- 1Key Laboratory of Receptors-Mediated Gene Regulation, The People’s Hospital of Hebi, School of Medicine, Henan University, Kaifeng, China
- 2State Key Laboratory of Chemical Resource Engineering, College of Life Science and Technology, Beijing University of Chemical Technology, Beijing, China
Hepatocyte growth factor (HGF) is a peptide-containing multifunctional cytokine that acts on various epithelial cells to regulate cell growth, movement and morphogenesis, and tissue regeneration of injured organs. HGF is sequestered by heparin-like protein in its inactive form and is widespread in the extracellular matrix of most tissues. When the liver loses its average mass, volume, or physiological and biochemical functions due to various reasons, HGF binds to its specific receptor c-Met (cellular mesenchymal-epithelial transition) and transmits the signals into the cells, and triggers the intrinsic kinase activity of c-Met. The downstream cascades of HGF/c-Met include JAK/STAT3, PI3K/Akt/NF-κB, and Ras/Raf pathways, affecting cell proliferation, growth, and survival. HGF has important clinical significance for liver fibrosis, hepatocyte regeneration after inflammation, and liver regeneration after transplantation. And the development of HGF as a biological drug for regenerative therapy of diseases, that is, using recombinant human HGF protein to treat disorders in clinical trials, is underway. This review summarizes the recent findings of the HGF/c-Met signaling functions in liver regeneration.
Introduction
Hepatocyte growth factor (HGF) is secreted by mesenchymal cells and is expressed in different type cells such as hepatic stellate cells (HSC), vascular endothelial cells (ECs), and Kupffer cells (KCs). HGF is a specific ligand for the tyrosine kinase receptor c-Met (Miyazawa et al., 1989; Matsumoto and Nakamura, 1992; Trusolino et al., 2010). The HGF/c-Met pathway plays a crucial role in the protection and regeneration of tissues. In various models built for injury and disease, HGF promotes cell survival and tissue regeneration, and improves chronic inflammation and fibrosis (Michalopoulos and Khan, 2005; Jangphattananont et al., 2019). Simultaneously, HGF is always acted as a potent inducer of lymphangiogenesis, angiogenesis, and tumor growth through promoting tumor cell invasion and metastasis (Cienfuegos et al., 2014; Wang et al., 2020).
In 1984, Russell et al. identified and purified HGF in rat platelets (Nakamura et al., 1984; Russell et al., 1984; Nakamura et al., 1987), and Gohda et al. purified human HGF from the plasma of fulminant hepatic failure patients in 1988 (Gohda et al., 1988). In 1989, Nakamura et al. cloned the cDNA of human HGF and clarified the primary structure of HGF, and identified HGF as a novel growth factor that has a unique structural characteristic (Miyazawa et al., 1989; Nakamura et al., 1989). Then, researchers gradually found that the fibroblast-derived factor scatters factor (SF) and HGF have the same structure and function. They finally confirmed that they are identical proteins encoded by a single gene (Stoker et al., 1987; Gherardi et al., 1989; Weidner et al., 1990; Naldini et al., 1991a; Shima et al., 1991; Weidner et al., 1991; Ahn et al., 2019).
The HGF gene is mapped on the long arm of chromosome 7 of q21.1, and alternative splicing results in multiple transcripts, at least one of which encodes an inactive pre-pro-HGF. Then, the pre-pro-HGF becomes pro-HGF after a cleavage process between Arg494 and Val495. Mesenchymal cells produced HGF, which is a specific paracrine factor. And it is secreted out of the cells in the precursor form (pro-HGF) and also activated by the proteolytic cleavage at the Arg-Val site (Naka et al., 1992; Mizuno et al., 1994). The active HGF consists of 697 or 692 amino acids as a heterodimeric composed of α-chain and β-chain (Kirchhofer et al., 2004). Four kringle domains constitute the α-chain, while serine protease-like structures constitute the β-chain (Figures 1A,B). HGF activators reported previously include serum proteases and cell membrane proteases, such as HGF activator (HGF-A), urokinase-type plasminogen activator (uPA), plasma kallikrein, coagulation factors XII and XI, metalloproteinases and heparin. HGF-A is the central protease responsible for activating pro-HGF in serum (Fajardo-Puerta et al., 2016). However, the studies in HGF-A knockout mice have shown that HGF-A gene deletion does not affect liver regeneration (Fukushima et al., 2018). In contrast, uPA plays a crucial role in HGF activation during liver regeneration (Roselli et al., 1998).
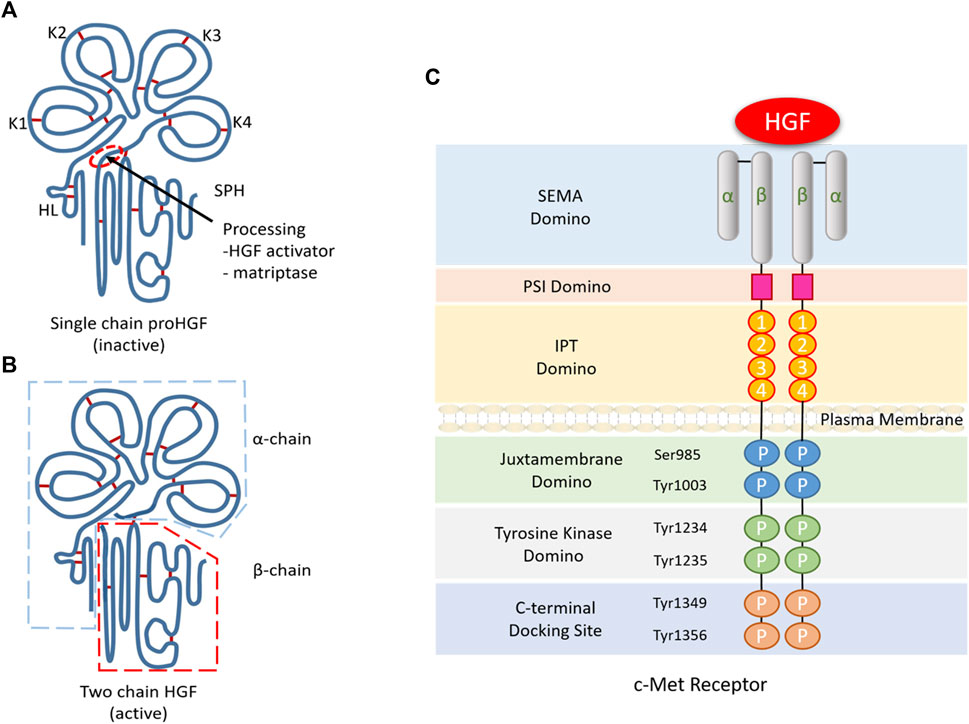
FIGURE 1. Structure and molecular signalling components of HGF/c-Met. (A). The α-chain of HGF is composed of an N-terminal hairpin loop (HL) attached four kringle domains (K1-K4), and the β-chain is composed of a serine protease homology domain (SPH) lacking proteolytic activity. (B). Active HGF is a heterodimeric molecule composed of an α-chain and a β-chain. (C). C-Met consists of a small α-chain and a larger β-chain, including extracellular, transmembrane and intracellular domains. The extracellular domain contains a large semaphore protein (SEMA) domain where HGF binds to c-Met. After the SEMA domain, a plexin, semaphorin and integrin-rich (PSI) domain and four immunoglobulin/plexin/transcriptional factors (IPT) domains make up the rest of the extracellular domains. Intracellularly, the C-terminal tail of c-Met β-chain containing two phosphorylation sites (Ser985 and Tyr1003), two tyrosine residues (Tyr1234 and Tyr1235) and a multisubstrate docking site (Tyr1349 and Tyr1356).
Unlike other growth factors and their receptors, HGF binds to the c-Met as a unique ligand (Bertotti and Comoglio, 2003; Birchmeier et al., 2003), which is also located on chromosome 7 (Bottaro et al., 1991; Naldini et al., 1991b; Dean et al., 1985). c-Met is a 190 kDa protein that contains a protein domain that binds to extracellular ligands, a transmembrane domain with tyrosine kinase activity, and a cytoplasmic domain. The c-Met has a 50 kDa β-chain and a 145 kDa α-chain for its mature form. The binding of HGF and c-Met can induce clustering of c-Met and phosphorylation of Y1234 and Y1235, and then phosphorylates Y1349 and Y1356 in the carboxy-terminal region (Figure 1C), which subsequently results in the adaptor molecule binding with it and transmitting downstream signals (Cecchi et al., 2012; Gherardi et al., 2012; Sakai et al., 2015). HGF has two individual MET-binding interfaces, which include a high affinity NK1 (N-terminal and first kringle domain) binding site and a low affinity β-chain binding site (Matsumoto et al., 2017).
The biological functions of HGF are extensive and diverse (Schmidt et al., 1995; Uehara et al., 1995; Samamé Pérez-Vargas et al., 2013; Cui, 2014; Maroun and Rowlands, 2014; Motoi et al., 2019; Mukai et al., 2020). In embryonic development, targeted destruction of HGF or c-Met could lead to mouse death in utero and damage the development of the liver and placenta (Schmidt et al., 1995; Uehara et al., 1995). Recombinant human HGF (rh-HGF) can significantly inhibit hepatocyte death and stabilize structural and vascular integrity in mice with acute liver failure (ALF) (Motoi et al., 2019). HGF receptor overexpression, mutation, amplification, or changes in its kinase activity is closely related to many different types of tumors (Samamé Pérez-Vargas et al., 2013; Cui, 2014; Maroun and Rowlands, 2014; Mukai et al., 2020).
HGF/c-Met signaling pathway is indispensable for liver regeneration and regular repair (Huh et al., 2004). Studies showed that the lack of c-Met leads to liver necrosis and jaundice, delays regeneration, and high mortality in rodents (Michalopoulos and DeFrances, 1997; Stolz et al., 1999; Borowiak et al., 2004; Huh et al., 2004). In the model of the normal rats and mice, injecting HGF into the portal vein caused hepatocyte proliferation and hepatomegaly (Liu et al., 1994a; Patijn et al., 1998). When recombinant human HGF-activator (rh-HGF-activator) was administered via the portal vein, compared with the control group, and the liver regeneration rate in the rh-HGF-activator-treated group was significantly higher (Kaibori et al., 2002). After partial hepatectomy (PHx), the tyrosine phosphorylation of c-Met occurs just in 5 min and increases gradually, then peaks at 60 min (Stolz et al., 1999). In vitro, the activated HGF binding to the c-Met drives the phosphorylation of c-Met tyrosine residues, then Wnt-independent nuclear translocation of β-catenin is induced by HGF (Monga et al., 2002). Subsequently, c-Met is internalized and degraded promptly by the ubiquitin-proteasome degradation pathway (Naka et al., 1993; Jeffers et al., 1997). In the initial stage of liver regeneration, HGF activates JAK/STAT3, PI3K/Akt/NF-κB, and Ras/Raf pathways via HGF/c-Met (Li et al., 2018a), and initiates cell proliferation.
In this review, we summarized the physiological and pathological mechanisms of the HGF/c-Met in liver regeneration. Specifically, we highlight the latest research on the HGF/c-Met axis, including the roles of non-coding RNAs in regulating of liver regeneration by HGF.
Changes of HGF After PHx
PHx in mice or rats is a mature model for the study of the mechanism of liver regeneration. After PHx (>70%) or liver injury, rapid recovery of liver volume and function and strict regulation at the beginning and end stages are unique features of the liver (Cienfuegos et al., 2014). After PHx on mice or rats, the residual liver tissue recovered to the same level both in mass and volume as before in just 1 week. Both single-chain HGF and two-chain (active form) HGF are present in normal liver, and the single-chain HGF is the dominant form, while the expression of HGF mRNA is almost undetectable (Pediaditakis et al., 2001). After PHx, the level of HGF in the plasma has increased 10 to 20 times (Lindroos et al., 1991; Liu et al., 1994a; Patijn et al., 1998). According to the changes in endogenous HGF expression levels, liver regeneration can be divided into two stages. In phase I, the depletion phase, 0–3 h after PHx, HGF was derived from the transcription of HGF gene in KCs and ECs of normal liver, with a decrease in the level of both single-chain HGF (inactive form) and active two-chain HGF (active form) as characteristic (Maher, 1993). In phase II, the production phase, from 3 to 48 h or more after PHx, HGF was newly synthesized by ECs and HSCs and characterized by a significant increase in both single-chain and two-chain HGF levels. In addition, only active two-chain HGF was detected in plasma during the first 3 h after PHx (Lindroos et al., 1991; Pediaditakis et al., 2001). At the end of the liver regeneration process, TGF-β plays a role in the extracellular matrix (ECM) reconstruction. And with the ECM rebuilds, TGF-β binds to HGF, blocking its activation and restoring the hepatocytes to stasis (Kogure et al., 2000; Ichikawa et al., 2001).
uPA Activation and HGF in Liver Regeneration
Another earliest biochemical change observed after PHx was the increased activity of uPA. Inactive pro-HGF was activated by uPA in the ECM (Shimizu et al., 2001). How do hemodynamic changes regulate the uPA activation is still unclear. However, uPA activation in such endothelial cells associated with the increased mechanical stress and turbulence have been documented (Sokabe et al., 2004).
We know that the microenvironment, including cells and ECM plays a crucial role in maintaining liver tissue homeostasis and regeneration (Fausto et al., 2006; Michalopoulos, 2010). The remodeling of ECM is accompanied by the renewal of many proteins. Urokinase is an activator for matrix remodeling and is detectable in tissues suffering wound healing and liver regeneration. After PHx, the uPA quantity remains relatively constant, but the uPA receptor (uPAR) dramatically increases after PHx, just beginning within 1 min. It enhances the activity of uPA in all remaining liver lobes (Mars et al., 1995; Kortlever and Bernards, 2006). Then, the uPAR is induced to activate the fibrinolytic cascade reaction, and the plasminogen is activated to plasmin within 15 min (Kim et al., 1997). Plasminase promotes the conversion of matrix metalloproteinases to active metalloproteinases at 30 min after PHx (Kim et al., 2000). Both metalloproteinase and plasmin play a role in matrix remodeling and the renewal of ECM. The remodeling of ECM triggers a signal impulse through integrin, which leads to activating the inactive HGF attached to the ECM into its active form. It causes local and systemic HGF receptor c-Met activation within 30 min to 1 h after PHx (Kim et al., 1997). So metalloproteinase and metalloproteinase tissue inhibitor levels are essential in regulating the release and activation of HGF during regeneration (Mohammed et al., 2005; Mohammed and Khokha, 2005).
A great deal of work focusing on the regulation of uPA activity in the initial stage of liver regeneration is underway. Recent reports show that the increased release of preformed HGF in the extracellular matrix during liver regeneration which supports liver regeneration, is due to the up-regulation of uPA by TLR3 (Stöß et al., 2020). And during hepatitis B virus infection, no matter in mouse liver tissues or human liver cell lines, HBx down-regulated expression of uPA by the uPA promoter epigenetic regulation, resulting in damage to liver regeneration (Park et al., 2013). Deletion of nuclear factor ImerC (NFI-C) leads to overexpression of plasminogen activator I (PAI-1) and subsequent inhibition of uPA activity and HGF signal, thus impairing hepatocyte proliferation (Edelmann et al., 2015). The hepatic hemodynamics changed immediately after PHx, and the portal vein pressure increased about three times theoretically (Marubashi et al., 2004). Still, the actual measurement showed that the portal vein pressure after PHx was only twice as much as that before operation (Xie et al., 2014). The increase of intravascular mechanical stress and turbulence promotes the activation of uPA in endothelial cells and other cells. This change regulates the production and release of HGF through β-1 integrin and vascular endothelial growth factor receptor 3 (VEGFR3) pathway. In addition, the permeability of the LSEC fenestra is heighten, and the nitric oxide secretion makes hepatocytes sensitive to HGF (Poisson et al., 2017).
HGF/C-Met Activates Multiple Signal Cascades in Liver Regeneration
HGF/c-Met axis has multiple effects in different cell types (Figure 2). The binding of C-Met to HGF initiates the receptor’s innate kinase activity, followed by the formation of a multisubstrate docking site for the intracellular junction protein, which recruits signaling molecules due to homologous dimerization and autophosphorylation at Tyr1234 and Tyr1235 and subsequent phosphorylation at Tyr1349 and Tyr1356 at the c-terminal tail (Birchmeier et al., 2003; Trusolino et al., 2010). Gab1 in the Gab family of docking proteins which can directly or indirectly bind to c-Met through Grb2, is the most critical downstream multi-adapter protein of c-Met (Birchmeier et al., 1997; Nguyen et al., 1997; Lock et al., 2002). The phosphorylated Gab1 creates additional binding sites for most downstream signaling molecules, which activate the different signaling pathways, including JAK/STAT3, phosphoinositide 3-kinase (PI3K)/Akt/NF-κB, Ras/Raf, and mitogen-activated protein kinase (MAPK) cascades.
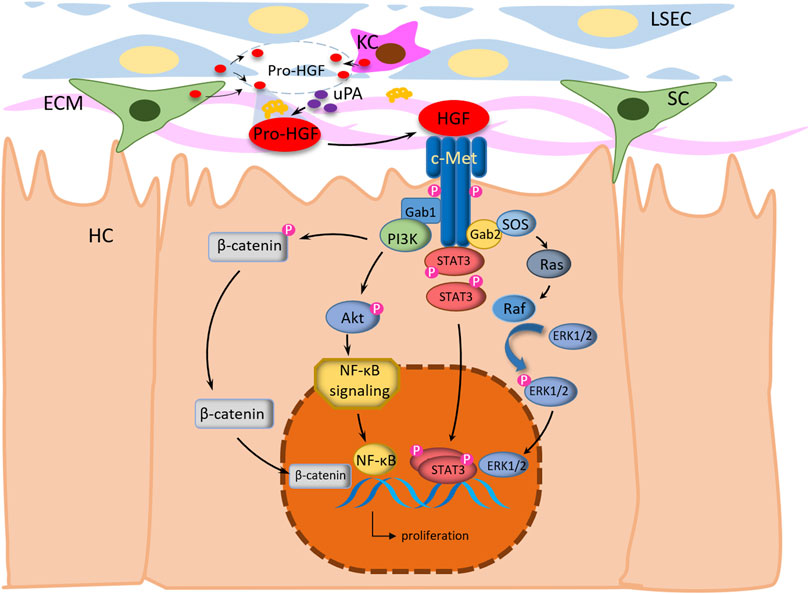
FIGURE 2. Molecular mechanism of HGF regulating liver regeneration after PHx. HGF is secreted by hepatic stellate cells (HSC), vascular endothelial cells (LECs) and Kupffer cells (KCs). Generally, HGF is sequestered by heparinlike proteoglycans in its inactive form and distributing widely in the extracellular matrix of most tissues. After PHx, inactive Pro-HGF is stored in the liver extracellular matrix (ECM) and can be activated by urokinase-type plasminogen activator (uPA). The activated HGF binding to the c-Met drives the phosphorylation of tyrosine residues and induces nuclear translocation of β-catenin in hepatocytes. In response to c-Met stimulation and the ensuing activation of PI3K, NF-κB signaling is activated, resulting in the nuclear translocation of NF-κB and transcription. Signal transducer and activator of transcription 3 (STAT3) monomers bind to c-Met and become trans-phosphorylated, followed by homodimerization and translocation into the nucleus for operate as a transcription factor. Meanwhile, the HGF/c-Met signaling activates Ras by the GRB2-SOS complex, then activates Raf to phosphorylate ERK1/2. Thus, HGF/c-Met signaling is transferred from extracellular to nucleus to activate transcription of a variety of transcription factors essential for liver regeneration.
Because of its rapid response to PHx, STAT3 is considered to be a trigger for liver regeneration. In addition, HGF promotes the phosphorylation of STAT3 to activate the STAT3 pathway (Li et al., 2018b). In mice, the activity of STAT3 DNA binding was observed within 30 min and peaked 3 h after PHx (Cressman et al., 1995). And this response is IL-6-dependent, and this STAT3 activation is almost inhibited in IL-6 deficient mice (Cressman et al., 1996). The STAT3 pathway regulates hepatocyte proliferation through holding cyclin D1/p21 and prevents cell death by up-regulating FLIP, Bcl-2, Bcl-xL, Ref1, and MnSOD (Fujiyoshi and Ozaki, 2011).
The PI3K/Akt pathway plays a vital role in regulating cell growth, migration, differentiation, and apoptosis (Vanhaesebroeck et al., 1999). When c-Met is activated by HGF, PI3K catalyzes the production of phosphatidylinositol-3,4,5-triphosphate after Gab1 coupled to the p85 subunit, which ultimately leads to phosphorylation of c-Met (Sheng et al., 2003; Watanabe et al., 2005).
In the Ras pathway, Erk1/2 as two mitogen-activated protein kinases to transmit mitotic signals, and the HGF/c-Met signaling pathway phosphorylates and activates Erk1/2 after PHx (Spector et al., 1997; Borowiak et al., 2004). Borowiak et al., 2004) found that when the liver regeneration was impaired, the phosphorylation of Erk1/2 was not detected in c-Met mutant mice. The results showed that specific signaling molecules, such as the protein kinase Akt, were fully activated by c-Met and other signaling receptors cooperators. The activation of ERK1/2 kinase was utterly dependent on c-Met during liver regeneration.
The MAPK signaling is activated in response to growth factors and cytokines to promote liver regeneration effectively and plays a dominant role in cellular responses, including proliferation, migration, and differentiation (Pagès et al., 1993; Cowley et al., 1994). HGF in PHx activates MAPK signaling to promote liver regeneration effectively (Xue et al., 2003). In addition, studies have shown that during the adult liver progenitor cells (HPC)/oval cells involved in the repair of chronically injured livers, the HGF/c-Met pathway plays a crucial contribution in regulating TGF-β-mediated epithelial-mesenchymal transition (EMT) responses. The coordination and balance of TGF-β and HGF promoted the optimal regenerative potential of HPCs (Almalé et al., 2019). These data indicate that the HGF/c-Met signaling system is essential for the regeneration of mature organs.
Regulation of HGF/C-Met Axis
The HGF/c-Met axis is strictly controlled by various mechanisms in normal tissues, and regulated by the amount of active HGF accurately. Two HGF protease inhibitors have been identified, HGF activator inhibitor (HAI)-1 and/or HAI-2, which control the activation of HGF ligand to limit the production of active HGF (Kaido et al., 2004; Kawaguchi and Kataoka, 2014). The HGF-specific antagonist NK4, which is similar to HGF, can bind to C-Met and competitively antagonize downstream phosphorylation induced by HGF (Date et al., 1998; Mizuno and Nakamura, 2013). In addition, the HGF/c-Met axis is regulated by the phosphorylation of the c-Met receptor. Many studies have shown that multiple pathways can negatively regulate the kinase activity of the c-Met receptor. For example, various protein tyrosine phosphatases negatively regulate the c-Met docking site by dephosphorylating c-Met receptor tyrosine residues (Trusolino et al., 2010). And c-Met monoubiquitination, internalization, and degradation could be triggered by casitas B lineage lymphoma ubiquitin ligase. Other studies have shown that changes in certain factors can regulate the expression of HGF and c-Met to regulate the HGF/c-Met axis. In vitro, the expression of HGF in hepatic stellate cells underwent a dramatic decrease under hypoxia, and the same as c-Met in hepatocytes (Corpechot et al., 2002). Human placental hydrolysate (hPH) treatment increased the secretion of HGF and promoted the proliferation of hepatocytes in rats after PHx (Lee et al., 2019). Gui et al. (2011) found that cytokine signaling inhibitory factor 1 (SOCS1) regulates hepatocyte proliferation through the HGF/c-Met axis. HGF stimulation-induced c-Met and Gab1 phosphorylation, cell migration, and proliferation in SOCS1 deficient mice hepatocytes. Later studies further showed that SOCS1 not only attenuates HGF/c-Met signaling but also regulates the expression of c-Met (Gui et al., 2015). In the model of chronic liver regeneration in rodents, Annalisa Addante et al. found that the bone morphogenetic protein (BMP)9 and HGF/c-Met signaling axes establish a signal crossover through ALK1 by modulating SMAD1 (pro-survival) and p38 mitogen-activated oval in kinase (p38MAPK; pro-apoptotic) determines the fate of oval cells (Addante et al., 2020). Another study showed that, the cellular transcription factor late SV40 factor (LSF) which is overexpressed in most of human hepatocellular carcinoma cases, is transcribed up-regulated osteopontin (OPN). Then, c-Met is activated via the potential interaction between OPN and its cell surface receptor CD44 (Yoo et al., 2011).
Under pathological conditions, many factors regulate cell proliferation by controlling the HGF/c-Met axis. Well known, the liver plays a vital and irreplaceable role in regulating physiological and biochemical balance. It is straightforward to cause pathological liver damage due to various factors such as inflammation and viral infection. Shubham et al. (2019) found that compared to ALF patients, in patients with acute chronic liver failure (ACLF), liver endothelial cells display a significant loss of CXC chemokine receptor type 7 DNA binding inhibitor 1 (CXCR7-ID1) dependent HGF expression, which correlates with the poor hepatocyte proliferation. Research proves that bacterial hepatocyte growth factor receptor agonist InlB321/15, a virulence factor produced by the pathogenic bacterium Listeria monocytogenes, improved hepatocyte proliferation and stimulated liver regeneration in animals with 70% hepatectomy (Kalinin et al., 2021).
In addition, some drugs, such as rosiglitazone and GW9662, the peroxisome proliferator-activated receptor gamma (PPARγ) agonist and antagonist, respectively, also regulate cell proliferation through the HGF/c-Met axis. Cheng et al. found that, PPARγ, a nuclear transcription factor with multiple biological functions, plays a important role in regulating cell cycle in liver regeneration (Cheng et al., 2018). They found that rosiglitazone inhibited liver regeneration while and GW9662 accelerated liver regeneration. Blocking the phosphorylation of c-Met by its inhibitor SGX523 could significantly eliminate the enhanced effect of PPARγ antagonist GW9662 on liver regeneration. Activation of HGF/c-Met pathways by phosphorylation of c-Met and ERK1/2 were inhibited in rosiglitazone-treated mice. It indicated that PPARγ hinders liver growth and hepatocyte proliferation by inhibiting the HGF/c-Met/ERK1/2 pathway. Researchers believe that these pathways may represent potential targets for liver disease in the future (Cheng et al., 2018). Schisandra Chinensis and Resina Draconis treatment significantly increased the expression levels of HGF, then promoted the recovery of liver function in liver regeneration model mice through the HGF/c-Met signaling pathway, and ameliorated acute liver injury by promoting liver cell proliferation (Li et al., 2018b; He et al., 2020). Another study showed that rapamycin could promote lens epithelial cells apoptosis by inhibiting HGF-induced phosphorylation of AKT/mTOR, ERK, and JAK2/STAT3 signaling molecules (Tian et al., 2014). At present, a synthetic small molecule, 1K1 has the effect of anti-fibrotic and promoting liver regeneration in rodents. It is an engineered form of naturally occurring HGF fragment NK1, which has the advantages of better stability and is easier to be produced and might be used as a therapeutic medicine for acute and chronic liver disease (Ross et al., 2012). Furthermore, age also plays a role in regulating HGF. It is well known that liver regeneration capacity will decrease with increasing age. Zhu et al. (2014) assessed 130 patients who underwent hepatectomy and found that HGF and c-Met expression in older patients was significantly lower than in younger patients. The average liver volume increase of young patients after 6 months of PHx is substantially more significant than that of elderly patients.
In recent years, there have been many reports on the roles of non-coding RNA (ncRNA) in regulating the HGF/c-Met axis, which are mainly focused on tumor-related studies. C-Met is the target gene of multiple miRNAs. These miRNAs mainly include the miR-34 family (including miR-34a, miR-34b, and miR-34c), miR-206, miR-1, and other tumor suppressor miRNAs. They inhibit cell cycle progression, epithelial-mesenchymal transition, metastasis, stem cell transformation, and angiogenesis and promote cell apoptosis by inhibiting the HGF/c-Met axis (Md Yusof et al., 2020). On the contrary, most lncRNAs targeting c-Met compete for endogenous RNAs (ceRNAs), decoys, or sponges, and combine with specific miRNAs to prevent the inhibition of c-Met, including ceRNAs: lnc-XIST, lnc-MALAT1, lnc-GAPLINC, lnc-HOTAIR targeting miR-34 (Md Yusof et al., 2020), LINC00240 targeting miR-4465 (Cui et al., 2019), LNCRNA ZFAS1 targeting miR-193a-3p (Bu et al., 2020), lncRNA UCA1 targeting miR-1271-5p (Yang and Chen, 2019), etc. However, the research on the mechanisms of ncRNAs in regulating the HGF/c-Met axis in the background of liver regeneration is still minimal. Recent studies have shown that HGF can up-regulate the expression of SNHG12 in regular liver cell lines and activate the Wnt/β-catenin signal pathway to promote hepatocyte proliferation and liver regeneration (Zhu et al., 2020).
The Roles of HGF/c-Met in Human Liver Regeneration
In human liver regeneration, limited by the means and purposes of clinical treatment, the researches on HGF are mainly carried out in the context of living donor liver transplantation rather than liver resection (Hoffmann et al., 2020). Studies have shown that HGF levels are significantly increased on days 1, 7, and 14 after living donor liver surgery and are related to the recipient liver volume on day 14 (Miki et al., 1996; de Jong et al., 2001; Dłuzniewska et al., 2002; Ninomiya et al., 2003; Efimova et al., 2005; Yoon et al., 2006; Justinger et al., 2008; Matsumoto et al., 2013; Sasturkar et al., 2016; Ray et al., 2018; Sparrelid et al., 2018). Matsumoto et al. (2013) reported that early-phase elevation of serum levels of various growth factors, including HGF, might be associated with the initiation of liver regeneration after hepatectomy in humans. At the same time, Tomiya et al. reported that serum HGF levels were increased after PHx, which was associated with hepatocellular dysfunction, necrosis, and systemic inflammation (Tomiya et al., 1992). On the other hand, Takeuchi et al. (1997) proved that the post hepatectomy bile HGF level might be more meaningful for the early assessment of post hepatectomy liver function, bile HGF level rather than serum HGF level is more closely related to the post hepatectomy liver failure (PHLF). Hoffmann et al. (2020) also suggested that bile HGF as a potentially functional marker of liver function after hepatectomy can be excreted from the liver at a higher concentration than that in serum (Appasamy et al., 1993; Liu et al., 1994b). Furthermore, Lehwald et al. (2014) showed that after hepatectomy, effective HSC mobilization and homing depends on HGF, and these findings have important implications for potential treatment strategies.
Discussion
The powerful regeneration potential of the liver is legendary, which is attributed to various precise and complicated network regulations. These factors cooperate to restore the metabolic and synthetic functions accurately and timely after liver injury or resection. HGF plays a vital role in the initial stage of liver repair.
HGF has been confirmed closely relating to liver repair after injury by various causes (chemical poison, infection, ischemia, physical injury or PHx, etc.). In animal experiments, HGF initiates liver regeneration at the beginning of the damage. The plasma HGF concentration changes throughout the repair progress, and HGF is maintained at a high concentration during the continuous regeneration phase until regeneration is complete. In clinical cases, the significant increase in HGF after live donor liver transplantation can be observed and related to the final liver regeneration volume. Without its specific receptor c-Met, HGF cannot play a role in liver regeneration. Furthermore, HGF combines with c-Met to trigger downstream cascade reactions and activate JAK/STAT3, PI3K/Akt/NF-κB, and Ras/Raf pathways, affecting cell proliferation, growth, and survival. The reports on HGF in human liver regeneration are minimal, and the use of HGF for drug development is challenging. Still, the preclinical studies currently have a large amount of data to support its therapeutic effects. Numerous clinical trials using rh-HGF protein or targeting HGF genes for clinical treatment are ongoing, which indicates that HGF may be a promising target for the therapies of human diseases.
Author Contributions
YZ and WY wrote the draft manuscript. W-DC and Y-DW edited and revised the manuscript.
Funding
This work is supported by the National Natural Science Foundation of China (Grant No. 81970551 and No. 81672433), the Fundamental Research Funds for the Central Universities (Grant No. PT2001) to Y-DW, the National Natural Science Foundation of China (Grant No. 81970726), Henan Provincial Natural Science Foundation (Grant No.182300410323), Program for Science & Technology Innovation Talents in Universities of Henan Province (HASTIT, Grant No. 13HASTIT024), Plan for Scientific Innovation Talent of Henan Province, and National College Student Innovation and Entrepreneurship Training Program (Grant No. 202010475032) to W-DC. Key project technology research Henan province department education (16A310006) to WY.
Conflict of Interest
The authors declare that the research was conducted in the absence of any commercial or financial relationships that could be construed as a potential conflict of interest.
Publisher’s Note
All claims expressed in this article are solely those of the authors and do not necessarily represent those of their affiliated organizations, or those of the publisher, the editors and the reviewers. Any product that may be evaluated in this article, or claim that may be made by its manufacturer, is not guaranteed or endorsed by the publisher.
References
Addante, A., Roncero, C., Lazcanoiturburu, N., Méndez, R., Almalé, L., García-Álvaro, M., et al. (2020). A Signaling Crosstalk between BMP9 and HGF/c-Met Regulates Mouse Adult Liver Progenitor Cell Survival. Cells 9, 752. doi:10.3390/cells9030752
Ahn, H. K., Kim, S., Kwon, D., Koh, J., Kim, Y. A., Kim, K., et al. (2019). MET Receptor Tyrosine Kinase Regulates the Expression of Co-stimulatory and Co-inhibitory Molecules in Tumor Cells and Contributes to PD-L1-Mediated Suppression of Immune Cell Function. Int. J. Mol. Sci. 20, 4287. doi:10.3390/ijms20174287
Almalé, L., García-Álvaro, M., Martínez-Palacián, A., García-Bravo, M., Lazcanoiturburu, N., Addante, A., et al. (2019). c-Met Signaling Is Essential for Mouse Adult Liver Progenitor Cells Expansion after Transforming Growth Factor-β-Induced Epithelial-Mesenchymal Transition and Regulates Cell Phenotypic Switch. Stem Cells 37, 1108–1118. doi:10.1002/stem.3038
Appasamy, R., Tanabe, M., Murase, N., Zarnegar, R., Venkataramanan, R., Van Thiel, D. H., et al. (1993). Hepatocyte Growth Factor, Blood Clearance, Organ Uptake, and Biliary Excretion in Normal and Partially Hepatectomized Rats. Lab. Invest. 68, 270–276.
Bertotti, A., and Comoglio, P. M. (2003). Tyrosine Kinase Signal Specificity: Lessons from the HGF Receptor. Trends. Biochem. Sci. 28, 527–533. doi:10.1016/j.tibs.2003.09.001
Birchmeier, C., Birchmeier, W., Gherardi, E., and Vande Woude, G. F. (2003). Met, Metastasis, Motility and More. Nat. Rev. Mol. Cel Biol. 4, 915–925. doi:10.1038/nrm1261
Birchmeier, W., Brinkmann, V., Niemann, C., Meiners, S., DiCesare, S., Naundorf, H., et al. (1997). Role of HGF/SF and C-Met in Morphogenesis and Metastasis of Epithelial Cells. Ciba Found. Symp. 212, 230–236. doi:10.1002/9780470515457.ch15
Borowiak, M., Garratt, A. N., Wüstefeld, T., Strehle, M., Trautwein, C., and Birchmeier, C. (2004). Met Provides Essential Signals for Liver Regeneration. Proc. Natl. Acad. Sci. U S A. 101, 10608–10613. doi:10.1073/pnas.0403412101
Bottaro, D. P., Rubin, J. S., Faletto, D. L., Chan, A. M., Kmiecik, T. E., Vande Woude, G. F., et al. (1991). Identification of the Hepatocyte Growth Factor Receptor as the C-Met Proto-Oncogene Product. Science 251, 802–804. doi:10.1126/science.1846706
Bu, W. J., Fang, Z., Li, W. L., Wang, X., Dong, M. J., Tao, Q. Y., et al. (2020). LINC00240 Sponges miR-4465 to Promote Proliferation, Migration, and Invasion of Hepatocellular Carcinoma Cells via HGF/c-MET Signaling Pathway. Eur. Rev. Med. Pharmacol. Sci. 24, 10452–10461. doi:10.26355/eurrev_202010_23397
Cecchi, F., Rabe, D. C., and Bottaro, D. P. (2012). Targeting the HGF/Met Signaling Pathway in Cancer Therapy. Expert Opin. Ther. Targets 16, 553–572. doi:10.1517/14728222.2012.680957
Cheng, Z., Liu, L., Zhang, X. J., Lu, M., Wang, Y., Assfalg, V., et al. (2018). Peroxisome Proliferator-Activated Receptor Gamma Negatively Regulates Liver Regeneration after Partial Hepatectomy via the HGF/c-Met/ERK1/2 Pathways. Sci. Rep. 8, 11894. doi:10.1038/s41598-018-30426-5
Cienfuegos, J. A., Rotellar, F., Baixauli, J., Martínez-Regueira, F., Pardo, F., and Hernández-Lizoáin, J. L. (2014). Liver Regeneration-the Best Kept Secret. A Model of Tissue Injury Response. Rev. Esp. Enferm. Dig. 106, 171–194.
Corpechot, C., Barbu, V., Wendum, D., Chignard, N., Housset, C., Poupon, R., et al. (2002). Hepatocyte Growth Factor and C-Met Inhibition by Hepatic Cell Hypoxia: a Potential Mechanism for Liver Regeneration Failure in Experimental Cirrhosis. Am. J. Pathol. 160, 613–620. doi:10.1016/S0002-9440(10)64881-X
Cowley, S., Paterson, H., Kemp, P., and Marshall, C. J. (1994). Activation of MAP Kinase Kinase Is Necessary and Sufficient for PC12 Differentiation and for Transformation of NIH 3T3 Cells. Cell 77, 841–852. doi:10.1016/0092-8674(94)90133-3
Cressman, D. E., Diamond, R. H., and Taub, R. (1995). Rapid Activation of the Stat3 Transcription Complex in Liver Regeneration. Hepatology 21, 1443–1449. doi:10.1002/hep.1840210531
Cressman, D. E., Greenbaum, L. E., DeAngelis, R. A., Ciliberto, G., Furth, E. E., Poli, V., et al. (1996). Liver Failure and Defective Hepatocyte Regeneration in Interleukin-6-Deficient Mice. Science 274, 1379–1383. doi:10.1126/science.274.5291.1379
Cui, J. J. (2014). Targeting Receptor Tyrosine Kinase MET in Cancer: Small Molecule Inhibitors and Clinical Progress. J. Med. Chem. 57, 4427–4453. doi:10.1021/jm401427c
Cui, X., Wang, Z., Liu, L., Liu, X., Zhang, D., Li, J., et al. (2019). The Long Non-coding RNA ZFAS1 Sponges miR-193a-3p to Modulate Hepatoblastoma Growth by Targeting RALY via HGF/c-Met Pathway. Front. Cel Dev. Biol. 7, 271. doi:10.3389/fcell.2019.00271
Date, K., Matsumoto, K., Kuba, K., Shimura, H., Tanaka, M., and Nakamura, T. (1998). Inhibition of Tumor Growth and Invasion by a Four-Kringle Antagonist (HGF/NK4) for Hepatocyte Growth Factor. Oncogene 17, 3045–3054. doi:10.1038/sj.onc.1202231
de Jong, K. P., von Geusau, B. A., Rottier, C. A., Bijzet, J., Limburg, P. C., de Vries, E. G., et al. (2001). Serum Response of Hepatocyte Growth Factor, Insulin-like Growth Factor-I, Interleukin-6, and Acute Phase Proteins in Patients with Colorectal Liver Metastases Treated with Partial Hepatectomy or Cryosurgery. J. Hepatol. 34, 422–427. doi:10.1016/s0168-8278(00)00030-1
Dean, M., Park, M., Le Beau, M. M., Robins, T. S., Diaz, M. O., Rowley, J. D., et al. (1985). The Human Met Oncogene Is Related to the Tyrosine Kinase Oncogenes. Nature 318, 385–388. doi:10.1038/318385a0
Dłuzniewska, J., Zolich, D., Polański, J., Zajac, L., Sitkiewicz, D., and Łukomska, B. (2002). Hepatocyte Growth Factor Levels in Liver and Blood, and Post-operative Liver Cell Proliferation in Patients with Benign and Malignant Liver Tumors after Partial Hepatectomy. Med. Sci. Monit. 8, CR690–6.
Edelmann, S., Fahrner, R., Malinka, T., Song, B. H., Stroka, D., and Mermod, N. (2015). Nuclear Factor I-C Acts as a Regulator of Hepatocyte Proliferation at the Onset of Liver Regeneration. Liver Int. 35, 1185–1194. doi:10.1111/liv.12697
Efimova, E. A., Glanemann, M., Nussler, A. K., Schumacher, G., Settmacher, U., Jonas, S., et al. (2005). Changes in Serum Levels of Growth Factors in Healthy Individuals after Living Related Liver Donation. Transpl. Proc 37, 1074–1075. doi:10.1016/j.transproceed.2004.12.170
Fajardo-Puerta, A. B., Mato Prado, M., Frampton, A. E., and Jiao, L. R. (2016). Gene of the Month: HGF. J. Clin. Pathol. 69, 575–579. doi:10.1136/jclinpath-2015-203575
Fausto, N., Campbell, J. S., and Riehle, K. J. (2006). Liver Regeneration. Hepatology 43, S45–S53. doi:10.1002/hep.20969
Fujiyoshi, M., and Ozaki, M. (2011). Molecular Mechanisms of Liver Regeneration and Protection for Treatment of Liver Dysfunction and Diseases. J. Hepatobiliary Pancreat. Sci. 18, 13–22. doi:10.1007/s00534-010-0304-2
Fukushima, T., Uchiyama, S., Tanaka, H., and Kataoka, H. (2018). Hepatocyte Growth Factor Activator: A Proteinase Linking Tissue Injury with Repair. Int. J. Mol. Sci. 19, 3435. doi:10.3390/ijms19113435
Gherardi, E., Birchmeier, W., Birchmeier, C., and Vande Woude, G. (2012). Targeting MET in Cancer: Rationale and Progress. Nat. Rev. Cancer 12, 89–103. doi:10.1038/nrc3205
Gherardi, E., Gray, J., Stoker, M., Perryman, M., and Furlong, R. (1989). Purification of Scatter Factor, a Fibroblast-Derived Basic Protein that Modulates Epithelial Interactions and Movement. Proc. Natl. Acad. Sci. U S A. 86, 5844–5848. doi:10.1073/pnas.86.15.5844
Gohda, E., Tsubouchi, H., Nakayama, H., Hirono, S., Sakiyama, O., Takahashi, K., et al. (1988). Purification and Partial Characterization of Hepatocyte Growth Factor from Plasma of a Patient with Fulminant Hepatic Failure. J. Clin. Invest. 81, 414–419. doi:10.1172/JCI113334
Gui, Y., Yeganeh, M., Donates, Y. C., Tobelaim, W. S., Chababi, W., Mayhue, M., et al. (2015). Regulation of MET Receptor Tyrosine Kinase Signaling by Suppressor of Cytokine Signaling 1 in Hepatocellular Carcinoma. Oncogene 34, 5718–5728. doi:10.1038/onc.2015.20
Gui, Y., Yeganeh, M., Ramanathan, S., Leblanc, C., Pomerleau, V., Ferbeyre, G., et al. (2011). SOCS1 Controls Liver Regeneration by Regulating HGF Signaling in Hepatocytes. J. Hepatol. 55, 1300–1308. doi:10.1016/j.jhep.2011.03.027
He, Z. Y., Lou, K. H., Zhao, J. H., Zhang, M., Zhang, L. C., Li, J., et al. (2020). Resina Draconis Reduces Acute Liver Injury and Promotes Liver Regeneration after 2/3 Partial Hepatectomy in Mice. Evid. Based Complement. Alternat Med. 2020, 2305784. doi:10.1155/2020/2305784
Hoffmann, K., Nagel, A. J., Tanabe, K., Fuchs, J., Dehlke, K., Ghamarnejad, O., et al. (2020). Markers of Liver Regeneration-The Role of Growth Factors and Cytokines: a Systematic Review. BMC Surg. 20, 31. doi:10.1186/s12893-019-0664-8
Huh, C. G., Factor, V. M., Sánchez, A., Uchida, K., Conner, E. A., and Thorgeirsson, S. S. (2004). Hepatocyte Growth Factor/c-Met Signaling Pathway Is Required for Efficient Liver Regeneration and Repair. Proc. Natl. Acad. Sci. U S A. 101, 4477–4482. doi:10.1073/pnas.0306068101
Ichikawa, T., Zhang, Y. Q., Kogure, K., Hasegawa, Y., Takagi, H., Mori, M., et al. (2001). Transforming Growth Factor Beta and Activin Tonically Inhibit DNA Synthesis in the Rat Liver. Hepatology 34, 918–925. doi:10.1053/jhep.2001.29132
Jangphattananont, N., Sato, H., Imamura, R., Sakai, K., Terakado, Y., Murakami, K., et al. (2019). Distinct Localization of Mature HGF from its Precursor Form in Developing and Repairing the Stomach. Int. J. Mol. Sci. 20, 2955. doi:10.3390/ijms20122955
Jeffers, M., Taylor, G. A., Weidner, K. M., Omura, S., and Vande Woude, G. F. (1997). Degradation of the Met Tyrosine Kinase Receptor by the Ubiquitin-Proteasome Pathway. Mol. Cel Biol. 17, 799–808. doi:10.1128/mcb.17.2.799
Justinger, C., Schlüter, C., Oliviera-Frick, V., Kopp, B., Rubie, C., and Schilling, M. K. (2008). Increased Growth Factor Expression after Hepatic and Pancreatic Resection. Oncol. Rep. 20, 1527–1531. doi:10.3892/or_00000175
Kaibori, M., Inoue, T., Oda, M., Naka, D., Kawaguchi, T., Kitamura, N., et al. (2002). Exogenously Administered HGF Activator Augments Liver Regeneration through the Production of Biologically Active HGF. Biochem. Biophys. Res. Commun. 290, 475–481. doi:10.1006/bbrc.2001.6170
Kaido, T., Oe, H., Yoshikawa, A., Okajima, A., and Imamura, M. (2004). Expressions of Molecules Associated with Hepatocyte Growth Factor Activation after Hepatectomy in Liver Cirrhosis. Hepatogastroenterology 51, 547–551.
Kalinin, E. V., Chalenko, Y. M., Sysolyatina, E. V., Midiber, K. Y., Gusarov, A. M., Kechko, O. I., et al. (2021). Bacterial Hepatocyte Growth Factor Receptor Agonist Stimulates Hepatocyte Proliferation and Accelerates Liver Regeneration in a Partial Hepatectomy Rat Model. Drug Dev. Res. 82, 123–132. doi:10.1002/ddr.21737
Kawaguchi, M., and Kataoka, H. (2014). Mechanisms of Hepatocyte Growth Factor Activation in Cancer Tissues. Cancers (Basel) 6, 1890–1904. doi:10.3390/cancers6041890
Kim, T. H., Mars, W. M., Stolz, D. B., and Michalopoulos, G. K. (2000). Expression and Activation of Pro-MMP-2 and Pro-MMP-9 during Rat Liver Regeneration. Hepatology 31, 75–82. doi:10.1002/hep.510310114
Kim, T. H., Mars, W. M., Stolz, D. B., Petersen, B. E., and Michalopoulos, G. K. (1997). Extracellular Matrix Remodeling at the Early Stages of Liver Regeneration in the Rat. Hepatology 26, 896–904. doi:10.1002/hep.510260415
Kirchhofer, D., Yao, X., Peek, M., Eigenbrot, C., Lipari, M. T., Billeci, K. L., et al. (2004). Structural and Functional Basis of the Serine Protease-like Hepatocyte Growth Factor Beta-Chain in Met Binding and Signaling. J. Biol. Chem. 279, 39915–39924. doi:10.1074/jbc.M404795200
Kogure, K., Zhang, Y. Q., Maeshima, A., Suzuki, K., Kuwano, H., and Kojima, I. (2000). The Role of Activin and Transforming Growth Factor-Beta in the Regulation of Organ Mass in the Rat Liver. Hepatology 31, 916–921. doi:10.1053/he.2000.6100
Kortlever, R. M., and Bernards, R. (2006). Senescence, Wound Healing and Cancer: the PAI-1 Connection. Cell Cycle 5, 2697–2703. doi:10.4161/cc.5.23.3510
Lee, T. H., Park, D. S., Jang, J. Y., Lee, I., Kim, J. M., Choi, G. S., et al. (2019). Human Placenta Hydrolysate Promotes Liver Regeneration via Activation of the Cytokine/Growth Factor-Mediated Pathway and Anti-oxidative Effect. Biol. Pharm. Bull. 42, 607–616. doi:10.1248/bpb.b18-00712
Lehwald, N., Duhme, C., Wildner, M., Kuhn, S., Fürst, G., Forbes, S. J., et al. (2014). HGF and SDF-1-Mediated Mobilization of CD133+ BMSC for Hepatic Regeneration Following Extensive Liver Resection. Liver Int. 34, 89–101. doi:10.1111/liv.12195
Li, N., Dou, Z., Liu, J., Chai, B., Li, Y., An, X., et al. (2018). Therapeutic Effect of HGF on NASH Mice through HGF/c-Met and JAK2-STAT3 Signalling Pathway. Ann. Hepatol. 17, 501–510. doi:10.5604/01.3001.0011.7395
Li, X., Sun, J., Fan, X., Guan, L., Li, D., Zhou, Y., et al. (2018b). Schisandrol B Promotes Liver Regeneration after Partial Hepatectomy in Mice. Eur. J. Pharmacol. 818, 96–102. doi:10.1016/j.ejphar.2017.10.044
Lindroos, P. M., Zarnegar, R., and Michalopoulos, G. K. (1991). Hepatocyte Growth Factor (Hepatopoietin A) Rapidly Increases in Plasma before DNA Synthesis and Liver Regeneration Stimulated by Partial Hepatectomy and Carbon Tetrachloride Administration. Hepatology 13, 743–750. doi:10.1002/hep.1840130422
Liu, M.-L., Mars, W. M., Zarnegar, R., and Michalopoulos, G. K. (1994). Collagenase Pretreatment and the Mitogenic Effects of Hepatocyte Growth Factor and Transforming Growth Factor-α in Adult Rat Liver. Hepatology 19, 1521–1527. doi:10.1002/hep.1840190630
Liu, M. L., Mars, W. M., Zarnegar, R., and Michalopoulos, G. K. (1994). Uptake and Distribution of Hepatocyte Growth Factor in Normal and Regenerating Adult Rat Liver. Am. J. Pathol. 144, 129–140. doi:10.1016/0378-1119(94)90376-x
Lock, L. S., Maroun, C. R., Naujokas, M. A., and Park, M. (2002). Distinct Recruitment and Function of Gab1 and Gab2 in Met Receptor-Mediated Epithelial Morphogenesis. Mol. Biol. Cel 13, 2132–2146. doi:10.1091/mbc.02-02-0031
Maher, J. J. (1993). Cell-specific Expression of Hepatocyte Growth Factor in Liver. Upregulation in Sinusoidal Endothelial Cells after Carbon Tetrachloride. J. Clin. Invest. 91, 2244–2252. doi:10.1172/JCI116451
Maroun, C. R., and Rowlands, T. (2014). The Met Receptor Tyrosine Kinase: a Key Player in Oncogenesis and Drug Resistance. Pharmacol. Ther. 142, 316–338. doi:10.1016/j.pharmthera.2013.12.014
Mars, W. M., Liu, M. L., Kitson, R. P., Goldfarb, R. H., Gabauer, M. K., and Michalopoulos, G. K. (1995). Immediate Early Detection of Urokinase Receptor after Partial Hepatectomy and its Implications for Initiation of Liver Regeneration. Hepatology 21, 1695–1701. doi:10.1002/hep.1840210631
Marubashi, S., Sakon, M., Nagano, H., Gotoh, K., Hashimoto, K., Kubota, M., et al. (2004). Effect of Portal Hemodynamics on Liver Regeneration Studied in a Novel Portohepatic Shunt Rat Model. Surgery 136, 1028–1037. doi:10.1016/j.surg.2004.03.012
Matsumoto, K., Miyake, Y., Umeda, Y., Matsushita, H., Matsuda, H., Takaki, A., et al. (2013). Serial Changes of Serum Growth Factor Levels and Liver Regeneration after Partial Hepatectomy in Healthy Humans. Int. J. Mol. Sci. 14, 20877–20889. doi:10.3390/ijms141020877
Matsumoto, K., and Nakamura, T. (1992). Hepatocyte Growth Factor: Molecular Structure, Roles in Liver Regeneration, and Other Biological Functions. Crit. Rev. Oncog. 3, 27–54.
Matsumoto, K., Umitsu, M., De Silva, D. M., Roy, A., and Bottaro, D. P. (2017). Hepatocyte Growth Factor/MET in Cancer Progression and Biomarker Discovery. Cancer Sci. 108, 296–307. doi:10.1111/cas.13156
Md Yusof, K., Rosli, R., Abdullah, M., and A Avery-Kiejda, K. (2020). The Roles of Non-coding RNAs in Tumor-Associated Lymphangiogenesis. Cancers (Basel) 12, 3290. doi:10.3390/cancers12113290
Michalopoulos, G. K., and DeFrances, M. C. (1997). Liver Regeneration. Science 276, 60–66. doi:10.1126/science.276.5309.60
Michalopoulos, G. K., and Khan, Z. (2005). Liver Regeneration, Growth Factors, and Amphiregulin. Gastroenterology 128, 503–506. doi:10.1053/j.gastro.2004.12.039
Michalopoulos, G. K. (2010). Liver Regeneration after Partial Hepatectomy: Critical Analysis of Mechanistic Dilemmas. Am. J. Pathol. 176, 2–13. doi:10.2353/ajpath.2010.090675
Miki, C., Iriyama, K., Strain, A., Harrison, J. D., Hirono, S., Gunson, B. K., et al. (1996). Clinical Significance of Serum Hepatocyte Growth Factor in Orthotopic Liver Transplantation. Surgery 119, 505–510. doi:10.1016/s0039-6060(96)80258-3
Miyazawa, K., Tsubouchi, H., Naka, D., Takahashi, K., Okigaki, M., Arakaki, N., et al. (1989). Molecular Cloning and Sequence Analysis of cDNA for Human Hepatocyte Growth Factor. Biochem. Biophys. Res. Commun. 163, 967–973. doi:10.1016/0006-291x(89)92316-4
Mizuno, K., Tanoue, Y., Okano, I., Harano, T., Takada, K., and Nakamura, T. (1994). Purification and Characterization of Hepatocyte Growth Factor (HGF)-converting Enzyme: Activation of Pro-HGF. Biochem. Biophys. Res. Commun. 198, 1161–1169. doi:10.1006/bbrc.1994.1164
Mizuno, S., and Nakamura, T. (2013). HGF-MET Cascade, a Key Target for Inhibiting Cancer Metastasis: the Impact of NK4 Discovery on Cancer Biology and Therapeutics. Int. J. Mol. Sci. 14, 888–919. doi:10.3390/ijms14010888
Mohammed, F. F., and Khokha, R. (2005). Thinking outside the Cell: Proteases Regulate Hepatocyte Division. Trends. Cel Biol. 15, 555–563. doi:10.1016/j.tcb.2005.08.009
Mohammed, F. F., Pennington, C. J., Kassiri, Z., Rubin, J. S., Soloway, P. D., Ruther, U., et al. (2005). Metalloproteinase Inhibitor TIMP-1 Affects Hepatocyte Cell Cycle via HGF Activation in Murine Liver Regeneration. Hepatology 41, 857–867. doi:10.1002/hep.20618
Monga, S. P., Mars, W. M., Pediaditakis, P., Bell, A., Mulé, K., Bowen, W. C., et al. (2002). Hepatocyte Growth Factor Induces Wnt-independent Nuclear Translocation of Beta-Catenin after Met-Beta-Catenin Dissociation in Hepatocytes. Cancer Res. 62, 2064–2071.
Motoi, S., Toyoda, H., Obara, T., Ohta, E., Arita, Y., Negishi, K., et al. (2019). Anti-Apoptotic Effects of Recombinant Human Hepatocyte Growth Factor on Hepatocytes Were Associated with Intrahepatic Hemorrhage Suppression Indicated by the Preservation of Prothrombin Time. Int. J. Mol. Sci. 20, 1821. doi:10.3390/ijms20081821
Mukai, S., Yamasaki, K., Fujii, M., Nagai, T., Terada, N., Kataoka, H., et al. (2020). Dysregulation of Type II Transmembrane Serine Proteases and Ligand-dependent Activation of MET in Urological Cancers. Int. J. Mol. Sci. 21, 2663. doi:10.3390/ijms21082663
Naka, D., Ishii, T., Yoshiyama, Y., Miyazawa, K., Hara, H., Hishida, T., et al. (1992). Activation of Hepatocyte Growth Factor by Proteolytic Conversion of a Single Chain Form to a Heterodimer. J. Biol. Chem. 267, 20114–20119. doi:10.1016/s0021-9258(19)88673-8
Naka, D., Shimomura, T., Yoshiyama, Y., Sato, M., Sato, M., Ishii, T., et al. (1993). Internalization and Degradation of Hepatocyte Growth Factor in Hepatocytes with Down-Regulation of the Receptor/c-Met. FEBS Lett. 329, 147–152. doi:10.1016/0014-5793(93)80212-d
Nakamura, T., Nawa, K., Ichihara, A., Kaise, N., and Nishino, T. (1987). Purification and Subunit Structure of Hepatocyte Growth Factor from Rat Platelets. FEBS Lett. 224, 311–316. doi:10.1016/0014-5793(87)80475-1
Nakamura, T., Nawa, K., and Ichihara, A. (1984). Partial Purification and Characterization of Hepatocyte Growth Factor from Serum of Hepatectomized Rats. Biochem. Biophys. Res. Commun. 122, 1450–1459. doi:10.1016/0006-291x(84)91253-1
Nakamura, T., Nishizawa, T., Hagiya, M., Seki, T., Shimonishi, M., Sugimura, A., et al. (1989). Molecular Cloning and Expression of Human Hepatocyte Growth Factor. Nature 342, 440–443. doi:10.1038/342440a0
Naldini, L., Vigna, E., Narsimhan, R. P., Gaudino, G., Zarnegar, R., Michalopoulos, G. K., et al. (1991). Hepatocyte Growth Factor (HGF) Stimulates the Tyrosine Kinase Activity of the Receptor Encoded by the Proto-Oncogene C-Met. Oncogene 6, 501–504.
Naldini, L., Weidner, K. M., Vigna, E., Gaudino, G., Bardelli, A., Ponzetto, C., et al. (1991). Scatter Factor and Hepatocyte Growth Factor Are Indistinguishable Ligands for the MET Receptor. EMBO J. 10, 2867–2878. doi:10.1002/j.1460-2075.1991.tb07836.x
Nguyen, L., Holgado-Madruga, M., Maroun, C., Fixman, E. D., Kamikura, D., Fournier, T., et al. (1997). Association of the Multisubstrate Docking Protein Gab1 with the Hepatocyte Growth Factor Receptor Requires a Functional Grb2 Binding Site Involving Tyrosine 1356. J. Biol. Chem. 272, 20811–20819. doi:10.1074/jbc.272.33.20811
Ninomiya, M., Harada, N., Shiotani, S., Hiroshige, S., Minagawa, R., Soejima, Y., et al. (2003). Hepatocyte Growth Factor and Transforming Growth Factor Beta1 Contribute to Regeneration of Small-For-Size Liver Graft Immediately after Transplantation. Transpl. Int. 16, 814–819. doi:10.1007/s00147-003-0628-9
Pagès, G., Lenormand, P., L'Allemain, G., Chambard, J. C., Meloche, S., and Pouysségur, J. (1993). Mitogen-Activated Protein Kinases P42mapk and P44mapk Are Required for Fibroblast Proliferation. Proc. Natl. Acad. Sci. U S A. 90, 8319–8323. doi:10.1073/pnas.90.18.8319
Park, E. S., Park, Y. K., Shin, C. Y., Park, S. H., Ahn, S. H., Kim, D. H., et al. (2013). Hepatitis B Virus Inhibits Liver Regeneration via Epigenetic Regulation of Urokinase-type Plasminogen Activator. Hepatology 58, 762–776. doi:10.1002/hep.26379
Patijn, G. A., Lieber, A., Schowalter, D. B., Schwall, R., and Kay, M. A. (1998). Hepatocyte Growth Factor Induces Hepatocyte Proliferation In Vivo and Allows for Efficient Retroviral-Mediated Gene Transfer in Mice. Hepatology 28, 707–716. doi:10.1002/hep.510280317
Pediaditakis, P., Lopez-Talavera, J. C., Petersen, B., Monga, S. P., and Michalopoulos, G. K. (2001). The Processing and Utilization of Hepatocyte Growth Factor/Scatter Factor Following Partial Hepatectomy in the Rat. Hepatology 34, 688–693. doi:10.1053/jhep.2001.27811
Poisson, J., Lemoinne, S., Boulanger, C., Durand, F., Moreau, R., Valla, D., et al. (2017). Liver Sinusoidal Endothelial Cells: Physiology and Role in Liver Diseases. J. Hepatol. 66, 212–227. doi:10.1016/j.jhep.2016.07.009
Ray, S., Mehta, N. N., Golhar, A., and Nundy, S. (2018). Post Hepatectomy Liver Failure - A Comprehensive Review of Current Concepts and Controversies. Ann. Med. Surg. (Lond) 34, 4–10. doi:10.1016/j.amsu.2018.08.012
Roselli, H. T., Su, M., Washington, K., Kerins, D. M., Vaughan, D. E., and Russell, W. E. (1998). Liver Regeneration Is Transiently Impaired in Urokinase-Deficient Mice. Am. J. Physiol. 275, G1472–G1479. doi:10.1152/ajpgi.1998.275.6.G1472
Ross, J., Gherardi, E., Mallorqui-Fernandez, N., Bocci, M., Sobkowicz, A., Rees, M., et al. (2012). Protein Engineered Variants of Hepatocyte Growth Factor/scatter Factor Promote Proliferation of Primary Human Hepatocytes and in Rodent Liver. Gastroenterology 142, 897–906. doi:10.1053/j.gastro.2011.12.006
Russell, W. E., McGowan, J. A., and Bucher, N. L. (1984). Partial Characterization of a Hepatocyte Growth Factor from Rat Platelets. J. Cel Physiol. 119, 183–192. doi:10.1002/jcp.1041190207
Sakai, K., Aoki, S., and Matsumoto, K. (2015). Hepatocyte Growth Factor and Met in Drug Discovery. J. Biochem. 157, 271–284. doi:10.1093/jb/mvv027
Samamé Pérez-Vargas, J. C., Biondani, P., Maggi, C., Gariboldi, M., Gloghini, A., Inno, A., et al. (2013). Role of cMET in the Development and Progression of Colorectal Cancer. Int. J. Mol. Sci. 14, 18056–18077. doi:10.3390/ijms140918056
Sasturkar, S. V., David, P., Sharma, S., Sarin, S. K., Trehanpati, N., and Pamecha, V. (2016). Serial Changes of Cytokines and Growth Factors in Peripheral Circulation after Right Lobe Donor Hepatectomy. Liver Transpl. 22, 344–351. doi:10.1002/lt.24373
Schmidt, C., Bladt, F., Goedecke, S., Brinkmann, V., Zschiesche, W., Sharpe, M., et al. (1995). Scatter Factor/Hepatocyte Growth Factor Is Essential for Liver Development. Nature 373, 699–702. doi:10.1038/373699a0
Sheng, H., Shao, J., Townsend, C. M., and Evers, B. M (2003). Phosphatidylinositol 3-Kinase Mediates Proliferative Signals in Intestinal Epithelial Cells. Gut 52, 1472–1478. doi:10.1136/gut.52.10.1472
Shima, N., Nagao, M., Ogaki, F., Tsuda, E., Murakami, A., and Higashio, K. (1991). Tumor Cytotoxic Factor/Hepatocyte Growth Factor from Human Fibroblasts: Cloning of its cDNA, Purification and Characterization of Recombinant Protein. Biochem. Biophys. Res. Commun. 180, 1151–1158. doi:10.1016/s0006-291x(05)81187-8
Shimizu, M., Hara, A., Okuno, M., Matsuno, H., Okada, K., Ueshima, S., et al. (2001). Mechanism of Retarded Liver Regeneration in Plasminogen Activator-Deficient Mice: Impaired Activation of Hepatocyte Growth Factor after Fas-Mediated Massive Hepatic Apoptosis. Hepatology 33, 569–576. doi:10.1053/jhep.2001.22650
Shubham, S., Kumar, D., Rooge, S., Maras, J. S., Maheshwari, D., Nautiyal, N., et al. (2019). Cellular and Functional Loss of Liver Endothelial Cells Correlates with Poor Hepatocyte Regeneration in Acute-On-Chronic Liver Failure. Hepatol. Int. 13, 777–787. doi:10.1007/s12072-019-09983-y
Sokabe, T., Yamamoto, K., Ohura, N., Nakatsuka, H., Qin, K., Obi, S., et al. (2004). Differential Regulation of Urokinase-type Plasminogen Activator Expression by Fluid Shear Stress in Human Coronary Artery Endothelial Cells. Am. J. Physiol. Heart Circ. Physiol. 287, H2027–H2034. doi:10.1152/ajpheart.00260.2004
Sparrelid, E., Johansson, H., Gilg, S., Nowak, G., Ellis, E., and Isaksson, B. (2018). Serial Assessment of Growth Factors Associated with Liver Regeneration in Patients Operated with Associating Liver Partition and Portal Vein Ligation for Staged Hepatectomy. Eur. Surg. Res. 59, 72–82. doi:10.1159/000488078
Spector, M. S., Auer, K. L., Jarvis, W. D., Ishac, E. J., Gao, B., Kunos, G., et al. (1997). Differential Regulation of the Mitogen-Activated Protein and Stress-Activated Protein Kinase Cascades by Adrenergic Agonists in Quiescent and Regenerating Adult Rat Hepatocytes. Mol. Cel Biol. 17, 3556–3565. doi:10.1128/mcb.17.7.3556
Stoker, M., Gherardi, E., Perryman, M., and Gray, J. (1987). Scatter Factor Is a Fibroblast-Derived Modulator of Epithelial Cell Mobility. Nature 327, 239–242. doi:10.1038/327239a0
Stolz, D. B., Mars, W. M., Petersen, B. E., Kim, T. H., and Michalopoulos, G. K. (1999). Growth Factor Signal Transduction Immediately after Two-Thirds Partial Hepatectomy in the Rat. Cancer Res. 59, 3954–3960.
Stöß, C., Laschinger, M., Wang, B., Lu, M., Altmayr, F., Hartmann, D., et al. (2020). TLR3 Promotes Hepatocyte Proliferation after Partial Hepatectomy by Stimulating uPA Expression and the Release of Tissue-Bound HGF. FASEB J. 34, 10387–10397. doi:10.1096/fj.202000904R
Takeuchi, E., Nimura, Y., Nagino, M., Kurumiya, Y., Maeda, A., Kamiya, J., et al. (1997). Human Hepatocyte Growth Factor in Bile: an Indicator of Posthepatectomy Liver Function in Patients with Biliary Tract Carcinoma. Hepatology 26, 1092–1099. doi:10.1053/jhep.1997.v26.pm0009362347
Tian, F., Dong, L., Zhou, Y., Shao, Y., Li, W., Zhang, H., et al. (2014). Rapamycin-Induced Apoptosis in HGF-Stimulated Lens Epithelial Cells by AKT/mTOR, ERK and JAK2/STAT3 Pathways. Int. J. Mol. Sci. 15, 13833–13848. doi:10.3390/ijms150813833
Tomiya, T., Tani, M., Yamada, S., Hayashi, S., Umeda, N., and Fujiwara, K. (1992). Serum Hepatocyte Growth Factor Levels in Hepatectomized and Nonhepatectomized Surgical Patients. Gastroenterology 103, 1621–1624. doi:10.1016/0016-5085(92)91186-8
Trusolino, L., Bertotti, A., and Comoglio, P. M. (2010). MET Signalling: Principles and Functions in Development, Organ Regeneration and Cancer. Nat. Rev. Mol. Cel Biol. 11, 834–848. doi:10.1038/nrm3012
Uehara, Y., Minowa, O., Mori, C., Shiota, K., Kuno, J., Noda, T., et al. (1995). Placental Defect and Embryonic Lethality in Mice Lacking Hepatocyte Growth Factor/Scatter Factor. Nature 373, 702–705. doi:10.1038/373702a0
Vanhaesebroeck, B., Jones, G. E., Allen, W. E., Zicha, D., Hooshmand-Rad, R., Sawyer, C., et al. (1999). Distinct PI(3)Ks Mediate Mitogenic Signalling and Cell Migration in Macrophages. Nat. Cel Biol. 1, 69–71. doi:10.1038/9045
Wang, H., Rao, B., Lou, J., Li, J., Liu, Z., Li, A., et al. (2020). The Function of the HGF/c-Met Axis in Hepatocellular Carcinoma. Front Cel Dev Biol 8, 55. doi:10.3389/fcell.2020.00055
Watanabe, H., Saito, H., Rychahou, P. G., Uchida, T., and Evers, B. M. (2005). Aging Is Associated with Decreased Pancreatic Acinar Cell Regeneration and Phosphatidylinositol 3-Kinase/Akt Activation. Gastroenterology 128, 1391–1404. doi:10.1053/j.gastro.2005.03.016
Weidner, K. M., Arakaki, N., Hartmann, G., Vandekerckhove, J., Weingart, S., Rieder, H., et al. (1991). Evidence for the Identity of Human Scatter Factor and Human Hepatocyte Growth Factor. Proc. Natl. Acad. Sci. U S A. 88, 7001–7005. doi:10.1073/pnas.88.16.7001
Weidner, K. M., Behrens, J., Vandekerckhove, J., and Birchmeier, W. (1990). Scatter Factor: Molecular Characteristics and Effect on the Invasiveness of Epithelial Cells. J. Cel Biol. 111, 2097–2108. doi:10.1083/jcb.111.5.2097
Xie, C., Wei, W., Zhang, T., Dirsch, O., and Dahmen, U. (2014). Monitoring of Systemic and Hepatic Hemodynamic Parameters in Mice. J. Vis. Exp. 92, e51955. doi:10.3791/51955
Xue, F., Takahara, T., Yata, Y., Kuwabara, Y., Shinno, E., Nonome, K., et al. (2003). Hepatocyte Growth Factor Gene Therapy Accelerates Regeneration in Cirrhotic Mouse Livers after Hepatectomy. Gut 52, 694–700. doi:10.1136/gut.52.5.694
Yang, Y., and Chen, L. (2019). Downregulation of lncRNA UCA1 Facilitates Apoptosis and Reduces Proliferation in Multiple Myeloma via Regulation of the miR-1271-5p/HGF axis. J. Chin. Med. Assoc. 82, 699–709. doi:10.1097/JCMA.0000000000000145
Yoo, B. K., Gredler, R., Chen, D., Santhekadur, P. K., Fisher, P. B., and Sarkar, D. (2011). c-Met Activation through a Novel Pathway Involving Osteopontin Mediates Oncogenesis by the Transcription Factor LSF. J. Hepatol. 55, 1317–1324. doi:10.1016/j.jhep.2011.02.036
Yoon, S. S., Kim, S. H., Gonen, M., Heffernan, N. M., Detwiller, K. Y., Jarnagin, W. R., et al. (2006). Profile of Plasma Angiogenic Factors before and after Hepatectomy for Colorectal Cancer Liver Metastases. Ann. Surg. Oncol. 13, 353–362. doi:10.1245/ASO.2006.03.060
Zhu, C., Ikemoto, T., Utsunomiya, T., Yamada, S., Morine, Y., Imura, S., et al. (2014). Senescence-Related Genes Possibly Responsible for Poor Liver Regeneration after Hepatectomy in Elderly Patients. J. Gastroenterol. Hepatol. 29, 1102–1108. doi:10.1111/jgh.12468
Keywords: HGF, c-Met, liver regeneration, uPA, cytokine
Citation: Zhao Y, Ye W, Wang Y-D and Chen W-D (2022) HGF/c-Met: A Key Promoter in Liver Regeneration. Front. Pharmacol. 13:808855. doi: 10.3389/fphar.2022.808855
Received: 04 November 2021; Accepted: 11 February 2022;
Published: 17 March 2022.
Edited by:
Leo A van Grunsven, Vrije University Brussel, BelgiumReviewed by:
Prasanna K. Santhekadur, JSS Academy of Higher Education and Research, IndiaCopyright © 2022 Zhao, Ye, Wang and Chen. This is an open-access article distributed under the terms of the Creative Commons Attribution License (CC BY). The use, distribution or reproduction in other forums is permitted, provided the original author(s) and the copyright owner(s) are credited and that the original publication in this journal is cited, in accordance with accepted academic practice. No use, distribution or reproduction is permitted which does not comply with these terms.
*Correspondence: Yan-Dong Wang, ydwangbuct2009@163.com; Wei-Dong Chen, wdchen666@163.com
†These authors have contributed equally to this work