- Physiology Unit, Laboratory of Malaria and Vector Research, National Institute of Allergy and Infectious Diseases, Rockville, MD, United States
Angiotensin-converting enzyme 2 (ACE2) is the established cellular receptor for SARS-CoV-2. However, it is unclear whether ACE1 inhibitors (e.g., lisinopril) or angiotensin receptor blockers (e.g., losartan) alter tissue ACE2 expression. This study sought to determine whether lisinopril or losartan, as monotherapies or in combination, changes tissue levels of ACE2 in healthy male and female mice. Mice received lisinopril (10 mg/kg/day), losartan (10 mg/kg/day), or both for 21 days via drinking water. A control group received water without drug. The ACE2 protein index (ACE2 protein/total protein) was determined on the small intestine, lung, kidney, and brain. Oral lisinopril increased the ACE2 protein index across all tissues (p < 0.0001 vs. control). In contrast, the combination of lisinopril plus losartan did not increase ACE2 levels in any tissue (p = 0.89 vs. control) and even decreased tissue expression of the Ace2 gene (p < 0.001 vs. control). Tissue ACE2 remained elevated in the mice 21 days after cessation of lisinopril (p = 0.02). Plasma ACE2 did not correlate with the ACE2 protein index in any tissue. A sex difference was observed: kidney ACE2 levels were higher in male than in female mice (p < 0.0001). Oral lisinopril increases ACE2, the cellular receptor for SARS-CoV-2, in tissues that are relevant to the transmission and pathogenesis of COVID-19. Remarkably, the addition of losartan prevented lisinopril-induced increases in ACE2 across tissues. These results suggest that ACE inhibitors and angiotensin receptor blockers interact to determine tissue levels of ACE2.
Introduction
Angiotensin-converting enzyme 2 (ACE2) is an established receptor and entry point for both SARS-CoV-1 and the novel SARS-CoV-2 coronavirus (Li et al., 2003; Kuba et al., 2005; Hoffmann et al., 2020). The spike proteins on the viral envelope bind the ACE2 receptor, and the virus replicates efficiently in cells expressing ACE2 (Li et al., 2003). Human tissue histological profiling reveals ACE2 to be highly expressed on lung alveolar epithelial cells and on enterocytes of the small intestine, as well as on arterial and venous endothelium (Hamming et al., 2004). SARS-CoV-2 can enter vascular endothelium in engineered human blood vessel organoids and human kidney organoids via ACE2 (Monteil et al., 2020). SARS-CoV-2 is also associated with endothelial inflammation (Cao and Li, 2020; Varga et al., 2020), which may give rise to the clinical findings of thromboembolism (Oudkerk et al., 2020) and disseminated intravascular coagulation (Tang et al., 2020).
Given the widespread abundance of ACE2 in tissue epithelial and endothelial cells and the role of ACE2 as the entry site for SARS-CoV-2, there has been much speculation regarding whether ACE inhibitors and/or angiotensin receptor blockers (ARBs) may alter ACE2 tissue abundance and thereby change the risk of transmission or development of severe complications (Diaz, 2020; Fang et al., 2020; Sommerstein, 2020). Recent clinical studies of patients with COVID-19 have not identified a clear relationship between ACE inhibitor use or ARB use and disease risk or severity (Baral et al., 2021; Bavishi et al., 2021; Morales et al., 2021), and current guidelines support continuance of ACE inhibitors or ARB during infection (Cohen et al., 2021; Lopes et al., 2021). The design of human trials and the development of clinical guidelines regarding ACE inhibitor and ARB use have been limited by the lack of preclinical data on how ACE inhibitors and ARBs change tissue abundance of ACE2 (Patel and Verma, 2020; Sommerstein et al., 2020; Vaduganathan et al., 2020). Therefore, the question of how these drugs may impact tissue expression and abundance of ACE2 remains of fundamental interest.
The primary goal of this study was to determine whether lisinopril, an oral ACE inhibitor, or losartan, an oral ARB, changes the tissue abundance of ACE2, and whether these changes resolve after cessation of the drug. The tissues studied were the lung and small intestine, which have been identified as portals of entry for SARS-CoV-2 and sites of primary disease pathogenesis (Ma et al., 2020; Ni et al., 2020; Guo et al., 2021); the kidney, selected for its role in the angiotensin pathway (Lores et al., 2020) and because renal failure is a complication of severe COVID-19 (Legrand et al., 2021); and the brain, due to the neurological symptoms and sequelae identified during acute and long-haul COVID-19 (Boldrini et al., 2021). The secondary goals of this study were to determine whether tissue ACE2 levels differ between tissues, whether plasma ACE2 correlates with tissue ACE2, and whether tissue ACE2 levels differ between male and female mice. The findings we present demonstrate that lisinopril raised ACE2 levels in tissues when given alone, but not when given in combination with losartan. ACE2 levels varied substantially between tissues, and plasma ACE2 did not correlate with tissue ACE2. We found kidney ACE2 levels to be greater in males than in females. Together, these results provide controlled experimental data demonstrating the impact of ACE inhibition and angiotensin receptor blockade on tissue ACE2 expression in mice and highlight a potential benefit of ACE inhibitor/ARB combination therapy in the setting of a SARS-CoV-2 pandemic.
Methods
Use of Laboratory Mice
The protocols used in this study were performed in accordance with the National Institutes of Health guide for the care and use of Laboratory animals (NIH Publications No. 8023, revised 1978). All experiments and protocols using laboratory mice were reviewed and approved by the NIAID Division of Intramural Research Animal Care and Use Committee.
Experimental Design
The experiment utilized a factorial design: five male and five female mice comprised each drug treatment group (lisinopril, losartan, lisinopril and losartan combined, or vehicle) at each time point (day 21 or day 42). These 40 male and 40 female 8-week-old C57Bl/6J mice (Jackson Laboratory) were fed standard chow and treated for 21 days with drinking water containing lisinopril (10 mg/kg/day; Exelan Pharmaceuticals), losartan (10 mg/kg/day; Aurobindo Pharma), combination (10 mg/kg/day of each drug), or no drug (vehicle control). On day 21, 40 animals were euthanized for collection of plasma and tissues, while the others transitioned to standard drinking water for an additional 21 days to assess whether drug-induced changes in ACE2 resolve after drug cessation.
A pilot study of five male and five female eight-week-old C57Bl/6J mice was performed to measure average daily drinking water intake. This value was used to calculate the initial drug concentration needed to achieve a 10 mg/kg/day dosage. Each week of drug treatment during the main study, every mouse was weighed (Supplementary Figure S2) and water consumption was measured on a per-cage basis (Supplementary Figure S3). This information was used to adjust the drug concentration weekly to maintain consistent dosing throughout the course of the study.
Tissue Collection and Processing
Each mouse was euthanized via bilateral thoracotomy while under anesthesia with 4% isoflurane. One mL of blood was drawn into an EDTA tube via cardiac puncture of the right ventricle. Twenty-five mL of cold phosphate-buffered saline was administered via transcardial perfusion to remove blood from tissues prior to collection. The small intestine, lung, kidney, and brain were collected and flash-frozen for protein extraction, stored in 10% formalin for histological examination, or stored in RNAlater for gene expression studies. Plasma was separated from whole blood by centrifugation and frozen.
Measurement of ACE2 Protein Index
The flash-frozen lung, small intestine, kidney, and brain were homogenized at 4°C (Precellys Cryolys Evolution, Bertin Instruments) in a lysis buffer (RIPA buffer, 1X, Cell Signaling). Tissue total protein concentration was measured by BCA assay (Pierce BCA). ACE2 tissue abundance was measured by ELISA (Abcam). To minimize the effects of inter-assay variation, all biospecimens from a given experimental day (21 or 42) were analyzed together on a single ELISA plate and BCA plate. The ACE2 protein index was calculated by dividing the ACE2 concentration by the total protein concentration of each specimen. ACE2 concentration in plasma (pg/ml) was measured by ELISA (Abcam).
Measurement of Ace2 Gene Expression
mRNA was extracted (RNeasy, Qiagen) from the small intestine, lung, kidney, and brain tissue sections; stored in RNAlater; and converted to cDNA (SuperScript IV VILO, Invitrogen). The expression of Ace2 and the reference gene Gapdh were measured by Reverse Transcriptase Droplet Digital PCR (Prime ddPCR assays, QX200, Bio-Rad). Gene expression was quantified as the transcript ratio of Ace2 to Gapdh.
Immunohistochemistry of Tissue Sections
The small intestine, lung, kidney, and brain tissue sections were fixed in 10% formalin, embedded into paraffin, sliced into 6- to 8-µm sections by microtome, and stained with immunohistochemical antibodies for ACE2 (Sino Biological, 1:1,000) to determine tissue prevalence and distribution.
Measurement of Plasma Renin Activity
The plasma renin activity was measured using a Fluorometric Renin Assay Kit (Abcam). The plasma renin activity was measured via cleavage of a fluorogenic substrate over 60 min at 37°C and reported as renin concentration equivalent (ng/ml) using a reference renin standard provided by Abcam. Fluorescence measurements (excitation/emission = 540/590) were made by a microplate reader (MD Gemini XPS).
Statistical Analyses
Data were tested for normality using the Shapiro–Wilk test. Tissue ACE2 data were transformed by the Box–Cox method before analysis. A multivariable analysis of variance was used to assess the effect of treatment on the tissue ACE2 protein index or Ace2/Gapdh ratio, with tissue type and sex as covariates. Each drug treatment group was compared against the vehicle control using Tukey’s post hoc tests, and adjusted p-values were reported.
Body weights and water consumption were measured weekly. The effect of treatment and time on body weight change and water consumption was assessed separately in male and female mice by repeated measures two-way ANOVA. The effect of treatment and sex on plasma renin activity was assessed separately at day 21 and day 42 by two-way ANOVA. Linear regression was used to measure the relationship between plasma ACE2 and the ACE2 protein index in each tissue; covariates were sex and treatment.
A post hoc multivariate model, prompted by visual inspection of the data, was used to test the effect of sex on the kidney ACE2 protein index across both cohorts and all treatment groups.
Supplemental Materials and Methods:
Detailed methodology and a comprehensive description of antibodies, PCR reaction components, and laboratory equipment and consumables are available in Supplementary Appendix A: Supplemental Materials and Methods.
Results
Tissue ACE2 Protein Index and Ace2 Gene Expression Differed by Tissue Type
To assess tissue-specific ACE2 abundance, the ACE2 protein index was analyzed in the small intestine, kidney, lung, and brain of male and female vehicle-treated mice at day 21. The ACE2 protein index differed significantly by tissue (p < 0.0001, two-way ANOVA); it was highest in the small intestine, followed by the kidney, lung, and brain (Figure 1A; Supplementary Table S1). To assess tissue-specific Ace2 gene expression, the Ace2/Gapdh transcript ratio was analyzed in the small intestine, kidney, lung, and brain of male and female vehicle-treated mice at day 21. The Ace2/Gapdh transcript ratio differed significantly by tissue (p < 0.0001, two-way ANOVA); it was highest in the small intestine, similar in the kidney and lung, and lowest in the brain (Figure 1B; Supplementary Table S2).
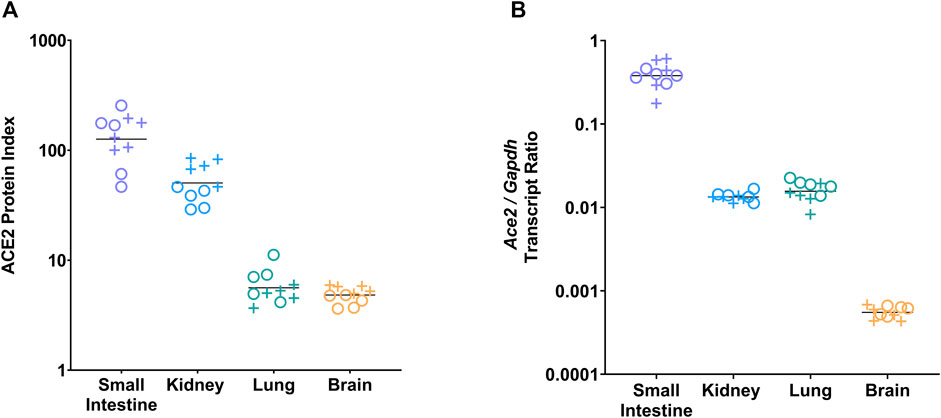
FIGURE 1. ACE2 protein index and Ace2 gene expression differed by tissue type. (A) ACE2 protein level was measured by ELISA and normalized to BCA-determined total protein concentration to generate tissue-specific ACE2 protein indices for the small intestine, kidney, lung, and brain of vehicle-treated male (+) and female (o) animals (5 males, 5 females per group). Tissue ACE2 protein index values were multiplied by 106 for display purposes. The ACE2 protein index differed by tissue (p < 0.0001 by two-way ANOVA). The ACE2 protein index was highest in the small intestine (padj < 0.0001 for the small intestine vs. kidney, lung, or brain by Tukey’s post hoc test), followed by the kidney (padj = 0.02 for kidney vs. lung, padj = 0.02 for kidney vs. brain). There was no difference between the lung and brain. (B) Ace2 gene expression was measured by ddPCR and normalized to Gapdh and presented as the Ace2/Gapdh transcript ratio for the small intestine, kidney, lung, and brain of vehicle-treated male (+) and female (o) animals. Ace2 expression differed by tissue (p < 0.0001 by two-way ANOVA). Ace2 expression was highest in the small intestine (padj < 0.0001 for small intestine vs. kidney, lung, or brain by Tukey’s post hoc test), and lowest in the brain (padj < 0.0001 for brain vs kidney or lung). There was no difference between the kidney and lung.
Given the abundance of ACE2 in tissues from vehicle-treated mice, we preserved tissue segments in 10% buffered formalin, and then sectioned and stained for ACE2 (Supplementary Figure S1). In the small intestine, ACE2 was detected along the microvilli of the small intestine in sections from vehicle-treated control and lisinopril-treated mice. In the kidney, ACE2 was detected along the lumen of renal tubules, consistent with tubular epithelium. In the lung, ACE2 was detected in the alveolar epithelium. In the brain, ACE2 localized to the vasculature.
Lisinopril Treatment Raised ACE2 Protein Index in Tissues, but the Combination of Lisinopril and Losartan Did Not
The ACE2 protein index was determined in the small intestine, kidney, lung, and brain of male and female mice after 21 days of treatment with lisinopril, losartan, combination, or vehicle (Figure 2 and Supplementary Table S1). To test the effect of treatment on tissue ACE2 protein index, analysis of variance was performed (Table 1). Treatment affected the ACE2 protein index (p < 0.0001). Lisinopril-treated mice had higher ACE2 protein indices than mice treated with vehicle (padj < 0.0001 by Tukey’s post hoc test). Losartan-treated mice had a non-significant increase in ACE2 compared to vehicle controls (padj = 0.058). In contrast, the combination of lisinopril plus losartan did not raise tissue levels of ACE2 (padj = 0.89 vs. vehicle control). Treatment had no effect on body weight (Supplementary Figure S2), water consumption (Supplementary Figure S3), or plasma renin activity (Supplementary Figure S4).
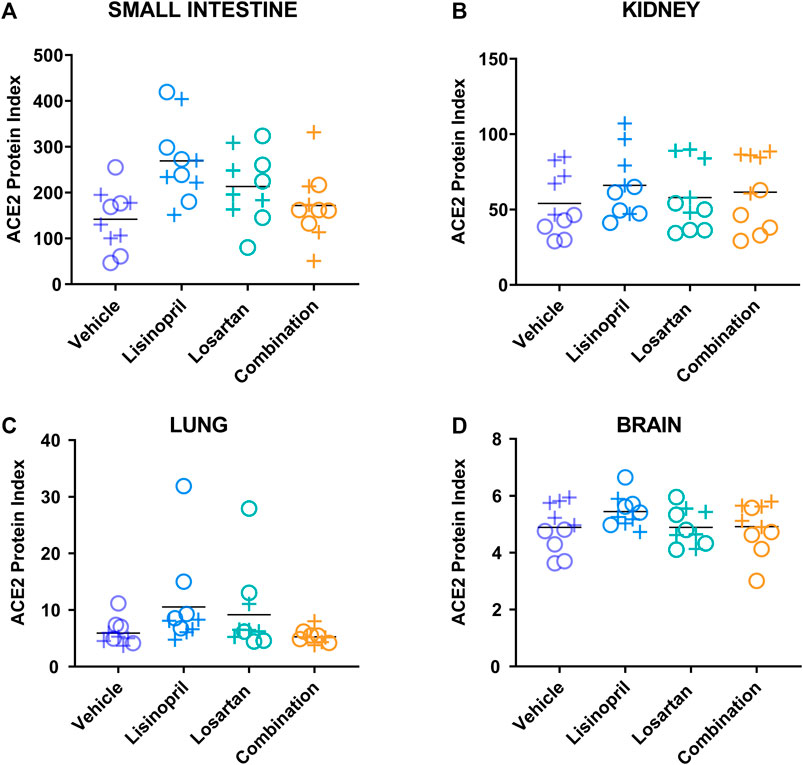
FIGURE 2. Lisinopril treatment raised the ACE2 protein index, but the combination of lisinopril and losartan did not. The ACE2 protein index was measured in the small intestine (A), kidney (B), lung (C), and brain (D) of male (+) and female (o) mice after 21 days of treatment (5 males, 5 females per group). The ACE2 protein index was different across treatment groups (p < 0.0001). Lisinopril-treated mice had higher ACE2 protein indices than in mice treated with the vehicle control (padj < 0.0001 by Tukey’s post hoc test), while mice treated with the combination of lisinopril plus losartan were not different from mice treated with the vehicle control (padj = 0.89). Tissue ACE2 protein index values were multiplied by 106 for display purposes.
Lisinopril and Losartan Combination Treatment Suppressed Ace2 Gene Expression in Tissue
To further explore the different effects of lisinopril vs. lisinopril/losartan combination on ACE2, we examined Ace2 gene expression in the small intestine, kidney, lung, and brain at day 21 (Figure 3 and Supplementary Table S2). Ace2 expression was highest in the small intestine (padj < 0.0001 against any other tissue). To assess the effect of treatment on tissue Ace2 gene expression, analysis of variance was performed that included terms for tissue type and sex (Table 2). Treatment affected Ace2 gene expression (p = 0.001); tissue type was a significant factor (p < 0.0001), but sex was not (p = 0.733). The primary treatment effect was the lowering of Ace2 expression by combination therapy compared to vehicle control (padj < 0.001) or lisinopril monotherapy (padj = 0.047). Neither lisinopril (padj = 0.60 by Tukey’s post hoc test) nor losartan (padj = 0.104) monotherapy changed Ace2 expression compared to vehicle.
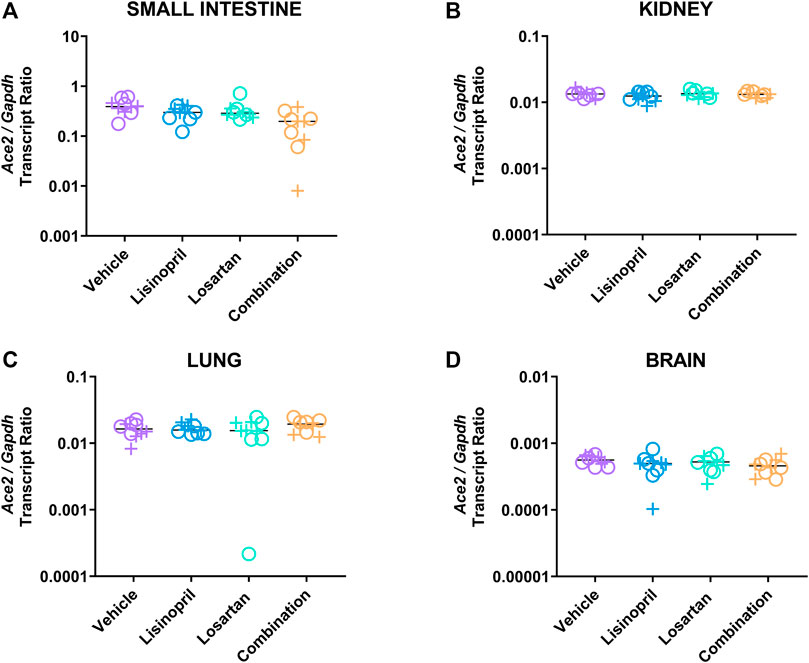
FIGURE 3. Lisinopril and losartan combination treatment suppressed Ace2 gene expression. Ace2 and Gapdh expression was measured in the small intestine of male (+) and female (o) mice by reverse transcriptase droplet digital PCR in the small intestine (A), kidney (B), lung (C), and brain (D) (5 males, 5 females per group). There was an effect of treatment (p = 0.001) and tissue (p < 0.0001), but no effect of sex (p = 0.733), on Ace2/Gapdh expression. Post hoc testing showed no effect of lisinopril or losartan on gene expression compared to vehicle. Combination-treated mice had a significantly lower Ace2/Gapdh transcript ratio than vehicle (padj < 0.001) or lisinopril monotherapy (padj = 0.047) by Tukey’s post hoc test. The effect was largest in the small intestine, which had the highest levels of expression (padj < 0.0001 vs. any tissue by Tukey’s test).
Drug-Induced Elevation of ACE2 Protein Index Persisted 21 Days After Discontinuation of Drug
To determine whether the treatment-induced changes in the ACE2 protein index were reversible, we measured tissue ACE2 protein index 21 days after cessation of drug treatment (Figure 4 and Supplementary Table S1). In the multivariate analysis that included treatment group, tissue type, and sex (Table 3), prior treatment was associated with higher ACE2 levels (p = 0.013 by ANOVA). Specifically, mice previously treated with lisinopril or losartan had higher tissue ACE2 levels than mice previously treated with the vehicle control (padj = 0.025, padj = 0.024, respectively, by Tukey’s post hoc test). ACE2 levels in mice previously treated with the combination of lisinopril and losartan were not different from mice previously treated with the vehicle control (p = 0.30).
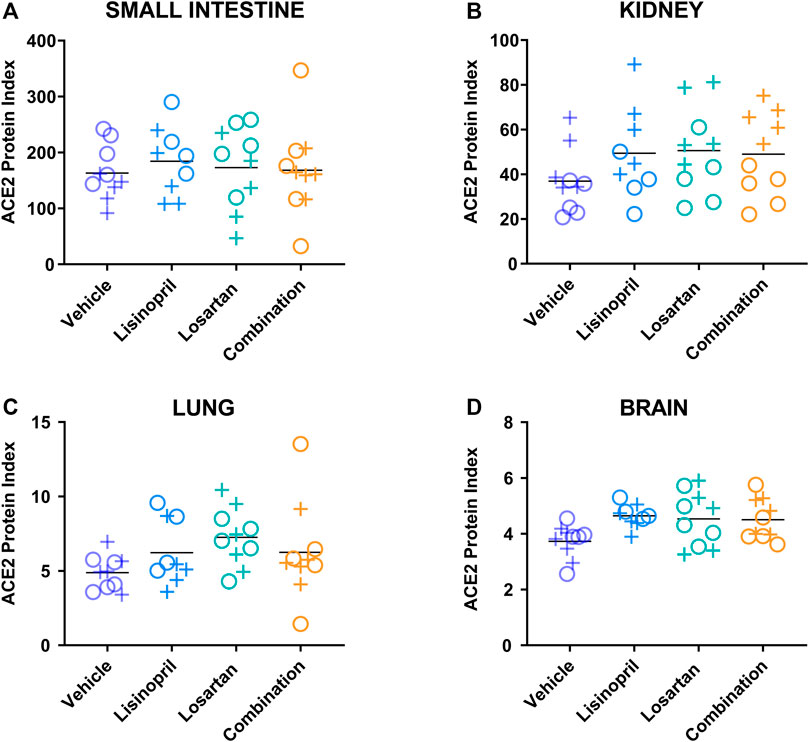
FIGURE 4. Drug-induced increases in the ACE2 protein index persisted 21 days after discontinuation of drug treatment. The ACE2 protein index was measured by ELISA in the (A) small intestine, (B) kidney, (C) lung, and (D) brain of male (+) and female (o) animals on day 42, 21 days after discontinuation of treatment with lisinopril, losartan, or lisinopril/losartan combination (n = 5 males, 5 females per group except losartan females day 42, n = 4). The ACE2 protein index values were multiplied by 106 for display purposes. The effect of treatment on the ACE2 protein index remained significant 21 days after drug cessation (p = 0.013 by ANOVA). Specifically, mice previously treated with lisinopril (padj = 0.025) or losartan (padj = 0.024) had greater ACE2 levels than mice treated with the vehicle control. Mice treated with the combination of lisinopril and losartan were not different from mice treated with vehicle control (p = 0.30).
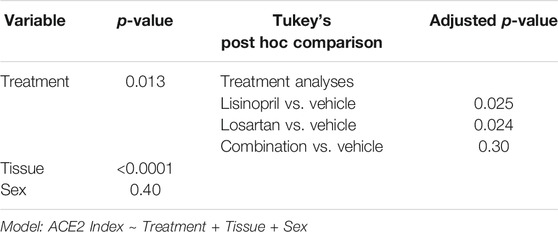
TABLE 3. Effect of drug treatment on the tissue ACE2 protein index on day 42, 21 days after drug cessation.
Plasma ACE2 Did Not Correlate With Tissue ACE2
Plasma ACE2 was measured in whole blood collected on day 21 and day 42 from male and female mice treated with lisinopril, losartan, combination, or vehicle. The relationships between plasma ACE2 and the tissue ACE2 protein index in the small intestine, lung, kidney, and brain were analyzed by linear regression to identify whether plasma ACE2 could serve as a biomarker of tissue ACE2 (Supplementary Figure S5). The linear regression analyses revealed that plasma ACE2 was not associated with tissue ACE2 in any tissue (small intestine p = 0.95; kidney p = 0.26; lung p = 0.90; brain p = 0.62).
Kidney ACE2 Levels Were Higher in Males vs. Females
The multivariate analysis of all treatment groups did not find sex to be a significant factor affecting tissue ACE2 levels at day 21 or day 42 (p = 0.17; p = 0.40, respectively). However, inspection of the data revealed a pattern of sex-based divergence in the kidney ACE2 levels in both the day 21 and day 42 cohorts (data shown in Figure 2 and Figure 4). This prompted a post hoc subgroup analysis focusing only on kidney ACE2 levels across both cohorts. The multivariate analysis of kidney ACE2 levels across both cohorts and all treatment groups revealed kidney ACE2 levels to be greater among males than females (p < 0.0001).
Discussion
ACE2 serves as the cognate receptor for the SARS-CoV-2 spike protein on the apical surface of epithelial and endothelial tissues in humans. As a key cellular entry point for the virus, ACE2 is important for both transmission of virus from person to person and tissue-specific pathology caused by local viral entry. High levels of plasma ACE2 have been associated with increased risk of severe illness from COVID-19 (Kragstrup et al., 2021). There has been much speculation about how ACE inhibitors or ARBs might alter expression of the ACE2 protein, and thereby potentially alter host susceptibility to infection with SARS-CoV-2 or the progression, severity, and tissue-specific pathology of COVID-19. However, few studies have directly measured the effect of ACE inhibition or angiotensin receptor blockade on ACE2 levels, especially outside the cardiovascular system.
To address the question of whether ACE inhibition and/or angiotensin receptor blockade alters tissue ACE2 expression, we measured tissue-specific changes in ACE2 abundance following treatment with an ACE inhibitor (lisinopril), an ARB (losartan), or the combination of both, compared to vehicle, in male and female mice. We found that 21 days of ACE inhibition with lisinopril increased tissue ACE2 expression compared to vehicle in analysis that included the small intestine, kidney, lung, and brain. However, this increase in tissue ACE2 was prevented when lisinopril was given in combination with losartan. These treatment-related increases in tissue ACE2 were still detectable 21 days after discontinuation of the drugs.
A secondary objective of this study was to assess for sex differences in tissue ACE2 abundance and in response to drug treatment. When all tissues were examined together, sex was not significantly associated with tissue ACE2 levels; however, a tissue subgroup analysis revealed kidney ACE2 levels to be significantly higher in male than in female mice among the drug-treated groups (p < 0.0001). Kidney ACE2 activity was previously reported to be greater in male vs. female mice in the absence of drug treatment (Liu et al., 2010), and a similar trend was observed in kidney tissue from human donors (Subramanian et al., 2020). Here, we described for the first time sex differences in kidney ACE2 in mice treated with ACE inhibitor and ARB.
Another secondary objective of this study was to test whether plasma ACE2 could serve as a biomarker for the tissue ACE2 protein index. We tested for association between plasma ACE2 and tissue ACE2 protein index by linear regression but did not find a significant relationship between plasma ACE2 and the ACE2 protein index in the small intestine, kidney, lung, or brain. Recently, clinical studies have used soluble ACE2 as a biomarker for activation of the renin–angiotensin aldosterone system or as a marker of tissue ACE2 (Ciaglia et al., 2020; Rieder et al., 2021); however, our results in mice suggest that plasma ACE2 is not a suitable biomarker for tissue ACE2.
We measured the renin activity in plasma from mice at day 21 and day 42 to test whether the lisinopril-induced increase in the tissue ACE2 index could be related to hemodynamic effects of drug treatment on the renin–angiotensin system. We did not observe an increase in the renin activity for any treatment group, suggesting that there were no hemodynamic changes induced by drug treatment. This observation was consistent with prior reports that ACE inhibitors and ARBs lower blood pressure in hypertensive mice but not in normotensive mice (Ferrington et al., 2011; Jackson et al., 2019; Keller et al., 2019; Liang et al., 2019).
Outside the cardiovascular system, we found that ACE2 was highly abundant around the lateral and apical margins of intestinal villi. Reports of fecal–oral transmission suggest that the intestinal tract may be a site of SARS-CoV-2 transmission (Amirian, 2020), as well as a route of viral entry into epithelial cells leading to gastrointestinal symptoms (Lehmann et al., 2021). There is increasing interest in intestinal infection as a route of viral spread (Guo et al., 2021); among people taking ACE inhibitors, associated increases in small intestine ACE2 could potentially increase the risk of SARS-CoV-2 viral infection.
This study is the first to systemically evaluate the effect of ACE inhibition and angiotensin receptor blockade on ACE2 protein abundance in these four tissues along with plasma; moreover, we assessed for sex differences and evaluated whether drug-induced changes in tissue ACE2 resolve after drug cessation. Previously, two preclinical studies examined the effect of ACE inhibitor and ARB treatment on Ace2 gene expression in cardiac and lung tissue from rats and mice. The first found that in cardiac tissue, 12 days of lisinopril or losartan monotherapy increased Ace2 gene expression, while the combination of lisinopril plus losartan did not (Ferrario et al., 2005). While we did not observe an increase in Ace2 gene expression after monotherapy with lisinopril or losartan, we did observe a decrease in Ace2 gene expression among mice treated with the combination of lisinopril and losartan compared to vehicle and lisinopril treatment, leading to similar differences in Ace2 gene expression between treatment groups. Our finding that lisinopril increased the tissue ACE2 protein index, but combination therapy prevented the increase agree with the principal finding of the previous study, and further extends this finding to the protein level and across several relevant tissues. A second study reported that 21 days of either oral captopril (an ACE inhibitor) or oral candesartan (an ARB) upregulated gene expression of Ace2 and increased ACE2 enzymatic activity in lung tissue from healthy rats. In that study, combination therapy was not evaluated. Interestingly, they reported a sharp increase in ACE2 gene expression in cultured human alveolar cells after 24 hours of exposure to captopril or candesartan that decreased to near baseline by 48 hours, despite the maintenance of elevated ACE2 protein. Another study found no changes in Ace2 expression in the lung, kidney, ileum, or heart after 14 days of treatment with an ACE inhibitor (Wu et al., 2020), while a study in diabetic mice found 8 weeks of ACE inhibitor treatment decreased Ace2 mRNA in the diabetic kidney but had no effect on Ace2 expression in the lung or heart (Vergara et al., 2021). Interestingly, that study also showed that diabetes increases ACE2 activity in kidney and lung. Our finding that elevated tissue ACE2 protein at day 21 in lisinopril-treated mice was not accompanied by a sustained increase in Ace2 gene expression is consistent with these prior observations. Furthermore, we observed elevated ACE2 protein at day 42 in the lisinopril- and losartan-treated groups compared to control even 21 days after cessation of drug. Taken together, the previous studies plus our present study indicate that ACE inhibitor or ARB monotherapy increases ACE2, as observed in rat tissue, mouse tissue, and cultured human cells, while combination therapy does not.
In contrast, another previous study in mice reported that an ACE inhibitor or ARB may actually reduce ACE2 in its membrane-bound form in the kidneys (Wysocki et al., 2020). This study examined the effects of 2 weeks of ACE inhibitor or ARB monotherapy on kidney and lung Ace2 gene expression, protein, and activity in tissue lysates from C57 mice. Similar to our results, no increase was found in Ace2 mRNA in the lung or kidney after ACE inhibitor or ARB treatment. In contrast, they found no difference in total kidney ACE2 from either drug, no change in lung ACE2 activity from either drug, but a significant decrease in membrane-bound ACE2 along with an increase in cytosolic ACE2 in the kidney after either ACE inhibitor or ARB treatment. This study used Western blot (as opposed to quantitative ELISA) to estimate ACE2 protein quantity in kidney and lung, and did not normalize to BCA-quantified total protein, making direct comparison to our study difficult. There was also no examination of drug withdrawal, and the sex of study animals was not reported, whereas we observed significant sex differences on the ACE2 protein index in the kidney.
Although neither ACE inhibitor nor ARB binds directly to ACE2, each may modulate ACE2 expression indirectly by changing the circulating levels of angiotensin-II, the major substrate for ACE2. ACE inhibitors decrease circulating levels of angiotensin-II; in contrast, ARBs increase circulating levels of angiotensin-II (Aronson and Ferner, 2020; Vaduganathan et al., 2020). However, treatment with lisinopril, losartan, or both did not change plasma renin activity in our study, suggesting that a more direct action of lisinopril on ACE2-producing cells may be responsible. The precise mechanism by which cells sense angiotensin-II and regulate ACE2 abundance and subcellular localization remains to be elucidated.
It is important to note the limitations of this study. All mice used in this study were healthy young adult mice. Hypertension and cardiovascular disease can impact tissue ACE2, and the findings could be different in the setting of cardiovascular disease. While lisinopril and losartan are representatives of their drug classes, other ACE inhibitors or ARBs may have different effects on ACE2 abundance. Lastly, while our findings in mice are consistent with the available results in rats, the effects of ACE inhibition and angiotensin receptor blockade on tissue ACE2 levels in humans may be different. A controlled study of tissue ACE2 in humans after initiating an ACE inhibitor, ARB, or combination therapy would be warranted to extend these findings into humans.
ACE2 expression is increased in the lungs of patients with COVID-19 comorbidities (Pinto et al., 2020), as well as in diabetes (Herman-Edelstein et al., 2021; Vergara et al., 2021) and heart failure (Khoury et al., 2021). It is possible that these comorbidities increase susceptibility to and severity of COVID-19 in part through increased tissue ACE2. In this context, our finding that ACE inhibitor and ARB combination therapy interact to decrease ACE2 gene expression and prevent increases in ACE2 protein levels may offer an avenue to reduce tissue ACE2 in people on ACE inhibitor or ARB monotherapy while still providing protection against cardiovascular or renal disease. While combination therapy of ACE inhibitor with ARB is not widely used, there is precedent for combination therapy for heart failure (Kuenzli et al., 2010), renal disease (Kunz et al., 2008), and among aged individuals (McAlister et al., 2011). It is important to note that human clinical studies have not identified ACE inhibitors or ARB medications to be risk factors for susceptibility to or poor outcome from COVID-19. An analysis of COVID-19 clinical outcomes among people taking ACE inhibitor or ARB monotherapy vs. combination therapy could provide valuable observational data on the potential benefits of combination therapy in reducing susceptibility or severity of COVID-19.
Conclusions
Lisinopril monotherapy increased ACE2 protein in key tissues affected by SARS-CoV-2, especially the lung and small intestine. In contrast, the combination of lisinopril with losartan prevented the lisinopril-induced increase in tissue ACE2 levels. These results demonstrate that ACE inhibition and angiotensin receptor blockade interact to determine tissue levels of ACE2, the SARS-CoV-2 receptor.
Data Availability Statement
The raw data supporting the conclusion of this article will be made available by the authors, without undue reservation.
Ethics Statement
The animal study protocol LMVR 21E was reviewed and approved by the NIAID Division of Intramural Research Animal Care and Use Committee. The study was carried out in a facility accredited by the American Association for Accreditation of Laboratory Animal Care.
Author Contributions
SB: conceptualization, methodology, validation, investigation, writing—original draft, review and editing, visualization, supervision, project administration, and funding acquisition; RS: validation, investigation, formal analysis, data curation, writing—review and editing, and visualization; ASM: investigation, data curation, writing—review and editing, and visualization; HA: conceptualization, methodology, validation, formal analysis, writing—review and editing, visualization, supervision, project administration, and funding acquisition.
Funding
This research was supported by the Intramural Research Program of the NIH, project numbers AI001195 and AI001275 (HA). The content of this publication does not necessarily reflect the views or policies of the U.S. Department of Health and Human Services, the National Institutes of Health, or the National Institute of Allergy and Infectious Diseases and nor does the mention of trade names, commercial products, or organizations imply endorsement by the U.S. government.
Conflict of Interest
The authors declare that the research was conducted in the absence of any commercial or financial relationships that could be construed as a potential conflict of interest.
Publisher’s Note
All claims expressed in this article are solely those of the authors and do not necessarily represent those of their affiliated organizations, or those of the publisher, the editors, and the reviewers. Any product that may be evaluated in this article, or claim that may be made by its manufacturer, is not guaranteed or endorsed by the publisher.
Acknowledgments
We would like to thank Dr. Ian Moore, DVM PhD, veterinary pathologist, and Kevin Bock, MS, histotechnologist, of the Infectious Disease Pathogenesis Section, Comparative Medicine Branch, National Institute of Allergy and Infectious Diseases, for their expertise in performing and interpreting the immunohistochemical staining of ACE2 protein in the small intestine, brain, lung, and kidney sections from mice in this project. The graphical abstract was created in part with BioRender (BioRender.com) with the academic publishing rights provided to the National Institute of Allergy and Infectious Diseases.
Supplementary Material
The Supplementary Material for this article can be found online at: https://www.frontiersin.org/articles/10.3389/fphar.2022.798349/full#supplementary-material
References
Amirian, E. S. (2020). Potential Fecal Transmission of SARS-CoV-2: Current Evidence and Implications for Public Health. Int. J. Infect. Dis. 95, 363–370. doi:10.1016/j.ijid.2020.04.057
Aronson, J. K., and Ferner, R. E. (2020). Drugs and the renin-angiotensin system in covid-19. BMJ: 369:m1313. doi:10.1136/bmj.m1313
Baral, R., Tsampasian, V., Debski, M., Moran, B., Garg, P., Clark, A., et al. (2021). Association between Renin-Angiotensin-Aldosterone System Inhibitors and Clinical Outcomes in Patients with COVID-19: A Systematic Review and Meta-Analysis. JAMA Netw. Open 4, e213594. doi:10.1001/jamanetworkopen.2021.3594
Bavishi, C., Whelton, P. K., Mancia, G., Corrao, G., and Messerli, F. H. (2021). Renin-angiotensin-system Inhibitors and All-Cause Mortality in Patients with COVID-19: a Systematic Review and Meta-Analysis of Observational Studies. J. Hypertens. 39, 784–794. doi:10.1097/HJH.0000000000002784
Boldrini, M., Canoll, P. D., and Klein, R. S. (2021). How COVID-19 Affects the Brain. JAMA Psychiatry 78, 682–683. doi:10.1001/jamapsychiatry.2021.0500
Cao, W., and Li, T. (2020). COVID-19: towards Understanding of Pathogenesis. Cell Res 30, 367–369. doi:10.1038/s41422-020-0327-4
Ciaglia, E., Vecchione, C., and Puca, A. A. (2020). COVID-19 Infection and Circulating ACE2 Levels: Protective Role in Women and Children. Front. Pediatr. 8, 206. doi:10.3389/fped.2020.00206
Cohen, J. B., Hanff, T. C., William, P., Sweitzer, N., Rosado-Santander, N. R., Medina, C., et al. (2021). Continuation versus Discontinuation of Renin-Angiotensin System Inhibitors in Patients Admitted to Hospital with COVID-19: a Prospective, Randomised, Open-Label Trial. Lancet Respir. Med. 9, 275–284. doi:10.1016/S2213-2600(20)30558-0
Diaz, J. H. (2020). Hypothesis: Angiotensin-Converting Enzyme Inhibitors and Angiotensin Receptor Blockers May Increase the Risk of Severe COVID-19. J. Trav. Med 27, taaa041. doi:10.1093/jtm/taaa041
Fang, L., Karakiulakis, G., and Roth, M. (2020). Antihypertensive Drugs and Risk of COVID-19? - Authors' Reply. Lancet Respir. Med. 8, e32–4. doi:10.1016/S2213-2600(20)30159-4
Ferrario, C. M., Jessup, J., Chappell, M. C., Averill, D. B., Brosnihan, K. B., Tallant, E. A., et al. (2005). Effect of Angiotensin-Converting Enzyme Inhibition and Angiotensin II Receptor Blockers on Cardiac Angiotensin-Converting Enzyme 2. Circulation 111, 2605–2610. doi:10.1161/CIRCULATIONAHA.104.510461
Ferrington, L., Miners, J. S., Palmer, L. E., Bond, S. M., Povey, J. E., Kelly, P. A., et al. (2011). Angiotensin II-Inhibiting Drugs Have No Effect on Intraneuronal Aβ or Oligomeric Aβ Levels in a Triple Transgenic Mouse Model of Alzheimer's Disease. Am. J. Transl. Res. 3, 197–208.
Guo, M., Tao, W., Flavell, R. A., and Zhu, S. (2021). Potential Intestinal Infection and Faecal-Oral Transmission of SARS-CoV-2. Nat. Rev. Gastroenterol. Hepatol. 18, 269–283. doi:10.1038/s41575-021-00416-6
Hamming, I., Timens, W., Bulthuis, M. L., Lely, A. T., Navis, G., and van Goor, H. (2004). Tissue Distribution of ACE2 Protein, the Functional Receptor for SARS Coronavirus. A First Step in Understanding SARS Pathogenesis. J. Pathol. 203, 631–637. doi:10.1002/path.1570
Herman-Edelstein, M., Guetta, T., Barnea, A., Waldman, M., Ben-Dor, N., Barac, Y. D., et al. (2021). Expression of the SARS-CoV-2 receptorACE2 in Human Heart Is Associated with Uncontrolled Diabetes, Obesity, and Activation of the Renin Angiotensin System. Cardiovasc. Diabetol. 20, 90. doi:10.1186/s12933-021-01275-w
Hoffmann, M., Kleine-Weber, H., Schroeder, S., Krüger, N., Herrler, T., Erichsen, S., et al. (2020). SARS-CoV-2 Cell Entry Depends on ACE2 and TMPRSS2 and Is Blocked by a Clinically Proven Protease Inhibitor. Cell 181, 271. doi:10.1016/j.cell.2020.02.052
Jackson, K. L., Head, G. A., Gueguen, C., Stevenson, E. R., Lim, K., and Marques, F. Z. (2019). Mechanisms Responsible for Genetic Hypertension in Schlager BPH/2 Mice. Front. Physiol. 10, 1311. doi:10.3389/fphys.2019.01311
Keller, K., Kane, A., Heinze-Milne, S., Grandy, S. A., and Howlett, S. E. (2019). Chronic Treatment with the ACE Inhibitor Enalapril Attenuates the Development of Frailty and Differentially Modifies Pro- and Anti-inflammatory Cytokines in Aging Male and Female C57BL/6 Mice. J. Gerontol. A. Biol. Sci. Med. Sci. 74, 1149–1157. doi:10.1093/gerona/gly219
Khoury, E. E., Knaney, Y., Fokra, A., Kinaneh, S., Azzam, Z., Heyman, S. N., et al. (2021). Pulmonary, Cardiac and Renal Distribution of ACE2, Furin, TMPRSS2 and ADAM17 in Rats with Heart Failure: Potential Implication for COVID-19 Disease. J. Cel. Mol. Med. 25, 3840–3855. doi:10.1111/jcmm.16310
Kragstrup, T. W., Singh, H. S., Grundberg, I., Nielsen, A. L., Rivellese, F., Mehta, A., et al. (2021). Plasma ACE2 Predicts Outcome of COVID-19 in Hospitalized Patients. PLOS ONE 16, e0252799. doi:10.1371/journal.pone.0252799
Kuba, K., Imai, Y., Rao, S., Gao, H., Guo, F., Guan, B., et al. (2005). A Crucial Role of Angiotensin Converting Enzyme 2 (ACE2) in SARS Coronavirus-Induced Lung Injury. Nat. Med. 11, 875–879. doi:10.1038/nm1267
Kuenzli, A., Bucher, H. C., Anand, I., Arutiunov, G., Kum, L. C., McKelvie, R., et al. (2010). Meta-Analysis of Combined Therapy with Angiotensin Receptor Antagonists versus ACE Inhibitors Alone in Patients with Heart Failure. PLOS ONE 5, e9946. doi:10.1371/journal.pone.0009946
Kunz, R., Friedrich, C., Wolbers, M., and Mann, J. F. (2008). Meta-analysis: Effect of Monotherapy and Combination Therapy with Inhibitors of the Renin Angiotensin System on Proteinuria in Renal Disease. Ann. Intern. Med. 148, 30–48. doi:10.7326/0003-4819-148-1-200801010-00190
Legrand, M., Bell, S., Forni, L., Joannidis, M., Koyner, J. L., Liu, K., et al. (2021). Pathophysiology of COVID-19-Associated Acute Kidney Injury. Nat. Rev. Nephrol. 17, 751–764. doi:10.1038/s41581-021-00452-0
Lehmann, M., Allers, K., Heldt, C., Meinhardt, J., Schmidt, F., Rodriguez-Sillke, Y., et al. (2021). Human Small Intestinal Infection by SARS-CoV-2 Is Characterized by a Mucosal Infiltration with Activated CD8+ T Cells. Mucosal Immunol. 14, 1381–1392. doi:10.1038/s41385-021-00437-z
Li, W., Moore, M. J., Vasilieva, N., Sui, J., Wong, S. K., Berne, M. A., et al. (2003). Angiotensin-converting Enzyme 2 Is a Functional Receptor for the SARS Coronavirus. Nature 426, 450–454. doi:10.1038/nature02145
Liang, L., Yuan, W., Qu, L., Li, H., Zhang, L., Fan, G. C., et al. (2019). Administration of Losartan Preserves Cardiomyocyte Size and Prevents Myocardial Dysfunction in Tail-Suspended Mice by Inhibiting P47phox Phosphorylation, NADPH Oxidase Activation and MuRF1 Expression. J. Transl. Med. 17, 279. doi:10.1186/s12967-019-2021-1
Liu, J., Ji, H., Zheng, W., Wu, X., Zhu, J. J., Arnold, A. P., et al. (2010). Sex Differences in Renal Angiotensin Converting Enzyme 2 (ACE2) Activity Are 17β-oestradiol-dependent and Sex Chromosome-independent. Biol. Sex. Differ. 1, 6. doi:10.1186/2042-6410-1-6
Lopes, R. D., Macedo, A. V. S., de Barros E Silva, P. G. M., Moll-Bernardes, R. J., Dos Santos, T. M., Mazza, L., et al. (2021). Effect of Discontinuing vs Continuing Angiotensin-Converting Enzyme Inhibitors and Angiotensin II Receptor Blockers on Days Alive and Out of the Hospital in Patients Admitted with COVID-19: A Randomized Clinical Trial. JAMA 325, 254–264. doi:10.1001/jama.2020.25864
Lores, E., Wysocki, J., and Batlle, D. (2020). ACE2, the Kidney and the Emergence of COVID-19 Two Decades after ACE2 Discovery. Clin. Sci. (Lond) 134, 2791–2805. doi:10.1042/CS20200484
Ma, C., Cong, Y., and Zhang, H. (2020). COVID-19 and the Digestive System. Am. J. Gastroenterol. 115, 1003–1006. doi:10.14309/ajg.0000000000000691
McAlister, F. A., Zhang, J., Tonelli, M., Klarenbach, S., Manns, B. J., and Hemmelgarn, B. R. (2011). The Safety of Combining Angiotensin-Converting-Enzyme Inhibitors with Angiotensin-Receptor Blockers in Elderly Patients: a Population-Based Longitudinal Analysis. CMAJ 183, 655–662. doi:10.1503/cmaj.101333
Monteil, V., Kwon, H., Prado, P., Hagelkrüys, A., Wimmer, R. A., Stahl, M., et al. (2020). Inhibition of SARS-CoV-2 Infections in Engineered Human Tissues Using Clinical-Grade Soluble Human ACE2. Cell 181, 905–913. doi:10.1016/j.cell.2020.04.004
Morales, D. R., Conover, M. M., You, S. C., Pratt, N., Kostka, K., Duarte-Salles, T., et al. (2021). Renin-angiotensin System Blockers and Susceptibility to COVID-19: an International, Open Science, Cohort Analysis. Lancet Digit. Health 3, e98–e114. doi:10.1016/S2589-7500(20)30289-2
Ni, W., Yang, X., Yang, D., Bao, J., Li, R., Xiao, Y., et al. (2020). Role of Angiotensin-Converting Enzyme 2 (ACE2) in COVID-19. Crit. Care 24, 422. doi:10.1186/s13054-020-03120-0
Oudkerk, M., Büller, H. R., Kuijpers, D., van Es, N., Oudkerk, S. F., McLoud, T., et al. (2020). Diagnosis, Prevention, and Treatment of Thromboembolic Complications in COVID-19: Report of the National Institute for Public Health of the Netherlands. Radiology 297, E216–E222. doi:10.1148/radiol.2020201629
Patel, A. B., and Verma, A. (2020). COVID-19 and Angiotensin-Converting Enzyme Inhibitors and Angiotensin Receptor Blockers. JAMA 323, 1769–1770. doi:10.1001/jama.2020.4812
Pedrosa, M. A., Valenzuela, R., Garrido-Gil, P., Labandeira, C. M., Navarro, G., Franco, R., et al. (2021). Experimental Data Using Candesartan and Captopril Indicate No Double-Edged Sword Effect in COVID-19. Clin. Sci. 135, 465–481. doi:10.1042/cs20201511
Pinto, B. G. G., Oliveira, A. E. R., Singh, Y., Jimenez, L., Gonçalves, A. N. A., Ogava, R. L. T., et al. (2020). ACE2 Expression Is Increased in the Lungs of Patients with Comorbidities Associated with Severe COVID-19. J. Infect. Dis. 222, 556–563. doi:10.1093/infdis/jiaa332
Rieder, M., Wirth, L., Pollmeier, L., Jeserich, M., Goller, I., Baldus, N., et al. (2021). Serum ACE2, Angiotensin II, and Aldosterone Levels Are Unchanged in Patients with COVID-19. Am. J. Hypertens. 34, 278–281. doi:10.1093/ajh/hpaa169
Sommerstein, R., Kochen, M. M., Messerli, F. H., and Gräni, C. (2020). Coronavirus Disease 2019 (COVID-19): Do Angiotensin-Converting Enzyme Inhibitors/Angiotensin Receptor Blockers Have a Biphasic Effect? J. Am. Heart Assoc. 9, e016509. doi:10.1161/JAHA.120.016509
Sommerstein, R. (2020). Rapid Reponse Re: Preventing a Covid-19 Pandemic: ACE Inhibitors as a Potential Risk Factor for Fatal Covid-19. 368:m810.
Subramanian, A., Vernon, K. A., Slyper, M., Waldman, J., Luecken, M. D., Gosik, K., et al. (2020). RAAS Blockade, Kidney Disease, and Expression of ACE2, the Entry Receptor for SARS-CoV-2, in Kidney Epithelial and Endothelial Cells, 167098. Available at: https://www.biorxiv.org/content/10.1101/2020.06.23.167098v2.doi:10.1101/2020.06.23.167098
Tang, N., Li, D., Wang, X., and Sun, Z. (2020). Abnormal Coagulation Parameters Are Associated with Poor Prognosis in Patients with Novel Coronavirus Pneumonia. J. Thromb. Haemost. 18, 844–847. doi:10.1111/jth.14768
Vaduganathan, M., Vardeny, O., Michel, T., McMurray, J. J. V., Pfeffer, M. A., and Solomon, S. D. (2020). Renin-Angiotensin-Aldosterone System Inhibitors in Patients with Covid-19. N. Engl. J. Med. 382, 1653–1659. doi:10.1056/NEJMsr2005760
Varga, Z., Flammer, A. J., Steiger, P., Haberecker, M., Andermatt, R., Zinkernagel, A. S., et al. (2020). Endothelial Cell Infection and Endotheliitis in COVID-19. The Lancet 395, 1417. doi:10.1016/s0140-6736(20)30937-5
Vergara, A., Jacobs-Cachá, C., Molina-Van den Bosch, M., Domínguez-Báez, P., Benito, B., García-Carro, C., et al. (2021). Effect of Ramipril on Kidney, Lung and Heart ACE2 in a Diabetic Mice Model. Mol. Cel. Endocrinol. 529, 111263. doi:10.1016/j.mce.2021.111263
Wu, C., Ye, D., Mullick, A. E., Li, Z., Danser, A. H. J., Daugherty, A., et al. (2020). Effects of Renin-Angiotensin Inhibition on ACE2 (Angiotensin-Converting Enzyme 2) and TMPRSS2 (Transmembrane Protease Serine 2) Expression: Insights into COVID-19. Hypertension 76, e29–e30. doi:10.1161/HYPERTENSIONAHA.120.15782
Keywords: angiotensin-converting enzyme 2, angiotensin-converting enzyme inhibitor, angiotensin receptor blocker, COVID-19, SARS-CoV-2
Citation: Brooks SD, Smith RL, Moreira AS and Ackerman HC (2022) Oral Lisinopril Raises Tissue Levels of ACE2, the SARS-CoV-2 Receptor, in Healthy Male and Female Mice. Front. Pharmacol. 13:798349. doi: 10.3389/fphar.2022.798349
Received: 21 October 2021; Accepted: 03 February 2022;
Published: 10 March 2022.
Edited by:
Maria Grazia Morgese, University of Foggia, ItalyReviewed by:
Asif Shajahan, University of Georgia, United StatesSilvia De Francia, University of Turin, Italy
Copyright © 2022 Brooks, Smith, Moreira and Ackerman. This is an open-access article distributed under the terms of the Creative Commons Attribution License (CC BY). The use, distribution or reproduction in other forums is permitted, provided the original author(s) and the copyright owner(s) are credited and that the original publication in this journal is cited, in accordance with accepted academic practice. No use, distribution or reproduction is permitted which does not comply with these terms.
*Correspondence: Hans C. Ackerman, hans.ackerman@nih.gov