- 1Department of Critical Care Medicine, Shanghai Children’s Hospital, Shanghai Jiao Tong University School of Medicine, Shanghai, China
- 2Institute of Antibiotics, Huashan Hospital, Fudan University, Shanghai, China
- 3Key Laboratory of Clinical Pharmacology of Antibiotics, National Health Commission of the People’s Republic of China, Shanghai, China
- 4Phase I Clinical Trial Center, Huashan Hospital, Fudan University, Shanghai, China
Background: Polymyxin B has become the last choice for patient with carbapenem-resistant bacterial infection. However, the optimal dosing of polymyxin B in critically ill children receiving continuous renal replacement therapy (CRRT) remains unclear.
Case Presentation: Two cases of critically ill pediatric patients (7 years old) with acute kidney injury requiring continuous renal replacement (CRRT) received polymyxin B treatment due to carbapenem-resistant organism bloodstream infections. Therapeutic drug monitoring (TDM) of polymyxin B was carried out by liquid chromatography tandem mass spectrometry (LC-MS/MS). The average steady-state plasma concentration (Css,avg) of 2–4 mg/L was set as the target level. Initial polymyxin B dose was 1 mg/kg every 12 h, and the Css,avg at 4–5th dosing were 1.76 and 1.06 mg/L for patient 1 and patient 2, respectively. TDM-guided polymyxin B dose was escalated to 2 mg/kg every 12 h for both patients, resulting in the Css,avg of 2.60 and 1.73 mg/L, and the infection was controlled subsequently. Css,avg of polymyxin B with the same dosing regimens and infusion length were different during CRRT and after termination of CRRT for both patients (2.60 mg/L vs. 4.94 mg/L with 2 mg/kg every 12 h in 2 h infusion for patient 1; and 1.73 mg/L vs. 3.53 mg/L with 2 mg/kg every 12 h in 2 h infusion for patient 2). The estimation of drug exposure (estimated by AUCss,12h at the same dose) during CRRT and cessation of CRRT showed that 45% and 51% of polymyxin B was cleared during CRRT.
Conclusion: Our study showed high clearance of polymyxin B through CRRT, and supplanted dosing of polymyxin B is necessary in pediatric patients undergoing CRRT.
Introduction
Carbapenem-resistant organisms (CROs) are global public health threat due to limited options of antimicrobials. Polymyxins are considered as a last option against CROs (Satlin et al., 2020). The two forms of polymyxins, polymyxin B and colistin, are clinically available. Notwithstanding the similar structures and antimicrobial activity, polymyxin B has some advantages such as direct administration in the active form and lower risk of nephrotoxicity (Tsuji et al., 2019). Furthermore, polymyxin B is the only available form of polymyxins in some countries, although colistin has been more broadly used than polymyxin B internationally (Satlin et al., 2020).
Pharmacokinetic (PK) studies are of paramount importance for suitable dosage of drugs, and to date, research on PK of polymyxin B is conducted mainly with adults (Sandri et al., 2013; Liu et al., 2021). International consensus guidelines recommended a target average steady-state plasma concentration (Css,avg) of 2–4 mg/L for the optimal use of polymyxin B. However, the guidelines have not provided the recommend dosage regimens in children (Tsuji et al., 2019). There are only a few studies that reported polymyxin B used in pediatric patients, with a dose of 1∼4 mg/kg per day (Shih and Gaik, 2014; Gothwal et al., 2016). The huge diversity of doses provided in these studies has made it hard to establish the optimal dose for pediatrics. Furthermore, continuous renal replacement therapy (CRRT) has been increasingly used in critically ill patients with fluid overload or acute kidney injury (AKI). Antibiotics dosage in patients with normal renal function cannot be directly applied to patients under CRRT because of the different pharmacokinetics (Willems et al., 2021). Clinical data also suggested that dose in patients undergoing CRRT could potentially lead to drug underexposure, resulting poor outcomes (Li et al., 2020). Nevertheless, there was a lack of comprehensive guidelines providing antibiotics strategies of polymyxin B for patients undergoing CRRT (Pistolesi et al., 2019). Two reports including three cases of adult patients receiving polymyxin B while CRRT was being operated suggested both continuous venovenous hemodialysis (CVVHD) and continuous venovenous hemofiltration (CVVHF) had a limited impact (no more than 12% clearance) on the removal of polymyxin B (Teng et al., 2008; Sandri et al., 2012). Contrarily, another study with three critical ill adults reported that 45% of colistin was removed during CRRT under the CVVHDF mode (Liu et al., 2020). Whether continuous venovenous hemodiafiltration (CVVHDF) would affect the clearance of polymyxin B in pediatric patients has not been reported. This report evaluates the extent of polymyxin B clearance by continuous hemodiafiltration in two critically ill pediatric patients.
Case Description
Patients
Case 1 was a 7-year-old female with a previous history of nephrotic syndrome. She was admitted to the nephrology department due to repeated puffiness for nearly 3 months, on January 23rd, 2021. Two weeks later, she was transferred to the pediatric intensive unit (PICU) for severe pneumocystis pneumonia, respiratory failure, sepsis, and AKI. She was received mechanical ventilation, sulfamethoxazole–trimethoprim, caspofungin, and CRRT. After 3 weeks, carbapenem-resistant K. Pneumoniae was isolated from both her urinary catheter and blood. Minimum inhibitory concentrations (MICs) and disk diffusion tests (DISK) of K. pneumoniae showed it was only susceptible to polymyxin B (15 mm of DISK and 0.5 mg/L of MIC) and tigecycline (2 mg/L of MIC). Tigecycline combined with fosfomycin was applied, but the efficacy was very limited. Therefore, tigecycline and fosfomycin were replaced by polymyxin B from March 5th 2021 (1 mg/kg every 12 h). On March 18th, due to the recovery of kidney function, CRRT ceased and polymyxin B therapy continued until April 5th.
Case 2 was a 7-year-old female with fulminant myocarditis. She was admitted to the PICU and given venoarterial extracorporeal membrane oxygenation (VA-ECMO) on June 30th 2021. The duration of extracorporeal cardiopulmonary resuscitation (ECPR) was about 70 min, leading to multiple organ dysfunction syndrome (MODS). In addition, CRRT was required (from June 30th to July 25th) for AKI. After 8 days, ECMO was weaned. However, the body temperature elevated between 38 and 40°C, accompanied by an increase in C-reactive protein (123 mg/L) and procalcitonin (16.95 ng/mL). Stenotrophomonas maltophilia (multidrug-resistant bacteria, susceptible to cefoperazone–sulbactam and polymyxin B) was identified by blood culture, abdominal fluid and in ECMO catheters. Meanwhile, Aspergillus fumigatus was cultured using bronchoalveolar lavage fluid. With cefoperazone–sulbactam combined with linezolid and voriconazole treatment, the clinical symptoms did not ameliorate. Therefore, polymyxin B (17 mm of DISK) was added from July 10th 2021 to August 4th 2021.
Continuous Renal Replacement Therapy Mode and Parameters
Two patients received CRRT with the CVVHDF mode (GAMBRO, Prismaflex, hemofilter M60). As the coagulation dysfunction, citric acid was used for anticoagulation for both the patients. For patient 1, 25 kg, the settings of CRRT were 30 mL/min for blood flow, 60 mL/h for citric acid, 100 mL/h for replacement fluid, 300 mL/h for dialysate fluid, and 60 mL/h for effluent. For patient 2, 20 kg, 40 mL/min for blood flow, 64 mL/h for citric acid, 300 mL/h for replacement fluid, 150 mL/h for dialysate fluid, and 80 mL/h for effluent was set.
Samples and Therapeutic Drug Monitoring
For both patients, trough and peak blood samples at steady state (about 0.5 h before and after the infusion of 4–5th dosing) were collected for TDM of polymyxin B. If the dosage regimens needed to be adjusted according to TDM, trough and peak blood samples after the third post-adjustment dose were collected. In the meantime, post-filtration blood samples were collected at the same time to evaluate the drug elimination during CRRT.
Polymyxins B1 and B2 were determined following a validated liquid chromatography tandem with mass spectrometry (LC-MS/MS) method (Liu et al., 2020). In brief, plasma samples were mixed with internal standard (polymyxin E2) and 30% ammonia and then loaded on a preconditioned (conditioned by methanol and water as per product instruction) 96-well Oasis WCX plate (30 mg, Waters Corporation, Milford, MA, United States). The WCX plate was washed and eluted by 30% acetonitrile in water (containing 6% formic acid, v/v). The elute was injected into LC-MS/MS (Shimadzu 30A series coupled with AB Sciex Triple Quad 5500 mass spectrometry platform) for analysis. Concentrations of polymyxin B were calculated by the sum of polymyxins B1 and B2 by the molar terms and molecular weight. The Css,avg of 2–4 mg/L (estimated by the average of peak and trough concentration) of polymyxin B was set as the target concentration.
AUC Estimates
Compartmental modeling using WinNonlin 8.0 was applied for PK parameters estimation and AUC calculation. Due to the limited data with Cmax and Ctrough for the second patient, an alternative equation-based approach for two PK samples methods was used for the AUC24h estimates (Pai et al., 2014) under similar eGFR. The equations are given as follows:
where Ke is the elimination rate constant, t2 is the drug administration interval, tinfusion is the infusion length, and n is the administration times during 24 h.
Results
Polymyxin B and Plasma Concentrations
After treatment with polymyxin B (1 mg/kg, every 12 h, Css,avg = 1.76 mg/L), C-reactive protein (CRP) of patient 1 decreased from 13 mg/L to 5 mg/L, and procalcitonin (PCT) fell from 18.04 ng/mL to 5.62 ng/mL. Nonetheless, the body temperature of patient 1 fluctuated around 38.0°C. When Css,avg ascended to 2.60 mg/L, CRP and PCT decreased to the normal range (CRP ≤ 5 mg/L, PCT 0.02 ng/ml) and the body temperature to normal. For patient 2, the levels of CRP and PCT did not decline until the Css,avg of polymyxin B reached 1.73 mg/L. The concentrations of polymyxin B at corresponding dosing regimens for the two patients and estimated glomerular filtration rate (eGFR, calculated by Schwartz formula) levels are presented in Table 1. In addition, the polymyxin B dose regimens with the concomitant drugs and C-reactive protein (CRP) and procalcitonin (PCT) levels at different times are shown in Figure 1.
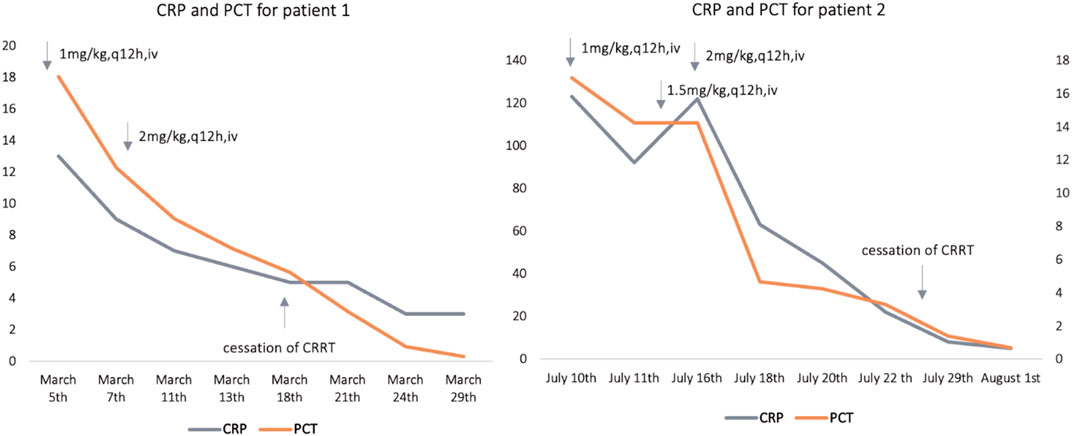
FIGURE 1. Polymyxin B dose regimens with the concomitant drugs and estimated C-reactive protein (CRP), and procalcitonin (PCT) levels at different time points; for patient 1, cessation of CRRT (continuous renal replacement) was on 18th March; for patient 2, cessation of CRRT was on 25th July. CRP: C-reactive protein (mg/L), PCT: procalcitonin (ng/mL).
Comparing Css,avg of polymyxin B with the same dosing regimens and infusion length, it showed significantly lower concentrations during CRRT than that after termination of CRRT for both patients (2.60 mg/L vs. 4.94 mg/L with 2 mg/kg every 12 h in 2 h infusion for patient 1; and 1.73 mg/L vs. 3.53 mg/L with 2 mg/kg every 12 h in 2 h infusion for patient 2).
Drug Removal by the Hemofilter Membrane
To assess whether CRRT could remove polymyxin B, blood samples from prefilter and postfilter were measured (Figure 2). The same type of hemofilter membrane was used for both patients. The concentrations of post-filtration and pre-filtration blood samples were 0.23 and 3.31 mg/L, respectively, which indicated 93.1% of polymyxin B was removed during the CVVHDF mode for patient 1. Two more pairs of pre- and post-filtration blood samples were evaluated for the drug removal during CRRT, and plasma concentrations showed 90.9–95.3% of polymyxin B was cleared. Similarly, 86.6% of polymyxin B was cleared for patient 2, with the concentrations of 0.43 and 3.19 mg/L (Table 2).
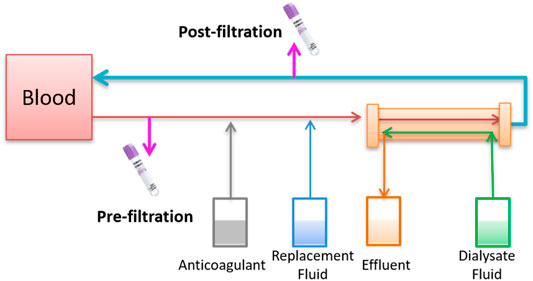
FIGURE 2. Schematic diagram of blood collection in the extracorporeal catheter prefilter and postfilter of CRRT.

TABLE 2. Concentration of polymyxin B of prefilter and postfilter at 0.5 h after infusion during CRRT.
Compartmental modeling was conducted using WinNonlin 8.0 for patient 1 whose samples were collected during and recess of CRRT. Drug exposure (estimated by AUCss,12h at the same dose) during CRRT and recess of CRRT through a preliminary pharmacokinetic modeling for patient 1 was estimated. The results showed that 45% of polymyxin B was removed during CRRT (Figure 3). By applying the equation-based method for calculating AUC24h for the first patient, 57% of polymyxin B was removed during CRRT, which was similar to that obtained using the compartmental modeling method. The same equation-based method was applied for the AUC24h estimation of the second patient. It was calculated that AUC24h was 30.2 mg•h/L and 60.6 mg•h/L for the 2 g and 2 h infusion of polymyxin B during CRRT and without CRRT, respectively, indicating 51% of polymyxin B lost during CRRT.
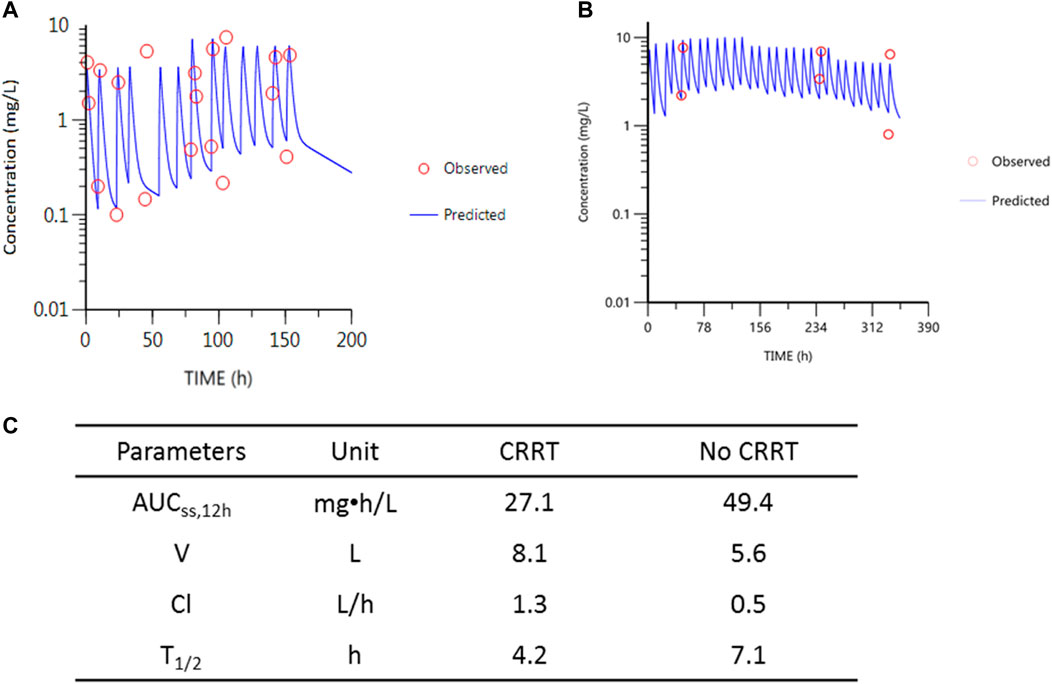
FIGURE 3. PK parameters of polymyxin B and the simulations for patient 1. (A) Observed concentrations vs. predicted concentrations during CRRT. (B) Observed concentrations vs. predicted concentrations after recess of CRRT. The last two samples in (B) were deviated from the blue line due to the dose adjustments from 1.5 mg/kg (with 0.5 mg/kg inhalation) to 1.0 mg/kg infusion. Red circles represent observed concentrations; blue line represents the predicted concentrations according to the two-compartmental PK model. (C) Table for the PK parameters during CRRT and without CRRT.
Discussion
The incidence of carbapenem-resistant organism infection is increasing globally, mainly carbapenem-resistant Enterobacteriaceae and Acinetobacter baumannii. Polymyxin is considered as the salvage therapy for aforementioned infections. The international consensus guideline recommended a polymyxin B dose of 1.25–1.5 mg/kg every 12 h for patients with severe infection patients. With doses of 2.5 and 3.0 mg/kg/day, 90% of adults would be expected to achieve a Css,avg of 1.8 and 2.2 mg/L, respectively (Tsuji et al., 2019). In addition, the consensus guideline suggested that polymyxin B dose need not be adjusted during CRRT. However, the guideline has not provided the recommended dosage regimens for children. In our study, to achieve the target Css,avg, a polymyxin B dose of 4.0 mg/kg/d was needed, which was far from the recommended doses in adults.
Young children show changes in drug absorption, disposition, metabolism, and excretion of drugs (Kearns et al., 2003; Downes et al., 2014). For example, in absorptive surfaces such as the gastrointestinal tract, skin, and pulmonary tree, it can influence the rate and extent of the bioavailability of drugs. Age-related changes in body composition alter the volume of distribution of antibiotics. Delayed maturation of drug-metabolizing enzyme activity and developmental changes in renal function account for the difference in drug clearance. Additionally, in critically ill patients, the hemodynamic alterations or renal dysfunction during critical illness pathophysiologically change the volume of distribution, protein binding, and drug clearance. In PICU patients, antibiotic concentrations are outside of the therapeutic window up to 95%, while these non-target concentrations in adult critically ill patients are up to 41% (Hartman et al., 2019). In our study, the clearance of polymyxin B (Cl) was 1.3 L/h (correspondingly 0.05 L/h/kg) during CRRT and 0.5 L/h (correspondingly 0.02 L/h/kg) without CRRT, which was in the range of Cl reported previously (0.02–0.07 L/h/kg in critically ill patients) (Sandri et al., 2013; Liu et al., 2021). The half-life (t1/2) of polymyxin B was different from that during CRRT and without CRRT (4.2 vs. 7.1 h), but in line with previous reports (healthy subjects (∼5 h) and critically ill patients (∼11.9 h) (Sandri et al., 2013; Liu et al., 2021). These suggested that changes in the dose of polymyxin B in these two patients had little to do with the special population of children themselves.
On the other hand, antibiotics with large molecular weight and high protein binding rate forming drug–protein complexes are not easily removed by CRRT (Pistolesi et al., 2019). Therefore, in theory, polymyxin B is difficult to be removed during CRRT because of its large molecular weight of more than 1,000 Da (e.g., 1,203, 1,189, and 1,203 for polymyxins B1, B2, and B1-Ile, respectively) and protein binding rate of 58% (Sandri et al., 2013). Two reports with three cases of adult patients suggested that only 5.6% and 12.2% of polymyxin B were removed by CVVHD and 5.0% was removed by CVVHF (Teng et al., 2008; Sandri et al., 2012). Conversely, another study with three critically ill adults reported that 45% of colistin was removed during CRRT under the CVVHDF mode (Nikolaos et al., 2012). In this study, both cases showed significant drug clearance of polymyxin B during CRRT with the CVVHDF mode. Comparing Css,avg of polymyxin B with the same dosing regimens and infusion length, it showed significantly lower concentrations during CRRT than that after termination of CRRT for both patients (2.60 mg/L vs. 4.94 mg/L with 2 mg/kg every 12 h in 2 h infusion for patient 1; and 1.73 mg/L vs. 3.53 mg/L with 2 mg/kg every 12 h in 2 h infusion for patient 2). This was also confirmed by the estimation of drug exposure (estimated by AUCss,12h at the same dose) during CRRT and recess of CRRT through a preliminary pharmacokinetic. Results showed that 45% and 51% of polymyxin B was clearance during CRRT which was much higher than that in the reported studies (no more than 12%) (Teng et al., 2008; Sandri et al., 2012). In addition, the plasma concentrations in prefilter and postfilter membranes showed 86.6–95.3% drug removal during filtration, suggesting the remarkable extracorporeal clearance. A previous study reported colistin absorption by the hemofilter, and the removal of dialysis contributed to its extracorporeal clearance (Nikolaos et al., 2012). In our study, we measured the concentration of the effluent fluid of CRRT, but due to the dilution effect and the large volume of the effluent (more than 5 L for 12 h), the concentration of polymyxin B was lower than the detection limit of the LC-MS/MS assay. Further studies are needed to evaluate the drug removal by adsorption of the hemofilter. Since this study only provided two cases, the exact clearance of polymyxin B through CRRT indicating supplanted dosing is necessary for pediatric patients undergoing CRRT.
Conclusion
CRRT (CVVHDF mode) significantly affects the drug concentrations and exposure of polymyxin B in the present two cases, indicating supplantation of polymyxin B is necessary in pediatrics undergoing CRRT. The TDM of polymyxin B is critically important for the dosing regimen optimization for patients undergoing CRRT. Larger PK studies are urgently needed to further refine dosing recommendations of polymyxin B in pediatric population receiving CRRT.
Data Availability Statement
The original contributions presented in the study are included in the article/Supplementary Material, further inquiries can be directed to the corresponding authors.
Ethics Statement
This study was reviewed and approved by the Huashan Hospital Institutional Review Board and Clinical Research Ethics Committee of Shanghai Children’s Hospital affiliated to Shanghai Jiao Tong University School of Medicine. The patients’ legal guardian provided their written informed consent to participate in this study. Written informed consent was obtained from the patients’ legal guardian for the publication of any potentially identifiable images or data included in this article.
Author Contributions
CX and XoL drafted the manuscript and collected data; YZ, YC, and JZ provided insights and edited the manuscript; and XH, YW, YF, HW, XnL, and BG helped collecting data and worked on the figures and tables.
Funding
This research was supported by the Shanghai Municipal Science and Technology Commission (Grant Number: 19411964900).
Conflict of Interest
The authors declare that the research was conducted in the absence of any commercial or financial relationships that could be construed as a potential conflict of interest.
Publisher’s Note
All claims expressed in this article are solely those of the authors and do not necessarily represent those of their affiliated organizations, or those of the publisher, the editors, and the reviewers. Any product that may be evaluated in this article, or claim that may be made by its manufacturer, is not guaranteed or endorsed by the publisher.
References
Downes, K. J., Hahn, A., Wiles, J., Courter, J. D., and Vinks, A. A. (2014). Dose Optimisation of Antibiotics in Children: Application of Pharmacokinetics/pharmacodynamics in Paediatrics. Int. J. Antimicrob. Agents 43 (3), 223–230. doi:10.1016/j.ijantimicag.2013.11.006
Gothwal, S., Meena, K., and Sharma, S. D. (2016). Polymyxin B Induced Generalized Hyperpigmentation in Neonates. Indian J. Pediatr. 83, 179–180. doi:10.1007/s12098-015-1798-z
Hartman, S. J. F., Brüggemann, R. J., Orriëns, L., Dia, N., Schreuder, M. F., and de Wildt, S. N. (2019). Pharmacokinetics and Target Attainment of Antibiotics in Critically Ill Children: A Systematic Review of Current Literature. Clin. Pharmacokinet. 59 (2), 173–205. doi:10.1007/s40262-019-00813-w
Kearns, G. L., Abdel-Rahman, S. M., Alander, S. W., Blowey, D. L., Leeder, J. S., and Kauffman, R. E. (2003). Developmental Pharmacology-Ddrug Disposition, Action, and Therapy in Infants and Children. N. Engl. J. Med. 349, 1157–1167. doi:10.1056/NEJMra035092
Li, L., Li, X., Xia, Y., Chu, Y., Zhong, H., Li, J., et al. (2020). Recommendation of Antimicrobial Dosing Optimization during Continuous Renal Replacement Therapy. Front. Pharmacol. 11, 786. doi:10.3389/fphar.2020.00786
Liu, X., Yu, Z., Wang, Y., Wu, H., Bian, X., Li, X., et al. (2020). Therapeutic Drug Monitoring of Polymyxin B by LC-MS/MS in Plasma and Urine. Bioanalysis 12, 845–855. doi:10.4155/bio-2020-0051
Liu, X., Chen, Y., Yang, H., Li, J., Yu, J., Yu, Z., et al. (2021). Acute Toxicity Is a Dose-Limiting Factor for Intravenous Polymyxin B: A Safety and Pharmacokinetic Study in Healthy Chinese Subjects. J. Infect. 82, 207–215. doi:10.1016/j.jinf.2021.01.006
Nikolaos, M., Marizoza, F., Markantonis, S. L., Zidianakis, B., Hroni, D., Boutzouka, E., et al. (2012). Colistin Pharmacokinetics in Intensive Care Unit Patients on Continuous Venovenous Haemodiafiltration: an Observational Study[J]. J. Antimicrob. Chemother. 67 (10), 2459–2462. doi:10.1093/jac/dks257
Pai, M. P., Neely, M., Rodvold, K. A., and Lodise, T. P. (2014). Innovative Approaches to Optimizing the Delivery of Vancomycin in Individual Patients. Adv. Drug Deliv. Rev. 77, 50–57. doi:10.1016/j.addr.2014.05.016
Pistolesi, V., Morabito, S., Di Mario, F., Regolisti, G., Cantarelli, C., and Fiaccadori, E. (2019). A Guide to Understanding Antimicrobial Drug Dosing in Critically Ill Patients on Renal Replacement Therapy. Antimicrob. Agents Chemother. 63, e00583-19. doi:10.1128/AAC.00583-19
Sandri, A. M., Landersdorfer, C. B., Jacob, J., Boniatti, M. M., Dalarosa, M. G., Falci, D. R., et al. (2013). Population Pharmacokinetics of Intravenous Polymyxin B in Critically Ill Patients: Implications for Selection of Dosage Regimens. Clin. Infect. Dis. 57, 524–531. doi:10.1093/cid/cit334
Sandri, A. M., Landersdorfer, C. B., Jacob, J., Boniatti, M. M., Dalarosa, M. G., Falci, D. R., et al. (2012). Pharmacokinetics of Polymyxin B in Patients on Continuous Venovenous Haemodialysis. J. Antimicrob. Chemother. 68, 674–677. doi:10.1093/jac/dks437
Satlin, M. J., Lewis, J. S., Weinstein, M. P., Patel, J., Humphries, R. M., Kahlmeter, G., et al. (2020). Clinical and Laboratory Standards Institute and European Committee on Antimicrobial Susceptibility Testing Position Statements on Polymyxin B and Colistin Clinical Breakpoints. Clin. Infect. Dis. 71, e523–e9. doi:10.1093/cid/ciaa121
Shih, L. K., and Gaik, C. L. (2014). Polymyxin B Induced Generalized Skin Hyperpigmentation in Infants. J. Pediatr. Neurosciences 6, e215. doi:10.17334/jps.10375
Teng, C. B., Koh, P. T., Lye, D. C., and Ang, B. S. (2008). Continuous versus Intermittent Infusion of Polymyxin B in the Treatment of Infections Caused by Multidrug-Resistant Gram-Negative Bacteria. Int. J. Antimicrob. Agents 31, 80–82. doi:10.1016/j.ijantimicag.2007.08.004
Tsuji, B. T., Pogue, J. M., Zavascki, A. P., Paul, M., Daikos, G. L., Forrest, A., et al. (2019). International Consensus Guidelines for the Optimal Use of the Polymyxins: Endorsed by the American College of Clinical Pharmacy (ACCP), European Society of Clinical Microbiology and Infectious Diseases (ESCMID), Infectious Diseases Society of America (IDSA), International Society for Anti-infective Pharmacology (ISAP), Society of Critical Care Medicine (SCCM), and Society of Infectious Diseases Pharmacists (SIDP). Pharmacotherapy 39, 10–39. doi:10.1002/phar.2209
Keywords: polymyxin B, CRRT, children, therapeutic drug monitoring, pharmacokinetic
Citation: Xu C, Liu X, Cui Y, Huang X, Wang Y, Fan Y, Wu H, Li X, Guo B, Zhang J and Zhang Y (2022) Case Report: Therapeutic Drug Monitoring of Polymyxin B During Continuous Renal Replacement Therapy in Two Pediatric Patients: Do Not Underestimate Extracorporeal Clearance. Front. Pharmacol. 13:822981. doi: 10.3389/fphar.2022.822981
Received: 26 November 2021; Accepted: 03 March 2022;
Published: 24 March 2022.
Edited by:
Raffaele Simeoli, Bambino Gesù Children’s Hospital (IRCCS), ItalyReviewed by:
Abdul Sami Shaikh, Shah Abdul Latif University, PakistanKamilia Abdelraouf, Hartford Hospital, United States
Copyright © 2022 Xu, Liu, Cui, Huang, Wang, Fan, Wu, Li, Guo, Zhang and Zhang. This is an open-access article distributed under the terms of the Creative Commons Attribution License (CC BY). The use, distribution or reproduction in other forums is permitted, provided the original author(s) and the copyright owner(s) are credited and that the original publication in this journal is cited, in accordance with accepted academic practice. No use, distribution or reproduction is permitted which does not comply with these terms.
*Correspondence: Yucai Zhang, zyucai2018@163.com; Jing Zhang, zhangj_fudan@aliyun.com
†These authors have contributed equally to this work
‡ORCID: Yucai Zhang, orcid.org/0000-0002-4905-3600