- 1Department of Rheumatology and Immunology, Xiangya Hospital, Central South University, Changsha, China
- 2Provincial Clinical Research Center for Rheumatic and Immunologic Diseases, Xiangya Hospital, Changsha, China
- 3National Clinical Research Center for Geriatric Disorders, Xiangya Hospital, Changsha, China
Systemic sclerosis (SSc) is a multisystem rheumatic disease characterized by vascular dysfunction, autoimmune abnormalities, and progressive organ fibrosis. A series of studies in SSc patients and fibrotic models suggest that immune cells, fibroblasts, and endothelial cells participate in inflammation and aberrant tissue repair. Furthermore, the growing number of studies on single-cell RNA sequencing (scRNA-seq) technology in SSc elaborate on the transcriptomics and heterogeneities of these cell subsets significantly. In this review, we summarize the current knowledge regarding immune cells and stromal cells in SSc patients and discuss their potential roles in SSc pathogenesis, focusing on recent advances in the new subtypes by scRNA-seq.
1 Introduction
Systemic sclerosis (SSc) is an autoimmune connective tissue disease that can be mainly divided into limited cutaneous SSc (lcSSc) and diffuse cutaneous SSc (dcSSc) according to the extent of skin involvement (LeRoy et al., 1988). In patients, lcSSc mainly manifests as a thickening and hardening of the distal acral skin, often related to positive anti-centromere antibodies. Whereas dcSSc in patients also involves the proximal limbs and trunk which are prone to develop visceral organ complications, commonly associated with positive anti-topoisomerase I (anti-Scl-70) or anti-RNA polymerase III antibodies (Steen 2005).
SSc has three major pathological characteristics, including vasculopathy, immune dysregulation, and connective tissue fibrosis. Dysfunction of endothelial cells (ECs) is a key event leading to SSc microangiopathy (Mostmans et al., 2017). Activated ECs are able to recruit inflammatory cells by secreting adhesion molecules and E-selectin or stimulate extracellular matrix (ECM) production by connective tissue growth factor (CTGF) or other pro-fibrotic mediators. Innate and adaptive immune responses play a prominent part in the progression of SSc (Lech and Anders 2013; Allanore et al., 2015). Substantial evidence uncovers the link between the immune system and fibrosis. For instance, specific serum autoantibodies and pro-fibrotic cytokines like IFN-α, IL-4, and IL-13 and the transforming growth factor-beta (TGF-β) secreted by inflammatory cells, as well as abnormal inflammatory signatures [such as type-I interferon (IFN) signature] appear in the blood or target organs of SSc patients (Allanore et al., 2015). In addition, in situ hybridization has found that fibroblasts adjacent to the infiltrated inflammatory cells are more likely to synthesize collagen, implying the recruitment to fibroblasts (Fleischmajer et al., 1977; Kähäri et al., 1988). In SSc, alteration of activation, proliferation, and differentiation in the fibroblast occupy kernel status in tissue fibrosis. These cells release different kinds of chemokines that promote or inhibit the recruitment of circulating immune cells. Moreover, the activation of fibroblasts prevents T-cell apoptosis and enhances the sustainability of T-cell function (Pilling et al., 1999; O'Reilly et al., 2012). Interactions between the fibroblasts and immune cells often lead to the amplification of ECM production. In addition, abundant works have emphasized the process on injured ECs transdifferentiating toward myofibroblasts known as endothelial-to-mesenchymal transition (EndMT), which is also responsible for the expansion of the fibroblast pool (Xu et al., 2011; Jimenez 2013; Ebmeier and Horsley 2015).
In recent years, droplet-based single-cell RNA sequencing (scRNA-seq) has been developed at a rapid pace. In SSc, scRNA-seq reveals novel or rare cell types with great precision and characterizes their developmental trajectory. Profiling cell landscapes by scRNA-seq depicts the entire cell compositions under the conditions of physiology and pathology. Moreover, scRNA-seq allows researchers to discern the potential roles of differentially expressed genes in individual cells and their involvement in signaling pathways which sheds light on the pathogenic mechanism of SSc. There are currently plentiful studies on scRNA-seq targeting monocytes, macrophages, dendritic cells (DCs), T cells, fibroblasts, and ECs in SSc.
In this review, we primarily focus on the recent insights into the characteristics and functions of immune cells and stromal cells in various tissues including the blood, lungs, and skin in SSc patients by scRNA-seq and traditional methods. Therefore, it will greatly benefit to facilitate our comprehension of pathogenic mechanisms and the development of subsequent treatments.
2 Innate Immune Cells
The innate immune system has been shown to be activated at various stages of SSc. Generally, pattern recognition receptors, such as Toll-like receptors (TLRs) in SSc, identify damage-associated molecular patterns and pathogen-associated molecular patterns, which trigger an inflammatory response (Martin 2014) and could lead to a fibrotic state ultimately (Dowson et al., 2017). Myeloid cells, such as monocytes, macrophages, and DCs, are representative of innate immunity and play a significant role in the onset and progression of SSc (Satoh et al., 2017; Ah Kioon et al., 2018; Bhandari et al., 2020). Monocytes participate in fibrosis progression by affecting inflammatory responses and differentiating into macrophage or fibroblast-like cells (Shi and Pamer 2011). The human monocytes can be divided into three subtypes according to the expression of membrane molecules: classical monocytes (CD14++CD16−), nonclassical monocytes (CD14+CD16++), and intermediate monocytes (CD14+CD16+) (Passlick et al., 1989; Auffray et al., 2007). Macrophages are significant mediators of tissue injury and the major source of TGF-β, which are involved in inflammation and fibrosis of SSc (Toledo and Pioli 2019). Traditionally, macrophages are divided into classically activated macrophages (M1) and alternately activated macrophages (M2), which have opposite functions of pro-inflammation and anti-inflammation (Mosser and Edwards 2008). DCs have two primary subtypes—conventional dendritic cells (cDCs) and plasmacytoid dendritic cells (pDCs), both derived from the common DC precursor (Liu et al., 2009). A third subset of DCs is derived from the monocyte precursor (Guilliams et al., 2014). cDCs are a class of powerful antigen-presenting cells and consist of two principal subsets (cDC1s and cDC2s) (Guilliams et al., 2014). These cells variably express the TLRs, which are able to initiate adaptive immune responses (Boltjes and van Wijk 2014). Moreover, pDCs play a critical role in innate immunity by producing type-I IFNs (Cella et al., 1999; Siegal et al., 1999; Guiducci et al., 2010; Swiecki and Colonna 2015).
2.1 Monocytes
Recently, both bulk RNA-seq and scRNA-seq had confirmed the presence of a cluster of monocytes (CD16+ monocytes) closely related to SSc pathogenesis. Bulk RNA-seq is found as an inflammatory gene module, including KLF10, JUNB, PLAUR, and JUND, in the monocytes of the peripheral blood in SSc when compared with the healthy control (HC) (Supplementary Table S1). ScRNA-seq was further used to explore the details of monocytes, and seven subsets (PM0–PM6) were finally identified. The PM0 cluster typically expressed the abovementioned inflammatory co-expression genes and had similar transcriptomics with IL1B+ FCN1hi monocytes in the lung tissue of SSc-associated interstitial lung disease (SSc-ILD) (Kobayashi et al., 2021) (Table 1). According to previous studies, IL1B could be a key pro-fibrotic mediator (Park et al., 2018), and IL1B+ FCN1hi cells were involved in skin and lung fibrosis, indicating that the cluster might be a new therapeutic target for SSc (Aran et al., 2019). Similar results by flow cytometry showed that in addition to the activated phenotypic profile of monocytes in the peripheral blood in SSc, the number of CD16+ monocytes were increased and related to ILD and modified Rodnan skin score (mRSS) (Lescoat et al., 2017; Schneider et al., 2021).
Recently, the whole-genome transcriptome analysis showed activated fibrotic pathway and increased fibronectin expression in circulating CD14+ monocytes of SSc patients. Interestingly, in a pro-fibrotic milieu, CD14+ monocytes were a source of ECM-producing cells, as they could differentiate into myofibroblast-like cells and produce type I collagen and α-SMA (Rudnik et al., 2021a) (Figure 1). One previous study described a monocyte population that secreted high levels of tissue inhibitor of metalloproteinases 1 (TIMP-1) protein, a potentially important regulator in fibrogenesis of SSc. TLR signaling had a key role in TIMP-1 secretion (Ciechomska et al., 2013). CD52 is a glycoprotein anchored to glycosylphosphatidylinositol (Xia et al., 1993; Hale 2001), and it is widely expressed on the monocytes, lymphocytes, and DCs (Ratzinger et al., 2003). Recently, a study has found that the expression of CD52 on circulating monocytes of SSc was reduced. The reduction of CD52 enhanced the adhesion between monocytes and ECs and then led to an increased recruitment of monocytes into the tissues. It is worth noting that the increased type-I IFN–related genes in SSc could downregulate the expression of CD52 in monocytes by histone deacetylase IIa (HDAC IIa). Targeting the IFN–HDAC–CD52 axis may bring a new strategy for early SSc patients (Rudnik et al., 2021b).
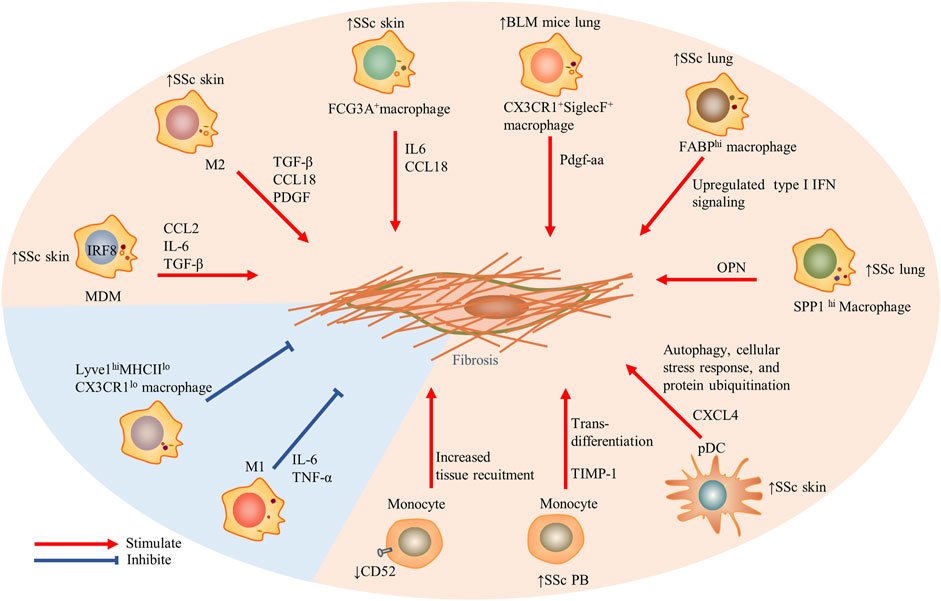
FIGURE 1. The role of innate immune cells including monocytes, macrophages, and dendritic cells in the fibrosis in SSc. Particularly, Lyve1hiMHCIIloCX3CR1lo macrophages restrained induced tissue fibrosis, and traditional M1 macrophages also prevented fibrosis. Red arrows indicate stimulation; blue blunted-ends indicate inhibition. SSc, systemic sclerosis; PB, peripheral blood; IRF, interferon regulatory factor 8; MDM, monocytes-derived macrophages; CCL, CC chemokine ligand; IL, interleukin; IFN, interferon; OPN, osteopontin; TIMP-1, tissue inhibitor of metalloproteinases 1; and Lyve1, lymphatic endothelium hyaluronan receptor-1.
2.2 Macrophages
Robert Lafyatis et al. found five clusters of macrophages in the skin lesions of SSc patients, three of which were parallelly described in HC, including CCR1+, MARCO+, and TREM2+ macrophages. Additional macrophage clusters associated with SSc were proliferating macrophages and FCGR3A+ macrophages, and the latter were probably derived from CCR1+ and MARCO+ macrophages based on pseudotime analysis. FCGR3A+ macrophages expressed M2 macrophage activation markers CD163 and MS4A4A and the macrophage scavenger receptor 1 (MSR1) and were characterized concomitantly by the enriched pathway–like “response to lipopolysaccharide” associated with M1 polarization (Xue et al., 2020; Xue et al., 2021).
The macrophages in the SSc-ILD lung tissue were divided into seven discrete subtypes (MP1–MP7). SPP1 expression at a higher prevalence was noted in the MP1 cluster, and the chemokine CCL18 was relatively enriched in the MP7 cluster. SPP1 encoded osteopontin, a potential predictor for lung function deterioration. The differentially upregulated genes of the two subtypes were mainly involved in the differentiation and migration of myeloid cells and responded to pro-inflammatory signals (Gao et al., 2020). Intriguingly, subsequent studies confirmed that in addition to SPP1hi macrophages, three other subsets including FABP4hi macrophages, monocyte-derived macrophages (FCN1hi macrophages), and proliferating macrophages also appeared in SSc-ILD lung tissues. Compared with idiopathic pulmonary fibrosis (a chronic pulmonary fibrosis disease) and the HC, the type-I IFN signaling was upregulated in SPP1hi and FABPhi macrophages of SSc-ILD. Concretely, the interferon-induced transmembrane proteins IF35, EGR1, and ISG15 were upregulated in the SPP1hi macrophages, while TBK1, PTPN11, and CHUK were upregulated in the FABP4hi macrophages (Valenzi et al., 2021).
The subpopulations of macrophages showed the greatest discrimination between mice and humans. Macrophages in the lung tissues of bleomycin-induced fibrosis mice were classified into three types by scRNA-seq: alveolar macrophages, intermediate macrophages (CX3CR1+SiglecF+), and interstitial macrophages. The transitional subpopulation highly expressing MHCII molecules was located in aggregates adjacent to infiltrated Pdgfra+ and Pdgfrb+ fibroblasts and might be the source of Pdgf-aa (a nutrient factor of fibroblasts) in the fibrosis niche. In other words, the paracrine interactions between the macrophages and fibroblasts played a positive role in tissue fibrosis (Aran et al., 2019). Recently, MHCIIhiCX3CR1hi macrophages also have been systematically studied in the lung, fat, dermis, and heart tissues of mice with a low expression of lymphatic endothelial hyaluronan receptor-1 (Lyve1). Lyve1loMHCIIhiCX3CR1hi macrophages were conserved in these tissues and located adjacent to the fibers. The other conserved macrophages were Lyve1hiMHCIIloCX3CR1lo macrophages with a role to restrain induced tissue fibrosis (Chakarov et al., 2019).
The traditional paradigm implies the M2 pro-fibrotic properties of monocytes/macrophages in the blood and target organs of SSc patients. M1 macrophages exerted their effects primarily at the stage of the inflammatory responses by producing IL-1β, TNF-α, and IL-6 (Gordon 2003; Mosser 2003). Infiltrated M2 macrophages in the skin and peripheral blood have been observed in SSc with the putative marker CD163 (Higashi–Kuwata et al., 2010; Bielecki et al., 2013). M2 macrophages produced pro-fibrotic mediators such as TGF-β, CCL18, and the platelet-derived growth factor to promote fibroblast activation and collagen release (Jaguin et al., 2015; Shapouri–Moghaddam et al., 2018). However, in addition to the M1 and M2 macrophages, the circulating macrophage of SSc patients also included a confluent M1/M2 phenotype which has been reported to be associated with a more severe phenotype (SSc-ILD) (Trombetta et al., 2018) and arthritis or myalgia (Mohamed et al., 2021). Monocytes in the peripheral blood in SSc might differentiate into monocytes-derived macrophages (MDMs) during exposure to the soluble factors in the plasma and secret activation markers such as CCL2, IL-6, and TGF-β. Their activation has a pro-fibrotic property (Bhandari et al., 2020), which possibly involves the key transcription factor—interferon regulatory factor 8 (IRF8), a regulator of differentiation and function of the myeloid cells. On the one hand, MDMs show an M2 phenotype after silencing IRF8; on the other hand, skin fibrosis of mice with myeloid cell–specific knockout of IRF8 increased after bleomycin treatment (Ototake et al., 2021).
2.3 Dendritic Cells
The results of scRNA-seq showed that there were six DC subpopulations in skin biopsies of the HC, including cDC1 (CLEC9A+ DCs), two subsets of cDC2 (CXorf21+ DCs and MCOLN2+ DCs), a novel DC subtype (LAMP3+ DCs), a cluster of proliferating DCs (KIAA0101+ DCs), and a Langerhans cell subset (Langerin+ DCs). FCN1+, the monocyte-derived DC marker, was related to cDC2 in the pseudo-time distribution and existed solely in dcSSc, which was associated with severe skin fibrosis and had been mentioned in previous studies (Kobayashi et al., 2021). In addition, pDCs derived from the lymphoid progenitor cells also appeared almost exclusively in the skin in dcSSc (Xue et al., 2020; Xue et al., 2021). The upregulated pathway, including autophagy, cellular stress response, and protein ubiquitination, showed the abnormal phenotypes of activation among the pDCs of SSc-ILD (Valenzi et al., 2021).
The assay of transposase-accessible chromatin using sequencing showed that the DCs occupied the greatest epigenetic difference between SSc skin lesions and the normal skin, and the disease-related single-nucleotide polymorphisms were significantly enriched in the DCs, indicating that it may be an important epigenetic driving factor of SSc (Liu et al., 2020). The proteome-wide analysis has proved that pDCs in SSc secreted chemokine CXCL4, a predictor of mRSS (van Bon et al., 2014). pDCs expressing IFNα and CXCL4 accumulated around the vessels of the skin in SSc (van Bon et al., 2014; Ah Kioon et al., 2018). In a recent study, CXCL4, as a self-antigen, promoted the activation of type-I IFN signals by pDCs and anti-CXCL4 antibodies by B cells, which maintained the vicious circle of SSc IFN-I signature (Lande et al., 2020). Epigenetic factors such as the recent miR-126 and miR-139-5p (Chouri et al., 2021) and the previous miR-618 (Rossato et al., 2017) also contributed to the characteristics of type-I IFN in disease. Moreover, xenotransplantation of human pDCs into bleomycin-induced nonobese diabetic/severe combined immunodeficiency mice increased IFN-induced response to TLR agonist significantly and further demonstrated the key role of pDC in immune response and fibrosis degree (Ross et al., 2021).
3 T Lymphocytes
T-cell heterogeneity is high in terms of expression and function of T-cell receptors. Antigen-specific oligoclonal T cells are related to the breakdown in self-tolerance (Laurent et al., 2018). Specific cytokine profiles have a significant impact on the functions of fibroblasts and ECs and can promote or inhibit collagen over-synthesis and vascular diseases (Hügle et al., 2013; Chizzolini and Boin 2015). T lymphocytes are divided into T helper (Th) cells, regulatory T (Treg) cells, cytotoxic T (CTL) cells, and γδ T cells. All Th cells express CD4, mainly including Th1, Th2, Th17, Th9, Th22, and T follicular helper (Tfh) cells, and each subpopulation developed from naive T cells which responded to diverse microenvironmental stimuli. Treg cells primarily maintain immune tolerance. CTL cells mainly express CD8 to identify and kill abnormal target cells such as cancer cells and infected cells, but a few can also express CD4. The receptors of γδ T cell are γ chain and δ chain which permit recognition of antigens without MHC restriction. In addition, a new subset of T cells in SSc skin likely promoting B-cell responses has been fully revealed by scRNA-seq which provides a fuller framework for understanding the contribution of T lymphocytes to autoimmunity (Gaydosik et al., 2021).
3.1 T-Cell Subsets by scRNA-Seq
Currently, only one study in 2021 analyzed the skin-resident and recirculating T-cell subsets and their heterogeneity in dcSSc by scRNA-seq. According to differences in transcriptome profiles, skin T lymphocytes were divided into nine clusters. In addition to traditional CD4+ T cells, CD8+ T cells, and Treg cells, T cell clusters expressing IL-26, proliferation genes, TRDC, and CXCL13 were also identified. Each T-cell cluster had activated and special molecular pathways. Especially, the mitochondrial, ERK/MAPK signaling, and oxidative phosphorylation pathways in CXCL13+ T cells were all upregulated. CXCL13+ T cells expanded in the skin of dcSSc with perivascular localization and were related to ILD significantly. Moreover, the subpopulation exhibited recirculating memory migratory phenotype (CCR7+SELL−), which implied a certain migration ability. CXCL13 is a B-cell chemoattractant that helps to form tertiary lymphoid structures (TLOs) (Ansel et al., 2000). In this study, CXCL13+ T cells were adjacent to CD20+ B cells and expressed Tfh-related genes with lower levels like ICOS and PD1 but not BCL6 and CXCR5. Therefore, it may be a special Tfh subset, and treatment for CXCL13+ T cells may prevent disease progression (Gaydosik et al., 2021) (Figure 2).
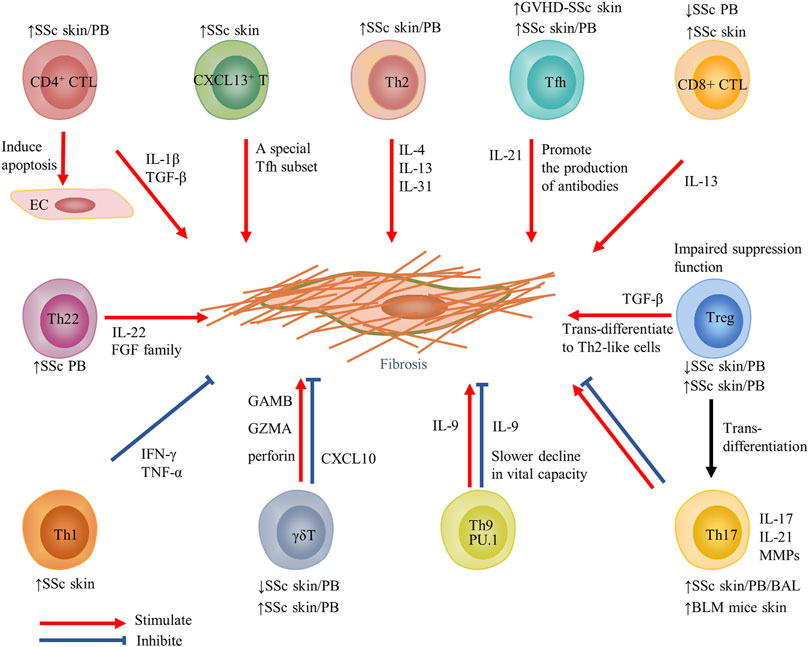
FIGURE 2. Contribution of T-cell subsets and their cytokines to fibrosis in SSc. Changes in T-cell subsets of PB or target organs in SSc and fibrosis models are displayed above or below the cell. Red arrows indicate stimulation; blue blunted-ends indicate inhibition. SSc, systemic sclerosis; PB, peripheral blood; EC, endothelial cells; IL, interleukin; Tfh, T follicular helper; GVHD, graft-versus-host disease; and MMP, matrix metalloproteinase.
3.2 Th1 and Th2 Cells
Th1 cells mainly secrete cytokines such as IFN-γ, TNF-α, and IL-2, while Th2 cells mainly secrete cytokines such as IL-4, IL-5, and IL-13 (Wynn 2004). At present, most researchers agree with the view of skewed Th2 pattern in SSc effector T cells (O'Reilly et al., 2012; Chizzolini and Boin 2015; Distler et al., 2019; Zhang et al., 2020; Worrell and O'Reilly 2020). Therefore, targeted delivery to restore the balance between Th1 and Th2 cytokines should be further researched for limiting fibrosis (Gourh et al., 2009). Generally, IFN-γ mainly inhibited collagen synthesis by reducing the activity of fibroblasts or restraining the effect of cytokines IL-4 and IL-13 (Rosenbloom et al., 1986). Compared with the HC, cytokines IL-4 and IL-13 in the serum of SSc patients were increased (Gasparini et al., 2020). IL-13 inhibited the expression of matrix metalloproteinase-1 (MMP-1) through the PKB/AKT pathway in skin fibroblasts of HC and SSc patients (Brown Lobbins et al., 2018). Latest reports have discovered the new Th2 cytokine IL-31 was elevated in plasma and target organs (skin and lung) of SSc patients (Yaseen et al., 2020). IL-31 promoted skin and lung fibrosis and enhanced Th2 immune responses, which were ameliorated by the blockade of IL-31 or anti-IL-31RA antibody (Kuzumi et al., 2021).
3.3 Th17 Cells
Naive T cells differentiate into Th17 cells in the presence of IL-6, IL-21, IL-23, or IL-1 (Harrington et al., 2005; Park et al., 2005; Bettelli et al., 2006), which involve inflammation and autoimmune disease by secreting IL-17, IL-22, and MMPs (Korn et al., 2009; Zambrano–Zaragoza et al., 2014). The proportion of Th17 in the blood, skin, and bronchoalveolar lavage (BAL) of patients of SSc was increased and associated with disease activity (Kubo et al., 2019; Robak et al., 2019; Fox et al., 2021). Due to the plasticity of Th17 cells, a subpopulation of Th17 cells expressed the adhesion molecule CD146 and had a stronger ability to bind and cross vascular endothelium in peripheral blood (Gabsi et al., 2019). Moreover, IFN-γ+IL-17+ Th17 cells which differentiate from Th17 in the presence of IL-12 have recently been shown to enhance the collagen secretion of fibroblasts by producing IL-21 (Annunziato et al., 2007; Xing et al., 2020). In addition, a previous study reported a significantly higher ratio of Th17/Treg cells in the peripheral blood of SSc patients than in HC, which supported the deflection towards Th17-mediated inflammatory processes (Yang et al., 2021). The conversion of Treg to Th17 cells might contribute to this bias as the CD4+CD25+FOXP3loCD45RA- Treg subset produced high levels of IL-17 (Liu et al., 2013).
The key cytokine IL-17 has been extensively studied controversially. IL-17 was both elevated in serum and skin during the early and active stages of SSc (Yang et al., 2014; Tezcan et al., 2021). Mechanically, IL-17 induced inflammation of ECs, and promoted fibroblast proliferation (Kurasawa et al., 2000; Xing et al., 2013). However, other sounds published their anti-fibrosis effect which reduced CTGF and type I collagen through the upregulation of miR-129-5p (Nakashima et al., 2012). More recently, a more refined organotypic model of human skin has been introduced; based on which IL-17 softened the skin by promoting inflammation and attenuating Wnt signaling (Dufour et al., 2020). It is to be noted that Th17 cells and IL-17 possessed a uniform effect in animal models which mediated fibrosis and SSc-like appearance in BLM mice and TSK-1 mice (Okamoto et al., 2012; Lei et al., 2016).
3.4 Th9 Cells
Th9 cells secrete IL-9 and IL-10 under the influence of pro-fibrosis factors (TGF-β, IL-4), and epithelial cytokine (thymic stromal lymphopoietin) (Veldhoen et al., 2008; Yao et al., 2013; Kaplan et al., 2015). IL-9 was highly expressed in the serum in both dcSSc and lcSSc and led to a lower degree of fibrosis and a slower decline in vital capacity at the earliest stage, which suggests IL-9 may be a protective molecule in pulmonary fibrosis (Yanaba et al., 2011). However, other studies elaborate increased IL-9 in the skin, and renal biopsy tissues were positively related to mRSS (Guggino et al., 2017). Furthermore, the Th9 transcription factor PU.1 activated the switch of pro-fibrotic fibroblast phenotype. When PU.1 was inactivated, skin and lung fibrosis in BLM-induced fibrotic mice became reduced (Wohlfahrt et al., 2019). The role of Th9 cells and IL-9 in the immune response of SSc individuals needs further research given the paucity of available results.
3.5 Th22 Cells
Although the existence of Th22 cells in SSc has been proved, less attention has been paid to their function. Th22 cells mainly produce IL-22 cytokine and express several members of the fibroblast growth factor (FGF) family, including FGF-1, FGF-5, and FGF-13 which play a role in tissue repair and fibrosis (Eyerich et al., 2009). It has been stressed that significantly increased circulating Th22 cells in SSc patients are related to SSc-ILD and CCR6 (a skin- and lung-homing chemokine receptor) (Truchetet et al., 2011). Furthermore, Th22 cells have been verified to participate in skin immunity because they promote keratinocyte activation induced by TNF and lead to fibroblasts obtaining a pro-inflammatory phenotype (Brembilla et al., 2016).
3.6 Tfh Cells
An increased frequency of Tfh cells related to disease severity in the peripheral blood of SSc patients has been observed, which promoted plasmablasts (CD19+CD27+CD38hi) differentiation and antibody production through the IL-21 signaling pathway (Ricard et al., 2019). Furthermore, it has been particularly emphasized that circulating Tfh cells unbalanced toward the Tfh 1 subset in SSc may alter the function of B cells through the IL-21 and IL-6 pathways. In addition, CD4+CXCR5+ Tfh cells also appeared in the skin of SSc patients (Ly et al., 2021). In the skin of graft-versus-host disease (GVHD)–SSc mice, increased ICOS+ Tfh-like cells promoted skin fibrosis in IL-21 and MMP-12–dependent manner (Taylor et al., 2018). Therefore, inhibition of the Tfh cell subset or their cytokines like IL-21 (ruxolitinib) may bring good news to SSc patients.
3.7 Treg Cells
To date, there has been little agreement on the proportions of circulating changes of SSc Tregs (Gu et al., 2008; Zhu and Paul 2008; Antiga et al., 2010; Mathian et al., 2012; Ugor et al., 2017). The possible intrinsic explanation is the decreased expression of runt-related transcription factor 1 (Runx1) mRNA. Runx1 mRNA and forkhead box transcription factor (FoxP3) form a transcription factor complex which is essential for eliciting the suppression function of Treg cells (Kataoka et al., 2015). In SSc patients, an inflammatory environment may be harmful to the immunosuppressive functions. For instance, the frequency of activated and inactivated Treg cells was lower in SSc than in the HC, but the immunosuppressive function of SSc Tregs was restored in vitro (Mathian et al., 2012). In addition, co-incubation of healthy Treg cells with the plasma of SSc patients could reduce the expression of CD69 and TGF-β of Tregs by some unknown factors in the plasma, thus reducing the functional response of Treg cells to effector T cells (Radstake et al., 2009). Some studies in the target organ of SSc also had confusing results (Klein et al., 2011; Yang et al., 2014). Particularly, the circulating Treg cells could migrate to the skin tissues and become Th2-like cells due to homing molecules that secreted a large number of cytokines IL-4 and IL-13, which might have driven the differentiation of fibroblasts and lead to fibrosis in SSc patients (MacDonald et al., 2015).
3.8 CD8+ CTL Cells
Compared with HC, the proportion of circulating CD8+ T cells in SSc patients in the early stage was decreased and related to the prolonged course of disease (Fox et al., 2021). Although according to a 2021 study, antigen-driven expansion of CD8+ T cells had a high temporal persistence in the blood of SSc patients (Servaas et al., 2021). In addition, the CD8+ T cells lacking CD28 expression in the peripheral blood in SSc and skin lesions have been detected. It is reasonable that cytotoxic CD8+CD28− T cells exhibited a pro-fibrotic phenotype because these cells were linked to the degree of skin fibrosis and significantly produced IL-13 (Li et al., 2017).
In recent years, genome-wide results in skin biopsies have identified CD8+ T cells–related genes (CD8A, GraK, GraH, and GraB) (Rice et al., 2016; Skaug et al., 2020). CD8+ T cells had infiltrated the skin of patients with scleroderma, particularly in the early stage, and their cytokine IL-13 promoted the activation of signal transducer and activator of transcription 6 (STAT6) signal through highly expressed receptors in the monocytes and fibroblasts (Fuschiotti et al., 2013). The growth of ECs in the skin in SSc was restricted. To some extent, this may be attributed to perforin and granzyme B expressed by CD8+ T cells (Kahaleh and Fan 1997; Li et al., 2017). When it comes to CD8+ T cells of SSc patients with pulmonary fibrosis, their proportion in the bronchoalveolar lavage fluid and lung tissue was significantly higher than for CD4+ T cells (Yurovsky et al., 1996; Luzina et al., 2009).
3.9 CD4+ CTL Cells
CD4+ CTL cells are a subset of CD4+ T cells with cytotoxicity, which have been widely studied in the context of chronic viral infections such as cytomegalovirus and human immunodeficiency virus. At present, significant studies gradually focused on their potential role in immune-mediated fibrosis, which promoted cytokine release, including IL-1β and TGF-β (Mattoo et al., 2016). The lymphocytes, also referred to as CD4+CD319+(SLAMF7+) lymphocytes, were increased significantly in the peripheral blood of early dcSSc patients compared to the HC (Fox et al., 2021). CD4+ CTL cells labeled GZMA have recently been found to be the dominant infiltrating cells in the skin of patients with SSc compared with Th1, Th2, Tfh, and Tregs. Furthermore, these cells may induce apoptosis of ECs accompanied by an excessive tissue repair process leading to fibrosis and tissue dysfunction (Maehara et al., 2020), indicating the source of tissue damage mediators first discovered in skin lesions in SSc decades ago (Kahaleh and Fan, 1997).
3.10 γδ T Cells
The rare γδ T cells still have a place in the immune responses of the skin, intestine, and lung tissues. There were a decreased number of γδ T cells in the peripheral blood mononuclear cells (PBMCs) of scleroderma individuals, especially in patients with anti-Scl-70 antibodies and shorter disease duration (Holcombe et al., 1995). In another research, activated circulating γδ T cells of SSc patients was increased, and they could upregulate COL1A2 mRNA in co-cultured fibroblasts (Ueda-Hayakawa et al., 2013). CD27 is a co-stimulatory receptor that was very vital to the development and function of the γδ T cells. The increased pathogenicity of the CD27+ γδ T cells had been verified because the subset upregulated the expression of granzyme B, granzyme A, or perforin (Henriques et al., 2016). In addition, the circulating Vdelta1+ T cells (a γδ T subset) tended to accumulate in the skin tissues of SSc due to their expression of adhesion molecules and activation markers (Giacomelli et al., 1998). While in bleomycin-induced pulmonary fibrosis mice, γδ T cells promoted the alleviation of fibrosis by producing CXCL10 (Pociask et al., 2011). Exploring the role and potential mechanism of the minority T cell in the pathogenesis of fibrosis will be helpful for γδ T cell–based immunotherapy.
4 B Cells
Abundant B-cell receptors (BCRs) repertoire is generated in the bone marrow through the rearrangement of immunoglobulin gene fragments (Zhang et al., 2004). B cells are a heterogeneous population distinguished by cytokine production spectra or membrane surface molecules (Perez–Andres et al., 2010). A proliferation-inducing ligand and B-cell–activating factor (BAFF) (TNF family member) are indispensable for B-cell maturation and long-term maintenance (Mackay et al., 2003). Once naive B cells are activated by antigen or Th cells, they will differentiate into memory B cells and plasma cells which are associated with fibrosis through the production of cytokines and classical disease-specific autoantibodies in SSc, while regulatory B cells (Bregs) maintain self-tolerance primarily by cytokine IL-10 (Lee et al., 2021).
4.1 B-Cell Subsets by scRNA-Seq
Systematic analysis of B cells in the blood or target organs of SSc patients by scRNA-seq is a largely explored domain. We briefly describe a finding in systemic lupus erythematosus (SLE) patients. Nine clusters of B cells were identified in the PBMCs which included switched memory, naive with interferon signature (naive-Ifn), non–switched memory, switched memory-Ifn, double-negative 2 (DN2), DN2-Ifn, activated memory, naive, and plasmablast. The strong interferon signature in SLE patients may have resulted from the DN2 subset. The CD52 gene, which had a role in maintaining B-cell homeostasis, was significantly elevated in multiple B-cell clusters from SLE patients, especially in the most depleted clusters of non–switched memory B cells (Zhu et al., 2018; Bhamidipati et al., 2020).
4.2 Memory B Cells
Although the CD19+CD27+ memory B cells in the peripheral blood of SSc patients were decreased, the expression of their activation markers (CD80, CD95, HLA-DR) changed in the opposite direction (Forestier et al., 2018). Furthermore, recent findings have emphasized that the frequency of CD19+IgD−CD27+CD38−CD95+–activated switched memory (ASM) B cells in the peripheral blood of patients with dcSSc was lower than that in the HC, mostly in individuals with anti–Scl-70 antibodies or pulmonary fibrosis, implying that the ASM B cells were associated with severe SSc (Simon et al., 2021). In fact, CD21low B cells were mainly composed of memory B cells such as the DN switched (CD27−IgD−) memory B cells and expressed high levels of activation markers. Beyond this, it was a possible indicator of new ulcers and visceral vascular damage (Marrapodi et al., 2020; Visentini et al., 2021).
4.3 Effector B Cells
Effector B cells (Beffs) participate in the immune response by secreting pro-fibrotic cytokine TGF-β (Dumoitier et al., 2017) and a variety of inflammatory markers, such as IL-4, IL-6, IL-12, TNF-α, and GM-CSF (Harris et al., 2000; Li et al., 2015; Shen and Fillatreau 2015). In the skin of SSc patients, infiltrated CD20+ B cells and CD138+ plasma cells were related to the early disease stage and disease progression (Bosello et al., 2018). The same discovery about the increases of B cells and plasma cells was verified by cutaneous transcriptome data (Whitfield et al., 2003; Streicher et al., 2018). As a classic pro-inflammatory cytokine, IL-6 promoted fibrosis by activating the downstream signaling molecule STAT3 and was also related to SSc disease severity (Sato et al., 2001). Tocilizumab (TCZ) is a humanized IL-6 receptor-α–blocking antibody (Mihara et al., 2011). Recently, the results of a phase 3 randomized controlled trial showed that TCZ stabilized the decline in forced vital capacity in SSc-ILD, whereas there was no change in mRSS (Khanna et al., 2021). The clinical end points of TCZ on skin fibrosis may be mediated only in part by the direct inhibition of fibrosis by TCZ (Khanna et al., 2018). In the bleomycin-induced scleroderma model, the BAFF inhibitor balanced the skew between Beffs and Bregs and alleviated skin and lung fibrosis (Matsushita et al., 2018). Consistent with this research, SSc patients treated with belimumab (binds with soluble BAFF with high affinity) achieved clinical improvements associated with reduced expression of B-cell activation and fibrosis-related genes in the skin (Gordon et al., 2018).
4.4 Regulatory B Cells
Compared with Treg cells, Bregs have relatively uniform alteration in SSc. In the peripheral blood of SSc patients, sufficient evidence indicates the decreased frequency of IL-10+ Bregs which is negatively related to the titers of anti-centromeric and anti-Scl-70 autoantibodies (Matsushita et al., 2016). The possible reasons for the altered quantities consists of TGF-β and IFN-γ both of which inhibited Bregs development (Iwata et al., 2011). In chronic GVHD (cGVHD) patients, Bregs enriched in the CD19+CD24hiCD38hi transitional and CD19+IgM+CD27+ memory B cells and exhibited decreased tendencies similar to less-produced IL-10 (Khoder et al., 2014). Recently, CD24hiCD27+ Bregs were shown to be involved in the regulation of disease severity due to their reduced frequency in SSc patients with pulmonary arterial hypertension (PAH) (Ricard et al., 2021). Interestingly, T-cell Ig and mucin domain protein 1 (TIM-1), a marker of the Bregs in mice, was also identified in the human IL-10+ Bregs as a unique marker. TIM-1+ B cells in the HC inhibited the pro-inflammatory ability of CD4+ T cells when compared with SSc patients (Aravena et al., 2017). Animal experiments yielded similar results. For instance, early adoptive transplantation of IL-10+ Bregs exhibited a suppressive role in the development of sclerodermatous cGVHD (Scl-cGVHD) mice (Le Huu et al., 2013).
5 Fibroblasts
The activity of fibroblasts and collagen production is closely related to the pathogenesis and severity of SSc, while there are no specific markers to define fibroblasts. Recently, scRNA-seq emphasized the intrinsic transcriptome changes in fibroblasts in the skin tissues of HC and SSc patients. A total of 10 SSc fibroblast subpopulations were identified by characteristic gene expression, including SFRP2hi fibroblasts (SFRP2/WIF fibroblasts, SFRP2/PRSS23 fibroblasts, PCOLCE2 fibroblast), APOE-defined cells (MYOC/FMO1/APOElow fibroblasts, CCL19/C7/APOEhi fibroblasts), CRABP1 fibroblasts, COL11A1 fibroblasts, POSTN/ASPN fibroblasts, ANGPTL7 fibroblasts, and proliferating fibroblasts. The major subpopulation was the SFRP2hi [secreted frizzled-related protein (SFRP) is related with Wnt signaling] fibroblasts located between the collagen bundles (Tabib et al., 2018). MYOC/FMO1/APOElow fibroblasts were distributed in the MYOC/FMO1/APOElow fibroblasts were distributed in the interstitial regions and around the blood vessels. While CCL19/C7/APOEhi fibroblasts showed a strong trend to SSc fibroblasts which differed from CCL19+ fibroblasts of HC. The CRABP1 fibroblasts, COL11A1 fibroblasts, and POSTN/ASPN fibroblasts located around hair follicles supported previous finding about papillary dermal fibroblasts regenerating hair follicles (Driskell et al., 2013). Of note, SFRP2/PRSS23 fibroblasts and ANGPTL7 fibroblasts appeared only in SSc skin lesions. The former was associated with collagen fibril organization and ECM organization by Gene Ontology (GO) analysis, and the latter expressed SFRP4 and represented myofibroblasts. Myofibroblasts–labeled SFRP2, SFRP4, and FNDC1 were converted from SFRP2hiWIF1+ precursor fibroblasts with the upregulation of transcriptome markers PRSS23 and THBS1 at the beginning. In addition, the transcription factors of fibroblasts (RUNX1, FOXP1, IRF7, STAT1, and CREB3L1) promoted the differentiation of fibroblasts into myofibroblasts by bioinformatics analysis (Tabib et al., 2018; Tabib et al., 2021).
The majority of the marker of pulmonary fibroblasts was not shared with dermal fibroblasts. MFAP5hi, SPINT2hi, few WIF1hi fibroblasts, and large proliferating myofibroblasts were identified between the lung tissues of the SSc-ILD and HC groups. It is noteworthy that MFAP5hi fibroblasts expressed higher SFRP, PCOLCE2, and CD55 than the skin SFRP2/DPP4 fibroblasts, while WIF1hi fibroblasts did not express SFRP2. In addition, myofibroblasts underwent the greatest phenotypic changes and upregulated the expression of collagen and other pro-fibrotic genes in SSc-ILD, which was pivotal to the pathologic mechanisms of fibrosis in SSc-ILD (Valenzi et al., 2019).
In the lung of bleomycin-induced fibrosis mice, researchers found ACTA2 was not the only defined marker for activated fibroblasts. For instance, Ltbp2, Col5a2, and Sparc had a stronger correlation with the fibroblast activation signals. This study similarly identified the largest subcluster of fibroblasts exhibiting a myofibroblast-related gene, including muscle contraction and the development of ECM (Peyser et al., 2019). While another study has divided the fibroblasts of the lungs in bleomycin and control mice into 12 clusters, seven clusters expressed higher COL1A1 and four clusters expressed ACTA2. The remaining were proliferative cells, which occupied unique anatomical locations. The study mainly found a unique type of highly expressing collagen triple helix repeat containing-1 (CTHRC1), which was a terminal state cluster and was detected in the multiple studies (Peyser et al., 2019; Valenzi et al., 2019; Tsukui et al., 2020). CTHRC1+ fibroblasts mainly existed in fibrotic lungs of mice and human and expressed the highest level of type I collagen and other ECM-producing genes such as TNC, POSTN, and COL3A1. Purified CTHRC1+ fibroblasts had better migration ability than other collagen-producing cells and could colonize in the lungs of mice treated with bleomycin (Tsukui et al., 2020).
6 Endothelial Cells
The mechanisms of EC damage and vascular disease progression in SSc are still not clear. ScRNA-seq–determined heparan sulfate proteoglycan 2 (HSPG2) and Apelin receptor (APLNR) were the two top injury markers of ECs in the skin in SSc. HSPF2 was implicated in TGF-β signaling (Iozzo et al., 1997; Sharma et al., 1998) and SSc-associated fibrosis (Laplante et al., 2005). Due to the limitation of only one patient and one control biopsy in this study, no specific subtypes of ECs were divided. It is noticeable that enriched genes in ECs of SSc patients were related to ECM formation, vascular injury, and EndMT by the Ingenuity Pathway Analysis (IPA) and Gene Set Enrichment Analysis (GSEA) approaches (Apostolidis et al., 2018). Moreover, scRNA-seq analysis of SSc-ILD implied the active expansion of ECs in the lung tissues due to their increased vasculogenesis, prostaglandin biosynthesis, and PDGFR-signaling (Valenzi et al., 2021).
There are also other possible explanations of endothelial dysfunction. A new study found that neuronal-related characteristics such as dysregulation of the neuronal genes ETV2 and NRXN1 in ECs may be a culprit for dysangiogenesis in SSc (Tsou et al., 2021). In addition, sufficient evidence underlined a close liaison between vasculopathy and the metabolism of SSc dermal fibroblasts. Extracellular acidosis derived from the released lactic acid by SSc fibroblasts might lead to the impairment of peripheral capillary networks through acidic upregulated MMP-12 (an inhibitor of angiogenesis) in ECs (Andreucci et al., 2021). Intriguingly, endothelial miR-150 showed a protective effect in an animal experiment which was an independent survival predictor of PAH patients. The possible explanation implicated PTEN-like mitochondrial phosphatase (PTPMT1), which improved mitochondrial function and reduced apoptosis of ECs (Russomanno et al., 2021).
7 Conclusion
In SSc, the immune system and stromal cells in the blood and target organs show significant numerical or functional changes leading to the pathogenetic phenotype. ScRNA-seq provides greater insights for identifying new cell subtypes and elaborating their complex roles in disease states, and we need to further explore those subgroups that have no well-characterized functions. With the combination of conventional methods, scRNA-seq analysis, and further integrative multi-omics analysis, we could understand individual cell behavior and cellular variety, and elucidate the pathogenic mechanism of SSc diseases quickly and systematically, significantly propelling precise medical interventions in the near future.
Author Contributions
BD wrote the first draft. BD, LD, and LZ revised the manuscript. HZ and HL revised final version and added extra information.
Funding
This study was funded by grants from National Natural Science Foundation of China (81671622,81771765, 81701621) and Hunan Provincial Natural Science Foundation (2019JJ40503).
Conflict of Interest
The authors declare that the research was conducted in the absence of any commercial or financial relationships that could be construed as a potential conflict of interest.
Publisher’s Note
All claims expressed in this article are solely those of the authors and do not necessarily represent those of their affiliated organizations, or those of the publisher, the editors and the reviewers. Any product that may be evaluated in this article, or claim that may be made by its manufacturer, is not guaranteed or endorsed by the publisher.
Supplementary Material
The Supplementary Material for this article can be found online at: https://www.frontiersin.org/articles/10.3389/fphar.2022.826839/full#supplementary-material
References
Ah Kioon, M. D., Tripodo, C., Fernandez, D., Kirou, K. A., Spiera, R. F., Crow, M. K., et al. (2018). Plasmacytoid Dendritic Cells Promote Systemic Sclerosis with a Key Role for TLR8. Sci. Transl Med. 10 (423), eaam8458. doi:10.1126/scitranslmed.aam8458
Allanore, Y., Simms, R., Distler, O., Trojanowska, M., Pope, J., Denton, C. P., et al. (2015). Systemic Sclerosis. Nat. Rev. Dis. Primers 1, 15002. doi:10.1038/nrdp.2015.2
Andreucci, E., Margheri, F., Peppicelli, S., Bianchini, F., Ruzzolini, J., Laurenzana, A., et al. (2021). Glycolysis-derived Acidic Microenvironment as a Driver of Endothelial Dysfunction in Systemic Sclerosis. Rheumatology (Oxford) 60 (10), 4508–4519. doi:10.1093/rheumatology/keab022
Annunziato, F., Cosmi, L., Santarlasci, V., Maggi, L., Liotta, F., Mazzinghi, B., et al. (2007). Phenotypic and Functional Features of Human Th17 Cells. J. Exp. Med. 204 (8), 1849–1861. doi:10.1084/jem.20070663
Ansel, K. M., Ngo, V. N., Hyman, P. L., Luther, S. A., Förster, R., Sedgwick, J. D., et al. (2000). A Chemokine-Driven Positive Feedback Loop Organizes Lymphoid Follicles. Nature 406 (6793), 309–314. doi:10.1038/35018581
Antiga, E., Quaglino, P., Bellandi, S., Volpi, W., Del Bianco, E., Comessatti, A., et al. (2010). Regulatory T Cells in the Skin Lesions and Blood of Patients with Systemic Sclerosis and Morphoea. Br. J. Dermatol. 162 (5), 1056–1063. doi:10.1111/j.1365-2133.2010.09633.x
Apostolidis, S. A., Stifano, G., Tabib, T., Rice, L. M., Morse, C. M., Kahaleh, B., et al. (2018). Single Cell RNA Sequencing Identifies HSPG2 and APLNR as Markers of Endothelial Cell Injury in Systemic Sclerosis Skin. Front. Immunol. 9, 2191. doi:10.3389/fimmu.2018.02191
Aran, D., Looney, A. P., Liu, L., Wu, E., Fong, V., Hsu, A., et al. (2019). Reference-based Analysis of Lung Single-Cell Sequencing Reveals a Transitional Profibrotic Macrophage. Nat. Immunol. 20 (2), 163–172. doi:10.1038/s41590-018-0276-y
Aravena, O., Ferrier, A., Menon, M., Mauri, C., Aguillón, J. C., Soto, L., et al. (2017). TIM-1 Defines a Human Regulatory B Cell Population that Is Altered in Frequency and Function in Systemic Sclerosis Patients. Arthritis Res. Ther. 19 (1), 8. doi:10.1186/s13075-016-1213-9
Auffray, C., Fogg, D., Garfa, M., Elain, G., Join-Lambert, O., Kayal, S., et al. (2007). Monitoring of Blood Vessels and Tissues by a Population of Monocytes with Patrolling Behavior. Science 317 (5838), 666–670. doi:10.1126/science.1142883
Bettelli, E., Carrier, Y., Gao, W., Korn, T., Strom, T. B., Oukka, M., et al. (2006). Reciprocal Developmental Pathways for the Generation of Pathogenic Effector TH17 and Regulatory T Cells. Nature 441 (7090), 235–238. doi:10.1038/nature04753
Bhamidipati, K., Silberstein, J. L., Chaichian, Y., Baker, M. C., Lanz, T. V., Zia, A., et al. (2020). CD52 Is Elevated on B Cells of SLE Patients and Regulates B Cell Function. Front. Immunol. 11, 626820. doi:10.3389/fimmu.2020.626820
Bhandari, R., Ball, M. S., Martyanov, V., Popovich, D., Schaafsma, E., Han, S., et al. (2020). Profibrotic Activation of Human Macrophages in Systemic Sclerosis. Arthritis Rheumatol. 72 (7), 1160–1169. doi:10.1002/art.41243
Bielecki, M., Kowal, K., Lapinska, A., Chyczewski, L., and Kowal-Bielecka, O. (2013). Increased Release of Soluble CD163 by the Peripheral Blood Mononuclear Cells Is Associated with Worse Prognosis in Patients with Systemic Sclerosis. Adv. Med. Sci. 58 (1), 126–133. doi:10.2478/v10039-012-0076-9
Boltjes, A., and van Wijk, F. (2014). Human Dendritic Cell Functional Specialization in Steady-State and Inflammation. Front. Immunol. 5, 131. doi:10.3389/fimmu.2014.00131
Bosello, S., Angelucci, C., Lama, G., Alivernini, S., Proietti, G., Tolusso, B., et al. (2018). Characterization of Inflammatory Cell Infiltrate of Scleroderma Skin: B Cells and Skin Score Progression. Arthritis Res. Ther. 20 (1), 75. doi:10.1186/s13075-018-1569-0
Brembilla, N. C., Dufour, A. M., Alvarez, M., Hugues, S., Montanari, E., Truchetet, M. E., et al. (2016). IL-22 Capacitates Dermal Fibroblast Responses to TNF in Scleroderma. Ann. Rheum. Dis. 75 (9), 1697–1705. doi:10.1136/annrheumdis-2015-207477
Brown Lobbins, M. L., Shivakumar, B. R., Postlethwaite, A. E., and Hasty, K. A. (2018). Chronic Exposure of Interleukin-13 Suppress the Induction of Matrix Metalloproteinase-1 by Tumour Necrosis Factor α in normal and Scleroderma Dermal Fibroblasts through Protein Kinase B/Akt. Clin. Exp. Immunol. 191 (1), 84–95. doi:10.1111/cei.13045
Cella, M., Jarrossay, D., Facchetti, F., Alebardi, O., Nakajima, H., Lanzavecchia, A., et al. (1999). Plasmacytoid Monocytes Migrate to Inflamed Lymph Nodes and Produce Large Amounts of Type I Interferon. Nat. Med. 5 (8), 919–923. doi:10.1038/11360
Chakarov, S., Lim, H. Y., Tan, L., Lim, S. Y., See, P., Lum, J., et al. (2019). Two Distinct Interstitial Macrophage Populations Coexist across Tissues in Specific Subtissular Niches. Science 363 (6432). doi:10.1126/science.aau0964
Chizzolini, C., and Boin, F. (2015). The Role of the Acquired Immune Response in Systemic Sclerosis. Semin. Immunopathol 37 (5), 519–528. doi:10.1007/s00281-015-0509-1
Chouri, E., Wang, M., Hillen, M. R., Angiolilli, C., Silva-Cardoso, S. C., Wichers, C. G. K., et al. (2021). Implication of miR-126 and miR-139-5p in Plasmacytoid Dendritic Cell Dysregulation in Systemic Sclerosis. J. Clin. Med. 10 (3), 491. doi:10.3390/jcm10030491
Ciechomska, M., Huigens, C. A., Hügle, T., Stanly, T., Gessner, A., Griffiths, B., et al. (2013). Toll-like Receptor-Mediated, Enhanced Production of Profibrotic TIMP-1 in Monocytes from Patients with Systemic Sclerosis: Role of Serum Factors. Ann. Rheum. Dis. 72 (8), 1382–1389. doi:10.1136/annrheumdis-2012-201958
Distler, J. H. W., Györfi, A. H., Ramanujam, M., Whitfield, M. L., Königshoff, M., and Lafyatis, R. (2019). Shared and Distinct Mechanisms of Fibrosis. Nat. Rev. Rheumatol. 15 (12), 705–730. doi:10.1038/s41584-019-0322-7
Dowson, C., Simpson, N., Duffy, L., and O'Reilly, S. (2017). Innate Immunity in Systemic Sclerosis. Curr. Rheumatol. Rep. 19 (1), 2. doi:10.1007/s11926-017-0630-3
Driskell, R. R., Lichtenberger, B. M., Hoste, E., Kretzschmar, K., Simons, B. D., Charalambous, M., et al. (2013). Distinct Fibroblast Lineages Determine Dermal Architecture in Skin Development and Repair. Nature 504 (7479), 277–281. doi:10.1038/nature12783
Dufour, A. M., Borowczyk-Michalowska, J., Alvarez, M., Truchetet, M. E., Modarressi, A., Brembilla, N. C., et al. (2020). IL-17A Dissociates Inflammation from Fibrogenesis in Systemic Sclerosis. J. Invest. Dermatol. 140 (1), 103–e8. doi:10.1016/j.jid.2019.05.026
Dumoitier, N., Chaigne, B., Régent, A., Lofek, S., Mhibik, M., Dorfmüller, P., et al. (2017). Scleroderma Peripheral B Lymphocytes Secrete Interleukin-6 and Transforming Growth Factor β and Activate Fibroblasts. Arthritis Rheumatol. 69 (5), 1078–1089. doi:10.1002/art.40016
Ebmeier, S., and Horsley, V. (2015). Origin of Fibrosing Cells in Systemic Sclerosis. Curr. Opin. Rheumatol. 27 (6), 555–562. doi:10.1097/BOR.0000000000000217
Eyerich, S., Eyerich, K., Pennino, D., Carbone, T., Nasorri, F., Pallotta, S., et al. (2009). Th22 Cells Represent a Distinct Human T Cell Subset Involved in Epidermal Immunity and Remodeling. J. Clin. Invest. 119 (12), 3573–3585. doi:10.1172/JCI40202
Fleischmajer, R., Perlish, J. S., and Reeves, J. R. (1977). Cellular Infiltrates in Scleroderma Skin. Arthritis Rheum. 20 (4), 975–984. doi:10.1002/art.1780200410
Forestier, A., Guerrier, T., Jouvray, M., Giovannelli, J., Lefèvre, G., Sobanski, V., et al. (2018). Altered B Lymphocyte Homeostasis and Functions in Systemic Sclerosis. Autoimmun. Rev. 17 (3), 244–255. doi:10.1016/j.autrev.2017.10.015
Fox, D. A., Lundy, S. K., Whitfield, M. L., Berrocal, V., Campbell, P., Rasmussen, S., et al. (2021). Lymphocyte Subset Abnormalities in Early Diffuse Cutaneous Systemic Sclerosis. Arthritis Res. Ther. 23 (1), 10. doi:10.1186/s13075-020-02383-w
Fuschiotti, P., Larregina, A. T., Ho, J., Feghali-Bostwick, C., and Medsger, T. A. (2013). Interleukin-13-producing CD8+ T Cells Mediate Dermal Fibrosis in Patients with Systemic Sclerosis. Arthritis Rheum. 65 (1), 236–246. doi:10.1002/art.37706
Gabsi, A., Heim, X., Dlala, A., Gati, A., Sakhri, H., Abidi, A., et al. (2019). TH17 Cells Expressing CD146 Are Significantly Increased in Patients with Systemic Sclerosis. Sci. Rep. 9 (1), 17721. doi:10.1038/s41598-019-54132-y
Gao, X., Jia, G., Guttman, A., DePianto, D. J., Morshead, K. B., Sun, K. H., et al. (2020). Osteopontin Links Myeloid Activation and Disease Progression in Systemic Sclerosis. Cell Rep Med 1 (8), 100140. doi:10.1016/j.xcrm.2020.100140
Gasparini, G., Cozzani, E., and Parodi, A. (2020). Interleukin-4 and Interleukin-13 as Possible Therapeutic Targets in Systemic Sclerosis. Cytokine 125, 154799. doi:10.1016/j.cyto.2019.154799
Gaydosik, A. M., Tabib, T., Domsic, R., Khanna, D., Lafyatis, R., and Fuschiotti, P. (2021). Single-cell Transcriptome Analysis Identifies Skin-specific T-Cell Responses in Systemic Sclerosis. Ann. Rheum. Dis. 80 (11), 1453–1460. doi:10.1136/annrheumdis-2021-220209
Giacomelli, R., Matucci-Cerinic, M., Cipriani, P., Ghersetich, I., Lattanzio, R., Pavan, A., et al. (1998). Circulating Vdelta1+ T Cells Are Activated and Accumulate in the Skin of Systemic Sclerosis Patients. Arthritis Rheum. 41 (2), 327–334. doi:10.1002/1529-0131(199802)41:2<327:AID-ART17>3.0.CO;2-S
Gordon, J. K., Martyanov, V., Franks, J. M., Bernstein, E. J., Szymonifka, J., Magro, C., et al. (2018). Belimumab for the Treatment of Early Diffuse Systemic Sclerosis: Results of a Randomized, Double-Blind, Placebo-Controlled, Pilot Trial. Arthritis Rheumatol. 70 (2), 308–316. doi:10.1002/art.40358
Gordon, S. (2003). Alternative Activation of Macrophages. Nat. Rev. Immunol. 3 (1), 23–35. doi:10.1038/nri978
Gourh, P., Agarwal, S. K., Divecha, D., Assassi, S., Paz, G., Arora-Singh, R. K., et al. (2009). Polymorphisms in TBX21 and STAT4 Increase the Risk of Systemic Sclerosis: Evidence of Possible Gene-Gene Interaction and Alterations in Th1/Th2 Cytokines. Arthritis Rheum. 60 (12), 3794–3806. doi:10.1002/art.24958
Gu, Y. S., Kong, J., Cheema, G. S., Keen, C. L., Wick, G., and Gershwin, M. E. (2008). The Immunobiology of Systemic Sclerosis. Semin. Arthritis Rheum. 38 (2), 132–160. doi:10.1016/j.semarthrit.2007.10.010
Guggino, G., Lo Pizzo, M., Di Liberto, D., Rizzo, A., Cipriani, P., Ruscitti, P., et al. (2017). Interleukin-9 Over-expression and T Helper 9 Polarization in Systemic Sclerosis Patients. Clin. Exp. Immunol. 190 (2), 208–216. doi:10.1111/cei.13009
Guiducci, C., Tripodo, C., Gong, M., Sangaletti, S., Colombo, M. P., Coffman, R. L., et al. (2010). Autoimmune Skin Inflammation Is Dependent on Plasmacytoid Dendritic Cell Activation by Nucleic Acids via TLR7 and TLR9. J. Exp. Med. 207 (13), 2931–2942. doi:10.1084/jem.20101048
Guilliams, M., Ginhoux, F., Jakubzick, C., Naik, S. H., Onai, N., Schraml, B. U., et al. (2014). Dendritic Cells, Monocytes and Macrophages: a Unified Nomenclature Based on Ontogeny. Nat. Rev. Immunol. 14 (8), 571–578. doi:10.1038/nri3712
Hale, G. (2001). The CD52 Antigen and Development of the CAMPATH Antibodies. Cytotherapy 3 (3), 137–143. doi:10.1080/146532401753174098
Harrington, L. E., Hatton, R. D., Mangan, P. R., Turner, H., Murphy, T. L., Murphy, K. M., et al. (2005). Interleukin 17-producing CD4+ Effector T Cells Develop via a Lineage Distinct from the T Helper Type 1 and 2 Lineages. Nat. Immunol. 6 (11), 1123–1132. doi:10.1038/ni1254
Harris, D. P., Haynes, L., Sayles, P. C., Duso, D. K., Eaton, S. M., Lepak, N. M., et al. (2000). Reciprocal Regulation of Polarized Cytokine Production by Effector B and T Cells. Nat. Immunol. 1 (6), 475–482. doi:10.1038/82717
Henriques, A., Silva, C., Santiago, M., Henriques, M. J., Martinho, A., Trindade, H., et al. (2016). Subset-specific Alterations in Frequencies and Functional Signatures of γδ T Cells in Systemic Sclerosis Patients. Inflamm. Res. 65 (12), 985–994. doi:10.1007/s00011-016-0982-6
Higashi-Kuwata, N., Jinnin, M., Makino, T., Fukushima, S., Inoue, Y., Muchemwa, F. C., et al. (2010). Characterization of Monocyte/macrophage Subsets in the Skin and Peripheral Blood Derived from Patients with Systemic Sclerosis. Arthritis Res. Ther. 12 (4), R128. doi:10.1186/ar3066
Holcombe, R. F., Baethge, B. A., Wolf, R. E., Betzing, K. W., and Stewart, R. M. (1995). Natural Killer Cells and Gamma delta T Cells in Scleroderma: Relationship to Disease Duration and Anti-scl-70 Antibodies. Ann. Rheum. Dis. 54 (1), 69–72. doi:10.1136/ard.54.1.69
Hügle, T., O'Reilly, S., Simpson, R., Kraaij, M. D., Bigley, V., Collin, M., et al. (2013). Tumor Necrosis Factor-Costimulated T Lymphocytes from Patients with Systemic Sclerosis Trigger Collagen Production in Fibroblasts. Arthritis Rheum. 65 (2), 481–491. doi:10.1002/art.37738
Iozzo, R. V., Pillarisetti, J., Sharma, B., Murdoch, A. D., Danielson, K. G., Uitto, J., et al. (1997). Structural and Functional Characterization of the Human Perlecan Gene Promoter. Transcriptional Activation by Transforming Growth Factor-Beta via a Nuclear Factor 1-binding Element. J. Biol. Chem. 272 (8), 5219–5228. doi:10.1074/jbc.272.8.5219
Iwata, Y., Matsushita, T., Horikawa, M., Dilillo, D. J., Yanaba, K., Venturi, G. M., et al. (2011). Characterization of a Rare IL-10-competent B-Cell Subset in Humans that Parallels Mouse Regulatory B10 Cells. Blood 117 (2), 530–541. doi:10.1182/blood-2010-07-294249
Jaguin, M., Fardel, O., and Lecureur, V. (2015). AhR-dependent Secretion of PDGF-BB by Human Classically Activated Macrophages Exposed to DEP Extracts Stimulates Lung Fibroblast Proliferation. Toxicol. Appl. Pharmacol. 285 (3), 170–178. doi:10.1016/j.taap.2015.04.007
Jimenez, S. A. (2013). Role of Endothelial to Mesenchymal Transition in the Pathogenesis of the Vascular Alterations in Systemic Sclerosis. ISRN Rheumatol. 2013, 835948. doi:10.1155/2013/835948
Kahaleh, M. B., and Fan, P. S. (1997). Mechanism of Serum-Mediated Endothelial Injury in Scleroderma: Identification of a Granular Enzyme in Scleroderma Skin and Sera. Clin. Immunol. Immunopathol 83 (1), 32–40. doi:10.1006/clin.1996.4322
Kähäri, V. M., Sandberg, M., Kalimo, H., Vuorio, T., and Vuorio, E. (1988). Identification of Fibroblasts Responsible for Increased Collagen Production in Localized Scleroderma by In Situ Hybridization. J. Invest. Dermatol. 90 (5), 664–670. doi:10.1111/1523-1747.ep12560826
Kaplan, M. H., Hufford, M. M., and Olson, M. R. (2015). The Development and In Vivo Function of T Helper 9 Cells. Nat. Rev. Immunol. 15 (5), 295–307. doi:10.1038/nri3824
Kataoka, H., Yasuda, S., Fukaya, S., Oku, K., Horita, T., Atsumi, T., et al. (2015). Decreased Expression of Runx1 and Lowered Proportion of Foxp3+ CD25+ CD4+ Regulatory T Cells in Systemic Sclerosis. Mod. Rheumatol. 25 (1), 90–95. doi:10.3109/14397595.2014.899736
Khanna, D., Denton, C. P., Lin, C. J. F., van Laar, J. M., Frech, T. M., Anderson, M. E., et al. (2018). Safety and Efficacy of Subcutaneous Tocilizumab in Systemic Sclerosis: Results from the Open-Label Period of a Phase II Randomised Controlled Trial (faSScinate). Ann. Rheum. Dis. 77 (2), 212–220. doi:10.1136/annrheumdis-2017-211682
Khanna, D., Lin, C. J. F., Furst, D. E., Wagner, B., Zucchetto, M., Raghu, G., et al. (2021). Long-Term Safety and Efficacy of Tocilizumab in Early Systemic Sclerosis-Interstitial Lung Disease: Open Label Extension of a Phase 3 Randomized Controlled Trial. Am. J. Respir. Crit. Care Med. doi:10.1164/rccm.202103-0714OC
Khoder, A., Sarvaria, A., Alsuliman, A., Chew, C., Sekine, T., Cooper, N., et al. (2014). Regulatory B Cells Are Enriched within the IgM Memory and Transitional Subsets in Healthy Donors but Are Deficient in Chronic GVHD. Blood 124 (13), 2034–2045. doi:10.1182/blood-2014-04-571125
Klein, S., Kretz, C. C., Ruland, V., Stumpf, C., Haust, M., Hartschuh, W., et al. (2011). Reduction of Regulatory T Cells in Skin Lesions but Not in Peripheral Blood of Patients with Systemic Scleroderma. Ann. Rheum. Dis. 70 (8), 1475–1481. doi:10.1136/ard.2009.116525
Kobayashi, S., Nagafuchi, Y., Okubo, M., Sugimori, Y., Shirai, H., Hatano, H., et al. (2021). Integrated Bulk and Single-Cell RNA-Sequencing Identified Disease-Relevant Monocytes and a Gene Network Module Underlying Systemic Sclerosis. J. Autoimmun. 116, 102547. doi:10.1016/j.jaut.2020.102547
Korn, T., Bettelli, E., Oukka, M., and Kuchroo, V. K. (2009). IL-17 and Th17 Cells. Annu. Rev. Immunol. 27, 485–517. doi:10.1146/annurev.immunol.021908.132710
Kubo, S., Nakayamada, S., Miyazaki, Y., Yoshikawa, M., Yoshinari, H., Satoh, Y., et al. (2019). Distinctive Association of Peripheral Immune Cell Phenotypes with Capillaroscopic Microvascular Patterns in Systemic Sclerosis. Rheumatology (Oxford) 58 (12), 2273–2283. doi:10.1093/rheumatology/kez244
Kurasawa, K., Hirose, K., Sano, H., Endo, H., Shinkai, H., Nawata, Y., et al. (2000). Increased Interleukin-17 Production in Patients with Systemic Sclerosis. Arthritis Rheum. 43 (11), 2455–2463. doi:10.1002/1529-0131(200011)43:11<2455:AID-ANR12>3.0.CO;2-K
Kuzumi, A., Yoshizaki, A., Matsuda, K. M., Kotani, H., Norimatsu, Y., Fukayama, M., et al. (2021). Interleukin-31 Promotes Fibrosis and T Helper 2 Polarization in Systemic Sclerosis. Nat. Commun. 12 (1), 5947. doi:10.1038/s41467-021-26099-w
Lande, R., Mennella, A., Palazzo, R., Pietraforte, I., Stefanantoni, K., Iannace, N., et al. (2020). Anti-CXCL4 Antibody Reactivity Is Present in Systemic Sclerosis (SSc) and Correlates with the SSc Type I Interferon Signature. Int. J. Mol. Sci. 21 (14), 5102. doi:10.3390/ijms21145102
Laplante, P., Raymond, M. A., Gagnon, G., Vigneault, N., Sasseville, A. M., Langelier, Y., et al. (2005). Novel Fibrogenic Pathways Are Activated in Response to Endothelial Apoptosis: Implications in the Pathophysiology of Systemic Sclerosis. J. Immunol. 174 (9), 5740–5749. doi:10.4049/jimmunol.174.9.5740
Laurent, P., Sisirak, V., Lazaro, E., Richez, C., Duffau, P., Blanco, P., et al. (2018). Innate Immunity in Systemic Sclerosis Fibrosis: Recent Advances. Front. Immunol. 9, 1702. doi:10.3389/fimmu.2018.01702
Le Huu, D., Matsushita, T., Jin, G., Hamaguchi, Y., Hasegawa, M., Takehara, K., et al. (2013). Donor-derived Regulatory B Cells Are Important for Suppression of Murine Sclerodermatous Chronic Graft-Versus-Host Disease. Blood 121 (16), 3274–3283. doi:10.1182/blood-2012-11-465658
Lech, M., and Anders, H. J. (2013). Macrophages and Fibrosis: How Resident and Infiltrating Mononuclear Phagocytes Orchestrate All Phases of Tissue Injury and Repair. Biochim. Biophys. Acta 1832 (7), 989–997. doi:10.1016/j.bbadis.2012.12.001
Lee, D. S. W., Rojas, O. L., and Gommerman, J. L. (2021). B Cell Depletion Therapies in Autoimmune Disease: Advances and Mechanistic Insights. Nat. Rev. Drug Discov. 20 (3), 179–199. doi:10.1038/s41573-020-00092-2
Lei, L., Zhao, C., Qin, F., He, Z. Y., Wang, X., and Zhong, X. N. (2016). Th17 Cells and IL-17 Promote the Skin and Lung Inflammation and Fibrosis Process in a Bleomycin-Induced Murine Model of Systemic Sclerosis. Clin. Exp. Rheumatol. 34 (5), 14–22.
LeRoy, E. C., Black, C., Fleischmajer, R., Jablonska, S., Krieg, T., Medsger, T. A., et al. (1988). Scleroderma (Systemic Sclerosis): Classification, Subsets and Pathogenesis. J. Rheumatol. 15 (2), 202–205.
Lescoat, A., Lecureur, V., Roussel, M., Sunnaram, B. L., Ballerie, A., Coiffier, G., et al. (2017). CD16-positive Circulating Monocytes and Fibrotic Manifestations of Systemic Sclerosis. Clin. Rheumatol. 36 (7), 1649–1654. doi:10.1007/s10067-017-3597-6
Li, G., Larregina, A. T., Domsic, R. T., Stolz, D. B., Medsger, T. A., Lafyatis, R., et al. (2017). Skin-Resident Effector Memory CD8+CD28- T Cells Exhibit a Profibrotic Phenotype in Patients with Systemic Sclerosis. J. Invest. Dermatol. 137 (5), 1042–1050. doi:10.1016/j.jid.2016.11.037
Li, R., Rezk, A., Miyazaki, Y., Hilgenberg, E., Touil, H., Shen, P., et al. (2015). Proinflammatory GM-CSF-Producing B Cells in Multiple Sclerosis and B Cell Depletion Therapy. Sci. Transl Med. 7 (310), 310ra166. doi:10.1126/scitranslmed.aab4176
Liu, K., Victora, G. D., Schwickert, T. A., Guermonprez, P., Meredith, M. M., Yao, K., et al. (2009). In Vivo analysis of Dendritic Cell Development and Homeostasis. Science 324 (5925), 392–397. doi:10.1126/science.1170540
Liu, Q., Zaba, L., Satpathy, A. T., Longmire, M., Zhang, W., Li, K., et al. (2020). Chromatin Accessibility Landscapes of Skin Cells in Systemic Sclerosis Nominate Dendritic Cells in Disease Pathogenesis. Nat. Commun. 11 (1), 5843. doi:10.1038/s41467-020-19702-z
Liu, X., Gao, N., Li, M., Xu, D., Hou, Y., Wang, Q., et al. (2013). Elevated Levels of CD4(+)CD25(+)FoxP3(+) T Cells in Systemic Sclerosis Patients Contribute to the Secretion of IL-17 and Immunosuppression Dysfunction. PLoS One 8 (6), e64531. doi:10.1371/journal.pone.0064531
Luzina, I. G., Todd, N. W., Nacu, N., Lockatell, V., Choi, J., Hummers, L. K., et al. (2009). Regulation of Pulmonary Inflammation and Fibrosis through Expression of Integrins alphaVbeta3 and alphaVbeta5 on Pulmonary T Lymphocytes. Arthritis Rheum. 60 (5), 1530–1539. doi:10.1002/art.24435
Ly, N. T. M., Ueda-Hayakawa, I., Nguyen, C. T. H., Huynh, T. N. M., Kishimoto, I., Fujimoto, M., et al. (2021). Imbalance toward TFH 1 Cells Playing a Role in Aberrant B Cell Differentiation in Systemic Sclerosis. Rheumatology (Oxford) 60 (3), 1553–1562. doi:10.1093/rheumatology/keaa669
MacDonald, K. G., Dawson, N. A., Huang, Q., Dunne, J. V., Levings, M. K., and Broady, R. (2015). Regulatory T Cells Produce Profibrotic Cytokines in the Skin of Patients with Systemic Sclerosis. J. Allergy Clin. Immunol. 135 (4), 946–e9. doi:10.1016/j.jaci.2014.12.1932
Mackay, F., Schneider, P., Rennert, P., and Browning, J. (2003). BAFF and APRIL: a Tutorial on B Cell Survival. Annu. Rev. Immunol. 21, 231–264. doi:10.1146/annurev.immunol.21.120601.141152
Maehara, T., Kaneko, N., Perugino, C. A., Mattoo, H., Kers, J., Allard-Chamard, H., et al. (2020). Cytotoxic CD4+ T Lymphocytes May Induce Endothelial Cell Apoptosis in Systemic Sclerosis. J. Clin. Invest. 130 (5), 2451–2464. doi:10.1172/JCI131700
Marrapodi, R., Pellicano, C., Radicchio, G., Leodori, G., Colantuono, S., Iacolare, A., et al. (2020). CD21low B Cells in Systemic Sclerosis: A Possible Marker of Vascular Complications. Clin. Immunol. 213, 108364. doi:10.1016/j.clim.2020.108364
Martin, S. F. (2014). Adaptation in the Innate Immune System and Heterologous Innate Immunity. Cell Mol Life Sci 71 (21), 4115–4130. doi:10.1007/s00018-014-1676-2
Mathian, A., Parizot, C., Dorgham, K., Trad, S., Arnaud, L., Larsen, M., et al. (2012). Activated and Resting Regulatory T Cell Exhaustion Concurs with High Levels of Interleukin-22 Expression in Systemic Sclerosis Lesions. Ann. Rheum. Dis. 71 (7), 1227–1234. doi:10.1136/annrheumdis-2011-200709
Matsushita, T., Hamaguchi, Y., Hasegawa, M., Takehara, K., and Fujimoto, M. (2016). Decreased Levels of Regulatory B Cells in Patients with Systemic Sclerosis: Association with Autoantibody Production and Disease Activity. Rheumatology (Oxford) 55 (2), 263–267. doi:10.1093/rheumatology/kev331
Matsushita, T., Kobayashi, T., Mizumaki, K., Kano, M., Sawada, T., Tennichi, M., et al. (2018). BAFF Inhibition Attenuates Fibrosis in Scleroderma by Modulating the Regulatory and Effector B Cell Balance. Sci. Adv. 4 (7), eaas9944. doi:10.1126/sciadv.aas9944
Mattoo, H., Mahajan, V. S., Maehara, T., Deshpande, V., Della-Torre, E., Wallace, Z. S., et al. (2016). Clonal Expansion of CD4(+) Cytotoxic T Lymphocytes in Patients with IgG4-Related Disease. J. Allergy Clin. Immunol. 138 (3), 825–838. doi:10.1016/j.jaci.2015.12.1330
Mihara, M., Ohsugi, Y., and Kishimoto, T. (2011). Tocilizumab, a Humanized Anti-interleukin-6 Receptor Antibody, for Treatment of Rheumatoid Arthritis. Open Access Rheumatol. 3, 19–29. doi:10.2147/OARRR.S17118
Mohamed, M. E., Gamal, R. M., El-Mokhtar, M. A., Hassan, A. T., Abozaid, H. S. M., Ghandour, A. M., et al. (2021). Peripheral Cells from Patients with Systemic Sclerosis Disease Co-expressing M1 and M2 Monocyte/macrophage Surface Markers: Relation to the Degree of Skin Involvement. Hum. Immunol. 82 (9), 634–639. doi:10.1016/j.humimm.2021.03.009
Mosser, D. M., and Edwards, J. P. (2008). Exploring the Full Spectrum of Macrophage Activation. Nat. Rev. Immunol. 8 (12), 958–969. doi:10.1038/nri2448
Mosser, D. M. (2003). The many Faces of Macrophage Activation. J. Leukoc. Biol. 73 (2), 209–212. doi:10.1189/jlb.0602325
Mostmans, Y., Cutolo, M., Giddelo, C., Decuman, S., Melsens, K., Declercq, H., et al. (2017). The Role of Endothelial Cells in the Vasculopathy of Systemic Sclerosis: A Systematic Review. Autoimmun. Rev. 16 (8), 774–786. doi:10.1016/j.autrev.2017.05.024
Nakashima, T., Jinnin, M., Yamane, K., Honda, N., Kajihara, I., Makino, T., et al. (2012). Impaired IL-17 Signaling Pathway Contributes to the Increased Collagen Expression in Scleroderma Fibroblasts. J. Immunol. 188 (8), 3573–3583. doi:10.4049/jimmunol.1100591
O'Reilly, S., Hügle, T., and van Laar, J. M. (2012). T Cells in Systemic Sclerosis: a Reappraisal. Rheumatology (Oxford) 51 (9), 1540–1549. doi:10.1093/rheumatology/kes090
Okamoto, Y., Hasegawa, M., Matsushita, T., Hamaguchi, Y., Huu, D. L., Iwakura, Y., et al. (2012). Potential Roles of interleukin-17A in the Development of Skin Fibrosis in Mice. Arthritis Rheum. 64 (11), 3726–3735. doi:10.1002/art.34643
Ototake, Y., Yamaguchi, Y., Asami, M., Komitsu, N., Akita, A., Watanabe, T., et al. (2021). Downregulated IRF8 in Monocytes and Macrophages of Patients with Systemic Sclerosis May Aggravate the Fibrotic Phenotype. J. Invest. Dermatol. 141 (8), 1954–1963. doi:10.1016/j.jid.2021.02.015
Park, H., Li, Z., Yang, X. O., Chang, S. H., Nurieva, R., Wang, Y. H., et al. (2005). A Distinct Lineage of CD4 T Cells Regulates Tissue Inflammation by Producing Interleukin 17. Nat. Immunol. 6 (11), 1133–1141. doi:10.1038/ni1261
Park, M. J., Moon, S. J., Lee, E. J., Jung, K. A., Kim, E. K., Kim, D. S., et al. (2018). IL-1-IL-17 Signaling Axis Contributes to Fibrosis and Inflammation in Two Different Murine Models of Systemic Sclerosis. Front. Immunol. 9, 1611. doi:10.3389/fimmu.2018.01611
Passlick, B., Flieger, D., and Ziegler-Heitbrock, H. W. (1989). Identification and Characterization of a Novel Monocyte Subpopulation in Human Peripheral Blood. Blood 74 (7), 2527–2534. doi:10.1182/blood.v74.7.2527.bloodjournal7472527
Perez-Andres, M., Paiva, B., Nieto, W. G., Caraux, A., Schmitz, A., Almeida, J., et al. (2010). Human Peripheral Blood B-Cell Compartments: a Crossroad in B-Cell Traffic. Cytometry B Clin. Cytom 78 (Suppl. 1), S47–S60. doi:10.1002/cyto.b.20547
Peyser, R., MacDonnell, S., Gao, Y., Cheng, L., Kim, Y., Kaplan, T., et al. (2019). Defining the Activated Fibroblast Population in Lung Fibrosis Using Single-Cell Sequencing. Am. J. Respir. Cel Mol Biol 61 (1), 74–85. doi:10.1165/rcmb.2018-0313OC
Pilling, D., Akbar, A. N., Girdlestone, J., Orteu, C. H., Borthwick, N. J., Amft, N., et al. (1999). Interferon-beta Mediates Stromal Cell rescue of T Cells from Apoptosis. Eur. J. Immunol. 29 (3), 1041–1050. doi:10.1002/(SICI)1521-4141(199903)29:03<1041:AID-IMMU1041>3.0.CO;2-#
Pociask, D. A., Chen, K., Choi, S. M., Oury, T. D., Steele, C., and Kolls, J. K. (2011). Γδ T Cells Attenuate Bleomycin-Induced Fibrosis through the Production of CXCL10. Am. J. Pathol. 178 (3), 1167–1176. doi:10.1016/j.ajpath.2010.11.055
Radstake, T. R., van Bon, L., Broen, J., Wenink, M., Santegoets, K., Deng, Y., et al. (2009). Increased Frequency and Compromised Function of T Regulatory Cells in Systemic Sclerosis (SSc) Is Related to a Diminished CD69 and TGFbeta Expression. PLoS One 4 (6), e5981. doi:10.1371/journal.pone.0005981
Ratzinger, G., Reagan, J. L., Heller, G., Busam, K. J., and Young, J. W. (2003). Differential CD52 Expression by Distinct Myeloid Dendritic Cell Subsets: Implications for Alemtuzumab Activity at the Level of Antigen Presentation in Allogeneic Graft-Host Interactions in Transplantation. Blood 101 (4), 1422–1429. doi:10.1182/blood-2002-04-1093
Ricard, L., Jachiet, V., Malard, F., Ye, Y., Stocker, N., Rivière, S., et al. (2019). Circulating Follicular Helper T Cells Are Increased in Systemic Sclerosis and Promote Plasmablast Differentiation through the IL-21 Pathway Which Can Be Inhibited by Ruxolitinib. Ann. Rheum. Dis. 78 (4), 539–550. doi:10.1136/annrheumdis-2018-214382
Ricard, L., Malard, F., Riviere, S., Laurent, C., Fain, O., Mohty, M., et al. (2021). Regulatory B Cell Imbalance Correlates with Tfh Expansion in Systemic Sclerosis. Clin. Exp. Rheumatol. 39 (4), 20–24.
Rice, L. M., Stifano, G., Ziemek, J., and Lafyatis, R. (2016). Local Skin Gene Expression Reflects Both Local and Systemic Skin Disease in Patients with Systemic Sclerosis. Rheumatology (Oxford) 55 (2), 377–379. doi:10.1093/rheumatology/kev335
Robak, E., Gerlicz-Kowalczuk, Z., Dziankowska-Bartkowiak, B., Wozniacka, A., and Bogaczewicz, J. (2019). Serum Concentrations of IL-17A, IL-17B, IL-17E and IL-17F in Patients with Systemic Sclerosis. Arch. Med. Sci. 15 (3), 706–712. doi:10.5114/aoms.2019.84738
Rosenbloom, J., Feldman, G., Freundlich, B., and Jimenez, S. A. (1986). Inhibition of Excessive Scleroderma Fibroblast Collagen Production by Recombinant Gamma-Interferon. Association with a Coordinate Decrease in Types I and III Procollagen Messenger RNA Levels. Arthritis Rheum. 29 (7), 851–856. doi:10.1002/art.1780290706
Ross, R. L., Corinaldesi, C., Migneco, G., Carr, I. M., Antanaviciute, A., Wasson, C. W., et al. (2021). Targeting Human Plasmacytoid Dendritic Cells through BDCA2 Prevents Skin Inflammation and Fibrosis in a Novel Xenotransplant Mouse Model of Scleroderma. Ann. Rheum. Dis. 80 (7), 920–929. doi:10.1136/annrheumdis-2020-218439
Rossato, M., Affandi, A. J., Thordardottir, S., Wichers, C. G. K., Cossu, M., Broen, J. C. A., et al. (2017). Association of MicroRNA-618 Expression with Altered Frequency and Activation of Plasmacytoid Dendritic Cells in Patients with Systemic Sclerosis. Arthritis Rheumatol. 69 (9), 1891–1902. doi:10.1002/art.40163
Rudnik, M., Hukara, A., Kocherova, I., Jordan, S., Schniering, J., Milleret, V., et al. (2021). Elevated Fibronectin Levels in Profibrotic CD14+ Monocytes and CD14+ Macrophages in Systemic Sclerosis. Front. Immunol. 12, 642891. doi:10.3389/fimmu.2021.642891
Rudnik, M., Rolski, F., Jordan, S., Mertelj, T., Stellato, M., Distler, O., et al. (2021). Regulation of Monocyte Adhesion and Type I Interferon Signaling by CD52 in Patients with Systemic Sclerosis. Arthritis Rheumatol. 73 (9), 1720–1730. doi:10.1002/art.41737
Russomanno, G., Jo, K. B., Abdul-Salam, V. B., Morgan, C., Endruschat, J., Schaeper, U., et al. (2021). miR-150-PTPMT1-cardiolipin Signaling in Pulmonary Arterial Hypertension. Mol. Ther. Nucleic Acids 23, 142–153. doi:10.1016/j.omtn.2020.10.042
Sato, S., Hasegawa, M., and Takehara, K. (2001). Serum Levels of Interleukin-6 and Interleukin-10 Correlate with Total Skin Thickness Score in Patients with Systemic Sclerosis. J. Dermatol. Sci. 27 (2), 140–146. doi:10.1016/s0923-1811(01)00128-1
Satoh, T., Nakagawa, K., Sugihara, F., Kuwahara, R., Ashihara, M., Yamane, F., et al. (2017). Identification of an Atypical Monocyte and Committed Progenitor Involved in Fibrosis. Nature 541 (7635), 96–101. doi:10.1038/nature20611
Schneider, L., Marcondes, N. A., Hax, V., da Silva Moreira, I. F., Ueda, C. Y., Piovesan, R. R., et al. (2021). Flow Cytometry Evaluation of CD14/CD16 Monocyte Subpopulations in Systemic Sclerosis Patients: a Cross Sectional Controlled Study. Adv. Rheumatol. 61 (1), 27. doi:10.1186/s42358-021-00182-8
Servaas, N. H., Zaaraoui-Boutahar, F., Wichers, C. G. K., Ottria, A., Chouri, E., Affandi, A. J., et al. (2021). Longitudinal Analysis of T-Cell Receptor Repertoires Reveals Persistence of Antigen-Driven CD4+ and CD8+ T-Cell Clusters in Systemic Sclerosis. J. Autoimmun. 117, 102574. doi:10.1016/j.jaut.2020.102574
Shapouri-Moghaddam, A., Mohammadian, S., Vazini, H., Taghadosi, M., Esmaeili, S. A., Mardani, F., et al. (2018). Macrophage Plasticity, Polarization, and Function in Health and Disease. J. Cel Physiol 233 (9), 6425–6440. doi:10.1002/jcp.26429
Sharma, B., Handler, M., Eichstetter, I., Whitelock, J. M., Nugent, M. A., and Iozzo, R. V. (1998). Antisense Targeting of Perlecan Blocks Tumor Growth and Angiogenesis In Vivo. J. Clin. Invest. 102 (8), 1599–1608. doi:10.1172/JCI3793
Shen, P., and Fillatreau, S. (2015). Antibody-independent Functions of B Cells: a Focus on Cytokines. Nat. Rev. Immunol. 15 (7), 441–451. doi:10.1038/nri3857
Shi, C., and Pamer, E. G. (2011). Monocyte Recruitment during Infection and Inflammation. Nat. Rev. Immunol. 11 (11), 762–774. doi:10.1038/nri3070
Siegal, F. P., Kadowaki, N., Shodell, M., Fitzgerald-Bocarsly, P. A., Shah, K., Ho, S., et al. (1999). The Nature of the Principal Type 1 Interferon-Producing Cells in Human Blood. Science 284 (5421), 1835–1837. doi:10.1126/science.284.5421.1835
Simon, D., Balogh, P., Erdő-Bonyár, S., Böröcz, K., Minier, T., Czirják, L., et al. (2021). Increased Frequency of Activated Switched Memory B Cells and its Association with the Presence of Pulmonary Fibrosis in Diffuse Cutaneous Systemic Sclerosis Patients. Front. Immunol. 12, 686483. doi:10.3389/fimmu.2021.686483
Skaug, B., Khanna, D., Swindell, W. R., Hinchcliff, M. E., Frech, T. M., Steen, V. D., et al. (2020). Global Skin Gene Expression Analysis of Early Diffuse Cutaneous Systemic Sclerosis Shows a Prominent Innate and Adaptive Inflammatory Profile. Ann. Rheum. Dis. 79 (3), 379–386. doi:10.1136/annrheumdis-2019-215894
Steen, V. D. (2005). Autoantibodies in Systemic Sclerosis. Semin. Arthritis Rheum. 35 (1), 35–42. doi:10.1016/j.semarthrit.2005.03.005
Streicher, K., Sridhar, S., Kuziora, M., Morehouse, C. A., Higgs, B. W., Sebastian, Y., et al. (2018). Baseline Plasma Cell Gene Signature Predicts Improvement in Systemic Sclerosis Skin Scores Following Treatment with Inebilizumab (MEDI-551) and Correlates with Disease Activity in Systemic Lupus Erythematosus and Chronic Obstructive Pulmonary Disease. Arthritis Rheumatol. 70 (12), 2087–2095. doi:10.1002/art.40656
Swiecki, M., and Colonna, M. (2015). The Multifaceted Biology of Plasmacytoid Dendritic Cells. Nat. Rev. Immunol. 15 (8), 471–485. doi:10.1038/nri3865
Tabib, T., Huang, M., Morse, N., Papazoglou, A., Behera, R., Jia, M., et al. (2021). Myofibroblast Transcriptome Indicates SFRP2hi Fibroblast Progenitors in Systemic Sclerosis Skin. Nat. Commun. 12 (1), 4384. doi:10.1038/s41467-021-24607-6
Tabib, T., Morse, C., Wang, T., Chen, W., and Lafyatis, R. (2018). SFRP2/DPP4 and FMO1/LSP1 Define Major Fibroblast Populations in Human Skin. J. Invest. Dermatol. 138 (4), 802–810. doi:10.1016/j.jid.2017.09.045
Taylor, D. K., Mittereder, N., Kuta, E., Delaney, T., Burwell, T., Dacosta, K., et al. (2018). T Follicular Helper-like Cells Contribute to Skin Fibrosis. Sci. Transl Med. 10 (431), eaaf5307. doi:10.1126/scitranslmed.aaf5307
Tezcan, D., Sivrikaya, A., Ergün, D., Özer, H., Eryavuz Onmaz, D., Körez, M. K., et al. (2021). Evaluation of Serum Interleukin-6 (IL-6), IL-13, and IL-17 Levels and Computed Tomography Finding in Interstitial Lung Disease Associated with Connective Tissue Disease Patients. Clin. Rheumatol. 40 (11), 4713–4724. doi:10.1007/s10067-021-05773-w
Toledo, D. M., and Pioli, P. A. (2019). Macrophages in Systemic Sclerosis: Novel Insights and Therapeutic Implications. Curr. Rheumatol. Rep. 21 (7), 31. doi:10.1007/s11926-019-0831-z
Trombetta, A. C., Soldano, S., Contini, P., Tomatis, V., Ruaro, B., Paolino, S., et al. (2018). A Circulating Cell Population Showing Both M1 and M2 Monocyte/macrophage Surface Markers Characterizes Systemic Sclerosis Patients with Lung Involvement. Respir. Res. 19 (1), 186. doi:10.1186/s12931-018-0891-z
Truchetet, M. E., Brembilla, N. C., Montanari, E., Allanore, Y., and Chizzolini, C. (2011). Increased Frequency of Circulating Th22 in Addition to Th17 and Th2 Lymphocytes in Systemic Sclerosis: Association with Interstitial Lung Disease. Arthritis Res. Ther. 13 (5), R166. doi:10.1186/ar3486
Tsou, P. S., Palisoc, P. J., Ali, M., Khanna, D., and Sawalha, A. H. (2021). Genome-Wide Reduction in Chromatin Accessibility and Unique Transcription Factor Footprints in Endothelial Cells and Fibroblasts in Scleroderma Skin. Arthritis Rheumatol. 73 (8), 1501–1513. doi:10.1002/art.41694
Tsukui, T., Sun, K. H., Wetter, J. B., Wilson-Kanamori, J. R., Hazelwood, L. A., Henderson, N. C., et al. (2020). Collagen-producing Lung Cell Atlas Identifies Multiple Subsets with Distinct Localization and Relevance to Fibrosis. Nat. Commun. 11 (1), 1920. doi:10.1038/s41467-020-15647-5
Ueda-Hayakawa, I., Hasegawa, M., Hamaguchi, Y., Takehara, K., and Fujimoto, M. (2013). Circulating γ/δ T Cells in Systemic Sclerosis Exhibit Activated Phenotype and Enhance Gene Expression of proalpha2(I) Collagen of Fibroblasts. J. Dermatol. Sci. 69 (1), 54–60. doi:10.1016/j.jdermsci.2012.10.003
Ugor, E., Simon, D., Almanzar, G., Pap, R., Najbauer, J., Németh, P., et al. (2017). Increased Proportions of Functionally Impaired Regulatory T Cell Subsets in Systemic Sclerosis. Clin. Immunol. 184, 54–62. doi:10.1016/j.clim.2017.05.013
Valenzi, E., Bulik, M., Tabib, T., Morse, C., Sembrat, J., Trejo Bittar, H., et al. (2019). Single-cell Analysis Reveals Fibroblast Heterogeneity and Myofibroblasts in Systemic Sclerosis-Associated Interstitial Lung Disease. Ann. Rheum. Dis. 78 (10), 1379–1387. doi:10.1136/annrheumdis-2018-214865
Valenzi, E., Tabib, T., Papazoglou, A., Sembrat, J., Trejo Bittar, H. E., Rojas, M., et al. (2021). Disparate Interferon Signaling and Shared Aberrant Basaloid Cells in Single-Cell Profiling of Idiopathic Pulmonary Fibrosis and Systemic Sclerosis-Associated Interstitial Lung Disease. Front. Immunol. 12, 595811. doi:10.3389/fimmu.2021.595811
van Bon, L., Affandi, A. J., Broen, J., Christmann, R. B., Marijnissen, R. J., Stawski, L., et al. (2014). Proteome-wide Analysis and CXCL4 as a Biomarker in Systemic Sclerosis. N. Engl. J. Med. 370 (5), 433–443. doi:10.1056/NEJMoa1114576
Veldhoen, M., Uyttenhove, C., van Snick, J., Helmby, H., Westendorf, A., Buer, J., et al. (2008). Transforming Growth Factor-Beta 'reprograms' the Differentiation of T Helper 2 Cells and Promotes an Interleukin 9-producing Subset. Nat. Immunol. 9 (12), 1341–1346. doi:10.1038/ni.1659
Visentini, M., Pellicano, C., Leodori, G., Marrapodi, R., Colantuono, S., Gigante, A., et al. (2021). CD21low B Cells Are Predictive Markers of New Digital Ulcers in Systemic Sclerosis. Clin. Exp. Immunol. 205 (2), 128–134. doi:10.1111/cei.13604
Whitfield, M. L., Finlay, D. R., Murray, J. I., Troyanskaya, O. G., Chi, J. T., Pergamenschikov, A., et al. (2003). Systemic and Cell Type-specific Gene Expression Patterns in Scleroderma Skin. Proc. Natl. Acad. Sci. U S A. 100 (21), 12319–12324. doi:10.1073/pnas.1635114100
Wohlfahrt, T., Rauber, S., Uebe, S., Luber, M., Soare, A., Ekici, A., et al. (2019). PU.1 Controls Fibroblast Polarization and Tissue Fibrosis. Nature 566 (7744), 344–349. doi:10.1038/s41586-019-0896-x
Worrell, J. C., and O'Reilly, S. (2020). Bi-directional Communication: Conversations between Fibroblasts and Immune Cells in Systemic Sclerosis. J. Autoimmun. 113, 102526. doi:10.1016/j.jaut.2020.102526
Wynn, T. A. (2004). Fibrotic Disease and the T(H)1/T(H)2 Paradigm. Nat. Rev. Immunol. 4 (8), 583–594. doi:10.1038/nri1412
Xia, M. Q., Hale, G., Lifely, M. R., Ferguson, M. A., Campbell, D., Packman, L., et al. (1993). Structure of the CAMPATH-1 Antigen, a Glycosylphosphatidylinositol-Anchored Glycoprotein Which Is an Exceptionally Good Target for Complement Lysis. Biochem. J. 293 (Pt 3), 633–640. doi:10.1042/bj2930633
Xing, X., Li, A., Tan, H., and Zhou, Y. (2020). IFN-γ+ IL-17+ Th17 Cells Regulate Fibrosis through Secreting IL-21 in Systemic Scleroderma. J. Cel Mol Med 24 (23), 13600–13608. doi:10.1111/jcmm.15266
Xing, X., Yang, J., Yang, X., Wei, Y., Zhu, L., Gao, D., et al. (2013). IL-17A Induces Endothelial Inflammation in Systemic Sclerosis via the ERK Signaling Pathway. PLoS One 8 (12), e85032. doi:10.1371/journal.pone.0085032
Xu, H., Zaidi, M., Struve, J., Jones, D. W., Krolikowski, J. G., Nandedkar, S., et al. (2011). Abnormal Fibrillin-1 Expression and Chronic Oxidative Stress Mediate Endothelial Mesenchymal Transition in a Murine Model of Systemic Sclerosis. Am. J. Physiol. Cel Physiol 300 (3), C550–C556. doi:10.1152/ajpcell.00123.2010
Xue, D., Tabib, T., Morse, C., and Lafyatis, R. (2020). Transcriptome Landscape of Myeloid Cells in Human Skin Reveals Diversity, Rare Populations and Putative DC Progenitors. J. Dermatol. Sci. 97 (1), 41–49. doi:10.1016/j.jdermsci.2019.11.012
Xue, D., Tabib, T., Morse, C., Yang, Y., Domsic, R., Khanna, D., et al. (2021). Expansion of FCGR3A + Macrophages, FCN1 + mo‐DC, and Plasmacytoid Dendritic Cells Associated with Severe Skin Disease in Systemic Sclerosis. Arthritis Rheumatol. doi:10.1002/art.41813
Yanaba, K., Yoshizaki, A., Asano, Y., Kadono, T., and Sato, S. (2011). Serum Interleukin 9 Levels Are Increased in Patients with Systemic Sclerosis: Association with Lower Frequency and Severity of Pulmonary Fibrosis. J. Rheumatol. 38 (10), 2193–2197. doi:10.3899/jrheum.110268
Yang, C., Lei, L., Pan, J., Zhao, C., Wen, J., Qin, F., et al. (2021). Altered CD4+ T Cell and Cytokine Levels in Peripheral Blood and Skin Samples from Systemic Sclerosis Patients and IL-35 in CD4+ T Cell Growth. Rheumatology (Oxford). doi:10.1093/rheumatology/keab359
Yang, X., Yang, J., Xing, X., Wan, L., and Li, M. (2014). Increased Frequency of Th17 Cells in Systemic Sclerosis Is Related to Disease Activity and Collagen Overproduction. Arthritis Res. Ther. 16 (1), R4. doi:10.1186/ar4430
Yao, W., Zhang, Y., Jabeen, R., Nguyen, E. T., Wilkes, D. S., Tepper, R. S., et al. (2013). Interleukin-9 Is Required for Allergic Airway Inflammation Mediated by the Cytokine TSLP. Immunity 38 (2), 360–372. doi:10.1016/j.immuni.2013.01.007
Yaseen, B., Lopez, H., Taki, Z., Zafar, S., Rosario, H., Abdi, B. A., et al. (2020). Interleukin-31 Promotes Pathogenic Mechanisms Underlying Skin and Lung Fibrosis in Scleroderma. Rheumatology (Oxford) 59 (9), 2625–2636. doi:10.1093/rheumatology/keaa195
Yurovsky, V. V., Wigley, F. M., Wise, R. A., and White, B. (1996). Skewing of the CD8+ T-Cell Repertoire in the Lungs of Patients with Systemic Sclerosis. Hum. Immunol. 48 (1-2), 84–97. doi:10.1016/0198-8859(96)00091-2
Zambrano-Zaragoza, J. F., Romo-Martínez, E. J., Durán-Avelar, Mde. J., García-Magallanes, N., and Vibanco-Pérez, N. (2014). Th17 Cells in Autoimmune and Infectious Diseases. Int. J. Inflam 2014, 651503. doi:10.1155/2014/651503
Zhang, J. H., Deng, J. H., Yao, X. L., Wang, J. L., and Xiao, J. H. (2020). CD4+CD25+ Tregs as Dependent Factor in the Course of Bleomycin-Induced Pulmonary Fibrosis in Mice. Exp. Cel Res 386 (1), 111700. doi:10.1016/j.yexcr.2019.111700
Zhang, M., Srivastava, G., and Lu, L. (2004). The Pre-B Cell Receptor and its Function during B Cell Development. Cell Mol Immunol 1 (2), 89–94.
Zhu, J., and Paul, W. E. (2008). CD4 T Cells: Fates, Functions, and Faults. Blood 112 (5), 1557–1569. doi:10.1182/blood-2008-05-078154
Zhu, L., Yin, Z., Ju, B., Zhang, J., Wang, Y., Lv, X., et al. (2018). Altered Frequencies of Memory B Cells in New-Onset Systemic Lupus Erythematosus Patients. Clin. Rheumatol. 37 (1), 205–212. doi:10.1007/s10067-017-3877-1
Glossary
AM alveolar macrophages
anti-Scl-70 anti-topoisomerase I
APLNR Apelin receptor
APRIL A proliferation-inducing ligand
ASM activated switched memory
ATAC-seq assay of transposase accessible chromatin with sequencing
BAFF B-cell–activating-factor
BCRs B-cell receptors
Beffs Effector B cells
Bregs regulatory B cells
cDCs conventional dendritic cells
CDP common DC precursor
CTGF connective tissue growth factor
CTHRC1 collagen triple helix repeat containing 1
DCs Dendritic cells
DN double-negative
ECM extracellular matrix
ECs endothelial cells
EndMT endothelial-to-mesenchymal transition
FGF fibroblast growth factor
FoxP3 forkhead box transcription factor
GO Gene Ontology;
GSEA Gene Set Enrichment Analysis
GVHD graft-versus-host disease
HC healthy control
HDAC IIa histone deacetylase IIa
HSPG2 heparan sulfate proteoglycan 2;
IFN type I interferon;
IM interstitial macrophages
IPA Ingenuity Pathway Analysis
IPF idiopathic pulmonary fibrosis
IRF8 interferon regulatory factor 8
ISH in situ hybridization
Lyve1 lymphatic endothelium hyaluronan receptor-1
MDMs monocytes-derived macrophages
MMP-1 matrix metalloproteinase-1
mRSS modified Rodnan skin score
MSR1 macrophage scavenger receptor 1
NOD/SCID nonobese diabetic/severe combined immunodeficiency
OPN osteopontin
PAH pulmonary arterial hypertension
PBMCs peripheral blood mononuclear cells
pDCs plasmacytoid dendritic cells
PTPMT1 PTEN-like mitochondrial phosphatase
Runx1 runt-related transcription factor 1
Scl-cGVHD sclerodermatous cGVHD
scRNA-seq single-cell RNA sequencing
SFRP secreted frizzled–related protein
SLE systemic lupus erythematosus
SNPs single-nucleotide polymorphisms
SSc Systemic sclerosis
SSc-ILD SSc-associated interstitial lung disease
TCRs T-cell receptors
Tfh T follicular helper
TGF-β transforming growth factor-beta
Th T helper
TIM-1 T-cell Ig and mucin domain protein 1
TLOs tertiary lymphoid structures
TLRs toll-like receptors
Tregs regulatory T cells
TSLP thymic stromal lymphopoietin
Keywords: systemic sclerosis, ScRNA-seq, immune cells, fibroblasts, endothelial cells
Citation: Dai B, Ding L, Zhao L, Zhu H and Luo H (2022) Contributions of Immune Cells and Stromal Cells to the Pathogenesis of Systemic Sclerosis: Recent Insights. Front. Pharmacol. 13:826839. doi: 10.3389/fphar.2022.826839
Received: 01 December 2021; Accepted: 04 January 2022;
Published: 03 February 2022.
Edited by:
Raffaele Strippoli, Sapienza University of Rome, ItalyReviewed by:
Toshiyuki Yamamoto, Fukushima Medical University, JapanSteven O'Reilly, STipe Therapeutics, Denmark
Copyright © 2022 Dai, Ding, Zhao, Zhu and Luo. This is an open-access article distributed under the terms of the Creative Commons Attribution License (CC BY). The use, distribution or reproduction in other forums is permitted, provided the original author(s) and the copyright owner(s) are credited and that the original publication in this journal is cited, in accordance with accepted academic practice. No use, distribution or reproduction is permitted which does not comply with these terms.
*Correspondence: Honglin Zhu, aG9uZ2xpbnpodUBjc3UuZWR1LmNu; Hui Luo, bHVvaHVpQGNzdS5lZHUuY24=