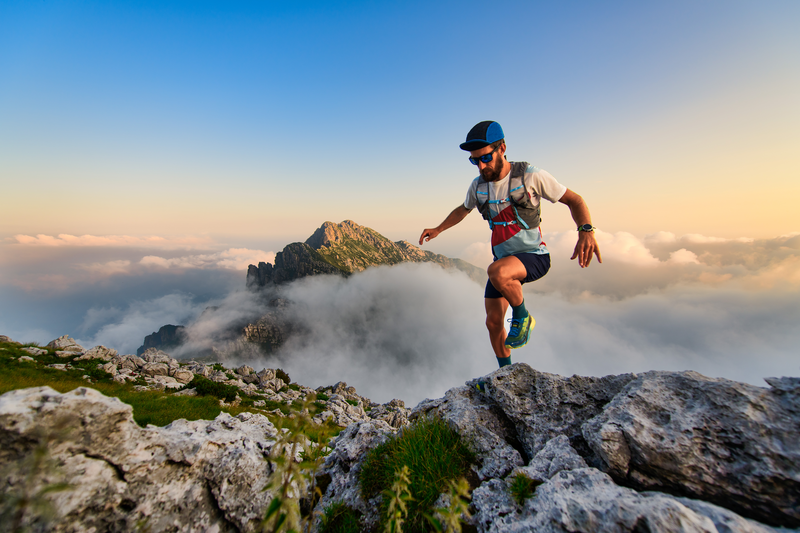
94% of researchers rate our articles as excellent or good
Learn more about the work of our research integrity team to safeguard the quality of each article we publish.
Find out more
ORIGINAL RESEARCH article
Front. Pharmacol. , 12 April 2022
Sec. Neuropharmacology
Volume 13 - 2022 | https://doi.org/10.3389/fphar.2022.812362
This article is part of the Research Topic Purinergic Signaling and Neuroinflammation View all 6 articles
Depression is a common and serious mental disorder. Data on its pathogenesis remain unclear and the options of drug treatments are limited. Here, we explored the role of pyroptosis, a novel pro-inflammatory programmed cell death process, in depression as well as the anti-depression effects and mechanisms of salidroside (Sal), a bioactive extract from Rhodiola rosea L. We established a corticosterone (CORT)-induced or lipopolysaccharide (LPS)-induced mice in vivo, and CORT, or nigericin (NLRP3 agonist)-induced PC12 cells in vitro. Our findings demonstrated that Sal profoundly mediated CORT or LPS-induced depressive behavior and improved synaptic plasticity by upregulating the expression of brain-derived neurotrophic factor (BDNF) gene. The data showed upregulation of proteins associated with NLRP3-mediated pyroptosis, including NLRP3, cleaved Caspase-1, IL-1β, IL-18, and cleaved GSDMD. The molecular docking simulation predicted that Sal would interact with P2X7 of the P2X7/NF-κB/NLRP3 signaling pathway. In addition, our findings showed that the NLRP3-mediated pyroptosis was regulated by P2X7/NF-κB/NLRP3 signaling pathway. Interestingly, Sal was shown to ameliorate depression via suppression of the P2X7/NF-κB/NLRP3 mediated pyroptosis, and rescued nigericin-induced pyroptosis in the PC12 cells. Besides, knock down of the NLRP3 gene by siRNA markedly increased the inhibitory effects of Sal on pyroptosis and proinflammatory responses. Taken together, our findings demonstrated that pyroptosis plays a crucial role in depression, and Sal ameliorates depression by suppressing the P2X7/NF-κB/NLRP3-mediated pyroptosis. Thus, our study provides new insights into the potential treatment options for depression.
Depression is a widespread mental health disorder, which is associated with disability and high mortality rates (Atique-Ur-Rehman and Neill, 2019). Typical clinical symptoms of depression include depressed mood, suppressed thinking and movement, as well as thoughts of worthlessness or even suicide (Su et al., 2018). It is a health problem that affects many people worldwide and leads to a significant social and economic burden (Malhi and Mann, 2018). Although multiple antidepressants are available for depression, there are still a series of limitations, including a low curative ratio, poor treatment compliance and serious side effects (Cosker et al., 2020). Thus, it is important to find more reliable treatments for depression.
Although the pathogenesis of depression is unclear, it is affected by various genetic, psychological, and physiological factors. Previous data showed that factors such as structural changes within the hippocampus, loss of BDNF, and excessive neuroinflammation are important in the development of depression (Haase and Brown, 2015). Recent evidence suggests that neuroinflammation is a key mediator of depression (Troubat et al., 2020). Other studies have shown that the expression of proinflammatory cytokines, especially interleukin (IL)-1β, is significantly enhanced in depressed patients (Pallavi et al., 2015). Increased inflammation leads to abnormal neuron changes and induces depressive symptoms (Felger 2018). Inflammation has been associated with a complex of NOD-like receptors (NLRs), and the NLRP3 receptor is an important molecular platform for processing and handling inflammatory responses in the intrinsic immune system (He et al., 2016). Activation of NLRP3 inflammasome releases inflammatory factors and initiates downstream inflammatory responses (Ramaswamy et al., 2013). Previous studies implicated that the NLRP3 inflammasome in the development of depression (Kaufmann et al., 2017; Su et al., 2017). Besides, other studies evaluated the IL-1β, TNF-α, and IL-6, and showed that they are overexpressed in patients with depression (Penninx et al., 2003; Thomas et al., 2005). Similarly, a previous systematic review and meta-analysis showed that the expression levels of IL-1β and IL-6 were significantly elevated in elderly patients with depression (Ng et al., 2018). In addition, there was significant increase of serum corticosterone and IL-1β, as well as higher levels of NLRP3 protein in hippocampus in the chronic stress-induced depression mice models (Zhang et al., 2015). Thus, NLRP3 inflammasome plays a crucial role in depression.
Previous data has shown that activation of the NLRP3 inflammasome can trigger caspase-1 associated pyroptosis, also referred to as “caspase-1 dependent programmed cell death”. Pyroptosis executor gasdermin D (GSDMD) is cleaved by activated caspase family proteins and releases the N-terminal, which creates holes in the cell membrane, leading to enhanced release of inflammatory products, cell rupture and death (Jorgensen et al., 2017). Recent studies have shown that NLRP3-regulated pyroptosis is involved in various neurodegenerative and metabolic disorders, such as Parkinson’s disease and diabetes as well as depression (Wang et al., 2019; Guan and Han, 2020; Li et al., 2020). However, data on the underlying mechanisms remain unclear.
The P2X7 receptor is distributed on cell membranes and is a P2X subtype closely associated with depression. After exposure to stressors, large amounts of ATP is released, which leads to activation of the P2X7R and NLRP3-mediated inflammatory responses (Bhattacharya and Jones, 2018). The release of inflammatory factors triggers neuroinflammation which can further lead to depressive-like behaviors. Besides, activated P2X7R can activate nuclear factor-κB (NF-κB) and promote maturation and excretion of inflammatory factors, such as IL-8 and IL-1β (Korcok et al., 2004; Thawkar and Kaur, 2019; Wang D. et al., 2020). In addition, it was shown that P2X7R, a key inflammatory switch can activate the NLRP3 inflammasome (Adinolfi et al., 2018).
Salidroside (Sal), a kind of phenylpropanoid glycoside found in the herb Rhodiola rosea L., has various pharmacological effects, including anti-inflammatory, antioxidant, and regulation of cognitive functions (Jin et al., 2016; Hu et al., 2020). Recent research has reported that Sal has protective effects on various diseases such as Alzheimer’s disease, epilepsy and stroke (Wang et al., 2015; Zhang et al., 2018; Wang H. et al., 2020). Data has shown that Sal exerts a potent antidepressant-like effect in chronic stress-depressed models or despair models and promotes neurogenesis in the hippocampus (Panossian et al., 2008; Vasileva et al., 2018). Although these findings demonstrate the ameliorative effect of Sal on depressive symptoms, data on its specific molecular mechanisms of Sal remain scant. This study aimed to explore the mechanisms of depression and the potential antidepressant-like effects of Sal.
Corticosterone (CORT, C104537), Fluoxetine (FLU, F131623) and TWEEN® 80 (T118633) were purchased from Aladdin (Shanghai, China). Lipopolysaccharide (LPS, L2630) was purchased from Sigma Aldrich (St. Louis, United States). Salidroside (Sal, MB5843-1) was purchased from Meilunbio (Dalian, China). Nigericin (Nig, HY-127019) was purchased from MedChemExpress (NewJersey, United States). The primary antibodies against P2X7, ASC, IL-18, IL-1β were purchased from Proteintech Group (Proteintech, United States). All chemicals and reagents were summarized in Supplementary Table S1.
The male C57BL/6 mice (8 weeks old, 20–22 g) were acquired from the Qinglongshan Farm (NO. SCXK 2020–0,005, Nanjing, China). To avoid the influence of female hormones on depressive behaviors, male mice were used in our study (Newhouse et al., 2015). The experimental animals were housed in cages with free access to food and water at the Laboratory Animal Research Center of China Pharmaceutical University. The laboratory temperature was controlled at 20–22°C with 50%–60% relative humidity, with a 12-h light-dark cycle. All animals are acclimatized to the laboratory environment for 1 week before the experiment. The protocol of experiments was supported by the Ethics Committee of China Pharmaceutical University.
Male C57BL6/J mice were weighed before the experiment, and were randomly divided into five groups (n = 10 per group): 1) the control group, 2) the CORT-injection group, 3) the CORT + fluoxetine treatment group (CORT + FLU), 4) the CORT +20 mg/kg Sal [CORT + Sal (20 mg/kg)] group, 5) the CORT +40 mg/kg Sal [CORT + Sal (40 mg/kg)] group. CORT was suspended in physiological saline containing 0.1% DMSO and 0.1% Tween-80, and the suspension was administered through subcutaneous injection (20 mg/kg) at a volume of 10 ml/kg once daily for 21 days to induce depression-like symptoms (Cavalcanti Capibaribe et al., 2019; Yokoyama et al., 2020). Based on the available studies, FLU (20 mg/kg) was selected for this experiment (Li et al., 2021). To determine the optimal dose of Sal, we relied on previous studies (Chen et al., 2012; Palmeri et al., 2016). Except control group, the other four groups all received CORT injection for 21 days. Sal and FLU were dissolved in saline and administrated by oral gavage 30 min prior to the CORT injection for 21 days. After 24 h of the last CORT administration, behavioral tests were conducted according to the time sequence of administration, including OFT, SPT, and FST. The experimental procedure is shown in .Supplementary Figure S1A. Subsequently, some mice were sacrificed and hippocampal tissue was stored at −80°C until further assays. The brain of other mice was removed and immersed in 4% PFA at 4°C for immunohistochemical examination and HE staining.
Mice were randomly divided into five groups (n = 10 per group): 1) the control group, 2) the LPS treated group, 3) the LPS +20 mg/kg FLU (LPS + FLU) group, 4) the LPS +20 mg/kg Sal [LPS + Sal (20 mg/kg)] group, 5) the LPS + 40 mg/kg Sal [LPS + Sal (40 mg/kg)] group. LPS (1 mg/kg) was dissolved in saline and injected intraperitoneally (10 ml/kg) for 5 days to induce acute depressive symptoms (Ali et al., 2020). Sal and FLU were dissolved in saline and administrated by oral gavage 30 min prior to the LPS injection for 5 days. Mice treated with a vehicle for the same period were used as the control group. The behavioral evaluations were given after the final LPS administration. The schedule of the experimental design was shown in Supplementary Figure S1B. Finally, some mice were sacrificed and hippocampal tissue was stored at −80°C until further assays. Another was sacrificed and the brain was fixed in 4% PFA at 4°C for immunohistochemical examination.
The behavioral tests were conducted on consecutive 3 days in the following description order. The first one was the open field test (OFT), followed by the sucrose preference test (SPT) and the last one was the forced swim test (FST).
Based on previous studies, moderately modified OFT was used to assess the locomotor capacity of mice (Zhao et al., 2019). Briefly, mice were acclimated to the experimental room for 1 h and were placed in a wooden box (45 × 45 × 45 cm), which was defined into 25 small squares. We artificially divide the nine grids as the central area and the remaining 16 squares against the walls as the peripheral area. The video recording system (ANY-Maze animal behavior analysis system) automatically records the locomotor activity of mice for 5 min. The observation index including distance, time and average velocity. After the test of each mouse, 75% alcohol was used to clean up the odor of the previous mouse and avoid affecting the results of the next experiment.
The absence of the ability to feel pleasure from pleasant activities is a classic symptom of human depression. On the other hand, depressed animals showed a decreased rate of preference for sucrose solution intake. The sucrose preference test was conducted using two bottles of either containing 1% sucrose solution or water respectively. Mice were acclimated to sucrose solution for 24 h. For the next 24 h, 1% sugar water was replaced by water. After the mice were starved of food and water for 24 h, the animals housed solely and had free access to the two bottles containing either 1% sucrose solution or water during the formal experiments. After 24 h, the ratio of sucrose consumption and total fluid intake was calculated as a sucrose preference ratio (Couch et al., 2016).
Mice were subjected to FST as noted in previous studies (Porsolt et al., 1977). Mice were placed in a beaker (height 21 cm, diameter 14 cm) filled with 15 cm depth of water (20–22°C). The total immobile time for the last 4 minutes of the 6-min total duration was calculated and analyzed by an observer blinded to the treatments.
To further explore the potential mechanism of Sal-mediated antidepressant related to pyroptosis processed by P2X7, molecular docking analysis was performed. The P2X7 protein (PDB code: 6U9W) structure was downloaded from the RCSB website (http://www.pdb.org) in PDB format. The 3D structure of Sal and A804598 (a typical P2X7R antagonist) was downloaded from https://pubchem.ncbi.nlm.nih.gov/. The P2X7 receptor was pretreated with pyMOL software to remove small molecule ligands, dehydrate, and hydrogenate. Then, both the small molecule and protein receptor formats are converted to PDBQT format. Finally, AutoDock Vina 1.1.2 was employed for molecular docking (Seeliger and de Groot, 2010).
The PC12 cells were cultured in DMEM (C11995500BT, Gibco) with 10% FBS, and placed in an incubator at 5% CO2 and 37°C. The concentration of CORT and Sal was selected by the CCK-8 assay. The cells were treated with CORT, and used to simulate an in vitro experimental model of depression in our study. To verify the neuroprotective benefits of Sal, the PC12 cells were exposed to Sal for 2 h and then treated with CORT (200 μM) for 24 h.
To determine whether NLRP3 can activate CORT-induced pyroptosis, the NLRP3 agonist nigericin (10 μM) was used in this study. The PC12 cells were divided into six groups for different treatments: 1) the Control group; 2) the 200 μM CORT (CORT) group; 3) the 10 μM nigericin (Nig group); 4) the 200 μM CORT +50 μM Sal (CORT + Sal) group; 5) the 10 μM Nig +50 μM Sal (Nig + Sal) group; 6) the 200 μM CORT +50 μM Sal +10 μM nigericin (CORT + Sal + Nig) group. The cells were treated with Sal (50 μM) for 2 h, and then incubated with CORT (200 μM) or Nig (10 μM) for 24 h.
NLRP3 siRNA and NC (negative control) siRNA provided by RiboBio (Guangzhou, China) were used in our study. The nucleotide sequences of siRNAs are listed in Supplementary Table S2. The PC12 cells were divided into six groups: the control group, the CORT group (200 μM), the Si-NC (100 nM) + CORT group, the Si-NLRP3 (100 nM) + CORT group, the CORT + Sal (50 μM) group, the Si-NLRP3 (100 nM) + CORT + Sal (50 μM) group. The PC12 cells were transfected with siRNAs by using riboFECTTMCP Reagent according to the manufacturer’s instructions. After transfection for 24 h, the cells were stimulated with CORT (200 μM) or Sal (50 μM) for 24 h. Finally, the cells were collected for various analysis.
The cell viability was quantified by the CCK-8 kit according to the manufacturer’s protocol. In brief, 10 µl of CCK-8 solution was added to each well of the 96-well plate and cultivated at 37°C for 1 h without light. The absorbance at 450 nm was detected by an enzyme marker. The cell viability of each well was computed according to the formula (Li L. et al., 2016): Cell Viability (%) = (Atest–Ablank)/(Acontrol–Ablank)*100%. The absorbance of different samples represented by Atest, absorbance of unprocessed cells is expressed as Acontrol, and Ablank is the absorbance of the well that is not inoculated with cells.
LDH, mainly located in the cytoplasm, can be released into the extracellular when cell membranes are disrupted (Sun et al., 2020). The release of LDH was determined by the LDH detection kit (Beyotime, China), according to the manufacturer’s instructions. Cytotoxicity was determined by a microplate reader at 490 nm.
The cell membrane integrity was detected by Calcein/PI Cell Viability/Cytotoxicity Assay Kit (Beyotime, China) according to the manufacturer’s instructions. Briefly, the cells were seeded in 24-well plates, then stained with Calcein-AM and PI mixed solution at 37°C for 30 min. The images were obtained using a fluorescent microscope (Olympus, Japan) (living cells appeared green and dead cells displayed red fluorescence) and the percentage of PI-positive cells was quantified by ImageJ software.
ELISA kits were used to determine the levels of BDNF and inflammatory cytokines (IL-18 and IL-1β) in our study according to the protocol’s guidelines.
Brain samples were fixed in 4% paraformaldehyde and embedded in paraffin for histopathological analysis (Khan et al., 2020). Serial 5 µm thick sections were obtained at the level of the hippocampus (Shal et al., 2019). HE staining to detect the morphological changes of the hippocampus.
Immunohistochemistry was carried out as previously reported with minor modifications (Aricioglu et al., 2019). Brain tissue was fixed with 4% paraformaldehyde for 24 h. Dehydrated, embedded in paraffin, and cut into slices of 5 µm thickness. After a series of operations, including deparaffinization, antigen repair and blocking, sections were incubated overnight with primary antibodies: anti-BDNF (Abcam, ab108319, 1:200), anti-P2X7 (Proteintech, 28207-1-AP, 1:50), anti-NLRP3 (Abcam, ab214185, 1:200) and anti-Cleaved caspase-1 (Thermo Fisher, PA5-99390, 1:200) at 4°C, followed by secondary antibodies incubation. Then, the slices were colored with diaminobenzidine (DAB). Finally, after dehydration and drying, sections were observed using a light microscope. The density values are analyzed by Image pro-plus software.
The expressions of P2X7, NLRP3 and Cleaved caspase-1 in PC12 cells were evaluated via immunofluorescence assay. Immunofluorescence was carried out as previously reported, with slight modifications (McFerran et al., 1998). In brief, PC12 cells were cultured on 24-well cell plates, fixed by 4% paraformaldehyde for 15 min. Cells were permeabilized with 0.1% Triton × 100 for 20 min and the blocking by 5% BSA for 30 min. The cells were incubated with the antibodies: anti-P2X7 (Proteintech, 28207-1-AP, 1:400), anti-NLRP3 (Abcam, ab214185, 1:400), Cleaved caspase-1 (Thermo Fisher, PA5-99390, 1:400) overnight at 4°C. The next day, the cells were washed three times in PBS followed by further incubation with a fluorescence-conjugated antibody for 2 h at room temperature. The nuclei were counterstained with DAPI. The images were photographed by inverted fluorescence microscopy and quantified by ImageJ software.
The hippocampus tissues and cells were homogenized in cold RIPA buffer containing various protease inhibitors. The equal amounts of protein were loaded and separated by 12% SDS-PAGE gels. After being transferred to PVDF and blocked by 5% skim milk powder for 2 h, and the membranes were incubated with antibodies targeting BDNF (Abcam, ab108319, 1:1000), P2X7 (Proteintech, 28207-1-AP, 1:1000), ASC (Proteintech, 10500-1-AP, 1:1000), NF-κB (CST, #8242, 1:1000), P-NF-κB (CST, #3033, 1:1000), NLRP3 (Abcam, ab214185, 1:1000), IL-18 (Proteintech, 10663-1-AP, 1:1000), IL-1β (Proteintech, 16806-1-AP, 1:1000), Cleaved GSDMD (CST, #10137, 1:1000), Cleaved caspase-1 (Thermo Fisher, PA5-99390, 1:1000), β-actin (Proteintech, 66009-1-AP, 1:5000) at 4°C overnight. After washing with TBST, the protein bands were incubated with the HRP-binding secondary antibody (1:1000) for 1 h at room temperature. Finally, blots were displayed with ECL reagent and quantified with ImageJ software.
Results are expressed as mean ± SEM. p < 0.05 means that the difference is statistically significant. All statistical analyses were analyzed by GraphPad Prism eight software. Statistical significance of various groups was analyzed by one-way ANOVA followed by Tukey’s test.
The exploratory and motor abilities of mice from each group were evaluated using OFT. Our results showed the motion trajectory plots of different experimental groups (Figure 1A). Mice treated with CORT exhibited significantly reduced locomotor activity compared to those in the control group. Administration of Sal (40 mg/kg) ameliorated the depression-like behaviors induced by CORT, increased total distance [F (4, 45) = 17.73, p < 0.01], elevated distance [F (4, 45) = 30.05, p < 0.01] and time [F (4, 45) = 7.188, p < 0.01] in the central zone, as well as increased average velocity [F (4, 45) = 27.58, p < 0.05] (Figure 1B). These data demonstrated that Sal can reverse depression-like behaviors in CORT mice.
FIGURE 1. Effects of Sal in CORT-induced depression in mice. (A) Representative tracks of different experimental groups in OFT. (B) Sal improved locomotor activity in the OFT (n = 10). (C) Sal ameliorated the consumption of sucrose solution in the SPT (n = 10). (D) Sal decreased the immobility time in the FST (n = 5). (E) HE staining to evaluate the pathological changes in the hippocampus. Cells have pyknotic nuclei with vacuolated cytoplasm (black arrows) and normal neuronal cells (red arrows) (magnification: × 400, Scale bar = 25 μm, n = 3). (F) The levels of BDNF in the hippocampus was examined using ELISA kits (n = 5). (G) Sal improved CORT-induced the decrease of BDNF in the hippocampus was determined by Western blot (n = 3). (H) Immunochemical staining and the relative density of BDNF in hippocampus CA1, CA3, and DG. Brown staining represents the expression of BDNF (black arrows). Original magnification: × 200, n = 3. ####p < 0.0001, ###p < 0.001, ##p < 0.01, #p < 0.05 versus the control group, ****p < 0.0001, ***p < 0.001, **p < 0.01, *p < 0.05 versus the CORT group.
Repeated administration of CORT significantly reduced the sucrose preference ratio in SPT compared with that in the control group (F (4, 45) = 7.636, p < 0.05) (Figure 1C). In contrast, treatment with Sal and FLU significantly increased the sucrose consumption. In addition, FST results showed that compared with the blank group, treatment with CORT obviously elevated the immobility time of mice [F (4, 20) = 7.565, p = 0.0003] (Figure 1D). However, there was a significant decline of the immobility time in FST in the groups exposed to FLU or different doses of Sal.
The HE-stained sections of the mouse hippocampus in the control group demonstrated that pyramidal cells of the CA1 region were uniform in size and neatly arranged, and many cells had intact structures. In contrast, analysis of the CORT group revealed that the number of nerve cells were significantly decreased, lacked regularity and nuclear pyknosis. These results suggested that the degrees of pathological damage in the hippocampal regions were caused by CORT. Interestingly, the hippocampal changes significantly reversed after Sal treatment [CA1: F (4, 10) = 8.891, p < 0.05; CA3: F (4, 10) = 14.98, p < 0.05; DG: F (4, 10) = 17.01, p < 0.05] (Figure 1E).
Stress reduces neurogenesis and impairs synaptic plasticity, which are attenuated by antidepressant treatment (Park 2019). BDNF is a neurotrophic factor widely dispersed in the central nervous system, whose increase is critical in mediating the antidepressant effect (Caviedes et al., 2017). We analyzed the effect of Sal on the expression of BDNF in the hippocampus. Our ELISA assay showed that exposure to CORT remarkably decreased BDNF levels in the hippocampus (Figure 1F). Administration of Sal (40 mg/kg) and FLU significantly improved BDNF protein levels in the hippocampus [F (4, 20) = 15.74, p < 0.05]. In addition, our findings showed that repeated CORT injections resulted in decreased BDNF protein levels in the hippocampus [F (4, 10) = 8.614, p < 0.01] (Figure 1G). In contrast, Sal and FLU upregulated the BDNF expression in depressed mice. Besides, immunohistochemical results demonstrated marked upregulation of BDNF in the group treated with Sal and FLU markedly increased [CA1: (F (4, 10) = 17.50, p < 0.05], CA3: [F (4, 10) = 27.17, p < 0.05], DG: [F (4, 10) = 12.33, p < 0.05)] (Figure 1H). Together, the data demonstrated that Sal can improve CORT-induced depressive symptoms.
We next evaluated the effect of Sal on pyroptosis-associated proteins using Western blot and ELISA. Our ELISA results showed significantly increased expression of IL-18 [F (4, 20) = 16.53, p < 0.0001] and IL-1β [F (4, 20) = 21.89, p < 0.0001] in the hippocampus of the CORT group compared to the control mice (Figure 2A). Treatment with Sal (20 mg/kg, 40 mg/kg) markedly reversed the protein expression changes. GSDMD is a newly discovered protein, which triggers pyroptosis and promotes the secretion of IL-18 and IL-1β (Li et al., 2019). In the Western blot analysis (Figure 2B), the CORT-stimulated mice showed remarkable upregulation of IL-18 [F (4, 10) = 7.872, p < 0.01], IL-1β [F (4, 10) = 8.986, p < 0.01] and cleaved GSDMD [F (4, 10) = 12.83, p < 0.001], which were all reversed by 40 mg/kg Sal and FLU. Collectively, our data illustrated that CORT triggers pyroptosis, which can be relieved by Sal.
FIGURE 2. Sal mitigates pyroptosis in CORT-induced depression in mice. (A) The levels of IL-1β and IL-18 in the hippocampus were detected by ELISA assay (n = 5). (B) The measurement of cleaved GSDMD, IL-1β and IL-18 in the hippocampus was detected by Western blot (n = 3). (C) Structural interactions of Sal with P2X7. 3D diagram of interaction between Sal and P2X7 showed the major binding sites and bonding forces. (D) Structural interactions of A-804598 (a P2X7R antagonist) with P2X7. 3D diagram of interaction between P2X7 and A-804598 showed the major binding sites and bonding forces. The Structural overview (left) and the close view (right). Dotted lines (yellow) represent hydrogen bonding interactions. ####p < 0.0001, ##p < 0.01 versus the control group; ***p < 0.001, **p < 0.01, *p < 0.05 versus the CORT group.
As shown in the Figure 2C, Sal binds to a relatively more spacious position at the GDP site of P2X7, and the amino acids interacting with it are SER543, ARG546, HIS547, TYR550, CYS548, LYS592, ARG551, ALA564, ASP565, ALA567, PHE566, ILE568, ARG578, SER589, ARG574, LEU569, PHE591, ILE568, and PRO594. Binding interactions of Sal and P2X7 protein target exhibited hydrogen-bonding interactions at ALA564, ARG574, ARG578 and SER589. Hydrophobic interactions at TYR550, CYS548, ALA564, ALA567, PHE566, ILE568, LEU569, PHE591, and ILE568. In addition, the binding energy of Sal and P2X7 protein receptor is −7.3 Kcal/mol. A-804598, a highly selective P2X7R antagonist, was shown effectively to bind with the P2X7 receptor at the binding site of the drug-binding pocket (Karasawa and Kawate, 2016). We also found that A-804598 could bind with P2X7R at ARG-574 site via hydrogen-bonding interactions. Hydrophobic interactions at TYR-550, LEU569, ALA-554, ALA-564, ALA-567 sites (Figure 2D). The molecular docking results showed that the binding affinity of A-804598 to P2X7 protein receptor is −7.4 Kcal/mol. It could be speculated that binding affinity of Sal with P2X7R is similar to that of A-804598 and P2X7R. Our data suggested that Sal could bind to P2X7 of P2X7/NF-κB/NLRP3 signal pathway.
We performed Western blot and immunohistochemical analyses to study the effect of Sal on the P2X7/NF-κB/NLRP3 signaling pathway in CORT-induced mice. Western blot assay showed increased expression of P2X7 [F (4, 10) = 9.862, p < 0.01], P-NF-κB [F (4, 10) = 10.75, p < 0.01], NLRP3 [F (4, 10) = 6.814, p < 0.01], ASC [F (4, 10) = 14.79, p < 0.001], Cleaved caspase-1 [F (4, 10) = 7.596, p < 0.01] in the CORT treated mice. However, Sal and FLU notably down-regulated the expression of the P2X7/NF-κB/NLRP3 signaling pathway-related proteins (Figure 3A). Besides, the increased cleaved of GSDMD and elevated caspase-1 activity in the CORT group were obviously blocked by Sal treatment (Figure 2B). These results indicated that Sal mitigated pyroptosis by inhibiting the P2X7/NF-κB/NLRP3 signaling pathway. Similarly, the immunohistochemical analyses showed that administration of CORT dramatically improved the production of P2X7 (CA1: (F (4, 10) = 8.321, p < 0.01), CA3: (F (4, 10) = 8.742, p < 0.01), DG: (F (4, 10) = 11.22, p < 0.001)), NLRP3 (CA1: (F (4, 10) = 13.23, p < 0.001), CA3: (F (4, 10) = 15.61, p < 0.001), DG: (F (4, 10) = 9.262, p < 0.01)) and cleaved Caspase-1 [CA1: (F (4, 10) = 12.82, p < 0.001), CA3: (F (4, 10) = 21.48, p < 0.001), DG: (F (4, 10) = 15.00, p < 0.001)], changes that were reversed by Sal and FLU (Figures 3B–D). Overall, our results demonstrated the correlation between pyroptosis and the P2X7/NF-κB/NLRP3 signaling pathway, and that Sal exerts its effect by suppressing the expression of proteins related with the signaling pathway.
FIGURE 3. Sal suppresses P2X7/NF-κB/NLRP3 signaling pathway in CORT-induced mice. (A) The expression of P2X7, P-NF-κB, and NLRP3 inflammasome-associated proteins in the hippocampus was checked by Western blot (n = 3). (B) The typical expression of P2X7 in the hippocampus was detected by immunohistochemical staining. (C) Sal reduced NLRP3 protein concentrations in the hippocampus was examined by immunohistochemical staining. (D) Sal decreased Cleaved caspase-1 expression in hippocampus CA1, CA3, and DG, measured by IHC assay. The typical positive staining of P2X7, NLRP3, and Cleaved caspase-1 were represented by brown staining (black arrows). Original magnification: ×200, n = 3. ####p < 0.0001, ###p < 0.001, ##p < 0.01 compared with the control group; **p < 0.01, *p < 0.05 compared with the CORT group.
LPS can cause inflammation and is widely used to induce depression-like behaviors (O'Connor et al., 2009; Sekio and Seki, 2014). In this study, LPS-induced acute mouse model was used to explore the ameliorative effects of Sal.
As shown in Figure 4A, LPS diminished the autonomous and exploratory behavior of mice in OFT, which were reversed by Sal and FLU. In our study, we showed that the distance traveled in the center [F (4, 45) = 5.472, p < 0.01], the time spent in the central zone [F (4, 45) = 6.046, p < 0.001], average velocity [F (4, 45) = 10.11, p < 0.0001], as well as the total distance [F (4, 45) = 10.00, p < 0.0001] were markedly decreased in mice administered with LPS than those in the normal group (Figure 4B).
FIGURE 4. Sal improves LPS-induced depression in mice. (A) Representative tracks of different experimental groups in the OFT. (B) Sal ameliorated locomotor activity in OFT, including the distance, time and average velocity during the test (n = 10). (C) Sal prevented the decrease of the consumption of sucrose solution in the SPT (n = 10). (D) Immobility duration in the FST (n = 5). (E) Sal improved LPS-induced the decrease of BDNF in the hippocampus was measured by ELISA (n = 5). (F) Sal improved LPS-induced the decrease of BDNF in the hippocampus was assayed by Western blot (n = 3). (G) The expression of BDNF in the hippocampus was detected by Immunochemical staining. Brown staining represents the expression of BDNF (black arrows). Original magnification: × 200, n = 3. ####p < 0.0001, ###p < 0.001, ##p < 0.01 contrast with the control group; ***p < 0.001, **p < 0.01, *p < 0.05 contrast with the LPS group.
In addition, the LPS-treated mice showed significantly decreased SPT [F (4, 45) = 7.419, p < 0.001] and extended inactivity time of FST [F (4, 20) = 10.71, p < 0.0001] in contrast with the control group (Figures 4C,D), phenomena that were restored by Sal and FLU. Thus, Sal prevented LPS-induced depressive-like behaviors.
The effect of Sal on BDNF expression in the LPS-induced mice was assessed by ELISA, Western blot and immunohistochemical analyses. The ELISA data showed that LPS treatment significantly suppressed the BDNF expression compared with that in the control group (Figure 4E). Conversely, pro-administration of Sal and FLU notably increased the expression of BDNF [F (4, 20) = 10.66, p < 0.01]. Furthermore, Western blot analyses demonstrated that LPS decreased hippocampus BDNF levels, while Sal treatment remarkably enhanced the production of hippocampus BDNF [F (4, 10) = 15.97, p < 0.01] (Figure 4F). Besides, immunohistochemical results showed that Sal reversed the LPS-induced suppression of BDNF in the hippocampus [CA1: (F (4, 10) = 9.960, p < 0.05), CA3: (F (4, 10) = 9.655, p < 0.05), DG: (F (4, 10) = 10.97, p < 0.05)] (Figure 4G). These results indicated that Sal could improve LPS-induced suppression of BDNF in the hippocampus.
The effect of Sal on pyroptosis-related cytokines, including IL-1β and IL-18, was evaluated using ELISA. The data showed that treatment with LPS dramatically increased the expression of IL-1β and IL-18. On the other hand, Sal inhibited the expression of IL-1β [F (4, 20) = 26.07, p < 0.05] and IL-18 [F (4, 20) = 12.38, p < 0.05] (Figure 5A). Similarly, Western blot analysis showed that Sal could inhibit the production of IL-1β [F (4, 10) = 12.35, p < 0.05], IL-18 [F (4, 10) = 8.367, p < 0.05], and the cleaved GSDMD [F (4, 10) = 6.542, p < 0.05] induced by LPS (Figure 5B). Our data demonstrated the inhibitory effects of Sal which relieve pyroptosis in LPS-induced mice.
FIGURE 5. Sal relieves pyroptosis in LPS-induced depression in mice. (A) The expression of IL-1β and IL-18 in the hippocampus was measured by ELISA assay (n = 5). (B) The concentrations of cleaved GSDMD, IL-1β and IL-18 proteins in the hippocampus were analyzed by Western blot (n = 3). ####p < 0.0001, ###p < 0.001, ##p < 0.01 compared with the normal group; ***p < 0.001, **p < 0.01, *p < 0.05 compared with the LPS group.
Mechanisms of the effects of Sal on pyroptosis in LPS-induced mice remain undefined. Western blot and immunohistochemical analyses of LPS-injected mice demonstrated increased expression of proteins such as P2X7 [F (4, 10) = 10.46, p < 0.01], P-NF-κB [F (4, 10) = 10.23, p < 0.05], NLRP3 [F (4, 10) = 9.431, p < 0.01], ASC [F (4, 10) = 6.874, p < 0.01], and Cleaved caspase-1 [F (4, 10) = 11.55, p < 0.001] in the LPS group. Sal markedly decreased the expression of proteins in the P2X7/NF-κB/NLRP3 signaling pathway (Figure 6A). In addition, the data showed that the P2X7 [CA1: (F (4, 10) = 8.530, p < 0.05); CA3: (F (4, 10) = 11.53, p < 0.01); DG: (F (4, 10) = 8.022, p < 0.05)], NLRP3 (CA1: (F (4, 10) = 10.96, p < 0.05); CA3: [F (4, 10) = 7.329, p < 0.05); DG: (F (4, 10) = 6.052, p < 0.05)] and Cleaved caspase-1 [CA1: (F (4, 10) = 11.56, p < 0.05); CA3: (F (4, 10) = 10.89, p < 0.05); DG: (F (4, 10) = 10.25, p < 0.05)] were notably up-regulated in the mice exposed to LPS. Conversely, administration of Sal and FLU effectively rescued the LPS-induced changes in the P2X7/NF-κB/NLRP3 cascade (Figures 6B–D).
FIGURE 6. Sal inhibits the P2X7/NF-κB/NLRP3 signaling pathway in LPS-induced mice. (A) The levels of proteins, including P2X7, P-NF-κB, NLRP3, ASC, Cleaved caspase-1 in the hippocampus, were determined by Western blot (n = 3). (B) The levels of P2X7 in the hippocampus were detected by IHC staining. (C) Immunohistochemical staining analysis of NLRP3 in the hippocampus. (D) Immunohistochemical results of Cleaved caspase-1 in the hippocampus. The typical positive staining of P2X7, NLRP3 and Cleaved caspase-1 were represented by brown staining (black arrows). Original magnification: × 200, n = 3. ###p < 0.001, ##p < 0.01 compared to the control group; **p < 0.01, *p < 0.05 compared to the LPS group.
We used PC12 cells, a cell line from pheochromocytoma of rat adrenal medulla. The cell line has typical neuron characteristics, high expression of glucocorticoid receptors and is widely used in neuropharmacology research (Li M. et al., 2016). Furthermore, a high concentration of corticosterone induces impairment in the PC12 cells, which has been widely used as an in vitro model to screen the underlying mechanisms of antidepressants (Zheng et al., 2011). In this study, we used PC12 cells exposed to 200 μM corticosterone to study the neuroprotective effect and potential mechanisms of Sal.
To explore the effect of Sal on cell viability, different concentrations of Sal were used in CCK-8 assay (Figure 7A). We selected the optimal concentration of corticosterone, which acts on the PC12 cells as assessed by the CCK-8 assay. The data showed that 100–800 μM CORT gradually decreased the cell viability with increase in concentration [F (4, 25) = 351.0, p < 0.01] (Figure 7B). 200 μM CORT reduced the cell viability to 56% and was then chosen in subsequent experiments. Compared with the model group, the cell survival rates of 10 μM and 50 μM Sal were 65% and 70%, respectively, which were significantly increased [F (4, 25) = 30.62, p < 0.05] (Figure 7C). 2 μM Sal also improved the cell viability, with no significant difference. In addition, our results showed that Sal treatment suppressed the release of LDH in the cell supernatant compared with the CORT group [F (4, 25) = 43.08, p < 0.001] (Figure 7D). Calcein-AM/PI staining data showed that CORT stimulation enhanced the number of PI-positive cells, which was remarkably suppressed following treatment with Sal (F (4, 10) = 6.902, p < 0.05) (Figure 7E). Further analyses revealed that CORT (200 μM) decreased the expression of BDNF in the PC12 cells, which is sync with the in vivo findings, while pretreatment with Sal remarkably restored the BDNF levels [F (4, 10) = 11.69, p < 0.05] (Figure 7F). These findings suggested that Sal could protect the PC12 cells against CORT-induced cytotoxicity.
FIGURE 7. Sal ameliorates CORT-induced PC12 cells. (A–C) The cell viability was measured by CCK-8 assay (n = 6). (D) The release of LDH was determined by LDH assay (n = 6). (E) Calcein-AM/PI staining images were captured by fluorescence microscopy, Calcein-AM (in green), PI (in red). Original magnification: ×200, Scale bar = 100 μm (n = 3). (F) Western blot analysis shown that Sal increased BDNF expression in CORT-induced PC12 cells (n = 3). (G) The expression of IL-1β and IL-18 in PC12 cell supernatant was detected by ELISA (n = 5). (H) The levels of IL-1β, IL-18 and cleaved GSDMD in supernatant of PC12 cell were analyzed by Western blot (n = 3). [(A) PC12 cells were cultured with various concentrations of Sal (2, 10, 50 μM) for 24 h (B) PC12 cells were treated with increasing doses of CORT (100–800 μM) for 24 h (C–H) PC12 cells were incubated with Sal (2, 10, 50 μM) for 2 h, and then cultured with 200 μM CORT for 24 h] ####p < 0.0001, ###p < 0.001, ##p < 0.01 compared to the control group; **p < 0.01, *p < 0.05 compared to the CORT group.
GSDMD-dependent membrane pore formation is an ultimate event of pyroptosis, which promotes the release of pro-inflammatory mediators, such as IL-18 and IL-1β (Zhang et al., 2020). We employed ELISA to analyze the levels of IL-1β [F (4, 20) = 11.45, p < 0.001] and IL-18 [F (4, 20) = 12.64, p < 0.001] in the PC12 cells as shown in Figure 7G. On the other hand, the expression of pyroptosis-related proteins was measured by Western blot assays (Figure 7H). In sync with the in vivo findings, the IL-1β [F (4, 10) = 10.92, p < 0.01], IL-18 [F (4, 10) = 17.93, p < 0.001] and cleaved GSDMD [F (4, 10) = 7.424, p < 0.01] proteins were upgraded in the PC12 cells, which were all significantly reversed by Sal. The cellular membrane integrality was evaluated by Calcein-AM/PI staining and LDH release. These results also indicated that CORT-induced pyroptosis in PC12 cells. Taken together, these findings demonstrated that the protective effects of Sal against CORT-induced cell injury was mediated through inhibiting of pyroptosis.
To further evaluate the potential molecular mechanisms of Sal in pyroptosis, we assayed the expression of proteins associated with the P2X7/NF-κB/NLRP3 signaling pathway in the PC12 cells. Western blot analysis showed that the expression levels of P2X7 [F (4, 10) = 9.217, p < 0.01], P- NF-κB [F (4, 10) = 18.23, p < 0.001], NLRP3 [F (4, 10) = 8.077, p < 0.05], ASC [F (4, 10) = 15.38, p < 0.01], and Cleaved caspase-1 [F (4, 10) = 7.748, p < 0.05] were significantly higher in the CORT-damaged PC12 cells, and the proteins were obviously down-regulated by Sal (Figure 8A). Our immunofluorescence results demonstrated that Sal-treatment greatly decreased the concentrations of P2X7 [F (2, 6) = 11.73, p < 0.05], NLRP3 [F (2, 6) = 16.85, p < 0.01] and Cleaved caspase-1 [F (2, 6) = 35.47, p < 0.01] in the PC12 cells (Figures 8B–D). These results indicated that Sal relieves pyroptosis in CORT-induced PC12 cells.
FIGURE 8. Sal ameliorates pyroptosis by inhibiting P2X7/NF-κB/NLRP3 signaling pathway in CORT-induced PC12 cells. (A) The protein expression of P2X7, P-NF-κB, NLRP3, ASC, Cleaved caspase-1 in PC12 cells was detected by Western blot (n = 3). PC12 cells were pre-treated with Sal (2, 10, 50 μM) for 2 h, and then stimulation with 200 μM CORT for 24 h. (B) Immunofluorescence staining of P2X7 in PC12 cells (n = 3). (C) Immunofluorescence detection of the expression of Cleaved caspase-1 in PC12 cells (n = 3). (D) The results of immunofluorescence demonstrated the expression level of NLRP3 in PC12 cells (n = 3). PC12 cells were pre-treated with Sal (50 μM) for 2 h, and then stimulation with 200 μM CORT for 24 h. Original magnification: ×200. (E) The protein levels of NLRP3, ASC, Cleaved caspase-1, IL-1β, IL-18, and Cleaved GSDMD were detected using Western blot (n = 3). ####p < 0.0001, ###p < 0.001, ##p < 0.01 versus the control group; ***p < 0.001, **p < 0.01 versus the CORT model group; &&p < 0.01, &p < 0.05 represent Nig + Sal group versus Nig group; @p < 0.05 represent CORT + Sal + Nig group compared with CORT + Sal group.
Based on the essentiality of NLRP3 in pyroptosis, we assessed the effect of nigericin (an NLRP3 agonist). Our Western blot assay showed that treatment with CORT and nigericin increased the expression of NLRP3 [F (5, 12) = 15.64, p < 0.001], ASC [F (5, 12) = 15.19, p < 0.01], Cleaved caspase-1 [F (5, 12) = 23.65, p < 0.001], IL-1β [F (5, 12) = 15.21, p < 0.01], IL-18 [F (5, 12) = 19.42, p < 0.01] and Cleaved GSDMD [F (5, 12) = 15.11, p < 0.001] in vitro. Nonetheless, these effects were all abolished by the Sal (Figure 8E). In addition, compared with the CORT + Sal group, the levels of NLRP3, ASC, Cleaved caspase-1, IL-1β and IL-18 were increased in the CORT + Sal + Nig group (All p < 0.05). Nig weakened the inhibitory effect of Sal on pyroptosis. Thus, these results demonstrated that the anti-pyroptosis effect of Sal is associated with NLRP3.
Next, to investigate the impact of NLRP3 on Sal-specific anti-pyroptosis effects, the PC12 cells were transfected with Si-NLRP3. NLRP3 was successfully knocked down by Si-RNA, as demonstrated by Western blot data (Figure 9A). We selected the Si-NLRP3 with the best knockdown effect for subsequent experiments. Our data showed that CORT decreased the viability of the cells, which was ameliorated by Si-NLRP3 [F (5, 30) = 21.02, p < 0.05] (Figure 9B). In addition, there was increased release of LDH following the CORT treatment. Furthermore, compared with the CORT group, Si-NLRP3 or Sal treatment significantly downregulated the LDH release [F (5, 30) = 39.81, p < 0.05] (Figure 9C). Our results showed increased PI positive stained cells in the CORT-group, effects that were abolished by Sal and Si-NLRP3 [F (5, 12) = 9.565, p < 0.05] (Figure 9D). As illustrated in Figure 9E, NLRP3 knocked down with SiRNA decreased the expression of pyroptosis-related proteins, including cleaved caspase-1 [F (5, 12) = 14.67, p < 0.01], IL-1β [F (5, 12) = 17.39, p < 0.01], IL-18 [F (5, 12) = 29.81, p < 0.01], and cleaved GSDMD [F (5, 12) = 14.44, p < 0.01] in the PC12 cells treated with CORT.
FIGURE 9. Sal and knockdown of NLRP3 attenuates CORT-induced pyroptosis in PC12 cells. (A) NLRP3 was knocked down by NLRP3 siRNA in PC12 cells, transfection efficiency of NLRP3 was detected by western blot (n = 3). (B) The viability of cells was detected by CCK-8 assay (n = 6). (C) Cell cytotoxicity in PC12 cells was detected by LDH cytotoxicity assay (n = 6). (D) Cell viability and cytotoxicity were measured by Calcein-AM/PI staining. Calcein-AM (in green), PI (in red). Original magnification: ×200, Scale bar = 100 μm, n = 3. (E) The levels of cleaved caspase-1, IL-1β, IL-18, and cleaved GSDMD in PC12 cells were assessed by western blot analysis (n = 3). ####p < 0.0001, ###p < 0.001, ##p < 0.01 compared with the control group; **p < 0.01, *p < 0.05 compared with the CORT group; ^^^^p < 0.0001, ^^^p < 0.001, ^^p < 0.01, ^p < 0.05 compared with Si-NC + CORT group.
Recent studies have reported that Sal exerts various pharmacological effects such as anti-inflammatory, antioxidant activities (Grech-Baran et al., 2015; Fan et al., 2020). Other studies have demonstrated that Sal can ameliorate cognitive and motor abilities by suppressing the dysfunction of hippocampal neurons (Gao et al., 2015; Palmeri, Mammana, Tropea, Gulisano and Puzzo 2016). In our study, we explored antidepressant-like effects of Sal and its underlying mechanisms. The data showed that Sal improves depression-like behavior in mice, inhibits pyroptosis and suppresses NLRP3-mediated pyroptosis via inhibition of P2X7/NF-κB/NLRP3 signaling pathway, as illustrated in Figure 10.
FIGURE 10. Illustration of the mechanism which Sal improves depression through suppressing NLRP3-mediated pyroptosis. The possible mechanism of Sal is as follows: Sal suppresses NLRP3-mediated pyroptosis through inhibiting P2X7/NF-κB/NLRP3 signaling pathway.
The pathogenesis of depression is very complex, and the proposed “neurotropism and plasticity” theory is gradually gaining attention in recent years. According to this theory, depression is caused by decreased neurotrophic factors, impaired synaptic plasticity and reduced neural plasticity (Duman et al., 2016). BDNF is involved in the growth and maintenance of neurons and is essential for cognitive function (Phillips 2017). Research has shown that the level of BDNF in the hippocampus and prefrontal cortex is significantly reduced in patients with depression (Taliaz et al., 2010). In this study, we showed that Sal significantly ameliorates CORT or LPS induced suppression of hippocampal BDNF expression. Besides, it has been reported that patients with depression have an overactive hypothalamic-pituitary-adrenal (HPA) axis and increased cortisol levels (Keller et al., 2017). Repeated injections of CORT in animals cause deregulation of the HPA axis, neuronal damage, cognitive and memory decline, and induce depression-like behavior (Zhao et al., 2008; Badr et al., 2020). Thus, CORT-induced model has been widely used as a chronic model of depression caused by stress (Kinlein et al., 2019). In addition, high concentrations of CORT have been used to induce injury in PC12 cells, which emulates pathological mechanisms of depression (Mao et al., 2012). Therefore, CORT-induced depression model is a reliable model for screening antidepressants and exploring their pharmacological mechanisms. Our in vivo results showed that CORT administration significantly decreased sucrose preference rate, increased the time of immobility in FST, and reduced motility in OFT. However, Sal administration profoundly improved the behavioral defects in SPT, OFT and FST, implying that Sal alleviates depression-like behavior in mice. Furthermore, our data showed that CORT induced damage in the PC12 cells, release of LDH and increased PI staining, which are reversed by Sal. In a previous study, fluoxetine, a typical selective serotonin reuptake inhibitor (SSRI), was shown to effectively reverse anxiety/depression-like behaviors (Alboni et al., 2017). In agreement, our findings showed that chronic treatment with fluoxetine also significantly increased the mobility time in the FST as well as the time spent in OFT. Thus, treatment with Sal and FLU effectively alleviated the depression-like behavior of mice and FLU was used as a positive control in this experiment.
Neuroinflammation is an essential occurrence in the pathogenesis of psychiatric diseases, which is associated with the pathogenesis of depression Galecki and Talarowska, 2018). Normal levels of inflammatory cytokines play a critical role in signaling, but excessive stress and long-term chronic inflammation can lead to neuroinflammatory, cellular damage, and exacerbation of neurodegenerative diseases as well as psychiatric disorders (Voet et al., 2019). Evidence has shown a strong correlation between depression and peripheral inflammatory markers in blood and cerebrospinal fluid (CSF). For instance, a recent cumulative meta-analysis reported that interleukin-6 (IL-6), IL-1β and C-reactive protein (CRP) are associated with depression (Haapakoski et al., 2015). Under physiological conditions, inflammasome-induced IL-1β expression is essential as a trophic support to memory formation (Yirmiya et al., 2002). However, at high levels, IL-1β becomes excitotoxic, which alters synaptic activity (Ross et al., 2003; Huang et al., 2011). Its expression and cleavage are influenced by NF-κB and NLRP3 (Wen et al., 2012; Zhong et al., 2016). Another study showed that NLRP3 inflammasome regulated the secretion of BDNF (Ward et al., 2019). This inverse correlation between the expression of BDNF and NLRP3 was also observed in the hippocampus of diabetic rats (Ye et al., 2018). It has been demonstrated that the NLRP3 inflammasome mediates depressive-like behaviors in animals (Zhang et al., 2014). Clinical data regarding the involvement of the NLRP3 inflammasome in patients with depression are scarce. A previous report showed that caspase-1, NLRP3 mRNA expression, and NLRP3 protein levels are increased in mononuclear blood cells in patients with depression (Alcocer-Gomez et al., 2014). In our study, mice exposed to CORT showed elevated levels of IL-18, IL-1β, and NLRP3, which were all attenuated by Sal intervention.
The NLRP3 inflammasome, an important component of the immune system, is one of the most intensively studied inflammasomes, and consists of NLRP3, apoptosis speck-like protein and pro-caspase-1. The NLRP3 inflammasome is present in microglia and astrocytes in the CNS (Song et al., 2017). An increase in expression of NLRP1 and NLRP3 inflammasome proteins and IL-1β and IL-18 proteins in neurons under ischemic conditions (Fann et al., 2013). A previous study also implied that the expression of NLRP3 in neurons increased in patients with Rasmussen’s encephalitis (V. et al., 2013). Activation of the NLRP3 inflammasome complex promotes activation of caspase-1, which further induces cleavage of GSDMD to a GSDMD-N terminal structure with pore-forming activity, eventually leading to cell rupture, and further inducing pyroptosis. In parallel, pro-IL-1β and pro-IL-18 are sheared by csapase-1 and can be excreted through the channels formed by the GSDMD-N terminal domain, thus triggering the pyroptosis-associated inflammatory cascade (Shi et al., 2015). Recent studies have shown that stress participates in the pathology of depression by activating the NLRP3 complex, which induces pyroptosis (Arioz 2019). Our in vivo and in vitro results indicated that Sal treatment significantly decreased pyroptosis-related protein levels including cleaved GSDMD, cleaved caspase-1, IL-1β, and IL-18. Therefore, the protective effect of Sal against depression-like behavior may be associated with the inhibition of pyroptosis. Pyroptosis is often accompanied by inflammation, which performs an essential role in the fight against infections. The activated NLRP3 inflammasome is one of the most important mediators of pyroptosis, which is involved in various inflammatory and immune diseases (Liang et al., 2020). Interestingly, we observed the characteristic hallmarks of pyroptosis in depression, which included increased activation of NLRP3 inflammasome and the release of IL-1β and IL-18. To evaluate whether NLRP3-mediated pyroptosis in depression, nigericin (an NLRP3 agonist) was added to activate NLRP3 in Sal-protected CORT-damaged PC12 cells. The data demonstrated that nigericin (an NLRP3 agonist) could reverse the protective effect of Sal in vitro. In addition, knockdown of the NLRP3 with siRNA significantly inhibited cell pyroptosis and improved cell viability. These results demonstrated that NLRP3 silencing and Sal treatment could prevent cell damage and ameliorate pyroptosis in PC12 cells. Our findings implied that NLRP3 may be a potential therapeutic target for depression and Sal can improve depressive symptoms by inhibiting the NLRP3-mediated pyroptosis.
It has been reported that NLRP3 inflammasome is activated by various stimuli, including ATP and K+ ionophores (Kelley et al., 2019). The P2X7 receptor, a subgroup of the P2X family distributed on cell membranes, is highly expressed in various neurons and immune cells and is closely associated with neuroinflammation and immunity (Kanellopoulos and Delarasse, 2019). P2X7 receptors are activated by ATP to induce overactivation of the NLRP3 inflammasome. As a key inflammation switch, P2X7 receptor participates in the development of depression by influencing the release of IL-1β (Yue et al., 2017). In our previous study, we have demonstrated that the activation of P2X7/NF-κB signaling pathway is involved in depression (Zhang et al., 2016). NF-κB, a key transcriptional activator of the NLRP3 inflammasome, is important in the activation and assembly of the inflammasome. NF-κB and NLRP3 are interconnected inflammatory pathways, and their interaction promotes the emission of inflammatory factors, leading to pyroptosis (Swanson et al., 2019). In addition, the NF-κB-mediated transcription can regulate the ability of the P2X7 receptor to initiate caspase-1 and fuel secretion of IL-18 (Kahlenberg et al., 2005). In our study, the molecular docking analysis predicted the interaction of Sal with P2X7, a upstream of P2X7/NF-κB/NLRP3. To study the underlying mechanisms of NLRP3-mediated pyroptosis, we analyzed the expression of P2X7R, ASC, and other proteins in hippocampus and cell suspensions by Western blot analysis. The data showed that the expression of proteins related with P2X7/NF-κB/NLRP3 signaling pathway-related and pyroptosis was significantly inhibited after Sal treatment. At the same time, there was improvement in the neurological function and histopathology. Our findings suggested that Sal could significantly alleviate depressive symptoms, and it may be dependent on the P2X7/NF-κB/NLRP3 pathway-mediated pyroptosis.
Previous studies demonstrated that LPS stimulation leads to pyroptosis and secretion of active IL-1β (Russo et al., 2021). In addition, the LPS-induced depression model was based on an inflammation-associated model of acute depression, and lower doses were more likely to trigger symptoms such as inflammatory response, reduced sucrose preference rate, and reduced exploratory activity in the OFT (Arioz 2019; Zheng et al., 2021). To further investigate the anti-inflammatory and antidepressant-like effect mechanisms of Sal, our study used a mice model with acute injection of LPS. The data showed that treatment with Sal led to decreased immobility and increased sucrose preference rate in the LPS-induced mice, and significantly improved exploratory and locomotor abilities in the OFT. In addition, the expression of NLRP3, ASC, Cleaved caspase-1 and pyroptosis-related proteins increased significantly under stimulation by LPS, which were ameliorated by Sal. Taken together, these data suggest that Sal reverses LPS-induced depressive behavior.
In conclusion, our findings indicated that NLRP3-mediated pyroptosis exerted an essential effect on depressive symptoms. Precisely, Sal can ameliorate pyroptosis via suppressing the P2X7/NF-κB/NLRP3 pathway. Furthermore, we revealed that Sal provides novel therapeutic strategies for the treatment of depression.
The original contributions presented in the study are included in the article/Supplementary Material, further inquiries can be directed to the corresponding authors.
The animal study was reviewed and approved by China Pharmaceutical University.
YUC, LZ, and TY conceived designed this study. YUC, YAC, YF, and YW conducted the experiments. YUC, MM, YZ, XZ processed the data. YUC, YAC, and LZ edited the initial manuscript. All the authors read and approved the final manuscript.
The authors declare that the research was conducted in the absence of any commercial or financial relationships that could be construed as a potential conflict of interest.
All claims expressed in this article are solely those of the authors and do not necessarily represent those of their affiliated organizations, or those of the publisher, the editors and the reviewers. Any product that may be evaluated in this article, or claim that may be made by its manufacturer, is not guaranteed or endorsed by the publisher.
We appreciate Jie Zhao, a staff member of Pharmaceutical Animal Experimental Center of China Pharmaceutical University, for her kind help with animal’s experiments.
The Supplementary Material for this article can be found online at: https://www.frontiersin.org/articles/10.3389/fphar.2022.812362/full#supplementary-material
ATP, Adenosine triphosphate; ANOVA, Analysis of variance; BDNF, Brain Derived Neurotrophic Factor; CCK-8, Cell Counting Kit-8; CORT, Corticosterone; Caspase-1, Cysteinyl aspartate specific proteinase; ELISA, Enzyme-linked immunosorbent assay; FLU, fluoxetine; FST, Forced swim test; GSDMD, Gasdermin D; HPA, Hypothalamic-pituitary-adrenal axis; IF, Immunofluorescence assay; IL-1β, Interleukin 1 beta; LPS, Lipopolysaccharide; NF-κB, Nuclear Factor Kappa B; Nig, Nigericin; NLRs, NOD-like receptors; OFT, Open field test; PFA, Paraformaldehyde; PVDF, Polyvinylidene difluoride; Sal, Salidroside; SPT, Sucrose preference test.
Adinolfi, E., Giuliani, A. L., De Marchi, E., Pegoraro, A., Orioli, E., and Di Virgilio, F. (2018). The P2X7 Receptor: A Main Player in Inflammation. Biochem. Pharmacol. 151, 234–244. doi:10.1016/j.bcp.2017.12.021
Alboni, S., van Dijk, R. M., Poggini, S., Milior, G., Perrotta, M., Drenth, T., et al. (2017). Fluoxetine Effects on Molecular, Cellular and Behavioral Endophenotypes of Depression Are Driven by the Living Environment. Mol. Psychiatry 22, 635–561. doi:10.1038/mp.2015.142
Alcocer-Gómez, E., de Miguel, M., Casas-Barquero, N., Núñez-Vasco, J., Sánchez-Alcazar, J. A., Fernández-Rodríguez, A., et al. (2014). NLRP3 Inflammasome Is Activated in Mononuclear Blood Cells from Patients with Major Depressive Disorder. Brain Behav. Immun. 36, 111–117. doi:10.1016/j.bbi.2013.10.017
Ali, T., Rahman, S. U., Hao, Q., Li, W., Liu, Z., Ali Shah, F., et al. (2020). Melatonin Prevents Neuroinflammation and Relieves Depression by Attenuating Autophagy Impairment through FOXO3a Regulation. J. Pineal Res. 69, e12667. doi:10.1111/jpi.12667
Aricioglu, F., Ozkartal, C. S., Bastaskin, T., Tüzün, E., Kandemir, C., Sirvanci, S., et al. (2019). Antidepressant-like Effects Induced by Chronic Blockade of the Purinergic 2X7 Receptor through Inhibition of Non-like Receptor Protein 1 Inflammasome in Chronic Unpredictable Mild Stress Model of Depression in Rats. Clin. Psychopharmacol. Neurosci. 17, 261–272. doi:10.9758/cpn.2019.17.2.261
Arioz, B. I., Tastan, B., Tarakcioglu, E., Tufekci, K. U., Olcum, M., Ersoy, N., et al. (2019). Melatonin Attenuates LPS-Induced Acute Depressive-like Behaviors and Microglial NLRP3 Inflammasome Activation through the SIRT1/Nrf2 Pathway. Front. Immunol. 10, 1511–1530. doi:10.3389/fimmu.2019.01511
Atique-Ur-Rehman, H., and Neill, J. C. (2019). Cognitive Dysfunction in Major Depression: From Assessment to Novel Therapies. Pharmacol. Ther. 202, 53–71. doi:10.1016/j.pharmthera.2019.05.013
Badr, A. M., Attia, H. A., and Al-Rasheed, N. (2020). Oleuropein Reverses Repeated Corticosterone-Induced Depressive-like Behavior in Mice: Evidence of Modulating Effect on Biogenic Amines. Sci. Rep. 10, 3336–3346. doi:10.1038/s41598-020-60026-1
Bhattacharya, A., and Jones, D. N. C. (2018). Emerging Role of the P2X7-NLRP3-IL1β Pathway in Mood Disorders. Psychoneuroendocrinology 98, 95–100. doi:10.1016/j.psyneuen.2018.08.015
Capibaribe, V. C. C., Vasconcelos Mallmann, A. S., Lopes, I. S., Oliveira, I. C. M., de Oliveira, N. F., Chaves, R. C., et al. (2019). Thymol Reverses Depression-like Behaviour and Upregulates Hippocampal BDNF Levels in Chronic Corticosterone-Induced Depression Model in Female Mice. J. Pharm. Pharmacol. 71, 1774–1783. doi:10.1111/jphp.13162
Caviedes, A., Lafourcade, C., Soto, C., and Wyneken, U. (2017). BDNF/NF-κB Signaling in the Neurobiology of Depression. Curr. Pharm. Des. 23, 3154–3163. doi:10.2174/1381612823666170111141915
Chen, S. F., Tsai, H. J., Hung, T. H., Chen, C. C., Lee, C. Y., Wu, C. H., et al. (2012). Salidroside Improves Behavioral and Histological Outcomes and Reduces Apoptosis via PI3K/Akt Signaling after Experimental Traumatic Brain Injury. PLoS One 7, e45763. doi:10.1371/journal.pone.0045763
Cosker, E., Schwan, R., Angioi-Duprez, K., Laprévote, V., and Schwitzer, T. (2020). New Insights on the Role of the Retina in Diagnostic and Therapeutic Strategies in Major Depressive Disorder. Neurosci. Biobehav Rev. 113, 262–272. doi:10.1016/j.neubiorev.2020.03.006
Couch, Y., Trofimov, A., Markova, N., Nikolenko, V., Steinbusch, H. W., Chekhonin, V., et al. (2016). Low-dose Lipopolysaccharide (LPS) Inhibits Aggressive and Augments Depressive Behaviours in a Chronic Mild Stress Model in Mice. J. Neuroinflammation 13, 108–125. doi:10.1186/s12974-016-0572-0
Duman, R. S., Aghajanian, G. K., Sanacora, G., and Krystal, J. H. (2016). Synaptic Plasticity and Depression: New Insights from Stress and Rapid-Acting Antidepressants. Nat. Med. 22, 238–249. doi:10.1038/nm.4050
Fan, F., Yang, L., Li, R., Zou, X., Li, N., Meng, X., et al. (2020). Salidroside as a Potential Neuroprotective Agent for Ischemic Stroke: a Review of Sources, Pharmacokinetics, Mechanism and Safety. Biomed. Pharmacother. 129, 110458. doi:10.1016/j.biopha.2020.110458
Fann, D. Y., Lee, S. Y., Manzanero, S., Tang, S. C., Gelderblom, M., Chunduri, P., et al. (2013). Intravenous Immunoglobulin Suppresses NLRP1 and NLRP3 Inflammasome-Mediated Neuronal Death in Ischemic Stroke. Cell Death Dis 4, e790. doi:10.1038/cddis.2013.326
Felger, J. C. (2018). Imaging the Role of Inflammation in Mood and Anxiety-Related Disorders. Curr. Neuropharmacol 16, 533–558. doi:10.2174/1570159X15666171123201142
Galecki, P., and Talarowska, M. (2018). Inflammatory Theory of Depression. Psychiatria Polska 52, 437–447. doi:10.12740/pp/76863
Gao, J., He, H., Jiang, W., Chang, X., Zhu, L., Luo, F., et al. (2015). Salidroside Ameliorates Cognitive Impairment in a D-Galactose-Induced Rat Model of Alzheimer's Disease. Behav. Brain Res. 293, 27–33. doi:10.1016/j.bbr.2015.06.045
Grech-Baran, M., Sykłowska-Baranek, K., and Pietrosiuk, A. (2015). Biotechnological Approaches to Enhance Salidroside, Rosin and its Derivatives Production in Selected Rhodiola Spp. In Vitro Cultures. Phytochem. Rev. 14, 657–674. doi:10.1007/s11101-014-9368-y
Guan, Y., and Han, F. (2020). Key Mechanisms and Potential Targets of the NLRP3 Inflammasome in Neurodegenerative Diseases. Front. Integr. Neurosci. 14, 37–46. doi:10.3389/fnint.2020.00037
Haapakoski, R., Mathieu, J., Ebmeier, K. P., Alenius, H., and Kivimäki, M. (2015). Cumulative Meta-Analysis of Interleukins 6 and 1β, Tumour Necrosis Factor α and C-Reactive Protein in Patients with Major Depressive Disorder. Brain Behav. Immun. 49, 206–215. doi:10.1016/j.bbi.2015.06.001
Haase, J., and Brown, E. (2015). Integrating the Monoamine, Neurotrophin and Cytokine Hypotheses of Depression-Aa central Role for the Serotonin Transporter? Pharmacol. Ther. 147, 1–11. doi:10.1016/j.pharmthera.2014.10.002
He, Y., Hara, H., and Núñez, G. (2016). Mechanism and Regulation of NLRP3 Inflammasome Activation. Trends Biochem. Sci. 41, 1012–1021. doi:10.1016/j.tibs.2016.09.002
Hu, R., Wang, M. Q., Ni, S. H., Wang, M., Liu, L. Y., You, H. Y., et al. (2020). Salidroside Ameliorates Endothelial Inflammation and Oxidative Stress by Regulating the AMPK/NF-κB/NLRP3 Signaling Pathway in AGEs-Induced HUVECs. Eur. J. Pharmacol. 867, 172797. doi:10.1016/j.ejphar.2019.172797
Huang, Y., Smith, D. E., Ibáñez-Sandoval, O., Sims, J. E., and Friedman, W. J. (2011). Neuron-specific Effects of Interleukin-1β Are Mediated by a Novel Isoform of the IL-1 Receptor Accessory Protein. J. Neurosci. 31, 18048–18059. doi:10.1523/JNEUROSCI.4067-11.2011
Jin, H., Pei, L., Shu, X., Yang, X., Yan, T., Wu, Y., et al. (2016). Therapeutic Intervention of Learning and Memory Decays by Salidroside Stimulation of Neurogenesis in Aging. Mol. Neurobiol. 53, 851–866. doi:10.1007/s12035-014-9045-6
Jorgensen, I., Rayamajhi, M., and Miao, E. A. (2017). Programmed Cell Death as a Defence against Infection. Nat. Rev. Immunol. 17, 151–164. doi:10.1038/nri.2016.147
Kahlenberg, J. M., Lundberg, K. C., Kertesy, S. B., Qu, Y., and Dubyak, G. R. (2005). Potentiation of Caspase-1 Activation by the P2X7 Receptor Is Dependent on TLR Signals and Requires NF-kappaB-Driven Protein Synthesis. J. Immunol. 175, 7611–7622. doi:10.4049/jimmunol.175.11.7611
Kanellopoulos, J. M., and Delarasse, C. (2019). Pleiotropic Roles of P2X7 in the Central Nervous System. Front Cell Neurosci 13, 401–419. doi:10.3389/fncel.2019.00401
Karasawa, A., and Kawate, T. (2016). Structural Basis for Subtype-specific Inhibition of the P2X7 Receptor. Elife 5. doi:10.7554/eLife.22153
Kaufmann, F. N., Costa, A. P., Ghisleni, G., Diaz, A. P., Rodrigues, A. L. S., Peluffo, H., et al. (2017). NLRP3 Inflammasome-Driven Pathways in Depression: Clinical and Preclinical Findings. Brain Behav. Immun. 64, 367–383. doi:10.1016/j.bbi.2017.03.002
Keller, J., Gomez, R., Williams, G., Lembke, A., Lazzeroni, L., Murphy, G. M., et al. (2017). HPA axis in Major Depression: Cortisol, Clinical Symptomatology and Genetic Variation Predict Cognition. Mol. Psychiatry 22, 527–536. doi:10.1038/mp.2016.120
Kelley, N., Jeltema, D., Duan, Y., and He, Y. (2019). The NLRP3 Inflammasome: An Overview of Mechanisms of Activation and Regulation. Int. J. Mol. Sci. 20. doi:10.3390/ijms20133328
Khan, A., Shal, B., Naveed, M., Nasir, B., Irshad, N., Ali, H., et al. (2020). Matrine Alleviates Neurobehavioral Alterations via Modulation of JNK-Mediated Caspase-3 and BDNF/VEGF Signaling in a Mouse Model of Burn Injury. Psychopharmacology (Berl) 237, 2327–2343. doi:10.1007/s00213-020-05537-5
Kinlein, S. A., Phillips, D. J., Keller, C. R., and Karatsoreos, I. N. (2019). Role of Corticosterone in Altered Neurobehavioral Responses to Acute Stress in a Model of Compromised Hypothalamic-Pituitary-Adrenal axis Function. Psychoneuroendocrinology 102, 248–255. doi:10.1016/j.psyneuen.2018.12.010
Korcok, J., Raimundo, L. N., Ke, H. Z., Sims, S. M., and Dixon, S. J. (2004). Extracellular Nucleotides Act through P2X7 Receptors to Activate NF-kappaB in Osteoclasts. J. Bone Miner Res. 19, 642–651. doi:10.1359/JBMR.040108
Li, D. X., Wang, C. N., Wang, Y., Ye, C. L., Jiang, L., Zhu, X. Y., et al. (2020). NLRP3 Inflammasome-dependent Pyroptosis and Apoptosis in hippocampus Neurons Mediates Depressive-like Behavior in Diabetic Mice. Behav. Brain Res. 391, 112684. doi:10.1016/j.bbr.2020.112684
Li, L., Lu, B., Fan, Q., Wei, L., Wu, J., Hou, J., et al. (2016a). Synthesis and pH-Responsive Self-Assembly Behavior of a Fluorescent Amphiphilic Triblock Copolymer mPEG-B-PCL-B-PDMAEMA-G-PC for the Controlled Intracellular Delivery of Doxorubicin. RSC Adv. 6, 27102–27112. doi:10.1039/c6ra01504b
Li, M., Zhou, J., Qian, J., Cheng, X., Wu, H., Li, L., et al. (2016b). Target Genes Involved in Corticosterone-Induced PC12 Cell Viability and Neurite Disorders: A Potential Molecular Mechanism of Major Depressive Disorder. Psychiatry Res. 235, 206–208. doi:10.1016/j.psychres.2015.11.044
Li, S., Wu, Y., Yang, D., Wu, C., Ma, C., Liu, X., et al. (2019). Gasdermin D in Peripheral Myeloid Cells Drives Neuroinflammation in Experimental Autoimmune Encephalomyelitis. J. Exp. Med. 216, 2562–2581. doi:10.1084/jem.20190377
Li, W., Ali, T., Zheng, C., Liu, Z., He, K., Shah, F. A., et al. (2021). Fluoxetine Regulates eEF2 Activity (Phosphorylation) via HDAC1 Inhibitory Mechanism in an LPS-Induced Mouse Model of Depression. J. Neuroinflammation 18, 38–57. doi:10.1186/s12974-021-02091-5
Liang, F., Zhang, F., Zhang, L., and Wei, W. (2020). The Advances in Pyroptosis Initiated by Inflammasome in Inflammatory and Immune Diseases. Inflamm. Res. 69, 159–166. doi:10.1007/s00011-020-01315-3
Malhi, G. S., and Mann, J. J. (2018). Depression. Lancet 392, 2299–2312. doi:10.1016/s0140-6736(18)31948-2
Mao, Q. Q., Huang, Z., Ip, S. P., Xian, Y. F., and Che, C. T. (2012). Protective Effects of Piperine against Corticosterone-Induced Neurotoxicity in PC12 Cells. Cell Mol Neurobiol 32, 531–537. doi:10.1007/s10571-011-9786-y
McFerran, B. W., Graham, M. E., and Burgoyne, R. D. (1998). Neuronal Ca2+ Sensor 1, the Mammalian Homologue of Frequenin, Is Expressed in Chromaffin and PC12 Cells and Regulates Neurosecretion from Dense-Core Granules. J. Biol. Chem. 273, 22768–22772. doi:10.1074/jbc.273.35.22768
Ng, A., Tam, W. W., Zhang, M. W., Ho, C. S., Husain, S. F., McIntyre, R. S., et al. (2018). IL-1β, IL-6, TNF- α and CRP in Elderly Patients with Depression or Alzheimer's Disease: Systematic Review and Meta-Analysis. Sci. Rep. 8, 12050–12062. doi:10.1038/s41598-018-30487-6
O'Connor, J. C., Lawson, M. A., André, C., Moreau, M., Lestage, J., Castanon, N., et al. (2009). Lipopolysaccharide-induced Depressive-like Behavior Is Mediated by Indoleamine 2,3-dioxygenase Activation in Mice. Mol. Psychiatry 14, 511–522. doi:10.1038/sj.mp.4002148
Pallavi, P., Sagar, R., Mehta, M., Sharma, S., Subramanium, A., Shamshi, F., et al. (2015). Serum Cytokines and Anxiety in Adolescent Depression Patients: Gender Effect. Psychiatry Res. 229, 374–380. doi:10.1016/j.psychres.2015.06.036
Palmeri, A., Mammana, L., Tropea, M. R., Gulisano, W., and Puzzo, D. (2016). Salidroside, a Bioactive Compound of Rhodiola Rosea, Ameliorates Memory and Emotional Behavior in Adult Mice. J. Alzheimers Dis. 52, 65–75. doi:10.3233/JAD-151159
Panossian, A., Nikoyan, N., Ohanyan, N., Hovhannisyan, A., Abrahamyan, H., Gabrielyan, E., et al. (2008). Comparative Study of Rhodiola Preparations on Behavioral Despair of Rats. Phytomedicine 15, 84–91. doi:10.1016/j.phymed.2007.10.003
Park, S. C. (2019). Neurogenesis and Antidepressant Action. Cell Tissue Res 377, 95–106. doi:10.1007/s00441-019-03043-5
Penninx, B. W., Kritchevsky, S. B., Yaffe, K., Newman, A. B., Simonsick, E. M., Rubin, S., et al. (2003). Inflammatory Markers and Depressed Mood in Older Persons: Results from the Health, Aging and Body Composition Study. Biol. Psychiatry 54, 566–572. doi:10.1016/s0006-3223(02)01811-5
Phillips, C. (20172017). Brain-Derived Neurotrophic Factor, Depression, and Physical Activity: Making the Neuroplastic Connection. Neural Plast. 2017, 7260130. doi:10.1155/2017/7260130
Porsolt, R. D., Bertin, A., and Jalfre, M. (1977). Behavioral Despair in Mice: a Primary Screening Test for Antidepressants. Arch. Int. Pharmacodyn Ther. 229, 327–336.
Ramaswamy, V., John, G. W., Sinclair, D. B., Edward, J., Richard, T-W., Wheatley, B. M., et al. (2013). Inflammasome Induction in Rasmussen's Encephalitis: Cortical and Associated white Matter Pathogenesis. J. Neuroinflammation 10, 152–162. doi:10.1186/1742-2094-10-152
Ross, F. M., Allan, S. M., Rothwell, N. J., and Verkhratsky, A. (2003). A Dual Role for Interleukin-1 in LTP in Mouse Hippocampal Slices. J. Neuroimmunol 144, 61–67. doi:10.1016/j.jneuroim.2003.08.030
Russo, A. J., Vasudevan, S. O., Méndez-Huergo, S. P., Kumari, P., Menoret, A., Duduskar, S., et al. (2021). Intracellular Immune Sensing Promotes Inflammation via Gasdermin D-Driven Release of a Lectin Alarmin. Nat. Immunol. 22, 154–165. doi:10.1038/s41590-020-00844-7
Seeliger, D., and de Groot, B. L. (2010). Ligand Docking and Binding Site Analysis with PyMOL and Autodock/Vina. J. Comput. Aided Mol. Des. 24, 417–422. doi:10.1007/s10822-010-9352-6
Sekio, M., and Seki, K. (2014). Lipopolysaccharide-induced Depressive-like Behavior Is Associated with α₁-adrenoceptor Dependent Downregulation of the Membrane GluR1 Subunit in the Mouse Medial Prefrontal Cortex and Ventral Tegmental Area. Int. J. Neuropsychopharmacol. 18, pyu005. doi:10.1093/ijnp/pyu005
Shal, B., Khan, A., Naveed, M., Ullah Khan, N., Ihsan-Ul-Haq, H., D AlSharari, S., et al. (2019). Effect of 25-methoxy Hispidol A Isolated from Poncirus Trifoliate against Bacteria-Induced Anxiety and Depression by Targeting Neuroinflammation, Oxidative Stress and Apoptosis in Mice. Biomed. Pharmacother. 111, 209–223. doi:10.1016/j.biopha.2018.12.047
Shi, J., Zhao, Y., Wang, K., Shi, X., Wang, Y., Huang, H., et al. (2015). Cleavage of GSDMD by Inflammatory Caspases Determines Pyroptotic Cell Death. Nature 526, 660–665. doi:10.1038/nature15514
Song, L., Pei, L., Yao, S., Wu, Y., and Shang, Y. (2017). NLRP3 Inflammasome in Neurological Diseases, from Functions to Therapies. Front. Cell Neurosci 11, 63. doi:10.3389/fncel.2017.00063
Su, M. H., Chen, H. C., Lu, M. L., Feng, J., Chen, I. M., Wu, C. S., et al. (2018). Risk Profiles of Personality Traits for Suicidality Among Mood Disorder Patients and Community Controls. Acta Psychiatr. Scand. 137, 30–38. doi:10.1111/acps.12834
Su, W. J., Zhang, Y., Chen, Y., Gong, H., Lian, Y. J., Peng, W., et al. (2017). NLRP3 Gene Knockout Blocks NF-Κb and MAPK Signaling Pathway in CUMS-Induced Depression Mouse Model. Behav. Brain Res. 322, 1–8. doi:10.1016/j.bbr.2017.01.018
Sun, Y., Huang, W.-m., Tang, P.-c., Zhang, X., Zhang, X.-y., Yu, B.-c., et al. (2020). Neuroprotective Effects of Natural Cordycepin on LPS-Induced Parkinson's Disease through Suppressing TLR4/NF-Κb/nlrp3-Mediated Pyroptosis. J. Funct. Foods 75, 104274–104287. doi:10.1016/j.jff.2020.104274
Swanson, K. V., Deng, M., and Ting, J. P. (2019). The NLRP3 Inflammasome: Molecular Activation and Regulation to Therapeutics. Nat. Rev. Immunol. 19, 477–489. doi:10.1038/s41577-019-0165-0
Taliaz, D., Stall, N., Dar, D. E., and Zangen, A. (2010). Knockdown of Brain-Derived Neurotrophic Factor in Specific Brain Sites Precipitates Behaviors Associated with Depression and Reduces Neurogenesis. Mol. Psychiatry 15, 80–92. doi:10.1038/mp.2009.67
Thawkar, B. S., and Kaur, G. (2019). Inhibitors of NF-Κb and P2X7/NLRP3/Caspase 1 Pathway in Microglia: Novel Therapeutic Opportunities in Neuroinflammation Induced Early-Stage Alzheimer's Disease. J. Neuroimmunol 326, 62–74. doi:10.1016/j.jneuroim.2018.11.010
Thomas, A. J., Davis, S., Morris, C., Jackson, E., Harrison, R., and O'Brien, J. T. (2005). Increase in Interleukin-1beta in Late-Life Depression. Am. J. Psychiatry 162, 175–177. doi:10.1176/appi.ajp.162.1.175
Troubat, R., Barone, P., Leman, S., Desmidt, T., Cressant, A., Atanasova, B., et al. (2020). Neuroinflammation and Depression: A Review. Eur. J. Neurosci. 53, 151–171. doi:10.1111/ejn.14720
Vasileva, L. V., Saracheva, K. E., Ivanovska, M. V., Petrova, A. P., Marchev, A. S., Georgiev, M. I., et al. (2018). Antidepressant-like Effect of Salidroside and Curcumin on the Immunoreactivity of Rats Subjected to a Chronic Mild Stress Model. Food Chem. Toxicol. 121, 604–611. doi:10.1016/j.fct.2018.09.065
Voet, S., Srinivasan, S., Lamkanfi, M., and van Loo, G. (2019). Inflammasomes in Neuroinflammatory and Neurodegenerative Diseases. EMBO Mol. Med. 11. doi:10.15252/emmm.201810248
Wang, D., Wang, H., Gao, H., Zhang, H., Zhang, H., Wang, Q., et al. (2020a). P2X7 Receptor Mediates NLRP3 Inflammasome Activation in Depression and Diabetes. Cell Biosci 10, 28–37. doi:10.1186/s13578-020-00388-1
Wang, H., Li, Q., Sun, S., and Chen, S. (2020b). Neuroprotective Effects of Salidroside in a Mouse Model of Alzheimer's Disease. Cell Mol Neurobiol 40, 1133–1142. doi:10.1007/s10571-020-00801-w
Wang, S., He, H., Chen, L., Zhang, W., Zhang, X., and Chen, J. (2015). Protective Effects of Salidroside in the MPTP/MPP(+)-Induced Model of Parkinson's Disease through ROS-NO-Related Mitochondrion Pathway. Mol. Neurobiol. 51, 718–728. doi:10.1007/s12035-014-8755-0
Wang, S., Yuan, Y. H., Chen, N. H., and Wang, H. B. (2019). The Mechanisms of NLRP3 Inflammasome/pyroptosis Activation and Their Role in Parkinson's Disease. Int. Immunopharmacol 67, 458–464. doi:10.1016/j.intimp.2018.12.019
Ward, R., Li, W., Abdul, Y., Jackson, L., Dong, G., Jamil, S., et al. (2019). NLRP3 Inflammasome Inhibition with MCC950 Improves Diabetes-Mediated Cognitive Impairment and Vasoneuronal Remodeling after Ischemia. Pharmacol. Res. 142, 237–250. doi:10.1016/j.phrs.2019.01.035
Wen, H., Ting, J. P., and O'Neill, L. A. (2012). A Role for the NLRP3 Inflammasome in Metabolic Diseases-Ddid Warburg Miss Inflammation? Nat. Immunol. 13, 352–357. doi:10.1038/ni.2228
Ye, T., Meng, X., Zhai, Y., Xie, W., Wang, R., Sun, G., et al. (2018). Gastrodin Ameliorates Cognitive Dysfunction in Diabetes Rat Model via the Suppression of Endoplasmic Reticulum Stress and NLRP3 Inflammasome Activation. Front. Pharmacol. 9, 1346–1359. doi:10.3389/fphar.2018.01346
Yirmiya, R., Winocur, G., and Goshen, I. (2002). Brain Interleukin-1 Is Involved in Spatial Memory and Passive Avoidance Conditioning. Neurobiol. Learn. Mem. 78, 379–389. doi:10.1006/nlme.2002.4072
Yokoyama, R., Higuchi, M., Tanabe, W., Tsukada, S., Naito, M., Yamaguchi, T., et al. (2020). (S)-norketamine and (2S,6S)-Hydroxynorketamine Exert Potent Antidepressant-like Effects in a Chronic Corticosterone-Induced Mouse Model of Depression. Pharmacol. Biochem. Behav. 191, 172876. doi:10.1016/j.pbb.2020.172876
Yue, N., Huang, H., Zhu, X., Han, Q., Wang, Y., Li, B., et al. (2017). Activation of P2X7 Receptor and NLRP3 Inflammasome Assembly in Hippocampal Glial Cells Mediates Chronic Stress-Induced Depressive-like Behaviors. J. Neuroinflammation 14, 102–117. doi:10.1186/s12974-017-0865-y
Zhang, K., Liu, J., You, X., Kong, P., Song, Y., Cao, L., et al. (2016). P2X7 as a New Target for Chrysophanol to Treat Lipopolysaccharide-Induced Depression in Mice. Neurosci. Lett. 613, 60–65. doi:10.1016/j.neulet.2015.12.043
Zhang, X., Du, Q., Yang, Y., Wang, J., Liu, Y., Zhao, Z., et al. (2018). Salidroside Alleviates Ischemic Brain Injury in Mice with Ischemic Stroke through Regulating BDNK Mediated PI3K/Akt Pathway. Biochem. Pharmacol. 156, 99–108. doi:10.1016/j.bcp.2018.08.015
Zhang, X., Zhang, P., An, L., Sun, N., Peng, L., Tang, W., et al. (2020). Miltirone Induces Cell Death in Hepatocellular Carcinoma Cell through GSDME-dependent Pyroptosis. Acta Pharm. Sin B 10, 1397–1413. doi:10.1016/j.apsb.2020.06.015
Zhang, Y., Liu, L., Liu, Y. Z., Shen, X. L., Wu, T. Y., Zhang, T., et al. (2015). NLRP3 Inflammasome Mediates Chronic Mild Stress-Induced Depression in Mice via Neuroinflammation. Int. J. Neuropsychopharmacol. 18, pyv006. doi:10.1093/ijnp/pyv006
Zhang, Y., Liu, L., Peng, Y. L., Liu, Y. Z., Wu, T. Y., Shen, X. L., et al. (2014). Involvement of Inflammasome Activation in Lipopolysaccharide-Induced Mice Depressive-like Behaviors. CNS Neurosci. Ther. 20, 119–124. doi:10.1111/cns.12170
Zhao, X., Cao, F., Liu, Q., Li, X., Xu, G., Liu, G., et al. (2019). Behavioral, Inflammatory and Neurochemical Disturbances in LPS and UCMS-Induced Mouse Models of Depression. Behav. Brain Res. 364, 494–502. doi:10.1016/j.bbr.2017.05.064
Zhao, Y., Ma, R., Shen, J., Su, H., Xing, D., and Du, L. (2008). A Mouse Model of Depression Induced by Repeated Corticosterone Injections. Eur. J. Pharmacol. 581, 113–120. doi:10.1016/j.ejphar.2007.12.005
Zheng, M., Liu, C., Pan, F., Shi, D., Ma, F., Zhang, Y., et al. (2011). Protective Effects of Flavonoid Extract from Apocynum Venetum Leaves against Corticosterone-Induced Neurotoxicity in PC12 Cells. Cell Mol Neurobiol 31, 421–428. doi:10.1007/s10571-010-9635-4
Zheng, Z.-H., Tu, J.-L., Li, X.-H., Hua, Q., Liu, W.-Z., Liu, Y., et al. (2021). Neuroinflammation Induces Anxiety- and Depressive-like Behavior by Modulating Neuronal Plasticity in the Basolateral Amygdala. Brain Behav. Immun. 91, 505–518. doi:10.1016/j.bbi.2020.11.007
Keywords: salidroside, depression, pyroptosis, NLRP3, P2X7
Citation: Chai Y, Cai Y, Fu Y, Wang Y, Zhang Y, Zhang X, Zhu L, Miao M and Yan T (2022) Salidroside Ameliorates Depression by Suppressing NLRP3-Mediated Pyroptosis via P2X7/NF-κB/NLRP3 Signaling Pathway. Front. Pharmacol. 13:812362. doi: 10.3389/fphar.2022.812362
Received: 10 November 2021; Accepted: 28 March 2022;
Published: 12 April 2022.
Edited by:
Francisco Lopez-Munoz, Camilo José Cela University, SpainReviewed by:
Sabrina F. Lisboa, University of São Paulo, BrazilCopyright © 2022 Chai, Cai, Fu, Wang, Zhang, Zhang, Zhu, Miao and Yan. This is an open-access article distributed under the terms of the Creative Commons Attribution License (CC BY). The use, distribution or reproduction in other forums is permitted, provided the original author(s) and the copyright owner(s) are credited and that the original publication in this journal is cited, in accordance with accepted academic practice. No use, distribution or reproduction is permitted which does not comply with these terms.
*Correspondence: Lingpeng Zhu, emh1bGluZ3BlbmdAbmptdS5lZHUuY24=; Mingxing Miao, bW14MDIyNEAxNjMuY29t; Tianhua Yan, MTAyMDA1MDgwNkBjcHUuZWR1LmNu
†These authors have contributed equally to this work
Disclaimer: All claims expressed in this article are solely those of the authors and do not necessarily represent those of their affiliated organizations, or those of the publisher, the editors and the reviewers. Any product that may be evaluated in this article or claim that may be made by its manufacturer is not guaranteed or endorsed by the publisher.
Research integrity at Frontiers
Learn more about the work of our research integrity team to safeguard the quality of each article we publish.