- 1Department of Pharmacology, Therapeutics and Toxicology, School of Medicine, Universitat Autònoma de Barcelona, Barcelona, Spain
- 2Institut de Neurociències, Universitat Autònoma de Barcelona, Barcelona, Spain
- 3Laboratory of Medicinal Chemistry (Associated Unit to CSIC), Department of Pharmacology, Toxicology and Medicinal Chemistry, Faculty of Pharmacy and Food Sciences, Institute of Biomedicine (IBUB), University of Barcelona, Barcelona, Spain
- 4Department of Psychiatry and Forensic Medicine, School of Medicine, Universitat Autònoma de Barcelona, Barcelona, Spain
- 5Department of Pharmacology, School of Medicine, Universidad de Valencia, Burjassot, Spain
- 6Estructura de Recerca Interdisciplinar en Biotecnologia i Biomedicina (ERI BIOTECMED), Universidad de Valencia, Valencia, Spain
- 7Pharmacology Section, Toxicology and Medicinal Chemistry, Faculty of Pharmacy and Food Sciences, Institut de Neurociències, University of Barcelona, Barcelona, Spain
Imidazoline receptors (IR) are classified into three receptor subtypes (I1R, I2R, and I3R) and previous studies showed that regulation of I2R signaling has neuroprotective potential. In order to know if I2R has a role in modulating vascular tone in health and disease, we evaluated the putative vasoactive effects of two recently synthesized I2R ligands, diethyl (1RS,3aSR,6aSR)-5-(3-chloro-4-fluorophenyl)-4,6-dioxo-1-phenyl-1,3a,4,5,6,6a-hexahydropyrrolo[3,4-c]pyrrole -1-phosphonate (B06) and diethyl [(1-(3-chloro-4-fluorobenzyl)-5,5-dimethyl-4-phenyl-4,5-dihydro-1H-imidazol-4-yl]phosphonate] (MCR5). Thoracic aortas from Oncins France 1 (3- to 4-months-old) and C57BL/6 (3- to 4- and 16- to 17-months-old mice) were mounted in tissue baths to measure isometric tension. In young mice of both strains, MCR5 induced greater relaxations than either B06 or the high-affinity I2R selective ligand 2-(2-benzofuranyl)-2-imidazoline (2-BFI), which evoked marginal responses. MCR5 relaxations were independent of I2R, as IR ligands did not significantly affect them, involved activation of smooth muscle KATP channels and inhibition of L-type voltage-gated Ca2+ channels, and were only slightly modulated by endothelium-derived nitric oxide (negatively) and prostacyclin (positively). Notably, despite the presence of endothelial dysfunction in old mice, MCR5 relaxations were preserved. In conclusion, the present study provides evidence against a functional contribution of I2R in the modulation of vascular tone in the mouse aorta. Moreover, the I2R ligand MCR5 is an endothelium-independent vasodilator that acts largely via I2R-independent pathways and is resistant to aging. We propose MCR5 as a candidate drug for the management of vascular disease in the elderly.
Introduction
Aging is a major risk factor in developing vascular disease and, consequently, strategies for achieving healthy vascular aging are encouraged. Endothelial dysfunction is defined as an impairment of endothelium-dependent vasodilation, a phenomenon that is frequently found in the aging vasculature (Ungvari et al., 2018). The endothelium is highly sensitive to changes in physical and chemical environmental conditions. Physiological levels of some endothelium-derived vasoactive mediators, such as NO and prostanoids, are crucial for normal endothelial function, and thus, their imbalance in situations of, for example, oxidative and nitrosative stress, could result in vascular dysfunction (El Assar et al., 2012). To address this problem, strategies usually seek to recover physiological levels of endothelium-derived mediators. Nevertheless, alternative approaches such as to potentiating endothelium-independent vasodilation could provide a complementary route to circumvent endothelial dysfunction.
Imidazoline receptors (IR) were identified around 40 years ago, but their molecular identity is not known. They recognize ligands containing an imidazoline nucleus or structurally related moiety (Bousquet et al., 1984; Ernsberger et al., 1987; Ernsberger et al., 1990). Subsequent studies classified these binding sites into three receptor subtypes (I1R, I2R, and I3R) according to different functional and binding studies (Michel and Ernsberger, 1992; Chan et al., 1994). The I2R selective ligand CR4056 has entered clinical trials to treat chronic pain (Ferrari et al., 2011; Li, 2017). In addition, stimulation of I2R has shown neuroprotective potential in stroke (Zhang et al., 2020). but few studies have focused on the potential effects of vascular I2R modulation. Some of them have found a link between I2R, vascular relaxation, and antihypertensive effects (Mar et al., 2013; Chen et al., 2014).
Agmatine, the putative endogenous non-selective IR ligand (Piletz et al., 1995; Santhanam et al., 2007; Mar et al., 2013; Chen et al., 2014) induces relaxation mediated by activation of I2R and opening of ATP-sensitive K+ (KATP) channels in the aorta of Wistar (Chen et al., 2014) and spontaneously hypertensive (Mar et al., 2013) rats. In contrast, I2R are not involved in agmatine-induced responses in the Sprague-Dawley rat aorta, which are mediated by NO, small conductance Ca2+-activated K+ channels, KATP and inward-rectifying K+ channels (Santhanam et al., 2007). The non-selectivity of agmatine may explain, at least partly, discrepancies in its mechanism of action across rat strains, as well as its multi-target effects (Santhanam et al., 2007; Mar et al., 2013; Chen et al., 2014). However, this evidence may also suggest that IR ligands may activate multiple pathways in the vasculature different from I2R, and these actions could contribute to their therapeutic effects. On the whole, modulation of I2R activity may have potentially interesting clinical applications in vascular disease. Notably, IR research has embarked on its second youth after recent evidence on the therapeutic potential of newly synthesized selective I2R ligands as neuroptotective agents (Ferrari et al., 2011; Abás et al., 2017; Griñán-Ferré et al., 2019; Abás et al., 2020; Vasilopoulou et al., 2020; Rodriguez-Arévalo et al., 2021; Vasilopoulou et al., 2021). These new tools may aid in identifying the relevance of I2R in vascular health and disease.
In the present study, we aimed to evaluate the potential vasoactive effects of two recently synthesized I2R ligands that belong to different chemical classes, diethyl [(1-(3-chloro-4-fluorobenzyl)-5,5-dimethyl-4-phenyl-4,5-dihydro-1H-imidazol-4-yl] phosphonate] (MCR5; Abás et al., 2017) and diethyl (1RS,3aSR,6aSR)-5-(3-chloro-4-fluorophenyl)-4,6-dioxo-1-phenyl-1,3a,4,5,6,6a-hexahydropyrrolo[3,4-c]pyrrole-1-phosphonate) (B06; Abás et al., 2020). The results show that MCR5 is the I2R compound that induces greater relaxations in the mouse aorta of two different mice strains. Notably, the high affinity I2R selective ligand 2-(2-benzofuranyl)-2-imidazoline (2-BFI) (Hudson et al., 1997) evoked marginal relaxations and agmatine did not relax. Thus, we confirmed that MCR5 relaxations are largely independent of I2R activation, are only slightly modulated by endothelium-derived factors, and are mostly mediated through activation of smooth muscle KATP and inhibition of L-type voltage-gated Ca2+ channels. Remarkably, we show that MCR5 relaxations are preserved in both endothelium-denuded arteries and in old mice despite the presence of endothelial dysfunction. We suggest that MCR5 could be used as a novel agent for treating vascular disease in the elderly.
Materials and Methods
Animals
Fifty-two male Oncins France 1 (OF1) non-consanguineous mice of 3- to 4-months of age, and thirty-seven (24 male/ 13 female) C57BL/6 consanguineous mice of 3- to 4- (n = 20) and 16- to 17- (n = 17) months of age were obtained from Charles River (Sant Cugat del Vallès, Spain). All animals were housed at the animals’ facility under constant temperature (20 ± 2°C) and humidity conditions, 12:12 h dark/light cycle, and provided with ad libitum food and water. All procedures were performed in accordance with Spanish legislation on “Protection of Animals Used for Experimental and Other Scientific Purposes” and the EU Council directive (2010/63/EU). The experiments were approved by the Ethics Committee of the Universitat Autònoma de Barcelona (approval code: FJA-eut/01).
Preparation of Isolated Aortic Rings
Mice were euthanized by decapitation under anesthesia (4% isoflurane mixed with 0.8 L/min O2). Descending thoracic aorta was removed and placed in oxygenated (95% O2, 5% CO2) ice-cold Krebs-Henseleit solution (KHS; NaCl 112 mM, KCl 4.7 mM, CaCl2 2.5 mM, KH2PO4 1.2 mM, MgSO4 1.2 mM, NaHCO3 25 mM and glucose 11.1 mM). Afterward, fat surrounding the arteries was quickly removed, and aortas were cut into 2 mm segments to analyze vascular reactivity.
Evaluation of Aortic Reactivity
Aortic segments were mounted onto a four-channel wire myograph (model 620M; Danish Myo Technology, Aarhus, Denmark) under isometric conditions, filled with ice-cold oxygenated KHS (5 ml), according to the protocol previously described (Muntsant et al., 2021). Briefly, aortic rings were left to equilibrate for 30 min at 37°C and were then stretched gradually to a basal tension of 14.7 mN (OF1 mice) or 6 mN (C57BL/6 mice; Chung et al., 2007). Optimal tension in OF1 mice was assessed in preliminary experiments, which showed that 14.7 mN produced good contraction and relaxation responses. After a 45-min stabilization period, arterial segments were exposed twice to a potassium-enriched KHS (containing 100 mM of KCl) to assess tissue viability. After several washes, aortic rings were left to equilibrate for 30 min and were then pre-contracted with 9,11-Dideoxy-11α,9α-epoxymethanoprostaglandin F2α (U46619), a thromboxane A2 stable analog, phenylephrine (Phe), an alpha 1 adrenergic receptor agonist, or 5-hydroxytryptamine (5-HT), a 5-HT receptor agonist, to reach around 70–100% of the contraction induced by KCl (100 mM). When precontraction reached a plateau, endothelium-dependent relaxations were assessed by adding cumulative concentrations (0.001–100 µM) of acetylcholine (ACh). After several washes and a resting period of 30 min, concentration-response curves (0.1–30 µM) to the non-selective IR ligand agmatine, and the I2R selective ligands 2-BFI (Hudson et al., 1997), B06 (Abás et al., 2020), and MCR5 (Abás et al., 2017) were performed. The maximum concentrations of these compounds that we could add in the bath were limited by the maximum vehicle (dimethyl sulfoxide; DMSO) concentration (0.2%) that did not produce any effect per se (results not shown). In a parallel set of experiments, concentration-response curves (0.001–1 mM) to the NO donor sodium nitroprusside or to ACh (0.001–100 µM) in the absence (control) or presence of MCR5 (1 and 3 µM)were performed.
In experiments aimed to assess the contribution of the endothelium on MCR5-induced relaxations, the endothelium was gently removed using a plastic cannula. Aortic segments were considered endothelium-denuded if relaxation to ACh was below 25%.
Before adding the inhibitors for mechanistic studies, preliminary experiments showed the reproducibility of two consecutive MCR5 relaxation curves (Supplementary Figure S1). Therefore, the study of MCR5 mechanisms of action was performed using the first curve as an internal control. The second curve was performed after preincubation (30 min) with the I2R ligand 2-(4,5-dihydro-1H-imidazol-2-yl) quinoline (BU224; 10 μM; Nutt et al., 1995) and the I2R and I1R antagonist idazoxan (10 μM; Coupry et al., 1987), the selective α2-adrenoceptor antagonist yohimbine (1 µM), the non-selective nitric oxide synthase inhibitor Nω-nitro-l-arginine methyl ester (L-NAME; 300 µM), the non-selective COX inhibitor indomethacin (10 µM), the inwardly rectifying potassium channel blocker BaCl2 (1 mM), the large and small conductance Ca2+ -activated K+ channels blockers, respectively, charybdotoxin (0.1 µM) and apamin (0.1 µM), the specific blocker of voltage-gated K+ channels 4-aminopyridine (1 mM), the ATP-sensitive K+ channels blocker glibenclamide (30 µM), or the Na+/K+-ATPase inhibitor ouabain (1 µM).
In experiments to study the contribution of voltage-gated Ca2+ channels, aortic rings were: 1) pre-contracted with KCl (100 mM) before performing concentration-response curves to MCR5; 2) contracted with KCl (8–300 mM) in the absence (control) or presence of the L-type Ca2+ channel blocker nifedipine (10 µM) or MCR5 (10 and 30 µM); 3) contracted with U46619 (0.001–1 µM) in the absence (control) or presence of nifedipine (10 µM) or MCR5 (10 and 30 µM); and 4) contracted with Bay K8644 (1 µM) in the absence (control) or presence of MCR5 (10 and 30 µM).
Measurement of Prostanoid Production
The release of prostanoids from aortic segments was determined by enzyme-linked immunosorbent assays (ELISA; Cayman Chemical, Ann Arbor, MI), as reported (Costa et al., 2019). Levels of prostacyclin (PGI2) and thromboxane A2 (TXA2) stable metabolites 6-Keto-PGF1α and TXB2, respectively, were assessed in the KHS collected in resting conditions (control) or after performing concentration-response curves to MCR5 in the absence or presence of indomethacin (10 µM). Samples were kept at room temperature for 30 min for complete hydrolysis of PGI2 and TXA2 into their stable metabolites. Prostanoid levels were assessed in duplicate and calculated according to the ELISA kit manufacturer instructions.
Statistical Analysis
Statistical analysis was performed with GraphPad Prism version 8.3 (San Diego, California, United States) software. Results are expressed as mean ± SEM (standard error of the mean) of the number (n) of animals in each group (shown in figure legends). Vasodilator responses to ACh, 2-BFI, B06, and MCR5 are expressed as a percentage of the tone generated by U46619, Phe, 5-HT, or KCl (100 mM) pre-contractions. Sigmoid curve fitting (variable slope) was performed by non-linear regression to obtain maximal responses (Emax) and sensitivity (pEC50) in the vasoconstrictor responses curves to KCl and U46619. Statistical analysis was carried out using one-way ANOVA or Kruskal-Wallis with Tukey’s or Dunn’s post hoc test, respectively, for a single factor. In case of two factors, two-way ANOVA corrected for multiple comparisons using Bonferroni post-test was used. Statistical significance was set as p ≤ 0.05.
Chemicals
Selective I2R ligands B06 and MCR5 were provided by Dr. Carmen Escolano from the laboratory of Medicinal Chemistry (Faculty of Pharmacy and Food Sciences, Universitat de Barcelona, Barcelona, Spain), and 2-BFI was purchased from Tocris (Bio-Techne R&D Systems S. LU, Madrid, Spain). ACh, sodium nitroprusside, U46619, Phe, 5-HT, BU224, idazoxan, L-NAME, indomethacin, 4-aminopyridine, glibenclamide, BaCl2, ouabain, Bay K8644, and agmatine were purchased from Merck (KGaA, Darmstadt, Germany). ACh, sodium nitroprusside, Phe, 5-HT, 4-aminopyridine, BaCl2, ouabain, Bay K8644, and agmatine were diluted in distilled water; 2-BFI, B06, MCR5, U46619, BU224, idazoxan and glibenclamide were diluted in DMSO (final bath concentration ≤ 0.2%); L-NAME was diluted in KHS; and indomethacin was diluted in 2% NaHCO3.
Results
MCR5 Induces Greater Relaxations Than B06 and 2-BFI
Neither of the compounds was able to induce aortic contractions. Figure 1 shows concentration-dependent relaxations to ACh (an endothelium-dependent agonist used as a relaxation control) vs. three structurally different I2R ligands in U46619-pre-contracted thoracic aortas from young OF1 and C57BL/6 mice. The results show that MCR5 induces greater relaxations, as compared to B06 and 2-BFI, following a similar pattern in both strains. Note that relaxations to 2-BFI, an I2R prototype ligand, were the lowest ones. Although MCR5 relaxations were less potent than ACh relaxations, maximum responses were similar (Figure 1).
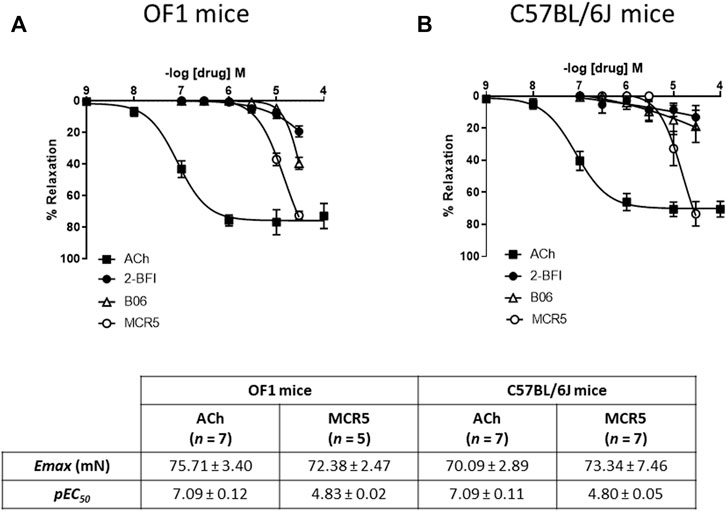
FIGURE 1. Comparison of I2R ligands relaxations. Concentration-response curves to acetylcholine (ACh) and I2R ligands in U46619-pre-contracted aortic rings from young OF1 (A) and C57BL/6J (B) mice. Maximum response (Emax) and potency (pEC50) of ACh and MCR5 relaxations are shown at the bottom. Results are mean ± SEM from n = 7 (ACh), 5-7 (2-BFI), 6 (B06), 5-7 (MCR5) mice.
MCR5 Relaxations Are Largely Non-mediated by I2R
Incubation with two different I2R ligands, idazoxan (I1R and I2R ligand; 10 μM; Figure 2A) and BU224 (I2R ligand; 10 μM; Figure 2B), only slightly decreased or increased, respectively, MCR5 relaxations. These results suggest that MCR5 relaxations are largely independent of I2R signaling. Of note, agmatine did not evoke any response in U46619-precontracted aortas from OF1 mice (results not shown). Newly synthesized imidazoline compounds are typically screened according to their ability to activate α2-adrenoceptors (Bousquet et al., 2020). We subsequently studied the effect of the selective α2-adrenoceptor antagonist yohimbine, which did not affect MCR5 relaxations (Figure 2C).
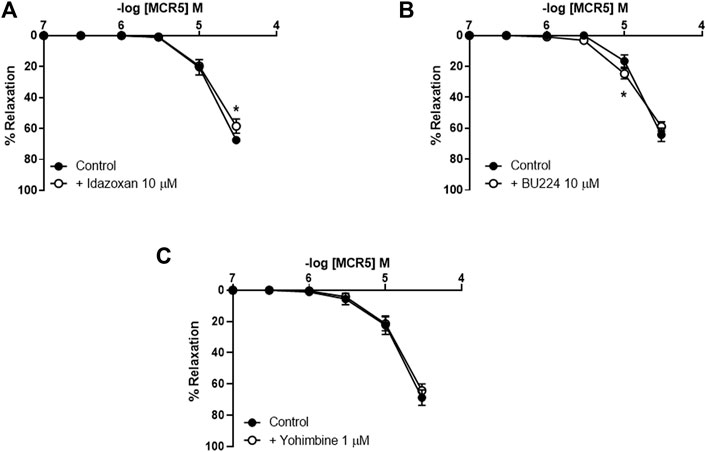
FIGURE 2. Effects of I2R modulators and antagonism of α2-adrenoceptors on MCR5 relaxations. Concentration-response curves to MCR5 in U46619-pre-contracted aortic rings from young OF1 mice in the absence (control) or presence of the I1R and I2R ligand idazoxan (10 µM) (A) the I2R ligand BU224 (10 µM) (B), or the α2-adrenoceptor antagonist yohimbine (1 µM) (C). Results are mean ± SEM from n = 7 (A), 8 (B), and 6 (C). ∗p < 0.05 by two-way repeated measures ANOVA with Bonferroni’s post hoc test.
MCR5 Relaxations Are Higher in KCl Pre-Contracted Vessels
Concentration-response curves to MCR5 were similar regardless of the use of Phe, 5-HT, or U46619 as precontractile agonists (Figure 3A). Only relaxations to 30 µM MCR5 were significantly lower (p < 0.001) in Phe- (53.26 ± 3.09%, n = 5) vs. U46619- (70.47 ± 4.83%, n = 5) pre-contracted arteries. Notably, MCR5 responses were markedly greater in KCl (100 mM)-pre-contracted arteries (Figure 3A). Neither idazoxan (10 µM) nor BU224 (10 µM) altered these relaxations (Figure 3B), confirming that I2R are not significantly involved in MCR5 responses.
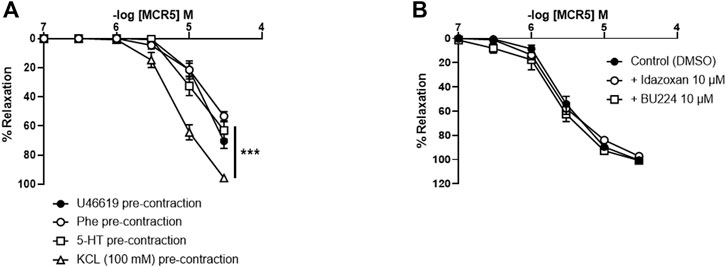
FIGURE 3. Effects of different pre-contractile agonists on MCR5 relaxations and influence of I2R modulation. Concentration-response curves to MCR5 in U46619-, phenylephrine (Phe)-, 5-hydroxytryptamine (5-HT)-, and KCL (100 mM)-pre-contracted aortic rings from young OF1 mice (A). Concentration-response relaxation curves to MCR5 in KCl (100 mM)-pre-contracted aortic rings in the absence (Control) or presence of idazoxan (10 µM) and BU224 (10 µM) (B). Results are mean ± SEM from n = 5 (A) and 3-4 (B) mice. ∗∗∗p < 0.001 by two-way repeated measures ANOVA with Bonferroni’s post hoc test.
MCR5 Blocks Smooth Muscle L-Type Voltage-Gated Ca2+ Channels
In view of the increased MCR5 relaxations in KCl pre-contracted aortas, we studied the potential role of the compound in blocking smooth muscle voltage-gated Ca2+ channels (Figure 4). Incubation with MCR5 10 or 30 µM caused a significant decrease in maximal contractions to KCl similar to that induced by the L-type Ca2+ channel blocker nifedipine (10 µM) (Figure 4A; Table 1). In addition, U46619 contractions were not significantly reduced by nifedipine, nor MCR5, but were slightly (p = 0.059) shifted to the right after nifedipine and MCR5 (30 µM) incubation (Figure 4B; Table 1). Moreover, MCR5 (30 µM) significantly reduced contractions induced by the L-type Ca2+ channel activator Bay K8644 (1 µM) (Figure 4C). Taken together, these results suggest that MCR5 blocks L-type voltage-gated Ca2+ channels.
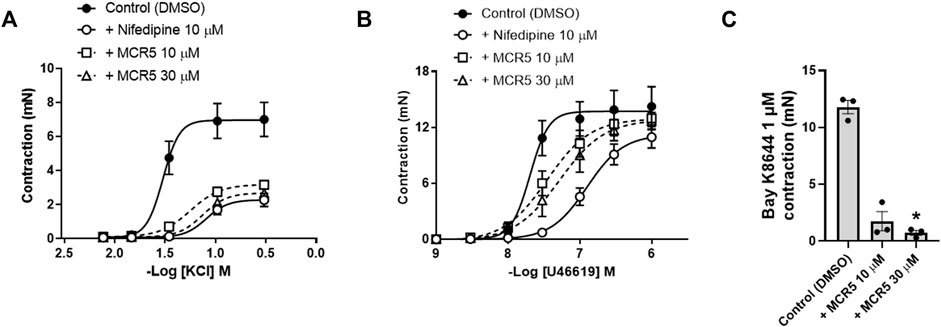
FIGURE 4. Influence of MCR5 on smooth muscle voltage-gated Ca2+ channels. Concentration-response contraction curves to KCl (A) and U46619 (B) in aortic rings from young OF1 mice in the absence (control) or presence of the L-type voltage-gated Ca2+ channel blocker nifedipine (10 µM) or MCR5 (10 and 30 µM). (C) Contractions to the L-type voltage-gated Ca2+ channel activator Bay K8644 (1 µM) in the absence (control) or presence of MCR5 (10 and 30 µM). Results are mean ± SEM from n = 4 (A,B) or n = 3 (C) mice. ∗p < 0.05 by Kruskal-Wallis with Dunn’s post hoc test.

TABLE 1. Maximum response (Emax) and potency (pEC50) of the concentration–response curves to KCL (n = 4) and U46619 (n = 4) contractions (mN) in aortic rings from young OF1 mice.
MCR5 Relaxations Are Modulated by KATP, Voltage-Gated K+ Channels, and the Na+/K+-ATPase
To investigate if relaxations were dependent upon K+ efflux, we pre-treated vessels with different K+ channel inhibitors. Neither inhibition of KCa channels with charybdotoxin (100 nM) and apamin (100 nM) (Figure 5A), nor inhibition of KIR channels with BaCl2 (1 mM) (Figure 5B) modified MCR5 relaxations. In contrast, inhibition of KATP channels with glibenclamide (30 µM) significantly reduced MCR5 responses (Figure 5C). Voltage-gated K+ channels blockade with 4-aminopiridine (1 mM) only slightly decreased maximal relaxations (Figure 5D). The activity of the Na+/K+-ATPase is another mechanism that contributes to vascular relaxation. Addition of the Na+/K+-ATPase inhibitor ouabain (1 μM) slightly decreased MCR5 relaxations (Figure 5E).
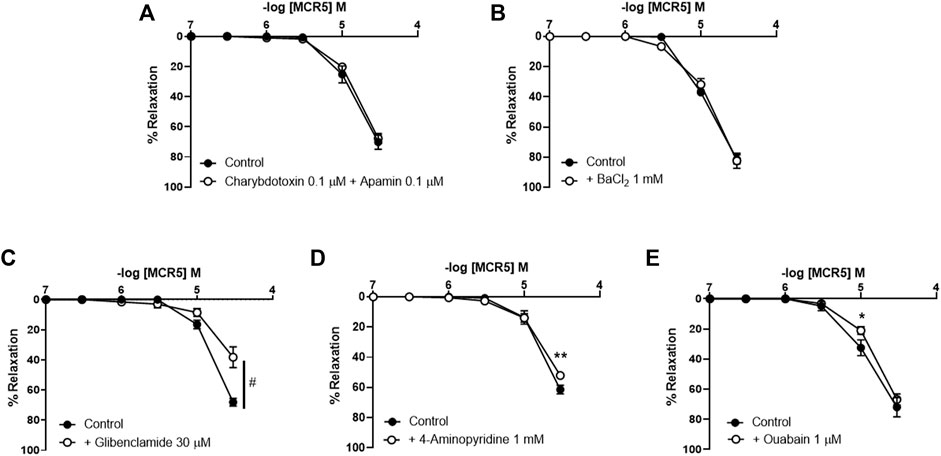
FIGURE 5. Effects of inhibition of K+ channels and the Na+/K+-ATPase on MCR5 relaxations. Concentration-response curves to MCR5 in U46619-pre-contracted aortic rings from young OF1 mice in the absence (control) or presence of the Ca2+-activated K+ channels inhibitors charybdotoxin (0.1 µM) and apamin (0.1 µM) (A), the inwardly rectifying K+ channel blocker BaCl2 (1 mM) (B), the KATP channel blocker glibenclamide (30 µM) (C), the voltage-gated K+ channel blocker 4-aminopyridine (1 mM) (D), and the Na+/K+-ATPase inhibitor ouabain (1 μM) (E). Results are mean ± SEM from n = 6 (A), 5 (B), 7 (C), 6 (D,E) mice. #p < 0.05 by two-way repeated measures ANOVA; ∗p < 0.05, ∗∗p < 0.01 by two-way repeated measures ANOVA with Bonferroni’s post hoc test.
MCR5 Relaxations Are Modulated by Endothelial-Derived NO and Prostacyclin
Inhibition of NO synthesis with L-NAME (300 µM) modestly increased MCR5-induced relaxations (Figure 6A), suggesting a slight negative influence related to NO, which was not mediated by. soluble guanylyl cyclase since ODQ (10 µM), an inhibitor of this enzyme, did not affect MCR5 relaxations (Figure 6B). Non-selective inhibition of COX with indomethacin (10 µM) significantly reduced MCR5 relaxations (Figure 7A). We next explored whether the endothelium could be the source of NOS- and COX-mediated products that affect, negatively or positively, respectively, MCR5 responses. As expected, removal of the endothelium almost abolished ACh vasodilation (Supplementary Figure S2). However, in the same aortic rings, the absence of endothelium did not modify MCR5 relaxations (Figure 7B). The observation that relaxations were unaltered after endothelium removal despite the negative feedback produced by endothelial-derived NO on MCR5 responses, raises the hypothesis that MCR5 releases an endothelium-derived COX-mediated relaxing factor, such as prostacyclin (PGI2). Interestingly, endothelium removal abolished the inhibitory effect of indomethacin on MCR5 relaxations (Figure 7B). Prostanoids release from aortic rings was determined in the KHS solution collected after concentration-response curves to MCR5. MCR5 tended (p = 0.06) to increase the release of PGI2 (Figure 7C), whereas thromboxane (TXA2) was not significantly increased (Figure 7D). Remarkably, indomethacin significantly (p < 0.05) reduced the PGI2/TXA2 ratio in the presence of MCR5 (Figure 7E). These results suggest that MCR5 releases endothelial-derived prostacyclin contributing to relaxation.
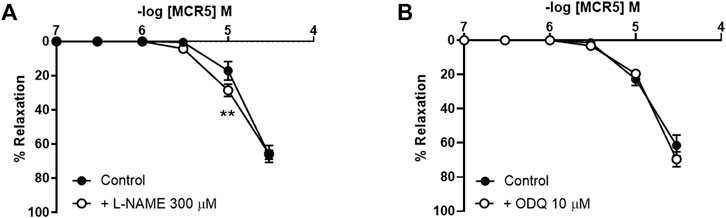
FIGURE 6. Effects of NO pathway inhibition on MCR5 relaxations. Concentration-response curves to MCR5 in U46619-pre-contracted aortic rings from young OF1 mice in the absence (control) or presence of the NO synthase inhibitor L-NAME (300 µM) (A) or the soluble guanylyl cyclase inhibitor ODQ (10 µM) (B). Results are mean ± SEM from n = 7 (A) and 5 (B) mice. ∗∗p < 0.01 by two-way repeated measures ANOVA with Bonferroni’s post hoc test.
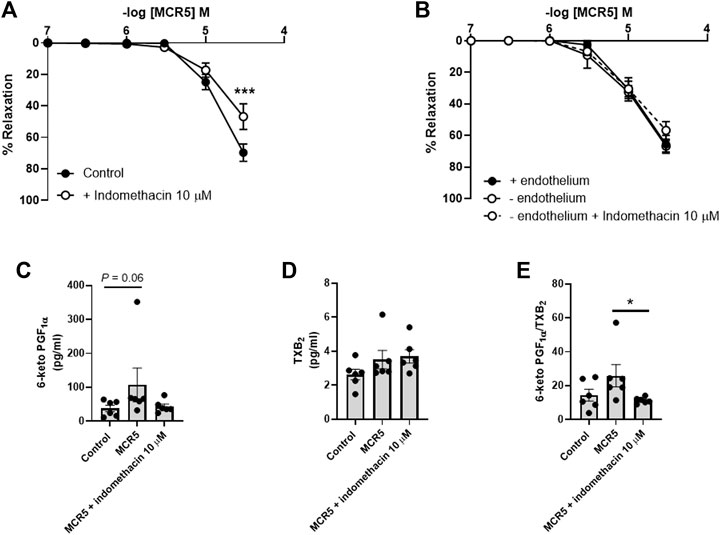
FIGURE 7. Effects of COX inhibition on MCR5-induced aortic relaxations and prostanoids production. Concentration-response curves to MCR5 in U46619-pre-contracted aortic rings from young OF1 mice in the absence (control) or presence of the COX inhibitor indomethacin (10 µM) (A). Concentration-response curves to MCR5 in endothelium denuded arteries (- endothelium) in the absence or presence of indomethacin (B). Levels of PGI2 and TXB2 metabolites, 6-ketoPGF1α (C) and TXB2 (D), respectively, and the 6-ketoPGF1α/TXB2 ratio (E), in the Krebs-Henseleit solution collected after concentration-response curves to MCR5 in U46619-pre-contracted aortic rings from young OF1 mice. Results are mean ± SEM from n = 7 (A), 4–6 (B), and 6 (C, D, and (E). ∗∗∗p < 0.001 by two-way repeated measures ANOVA with Bonferroni’s post hoc test (A); ∗p < 0.05 by Kruskal-Wallis with Dunn’s post hoc test (C,E).
MCR5 Relaxations Are Not Impaired in Old Mice Despite the Presence of Endothelial Dysfunction
To study the potential relevance of MCR5 in aging-related vasodilator dysfunction, we used aortic rings from young (3–4 months) and old (16–17 months) C57BL/6 mice (Figure 8). We firstly evaluated ACh relaxations, which were significantly impaired in old compared to young mice (Figure 8A). However, endothelium-independent relaxations to the NO donor sodium nitroprusside were not altered in old mice (Supplementary Figure S3), suggesting that direct smooth muscle relaxation is not affected by aging. In addition, these results indicate that impairments of ACh responses in old mice are a result of endothelial dysfunction. Remarkably, relaxations to MCR5 were not affected by aging (Figure 8B).
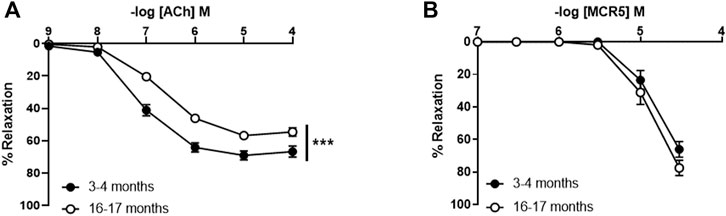
FIGURE 8. Influence of aging on aortic relaxations. Concentration-response curves to acetylcholine (ACh) in U46619-pre-contracted aortic rings from 3- to 4-month-old (young) and 16- to 17-months-old (old) C57BL/6 mice (A). Concentration-response curves to MCR5 (B). Results are mean ± SEM from n = 13–15 (A,B) mice. ∗∗∗p < 0.001 by two-way repeated measures ANOVA with Bonferroni’s post hoc test.
To know if MCR5, additionally to its endothelium independent vasorelaxant activity, could improve the age-related endothelial dysfunction, we further studied whether concentrations of MCR5 that evoked marginal relaxations (1 or 3 µM) may improve ACh responses. The results show that MCR5 does not modify ACh vasodilations (Supplementary Figure S4).
Discussion
The development of new strategies to modulate vascular responses is important to discover pharmacological tools to face vascular disease. In the present study, we explored the putative vasoactive properties of selective I2R ligands that have previously shown neuroprotective activity in age-related neurodegenerative disorders such as Alzheimer’s disease (Abás et al., 2017; Griñán-Ferré et al., 2019; Abás et al., 2020). The results not only might explain some of the mechanisms involved in neuroprotection, but also might pave the way towards the identification of I2R modulatory molecules with therapeutic potential against vascular disease in the elderly. Nevertheless, our findings indicate that I2R might not have an important role in the modulation of vascular tone in the mouse aorta.
Previous studies reported that both I1R and I2R are present in the vasculature (Regunathan et al., 1995; Mar et al., 2013; Sarac et al., 2015). The vascular effects of I1R activation have been primarily related to the central regulation of blood pressure (Ernsberger et al., 1995), whereas vascular I2R signaling is associated with vasodilation and antihypertensive effects (Mar et al., 2013; Chen et al., 2014). Compounds B06 and MCR5 have been recently recognized as selective I2R ligands with neuroprotective potential in Alzheimer’s disease (Abás et al., 2017; Griñán-Ferré et al., 2019; Abás et al., 2020). In the present study, we studied the putative vasoactive actions of these compounds in two different mice strains (OF1 and C57BL/6J). We found that although both compounds cause aortic dilation, MCR5 was the one that induced greater relaxations in both mice strains. Surprisingly, these responses were largely non-mediated by I2R signaling. Contrasting results were reported regarding the mechanism of vasodilatation induced by the non-selective IR ligand agmatine across rat strains (Santhanam et al., 2007; Mar et al., 2013; Chen et al., 2014). Here, in the mouse aorta, agmatine did not evoke any response and relaxations induced by the selective I2R ligand MCR5 were largely independent of I1R, I2R and α2-adrenoceptors, since idazoxan (I1R and I2R, but not I3R ligand; Coupry et al., 1987) or BU224 (I2R ligand; Nutt et al., 1995) only slightly affected them, and yohimbine (α2-adrenoceptor antagonist) did not alter them. This argument was further substantiated by the fact that relaxations induced by the I2R prototype ligand 2-BFI (Hudson et al., 1997), by B06, another selective I2R ligand that belongs to a different chemical class compared to MCR5 (Abas et al., 2020), and by agmatine, a non-selective IR ligand, were minimal or absent providing additional evidence in support of a marginal contribution of I2R signalling in MCR5 relaxations in the mouse aorta, at least in the current study experimental conditions. Indeed, these findings raise the question whether I2R are expressed in the mouse aorta. Unfortunately, this cannot be answered until IR molecular identity is known.
Potassium channels exert an important contribution to the regulation of vascular tone (Tykocki et al., 2017). The activation of KATP channels induces hyperpolarization, closing of voltage-gated Ca2+ channels and Ca2+ influx, and consequently, relaxes smooth muscle. Note that KATP channels have been consistently involved in agmatine responses in the rat aorta (Santhanam et al., 2007; Mar et al., 2013; Chen et al., 2014) and here, we found that MCR5 relaxation of the mouse aorta was partly mediated via activation of KATP, since it was attenuated by glibenclamide. It is proposed that I3R receptors represent a binding site present in the Kir6.2 subunit of KATP channels in pancreatic beta cells (Ishida-Takahashi et al., 1996; Proks and Ashcroft, 1997; Bousquet et al., 2020). We can speculate that MCR5 induces vasodilatation by binding to a putative vascular I3R receptor or directly to vascular KATP channels, activating K+ efflux, and promoting membrane hyperpolarization. This change in membrane potential may affect the open probability of voltage-gated Ca2+channels, facilitating vasodilatation. The possibility that MCR5 acts through I3R remains open, but the lack of previous evidence on the presence and functional role of I3R in vessels does not support this hypothesis at this stage.
Participation of other K+ channels as Ca2+-activated K+ channels or inwardly rectifying K+ channels can be discarded, as selective inhibitors as charybdotoxin and apamin, or BaCl2, respectively, did not alter MCR5 vasodilator activity. Only a minor contribution of voltage-gated K+ channels was observed. However, at this stage, we cannot discard that the effect of 4-aminopiridine on MCR5 relaxations might be a consequence of voltage-gated Ca2+ channel activation (Wu et al., 2009). In fact, we found that inhibition of voltage-gated Ca2+ channels was involved in MCR5 relaxations, an effect that was independent of I2R, but might be a consequence of KATP channels opening related to I3R. Voltage-gated Ca2+ channels greatly contribute to the control of resting membrane potential in aortic smooth muscle cells (Tammaro et al., 2004; Tykocki et al., 2017). The extent of the inhibitory effect of MCR5 and nifedipine was similar in KCl-induced contraction-response curves, but with some differences in U46619-induced contractions. The main mechanism associated with KCl-induced contractions is the opening of L-type voltage-gated Ca2+-channels, which suggests an involvement of this type of channel on MCR5 vasodilatations. Consistently, MCR5 greatly reduced contractions evoked by the L-type voltage-gated Ca2+-channel activator Bay K8644. These results agree, at least in part, with previous evidence showing that agmatine blocks L-type voltage-gated Ca2+ channels and other types of voltage-gated Ca2+ channels in cultured rat hippocampal neurons (Weng et al., 2003; Zheng et al., 2004). However, other mechanisms such as increased reactive oxygen species production, KATP channel dysfunction and impaired NO-dependent vasodilation contribute to the thromboxane A2 analogue U46619 vascular smooth muscle contractions (Santos et al., 2021). This evidence may explain slight differences found between the inhibitory effect of nifedipine and MCR5 on U46619 versus KCl concentration-response curves.
Vascular tone is a tightly regulated phenomenon that involves multiple signalling pathways, whose deregulation results in the development of vascular pathology. The ability of MCR5 to induce robust relaxations in endothelium-denuded arteries suggests that this compound mostly relaxes the mouse aorta through endothelium-independent mechanisms. Besides, numerous vasoconstrictors and vasodilators can modulate, either directly or indirectly, smooth muscle ion channel activity and, consequently, vascular tone.
In physiological conditions, endothelial cells regulate vascular tone by producing a negative feedback on vascular smooth muscle contractility (Mohrman and Heller, 2014). An important relaxation mediator produced by endothelial cells is NO, which is synthesized in response to a wide variety of stimuli. In addition, the endothelium regulates basal tone not only through the production of NO, but also through the release of other endothelium-derived relaxing and contracting factors able to influence vascular smooth muscle contraction in a paracrine fashion (Mohrman and Heller, 2014). For instance, prostanoids, lipid mediators produced by COX, are potent endothelium- and smooth muscle-derived vasodilators and vasoconstrictors that can actively modulate vascular tone (Wong et al., 2010). MCR5 vasodilatation was not mediated by NO, since L-NAME did not reduce relaxations, but slightly increased them. The fact that the soluble guanylyl cyclase inhibitor ODQ did not reproduce this effect suggests that the slight NO negative influence on MCR5 relaxations was not mediated by a downstream signaling pathway of this enzyme but could be related to endothelial mechanisms. Non-selective COX inhibition with indomethacin decreased either MCR5 relaxations or MCR5-induced increase in prostacyclin levels. These results demonstrate that MCR5 relaxations are positively modulated by COX activation in the aorta of OF1 mice. Notably, the inhibitory effect of indomethacin was not present after endothelium removal, suggesting that the compound may activate endothelial COX. Altogether, these results provide evidence in support of an opposed modulatory effect of NO and prostacyclin endothelial pools on MCR5 relaxations.
The classical approach to protecting dysfunctional vessels is to administer compounds with anti-oxidant or anti-inflammatory activities, which, among other beneficial effects, are able to restore physiological levels of vasoactive factors. However, some treatments with these compounds can cause adverse effects or their effectiveness have not been demonstrated in the clinical setting (Costa et al., 2021). Interestingly, vasodilation through endothelium-independent mechanisms could also represent a strategy to warrant physiological responses in adverse environmental conditions that have the potential to affect endothelial function, like in those reported in vascular aging (El Assar et al., 2012; Ungvari et al., 2018). Along these lines, endothelium-independent relaxations induced by sodium nitroprusside were preserved in old mice, suggesting that strategies that promote direct smooth muscle relaxation might be more resistant to aging-related vascular dysfunction. Importantly, we found that MCR5 responses were conserved in old mice in spite of impaired ACh relaxations. Therefore, present findings demonstrate that MCR5 relaxations are protected in the setting of endothelial dysfunction, likely because of they are largely independent of endothelium-derived signalling.
The present study has some limitations. First, it is not possible to identify I2R in vessels since their molecular identity is unknown; and second, the lack of a direct evidence about the proposed relationship between MCR5 activity and KATP and L-type voltage-gated Ca2+ channels. Finally, it would be interesting to know the putative vasoactive effects of MCR5 in small resistance peripheral and cerebral arteries, which may provide relevant information about the impact of the compound on, for instance, blood pressure or cerebrovascular autoregulation, respectively.
In our hands, MCR5 showed a pleiotropic action related primarily with endothelium independent routes that explained its vascular effects. These actions included activation of smooth muscle KATP and inhibition of L-type voltage-gated Ca2+ channels. Furthermore, vasodilation was slightly modulated by endothelial nitric oxide (negatively) and prostacyclin (positively), effects that compensate each other. Importantly, MCR5 relaxations were preserved either in endothelium-denuded vessels or in old mice, suggesting that this compound may have therapeutic potential in the setting of aging-related endothelial dysfunction. Overall, the present study adds knowledge on the understanding of the effects of I2R ligands in vessels and provides evidence against a functional contribution of I2R in the modulation of vascular tone in the mouse aorta. MCR5 is a I2R ligand with an interesting vasodilator activity largely mediated through I2R- and endothelium-independent mechanisms. This vasorelaxant activity is resistant to aging-related dysfunction, suggesting that MCR5 may be an interesting tool for treating vascular disease associated with aging.
Data Availability Statement
The raw data supporting the conclusion of this article will be made available by the authors, without undue reservation.
Ethics Statement
The animal study was reviewed and approved by Ethics Committee of the Universitat Autònoma de Barcelona (approval code: FJA-eut/01).
Author Contributions
FJ-A, MP, and CE: conceptualization. FJ-A: drafted the manuscript. FJ-A, AC, AB, LG-L, PD, BP, MP, and CE: made a substantial contribution to the acquisition of data and data interpretation. FJ-A, AC, AB, LG-L, PD, BP, MP, and CE: edited the manuscript critically for important intellectual content, and approved the version to be published.
Funding
This work has been supported by Generalitat de Catalunya (2017-SGR-645) and Ministerio de Ciencia e Innovación (PID 2020-113634RB-C22 and PID 2019-107991RB-I00).
Conflict of Interest
The authors declare that the research was conducted in the absence of any commercial or financial relationships that could be construed as a potential conflict of interest.
Publisher’s Note
All claims expressed in this article are solely those of the authors and do not necessarily represent those of their affiliated organizations, or those of the publisher, the editors and the reviewers. Any product that may be evaluated in this article, or claim that may be made by its manufacturer, is not guaranteed or endorsed by the publisher.
Supplementary Material
The Supplementary Material for this article can be found online at: https://www.frontiersin.org/articles/10.3389/fphar.2022.826837/full#supplementary-material
References
Abás, S., Erdozain, A. M., Keller, B., Rodríguez-Arévalo, S., Callado, L. F., García-Sevilla, J. A., et al. (2017). Neuroprotective Effects of a Structurally New Family of High Affinity Imidazoline I2 Receptor Ligands. ACS Chem. Neurosci. 8, 737–742. doi:10.1021/acschemneuro.6b00426
Abás, S., Rodríguez-Arévalo, S., Bagán, A., Griñán-Ferré, C., Vasilopoulou, F., Brocos-Mosquera, I., et al. (2020). Bicyclic α-Iminophosphonates as High Affinity Imidazoline I2 Receptor Ligands for Alzheimer's Disease. J. Med. Chem. 63, 3610–3633. doi:10.1021/acs.jmedchem.9b02080
Bousquet, P., Feldman, J., and Schwartz, J. (1984). Central Cardiovascular Effects of Alpha Adrenergic Drugs: Differences between Catecholamines and Imidazolines. J. Pharmacol. Exp. Ther. 230, 232–236.
Bousquet, P., Hudson, A., García-Sevilla, J. A., and Li, J. X. (2020). Imidazoline Receptor System: The Past, the Present, and the Future. Pharmacol. Rev. 72, 50–79. doi:10.1124/pr.118.016311
Chan, S. L., Brown, C. A., Scarpello, K. E., and Morgan, N. G. (1994). The Imidazoline Site Involved in Control of Insulin Secretion: Characteristics that Distinguish it from I1- and I2-Sites. Br. J. Pharmacol. 112, 1065–1070. doi:10.1111/j.1476-5381.1994.tb13191.x
Chen, M. F., Tsai, J. T., Chen, L. J., Wu, T. P., Yang, J. J., Yin, L. T., et al. (2014). Characterization of Imidazoline Receptors in Blood Vessels for the Development of Antihypertensive Agents. Biomed. Res. Int. 2014, 182846. doi:10.1155/2014/182846
Chung, A. W., Au Yeung, K., Cortes, S. F., Sandor, G. G., Judge, D. P., Dietz, H. C., et al. (2007). Endothelial Dysfunction and Compromised eNOS/Akt Signaling in the Thoracic Aorta during the Progression of Marfan Syndrome. Br. J. Pharmacol. 150, 1075–1083. doi:10.1038/sj.bjp.0707181
Costa, T. J., Barros, P. R., Arce, C., Santos, J. D., da Silva-Neto, J., Egea, G., et al. (2021). The Homeostatic Role of Hydrogen Peroxide, Superoxide Anion and Nitric Oxide in the Vasculature. Free Radic. Biol. Med. 162, 615–635. doi:10.1016/j.freeradbiomed.2020.11.021
Costa, T. J., Jiménez-Altayó, F., Echem, C., Akamine, E. H., Tostes, R., Vila, E., et al. (2019). Late Onset of Estrogen Therapy Impairs Carotid Function of Senescent Females in Association with Altered Prostanoid Balance and Upregulation of the Variant ERα36. Cells 8, 1217. doi:10.3390/cells8101217
Coupry, I., Podevin, R. A., Dausse, J. P., and Parini, A. (1987). Evidence for Imidazoline Binding Sites in Basolateral Membranes from Rabbit Kidney. Biochem. Biophys. Res. Commun. 147, 1055–1060. doi:10.1016/s0006-291x(87)80177-8
El Assar, M., Angulo, J., Vallejo, S., Peiró, C., Sánchez-Ferrer, C. F., and Rodríguez-Mañas, L. (2012). Mechanisms Involved in the Aging-Induced Vascular Dysfunction. Front. Physiol. 3, 132. doi:10.3389/fphys.2012.00132
Ernsberger, P., Giuliano, R., Willette, R. N., and Reis, D. J. (1990). Role of Imidazole Receptors in the Vasodepressor Response to Clonidine Analogs in the Rostral Ventrolateral Medulla. J. Pharmacol. Exp. Ther. 253, 408–418.
Ernsberger, P., Graves, M. E., Graff, L. M., Zakieh, N., Nguyen, P., Collins, L. A., et al. (1995). I1-Imidazoline Receptors. Definition, Characterization, Distribution, and Transmembrane Signaling. Ann. N. Y. Acad. Sci. 763, 22–42. doi:10.1111/j.1749-6632.1995.tb32388.x
Ernsberger, P., Meeley, M. P., Mann, J. J., and Reis, D. J. (1987). Clonidine Binds to Imidazole Binding Sites as Well as Alpha 2-adrenoceptors in the Ventrolateral Medulla. Eur. J. Pharmacol. 134, 1–13. doi:10.1016/0014-2999(87)90125-7
Ferrari, F., Fiorentino, S., Mennuni, L., Garofalo, P., Letari, O., Mandelli, S., et al. (2011). Analgesic Efficacy of CR4056, a Novel Imidazoline-2 Receptor Ligand, in Rat Models of Inflammatory and Neuropathic Pain. J. Pain Res. 4, 111–125. doi:10.2147/JPR.S18353
Griñán-Ferré, C., Vasilopoulou, F., Abás, S., Rodríguez-Arévalo, S., Bagán, A., Sureda, F. X., et al. (2019). Behavioral and Cognitive Improvement Induced by Novel Imidazoline I2 Receptor Ligands in Female SAMP8 Mice. Neurotherapeutics 16, 416–431. doi:10.1007/s13311-018-00681-5
Hudson, A. L., Chapleo, C. B., Lewis, J. W., Husbands, S., Grivas, K., Mallard, N. J., et al. (1997). Identification of Ligands Selective for Central I2-Imidazoline Binding Sites. Neurochem. Int. 30, 47–53. doi:10.1016/s0197-0186(96)00037-x
Ishida-Takahashi, A., Horie, M., Tsuura, Y., Ishida, H., Ai, T., and Sasayama, S. (1996). Block of Pancreatic ATP-Sensitive K+ Channels and Insulinotrophic Action by the Antiarrhythmic Agent, Cibenzoline. Br. J. Pharmacol. 117, 1749–1755. doi:10.1111/j.1476-5381.1996.tb15349.x
Li, J. X. (2017). Imidazoline I2 Receptors: An Update. Pharmacol. Ther. 178, 48–56. doi:10.1016/j.pharmthera.2017.03.009
Mar, G. Y., Chou, M. T., Chung, H. H., Chiu, N. H., Chen, M. F., and Cheng, J. T. (2013). Changes of Imidazoline Receptors in Spontaneously Hypertensive Rats. Int. J. Exp. Pathol. 94, 17–24. doi:10.1111/iep.12000
Michel, M. C., and Ernsberger, P. (1992). Keeping an Eye on the I Site: Imidazoline-Preferring Receptors. Trends Pharmacol. Sci. 13, 369–370.
Mohrman, D. E., and Heller, L. J. (2014). “Vascular Control,” in Cardiovascular Physiology. Editors D. E. Mohrman, and L.J. Heller (New York: McGraw-Hill Education), 126–156.
Muntsant, A., Jiménez-Altayó, F., Puertas-Umbert, L., Jiménez-Xarrie, E., Vila, E., and Giménez-Llort, L. (2021). Sex-Dependent End-Of-Life Mental and Vascular Scenarios for Compensatory Mechanisms in Mice with Normal and AD-Neurodegenerative Aging. Biomedicines 9, 111. doi:10.3390/biomedicines9020111
Nutt, D. J., French, N., Handley, S., Hudson, A., Husbands, S., Jackson, H., et al. (1995). Functional Studies of Specific Imidazoline-2 Receptor Ligands. Ann. N. Y. Acad. Sci. 763, 125–139. doi:10.1111/j.1749-6632.1995.tb32397.x
Piletz, J. E., Chikkala, D. N., and Ernsberger, P. (1995). Comparison of the Properties of Agmatine and Endogenous Clonidine-Displacing Substance at Imidazoline and Alpha-2 Adrenergic Receptors. J. Pharmacol. Exp. Ther. 272, 581–587.
Proks, P., and Ashcroft, F. M. (1997). Phentolamine Block of KATP Channels Is Mediated by Kir6.2. Proc. Natl. Acad. Sci. U. S. A. 94, 11716–11720. doi:10.1073/pnas.94.21.11716
Regunathan, S., Youngson, C., Wang, H., and Reis, D. J. (1995). Imidazoline Receptors in Vascular Smooth Muscle and Endothelial Cells. Ann. N. Y. Acad. Sci. 763, 580–590. doi:10.1111/j.1749-6632.1995.tb32453.x
Rodriguez-Arévalo, S., Bagán, A., Griñán-Ferré, C., Vasilopoulou, F., Pallàs, M., et al. (2021). Benzofuranyl-2-imidazoles as Imidazoline I2 Receptor Ligands for Alzheimer's Disease. Eur. J. Med. Chem. 222, 113540. doi:10.1016/j.ejmech.2021.113540
Santhanam, A. V., Viswanathan, S., and Dikshit, M. (2007). Activation of Protein Kinase B/Akt and Endothelial Nitric Oxide Synthase Mediates Agmatine-Induced Endothelium-Dependent Relaxation. Eur. J. Pharmacol. 572, 189–196. doi:10.1016/j.ejphar.2007.06.031
Santos, J. D., Paulo, M., Vercesi, J. A., and Bendhack, L. M. (2021). Thromboxane-prostanoid Receptor Activation Blocks ATP-Sensitive Potassium Channels in Rat Aortas. Clin. Exp. Pharmacol. Physiol. 48, 1537–1546. doi:10.1111/1440-1681.13557
Sarac, B., Korkmaz, O., Altun, A., Bagcivan, I., Göksel, S., Yildirim, S., et al. (2015). Investigation of the Vasorelaxant Effects of Moxonidine and its Relaxation Mechanism on the Human Radial Artery when Used as a Coronary Bypass Graft. Interact. Cardiovasc. Thorac. Surg. 21, 342–345. doi:10.1093/icvts/ivv136
Tammaro, P., Smith, A. L., Hutchings, S. R., and Smirnov, S. V. (2004). Pharmacological Evidence for a Key Role of Voltage-Gated K+ Channels in the Function of Rat Aortic Smooth Muscle Cells. Br. J. Pharmacol. 143, 303–317. doi:10.1038/sj.bjp.0705957
Tykocki, N. R., Boerman, E. M., and Jackson, W. F. (2017). Smooth Muscle Ion Channels and Regulation of Vascular Tone in Resistance Arteries and Arterioles. Compr. Physiol. 7, 485–581. doi:10.1002/cphy.c160011
Ungvari, Z., Tarantini, S., Kiss, T., Wren, J. D., Giles, C. B., Griffin, C. T., et al. (2018). Endothelial Dysfunction and Angiogenesis Impairment in the Ageing Vasculature. Nat. Rev. Cardiol. 15, 555–565. doi:10.1038/s41569-018-0030-z
Vasilopoulou, F., Bagan, A., Rodriguez-Arevalo, S., Escolano, C., Griñán-Ferré, C., and Pallàs, M. (2020). Amelioration of BPSD-Like Phenotype and Cognitive Decline in SAMP8 Mice Model Accompanied by Molecular Changes after Treatment with I2-Imidazoline Receptor Ligand MCR5. Pharmaceutics 12, 475. doi:10.3390/pharmaceutics12050475
Vasilopoulou, F., Rodríguez-Arévalo, S., Bagán, A., Escolano, C., Griñán-Ferré, C., and Pallàs, M. (2021). Disease-modifying Treatment with I2 Imidazoline Receptor Ligand LSL60101 in an Alzheimer's Disease Mouse Model: A Comparative Study with Donepezil. Br. J. Pharmacol. 178, 3017–3033. doi:10.1111/bph.15478
Weng, X. C., Gai, X. D., Zheng, J. Q., and Li, J. (2003). Agmatine Blocked Voltage-Gated Calcium Channel in Cultured Rat Hippocampal Neurons. Acta Pharmacol. Sin. 24, 746–750.
Wong, S. L., Wong, W. T., Tian, X. Y., Lau, C. W., and Huang, Y. (2010). Prostaglandins in Action Indispensable Roles of Cyclooxygenase-1 and -2 in Endothelium-dependent Contractions. Adv. Pharmacol. 60, 61–83. doi:10.1016/B978-0-12-385061-4.00003-9
Wu, Z. Z., Li, D. P., Chen, S. R., and Pan, H. L. (2009). Aminopyridines Potentiate Synaptic and Neuromuscular Transmission by Targeting the Voltage-Activated Calcium Channel Beta Subunit. J. Biol. Chem. 284, 36453–36461. doi:10.1074/jbc.M109.075523
Zhang, L., Xu, S., Wu, X., Chen, J., Guo, X., Cao, Y., et al. (2020). Combined Treatment with 2-(2-Benzofu-Ranyl)-2-Imidazoline and Recombinant Tissue Plasminogen Activator Protects Blood-Brain Barrier Integrity in a Rat Model of Embolic Middle Cerebral Artery Occlusion. Front. Pharmacol. 11, 801. doi:10.3389/fphar.2020.00801
Keywords: imidazoline receptor 2 ligands, mouse aorta, endothelium-independent vasodilatation, vascular aging, endothelial dysfunction, potassium and calcium ion channels, L-type voltage-gated Ca2+ channels, KATP channels
Citation: Jiménez-Altayó F, Cabrera A, Bagán A, Giménez-Llort L, D’Ocon P, Pérez B, Pallàs M and Escolano C (2022) An Imidazoline 2 Receptor Ligand Relaxes Mouse Aorta via Off-Target Mechanisms Resistant to Aging. Front. Pharmacol. 13:826837. doi: 10.3389/fphar.2022.826837
Received: 01 December 2021; Accepted: 26 April 2022;
Published: 12 May 2022.
Edited by:
Heike Wulff, University of California, Davis, United StatesReviewed by:
Igor Vorobyov, University of California, Davis, United StatesUlf Simonsen, Aarhus University, Denmark
Copyright © 2022 Jiménez-Altayó, Cabrera, Bagán, Giménez-Llort, D’Ocon, Pérez, Pallàs and Escolano. This is an open-access article distributed under the terms of the Creative Commons Attribution License (CC BY). The use, distribution or reproduction in other forums is permitted, provided the original author(s) and the copyright owner(s) are credited and that the original publication in this journal is cited, in accordance with accepted academic practice. No use, distribution or reproduction is permitted which does not comply with these terms.
*Correspondence: Francesc Jiménez-Altayó, francesc.jimenez@uab.cat