- 1Faculty of Life Science and Technology, Kunming University of Science and Technology, Kunming, China
- 2Yunnan Key Laboratory of Panax notoginseng, Kunming University of Science and Technology, Kunming, China
- 3Leiden University–European Center for Chinese Medicine and Natural Compounds, Institute of Biology Leiden, Leiden University, Leiden, Netherlands
- 4SU Biomedicine BV, Leiden, Netherlands
- 5Center for Drug Discovery & Technology Development of Yunnan Traditional Medicine, Kunming, China
Chinese herbal medicines (CHMs), with a wide range of bioactive components, are considered to be an important source for new drug discovery. However, the process to isolate and obtain those bioactive components to develop new drugs always consumes a large amount of organic solvents with high toxicity and non-biodegradability. Natural deep eutectic solvents (NADES), a new type of green and designable solvents composed of primary plant-based metabolites, have been used as eco-friendly substitutes for traditional organic solvents in various fields. Due to the advantages of easy preparation, low production cost, low toxicity, and eco-friendliness, NADES have been also applied as extraction solvents, media, and drug delivery agents in CHMs in recent years. Besides, the special properties of NADES have been contributed to elucidating the traditional processing (also named Paozhi in Chinese) theory of CHMs, especially processing with honey. In this paper, the development process, preparation, classification, and applications for NADES in CHMs have been reviewed. Prospects in the future applications and challenges have been discussed to better understand the possibilities of the new solvents in the drug development and other uses of CHMs.
1 Introduction
Chinese herbal medicines (CHMs) have been used to prevent and treat diseases based on the theory of traditional Chinese medicine (TCM) since ancient times. They are mainly derived from different parts of medicinal plants, including roots, stems, leaves, fruits, etc. On the one hand, CHMs are an important source of various bioactive components (Rácz et al., 2012). Youyou Tu, a Chinese scientist who discovered artemisinin used to treat malaria, won the Nobel Prize in Physiology or Medicine in 2015. Her discovery was based on TCM knowledge and considered to be a breakthrough in 20th tropical medicine, saving millions of lives in South China, Southeast Asia, Africa, and South America. Therefore, she stated that the discovery of artemisinin against malaria, is a gift from TCM. On the other hand, to isolate and obtain those bioactive components, or to develop new drugs from CHMs, often consumes large amount of organic solvents, most of which are volatile, flammable, and corrosive. Some of them are even highly toxic, carcinogenic, and non-biodegradable, which would be harmful for both the environment and ecosystem (Bushnell et al., 2007).
In the 21st century, a new type of green solvents has been given increased attention. They are different from conventional organic solvents with toxicity, flammability, or pungent odor. Instead, they are composed of components from natural products, which are easy to access, low-cost, non-toxic, and eco-friendly with good biocompatibility and biodegradability. These green solvents were firstly named as “natural deep eutectic solvents (NADES)” in 2011 and considered to be new substitutes for traditional organic solvents and hazardous solvents (Choi et al., 2011). NADES are generated by mixing the hydrogen bond acceptor (HBA) and hydrogen bond donor (HBD) together in a certain proportion. Both HBA and HBD in the binary system of NADES originate from biosynthetically primordial metabolites, including sugars, amino acids, choline, and some organic acids (Liu et al., 2018). The melting point of the mixture is lower than those of the individual components, which is the most typical feature of NADES. Hydrogen bondings and van der walls interactions are the main driving forces of this phenomenon (Espino et al., 2016).
Although the research of NADES is comparatively in its infancy, they have gradually become more popular and been used in various fields, such as electrochemistry, nanotechnology, catalysis, and biomedical research (He et al., 2019). Since the properties of NADES depend largely on the components and could be adjustable, they have been recently applied to extract and isolate various natural bioactive compounds from CHMs, including phenols, flavonoids, terpenoids, and alkaloids (Duan et al., 2016; Zeng et al., 2018). Also, they have been used as adjuncts to improve the stability, oral bioavailability, and skin permeability of some CHMs, thereby enhancing the therapeutic effect of these drugs (Xuan et al., 2021). Interestingly, the properties of NADES have even provided evidences of molecular interactions for elucidating traditional Paozhi theory of processing CHMs with honey. To understand the current status and foresee new possibilities of combing the novel solvents and traditional medicines, this study provides an overview of applications for NADES in CHMs. We hope to provide a clue for developing and utilizing CHMs from a new direction, and developing new drugs from natural resources in a more efficient, eco-friendly, and sustainable way.
2 “Green” solvents and development of NADES
The idea of “green” solvents expresses the goal to minimize the environmental impact resulting from the use of solvents in chemical production (Capello et al., 2007). Ionic liquids (ILs), known as the initial green solvents, were reported by Walden in 1914 for the first time (Walden, 1914) (Figure 1). ILs are liquid molten salts at temperatures below 100°C or even at room temperature, which are formed from systems composed generally of organic cations and organic or inorganic anions. It is well known that ILs are non-flammable, non-volatile, and stable in air and water (Brennecke and Maginn, 2001). The preparation of ILs mainly consists of two steps: i) protonation of amines to cations, and ii) using Lewis acids to treat halide salts or by anion decomposition reactions. However, the byproducts and wastes generated from ILs make it less “green”. They often cause the persistent pollutants in the wastewater which are difficult to be removed because of the high stability of ILs in water. Meanwhile, some studies have shown that the compounds of imidazole structure as constituents of some ILs showed significant toxicity (Romero et al., 2008).
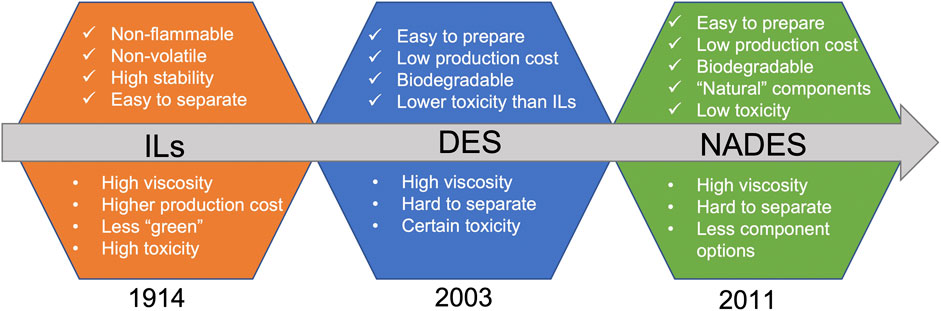
FIGURE 1. Timeline of reported developments and properties of ILs, DES, and NADES. DES, deep eutectic solvents; ILs, ironic liquids; NADES, natural deep eutectic solvents.
In order to overcome the drawbacks of ILs, deep eutectic solvents (DES) were introduced as the alternatives and were first proposed in 2003 (Abbott et al., 2003). DES are generally synthesized by gently heating the mixture while simply mixing the ingredients that can form clear and stable liquid solutions at room temperature, in a shorter amount of time (Florindo, et al., 2014). Carboxy, hydroxyl, and carbonyl are the most abundant functional groups of DES components. For example, choline chloride (ChCl), acetylcholine chloride, and various amino acids can be used as HBAs (Smith et al., 2014) and other compounds such as urea, lactic acid (LA), and citric acid (CA) (Abbott et al., 2004) are often used as the HBDs. Different from ILs that the ionic interactions are the main interaction forces, the components of DES interact with each other through the hydrogen bondings (Kelley et al., 2013). The reasons that ILs can be replaced by DES mainly include the easily available and cheap ingredients, and the easy and time-saving preparation process. However, subsequent studies have shown that the potential toxicity and cytotoxicity may be imparted by various DES. For instance, Hayyan et al. (2013) found that the cytotoxicity of some phosphonium-based DES was higher than their individual components and that their overall toxicity was varied depending on the structures of the latter. Therefore, the physicochemical properties, biological effects, and toxicological profiles of DES could be potentially adjusted by using different types of components (Juneidi et al., 2016).
In 2011, Choi et al. proposed the concept of NADES, which solely consist of natural components, i.e., primary metabolites (e.g., sugars, amino acids, organic acids, polyols, and tertiary amines) (Choi et al., 2011). The nuclear magnetic resonance metabolomics showed that in all organisms certain ingredients for NADES are present as major compounds, often keeping a constant molar ratio to some others. This brought the hypothesis that “Everywhere in living systems NADES occur and form a third liquid phase of intermediate polarity”. Apart from water and lipids, NADES aid certain cells in biosynthesis and storage of bioproducts, cryoprotection, and drought resistance (Choi et al., 2011). Compared to conventional organic solvents, NADES are considered as “green” solvents in terms of the biodegradability and sustainability, and the toxicity is generally lower than that of traditional DES (Fuad et al., 2021). Currently, NADES have been proposed as potential excipients in pharmaceutical preparations and drug delivery systems, particularly because of their solubilizing properties, varying viscosities, and built-in bioactivities (Grønlien et al., 2020).
3 Preparation of NADES
NADES are prepared by mixing two or more common natural products in a specific molar ratio that form a liquid (Figure 2). In general, the components of NADES are commercially available. For components with higher melting points, a small amount of water is usually added into the NADES mixture by a certain molar ratio. Then the mixture is heated while stirred at a certain temperature until a clear and homogeneous liquid is obtained. In the last stage, the residual water can be removed through evaporation in vacuum or the combined use of other techniques such as freeze drying (Oomen et al., 2020).
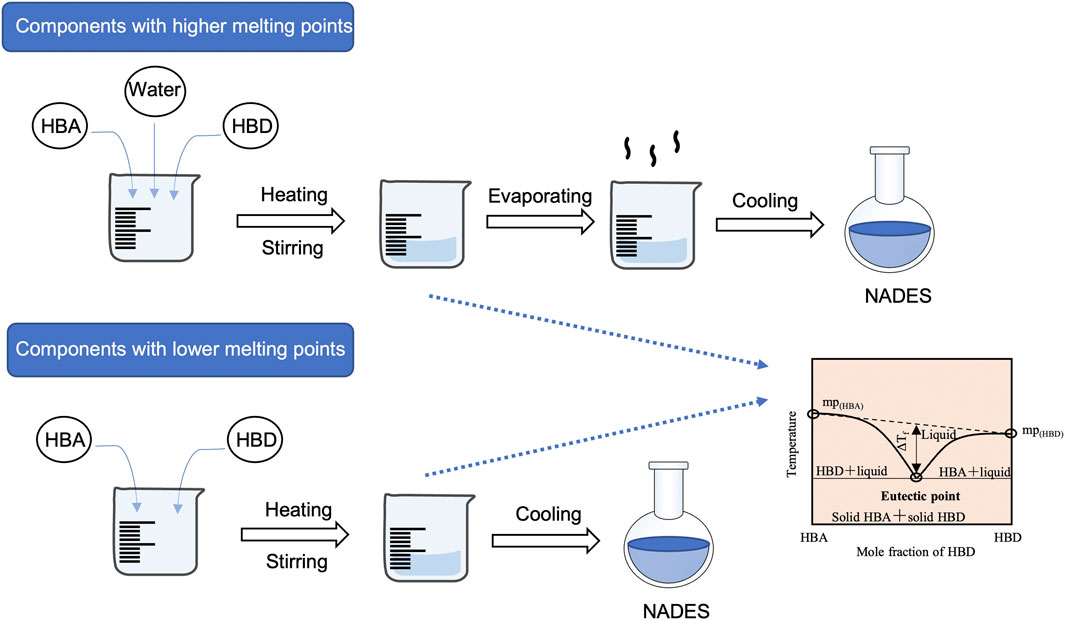
FIGURE 2. Preparation of NADES with components with higher/lower melting points. HBA, hydrogen bond acceptor; HBD, hydrogen bond donor; NADES, natural deep eutectic solvents.
For NADES components with lower melting points, the water is not necessary and the NADES can be prepared by directly mixing and constantly stirring two or three components in certain molar ratios under proper temperatures (e.g. ChCl and LA at the molar ratio of 1:1 at 60°C). Sometimes the heating should be performed with magnetic agitation to obtain a homogeneous transparent liquid. After cooling the mixture to room temperature, the transparency and homogeneity of the mixture could indicate that the designed ratio is feasible. In addition, all NADES prepared need to be kept in a desiccator before use (Xie et al., 2019).
Due to the difference in the properties of various components to prepare NADES, the modification to the preparation process is usually inevitable. Parameters such as the heating time, heating temperature, heating stability, and water ratio should be adjusted according to different properties of the components.
4 Classification of NADES
The hydrophilicity and hydrophobicity of NADES are essential indicators for evaluating their properties and applications. NADES without a clear prefix are identified as hydrophilic NADES (Figure 3) due to their prominent position in various studies and the richer source from the nature. For example, the primary metabolites of sugars [e.g., glucose (Glu), fructose (Fru), sucrose (Suc), and trehalose] in nature, amino acids [e.g., proline (Pro)], and organic acids [e.g., malic acid (MA) and CA], etc., are all candidates for the hydrophilic and designable eutectic compositions. The density of hydrophilic NADES is reported to be related to the molecular weight of HBAs and HBDs. The higher the molecular weight of the components is, the higher the density is (Fuad et al., 2021). For example, the hydrophilic NADES containing sugars and organic acids have relatively higher density, owing to their higher molecular weight. In comparison, the density of hydrophilic NADES containing alcohols is usually lower. Also, hydrophilic NADES often have higher viscosity. According to different types of HBDs, the viscosity of ChCl-based NADES and betaine (Bet)-based NADES was increased as follows: alcohols < organic acids and sugar. But NADES containing LA often have a relatively low viscosity (Freitas et al., 2022).
In contrast, the studies on hydrophobic NADES (Figure 4) are much less than that on hydrophilic NADES. van Osch et al. (2019) proposed four criteria for judging and evaluating the hydrophobic NADES, including i) the viscosity less is than 100 mPa s; ii) the difference between the density of NADES and the density of water is more than 50 kg m−3; iii) when NADES are mixed with water, a low transfer rate of NADES to the water phase should be presented; and iv) there is little or no change in pH value. The substances commonly used in the synthesis of hydrophobic NADES involve menthol (Men), thymol, coumarin, camphor, etc. (Martins et al., 2018), which have lower solubility in the water phase. The hydrophilicity and hydrophobicity of NADES depend on the carbon chain length of HBA and HBD. The longer the carbon chain contained in the structure is, the stronger the most hydrophobic effect expresses (Florindo et al., 2019). For example, L-Men, belonging to hydrophobic natural component with long alkyl chains, was often used as HBA to synthesize hydrophobic NADES (Ribeiro et al., 2015). Some bioactive compounds in CHMs, e.g. carthamin and rutin, showed poor solubility in water (Dai et al., 2014; Zhao, et al., 2015). Therefore, the hydrophobic NADES were used as the medium for dissolving and extracting them.
5 Current applications for NADES in CHMs
5.1 Extraction of active components in CHMs
Water, as the most widely used and easily accessed solvent in the extracting process of CHMs, is a good candidate to extract active components with strong polarity (Lu and Jiang, 2013). However, there are multiple bioactive components in CHMs with strong lipophilicity which exhibit very low solubility in water (Wang et al., 2018). Other traditional organic solvents such as methanol, ethanol, and ethyl acetate are also common solvents for extracting bioactive substances (Chemat et al., 2019). But many of them are volatile, flammable, explosive, and even toxic (Basant et al., 2016). In recent years, NADES have been designed to extract various bioactive compounds from CHMs due to their better biocompatibility, higher solubility, and subsequently higher extraction yields sometimes (Table 1). By investigating results in Table 1, we can find that the type of NADES components, molar ratio, water content, and several extraction conditions are major factors that would affect the extraction yield.
5.1.1 NADES components
According to Table 1, the ChCl-containing NADES were the most frequently-used solvents to extract bioactive compounds from CHMs, indicating that ChCl, especially as HBA, played an important role in this application. Additionally, more than half of the NADES were prepared with organic acids (e.g. MA, CA, and LA), which were more favorable for the extraction of alkaloids. The extraction yields using NADES with organic acids as HBDs were relatively higher than those containing sugars or alcohols (Li et al., 2020). Also, the organic acid-containing NADES exhibited an excellent extraction capacity when used to extract steroidal saponins (Yang et al., 2021) and phenolics (Dai et al., 2013), which are two representative components with various activities in CHMs. For extracting phenolic metabolites in the CHM of Carthamus tinctorius flower, Pro-MA and LA-Glu were found to be more efficient than 40% ethanol in the extraction process (Dai et al., 2014), which might be attributed to the formation of strong hydrogen bonds between molecules of NADES and phenolic compounds.
5.1.2 Molar ratio of NADES components
The physicochemical properties of NADES, e.g. viscosity, surface tension, and polarity, would change along with the variation in the molar ratios of NADES components, which often affect the extraction efficiency (Tian et al., 2008; Guo et al., 2019). In most cases in Table 1, NADES had the positive properties when the molar ratio of HBA and HBD was 1:1 or 1:2 when extracting bioactive components from CHMs. For example, ChCl-PG of 9 M ratios, i.e. 1:0.2,1:0.25, 1:0.33, 1:0.5, 1:1, 1:2, 1:3, 1:4, and 1:5 were prepared to extract ferulic acid from Ligusticum chuanxiong Hort. The results showed that ChCl-PG at the ratio of 1:2 had the highest extraction rate (Xie et al., 2019). The thermodynamic analysis showed that the hydrogen bond in NADES was the main force in the extraction process (Fan et al., 2020). And NADES with components at a certain ratio often came with a suitable viscosity, which thereby brought an increased number of hydrogen bonds and stronger force during the extraction. However, the optimized molar ratios for different NADES components were varied. Different ratios of the targeted NADES should be initially prepared to determine the optimal one.
5.1.3 Water content of NADES
Generally, NADES have higher viscosity than conventional solvents. And the water content of NADES is a key factor affecting the viscosity and the followed extraction yield (Craveiro et al., 2016; Wang et al., 2020b). NADES with higher viscosity would hinder the dissolution of bioactive components. In order to improve the extraction efficiency of NADES, a certain amount of water could be added to reduce the viscosity. For example, the extraction yield of phenolic compounds was increased along with the increased water content of Suc-ChCl from 10% to 25% (Dai et al., 2013). However, the water content of NADES and the extraction yield of target compounds were not in a linear relationship in most cases. The optimal extraction capacity of NADES could be only reached at a specific water content. Xie et al. investigated the extraction capacity of ChCl-PG (1:2) with a water content from 0% to 60%. The results showed that the extraction yield of ferulic acid was initially increased and then decreased, reaching a maximum extraction yield when the water content was 30% (Xie et al., 2019). The reason might be that the excessive water in NADES would increase the polarity of the solvent, whereas some compounds were difficult to be dissolved in solvents of high polarity (Wu et al., 2018). In addition, some research revealed that when the water content of NADES reached or exceeded 50%, it would negatively affect the stability of NADES. In such system, hydrogen bonds would be broken and the NADES components were completely dissociated and hydrated. Consequently, the physical and chemical properties of NADES would be altered. And the extraction yields of the target compounds were then decreased (Cui et al., 2018).
5.1.4 Extraction technique
UAE was the most used extraction technique to assist NADES in extracting bioactive components from plants. This might be due to its advantages of energy saving, high efficiency, safety, and environmental friendliness compared with other extraction methods (Cunha and Fernandes, 2018). According to results in Table 1, UAE was applied in more than 75% of the studies to extract components from CHMs with NADES. The higher extraction rate was considered to be related to the damaged structure of CHMs by the strong mechanical effect of ultrasound, making it more favorable for solubilizing large quantities of compounds into NADES (Li et al., 2020). Besides UAE, MAE was another commonly used extraction method, by which the microwaves with extremely high frequencies could penetrate the solvent and reach the cells inside the solid raw materials (Chan et al., 2016). The advantage of this method was that high levels of the target compounds could be obtained in a much shorter amount of time. The water in cells absorbed the energy from microwaves, which increased the temperature and pressure inside cells, resulting in cell disruption and dissolution of active components. However, MAE was frequently performed under higher temperature, which could affect the stability of active components (Zuo et al., 2021).
5.1.5 Extraction temperature
The extraction temperature often has a significant influence on the extraction yield of components from CHMs. Apart from adding a certain percentage of water, increasing the temperature was also a way to reduce the viscosity of NADES (Liu et al., 2018). Along with the increase of temperature, the energy of the molecules would be increased, which enabled them to dissolve and diffuse faster in the liquid media (Francisco et al., 2013). However, for the active molecules with poor thermal stability, they could be decomposed or even inactivated at higher temperatures. For example, the important peroxide group in the structure of artemisinin was decomposed under the influence of high temperature, which led to a lower extraction yield (Pan et al., 2021). Therefore, the determination of a suitable temperature is necessary in the extraction process.
5.1.6 Extraction time
In most cases, the yield of target compounds could be increased with a longer extraction time, and then maintained constant. For example, after the extraction time over 30 min, the extraction yield of artemisinin was hardly increased (Pan et al., 2021). The extraction yield of coumarin also reached the maximum at 30 min, and then was almost constant at 40 min and 50 min. This might be due to that the target bioactive components were completely extracted, or the compounds in the solvent were saturated. In some cases, there would be a declined phase, which mainly depended on the properties of the target bioactive components. When the extraction time or the exposure duration of active compounds to air was too long, they could be oxidized or decomposed, thereby resulting in a reduction of the extraction yield. The study from Xie et al. (2019) confirmed that a long extraction time was not suitable for the extraction of ferulic acid. Also, the energy consumption was another factor to consider to determine the extraction time. The extraction time in most of the studies summarized in Table 1 was less than 60 min.
5.1.7 Liquid-solid ratio
Studies have shown that a higher liquid-solid ratio could generally produce a positive effect on the extraction yield of compounds. With the increase of liquid-solid ratio, the solid matrix would have more contact with the solvent, which was beneficial for the dissolution of the target compounds. However, such positive effect could only be maintained at a certain range of the liquid-solid ratio. For example, the ratio of Bet-tartaric acid-water (1:1:1) to Coptis chinensis rhizome powder at 35 mL/g obtained the highest extraction yield of alkaloids. And then the adding of NADES failed to increase the extraction yield significantly (Li et al., 2020). Similar result could be found in another study, that the extraction yield of GA was increased with the increase of liquid-solid ratio from 10 to 30 mL/g. However, when the liquid-solid ratio reached 40 mL/g, the extraction yield was decreased, which might be due to a cavitation effect between the excessive solvent and the solid powder (Lanjekar and Rathod, 2021). Moreover, the higher ratio of solvent is not cost effective in the industrial scale.
5.2 Improving the bioavailability of CHMs
5.2.1 Improving the oral bioavailability of active components from CHMs
Oral administration is the most common way of administration of CHMs. However, some active components from CHMs with low bioavailability can be poorly absorbed by human body when taken orally. For example, paeoniflorin showed strong anti-depression activity. But its oral bioavailability was determined as 2.32% (Yu et al., 2019). According to the Biopharmaceutics Classification System II substances, the bioavailability may be enhanced by increasing the solubility and dissolution rate of the drug in the gastrointestinal fluids (Khadka et al., 2014). NADES are green solvents which have been applied in increasing the solubility and oral bioavailability of some components through the hydrogen-bond interaction with drugs.
Chen et al. (2017) evaluated the influence of ChCl-GL (1:2) on the pharmacokinetics of SAB, one of the active components from Salvia miltiorrhiza Bge. used to relieve the pain and calm the mind in TCM. They found that compared with water, ChCl-GL could promote the absorption of SAB, showing a higher peak concentration and shorter peak time than dissolving SAB in water. Another example is about berberine, a quaternary benzylisoquinoline alkaloid in many Chinese medicinal plants such as Berberis spp. and Coptis spp. Despite its demonstrated therapeutic efficacies in anti-inflammatory, anti-microbial, and cholesterol-lowering treatments, the orally administered berberine can be poorly absorbed and rapidly undergone extensive metabolism (Spinozzi et al., 2014). Sut et al. (2017) investigated the pharmacokinetic profiles of berberine solubilized in three considered NADES after orally administered to mice. The results revealed a 2–20 folds increase of berberine level in blood concentration when treating with NADES-berberine compared with the treatment of water-berberine. The similar trend was found in another pioneering work by Farggian et al. (Faggian et al., 2016), that the NADES of Pro-glutamic acid significantly enhanced the maximum concentration and the area under curve of rutin in Balb/c mice. It revealed an increase in the relative bioavailability of rutin in the NADES formulation of approximately 100% when compared with its behavior in the corresponding aqueous solution. Also, 25 different NADES were prepared for the extraction of PUE and its two natural derivatives from the dried root of Pueraria lobata (Willd.) Ohwi (Huang et al., 2021). According to the results, the optimized NADES increased the extraction yields of PUE and its derivatives by 2.2–3.4 folds compared with water. Furthermore, the relative oral bioavailability of PUE in NADES was 323%, which was even higher than the nanocrystal self-stabilized Pickering emulsion and self-microemulsifying drug-delivery systems of PUE (Qiao et al., 2018; Zhang et al., 2018).
5.2.2 Promoting the skin-permeation of CHMs
Among various administration routes of drugs, the transdermal drug delivery system (TDDS) is favored due to its advantages of improved bioavailability, more uniform plasma levels, reduced side effects and first-pass drug degradation effects because of the maintenance of plasma levels up to the end of the dosing interval compared with a decline in plasma levels by conventional oral dosage forms (Patel et al., 2012). It has been reported that NADES can significantly enhance the percutaneous absorption of small molecules as well as macromolecular drugs, such as proteins, small interfering RNAs, and polysaccharides in TDDS systems (Berton et al., 2017; Qu et al., 2019).
Inspired by these applications, Xiao et al. (2022) developed a novel hydrogel TDDS incorporating an amino acid-based NADES for the CHM product of “Sanwujiaowan”, which is original prepared as pills for oral administration to treat rheumatoid arthritis and rheumatoid myositis. Sanwujiaowan is composed of the extracts of five CHM ingredients, some of which contain components with certain degree of toxicity. By preparing the NADES-extract complex, it was found that there were excellent dissolution and skin permeability of the components in the extracts. Moreover, the consequent hydrogel with the NADES-extract complex exerted an enhanced therapeutic effect that significantly reduced the inflammatory response with systemic toxicity of the extracts. Wang et al. (2017) prepared a microemulsion based on the NADES of paeonol and Men, which are active components from the root bark of Paeonia suffruticosa Andr. and the herb of Mentha haplocalycis Briq, respectively. The results showed that the microemulsion-based gel formulations facilitated the drug permeation compared with the simple mixture gel of paeonol and Men without forming a NADES system.
5.3 Improving the stability of active components in CHMs
NADES could significantly enhance the stability of some bioactive components in CHMs, e.g. phenolic compounds (Dai et al., 2013), without weaking their activities. Carthamus tinctorius L. is a CHM widely used in the clinic to treat cardiovascular diseases, which owns efficacies of invigorating the circulation of blood, dissipating blood stasis, and relieving pains (Kim and Paik, 1997). Carthamin is one of the major active components in Carthamus tinctorius L, which has strong antioxidant activity (Jeliński et al., 2019). However, carthamin is unstable in aqueous solutions (Sut et al., 2017) and also sensitive to the change of temperature (Ji et al., 2016). It was found that the sugar-based NADES with lower water content could significantly improve the stability of carthamin under various conditions such as high temperature, light exposure, and long storage time, compared with other solvents of water and 40% ethanol (Dai et al., 2014). This increased stability was attributed to the high viscosity and low mobility of NADES. The free carthamin molecules were encapsulated tightly, thereby restricting the movement of the solute molecules and enhancing their stability, which was consistent with previous conclusion that NADES containing sugar generally possessed higher viscosity. Similarly, Wikene et al. (2015) investigated the effect of NADES on the stability of curcumin, an active component in the CHM of Curcuma longa L, which is sensitive to light and temperature under normal conditions. They synthesized several NADES and found that the hydrolytic stability of curcumin in NADES was comparable to or up to 2–10 times higher than in cyclodextrins and up to >1300 times higher than in the buffer solution at pH 8.
5.4 Elucidation of possible molecular bases for traditional processing theory of CHMs
Processing of CHMs, also named Paozhi, is a treatment to improve the medical applications of CHMs in TCM. Its functions include altering the flavor, moderating the medicinal properties, and eliminating the side effects or toxicities of the crude materials. The importance of processing materials has already been mentioned in the Huang Di Nei Jing (The Yellow Emperor’s Internal Classic, 475–221 B.C.) and Shen Nong Ben Cao Jing (Divine Husbandman’s Classic of the Materia Medica, c. 220 A.D.) (Chang et al., 2011). In the current TCM practice, all the materials are strictly required to be properly processed before using for therapeutic application. The techniques of Paozhi include cutting, crushing, steaming, calcining, stir-frying, with or without adjuvants (Dai et al., 2021). Honey is one of the most common adjuvants in the stir-frying of Paozhi, which could keep its liquidity even with a low water content and was hypothesized to be a sugar-based NADES. The stir-frying with honey often begins with the dilution of boiled water. Then the honey solution is added to the cleaned and cut plant materials, mixed thoroughly, kept for a while and then stir-fried with gentle heat until the materials get a specific color (Chinese Pharmacopoeia Committee, 2020). This process has the following effects according to TCM theory: 1) moistening lungs for relieving cough (e.g. Stemono Radix); 2) enhancing the tonifying effects on spleen and nourishing qi (e.g. Astragali Radix); 3) moderating the properties of raw materials (e.g. Ephedra Herba); and 4) correcting the flavor and eliminating the side effects of drugs (e.g. Aristolochia Fructus) (Jin, 1988).
To prove the hypothesis that honey behaves like a NADES in the process of Paozhi, Dai et al. (2021) processed Astragali Radix with or without honey in different ways. They found that the level of isoflavonoids and saponins were far more increased by honey and NADES treated samples than in the decoction of raw materials. It indicated that the artificial honey using a sugar-based NADES, had the same effect as real honey, which supported the hypothesis that honey, similar as NADES, could improve the solubility of medium polar plant secondary metabolites present in the plant materials. Consequently, the bioeffects of honey and NADES on estrogen receptor, androgen receptor, and antioxidant-related factors were significantly improved than those of the raw material decoction, which was consistent with the traditional function of honey in Paozhi. Based on a series of studies, it was suggested that the mechanisms of honey in the stir-frying process, behaving like NADES, might promote the conversion of aglucones to glucosides, inhibit the hydrolysis of the glucosides, inhibit acetylation of isoflavonoids and astragalosides, increase deacetylation of acetyl-containing isoflavonoids and astragalosides, inhibit the hydrolysis of the malonylated and/or acetylated glucosides, and improve the extraction of the isoflavonoids glucosides if compared to the aglycones (Ma et al., 2009; Zheng et al., 2014).
6 Prospects of future applications for NADES in CHMs and challenges
6.1 Prospects of future applications
6.1.1 Development of active pharmaceutical ingredient (API)-NADES formulations
CHMs are great source of multiple APIs which have potential to be developed as drugs. Unlike the previous reviewed applications (Figure 5) that NADES were mostly used as solvents for APIs in CHMs, it is promising that APIs from CHMs could be directly used as NADES phase-forming constituents by acting as HBDs and/or HBAs, to exhibit higher therapeutic effects or improved properties through their own eutectic systems, i.e. API-NADES. The formulation of API-NADES could usually improve the solubility and permeability of the APIs in comparison to their isolated solid forms, as the liquid state of the APIs confers them an enhanced bioavailability and thus, better efficacy (Roda et al., 2020). In the study from Wang et al. (2017), the permeation performance of paeonol was significantly increased in its eutectic mixture with methanol. The reasons might be that: i) the NADES mixture may cause the leaching of the lipids in the skin and thereby formation of pores, and/or ii) it may depress the melting point of drug to below skin temperature thereby increase the drug solubility (Park et al., 2012). Due to the property as a permeation enhancer, Men has been widely used in API-NADES formulations with chemical drugs such as ibuprofen and aspirin (Yong et al., 2003; Aroso et al., 2016), which increased folds of permeation capability of those drugs compared to the pure form of them.
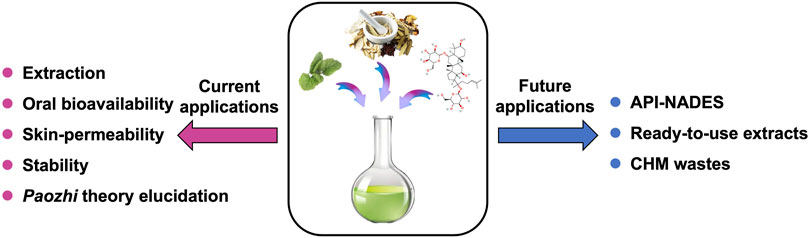
FIGURE 5. Current and future applications for NADES in CHMs. CHMs, Chinese herbal medicines; NADES, natural deep eutectic solvents.
In some other cases, the formulation could also improve the stability of APIs and even promote a controlled release to achieve the therapeutic effect. In the work from Mano et al. (2017), the API-NADES composed by ChCl-mandelic acid (1:2) and encapsulated in gelatin showed a fast-dissolving release profile in phosphate buffered saline without cytotoxicity. The formulation also maintained the anti-bacterial effect of mandelic acid on both Gram-positive and negative bacteria. Inspired by these applications, it is rather promising to develop API-NADES formulations based on various components from CHMs to improve their targeted properties, such as solubility, bioavailability, stability, or fast drug delivery capacity to the target site.
6.1.2 Development of ready-to-use NADES extract of CHMs
NADES represent a new green strategy to overcome the toxicity and environmental disadvantages of conventional organic solvents, which have been demonstrated as efficient as conventional organic solvents for the extraction of multiple components from fruits, seeds, flowers, leaves, and other parts of plants (da Silva et al., 2021). In the meantime, the solvents in many of those NADES extracts did not need to be removed, and no toxicity was observed from the NADES per se. Thus, such NADES mixtures have the potential to be used as ready-to-use extracts for the development of functional foods, nutraceuticals, or cosmetic products.
In the study from Komaikul et al. (2021), they extracted stilbenoids from Morus alba callus by using a selected NADES of chloride-GL mixture. The NADES extract of Morus alba callus showed comparable anti-melanogenesis activity compared with methanol extract and no irritation effect on reconstructed human epidermis. After 6 months of storage, the stilbenoids in the NADES remained stable at the storage temperature of 4°C. It suggested NADES could be advantageous for the preparation of skin-lightening cosmetics without removing the solvent. Besides being an eco-friendly and non-irritable solvent for the extraction of active phytochemicals, some NADES showed potential to be used as a ready-to-use vehicle for increasing oral absorption of bioactive compounds. For example, da Silva et al. (2021) investigated if a ready-to-use extract obtained using a NADES affects the pharmacokinetic profile of blueberry phenolic compounds compared to organic solvent-extracted compounds. The non-compartmental pharmacokinetic analysis revealed that the NADES extract increased the bioavailability of anthocyanins by 140% compared to the organic solvent extract. And the stability of phenolic compounds in NADES was increased during in vitro digestion by delaying gastric chyme neutralization.
Those strategies could be referred for the development of products based on CHMs. For CHMs, a large number of them have been investigated for the development of cosmetic products with anti-oxidative, anti-wrinkle, skin lightening and other types of skin-care functions; or pharmaceuticals to treat or prevent various diseases. Therefore, the combination of CHMs or their components with NADES, especially the preparation of related ready-to-use extracts, could be a direction for the further development of products used in the cosmeceutical or pharmaceutical, or even nutraceutical industries.
6.1.3 Utilization of CHMs waste with NADES
Based on TCM theory, only certain parts of medicinal herbs are harvested and processed as CHMs in the clinic. For example, the main root of Panax ginseng Meyer is traditionally and widely accepted as the medicinal part of this CHM, whereas other parts, e.g. fibrous roots, leaves, flower buds, and fruits, were hardly used. However, according to the chemical analysis, the content of ginsenosides, the major active components of Panax ginseng Meyer, was higher in the leaf and fibrous roots (Shi et al., 2007). Similar results were found in Panax notoginseng (Burk.) F. H. Chen, the protopanaxadiol type saponins were rich in the leaves and flowers, whereas rare in the underground parts (Wan et al., 2006). Those findings suggested that the sustainable development and rational utilization of CHMs waste or non-medicinal parts would be necessary and might increase the economic value of related industries.
To recover active components from agro-industrial wastes or convert those wastes into a wide range of bio-based products based on the biorefinery concept, NADES have been employed in a number of studies and shown an advantageous potential to utilize the biowastes. In the study of Bertolo et al. (2021), NADES were used as alternative solvents to recover the phenolic compounds from the pomegranate peel waste. Under optimal extraction conditions, the phenolic recovery rate with ChCl and LA was increased by 54.6%, when compared with the non-optimized conditions, and by 84.2%, when compared with the ethanolic extraction. In another biorefinery research, Kumar et al. (2018) conducted a comprehensive evaluation of an integrated process for cellulosic ethanol production from a NADES based pretreated rice straw. They obtained the maximum ethanol yield with a theoretical conversion efficiency of 79.9%. These studies demonstrated the potential of NADES as an effective environmentally friendly alternative for obtaining valuable compounds from agro-industrial waste, and the applicability to be used in the economically viable integrated biorefinery process. Considering the commonality in the material base and processing method of many CHMs waste and agro-industrial one, those applications with NADES could be learned for the better utilization of CHMs waste in the future.
6.2 Challenges of applying NADES in CHMs
Even though NADES offer several benefits as compared with traditional solvents, e.g. lower toxicity, higher extractability of diverse compounds with varied polarity, and capacity to improve the bioactivity and stability, there are still challenges and limits when used in CHMs due to their inherent properties.
Due to the high viscosity and low vapor pressure of NADES, the recycling and reusing of NADES after the extraction process is the major challenge to develop a cost-effective extraction process, which might impede its application at industrial scale or in continuous-flow reactions. In most cases, the resins or molecular sieves were used to recover active components from the NADES extract, which also involved the use of large number of alternative solvents. When those alternative solvents were organic ones, this process seems to, eventually, counterbalance the benefits of using NADES. Sometimes the addition of antisolvents (ethanol or water) is probably the most valuable method since it does not require a specific equipment or additional investment and allows the processing in a sustainable way. After extracting the solutes by precipitation or by forming an ethanol- or water-insoluble layer, the initial NADES could be recovered by removing ethanol or water through evaporation. Yet, it cannot be used for ethanol- or water-soluble NADES. Therefore, further research in the final recycling or purification step should be a future focus to implement an efficient, eco-compatible, and sustainable extraction of APIs from CHMs with NADES as the solvents. Another possible solution could be the development of ready-to-use extracts as what has been proposed in Section 6.1.2, which does not need to remove the solvents.
Moreover, even though the components of NADES are generally considered to be safe and non-toxic, there is no comprehensive toxicity evaluation in vivo of different NADES yet. According to an acute toxicity assessment by using mice (Mbous et al., 2017), the investigated NADES exhibited a certain degree of hepatotoxic effect. This was attributed to the viscosity of these solvents, which might have failed to circulate properly in mice, and then blocked and halted blood flow. Another risk might be induced by the increased permeability of NADES. Based on the results from the same study above, the NADES treated cells had an increased porosity compared with the control cells without NADES treatment, suggesting an increased capacity of NADES to perforate cellular membranes. On the one hand, this property might increase the therapeutic effects of possible NADES-CHMs extracts as discussed before. On the other hand, it could be also possible to increase the toxicity of those formulations due to the increased permeability of NADES. Therefore, besides the accurate selection of HBA and HBD for optimizing the extraction of targeted APIs in a CHM, the toxicity evaluation of the NADES and the corresponding NADES-CHM extract should be also an integral part in further studies.
7 Conclusion
CHMs have been used to prevent and treat diseases for thousands of years, of which the medical claims are supported by clinical evidence of a long history. With the development of extraction and purification technology, more and more bioactive compounds have been identified and obtained from CHMs, making them an important resource for the discovery of APIs. In the meantime, CHMs have their own unique features. For example, they are administered under the guidance of TCM theory and often given in formulars. NADES, as novel green solvents, are mostly used to extract multiple phytochemicals and food components, showing advantages over the organic solvents, such as enhanced bioactivity of bioactive compounds and their higher stability in NADES under various storage conditions. Their special properties offer the possibility for the development of CHMs as well as related products, including extracting APIs in a more effective way, increasing the stability, bioavailability, and skin-permeability of components or extracts from CHMs, and even shedding light on the elucidation of traditional Paozhi theory. The applications of NADES in cosmeceutical, pharmaceutical, and nutraceutical areas also inspired those in CHMs, such as the preparation of API-NADES formulations, development of ready-to-use CHM-NADES extracts, and utilization of non-medicinal parts from CHM plants. Apart from that, the challenges coexist with the opportunities, which reminds us to pay more attention on research of recycling or recovering the solvents, and the necessary toxicity assessment in the future.
Author contributions
ML, CR, and YX wrote this paper; XY, MW, and BY participated in the revision work; CW provided the technical support; ML, CR, YX, and LG did the literature studies; YX and XC supervised the project. All the authors read and approve the final manuscript.
Funding
This work was supported by the Fund of Yunnan Quality and Technology Supervision Bureau (KKPT202126008), National Natural Science Foundation of China (82260698); Key R&D Project of Yunnan Provincial Science and Technology Department (202003AC100013), and Fund of Yunnan Key Laboratory of Innovative Application of Chinese Medicines (02205AG070005).
Conflict of interest
Author MW was employed by the company SU Biomedicine BV.
The remaining authors declare that the research was conducted in the absence of any commercial or financial relationships that could be construed as a potential conflict of interest.
Publisher’s note
All claims expressed in this article are solely those of the authors and do not necessarily represent those of their affiliated organizations, or those of the publisher, the editors and the reviewers. Any product that may be evaluated in this article, or claim that may be made by its manufacturer, is not guaranteed or endorsed by the publisher.
Abbreviations
API, active pharmaceutical ingredient; Bet, betaine; CA, citric acid; ChCl, choline chloride; CHMs, Chinese herbal medicines; DES, deep eutectic solvents; Fru, fructose; GA, glycyrrhizic acid; GL, glycerol; Glu, glucose; Gly, glycerin; HBA, hydrogen bond acceptor; HBD, hydrogen bond donor; ILs, ionic liquids; LA, lactic acid; MA, malic acid; MAE, microwave-assistance extraction; Men, menthol; NADES, natural deep eutectic solvents; PG, propanediol; Pro, proline; PUE, puerarin; SAB, salvianoli cacid B; Suc, sucrose; TCM, traditional Chinese medicine; TDDS, transdermal drug delivery system; UAE, ultrasound-assistance extraction.
References
Abbott, A. P., Boothby, D., Capper, G., Davies, D. L., and Rasheed, R. K. (2004). Deep eutectic solvents formed between choline chloride and carboxylic acids: Versatile alternatives to ionic liquids. J. Am. Chem. Soc. 126, 9142–9147. doi:10.1021/ja048266j
Abbott, A. P., Capper, G., Davies, D. L., Rasheed, R. K., and Tambyrajah, V. (2003). Novel solvent properties of choline chloride/urea mixturesElectronic supplementary information (ESI) available: Spectroscopic data. See http://www.rsc.org/suppdata/cc/b2/b210714g/. Chem. Comm., 70–71. doi:10.1039/b210714g
Aggarwal, B. B., Bhatt, I. D., and Ichikawa, H. (2007). Curcumin–biological and medicinal properties. Turmeric: The Genus Curcuma. Boca Raton, FLLondon: CRC Press, 297–368.
Allegra, A., Innao, V., Russo, S., Gerace, D., Alonci, A., and Musolino, C. (2017). Anticancer activity of curcumin and its analogues: Preclinical and clinical studies. Cancer Invest. 35, 1–22. doi:10.1080/07357907.2016.1247166
Aroso, I. M., Silva, J. C., Mano, F., Ferreira, A. S. D., Dionísio, M., Sá-Nogueira, I., et al. (2016). Dissolution enhancement of active pharmaceutical ingredients by therapeutic deep eutectic systems. Eur. J. Pharm. Biopharm. 98, 57–66. doi:10.1016/j.ejpb.2015.11.002
Basant, N., Gupta, S., and Singh, K. P. (2016). Predicting the acute neurotoxicity of diverse organic solvents using probabilistic neural networks based QSTR modeling approaches. Neurotoxicology 53, 45–52. doi:10.1016/j.neuro.2015.12.013
Bertolo, M. R. V., Martins, V. C. A., Plepis, A. M. G., and Bogusz, S. (2021). Utilization of pomegranate peel waste: Natural deep eutectic solvents as a green strategy to recover valuable phenolic compounds. J. Clean. Prod. 327, 129471. doi:10.1016/j.jclepro.2021.129471
Berton, P., Di Bona, K. R., Yancey, D., Rizvi, S. A. A., Gray, M., Gurau, G., et al. (2017). Transdermal bioavailability in rats of lidocaine in the forms of ionic liquids, salts, and deep eutectic. ACS Med. Chem. Lett. 8, 498–503. doi:10.1021/acsmedchemlett.6b00504
Brennecke, J. F., and Maginn, E. J. (2001). Ionic Liquids: Innovative fluids for chemical processing. AIChE J. 47, 2384–2389. doi:10.1002/aic.690471102
Bushnell, P. J., Boyes, W. K., Shafer, T. J., Bale, A. S., and Benignus, V. A. (2007). Approaches to extrapolating animal toxicity data on organic solvents to public health. Neurotoxicology 28, 221–226. doi:10.1016/j.neuro.2006.03.013
Cai, S. Q., Wang, R., Yang, X., Shang, M., Ma, C., and Shoyama, Y. (2006). Antiviral flavonoid-type C-glycosides from the flowers of Trollius chinensis. Chem. Biodivers. 3, 343–348. doi:10.1002/cbdv.200690037
Cao, H., Ren, M., Guo, L., Shang, H., Zhang, J., Song, Y., et al. (2010). JinQi-jiangtang tablet, a Chinese patent medicine, for pre-diabetes: A randomized controlled trial. Trials 11, 27. doi:10.1186/1745-6215-11-27
Capello, C., Fischer, U., and Hungerbühler, K. (2007). What is a green solvent? A comprehensive framework for the environmental assessment of solvents. Green Chem. 9, 927–934. doi:10.1039/B617536H
Chan, C. H., Yeoh, H. K., Yusoff, R., and Ngoh, G. C. (2016). A first-principles model for plant cell rupture in microwave-assisted extraction of bioactive compounds. J. Food Eng. 188, 98–107. doi:10.1016/j.jfoodeng.2016.05.017
Chang, S. J., Huang, S. H., Lin, Y. J., Tsou, Y. Y., and Lin, C. W. (2014). Antiviral activity of Rheum palmatum methanol extract and chrysophanol against Japanese encephalitis virus. Arch. Pharm. Res. 37, 1117–1123. doi:10.1007/s12272-013-0325-x
Chang, W. T., Choi, Y. H., Van der Heijden, R., Lee, M. S., Lin, M. K., Kong, H., et al. (2011). Traditional processing strongly affects metabolite composition by hydrolysis in Rehmannia glutinosa roots. Chem. Pharm. Bull. 59, 546–552. doi:10.1248/cpb.59.546
Chemat, F., Vian, M. A., Ravi, H. K., Khadhraoui, B., Hilali, S., Perino, S., et al. (2019). Review of alternative solvents for green extraction of food and natural products: Panorama, principles, applications and prospects. Molecules 24, 3007. doi:10.3390/molecules24163007
Chen, J., Wang, Q., Liu, M., and Zhang, L. (2017). The effect of deep eutectic solvent on the pharmacokinetics of salvianolic acid B in rats and its acute toxicity test. J. Chromatogr. B 1063, 60–66. doi:10.1016/j.jchromb.2017.08.016
Chen, R., Li, D., Ruan, G., Liu, S., Sun, K., Huang, S., et al. (2019). Green and efficient extraction of flavoniods and saponins from Abrus cantoniensis Hance by deep eutectic solvents. Nat. Prod. Res. Dev. 31, 1632–1640. doi:10.16333/j.1001-6880.2019.9.022
Chen, Y., Zhang, N., Ma, J., Zhu, Y., Wang, M., Wang, X., et al. (2016). A Platelet/CMC coupled with offline UPLC-QTOF-MS/MS for screening antiplatelet activity components from aqueous extract of Danshen. J. Pharm. Biomed. Anal. 117, 178–183. doi:10.1016/j.jpba.2015.06.009
Chinese Pharmacopoeia Committee (2020). Pharmacopoeia of the people’s Republic of China Part I. Beijing: Chinese Medical Science and Technology Press.
Choi, Y. H., van Spronsen, J., Dai, Y., Verberne, M., Hollmann, F., Arends, I. W. C. E., et al. (2011). Are natural deep eutectic solvents the missing link in understanding cellular metabolism and physiology? Plant Physiol. 156, 1701–1705. doi:10.1104/pp.111.178426
Craveiro, R., Aroso, I., Flammia, V., Carvalho, T., Viciosa, M. T., Dionísio, M., et al. (2016). Properties and thermal behavior of natural deep eutectic solvents. J. Mol. Liq. 215, 534–540. doi:10.1016/j.molliq.2016.01.038
Cui, Q., Liu, J., Wang, L., Kang, Y., Meng, Y., Jiao, J., et al. (2018). Sustainable deep eutectic solvents preparation and their efficiency in extraction and enrichment of main bioactive flavonoids from sea buckthorn leaves. J. Clean. Prod. 184, 826–835. doi:10.1016/j.jclepro.2018.02.295
Cunha, S. C., and Fernandes, J. O. (2018). Extraction techniques with deep eutectic solvents. Trends Anal. Chem. 105, 225–239. doi:10.1016/j.trac.2018.05.001
da Silva, D. T., Smaniotto, F. A., Costa, I. F., Baranzelli, J., Muller, A., Somacal, S., et al. (2021). Natural deep eutectic solvent (NADES): A strategy to improve the bioavailability of blueberry phenolic compounds in a ready-to-use extract. Food Chem. 364, 130370. doi:10.1016/j.foodchem.2021.130370
Dai, Y., Choi, Y. H., and Verpoorte, R. (2021). Honey in traditional Chinese medicine: A guide to future applications of NADES to medicines. Adv. Botanical Res. 97, 361–384. doi:10.1016/bs.abr.2020.09.011
Dai, Y., Verpoorte, R., and Choi, Y. H. (2014). Natural deep eutectic solvents providing enhanced stability of natural colorants from safflower (Carthamus tinctorius). Food Chem. 159, 116–121. doi:10.1016/j.foodchem.2014.02.155
Dai, Y., Witkamp, G. J., Verpoorte, R., and Choi, Y. H. (2013). Natural deep eutectic solvents as a new extraction media for phenolic metabolites in Carthamus tinctorius L. Anal. Chem. 85, 6272–6278. doi:10.1021/ac400432p
Doldolova, K., Bener, M., Lalikoğlu, M., Aşçı, Y. S., Arat, R., and Apak, R. (2021). Optimization and modeling of microwave-assisted extraction of curcumin and antioxidant compounds from turmeric by using natural deep eutectic solvents. Food Chem. 353, 129337. doi:10.1016/j.foodchem.2021.129337
Duan, L., Dou, L. L., Guo, L., Li, P., and Liu, E. H. (2016). Comprehensive evaluation of deep eutectic solvents in extraction of bioactive natural products. ACS Sustain. Chem. Eng. 4, 2405–2411. doi:10.1021/acssuschemeng.6b00091
Duan, L., Zhang, W. H., Zhang, Z. H., Liu, E. H., and Guo, L. (2018). Evaluation of natural deep eutectic solvents for the extraction of bioactive flavone C-glycosides from Flos Trollii. Microchem. J. 145, 180–186. doi:10.1016/j.microc.2018.10.031
Espino, M., Fernández, M. D. L. A., Gomez, F. J. V., and Silva, M. F. (2016). Natural designer solvents for greening analytical chemistry. Trends Anal. Chem. 76, 126–136. doi:10.1016/j.trac.2015.11.006
Faggian, M., Sut, S., Perissutti, B., Baldan, V., Grabnar, I., and Dall’Acqua, S. (2016). Natural deep eutectic solvents (NADES) as a tool for bioavailability improvement: Pharmacokinetics of rutin dissolved in proline/glycine after oral administration in rats: Possible application in nutraceuticals. Molecules 21, 1531. doi:10.3390/molecules21111531
Fan, Y., Luo, Q., Wei, J., Lin, R., Lin, L., Li, Y., et al. (2018). Mechanism of salvianolic acid B neuroprotection against ischemia/reperfusion induced cerebral injury. Brain Res. 1679, 125–133. doi:10.1016/j.brainres.2017.11.027
Fan, Y., Wu, H., Cai, D., Yang, T., and Yang, L. (2020). Effective extraction of harmine by menthol/anise alcohol-based natural deep eutectic solvents. Sep. Purif. Technol. 250, 117211. doi:10.1016/j.seppur.2020.117211
Florindo, C., Branco, L. C., and Marrucho, I. M. (2019). Quest for green-solvent design: From hydrophilic to hydrophobic (deep) eutectic solvents. ChemSusChem 12, 1549–1559. doi:10.1002/cssc.201900147
Florindo, C., Oliveira, F. S., Rebelo, L. P. N., Fernandes, A. M., and Marrucho, I. M. (2014). Insights into the synthesis and properties of deep eutectic solvents based on cholinium chloride and carboxylic acids. ACS Sustain. Chem. Eng. 2, 2416–2425. doi:10.1021/sc500439w
Francisco, M., Van Den Bruinhorst, A., and Kroon, M. C. (2013). Low-transition-temperature mixtures (LTTMs): A new generation of designer solvents. Angew. Chem. Int. Ed. 52, 3074–3085. doi:10.1002/anie.201207548
Freitas, D. S., Rocha, D., Castro, T. G., Noro, J., Castro, V. I. B., Teixeira, M. A., et al. (2022). Green extraction of cork bioactive compounds using natural deep eutectic mixtures. ACS Sustain. Chem. Eng. 10, 7974–7989. doi:10.1021/acssuschemeng.2c01422
Fuad, F. M., Nadzir, M. M., and Harun, A. (2021). Hydrophilic natural deep eutectic solvent: A review on physicochemical properties and extractability of bioactive compounds. J. Mol. Liq. 339, 116923. doi:10.1016/j.molliq.2021.116923
Grønlien, K. G., Pedersen, M. E., and Tønnesen, H. H. (2020). A natural deep eutectic solvent (NADES) as potential excipient in collagen-based products. Int. J. Biol. Macromol. 156, 394–402. doi:10.1016/j.ijbiomac.2020.04.026
Guo, N., Kou, P., Jiang, Y. W., Wang, L. T., Niu, L. J., Liu, Z. M., et al. (2019). Natural deep eutectic solvents couple with integrative extraction technique as an effective approach for mulberry anthocyanin extraction. Food Chem. 296, 78–85. doi:10.1016/j.foodchem.2019.05.196
Guo, Q., Zhao, L., You, Q., Yang, Y., Gu, H., Song, G., et al. (2007). Anti-hepatitis B virus activity of wogonin in vitro and in vivo. Antivir. Res. 74, 16–24. doi:10.1016/j.antiviral.2007.01.002
Hayyan, M., Hashim, M. A., Al-Saadi, M. A., Hayyan, A., AlNashef, I. M., and Mirghani, M. E. (2013). Assessment of cytotoxicity and toxicity for phosphonium-based deep eutectic solvents. Chemosphere 93, 455–459. doi:10.1016/j.chemosphere.2013.05.013
He, X., Yang, J., Huang, Y., Zhang, Y., Wan, H., and Li, C. (2019). Green and efficient ultrasonic-assisted extraction of bioactive components from Salvia miltiorrhiza by natural deep eutectic solvents. Molecules 25, 140. doi:10.3390/molecules25010140
Huang, Y., Yang, J., Zhao, Y., Yu, L., He, Y., Wan, H., et al. (2021). Screening, optimization, and bioavailability research of natural deep eutectic solvent extracts from radix pueraria. Molecules 26, 729. doi:10.3390/molecules26030729
Jeliński, T., Przybyłek, M., and Cysewski, P. (2019). Natural deep eutectic solvents as agents for improving solubility, stability and delivery of curcumin. Pharm. Res. 36, 1–10. doi:10.1007/s11095-019-2643-2
Ji, L., Du, Q., Li, Y. T., and Hu, W. (2016). Puerarin inhibits the inflammatory response in atherosclerosis via modulation of the nf-κb pathway in a rabbit model. Pharmacol. Rep. 68, 1054–1059. doi:10.1016/j.pharep.2016.06.007
Jin, S. Y. (1988). Science of processing Chinese materia medica. Jiangsu: Jiangsu Science and Technology Press.
Juneidi, I., Hayyan, M., and Mohd Ali, O. (2016). Toxicity profile of choline chloride-based deep eutectic solvents for fungi and Cyprinus carpio fish. Environ. Sci. Pollut. Res. 23, 7648–7659. doi:10.1007/s11356-015-6003-4
Kelley, S. P., Narita, A., Holbrey, J. D., Green, K. D., Reichert, W. M., and Rogers, R. D. (2013). Understanding the effects of ionicity in salts, solvates, co-crystals, ionic co-crystals, and ionic liquids, rather than nomenclature, is critical to understanding their behavior. Cryst. Growth Des. 13, 965–975. doi:10.1021/cg4000439
Khadka, P., Ro, J., Kim, H., Kim, I., Kim, J. T., Kim, H., et al. (2014). Pharmaceutical particle technologies: An approach to improve drug solubility, dissolution and bioavailability. Asian J. Pharm. Sci. 9, 304–316. doi:10.1016/j.ajps.2014.05.005
Kim, J. B., and Paik, Y. S. (1997). Stability of carthamin from Carthamus tinctorius in aqueous solution: pH and temperature effects. Arch. Pharm. Res. 20, 643–646. doi:10.1007/bf02975225
Kim, S. J., Kim, M. C., Lee, B. J., Park, D. H., Hong, S. H., and Um, J. Y. (2010). Anti-Inflammatory activity of chrysophanol through the suppression of NF-kappaB/caspase-1 activation in vitro and in vivo. Molecules 15, 6436–6451. doi:10.3390/molecules15096436
Komaikul, J., Mangmool, S., Putalun, W., and Kitisripanya, T. (2021). Preparation of readily-to-use stilbenoids extract from Morus alba callus using a natural deep eutectic solvent. Cosmetics 8, 91. doi:10.3390/cosmetics8030091
Kong, W., Wei, J., Abidi, P., Lin, M., Inaba, S., Li, C., et al. (2004). Berberine is a novel cholesterol-lowering drug working through a unique mechanism distinct from statins. Nat. Med. 10, 1344–1351. doi:10.1038/nm1135
Kowalczyk, E., Krzesiński, P., Kura, M., Niedworok, J., Kowalski, J., and Błaszczyk, J. (2006). Pharmacological effects of flavonoids from Scutellaria baicalensis. Przegląd Lek. 63, 95–96.
Kumar, A. K., Sharma, S., Shah, E., and Patel, A. (2018). Technical assessment of natural deep eutectic solvent (NADES) mediated biorefinery process: A case study. J. Mol. Liq. 260, 313–322. doi:10.1016/j.molliq.2018.03.107
Lanjekar, K. J., and Rathod, V. K. (2021). Application of ultrasound and natural deep eutectic solvent for the extraction of glycyrrhizic acid from Glycyrrhiza glabra: Optimization and kinetic evaluation. Ind. Eng. Chem. Res. 60, 9532–9538. doi:10.1021/acs.iecr.1c00862
Li, C., Lin, G., and Zuo, Z. (2011). Pharmacological effects and pharmacokinetics properties of Radix Scutellariae and its bioactive flavones. Biopharm. Drug Dispos. 32, 427–445. doi:10.1002/bdd.771
Li, J., Ding, L., Song, B., Xiao, X., Qi, M., Yang, Q., et al. (2016). Emodin improves lipid and glucose metabolism in high fat diet-induced obese mice through regulating SREBP pathway. Eur. J. Pharmacol. 770, 99–109. doi:10.1016/j.ejphar.2015.11.045
Li, Y., Hsieh, Y., Pan, Z., Zhang, L., Yu, W., Wang, B., et al. (2020). Extraction of alkaloids from coptidis rhizoma via betaine-based deep eutectic solvents. ChemistrySelect 5, 4973–4978. doi:10.1002/slct.202000865
Liao, Y., Liang, F., Liu, H., Zheng, Y., Li, P., Peng, W., et al. (2018). Safflower yellow extract inhibits thrombus formation in mouse brain arteriole and exerts protective effects against hemorheology disorders in a rat model of blood stasis syndrome. Biotechnol. Biotechnol. Equip. 32, 487–497. doi:10.1080/13102818.2018.1429310
Liu, Y., Brent Friesen, J., McAlpine, J. B., Lankin, D. C., Chen, S. N., and Pauli, G. F. (2018). Natural deep eutectic solvents: Properties, applications, and perspectives. J. Nat. Prod. 81, 679–690. doi:10.1021/acs.jnatprod.7b00945
Lu, Q. Y., Ma, J. Q., Duan, Y. Y., Sun, Y., Yu, S., Li, B., et al. (2019). Carthamin yellow protects the heart against ischemia/reperfusion injury with reduced reactive oxygen species release and inflammatory response. J. Cardiovasc. Pharmacol. 74, 228–234. doi:10.1097/fjc.0000000000000710
Lu, Y., and Jiang, J. G. (2013). Application of enzymatic method in the extraction and transformation of natural botanical active ingredients. Appl. Biochem. Biotechnol. 169, 923–940. doi:10.1007/s12010-012-0026-9
Ma, C. M., Wei, Y., Wang, Z. G., and Hattori, M. (2009). Triterpenes from Cynomorium songaricium-analysis of HCV protease inhibitory activity, quantification, and content change under the influence of heating. J. Nat. Med. 63, 9–14. doi:10.1007/s11418-008-0267-7
Mano, F., Martins, M., Sá-Nogueira, I., and Borges, J. P. (2017). Production of electrospun fast-dissolving drug delivery systems with therapeutic eutectic systems encapsulated in gelatin. AAPS PharmSciTech 18, 2579–2585. doi:10.1208/s12249-016-0703-z
Martins, M. A. R., Crespo, E. A., Pontes, P. V., Silva, L. P., Bulow, M., Maximo, G. J., et al. (2018). Tunable hydrophobic eutectic solvents based on terpenes and monocarboxylic acids. ACS Sustain. Chem. Eng. 6, 8836–8846. doi:10.1021/acssuschemeng.8b01203
Mbous, Y. P., Hayyan, M., Wong, W. F., Looi, C. Y., and Hashim, M. A. (2017). Unraveling the cytotoxicity and metabolic pathways of binary natural deep eutectic solvent systems. Sci. Rep. 7, 41257. doi:10.1038/srep41257
Oomen, W. W., Begines, P., Mustafa, N. R., Wilson, E. G., Verpoorte, R., and Choi, Y. H. (2020). Natural deep eutectic solvent extraction of flavonoids of Scutellaria baicalensis as a replacement for conventional organic solvents. Molecules 25, 617. doi:10.3390/molecules25030617
Ou-Yang, S. H., Jiang, T., Zhu, L., and Yi, T. (2018). Dioscorea nipponica makino: A systematic review on its ethnobotany, phytochemical and pharmacological profiles. Chem. Cent. J. 12, 57. doi:10.1186/s13065-018-0423-4
Pan, Z., Bo, Y., Liang, Y., Lu, B., Zhan, J., Zhang, J., et al. (2021). Intermolecular interactions in natural deep eutectic solvents and their effects on the ultrasound-assisted extraction of artemisinin from Artemisia annua. Artemisia annua J. Mol. Liq. 326, 115283. doi:10.1016/j.molliq.2021.115283
Pandey, N., and Pandey-Rai, S. (2016). Updates on artemisinin: An insight to mode of actions and strategies for enhanced global production. Protoplasma 253, 15–30. doi:10.1007/s00709-015-0805-6
Park, C. W., Kim, J. Y., Rhee, Y. S., Oh, T. O., Ha, J. M., Choi, N. Y., et al. (2012). Preparation and valuation of a topical solution containing eutectic mixture of itraconazole and phenol. Arch. Pharm. Res. 35, 1935–1943. doi:10.1007/s12272-012-1110-y
Patel, D., Chaudhary, S. A., Parmar, B., and Bhura, N. (2012). Transdermal drug delivery system: A review. Pharma Innovation 1. 66 Available at: www.thepharmajournal.com.
Qiao, J., Ji, D., Sun, S., Zhang, G., Liu, X., Sun, B., et al. (2018). Oral bioavailability and lymphatic transport of pueraria flavone-loaded self-emulsifying drug-delivery systems containing sodium taurocholate in rats. Pharmaceutics 10, 147. doi:10.3390/pharmaceutics10030147
Qu, W., Hakkinen, R., Allen, J., D'Agostino, C., and Abbott, A. P. (2019). Globular and fibrous proteins modified with deep eutectic solvents: Materials for drug delivery. Molecules 24, 3583. doi:10.3390/molecules24193583
Rácz, G., Olasz, Á., Rozsnyik, Z., and Ferenczi, G. (2012). A modern approach to traditional Chinese medicine. IFAC Proc. Vol. 45, 196–200. doi:10.3182/20120829-3-HU-2029.00049
Ribeiro, B. D., Florindo, C., Iff, L. C., Coelho, M. A. Z., and Marrucho, I. M. (2015). Menthol-based eutectic mixtures: Hydrophobic low viscosity solvents. ACS Sustain. Chem. Eng. 3, 2469–2477. doi:10.1021/acssuschemeng.5b00532
Roda, A., Santos, F., Matias, A. A., Paiva, A., and Duarte, A. R. C. (2020). Design and processing of drug delivery formulations of therapeutic deep eutectic systems for tuberculosis. J. Supercrit. Fluids 161, 104826. doi:10.1016/j.supflu.2020.104826
Romero, A., Santos, A., Tojo, J., and Rodriguez, A. (2008). Toxicity and biodegradability of imidazolium ionic liquids. J. Hazard. Mat. 151, 268–273. doi:10.1016/j.jhazmat.2007.10.079
Shi, W., Wang, Y., Li, J., Zhang, H., and Ding, L. (2007). Investigation of ginsenosides in different parts and ages of Panax ginseng. Food Chem. 102, 664–668. doi:10.1016/j.foodchem.2006.05.053
Shikov, A. N., Kosman, V. M., Flissyuk, E. V., Smekhova, I. E., Elameen, A., and Pozharitskaya, O. N. (2020). Natural deep eutectic solvents for the extraction of phenyletanes and phenylpropanoids of Rhodiola rosea L. Molecules 25, 1826. doi:10.3390/molecules25081826
Smith, E. L., Abbott, A. P., and Ryder, K. S. (2014). Deep eutectic solvents (DESs) and their applications. Chem. Rev. 114, 11060–11082. doi:10.1021/cr300162p
Spinozzi, S., Colliva, C., Camborata, C., Roberti, M., Ianni, C., Neri, F., et al. (2014). Berberine and its metabolites: relationship between physicochemical properties and plasma levels after administration to human subjects. J. Nat. Prod. 77 (4), 776–772. doi:10.1021/np400607k
Srikrishna, D., Godugu, C., and Dubey, P. K. (2018). A review on pharmacological properties of coumarins. Mini-Rev. Med. Chem. 18, 113–141. doi:10.2174/1389557516666160801094919
Sut, S., Faggian, M., Baldan, V., Poloniato, G., Castagliuolo, I., Grabnar, I., et al. (2017). Natural deep eutectic solvents (NADES) to enhance berberine absorption: An in vivo pharmacokinetic study. Molecules 22, 1921. doi:10.3390/molecules22111921
Tian, M., Yan, H., and Row, K. H. (2008). Extraction of glycyrrhizic acid and glabridin from licorice. Int. J. Mol. Sci. 9, 571–577. doi:10.3390/ijms9040571
van Osch, D. J. G. P., Dietz, C., Van Spronsen, J., Gallucci, F., Martin, V. S. A., Tuinier, R., et al. (2019). A search for natural hydrophobic deep eutectic solvents based on natural components. ACS Sustain. Chem. Eng. 7, 2933–2942. doi:10.1021/acssuschemeng.8b03520
Walden, P. (1914). Ueber die Molekulargrösse und elektrische Leitfähigkeit einiger geschmolzenen salze. Bull. Acad. Imper Sci. 8, 405–422.
Wan, J. B., Yang, F. Q., Li, S. P., Wang, Y. T., and Cui, X. M. (2006). Chemical characteristics for different parts of Panax notoginseng using pressurized liquid extraction and HPLC-ELSD. J. Pharm. Biomed. Anal. 41, 1596–1601. doi:10.1016/j.jpba.2006.01.058
Wang, H., Ma, X., Cheng, Q., Xi, X., and Zhang, L. (2018). Deep eutectic solvent-based microwave-assisted extraction of baicalin from scutellaria baicalensis georgi. J. Chem. 5, 1–10. doi:10.1155/2018/9579872
Wang, W., Cai, Y., Liu, Y., Zhao, Y., Feng, J., and Liu, C. (2017). Microemulsions based on paeonol-menthol eutectic mixture for enhanced transdermal delivery: Formulation development and in vitro evaluation. Artif. Cells Nanomed. Biotechnol. 45, 1241–1246. doi:10.1080/21691401.2016.1226178
Wang, Y., Hu, Y., Wang, H., Tong, M., and Gong, Y. (2020a). Green and enhanced extraction of coumarins from Cortex Fraxini by ultrasound-assisted deep eutectic solvent extraction. J. Sep. Sci. 43, 3441–3448. doi:10.1002/jssc.202000334
Wang, Y., Peng, B., Zhao, J., Wang, M., and Zhao, L. (2020b). Efficient extraction and determination of prenylflavonol glycosides in Epimedium pubescens Maxim. using deep eutectic solvents. Phytochem. Anal. 31, 375–383. doi:10.1002/pca.2904
Wikene, K. O., Bruzell, E., and Tønnesen, H. H. (2015). Characterization and antimicrobial phototoxicity of curcumin dissolved in natural deep eutectic solvents. Eur. J. Pharm. Sci. 80, 26–32. doi:10.1016/j.ejps.2015.09.013
Wu, Y. C., Wu, P., Li, Y. B., Liu, T. C., Zhang, L., and Zhou, Y. H. (2018). Natural deep eutectic solvents as new green solvents to extract anthraquinones from Rheum palmatum L. Rheum. Palmatum L. RSC Adv. 8, 15069–15077. doi:10.1039/c7ra13581e
Xiao, S., Wang, L., Han, W., Gu, L., Cui, X., and Wang, C. (2022). Novel deep eutectic solvent–hydrogel systems for synergistic transdermal delivery of Chinese herb medicine and local treatments for rheumatoid arthritis. Pharm. Res. 39, 2431–2446. doi:10.1007/s11095-022-03239-5
Xie, Y., Liu, H., Lin, L., Zhao, M., Wu, Y., Zhang, Y., et al. (2019). Application of natural deep eutectic solvents to extract ferulic acid from Ligusticum Chuanxiong Hort with microwave assistance. RSC Adv. 9, 22677–22684. doi:10.1039/c9ra02665g
Xuan, J., Wu, X., Qi, J., and Zhuang, J. (2021). Application of natural deep eutectic solvents in pharmaceutics. Acta Pharm. Sin. 56, 146–157. doi:10.16438/j.0513-4870.2020-1148
Yang, G. Y., Song, J. N., Chang, Y. Q., Wang, L., Zheng, Y. G., Zhang, D., et al. (2021). Natural deep eutectic solvents for the extraction of bioactive steroidal saponins from Dioscoreae Nipponicae Rhizoma. Molecules 26, 2079. doi:10.3390/molecules26072079
Yang, L., Li, L., Hu, H., Wan, J., and Li, P. (2019a). Natural deep eutectic solvents for simultaneous extraction of multi-bioactive components from Jinqi Jiangtang preparations. Pharmaceutics 11, 18. doi:10.3390/pharmaceutics11010018
Yang, X. J., Zhang, P., Lv, W., Zhou, T., Li, P., and Zhao, M. (2019b). Aggregation behavior of imidazolium-based amino acid ionic liquid surfactants in aqueous solution: The effect of amino acid counterions. J. Surfactants Deterg. 22, 515–523. doi:10.1002/jsde.12270
Yong, C. S., Jung, S. H., Rhee, J. D., Choi, H. G., Lee, B. J., Kim, D. C., et al. (2003). Improved solubility and in vitro dissolution of Ibuprofen from poloxamer gel using eutectic mixture with menthol. Drug Deliv. 10, 179–183. doi:10.1080/713840406
Yu, J. B., Zhao, Z. X., Peng, R., Pan, L. B., Fu, J., Ma, S. R., et al. (2019). Gut microbiota-based pharmacokinetics and the antidepressant mechanism of paeoniflorin. Front. Pharmacol. 10, 268–279. doi:10.3389/fphar.2019.00268
Zang, Y. Y., Yang, X., Chen, Z. G., and Wu, T. (2020). One-pot preparation of quercetin using natural deep eutectic solvents. Process Biochem. 89, 193–198. doi:10.1016/j.procbio.2019.10.019
Zeng, Y. J., Xu, P., Yang, H. R., Zong, M. H., and Lou, W. Y. (2018). Purification of anthocyanins from saskatoon berries and their microencapsulation in deep eutectic solvents. LWT 95, 316–325. doi:10.1016/j.lwt.2018.04.087
Zhang, J., Zhang, J., Wang, S., and Yi, T. (2018). Development of an oral compound pickering emulsion composed of nanocrystals of poorly soluble ingredient and volatile oils from traditional Chinese medicine. Pharmaceutics 10, 170. doi:10.3390/pharmaceutics10040170
Zhang, X., Zhou, Y., and Gu, Y. E. (2019). Tanshinone IIA induces apoptosis of ovarian cancer cells in vitro and in vivo through attenuation of PI3K/AKT/JNK signaling pathways. Oncol. Lett. 17, 1896–1902. doi:10.3892/ol.2018.9744
Zhao, B. Y., Xu, P., Yang, F. X., Wu, H., Zong, M. H., and Lou, W. Y. (2015). Biocompatible deep eutectic solvents based on choline chloride: Characterization and application to the extraction of rutin from Sophora japonica. Sophora Jpn. ACS Sustain. Chem. Eng. 3, 2746–2755. doi:10.1021/acssuschemeng.5b00619
Zheng, N., Shi, Y. L., Ji, Y. X., and Lu, W. (2014). Stability and transformation of astragaloside Ⅳ, Ⅲ and Ⅰ. Cent. South Pharm. 12, 1062. doi:10.7539/j.issn.1672-2981.2014.11.002
Keywords: Chinese herbal medicines, NADES, extraction, green solvent, traditional processing (Paozhi)
Citation: Li M, Rao C, Ye X, Wang M, Yang B, Wang C, Guo L, Xiong Y and Cui X (2023) Applications for natural deep eutectic solvents in Chinese herbal medicines. Front. Pharmacol. 13:1104096. doi: 10.3389/fphar.2022.1104096
Received: 21 November 2022; Accepted: 28 December 2022;
Published: 09 January 2023.
Edited by:
Vincent Kam Wai Wong, Macau University of Science and Technology, Macao SAR, ChinaReviewed by:
Zhijian Tan, Institute of Bast Fiber Crops (CAAS), ChinaFilipa Santos, New University of Lisboa, Portugal
Copyright © 2023 Li, Rao, Ye, Wang, Yang, Wang, Guo, Xiong and Cui. This is an open-access article distributed under the terms of the Creative Commons Attribution License (CC BY). The use, distribution or reproduction in other forums is permitted, provided the original author(s) and the copyright owner(s) are credited and that the original publication in this journal is cited, in accordance with accepted academic practice. No use, distribution or reproduction is permitted which does not comply with these terms.
*Correspondence: Yin Xiong, eWhzaXVuZ0AxNjMuY29t; Xiuming Cui, c2FucWkzN0B2aXAuc2luYS5jb20=