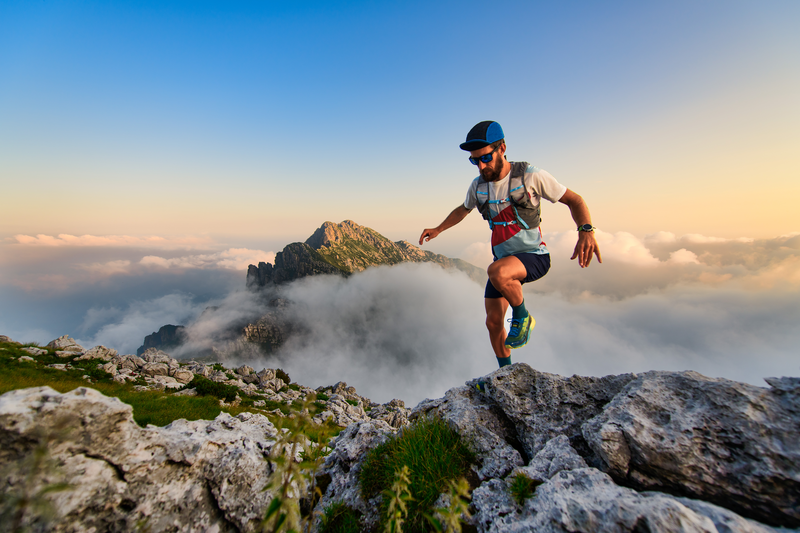
94% of researchers rate our articles as excellent or good
Learn more about the work of our research integrity team to safeguard the quality of each article we publish.
Find out more
REVIEW article
Front. Pharmacol. , 24 February 2022
Sec. Cardiovascular and Smooth Muscle Pharmacology
Volume 13 - 2022 | https://doi.org/10.3389/fphar.2022.810587
This article is part of the Research Topic Novel Perspectives For The Therapy And Prevention Of Heart Failure View all 13 articles
Heart failure (HF) is one of the main public health problems at present. Although some breakthroughs have been made in the treatment of HF, the mortality rate remains very high. However, we should also pay attention to improving the quality of life of patients with HF. Traditional Chinese medicine (TCM) has a long history of being used to treat HF. To demonstrate the clinical effects and mechanisms of TCM, we searched published clinical trial studies and basic studies. The search results showed that adjuvant therapy with TCM might benefit patients with HF, and its mechanism may be related to microvascular circulation, myocardial energy metabolism, oxidative stress, and inflammation.
Heart failure (HF) is a clinical syndrome characterized by with dyspnea, fatigue, and hydroncus, which is caused by decreased cardiac output or elevated endocardial pressure resulting from a change in the cardiac structure or function (McDonagh et al., 2021). As shown in the 2017 Global Burden of Disease Study, the number of patients with HF is about 64.34 million, which makes HF an epidemic public health problem (Disease et al., 2018). The morbidity of HF in adults from developed countries is between 1 and 2% (Groenewegen et al., 2020). Data from the USA in 2019 showed that 6.2 million people aged above 20 years old have HF, and HF morbidity is estimated to increase by 46% from 2012 to 2030 (Benjamin et al., 2019). The 2012–2015 China Hypertension Survey found that the morbidity of HF in adults aged 35 years and over was 1.3% (Wang Z et al., 2018). Moreover, the incidence of heart failure increases with age. Data from the Heart Failure Association of the European Society indicates that 1-year all-cause mortality of acute heart failure (AHF) and chronic heart failure (CHF) are 23.6 and 6.4%, respectively (Crespo-Leiro et al., 2016). The INTERnational Congestive Heart Failure (INTER-CHF) prospective cohort study showed that HF 1-year all-cause mortality was 16.5%, which in Africa was 34%, in Southeast Asia was 15%, in China was 7%, in South America was 9%, and in the Middle East was 9% (Dokainish et al., 2017).
In clinical practice, HF can be divided into HF with reduced ejection fraction [HFrEF, left ventricular ejection fraction (LVEF) ≤ 40%] and HF with preserved ejection fraction (HFpEF, LVEF ≥ 50%) based on the LVEF (Bozkurt et al., 2021). Research into the treatment of HFrEF has formed a new quadruple chemotherapy consisting of angiotensin-converting enzyme inhibitors, beta-blockers, mineralocorticoid receptor antagonists, and sodium-dependent glucose transporters 2 inhibitors in medication (McMurray et al., 2014; McMurray et al., 2019; Packer et al., 2020). Although these treatments show prognostic benefits, the 5-years mortality after hospitalization of patients with HFrEF is still high, even up to 75.3% (Shah et al., 2017). Among all research aimed at HFpEF published to date, only the EMPEROR-Preserved Trial proved that medicine could improve prognosis (Anker et al., 2021). Previous guidelines defined HF (LVEF = 41–49%) as HF with a mid-range ejection fraction (Ponikowski et al., 2016). Meanwhile, the latest European Society of Cardiology guidelines defined it as HF with mildly reduced ejection fraction (HFmrEF); however, we lack prospective experimental evidence for patients with HFmrEF. All analyses and suggestions are based on post hoc analyses of HFrEF and HFpEF (McDonagh et al., 2021). Therefore, the treatment of HF still faces great challenges. One the one hand, we need to focus on improving life qualities of patients with HF, in addition to its notable morbidity and mortality (Fiuzat et al., 2020). On the other hand, medicine exploitation needs new therapeutic targets, such as microvascular circulation, myocardial energy metabolism, inflammation, and mitochondrial function (Reddy et al., 2020; Ghionzoli et al., 2021).
Traditional Chinese Medicine (TCM), based on its own theory, has resulted in Chinese medicinal herbs being used widely in the therapy of HF. For example, many anti-HF prescriptions are recorded in textbooks (such as Zhenwu decoction, Shengmai powder, and Lingguizhugan decoction), while only a few of them have been studied strictly (Fu et al., 2010). Chinese medicinal herbs have been used for a long time to cure heart failure. By contrast, research into the effects and mechanisms of TCM are still in the initial stage. In this article, we summarize recent development in this field, with the aim of clarifying the mechanisms of Chinese medicinal herbs in therapy for HF and to provide new directions for the development and clinical application of HF therapeutic drugs.
Considering the large number of studies about TCM treatment in HF, we only searched two databases, the Chinese Clinical Trial Registry (http://www.chictr.org.cn) and ClinicalTrials.gov (http://www.clinicaltrials.gov), and then obtained relevant data from the China National Knowledge Infrastructure (https://www.cnki.net/) and PubMed (https://pubmed.ncbi.nlm.nih.gov/), which resulted in 13 studies being selected (Table 1). The search date was August 28, 2021. Two investigators (Anzhu Wang and Pingping Huang) screened the literature separately, and a third investigator (Xiao-Chang Ma) checked the search results. Two investigators (Anzhu Wang and Pingping Huang) used the Cochrane risk of bias assessment tool to assess the risk of bias for the included studies. Disagreements in the process were resolved through discussion and consultation with a third investigator (Xiaochang Ma). The search process and quality assessment were placed in Supplementary Figures S1, S2.
In 2013, Li et al. (2013) reported a multicenter, randomized, double-blind, parallel-group, placebo-controlled study on the efficacy and safety of Qili Qiangxin capsules in 512 patients with CHF. After 12 weeks of treatment, the level of N-terminal pro-B-type natriuretic peptide (NT-proBNP) in the two groups was significantly lower than baseline. However, reduction in the Qili Qiangxin capsules group was markedly larger than that in placebo group [240.15 pg/ml (−23.15, 1113.85) vs. 0.00 pg/ml (−286.00, 800.00), p = 0.002]. The therapeutic effects of Qili Qiangxin capsules were clearly better than that of the placebo (p < 0.05) in terms of composite cardiac events, New York Heart Association (NYHA) functional classification, LVEF, 6-min walking distance (6MWD), and Quality of life assessment using the Minnesota Living with Heart Failure Questionnaire (MLHFQ). In terms of safety, the differences between two groups for serious adverse events (SAEs) and adverse events (AEs) were not statistically significant (p < 0.05). Elevated levels of circulating NT-proBNP contribute to the diagnosis of HF and are linked to increased mortality and morbidity in HF patients (McDonagh et al., 2021). The findings revealed that Qili Qiangxin capsules can significantly lower NT-proBNP levels, indicating that patients may have a better prognosis with long-term therapy. To test this theory, a large, randomized, controlled trial with all-cause death as the outcome is required.
In 2020, Mao et al. (2020) reported a prospective randomized, double-blind, multicenter, placebo-controlled study on the efficacy and safety of Qishen Yiqi dripping pills that enrolled 640 patients with ischemic heart failure (IHF). After 6 months of treatment, the level of 6MWD in the two groups was significantly higher than baseline. However, increase in the Qishen Yiqi dripping pills group was obviously greater than that in the placebo group (336.15 ± 100.84 m to 374.47 ± 103.09 m vs. 334.40 ± 100.27 m to 340.71 ± 104.57 m, p < 0.001). Compared with those in the placebo group, the MLHFQ grades of Qishen Yiqi dripping pills group were better and the NYHA functional classification was ameliorated (p < 0.05). Although Qishen Yiqi dripping pills increased LVEF and BNP during a 6-month period, the effects were not substantially larger than those seen in the placebo group. This was also linked to a lack of meaningful difference in composite clinical events at 6 months and 1 year (follow-up), despite a tendency for decreased HF hospitalizations at 6 months. To thoroughly assess the impact of Qishen Yiqi dripping pills on clinical events in IHF, longer-term medication and follow-up may be required. In terms of safety, AEs in the Qishen Yiqi dripping pills group were less common and milder.
In 2016, Xian et al. (2016) delivered a randomized, double-blind, multicenter, placebo-controlled study on the efficacy and safety of Shenmai injection in 240 patients with CHF. After 7 days of treatment, the levels of the NYHA functional classification in the two groups were lower than baseline. However, the proportion of improved patients in the Shenmai injection group was significantly bigger than placebo group (NYHA Ⅰ: 22.8 vs. 8.8%, NYHA Ⅱ: 12.3 vs. 14.9%, NYHA Ⅲ: 28.1 vs. 19.3%, and NYHA Ⅳ: 7.0 vs. 4.4%, p = 0.001). In 6MWD, the short-form 36 (SF-36) hearth survey score and the TCM syndrome score, Shenmai injection therapy was more effective than the placebo (p < 0.05). For safety, treatment with Shenmai injection within 1 week was well tolerated with no apparent safety concerns (p > 0.05). It is worth noting that, despite indications of improvements in these endpoints, there is no evidence in this trial to demonstrate any benefits in long-term results of Shenmai injection. If a long-term duration is envisaged, more detailed study should be conducted.
In 2020, Wang et al. (2020) initiated a controlled experiment in which 120 patients with HF requiring further treatment in the hospital were randomly assigned to one of three groups: Shenmai injection, trimetazidine, or placebo. After 7 days of treatment, compared with the control group, Shenmai injection could inhibit the production of free fatty acids (FFAs, 452.88 ± 226.62 vs. 571.42 ± 209.40 μmol/L, p < 0.05) and branched-chain amino acids (BCAAs, 0.55 ± 0.17 vs. 0.47 ± 0.17 nmol/μL, p < 0.05), improved the NYHA functional classification, and raised the level of TCM score associated brain natriuretic peptide (BNP, p < 0.05). The advantage of Shenmai injection in HF may be due to an improvement in energy metabolism that was more noticeable than that seen after trimetazidine therapy. However, the number of serological metabolic indexes was limited, and there were no direct associations between metabolic indexes and enhanced cardiac function, nor were there any links between metabolic indexes. As a result, greater study into changes in serological metabolism after Shenmai injection therapy in HF is needed to corroborate these findings.
In 2019, Wang et al. (2019) reported a randomized, double-blinded, multicenter, placebo-controlled trial of Shenfu Injection in 160 patients with CHF during the acute phase of symptom aggravation (Yang and Qi Deficiency Syndrome). After 7 days of treatment, compared with the control group, Shenfu Injection could improve the NYHA classification (78.38 vs. 61.43%, p = 0.0026), and increase the TCM syndrome score (89.19 vs. 60.00%, p < 0.001), Lee’s HF score, and 6MWD (p < 0.05). In terms of safety, treatment with Shenfu Injection within 1 week showed no statistical differences in the occurrence of AEs and adverse drug reactions (ADRs) (p > 0.05). However, this study was limited to a few places, and the observation duration was brief. Furthermore, we found no significant differences in several endpoint markers, such as composite cardiac events (CCEs) or fatalities, across these groups.
In 2011, Xu et al. (2011) reported a randomized controlled trial (RCT) of Huangqi injection in 128 patients with acute decompensated CHF(Qi deficiency syndrome). After 7 days of treatment, compared with the control group, Huangqi injection could improve the LVEF (37.98 ± 12.77 vs. 31.06 ± 10.36, p = 0.003), strengthen dyspnea, and increase the NYHA functional classification and Tei index (p < 0.05). In terms of safety, Huangqi injection was well tolerated, with no AEs. In addition, this trial designed the application dose of Huangqi injection at 40 ml/d, which was double the conventional dosage, suggesting that the application of Huangqi injection for the treatment of AHF can be increased, although fluid intake must be tightly regulated. Currently, there are no thorough pharmacodynamic and pharmacokinetic studies as well as large-scale RCTs on Huangqi injection for the treatment of HF, which need to be examined further.
In 2017, Wang et al. (2017b) reported a randomized, double-blind, multicenter placebo-controlled trial of Shensong Yangxin capsules in 465 patients with CHF with frequent ventricular premature complexes (VPCs). After 12 weeks of treatment, compared with the placebo group, the Shensong Yangxin capsules group had significantly fewer total number of VPCs on a 24-h ambulatory electrocardiogram (1538 ± 2187 vs. 2746 ± 3889, p < 0.05). In addition, it also increased NT-proBNP, LVEF, NYHA functional classification, 6MWD, and the MLHFQ score (p < 0.05). In terms of safety, the differences between two groups for SAEs were not statistically significant (p > 0.05). On the basis of conventional CHF therapy, Shensong Yangxin capsules were shown to have the advantages of reducing VPCs and enhancing cardiac function with good compliance in this research. However, because the frequency of VPCs varies daily or on a regular basis, a longer period of ECG, such as 72 h or even 7 days, is seen to be more compelling. Because the 12-weeks timeframe in this study was so short, a bigger size and longer duration of a well-designed clinical trial should be conducted for a more thorough examination of Shensong Yangxin capsules in CHF patients with VPCs.
In 2105, Xian et al. (2015) reported a randomized, double-blind, multicenter, placebo-controlled study of Yangxinkang Tablets in 228 patients with CHF. After 4 weeks of treatment, compared with the placebo group, Yangxinkang Tablets could improve the NYHA functional classification, Chinese medicine (CM) syndromes, the symptom score, the Sign Score, and quality of life measured by the MLHFQ score (p < 0.05). No prominent ADRs were noted during the study. Yangxinkang tablets were shown to relieve clinical symptoms in CHF patients in this trial, although the lack of laboratory markers made the findings less reliable.
In 2021, Li X. X et al. (2021) reported a single-center, prospective, randomized, controlled clinical trial of Qishen Taohong granules in 76 patients with CHF. After 4 weeks of treatment, compared with trimetazidine, the Qishen Taohong granules could improve the NYHA classification (74.29 vs. 54.29%, p < 0.05), quality of life measured using the CHF Integrated Chinese and Western Medicine Survival Scale (CHFQLS; 13.82 ± 6.04 vs. 7.49 ± 2.28, p < 0.05). Treatment with Qishen Taohong granules also showed a superior performance in 6MWT, CM syndrome, shortness of breath, fatigue, gasping, general edema, and NT-proBNP levels (p < 0.05). No remarkable AEs was revealed during the study. The findings of this study should be interpreted with care due to the small sample size and short observation period. Furthermore, because this study lacked quantitative markers directly connected to energy metabolism, it was unable to evaluate if improved qi deficiency symptoms equated to improved energy metabolism.
In 2020, Tang (2020) reported a multicenter, randomized, double-blind, placebo-controlled study of Yangxinxue granules in 108 patients with CHF. After 6 months of treatment, as well as 6 months of follow-up, although the differences between two groups for 1-year cardiovascular-related disease mortality were not statistically significant (13.33 vs. 6.52%, p > 0.05), the attack rate and rehospitalization rate were much lower for patients with AHF in the Yangxinxue granule group (p < 0.05). Moreover, the Yangxinxue granules could reduce the BNP level, and improve the 6MWT and MLHFQ scores (p < 0.05). No significant ADRs were noted during the study (p > 0.05). In addition to the standard treatment, Yangxinxue granules could significantly reduce the number of episodes of AHF and BNP levels, indicating that Yangxinxue granules had definite clinical efficacy in the treatment of CHF. The study included four research centers, but all were located in China’s Sichuan province and may not reflect regional differences.
In 2017, Wang et al. (2017a) reported a prospective, single-blind, randomized, controlled, and multicenter clinical trial of TCM preparations in 340 patients with CHF. During hospitalization, the control group received polarized solution and the treatment group received Danhong injection with Shenfu injection or Shenmai injection. After discharge, all patients were treated with Qiliqiangxin capsules and Buyiqiangxin tablets or a placebo for 6 months. After 6 months, the Kaplan-Meier curves revealed a significantly improved trend in the treatment group’s cumulative survival rate compared to the control group. Although the treatment group ceased taking TCM and got the same therapy as the control group after 6 months, the treatment group’s cumulative survival rate remained higher than the control group until the 12th month (p = 0.208). More research is needed to identify whether this favorable trend is the result of TCM’s delayed effect or of other reasons. There was no significant difference between the groups in BNP, Lee’s HF scores and LVEF (p > 0.05). The treatment group showed greater improvement in 6MWT and MLHFQ (p < 0.05). No significant ADRs were noted during the study (p > 0.05).
In 2020, Liu (2020) reported a randomized, controlled clinical trial of Yangyin Shuxin prescription in 60 patients with HFpEF. The study evaluated the diastolic function of patients using various methods including two-dimensional ultrasound echocardiography, pulsed-wave doppler, tissue doppler imaging and speckle tracking imaging. After 14 ± 3 days of treatment, the mean difference in untwisting rate was 0.14% (95% CI: 0.07–0.21%, p < 0.001) and the mean difference in left atrial volume index was −4.99 ml/m2 (95% CI: −7.09 to −2.99 ml/m2, p < 0.001) between the two groups on the basis of conventional treatment, suggesting that Yangyin Shuxin prescription could improve diastolic function in patients with HFpEF. This research’s diagnostic criteria correspond to various guidelines, but there were issues such as a limited number of patients, a short observation duration, and insufficient persuasive power of outcome indicators, necessitating a more well-designed clinical investigation.
In 2017, Gao et al. (2017) reported a randomized, double-blind controlled clinical trial of Chinese herbal medicine granules in 64 patients with HFpEF of Qi deficiency and blood stasis syndrome. After 28 days of treatment, compared with the placebo group, Chinese herbal medicine granules could improve the LVEF and 6MWT (p < 0.05). By comparing metabolic profiles, 9 biomarkers, including 2-arachidonoylglycerophosphocholine, lysophosphatidylethanolamine (LPE) 16:0, phosphatidylserine 21:0, LPE 20:4, LPE 18:0, linoleic acid, LPE 18:2, 4-hydroxybenzenesulfonic acid, and LPE 22:6, may be particularly important for the effect of Chinese herbal medicine granules. This study used a metabolomics approach to validate the efficacy of herbal medicines, which can provide ideas for other studies.
Ischemic heart disease (IHD) commonly leads to HF (Pagliaro et al., 2020). Besides atherosclerosis and obstructive atherothrombotic events in epicardial coronary arteries, myocardial ischemia also has a close connection with coronary microvascular dysfunction (CMD) (Marzilli et al., 2012; Ford et al., 2018). CMD, characterized by coronary flow reserve (CFR) injury, means that the structure and function of coronary microcirculation are affected (Padro et al., 2020). As for obstructive coronary syndrome, although revascularization could recover the blood flow, CMD in distal coronary vasculature might bring about limited or incomplete recovery of damaged cardiomyocytes. Furthermore, myocardial fibrosis and adverse ventricular remodeling might result in HfrEF (Elgendy et al., 2019). In contrast to HFrEF resulting from myocardial scar and following left ventricular dilatation, the pathophysiological mechanisms of HFpEF are more complicated (Lam et al., 2018). As a result of CMD, heterogeneity in blood flow and oxygen levels would increase. Long term of CFR injury would result in damage to cardiac muscle cells and interstitial fibrosis (Yang et al., 2020). Thus, a wealth of supporting evidence suggests that CMD is the potential mechanism HFpEF and is a therapeutic target (Shah et al., 2018; Taqueti et al., 2018; Ahmad et al., 2021). The endothelium plays an important part in CMD. Endothelial structure and function are distinct in different angiosomes, whereas all of them could secrete and regulate vascular tension and substance permeability, especially in heart muscle capillaries, which are connected directly with endothelial cells and adjacent cardiomyocytes (Brutsaert, 2003; Zuchi et al., 2020). In addition, CMD is also associated with smooth muscle cell dysfunction, microvascular spasm/sympathetic dysfunction and altered microvascular remodeling (Pries and Reglin, 2017).
Many TCM composite preparations include Astragulus embranaceus (Fisch.) Bge., which could affect endothelial function. Further research of the effective ingredient in Astragulus embranaceus (Fisch.) Bge. also proved its function (Tse et al., 2012; Hu et al., 2018). For example, Astragaloside IV (PubChem CID: 13943297) can not only improve vascular endothelial dysfunction induced by hyperglycemia via inhibiting the Toll-like receptor 4 (TLR4)/nuclear factor kappa-B(NF-κB) signaling pathway (Leng et al., 2018), but also inhibits vascular endothelial dysfunction via the phosphatidylinositol 3-kinase (PI3K)/protein kinase B (AKT)/endothelial nitric oxide synthase (eNOS) signaling pathway in rat aortic rings (Lin et al., 2018). Calycosin (PubChem CID: 5280448) can also promote angiogenesis, via a mechanism that involves vascular endothelial growth factor (VEGF)-vascular endothelial growth factor receptor 2 (VEGFR2) and mitogen-activated protein kinase (MAPK) signaling pathways (Tang et al., 2010; Li et al., 2011). Astragalus polysaccharide (PubChem CID: 2782115) can restrain apoptosis of human cardiac microvascular endothelial cells undergoing hypoxia and reoxygenation by activating the PI3K/AKT signaling pathway (Xie et al., 2016), (Table 2).
Ginsenoside Rg3 (PubChem CID: 9918693) isolated from Panax ginseng C. A. Mey. undertakes key roles of relaxing vessels and exerting a cytoprotective effect via induction of inducible nitric oxide synthase (iNOS) by activating NF-κB (Kim et al., 2003). Rg3 can antagonize adriamycin-induced cardiotoxicity by improving endothelial dysfunction via upregulating the nuclear factor-erythroid 2-related factor-2 (NRF2)-antioxidant responsive element (ARE) pathway through activation of AKT (Wang et al., 2015). Under normal cellular oxygen conditions in human umbilical vein endothelial cells, Ginsenoside Rg1 (PubChem CID: 441923) is a valid stimulator of hypoxia-inducible factor, which is an important transcription regulator for numerous angiogenic factors, through activating PI3K/AKT/ribosomal protein S6 kinase B1 (p70S6K) signaling (Leung et al., 2011), (Table 2).
Studies on effective ingredients of Salvia miltiorrhiza Bge. are numerous. The direct vasorelaxation induced by Tanshinone IIa (PubChem CID: 164676) is mediated by the nongenomic action of the estrogen receptor through endothelial nitric oxide synthase activation and Ca2+ mobilization (Fan et al., 2011). Tanshinone IIa also attenuates hypoxia/reoxygenation (H/R)-induced apoptosis via inhibiting the Janus kinase 2 (JAK2)/signal transducer and activator of transcription 3 (STAT3) signaling pathway (Cui et al., 2016) and protects human coronary artery endothelial cells from ferroptosis by activating the NRF2 pathway (He L et al., 2021). Ursolic acid (PubChem CID: 64945) exerts beneficial effects by upregulation of eNOS and a parallel downregulation of nicotinamide adenine dinucleotide phosphate (NADPH) oxidase 4 (NOX4), leading to an increase in bioactive nitric oxide (NO) (Steinkamp-Fenske et al., 2007). Magnesium lithospermate B (PubChem CID: 6918234) also can activate eNOS, which in turn enhances vascular nitric oxide production, which is medicated via the PI3K/AKT pathway (Liu Y. L et al., 2019), (Table 2).
Panax notoginseng saponins, which are the major active components of Panax notoginseng (Burk.) F. H. Chen, are a kind of chemical mixture containing different dammarane-type saponins, such as Notoginsenoside R1, and Ginsenosides Rb1, Rd, Re, and Rg1 (Xu et al., 2019). The pro-angiogenic and endothelial protection effects of Panax notoginseng saponins have been demonstrated in vitro and in vivo experimental models by upregulating sirtuin 1 (SIRT1) and antioxidants, and enhancing autophagy through phosphorylation of 5′-adenosine monophosphate-activated protein kinase (AMPK) Thr172 and calcium/calmodulin-dependent protein kinase II (CaMKII) Thr287, and eNOS-dependent pathways (Wang D et al., 2017; Bo et al., 2020; Wang D et al., 2021). Notoginsenoside R1 (PubChem CID: 441934) plays an important role among Panax notoginseng saponin active component by activating the angiogenin 2 (ANG2)/tyrosine kinase with immunoglobin and epidermal growth factor homology domain 2 (TIE2) pathway (Zhong et al., 2020) and PI3K/AKT pathway (Fang et al., 2018) to promote angiogenesis and activating NRF2 in endothelial cells to suppressing monocyte adhesion (Fan et al., 2016), (Table 2).
Tetramethylpyrazine (PubChem CID: 14296), the predominant active ingredient in Ligusticum chuanxiong Hort. or Schisandra sphenantheraRehd.et Wils., can suppress angiotensin II-induced soluble epoxide hydrolase (sEH) expression in the coronary endothelium via an anti-endoplasmic reticulum (ER) stress mechanism (Mak et al., 2017) and attenuates the endotheliotoxicity of doxorubicin via tyrosine 3-monooxygenase/tryptophan 5-monooxygenase activation protein gamma (14-3-3γ)/B-cell lymphoma-2 (BCL-2) (Yang et al., 2019). Gomisin A (PubChem CID: 634470) contained in Schisandra chinensis (Turcz.) Baill. induces Ca2+-dependent activation of eNOS in human coronary artery endothelial cells, events that are linked to NO production and thereby endothelial-dependent vasorelaxation (Park et al., 2009). Saikosaponin C (PubChem CID: 131801344) in Bupleurum chinense DC can induce endothelial cell growth, migration, and capillary tube formation via activating MMP-2, VEGF, MAPK, and ERK (Shyu et al., 2004), (Table 2).
The heart is an organ with high energy demands. The adenosine-triphosphate (ATP) required for cardiac contraction, relaxation, and basal metabolism in healthy adults is mainly provided by mitochondrial oxidative phosphorylation, and a small part comes from glycolysis (Stanley et al., 2005). There are a variety of energy substrates that can be used by cardiomyocytes. Approximately 70–90% of cardiac ATP is a result of the oxidation of fatty acids and the remaining 10–30% comes from the metabolism of glucose, lactate, ketone bodies, and certain amino acids (Doenst et al., 2013). Changes in energy metabolism during HF are mainly related to changes in the metabolic substrates and mitochondrial changes (He Y et al., 2021). The metabolic changes of cardiomyocytes not only depend on the severity and type of HF, but also are related to different underlying diseases (Lopaschuk et al., 2021). Generally, in the early stage of HF, the utilization of fatty acids remains the same or increases slightly as the severity of HF progresses, the rate of myocardial fatty acid oxidation decreases (Bertero and Maack, 2018b). Glucose metabolism in HF is characterized by an increased glucose uptake and glycolysis rate, without accompanying increase in glucose oxidation (De Jong and Lopaschuk, 2017). In the late stage of HF, the reduced sensitivity of the myocardium to insulin can lead to impaired myocardial glucose uptake (Lin et al., 2017). Ketone bodies, as substrates of cardiometabolic metabolism, have received extensive attention in recent years (Selvaraj et al., 2020). The utilization of ketone bodies increases as the use of fatty acid and glucose decreases (Bedi et al., 2016). Clinical studies have shown that exogenous ketone body supplementation can improve heart function in patients with HF (Nielsen et al., 2019). Most of the ATP (about 95%) consumed by the heart comes from oxidative metabolism in the mitochondria (Manolis et al., 2021). Changes in metabolic substrates during HF mean that the function and structure of mitochondria also change (Bayeva et al., 2013; Chaanine et al., 2019). On the one hand, after mitochondrial damage, oxidative phosphorylation is reduced, and the production of high-energy phosphoric acid compounds in the respiratory chain is impeded, resulting in insufficient bioenergy and aggravating the progression of HF (Zhou and Tian, 2018). On the other hand, other changes in mitochondrial function and structure, such as impaired mitochondrial electron transport chain activity, increased formation of reactive oxygen species, aberrant mitochondrial dynamics, and altered ion homeostasis, are also closely related to the occurrence of HF (Brown et al., 2017).
In the Langendorff system, total ginsenosides of Panax ginseng C. A. Mey. may modulate tricarboxylic acid cycle protein expression, such as L-lactate dehydrogenase B chain (LDHB), glycerol-3-phosphate dehydrogenase (G3PD), pyruvate dehydrogenase complex (PDC)and aldose reductase (AR), to improve cardiac energy metabolism in ischemic rat heart tissues (Wang et al., 2012). Panax ginseng polysaccharide protected mitochondria by inhibiting mitochondrial injury and swelling in a concentration-dependent manner, and improved energy metabolism (Li et al., 2009). The SIRT1 signaling pathway could improve glucose aerobic metabolism and mitochondrial biosynthesis and ginsenoside Rc acts as a SIRT1 activator (Huang et al., 2021). Ginsenoside Rg1 adjusts energy metabolism via regulating the expression of associated proteins, thus increasing the activity of mitochondria respiratory complexes and the expression of ATP synthase, H+ transporting, mitochondrial F1 complex, delta subunit (ATP5D), which might be the result of binding to Ras homolog family member A (RHOA) and inactivating RHOA/Rho associated coiled-coil containing protein kinase 1 (ROCK1) signaling (Li et al., 2018). Ginsenoside Rg1 an also promote autophagy, and inhibits apoptosis by weakening the interaction between Beclin1 and BCL-2 (Li et al., 2016). Ginsenoside Rb3(PubChem CID: 12912363) regulates energy metabolism and inhibits myocardial fibrosis by regulating peroxisome proliferator activated receptor a (PPARα) (Chen et al., 2019, Zhang Y et al., 2021). Ginsenoside Rb1(PubChem CID: 9898279) might regulate calcium signaling by alleviating protein O-GlcNAcylation to improve diabetic cardiomyopathy (Qin et al., 2019), (Table 3).
Aconitum carmichaelii Debx is a TCM that is, used extensively in HF as the processed product of Aconitum carmichaelii Debeaux tubers (Zhou W et al., 2021). Aconitum alkaloids, which include C19-diterpenoid alkaloids (mainly comprising aconitine, mesaconitine, and hypaconitine) and C20-diterpenoid alkaloids (predominantly benzoylmesaconitine, benzoylaconitine, and benzoylhypacoitine) and other alkaloids (mainly comprising higenamine and salsolinol) have versatile chemical structures (Zhou et al., 2015). Their cardiotoxicity and neurotoxicity hinder the use of Aconitum alkaloids; however, in TCM’s theory that even toxic substances are powerful medicines and can show reduced toxicity by proper methods (Liu et al., 2017; Zong et al., 2019; Mi et al., 2021). Emerging evidence also shows that Aconitum alkaloids could improve energy metabolism and mitochondrial function to generate cardioprotective effects. Aconitum alkaloids could reduce glucose levels, and increase creatine, adenosine monophosphate (AMP), Guanosine monophosphate (GMP), Adenosine diphosphate (ADP), and ATP levels in myocardial infarction rats (Wu H et al., 2019). Salsolinol (PubChem CID: 91588) attenuates doxorubicin-induced CHF in rats via a mechanism that might be related to the mitochondrial calcium uniporter (MCU) pathway (Wen et al., 2019). Aconitine (PubChem CID: 245005) alleviated the energy metabolic dysfunction of a myocardial injury model induced by Angiotensin II in H9c2 cells by activating SIRT3 to deacetylate cyclophilin D (CYPD) (Wang N. N et al., 2021). A combination of Zingiber officinale Rosc. and Aconitum carmichaelii Debx. showed an enhancing effect (Yu et al., 2012; Wen et al., 2020a). The potential mechanism of this effect might be related to mitochondrial energy metabolism, and it can be mediated by MCU in AHF rats induced by propafenone hydrochloride (Zhang et al., 2017) and improved by the liver kinase B1 (LKB1)/AMPKα/SIRT1 signaling pathway in doxorubicin (DOX)-induced CHF rats (Wen et al., 2020b), (Table 3).
Astragaloside IV can increase the ratio of ATP/AMP, and decrease the content of FFAs in heart tissue of rats compared with isoproterenol alone via NF-κB/peroxisome proliferator-activated receptor gamma coactivator-1 alpha (PGC1α) signaling-mediated energy biosynthesis (Zhang et al., 2015). The function of Astragaloside IV in improving fatty acid utilization might be connected with PPARα, medium-chain acyl-CoA dehydrogenase (MCAD), and mast cell protease 1 (MCPT1) (Dong et al., 2017; Tang et al., 2018). Astragaloside IV prevents ischemia/reperfusion (I/R) injury by maintaining the mitochondrial membrane potential, inhibiting mitochondrial permeability transition pore opening, and improving energy metabolism via inactivating glycogen synthase kinase 3 beta (GSK-3beta) and hairy and enhancer of split-1 (HES1), thus improving BCL-2-mediated mitochondrial function (He et al., 2012; Tu et al., 2013; Huang et al., 2016; Luo et al., 2019). Formononetin (PubChem CID: 5280378) has beneficial effects on obesity and insulin resistance by modulating PPARγ activity (Nie et al., 2018). Astragalus polysaccharide attenuates iso-induced cardiac hypertrophy through regulating tumor necrosis factor-α (TNF-α)/PGC1α signaling, resulting in decreased FFA contents (Luan et al., 2015), (Table 3).
Tanshinone IIa blocks mitochondrial damage via activating the SIRT1/PGC1α pathway in acute cardiac microcirculation I/R injury (Zhong et al., 2019). Cryptotanshinone (PubChem CID: 160254) protects against adriamycin-induced mitochondrial dysfunction in cardiomyocytes by increasing the activities of complexes, except complex II, and promotes mitochondrial biogenesis-relative factors PGC1α, NRF-1, and TFAM (Zhang et al., 2016), (Table 3).
Oxidative stress is defined as an imbalance between the generation of reactive oxygen species (ROS) and the endogenous antioxidant defense mechanisms (Zhou Y et al., 2021). At low concentrations, ROS modulates critical functions in cell homeostasis, whereas an overabundance of ROS plays a crucial role of worsening of the systolic and diastolic function and HF (Munzel et al., 2015). ROS production in the heart is primarily achieved by the mitochondria, NADPH oxidases, xanthine oxidase, and uncoupled nitric oxide synthase (NOS) (Munzel et al., 2017). Although pre-clinical studies have shown the beneficial effects of antioxidant strategies on HF, clinical studies in patients with HF have not achieved ideal results. A meta-analysis of 50 RCTs showed that supplementation with vitamins and antioxidants was not associated with reductions in the risk of major cardiovascular events (Myung et al., 2013). The future of anti-oxidative stress therapies might lie in increasing the endogenous antioxidant capacity or targeting mitochondrial ROS (Bertero and Maack, 2018a; Zhang et al., 2020). Study of TCM in oxidative stress might provide new ideas for the antioxidant treatment of HF (Table 3).
Berberine (PubChem CID: 2353), Palmatine (PubChem CID: 19009), and Coptisine (PubChem CID: 72322) are the main components of Coptis chinensis Franch. (Meng et al., 2018). Berberine protects rat hearts from I/R injury by decreasing the level of ROS and malondialdehyde (MDA), improving mitochondrial dysfunction via increasing MMP and complex I activity, as well as activating the SIRT1/tumor protein p53 (p53) signaling pathway (Liu D. Q et al., 2019). Berberine also ameliorates the doxorubicin-induced oxidative insult and mitochondrial damage as a modulator of SIRT3 or the SIRT1/Src-homology-2-domain-containing transforming protein 1 (p66Shc)-mediated pathway (Coelho et al., 2017; Wu Y. Z et al., 2019). Coptisine reduces oxidative stress by activating AKT and the JNK/NRF2/NAD(P)H quinone dehydrogenase 1 (NQO1) pathway (Hu et al., 2017), and Palmatine can inhibit the increase of lactate dehydrogenase (LDH), Creatine kinase (CK), and MDA and halt the decline of superoxide dismutase (SOD) and catalase (CAT) in I/R cardiac tissues (Kim et al., 2009), (Table 4).
Salidroside (PubChem CID: 159278) has been identified as one of the most active ingredients isolated from Rhodiola crenulate (Hook.f. et Thoms.) H. Ohba, which reduces oxidative stress to fight against cardiovascular diseases (Tao et al., 2019; Zhao et al., 2021). Salidroside protected cardiomyocytes against hydrogen peroxide-induced injury via an PI3K-AKT dependent pathway and increaed the expression and activities of endogenous PI3K-dependent antioxidant enzymes (Zhu et al., 2011). In doxorubicin-induced cardiotoxicity, salidroside suppressed the excessive oxidative stress by inhibiting NOX1 and augmented the endogenous antioxidative enzymes, catalase, and manganese superoxide dismutase (Wang et al., 2013). Salidroside protects against diabetes-induced cardiac dysfunction by modulating the mechanistic target of rapamycin (mTOR), AMPK, and AKT/heme oxygenase-1(HO-1) signaling pathways (Hao et al., 2021; Ni et al., 2021), (Table 4).
Over 67 compounds been isolated from Polygonum cuspidatum Sieb. et Zucc. and identified, among them, Polydatin (PubChem CID: 5281718) and Resveratrol (PubChem CID: 445154) have attracted wide attention in the field of oxidative stress (Peng et al., 2013). Polydatin protects myocardial function in diabetic rats via inhibition of NADPH oxidase and NF-κB activities (Tan et al., 2020), and also alleviates oxidative/nitrative stress damage via the Notch receptor 1 (Notch1)/heat stable protein 1(HS1)-phosphatase and tensin homolog (PTEN)/AKT signaling pathway in diabetic myocardial I/R injury (Yu et al., 2018). Polydatin prevents myocardial hypertrophy mediated by inhibition of ROS-dependent ROCK activation (Dong et al., 2015). In models of cardiac I/R injury, pharmacological agent-induced cardiotoxicity, obesity, long-term nicotine intake, and diabetes, Resveratrol activates the antioxidant genes such as those encoding HO-1, SOD, CAT, and glutathione (GSH), which can help to promote the balance between oxidative stress and antioxidants, especially in the mitochondria (Kovacic and Somanathan, 2010; Li et al., 2012; Ahmad and Hoda, 2020; Ramalingam et al., 2021). Parkinsonism associated deglycase (DJ-1) preserves mitochondrial complex I activity, thus playing an important role in Resveratrol-mediated cardioprotective effects against I/R-induced oxidative stress damage (Zhang et al., 2018). For the myocardial damage caused by diabetes, Resveratrol can inhibit the high-mobility group box 1tbox1 (HMGB 1)-mediated signaling pathway (Wu et al., 2016), activate SIRT1 leading to deacetylation of both NF-kB/p65 and histone 3 (H3) (Bagul et al., 2015), improve AMPK-related pathways (Guo et al., 2015), and enhance autophagy via the SIRT1/Forkhead box O1(FOXO1)/member RAS oncogene family (RAB7) axis (Wang et al., 2014), (Table 4).
Curcumin (PubChem CID: 969516) is an important compound in Curcuma Longa L., which is the golden spice in treating cardiovascular diseases (Li et al., 2020). Chemotherapeutic drugs induce cardiotoxicity, limiting their clinical application, and curcumin rescues DOX-induced cardiac injury by suppressing oxidative stress and improving mitochondrial function via regulation of the mode of cell death (autophagy, apoptosis, and pyroptosis) via an mTOR or 14-3-3γ-dependent pathway (He et al., 2018; Liu Q et al., 2019; Yu et al., 2020). Curcumin acts as an agonist of SIRT1 to protect against myocardial infarction-induced cardiac fibrosis (Xiao et al., 2016). The protection provided by curcumin in myocardial damage induced by metabolic disorders might be associated with activating Nrf2, inactivating NF-κB, and modulating the SIRT1-FOXO1 and PI3K-AKT pathways (Zeng et al., 2015; Ren et al., 2020), (Table 4).
The innate immune system is activated by a variety of cardiac disease states that lead to cardiac injury through the interaction between damage-associated molecular patterns (DAMPs) or pathogen-associated molecular patterns (PAMPs) and pattern-recognition receptors (PRRs), most commonly TLR4 (Dutka et al., 2020). Activation of PRRs induces a variety of non-cellular effectors (pro-inflammatory cytokine, chemokines, and inflammasome assembly) and cellular effectors (neutrophils, monocytes, and macrophages) in the heart, especially NF-kB (Adamo et al., 2020). Adaptive immunity is activated by the innate immune system, resulting in the recruitment of B cells and T cells to injured cardiomyocytes (Rhee and Lavine, 2020). On a myocardial level, inflammation promotes myofibroblast formation and interstitial collagen deposition, and influences multiple peripheral organ systems to exacerbate the development of HF (Murphy et al., 2020). Similar to the clinical studies of oxidative stress, the results of other anti-inflammatory treatments are not satisfactory, except in the CANTOS trial (Everett et al., 2020). The study of inflammation in TCM might provide new ideas for the treatment of HF.
Paeonia lactiflora Pall. and Paeonia veitchii Lynch belong to the genus Paeonia, from which more than 400 compounds have been structurally identified (Yan et al., 2018; Li P et al., 2021). Paeoniflorin (PubChem CID: 442534) is unique to the genus Paeonia and several studies have reported its anti-inflammatory effects (Jiao et al., 2021). Paeoniflorin decreased the levels of tumor necrosis factor-α (TNFα) and interleukin-1β (IL)-1β in a mouse pressure overload-induced cardiac remodeling model by inhibiting NF-κB pathways (Zhou et al., 2013). Paeoniflorin reduces TNFα, IL-6, and monocyte chemoattractant protein (MCP)-1 levels and plays a cardioprotective role in spontaneous hypertensive rats via the MAPK signaling pathway (Liu X et al., 2019). The levels of inflammatory cytokines of TNF-α, IL-1β, IL-6, IL-12, MCP-1, and interferon (IFN)-γ can be decreased by paeoniflorin in endotoxemic mice to improve cardiac function via activation of PI3K/AKT signaling (Zhai and Guo, 2016). Gallic acid is a tannin of the genus Paeonia. Gallic acid (PubChem CID: 370) protects cardiac dysfunction by reducing the level of IL-6 and TNF-α in particulate matter-induced rat model (Radan et al., 2019) and ameliorates angiotensin II-induced atrial fibrillation by inhibiting immunoproteasome-mediated PTEN degradation in mice (Han D et al., 2020). Paeonol (PubChem CID: 11092) is another compound in the genus Paeonia, especially Paeonia suffruticosa Andr., which has a cardioprotective effect in epirubicin-induced cardiotoxicity via increasing MicroRNA-1 (miR-1) to suppress the PI3K/AKT/mTOR and NF-kB signaling pathways (Wu et al., 2018) and reducing inflammatory damage in I/R injury rats (Ma et al., 2016), (Table 5).
Hyperoside (PubChem CID: 5281643), a flavonoid from Crataegus pinnatifida Bge. var. major N.E.Br., suppresses vascular inflammatory responses in diabetic complications and atherosclerosis by downregulating mitogen-activated protein kinases (p38 MAPK, JNK, and ERK) and NF-κB signaling (Ku et al., 2014; Jang et al., 2018). Hyperoside also plays a protective role against heart damage caused by other diseases. Hyperoside ameliorated myocardial hypertrophy, collagen volume fraction, and cardiomyocyte inflammation in the myocardial infarction mice by regulating autophagy via the nucleotide-binding domain and leucine-rich repeat related (NLR) family, pyrin domain containing 1(NLRP1) inflammation pathway (Yang et al., 2021). Hyperoside decreased the levels of inflammatory factors, including IL-1β, IL-6, TNF-α, and MCP-1 in an angiotensin II-induced cardiomyocyte hypertrophy model to improve cardiac function via AKT signaling (Wang X et al., 2018). Hyperoside treated cardiac dysfunction in a mouse model of sepsis by regulating cardiomyocyte viability and inflammation via suppressing miR-21 (Zhang J et al., 2021), (Table 5).
The anti-inflammatory effects of Cinnamomum cassia Presl extracts have been used to treat a variety of diseases, and research on its anti-inflammatory mechanism has found that it might be closely related to NF-κB, and Toll-like receptors TLR2 and TLR4 (Reddy et al., 2004; Schink et al., 2018). More than 160 components have been isolated and identified from Cinnamomum cassia Presl. Phenylpropanoids are the main bioactive components, including 2-Methoxycinnamaldehyde (PubChem CID: 641298), Cinnamaldehyde (PubChem CID: 637511) (Zhang et al., 2019). 2-Methoxycinnamaldehyde acid inhibits vascular cell adhesion molecule-1(VCAM-1) and NF-κB expression, which are activated by TNF-α in endothelial cells and inhibited by HO-1 induction, thus the heart function of rats with I/R injury can be protected (Hwa et al., 2012). Cinnamaldehyde inhibits the activation of NLRP3 inflammasomes by attenuating the cluster of differentiation 36 (CD36)-mediated TLR4/6-IRAK (IRAK) 4/1 signaling, thereby reducing fructose-induced cardiac inflammation and fibrosis (Kang et al., 2016). Cinnamaldehyde can also improve lipopolysaccharide-induced cardiac dysfunction through the TLR4-NOX4 pathway (Zhao et al., 2016). Cinnamaldehyde and its derivative cinnamic acid can reduce TNF-α and IL-6 in rats with acute myocardial ischemia induced by isoproterenol (Song et al., 2013), which also directly reduces the inflammation of viral myocarditis induced by coxsackie virus B3 by inhibiting the TLR4-NF-κB signal transduction pathway (Ding et al., 2010), (Table 5).
In this review, we retrospectively analyzed clinical studies on the intervention of TCM in HF, and discussed the mechanisms of some commonly used TCMs and their components in the treatment of HF. From the perspective of clinical research, TCM has the advantages of good curative effect and low levels of side effects in the treatment of HF, which can make up for the shortcomings of current treatment methods to a certain extent, and the two sides can produce complementary advantages. Basic research has shown that TCM can play its role in many ways, such as microcirculation improvements, energy metabolism promotion, anti-inflammation, and anti-oxidation. However, it should be noted that the compositions of TCM are complex. On the one hand, a variety of chemical components can affect organisms through many biological reactions. On the other hand, this kind of diversity makes it possible for different active ingredients in TCM to be synergistic, enhancing, and antagonistic. In the basic theories of TCM, the principles of drug application are also mentioned. However, currently, it is unclear which combinations of active ingredients have synergistic and antagonistic effects, or which combinations might increase toxicity, not to mention the effective or optimal dose of each active ingredient in the combination.
HF comprises a group of clinical syndromes with complex pathological mechanisms, involving multiple signaling pathways and targets. Natural medicines or their active ingredients can act on a variety of pathways and targets to effectively treat diseases, which is also the advantage of the application of TCM. To clarify the mechanism of TCM, it is necessary to study the pathways and targets of each active ingredient alone and in different combinations. It is also necessary to find a suitable breakthrough point and establish a reasonable pharmacological model based on genomics, proteomics, functional metabolomics, TCM pharmacology, and other -omics research (Han Y et al., 2020; Ma et al., 2020; Wang T et al., 2021). Determining the mechanism of the effective ingredients can not only explain how the various ingredients in TCM work individually or in combination, but also, and more importantly, it can discover new mechanisms and synergistic effects of the effective ingredients, which is conducive to innovation of TCM and the development of TCM theories.
A large number RCTs of integrated TCM and western medicine are reported every year; however, the quality of these studies is uneven. On the one hand, clinical research in TCM should carry out randomized, double-blind, placebo-controlled large-sample, multi-center RCT studies. During the implementation of RCTs, patients need to be included in strict accordance with the latest diagnostic criteria. The design of the placebo should be completely consistent with TCM in appearance and smell etc. Curative effect indicators should pay more attention on hard endpoints of cardiovascular events or choose internationally recognized intermediate endpoints as the primary endpoints to carry out long-term follow-up. On the other hand, the advantage of TCM treatment lies in individualized therapy based on syndrome differentiation. The direction of our future efforts is to form an RCT research method for TCM treatment and enable TCM with RCT evidence to enter clinical practice.
The results of RCTs indicate that as an adjuvant treatment to conventional drugs, TCM might be beneficial to patients with HF. Recent studies on the mechanism of HF in vitro and in animal models have shown that TCM has microcirculation improvement, energy metabolism promotion, anti-inflammation, and anti-oxidation effects.
AW: Conceptualization, Methodology, Validation, Formal analysis, Investigation, Data Curation, Writing—original draft, Visualization. WZ: Conceptualization, Methodology, Writing—review and editing. KY: Conceptualization, Methodology, Writing—review and editing. PH: Investigation, Formal analysis, Data Curation. HZ: Investigation, Data Curation. ZZ: Formal analysis, Visualization. DZ: Writing—review and editing, Supervision. XM: Conceptualization, Writing—review and editing, Supervision, Project administration.
This work was supported by the National Key Research and Development Program of China (No. 2018YFC1707410-02).
The authors declare that the research was conducted in the absence of any commercial or financial relationships that could be construed as a potential conflict of interest.
All claims expressed in this article are solely those of the authors and do not necessarily represent those of their affiliated organizations, or those of the publisher, the editors and the reviewers. Any product that may be evaluated in this article, or claim that may be made by its manufacturer, is not guaranteed or endorsed by the publisher.
We would like to acknowledge Hong Liu, Qingzhou Station Primary School, Weifang, China for writing assistance and ETCM (http://www.tcmip.cn/ETCM/index.php/Home/Index/) for providing photographs of TCM decoction pieces.
The Supplementary Material for this article can be found online at: https://www.frontiersin.org/articles/10.3389/fphar.2022.810587/full#supplementary-material
Adamo, L., Rocha-Resende, C., Prabhu, S. D., and Mann, D. L. (2020). Reappraising the Role of Inflammation in Heart Failure. Nat. Rev. Cardiol. 17 (5), 269–285. doi:10.1038/s41569-019-0315-x
Ahmad, A., Corban, M. T., Toya, T., Verbrugge, F. H., Sara, J. D., Lerman, L. O., et al. (2021). Coronary Microvascular Dysfunction Is Associated with Exertional Haemodynamic Abnormalities in Patients with Heart Failure with Preserved Ejection Fraction. Eur. J. Heart Fail 23 (5), 765–772. doi:10.1002/ejhf.2010
Ahmad, I., and Hoda, M. (2020). Molecular Mechanisms of Action of Resveratrol in Modulation of Diabetic and Non-diabetic Cardiomyopathy. Pharmacol. Res. 161 (11), 105112–112. doi:10.1016/j.phrs.2020.105112
Anker, S. D., Butler, J., Filippatos, G., Ferreira, J. P., Bocchi, E., Böhm, M., et al. (2021). Empagliflozin in Heart Failure with a Preserved Ejection Fraction. N. Engl. J. Med. 385 (16), 1451–1461. doi:10.1056/NEJMoa2107038
Bagul, P. K., Deepthi, N., Sultana, R., and Banerjee, S. K. (2015). Resveratrol Ameliorates Cardiac Oxidative Stress in Diabetes through Deacetylation of NFkB-P65 and Histone 3. J. Nutr. Biochem. 26 (11), 1298–1307. doi:10.1016/j.jnutbio.2015.06.006
Bayeva, M., Gheorghiade, M., and Ardehali, H. (2013). Mitochondria as a Therapeutic Target in Heart Failure. J. Am. Coll. Cardiol. 61 (6), 599–610. doi:10.1016/j.jacc.2012.08.1021
Bedi, K. C., Snyder, N. W., Brandimarto, J., Aziz, M., Mesaros, C., Worth, A. J., et al. (2016). Evidence for Intramyocardial Disruption of Lipid Metabolism and Increased Myocardial Ketone Utilization in Advanced Human Heart Failure. Circulation 133 (8), 706–716. doi:10.1161/CIRCULATIONAHA.115.017545
Benjamin, E. J., Muntner, P., Alonso, A., Bittencourt, M. S., Callaway, C. W., Carson, A. P., et al. (2019). Heart Disease and Stroke Statistics-2019 Update: A Report from the American Heart Association. Circulation 139 (10), e56–528. doi:10.1161/CIR.0000000000000659
Bertero, E., and Maack, C. (2018a). Calcium Signaling and Reactive Oxygen Species in Mitochondria. Circ. Res. 122 (10), 1460–1478. doi:10.1161/CIRCRESAHA.118.310082
Bertero, E., and Maack, C. (2018b). Metabolic Remodelling in Heart Failure. Nat. Rev. Cardiol. 15 (8), 457–470. doi:10.1038/s41569-018-0044-6
Bo, Y., Jian, Z., Zhi-Jun, S., Quing, W., Hua, Z., Chuan-Wei, L., et al. (2020). Panax Notoginseng Saponins Alleviates Advanced Glycation End Product-Induced Apoptosis by Upregulating SIRT1 and Antioxidant Expression Levels in HUVECs. Exp. Ther. Med. 20 (5), 99. doi:10.3892/etm.2020.9229
Bozkurt, B., Coats, A. J. S., Tsutsui, H., Abdelhamid, C. M., Adamopoulos, S., Albert, N., et al. (2021). Universal Definition and Classification of Heart Failure: a Report of the Heart Failure Society of America, Heart Failure Association of the European Society of Cardiology, Japanese Heart Failure Society and Writing Committee of the Universal Definition of Heart Failure: Endorsed by the Canadian Heart Failure Society, Heart Failure Association of India, Cardiac Society of Australia and New Zealand, and Chinese Heart Failure Association. Eur. J. Heart Fail 23 (3), 352–380. doi:10.1002/ejhf.2115
Brown, D. A., Perry, J. B., Allen, M. E., Sabbah, H. N., Stauffer, B. L., Shaikh, S. R., et al. (2017). Expert Consensus Document: Mitochondrial Function as a Therapeutic Target in Heart Failure. Nat. Rev. Cardiol. 14 (4), 238–250. doi:10.1038/nrcardio.2016.203
Brutsaert, D. L. (2003). Cardiac Endothelial-Myocardial Signaling: its Role in Cardiac Growth, Contractile Performance, and Rhythmicity. Physiol. Rev. 83 (1), 59–115. doi:10.1152/physrev.00017.2002
Chaanine, A. H., Joyce, L. D., Stulak, J. M., Maltais, S., Joyce, D. L., Dearani, J. A., et al. (2019). Mitochondrial Morphology, Dynamics, and Function in Human Pressure Overload or Ischemic Heart Disease with Preserved or Reduced Ejection Fraction. Circ. Heart Fail 12 (2), e005131. doi:10.1161/CIRCHEARTFAILURE.118.005131
Chen, X., Wang, Q., Shao, M., Ma, L., Guo, D., Wu, Y., et al. (2019). Ginsenoside Rb3 Regulates Energy Metabolism and Apoptosis in Cardiomyocytes via Activating PPARα Pathway. Biomed. Pharmacother. 120, 109487. doi:10.1016/j.biopha.2019.109487
Coelho, A. R., Martins, T. R., Couto, R., Deus, C., Pereira, C. V., Simões, R. F., et al. (2017). Berberine-induced Cardioprotection and Sirt3 Modulation in Doxorubicin-Treated H9c2 Cardiomyoblasts. Biochim. Biophys. Acta Mol. Basis Dis. 1863 (11), 2904–2923. doi:10.1016/j.bbadis.2017.07.030
Crespo-Leiro, M. G., Anker, S. D., Maggioni, A. P., Coats, A. J., Filippatos, G., Ruschitzka, F., et al. (2016). European Society of Cardiology Heart Failure Long-Term Registry (ESC-HF-LT): 1-year Follow-Up Outcomes and Differences across Regions. Eur. J. Heart Fail 18 (6), 613–625. doi:10.1002/ejhf.566
Cui, Z. T., Liu, J. P., and Wei, W. L. (2016). The Effects of Tanshinone IIA on Hypoxia/reoxygenation-Induced Myocardial Microvascular Endothelial Cell Apoptosis in Rats via the JAK2/STAT3 Signaling Pathway. Biomed. Pharmacother. 83, 1116–1126. doi:10.1016/j.biopha.2016.07.054
De Jong, K. A., and Lopaschuk, G. D. (2017). Complex Energy Metabolic Changes in Heart Failure with Preserved Ejection Fraction and Heart Failure with Reduced Ejection Fraction. Can J. Cardiol. 33 (7), 860–871. doi:10.1016/j.cjca.2017.03.009
Ding, Y., Qiu, L., Zhao, G., Xu, J., and Wang, S. (2010). Influence of Cinnamaldehyde on Viral Myocarditis in Mice. Am. J. Med. Sci. 340 (2), 114–120. doi:10.1097/MAJ.0b013e3181dd3b43
Disease, G. B. D., Injury, I., and Prevalence, C. (2018). Global, Regional, and National Incidence, Prevalence, and Years Lived with Disability for 354 Diseases and Injuries for 195 Countries and Territories, 1990-2017: a Systematic Analysis for the Global Burden of Disease Study 2017. Lancet 392 (10159), 1789–1858. doi:10.1016/S0140-6736(18)32279-7
Doenst, T., Nguyen, T. D., and Abel, E. D. (2013). Cardiac Metabolism in Heart Failure: Implications beyond ATP Production. Circ. Res. 113 (6), 709–724. doi:10.1161/CIRCRESAHA.113.300376
Dokainish, H., Teo, K., Zhu, J., Roy, A., AlHabib, K. F., ElSayed, A., et al. (2017). Global Mortality Variations in Patients with Heart Failure: Results from the International Congestive Heart Failure (INTER-CHF) Prospective Cohort Study. Lancet Glob. Health 5 (7), e665–e672. doi:10.1016/S2214-109X(17)30196-1
Dong, M., Ding, W., Liao, Y., Liu, Y., Yan, D., Zhang, Y., et al. (2015). Polydatin Prevents Hypertrophy in Phenylephrine Induced Neonatal Mouse Cardiomyocytes and Pressure-Overload Mouse Models. Eur. J. Pharmacol. 746, 186–197. doi:10.1016/j.ejphar.2014.11.012
Dong, Z., Zhao, P., Xu, M., Zhang, C., Guo, W., Chen, H., et al. (2017). Astragaloside IV Alleviates Heart Failure via Activating PPARα to Switch Glycolysis to Fatty Acid β-oxidation. Sci. Rep. 7 (1), 2691. doi:10.1038/s41598-017-02360-5
Dutka, M., Bobiński, R., Ulman-Włodarz, I., Hajduga, M., Bujok, J., Pająk, C., et al. (2020). Various Aspects of Inflammation in Heart Failure. Heart Fail Rev. 25 (3), 537–548. doi:10.1007/s10741-019-09875-1
Elgendy, I. Y., Mahtta, D., and Pepine, C. J. (2019). Medical Therapy for Heart Failure Caused by Ischemic Heart Disease. Circ. Res. 124 (11), 1520–1535. doi:10.1161/CIRCRESAHA.118.313568
Everett, B. M., MacFadyen, J. G., Thuren, T., Libby, P., Glynn, R. J., and Ridker, P. M. (2020). Inhibition of Interleukin-1β and Reduction in Atherothrombotic Cardiovascular Events in the CANTOS Trial. J. Am. Coll. Cardiol. 76 (14), 1660–1670. doi:10.1016/j.jacc.2020.08.011
Fan, G., Zhu, Y., Guo, H., Wang, X., Wang, H., and Gao, X. (2011). Direct Vasorelaxation by a Novel Phytoestrogen Tanshinone IIA Is Mediated by Nongenomic Action of Estrogen Receptor through Endothelial Nitric Oxide Synthase Activation and Calcium Mobilization. J. Cardiovasc. Pharmacol. 57 (3), 340–347. doi:10.1097/FJC.0b013e31820a0da1
Fan, J., Liu, D., He, C., Li, X., and He, F. (2016). Inhibiting Adhesion Events by Panax Notoginseng Saponins and Ginsenoside Rb1 Protecting Arteries via Activation of Nrf2 and Suppression of P38 - VCAM-1 Signal Pathway. J. Ethnopharmacol 192, 423–430. doi:10.1016/j.jep.2016.09.022
Fang, H., Yang, S., Luo, Y., Zhang, C., Rao, Y., Liu, R., et al. (2018). Notoginsenoside R1 Inhibits Vascular Smooth Muscle Cell Proliferation, Migration and Neointimal Hyperplasia through PI3K/Akt Signaling. Sci. Rep. 8 (1), 7595. doi:10.1038/s41598-018-25874-y
Fiuzat, M., Lowy, N., Stockbridge, N., Sbolli, M., Latta, F., Lindenfeld, J., et al. (2020). Endpoints in Heart Failure Drug Development: History and Future. JACC Heart Fail 8 (6), 429–440. doi:10.1016/j.jchf.2019.12.011
Ford, T. J., Corcoran, D., and Berry, C. (2018). Stable Coronary Syndromes: Pathophysiology, Diagnostic Advances and Therapeutic Need. Heart 104 (4), 284–292. doi:10.1136/heartjnl-2017-311446
Fu, S., Zhang, J., Gao, X., Xia, Y., Ferrelli, R., Fauci, A., et al. (2010). Clinical Practice of Traditional Chinese Medicines for Chronic Heart Failure. Heart Asia 2 (1), 24–27. doi:10.1136/ha.2009.001123
Gao, K., Zhao, H., Gao, J., Wen, B., Jia, C., Wang, Z., et al. (2017). Mechanism of Chinese Medicine Herbs Effects on Chronic Heart Failure Based on Metabolic Profiling. Front Pharmacol. 8, 864. doi:10.3389/fphar.2017.00864
Ghionzoli, N., Gentile, F., Del Franco, A. M., Castiglione, V., Aimo, A., Giannoni, A., et al. (2021). Current and Emerging Drug Targets in Heart Failure Treatment. Heart Fail Rev. [online ahead of print]. doi:10.1007/s10741-021-10137-2
Groenewegen, A., Rutten, F. H., Mosterd, A., and Hoes, A. W. (2020). Epidemiology of Heart Failure. Eur. J. Heart Fail 22 (8), 1342–1356. doi:10.1002/ejhf.1858
Guo, S., Yao, Q., Ke, Z., Chen, H., Wu, J., and Liu, C. (2015). Resveratrol Attenuates High Glucose-Induced Oxidative Stress and Cardiomyocyte Apoptosis through AMPK. Mol. Cell Endocrinol 412, 85–94. doi:10.1016/j.mce.2015.05.034
Han, D., Zhang, Q. Y., Zhang, Y. L., Han, X., Guo, S. B., Teng, F., et al. (2020). Gallic Acid Ameliorates Angiotensin II-Induced Atrial Fibrillation by Inhibiting Immunoproteasome- Mediated PTEN Degradation in Mice. Front Cell Dev Biol 8, 594683. doi:10.3389/fcell.2020.594683
Han, Y., Sun, H., Zhang, A., Yan, G., and Wang, X. J. (2020). Chinmedomics, a New Strategy for Evaluating the Therapeutic Efficacy of Herbal Medicines. Pharmacol. Ther. 216, 107680. doi:10.1016/j.pharmthera.2020.107680
Hao, W., Li, N., Mi, C., Wang, Q., and Yu, Y. (2021). Salidroside Attenuates Cardiac Dysfunction in a Rat Model of Diabetes. Diabet Med., e14683. [online ahead of print]. doi:10.1111/dme.14683
He, H., Luo, Y., Qiao, Y., Zhang, Z., Yin, D., Yao, J., et al. (2018). Curcumin Attenuates Doxorubicin-Induced Cardiotoxicity via Suppressing Oxidative Stress and Preventing Mitochondrial Dysfunction Mediated by 14-3-3γ. Food Funct. 9 (8), 4404–4418. doi:10.1039/c8fo00466h
He, L., Liu, Y. Y., Wang, K., Li, C., Zhang, W., Li, Z. Z., et al. (2021). Tanshinone IIA Protects Human Coronary Artery Endothelial Cells from Ferroptosis by Activating the NRF2 Pathway. Biochem. Biophys. Res. Commun. 575, 1–7. doi:10.1016/j.bbrc.2021.08.067
He, Y., Huang, W., Zhang, C., Chen, L., Xu, R., Li, N., et al. (2021). Energy Metabolism Disorders and Potential Therapeutic Drugs in Heart Failure. Acta Pharm. Sin B 11 (5), 1098–1116. doi:10.1016/j.apsb.2020.10.007
He, Y., Xi, J., Zheng, H., Zhang, Y., Jin, Y., and Xu, Z. (2012). Astragaloside IV Inhibits Oxidative Stress-Induced Mitochondrial Permeability Transition Pore Opening by Inactivating GSK-3β via Nitric Oxide in H9c2 Cardiac Cells. Oxid Med. Cell Longev 2012, 935738. doi:10.1155/2012/935738
Hu, G., Yang, P., Zeng, Y., Zhang, S., and Song, J. (2018). Danggui Buxue Decoction Promotes Angiogenesis by Up-Regulation of VEGFR1/2 Expressions and Down-Regulation of sVEGFR1/2 Expression in Myocardial Infarction Rat. J. Chin Med. Assoc. 81 (1), 37–46. doi:10.1016/j.jcma.2017.06.015
Hu, Y. R., Ma, H., Zou, Z. Y., He, K., Xiao, Y. B., Wang, Y., et al. (2017). Activation of Akt and JNK/Nrf2/NQO1 Pathway Contributes to the Protective Effect of Coptisine against AAPH-Induced Oxidative Stress. Biomed. Pharmacother. 85, 313–322. doi:10.1016/j.biopha.2016.11.031
Huang, H., Lai, S., Wan, Q., Qi, W., and Liu, J. (2016). Astragaloside IV Protects Cardiomyocytes from Anoxia/reoxygenation Injury by Upregulating the Expression of Hes1 Protein. Can J. Physiol. Pharmacol. 94 (5), 542–553. doi:10.1139/cjpp-2015-0457
Huang, Q., Su, H., Qi, B., Wang, Y., Yan, K., Wang, X., et al. (2021). A SIRT1 Activator, Ginsenoside Rc, Promotes Energy Metabolism in Cardiomyocytes and Neurons. J. Am. Chem. Soc. 143 (3), 1416–1427. doi:10.1021/jacs.0c10836
Hwa, J. S., Jin, Y. C., Lee, Y. S., Ko, Y. S., Kim, Y. M., Shi, L. Y., et al. (2012). 2-methoxycinnamaldehyde from Cinnamomum cassia Reduces Rat Myocardial Ischemia and Reperfusion Injury In Vivo Due to HO-1 Induction. J. Ethnopharmacol 139 (2), 605–615. doi:10.1016/j.jep.2011.12.001
Jang, S. A., Park, D. W., Sohn, E. H., Lee, S. R., and Kang, S. C. (2018). Hyperoside Suppresses Tumor Necrosis Factor α-mediated Vascular Inflammatory Responses by Downregulating Mitogen-Activated Protein Kinases and Nuclear Factor-Κb Signaling. Chem. Biol. Interact 294, 48–55. doi:10.1016/j.cbi.2018.08.013
Jiao, F., Varghese, K., Wang, S., Liu, Y., Yu, H., Booz, G. W., et al. (2021). Recent Insights into the Protective Mechanisms of Paeoniflorin in Neurological, Cardiovascular, and Renal Diseases. J. Cardiovasc. Pharmacol. 77 (6), 728–734. doi:10.1097/FJC.0000000000001021
Kang, L. L., Zhang, D. M., Ma, C. H., Zhang, J. H., Jia, K. K., Liu, J. H., et al. (2016). Cinnamaldehyde and Allopurinol Reduce Fructose-Induced Cardiac Inflammation and Fibrosis by Attenuating CD36-Mediated TLR4/6-Irak4/1 Signaling to Suppress NLRP3 Inflammasome Activation. Sci. Rep. 6, 27460. doi:10.1038/srep27460
Kim, N. D., Kim, E. M., Kang, K. W., Cho, M. K., Choi, S. Y., and Kim, S. G. (2003). Ginsenoside Rg3 Inhibits Phenylephrine-Induced Vascular Contraction through Induction of Nitric Oxide Synthase. Br. J. Pharmacol. 140 (4), 661–670. doi:10.1038/sj.bjp.0705490
Kim, Y. M., Ha, Y. M., Jin, Y. C., Shi, L. Y., Lee, Y. S., Kim, H. J., et al. (2009). Palmatine from Coptidis Rhizoma Reduces Ischemia-Reperfusion-Mediated Acute Myocardial Injury in the Rat. Food Chem. Toxicol. 47 (8), 2097–2102. doi:10.1016/j.fct.2009.05.031
Kovacic, P., and Somanathan, R. (2010). Multifaceted Approach to Resveratrol Bioactivity: Focus on Antioxidant Action, Cell Signaling and Safety. Oxid Med. Cell Longev 3 (2), 86–100. doi:10.4161/oxim.3.2.11147
Ku, S. K., Kwak, S., Kwon, O. J., and Bae, J. S. (2014). Hyperoside Inhibits High-Glucose-Induced Vascular Inflammation In Vitro and In Vivo. Inflammation 37 (5), 1389–1400. doi:10.1007/s10753-014-9863-8
Lam, C. S. P., Voors, A. A., de Boer, R. A., Solomon, S. D., and van Veldhuisen, D. J. (2018). Heart Failure with Preserved Ejection Fraction: from Mechanisms to Therapies. Eur. Heart J. 39 (30), 2780–2792. doi:10.1093/eurheartj/ehy301
Leng, B., Tang, F., Lu, M., Zhang, Z., Wang, H., and Zhang, Y. (2018). Astragaloside IV Improves Vascular Endothelial Dysfunction by Inhibiting the TLR4/NF-Κb Signaling Pathway. Life Sci. 209, 111–121. doi:10.1016/j.lfs.2018.07.053
Leung, K. W., Ng, H. M., Tang, M. K., Wong, C. C., Wong, R. N., and Wong, A. S. (2011). Ginsenoside-Rg1 Mediates a Hypoxia-independent Upregulation of Hypoxia-Inducible Factor-1α to Promote Angiogenesis. Angiogenesis 14 (4), 515–522. doi:10.1007/s10456-011-9235-z
Li, D., Wang, J., Hou, J., Fu, J., Chang, D., Bensoussan, A., et al. (2016). Ginsenoside Rg1 Protects Starving H9c2 Cells by Dissociation of Bcl-2-Beclin1 Complex. BMC Complement Altern. Med. 16, 146. doi:10.1186/s12906-016-1112-2
Li, H., Sureda, A., Devkota, H. P., Pittalà, V., Barreca, D., Silva, A. S., et al. (2020). Curcumin, the golden Spice in Treating Cardiovascular Diseases. Biotechnol. Adv. 38, 107343. doi:10.1016/j.biotechadv.2019.01.010
Li, H., Xia, N., and Förstermann, U. (2012). Cardiovascular Effects and Molecular Targets of Resveratrol. Nitric Oxide 26 (2), 102–110. doi:10.1016/j.niox.2011.12.006
Li, L., Pan, C. S., Yan, L., Cui, Y. C., Liu, Y. Y., Mu, H. N., et al. (2018). Ginsenoside Rg1 Ameliorates Rat Myocardial Ischemia-Reperfusion Injury by Modulating Energy Metabolism Pathways. Front Physiol. 9, 78. doi:10.3389/fphys.2018.00078
Li, P., Shen, J., Wang, Z., Liu, S., Liu, Q., Li, Y., et al. (2021). Genus Paeonia: A Comprehensive Review on Traditional Uses, Phytochemistry, Pharmacological Activities, Clinical Application, and Toxicology. J. Ethnopharmacol 269, 113708. doi:10.1016/j.jep.2020.113708
Li, S., Lou, S., Lei, B. U., Chan, T. F., Kwan, Y. W., Chan, S. W., et al. (2011). Transcriptional Profiling of Angiogenesis Activities of Calycosin in Zebrafish. Mol. Biosyst. 7 (11), 3112–3121. doi:10.1039/c1mb05206c
Li, X., Zhang, J., Huang, J., Ma, A., Yang, J., Li, W., et al. (2013). A Multicenter, Randomized, Double-Blind, Parallel-Group, Placebo-Controlled Study of the Effects of Qili Qiangxin Capsules in Patients with Chronic Heart Failure. J. Am. Coll. Cardiol. 62 (12), 1065–1072. doi:10.1016/j.jacc.2013.05.035
Li, X. T., Chen, R., Jin, L. M., and Chen, H. Y. (2009). Regulation on Energy Metabolism and protection on Mitochondria of Panax Ginseng Polysaccharide. Am. J. Chin Med. 37 (6), 1139–1152. doi:10.1142/S0192415X09007454
Li, X. X., Wu, Y., Fan, Z. J., Cui, J., Li, D., Lin, Q., et al. (2022). Qishen Taohong Granule () as Adjuvant Therapy for Improving Cardiac Function and Quality of Life in Patients with Chronic Heart Failure: A Randomized Controlled Trial. Chin J. Integr. Med. 28 (1), 12–19. doi:10.1007/s11655-021-2866-z
Lin, C. H., Cheng, Y. C., Nicol, C. J., Lin, K. H., Yen, C. H., and Chiang, M. C. (2017). Activation of AMPK Is Neuroprotective in the Oxidative Stress by Advanced Glycosylation End Products in Human Neural Stem Cells. Exp. Cell Res 359 (2), 367–373. doi:10.1016/j.yexcr.2017.08.019
Lin, X. P., Cui, H. J., Yang, A. L., Luo, J. K., and Tang, T. (2018). Astragaloside IV Improves Vasodilatation Function by Regulating the PI3K/Akt/eNOS Signaling Pathway in Rat Aorta Endothelial Cells. J. Vasc. Res. 55 (3), 169–176. doi:10.1159/000489958
Liu, D. Q., Chen, S. P., Sun, J., Wang, X. M., Chen, N., Zhou, Y. Q., et al. (2019). Berberine Protects against Ischemia-Reperfusion Injury: A Review of Evidence from Animal Models and Clinical Studies. Pharmacol. Res. 148, 104385. doi:10.1016/j.phrs.2019.104385
Liu, Q., Yuan, J. W., Zhang, F., Qiao, F., Sui, X. F., and Liu, C. H. (2019). Curcumin Protects Rat H9C2 Cardiomyocytes against Doxorubicin Toxicity by Modulating Oxidative Stress and Apoptosis. J. Biol. Regul. Homeost Agents 33 (6), 1849–1854. doi:10.23812/19-365-L10.23812/19-365-L
Liu, S., Li, F., Li, Y., Li, W., Xu, J., and Du, H. (2017). A Review of Traditional and Current Methods Used to Potentially Reduce Toxicity of Aconitum Roots in Traditional Chinese Medicine. J. Ethnopharmacol 207, 237–250. doi:10.1016/j.jep.2017.06.038
Liu, W. H. (2020). Effectiveness of Yangyin Shuxin Decoction on Diastolic Function and Exercise Capacity of Patients with Heart Failure. Tianjin: Tianjin University of Chinese Medicine.
Liu, X., Chen, K., Zhuang, Y., Huang, Y., Sui, Y., Zhang, Y., et al. (2019). Paeoniflorin Improves Pressure Overload-Induced Cardiac Remodeling by Modulating the MAPK Signaling Pathway in Spontaneously Hypertensive Rats. Biomed. Pharmacother. 111, 695–704. doi:10.1016/j.biopha.2018.12.090
Liu, Y. L., Zhou, X. Y., and Xuan, L. J. (2019). Magnesium Lithospermate B Ameliorates Microcirculation Perfusion in Rats by Promoting Vascular NO Production via Activating the PI3K/AKT Pathway. Acta Pharmacol. Sin 40 (8), 1010–1018. doi:10.1038/s41401-018-0203-7
Lopaschuk, G. D., Karwi, Q. G., Tian, R., Wende, A. R., and Abel, E. D. (2021). Cardiac Energy Metabolism in Heart Failure. Circ. Res. 128 (10), 1487–1513. doi:10.1161/CIRCRESAHA.121.318241
Luan, A., Tang, F., Yang, Y., Lu, M., Wang, H., and Zhang, Y. (2015). Astragalus Polysaccharide Attenuates Isoproterenol-Induced Cardiac Hypertrophy by Regulating TNF-Α/pgc-1α Signaling Mediated Energy Biosynthesis. Environ. Toxicol. Pharmacol. 39 (3), 1081–1090. doi:10.1016/j.etap.2015.03.014
Luo, Y., Wan, Q., Xu, M., Zhou, Q., Chen, X., Yin, D., et al. (2019). Nutritional Preconditioning Induced by Astragaloside Ⅳ on Isolated Hearts and Cardiomyocytes against Myocardial Ischemia Injury via Improving Bcl-2-Mediated Mitochondrial Function. Chem. Biol. Interact 309, 108723. doi:10.1016/j.cbi.2019.06.036
Ma, L., Chuang, C. C., Weng, W., Zhao, L., Zheng, Y., Zhang, J., et al. (2016). Paeonol Protects Rat Heart by Improving Regional Blood Perfusion during No-Reflow. Front Physiol. 7, 298. doi:10.3389/fphys.2016.00298
Ma, X., Meng, Y., Wang, P., Tang, Z., Wang, H., and Xie, T. (2020). Bioinformatics-assisted, Integrated Omics Studies on Medicinal Plants. Brief Bioinform 21 (6), 1857–1874. doi:10.1093/bib/bbz132
Mak, S. K., Yu, C. M., Sun, W. T., He, G. W., Liu, X. C., and Yang, Q. (2017). Tetramethylpyrazine Suppresses Angiotensin II-Induced Soluble Epoxide Hydrolase Expression in Coronary Endothelium via Anti-ER Stress Mechanism. Toxicol. Appl. Pharmacol. 336, 84–93. doi:10.1016/j.taap.2017.10.016
Manolis, A. S., Manolis, A. A., Manolis, T. A., Apostolaki, N. E., Apostolopoulos, E. J., Melita, H., et al. (2021). Mitochondrial Dysfunction in Cardiovascular Disease: Current Status of Translational Research/clinical and Therapeutic Implications. Med. Res. Rev. 41 (1), 275–313. doi:10.1002/med.21732
Mao, J., Zhang, J., Lam, C. S. P., Zhu, M., Yao, C., Chen, S., et al. (2020). Qishen Yiqi Dripping Pills for Chronic Ischaemic Heart Failure: Results of the CACT-IHF Randomized Clinical Trial. ESC Heart Fail 7 (6), 3881–3890. doi:10.1002/ehf2.12980
Marzilli, M., Merz, C. N., Boden, W. E., Bonow, R. O., Capozza, P. G., Chilian, W. M., et al. (2012). Obstructive Coronary Atherosclerosis and Ischemic Heart Disease: an Elusive Link! J. Am. Coll. Cardiol. 60 (11), 951–956. doi:10.1016/j.jacc.2012.02.082
McDonagh, T. A., Metra, M., Adamo, M., Gardner, R. S., Baumbach, A., Böhm, M., et al. (2021). 2021 ESC Guidelines for the Diagnosis and Treatment of Acute and Chronic Heart Failure. Eur. Heart J. 42 (36), 3599–3726. doi:10.1093/eurheartj/ehab368
McMurray, J. J., Packer, M., Desai, A. S., Gong, J., Lefkowitz, M. P., Rizkala, A. R., et al. (2014). Angiotensin-neprilysin Inhibition versus Enalapril in Heart Failure. N. Engl. J. Med. 371 (11), 993–1004. doi:10.1056/NEJMoa1409077
McMurray, J. J. V., Solomon, S. D., Inzucchi, S. E., Køber, L., Kosiborod, M. N., Martinez, F. A., et al. (2019). Dapagliflozin in Patients with Heart Failure and Reduced Ejection Fraction. N. Engl. J. Med. 381 (21), 1995–2008. doi:10.1056/NEJMoa1911303
Meng, F. C., Wu, Z. F., Yin, Z. Q., Lin, L. G., Wang, R., and Zhang, Q. W. (2018). Coptidis Rhizoma and its Main Bioactive Components: Recent Advances in Chemical Investigation, Quality Evaluation and Pharmacological Activity. Chin Med. 13, 13. doi:10.1186/s13020-018-0171-3
Mi, L., Li, Y.-C., Sun, M.-R., Zhang, P.-L., Li, Y., and Yang, H. (2021). A Systematic Review of Pharmacological Activities, Toxicological Mechanisms and Pharmacokinetic Studies on Aconitum Alkaloids. Chin. J. Nat. medicines 19 (7), 505–520. doi:10.1016/S1875-5364(21)60050-X
Münzel, T., Camici, G. G., Maack, C., Bonetti, N. R., Fuster, V., and Kovacic, J. C. (2017). Impact of Oxidative Stress on the Heart and Vasculature: Part 2 of a 3-Part Series. J. Am. Coll. Cardiol. 70 (2), 212–229. doi:10.1016/j.jacc.2017.05.035
Münzel, T., Gori, T., Keaney, J. F., Maack, C., and Daiber, A. (2015). Pathophysiological Role of Oxidative Stress in Systolic and Diastolic Heart Failure and its Therapeutic Implications. Eur. Heart J. 36 (38), 2555–2564. doi:10.1093/eurheartj/ehv305
Murphy, S. P., Kakkar, R., McCarthy, C. P., and Januzzi, J. L. (2020). Inflammation in Heart Failure: JACC State-Of-The-Art Review. J. Am. Coll. Cardiol. 75 (11), 1324–1340. doi:10.1016/j.jacc.2020.01.014
Myung, S. K., Ju, W., Cho, B., Oh, S. W., Park, S. M., Koo, B. K., et al. (2013). Efficacy of Vitamin and Antioxidant Supplements in Prevention of Cardiovascular Disease: Systematic Review and Meta-Analysis of Randomised Controlled Trials. BMJ 346 (18), f10. doi:10.1136/bmj.f10
Ni, J., Li, Y., Xu, Y., and Guo, R. (2021). Salidroside Protects against Cardiomyocyte Apoptosis and Ventricular Remodeling by AKT/HO-1 Signaling Pathways in a Diabetic Cardiomyopathy Mouse Model. Phytomedicine 82, 153406. doi:10.1016/j.phymed.2020.153406
Nie, T., Zhao, S., Mao, L., Yang, Y., Sun, W., Lin, X., et al. (2018). The Natural Compound, Formononetin, Extracted from Astragalus Membranaceus Increases Adipocyte Thermogenesis by Modulating PPARγ Activity. Br. J. Pharmacol. 175 (9), 1439–1450. doi:10.1111/bph.14139
Nielsen, R., Møller, N., Gormsen, L. C., Tolbod, L. P., Hansson, N. H., Sorensen, J., et al. (2019). Cardiovascular Effects of Treatment with the Ketone Body 3-Hydroxybutyrate in Chronic Heart Failure Patients. Circulation 139 (18), 2129–2141. doi:10.1161/CIRCULATIONAHA.118.036459
Packer, M., Anker, S. D., Butler, J., Filippatos, G., Pocock, S. J., Carson, P., et al. (2020). Cardiovascular and Renal Outcomes with Empagliflozin in Heart Failure. N. Engl. J. Med. 383 (15), 1413–1424. doi:10.1056/NEJMoa2022190
Padro, T., Manfrini, O., Bugiardini, R., Canty, J., Cenko, E., De Luca, G., et al. (2020). ESC Working Group on Coronary Pathophysiology and Microcirculation Position Paper on 'coronary Microvascular Dysfunction in Cardiovascular Disease'. Cardiovasc. Res. 116 (4), 741–755. doi:10.1093/cvr/cvaa003
Pagliaro, B. R., Cannata, F., Stefanini, G. G., and Bolognese, L. (2020). Myocardial Ischemia and Coronary Disease in Heart Failure. Heart Fail Rev. 25 (1), 53–65. doi:10.1007/s10741-019-09831-z
Park, J. Y., Shin, H. K., Choi, Y. W., Lee, Y. J., Bae, S. S., Han, J., et al. (2009). Gomisin A Induces Ca2+-dependent Activation of eNOS in Human Coronary Artery Endothelial Cells. J. Ethnopharmacol 125 (2), 291–296. doi:10.1016/j.jep.2009.06.028
Peng, W., Qin, R., Li, X., and Zhou, H. (2013). Botany, phytochemistry, pharmacology, and potential application of Polygonum cuspidatum Sieb.et Zucc. a review. J. Ethnopharmacol 148 (3), 729–745. doi:10.1016/j.jep.2013.05.007
Ponikowski, P., Voors, A. A., Anker, S. D., Bueno, H., Cleland, J. G., Coats, A. J., et al. (2016). 2016 ESC Guidelines for the Diagnosis and Treatment of Acute and Chronic Heart Failure: The Task Force for the Diagnosis and Treatment of Acute and Chronic Heart Failure of the European Society of Cardiology (ESC). Developed with the Special Contribution of the Heart Failure Association (HFA) of the ESC. Eur. J. Heart Fail 18 (27), 891–975. doi:10.1093/eurheartj/ehw12810.1002/ejhf.592
Pries, A. R., and Reglin, B. (2017). Coronary Microcirculatory Pathophysiology: Can We Afford it to Remain a Black Box? Eur. Heart J. 38 (7), 478–488. doi:10.1093/eurheartj/ehv760
Qin, L., Wang, J., Zhao, R., Zhang, X., and Mei, Y. (2019). Ginsenoside-Rb1 Improved Diabetic Cardiomyopathy through Regulating Calcium Signaling by Alleviating Protein O-GlcNAcylation. J. Agric. Food Chem. 67 (51), 14074–14085. doi:10.1021/acs.jafc.9b05706
Radan, M., Dianat, M., Badavi, M., Mard, S. A., Bayati, V., and Goudarzi, G. (2019). Gallic Acid Protects Particulate Matter (PM10) Triggers Cardiac Oxidative Stress and Inflammation Causing Heart Adverse Events in Rats. Environ. Sci. Pollut. Res. Int. 26 (18), 18200–18207. doi:10.1007/s11356-019-05223-w
Ramalingam, A., Budin, S. B., Mohd Fauzi, N., Ritchie, R. H., and Zainalabidin, S. (2021). Targeting Mitochondrial Reactive Oxygen Species-Mediated Oxidative Stress Attenuates Nicotine-Induced Cardiac Remodeling and Dysfunction. Sci. Rep. 11 (1), 13845. doi:10.1038/s41598-021-93234-4
Reddy, A. M., Seo, J. H., Ryu, S. Y., Kim, Y. S., Kim, Y. S., Min, K. R., et al. (2004). Cinnamaldehyde and 2-methoxycinnamaldehyde as NF-kappaB Inhibitors from Cinnamomum cassia. Planta Med. 70 (9), 823–827. doi:10.1055/s-2004-827230
Reddy, Y. N. V., Borlaug, B. A., O'Connor, C. M., and Gersh, B. J. (2020). Novel Approaches to the Management of Chronic Systolic Heart Failure: Future Directions and Unanswered Questions. Eur. Heart J. 41 (18), 1764–1774. doi:10.1093/eurheartj/ehz364
Ren, B. C., Zhang, Y. F., Liu, S. S., Cheng, X. J., Yang, X., Cui, X. G., et al. (2020). Curcumin Alleviates Oxidative Stress and Inhibits Apoptosis in Diabetic Cardiomyopathy via Sirt1-Foxo1 and PI3K-Akt Signalling Pathways. J. Cell Mol Med 24 (21), 12355–12367. doi:10.1111/jcmm.15725
Rhee, A. J., and Lavine, K. J. (2020). New Approaches to Target Inflammation in Heart Failure: Harnessing Insights from Studies of Immune Cell Diversity. Annu. Rev. Physiol. 82, 1–20. doi:10.1146/annurev-physiol-021119-034412
Schink, A., Naumoska, K., Kitanovski, Z., Kampf, C. J., Fröhlich-Nowoisky, J., Thines, E., et al. (2018). Anti-inflammatory Effects of Cinnamon Extract and Identification of Active Compounds Influencing the TLR2 and TLR4 Signaling Pathways. Food Funct. 9 (11), 5950–5964. doi:10.1039/c8fo01286e
Selvaraj, S., Kelly, D. P., and Margulies, K. B. (2020). Implications of Altered Ketone Metabolism and Therapeutic Ketosis in Heart Failure. Circulation 141 (22), 1800–1812. doi:10.1161/CIRCULATIONAHA.119.045033
Shah, K. S., Xu, H., Matsouaka, R. A., Bhatt, D. L., Heidenreich, P. A., Hernandez, A. F., et al. (2017). Heart Failure with Preserved, Borderline, and Reduced Ejection Fraction: 5-Year Outcomes. J. Am. Coll. Cardiol. 70 (20), 2476–2486. doi:10.1016/j.jacc.2017.08.074
Shah, S. J., Lam, C. S. P., Svedlund, S., Saraste, A., Hage, C., Tan, R. S., et al. (2018). Prevalence and Correlates of Coronary Microvascular Dysfunction in Heart Failure with Preserved Ejection Fraction: PROMIS-HFpEF. Eur. Heart J. 39 (37), 3439–3450. doi:10.1093/eurheartj/ehy531
Shyu, K. G., Tsai, S. C., Wang, B. W., Liu, Y. C., and Lee, C. C. (2004). Saikosaponin C Induces Endothelial Cells Growth, Migration and Capillary Tube Formation. Life Sci. 76 (7), 813–826. doi:10.1016/j.lfs.2004.10.003
Song, F., Li, H., Sun, J., and Wang, S. (2013). Protective Effects of Cinnamic Acid and Cinnamic Aldehyde on Isoproterenol-Induced Acute Myocardial Ischemia in Rats. J. Ethnopharmacol 150 (1), 125–130. doi:10.1016/j.jep.2013.08.019
Stanley, W. C., Recchia, F. A., and Lopaschuk, G. D. (2005). Myocardial Substrate Metabolism in the normal and Failing Heart. Physiol. Rev. 85 (3), 1093–1129. doi:10.1152/physrev.00006.2004
Steinkamp-Fenske, K., Bollinger, L., Völler, N., Xu, H., Yao, Y., Bauer, R., et al. (2007). Ursolic Acid from the Chinese Herb Danshen (Salvia Miltiorrhiza L.) Upregulates eNOS and Downregulates Nox4 Expression in Human Endothelial Cells. Atherosclerosis 195 (1), e104–11. doi:10.1016/j.atherosclerosis.2007.03.028
Tan, Y. Y., Chen, L. X., Fang, L., and Zhang, Q. (2020). Cardioprotective Effects of Polydatin against Myocardial Injury in Diabetic Rats via Inhibition of NADPH Oxidase and NF-Κb Activities. BMC Complement Med. Ther. 20 (1), 378. doi:10.1186/s12906-020-03177-y
Tang, B., Zhang, J. G., Tan, H. Y., and Wei, X. Q. (2018). Astragaloside IV Inhibits Ventricular Remodeling and Improves Fatty Acid Utilization in Rats with Chronic Heart Failure. Biosci. Rep. 38 (3). doi:10.1042/BSR20171036
Tang, D. (2020). A Multicenter,randomized,double-Blind,placebo-Controlled Study of the Efficacy and Safety of Yangxinxue Granule in the Treatment of Chronic Heart Failure. Chengdu: Chengdu University of TCM.
Tang, J. Y., Li, S., Li, Z. H., Zhang, Z. J., Hu, G., Cheang, L. C., et al. (2010). Calycosin Promotes Angiogenesis Involving Estrogen Receptor and Mitogen-Activated Protein Kinase (MAPK) Signaling Pathway in Zebrafish and HUVEC. PloS one 5 (7), e11822. doi:10.1371/journal.pone.0011822
Tao, H., Wu, X., Cao, J., Peng, Y., Wang, A., Pei, J., et al. (2019). Rhodiola Species: A Comprehensive Review of Traditional Use, Phytochemistry, Pharmacology, Toxicity, and Clinical Study. Med. Res. Rev. 39 (5), 1779–1850. doi:10.1002/med.21564
Taqueti, V. R., Solomon, S. D., Shah, A. M., Desai, A. S., Groarke, J. D., Osborne, M. T., et al. (2018). Coronary Microvascular Dysfunction and Future Risk of Heart Failure with Preserved Ejection Fraction. Eur. Heart J. 39 (10), 840–849. doi:10.1093/eurheartj/ehx721
Tse, H. Y., Hui, M. N., Li, L., Lee, S. M., Leung, A. Y., and Cheng, S. H. (2012). Angiogenic Efficacy of Simplified 2-herb Formula (NF3) in Zebrafish Embryos In Vivo and Rat Aortic Ring In Vitro. J. Ethnopharmacol 139 (2), 447–453. doi:10.1016/j.jep.2011.11.031
Tu, L., Pan, C. S., Wei, X. H., Yan, L., Liu, Y. Y., Fan, J. Y., et al. (2013). Astragaloside IV Protects Heart from Ischemia and Reperfusion Injury via Energy Regulation Mechanisms. Microcirculation 20 (8), 736–747. doi:10.1111/micc.12074
Wang, B., Yang, Q., Sun, Y. Y., Xing, Y. F., Wang, Y. B., Lu, X. T., et al. (2014). Resveratrol-enhanced Autophagic Flux Ameliorates Myocardial Oxidative Stress Injury in Diabetic Mice. J. Cell Mol Med 18 (8), 1599–1611. doi:10.1111/jcmm.12312
Wang, D., Jie, Q., Liu, B., Li, Y., Dai, L., Luo, J., et al. (2017). Saponin Extract from Panax notoginseng Promotesangiogenesis through AMPK- and eNOS-dependent P-athways in HUVECs. Mol. Med. Rep. 16 (4), 5211–5218. doi:10.3892/mmr.2017.7280
Wang, D., Lv, L., Xu, Y., Jiang, K., Chen, F., Qian, J., et al. (2021). Cardioprotection of Panax Notoginseng Saponins against Acute Myocardial Infarction and Heart Failure through Inducing Autophagy. Biomed. Pharmacother. 136, 111287. doi:10.1016/j.biopha.2021.111287
Wang, J. R., Zhou, H., Yi, X. Q., Jiang, Z. H., and Liu, L. (2012). Total Ginsenosides of Radix Ginseng Modulates Tricarboxylic Acid Cycle Protein Expression to Enhance Cardiac Energy Metabolism in Ischemic Rat Heart Tissues. Molecules 17 (11), 12746–12757. doi:10.3390/molecules171112746
Wang, N. N., Xu, H. H., Zhou, W., Yang, H. X., Wang, J., Ma, Z. C., et al. (2021). Aconitine Attenuates Mitochondrial Dysfunction of Cardiomyocytes via Promoting Deacetylation of Cyclophilin-D Mediated by Sirtuin-3. J. Ethnopharmacol 270, 113765. doi:10.1016/j.jep.2020.113765
Wang, S. M., Ye, L. F., and Wang, L. H. (2020). Shenmai Injection Improves Energy Metabolism in Patients with Heart Failure: A Randomized Controlled Trial. Front Pharmacol. 11, 459. doi:10.3389/fphar.2020.00459
Wang, T., Liu, J., Luo, X., Hu, L., and Lu, H. (2021). Functional Metabolomics Innovates Therapeutic Discovery of Traditional Chinese Medicine Derived Functional Compounds. Pharmacol. Ther. 224, 107824. doi:10.1016/j.pharmthera.2021.107824
Wang, X., Chen, L., Wang, T., Jiang, X., Zhang, H., Li, P., et al. (2015). Ginsenoside Rg3 Antagonizes Adriamycin-Induced Cardiotoxicity by Improving Endothelial Dysfunction from Oxidative Stress via Upregulating the Nrf2-ARE Pathway through the Activation of Akt. Phytomedicine 22 (10), 875–884. doi:10.1016/j.phymed.2015.06.010
Wang, X., Hou, Y., Mao, J., Zhang, Y., Li, Z., Zhao, Y., et al. (2017a). Western Medication Plus Traditional Chinese Medicine Preparations in Patients with Chronic Heart Failure: a Prospective, Single-Blind, Randomized, Controlled, and Multicenter Clinical Trial. J. Tradit Chin Med. 37 (6), 756–766.
Wang, X., Hu, D., Dang, S., Huang, H., Huang, C. X., Yuan, M. J., et al. (2017b). Effects of Traditional Chinese Medicine Shensong Yangxin Capsules on Heart Rhythm and Function in Congestive Heart Failure Patients with Frequent Ventricular Premature Complexes: A Randomized, Double-Blind, Multicenter Clinical Trial. Chin Med. J. (Engl) 130 (14), 1639–1647. doi:10.4103/0366-6999.209906
Wang, X., Liu, Y., Xiao, L., Li, L., Zhao, X., Yang, L., et al. (2018). Hyperoside Protects against Pressure Overload-Induced Cardiac Remodeling via the AKT Signaling Pathway. Cell Physiol Biochem 51 (2), 827–841. doi:10.1159/000495368
Wang, X., Zhao, Z., Mao, J., Du, T., Chen, Y., Xu, H., et al. (20192019). Randomized, Double-Blinded, Multicenter, Placebo-Controlled Trial of Shenfu Injection for Treatment of Patients with Chronic Heart Failure during the Acute Phase of Symptom Aggravation (Yang and Qi Deficiency Syndrome). Evid. Based Complement Alternat Med. 2019, 9297163. doi:10.1155/2019/9297163
Wang, X. L., Wang, X., Xiong, L. L., Zhu, Y., Chen, H. L., Chen, J. X., et al. (2013). Salidroside Improves Doxorubicin-Induced Cardiac Dysfunction by Suppression of Excessive Oxidative Stress and Cardiomyocyte Apoptosis. J. Cardiovasc. Pharmacol. 62 (6), 512–523. doi:10.1097/FJC.0000000000000009
Wang, Z., Chen, Z., Zhang, L., Wang, X., Hao, G., Zhang, Z., et al. (2018). Status of Hypertension in China: Results from the China Hypertension Survey, 2012-2015. Circulation 137 (22), 2344–2356. doi:10.1161/CIRCULATIONAHA.117.032380
Wen, J., Ma, X., Niu, M., Hao, J., Huang, Y., Wang, R., et al. (2020a). Metabolomics Coupled with Integrated Approaches Reveal the Therapeutic Effects of Higenamine Combined with [6]-gingerol on Doxorubicin-Induced Chronic Heart Failure in Rats. Chin Med. 15 (1), 120. doi:10.1186/s13020-020-00403-0
Wen, J., Zhang, L., Liu, H., Wang, J., Li, J., Yang, Y., et al. (2019). Salsolinol Attenuates Doxorubicin-Induced Chronic Heart Failure in Rats and Improves Mitochondrial Function in H9c2 Cardiomyocytes. Front Pharmacol. 10, 1135. doi:10.3389/fphar.2019.01135
Wen, J., Zhang, L., Wang, J., Wang, J., Wang, L., Wang, R., et al. (2020b). Therapeutic Effects of Higenamine Combined with [6]-gingerol on Chronic Heart Failure Induced by Doxorubicin via Ameliorating Mitochondrial Function. J. Cell Mol Med 24 (7), 4036–4050. doi:10.1111/jcmm.15041
Wu, H., Liu, X., Gao, Z. Y., Dai, Z. F., Lin, M., Tian, F., et al. (2019). Anti-Myocardial Infarction Effects of Radix Aconiti Lateralis Preparata Extracts and Their Influence on Small Molecules in the Heart Using Matrix-Assisted Laser Desorption/Ionization-Mass Spectrometry Imaging. Int. J. Mol. Sci. 20 (19). doi:10.3390/ijms20194837
Wu, H., Sheng, Z. Q., Xie, J., Li, R., Chen, L., Li, G. N., et al. (20162016). Reduced HMGB 1-Mediated Pathway and Oxidative Stress in Resveratrol-Treated Diabetic Mice: A Possible Mechanism of Cardioprotection of Resveratrol in Diabetes Mellitus. Oxid Med. Cell Longev 2016, 9836860. doi:10.1155/2016/9836860
Wu, J., Sun, C., Wang, R., Li, J., Zhou, M., Yan, M., et al. (2018). Cardioprotective Effect of Paeonol against Epirubicin-Induced Heart Injury via Regulating miR-1 and PI3K/AKT Pathway. Chem. Biol. Interact 286, 17–25. doi:10.1016/j.cbi.2018.02.035
Wu, Y. Z., Zhang, L., Wu, Z. X., Shan, T. T., and Xiong, C. (2019). Berberine Ameliorates Doxorubicin-Induced Cardiotoxicity via a SIRT1/p66Shc-Mediated Pathway. Oxid Med. Cell Longev 2019, 2150394. doi:10.1155/2019/2150394
Xian, S., Yang, Z., Lee, J., Jiang, Z., Ye, X., Luo, L., et al. (2016). A Randomized, Double-Blind, Multicenter, Placebo-Controlled Clinical Study on the Efficacy and Safety of Shenmai Injection in Patients with Chronic Heart Failure. J. Ethnopharmacol 186, 136–142. doi:10.1016/j.jep.2016.03.066
Xian, S. X., Yang, Z. Q., Ren, P. H., Ye, X. H., Ye, S. L., Wang, Q. H., et al. (2015). Effect of Yangxinkang Tablets on Chronic Heart Failure: A Multi-center Randomized Double-Blind Placebo-Controlled Trial. Chin J. Integr. Med. 21 (10), 733–742. doi:10.1007/s11655-015-2170-x
Xiao, J., Sheng, X., Zhang, X., Guo, M., and Ji, X. (2016). Curcumin Protects against Myocardial Infarction-Induced Cardiac Fibrosis via SIRT1 Activation In Vivo and In Vitro. Drug Des. Devel Ther. 10, 1267–1277. doi:10.2147/DDDT.S104925
Xie, L., Wu, Y., Fan, Z., Liu, Y., and Zeng, J. (2016). Astragalus Polysaccharide Protects Human Cardiac Microvascular Endothelial Cells from Hypoxia/reoxygenation Injury: The Role of PI3K/AKT, Bax/Bcl-2 and Caspase-3. Mol. Med. Rep. 14 (1), 904–910. doi:10.3892/mmr.2016.5296
Xu, C., Wang, W., Wang, B., Zhang, T., Cui, X., Pu, Y., et al. (2019). Analytical Methods and Biological Activities of Panax Notoginseng Saponins: Recent Trends. J. Ethnopharmacol 236, 443–465. doi:10.1016/j.jep.2019.02.035
Xu, H. C., Cao, A. Q., Liu, S. J., Zhou, W. B., Pan, G. M., and Huang, G. B. (2011). A Randomized Controlled Trial of Astragalus Injection in the Treatment of Patients with Acute Decompensated Chronic Heart Failure and Qi-Deficiecy. J. Liaoning Univ. Traditional Chin. Med. 13 (01), 101–105. https://oversea.cnki.net/index/
Yan, B., Shen, M., Fang, J., Wei, D., and Qin, L. (2018). Advancement in the Chemical Analysis of Paeoniae Radix (Shaoyao). J. Pharm. Biomed. Anal 160, 276–288. doi:10.1016/j.jpba.2018.08.009
Yang, B., Li, H., Qiao, Y., Zhou, Q., Chen, S., Yin, D., et al. (2019). Tetramethylpyrazine Attenuates the Endotheliotoxicity and the Mitochondrial Dysfunction by Doxorubicin via 14-3-3γ/Bcl-2. Oxid Med. Cell Longev 2019, 5820415. doi:10.1155/2019/5820415
Yang, J. H., Obokata, M., Reddy, Y. N. V., Redfield, M. M., Lerman, A., and Borlaug, B. A. (2020). Endothelium-dependent and Independent Coronary Microvascular Dysfunction in Patients with Heart Failure with Preserved Ejection Fraction. Eur. J. Heart Fail 22 (3), 432–441. doi:10.1002/ejhf.1671
Yang, Y., Li, J., Rao, T., Fang, Z., and Zhang, J. (2021). The Role and Mechanism of Hyperoside against Myocardial Infarction in Mice by Regulating Autophagy via NLRP1 Inflammation Pathway. J. Ethnopharmacol 276, 114187. doi:10.1016/j.jep.2021.114187
Yu, H. Y., Wang, S. J., Teng, J. L., Ji, X. M., Wu, Z. C., Ma, Q. C., et al. (2012). Effects of Radix Aconiti Lateralis Preparata and Rhizoma Zingiberis on Energy Metabolism and Expression of the Genes Related to Metabolism in Rats. Chin J. Integr. Med. 18 (1), 23–29. doi:10.1007/s11655-012-0964-7
Yu, L., Li, Z., Dong, X., Xue, X., Liu, Y., Xu, S., et al. (20182018). Polydatin Protects Diabetic Heart against Ischemia-Reperfusion Injury via Notch1/Hes1-Mediated Activation of Pten/Akt Signaling. Oxid Med. Cell Longev 2018, 2750695. doi:10.1155/2018/2750695
Yu, W., Qin, X., Zhang, Y., Qiu, P., Wang, L., Zha, W., et al. (2020). Curcumin Suppresses Doxorubicin-Induced Cardiomyocyte Pyroptosis via a PI3K/Akt/mTOR-dependent Manner. Cardiovasc. Diagn. Ther. 10 (4), 752–769. doi:10.21037/cdt-19-707
Zeng, C., Zhong, P., Zhao, Y., Kanchana, K., Zhang, Y., Khan, Z. A., et al. (2015). Curcumin Protects Hearts from FFA-Induced Injury by Activating Nrf2 and Inactivating NF-Κb Both In Vitro and In Vivo. J. Mol. Cell Cardiol 79, 1–12. doi:10.1016/j.yjmcc.2014.10.002
Zhai, J., and Guo, Y. (2016). Paeoniflorin Attenuates Cardiac Dysfunction in Endotoxemic Mice via the Inhibition of Nuclear Factor-Κb. Biomed. Pharmacother. 80, 200–206. doi:10.1016/j.biopha.2016.03.032
Zhang, C., Fan, L., Fan, S., Wang, J., Luo, T., Tang, Y., et al. (2019). Cinnamomum cassia Presl: A Review of its Traditional Uses, Phytochemistry, Pharmacology and Toxicology. Molecules 24 (19), 3473. doi:10.3390/molecules24193473
Zhang, J., Liu, Y., and Liu, L. (2021). Hyperoside Prevents Sepsis-Associated Cardiac Dysfunction through Regulating Cardiomyocyte Viability and Inflammation via Inhibiting miR-21. Biomed. Pharmacother. 138, 111524. doi:10.1016/j.biopha.2021.111524
Zhang, L., Lu, X., Wang, J., Li, P., Li, H., Wei, S., et al. (2017). Zingiberis Rhizoma Mediated Enhancement of the Pharmacological Effect of Aconiti Lateralis Radix Praeparata against Acute Heart Failure and the Underlying Biological Mechanisms. Biomed. Pharmacother. 96, 246–255. doi:10.1016/j.biopha.2017.09.145
Zhang, S., Tang, F., Yang, Y., Lu, M., Luan, A., Zhang, J., et al. (2015). Astragaloside IV Protects against Isoproterenol-Induced Cardiac Hypertrophy by Regulating NF-Κb/pgc-1α Signaling Mediated Energy Biosynthesis. PloS one 10 (3), e0118759. doi:10.1371/journal.pone.0118759
Zhang, Y., Chen, L., Li, F., Wang, H., Yao, Y., Shu, J., et al. (2016). Cryptotanshinone Protects against Adriamycin-Induced Mitochondrial Dysfunction in Cardiomyocytes. Pharm. Biol. 54 (2), 237–242. doi:10.3109/13880209.2015.1029052
Zhang, Y., Ji, H., Qiao, O., Li, Z., Pecoraro, L., Zhang, X., et al. (2021). Nanoparticle Conjugation of Ginsenoside Rb3 Inhibits Myocardial Fibrosis by Regulating PPARα Pathway. Biomed. Pharmacother. 139, 111630. doi:10.1016/j.biopha.2021.111630
Zhang, Y., Li, X. R., Zhao, L., Duan, G. L., Xiao, L., and Chen, H. P. (2018). DJ-1 Preserving Mitochondrial Complex I Activity Plays a Critical Role in Resveratrol-Mediated Cardioprotection against Hypoxia/reoxygenation-Induced Oxidative Stress. Biomed. Pharmacother. 98, 545–552. doi:10.1016/j.biopha.2017.12.094
Zhang, Y., Murugesan, P., Huang, K., and Cai, H. (2020). NADPH Oxidases and Oxidase Crosstalk in Cardiovascular Diseases: Novel Therapeutic Targets. Nat. Rev. Cardiol. 17 (3), 170–194. doi:10.1038/s41569-019-0260-8
Zhao, C. C., Wu, X. Y., Yi, H., Chen, R., and Fan, G. (2021). The Therapeutic Effects and Mechanisms of Salidroside on Cardiovascular and Metabolic Diseases: An Updated Review. Chem. Biodiversity 18 (7), e2100033. doi:10.1002/cbdv.202100033
Zhao, H., Zhang, M., Zhou, F., Cao, W., Bi, L., Xie, Y., et al. (2016). Cinnamaldehyde Ameliorates LPS-Induced Cardiac Dysfunction via TLR4-NOX4 Pathway: The Regulation of Autophagy and ROS Production. J. Mol. Cell Cardiol 101, 11–24. doi:10.1016/j.yjmcc.2016.10.017
Zhong, J., Lu, W., Zhang, J., Huang, M., Lyu, W., Ye, G., et al. (2020). Notoginsenoside R1 Activates the Ang2/Tie2 Pathway to Promote Angiogenesis. Phytomedicine 78, 153302. doi:10.1016/j.phymed.2020.153302
Zhong, J., Ouyang, H., Sun, M., Lu, J., Zhong, Y., Tan, Y., et al. (2019). Tanshinone IIA Attenuates Cardiac Microvascular Ischemia-Reperfusion Injury via Regulating the SIRT1-Pgc1α-Mitochondrial Apoptosis Pathway. Cell Stress Chaperones 24 (5), 991–1003. doi:10.1007/s12192-019-01027-6
Zhou, B., and Tian, R. (2018). Mitochondrial Dysfunction in Pathophysiology of Heart Failure. J. Clin. Invest 128 (9), 3716–3726. doi:10.1172/JCI120849
Zhou, G., Tang, L., Zhou, X., Wang, T., Kou, Z., and Wang, Z. (2015). A Review on Phytochemistry and Pharmacological Activities of the Processed Lateral Root of Aconitum Carmichaelii Debeaux. J. Ethnopharmacol 160, 173–193. doi:10.1016/j.jep.2014.11.043
Zhou, H., Yang, H. X., Yuan, Y., Deng, W., Zhang, J. Y., Bian, Z. Y., et al. (2013). Paeoniflorin Attenuates Pressure Overload-Induced Cardiac Remodeling via Inhibition of TGFβ/Smads and NF-Κb Pathways. J. Mol. Histol. 44 (3), 357–367. doi:10.1007/s10735-013-9491-x
Zhou, W., Liu, H., Qiu, L. Z., Yue, L. X., Zhang, G. J., Deng, H. F., et al. (2021). Cardiac Efficacy and Toxicity of Aconitine: A New Frontier for the Ancient Poison. Med. Res. Rev. 41 (3), 1798–1811. doi:10.1002/med.21777
Zhou, Y., Murugan, D. D., Khan, H., Huang, Y., and Cheang, W. S. (2021). Roles and Therapeutic Implications of Endoplasmic Reticulum Stress and Oxidative Stress in Cardiovascular Diseases. Antioxidants (Basel) 10 (8), 1167. doi:10.3390/antiox10081167
Zhu, Y., Shi, Y. P., Wu, D., Ji, Y. J., Wang, X., Chen, H. L., et al. (2011). Salidroside Protects against Hydrogen Peroxide-Induced Injury in Cardiac H9c2 Cells via PI3K-Akt Dependent Pathway. DNA Cell Biol 30 (10), 809–819. doi:10.1089/dna.2010.1183
Zong, X., Yan, X., Wu, J. L., Liu, Z., Zhou, H., Li, N., et al. (2019). Potentially Cardiotoxic Diterpenoid Alkaloids from the Roots of Aconitum Carmichaelii. J. Nat. Prod. 82 (4), 980–989. doi:10.1021/acs.jnatprod.8b01039
Zuchi, C., Tritto, I., Carluccio, E., Mattei, C., Cattadori, G., and Ambrosio, G. (2020). Role of Endothelial Dysfunction in Heart Failure. Heart Fail Rev. 25 (1), 21–30. doi:10.1007/s10741-019-09881-3
14-3-3γ Tyrosine 3-monooxygenase/tryptophan 5-monooxygenase activation protein gamma
6MWD 6-min walking distance
ADP Adenosine diphosphate
ADRs Adverse drug reactions
AEC Adenylate energy charge
AEs Adverse events
AHF Acute heart failure
AKT Protein kinase B
AMP Adenosine monophosphate
AMPK 5′-adenosine monophosphat-activated protein kinase
ANG2 Angiogenin 2
AR Aldose reductase
ARE Antioxidant responsive element
ATP Adenosine-triphosphate
ATP5D ATP synthase, H+ transporting, mitochondrial F1 complex, delta subunit
BCAAs Branched-chain amino acids
BCL-2 B-cell lymphoma-2
BNP Brain natriuretic peptide
CaMKII Calcium/calmodulin-dependent protein kinase II
CAT Catalase
CCEs Composite cardiac events
CD36 Cluster of differentiation 36
CFR Coronary flow reserve
CHF Chronic heart failure
CHFQLS Quality of Life measured by the CHF Integrated Chinese and Western Medicine Survival Scale
CK Creatine kinase
CM Chinese medicine
CMD Coronary microvascular dysfunction
CYPD Cyclophilin D
DAMPs Damage-associated molecular patterns
DJ-1 Parkinsonism associated deglycase
DOX Doxorubicin
ECG Electrocardiograph
eNOS Endothelial nitric oxide synthase
ERK Extracellular-regulated kinase
FFAs Free fatty acids
FOXO1 Forkhead box O1
G3PD Glycerol-3-phosphate dehydrogenase
GMP Guanosine monophosphate
GSH Glutathione
GSK-3beta Glycogen synthase kinase 3 beta
H/R Hypoxia/reoxygenation
H3 Histone 3
HES1 Hairy and enhancer of split-1
HF Heart Failure
HFmrEF HF with mildly reduced ejection fraction
HFpEF HF with preserved ejection fraction
HFrEF HF with reduced ejection fraction
HMGB1 High-mobility group box 1
HO-1 Heme oxygenase-1
HS1 Heat stable protein 1
I/R Ischemia/reperfusion
IFN Interferon
IHD Ischemic heart disease
IHF Ischemic heart failure
IL Interleukin
iNOS Inducible nitric oxide synthase
INTER-CHF INTERnational Congestive Heart Failure
IRAK IL-1R-associated kinase
JAK2 Janus kinase 2
JNK c-Jun N-terminal kinase
LA Lactic acid
LDH Lactate dehydrogenase
LDHB L-lactate dehydrogenase B chain
LKB1 Liver kinase B1
LPE Lysophosphatidylethanolamine
LVED Left ventricular end-diastolic diameter
LVEDD Left ventricular end diastolic diameter
LVEF Left ventricular ejection fraction
LVIDd Left ventricular internal diastolic diameter
LVIDs Left ventricular internal dimension systole
MAPK Mitogen-activated protein kinase
MCAD Medium-chain acyl-CoA dehydrogenase
MCP Monocyte chemoattractant protein
MCPT1 Mast cell protease 1
MCU Mitochondrial calcium uniporter
MDA Malondialdehyde
MI Myocardial infarction
miR MicroRNA
MLHFQ Minnesota Living with Heart Failure Questionnaire
MMP Matrix metalloproteinase
mTOR Mechanistic target of rapamycin
NADPH Nicotinamide Adenine Dinucleotide Phosphate
NF-κB Nuclear factor kappa-B
NLRP3 Nucleotide-binding domain and leucine-rich repeat related family, pyrin domain containing 3
NO Nitric oxide
NOS Nitric oxide synthase
Notch1 Notch receptor 1
NOX NADPH oxidase
NQO1 NAD(P)H quinone dehydrogenase 1
NRF Nuclear factor-erythroid 2-related factor
NT-proBNP N-terminal pro-B-type natriuretic peptide
NYHA New York Heart Association
O-GlcNAcylation O-linked β--acetylglucosamine modification
p53 Tumor protein p53
p66Shc Src-homology-2-domain-containing transforming protein 1
p70S6K Ribosomal protein S6 kinase B1
PA Pyroracemic acid
PAMPs Pathogen-associated molecular patterns
PDC Pyruvate dehydrogenase complex
PGC1α Peroxisome proliferator-activated receptor gamma coactivator-1 alpha
PI3K Phosphatidylinositol 3-kinase
PPAR Peroxisome proliferator-activated receptor
PRRS Pattern-recognition receptors
PTEN Phosphatase and tensin homolog
RAB7 Member RAS oncogene family
RCT Randomized controlled trial
RHOA Ras homolog family member A
ROCK1 Rho associated coiled-coil containing protein kinase 1
ROS Reactive oxygen species
SAEs Serious adverse events
sEH Soluble epoxide hydrolase
SF-36 Short-form 36
SIRT Sirtuin
SOD Superoxide dismutase
STAT3 Signal transducer and activator of transcription 3
TAP Total adenylate pool
TCM Traditional Chinese Medicine
TFAM Transcription factor A, mitochondrial
TIE2 Tyrosine kinase with immunoglobin and epidermal growth factor homology domain 2
TLR Toll-like receptor
TNF-α Tumor necrosis factor-α
VCAM-1 Vascular cell adhesion molecule-1
VEGF Vascular endothelial growth factor
VEGFR2 Vascular endothelial growth factor receptor 2
VPCs Ventricular premature complexes
Keywords: heart failure, traditional Chinese medicine, microvascular circulation, myocardial energy metabolism, inflammation, oxidative stress
Citation: Wang A, Zhao W, Yan K, Huang P, Zhang H, Zhang Z, Zhang D and Ma X (2022) Mechanisms and Efficacy of Traditional Chinese Medicine in Heart Failure. Front. Pharmacol. 13:810587. doi: 10.3389/fphar.2022.810587
Received: 07 November 2021; Accepted: 24 January 2022;
Published: 24 February 2022.
Edited by:
Francesco Rossi, University of Campania Luigi Vanvitelli, ItalyReviewed by:
Alexander E. Berezin, Zaporizhia State Medical University, UkraineCopyright © 2022 Wang, Zhao, Yan, Huang, Zhang, Zhang, Zhang and Ma. This is an open-access article distributed under the terms of the Creative Commons Attribution License (CC BY). The use, distribution or reproduction in other forums is permitted, provided the original author(s) and the copyright owner(s) are credited and that the original publication in this journal is cited, in accordance with accepted academic practice. No use, distribution or reproduction is permitted which does not comply with these terms.
*Correspondence: Xiaochang Ma, bWF4aWFvY2hhbmdAeDI2Mw==
Disclaimer: All claims expressed in this article are solely those of the authors and do not necessarily represent those of their affiliated organizations, or those of the publisher, the editors and the reviewers. Any product that may be evaluated in this article or claim that may be made by its manufacturer is not guaranteed or endorsed by the publisher.
Research integrity at Frontiers
Learn more about the work of our research integrity team to safeguard the quality of each article we publish.