- 1Engineering Research Center of Cell and Therapeutic Antibody, MOE, School of Pharmacy, Shanghai Jiao Tong University, Shanghai, China
- 2Jecho Institute, Co. Ltd., Shanghai, China
- 3Jecho Biopharmaceuticals Co. Ltd., Tianjin, China
- 4School of Science, and School of Interprofessional Health Studies, Faculty of Health and Environmental Sciences, Auckland University of Technology, Auckland, New Zealand
- 5Xinjiang Key Laboratory of Biological Resources and Genetic Engineering, College of Life Science and Technology, Xinjiang University, Urumqi, China
- 6Key Laboratory of Medical Molecular Virology (MOE/NHC/CAMS), School of Basic Medical Sciences, Fudan University, Shanghai, China
- 7Jecho Laboratories, Inc., Frederick, MD, United States
Background: Colorectal cancer is a commonly diagnosed cancer with high mortality worldwide. Postoperative recidivation and metastasis still are the main challenges in clinical treatments. Thus, it is urgent to develop new therapies against colorectal cancer. Epithelial Cell Adhesion Molecule (EpCAM) is overexpressed in colorectal cancer cells and strongly associated with cancer development. Bispecific antibody (BsAb) is a kind of promising immunotherapy, which could recognize T cells and cancer cells simultaneously to achieve the anti-tumor effects.
Methods: A bispecific antibody targeting EpCAM and CD3 with IgG format was genereated by split intein based on the Bispecific Antibody by Protein Splicing” platform. In vitro, the affinity of CD3×EpCAM BsAb was determined by Biolayer interferometry, its cytotoxicity was detected by LDH release assay, T cell recruitment and activation was detected by Flow Cytometry. In vivo, its pharmacokinetic parameters were detected, and anti-tumor effects were evaluated on the tumor cell xenograft mouse model.
Results: The results showed that the CD3×EpCAM BsAb could activate and recruit T cells via binding colorectal cells and T cells, which could lead to more potent cytotoxicity to various colorectal cell lines than its parent EpCAM monoclonal antibody (mAb) in vitro. The CD3×EpCAM BsAb had similar pharmacokinetic parameters with EpCAM mAb and inhibits tumor growth on the SW480 tumor cell xenograft mouse model.
Conclusion: The CD3×EpCAM BsAb could be a promising candidate for colorectal cancer treatment.
Introduction
Colorectal cancer (CRC) is one of the most common cancers with a low 5-years survival rate worldwide (Siegel et al., 2021; Sung et al., 2021). CRC develops from normal mucosa to an invasive tumor through different stages (Argilés et al., 2020). Even though surgery and chemotherapy are standard treatments, chemotherapy could negatively affect normal tissue (Nordlinger et al., 2009). Meanwhile, patients with metastatic colorectal cancer have a poor prognosis, and tumor recurrence is possible within 5 years after surgery (Seo et al., 2013; Van Cutsem et al., 2016). Therefore, a novel therapeutic for colorectal cancer is urgently needed.
In recent years, immunotherapies for CRC have been developed, including bispecific antibodies (BsAb), checkpoint inhibitors, cancer vaccines, and oncolytic virus therapy (Ciardiello et al., 2019). Engineered antibodies are an important part of immunotherapy (Zhu, 2012). The bispecific antibody is an engineering antibody with two different binding targets or epitopes for multiple applications (Brinkmann and Kontermann, 2021). It could exhibit a novel action or mechanism that cannot be achieved by combining separate antibodies with the same targets (Labrijn et al., 2019), such as redirecting T cells to tumor cells (Bargou et al., 2008), activating or inhibiting two receptors (Schaefer et al., 2011), serving as cofactor mimetic (Sampei et al., 2013), or even delivering a protein across the blood-brain barrier (Yu et al., 2011; Yu et al., 2014). Recruiting effector cells to eliminate tumor cells was the typical application of BsAb in immunotherapies. The European Medicines Agency approved the first commercial bispecific antibody targeting CD3 and EpCAM for malignant ascites, followed by FDA approval of Blinatumomab targeting CD3 and CD19 for acute lymphoblastic leukemia, Emicizumab targeting factors IXa and X for Hemophilia A, and Amiivantamab targeting EGFR and cMet for non-small cell lung cancer (Heiss et al., 2010; Blair, 2019; Stein et al., 2019; Neijssen et al., 2021). However, compared to the success of hematological malignancies, solid tumor treatment is still complex in clinical trials.
Important expression characteristics and functional roles contribute to EpCAM (CD326) to an attractive target for immunotherapy (Chaudry et al., 2007; Eyvazi et al., 2018; Song et al., 2021). On the one hand, it is a highly expressed glycoprotein on most carcinomas and slightly expressed on normal tissues. On the other hand, it is crucial for tissue morphogenesis and stable adhesion formation, and it is associated with epithelial cell proliferation, differentiation, and migration (Munz et al., 2004; Went et al., 2006). Compared with the anti-EpCAM monoclonal antibody (de Bono et al., 2004), CD3×EpCAM BsAb (Catumaxomab and MT110) exhibited a better efficacy against EpCAM-positive cancer in clinical trials (Heiss et al., 2010; Münz et al., 2010; Kebenko et al., 2018). Nevertheless, while catumaxomab can drive the immune system to kill tumor cells, it also has serious side effects due to its Fc region’s off-target binding to FcγR+ Kuffer cells (Borlak et al., 2016).
The property of the bispecific antibody is deeply affected by the structure and target (Nie et al., 2020). Fragment-based BsAb has intense penetration and epitope accessibility with a short plasma half-life (Fan et al., 2015). For instance, the half-life of Blinatumomab is only 1.25 ± 0.63 h (Klinger et al., 2012). By contrast, IgG-like BsAb with a natural structure could be flexible and desirable for therapeutic clinical application; however, heavy/light chain miss pairing remains an issue. Several alternative strategies were applied to generate bispecific antibodies (Han et al., 2019; Zhou et al., 2020). In our study, the “Bispecific Antibody by Protein Trans-Splicing” (BAPTS) platform was introduced to maintain the correct pairing of chains for the binding affinity of the Fab domain (Han et al., 2017). Split inteins comprise N-terminal fragment (InteinN) and C-terminal fragment (InteinC) and are inactive when separated. The trans-splicing reaction could be carried out under native conditions, including reconstitution of intein fold, excision of the intein, and ligation of exteins. In the BAPTS platform, the bispecific antibody was divided into two antibody fragments attached with InteinN and InteinC at the hinge region of the antibody. The trans-splicing reaction occurred between two antibody fragments under mild reducing conditions, resulting in a mature bispecific antibody with correct chain pairing. In addition, the bispecific antibody remains an IgG-like format without an extra linker.
In our previous work, CD3×HER2, CD3×EGFR, HER2×EGFR and CD3×PRLR BsAbs were produced based on the “BAPTS” platform (Han et al., 2017; Zhou et al., 2020). The CD3×EpCAM BsAb was generated with natural human IgG1 structure with the same technology. The CD3×EpCAM BsAb eliminated the tumor cells with high EpCAM expression level, activated T cells and induced T cells redirected to tumor cells in vitro. It could inhibit the growth of solid tumors derived from the SW480 cell line and maintain a similar half-life with EpCAM mAb in vivo.
Materials and Methods
Mice and Cell Culture
Female NOD/SCID mice and Balb/c mice were purchased from Beijing Charles River Laboratories. The experimental protocols were approved by the Institutional Animal Care and Use Committee of Shanghai Jiao Tong University (A2018041). SW480 and Jurkat cells were purchased from the Chinese Type Culture Collection, and Caco-2 and HT-29 cells were purchased from the American Type Culture Collection. All tumor cell lines were cultured according to manufactures’ instructions.
Transient Antibody Expression in HEK293E Cells
Anti-CD3 and anti-EpCAM variable heavy chain (VH) and variable light chain (VL) sequences were synthesized and extended with human IgG1 framework with mutations of L234A, L235A, and P329G (LALA-PG), and then cloned into expression vector pM09 separately via One Step Cloning Kit (Vazyme Biotech, Nanjing, China) (30). Fragment A contained anti-CD3 light chain, anti-CD3 heavy chain, and inteinC fused CH2-3 construct, and Fragment B contained anti-EpCAM light chain and anti-EpCAM VH and CH1 in tandem with inteinN construct.
Transient gene expression (TGE) was used to produce antibody fragments and monoclonal antibodies as previously described (Ding et al., 2017). In brief, two antibody fragments conjunct with split intein against CD3 and EpCAM were expressed via the transient transfection system in HEK293E cells. Plasmids were prepared through the TM Endo-free Plasmid Maxi Kit (Omega Bio Tek, Norcross, GA, United States). HEK293E cells were seeded at a density of 3×106 cells/mL on the day before transfection and were centrifuged and adjusted at 6×106 cells/mL using Freestyle 293 medium (Thermo Fisher Scientific, Shanghai, China) on the day of transfection. Fragment A and B expression vectors were diluted into 40 μg/ml using freestyle 293 medium and gently mixed with 25 kDa linear-polyethyleneimine (PEI, 1 mg/ml, 23966-1, Polysciences, Warrington, PA, United States) (DNA: PEI = 1:5, w: w). Plasmid and PEI complexes were incubated for 10–15 min and added to the cell medium. After 4 h, the transfection system was supplemented with SFM4 HEK293 (Cytiva, Logan, UT, United States) medium equal to freestyle 293 medium in volume and valproic acid (VPA, Sigma Aldrich, Shanghai, China) to 3 mM; after 24 h, 20% Tryptone N1 (Organotechnie, La Courneuve, France) was added into to the culture at 0.5% and anti-clumping agent (Thermo Fisher Scientific, Shanghai, China) to 0.1%. The supernatant was taken for purification when the cell viability was near 50%.
Bispecific Antibody Generation and Purification
The CD3 and EpCAM fragments were purified by Capto L chromatography (Cytiva, Uppsala, Sweden) through the Akta150 system (Cytiva, Uppsala, Sweden). The supernatant was centrifuged and flirted through a 0.45 μm filter membrane. The column was loaded with binding buffer (20 mM sodium phosphate, 150 mM NaCl, pH 7.4), supernatants, wash buffer (100 mM sodium citrate, pH 5.0), and eluted with elution buffer (100 mM sodium citrate, pH 2.8). The elution was neutralized to pH 7.0 with Tris-HCl buffer (1 M, pH 8.0). Fragment A and B samples were dialyzed into phosphate buffer (PBS, pH 7.4) followed by splicing reaction catalyzed mediated by split intein.
After dialyzing all of the fragments in PBS buffer and adding 2 mM dithiothreitol (DTT, Sigma Aldrich, Shanghai, China), 100 mM fragment A was combined with 375 mM fragment B and incubated at 37°C for 2 h. The mixture was dialyzed to PBS to remove DTT, sterilized using a 0.22 μm filter (Millipore, Shanghai, China), and the oxidation reaction was carried out at room temperature for 2–3 days. The CD3×EpCAM BsAb was isolated through Protein A affinity chromatography (Cytiva, Uppsala, Sweden). The eluates were adjusted to pH 7.0 using Tris-HCl buffer, dialyzed to PBS, and sterilized through a 0.22 μm filter (Millipore, Shanghai, China).
Affinity Measurement of the CD3×EpCAM BsAb
Biolayer light interferometry (BLI, OctectRED96, ForteBio Analytics, Shanghai, China) was used to evaluate CD3×EpCAM BsAb affinity to EpCAM. Biotinylated EpCAM antigen (Sino Biological, Beijing, China) immobilized to the pre-hydrated streptavidin (SA, 18–5,020, Sartorius) sensors. SA sensors were balanced in PBST +0.1%Tween 20 (assay buffer). Then 50 nM CD3×EpCAM BsAb associated with antigen for 300 s and dissociated in 0.1%PBST for 600 s. The SA sensors were regenerated by 10 mM glycine, pH 3.0 for 10 s, and neutralized in assay buffer for 10 s, repeated three times. CD3×EpCAM BsAb concentration series at 500 nM, 300 nM, 200 nM, 100 nM was measured another four times. The shaking speed was 1,000 rpm. A 1:1 curve fitting model analyzed the association and dissociation results to fit the association constant (kon), dissociation constant (kdis), and affinity constant (KD).
In Vitro Cytotoxicity Assays
Tumor cells were seeded in culture medium RPMI 1640 (Thermo Fisher Scientific, Shanghai, China) with 10% FBS (Thermo Fisher Scientific, Shanghai, China) at a density of 1×104 cells/well on a 96-well flat-bottom cell culture plate (Corning, New York, NY, United States) and cultured overnight. In RPMI 1640/2% inactivated FBS, 10-fold serial gradient dilution of CD3×EpCAM BsAb was performed starting with a 15 μg/ml concentration. Samples were added to corresponding wells at a final volume of 50 μl. Peripheral blood mononuclear cells (PBMC) were isolated from health volunteers through Ficoll plus (Cytiva, Logan, UT, United States) density centrifugation by standard procedures. In RPMI 1640 with 10% inactivated FBS medium, PBMCs were adjusted to 1×105 cells/well added into the plate at an effector cell: tumor cell (E: T) ratio of 10:1. The cytotoxicity assay was detected after plates were incubated at 37°C for 32–48 h from supernatant samples using CytoTox 96® Non-Radioactive LDH Kit (Promega, Madison, WI, United States). All tests were repeated in triplicate and nonlinear regression analysis to fit dose-response curves and were assayed with GraphPad Prism Version 8.0.
Analysis of T-Cell Redirection
CD3+ Jurkat cells were labeled with PKH26 (Sigma Aldrich, Shanghai, China) as model cells, and SW480 cells were labeled with carboxyfluorescein succinimidyl amino ester (CFSE, Invitrogen, Carlsbad, CA, United States) as target cells, according to manufactures’ instructions. The two kinds of labeled cells were incubated at equal ratios and then added with 150 ng/ml EpCAM mAb, CD3 mAb, CD3×EpCAM BsAb and control antibody for 30 min at 4°C respectively. CFSE+/PKH26+ cells were detected by flow cytometry.
Analysis of T-Cell Activation
Freshly prepared peripheral blood mononuclear cells (PBMC) were treated with CD3×EpCAM BsAb, CD3 mAb, EpCAM mAb and control antibody at 150 ng/ml and incubated with or without target cells in 96-well plates. The early activation marker CD69 was detected after 24 h and late activation marker CD25 was detected after 90 h. The PBMC were collected and stained with CD8-FITC (Sino Biological, Beijing, China), CD4-PE (Sino Biological, Beijing, China), and CD69-APC (BD biosciences, San Jose, CA, United States) or CD25-APC (Sino Biological, Beijing, China) by standard flow cytometry.
Pharmacokinetic Studies
Five male Balb/c mice (6–8 weeks) per group were intraperitoneally (i.p.) treated with CD3×EpCAM BsAb or EpCAM mAb at a dose of 10 mg/kg. Blood samples were diluted 2000-fold for the quantitative assay through enzyme-linked immunosorbent assay (ELISA) standard procedure. In brief, goat anti-human IgG kappa chain-specific antibody (Millipore, Shanghai, China) was coated on 96 well high-affinity protein-binding flat-bottom plates overnight. CD3×EpCAM BsAb or EpCAM mAb were captured by anti-human IgG kappa antibody and then were detected by goat anti-human Fc HRP antibody (Sigma Aldrich, Shanghai, China) for the measurements by TMB solution over OD450 absorption. PK parameters were assayed with a non-compartmental analysis model using the PK solver software.
Xenograft Tumor for Pharmacodynamics Studies
Human effector cells were isolated from healthy donors using Ficoll density centrifugation. In vivo experiments were performed in female NOD/SCID mice (6–8 weeks). Thirty mice were divided into five groups for double-blind and randomized treatment. For each mouse, 6×106 SW480 cells were mixed with 2×106 inactivated PBMCs in a final volume of 100 μl. Each mouse was subcutaneously implanted with PBMC and SW480 cells in an E: T ratio of 1:3 on the right flank. Six mice per group were intraperitoneally injected with CD3×EpCAM BsAb, mAb, and PBS as blank control at indicated doses on the second day. All mice were treated once a week by intraperitoneal administration. Tumor sizes were measured using a caliper every three days and calculated according to the formulation.
Statistical Analysis
The GraphPad was applied to calculate EC50 values from nonlinear regression analysis. PK solver calculated the pharmacokinetic parameters using noncompartmental analysis.
Results
Designing of Bispecific Antibody Molecule Assembly
We constructed CD3 and EpCAM fragment expression vectors based on the “BAPTS” platform (Figure 1). Two fragments were expressed in mammalian cells separately (CD3 light chain, CD3 heavy chain, and inteinC for fragment A and EpCAM light chain, EpCAM InteinN chain for fragment B. The Antibody-Dependent Cell-mediated Cytotoxicity (ADCC) activity of CD3×EpCAM BsAb was silenced, and “Knobs-into-Holes” were adopted through Fc engineering methods.
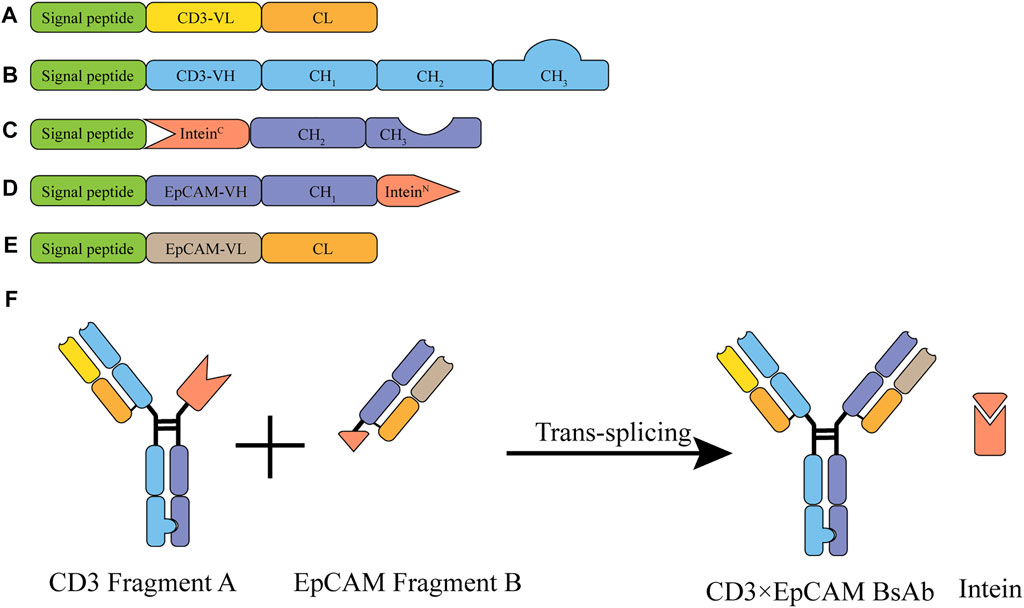
FIGURE 1. Illustration of the CD3×EpCAM BsAb bispecific antibody generated by BAPTS platform. (A) CD3 variable and constant domains for the CD3 light chain. (B) CD3 variable and constant domains for the CD3 heavy chain. (C) CH2 and CH3 fused with InteinC for IntcFc chain. (D) EpCAM variable domain fused with InteinN for HN chain. (E) Variable and constant domains for the EpCAM light chain. (F) Schematic illustration of CD3×EpCAM BsAb generated by BAPTS platform.
Generation of CD3×EpCAM BsAb
After being caught by Capto L affinity chromatography, fragment A and B molecular weight were measured by SDS-PAGE at about 170 and 55 kDa. Fragment A comprised three peptides, CD3 light chain, CD3 heavy chain, and IntCFcH chain (Figure 2A, Supplementary Figure S1A). Fragment B comprised two peptides, EpCAM light chain and HN chain (Figure 2A, Supplementary Figure S1B).
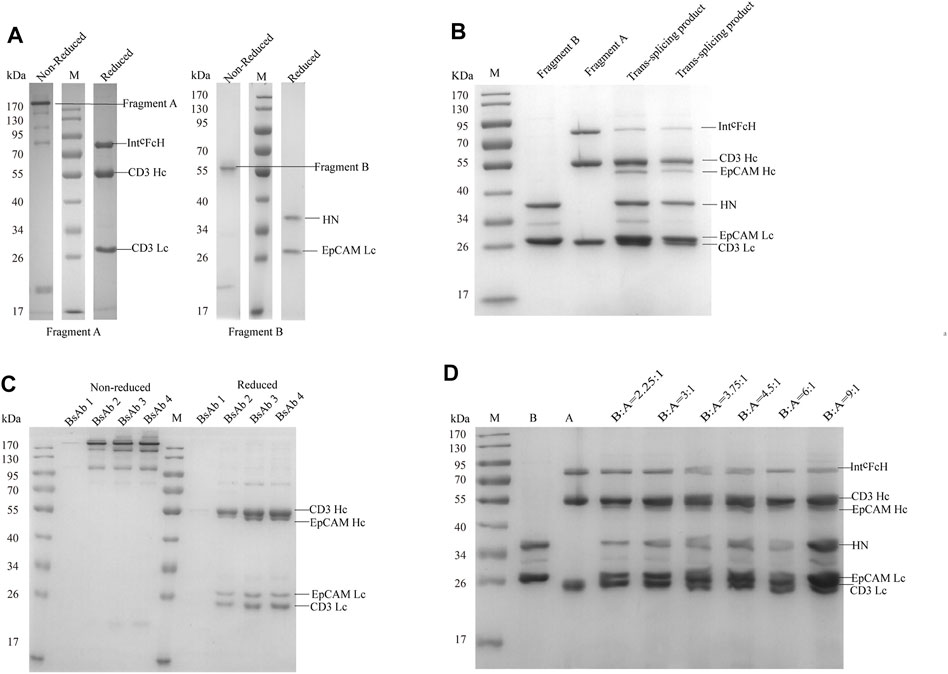
FIGURE 2. SDS-PAGE analysis of CD3×EpCAM bispecific antibody. (A) SDS-PAGE analysis of CD3×EpCAM bispecific antibody CD3 fragment A and EpCAM fragment B under non-reduced and reduced conditions. (B) Trans-splicing reaction between CD3 fragment A and EpCAM fragment B at 37°C with 1.5 mM DTT for 2 h (C) CD3×EpCAM BsAb under non-reduced and reduced conditions. (D) Trans-splicing reaction condition optimization between the different fragments A and B ratios with 1.5 mM DTT.
When two fragments were reduced with 1.5 mM DTT, the trans-splicing reaction mediated by Npu DnaE split intein occurred. A new peptide bond was formed in the flanking extein, and SDS-PAGE analysis showed the heavy chain binding to EpCAM at 55 kDa formed (Figure 2B). Then, the dimeric light chain and extra fragment B were removed through Protein A affinity chromatography (Figure 2C). Additionally, a 3.75:1 ratio of fragment B to fragment A (mol: mol) was optimized for trans-splicing reaction. (Figure 2D). At the same time, an EpCAM parental monoclonal antibody was produced as a control (Supplementary Figure S1C).
In Vitro Cytotoxicity of CD3×EpCAM BsAb
BLI was used to evaluate the affinity of CD3×EpCAM BsAb for EpCAM. The biotinylated extracellular domain of EpCAM was loaded on the streptavidin sensor and yielded a kon of 2.70 × 104 M−1s−1, a kdis of 1.05 × 10−3 s−1, fitting a KD of 3.98 × 10−8 M (Figure 3A, Supplementary Figure S2). In addition, the parental antibody was characterized at a KD of nM (Table 1).
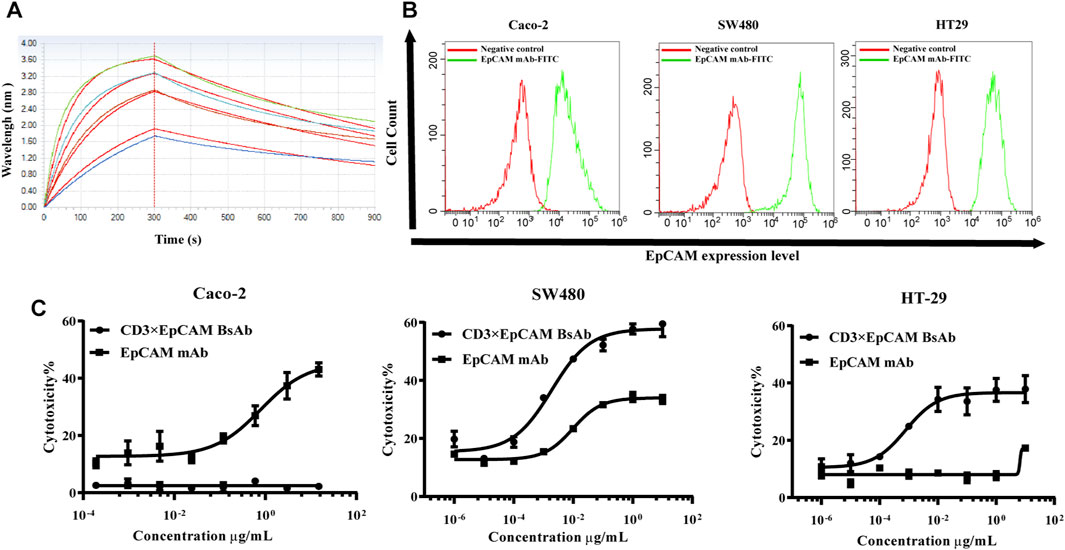
FIGURE 3. Binding activity to EpCAM and in vitro cytotoxicity analysis of CD3×EpCAM BsAb. (A) Affinity analysis of CD3×EpCAM BsAb to EpCAM detected by BLI. (B) Expression of EpCAM on different cell lines detected by flow cytometry. (C) In vitro cytotoxicity assay of CD3×EpCAM bispecific antibody using LDH release assay. The dose-response curve was fitted through Graphpad Prism version 8.0. Data points in the curve represent the mean of three samples; error bars, SD.
Cell surface expression level of EpCAM on our panel of cancer cells was tested using flow cytometry. The highest expression level was Caco-2, followed by HT-29 and SW480. The in vitro bioactivity of CD3×EpCAM BsAb was tested using an LDH-release cytotoxicity assay. As effector cells, PBMCs were obtained from healthy donors, and as target cells, a group of tumor cells with different EpCAM expression levels were chosen (Figure 3B). The cells could be killed only in the presence of PMCM and an E:T ratio of 10:1 was taken for the cytotoxicity assay (Supplementary Figure S3A,B). A dose-dependent decrease cytotoxicity effect caused by CD3×EpCAM BsAb was observed at an E:T ratio of 10:1 (Figure 3C). The results showed that tumor cells with the high expression level of EpCAM were sensitive to the BsAb but not to parental EpCAM mAb. And EpCAM negative cell line, Jurkat cell, could not be killed by both CD3×EpCAM BsAb and EpCAM mAb (Supplementary Figure S3C,D). The maximal killing activity of CD3×EpCAM BsAb to different cell lines Caco-2, SW480, and HT-29 were listed (Table 2).
In Vitro T Cell Recruitment and Activation Mediated by CD3×EpCAM BsAb
The mechanism of CD3×EpCAM BsAb mediated cytotoxicity of cancer cells was investigated (Brischwein et al., 2007; Massafra et al., 2021). The binding ability of CD3×EpCAM to objected cells was tested through flow cytometry. After incubation with CD3×EpCAM BsAb at 100 ng/ml, redirected T cells around tumor cells were observed (Figure 4A), and CFSE+/PKH26+ cells were more efficiently assembled than CD3 mAb or EpCAM mAb groups. Cell aggression was observed, indicating that compared with EpCAM mAb or CD3 mAb, T cells were significantly redirected to tumor cells by CD3×EpCAM BsAb. (Figure 4B).
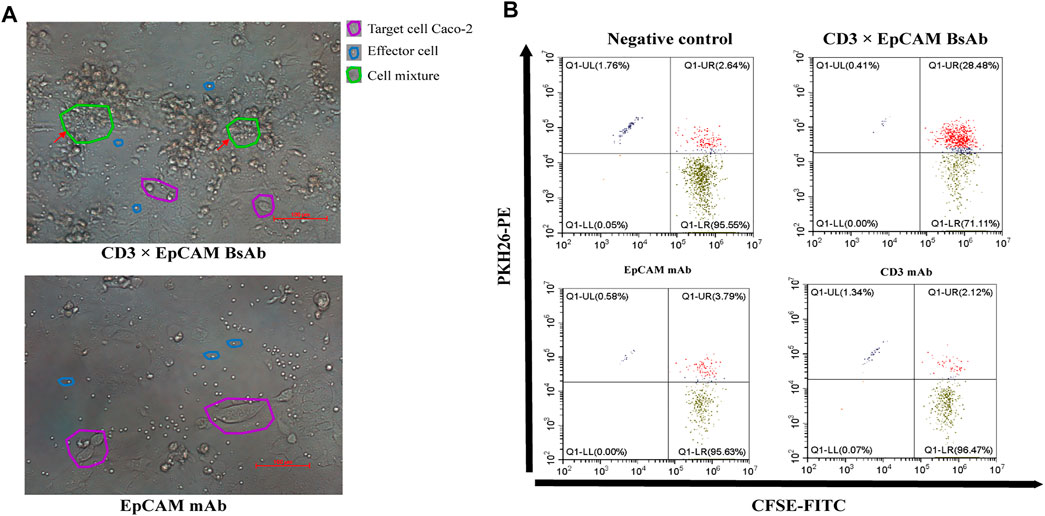
FIGURE 4. T cell recruitment mediated by CD3×EpCAM BsAb. (A) The recruitment of T cells to tumor cells mediated by 150 ng/ml CD3×EpCAM BsAb and EpCAM mAb at an E: T ratio of 10:1 after an incubation of 24 h. Images were obtained under 20 × magnification, and scale bars 100 μm. (B) The recruitment analysis of CD3+ cells to tumor cells by CD3×EpCAM BsAb by flow cytometry.
Meantime, the early activation marker CD69 and late activation marker CD25 of CD8 or CD4 positive T cells was also determined by flow cytometry. In the presence of the target cell, activation maker CD69 of T cells was upregulated obviously by CD3×EpCAM BsAb (Figure 5A). In comparison to CD3 mAb or EpCAM mAb, CD3×EpCAM BsAb efficiently activated T cells, resulting in increased CD69 and CD25 expression on CD8+ and CD4+ T cells (Figures 5B,C). The results also showed the CD107a could be upregulated by CD3×EpCAM BsAb on CD4+ and CD8+ T cells (Supplementary Figure S4).
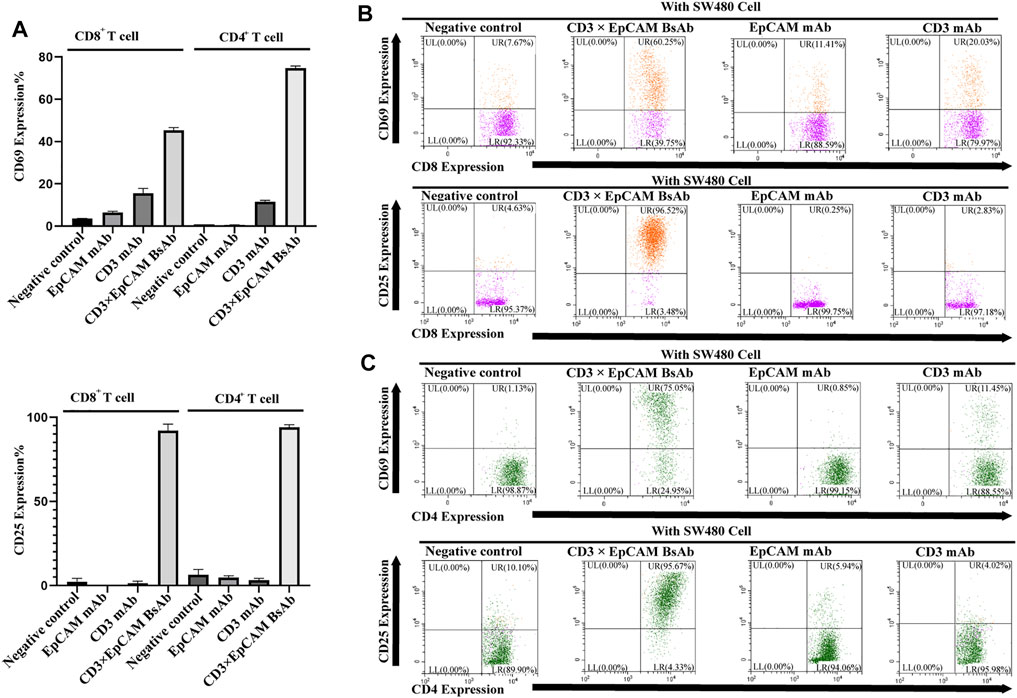
FIGURE 5. T cell activation analysis mediated by CD3×EpCAM BsAb detected by flow cytometry. (A) The activation expression level of CD69 on T cells was detected after incubation for 24 h and CD25 for 90 h (E: T = 10:1). (B) The activation analysis of CD8+ cells to tumor cells by CD3×EpCAM BsAb, EpCAM mAb, and CD3 mAb. (C) The cell activation analysis of CD4+ cells to tumor cells by CD3×EpCAM BsAb, EpCAM mAb, and CD3 mAb.
In Vivo Bioactivity Study
We performed PK and PD experiments to investigate the in vivo bioactivity of CD3×EpCAM BsAb. Balb/c mice were i. p. treated with a single dose of 10 mg/kg CD3×EpCAM bispecific antibody or parental EpCAM mAb, respectively. The blood samples were gathered at a series of time points and quantified by Elisa assay (Figure 6A). CD3×EpCAM bispecific antibody parameters were consistent with EpCAM mAb as excepted (Table 3). It is worth noting that the CD3×EpCAM bispecific antibody was stable with an in vivo half-life of about 14 days. The CD3×EpCAM BsAb is lack of cross-reactivity to muEpCAM, so the EpCAM related toxicity and elimination could not be reflected in the mouse model.
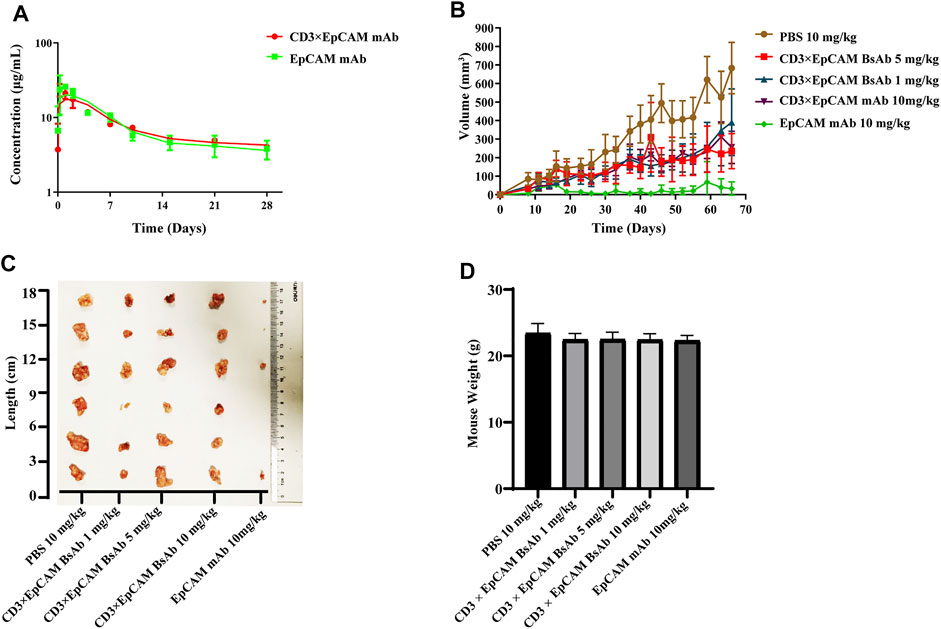
FIGURE 6. In vivo bioactivity assay of CD3×EpCAM BsAb in xenograft NOD/SCID mouse model. (A) In vivo PK analysis of CD3×EpCAM BsAb and EpCAM mAb in Balb/c mice (N = 5). (B) Tumor growth inhibition efficacy of CD3×EpCAM BsAb. Mice were implanted subcutaneously with 6×106 SW480 cells mixed with 2×106 unstimulated human PBMC (E: T = 1:3). Mice (N = 6) were treated through intraperitoneal injection with PBS vehicle control, EpCAM mAb, or CD3×EpCAM BsAb at different dosages per mouse per week. (C) Excised tumor results showed on double-blind order. (D) Weight comparison of different groups. Error bar, SD.
The anti-tumor bioactivity of CD3×EpCAM BsAb was evaluated on xenograft NOD/SCID mouse model. The NOD/SCID mice were implanted subcutaneously with a 3:1 mixture of SW480 cells and unstimulated human PBMCs and treated with CD3×EpCAM BsAb or EpCAM mAb through intraperitoneal injection every week. After 66 days, the results revealed that the experimental groups could reduce the growth of the SW480 tumor, although there was no dose-dependent inhibition in the 1 mg/kg, 5 mg/kg, and 10 mg/kg groups (Figures 6B,C). Unexpectedly, EpCAM mAb at a dosage of 10 mg/kg resulted in considerable tumor elimination. In addition, as a sign of toxicity, there was a weight loss of mice in both CD3×EpCAM BsAb and EpCAM mAb groups (Figure 6D).
Discussion
Colorectal cancer is still a commonly diagnosed cancer with high mortality (Arnold et al., 2017), and an increasing number of novel therapeutics were explored into the clinical stage. This study generated a kind of IgG format bispecific antibody targeting CD3 and EpCAM without an extra linker based on the “BAPTS” platform. Furthermore, the CD3×EpCAM BsAb characterizations in vitro and in vivo bioactivity, specificity, affinity, and stability were demonstrated.
EpCAM is an attractive target overexpressed on colorectal tumor cells and slightly expressed on normal tissues (Moldenhauer et al., 1987). In order to evaluate the safety, tolerability, pharmacokinetics and pharmacodynamics of solitomab (MT110, AMG 110), a phase I trial in patients with advanced solid tumors was investigated. Diarrhea and transient abnormal liver parameters were dose-limiting toxicities (DLT), leading to a maximum tolerated dose of 48 μg/d (Kebenko et al., 2018). It’s noticed that EpCAM-related toxicity on non-pathological epithelial tissue, especially like hepatocytes and duodenal, when design an anti-EpCAM-antibody based therapy (Schmelzer and Reid, 2008). Besides, a half-life-extended bispecific T-cell engager molecule format was developed by Amgen, which possessed of the Fc region and may change the administration method (Giffin et al., 2021). CD3×EpCAM BsAb exhibited superior cytotoxicity than EpCAM mAb on colorectal cell lines with different EpCAM expression levels in vitro (Figure 3B). For safety reasons, the affinity to EpCAM of CD3×EpCAM BsAb was the nM scale in our study (Figure 3A), which could elicit a robust and toxic immune response when binding to EpCAM positive cells (Ruf et al., 2007). The redirection and activation of T cells were confirmed. T cells were bridged with tumor cells through the BsAb rather than the parent monoclonal antibody. T cells were activated by binding of CD3ε without major histocompatibility complex (MHC) restriction, leading to the increased release of granzyme B and perforin (Offner et al., 2006; Bargou et al., 2008). More research could investigate co-simulation or co-inhibitory receptor expression after bispecific antibody treatment to better understand the T activation and cytotoxicity mechanism (Skokos et al., 2020). SW480 xenograft mouse model was established to evaluate the efficacy of CD3×EpCAM BsAb (Schlereth et al., 2005). The growth of the tumor in the NOD/SCID model was inhibited under different dosages of CD3×EpCAM BsAb (Figure 6B). The anti-tumor response among different dosages of CD×EpCAM BsAb was similar in the early stage, while 10 mg/kg CD3×EpCAM BsAb group did not show a superior in vivo bioactivity. We considered it might be because the extra CD3 fragment blocked the CD3 antigen on human PBMC in the system or T cell exhaustion on the early stage of administration (Wherry and Kurachi, 2015). The NOD/SCID mouse was widely used in the pre-clinical stage of immunotherapy (Amann et al., 2009; Chen et al., 2021; Sun et al., 2021). However, human immune cells with a limited half-life in the treatment cycle might hard to infiltrate into tumor tissues (Shultz et al., 2005). As the bispecific antibody is specific for human EpCAM and could not bind muEpCAM expressed on mouse normal tissues, the toxicity and distribution could not be reflected in the mouse model. It was worth mentioning that the 10 mg/kg EpCAM mAb group eliminated the tumor growth on the xenograft mouse model. That might be due to EpCAM mAb with low dissociation constant to EpCAM (kdis = 10−7 s−1) could specifically target tumor cells and then lead to a more prolonged and robust ADCC activity in this model. EpCAM mAb processing bivalent antigen-binding domain could bind to EpCAM more easily than CD3×EpCAM BsAb with single binding valency in this model. Thus, bispecific antibodies with bivalent binding domain to tumor antigen might be more potent to eliminate tumors (Santich et al., 2020). Immune checkpoint inhibitors, including cytotoxic T cell lymphocyte antigen-4 antibody and programmed death-1 antibody, could block the receptor to down-regulate T cells activation and were used to treat colorectal cancer (Chang et al., 2017; Pan et al., 2021). Combination CD3×EpCAM BsAb with the immune checkpoint inhibitor with CD3×EpCAM BsAb might be more helpful to improve the anti-tumor efficacy in future work.
Data Availability Statement
The original contributions presented in the study are included in the article/Supplementary Material, further inquiries can be directed to the corresponding author.
Ethics Statement
The animal study was reviewed and approved by Institutional Animal Care and Use Committee of Shanghai Jiao Tong University.
Author Contributions
LW and YQ performed the experiments and drafted the manuscript. HZ and LH designed the strategy, developed the methodology and prepared the CD3 fragment. YK, ZP, and JC provided reagents, performed some experiments and discussed data. JuL, TY, and JiL reviewed and revised the manuscript. JZ discussed strategy, reviewed data and revised manuscript. BZ designed the strategy, discussed data, revised the manuscript. All authors read and approved the final manuscript.
Funding
This project was supported by the National Natural Science Foundation of China (grant number: 81773621 and 82073751), the National Science and Technology Major Project “Key New Drug Creation and Manufacturing Program” of China (grant number: 2019ZX09732001-019) and New Zealand Ministry of Education, New Zealand-China Tripartite Partnership Fund (grant number: AUT-13772).
Conflict of Interest
LH is an employee of Jecho Biopharmaceutical Institute, Shanghai and Jecho Biopharmaceuticals Co., Ltd., Tianjin, China; JZ is an employee of Jecho Biopharmaceuticals Co., Ltd., Tianjin, China; Jecho Laboratories, Inc., Frederick, MD, United States; and Jecho Biopharmaceutical Institute, Shanghai, China.
The remaining authors declare that the research was conducted in the absence of any commercial or financial relationships that could be construed as a potential conflict of interest.
Publisher’s Note
All claims expressed in this article are solely those of the authors and do not necessarily represent those of their affiliated organizations, or those of the publisher, the editors, and the reviewers. Any product that may be evaluated in this article, or claim that may be made by its manufacturer, is not guaranteed or endorsed by the publisher.
Supplementary Material
The Supplementary Material for this article can be found online at: https://www.frontiersin.org/articles/10.3389/fphar.2022.803059/full#supplementary-material
Supplementary Figure S1 | (A) CD3 fragment A the EpCAM fragment B under non-reduced and reduced conditions. (B) EpCAM fragment B under non-reduced and reduced conditions. (C) EpCAM mAb under non-reduced and reduced conditions. Lanes: 1, cell culture supernatant; 2, protein L flow through; 3 to 6, different fractions of protein L elution. Lanes 7 to 12 are the corresponding samples of lanes 1 to 6 analyzed under reduced condition. Lanes 13 to 15, different fractions of protein A elution. Lanes 16 to 17 are the corresponding samples of lanes 13 to 15 analyzed under reduced condition.
Supplementary Figure S2 | Subtracted data process of CD3×EpCAM BsAb in the binding test to EpCAM.
Supplementary Figure S3 | In vitro cytotoxicity assay of EpCAM negative cells and optimization. (A) In vitro cytotoxicity assay in the presence of T cells and target cells. (B) In vitro cytotoxicity assay of CD3×EpCAM BsAb to SW480 cells at a concentration of 1.5 mg/ml in different E:T ratio. (C) Expression of EpCAM on Jurkat cells detected by flow cytometry. (D) In vitro cytotoxicity assay of CD3×EpCAM BsAb and EpCAM mAb to Jurkat cells detected by LDH release assay.
Supplementary Figure S4 | T cell activation maker CD107a analysis detected by flow cytometry. (A) The CD107a detection of CD8+ cells to tumor cells by CD3×EpCAM BsAb, EpCAM mAb, and CD3 mAb. (B) The CD107a detection of CD4+ cells to tumor cells by CD3×EpCAM BsAb, EpCAM mAb, and CD3 mAb.
References
Amann, M., D'Argouges, S., Lorenczewski, G., Brischwein, K., Kischel, R., Lutterbuese, R., et al. (2009). Antitumor Activity of an EpCAM/CD3-Bispecific BiTE Antibody during Long-Term Treatment of Mice in the Absence of T-Cell Anergy and Sustained Cytokine Release. J. Immunother. 32 (5), 452–464. doi:10.1097/CJI.0b013e3181a1c097
Argilés, G., Tabernero, J., Labianca, R., Hochhauser, D., Salazar, R., Iveson, T., et al. (2020). Localised colon Cancer: ESMO Clinical Practice Guidelines for Diagnosis, Treatment and Follow-Up. Ann. Oncol. 31 (10), 1291–1305. doi:10.1016/j.annonc.2020.06.022
Arnold, M., Sierra, M. S., Laversanne, M., Soerjomataram, I., Jemal, A., and Bray, F. (2017). Global Patterns and Trends in Colorectal Cancer Incidence and Mortality. Gut 66 (4), 683–691. doi:10.1136/gutjnl-2015-310912
Bargou, R., Leo, E., Zugmaier, G., Klinger, M., Goebeler, M., Knop, S., et al. (2008). Tumor Regression in Cancer Patients by Very Low Doses of a T Cell-Engaging Antibody. Science 321 (5891), 974–977. doi:10.1126/science.1158545
Blair, H. A. (2019). Emicizumab: A Review in Haemophilia A. Drugs 79 (15), 1697–1707. doi:10.1007/s40265-019-01200-2
Borlak, J., Länger, F., Spanel, R., Schöndorfer, G., and Dittrich, C. (2016). Immune-mediated Liver Injury of the Cancer Therapeutic Antibody Catumaxomab Targeting EpCAM, CD3 and Fcγ Receptors. Oncotarget 7 (19), 28059–28074. doi:10.18632/oncotarget.8574
Brinkmann, U., and Kontermann, R. E. (2021). Bispecific Antibodies. Science 372 (6545), 916–917. doi:10.1126/science.abg1209
Brischwein, K., Parr, L., Pflanz, S., Volkland, J., Lumsden, J., Klinger, M., et al. (2007). Strictly Target Cell-dependent Activation of T Cells by Bispecific Single-Chain Antibody Constructs of the BiTE Class. J. Immunother. 30 (8), 798–807. doi:10.1097/CJI.0b013e318156750c
Chang, C. H., Wang, Y., Li, R., Rossi, D. L., Liu, D., Rossi, E. A., et al. (2017). Combination Therapy with Bispecific Antibodies and PD-1 Blockade Enhances the Antitumor Potency of T Cells. Cancer Res. 77 (19), 5384–5394. doi:10.1158/0008-5472.Can-16-3431
Chaudry, M. A., Sales, K., Ruf, P., Lindhofer, H., and Winslet, M. C. (2007). EpCAM an Immunotherapeutic Target for Gastrointestinal Malignancy: Current Experience and Future Challenges. Br. J. Cancer 96 (7), 1013–1019. doi:10.1038/sj.bjc.6603505
Chen, J., Pan, Z., Han, L., Zhou, Y., Zong, H., Wang, L., et al. (2021). A Novel Bispecific Antibody Targeting CD3 and Lewis Y with Potent Therapeutic Efficacy against Gastric Cancer. Biomedicines 9 (8), 1059. doi:10.3390/biomedicines9081059
Ciardiello, D., Vitiello, P. P., Cardone, C., Martini, G., Troiani, T., Martinelli, E., et al. (2019). Immunotherapy of Colorectal Cancer: Challenges for Therapeutic Efficacy. Cancer Treat. Rev. 76, 22–32. doi:10.1016/j.ctrv.2019.04.003
de Bono, J. S., Tolcher, A. W., Forero, A., Vanhove, G. F., Takimoto, C., Bauer, R. J., et al. (2004). ING-1, a Monoclonal Antibody Targeting Ep-CAM in Patients with Advanced Adenocarcinomas. Clin. Cancer Res. 10 (22), 7555–7565. doi:10.1158/1078-0432.Ccr-04-0729
Ding, K., Han, L., Zong, H., Chen, J., Zhang, B., and Zhu, J. (2017). Production Process Reproducibility and Product Quality Consistency of Transient Gene Expression in HEK293 Cells with Anti-PD1 Antibody as the Model Protein. Appl. Microbiol. Biotechnol. 101 (5), 1889–1898. doi:10.1007/s00253-016-7973-y
Eyvazi, S., Farajnia, S., Dastmalchi, S., Kanipour, F., Zarredar, H., and Bandehpour, M. (2018). Antibody Based EpCAM Targeted Therapy of Cancer, Review and Update. Curr. Cancer Drug Targets 18 (9), 857–868. doi:10.2174/1568009618666180102102311
Fan, G., Wang, Z., Hao, M., and Li, J. (2015). Bispecific Antibodies and Their Applications. J. Hematol. Oncol. 8, 130. doi:10.1186/s13045-015-0227-0
Giffin, M. J., Cooke, K., Lobenhofer, E. K., Estrada, J., Zhan, J., Deegen, P., et al. (2021). AMG 757, a Half-Life Extended, DLL3-Targeted Bispecific T-Cell Engager, Shows High Potency and Sensitivity in Preclinical Models of Small-Cell Lung Cancer. Clin. Cancer Res. 27 (5), 1526–1537. doi:10.1158/1078-0432.Ccr-20-2845
Han, L., Chen, J., Ding, K., Zong, H., Xie, Y., Jiang, H., et al. (2017). Efficient Generation of Bispecific IgG Antibodies by Split Intein Mediated Protein Trans-splicing System. Sci. Rep. 7 (1), 8360. doi:10.1038/s41598-017-08641-3
Han, L., Zong, H., Zhou, Y., Pan, Z., Chen, J., Ding, K., et al. (2019). Naturally Split Intein Npu DnaE Mediated Rapid Generation of Bispecific IgG Antibodies. Methods 154, 32–37. doi:10.1016/j.ymeth.2018.10.001
Heiss, M. M., Murawa, P., Koralewski, P., Kutarska, E., Kolesnik, O. O., Ivanchenko, V. V., et al. (2010). The Trifunctional Antibody Catumaxomab for the Treatment of Malignant Ascites Due to Epithelial Cancer: Results of a Prospective Randomized Phase II/III Trial. Int. J. Cancer 127 (9), 2209–2221. doi:10.1002/ijc.25423
Kebenko, M., Goebeler, M. E., Wolf, M., Hasenburg, A., Seggewiss-Bernhardt, R., Ritter, B., et al. (2018). A Multicenter Phase 1 Study of Solitomab (MT110, AMG 110), a Bispecific EpCAM/CD3 T-Cell Engager (BiTE®) Antibody Construct, in Patients with Refractory Solid Tumors. Oncoimmunology 7 (8), e1450710. doi:10.1080/2162402X.2018.1450710
Klinger, M., Brandl, C., Zugmaier, G., Hijazi, Y., Bargou, R. C., Topp, M. S., et al. (2012). Immunopharmacologic Response of Patients with B-Lineage Acute Lymphoblastic Leukemia to Continuous Infusion of T Cell-Engaging CD19/CD3-Bispecific BiTE Antibody Blinatumomab. Blood 119 (26), 6226–6233. doi:10.1182/blood-2012-01-400515
Labrijn, A. F., Janmaat, M. L., Reichert, J. M., and Parren, P. W. H. I. (2019). Bispecific Antibodies: a Mechanistic Review of the Pipeline. Nat. Rev. Drug Discov. 18 (8), 585–608. doi:10.1038/s41573-019-0028-1
Massafra, V., Tundo, S., Dietzig, A., Ducret, A., Jost, C., Klein, C., et al. (2021). Proteolysis-Targeting Chimeras Enhance T Cell Bispecific Antibody-Driven T Cell Activation and Effector Function through Increased MHC Class I Antigen Presentation in Cancer Cells. J. Immunol. 207 (2), 493–504. doi:10.4049/jimmunol.2000252
Moldenhauer, G., Momburg, F., Möller, P., Schwartz, R., and Hämmerling, G. J. (1987). Epithelium-specific Surface Glycoprotein of Mr 34,000 Is a Widely Distributed Human Carcinoma Marker. Br. J. Cancer 56 (6), 714–721. doi:10.1038/bjc.1987.276
Münz, M., Kieu, C., Mack, B., Schmitt, B., Zeidler, R., and Gires, O. (2004). The Carcinoma-Associated Antigen EpCAM Upregulates C-Myc and Induces Cell Proliferation. Oncogene 23 (34), 5748–5758. doi:10.1038/sj.onc.1207610
Münz, M., Murr, A., Kvesic, M., Rau, D., Mangold, S., Pflanz, S., et al. (2010). Side-by-side Analysis of Five Clinically Tested Anti-EpCAM Monoclonal Antibodies. Cancer Cel Int 10, 44. doi:10.1186/1475-2867-10-44
Neijssen, J., Cardoso, R. M. F., Chevalier, K. M., Wiegman, L., Valerius, T., Anderson, G. M., et al. (2021). Discovery of Amivantamab (JNJ-61186372), a Bispecific Antibody Targeting EGFR and MET. J. Biol. Chem. 296, 100641. doi:10.1016/j.jbc.2021.100641
Nie, S., Wang, Z., Moscoso-Castro, M., D'Souza, P., Lei, C., Xu, J., et al. (2020). Biology Drives the Discovery of Bispecific Antibodies as Innovative Therapeutics. Antib Ther. 3 (1), 18–62. doi:10.1093/abt/tbaa003
Nordlinger, B., Van Cutsem, E., Gruenberger, T., Glimelius, B., Poston, G., Rougier, P., et al. (2009). Combination of Surgery and Chemotherapy and the Role of Targeted Agents in the Treatment of Patients with Colorectal Liver Metastases: Recommendations from an Expert Panel. Ann. Oncol. 20 (6), 985–992. doi:10.1093/annonc/mdn735
Offner, S., Hofmeister, R., Romaniuk, A., Kufer, P., and Baeuerle, P. A. (2006). Induction of Regular Cytolytic T Cell Synapses by Bispecific Single-Chain Antibody Constructs on MHC Class I-Negative Tumor Cells. Mol. Immunol. 43 (6), 763–771. doi:10.1016/j.molimm.2005.03.007
Pan, Z., Chen, J., Xiao, X., Xie, Y., Jiang, H., Zhang, B., et al. (2021). Characterization of a Novel Bispecific Antibody Targeting Tissue Factor-Positive Tumors with T Cell Engagement. Acta Pharm. Sinica B. doi:10.1016/j.apsb.2021.10.028
Ruf, P., Gires, O., Jäger, M., Fellinger, K., Atz, J., and Lindhofer, H. (2007). Characterisation of the New EpCAM-specific Antibody HO-3: Implications for Trifunctional Antibody Immunotherapy of Cancer. Br. J. Cancer 97 (3), 315–321. doi:10.1038/sj.bjc.6603881
Sampei, Z., Igawa, T., Soeda, T., Okuyama-Nishida, Y., Moriyama, C., Wakabayashi, T., et al. (2013). Identification and Multidimensional Optimization of an Asymmetric Bispecific IgG Antibody Mimicking the Function of Factor VIII Cofactor Activity. PLoS One 8 (2), e57479. doi:10.1371/journal.pone.0057479
Santich, B. H., Park, J. A., Tran, H., Guo, H. F., Huse, M., and Cheung, N. V. (2020). Interdomain Spacing and Spatial Configuration Drive the Potency of IgG-[L]-scFv T Cell Bispecific Antibodies. Sci. Transl Med. 12 (534), eaax1315. doi:10.1126/scitranslmed.aax1315
Schaefer, G., Haber, L., Crocker, L. M., Shia, S., Shao, L., Dowbenko, D., et al. (2011). A Two-In-One Antibody against HER3 and EGFR Has superior Inhibitory Activity Compared with Monospecific Antibodies. Cancer Cell 20 (4), 472–486. doi:10.1016/j.ccr.2011.09.003
Schlereth, B., Fichtner, I., Lorenczewski, G., Kleindienst, P., Brischwein, K., da Silva, A., et al. (2005). Eradication of Tumors from a Human colon Cancer Cell Line and from Ovarian Cancer Metastases in Immunodeficient Mice by a Single-Chain Ep-CAM-/cd3-Bispecific Antibody Construct. Cancer Res. 65 (7), 2882–2889. doi:10.1158/0008-5472.Can-04-2637
Schmelzer, E., and Reid, L. M. (2008). EpCAM Expression in normal, Non-pathological Tissues. Front. Biosci. 13, 3096–3100. doi:10.2741/2911
Seo, S. I., Lim, S. B., Yoon, Y. S., Kim, C. W., Yu, C. S., Kim, T. W., et al. (2013). Comparison of Recurrence Patterns between ≤5 Years and >5 Years after Curative Operations in Colorectal Cancer Patients. J. Surg. Oncol. 108 (1), 9–13. doi:10.1002/jso.23349
Shultz, L. D., Lyons, B. L., Burzenski, L. M., Gott, B., Chen, X., Chaleff, S., et al. (2005). Human Lymphoid and Myeloid Cell Development in NOD/LtSz-scid IL2R Gamma Null Mice Engrafted with Mobilized Human Hemopoietic Stem Cells. J. Immunol. 174 (10), 6477–6489. doi:10.4049/jimmunol.174.10.6477
Siegel, R. L., Miller, K. D., Fuchs, H. E., and Jemal, A. (2021). Cancer Statistics, 2021. CA A. Cancer J. Clin. 71 (1), 7–33. doi:10.3322/caac.21654
Skokos, D., Waite, J. C., Haber, L., Crawford, A., Hermann, A., Ullman, E., et al. (2020). A Class of Costimulatory CD28-Bispecific Antibodies that Enhance the Antitumor Activity of CD3-Bispecific Antibodies. Sci. Transl Med. 12 (525), eaaw7888. doi:10.1126/scitranslmed.aaw7888
Song, L., Xue, J., Zhang, J., Li, S., Liu, D., and Zhou, T. (2021). Mechanistic Prediction of First-In-Human Dose for Bispecific CD3/EpCAM T-Cell Engager Antibody M701, Using an Integrated PK/PD Modeling Method. Eur. J. Pharm. Sci. 158, 105584. doi:10.1016/j.ejps.2020.105584
Stein, A. S., Kantarjian, H., Gökbuget, N., Bargou, R., Litzow, M. R., Rambaldi, A., et al. (2019). Blinatumomab for Acute Lymphoblastic Leukemia Relapse after Allogeneic Hematopoietic Stem Cell Transplantation. Biol. Blood Marrow Transpl. 25 (8), 1498–1504. doi:10.1016/j.bbmt.2019.04.010
Sun, R., Zhou, Y., Han, L., Pan, Z., Chen, J., Zong, H., et al. (2021). A Rational Designed Novel Bispecific Antibody for the Treatment of GBM. Biomedicines 9 (6), 640. doi:10.3390/biomedicines9060640
Sung, H., Ferlay, J., Siegel, R. L., Laversanne, M., Soerjomataram, I., Jemal, A., et al. (2021). Global Cancer Statistics 2020: GLOBOCAN Estimates of Incidence and Mortality Worldwide for 36 Cancers in 185 Countries. CA Cancer J. Clin. 71 (3), 209–249. doi:10.3322/caac.21660
Van Cutsem, E., Cervantes, A., Adam, R., Sobrero, A., Van Krieken, J. H., Aderka, D., et al. (2016). ESMO Consensus Guidelines for the Management of Patients with Metastatic Colorectal Cancer. Ann. Oncol. 27 (8), 1386–1422. doi:10.1093/annonc/mdw235
Went, P., Vasei, M., Bubendorf, L., Terracciano, L., Tornillo, L., Riede, U., et al. (2006). Frequent High-Level Expression of the Immunotherapeutic Target Ep-CAM in colon, Stomach, Prostate and Lung Cancers. Br. J. Cancer 94 (1), 128–135. doi:10.1038/sj.bjc.6602924
Wherry, E. J., and Kurachi, M. (2015). Molecular and Cellular Insights into T Cell Exhaustion. Nat. Rev. Immunol. 15 (8), 486–499. doi:10.1038/nri3862
Yu, Y. J., Atwal, J. K., Zhang, Y., Tong, R. K., Wildsmith, K. R., Tan, C., et al. (2014). Therapeutic Bispecific Antibodies Cross the Blood-Brain Barrier in Nonhuman Primates. Sci. Transl Med. 6 (261), 261ra154. doi:10.1126/scitranslmed.3009835
Yu, Y. J., Zhang, Y., Kenrick, M., Hoyte, K., Luk, W., Lu, Y., et al. (2011). Boosting Brain Uptake of a Therapeutic Antibody by Reducing its Affinity for a Transcytosis Target. Sci. Transl Med. 3 (84), 84ra44. doi:10.1126/scitranslmed.3002230
Zhou, Y., Zong, H., Han, L., Xie, Y., Jiang, H., Gilly, J., et al. (2020). A Novel Bispecific Antibody Targeting CD3 and Prolactin Receptor (PRLR) against PRLR-Expression Breast Cancer. J. Exp. Clin. Cancer Res. 39 (1), 87. doi:10.1186/s13046-020-01564-4
Keywords: colorectal cancer, bispecific antibody, split intein, epcam, CD3
Citation: Wang L, Qiao Y, Zong H, Han L, Ke Y, Pan Z, Chen J, Lu J, Li J, Ying T, Zhang B and Zhu J (2022) IgG-like Bispecific Antibody CD3×EpCAM Generated by Split Intein Against Colorectal Cancer. Front. Pharmacol. 13:803059. doi: 10.3389/fphar.2022.803059
Received: 27 October 2021; Accepted: 31 January 2022;
Published: 23 February 2022.
Edited by:
Amit Kumar Nayak, Seemanta Institute of Pharmaceutical Sciences (SIPS), IndiaReviewed by:
Shahryar Khoshtinat Nikkhoi, Rutgers, The State University of New Jersey, United StatesChristian Klein, Roche Innovation Center Zurich, Switzerland
Copyright © 2022 Wang, Qiao, Zong, Han, Ke, Pan, Chen, Lu, Li, Ying, Zhang and Zhu. This is an open-access article distributed under the terms of the Creative Commons Attribution License (CC BY). The use, distribution or reproduction in other forums is permitted, provided the original author(s) and the copyright owner(s) are credited and that the original publication in this journal is cited, in accordance with accepted academic practice. No use, distribution or reproduction is permitted which does not comply with these terms.
*Correspondence: Baohong Zhang, bhzhang@sjtu.edu.cn
†These authors have contributed equally to this work