. Accompanying control group, accompanying model group, SMYAT group and MLN group of urine samples were collected at 25th day of experiment and then centrifuged with of 13,000 rpm/min in 4°C for 10 min to obtain urine samples. Before UPLC-MS analysis, the urine supernatant was stored at −80°C.
Before analysis, the urine samples were thawed in an ice bath, 500 μL of urine was taken and added to 500 μL of ultra-pure water, placed in a vortex mixer for 30 s, centrifuged at 13,000 rpm for 15 min at 4°C; and filtered over 0.22 μm, then the supernatant were injected into the UPLC-MS.
The accompanying control group, accompanying model group, SMYAT group and MLN group of blood samples were collected from the abdominal aorta of rats (on the 25th day of the experiment, 1 h after the last administration). Sodium citrate and EDTA-K2 were used for anticoagulation. The whole blood viscosity and plasma viscosity and other indexes of rats in each group were determined. In each group, the femoral artery tissues below 2–3 cm and around the artery were taken from the affected limb. After washing with normal saline, the femoral artery was fixed with 10% formalin solution for 24 h for histopathological observation.
Chromatograph condition: Acquity™ UPLC liquid chromatography (Waters, USA); chromatographic column used was an ACQUITY HSS T3 chromatographic column (100 mm × 2.1 mm i.d., 1.8 μm. Waters, USA); the column temperature is set at 40°C; the sample bin temperature is set at 4°C; meanwhile mobile phase for gradient elution consist of phase A (acetonitrile containing 0.1% formic acid) and phase B (water with 0.1% formic acid). The gradient was as follows: 0–1 min, 1%A; 1–6 min, 1–30%A; 6–8 min, 30–70%A; 8–9 min, 70–99%A; 9–10 min, 99%A; 10–10.1 min, 99–1%A; 10.1–14 min, 1%A. In addition, equal volume of each sample was mixed into a quality control sample (QC) for monitoring the stability of the instrument in the experiment, and one QC solution was run every ten samples, which were crucial for optimization during metabolomic analytical method development.
Synapt TM G2-Si mass spectrometer (Waters, USA). MS system using ESI as an ion source to operate in ESI+ and ESI-modes with a scanning range of 50–1,200 m/z. The desolvation temperature was 400°C, the source temperature was 110°C, desolvation gas flow was 800 L/h, and cone gas flow was 50 L/h. Sampling cone voltage was maintained at 20 V; Leucine enkaphalin was used as the reference compound {positive ion mode [(M + H)+ = 556.2771] and (M−H)− = 554.2615}. All the above parameters are set using Masslynx v4.1 workstation (waters, United States).
In order to more accurately find the urine biomarkers in TAO model rats original urine files were imported into Progensis QI software for analysis and processing, andpeak matching and normalization for each peak was performed. Meanwhile, we used Ezinfo 3.0 software, including PCA and OPLS-DA. SPSS 22.0 for Windows was used for the statistical analysis. The variable importance for the projection (VIP) value >2 in OPLS-DA, and p < 0.05 for Students t-test were selected as potential urine biomarkers.
The identification of biomarkers is to lock the Rt_m/z of important metabolite ions to help confirm the molecular mass of metabolites and perform elemental composition analysis to determine possible chemical formulas. Based on the possible chemical formulas, accurate masses and secondary mass spectrometry data (MS/MS) of ions, the Human Metabolome Database (HMDB) and Metaboanalyst were used to match the mass spectrometry information. The MassFragment application manager was used to facilitate the chemically intelligent peak matching algorithms MS/MS fragment ion analysis process and to determine the final biomarker. The results of the secondary ion fragmentation information matching of these 35 compounds are shown in Supplementary Material S3.
The urine datasets collected on the 10th day were further subjected to multivariate statistical analysis using EZinfo 3.0 software. The PCA score plots (Figure 1A) showed an obvious separation between the TAO model and control model. The OPLS-DA was employed to find the potential biomarkers (Figure 1B). The R2Y and Q2 values of the model [R2Y (cum) = 0.991607, Q2 (cum) = 0.905193 in positive mode and R2Y (cum) = 0.993259, Q2 (cum) = 0.942307] in negative mode indicated that the models have good quality and predictability. The OPLS-DA score plots showed that there was a significant difference between the TAO model groups and control groups, suggesting that the TAO model group had obvious urinary biochemical disorders and its metabolic profile had changed significantly. Screen out potential urine markers, and by using related databases, such as HMDB, KEGG, etc. Meanwhile, we combined the fragment information of the secondary mass spectrometer to obtain the chemical information of potential biomarkers, see Supplementary Table S1 for details. The content of these potential biomarkers in the 10th day model group and the 10th day control group were statistically analyzed (Figure 2). We constructed a PLS-DA model with the obtained differential metabolic markers (Figure 3A). Figure 3B showed top significant features of the metabolite markers based the VIP projection. Correlation analysis of differential metabolites are marked on the hierarchical clustering plot (Figure 3C), and the obtained differential metabolic markers are analyzed by heat mapwith results shown in Figure 3D.
FIGURE 1. Metabolomic profiling of TAO. PCA model results for TAO model group in positive mode (A); OPLS-DA model results for TAO model group in positive mode (B); Black spot represents the control group and red spot represents the model group.
FIGURE 2. Change trend of biomarker content in control group and model group. *Significant difference between the control group and model group (p < 0.05). **Very significant difference between the control group and model group (p < 0.01).
FIGURE 3. Systems analysis of metabolomic alterations of the model and control samples with MetaboAnalyst’s data annotation tools: (A) PLS-plot revealed differences between the two groups. (B) Correlation analysis of the 10 differential metabolites are marked on the plot. Three clusters were identified representing the different groups of metabolites. Top significant features of the metabolite markers based the VIP projection (B). (C) Hierarchical clustering of the differential metabolites. (D) Heatmap visualization constructed based on the differential metabolites of importance for the urine of model. The heatmaps were constructed based on the potential candidates of importance, which were extracted with OPLS-DA analysis. Rows: metabolites; columns: samples; Variable differences are revealed between the control and model groups.
MetPA (Metabolomics Pathway Analysis) is used to analyze metabolic pathways in metabolomics data. MetPA analysis was performed on the characterized 35 biomarkers related to thromboangiitis obliterans model, and 17 related metabolic pathways were obtained including vitamin B6 metabolism, cysteine and methionine metabolism, tyrosine metabolism, phenylalanine metabolism, and so forth. The results show that these endogenous metabolites are closely related to the thromboangiitis obliterans model. The metabolic pathway is shown in Figure 4.
FIGURE 4. Main metabolic pathways of potential biomarkers. (1) beta-Alanine metabolism; (2) Phenylalanine metabolism; (3) Histidine metabolism; (4) Cysteine and methionine metabolism; (5) Citrate cycle (TCA cycle); (6) Steroid hormone biosynthesis; (7) Pantothenate and CoA biosynthesis; (8) Glyoxylate and dicarboxylate metabolism; (9) Tyrosine metabolism; (10) Tryptophan metabolism; (11) Vitamin B6 metabolism; (12) Ascorbate and aldarate metabolism; (13) Propanoate metabolism; (14) Glutathione metabolism; (15) Arginine and proline metabolism; (16) Pyrimidine metabolism; (17) Aminoacyl-tRNA biosynthesis.
Rats in each group were in good condition, lively and active prior to the start of the experiment. After modeling, except for control group, the other groups of rats showed obvious ischemic symptoms, such as lower skin temperature, obvious swelling of foot and paw, weakened arterial pulsation, limping and dragging walking in varying degrees. On the 10th–25th day, the condition of the affected limbs of the rats in each treatment group was gradually improved, among them, the SMYAT group had a significant effect, the skin temperature returned to normal, the swelling of the paws subsided, and the arterial pulsation recovered, while the state of the rats in the following model group remained the same as that after modeling, and there was no improvement trend.
The results in Figure 5A, show low shear whole blood viscosity and that the blood plasma viscosity of the thromboangiitis obliterans model rats had changed significantly compared with the control group. After treatment with SMYAT, all the indexes had a significant decrease, which showed that SMYAT had a good effect on regulating blood viscosity and treating thrombosis.
FIGURE 5. (A) Effects of administration on plasma viscosity and whole blood viscosity of rats in each group; (B) Effects of administration on related indexes of red blood cells of rats in each group; *p < 0.05, **p < 0.01 compared with control group; #p < 0.05, ##p < 0.01 compared with model group.
The results in Figure 5B, show the hematocrit, erythrocyte aggregation index and erythrocyte rigidity index of the thromboangiitis obliterans model rats changed significantly compared with the control group. After treatment with SMYAT, the erythrocyte rigidity index, erythrocyte aggregation index and erythrocyte deformation index have been significantly adjusted, indicating that SMYAT has a significant regulatory effect on red blood cells and other indicators, and can achieve good therapeutic effects.
We evaluated the effect of SMYAT on the histopathology of TAO model rats (Figure 6), and observation of rat femoral artery sections showed that there were no pathological changes in the control group, the vascular endothelium was smooth and orderly, and the endothelial cells were neatly arranged (Figure 6A). The femoral artery sections in the TAO model group found obvious pathological changes, such as vascular thinning, endothelial cell deformation, vascular endothelial cells and smooth muscle cells disorderly arranged, and a large number of inflammatory cell infiltration (Figure 6B). In the SMYAT treatment group, the degree of femoral artery lesions was improved, the deformation of endothelial cells was improved, and the arrangement of vascular endothelial cells and smooth muscle cells was restored, and the injure condition was restored and maintained a stable state (Figure 6C). In MLN group, the femoral artery became slightly thinner, intimal peeling phenomenon relieved, and the inflammatory cell infiltration in the blood vessel wall and surrounding tissues gradually reduced (Figure 6D).
FIGURE 6. Femoral artery sections of rats in each group on the 25th day of the experiment (×400); (A): control group; (B): model group; (C): SMYAT group; (D): MLN group.
The PCA was carried out on the urine data by collected on the 25th day from the four different groups, and the plots obtained (Figure 7), show that there are obvious clusters between each group of data, they are distributed in different positions, especially between the control group and the TAO model group which are far away and can be distinguished significantly. The position of the SMYAT treatment group gradually approached the control groupwhich shows that SMYAT could improve the abnormal metabolic network of TAO model rats.
FIGURE 7. The PCA score plot of control, model, SMYAT, and MLN groups in urine metabolism profile. (A) Positive ion mode and (B) negative ion mode. Black spot represents the control group, red spot represents the model group, green spot represents the SMYAT group and blue spot represents the MLN group.
On the 25th day of the experiment, the regulation of biomarkers in rats with thromboangiitis obliterans model by SMYAT was analyzed, among the 35 potential biomarkers that were characterized, SMYAT could be adjusted to 22 biomarkers; respectively including pyranuronic acid, DL-2-aminooctanoic acid, imidazolepropionic acid, L-prolyl-L-prolin, 4-pyridoxic acid, normetanephrine, 2-indolecarboxylic acid, S-[2- (carboxymethyl)-2, 5-dihydroxy-3-cyclohexen-1-yl]cysteine, 3-methylene-indolenine, 4-ethylphenylsulfate, coproporphyrin, hydroxyprolyl-leucine, N-methylnicotinamide, beta-alanine, 3-indole carboxylic acid glucuronide, urocanic acid, phenylacetaldehyde, cis-aconitic acid, estrone glucuronide, 5-methoxyindoleacetate, 11b-hydroxyprogesterone, and L-methionine (Figure 8).
FIGURE 8. *Significant difference between the control group and model group (p < 0.05). **Very significant difference between the control group and model group (p < 0.01). # Significant difference between SMYAT and model groups, p < 0.05. ## Very significant difference compared between SMYAT and model groups, p < 0.01.
Based on the HMDB, KEGG and the metaboanalyst platform, the metabolic network of SMYAT on thromboangiitis obliterans rats was mapped to clarify its potential mechanism (Figure 9).
Thromboangiitis obliterans is a common vascular disease in clinics, which mainly affects small and medium-sized arteries and veins (Klein-Weigel et al., 2015). Amputation is needed in severe cases. Because its exact pathogenesis and etiology are not clear, there is no radical treatment, causing great pain to patients, but the research on it has not stopped. In this study, metabolomics provides a powerful approach to analyze the urine of TAO model rats and control group rats to study the difference of endogenous small molecule metabolic profiles. On this basis, we found the biomarkers and metabolic pathways regulated by SMYAT, so as to provide ideas for the research on the mechanism of SMYAT in the treatment of thromboangiitis obliterans. In this study, a total of 35 biomarkers of thromboangiitis obliterans model were characterized. In the visualized thermogram of the biomarkers of the thromboangiitis obliterans model, it can be clearly seen that these 35 urine biomarkers can clearly distinguish the control group from the TAO model group, suggesting that the biomarkers we found are significantly related to thromboangiitis obliterans. Among them, 22 biomarkers were adjusted by SMYAT. According to the results of treatment, the affected limbs of rats in the SMYAT treatment group were recovered in varying degrees, the hemorheology and other indicators significantly adjusted back, and the degree of femoral artery lesions improved. This study can show that the metabolomics method can accurately analyze the potential biomarkers and metabolic pathways, so as to achieve a specific, targeted, therapeutic effect. SMYAT can regulate multiple metabolic pathways such as cysteine and methionine metabolism, phenylalanine metabolism, tyrosine metabolism and vitamin B6 metabolism in rats with thromboangiitis obliterans, and achieve good therapeutic effects.
Methionine is an alpha-amino acid, and is found in all organisms ranging from bacteria to plants to animals. It is classified as an aliphatic, non-polar amino acid. In addition to being a substrate for protein synthesis, methionine is an intermediate in transmethylation reactions, serving as the major methyl group donor in vivo, including the methyl groups for DNA and RNA intermediates. Acute doses of methionine can lead to acute increases in plasma homocysteine, which can be used as an index of the susceptibility to cardiovascular disease. When present in sufficiently high levels, methionine can act as an atherogen and a metabotoxin. An atherogen is a compound that, when present at chronically high levels, causes atherosclerosis and cardiovascular disease. Methionine oxidation metabolism has a promoting effect on vascular diseases such as atherosclerosis and thrombosis (Gu et al., 2015). Studies have shown that increased homocysteine levels are related to thrombosis. In vivo, cysteine is derived from the amino acid containing thiol group formed by methionine metabolism (Undas et al., 2005; Qureshi et al., 2019), and the methionine content is significantly increased in rats with thromboangiitis obliterans, resulting in the metabolic disorder of cysteine and the methionine metabolic pathway, thus promoting thrombosis. After treatment with SMYAT, the content of methionine was significantly reduced. It reminds us that maintaining a stable methionine content plays an important role in preventing thrombosis. The content of normetanephrine in TAO model rats was significantly increased, and normetanephrine was a metabolite produced by catechol-O-methyl transferase. Within humans, normetanephrine participates in a number of enzymatic reactions. It is also involved in the metabolic disorder called transient tyrosinemia. Phenylalanine and tyrosine are used as the precursor of catecholamine and participate in the synthesis of normetanephrine and other substances. In this TAO model, the tyrosine metabolism pathway is disordered, which led to the increase of normetanephrine content. Normetanephrine has a regulatory effect on the degree of vascular tension, which affects the model state (Desjars and Pinaud, 1989). High levels of norepinephrine can reduce blood supply, aggravate ischemia, expand the scope of infarction, and promote thrombosis. After treatment with SMYAT, normetanephrine content is significantly regulated, and the state of blood vessels is improved to prevent thrombosis. The content of hydroxyprolyl-leucine in TAO model rats significantly increased and hydroxyprolyl-leucine was a dipeptide composed of hydroxyproline and leucine. Most of these substances have cell signaling effects and are intermediate products of amino acid degradation pathways. The content of hydroxyprolyl-leucine significantly decreased after treatment with SMYAT, suggesting that the therapeutic effect of SMYAT might be related to the regulation of the hydroxyprolyl-leucine content. 4-ethylphenylsulfate is an organic compound, called phenylsulfate benzene sulfate is a compound containing sulfuric acid group which combines with phenyl. The component in TAO model group is significantly increased and it is significantly regulated after giving SMYAT. Vitamin B6 is a term for a group of interconvertible molecules containing pyridoxine, pyridoxal, pyridoxamine, and their phosphates (Kalle et al., 2016). Vitamin B6 is the main component of a coenzyme in the human body and participates in a variety of metabolic reactions. Vitamin B6 is finally metabolized into 4-pyridoxic through a series of processes in the body (Stanulović et al., 1976). The ratio of 4-pyridoxic acid to pyridoxine is related to cardiovascular disease. It is believed that vitamin B6 in plasma can be metabolized to 4-pyridoxic acid, which increases the risk factors of blood vessels (Obeid et al., 2019). In this research, the vitamin B6 metabolism in TAO model rats was disordered, and the content of 4-pyridoxic acid increased. 4-pyridoxic acid will accumulate in the body, thereby increasing the risk of thrombosis, and it is significantly regulated after giving SMYAT. Many of the biomarkers we have found are related to thrombosis and have been significantly adjusted. This also shows that SMYAT has a significant effect in the treatment of this disease and has the value of in-depth analysis.
Metabolomics technology is used to characterize the metabolic profile of syndromes and find biomarkers from the level of endogenous small molecule metabolism (Liang et al., 2015c; Qiu et al., 2017, 2020). Because of its high sensitivity, it is very effective in the identification of biomarkers, so it also reflects a new strategy for the diagnosis of thromboangiitis obliterans. This study is the first to apply the technology of urine metabolomics to the study of the mechanism of thromboangiitis obliterans, which provides an innovative idea for the study of the mechanism of thromboangiitis obliterans. SMYAT has a good regulatory effect on thromboangiitis obliterans. Based on UPLC-MS/MS technology, the metabolomics method was used to evaluate the overall therapeutic effect of SMYAT and study its potential molecular mechanism. This experiment provides strong evidence for the mechanism of SMYAT in treating thromboangiitis obliterans and a reliable method for exploring the mechanism of traditional Chinese medicine.
In our research, the rat model of thromboangiitis obliterans was established by injection of sodium laurate, and the effect of SMYAT was evaluated by combining the evaluation indexes of hemorheology, histopathology, and metabolomic methods. In the rat model of thromboangiitis obliterans, there are 35 endogenous urinary metabolites as potential biomarkers. The metabolic pathways include vitamin B6 metabolism, phenylalanine metabolism, cysteine and methionine metabolism and tyrosine metabolism, and so forth. These findings add to our understanding of the pathogenesis of thromboangiitis obliterans and reveal the molecular basis of the efficacy of SMYAT in the treatment of thromboangiitis obliterans through metabolomic techniques. SMYAT can improve thromboangiitis obliterans and is a promising candidate drug for thromboangiitis obliterans.
The original contributions presented in the study are included in the article/Supplementary Material, further inquiries can be directed to the corresponding authors.
The animal study was reviewed and approved by the ethics committee of Heilongjiang University of Chinese Medicine.
X-JW and A-HZ designed the experiments; H-YL, L-WH, and J-RX, performed the experiment; H-YL, HS, L-WH, SQ, and FW analyzed the data; H-YL, wrote the paper. All the authors read and approved the final manuscript.
This work was supported by grants from the Key Program of Natural Science Foundation of State (Grant No. 81430093), Natural Science Foundation of Heilongjiang Province (YQ2019H030), Scientific and Technology Development Program of Guangxi (AD18126013), the Ba Gui Scholars program of Guangxi, the Central Government Guides Local Science and Technology Development Fund Projects (ZY21195044) and Heilongjiang Touyan Innovation Team Program.
The authors declare that the research was conducted in the absence of any commercial or financial relationships that could be construed as a potential conflict of interest.
All claims expressed in this article are solely those of the authors and do not necessarily represent those of their affiliated organizations, or those of the publisher, the editors and the reviewers. Any product that may be evaluated in this article, or claim that may be made by its manufacturer, is not guaranteed or endorsed by the publisher.
The Supplementary Material for this article can be found online at: https://www.frontiersin.org/articles/10.3389/fphar.2022.827733/full#supplementary-material
Asada, Y., Yamashita, A., Sato, Y., and Hatakeyama, K. (2018). Thrombus Formation and Propagation in the Onset of Cardiovascular Events. J. Atheroscler. Thromb. 25 (8), 653–664. doi:10.5551/jat.RV17022
Desjars, P., and Pinaud, M. (1989). Norepinephrine in Hyperkinetic Septic Shock: from a Misunderstood Process to Justified Rehabilitation. Ann. Fr Anesth. Reanim 8 (1), 1–3. doi:10.1016/s0750-7658(89)80134-0
Fazeli, B., and Ravari, H. (2015). Mechanisms of Thrombosis, Available Treatments and Management Challenges Presented by Thromboangiitis Obliterans. Curr. Med. Chem. 22 (16), 1992–2001. doi:10.2174/0929867322666150429112111
Filla, L. A., and Edwards, J. L. (2016). Metabolomics in Diabetic Complications. Mol. Biosyst. 12 (4), 1090–1105. doi:10.1039/c6mb00014b
Gu, S. X., Stevens, J. W., and Lentz, S. R. (2015). Regulation of Thrombosis and Vascular Function by Protein Methionine Oxidation. Blood 125 (25), 3851–3859. doi:10.1182/blood-2015-01-544676
Guo, R., Luo, X., Liu, J., Liu, L., Wang, X., and Lu, H. (2020). Omics Strategies Decipher Therapeutic Discoveries of Traditional Chinese Medicine against Different Diseases at Multiple Layers Molecular-Level. Pharmacol. Res. 152, 104627. doi:10.1016/j.phrs.2020.104627
Harpole, M., Davis, J., and Espina, V. (2016). Current State of the Art for Enhancing Urine Biomarker Discovery. Expert Rev. Proteomics 13 (6), 609–626. doi:10.1080/14789450.2016.1190651
Highlander, P., Southerland, C. C., VonHerbulis, E., and Gonzalez, A. (2011). Buerger Disease (Thromboangiitis Obliterans): a Clinical Diagnosis. Adv. Skin Wound Care 24 (1), 15–17. doi:10.1097/01.ASW.0000392923.37852.43
Johnson, C. H., Ivanisevic, J., and Siuzdak, G. (2016). Metabolomics: beyond Biomarkers and towards Mechanisms. Nat. Rev. Mol. Cel Biol 17 (7), 451–459. doi:10.1038/nrm.2016.25
Kalle, S., Tanner, R., Arund, J., Tomson, R., Luman, M., and Fridolin, I. (2016). 4-Pyridoxic Acid in the Spent Dialysate: Contribution to Fluorescence and Optical Monitoring. PLoS One 11 (119), e0162346. doi:10.1371/journal.pone.0162346
Khamis, M. M., Adamko, D. J., and El-Aneed, A. (2017). Mass Spectrometric Based Approaches in Urine Metabolomics and Biomarker Discovery. Mass. Spectrom. Rev. 36 (2), 115–134. doi:10.1002/mas.21455
Klein-Weigel, P., Volz, T. S., and Richter, J. (2015). Thromboangiitis Obliterans (Buerger's Disease): Update 2015. Dtsch Med. Wochenschr 140 (20), 1486–1489. doi:10.1055/s-0041-106246
Laíns, I., Gantner, M., Murinello, S., Lasky-Su, J. A., Miller, J. W., Friedlander, M., et al. (2019). Metabolomics in the Study of Retinal Health and Disease. Prog. Retin. Eye Res. 69, 57–79. doi:10.1016/j.preteyeres.2018.11.002
Li, M. D., Wang, Y. F., Yang, M. W., Hong, F. F., and Yang, S. L. (2020). Risk Factors, Mechanisms and Treatments of Thromboangiitis Obliterans: An Overview of Recent Research. Curr. Med. Chem. 27 (35), 6057–6072. doi:10.2174/0929867326666190816233042
Li, N., Qu, X. B., Lin, S., Li, H., Lin, H., Li, J., et al. (2013). Protective Effect of Simiao Yongan Decoction on Rats with Thromboangiitis Obliterans. Chin. J. Exp. Traditional Med. Formulae 19 (08), 225–227.
Li, Y.-F., Qiu, S., Gao, L.-J., and Zhang, A.-H. (2018). Metabolomic Estimation of the Diagnosis of Hepatocellular Carcinoma Based on Ultrahigh Performance Liquid Chromatography Coupled with Time-Of-Flight Mass Spectrometry. RSC Adv. 8 (17), 9375–9382. doi:10.1039/c7ra13616a
Liang, Q., Liu, H., Xie, L.-X., Li, X., and Zhang, A.-H. (2017). High-throughput Metabolomics Enables Biomarker Discovery in Prostate Cancer. RSC Adv. 7 (5), 2587–2593. doi:10.1039/c6ra25007f
Liang, Q., Liu, H., Zhang, T., Jiang, Y., Xing, H., and Zhang, A.-H. (2016b). Discovery of Serum Metabolites for Diagnosis of Progression of Mild Cognitive Impairment to Alzheimer's Disease Using an Optimized Metabolomics Method. RSC Adv. 6 (5), 3586–3591. doi:10.1039/c5ra19349d
Liang, Q., Liu, H., Zhang, T., Jiang, Y., Xing, H., and Zhang, A.-H. (2015b). Metabolomics-based Screening of Salivary Biomarkers for Early Diagnosis of Alzheimer's Disease. RSC Adv. 5 (116), 96074–96079. doi:10.1039/c5ra19094k
Liang, Q., Liu, H., Zhang, T., Jiang, Y., and Zhang, A.-H. (2016a). Untargeted Lipidomics Study of Coronary Artery Disease by FUPLC-Q-TOF-MS. Anal. Methods 8 (6), 1229–1234. doi:10.1039/c5ay02258d
Liang, Q., Wang, C., Li, B., and Zhang, A.-H. (2015a). Lipidomics Analysis Based on Liquid Chromatography Mass Spectrometry for Hepatocellular Carcinoma and Intrahepatic Cholangiocarcinoma. RSC Adv. 5 (78), 63711–63718. doi:10.1039/c5ra09589a
Liang, Q., Wang, C., Li, B., and Zhang, A.-H. (2015c). Metabolomics of Alcoholic Liver Disease: A Clinical Discovery Study. RSC Adv. 5 (98), 80381–80387. doi:10.1039/c5ra13417j
Liang, Q., Yu, Q., Wu, H., Zhu, Y.-Z., and Zhang, A.-H. (2014). Metabolite Fingerprint Analysis of Cervical Cancer Using LC-QTOF/MS and Multivariate Data Analysis. Anal. Methods 6 (12), 3937–3942. doi:10.1039/c4ay00399c
Liu, J., Tian, S., and Miao, M. S. (2019). Effect of Essential Oil of Sanshencao on Thromboangiitis Obliterans Model in Rats. Chin. J. Exp. Traditional Med. Formulae 25 (04), 18–22.
Liu, X.-y., Zhang, A.-h., Fang, H., Li, M.-x., Song, Q., Su, J., et al. (2018a). Serum Metabolomics Strategy for Understanding the Therapeutic Effects of Yin-Chen-Hao-Tang against Yanghuang Syndrome. RSC Adv. 8, 7403–7413. doi:10.1039/c7ra11048k
Liu, Y., Wei, M., Yue, K., Wang, R., Ma, Y., Men, L., et al. (2018b). Non-target Metabonomic Method provided New Insights on the Therapeutical Mechanism of Gancao Fuzi Decoction on Rheumatoid Arthritis Rats. J. Chromatogr. B Analyt Technol. Biomed. Life Sci. 1105, 93–103. doi:10.1016/j.jchromb.2018.11.015
Liu, Z., Zhang, Y., Zhang, R., Gu, L., and Chen, X. (2017). Promotion of Classic Neutral Bile Acids Synthesis Pathway Is Responsible for Cholesterol-Lowing Effect of Si-Miao-Yong-An Decoction: Application of LC-MS/MS Method to Determine 6 Major Bile Acids in Rat Liver and Plasma. J. Pharm. Biomed. Anal. 135, 167–175. doi:10.1016/j.jpba.2016.12.021
Munnix, I. C., Cosemans, J. M., Auger, J. M., and Heemskerk, J. W. (2009). Platelet Response Heterogeneity in Thrombus Formation. Thromb. Haemost. 102 (6), 1149–1156. doi:10.1160/TH09-05-0289
Obeid, R., Geisel, J., and Nix, W. A. (2019). 4-Pyridoxic Acid/Pyridoxine Ratio in Patients with Type 2 Diabetes Is Related to Global Cardiovascular Risk Scores. Diagnostics (Basel) 9 (1), 28. doi:10.3390/diagnostics9010028
Peng, L., Li, M., Xu, Y. Z., Zhang, G. Y., Yang, C., Zhou, Y. N., et al. (2012). Effect of Si-Miao-Yong-An on the Stability of Atherosclerotic Plaque in a Diet-Induced Rabbit Model. J. Ethnopharmacol 143 (1), 241–248. doi:10.1016/j.jep.2012.06.030
Piazza, G., and Creager, M. A. (2010). Thromboangiitis Obliterans. Circulation 121 (16), 1858–1861. doi:10.1161/CIRCULATIONAHA.110.942383
Qiu, S., Zhang, A., Zhang, T., Sun, H., Guan, Y., Yan, G., et al. (2017). Dissect New Mechanistic Insights for Geniposide Efficacy on the Hepatoprotection Using Multiomics Approach. Oncotarget 8 (65), 108760–108770. doi:10.18632/oncotarget.21897
Qiu, S., Zhang, A.-h., Guan, Y., Sun, H., Zhang, T.-l., Han, Y., et al. (2020). Functional Metabolomics Using UPLC-Q/TOF-MS Combined with Ingenuity Pathway Analysis as a Promising Strategy for Evaluating the Efficacy and Discovering Amino Acid Metabolism as a Potential Therapeutic Mechanism-Related Target for Geniposide against Alcoholic Liver Disease. RSC Adv. 10 (5), 2677–2690. doi:10.1039/c9ra09305b
Quintas, A., and Albuquerque, R. (2008). Buerger's Disease: Current Concepts. Rev. Port Cir Cardiotorac Vasc. 15 (1), 33–40.
Qureshi, S. S., Gupta, J. K., Goyal, A., and Narayan Yadav, H. (2019). A Novel Approach in the Management of Hyperhomocysteinemia. Med. Hypotheses 129, 109245. doi:10.1016/j.mehy.2019.109245
Roberts, L. D., Souza, A. L., Gerszten, R. E., and Clish, C. B. (2012). Targeted Metabolomics. Curr. Protoc. Mol. Biol. 30, Unit–24. doi:10.1002/0471142727.mb3002s98
Stanulović, M., Jeremić, V., Leskovac, V., and Chaykin, S. (1976). New Pathway of Conversion of Pyridoxal to 4-pyridoxic Acid. Enzyme 21 (4), 357–369. doi:10.1159/000458879
Undas, A., Brozek, J., and Szczeklik, A. (2005). Homocysteine and Thrombosis: from Basic Science to Clinical Evidence. Thromb. Haemost. 94 (5), 907–915. doi:10.1160/TH05-05-0313
Wang, S., Bai, M., and Miao, M. S. (2021). Analysis of Animal Models of Thromboangiitis Obliterans Based on the Characteristics of Clinical Symptoms in Chinese and Western Medicine. Chin. J. Exp. Traditional Med. Formulae 27 (08), 235–240.
Wang, T., Sun, H. G., Hua, Y. L., Li, P. L., and Wei, Y. M. (2016). Urine Metabonomic Study for Blood-Replenishing Mechanism of Angelica Sinensis in a Blood-Deficient Mouse Model. Chin. J. Nat. Med. 14 (3), 210–219. doi:10.1016/S1875-5364(16)30018-8
Wang, X., Sun, H., Zhang, A., Sun, W., Wang, P., and Wang, Z. (2011). Potential Role of Metabolomics Apporoaches in the Area of Traditional Chinese Medicine: as Pillars of the Bridge between Chinese and Western Medicine. J. Pharm. Biomed. Anal. 55 (5), 859–868. doi:10.1016/j.jpba.2011.01.042
Yang, Z. L., Liu, Z., Meng, Y. H., and Ma, H. Q. (2020). Application of Simiao Yongan Decoction in Cardiovascular Diseases and Research Progress of its Mechanism. Chin. J. Integr. Med. Cardio-Cerebrovascular Dis. 18 (01), 85–88.
Zhang, A., Sun, H., Han, Y., Yuan, Y., Wang, P., Song, G., et al. (2012a). Exploratory Urinary Metabolic Biomarkers and Pathways Using UPLC-Q-TOF-HDMS Coupled with Pattern Recognition Approach. Analyst 137 (13718), 4200–4208. doi:10.1039/c2an35780a
Zhang, A., Sun, H., and Wang, X. (2012b). Saliva Metabolomics Opens Door to Biomarker Discovery, Disease Diagnosis, and Treatment. Appl. Biochem. Biotechnol. 168 (6), 1718–1727. doi:10.1007/s12010-012-9891-5
Zhang, A., Sun, H., Wu, X., and Wang, X. (2012c). Urine Metabolomics. Clin. Chim. Acta 414, 65–69. doi:10.1016/j.cca.2012.08.016
Zhang, A., Sun, H., Yan, G., Wang, P., and Wang, X. (2016). Mass Spectrometry-Based Metabolomics: Applications to Biomarker and Metabolic Pathway Research. Biomed. Chromatogr. 30 (1), 7–12. doi:10.1002/bmc.3453
Zhang, A., Wang, H., Sun, H., Zhang, Y., An, N., Yan, G., et al. (2015). Metabolomics Strategy Reveals Therapeutical Assessment of Limonin on Nonbacterial Prostatitis. Food Funct. 6 (11), 3540–3549. doi:10.1039/c5fo00489f
Zhang, A.-H., Sun, H., Yan, G.-L., Han, Y., Zhao, Q.-Q., and Wang, X.-J. (2019a). Chinmedomics: A Powerful Approach Integrating Metabolomics with Serum Pharmacochemistry to Evaluate the Efficacy of Traditional Chinese Medicine. Engineering 5, 60–68. doi:10.1016/j.eng.2018.11.008
Zhang, A., Fang, H., Wang, Y., Yan, G., Sun, H., Zhou, X., et al. (2017). Discovery and Verification of the Potential Targets from Bioactive Molecules by Network Pharmacology-Based Target Prediction Combined with High-Throughput Metabolomics. RSC Adv. 7, 51069–51078. doi:10.1039/c7ra09522h
Zhang, A. H., Ma, Z. M., Kong, L., Gao, H. L., Sun, H., Wang, X. Q., et al. (2020). High-throughput Lipidomics Analysis to Discover Lipid Biomarkers and Profiles as Potential Targets for Evaluating Efficacy of Kai-Xin-San against APP/PS1 Transgenic Mice Based on UPLC-Q/TOF-MS. Biomed. Chromatogr. 34 (2), e4724. doi:10.1002/bmc.4724
Zhang, A. H., Ma, Z. M., Sun, H., Zhang, Y., Liu, J. H., Wu, F. F., et al. (2019b). High-Throughput Metabolomics Evaluate the Efficacy of Total Lignans from Acanthophanax Senticosus Stem against Ovariectomized Osteoporosis Rat. Front. Pharmacol. 10, 553. doi:10.3389/fphar.2019.00553
Zhang, A. H., Yu, J. B., Sun, H., Kong, L., Wang, X. Q., Zhang, Q. Y., et al. (2018). Identifying Quality-Markers from Shengmai San Protects against Transgenic Mouse Model of Alzheimer's Disease Using Chinmedomics Approach. Phytomedicine 45, 84–92. doi:10.1016/j.phymed.2018.04.004
Zhang, A., Sun, H., Qiu, S., and Wang, X. (2013). Advancing Drug Discovery and Development from Active Constituents of Yinchenhao Tang, a Famous Traditional Chinese Medicine Formula. Evidence-Based Complement. Altern. Med. 2013, 1–6. doi:10.1155/2013/257909
Zhao, Y., Jiang, Y., Chen, Y., Zhang, F., Zhang, X., Zhu, L., et al. (2020). Dissection of Mechanisms of Chinese Medicinal Formula Si-Miao-Yong-An Decoction Protects against Cardiac Hypertrophy and Fibrosis in Isoprenaline-Induced Heart Failure. J. Ethnopharmacology 248, 112050. doi:10.1016/j.jep.2019.112050
Zheng, L., Wang, M. Y., Chen, Z., and Li, X. B. (2019). Research Progress of Simiao Yongan Decoction. Chin. Traditional Patent Med. 41 (06), 1365–1370.
Keywords: metabolomics, biomarker, therapeutic effect and mechanism, pathway, metabolites
Citation: Li H-Y, Sun H, Zhang A-H, He L-W, Qiu S, Xue J-R, Wu F and Wang X-J (2022) Therapeutic Effect and Mechanism of Si-Miao-Yong-An-Tang on Thromboangiitis Obliterans Based on the Urine Metabolomics Approach. Front. Pharmacol. 13:827733. doi: 10.3389/fphar.2022.827733
Received: 02 December 2021; Accepted: 12 January 2022;
Published: 22 February 2022.
Edited by:
Wenzhi Yang, Tianjin University of Traditional Chinese Medicine, ChinaReviewed by:
Wei Zhang, Macau University of Science and Technology, Macao SAR, ChinaCopyright © 2022 Li, Sun, Zhang, He, Qiu, Xue, Wu and Wang. This is an open-access article distributed under the terms of the Creative Commons Attribution License (CC BY). The use, distribution or reproduction in other forums is permitted, provided the original author(s) and the copyright owner(s) are credited and that the original publication in this journal is cited, in accordance with accepted academic practice. No use, distribution or reproduction is permitted which does not comply with these terms.
*Correspondence: Ai-Hua Zhang, YWlodWF0Y21AMTYzLmNvbQ==; Xi-Jun Wang, eGlqdW53QHNpbmEuY29t
Disclaimer: All claims expressed in this article are solely those of the authors and do not necessarily represent those of their affiliated organizations, or those of the publisher, the editors and the reviewers. Any product that may be evaluated in this article or claim that may be made by its manufacturer is not guaranteed or endorsed by the publisher.
Research integrity at Frontiers
Learn more about the work of our research integrity team to safeguard the quality of each article we publish.