- 1Division of Clinical Toxicology and Poison Control Centre Munich, Department of Internal Medicine II, TUM School of Medicine, Technical University of Munich, Munich, Germany
- 2Bundeswehr Institute of Pharmacology and Toxicology, Munich, Germany
A 63-year-old male was admitted to a district hospital after ingesting ethanol and pirimiphos-methyl (PM) with suicidal intentions. History included alcoholic cirrhosis with alcoholism, adiposity, diabetes with cerebral microangiopathy, chronic renal insufficiency, heparin-induced thrombocytopenia, and status post necrotizing fasciitis. Emergency medical service reported an alert patient without signs of cholinergic crisis; activated charcoal and atropine were administered. Upon hospital arrival, he received fluid resuscitation, activated charcoal, and atropine. He was transferred to a toxicology unit the next day. On admission, he had no cholinergic signs (dry mucous membranes, warm skin, and mydriatic pupils) requiring small atropine doses (0.5 mg per hour). Four hours after admission, he developed bradycardia and respiratory distress, necessitating intubation. He received atropine by continuous infusion for 7 days (248 mg total) and obidoxime (bolus and continuous infusion). PM, pirimiphos-methyl-oxon (PMO), and phosphorylated tyrosine (Tyr) adducts derived from human serum albumin were analyzed in vivo. Cholinesterase status (acetylcholinesterase (AChE), butyrylcholinesterase (BChE), inhibitory activity of patient plasma and reactivatability, and phosphorylated BChE-derived nonapeptides) was measured in vivo. Obidoxime and atropine were monitored. PM and PMO were detectable, PM with maximum concentration ∼24 h post admission (p.a.) and PMO at ∼18 h p.a. Tyr adducts were detectable. AChE in vivo was suppressed on admission, increased continuously after starting obidoxime, and reached maximum activity after ∼30 h. AChE in vivo and reactivatability remained at the same level until the end of monitoring. BChE was already suppressed on admission; termination of the antidote treatment was possible after BChE had recovered to 1/5th of its normal value and extubation was possible after BChE had recovered to 2/5th. While a substantial part of BChE was already aged on admission, aging continued peaking at ∼24 h p.a. After initiating obidoxime treatment, plasma levels increased until obidoxime plasma levels reached a steady state. On admission, plasma atropine level was low; it increased with the start of the continuous infusion. Afterward, the level dropped to a steady state. The clinical course was characterized by bouts of pneumonia, necessitating re-intubation and prolonged ventilation, sepsis, delirium, and a peripheral neuropathy. After psychiatric evaluation, the patient was discharged to a neurological rehabilitation facility after 77 days of hospital care.
Introduction
Pirimiphos-methyl (PM) is an organophosphate (OP) which is used as an insecticide and marketed as Actellic® 50 by Syngenta (Syngenta. https, 2021). At the beginning of the millennium, OPs and other pesticides were responsible for 250,000–370,000 deaths worldwide per year due to intentional ingestions (Gunnell and Eddleston, 2003; Gunnell et al., 2007a). However, to the best of our knowledge, a severe case of PM poisoning with corresponding diagnostic investigations has not been published yet. Nowadays, due to stricter regulations, the death toll has decreased to approximately 100,000 deaths per year worldwide (Gunnell et al., 2007b; Mew et al., 2017). In 2015, a patient with severe PM intoxication was treated in a specialized toxicology unit at a tertiary care center of a university hospital in Germany. The case is described in the following.
As is typical for other OPs, the toxicity of PM is due to the phosphorylation of the enzyme acetylcholinesterase (AChE) which is inhibited by the OP (John et al., 2020). Phosphorylation in organophosphates describes the covalent binding of the phosphoryl moiety of the OP to any reactive center, e.g., at the side chains of certain amino acids, thus forming adducts (John et al., 2020). Similar to AChE, the circulating enzyme butyrylcholinesterase (BChE)—also known as pseudo-cholinesterase–is also a target of phosphorylation and inhibition (John et al., 2020). Prior to phosphorylation, the thio-pesticide PM (containing a P=S double bond) is transformed into its oxon-variant (containing a P=O double bond). In vivo, PM undergoes cytochrome P450-mediated desulfuration via cytochrome P450, yielding pirimiphos-methyl-oxon (PMO, Figure 1). This poison belongs to the class of oxono-pesticides which phosphorylate the serine residues of the active site of AChE (John et al., 2020). As a result, acetylcholine accumulates in the synaptic cleft of sympathetic and parasympathetic preganglionic synapses, central synapses, parasympathetic postganglionic synapses, and skeletal muscle afferent nerve endings. Accordingly, it stimulates muscarinic and nicotinic receptors causing a cholinergic crisis. For therapeutic intervention, phosphorylated AChE can be reactivated by oxime antidotes (e.g., obidoxime or pralidoxime) that cleave the phosphoryl moiety and restore the functional enzyme (John et al., 2020). Reactivation will be successful as long as the bound phosphoryl moiety has not already been “aged.” Aging is a chemical process of hydrolysis of at least one alkoxy group of the serine-bound phosphoryl moiety. Such aged adducts cannot be reactivated by oximes anymore, leaving the enzymes irreversibly inhibited (John et al., 2020). For biomedical analysis, adducts of BChE are analyzed, documenting exposure to OP (John et al., 2010a; John et al., 2020; John and Thiermann, 2021).
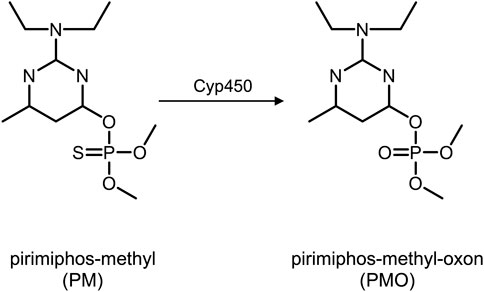
FIGURE 1. Pirimiphos-methyl (left) and pirimiphos-methyl-oxon (right) after metabolization via Cytochrome P450. Abbreviations: PM, pirimiphos-methyl; PMO, pirimiphos-methyl-oxon; Cyp450, Cytochrome P450.
In addition, adduction of other serum proteins, especially of human serum albumin (HSA), is observed after OP poisoning (von der Wellen et al., 2018). To document poison incorporation, these adducts are proteolyzed with diverse enzymes yielding biomarker adducts, e.g., phosphorylated tyrosine (Tyr) (John et al., 2020; von der Wellen et al., 2018). Adducts of PM should yield a biomarker consisting of Tyr adducted with a dimethyl thiophosphate moiety (Tyr-DMTP), whereas PMO should yield Tyr adducted with a dimethyl phosphate moiety (Tyr-DMP). Such Tyr adducts can be monitored by liquid chromatography-electrospray ionization tandem-mass spectrometry (LC-ESI MS/MS), allowing their selective and sensitive detection.
Materials and methods
Patient data
Patient data were extracted from the hospital charts of the district hospital in Dachau, Germany, the emergency medical service (EMS) and the toxicological intensive care unit (ICU) of the Klinikum rechts der Isar, Technical University of Munich, Germany. Patient blood samples were analyzed at the respective clinical laboratories, and further samples were transferred to the Bundeswehr Institute of Pharmacology and Toxicology for specific analysis of the pesticides, cholinesterase status, and antidote concentrations in plasma. Patient consent for publication was obtained.
Results from the respective clinical laboratories were available to the treating physicians with the daily lab results. Data from the Bundeswehr were made available to the treating physicians as soon as measurements were completed.
Unfortunately, it was not possible to determine the exact time when the patient ingested the PM. Therefore, we chose either the admission to the tertiary care center or the time of the first blood sample as references for time, since these were the times which could be clearly determined.
Cholinesterase and standard laboratory tests
BChE and standard laboratory tests (e.g., sodium, creatinine, leucocytes, C-reactive protein, pH, and lactate) were performed in the respective clinical laboratories.
Sample storage and transfer
Patient EDTA blood samples were taken repeatedly, 0.2 ml was bedside diluted with 3.8 ml ice-cold distilled water, and plasma was collected after centrifugation. Whole blood dilution and plasma were immediately frozen (−20°C) until analysis. Blood was taken on admission and three times per day on the following days at 8 a.m., 4 p.m., and midnight.
Detection of pesticides
PM and PMO were monitored by LC-ESI MS/MS in a similar way as described before, for analysis of the pesticides dimethoate and omethoate (John et al., 2010a).
Detection of phosphorylated tyrosine adducts
Phosphorylated tyrosine (Tyr) adducts derived from modified plasma proteins predominantly from HSA were analyzed by micro liquid chromatography-electrospray ionization high-resolution tandem-mass spectrometry (µLC-ESI MS/HR MS) as described before (von der Wellen et al., 2018).
Detection of BChE adducts
For analysis of phosphorylated BChE-derived nonapeptides, a µLC-ESI MS/MS method was applied as described before (John et al., 2015).
Determination of cholinesterase status
AChE and BChE activity were determined with an UV-VIS spectrophotometer via modified Ellman assay as described before (Worek et al., 1999a), and the AChE and BChE activity, reactivatability of AChE and inhibitory activity of plasma were also measured (Worek et al., 2005; Worek et al., 2010).
Determination of antidotes obidoxime and atropine
Obidoxime was measured via reversed-phase ion-pair chromatography-diode array detection (RPIPC-DAD) as described recently (Kranawetvogl et al., 2020). Atropine was monitored by LC-ESI MS/MS in multiple reaction monitoring mode (John et al., 2010b).
Clinical case
A 63-year-old male was admitted to a district hospital after ingesting 700 ml vodka and two swallows of PM solution (500 g PM/L, Actellic® 50, Syngenta; approximately 25 g of PM) with suicidal intention.
His medical history included Child–Pugh Class B alcoholic liver cirrhosis with active alcoholism, esophageal varices grade II, status post epileptic seizure during alcohol withdrawal, adiposity, diabetes type II with cerebral microangiopathy and chronic renal insufficiency, necrotizing fasciitis of the left leg, and heparin-induced thrombocytopenia.
Upon arrival of the EMS, he was fully alert with no evident signs of cholinergic excess. Preclinically, EMS administered 50 g of oral activated charcoal and 0.5 mg atropine intravenously. He had one episode of vomiting. Arrival of the EMS on scene (6 p.m.) was approximately 3–4 h after ingestion (approximately 2–3 p.m.), and they brought the patient to the intensive care unit (ICU) of the district hospital approximately 4–5 h post ingestion of PM (transport time approximately 1 h).
Upon arrival at the district hospital as primary care center at 7 p.m., he received fluid resuscitation, another 25 g of activated charcoal, and further atropine (0.5 mg every 30 min) and was admitted to the local ICU. His blood ethanol level was 2 g/L. Arterial blood gas analysis showed an acidosis with a pH of 7.19 and highly elevated lactate of 25 mmol/L. He received sodium bicarbonate which resolved the acidosis, and the lactate was cleared with fluid resuscitation. On the next day, he was transferred to the ICU of our toxicology unit as tertiary care center and arrived there at 2:30 p.m.
Upon arrival at our ICU, the patient had no cholinergic signs (he presented with dry mucous membranes, warm skin, and mydriatic pupils), requiring only small doses of atropine. His initial vital parameters were stable (heart rate, 105/min; blood pressure, 155/75 mmHg; SpO2, 99% with 4L/min O2 via nasal cannula). Four hours after admission, he developed bradycardia and respiratory distress and was intubated. He received atropine by continuous infusion at a maximum dose of 10 mg/h for 7 days and obidoxime 250 mg as initial bolus and 750 mg/24 h as a continuous infusion for 7 days. Initially, epinephrine was used to stabilize the patient with a maximum dose of 500 μg/h; due to bradycardia, he repeatedly received dobutamine as continuous infusion within the following days (Table 1).
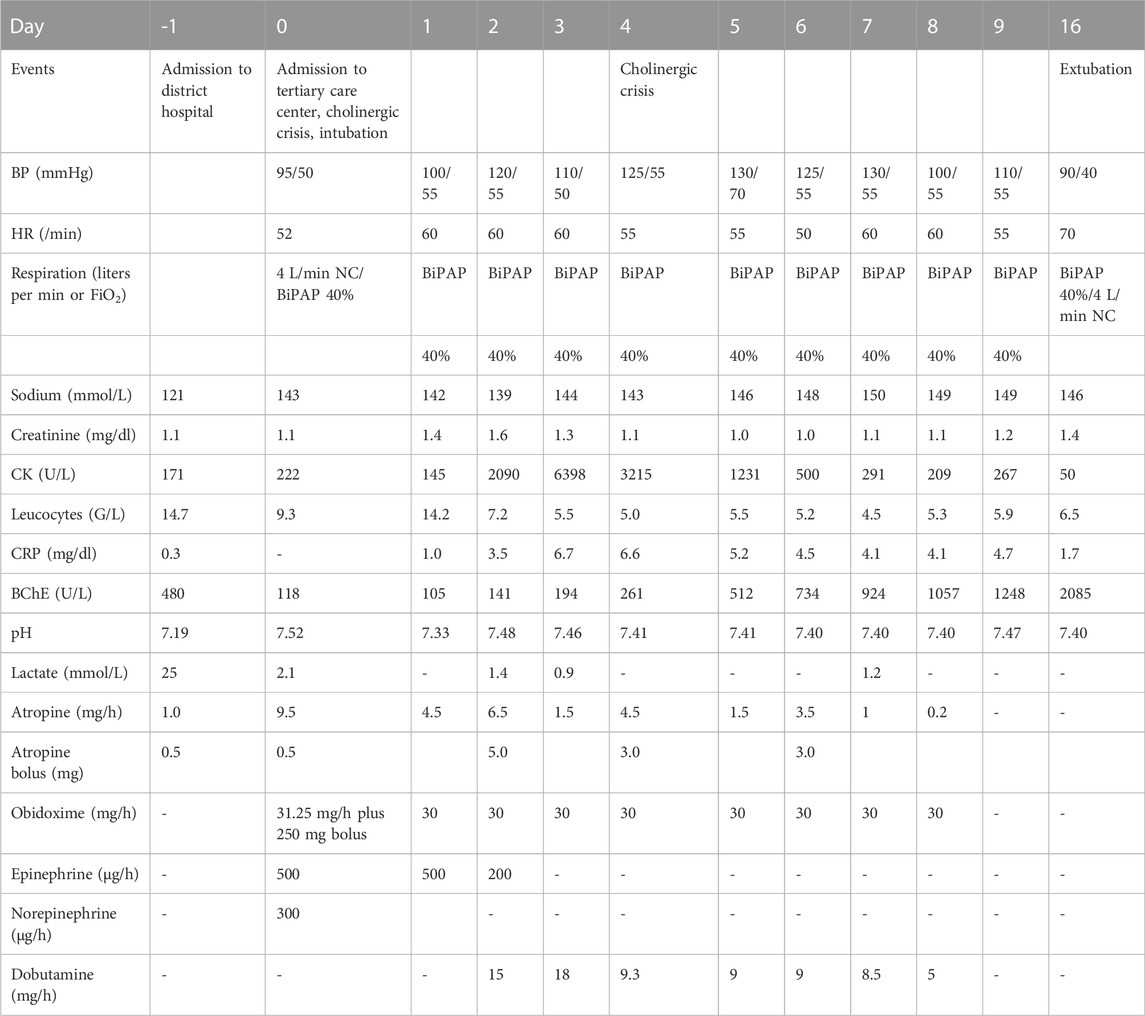
TABLE 1. Clinical course of patient from admission to primary care center until extubation, showing the clinical course of the intoxication. Blood pressure and heart rate are given as the lowest daily value, medications as their highest infusion rate on the respective day, O2 is displayed in liters per minute, and FiO2 in percent (also highest daily flow rates/percentages). Laboratory results from the respective clinical laboratories. Cumulative atropine = 248 mg (12 mg as bolus, 236 mg as continuous infusion), BP = blood pressure, HR = heart rate, CRP = C-reactive protein, CK = creatinine kinase, BChE = butyrylcholinesterase, NC = nasal cannula, BiPAP = bilevel positive airway pressure ventilation, FiO2 = fraction of inspired oxygen, and G = giga. Reference levels: sodium = 135–145 mmol/L; creatinine = 0.7–1. mg/dl; CK<174U/L; leucocytes = 4.0-9-0G/L; CRP<0.5 mg/dl; BChE = 5320–12920U/L; pH = 7.35–7.45; lactate<2.4 mmol/L.
The subsequent clinical course was complicated by bouts of pneumonia, necessitating prolonged ventilatory support. Extubation was possible on day 16 after admission to our ICU; however, re-intubation and tracheotomy were necessary. The further course was complicated by severe delirium and catheter-associated sepsis with a septic shock, which required mechanical ventilation for another 17 days (week 4 and 5).
Due to profound peripheral neuropathy, the patient was discharged—after psychiatric evaluation—to a neurological rehabilitation facility after 77 days of cumulated ICU and hospital care.
Results
Detection of pesticides
PM was detectable in plasma immediately and showed its maximum concentration ∼18 h post admission to our ICU. It was still detectable 160 h post admission.
The highest peak area was at ∼10 h after first sample, half of the highest peak area was reached at ∼90 h after first sample. Therefore, the approximated half-life in this overdose was calculated at 80 h (Figure 2A). PMO was also detectable on admission and peaked at ∼18 h post admission. It also was still detectable at 160 h post admission (Figure 2B).
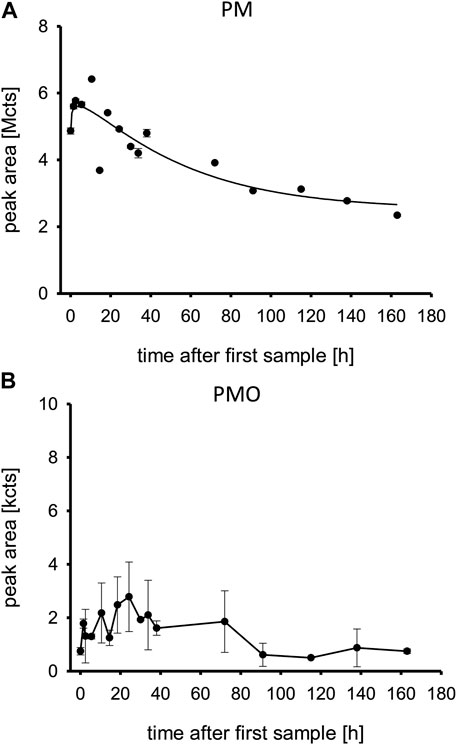
FIGURE 2. (A,B) Pirimiphos-methyl and pirimiphos-methyl-oxon in patient plasma. Time is shown in hours after first blood sample, which was approximately upon admission to the tertiary care center ICU. Abbreviations: PM, pirimiphos-methyl; PMO, pirimiphos-methyl-oxon; Mcts, mega-counts; kcts, kilo-counts.
Detection of Tyr adducts
The relative concentration-time profiles of the Tyr-DMP and Tyr-DMTP are displayed in Figure 3. While Tyr-DMP peaked early and decreased over time, Tyr-DMTP remained at a steady state in very low relative concentrations during the first 160 h post admission.
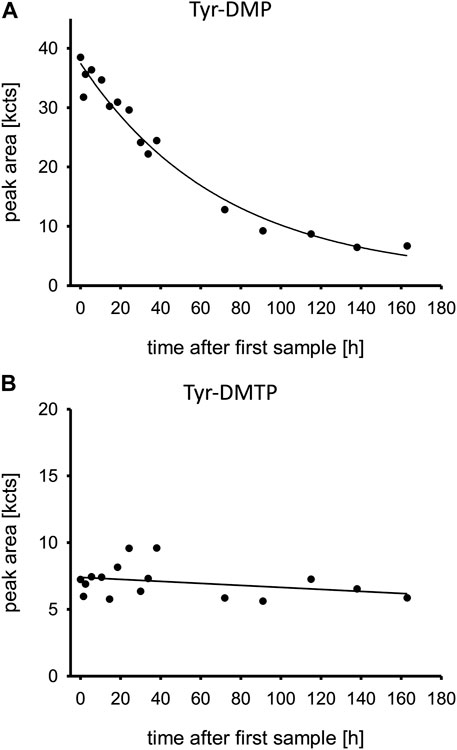
FIGURE 3. (A,B) Oxono-tyrosine and thiono-tyrosine adducts to human serum albumin. Time is shown in hours after first blood sample, which was approximately upon admission to the tertiary care center ICU. Abbreviations: Tyr-DMP, tyrosine dimethyl thiophosphate moiety; Tyr-DMTP, tyrosine dimethyl phosphate moiety; kcts, kilo-counts.
Cholinesterase
BChE was already suppressed at admission in the primary care center; the BChE measured by the clinical laboratory is shown in Table 1. After having passed its nadir on day 1 after admission to the tertiary care center, termination of the antidote treatment was possible after BChE had recovered to 1/5th of its physiological value. Extubation was possible after BChE had recovered to 2/5th of its normal value (Table 1).
Determination of cholinesterase status
AChE in vivo and its reactivatability are shown in Figure 4A, including the inhibitory activity of the patient’s plasma (Figure 4C). AChE in vivo was suppressed at admission, increased continuously after start of obidoxime therapy, and reached its maximum activity after ∼30 h. AChE in vivo and reactivatability remained at the same level until the end of monitoring of the enzyme activities.
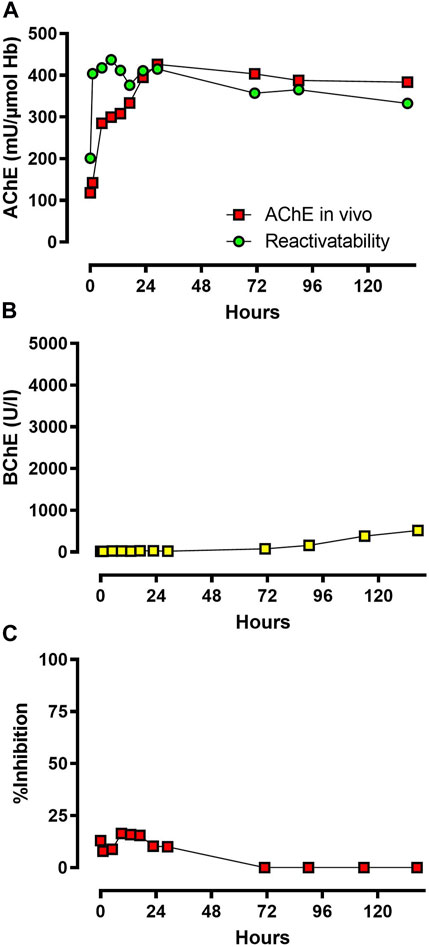
FIGURE 4. (A,B) and (C): AChE in vivo and reactivatability, BChE in vivo, and inhibitory activity of patient plasma. Time is shown in hours after admission to the toxicological unit. Abbreviations: AChE, acetylcholinesterase; BChE, butyrylcholinesterase.
BChE activity measured by UV-VIS spectrophotometer via modified Ellman assay was markedly suppressed at admission and increased slowly after ∼70 h, which correlates with the gradual decrease of inhibitory activity (Figure 4B).
Detection of BChE adduct
The serum levels of the aged BChE adduct containing a bound monomethyl phosphate moiety (BChE-MMP) are shown in Figure 5. While a substantial part of the BChE was already aged at admission, the aging continued with a peak at 24 h post admission and slowly declined afterward. Surprisingly, the non-aged adduct was not detected.
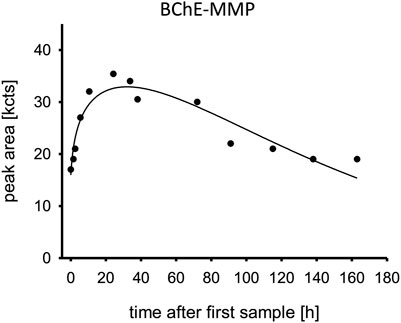
FIGURE 5. Aged butylcholinesterase. Time is shown in hours after first blood sample, which was approximately upon admission to the tertiary care center ICU. Abbreviation: BChE-MMP, butylcholinesterase monomethyl phosphate moiety; kcts, kilo-counts.
Determination of antidotes obidoxime and atropine
Obidoxime levels are displayed in Figure 6A. After the obidoxime bolus of 250 mg, it further increased under continuous infusion until it reached a steady state. This continued until the obidoxime infusion was stopped. On admission, plasma atropine levels were low, corresponding with the applied low doses of 0.5 mg every 30 min as shown in Figure 6B. The plasma level increased with the start of the continuous infusions of 9.5 mg/h. Afterward, the level dropped to a steady state until atropine infusions were discontinued.
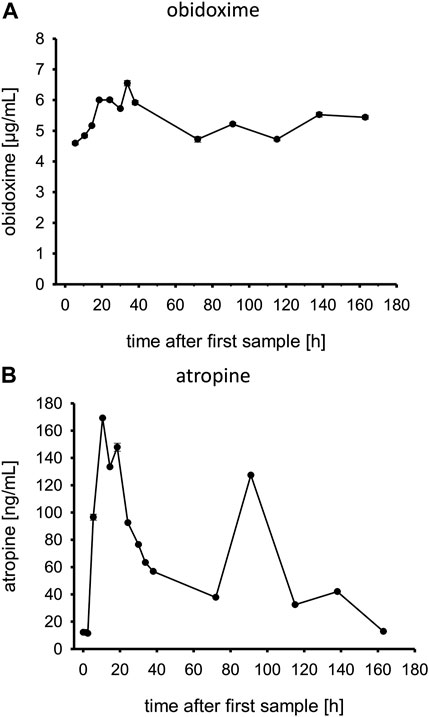
FIGURE 6. (A,B) Obidoxime and atropine levels in patient plasma. Time is shown in hours after first blood sample, which was approximately upon admission to the tertiary care center ICU.
Discussion
Initial presentation and onset of symptoms
Upon admission to the primary care center and upon transfer to the tertiary care center, the patient did not shown a full cholinergic syndrome, though treatment with atropine was necessary with initially rather low doses compared to the usually applied doses for severe organophosphate poisoning. Treatment with obidoxime was not initiated by the district hospital due to the lack of cholinergic signs and unavailability of this antidote. According to the clinical signs, the development of severe cholinergic crisis took more than 27 h after ingestion of PM, indicating a slow decrease of AChE activity. On admission, AChE activity was markedly but not completely inhibited, which fits the clinical picture. Obidoxime was chosen as an antidote in combination with atropine since it has been shown to be safe and a potent reactivator of OP-inhibited AChE in other cases of OP poisoning (Eyer et al., 2009).
Antidote therapy: Obidoxime and atropine
An obidoxime bolus and continuous infusion over 7 days resulted in a rapid increase of AChE activity which remained at a sufficiently high level. We applied obidoxime in the standard dosing regimen. The plasma obidoxime levels in steady state reached ∼5 μg/ml (approx. 15 μmol/L) which is the recommended level (Figure 6A) (Eyer et al., 2009).
Atropine plasma levels correlated well with the given atropine doses. At ∼90 h post admission to the tertiary care center, the patient developed another episode of cholinergic syndrome, requiring increased atropine infusion rates and an atropine bolus (Table 1) which can also be seen in the plasma levels (Figure 6B). Ultimately, it remains unclear why the patient had renewed cholinergic symptoms after about 90 h, which resulted in a temporary increase in dosage of atropine. In any case, neither the cholinesterase status (AChE; BChE) nor the concentrations of PM or PMO at that time suggest that there was a significant increase in the load of OP (e.g., late reabsorption) that justified a renewed more intensive therapy with atropine. The decision to adjust the atropine dosage was made clinically, without awareness of the analytical results from, e.g., PM or PMO.
In general, the patient required prolonged atropine application with high continuous infusion rates and repeated boluses in high doses. The cumulative applied atropine was 248 mg (12 mg as bolus, 236 mg as continuous infusion), which is unusually high for an OP intoxication and could be a noteworthy peculiarity in PM poisoning. In OP poisoning, atropine is dosed according to clinical symptoms. Those high doses usually show no relevant side effects, since all atropine is required to antagonize the muscarinergic effects of the OP. Our patient never experienced episodes of over-atropinization, such as tachycardia or other anticholinergic symptoms, despite those high doses.
AChE activity was analyzed off-site, and the results were reported to the clinic with some delay. Therefore, the discontinuation of obidoxime was decided by the clinical signs. Determination of BChE activity is generally used in hospitals since assays for AChE activity are usually not available. In these cases, an increase of BChE activity indicates the absence of an inhibitor in blood which is one parameter for deciding to stop oxime therapy.
As expected, obidoxime had no effect on BChE activity, which increased only after inhibitory activity was absent due to de novo synthesis. Spontaneous reactivation of dimethyl-OP-inhibited BChE is possible at a rather low rate. However, in this case, BChE activity increased (by de novo synthesis and spontaneous reactivation) only after loss of inhibitory activity, i.e., presence of PMO, starting on day 3 (Worek et al., 1999b). The Tyr-DMP concentration decreased in the days post admission, the patient plasma still had inhibitory activity (remaining pesticide), and aging of the BChE continued despite treatment with obidoxime. Even though BChE showed significant aging, reactivatability of AChE was nevertheless rather high, suggesting that BChE has a limited clinical relevance.
Pesticides
The high concentration of PM in comparison to PMO documents its high resistance in the circulation and a notably low biotransformation process to PMO, potentially caused by impaired liver activity due to the patient’s underlying Child–Pugh Class B alcoholic liver cirrhosis. The low relative concentrations of PMO compared to PM document that most of this PM biotransformation product has been either eliminated by urine or reacted with diverse endogenous molecules including, e.g., cholinesterases and HSA.
Our measurements documented that although the patient did not yet show severe signs of cholinergic crisis, he already had high plasma pesticide levels (Figure 2A), and a large amount of the BChE had already aged irreversibly (Figure 5). The AChE was initially suppressed, but it still showed reactivatability and rapidly increased after treatment with obidoxime was started. AChE reached its maximum 30 h post treatment initiation. Consequently, the patient’s clinical condition initially deteriorated, and he required mechanical ventilation and circulatory support with catecholamines. Our laboratory measurements cannot sufficiently explain the renewed cholinergic crisis at day 4.
Tyr and BChE adducts
The quite rapid decrease of Tyr-DMP was unexpected as phosphorylated Tyr from HSA was thought to be stable for some weeks as determined by the natural protein turn-over (John et al., 2020). Accordingly, the decrease of this DMP adduct might have been due to spontaneous hydrolysis (cleavage of the entire phosphoryl moiety) or any process of enzymatic dephosphorylation not unraveled so far. The low concentrations of Tyr-DMTP illustrate a low reactivity with HSA despite the high PM concentrations. In addition, the rapid decrease of Tyr-DMP may also be caused by pathological renal loss of HSA in general.
The rapid decrease of the BChE-MMP concentration is explained by the natural protein turn-over (John et al., 2020) and the spontaneous reactivation yielding functional BChE (Worek et al., 2005; Worek et al., 2010). The kinetics of aging of AChE and BChE are different, leading to different levels of aged AChE and BChE (Worek et al., 1999b).
Clinical course
Even though the laboratory findings improved with treatment, the patient’s condition remained critical for 4 days, requiring high doses of atropine and only improved slowly afterward. He had a complicated further clinical course, also partly due to his comorbidities. His initial pneumonia was due to aspiration and resolved slowly despite broad spectrum antibiotic treatment and lung protective ventilation and required tracheotomy. Nevertheless, he may have benefitted from an earlier application of obidoxime, although there is still a controversial debate about the clinical impact of oxime therapy alongside best supportive critical care, including atropine (Eddleston, 2022).
Although the acute toxicity of organophosphates is largely explainable by AChE inhibition and the lasting effects of poisoning by direct excitotoxicity or indirect consequences of the cholinergic syndrome, effects at lower levels of exposure could not be predicted from this mechanism. Carter et al. found significant adduction of partially characterized protein targets in both rat brain and thymus by azamethiphos, chlorfenvinphos, chlorpyrifos-oxon, diazinon-oxon, dichlorvos, and malaoxon, in vitro and PM in vivo (Carter et al., 2007).
Limitations
As with all case reports, this case shows data from only one patient, so it is difficult to draw general conclusions from it.
Laboratory findings by the Bundeswehr Institute of Pharmacology and Toxicology were made available to the treating physicians with some delay (inherent to the complex analytical process); this could have influenced their clinical decision-making if they had been available earlier.
Conclusion
We describe a case of severe PM intoxication with a complicated and prolonged clinical course. Our patient required rather high doses of atropine. Despite delayed obidoxime treatment, AChE activity recovered to large extent. The support by a specialized laboratory allowed the determination of the cholinesterase status (AChE and BChE activity, reactivatability, and inhibitory activity of the patient’s plasma) and the analysis of BChE and albumin adducts to verify the intake of PM and its conversion into PMO. While these measurements were not available in time for treatment decisions, which were made clinically, they give a comprehensive insight into a rather rare poisoning. For optimal care, patients with organophosphate poisoning should be admitted to a hospital specializing in clinical toxicology.
Our clinical and analytical finding in this rare case of PM poisoning suggests the following:
- Patients with PM poisoning should be observed in an ICU for at least 48 h because a delayed onset of cholinergic crisis is possible with this agent
- PM has an in vivo half-life of ∼80 h in overdose
- AChE could have been reactivated by antidote therapy in this case, even though the antidote was not given earlier than 24 h post ingestion
Data availability statement
The datasets for this article are not publicly available due to concerns regarding participant/patient anonymity. Requests to access the datasets should be directed to the corresponding author.
Ethics statement
The patients/participants provided their written informed consent to participate in this study. Patient consent for publication was obtained.
Author contributions
TZ wrote the manuscript and treated the patient, CR contributed to the manuscript and treated the patient, JW performed laboratory analysis, DH performed laboratory analysis, HJ contributed to the manuscript and performed laboratory analysis, FW contributed to the manuscript and performed laboratory analysis, and FE contributed to the manuscript and treated the patient.
Conflict of interest
The authors declare that the research was conducted in the absence of any commercial or financial relationships that could be construed as a potential conflict of interest.
Publisher’s note
All claims expressed in this article are solely those of the authors and do not necessarily represent those of their affiliated organizations, or those of the publisher, the editors, and the reviewers. Any product that may be evaluated in this article, or claim that may be made by its manufacturer, is not guaranteed or endorsed by the publisher.
Abbreviations
AChE, acetylcholinesterase; BChE, butyrylcholinesterase; BChE-MMP, aged BChE adduct containing a bound monomethyl phosphate moiety; EMS, emergency medical service; h, hours; HSA, human serum albumin; ICU, intensive care unit; LC-ESI MS/MS, liquid chromatography-electrospray ionization tandem-mass spectrometry; µLC-ESI MS/HR MS, micro liquid chromatography-electrospray ionization high-resolution tandem-mass spectrometry; mg, milligram, milligrams; OP, organophosphate; p.a., post admission; PM, pirimiphos-methyl; PMO, pirimiphos-methyl-oxon; RPIPC-DAD, reversed-phase ion-pair chromatography-diode array detection; Tyr, tyrosine; Tyr-DMP, tyrosine adducted with a dimethyl phosphate moiety; Tyr-DMTP, tyrosine adducted with a dimethyl thiophosphate moiety.
References
Carter, W. G., Tarhoni, M., Rathbone, A. J., and Ray, D. E. (2007). Differential protein adduction by seven organophosphorus pesticides in both brain and thymus. Hum. Exp. Toxicol. 26 (4), 347–353. doi:10.1177/0960327107074617
Eddleston, M. (2022). CON: Oximes should not be used routinely in organophosphorus insecticide poisoning. Br. J. Clin. Pharmacol. 88, 5070–5073. doi:10.1111/bcp.15217
Eyer, F., Worek, F., Eyer, P., Felgenhauer, N., Haberkorn, M., Zilker, T., et al. (2009). Obidoxime in acute organophosphate poisoning: 1 - clinical effectiveness. Clin. Toxicol. (Phila). 47 (8), 798–806. doi:10.1080/15563650903206828
Gunnell, D., Eddleston, M., Phillips, M. R., and Konradsen, F. (2007). The global distribution of fatal pesticide self-poisoning: Systematic review. BMC Public Health 7, 357. doi:10.1186/1471-2458-7-357
Gunnell, D., and Eddleston, M. (2003). Suicide by intentional ingestion of pesticides: A continuing tragedy in developing countries. Int. J. Epidemiol. 32 (6), 902–909. doi:10.1093/ije/dyg307
Gunnell, D., Fernando, R., Hewagama, M., Priyangika, W. D., Konradsen, F., and Eddleston, M. (2007). The impact of pesticide regulations on suicide in Sri Lanka. Int. J. Epidemiol. 36 (6), 1235–1242. doi:10.1093/ije/dym164
John, H., Balszuweit, F., Steinritz, D., Kehe, K., Worek, F., and Thiermann, H. (2020). “Toxicokinetic aspects of nerve agents and vesicants,” in Handbook of toxicology of chemical warfare agents”. 3rd ed (San Diego: Elsevier Inc./Academic Press).
John, H., Binder, T., Hochstetter, H., and Thiermann, H. (2010). LC-ESI MS/MS quantification of atropine and six other antimuscarinic tropane alkaloids in plasma. Anal. Bioanal. Chem. 396 (2), 751–763. doi:10.1007/s00216-009-3209-7
John, H., Breyer, F., Schmidt, C., Mizaikoff, B., Worek, F., and Thiermann, H. (2015). Small-scale purification of butyrylcholinesterase from human plasma and implementation of a μLC-UV/ESI MS/MS method to detect its organophosphorus adducts. Drug Test. Anal. 7 (10), 947–956. doi:10.1002/dta.1792
John, H., Eddleston, M., Clutton, R. E., Worek, F., and Thiermann, H. (2010). Simultaneous quantification of the organophosphorus pesticides dimethoate and omethoate in porcine plasma and urine by LC-ESI-MS/MS and flow-injection-ESI-MS/MS. J. Chromatogr. B Anal. Technol. Biomed. Life Sci. 878 (17-18), 1234–1245. doi:10.1016/j.jchromb.2010.01.003
John, H., and Thiermann, H. (2021). Poisoning by organophosphorus nerve agents and pesticides: An overview of the principle strategies and current progress of mass spectrometry-based procedures for verification. J. Mass Spectrom. Adv. Clin. Lab. 19, 20–31. doi:10.1016/j.jmsacl.2021.01.002
Kranawetvogl, T., Steinritz, D., Thiermann, H., and John, H. (2020). A novel high-performance liquid chromatography with diode array detector method for the simultaneous quantification of the enzyme-reactivating oximes obidoxime, pralidoxime, and HI-6 in human plasma. Drug Test. Anal. 12 (7), 938–947. doi:10.1002/dta.2800
Mew, E. J., Padmanathan, P., Konradsen, F., Eddleston, M., Chang, S. S., Phillips, M. R., et al. (2017). The global burden of fatal self-poisoning with pesticides 2006-15: Systematic review. J. Affect Disord. 219, 93–104. doi:10.1016/j.jad.2017.05.002
Syngenta. https://www.syngenta.co.uk/sites/g/files/zhg151/f/media/2021/04/15/actellic_50ec_v16.pdf?token=1618482909last accessed 2022-09-14 [
von der Wellen, J., Winterhalter, P., Siegert, M., Eyer, F., Thiermann, H., and John, H. (2018). A toolbox for microbore liquid chromatography tandem-high-resolution mass spectrometry analysis of albumin-adducts as novel biomarkers of organophosphorus pesticide poisoning. Toxicol. Lett. 292, 46–54. doi:10.1016/j.toxlet.2018.04.025
Worek, F., Aurbek, N., Herkert, N. M., John, H., Eddleston, M., Eyer, P., et al. (2010). Evaluation of medical countermeasures against organophosphorus compounds: The value of experimental data and computer simulations. Chem. Biol. Interact. 187 (1-3), 259–264. doi:10.1016/j.cbi.2009.11.009
Worek, F., Diepold, C., and Eyer, P. (1999). Dimethylphosphoryl-inhibited human cholinesterases: Inhibition, reactivation, and aging kinetics. Arch. Toxicol. 73 (1), 7–14. doi:10.1007/s002040050580
Worek, F., Koller, M., Thiermann, H., and Szinicz, L. (2005). Diagnostic aspects of organophosphate poisoning. Toxicology 214 (3), 182–189. doi:10.1016/j.tox.2005.06.012
Keywords: organophosphate, pesticide, obidoxime, atropine, cholinesterase
Citation: Zellner T, Rabe C, Wellen-Pawlowski Jvd, Hansen D, John H, Worek F and Eyer F (2022) A case report of severe pirimiphos-methyl intoxication: Clinical findings and cholinesterase status. Front. Pharmacol. 13:1102160. doi: 10.3389/fphar.2022.1102160
Received: 18 November 2022; Accepted: 12 December 2022;
Published: 23 December 2022.
Edited by:
Eleonore Fröhlich, Medical University of Graz, AustriaReviewed by:
Rudolph Johnson, Centers for Disease Control and Prevention (CDC), United StatesJiri Kassa, University of Defence, Czechia
Jaroslav Pejchal, University of Defence, Czechia
Dragana Loncar Stojiljkovic, ICVD Dedunje, Serbia
Timothy Marrs, Dr Timothy Marrs, United Kingdom
Copyright © 2022 Zellner, Rabe, Wellen-Pawlowski, Hansen, John, Worek and Eyer. This is an open-access article distributed under the terms of the Creative Commons Attribution License (CC BY). The use, distribution or reproduction in other forums is permitted, provided the original author(s) and the copyright owner(s) are credited and that the original publication in this journal is cited, in accordance with accepted academic practice. No use, distribution or reproduction is permitted which does not comply with these terms.
*Correspondence: Tobias Zellner, tobias.zellner@tum.de