- 1State Key Laboratory of Southwestern Chinese Medicine Resources, Pharmacy School, Chengdu University of Traditional Chinese Medicine, Chengdu, China
- 2TCM Regulating Metabolic Diseases Key Laboratory of Sichuan Province, Hospital of Chengdu University of Traditional Chinese Medicine, Chengdu, China
- 3State Key Laboratory of Innovation Medicine and High Efficiency and Energy Saving Pharmaceutical Equipment, Jiangxi University of Traditional Chinese Medicine, Nanchang, China
Type 2 diabetes mellitus (T2DM) and heart failure (HF) are diseases characterized by high morbidity and mortality. They often occur simultaneously and increase the risk of each other. T2DM complicated with HF, as one of the most dangerous disease combinations in modern medicine, is more common in middle-aged and elderly people, making the treatment more difficult. At present, the combination of blood glucose control and anti-heart failure is a common therapy for patients with T2DM complicated with HF, but their effect is not ideal, and many hypoglycemic drugs have the risk of heart failure. Abnormal insulin signaling pathway, as a common pathogenic mechanism in T2DM and HF, could lead to pathological features such as insulin resistance (IR), myocardial energy metabolism disorders, and vascular endothelial disorders. The therapy based on the insulin signaling pathway may become a specific therapeutic target for T2DM patients with HF. Here, we reviewed the mechanisms and potential drugs of insulin signaling pathway in the treatment of T2DM complicated with HF, with a view to opening up a new perspective for the treatment of T2DM patients with HF and the research and development of new drugs.
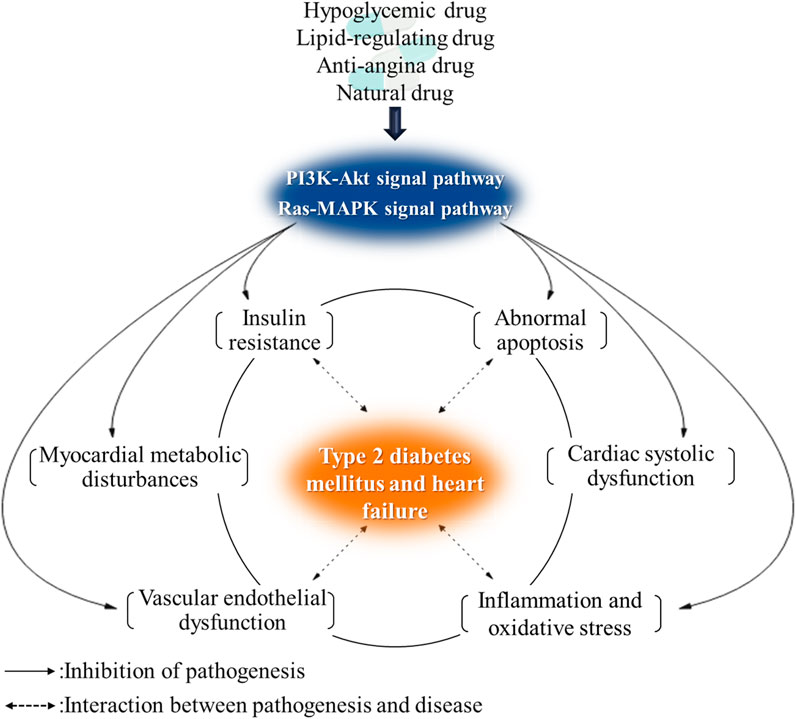
Graphical Abstract. The insulin signaling pathway is a common signaling pathway to regulate myocardial energy metabolism and IR. The regulation of insulin signaling pathway will become an effective treatment for T2DM complicated with HF.
Introduction
Type 2 diabetes mellitus (T2DM) and heart failure (HF) are common chronic diseases, which often occur simultaneously. About 415 million people worldwide suffer from diabetes, with T2DM accounting for more than 90% (Chatterjee et al., 2017). The annual meeting of the EASD (European Association for the Study of Diabetes) in 2004 pointed out that when T2DM occurs, patients have a first-degree HF. T2DM accelerates physiological cardiac aging through hyperglycemia and hyperinsulinemia (Sciacqua et al., 2021). Thus, T2DM complicated with HF is the final outcome in elderly patients with T2DM. The clinical incidence and mortality of T2DM complicated with HF are significantly higher than those of the general population, 4–8 times those of non-diabetic patients (Bell and David, 2004). Compared with patients without T2DM, patients with T2DM complicated with HF have more obvious clinical symptoms and signs, and their cardiac function classification, quality of life, and prognosis are worse (Seferović et al., 2018). Paolisso et al. (2021) also found patients with hyperglycemia versus normoglycemia had higher levels of inflammatory markers and B-type natriuretic peptide and lower left ventricular ejection fraction, and higher rates of heart failure and death. Acute hyperglycemia could induce a more severe depression of cardiac pump, with cardiac denervation, increased rate of acute HF, and worse prognosis at follow-up (Paolisso et al., 2021). Meanwhile, the intersection of T2DM and HF is mainly concentrated in elderly patients, which also increases the difficulty of its treatment.
Until recently, T2DM and HF have been managed independently. There is no effective therapy for T2DM complicated with HF, which is usually combined with blood glucose control and anti-HF therapy. The therapeutic drugs are very limited, and the effect is poor. Many hypoglycemic drugs have been found to have potential cardiovascular risks, which are not conducive to HF. And conventional drugs for HF treatment are also not effective in the treatment of DM, and it is still unclear whether they will aggravate the condition of diabetes or benefit the prognosis of diabetes. Therefore, it is necessary to further explore the treatment plan of DM combined with HF. Recently, sodium-dependent glucose transporter 2 inhibitors (SGLT2i), an oral glucose-lowering agent, have hit two birds (T2DM and HF) with a single stone (Santos-Ferreira et al., 2020). SGLT2i significantly reduced the rates of HF hospitalization in multiple cardiovascular outcome trials (CVOTs) (Marfella et al., 2021). Based on this evidence, the recent trend of SGLT2i has shifted from the prevention of HF in T2DM patients to the treatment of HF regardless of the presence of T2DM (Marfella et al., 2021). This also brings hope for drug therapy of T2DM complicated with HF.
The main pathological mechanism of T2DM complicated with HF is energy metabolism disorders and severe insulin resistance (IR). Early intervention to correct the metabolic disorder is an effective way to prevent the deterioration of the disease (Xiaoli and Shu, 2010). The insulin signaling pathway is a common signaling pathway to regulate myocardial energy metabolism and IR. Regulating this signaling pathway can improve a series of physiological and pathological mechanisms such as abnormal myocardial energy metabolism of HF, IR and vascular endothelial dysfunction, inflammation, and oxidative stress. Furthermore, it has the effect of reducing blood sugar and improving heart function, which is the best way to treat T2DM complicated with HF. In this paper, we reviewed the mechanisms and potential drugs of insulin signaling pathway in the treatment of T2DM complicated with HF to provide a comprehensive and detailed reference for relevant studies, and to contribute to the further research and development of new drugs for T2DM patients with HF.
Insulin Signaling Pathway
There are two main pathways of insulin receptor signal transduction: 1) phosphatidylinositol 3-kinase/protein kinase B (PI3K/Akt) pathway, which is involved in the survival, growth, and proliferation of cells and protein synthesis and 2) RAS/mitogen-activated protein kinase (RAS-MAPK) pathway, which mainly regulates cell differentiation.
Normal Insulin Signaling Pathway
The insulin signaling pathway includes PI3K/Akt and RAS-MAPK signaling pathways. The binding of insulin or insulin-like growth factor (IGF-1) to the corresponding receptor can phosphorylate insulin substrate-1/2 (IRS-1/2), thus activating PI3K/Akt and RAS-MAPK signaling pathways (Figure 1). The PI3K/Akt signaling pathway is the most classical insulin signaling pathway, in which AKT phosphorylation is the key role. The phosphorylation of Akt leads to the phosphorylation of many downstream targets involved in cell growth and metabolism, including glycogen synthase kinase-3β (GSK-3β), glucose transporter-4 (GLUT-4), forkhead transcription factor O1 (FoxO1), endothelial nitric oxide synthase (eNOS), nuclear factor-κB (NF-κB), P21, and mammalian target of rapamycin (mTOR), thereby mediating the cell growth and proliferation, cell cycle regulation, and glucose metabolism regulation induced by insulin and various growth factors (Plotnikov et al., 2011). The RAS-MAPK signaling pathway can be indirectly activated by phosphorylation of IRS-1/2, thereby activating extracellular signal–regulated kinases (ERK1/2), c-Jun N-terminal kinase (JNK), and p38MAPK. These signals can activate downstream related factors and regulate many physiological processes such as cell growth, proliferation, differentiation, apoptosis, and immune response (Plotnikov et al., 2011).
(After insulin and IGF-1 activate, IRS, PI3K, and RAS are activated, and phosphorylation of PI3K activates Akt. These two important insulin signaling pathways can mediate cell growth, proliferation, differentiation, apoptosis, and immune response and regulate glucose metabolism and the energy metabolism, structure, and function of the heart.)
The Change of Insulin Signaling Pathway in T2DM Patients With HF
In T2DM patients with HF, the insulin signaling pathway is obviously abnormal and accompanied by IR. In the early stage of T2DM with HF, the insulin signaling pathway is not significantly impaired. In the middle and late stages, the impairment of insulin signaling pathway is aggravated, accompanied by the synchronous aggravation of IR. In end-stage HF, the Akt signaling pathway is severely inhibited and the MAPK signaling pathway is partially active, accompanied by severe IR (Riehle and Abel, 2016).
Schulze et al. (Chokshi et al., 2012) found that myocardial Akt phosphorylation was significantly inhibited in the left ventricular samples from patients with advanced HF. After implanting the left ventricular assist device, the patient’s left ventricular function was significantly restored and the PI3K/Akt signaling cascade was activated. In addition, the activation of JNK and p38MAPK could induce apoptosis and necrosis, affect myocardial contractility, and deteriorate cardiac function (Bin, 2008). In both HF animal models and HF patients, the activities of JNK and p38MAPK in the failed myocardium are significantly higher than those in the healthy heart; also, the activities of JNK and p38MAPK in severe HF patients are also significantly higher than those in mild HF patients (Cook et al., 1999; Arabacilar and Marber, 2015). Significant improvement in cardiac function was observed after using p38MAPK inhibitors (Riba et al., 2017). These studies showed that the expression of insulin signaling pathway is abnormal during HF, especially in the end-stage HF. Meanwhile, the recovery of ventricular function is related to the activation of Akt signaling pathway and the inhibition of JNK and p38MAPK signaling pathways.
Mechanism of Treating T2DM Complicated With HF Based on Insulin Signaling Pathway
T2DM complicated with HF has a complicated pathogenesis and involves multiple pathological mechanisms, including IR, myocardial energy metabolism disorders, vascular endothelial dysfunction, abnormal apoptosis of myocardial cells, abnormal calcium circulation of the myocardium, excessive inflammation of the myocardium, and oxidative stress. Regulating the insulin signaling pathway can activate/inhibit multiple targets, mediate the above-mentioned multiple mechanisms such as glucose and lipid metabolism, apoptosis, and inflammatory factor secretion, and improve T2DM complicated with HF.
Insulin Signaling Pathway and IR
IR refers to a state in which insulin secretion is normal, but the sensitivity of tissues, organs, and cells to insulin is reduced and the role of insulin in promoting glucose uptake and utilization in target organs is reduced (Semenkovich, 2016). IR is a pathological feature of T2DM, as well as the main metabolic feature of pathophysiology of HF. Swan et al. (1997) found that the severity of chronic HF is significantly associated with increased IR, and patients with T2DM complicated with HF may have both systemic and cardiac IR. Furthermore, IR and T2DM complicated with HF are mutually causal, forming a vicious circle and worsening the disease (Figure 2).
The combined effect of systemic IR and myocardial IR can lead to a series of pathological injuries, such as impaired calcium signaling (Roe et al., 2013), substrate metabolism disorders, mitochondrial dysfunction (Qi et al., 2013) and oxidative stress, ER stress, and impaired cardiomyocyte endothelial nitric oxide (NO) signaling (Falskov et al., 2011). These pathological injuries could damage the heart, resulting in impaired calcium processing and contractility of the myocardium, decreased cardiac energy efficiency, myocardial cell apoptosis, and cardiac fibrosis (Aroor et al., 2012), accelerating the deterioration of T2DM complicated with HF (Nagoshi et al., 2011). Meanwhile, they can also aggravate IR, triggering a vicious cycle. With the development of T2DM complicated with HF, IR also aggravated. Especially in end-stage HF, patients are accompanied by severe IR. Therefore, improving IR can be a key entry point for treating T2DM complicated with HF.
Studies have shown that mitochondria play a major role in the pathogenesis of IR. An increase in mitochondrial oxidants is a consistent feature of IR in vitro and in vivo and in humans and can rapidly impair insulin-regulated GLUT4 translocation and significantly contribute to IR (Fazakerley et al., 2018). The mitochondrial oxidative stress and mitochondrial dysfunction are all related to IR (Fazakerley et al., 2018). Furthermore, the abnormality of insulin signaling pathway plays a key role in the occurrence and development of IR. IR can be induced by any abnormal insulin signal site, such as degradation, phosphorylation, and distribution of IRS, blocked activation and abnormal expression of PI3K, abnormal expression of PKD, Akt, GSK-3, and glucose transporters, reduction and activity disorder of glucose transporters, and activation of FoxO1 (Goedeke et al., 2019; Park et al., 2019). Therefore, it is beneficial for the early prevention and prognosis of T2DM complicated with HF to improve IR and break the vicious cycle of T2DM with HF by regulating the insulin signaling pathway.
(IR can induce HF through myocardial metabolic disorders, endothelial dysfunction, impaired calcium signaling transduction pathway, hormone endocrine abnormalities, inflammation, and oxidative stress. HF can also cause hyper-activation of the neuroendocrine system and inflammatory cytokine increases, which increase the free fatty acid (FFA) level and damage the insulin signaling pathway, thus leading to IR. HF and IR will form a vicious cycle, aggravating the disease.)
Insulin Signaling Pathway and Energy Metabolism Disorders
There are severe systemic and myocardial glucose and lipid metabolism disorders in T2DM patients complicated with HF, resulting in significant abnormalities of myocardial energy metabolism and dysfunction of myocardial contraction. HF is a progressive disease. The metabolic substrates show different metabolic states in different stages and degrees of HF. In the early stage of HF, cardiomyocytes are in the compensatory stage, where the oxidative metabolic rate of FA remains normal or slightly increases, and glucose oxidation may increase to supplement myocardial energy deficiency (Aroor et al., 2012). In the early and middle stages of HF, there is no significant change in the utilization of myocardial energy substrates, and myocardial mitochondria may increase for compensation to maintain the overall quantity balance (Wang et al., 2009). In the late stage of HF, the heart loses its metabolic flexibility, where FA oxidation metabolism is significantly down-regulated, and the heart relies to a greater extent on glucose oxidation as a priority metabolic substrate (Aroor et al., 2012). Severe myocardial blood oxygen deficiency leads to IR. And glucose aerobic oxidation is reduced, and the energy supply mode is changed to glycolysis (Aroor et al., 2012). The ventricular remodeling process progresses to the decompensated state, myocardial energy is in a state of lack, and pyruvate produced by glycolysis is converted to lactic acid under hypoxia, further aggravating HF (Aroor et al., 2012). In addition, cardiomyocyte apoptosis and autophagy aggravate in the late stage of HF, and the cells cannot continue to maintain the compensatory effect of synthesizing mitochondria, which significantly reduces mitochondria and exacerbates energy metabolism disorders (Wang et al., 2009).
The insulin signaling pathway can improve abnormal energy metabolism in the whole body and myocardium by regulating the conversion of metabolic substrates and mitochondrial function. After activation of Akt, GLUT4 can be directly transferred from the cytosol to the plasma membrane to enhance glucose uptake of myocardial cells, as well as regulating the expression of genes such as GSK-3 and FoxO1 to promote myocardial glucose uptake and inhibit glycogen synthesis (Melenovsky et al., 2011; Jiménez-Osorio et al., 2016). In addition, stimulation of glucose oxidation not only increases energy supply in ischemic heart disease and HF but also increases heart function due to better coupling of glycolysis and glucose oxidation (Lopaschuk et al., 2010). Meanwhile, regulating the expression of GSK-3β, mTOR, and NF-κB can improve mitochondrial function and regulate myocardial mitochondrial metabolism. Parra et al. (2014) have confirmed that the activation of Akt–mTOR–NF-κB signaling pathway increased the Opa-1 protein level in myocardial cells both in vitro and in vivo, promoted mitochondrial fusion, increased the mitochondrial membrane potential, and increased the intracellular ATP level and oxygen consumption, thereby acutely regulating mitochondrial metabolism in myocardial cells.
Therefore, when cardiac energy metabolism has not been unbalanced in the early and middle stages of HF, the regulation of insulin signaling pathway could increase the aerobic oxidation of glucose and inhibit the acceleration of cardiomyocyte apoptosis to prevent mitochondrial dysfunction, thus effectively preventing the deterioration of HF. In the late stage of HF, the body has severe energy metabolism disorder accompanied by IR. The regulation of insulin signaling pathway could increase the aerobic oxidation of glucose, inhibit the aerobic oxidation of fatty acids, improve insulin sensitivity, improve IR, and improve mitochondrial function, which are the treatments for energy metabolism in the late stage of HF.
Insulin Signaling Pathway and Vascular Endothelial Dysfunction
Vascular endothelial dysfunction (VED), a common pathological mechanism of diabetes and cardiovascular disease, directly affects the prognosis and survival of patients in the course of diabetes and cardiovascular disease. VED occurs early in the chain of atherosclerotic process and is a main determinant of acute coronary events, infarction, and reduction of cardiac pump (Harjola et al., 2020). VED can increase the whole systemic peripheral resistance and afterload through peripheral effects and aggravate the cardiac function impairment through central effects such as myocardial ischemia and inducible nitric oxide synthase (iNOS)-induced damaging effects (Penna et al., 2006). Nemoto et al. (2019) found that impaired endothelial function results in severe coronary artery stenosis as well as plaque vulnerability, while chronic hyperglycemia aggravates VED, resulting in more diffuse coronary artery lesions and worse clinical outcomes (Kang et al., 2018). Meanwhile, coronary heart disease and infarction are precipitants of acute HF, and VED under hyperglycemia is highly likely to cause acute HF and seriously increases cardiac mortality (Driscoll et al., 2017). Studies have also confirmed a higher rate of coronary endothelial dysfunction in DM patients, which is also the reason for the high incidence of HF and poor clinical outcomes in DM patients (Sardu et al., 2019a).
VED is mainly characterized by a reduction in the bioavailability of nitric oxide (NO) and excess production of reactive oxygen species (Quyyumi et al., 1995; Ren et al., 2017). Many diabetes and cardiovascular risk factors contribute to VED, such that hyperglycemia and IR in pre-DM might lead to endothelial dysfunction and worse prognosis by alterations in vasomotor tone and by the overproduction of inflammatory molecules and reactive oxygen species. And various cytokines produced in inflammation inhibit NO production and endothelial vasodilation by down-regulating the PI3K signaling pathway. The stabilization of vascular endothelial cell function and myocardial vasomotor capacity could be maintained by activating the Akt–eNOS signal axis and RAS–MAPK (ERK1/2)–ET-1 signaling pathway to mediate the production of vasodilator NO and vasoconstrictor ET-1, as well as maintaining the balance of NO and ET-1 (Kang et al., 2017). Akt1 was confirmed to be the key kinase of eNOS in the endothelial-specific Akt1-deleted adult mouse model, and eNOS function impairment reduced NO secretion and affected endothelial relaxation (Lee et al., 2018). In addition, it can regulate the insulin signaling pathway (GSK-3β, NF-κB, p38MAPK) to improve blood glucose homeostasis, IR, inflammation, and oxidative stress, thereby alleviating VED.
Insulin Signaling Pathway and Apoptosis
T2DM is characterized by pancreatic β cell dysfunction, including hypofunction and the decrease of β-cells (Zhang et al., 2011). Studies have shown that β-cell replication can compensate for peripheral IR in patients with T2DM. However, the decreased expression of insulin receptor and the dysfunction of insulin signal transduction accelerated β apoptosis, which leads to the failure of β-cell proliferation and cannot compensate for peripheral IR to aggravate T2DM (Folli et al., 2011). Additionally, cardiomyocyte apoptosis and autophagy also occur at the end stage of HF, resulting in the reduction of the number of mitochondria and cardiomyocytes, which further accelerates the development of HF (Xiaomei and Guoliang, 2014).
The PI3K/Akt signaling pathway and MAPK signaling pathway can regulate the apoptosis of β-cells and cardiomyocytes, which is beneficial to improve islet function and slow the development of HF. The PI3K/AKT signaling pathway could affect the activation of a variety of downstream effector molecules, such as Bcl-2/Bcl-XL–associated death promoter (Shultz et al., 2010), FoxO1 (Gardino and Yaffe, 2011; Battiprolu et al., 2012; Qi et al., 2013; Guo, 2014), Caspase-9 (Shultz et al., 2010), and nuclear factor-κB (NF-κB), to produce anti-apoptotic effect in cells and promote cell survival. Both in vitro and in vivo experiments have shown that the activation of Akt signaling pathway can slow the pancreatic islet apoptosis of rats, prolong the survival of pancreatic islets, and remarkably improve its function of synthesizing and secreting insulin (Richards et al., 2005; Wang et al., 2011). In addition, Shiojima and Walsh (2002) showed that the activation of Akt can inhibit apoptosis of endothelial cells and promote neovascularization. The activation of JNK and p38MAPK signaling pathways promotes apoptosis, while the activation of ERK signaling pathway inhibits apoptosis.
Insulin Signaling Pathway and Myocardial Contraction
A character of HF is increased leakage of Ca2+ from the sarcoplasmic reticulum (SR) via type 2 ryanodine receptor (RyR2) (Cho et al., 2016). The leaked Ca2+ in SR will be redistributed into the cytosol, thus increasing the cytoplasmic Ca2+ concentration, decreasing the electrochemical gradient inside and outside the cell, and impairing myocardial contractility (Cho et al., 2016). SR Ca2+ ATPase 2a (SERCA2a) is an important ATPase that can pump Ca2+ back into the SR of myocardial cells to mediate myocardial relaxation (Gorski et al., 2015). The decrease of myocardial SERCA level will severely impair systolic and diastolic functions. The activation of insulin signaling pathway can indirectly up-regulate the cellular calcium signaling pathway and regulate the intracellular Ca2+ concentration, thereby improving the myocardial contraction (Kim et al., 2008). Li et al. (2012) found that the mice with conditional cardiomyocyte-specific SERCA2 knockout would develop the end-stage HF with elevated [Na (+)] (i) and intracellular acidosis during 4 or 7 weeks. Studies have found that the activation of PI3K/Akt signaling pathway can enhance the function of L-type Ca2+ channels, up-regulate the activity of SERCA2a, regulate the calcium signaling pathway, increase the Ca2+ concentration, and maintain the normal heart beating (Aroor et al., 2012). A clinical trial (Jessup et al., 2011) also confirmed that the up-regulation of SERCA2a expression can improve myocardial contractility and is beneficial to HF treatment.
Insulin Signaling Pathways and Inflammatory Reaction
Both T2DM and HF are regarded as inflammatory diseases. In patients with T2DM complicated with HF, the inflammatory markers such as TNF-α, IL-6, and CRP are significantly higher than those in healthy people (Pickup et al., 2000; Leinonen et al., 2003). In HFrEF and HFpEF patients, elevated circulating levels of pro-inflammatory cytokines and cytokine receptors were associated with mortality (Kenny and Abel, 2019). Therefore, early anti-inflammatory therapy can effectively prevent T2DM complicated with HF. PI3K/Akt/NF-κB, p38MAPK, and other insulin signaling pathways can regulate the production of inflammatory factors and ROS, play anti-inflammatory and antioxidant roles, and improve myocardial inflammation. Related studies (Maier et al., 2012) have shown that the NF-κB expression in patients with HF is significantly higher than that in healthy people, and the activity of NF-κB increased with the aggravation of HF. Watanabe et al. (2013) found that the inhibitor of NF-κB kinase (IKK) significantly alleviated myocarditis in myocarditis rats, as well as alleviating heart injury.
It has a close relationship of pathological mechanisms between T2DM and HF and can interact among IR, energy metabolism disorders, inflammation, oxidative stress, and endothelial dysfunction. Taking the regulation of insulin signaling pathway as the starting point, it can regulate a variety of pathological mechanisms of T2DM and HF at the same time, so as to delay the development of T2DM complicated with HF and improve the condition.
Potential Drugs for the Treatment of T2DM Complicated With HF Based on Insulin Signaling Pathway
At present, marketed hypoglycemic drugs, lipid-lowering drugs, and anti-anginal drugs have found some drugs could regulate the insulin signaling pathway. These drugs can improve glucose and lipid metabolism, myocardial energy metabolism, and heart function of patients through the insulin signaling pathway.
Synthetic Drugs
Hypoglycemic Drugs
Biguanides (metformin), glucagon-like peptide-1 (GLP-1) receptor agonists, and dipeptidyl peptidase-IV (DPP-4) inhibitors in hypoglycemic drugs can treat T2DM complicated with HF through the insulin signaling pathway and have little cardiovascular safety risk. So, they can be considered potential therapeutic agents for T2DM complicated with HF in the future. Table 1 summarizes the clinical research of the effect of these hypoglycemic drugs on T2DM and HF.
Biguanides
Biguanides are classic oral hypoglycemic drugs, mainly including phenformin and metformin, but phenformin has been discontinued due to its severe lactic acidosis. Studies have confirmed that metformin is the first choice for the treatment of T2DM complicated with HF. Metformin could improve left ventricular remodeling and function by activating the AMP-activated protein kinase (AMPK) to increase glucose uptake and fatty acid oxidation, enhance insulin sensitivity, and reduce IR, thereby preventing the progression of HF (Nesti and Natali, 2017). Meanwhile, metformin can inhibit the activation of NF-κB by activating AMPK, thereby reducing the expression and release of inflammatory factors and adhesion molecules and thus reducing the incidence of inflammatory cardiovascular diseases (Kinsara and Ismail, 2018).
At present, there has been sufficient clinical evidence to prove that metformin can significantly reduce the glycosylated hemoglobin (HbA1c) level, mortality, all-cause rehospitalization rate, and cardiovascular rehospitalization rate in patients with DM complicated with HF (Stolarikova et al., 2018; Romero et al., 2013). Furthermore, metformin could also significantly improve the cardiac function and prognosis of patients. Especially in patients with end-stage HF, the one-year survival rate of the metformin group was significantly improved compared with that of the non-metformin group (Table 1) (Miaomiao and Shuang, 2018). Metformin therapy may reduce the high risk of cardiovascular events and the exacerbation of diabetic cardiomyopathy in pre-DM patients by reducing coronary endothelial dysfunction (Sardu et al., 2019).
In conclusion, although metformin can be used safely and effectively in T2DM patients complicated with HF, it should be noted that currently metformin is forbidden to be used in patients with acute or unstable HF. Patients with impaired liver and kidney function should be cautious about taking long-term use of metformin, as metformin can significantly limit liver and kidney clearance and may cause lactate poisoning (2018). But metformin can also be used in patients with stable congestive HF if patients’ renal function is normal (2018b). Patients with gastrointestinal reactions and other adverse reactions should be prevented and treated on time, as well as paying attention to regular examination of patients’ liver and kidney function.
Glucagon-Like Peptide-1 Receptor Agonists
In recent years, GLP-1 receptor agonists (GLP-1 RAs) have become the most attractive drug for diabetes. At present, exenatide and liraglutide have discovered the potential to treat T2DM complicated with HF. Other GLP-1 RAs have little cardiovascular research. Exenatide could up-regulate the expression of GLUT-4 and increase phosphorylation of AMPK and Akt to increase the glucose uptake of cardiomyocytes, thereby improving cardiac systolic function (Vyas et al., 2011). Exenatide can also enhance SR reuptake of Ca2+ by enhancing SERCA2a expression to improve calcium circulation (Harikumar et al., 2012). Liraglutide can mediate metabolism, vascular endothelial disorders, inflammation, endoplasmic reticulum stress, and other multiple pathways by inhibiting the activity of PPARγ, increasing the expression of AMPK (Koshibu et al., 2019), and inhibiting the JNK signaling pathway and caspase-12–mediated apoptosis signaling pathway (Li et al., 2019). In addition, serum-reduced GLP-1 levels were positively associated with IR (Ahmed et al., 2017). Studies have also confirmed that GLP-1 RAs (liraglutide) can reverse the increased phosphorylation of IRS1 serines, IKKα/β, and JNK in the model of IR and thus reverse the inhibited downstream mediators of IRS1 such as Akt, AS160, and GLUT4 translocation (Li et al., 2018). And liraglutide can also reduce the NF-KB activation, thus reducing inflammatory cytokines (Li et al., 2018). Importantly, GLP-1 may improve insulin sensitivity, glycemic control, and endothelial function, increase muscle microvascular perfusion, and stimulate angiogenesis, and these effects are retained in IR (Hagve et al., 2019; Love et al., 2020).
At present, GLP-1 agonists have been used for the treatment of chronic IR. They have beneficial cardiovascular effects for T2DM patients and are very suitable for T2DM patients with cardiomyopathy (Hagve et al., 2019; Love et al., 2020). Intervention of GLP-1 RA to CRTd therapy in diabetic patients significantly improved LVEF and the 6 min walking test, reduced the arrhythmic burden, and reduced hospital admissions for heart failure worsening by increasing the CRTd responder rate (Sardu et al., 2018). Clinical studies of exenatide and liraglutide show that GLP-1 RAs can reduce blood glucose as much as traditional hypoglycemic agents, and both can reduce hemoglobin A1c (Monami et al., 2014). Moreover, the incidence of hypoglycemia is much lower than that of sulfonylurea, insulin, and other drugs (Monami et al., 2014). However, it is unstable for these two drugs to improve left ventricular ejection fraction (LVEF) and other systolic function indicators in the clinical treatment of HF. In some clinic studies, it was found that exenatide and liraglutide not only failed to improve HF but even increased the heart rate (HR) and cardiovascular adverse events of patients (Table 1). Therefore, the cardiovascular safety of GLP-1 RA needs to be verified by more clinical studies.
Lipid-Lowering Drugs
Hyperlipidemia is closely related to atherosclerosis and is a risk factor for most cardiovascular diseases. Clinical studies have found that some lipid-lowering drugs can effectively treat HF and also have a certain effect on T2DM. Therefore, we discuss the commonly used lipid-lowering drugs based on the insulin signaling pathway—statins and fibrates.
Statins
Statins are the most important and widely used lipid-lowering drugs in the clinic. They are also widely used in atherosclerosis and are the most effective drugs for the prophylaxis and treatment of coronary heart disease. Most statins promote the production of NO in the myocardial vascular endothelium and improve vascular endothelial dysfunction in the IR state by up-regulating the expressions of Akt and P-Akt and PI3K/Akt/e NOS signaling pathway, thereby exerting vascular protection (Jie et al., 2014). Atorvastatin also can increase the activation of AMPK and inhibit FoxO1 to achieve the anti-myocardial hypertrophy effect (Park et al., 2018; Yu et al., 2018).
Statins have a remarkable clinical effect on HF (Table 2), which could significantly improve cardiac indicators (such as LVEF and HR), reduce the levels of myocardial inflammatory factors and risk of HF, and increase the risk of T2DM (Cederberg et al., 2015; Elmadbouh et al., 2015). Clinical trials have also found that statins cause the reduction of insulin sensitivity and IR and are associated with an increased risk of diabetes. Compared with placebo, statin therapy was associated with a 9% increased risk for incident diabetes (Sattar et al., 2010). Henna Cederberg (Cederberg et al., 2015) and Yaluri et al. (2016) found that both simvastatin and rosuvastatin can lead to insulin sensitivity and IR. Pravastatin, rosuvastatin, and other statins were also found to be related to the increase of DM (Culver et al., 2012; Ridker et al., 2012). Some studies also found that cardiovascular benefits of statins are far greater than those of DM. For instance, statins significantly reduced the primary major adverse cardiac endpoint in both high-risk DM participants and low-risk DM participants (Yandrapalli et al., 2019). The reason for this effect may be the difference in the dosage of statins. Considering the therapeutic effects of statins on HF and the potential risk of diabetes, it is clear that statins have a good prospect in the treatment of simple HF, which is worthy of further research. However, they are not suitable for the treatment of T2DM complicated with HF.
Fibrates
At present, fenofibrate (FF) is the only fibrate drug found to be able to treat HF by the insulin signaling pathway. It can enhance the oxidative metabolism of free fatty acids by up-regulating the expression of myocardial PPARα and FoxO1 (Ramakrishnan et al., 2016) to improve the energy metabolism of failed myocardium, inhibit ventricular remodeling (Li et al., 2015), and inhibit cardiomyocyte hypertrophy in multiple ways (Le et al., 2012). Meanwhile, FF down-regulates the activity of phosphokinase and phosphatase-1 in mitochondria, thus prolonging insulin action to improve its sensitivity and alleviate IR (Huang et al., 2017). Clinical studies have shown that FF has a good effect on HF patients (Table 2), which can significantly improve cardiac function indicators and myocardial systolic and diastolic functions. However, there is no clinical study of FF in the treatment of T2DM complicated with HF.
The bioavailability of FF is low, the patient’s compliance is poor, and there are large individual differences (Chang and Guangyu, 2021). In order to solve the problem of low bioavailability of FF, micro-powder technology, nanocrystallization technology, self-emulsifying technology, and slow and controlled release technology have been developed to improve the dissolution of FF dosage form. For example, the Fournier Laboratories developed the FF suprabioavailable tablet which can increase bioavailability by 25% via combining micronization technology with a new micro-coating process (Guichard et al., 2000). FF has shown good safety in long-term clinical application, with a low incidence of adverse reactions, most of which are mild. Considering its efficacy and safety, FF is expected to be a therapeutic agent for T2DM complicated with HF, but its clinical study in T2DM complicated with HF needs to be expanded.
Dipeptidyl Peptidase-IV Inhibitors
Dipeptidyl peptidase-IV (DPP-4) inhibitors are hypoglycemic drugs, commonly used in patients with T2DM. Some DPP-4 inhibitors have a protective effect on the heart. For example, sitagliptin can increase IRS-2 mRNA, inhibit PI3K-p85α protein expression, improve the IRS-2/PI3K signaling pathway, reduce IR, activate the AMPK signaling pathway, and inhibit the MAPK signaling pathway and NF-κB signaling pathway to reduce inflammatory response and cardiovascular diseases (Zheng et al., 2018). However, clinical studies (Table 1) show that DPP-4 inhibitors are generally not safe and have a potential cardiovascular risk profile. For instance, alogliptin has a higher risk of HF (Guo et al., 2017). Although sitagliptin and saxagliptin did not increase the risk of HF, they will increase the hospitalization rate of HF patients (Bowes et al., 2019). Furthermore, some studies illustrated that sitagliptin increases the risk of HF and the hospitalization rate of HF in DM patients (Weir et al., 2014). In 2013, the European Congress of Cardiology conducted a study, and the results showed that 20.1% (1,672) patients treated with saxagliptin and 19.6% (1,643) patients treated with placebo experienced cardiovascular adverse events. 3.5% (289) patients in the group treated with saxagliptin and 2.8% (228) patients in the group treated with placebo were hospitalized due to HF. The results suggested that saxagliptin did not increase the occurrence of ischemic cardiovascular events, but it inclined to increased hospitalization for HF in patients with the already present HF (Spinar and Smahelová, 2013). Based on the cardiovascular safety of DPP-4 inhibitors, sitagliptin, saxagliptin, and vildagliptin with low cardiovascular risk are recommended to be used as second-line or third-line additional treatment for T2DM combined with HF, which cannot increase the risk of HF, hypoglycemia, or death.
Diabetes is a common pathogenesis basis and risk factor of HF, and its complication rate is high clinically. Hypoglycemic drugs that treat HF through the insulin signaling pathway will be suitable for treating T2DM complicated with HF, which greatly reduces the incidence risk of HF and improves the therapeutic effect compared with simple HF treatment. Although hypoglycemic agents can regulate metabolism through the insulin signaling pathway, not all are suitable for the treatment of HF. Some hypoglycemic agents can cause adverse effects on the cardiovascular system, such as causing arrhythmia and HF. Furthermore, some drugs can treat HF, but there is a lack of clinical research on the treatment of T2DM combined with HF, and their safety needs to be investigated. Therefore, the hypoglycemic agents for HF need to be strictly screened.
Anti-Anginal—Trimetazidine
The therapeutic effect of anti-anginal drugs is worthy of affirmation for cardiovascular disease. Trimetazidine (TMZ), the first-line anti-angina drug, can treat HF through the insulin signaling pathway. Recent guidelines published by ESC 2016 have recommended TMZ in patients with HF with ongoing angina (Dalal and Mishra, 2017). TMZ can prevent reperfusion-mediated heart injury and dysfunction by activating insulin signaling pathways such as p38MAPK and Akt and regulating the expression of miRNA-21 in cardiomyocytes both in vivo and in vitro, thereby reducing cardiomyocyte apoptosis and enhancing anti-inflammatory and antioxidant capacity (Khan et al., 2010; Gao et al., 2011). Clinical studies of TMZ in HF treatment (Table 3) showed that it can indeed significantly improve the blood glucose and left ventricle function of patients and can significantly improve HbA1c, the blood glucose level, LVEF, the myocardial velocity of the left ventricle (LV) and right ventricle (RV), and other indicators. For T2DM patients with HF, the clinical effect of TMZ is more significant.
In clinics, TMZ ordinary tablets and sustained-release tablets were commonly used. Compared with ordinary tablets, sustained-release tablets can delay the release of TMZ, ensure a uniform and constant blood concentration, avoid peaking the problem of blood concentration, and make the anti-myocardial ischemia effect more durable, effectively covering the early morning dangerous period (Meirong et al., 2012). In addition, TMZ is well tolerated, with only a few cases of adverse reactions (such as nausea and vomiting). Considering the efficacy and safety, the clinical research of TMZ in HF is relatively mature, and it is recommended for the treatment of T2DM complicated with HF.
Among the above synthetic drugs, metformin and trimetazidine have good efficacy and high safety in treating T2DM complicated with HF, which can be extended to the clinical treatment of T2DM complicated with HF after improvement. Although GLP-1 agonists can be partially effective in T2DM complicated with HF, the efficacy is poor. Therefore, more effective derivatives or prodrugs need to be found. Metformin, trimetazidine, and fenofibrate have good efficacy in the treatment of HF, but there are defects such as low bioavailability and gastrointestinal adverse reactions. Therefore, they can be modified in the structure or dosage form to improve their bioavailability and reduce adverse reactions. For example, metformin has poor lipophilic property and low bioavailability and may cause gastrointestinal discomfort in some patients (Mingji, 2014). In order to improve the lipophilic property, bioavailability, and safety of metformin, it is necessary to modify the structure of metformin by introducing some lipophilic groups. Huttunen et al. (2013) synthesized several lipophilic sulfenamide prodrugs of metformin, and the oral absorption of these drugs was greatly improved compared with that of metformin. The bioavailability of prodrug 2a in rats is increased from 43 to 65%, and with increased oral absorption, the dose of metformin could be reduced, thereby reducing some of the adverse reactions (Huttunen et al., 2009). In addition, the absorbability of different dosage forms of FF in vivo is quite different. The bioavailability of common dosage form FF is 60%–90%, while the bioavailability of FF can be increased by 30% after micronization (Kai et al., 2010).
Natural Products
Natural drugs and their active components have the advantages of multiple targets, comprehensive curative effect, small side effects, high safety, etc., which can make up for the defects of synthetic drugs such as single action and more adverse reactions. At least 16 natural products have been found to be capable of treating DM or HF via the insulin signaling pathway, such as astragaloside IV (Shi-Guang et al., 2015; Zhiwei et al., 2017), tanshinone IIA (Hwang et al., 2013; Yuan et al., 2014), and salvianolic acid A (Chen et al., 2016; Xue-Li et al., 2019), but only sodium ferulate (SF), tetramethylpyrazine (TMP), and resveratrol; TMP and resveratrol can be used for both DM and HF. These three natural products have been clinically used in T2DM and HF, respectively (Table 4), and they have great potential for the treatment of T2DM complicated with HF.
Sodium Ferulate
SF, with the chemical name 3-methoxy-4-hydroxycinnamate sodium, is the sodium salt of ferulate acid, which is the main component of Angelica sinensis (Oliv.) Diels and Ligusticum chuanxiong Hort. Studies have confirmed that SF can induce mkP-1 protein expression to alleviate myocardial hypertrophy caused by angina pectoris by inhibiting the expression of PKC-β, RAF-1, and p-ERK1/2 protein and regulating MAPK/ERK and JNK pathways (Luo et al., 2019; Hu et al., 2017). For the clinical treatment of cardiovascular diseases, SF sodium chloride injection and SF glucose injection are mainly used in the adjuvant treatment of vascular diseases such as atherosclerosis, coronary heart disease, and vasculitis. Clinical data also demonstrate that SF works well in the treatment of T2DM and HF (Table 4), and SF, with low toxicity and good safety, is easy to be metabolized by the human body. However, SF aqueous solution is unstable to light and easily decomposes, which may cause adverse reactions due to its impurities (Yanhua et al., 2011). Therefore, it is suggested to take measures to avoid light to increase drug stability and reduce adverse drug reactions.
Tetramethylpyrazine
TMP is an alkaloid extracted from Ligusticum chuanxiong Hort. Studies have shown that TMP mainly activates the Akt and AMPK signaling pathways, up-regulates the expressions of GLUT-4 and eNOS to mediate the recovery of insulin signal transduction, reduces IR, increases the utilization of glucose by cardiomyocytes, and regulates apoptosis and autophagy, thereby improving cardiac function (Yanhua et al., 2011; Lv et al., 2012; Rai et al., 2019). At present, TMP phosphate tablets, TMP phosphate capsules, TMP phosphate injection (only for intramuscular injection), TMP hydrochloride injection, and other dosage forms are mainly used clinically. The combination of TMP injection and Astragalus membranaceus (Fisch.) had a better effect on HF and can significantly improve the symptoms of HF (Zhen and Faxiang, 2011). Salvia miltiorrhiza and ligustrazine injection can also effectively improve the left ventricular function and myocardial systolic function in the treatment of chronic congestive HF with high safety (Wenhhhe, 2016).
There are many TMP derivatives, including TMP ether derivatives, ester derivatives, piperazine derivatives, and stilbene derivatives (such as resveratrol), most of which show a certain cardiovascular protection (Zou et al., 2018). For example, TMP-amide derivatives have protective effects on injured vascular endothelial cells; TMP-benzyl alcohol ether derivatives mostly protect against ischemia–reperfusion injury and improve vasodilation (Zhen and Faxiang, 2011; Wenhhhe, 2016). Many studies have modified the structure of TMP and found that the pyrazine ring (parent nucleus) of TMP may be its pharmacodynamic group, and the side chain is its pharmacokinetic group, which mainly affects the absorption, distribution, and metabolism of TMP in vivo (Zou et al., 2018). On the basis of retaining the parent nucleus, the side chain of TMP can be modified to obtain cardiovascular drugs with lower toxicity, better efficacy, better water solubility, and higher bioavailability (Chen et al., 2011). Chen et al. (2011) synthesized a series of novel ligustrazinyloxy-cinnamic acid derivatives, several of which showed enhanced inhibitory effect on platelet aggregation compared with TMP.
Resveratrol
Resveratrol, a representative drug of ligustrazine-stilbene derivatives, has pharmacological effects on regulating the blood lipid level, preventing oxidation of low-density lipoprotein and anti-platelet agglutination, and reducing the incidence of sudden heart disease (Li et al., 2016). Studies confirmed that resveratrol mainly activates p38MAPK-β, Akt, NF-κB, and PI3K/Akt/mTOR signaling pathways and inhibits PI3K/Akt/FoxO3a and p38MAPK-α signaling pathways to regulate apoptosis and related inflammatory factors, thereby exerting myocardial protection (Das et al., 2006; Wu et al., 2017; Xiuling et al., 2019). Although resveratrol is highly absorbed when given orally, it has a very low bioavailability due to rapid metabolism of its glucuronide and sulfate conjugates (Walle et al., 2004). Therefore, its effective dosage is not accurate. When the dosage is low (5 μmol/L–20 μmol/L), it can protect the myocardium from ROS damage. It will promote oxidation and cause adverse reactions at higher dosages, such as liver and kidney toxicity (Zordoky et al., 2015). A study has shown that resveratrol has been shown to inhibit angiogenesis at 30 mg/kg/day or higher doses (Zordoky et al., 2015).
In summary, SF, TMP, and resveratrol have low side effects, high safety, and mature clinical application, so we can consider increasing their clinical verification as potential therapeutic drugs for T2DM complicated with HF in the future. Many in vitro studies have shown that promising active components of natural drugs have been abandoned due to extremely low or even no activity or high toxicity in vivo. The reason for low activity in vivo may be due to its unsatisfactory pharmacokinetic properties, such as strong first-pass effect, low bioavailability, short half-life, rapid metabolism, and difficulty in entering target organs through biofilm (Shu, 2005). The bioavailability in the active ingredients of natural drugs is low, and the clinical dosage needs to be increased. It will inevitably increase the clinical risk and the number/amount of patients taking the drugs and is not conducive to clinical safety and patient compliance. The same is true of sodium ferulate, ligustrazine, and resveratrol. At present, these defects can be improved through structural modification and dosage form change. For example, Peng et al. (Xiaoyun et al., 2005) prepared ligustrazine phosphate dropping pills, which significantly improved its bioavailability and inevitably reduced its clinical risk. Peñalva et al. (2018) wrapped resveratrol with casein nanoparticles and found that its oral bioavailability is 26.5%, which was 10 times higher than when the resveratrol was administered as an oral solution. The modification of dosage form for natural drugs can improve its bioavailability, reduce the number and dose of drugs taken by patients every day, and improve patients’ compliance. Meanwhile, this way can also reduce clinical risk and improve efficacy, which is suitable for long-term medication of T2DM complicated with HF and other cardiovascular diseases.
Other Drugs
Many new drugs currently in the research and development stage have the potential to treat cardiovascular diseases through the insulin signaling pathway. Poxel SA’s Imeglimin, a new T2DM drug, has successfully completed phase 3 development in Japan. Marianne et al. (Lachaux et al., 2020) found that Imeglimin immediately countered metabolic syndrome–related cardiac diastolic and vascular dysfunction in a rat model with human metabolic syndrome by reducing oxidative stress/increased NO bioavailability and improving myocardial perfusion. After 90-day treatment, myocardial and kidney structures in rats were improved after 90-day treatment (Lachaux et al., 2020). Esperion’s bempedoic acid, a new type of non-statin lipid-lowering drug, affects glucose and lipid metabolism and inhibits fatty acid and cholesterol synthesis by activating AMPK and has completed a phase 3 clinical trial. Masson et al. (2020) found that bempedoic acid significantly can reduce the levels of all atherogenic lipid markers, including LDL-C, non-HDL-C, and apolipoprotein B, and prevent atherosclerosis. Therefore, we can explore potential drugs to reduce cardiovascular risk in new drugs such as hypoglycemic drugs and lipid-lowering drugs, which also provides direction and convenience for the research and development of drugs based on the insulin signaling pathway to treat T2DM complicated with HF.
Integration Analysis of Drug, Target, and Metabolic Pathway
After summarizing the action pathways, targets, and metabolic pathways of synthetic drugs, natural drugs, and a few derivatives mentioned above, it was found that most drugs regulate glucose metabolism, fatty acid oxidation, vascular endothelial function, and apoptosis mainly by acting on several signaling pathways or targets such as PI3K/Akt, AMPK, eNOS, NF-κB, and p38MAPK. Cascading the main signaling pathways (Figure 3), it is found that the activation of Akt is the core that affects other pathways and gene expression. Through the action of Akt on AMPK, MAPK, eNOS, NF-κB, and other pathways and targets, it can affect glycolysis, cell growth, protein synthesis, and other metabolic pathways. There is a direct or indirect relationship between each target and pathway, and they are inseparable, so we can consider the combination of drugs to exert the maximum efficacy and appropriately reduce the adverse reactions. For example, Pan et al. (Jing et al., 2019) used metformin combined with sitagliptin to treat T2DM patients with HF. After three-month treatment, they found that the combined treatment had significant therapeutic effect, which could improve cardiac function and effectively reduce blood glucose and the level of related inflammatory factors (Jing et al., 2019). Moreover, the prognosis was good, and the clinical effect was better than that of metformin alone. Feng et al. (Yanling, 2021) used atorvastatin combined with metformin to treat patients with diabetic cardiomyopathy, and the results showed that atorvastatin combined with metformin treatment could reduce the blood glucose level, improve heart function, and regulate blood lipids. In addition, many targets and pathways involved in Figure 3 can be used as new directions for the future development of T2DM complicated with HF drugs, such as the continued development of PPAR agonists and GLP-1 agonists.
(Figure 3 involves PI3K/Akt, AMPK, VEGF, NF-κB, MAPK, and mTOR signaling pathways. It can be seen that the core of insulin signaling pathways is the activation of Akt. By activating the PI3K/Akt signaling pathway, it acts on pathways and targets such as AMPK, MAPK, eNOS, and NF-κB and affects metabolic pathways such as glycolysis, cell growth, and protein synthesis to regulate heart function.)
Conclusion and Prospects
It is certain that the insulin signaling pathway is a promising therapeutic direction for T2DM patients with HF. It can improve myocardial energy metabolism and IR and regulate cell apoptosis, inflammatory response, vascular endothelial function, and other ways to improve T2DM, thus protecting the heart and improving heart function. Different from the current combination therapy for T2DM complicated with HF, the regulation of insulin signaling pathway can provide targeted treatment for the special combination, improve the common physiological and pathological characteristics of the two diseases, delay the development of T2DM complicated with HF, and ensure a good prognosis. The synthetic drugs in this article are mostly listed drugs and have basically mature drug research. They can regulate blood sugar and blood lipids while treating HF. Compared with the previous drugs, natural drugs and their active ingredients not only can improve patient’s cardiac function and prognosis but also are safe and suitable for long-term treatment of HF patients.
Notably, it has certain defects for drug therapy based on the insulin signaling pathway. Regulating the insulin signaling pathway to treat T2DM complicated with HF mainly aims to improve myocardial energy metabolism disorder, so as to ensure adequate heart energy and recover heart function, which is a chronic treatment. In different types and stages of HF, myocardial energy metabolism presents different states. The treatment of HF by regulating the insulin signaling pathway should be adjusted according to the disease progress. Acute HF is a severe stress state with severe hyperglycemia (Hongji, 2018). Metabolic therapy based on insulin signaling is not applicable to T2DM complicated with acute HF, which is in critical condition and needs urgent treatment. This therapy is suitable for T2DM complicated with chronic HF or T2DM complicated with acute HF in the stable phase. Chronic HF can be divided into four stages, including A, B, C, and D. Drug therapy of insulin signaling pathway can be considered for A, B, C stages, especially in A and B stages. The reason is that stage A is mainly for the treatment of HF risk factors, and stage B is mainly to prevent and improve ventricular remodeling and prevent aggravation of heart failure symptoms (Osuna et al., 2018). In these two stages, the use of insulin signaling pathway drugs to treat the energy metabolism disorder and slow down the development of IR can effectively prevent the development of HF to the next stage. In the C and D stages, the disease is further aggravated, especially in the D stage. Most powerful drugs in conjunction with the application of mechanical assist devices, heart transplantation, ultrafiltration, and other treatment methods were used in these two stages, so that drug therapy based on the insulin signaling pathway is not suitable in two stages. Therefore, it is suitable to use insulin signaling pathway drug therapy in the early term and mid-term of T2DM complicated with HF, which can effectively control blood sugar and prevent the further development of HF.
The insulin signaling pathway has not yet been considered in the treatment of HF because it still has several problems that need to be clarified. First is the effect of excessive activation of insulin signaling pathway on HF. Studies have shown that over-expression of Akt can cause pathological myocardial hypertrophy (Dorn and Force, 2005). Whether the over-expression of other genes can harm the myocardium has not been clearly investigated. But as a potential risk factor, excessive activation of insulin signaling pathway may worsen heart failure. Second is the regulation of systemic insulin signaling pathway. While drugs regulate the myocardial insulin signaling pathway and improve myocardial IR, the systemic insulin signaling pathway is also affected. The disorder of systemic metabolic environment may trigger other diseases or affect the prognosis of HF. Third, although the drugs mentioned in this review can act through the insulin signaling pathway, most of them lack clinical trials in T2DM complicated with HF and cannot directly prove that they can treat HF through the insulin signaling pathway. In particular, clinical data on natural drugs are very scarce, with only a small amount in China. Moreover, due to the poor absorption and low bioavailability of natural drugs, further structural modifications are needed to obtain drugs more suitable for clinical use. Fourth, patients with T2DM complicated with HF need long-term medication and long-term treatment. Most of the drugs mentioned in this article have a short medication time in clinical trials, so the long-term effects and safety of these drugs need further research and demonstration. In the future, medical treatment of HF will be promoted along the direction of insulin signaling pathway. With more perfect and optimized research results and the advent of new drugs, the pattern of drug treatment dominated by the “Golden Triangle” in the past will gradually change, and the treatment of HF will usher in a more diversified landscape.
Author Contributions
DZ proposed the concept of the review. HY collected and analyzed documentation, prepared tables, and drew the figures. YH drafted the manuscript. HY, YH, CZ, FW, MY, JL, RX, and DZ critically revised the manuscript. All authors contributed to discussions and gave final approval of the submitted manuscript.
Funding
This work was supported by grants from the National Natural Science Foundation of China (81973493), Science and Technology Research Special Project of Sichuan Administration of Traditional Chinese Medicine (2021MS016), and Open Fund Project of State Key Laboratory of Innovative Drug and Efficient Energy-Saving Pharmaceutical Equipment, Jiangxi University of Chinese Medicine (GZSYS202005).
Conflict of Interest
The authors declare that the research was conducted in the absence of any commercial or financial relationships that could be construed as a potential conflict of interest.
Publisher’s Note
All claims expressed in this article are solely those of the authors and do not necessarily represent those of their affiliated organizations, or those of the publisher, the editors, and the reviewers. Any product that may be evaluated in this article, or claim that may be made by its manufacturer, is not guaranteed or endorsed by the publisher.
References
Ahmed, R. H., Huri, H. Z., Muniandy, S., Al-Hamodi, Z., Al-Absi, B., Alsalahi, A., et al. (2017). Altered Circulating Concentrations of Active Glucagon-like Peptide (GLP-1) and Dipeptidyl Peptidase 4 (DPP4) in Obese Subjects and Their Association with Insulin Resistance. Clin. Biochem. 50 (13-14), 746–749. doi:10.1016/j.clinbiochem.2017.03.008
Arabacilar, P., and Marber, M. (2015). The Case for Inhibiting P38 Mitogen-Activated Protein Kinase in Heart Failure. Front. Pharmacol. 6, 102. doi:10.3389/fphar.2015.00102
Aroor, A. R., Mandavia, C. H., and Sowers, J. R. (2012). Insulin Resistance and Heart Failure: Molecular Mechanisms. Heart Fail. Clin. 8 (4), 609–617. doi:10.1016/j.hfc.2012.06.005
Arturi, F., Succurro, E., Miceli, S., Cloro, C., Ruffo, M., Maio, R., et al. (2017). Liraglutide Improves Cardiac Function in Patients with Type 2 Diabetes and Chronic Heart Failure. Endocrine 57 (3), 464–473. doi:10.1007/s12020-016-1166-4
Battiprolu, P. K., Hojayev, B., Jiang, N., Wang, Z. V., Luo, X., Iglewski, M., et al. (2012). Metabolic Stress-Induced Activation of FoxO1 Triggers Diabetic Cardiomyopathy in Mice. J. Clin. Invest. 122 (3), 1109–1118. doi:10.1172/JCI60329
Bell, H., and David, S. (2004). Heart Failure: A Serious and Common Comorbidity of Diabetes. Clin. Diabetes 19 (7), 39. doi:10.2337/diaclin.22.2.61
Bin, Z. (2008). JNK Mediated the Apoptosis of Islet β Cells Induced by High Glucose and Palmitic Acid by Inhibiting Insulin Signaling Pathway. Shanghai: Shanghai Jiao Tong University.
Bowes, C. D., Lien, L. F., and Butler, J. (2019). Assessment of Heart Failure in Diabetes Cardiovascular Outcomes Trials: Is what We Are Currently Capturing Adequate. Curr. Diab Rep. 19 (7), 39. doi:10.1007/s11892-019-1154-1
Cederberg, H., Stančáková, A., Yaluri, N., Modi, S., Kuusisto, J., and Laakso, M. (2015). Increased Risk of Diabetes with Statin Treatment Is Associated with Impaired Insulin Sensitivity and Insulin Secretion: a 6 Year Follow-Up Study of the METSIM Cohort. Diabetologia 58 (5), 1109–1117. doi:10.1007/s00125-015-3528-5
Chang, S., and Guangyu, W. (2021). Efficacy Analysis of Metformin Combined with Fenofibrate in the Treatment of Type 2 Diabetes. Chin. J. Mod. Drug Appl. 15 (07), 198. doi:10.3969/j.issn.1671-2560.2005.10.008
Chatterjee, S., Khunti, K., and Davies, M. J. (2017). Type 2 Diabetes. Lancet 389 (10085), 2239–2251. doi:10.1016/S0140-6736(17)30058-2
Chen, H., Li, G., Zhan, P., and Liu, X. (2011). Ligustrazine Derivatives. Part 5: Design, Synthesis and Biological Evaluation of Novel Ligustrazinyloxy-Cinnamic Acid Derivatives as Potent Cardiovascular Agents. Eur. J. Med. Chem. 46 (11), 5609–5615. doi:10.1016/j.ejmech.2011.09.030
Chen, Q., Xu, T., Li, D., Pan, D., Wu, P., Luo, Y., et al. (2016). JNK/PI3K/Akt Signaling Pathway Is Involved in Myocardial Ischemia/reperfusion Injury in Diabetic Rats: Effects of Salvianolic Acid A Intervention. Am. J. Transl. Res. 8 (6), 2534.
Cho, G. W., Altamirano, F., and Hill, J. A. (2016). Chronic Heart Failure: Ca(2+), Catabolism, and Catastrophic Cell Death. Biochim. Biophys. Acta 1862 (4), 763–777. doi:10.1016/j.bbadis.2016.01.011
Chokshi, A., Drosatos, K., Cheema, F. H., Ji, R., Khawaja, T., Yu, S., et al. (2012). Ventricular Assist Device Implantation Corrects Myocardial Lipotoxicity, Reverses Insulin Resistance, and Normalizes Cardiac Metabolism in Patients with Advanced Heart Failure. Circulation 125 (23), 2844–2853. doi:10.1161/CIRCULATIONAHA.111.060889
Cook, S. A., Sugden, P. H., and Clerk, A. (1999). Activation of C-Jun N-Terminal Kinases and P38-Mitogen-Activated Protein Kinases in Human Heart Failure Secondary to Ischaemic Heart Disease. J. Mol. Cel. Cardiol. 31 (8), 1429–1434. doi:10.1006/jmcc.1999.0979
Culver, A. L., Ockene, I. S., Balasubramanian, R., Olendzki, B. C., Sepavich, D. M., Wactawski-Wenhhhde, J., et al. (2012). Statin Use and Risk of Diabetes Mellitus in Postmenopausal Women in the Women's Health Initiative. Arch. Intern. Med. 172 (2), 144–152. doi:10.1001/archinternmed.2011.625
Dalal, J. J., and Mishra, S. (2017). Modulation of Myocardial Energetics: An Important Category of Agents in the Multimodal Treatment of Coronary Artery Disease and Heart Failure. Indian Heart J. 69 (3), 393–401. doi:10.1016/j.ihj.2017.04.001
Das, S., Fraga, C. G., and Das, D. K. (2006). Cardioprotective Effect of Resveratrol via HO-1 Expression Involves P38 Map Kinase and PI-3-Kinase Signaling, but Does Not Involve NFkappaB. Free Radic. Res. 40 (10), 1066–1075. doi:10.1080/10715760600833085
David, M., and Droogan, C. (2013). Role Of Metformin In Early Stages Of Heart Failure: Rome Hf Study. J. Am. Coll. Cardiol. doi:10.1016/s0735-1097(13)60704-4
Dorn, G. N., and Force, T. (2005). Protein Kinase Cascades in the Regulation of Cardiac Hypertrophy. J. Clin. Invest. 115 (3), 527–537. doi:10.1172/JCI24178
Elmadbouh, I., Mansour, M., Nabeh, M., Faried, W., Abdelsabour, A., and Omar, A. (2015). Atorvastatin Improves Cardiac Function and Remodeling in Chronic Non-ischemic Heart Failure: A Clinical and Pre-clinical Study. Egypt. Heart J. 67 (4), 289–298. doi:10.1016/j.ehj.2014.11.003
Falskov, B., Hermann, T. S., Rask-Madsen, C., Major-Pedersen, A., Christiansen, B., Raunsø, J., et al. (2011). The Effect of Chronic Heart Failure and Type 2 Diabetes on Insulin-Stimulated Endothelial Function Is Similar and Additive. Vasc. Health Risk Manag. 7, 771–776. doi:10.2147/VHRM.S25724
Fazakerley, D. J., Minard, A. Y., Krycer, J. R., Thomas, K. C., Stöckli, J., Harney, D. J., et al. (2018). Mitochondrial Oxidative Stress Causes Insulin Resistance without Disrupting Oxidative Phosphorylation. J. Biol. Chem. 293 (19), 7315–7328. doi:10.1074/jbc.RA117.001254
Fengxiang, G. (2014). Clinical Observation on Therapeutic Effect of Sodium Ferulate Injection on 60 Cases of Diabetic Cardiomyopathy. Guide China Med. 12 (33), 220. doi:10.15912/j.cnki.gocm.2014.33.173
Folli, F., Okada, T., Perego, C., Gunton, J., Liew, C. W., Akiyama, M., et al. (2011). Altered Insulin Receptor Signalling and β-cell Cycle Dynamics in Type 2 Diabetes Mellitus. PLoS One 6 (11), e28050. doi:10.1371/journal.pone.0028050
Fudim, M., White, J., Pagidipati, N. J., Lokhnygina, Y., and Mentz, R. J. (2019). Effect of Once-Weekly Exenatide in Patients with Type 2 Diabetes with and without Heart Failure and Heart Failure-Related Outcomes: Insights from the EXSCEL Trial. Circulation 140 (20), 1613-1622. doi:10.1161/CIRCULATIONAHA.119.041659
Gao, D., Ning, N., Niu, X., Hao, G., and Meng, Z. (2011). Trimetazidine: a Meta-Analysis of Randomised Controlled Trials in Heart Failure. Heart 97 (4), 278–286. doi:10.1136/hrt.2010.208751
Gardino, A. K., and Yaffe, M. B. (2011). 14-3-3 Proteins as Signaling Integration Points for Cell Cycle Control and Apoptosis. Semin. Cel Dev. Biol. 22 (7), 688–695. doi:10.1016/j.semcdb.2011.09.008
Goedeke, L., Perry, R. J., and Shulman, G. I. (2019). Emerging Pharmacological Targets for the Treatment of Nonalcoholic Fatty Liver Disease, Insulin Resistance, and Type 2 Diabetes. Annu. Rev. Pharmacol. Toxicol. 59, 65–87. doi:10.1146/annurev-pharmtox-010716-104727
Gorski, P. A., Ceholski, D. K., and Hajjar, R. J. (2015). Altered Myocardial Calcium Cycling and Energetics in Heart Failure-Aa Rational Approach for Disease Treatment. Cell Metab 21 (2), 183–194. doi:10.1016/j.cmet.2015.01.005
Guichard, J. P., Blouquin, P., and Qing, Y. (2000). A New Formulation of Fenofibrate: Suprabioavailable Tablets. Curr. Med. Res. Opin. 16 (2), 134–138. doi:10.1185/0300799009117017
Gunes, Y., Guntekin, U., Tuncer, M., and Sahin, M. (2009). Improved Left and Right Ventricular Functions with Trimetazidine in Patients with Heart Failure: a Tissue Doppler Study. Heart Vessels 24 (4), 277–282. doi:10.1007/s00380-008-1118-x
Guo, S. (2014). Insulin Signaling, Resistance, and the Metabolic Syndrome: Insights from Mouse Models into Disease Mechanisms. J. Endocrinol. 220 (2), T1–T23. doi:10.1530/JOE-13-0327
Guo, W. Q., Li, L., Su, Q., Dai, W. R., and Ye, Z. L. (2017). Effect of Dipeptidyl Peptidase-4 Inhibitors on Heart Failure: A Network Meta-Analysis. Value Health 20 (10), 1427–1430. doi:10.1016/j.jval.2017.04.010
Gürgün, C., Ildizli, M., Yavuzgil, O., Sin, A., Apaydin, A., Cinar, C., et al. (2008). The Effects of Short Term Statin Treatment on Left Ventricular Function and Inflammatory Markers in Patients with Chronic Heart Failure. Int. J. Cardiol. 123 (2), 102–107. doi:10.1016/j.ijcard.2006.11.152
Hagve, M., Gjessing, P. F., Hole, M. J., Jansen, K. M., Fuskevåg, O. M., Mollnes, T. E., et al. (2019). Perioperative Infusion of Glucagon-like Peptide-1 Prevents Insulin Resistance after Surgical Trauma in Female Pigs. Endocrinology 160 (12), 2892–2902. doi:10.1210/en.2019-00374
Harikumar, K. G., Wootten, D., Pinon, D. I., Koole, C., Ball, A. M., Furness, S. G., et al. (2012). Glucagon-like Peptide-1 Receptor Dimerization Differentially Regulates Agonist Signaling but Does Not Affect Small Molecule Allostery. Proc. Natl. Acad. Sci. U S A. 109 (45), 18607–18612. doi:10.1073/pnas.1205227109
Hongji, C. (2018). Correlation between Admission Glucose Level and Prognosis in Patients with Acute Heart Failure. Guangzhou: Southern Medical University.
Hoseini, A., Namazi, G., Farrokhian, A., Reiner, Ž., Aghadavod, E., Bahmani, F., et al. (2019). The Effects of Resveratrol on Metabolic Status in Patients with Type 2 Diabetes Mellitus and Coronary Heart Disease. Food Funct. 10 (9), 6042–6051. doi:10.1039/c9fo01075k
Hu, B., Song, J. T., Ji, X. F., Liu, Z. Q., Cong, M. L., and Liu, D. X. (2017). Sodium Ferulate Protects against Angiotensin II-Induced Cardiac Hypertrophy in Mice by Regulating the MAPK/ERK and JNK Pathways. Biomed. Res. Int. 2017, 3754942. doi:10.1155/2017/3754942
Huang, W. P., Yin, W. H., Chen, J. S., Huang, P. H., and Lin, S. J. (2020). Fenofibrate Reverses Dysfunction of EPCs Caused by Chronic Heart Failure. J. Cardiovasc. Transl. 13 (2). doi:10.1007/s12265-019-09889-y
Huang, X., Wang, Y., Zhang, Z., Wang, Y., Chen, X., Wang, Y., et al. (2017). Ophiopogonin D and EETs Ameliorate Ang II-Induced Inflammatory Responses via Activating PPARα in HUVECs. Biochem. Biophys. Res. Commun. 490 (2), 123–133. doi:10.1016/j.bbrc.2017.06.007
Huttunen, K. M., Leppänen, J., Laine, K., Vepsäläinen, J., and Rautio, J. (2013). Convenient Microwave-Assisted Synthesis of Lipophilic Sulfenamide Prodrugs of Metformin. Eur. J. Pharm. Sci. 49 (4), 624–628. doi:10.1016/j.ejps.2013.05.023
Huttunen, K. M., Mannila, A., Laine, K., Kemppainen, E., Leppänen, J., Vepsäläinen, J., et al. (2009). The First Bioreversible Prodrug of Metformin with Improved Lipophilicity and Enhanced Intestinal Absorption. J. Med. Chem. 52 (14), 4142–4148. doi:10.1021/jm900274q
Hwang, S. L., Yang, J. H., Jeong, Y. T., Kim, Y. D., Li, X., Lu, Y., et al. (2013). Tanshinone IIA Improves Endoplasmic Reticulum Stress-Induced Insulin Resistance through AMP-Activated Protein Kinase. Biochem. Biophysical Res. Commun. 430 (4), 1246–1252. doi:10.1016/j.bbrc.2012.12.066
Investigators, G. H. (2008). Effect of Rosuvastatin in Patients with Chronic Heart Failure (The GISSI-HF Trial): a Randomised, Double-Blind, Placebo-Controlled Trial. Lancet 372 (9645), 1231–1239. doi:10.1016/s0140-6736(08)61240-4
Jessup, M., Greenberg, B., Mancini, D., Cappola, T., Pauly, D. F., Jaski, B., et al. (2011). Calcium Upregulation by Percutaneous Administration of Gene Therapy in Cardiac Disease (CUPID): a Phase 2 Trial of Intracoronary Gene Therapy of Sarcoplasmic Reticulum Ca2+-ATPase in Patients with Advanced Heart Failure. Circulation 124 (3), 304–313. doi:10.1161/CIRCULATIONAHA.111.022889
Jie, L., Qun, L., and Jian, K. (2014). GW25-e1124 Atorvastatin Improve Endothelial Function of Insulin Resistance Endothelial Cells Directly. J. Am. Coll. Cardiol. 64 (16), C89. doi:10.1016/j.jacc.2014.06.417
Jiménez-Osorio, A. S., Monroy, A., and Alavez, S. (2016). Curcumin and Insulin Resistance-Molecular Targets and Clinical Evidences. Biofactors 42 (6), 561–580. doi:10.1002/biof.1302
Jin, L., Junhong, W., and Nannan, F. (2014). Clinical Observation on the Efficacy and Safety of Sitagliptin Phosphate in Patients with Type 2 Diabetes Mellitus Combined with Heart Failure. J Chinese Physician 16 (12), 1694. doi:10.3760/cma.j.issn.1008-1372.2014.12.035
Jing, P., Jing, H., and Yu, G. (2019). Efficacy of Metformin Combined with Sitagliptin in the Treatment of Type 2 Diabetes Combined with Heart Failure. J. Prev. Med. Chin. People's Liberation Arm. 37 (10), 162. doi:10.13704/j.cnki.jyyx.2019.10.085
Jorsal, A., Kistorp, C., Holmager, P., Tougaard, R. S., Nielsen, R., Hänselmann, A., et al. (2017). Effect of Liraglutide, a Glucagon-like Peptide-1 Analogue, on Left Ventricular Function in Stable Chronic Heart Failure Patients with and without Diabetes (LIVE)-a Multicentre, Double-Blind, Randomised, Placebo-Controlled Trial. Eur. J. Heart Fail. 19 (1), 69–77. doi:10.1002/ejhf.657
Kai, C., Shen, X., Yao, T., and Haibo, H. (2010). Analysis of Adverse Reaction Induced by Fenofibrate. West China Med. J. 25 (08), 1536.
Kang, K. W., Choi, B. G., and Rha, S. W. (2018). Impact of Insulin Resistance on Acetylcholine-Induced Coronary Artery Spasm in Non-diabetic Patients. Yonsei Med. J. 59 (9), 1057–1063. doi:10.3349/ymj.2018.59.9.1057
Kang, Y. M., Kim, F., and Lee, W. J. (2017). Role of NO/VASP Signaling Pathway against Obesity-Related Inflammation and Insulin Resistance. Diabetes Metab. J. 41 (2), 89–95. doi:10.4093/dmj.2017.41.2.89
Katircibasi, M. T., Canatar, T., Kocum, H. T., Erol, T., Tekin, G., Demircan, S., et al. (2005). Decreased Heart Rate Recovery in Patients with Heart Failure: Effect of Fluvastatin Therapy. Int. Heart J. 46 (5), 845–854. doi:10.1536/ihj.46.845
Kenny, H. C., and Abel, E. D. (2019). Heart Failure in Type 2 Diabetes Mellitus. Circ. Res. 124 (1), 121–141. doi:10.1161/CIRCRESAHA.118.311371
Khan, M., Meduru, S., Mostafa, M., Khan, S., Hideg, K., and Kuppusamy, P. (2010). Trimetazidine, Administered at the Onset of Reperfusion, Ameliorates Myocardial Dysfunction and Injury by Activation of P38 Mitogen-Activated Protein Kinase and Akt Signaling. J. Pharmacol. Exp. Ther. 333 (2), 421–429. doi:10.1124/jpet.109.165175
Kim, S. J., Abdellatif, M., Koul, S., and Crystal, G. J. (2008). Chronic Treatment with Insulin-like Growth Factor I Enhances Myocyte Contraction by Upregulation of Akt-SERCA2a Signaling Pathway. Am. J. Physiol. Heart Circ. Physiol. 295 (1), H130–H135. doi:10.1152/ajpheart.00298.2008
Kinsara, A. J., and Ismail, Y. M. (2018). Metformin in Heart Failure Patients. Indian Heart J. 70 (1), 175–176. doi:10.1016/j.ihj.2017.05.009
Kjekshus, J., Pedersen, T. R., Olsson, A. G., Faergeman, O., and Pyörälä, K. (1997). The Effects of Simvastatin on the Incidence of Heart Failure in Patients with Coronary Heart Disease. J. Card. Fail. 3 (4), 249–254. doi:10.1016/s1071-9164(97)90022-1
Koshibu, M., Mori, Y., Saito, T., Kushima, H., Hiromura, M., Terasaki, M., et al. (2019). Antiatherogenic Effects of Liraglutide in Hyperglycemic Apolipoprotein E-Null Mice via AMP-Activated Protein Kinase-independent Mechanisms. Am. J. Physiol. Endocrinol. Metab. 316 (5), E895–E907. doi:10.1152/ajpendo.00511.2018
Kyhl, K., Lønborg, J., Vejlstrup, N., Kelbæk, H., Helqvist, S., Holmvang, L., et al. (2016). A Post Hoc Analysis of Long-Term Prognosis after Exenatide Treatment in Patients with ST-Segment Elevation Myocardial Infarction. EuroIntervention 12 (4), 449–455. doi:10.4244/EIJV12I4A78
Lachaux, M., Soulié, M., Hamzaoui, M., Bailly, A., and Mulder, P. (2020). Short-and Long‐term Administration of Imeglimin Counters Cardiorenal Dysfunction in a Rat Model of Metabolic Syndrome. Endocrinol. Diabetes Metab.
Le, K., Li, R., Xu, S., Wu, X., Huang, H., Bao, Y., et al. (2012). PPARα Activation Inhibits Endothelin-1-Induced Cardiomyocyte Hypertrophy by Prevention of NFATc4 Binding to GATA-4. Arch. Biochem. Biophys. 518 (1), 71–78. doi:10.1016/j.abb.2011.11.024
Lee, M. Y., Gamez-Mendez, A., Zhang, J., Zhuang, Z., Vinyard, D. J., Kraehling, J., et al. (2018). Endothelial Cell Autonomous Role of Akt1: Regulation of Vascular Tone and Ischemia-Induced Arteriogenesis. Arterioscler Thromb. Vasc. Biol. 38 (4), 870–879. doi:10.1161/ATVBAHA.118.310748
Leinonen, E., Hurt-Camejo, E., Wiklund, O., Hultén, L. M., Hiukka, A., and Taskinen, M. R. (2003). Insulin Resistance and Adiposity Correlate with Acute-phase Reaction and Soluble Cell Adhesion Molecules in Type 2 Diabetes. Atherosclerosis 166 (2), 387–394. doi:10.1016/s0021-9150(02)00371-4
Li, L., Louch, W. E., Niederer, S. A., Aronsen, J. M., Christensen, G., Sejersted, O. M., et al. (2012). Sodium Accumulation in SERCA Knockout-Induced Heart Failure. Biophys. J. 102 (9), 2039–2048. doi:10.1016/j.bpj.2012.03.045
Li, P., Luo, S., Pan, C., and Cheng, X. (2015). Modulation of Fatty Acid Metabolism Is Involved in the Alleviation of Isoproterenol-Induced Rat Heart Failure by Fenofibrate. Mol. Med. Rep. 12 (6), 7899–7906. doi:10.3892/mmr.2015.4466
Li, Q., Xue, A. Y., Li, Z. L., and Yin, Z. (2019). Liraglutide Promotes Apoptosis of HepG2 Cells by Activating JNK Signaling Pathway. Eur. Rev. Med. Pharmacol. Sci. 23 (8), 3520–3526. doi:10.26355/eurrev_201904_17719
Li, Y., Guoda, L., Lingyan, F., and Yanyu, L. (2016). Advances in Cardiovascular Protective Mechanisms and Clinical Studies of Resveratrol. Chin. J. Cardiovasc. Med. 21 (01), 76. doi:10.3969/j.issn.1007-5410.2016.01.020
Li, Z., Zhu, Y., Li, C., Tang, Y., Jiang, Z., Yang, M., et al. (2018). Liraglutide Ameliorates Palmitate-Induced Insulin Resistance through Inhibiting the IRS-1 Serine Phosphorylation in Mouse Skeletal Muscle Cells. J. Endocrinol. Invest. 41 (9), 1097–1102. doi:10.1007/s40618-018-0836-x
Liang, B., and Gu, N. (2020). Liraglutide in the Treatment of Heart Failure: Insight from FIGHT and LIVE. Cardiovasc. Diabetol. 19 (1), 106. doi:10.1186/s12933-020-01088-3
Lopaschuk, G. D., Ussher, J. R., Folmes, C. D., Jaswal, J. S., and Stanley, W. C. (2010). Myocardial Fatty Acid Metabolism in Health and Disease. Physiol. Rev. 90 (1), 207–258. doi:10.1152/physrev.00015.2009
Love, K. M., Liu, J., Regensteiner, J. G., Reusch, J., and Liu, Z. (2020). GLP-1 and Insulin Regulation of Skeletal and Cardiac Muscle Microvascular Perfusion in Type 2 Diabetes. J. Diabetes 12 (7), 488–498. doi:10.1111/1753-0407.13045
Luo, M., Chen, P. P., Yang, L., Wang, P., Lu, Y. L., Shi, F. G., et al. (2019). Sodium Ferulate Inhibits Myocardial Hypertrophy Induced by Abdominal Coarctation in Rats: Involvement of Cardiac PKC and MAPK Signaling Pathways. Biomed. Pharmacother. 112, 108735. doi:10.1016/j.biopha.2019.108735
Lv, L., Jiang, S. S., Xu, J., Gong, J. B., and Cheng, Y. (2012). Protective Effect of Ligustrazine against Myocardial Ischaemia Reperfusion in Rats: the Role of Endothelial Nitric Oxide Synthase. Clin. Exp. Pharmacol. Physiol. 39 (1), 20–27. doi:10.1111/j.1440-1681.2011.05628.x
Maier, H. J., Schips, T. G., Wietelmann, A., Krüger, M., Brunner, C., Sauter, M., et al. (2012). Cardiomyocyte-specific IκB Kinase (IKK)/NF-κB Activation Induces Reversible Inflammatory Cardiomyopathy and Heart Failure. Proc. Natl. Acad. Sci. U S A. 109 (29), 11794–11799. doi:10.1073/pnas.1116584109
Marfella, R., D'Onofrio, N., Trotta, M. C., Sardu, C., Scisciola, L., Amarelli, C., et al. (2021). Sodium/glucose Cotransporter 2 (SGLT2) Inhibitors Improve Cardiac Function by Reducing JunD Expression in Human Diabetic Hearts. Metabolism 127, 154936. doi:10.1016/j.metabol.2021.154936
Masson, W., Lobo, M., Lavalle-Cobo, A., and Molinero, G. (2020). Effect of Bempedoic Acid on Atherogenic Lipids and Inflammation: A Meta-Analysis. Clínica e Investigación en Arteriosclerosis 33(3), 117–126. doi:10.1016/j.arteri.2020.09.002
Meirong, W., Xiaoqing, W., Hailong, X., Wang, Y., Honghong, C., and Jianying, L. (2012). Preparation and Sustained-Release Properties of Trimetazidine Solid Dispersion. Chin. J. New Drugs 21 (12), 1401.
Meiszterics, Z., Kónyi, A., Hild, G., Sárszegi, Z., and Gaszner, B. (2017). Effectiveness and Safety of Anti-ischemic Trimetazidine in Patients with Stable Angina Pectoris and Type 2 Diabetes. J. Comp. Eff. Res. 6 (8), 649–657. doi:10.2217/cer-2017-0011
Melenovsky, V., Benes, J., Skaroupkova, P., Sedmera, D., Strnad, H., Kolar, M., et al. (2011). Metabolic Characterization of Volume Overload Heart Failure Due to Aorto-Caval Fistula in Rats. Mol. Cel. Biochem. 354 (1-2), 83–96. doi:10.1007/s11010-011-0808-3
Miaomiao, A., and Shuang, Y. (2018). Application Progress of Hypoglycemic Drugs in Patients with Type 2 Diabetes Mellitus Combined with Heart Failure. Med. Recapitulat 24 (06), 1160. doi:10.3969/j.issn.1006-2084.2018.06.023
Mingji, W. (2014). Adverse Reactions of Metformin and Key Points of Clinical Pharmaceutical Care. China Pharm. 23 (No.37116), 62.
Mitrohina, O. S., and Kuryata, O. V. (2008). Effects Of Simvastatin On Insulin Resistance In The Elderly Patients With Chronic Heart Failure And Preserved Systolic Function. Atherosclerosis 9 (1), 203. doi:10.1016/s1567-5688(08)70810-9
Monami, M., Dicembrini, I., Nardini, C., Fiordelli, I., and Mannucci, E. (2014). Effects of Glucagon-like Peptide-1 Receptor Agonists on Cardiovascular Risk: a Meta-Analysis of Randomized Clinical Trials. Diabetes Obes. Metab. 16 (1), 38–47. doi:10.1111/dom.12175
Nagoshi, T., Yoshimura, M., Rosano, G. M., Lopaschuk, G. D., and Mochizuki, S. (2011). Optimization of Cardiac Metabolism in Heart Failure. Curr. Pharm. Des. 17 (35), 3846–3853. doi:10.2174/138161211798357773
Nathanson, D., Ullman, B., Löfström, U., Hedman, A., Frick, M., Sjöholm, A., et al. (2012). Effects of Intravenous Exenatide in Type 2 Diabetic Patients with Congestive Heart Failure: a Double-Blind, Randomised Controlled Clinical Trial of Efficacy and Safety. Diabetologia 55 (4), 926–935. doi:10.1007/s00125-011-2440-x
Nemoto, T., Minami, Y., Yamaoka-Tojo, M., Sato, T., Muramatsu, Y., Kakizaki, R., et al. (2019). Impaired Flow-Mediated Dilation and Severity and Vulnerability of Culprit Plaque in Patients with Coronary Artery Disease. Int. Heart J. 60 (3), 539–545. doi:10.1536/ihj.18-531
Nesti, L., and Natali, A. (2017). Metformin Effects on the Heart and the Cardiovascular System: A Review of Experimental and Clinical Data. Nutr. Metab. Cardiovasc. Dis. 27 (8), 657–669. doi:10.1016/j.numecd.2017.04.009
Osuna, P. M., Brown, S. J., Tabatabai, L. S., and Hamilton, D. J. (2018). Stage-Based Management of Type 2 Diabetes Mellitus with Heart Failure. Methodist Debakey Cardiovasc. J. 14 (4), 257–265. doi:10.14797/mdcj-14-4-257
Paolisso, P., Bergamaschi, L., Rambaldi, P., Gatta, G., Foà, A., Angeli, F., et al. (2021). Impact of Admission Hyperglycemia on Heart Failure Events and Mortality in Patients with Takotsubo Syndrome at Long-Term Follow-Up: Data from HIGH-GLUCOTAKO Investigators. Diabetes Care 44 (9), 2158–2161. doi:10.2337/dc21-043310.2337/dci21-0042
Park, J. E., Park, J. Y., Seo, Y., and Han, J. S. (2019). A New Chromanone Isolated from Portulaca Oleracea L. Increases Glucose Uptake by Stimulating GLUT4 Translocation to the Plasma Membrane in 3T3-L1 Adipocytes. Int. J. Biol. Macromol. 123, 26–34. doi:10.1016/j.ijbiomac.2018.10.206
Park, J., Hwang, I., Kim, S. J., Youn, S. W., Hur, J., and Kim, H. S. (2018). Atorvastatin Prevents Endothelial Dysfunction in High Glucose Condition through Skp2-Mediated Degradation of FOXO1 and ICAM-1. Biochem. Biophys. Res. Commun. 495 (2), 2050–2057. doi:10.1016/j.bbrc.2017.08.023
Parra, V., Verdejo, H. E., Iglewski, M., Del, C. A., Troncoso, R., Jones, D., et al. (2014). Insulin Stimulates Mitochondrial Fusion and Function in Cardiomyocytes via the Akt-mTOR-Nfκb-Opa-1 Signaling Pathway. Diabetes 63 (1), 75–88. doi:10.2337/db13-0340
Peñalva, R., Morales, J., González-Navarro, C., Larrañeta, E., Quincoces, G., Peñuelas, I., et al. (2018). Increased Oral Bioavailability of Resveratrol by its Encapsulation in Casein Nanoparticles. Int. J. Mol. ences 19 (9).
Penna, C., Rastaldo, R., Mancardi, D., Cappello, S., Pagliaro, P., Westerhof, N., et al. (2006). Effect of Endothelins on the Cardiovascular System. J Cardiovasc Med (Hagerstown) 7 (9), 645–652. doi:10.2459/01.JCM.0000242996.19077.ba
Pickup, J. C., Chusney, G. D., Thomas, S. M., and Burt, D. (2000). Plasma Interleukin-6, Tumour Necrosis Factor Alpha and Blood Cytokine Production in Type 2 Diabetes. Life Sci. 67 (3), 291–300. doi:10.1016/s0024-3205(00)00622-6
Pinchuk, T. V., Fedulaev, Y. N., Khairetdinova, G. A., Kozina, A. A., and Ahmedzhanov, N. M. (2015). Effect Of Simvastatin Therapy On Indicators Of Transmitral Blood Flow In Patients With Diastolic Heart Failure. Rational Pharmacother. Cardiol. 9 (6), 655. doi:10.20996/1819-6446-2013-9-6-655-659
Plotnikov, A., Zehorai, E., Procaccia, S., and Seger, R. (2011). The MAPK Cascades: Signaling Components, Nuclear Roles and Mechanisms of Nuclear Translocation. Biochim. Biophys. Acta 1813 (9), 1619–1633. doi:10.1016/j.bbamcr.2010.12.012
Qi, Y., Xu, Z., Zhu, Q., Thomas, C., Kumar, R., Feng, H., et al. (2013). Myocardial Loss of IRS1 and IRS2 Causes Heart Failure and Is Controlled by P38α MAPK during Insulin Resistance. Diabetes 62 (11), 3887–3900. doi:10.2337/db13-0095
Rai, U., Kosuru, R., Prakash, S., Singh, S. P., Birla, H., Tiwari, V., et al. (2019). Tetramethylpyrazine Prevents Diabetes by Activating PI3K/Akt/GLUT-4 Signalling in Animal Model of Type-2 Diabetes. Life Sci. 236, 116836. doi:10.1016/j.lfs.2019.116836
Ramakrishnan, S. K., Russo, L., Ghanem, S. S., Patel, P. R., Oyarce, A. M., Heinrich, G., et al. (2016). Fenofibrate Decreases Insulin Clearance and Insulin Secretion to Maintain Insulin Sensitivity. J. Biol. Chem. 291 (46), 23915–23924. doi:10.1074/jbc.M116.745778
Riba, A., Deres, L., Sumegi, B., Toth, K., Szabados, E., and Halmosi, R. (20172017). Cardioprotective Effect of Resveratrol in a Postinfarction Heart Failure Model. Oxid. Med. Cel. Longev., 6819281. doi:10.1155/2017/6819281
Richards, S. K., Parton, L. E., Leclerc, I., Rutter, G. A., and Smith, R. M. (2005). Over-expression of AMP-Activated Protein Kinase Impairs Pancreatic {beta}-Cell Function In Vivo. J. Endocrinol. 187 (2), 225–235. doi:10.1677/joe.1.06413
Ridker, P. M., Pradhan, A., Macfadyen, J. G., Libby, P., and Glynn, R. J. (2012). Cardiovascular Benefits and Diabetes Risks of Statin Therapy in Primary Prevention: an Analysis from the JUPITER Trial. Lancet 380 (9841), 565–571. doi:10.1016/S0140-6736(12)61190-8
Riehle, C., and Abel, E. D. (2016). Insulin Signaling and Heart Failure. Circ. Res. 118 (7), 1151–1169. doi:10.1161/CIRCRESAHA.116.306206
Roe, N. D., He, E. Y., Wu, Z., and Ren, J. (2013). Folic Acid Reverses Nitric Oxide Synthase Uncoupling and Prevents Cardiac Dysfunction in Insulin Resistance: Role of Ca2+/calmodulin-Activated Protein Kinase II. Free Radic. Biol. Med. 65, 234–243. doi:10.1016/j.freeradbiomed.2013.06.042
Rogers, J. K., Jhund, P. S., Perez, A. C., B Hm, M., Cleland, J. G., Gullestad, L., et al. (2014). Effect of Rosuvastatin on Repeat Heart Failure Hospitalizations: the CORONA Trial (Controlled Rosuvastatin Multinational Trial in Heart Failure). Jacc Heart Fail. 2 (3), 289–297. doi:10.1016/j.jchf.2013.12.007
Romero, S. P., Andrey, J. L., Garcia-Egido, A., Escobar, M. A., Perez, V., Corzo, R., et al. (2013). Metformin Therapy and Prognosis of Patients with Heart Failure and New-Onset Diabetes Mellitus. A Propensity-Matched Study in the Community. Int. J. Cardiol. 166 (2), 404–412. doi:10.1016/j.ijcard.2011.10.141
Santos-Ferreira, D., Gonçalves-Teixeira, P., and Fontes-Carvalho, R. (2020). SGLT-2 Inhibitors in Heart Failure and Type-2 Diabetes: Hitting Two Birds with One Stone. Cardiology 145 (5), 311–320. doi:10.1159/000504694
Sardu, C., Paolisso, P., Sacra, C., Mauro, C., Minicucci, F., Portoghese, M., et al. (2019). Effects of Metformin Therapy on Coronary Endothelial Dysfunction in Patients with Prediabetes with Stable Angina and Nonobstructive Coronary Artery Stenosis: The CODYCE Multicenter Prospective Study. Diabetes Care 42 (10), 1946–1955. doi:10.2337/dc18-2356
Sardu, C., Paolisso, P., Sacra, C., Santamaria, M., de Lucia, C., Ruocco, A., et al. (2018). Cardiac Resynchronization Therapy with a Defibrillator (CRTd) in Failing Heart Patients with Type 2 Diabetes Mellitus and Treated by Glucagon-like Peptide 1 Receptor Agonists (GLP-1 RA) Therapy vs. Conventional Hypoglycemic Drugs: Arrhythmic burden, Hospitalizations for Heart Failure, and CRTd Responders Rate. Cardiovasc. Diabetol. 17 (1), 137. doi:10.1186/s12933-018-0778-9
Sattar, N., Preiss, D., Murray, H. M., Welsh, P., Buckley, B. M., de Craen, A. J., et al. (2010). Statins and Risk of Incident Diabetes: a Collaborative Meta-Analysis of Randomised Statin Trials. Lancet 375 (9716), 735–742. doi:10.1016/S0140-6736(09)61965-6
Sciacqua, A., Succurro, E., Armentaro, G., Miceli, S., Pastori, D., Rengo, G., et al. (2021). Pharmacological Treatment of Type 2 Diabetes in Elderly Patients with Heart Failure: Randomized Trials and beyond. Heart Fail. Rev. doi:10.1007/s10741-021-10182-x
Seferović, P. M., Petrie, M. C., Filippatos, G. S., Anker, S. D., Rosano, G., Bauersachs, J., et al. (2018). Type 2 Diabetes Mellitus and Heart Failure: a Position Statement from the Heart Failure Association of the European Society of Cardiology. Eur. J. Heart Fail. 20 (5), 853–872. doi:10.1002/ejhf.1170
Semenkovich, C. F. (2016). Insulin Resistance and a Long, Strange Trip. N. Engl. J. Med. 374 (14), 1378–1379. doi:10.1056/NEJMe1600962
Shi-Guang, W., Yan, X., Hao, X., and Wang, X-H. (2015). Astragaloside IV Prevents Lipopolysaccharide-Induced Injury in H9C2 Cardiomyocytes. Chin. J. Nat. Medicines 13 (2), 127–132. doi:10.1016/S1875-5364(15)60016-4
Shiojima, I., and Walsh, K. (2002). Role of Akt Signaling in Vascular Homeostasis and Angiogenesis. Circ. Res. 90 (12), 1243–1250. doi:10.1161/01.res.0000022200.71892.9f
Shu, X. (2005). Study on Photolysis Kinetics of Sodium Ferulate and its Sustained-Release Preparation in Vivo.
Shultz, J. C., Goehe, R. W., Wijesinghe, D. S., Murudkar, C., Hawkins, A. J., Shay, J. W., et al. (2010). Alternative Splicing of Caspase 9 Is Modulated by the Phosphoinositide 3-kinase/Akt Pathway via Phosphorylation of SRp30a. Cancer Res. 70 (22), 9185–9196. doi:10.1158/0008-5472.CAN-10-1545
Song, L. (2007). Clinical Observation of 31 Cases of Chronic Pulmonary Heart Disease Heart Failure Treated with Sodium Ferulate. J. Xianning Coll. Med. Edition (01), 37.
Spinar, J., and Smahelová, A. (2013). SAVOR TIMI 53 - Saxagliptin and Cardiovascular Outcomes in Patients with Type 2 Diabetes Mellitus. Vnitr Lek 59 (11), 1003. doi:10.1056/NEJMoa1307684
Stolarikova, E., Kopecky, J., Velebova, K., Veleba, J., Belinova, L., Malinska, H., et al. (2018). The Effect of Metformin on Energetic Metabolism in Patients with Type 2 Diabetes/prediabetes and Chronic Heart Failure. Atherosclerosis 275. doi:10.1016/j.atherosclerosis.2018.06.600
Swan, J. W., Anker, S. D., Walton, C., Godsland, I. F., Clark, A. L., Leyva, F., et al. (1997). Insulin Resistance in Chronic Heart Failure: Relation to Severity and Etiology of Heart Failure. J. Am. Coll. Cardiol. 30 (2), 527–532. doi:10.1016/s0735-1097(97)00185-x
Tekin, A., Sezgin, N., and Müderrisolu, H. (2016). PP-164 Short-Term Effects of Fluvastatin Therapy on Plasma Interleukin-10 Levels in Patients with Chronic Heart Failure. Am. J. Cardiol. 117, S99. doi:10.1016/j.amjcard.2016.04.257
Vyas, A. K., Yang, K. C., Woo, D., Tzekov, A., Kovacs, A., Jay, P. Y., et al. (2011). Exenatide Improves Glucose Homeostasis and Prolongs Survival in a Murine Model of Dilated Cardiomyopathy. PLoS One 6 (2), e17178. doi:10.1371/journal.pone.0017178
Walle, T., Hsieh, F., Delegge, M. H., Oatis, J. J., and Walle, U. K. (2004). High Absorption but Very Low Bioavailability of Oral Resveratrol in Humans. Drug Metab. Dispos. 32 (12), 1377–1382. doi:10.1124/dmd.104.000885
Wang, G., Cao, R., Chang, Y., Zhang, J., Du, Y., and Lv, J. (2005). Clinical Observation of Resveratrol Improving Prognosis of Chronic Heart Failure. Chin. Remedies Clin. (10), 755. doi:10.3969/j.issn.1671-2560.2005.10.008
Wang, J., Bai, L., Li, J., Sun, C., Zhao, J., Cui, C., et al. (2009). Proteomic Analysis of Mitochondria Reveals a Metabolic Switch from Fatty Acid Oxidation to Glycolysis in the Failing Heart. Sci. China C Life Sci. 52 (11), 1003–1010. doi:10.1007/s11427-009-0140-2
Wang, K. L., Liu, C. J., Chao, T. F., Huang, C. M., Wu, C. H., Chen, S. J., et al. (2014). Sitagliptin and the Risk of Hospitalization for Heart Failure: a Population-Based Study. Int. J. Cardiol. 177 (1), 86–90. doi:10.1016/j.ijcard.2014.09.038
Wang, X., Liu, Y., Yang, Z., Zhang, Z., Zhou, W., Ye, Z., et al. (2011). Glucose Metabolism-Related Protein 1 (GMRP1) Regulates Pancreatic Beta Cell Proliferation and Apoptosis via Activation of Akt Signalling Pathway in Rats and Mice. Diabetologia 54 (4), 852–863. doi:10.1007/s00125-011-2048-1
Watanabe, R., Azuma, R. W., Suzuki, J., Ogawa, M., Itai, A., Hirata, Y., et al. (2013). Inhibition of NF-Κb Activation by a Novel IKK Inhibitor Reduces the Severity of Experimental Autoimmune Myocarditis via Suppression of T-Cell Activation. Am. J. Physiol. Heart Circ. Physiol. 305 (12), H1761–H1771. doi:10.1152/ajpheart.00159.2013
Weir, D. L., Mcalister, F. A., Senthilselvan, A., Minhas-Sandhu, J. K., and Eurich, D. T. (2014). Sitagliptin Use in Patients with Diabetes and Heart Failure: a Population-Based Retrospective Cohort Study. JACC Heart Fail. 2 (6), 573–582. doi:10.1016/j.jchf.2014.04.005
Wenhe, S. (2016). Clinical Effect of Salvia Miltiorrhiza Ligustrazine Injection on Patients with Chronic Congestive Heart Failure. China J. Pharm. Econ. 11 (03), 53. doi:10.12010/j.issn.1673-5846.2016.03.023
Wu, Z., Huang, A., Yan, J., Liu, B., Liu, Q., Zhang, J., et al. (2017). Resveratrol Ameliorates Cardiac Dysfunction by Inhibiting Apoptosis via the PI3K/Akt/FoxO3a Pathway in a Rat Model of Diabetic Cardiomyopathy. J. Cardiovasc. Pharmacol. 70 (3), 184–193. doi:10.1097/FJC.0000000000000504
Xiaoli, J., and Shu, C. (2010). Energy Metabolism Support Therapy in Diabetic Patients with Heart Failure. Pract. J. Clin. Med. 7 (05), 150.
Xiaomei, W., and Guoliang, L. (2014). Effect of High Glucose Toxicity on Islet β Cells and its Clinical Countermeasures. J. Pract. Diabetology 10 (06), 15.
Xiaoyan, Z., Jinlin, S., Xukai, W., Hailong, W., Bo, W., and Peipei, J. (2013). Effects of Fenofibrate on Myocardial Fibrosis and Cardiac Function in Patients with Chronic Heart Failure. Chin. J. Mod. Med. 23 (29), 46.
Xiaoyun, P., Yunzhi, Q., and Sufen, L. (2005). Study on Bioavailability of Ligustrazine Phosphate Dropping Pills. Pharmacol. Clin. Chin. Materia Med. (01), 19.
Xiuling, , Shang, K., Lin, Ro., and Pengli, Zh. (2019). Resveratrol Protects the Myocardium in Sepsis by Activating the Phosphatidylinositol 3-Kinases (PI3K)/AKT/Mammalian Target of Rapamycin (mTOR) Pathway and Inhibiting the Nuclear Factor-Κb (NF-Κb) Signaling Pathway. Med. Sci. monitor: Int. Med. J. Exp. Clin. Res. 25, 9290–9298. doi:10.12659/MSM.918369
Xue-Li, N., Li, J-P., FanLiu, J. X, and Liang, N. (2019). Salvianolic Acid A Protects Neonatal Cardiomyocytes against Hypoxia/reoxygenation-Induced Injury by Preserving Mitochondrial Function and Activating Akt/GSK-3β Signals. Chin. J. Integr. Med. 25(1), 23–30. doi:10.1007/s11655-016-2747-z
Yaluri, N., Modi, S., and Kokkola, T. (2016). Simvastatin Induces Insulin Resistance in L6 Skeletal Muscle Myotubes by Suppressing Insulin Signaling, GLUT4 Expression and GSK-3β Phosphorylation. Biochem. Biophys. Res. Commun. 480 (2), 194–200. doi:10.1016/j.bbrc.2016.10.026
Yandrapalli, S., Malik, A., Guber, K., Rochlani, Y., Pemmasani, G., Jasti, M., et al. (2019). Statins and the Potential for Higher Diabetes Mellitus Risk. Expert Rev. Clin. Pharmacol. 12 (9), 825–830. doi:10.1080/17512433.2019.1659133
Yanhua, Z., Yonghong, Z., and Jiang, Y. (2011). Study on Compatibility Stability of Sodium Ferulate Injection and Common Clinical Liquids. Acta Academiae Medicinae CPAPF 20 (9), 720. doi:10.3969/j.issn.1008-5041.2011.09.011
Yanling, F. (2021). Effects of Atorvastatin Combined with Metformin on Cardiac Remodeling and Blood Lipid Levels in Patients with Diabetic Cardiomyopathy. Heilongjiang Med. J. 34 (02), 370.
Yu, B., Liu, D., Zhang, H., Xie, D., Nie, W., Shi, K., et al. (2018). Anti-hypertrophy Effect of Atorvastatin on Myocardium Depends on AMPK Activation-Induced miR-143-3p Suppression via Foxo1. Biomed. Pharmacother. 106, 1390–1395. doi:10.1016/j.biopha.2018.07.064
Yuan, X., Jing, S., Lingzhen, W. U., Chen, L., and Fang, J. (2014). Pharmacological Postconditioning with Tanshinone IIA Attenuates Myocardial Ischemia-Reperfusion Injury in Rats by Activating the Phosphatidylinositol 3-kinase Pathway. Exp. Ther. Med. 8 (3), 973–977. doi:10.3892/etm.2014.1820
Zhang, L., Yang, Y., Wang, Y., and Gao, X. (2011). Astragalus Membranaceus Extract Promotes Neovascularisation by VEGF Pathway in Rat Model of Ischemic Injury. Pharmazie 66 (2), 144. doi:10.1691/ph.2011.0738
Zhao, C., Luo, G., Li, C., and Yan, Z. (1989). The Hemorheological Study of Ligustrazine Treatment in Diabetic Subjects. Clin. Hemorheol. Micro. 9 (4), 615.
Zhen, C., and Faxiang, Z. (2011). Effect of Astragalus Membranaceus and Ligustrazine Injection on Chronic Congestive Heart Failure. J. Med. Forum 032 (006), 149.
Zheng, W., Zhou, J., Song, S., Kong, W., Xia, W., Chen, L., et al. (2018). Dipeptidyl-Peptidase 4 Inhibitor Sitagliptin Ameliorates Hepatic Insulin Resistance by Modulating Inflammation and Autophagy in Ob/ob Mice. Int. J. Endocrinol. 2018, 8309723. doi:10.1155/2018/8309723
Zhiming, Z., and Zhonghua, S. (2011). Fenofibrate in the Treatment of 16 Elderly Patients with Chronic Heart Failure. Chin. J. Gerontol. 31 (14), 2782. doi:10.3969/j.issn.1005-9202.2011.14.092
Dong, Z., Zhao, P., Xu, M., Zhang, C., Guo, W., Chen, H., et al. (2017). Astragaloside IV Alleviates Heart Failure via Activating PPARα to Switch Glycolysis to Fatty Acid β-oxidation. Sci. Rep.-UK. 7 (1), 2691. doi:10.1038/s41598-017-02360-5
Zhou, X., Chen, J., and Guo, Y. (2014). Is Treatment with Trimetazidine Beneficial in Patients with Chronic Heart Failure. PLoS One 9 (5), e94660. doi:10.1371/journal.pone.0094660
Zordoky, B. N., Robertson, I. M., and Dyck, J. R. (2015). Preclinical and Clinical Evidence for the Role of Resveratrol in the Treatment of Cardiovascular Diseases. Biochim. Biophys. Acta 1852 (6), 1155–1177. doi:10.1016/j.bbadis.2014.10.016
Zou, J., Gao, P., Hao, X., Xu, H., Zhan, P., and Liu, X. (2018). Recent Progress in the Structural Modification and Pharmacological Activities of Ligustrazine Derivatives. Eur. J. Med. Chem. 147, 150–162. doi:10.1016/j.ejmech.2018.01.097
Keywords: type 2 diabetes, heart failure, insulin signaling pathway, myocardial energy metabolism, insulin resistance, drugs
Citation: Ye H, He Y, Zheng C, Wang F, Yang M, Lin J, Xu R and Zhang D (2022) Type 2 Diabetes Complicated With Heart Failure: Research on Therapeutic Mechanism and Potential Drug Development Based on Insulin Signaling Pathway. Front. Pharmacol. 13:816588. doi: 10.3389/fphar.2022.816588
Received: 16 November 2021; Accepted: 31 January 2022;
Published: 03 March 2022.
Edited by:
Francesco Rossi, University of Campania Luigi Vanvitelli, ItalyReviewed by:
Celestino Sardu, University of Campania Luigi Vanvitelli, ItalyTing C. Zhao, Boston University, United States
Copyright © 2022 Ye, He, Zheng, Wang, Yang, Lin, Xu and Zhang. This is an open-access article distributed under the terms of the Creative Commons Attribution License (CC BY). The use, distribution or reproduction in other forums is permitted, provided the original author(s) and the copyright owner(s) are credited and that the original publication in this journal is cited, in accordance with accepted academic practice. No use, distribution or reproduction is permitted which does not comply with these terms.
*Correspondence: Junzhi Lin, bGluanVuemhpQGNkdXRjbS5lZHUuY24=; Runchun Xu, MTk5NzIwMTZAY2R1dGNtLmVkdS5jbg==; Dingkun Zhang, emhhbmdkaW5na3VuQGNkdXRjbS5lZHUuY24=
†These authors have contributed equally to this work