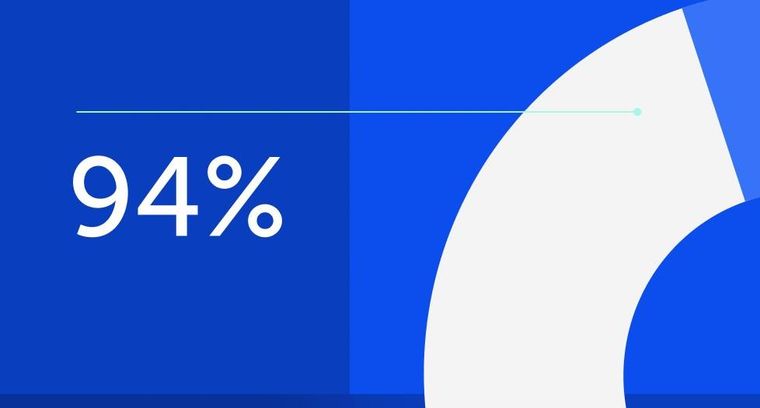
94% of researchers rate our articles as excellent or good
Learn more about the work of our research integrity team to safeguard the quality of each article we publish.
Find out more
ORIGINAL RESEARCH article
Front. Pharmacol., 09 January 2023
Sec. Ethnopharmacology
Volume 13 - 2022 | https://doi.org/10.3389/fphar.2022.1096137
This article is part of the Research TopicTraditional Medicine and Chronic Inflammatory DiseasesView all 16 articles
PM2.5 is an important environmental problem threatening human health at present, which poses serious harm to human body after inhalation. J. cannabifolia is a traditional Chinese medicine which exhibits anti-inflammatory effect. This study aimed to investigate the inhibitory effect of main phenolic acid components of J. cannabifolia on inflammation caused by PM2.5. Effect of PM2.5 on cell activity and apoptosis were determined by MTT, flow cytometry and calcein AM/PI staining. PHBA, PHPAA, and mixture of PHBA and PHPAA of different concentrations were given to RAW264.7 cells pretreated with PM2.5. The effect of drugs on cellular inflammatory factors was detected by ELISA. The expressions of TLRs related signal pathway at protein and gene levels were detected by western blot and qRT-PCR. The results showed that PM2.5 had no effect on cell activity and apoptosis within the determined concentration range. PHBA and PHPAA could markly inhibit the level of IL-1β, IL-6, and TNF-α in RAW264.7 cells. Furthermore, the expressions of TLR2, TLR4, MyD88, IRAK1, TRAF6, TAK1, IKKβ, and NF-κB induced by PM2.5 were markedly inhibited by PHBA and PHPAA at protein and gene levels. This study demonstrated that PHBA and PHPAA could attenuated inflammation caused by PM2.5 through suppressing TLRs related signal pathway.
In recent years, some cities in China have experienced haze weather with particulate matter as the main pollution feature, and the ambient air quality is facing a severe test, which is extremely adverse to the national health and socio-economic development (Lin et al., 2018). PM10 (diameter of inhalable particles which are less than 10 μm) and PM2.5 (diameter of inhalable particles which are less than 2.5 μm and can enter the lung) are the main pollutant (Huang et al., 2014). The particle size of PM2.5 is smaller, resulting in a larger surface area, which makes it easy to be absorbed. At the same time, PM2.5 can stay in the atmosphere for a long time, and can travel a long distance (Han et al., 2015; Ji and Zhao., 2015). Therefore, PM2.5 has a great impact on people’s health and air quality.
The sources of PM2.5 are very complex, mainly including emissions of combustibles, particles generated in chemical processes, human activities, equipment operation, cleaning and cooking (Ji and Zhao., 2015; Li et al., 2016; Zhou et al., 2016). PM2.5 is a major threat to human health and can affect many systems of the body, including the central nervous system, blood system, metabolic immune system, urogenital system, digestive system and skin (Boothe et al., 2014; Park and Wang., 2014; Piao et al., 2018; Geng et al., 2019; Jeong et al., 2019; Shaffer et al., 2019). Therefore, it is very important for human health to develop new drugs to treat PM2.5.
Jacobaea cannabifolia (Less.) E.Wiebe is a plant of Senecio genus, which is a genuine medicinal material in Northeast China. J. cannabifolia has anti-inflammatory, bacteriostatic, anti-virus, immune regulation, anti-tumor, and other effects (Li et al., 2005; Chen et al., 2015). It is clinically used to treat acute and chronic bronchitis, asthmatic bronchitis and acute respiratory tract infection. The main metabolites of the herb are flavonoids, phenolic acids, alkaloids, volatile oils, glycosides, and tannins. P-hydroxybenzoic acid (PHBA) and p-hydroxyphenylacetic acid (PHPAA) (Figure 1) are the main phenolic acids in J. cannabifolia and they have anti-inflammatory and other activities (Zhao et al., 2013; Sun et al., 2014).
This study aimed to explore the effect of PHBA and PHPAA on inflammation induced by PM2.5 and its possible mechanism, to provide an experimental basis for PHBA and PHPAA to become clinical therapeutic drugs for lung injury caused by PM2.5.
PHBA (≥99%) and PHPPA (98%) were provided by Sigma Chemical Co. (St. Louis, MO, United States). PM2.5 particles were provided by Hong-Hai Yu, senior engineer of Huadian Electric Power Research Institute Co., Ltd. Northeast Branch. Mouse tumor necrosis factor-α (TNF-α), interleukin-6 (IL-6) and interleukin-1β (IL-1β) ELISA kits were purchased from Biyuntian Biotechnology Research Institute. TLR4, TLR2, TRAF6, NF-κB, MyD88, IRAK1, TAK1, and IKKβ polyclonal antibody IgG were obtained from Proteintech Group Inc (Chicago, IL, United States).
RAW264.7 murine macrophage cells were purchased from cell bank of Chinese Academy of Science, Shanghai. Cells were maintained in Dulbecco’s modified Eagle’s medium (Hyclone, Logan, UT) supplemented with 5% FBS (Gibco, United States), 1% streptomycin and penicillin (Hyclone) at 37°C in 5% CO2 in a humidified atmosphere incubator.
The cell viability was determined by MTT method after treating with PM2.5. Collect RAW264.7 cells growing in logarithmic phase, add culture medium to make cell suspension, adjust cell concentration, and add 100 μl cell suspension to each well, and adjust the cell density to 1.0 × 104/well. The cells were incubated in an environment of 5% CO2 and 37°C. When the cells covered the bottom of 96 wells, a series of concentrations of PM2.5 were added. After incubation for 48 h, add 10 μl MTT to each hole, culture the cells for 4 h, discard the culture medium, and carefully rinse it out with PBS for 2–3 times, then add 100 μl DMSO to each hole. Vibrate the shaking table at a low speed for 10 min, set the OD value to 490 nm on the microplate reader and conduct a light absorption measurement for each test hole, and calculate the cell survival rate. Survival % = (A490 nm for treated cells/A490 nm for control cells) × 100%, where A490 nm represents the absorbance value.
RAW264.7 cells were pretreated with PM2.5 (0, 10, 20, 40, 80, 100 μg/ml) for 48 h. Cells were digest with trypsin, collected by centrifugation and washed twice with PBS. 1 × 106 cells were suspended in 500 μl binding buffer followed by the addition of 10 μl Annexin V-FITC. After incubation at room temperature in dark for 15 min, add 5 μl PI and the cells were then analyzed using a flow cytometer (BD Accuri, US).
RAW264.7 cells were seeded in a 6-well plate at a density of 1 × 106cells/well and cultured overnight in a 5% CO2 incubator at 37°C to adhere. The cells were incubated with different concentrations of PM2.5 for 48 h. According to the instructions, the cells were gently washed with PBS twice. A small amount of about 200 μl suspension was added to the test tube, and then an appropriate amount of 100 μl dye solution was added. Incubate the cell suspension at 37°C for 15 min and then the fluorescence microscope was used to observe the cell staining.
Determination of the effect of PM2.5 on the production of inflammatory factors in RAW264.7 cells by ELISA. RAW264.7 cells were seeded in a 96-well plate at a density of 1 × 104cells/well and cultured overnight in a 5% CO2 incubator at 37°C. After cell adhesion, different concentrations of PM2.5 (0, 10, 20, 40, 80, and 100 μg/ml) were added into the culture medium and the cells were continued to culture for 48 h. Then, the concentration of IL-1β, IL-6, and TNF-α in each was measured according to the ELISA instruction with a microplate reader at 450 nm. The effects of PHBA and PHPAA on inflammatory cytokines induced by PM2.5 were also determined using ELISA method and the duration of drugs was 24 h. The concentrations of PHBA or PHPAA were 10, 100, and 1,000 μM. In the mixture of PHBA and PHPAA, the molar ratio was 1:1.
RAW264.7 cells (2 × 106 cells/well) were seeded in a 6-well plate, pretreated with PM2.5 (80 μg/L) for 48 h, and then treated with PHBA, PHPAA or mixture of PHBA and PHPAA for 24 h. Trizol reagent (Invitrogen Life Technologies, Carlsbad, CA, United States) was used to extract the total RNA of the cells pretreated with PM2.5. Detection of mRNA expression with Applied Biosystems ViiATM 7 Real-Time PCR system. cDNA synthesis was performed using a PrimeScript™ RT Reagent Kit according to the manufacturer’s instructions. After reverse transcription, the cDNA was amplified using SYBR-Green Premix (Takara, Otsu, Japan). Level of TLR2, TLR4, MyD88, IRAK1, TRAF6, TAK1, IKKβ, and NF-κB mRNA expressions were examined, and GAPDH was used as an internal control. Data were analyzed by the 2−ΔΔCT method. Sequences of primers used for qRT-PCR are list in Table 1.
After overnight culture in a 6-well plate (2 × 106 cells/well, 3 ml medium/plate), the cells were pre-treated with PM2.5 for 48 h and drugs for an additional 24 h, cells were harvested and lysed in lysis buffer for 15 min on ice. After incubation, lysates were centrifuged and supernatant was collected. The protein concentration was measured by the bicinchoninic acid (BCA) method. 20 μg protein sample was separated on 10% SDS-PAGE and transferred to PVDF membranes. At room temperature, the membrane was sealed with 5% skimmed milk powder dissolved in triple buffered saline containing .1% Tween-20 (TBST) for 1 h. Then incubate the membrane with the primary antibody that recognized TLR2, TLR4, MyD88, IRAK1, TRAF6, TAK1, IKKβ, NF-κB, and GAPDH in a shaking incubator at 4°C overnight, wash it with TBST three times, and incubate it with the secondary antibody conjugated with peroxidase diluted in the closed solution at room temperature for 1 h. After washing, proteins of interest were detected using ECL detection reagent.
Statistical analyses were performed using the SPSS 24.0 software. The data were expressed as the Mean ± SD. Multiple comparisons were evaluated by one-way analysis of variance (ANOVA) with Dunnett’s posttest. Statistical significance was accepted at p < .05 or p < .01.
After administration of PM2.5 at different concentrations, the survival rate of RAW264.7 cells decreased, but there was no statistical difference compared with the control group, indicating that PM2.5 within the measurement range could not inhibit the growth of RAW264.7 cells (Figure 2). It was found by flow cytometry that compared with the control group, PM2.5 of all concentrations did not induce apoptosis (Figure 3). Fluorescence staining results showed that the number of dead cells (red fluorescence) did not increase significantly after the action of PM2.5 of various concentrations. Hochest staining results showed that the nuclear morphology of each PM2.5 group did not change significantly, and no apoptotic cells were observed (Figure 4).
The inflammatory factors produced by RAW264.7 cells stimulated by PM2.5 were detected by ELISA, and the results showed that 80 μg/ml and 100 μg/mL PM2.5 produced significantly higher IL-1β, IL-6, and TNF- α than that of the control group (p < .01). Compared with the control group, there was no difference of IL-1β concentration produced by RAW264.7 cells that stimulated by 10, 20 or 40 μg/mL PM2.5(p>.05) (Figure 5). Through microscopic observation, high concentration of PM2.5 will cause unclear observation field of vision, so 80 μg/ml of PM2.5 was applied in subsequent experiments.
FIGURE 5. Inflammatory cytokine levels of (A) IL-1β, (B) IL-6 and (C) TNF-α in RAW264.7 cells stimulates by PM2.5. Values are means ± SD from three separate experiments. Compared with control group, **p < .01.
Compared with the control group, PM2.5 significantly increased the production of inflammatory factors (p < .01). After different concentrations of PHBA or PHPAA (10, 100, 1,000 μM) treatment, the content of IL-1 β, IL-6, and TNF- α significantly decreased (p < .01). After combined treatment of PHBA and PHPAA (the molar ratio of PHBA and PHPAA was 1:1), the content of IL-1 β, IL-6, and TNF- α significantly decreased (p < .01) (Figure 6).
FIGURE 6. Inhibition of PHBA and PHPAA on inflammatory cytokines produced by PM2.5. (A–C), Relative levels of IL-1β, IL-6, and TNF-α levels at 48 h assessed by ELISA. Values are means ± SD from three separate experiments. Compared with PM2.5 stimulation group, *p < .05, **p < .01.
Compared with the control group, the protein expression of TLRs (TLR2 and TLR4) and related receptors MyD88, IRAK1, TRAF6, TAK1, IKKβ, and NF-κB increased significantly after PM2.5 stimulation (p < .01) (Figure 7). After treating RAW264.7 cells stimulated by PM2.5 with different concentrations of PHBA, the expressions of TLR2, TLR4, MyD88, IRAK1, TRAF6, TAK1, IKKβ, and NF-κB decreased, and the changes showed a dose-effect relationship. However, when treated with a high concentration of PHBA (1,000 μM), the protein expression of TLR2 increased (Figure 7). Treating RAW264.7 cells stimulated by PM2.5 with different concentrations of PHPAA (10, 100, 1,000 μM) could make the protein expression of TLR2, TLR4, MyD88, IRAK1, TRAF6, TAK1, IKKβ, and NF-κB significantly decreased (p < .01). The protein expressions of TLR4, MyD88, IRAK1, TRAF6, TAK1, IKKβ, and NF-κB were increased when PHPAA was treated with high concentration (1,000 μM) (Figure 7). The combination of PHBA and PHPAA (1:1) also significantly decreased the expression of TLRs pathway proteins (p < .01), but increased the expression of TLR2, TLR4, IRAK1 and NF-κB proteins at high concentrations (1000 μM) (Figure 7).
FIGURE 7. Protein expression of TLRs related pathways. (A), Representative immunoblots of TLR2, TLR4, MyD88, IRAK1, TRAF6, TAK1, IKKβ, and NF-κB. (B1–B8), WB results of TLR2, TLR4, MyD88, IRAK1, TRAF6, TAK1, IKKβ, and NF-κB in cell at 48 h. Values are means ± SD from three separate experiments. Compared with PM2.5 stimulation group, *p < .05, **p < .01.
The gene expressions of TLRs and its downstream related pathway were determined by RT-qPCR. PM2.5 can significantly up-regulate the gene expression of TLR2, TLR4, MyD88, IRAK1, TRAF6, TAK1, IKK ß and NF- κ B(p<.01) (Figure 8, Table2). After treating RAW264.7 cells stimulated by PM2.5 with drugs for 24 h, the TLRs and its downstream pathway related genes were detected. The results showed that PHBA could significantly down regulate the TLRs related pathway genes in a dose effect relationship (p < .01) (Figure 8; Table2). After the cells stimulated by PM2.5 were treated with PHPAA, the gene expression of TLRs pathway was significantly decreased (p < .01). When PHPAA concentration is high (1,000 μM), the expression of related genes TLR4, MyD88, IRAK1, TRAF6, TAK1, IKK ß, and NF- κB increased (Figure 8; Table3). When the mixture of PHBA and PHPAA (1:1) was used, the gene expression of TLRs pathway was significantly decreased (p < .01). When the mixture is at high concentration (1,000 μM), the expression of TLR2, TLR4, IRAK1 and NF-κB significantly increased (Figure 8; Table4).
FIGURE 8. Gene expression of TLRs related pathways. Values are means ± SD from three separate experiments. Compared with PM2.5 stimulation group, *p < .05, **p < .01.
PM2.5 has a great impact on the central nervous system, blood system, metabolic immune system, digestive system, skin and other human bodies, and may lead to a variety of diseases. PM2.5 can destroy the integrity of the blood brain barrier (BBB) and make it easy for peripheral systemic inflammation to pass through the BBB to reach the central nervous system (Shou et al., 2019). The study found that the concentration of PM2.5 had a certain toxic effect on children’s bone marrow stromal cells, which affected the hematopoietic microenvironment of bone marrow. PM2.5 may activate PINK1/Parking pathway signal and induce mitochondrial autophagy by increasing ROS, and further activate HSCs (hepatic stellate cells) to cause liver fibrosis (Qiu et al., 2019). Cholesterol and squalene are key substances that affect the skin barrier function. PM2.5 can cause barrier disorder by increasing cholesterol synthesis, leading to the temporary accumulation of epidermal cholesterol and the reduction of squalene (Liao et al., 2019). It can be seen that PM2.5 is harmful to human health. It is very important to find effective drugs to treat PM2.5 as soon as possible.
It has been reported that PM2.5 can activate the inflammatory axis of vascular endothelial cell COX-2/PGES/PGE2, and promote cell apoptosis and inflammatory response (Yin et al., 2017). PM2.5 may induce apoptosis by increasing lipid accumulation, ROS level and activating mitochondrial pathway of macrophages. However, the results of MTT, flow cytometry and Calcein AM/PI staining showed that PM2.5 used in our study had no cytotoxicity to RAW264.7 and had no effect on apoptosis. The experimental results obtained in this study are different from those in related papers, which may be related to the physical and chemical properties of PM2.5 collected, processing and preservation methods of PM2.5.
It is reported that PM2.5 can cause airway inflammation and lung injury in mice, and can also produce cellular inflammation and secrete inflammatory factors from RAW264.7 cells (He et al., 2017; Ogino et al., 2018). In this study, PM2.5 was used to stimulate RAW264.7 cells, and the related inflammatory factor IL-1β, IL-6, and TNF-α secreted by cells significantly increased. However, when PHBA, PHPAA, the mixture of PHBA and PHPAA (1:1) were given in advance, the detection results of inflammatory factors secreted by cells were significantly reduced. It suggests that PHBA and PHPAA can repair the damage caused by PM2.5 through inhibiting inflammatory factors.
In the induction of inflammatory response, TLRs play a role mainly through MyD88 and TRIF mediated pathways (Deguine and Barton., 2014). When sensing external stimuli, TLRs allow MyD88 to dock with MAL (MyD88 adapter-like). MAL is a bridging linker, often involved in TLR4 signal pathway, while TLR2 signal pathway is less involved, and interacts with MyD88 through TIR. In addition to the TIR domain, MyD88 also contains a dead domain, which can help it interact with IRAK4 (Janssens and Beyaert., 2003; Lim and Staudt., 2013). The interaction between these domains produces a large polymer, and the nitration of this polymer leads to the activation and dimerization of TRAF6 (Lin et al., 2010). TRAF6 mediates the ubiquitination of TAK1. The lack of TAK1 reduces the inflammatory signal of TLRs, but this phenomenon is not observed when TAB protein is lacking. TAK1 signal then activate NF- κ B and MAPK, respectively. NF-κB is a molecular center of inflammation signal, and activated by phosphorylated IKK α And IKK ß (Kawai and Akira., 2007). TRF signal is a separate branch of TLR signal, which can only be continued through TLR3 and TLR4, where TRIF interacts with TRAF3 and TRAF6 (Akira et al., 2006). In this study, the protein and gene of TLR pathway related factors were determined. It was found that PM2.5 can increase the expression of TLR2/4→MyD88→IRAK1 (TRAF6) →TAK1→IKKβ→NF-κB protein and gene in TLR pathway and eventually lead to inflammation (Figure 9). When PHBA, PHPAA and the mixture of PHBA and PHPAA (1:1) were added to PM2.5-stimulated RAW264.7 cells, the above three factors could reduce the expression of TLRs related pathway proteins and genes. These results indicate that PHBA and PHPAA can reduce inflammation caused by PM2.5 by regulating TLRs and their related pathway signal transduction factors.
In this study, we measured the protein and gene of TLR pathway related factors and found that PM2.5 can increase the protein and gene expression of TLR pathway and eventually lead to inflammation. PHBA, PHPAA and the mixture of PHBA and PHPAA (1:1) can reduce the expression of TLRs related pathway proteins and genes of RAW264.7 cells stimulated by PM2.5, indicating that PHBA and PHPAA can reduce the inflammation produced by PM2.5 by regulating TLRs and its related pathway signal transduction factors.
PM2.5 can produce inflammatory reaction and secrete inflammatory cytokines. The molecular mechanism of inflammation produced by PM2.5 is related to TLRs and its related pathways, and TLRs pathway can become a potential new target for treating inflammation produced by PM2.5. PHBA, PHPAA and their combination can reduce the inflammatory reaction produced by PM2.5. The mechanism of action is related to the inhibition of TLRs and its related pathways, indicating that TLRs signal pathway may be a potential pathway for PHBA and PHPAA to treat inflammation induced by PM2.5.
The original contributions presented in the study are included in the article/Supplementary Material, further inquiries can be directed to the corresponding author.
Study design: B-LX and X-BW; data collection: B-LX, Y-YW, L-LJ, and ZL, D-RL, HZ, and S-LL; Analysis and interpretation: B-LX, Y-YW, and L-LJ; Statistical analysis: Y-YW; Drafting manuscript: B-LX; Revision manuscript: X-BW.
The authors thanks Hong-Hai YU from Huadian Electric Power Research Institute Co., LTD. Northeast Branch provided PM2.5 in the experiment.
The authors declare that the research was conducted in the absence of any commercial or financial relationships that could be construed as a potential conflict of interest.
All claims expressed in this article are solely those of the authors and do not necessarily represent those of their affiliated organizations, or those of the publisher, the editors and the reviewers. Any product that may be evaluated in this article, or claim that may be made by its manufacturer, is not guaranteed or endorsed by the publisher.
Akira, S., Uematsu, S., and Takeuchi, O. (2006). Pathogen recognition and innate immunity. Cell 124 (4), 783–801. doi:10.1016/j.cell.2006.02.015
Boothe, V. L., Boehmer, T. K., Wendel, A. M., and Yip, F. Y. (2014). Residential traffic exposure and childhood leukemia: A systematic review and meta-analysis. Am. J. Prev. Med. 46 (4), 413–422. doi:10.1016/j.amepre.2013.11.004
Chen, P., Wang, Y., Chen, L., Jiang, W., Niu, Y., Shao, Q., et al. (2015). Comparison of the anti-inflammatory active constituents and hepatotoxic pyrrolizidine alkaloids in two Senecio plants and their preparations by LC-UV and LC-MS. J. Pharm. Biomed. Anal. 10 (115), 260–271. doi:10.1016/j.jpba.2015.07.014
Deguine, J., and Barton, G. M. (2014). MyD88: A central player in innate immune signaling. F1000Prime Rep. 4, 697. doi:10.12703/P6-97
Geng, J., Liu, H., Ge, P., Hu, T., Zhang, Y., Zhang, X., et al. (2019). PM2.5 promotes plaque vulnerability at different stages of atherosclerosis and the formation of foam cells via TLR4/MyD88/NFκB pathway. Ecotoxicol. Environ. Saf. 30 (176), 76–84. doi:10.1016/j.ecoenv.2019.03.068
Han, Y., Qi, M., Chen, Y., Shen, H., Liu, J., Huang, Y., et al. (2015). Influences of ambient air PM- concentration and meteorological condition on the indoor PM₂.₅ concentrations in a residential apartment in Beijing using a new approach. Environ. Pollut. 205, 307–314. doi:10.1016/j.envpol.2015.04.026
He, M., Ichinose, T., Yoshida, S., Ito, T., He, C., Yoshida, Y., et al. (2017). PM2.5-induced lung inflammation in mice: Differences of inflammatory response in macrophages and type II alveolar cells. J. Appl. Toxicol. 37 (10), 1203–1218. doi:10.1002/jat.3482
Huang, X. F., Yun, H., Gong, Z. H., Li, X., He, L. Y., Zhang, Y. H., et al. (2014). Source apportionment and secondary organic aerosol estimation of PM2.5 in an urban atmosphere in China. Sci. China(Earth Sci. 57 (06), 1352–1362. doi:10.1007/s11430-013-4686-2
Janssens, S., and Beyaert, R. (2003). Functional diversity and regulation of different interleukin-1 receptor-associated kinase (IRAK) family members. Mol. Cell 11 (2), 293–302. doi:10.1016/s1097-2765(03)00053-4
Jeong, S., Park, S. A., Park, I., Kim, P., Cho, N. H., Hyun, J. W., et al. (2019). PM2.5 exposure in the respiratory system induces distinct inflammatory signaling in the lung and the liver of mice. J. Immunol. Res. 1, 3486841. doi:10.1155/2019/3486841
Ji, W., and Zhao, B. (2015). Contribution of outdoor-originating particles, indoor-emitted particles and indoor secondary organic aerosol (soa) to residential indoor PM2.5 concentration: A model-based estimation. Build. Environ. 90, 196–205. doi:10.1016/j.buildenv.2015.04.006
Kawai, T., and Akira, S. (2007). Signaling to NF-kappaB by toll-like receptors. Trends Mol. Med. 13 (11), 460–469. doi:10.1016/j.molmed.2007.09.002
Li, L. J., Wang, J. Y., Wang, Y., Wang, L. J., Wang, H. Y., and Wang, B. X. (2005). Anti-virus activities of the extract and effective components isolated from Senecio cannabifolius less. China J. Basic Med. TCM. 11 (8), 585–587.
Li, T., Cao, S., Fan, D., Zhang, Y., Wang, B., Zhao, X., et al. (2016). Household concentrations and personal exposure of PM2.5 among urban residents using different cooking fuels. Sci. Total Environ. 1, 548–549. doi:10.1016/j.scitotenv.2016.01.038
Liao, Z., Nie, J., and Sun, P. (2019). The impact of particulate matter (PM2.5) on skin barrier revealed by transcriptome analysis: Focusing on cholesterol metabolism. Toxicol. Rep. 25 (7), 1–9. doi:10.1016/j.toxrep.2019.11.014
Lim, K. H., and Staudt, L. M. (2013). Toll-like receptor signaling. Cold Spring Harb. Perspect. Biol. 5 (1), a011247. doi:10.1101/cshperspect.a011247
Lin, S. C., Lo, Y. C., and Wu, H. (2010). Helical assembly in the MyD88-IRAK4-IRAK2 complex in TLR/IL-1R signalling. Nature 17 (7300), 465885–465890. doi:10.1038/nature09121
Lin, Y., Zou, J., Yang, W., and Li, C. Q. (2018). A review of recent advances in research on PM2.5 in China. Int. J. Environ. Res. Public Health 15 (3), 438. doi:10.3390/ijerph15030438
Ogino, K., Nagaoka, K., Ito, T., Takemoto, K., Okuda, T., Nakayama, S. F., et al. (2018). Involvement of PM2.5-bound protein and metals in PM2.5-induced allergic airway inflammation in mice. Inhal. Toxicol. 30 (13-14), 498–508. doi:10.1080/08958378.2018.1561769
Park, S. K., and Wang, W. (2014). Ambient air pollution and type 2 diabetes: A systematic review of epidemiologic research. Curr. Environ. Health Rep. 1 (3), 275–286. doi:10.1007/s40572-014-0017-9
Piao, M. J., Ahn, M. J., Kang, K. A., Ryu, Y. S., Hyun, Y. J., Shilnikova, K., et al. (2018). Particulate matter 2.5 damages skin cells by inducing oxidative stress, subcellular organelle dysfunction, and apoptosis. Arch. Toxicol. 92 (6), 2077–2091. doi:10.1007/s00204-018-2197-9
Qiu, Y. N., Wang, G. H., Zhou, F., Hao, J. J., Tian, L., Guan, L. F., et al. (2019). PM2.5 induces liver fibrosis via triggering ROS-mediated mitophagy. Ecotoxicol. Environ. Saf. 167, 178–187. doi:10.1016/j.ecoenv.2018.08.050
Shaffer, R. M., Sheppard, L., Peskind, E. R., Zhang, J., Adar, S. D., and Li, G. (2019). Fine particulate matter exposure and cerebrospinal fluid markers of vascular injury. J. Alzheimers Dis. 71 (3), 1015–1025. doi:10.3233/JAD-190563
Shou, Y., Huang, Y., Zhu, X., Liu, C., Hu, Y., and Wang, H. (2019). A review of the possible associations between ambient PM2.5 exposures and the development of Alzheimer's disease. Ecotoxicol. Environ. Saf. 15 (174), 344–352. doi:10.1016/j.ecoenv.2019.02.086
Sun, J. D., Wang, X. B., Jiang, S., Xi, R. G., and Tian, Y. (2014). Preventive effect of Senecio cannabifolius Less. on perfluoroisobutylene inhalation-induced acute lung injury in rats. J. Int. Pharm. Res. 41 (4), 444. doi:10.13220/j.cnki.jipr.2014.04.01
Yin, J., Xia, W., Li, Y., Guo, C., Zhang, Y., Huang, S., et al. (2017). COX-2 mediates PM2.5-induced apoptosis and inflammation in vascular endothelial cells. Am. J. Transl. Res. 9 (9), 3967–3976.
Zhao, G. Y., Wang, X. B., Xi, R. G., Jiang, S., and Wu, L. J. (2013). Protective effect of Senecio cannabifolius less. On acute lung injury induced by perfluoroisobutylene on mice. Pharm. J. Chin. People's Lib. Army. 29 (01), 13–16.
Keywords: PM2.5, Jacobaea cannabifolia (Less.), PHBA, PHPAA, toll-like receptors
Citation: Xu B-L, Wang Y-Y, Jiang L-L, Liu Z, Liu D-R, Zhao H, Li S-L and Wang X-B (2023) Inhibitory effect of main phenolic acid components of Jacobaea cannabifolia (Less.) on inflammation caused by PM2.5. Front. Pharmacol. 13:1096137. doi: 10.3389/fphar.2022.1096137
Received: 11 November 2022; Accepted: 12 December 2022;
Published: 09 January 2023.
Edited by:
Zheng Xiang, Liaoning University, ChinaReviewed by:
Hongxun Tao, Jiangsu University, ChinaCopyright © 2023 Xu, Wang, Jiang, Liu, Liu, Zhao, Li and Wang. This is an open-access article distributed under the terms of the Creative Commons Attribution License (CC BY). The use, distribution or reproduction in other forums is permitted, provided the original author(s) and the copyright owner(s) are credited and that the original publication in this journal is cited, in accordance with accepted academic practice. No use, distribution or reproduction is permitted which does not comply with these terms.
*Correspondence: Xiao-Bo Wang, d3hiYmVuc29uMDY1M0BzaW5hLmNvbQ==
Disclaimer: All claims expressed in this article are solely those of the authors and do not necessarily represent those of their affiliated organizations, or those of the publisher, the editors and the reviewers. Any product that may be evaluated in this article or claim that may be made by its manufacturer is not guaranteed or endorsed by the publisher.
Research integrity at Frontiers
Learn more about the work of our research integrity team to safeguard the quality of each article we publish.