- 1Department of Biochemistry and Molecular Medicine, School of Medicine, University of California Davis, Sacramento, CA, United States
- 2Centre National de la Recherche Scientifique, SYS2DIAG-ALCEN, Cap Delta, Montpellier, France
- 3Center for Health and the Environment, University of California Davis, Davis, CA, United States
- 4Department of Environmental Toxicology, University of California Davis, Davis, CA, United States
Activation of the aryl hydrocarbon receptor (AhR) through environmental exposure to chemicals including polycyclic aromatic hydrocarbons (PAHs) and polychlorinated dibenzo-p-dioxins (PCDDs) can lead to severe adverse health effects and increase the risk of breast cancer. This review considers several mechanisms which link the tumor promoting effects of environmental pollutants with the AhR signaling pathway, contributing to the development and progression of breast cancer. We explore AhR’s function in shaping the tumor microenvironment, modifying immune tolerance, and regulating cancer stemness, driving breast cancer chemoresistance and metastasis. The complexity of AhR, with evidence for both oncogenic and tumor suppressor roles is discussed. We propose that AhR functions as a “molecular bridge”, linking disproportionate toxin exposure and policies which underlie environmental injustice with tumor cell behaviors which drive poor patient outcomes.
Introduction—Environmental exposure and breast cancer
Air pollution and occupational exposure studies have reported positive associations with the risk of developing breast cancer (Amadou et al., 2021). Air pollution and ambient particulate matter (PM) contain a complex mixture of compounds, including polycyclic aromatic hydrocarbons (PAHs) and various metals (e.g. iron, nickel, copper), which may induce reactive oxygen species (ROS) and inflammation (Rückerl et al., 2007; Grunig et al., 2014) and stimulate the progression of breast cancer (Romaniuk et al., 2017). PAHs are generated during combustion processes and derive from various sources such as indoor fireplaces, wildfires, industrial activities, and vehicular traffic and the exposure to PAHs has been identified as a risk factor for breast cancer (Lichtiger et al., 2021; Gamboa-Loira et al., 2022). Importantly, a stronger association of breast cancer risk was found with traffic related air pollution (TRAP) and higher PAH exposure intensity and duration of exposure (Brody et al., 2007; Nie et al., 2007; Mordukhovich et al., 2016; Large and Wei 2017; Shen et al., 2017; Lee et al., 2019). Vehicular traffic is a major ambient source of PAH exposure and the PAH benzo[a]pyrene (BaP) is classified as a human carcinogen by the International Agency for Research on Cancer (IARC, 2010). Furthermore, BaP and other PAHs have been identified as ligands of the aryl hydrocarbon receptor (AhR), a ligand-activated transcription factor, belonging to the bHLH-PAS family, which regulates multiple target genes and is best known for its role as a xenobiotic receptor (Boonen et al., 2020; Vogel et al., 2020). The activation of the AhR signaling pathway via environmental pollutants including dioxins and PAHs has been associated with the development of breast cancer (Birnbaum and Fenton 2003; La Merrill et al., 2010; Murray et al., 2014; Warner et al., 2011; Kolluri et al., 2017; Donovan et al., 2018; Narasimhan et al., 2018; Gearhart-Serna et al., 2020). Reports from our team and other groups suggest an important role of AhR as an immune-modulator and mediator of toxic responses triggered by particulate matter (PM) derived from TRAP (O'Driscoll et al., 2019; O'Driscoll and Mezrich, 2018; Castaneda et al., 2018; Yuan et al., 2020).
Recent studies confirmed an increased risk of breast cancer with vehicular-specific PM exposure among African American and Japanese American women living near major roads, highlighting the link between environmental injustice and health disparities (Cheng et al., 2020; Niehoof et al., 2020). Indeed, residential proximity to major roadways is a recognized risk factor beyond breast cancer, in cardiovascular disease (Hart et al., 2014; Kirwa et al., 2014; Kingsley et al., 2015; Kubil et al., 2018) and renal disease (Lue et al., 2013). Further, it disproportionately impacts racial and ethnic minoritized groups and those of lower socioeconomic status, the legacy of the widespread practice of redlining in the United States (Hwa Jung et al., 2022; Swope et al., 2022). While the Fair Housing Act of 1968 prohibited racial discrimination in housing and lending, exclusionary zoning and other practices such as gentrification has perpetuated residential segregation (https://www.brookings.edu/research/neighborhood-segregation-persists-for-black-latino-or-hispanic-and-asian-americans/). In a study of Hillsborough County in Florida, Stuart et al. (2009) found that blacks, Hispanics, and people living below the poverty line are much more likely to reside close to sources of air pollution but further from air quality monitoring sites while whites were found to live closer to monitoring sites but significantly further from pollution sources. Wu et al. (2014) found that particulate matter (PM) collected near a major Los Angeles freeway (compared to an urban background location) induced significantly higher production of the cytokines IL-6, IL-8, and TNF-α, suggesting a link between AhR activation, AhR-driven inflammation (Vogel et al., 2011; Vacher et al., 2018; Wu et al., 2021) and proximity to pollution. The interaction between environmental exposure, socio-economic related stress and psychosocial stress in under-resourced neighborhoods has been termed the environmental “riskscape” by Morello-Frosch & Shenassa, 2006 (Morello-Frosch & Lopez, 2006). As noted by Morello-Frosch, the Institute of Medicine recognizes this as a type of “double jeopardy” in which elevated stress impairs the ability of individuals living in under-resourced neighborhoods to endure the myriad health consequences of chronic environmental exposures (https://www.scientificamerican.com/article/end-double-jeopardy/#).
Role of AhR in breast cancer
Approximately 2 decades ago, AhR was found to be overexpressed in mammary cancer in rats (Trombino et al., 2000) sparking curiosity as to its role in breast cancer progression. Several studies have since shown that chemical exposure and AhR activation affect processes of mammary gland differentiation, disrupting pregnancy-related differentiation and milk production, and increasing the risk of breast cancer (Warner et al., 2002; Vorderstrasse et al., 2004; Lew et al., 2011; Belton et al., 2018; Kay et al., 2022). Further studies have elucidated AhR’s molecular contribution to carcinogenic progression and ratified the oncogenic role of AhR in breast cancer cells (Wang et al., 2017; Wang et al., 2020). In support of its role as a breast cancer oncogene, AhR activation is sufficient to transform human mammary epithelial cells and promote their migration, invasion and epithelial-to-mesenchymal transition (EMT) (Brooks and Eltom 2011). Work from our group showed that chronic exposure of MCF10AT1 and MCF-7 cells to estradiol (E2) resulted in AhR overexpression and downregulation of estrogen receptor alpha (ERα) and progesterone receptor (Zou and Matsumura 2003; Wong et al., 2009) accompanied by increased proliferation, invasion, and apoptosis resistance. The resistance to apoptosis was also demonstrated in human breast cancer cell lines treated with the prototypical AhR ligand TCDD when apoptosis was induced by chemotherapeutics (doxorubicin, lapatinib and paclitaxel) (Bekki et al., 2015). Treatment with PAH mixtures which bind to and activate AhR also increased cell proliferation and expression of antiapoptotic proteins in MCF-7 cells via AhR signaling (Gearhart-Serna et al., 2020).
Several studies have reported AhR overexpression in human breast cancer (Li et al., 2014; D’Amato et al., 2015; Vacher et al., 2018). Using samples from breast cancer patients, we found that AhR is frequently over-expressed in ER-negative human breast tumors, and this is closely correlated with elevated expression of the NF-кB subunit RelB and inflammatory markers such as IL-8 (CXCL1 in mouse) and COX-2 (Vogel et al., 2011). This was also observed by Vacher et al. with significant overexpression of cytokines, including IL-8, in AhR high expressing tumors (Vacher et al., 2018). We demonstrated that C/EBPβ serves as a key transactivator for AhR-mediated COX-2 gene induction (Vogel et al., 2000; Vogel et al., 2004). Interestingly, COX-2, CXCL1, and IL-8 have been identified as critical genes that mediate breast cancer invasion and metastasis to lung and lymph nodes (Freund et al., 2003; Minn et al., 2005; Ahmed et al., 2021). A recent report suggests that inhibition of COX-2 expression reduces mammary tumor multiplicity and size in the polyoma middle T antigen (PyMT) mouse model (Esbona et al., 2016). In our recent study we demonstrated that overexpression of AhRR (Aryl Hydrocarbon Receptor Repressor) suppresses AhR-driven (TCDD-stimulated) growth of syngeneic mammary tumors as well the onset, growth and metastasis of spontaneous mammary tumors in PyMT mice (Vogel et al., 2021). In human breast cancer, high expression of AhRR, the dedicated AhR repressor, independently predicts prolonged metastasis-free survival (Vacher et al., 2018), in agreement with our findings in PyMT mice (Vogel et al., 2021). Interestingly, knockdown of AhRR in normal human mammary epithelial cells resulted in anchorage-independent cell growth suggesting that the AhRR may function as a tumor suppressor gene (Zudaire et al., 2008).
In a mouse model of BRCA1-associated breast cancer, AhR was found to transcriptionally induce the EGF receptor ligand, Amphiregulin, driving tumor growth and macrophage infiltration. Of note, this was inhibited by the combination of an AhR inhibitor and an EGF receptor inhibitor, suggesting new therapeutic possibilities for this type of breast cancer (Kubli et al., 2019). The relationship between AhR activation and breast cancer-related death was recently assessed using an artificial intelligence tool to analyze the scientific literature, with strong evidence that AhR activation is an adverse outcome pathway in breast cancer (Benoit et al., 2022).
Interestingly, many studies have also provided evidence for a tumor suppressor role for AhR, with evidence that AhR can inhibit tumor growth (Fritz et al., 2007; Jin et al., 2014; Feng et al., 2020) while inhibition of AhR or AhR deficiency promotes tumor development (Abdelrahim et al., 2003; Safe et al., 2017). For example, in the ApcS580/+; KrasG12D/+ mouse model of colon tumorigenesis, intestinal epithelial specific AhR knockout promoted tumorigenesis through enhanced Wnt signaling (Han et al., 2021). In p53 deficient mice, AhR knockout significantly increased incidence of thymic lymphomas and sarcomas and decreased survival (Phillips et al., 2022). In a mouse model of sonic hedgehog type-medulloblastoma, AhR deletion in cerebellar granule cell progenitors accelerated tumorigenesis through increased TGFβ-SMAD3 signaling (Sarić et al., 2020) with high AhRR expression linked to decreased patient survival. Further, in an unbiased functional genomics screen, AhR was identified as metastasis suppressor in a lung cancer model (Nothdurft et al., 2020). In in vitro studies, AhR was demonstrated to cooperate with the Rb tumor suppressor to prevent S-phase cell cycle entry (Puga et al., 2000) while activation of AhR by the prototypical ligand TCDD inhibited the growth of MCF7 breast cancer cells (Vogel and Abel 1995). David Sherr’s team investigated AhR agonists and antagonists in a direct comparison and concluded that the sustained activation of AhR drives the later, more lethal stages of some cancers, but that AhR agonists under some circumstances can counteract tumor development and may also serve as cancer therapeutics (Narasimhan et al., 2018). In this vein, O’Donnell (O’Donnell et al., 2021) and others (Rowland et al., 2019) have pursued SMAhRTs, Select Modulators of AhR-regulated Transcription, to specifically exploit the anti-cancer functions of AhR. Notably, they identified a modulator which induced AhR-dependent Fas ligand expression and breast and liver tumor cell apoptosis without increasing expression of the prototypical AhR target gene, CYP1A1, suggesting that AhR transcriptional activity can be fine-tuned, to specifically unlock its function as a tumor suppressor.
Cytokines and chemokines in breast cancer and the tumor microenvironment
The tumor microenvironment (TME) corresponds to the fact that tumor cells are surrounded in close proximity by a number of non - cancerous cells including cancer associated fibroblasts (CAFs), mesenchymal stem cells (MSCs), adipocytes, myeloid-derived suppressor cells (MDSCs), tumor associated macrophages (TAMs), tumor associated neutrophils (TANs), tumor infiltrating lymphocytes (TILs), and endothelial cells (Joyce and Pollard 2009; Lazennec and Lam 2016; Binnewies et al., 2018). In addition to direct contact with tumor cells, TME cells will interact with tumor cells though a number of different soluble factors including cytokines and chemokines, which will reshape TME to support cancer initiation, progression, and metastasis (Ali and Lazennec 2007; Lazennec and Richmond 2010; Mancini et al., 2021) (Figure 1).
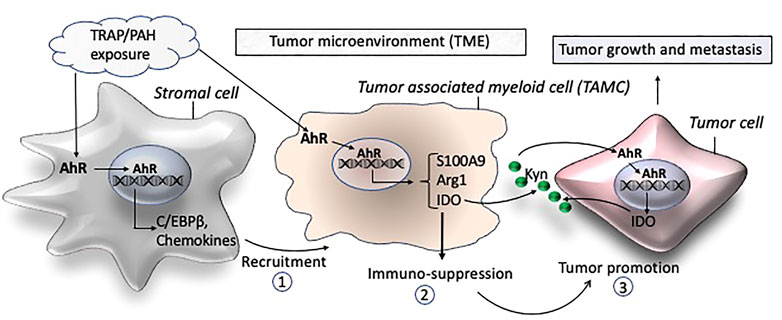
FIGURE 1. Schematic of proposed mechanisms by which traffic-associated air pollution (TRAP) activates AhR to induce the accumulation of TAMCs (1) and expression of immune suppressive factors (2) leading to a tumor promoting microenvironment (3) and growth and metastasis of breast cancer.
In breast cancer, many chemokines and cytokines have been analyzed and identified as important factors contributing to the development of breast tumors (Narita et al., 2016; Masih et al., 2022). In particular the CXCR4/CXCL12 axes has been reported to control breast cancer metastasis and the involvement of CAFs (Muller et al., 2001; Orimo et al., 2005). The CAF-driven CXCR4/CXCL12 axis may also stimulate the accumulation of protumorigenic lipid associated macrophages which supports an immunosuppressive microenvironment in breast cancer (Timperi et al., 2022).
CCL2 and CCL5 have also retained attention in breast cancer, as they are expressed by cancer cells and promote the recruitment of TAMs and metastasis by inducing Th2 polarization of CD4+ T cells (Chavey et al., 2007; Soria and Ben-Baruch 2008; Zhang et al., 2015; Brummer et al., 2018). In addition, the ligands of CXCR2 (CXCL1, 2, 3, 5, 6, 7, 8) have been shown in a number of studies to be involved in the aggressiveness of triple negative breast cancers (TNBC) (Bieche et al., 2007; Chavey et al., 2007; Acharyya et al., 2012). The genes of these chemokines are encoded by a small region of chromosome 4q21 and have been found to be coregulated in TNBC (Bieche et al., 2007). Moreover, cancer cells expressing high levels of CXCL1 and CXCL2 acquire an advantage in terms of survival in metastatic sites and favor the recruitment of TANs (Acharyya et al., 2012). Interestingly, CXCR2 itself is also playing a major role in the aggressiveness of TNBC, in particular through its expression on TANs (Boissiere-Michot et al., 2020; Boissiere-Michot et al., 2021). Although the levels of CXCR2-expressing neutrophils is correlated to high grade breast cancers, its role is rather to counteract tumor progression (Boissiere-Michot et al., 2021), as it is correlated with a better survival of the patients and its deletion favors tumor growth and metastasis (Timaxian et al., 2021). There are many links between AhR and CXCR ligands in particular. For instance, we have shown that the complex of AhR and NFkB RelB was able to bind to a specific binding elements of chemokines including the CXCL8 promoter and to promote its activation though protein kinase A (Vogel et al., 2007). RelB/AhR complex is also involved in the overexpression of CXCL8 in breast cancer (Vogel et al., 2011; Bekki et al., 2015). A significant elevated level of CXCL8 mRNAs expression (56-fold) has also been found in tissue samples of high stage compared to low stage patients and adipose-derived stem cells (Razmkhah et al., 2010). AhR may also interact with NFkB RelA causing the upregulation of c-myc and stimulation of tumorigenesis in MCF-7 cells (Kim et al., 2000). Further HER2 overexpression in MCF-7 cells resulted in pro-inflammatory signaling and induction of IL-6 enhancing mammosphere formation in an AhR-dependent manner (Zhao et al., 2013). The role of AhR as a mediator of chronic inflammation in breast cancer has been recently reviewed elsewhere (Guarnieri 2020). Moreover, a recent study by Kubli et al. has shown that AhR was induced by reactive oxygen species (ROS) in mammary epithelial cells, which in turn enhance AREG (amphiregulin) production (Kubli et al., 2019). In basal-like and BRCA1-related breast cancers, ROS expression was correlated with AhR levels and the expression of the chemokines CXCL1, CXCL2, and CCL5. Targeting AhR or AREG reduced the recruitment of macrophages in tumors in mouse models and AREG expression was associated with the density of macrophages in human tumors. Another cytokine upregulated by AhR activation is IL-22 which is an important factor controlling host defense and gut immunity. However, dysregulation of IL-22 may contribute to the development of TNBC and the pathology in breast cancer (Kim et al., 2014; Voigt et al., 2017; Wang et al., 2018; Katara et al., 2020). IL-22 has also been described to mediate macrophage infiltration in the TME and the migration of breast cancer cells (Kim et al., 2020). Results from MCF-7 cells co-cultured with preadipocytes and an in vivo zebrafish model showed that prototypical AhR ligand TCDD enhanced the invasive and metastatic potential of MCF-7 cells implicating the importance of AhR in the TME (Koual et al., 2021).
AhR as a critical player in the tumor microenvironment of breast cancer
The development of metastatic disease, which accounts for greater than 90% of cancer mortality, requires collaboration between tumor cells and their environment. Recent studies reveal that the TME possesses remarkable cellular heterogeneity with an important role of immune cells in the development and progression of breast cancer (Ben-Baruch 2003; Place et al., 2011). The TME also consists of an acellular component (e.g., soluble cytokines, chemokines, and growth factors), that forms part of the stromal structure as described above. TAMs and MDSCs are tumor-associated myeloid cells (TAMCs) and have been identified as key players in breast cancer progression and metastasis (Cha and Koo 2020). MDSCs are myeloid cells at earlier stages of differentiation and serve as precursor of TAMs (Coffelt et al., 2009). Their presence and frequency have been directly correlated with tumor aggressiveness and is associated with poor survival rates in breast cancer (Leek et al., 1996; Mukhtar et al., 2011a; Mahmoud et al., 2012; Zhang et al., 2012; Zhao et al., 2017; Qiu et al., 2018). They have been found to drive cancer progression via immune regulatory functions creating a tolerogenic environment allowing the tumor to progress (Figure 1). TAMCs inhibit tumor immune responses by blocking T cell functions and proliferation, but they also directly trigger tumor growth by promoting cancer stemness, angiogenesis, EMT and metastasis formation. In breast cancer patients, levels of MDSCs in peripheral blood were found to be about 10-fold higher compared to healthy control individuals (Safarzadeh et al., 2019). Moreover, they found a direct relationship between MDSC levels and tumor stage of breast cancer patients. The study underlines the importance of MDSCs in tumor progression and invasion which was supported by Diaz-Montero et al. (Diaz-Montero et al., 2009) showing that MDSC levels are associated with the clinical stage and metastatic disease burden in patients with breast cancer. MDSCs possess strong immunosuppressive activities and interact with other immune cells to regulate their functions. The number and abundance of TAMs and MDSCs is considered to be an important factor in the clinical success of cancer immunotherapy, underlining their critical role in suppression of immunity in breast cancer patients (Gnant et al., 2011; Gomez-Roca et al., 2015).
AhR plays a critical role in carcinogenesis and tumor immunity (Murray et al., 2014; Xue et al., 2018). Activation of AhR via Kynurenine (Kyn) produced by the immunosuppressive enzyme indoleamine 2, 3-dioxygenase (IDO) in glioblastoma cells has been found to induce the accumulation of TAMs (Takenaka et al., 2019; McKay et al., 2021). They reported that the AhR ligand Kyn is able to activate AhR in TAMs, leading to an increased expression of the chemokine receptor CCR2 by TAMs, which enhances the recruitment of TAMs in response to CCL2. Moreover, AhR stimulates the production of the exonucleotidase CD39 by TAMs, interfering with the function of cytotoxic CD8+ T cells (Takenaka et al., 2019). In melanoma patients, high levels of IDO1 are associated with high levels of Kyn and immunosuppression (Campesato et al., 2020). Using a melanoma model, it was shown that tumors expressing high levels of IDO1 present an enrichment of TAMs and selective inhibition of AhR decreases tumor progression, by inhibiting the immunosuppression mediated by IDO1. Another link of AhR with immune response in cancer is highlighted by the fact that AhR mediates the induction of the poliovirus receptor CD155 by IL-4 and LPS in macrophages, as CD155 is suppressing T cell function (McKay et al., 2021). In the same line, the inhibition of AhR activity in a model of pancreatic cancer promotes the infiltration of CD8+ T cells and improves the response to immune therapy (Hezaveh et al., 2022). This study also showed that AhR is highly expressed in TAMs, involved in their polarization, and associated with a reduction of iNOS, CCL4, and TNFα levels. Further, Neamah et al. (2019) found that treatment with the AhR ligand TCDD induces the accumulation of MDSCs in the peritoneal cavity. Interestingly, we found an accumulation of CD11b+ F4/80+ and CD11b+ F4/80- Ly6G + cell subsets in adipose tissue associated with a significant increase of the chemokine CXCL5 in TCDD-treated mice (Vogel et al., 2016) which indicates accumulation of TAMs and MDSCs (Ugel et al., 2015). Although TAMs and MDSCs are regarded as separate populations, some markers including CD11b are shared among TAMs and MDSCs (Ugel et al., 2015). There are specific markers (e.g., Ly6G and Ly6C) that can be used to distinguish them. Further, MDSCs and TANs express high levels of S100A9 and the immunosuppressive enzymes IDO and arginase 1 (Arg1) which are specific for their immune-suppressive activity in TME of breast cancer (Fridlender et al., 2009; Ostrand-Rosenberg 2016).
The polarization of TAMs and MDSCs within the TME is highly dependent on the local milieu of immune regulatory factors (e.g., C/EBPβ and S100A9) and cytokines and chemokines which can originate from stromal cells (Figure 1). Recently, we identified C/EBPβ as a critical transcription factor in AhR-dependent induction of S100A9 after treatment with PM rich in PAHs (Dahlem et al., 2020). The S100 calcium binding protein S100A9 has been shown to play a critical role in mediating the expansion of MDSCs in breast cancer models (Zhao F et al., 2012). Moreover, S100A9 can act as a transcriptional coactivator during breast cancer development (Song and Struhl 2021) and promotes the immune-suppressive activity of MDSCs (Ostrand-Rosenberg 2016). Regardless of any direct lineage link and distinction between MDSCs, TANs and TAMs, the most important criteria for their role in carcinogenesis are their immune-suppressive and pro-tumoral activities. Importantly, the AhR has been demonstrated to regulate the expression of immune-regulatory markers including Arg1, IDO, IL-10, COX-2, C/EBPβ, and S100A9 (Vogel et al., 2008; Bankoti et al., 2010; Benson and Shepherd 2011; Simones and Shepherd 2011; Vogel et al., 2013; Neamah et al., 2019; Dahlem et al., 2020), which are critical factors in the pathogenesis of breast cancer (Yu et al., 2013; Yu et al., 2014; Dey et al., 2021). Moreover, TCDD increased the activity of the immunosuppressive enzyme IDO which mediates tumor immunity in breast cancer cells (Bekki et al., 2015). Interestingly, AhR as well as NFkB RelB have been shown to induce IDO expression (Vogel et al., 2008; Yu et al., 2014), which is also critically involved in the immunosuppressive mechanisms of myeloid-derived suppressor cells (MDSCs) in breast cancer (Yu et al., 2013). The number and frequency of TAMs and MDSCs have been directly correlated with tumor aggressiveness, and indirectly correlated with clinical outcome in breast cancer (Mukhtar et al., 2011b). The literature also shows that accumulation of TAMCs is a significant prognostic factor in breast cancer (Zhao et al., 2017). A significant heterogeneity of TAMCs in mammary tumors has been described (Movahedi et al., 2010) and the activation of AhR has been shown to activate TAMs (Takenaka et al., 2019) and induce the accumulation of MDSCs (Neamah et al., 2019). The mechanisms that are driving the polarization of immune-suppressive TAMCs in the TME by AhR signaling activated through the exposure to PM, PAHs, and dioxin like chemicals are not clear yet. In summary, data from the literature strongly suggest AhR’s critical role in the microenvironment of mammary tumorigenesis promoting tumor progression and metastasis.
The intersection between environmental exposure and cancer stemness
Breast cancer stem cells (BCSCs), a small but highly plastic subpopulation of tumor cells, have taken center stage in the interplay between chemoresistance, recurrence, and metastasis (Shan et al., 2021). BCSCs, capable of both self-renewal and recapitulation of tumor heterogeneity, are multidrug-resistant (MDR) and highly immune-evasive. MDR is due in part to robust expression of the ABCG2 efflux protein, also known as Breast Cancer Resistance Protein (BCRP) (Zhou et al., 2001; Zattoni et al., 2022), a direct transcriptional target of AhR (Tan et al., 2010). Substantial efforts have focused on strategies which will lead to the effective elimination of BCSCs, however it is recognized that standard endocrine and chemotherapy regimens paradoxically enrich for BCSCs with mesenchymal features, driving tumor recurrence (Li et al., 2008; Creighton et al., 2009; Famta et al., 2022).
AhR has been implicated in cancer stemness and immune evasion in various tumor types serving as a “molecular bridge” between environmental exposure and poor patient prognosis. In lung and nasopharyngeal carcinoma cells, AhR was shown to drive the expression of a panel of stemness genes, including ABCG2 (Yan et al., 2018). Interestingly, ABCG2 has been directly implicated in expanding the stem population in osteosarcoma cells (Zhou et al., 2001). In non-small cell lung carcinoma, the deubiquitinase UCHL3 promoted cancer stemness through stabilization of AhR (Ouyang et al., 2020). Recently, activation of AhR by the endogenous ligand kynurenine was linked to colon cancer stemness, immune evasion through PD-L1 induction and metastasis (Miyazaki et al., 2022). In an oral squamous cell carcinoma model, tumor cell- and immune cell-expressed AhR collaborated to promote tumor immune evasion with AhR knockout in tumor cells restoring anti-tumor immunity (Kenison et al., 2021).
In breast cancer, tranilast, a tryptophan metabolite and AhR agonist, was shown to inhibit the BCSC population in MDA-MB-231 (triple negative) breast cancer cells and abrogate metastasis in a tail vein injection model (Prud’homme et al., 2010), in an AhR dependent manner. In agreement with these findings, several studies reported that AhR activation inhibits the BCSC population (Saito et al., 2021; Yamashita et al., 2021). In MCF7 (ER+) cells expressing a constitutively active AhR or treated with the AhR agonists 3-Methylcholanthrene (3 MC) or β-naphthoflavone (β-NF), the BCSC population was decreased (Zhao S et al., 2012). Most recently, camalexin, an indole phytoalexin and AhR agonist was shown to decrease the BCSC population of MCF7 and T47D (ER+) breast cancer cells (Yamashita et al., 2022). Conversely, AhR activation by the potent agonists TCDD and DMBA was found to increase the breast cancer stem cell population and was implicated in doxorubicin resistance of MCF-7 breast cancer cells (Al-Dhfyan et al., 2017). In Tamoxifen-resistant MCF7 cells, AhR antagonism inhibited the BCSC population and also inhibited tumor growth (Dubrovska et al., 2012). In Hs578T (triple negative) and SUM149 (inflammatory) breast cancer cells, AhR was shown to augment the BCSC population, and its inhibition decreased tumor growth and sensitized cells to both adriamycin and paclitaxel (Stanford et al., 2016). This study also found a significant correlation between AhR activity and “cancer stem cell- and migration/invasion-associated gene sets” in an analysis of 79 human breast cancer cells lines and more than 1,850 human breast cancers. In inflammatory breast cancer, AhR was linked to BCSC maintenance through the Wnt5a/β-catenin signaling pathway (Mohamed et al., 2018). AhR crosstalk with Wnt/β-catenin signaling in the regulation of CSCs has been reported in several studies (Al-Dhfyan et al., 2017; Akhtar et al., 2022).
The role of AhR in cancer stemness and breast cancer stemness more specifically is complex, influenced by mode of AhR activation, engagement with various signaling pathways and cell context. Nevertheless, the collective evidence strongly suggests that AhR activation by environmental toxins and endogenous ligands (Ala 2021) aligns with chemoresistance, recurrence and metastasis, the hallmarks of cancer stemness. This places AhR at the intersection between racial/ethnic and socioeconomic disparities in toxin exposure in under-resourced neighborhoods, as discussed previously, and cancer stemness, undermining response to cancer therapy, worsening the riskscape that an individual must navigate. In a recent review by Lagunas-Rangel, the authors pose the question “Can Exposure to Environmental Pollutants Be Associated with Less Effective Chemotherapy in Cancer Patients?” The authors summarize evidence which strongly supports this hypothesis, which includes toxins which activate AhR (Lagunas-Rangel et al., 2022). Therachiyil examines this from the perspective of gynecological cancers. (Therachiyil et al., 2022).
Conclusion
Collectively, the body of literature indicates that the role of AhR in cancer is complex, with ample evidence for both an oncogenic and tumor suppressor function, depending on cell and tissue context and mode of AhR activation. However, exposure studies indicate that environmental pollutant-mediated activation of AhR is consistently oncogenic, highlighting the potential for cautious therapeutic intervention. The data from human and in vivo studies, as well as in vitro experiments suggest that exposure to environmental pollutants especially PAHs and dioxin-like chemicals, potent ligands for AhR, increases breast cancer risk and worsens outcome through chemoresistance, immune evasion, EMT, tumor cell proliferation, and metastasis, linked functional outcomes of cancer stemness (Figure 2). Some critical questions remain, including how AhR activation modulates the tumor microenvironment. This review also highlights the role of AhR at the interface between historical and existing systemic practices - which reinforce residential segregation and environmental injustice - and the molecular drivers of aggressive tumor biology. While policies and molecules are not frequently in the same conversation, greater dialogue is needed and opportunities for “upstream” disease prevention through systemic change should be prioritized.
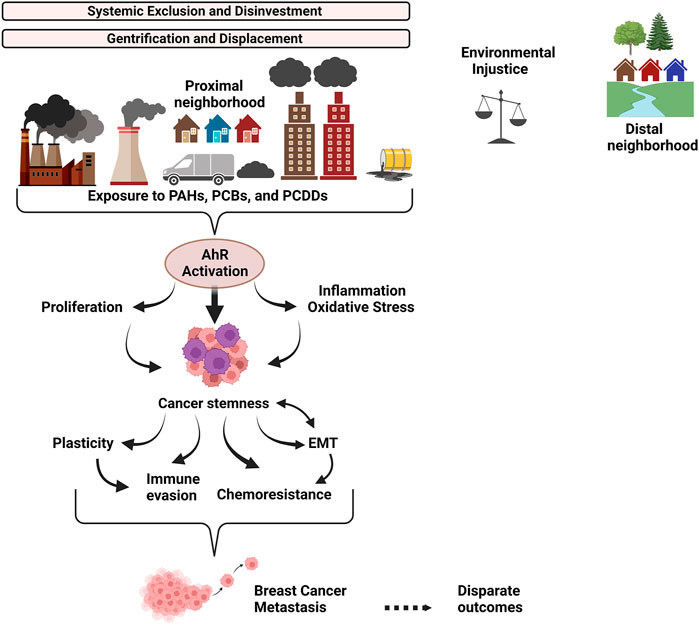
FIGURE 2. Overview of the link between systemic exclusion, environmental injustice, and AhR-driven tumor biology. Discriminatory housing and lending policies (ex: redlining) drove neighborhood racial/ethnic and socioeconomic segregation which persists today due to ongoing systemic discrimination and gentrification. Resources were and are disproportionately allocated to wealthier neighborhoods, contributing to neighborhood disinvestment. The proximal neighborhood has more traffic, pollution-generating factories and dump sites. It has less green space for stress relief and exercise, worsening the riskscape that individuals in the proximal neighborhood must navigate. Individuals living in the proximal neighborhood are chronically exposed to environmental toxins, tipping the scales of environmental justice against them. Polycyclic aromatic hydrocarbons (PAHs), Polychlorinated dibenzo-p-dioxins (PCDDs), and Polychlorinated biphenyls (PCBs) are generated by combustion processes as components of ambient particulate matter (PM) derived from urban areas and industrial activities. PAHs, PCDDs and PCBs robustly activate the AhR signaling pathway, promoting cancer stemness and interrelated functional outcomes, including plasticity, chemoresistance, EMT and immune evasion, which synergize to drive breast cancer metastasis and disparate outcomes for individuals in proximal neighborhoods.
Author contributions
Review concept: CS, GL, and CV. Review design: CS and CV. Manuscript preparation: CS, GL, and CV. Manuscript editing: CS and CV. Manuscript review: CS, GL, and CV.
Funding
This work was supported by pilot funding from the Molecular Oncology Program at the UC Davis Comprehensive Cancer Center and R21ES030419, R01ES032827 (CV).
Acknowledgments
CS gratefully acknowledges the dedicated mentorship of Dr. Jann Murray-Garcia.
Conflict of interest
The authors declare that the research was conducted in the absence of any commercial or financial relationships that could be construed as a potential conflict of interest.
Publisher’s note
All claims expressed in this article are solely those of the authors and do not necessarily represent those of their affiliated organizations, or those of the publisher, the editors and the reviewers. Any product that may be evaluated in this article, or claim that may be made by its manufacturer, is not guaranteed or endorsed by the publisher.
References
Abdelrahim, M., Smith, R., and Safe, S. (2003). Aryl hydrocarbon receptor gene silencing with small inhibitory RNA differentially modulates Ah-responsiveness in MCF-7 and HepG2 cancer cells. Mol. Pharmacol. 63 (6), 1373–1381. doi:10.1124/mol.63.6.1373
Acharyya, S., Oskarsson, T., Vanharanta, S., Malladi, S., Kim, J., Morris, P. G., et al. (2012). A CXCL1 paracrine network links cancer chemoresistance and metastasis. Cell 150 (1), 165–178. doi:10.1016/j.cell.2012.04.042
Ahmed, S., Mohamed, H. T., El-Husseiny, N., El Mahdy, M. M., Safwat, G., Diab, A. A., et al. (2021). IL-8 secreted by tumor associated macrophages contribute to lapatinib resistance in HER2-positive locally advanced breast cancer via activation of Src/STAT3/ERK1/2-mediated EGFR signaling. Biochim. Biophys. Acta. Mol. Cell Res. 1868 (6), 118995. doi:10.1016/j.bbamcr.2021.118995
Akhtar, S., Hourani, S., Therachiyil, L., Al-Dhfyan, A., Agouni, A., Zeidan, A., et al. (2022). Epigenetic regulation of cancer stem cells by the aryl hydrocarbon receptor pathway. Semin. Cancer Biol. 83, 177–196. doi:10.1016/j.semcancer.2020.08.014
Al-Dhfyan, A., Alhoshani, A., and Korashy, H. M. (2017). Aryl hydrocarbon receptor/cytochrome P450 1A1 pathway mediates breast cancer stem cells expansion through PTEN inhibition and β-Catenin and Akt activation. Mol. Cancer 16 (1), 14. doi:10.1186/s12943-016-0570-y
Ala, M. (2021). The footprint of kynurenine pathway in every cancer: A new target for chemotherapy. Eur. J. Pharmacol. 896, 896173921. doi:10.1016/j.ejphar.2021.173921
Ali, S., and Lazennec, G. (2007). Chemokines: Novel targets for breast cancer metastasis. Cancer Metastasis Rev. 26 (3-4), 401–420. doi:10.1007/s10555-007-9073-z
Amadou, A., Praud, D., Coudon, T., Deygas, F., Grassot, L., Faure, E., et al. (2021). Risk of breast cancer associated with long-term exposure to benzo[a]pyrene (BaP) air pollution: Evidence from the French E3N cohort study. Environ. Int. 149, 106399. doi:10.1016/j.envint.2021.106399
Bankoti, J., Rase, B., Simones, T., and Shepherd, D. M. (2010). Functional and phenotypic effects of AhR activation in inflammatory dendritic cells. Toxicol. Appl. Pharmacol. 246 (1-2), 18–28. doi:10.1016/j.taap.2010.03.013
Bekki, K., Vogel, H., Li, W., Ito, T., Sweeney, C., Haarmann-Stemmann, T., et al. (2015). The aryl hydrocarbon receptor (AhR) mediates resistance to apoptosis induced in breast cancer cells. Pestic. Biochem. Physiol. 120, 5–13. doi:10.1016/j.pestbp.2014.12.021
Belton, K. R., Tian, Y., Zhang, L., Anitha, M., Smith, P. B., Perdew, G. H., et al. (2018). Metabolomics reveals aryl hydrocarbon receptor activation induces liver and mammary gland metabolic dysfunction in lactating mice. J. Proteome Res. 17 (4), 1375–1382. doi:10.1021/acs.jproteome.7b00709
Ben-Baruch, A. (2003). Host microenvironment in breast cancer development: Inflammatory cells, cytokines and chemokines in breast cancer progression: Reciprocal tumor-microenvironment interactions. Breast Cancer Res. 5 (1), 31–36. doi:10.1186/bcr554
Benoit, L., Jornod, F., Zgheib, E., Tomkiewicz, C., Koual, M., Coustillet, T., et al. (2022). Adverse outcome pathway from activation of the AhR to breast cancer-related death. Environ. Int. 165, 107323. doi:10.1016/j.envint.2022.107323
Benson, J. M., and Shepherd, D. M. (2011). Dietary ligands of the aryl hydrocarbon receptor induce anti-inflammatory and immunoregulatory effects on murine dendritic cells. Toxicol. Sci. 124 (2), 327–338. doi:10.1093/toxsci/kfr249
Bieche, I., Chavey, C., Andrieu, C., Busson, M., Vacher, S., Le Corre, L., et al. (2007). CXC chemokines located in the 4q21 region are up-regulated in breast cancer. Endocr. Relat. Cancer 14 (4), 1039–1052. doi:10.1677/erc.1.01301
Binnewies, M., Roberts, E. W., Kersten, K., Chan, V., Fearon, D. F., Merad, M., et al. (2018). Understanding the tumor immune microenvironment (TIME) for effective therapy. Nat. Med. 24 (5), 541–550. doi:10.1038/s41591-018-0014-x
Birnbaum, L. S., and Fenton, S. E. (2003). Cancer and developmental exposure to endocrine disruptors. Environ. Health Perspect. 111 (4), 389–394. doi:10.1289/ehp.5686
Boissiere-Michot, F., Jacot, W., Fraisse, J., Gourgou, S., Timaxian, C., and Lazennec, G. (2020). Prognostic value of CXCR2 in breast cancer. Cancers (Basel) 12 (8), 2076. doi:10.3390/cancers12082076
Boissiere-Michot, F., Jacot, W., Massol, O., Mollevi, C., and Lazennec, G. (2021). CXCR2 levels correlate with immune infiltration and a better prognosis of triple-negative breast cancers. Cancers (Basel) 13 (10), 2328. doi:10.3390/cancers13102328
Boonen, I., Van Heyst, A., Van Langenhove, K., Van Hoeck, E., Mertens, B., Denison, M. S., et al. (2020). Assessing the receptor-mediated activity of PAHs using AhR-ERα- and PPARγ- CALUX bioassays. Food Chem. Toxicol. 145, 111602. doi:10.1016/j.fct.2020.111602
Brody, J. G., Moysich, K. B., Humblet, O., Attfield, K. R., Beehler, G. P., and Rudel, R. A. (2007). Environmental pollutants and breast cancer: Epidemiologic studies. Cancer 109 (12), 2667–2711. doi:10.1002/cncr.22655
Brooks, J., and Eltom, S. E. (2011). Malignant transformation of mammary epithelial cells by ectopic overexpression of the aryl hydrocarbon receptor. Curr. Cancer Drug Targets 11 (5), 654–669. doi:10.2174/156800911795655967
Brummer, G., Acevedo, D. S., Hu, Q., Portsche, M., Fang, W. B., Yao, M., et al. (2018). Chemokine signaling facilitates early-stage breast cancer survival and invasion through fibroblast-dependent mechanisms. Mol. Cancer Res. 16 (2), 296–308. doi:10.1158/1541-7786.MCR-17-0308
Campesato, L. F., Budhu, S., Tchaicha, J., Weng, C. H., Gigoux, M., Cohen, I. J., et al. (2020). Blockade of the AHR restricts a Treg-macrophage suppressive axis induced by L-Kynurenine. Nat. Commun. 11 (1), 4011. doi:10.1038/s41467-020-17750-z
Castañeda, A. R., Pinkerton, K. E., Bein, K. J., Magaña-Méndez, A., Yang, H. T., Ashwood, P., et al. (2018). Ambient particulate matter activates the aryl hydrocarbon receptor in dendritic cells and enhances Th17 polarization. Toxicol. Lett. 292, 85–96. doi:10.1016/j.toxlet.2018.04.020
Cha, Y. J., and Koo, J. S. (2020). Role of tumor-associated myeloid cells in breast cancer. Cells 9 (8), 1785. doi:10.3390/cells9081785
Chavey, C., Bibeau, F., Gourgou-Bourgade, S., Burlinchon, S., Boissiere, F., Laune, D., et al. (2007). Oestrogen receptor negative breast cancers exhibit high cytokine content. Breast Cancer Res. 9 (1), R15. doi:10.1186/bcr1648
Cheng, I., Tseng, C., Wu, J., Yang, J., Conroy, S. M., Shariff-Marco, S., et al. (2020). Association between ambient air pollution and breast cancer risk: The multiethnic cohort study. Int. J. Cancer 146 (3), 699–711. doi:10.1002/ijc.32308
Coffelt, S. B., Hughes, R., and Lewis, C. E. (2009). Tumor-associated macrophages: Effectors of angiogenesis and tumor progression. Biochim. Biophys. Acta 1796, 11–18. doi:10.1016/j.bbcan.2009.02.004
Creighton, C. J., Li, X., Landis, M., Dixon, J. M., Neumeister, V. M., Sjolund, A., et al. (2009). Residual breast cancers after conventional therapy display mesenchymal as well as tumor-initiating features. Proc. Natl. Acad. Sci. U. S. A. 106 (33), 13820–13825. doi:10.1073/pnas.0905718106
D'Amato, N. C., Rogers, T. J., Gordon, M. A., Greene, L. I., Cochrane, D. R., Spoelstra, N. S., et al. (2015). A TDO2-AhR signaling axis facilitates anoikis resistance and metastasis in triple-negative breast cancer. Cancer Res. 75 (21), 4651–4664. doi:10.1158/0008-5472.CAN-15-2011
Dahlem, C., Kado, S. Y., He, Y., Bein, K., Wu, D., Haarmann-Stemmann, T., et al. (2020). AHR signaling interacting with nutritional factors regulating the expression of markers in vascular inflammation and atherogenesis. Int. J. Mol. Sci. 21 (21), 8287. doi:10.3390/ijms21218287
Demicco, E. G., Kavanagh, K. T., Romieu-Mourez, R., Wang, X., Shin, S. R., Landesman-Bollag, E., et al. (2005). RelB/p52 NF-kappaB complexes rescue an early delay in mammary gland development in transgenic mice with targeted superrepressor IkappaB-alpha expression and promote carcinogenesis of the mammary gland. Mol. Cell. Biol. 25 (22), 10136–10147. doi:10.1128/MCB.25.22.10136-10147.2005
Dey, S., Mondal, A., DuHadaway, J. B., Sutanto-Ward, E., Laury-Kleintop, L. D., Thomas, S., et al. (2021). Ido1 signaling through GCN2 in a subpopulation of gr-1+ cells shifts the ifnγ/IL6 balance to promote neovascularization. Cancer Immunol. Res. 9, 514–528. doi:10.1158/2326-6066.CIR-20-0226
Diaz-Montero, C. M., Salem, M. L., Nishimura, M. I., Garrett-Mayer, E., Cole, D. J., and Montero, A. J. (2009). Increased circulating myeloid-derived suppressor cells correlate with clinical cancer stage, metastatic tumor burden, and doxorubicin-cyclophosphamide chemotherapy. Cancer Immunol. Immunother. 58 (1), 49–59. doi:10.1007/s00262-008-0523-4
Donovan, M. G., Selmin, O. I., and Romagnolo, D. F. (2018). Aryl hydrocarbon receptor diet and breast cancer risk. Yale J. Biol. Med. 91 (2), 105–127.
Dubrovska, A., Hartung, A., Bouchez, L. C., Walker, J. R., Reddy, V. A., Cho, C. Y., et al. (2012). CXCR4 activation maintains a stem cell population in tamoxifen-resistant breast cancer cells through AhR signalling. Br. J. Cancer 107 (1), 43–52. doi:10.1038/bjc.2012.105
Esbona, K., Inman, D., Saha, S., Jeffery, J., Schedin, P., Wilke, L., et al. (2016). COX-2 modulates mammary tumor progression in response to collagen density. Breast Cancer Res. 18 (1), 35. doi:10.1186/s13058-016-0695-3
Famta, P., Shah, S., Jain, N., Kumar, K. C., Bagasariya, D., Khatri, D. K., et al. (2022). Tumor-promoting aftermath post-chemotherapy: A focus on breast cancer. Life Sci. 310, 121125. doi:10.1016/j.lfs.2022.121125
Feng, J., Zheng, T., Hou, Z., Lv, C., Xue, A., Han, T., et al. (2020). Luteolin, an aryl hydrocarbon receptor ligand, suppresses tumor metastasis in vitro and in vivo. Oncol. Rep. 44 (5), 2231–2240. doi:10.3892/or.2020.7781
Freund, A., Chauveau, C., Brouillet, J. P., Lucas, A., Lacroix, M., Licznar, A., et al. (2003). IL-8 expression and its possible relationship with estrogen-receptor-negative status of breast cancer cells. Oncogene 22 (2), 256–265. doi:10.1038/sj.onc.1206113
Fridlender, Z. G., Sun, J., Kim, S., Kapoor, V., Cheng, G., Ling, L., et al. (2009). Polarization of tumor-associated neutrophil phenotype by TGF-beta: "N1" versus "N2" TAN. Cancer Cell 16 (3), 183–194. doi:10.1016/j.ccr.2009.06.017
Fritz, W. A., Lin, T. M., Cardiff, R. D., and Peterson, R. E. (2007). The aryl hydrocarbon receptor inhibits prostate carcinogenesis in TRAMP mice. Carcinogenesis 28 (2), 497–505. doi:10.1093/carcin/bgl179
Gamboa-Loira, B., López-Carrillo, L., Mar-Sánchez, Y., Stern, D., and Cebrián, M. E. (2022). Epidemiologic evidence of exposure to polycyclic aromatic hydrocarbons and breast cancer: A systematic review and meta-analysis. Chemosphere 290, 133237. doi:10.1016/j.chemosphere.2021.133237
Gearhart-Serna, L. M., Davis, J. B., Jolly, M. K., Jayasundara, N., Sauer, S. J., Di Giulio, R. T., et al. (2020). A polycyclic aromatic hydrocarbon-enriched environmental chemical mixture enhances AhR, antiapoptotic signaling and a proliferative phenotype in breast cancer cells. Carcinogenesis 41 (12), 1648–1659. doi:10.1093/carcin/bgaa047
Gnant, M., Mlineritsch, B., Stoeger, H., Luschin-Ebengreuth, G., Heck, D., Menzel, C., et al. (2011). Adjuvant endocrine therapy plus zoledronic acid in premenopausal women with early-stage breast cancer: 62-month follow-up from the ABCSG-12 randomised trial. Lancet. Oncol. 12 (7), 631–641. doi:10.1016/S1470-2045(11)70122-X
Gomez-Roca, C. A., Cassier, P. A., Italiano, A., Cannarile, M., Ries, C., Brillouet, A., et al. (2015). Phase I study of RG7155, a novel anti-CSF1R antibody, in patients with advanced/metastatic solid tumors. J. Clin. Oncol. 33, 3005. doi:10.1200/jco.2015.33.15_suppl.3005
Grunig, G., Marsh, L. M., Esmaeil, N., Jackson, K., Gordon, T., Reibman, J., et al. (2014). Perspective: Ambient air pollution: Inflammatory response and effects on the lung's vasculature. Pulm. Circ. 4 (1), 25–35. doi:10.1086/674902
Guarnieri, T. (2020). Aryl hydrocarbon receptor connects inflammation to breast cancer. Int. J. Mol. Sci. 21 (15), 5264. doi:10.3390/ijms21155264
Han, H., Davidson, L. A., Hensel, M., Yoon, G., Landrock, K., Allred, C., et al. (2021). Loss of aryl hydrocarbon receptor promotes colon tumorigenesis in ApcS580/+; KrasG12D/+ mice. Mol. Cancer Res. 19 (5), 771–783. doi:10.1158/1541-7786.MCR-20-0789
Hart, J. E., Chiuve, S. E., Laden, F., and Albert, C. M. (2014). Roadway proximity and risk of sudden cardiac death in women. Circulation 130 (17), 1474–1482. doi:10.1161/CIRCULATIONAHA.114.011489
Hezaveh, K., Shinde, R. S., Klotgen, A., Halaby, M. J., Lamorte, S., Ciudad, M. T., et al. (2022). Tryptophan-derived microbial metabolites activate the aryl hydrocarbon receptor in tumor-associated macrophages to suppress anti-tumor immunity. Immunity 55 (2), 324–340.e8. doi:10.1016/j.immuni.2022.01.006
Hwa Jung, K., Pitkowsky, Z., Argenio, K., Quinn, J. W., Bruzzese, J. M., Miller, R. L., et al. (2022). The effects of the historical practice of residential redlining in the United States on recent temporal trends of air pollution near New York City schools. Environ. Int. 169, 107551. doi:10.1016/j.envint.2022.107551
IARC Working Group on the Evaluation of Carcinogenic Risks to Humans (2010). Some non-heterocyclic polycyclic aromatic hydrocarbons and some related exposures. IARC Monogr. Eval. Carcinog. Risks Hum. 92, 1–853. PMID: 21141735; PMCID: PMC4781319.
Jin, U. H., Lee, S. O., Pfent, C., and Safe, S. (2014). The aryl hydrocarbon receptor ligand omeprazole inhibits breast cancer cell invasion and metastasis. BMC Cancer 14, 498. doi:10.1186/1471-2407-14-498
Joyce, J. A., and Pollard, J. W. (2009). Microenvironmental regulation of metastasis. Nat. Rev. Cancer 9 (4), 239–252. doi:10.1038/nrc2618
Katara, G. K., Kulshrestha, A., Schneiderman, S., Riehl, V., Ibrahim, S., and Beaman, K. D. (2020). Interleukin-22 promotes development of malignant lesions in a mouse model of spontaneous breast cancer. Mol. Oncol. 14 (1), 211–224. doi:10.1002/1878-0261.12598
Kay, J. E., Cardona, B., Rudel, R. A., Vandenberg, L. N., Soto, A. M., Christiansen, S., et al. (2022). Chemical effects on breast development, function, and cancer risk: Existing knowledge and new opportunities. Curr. Environ. Health Rep. doi:10.1007/s40572-022-00376-2
Kenison, J. E., Wang, Z., Yang, K., Snyder, M., Quintana, F. J., and Sherr, D. H. (2021). The aryl hydrocarbon receptor suppresses immunity to oral squamous cell carcinoma through immune checkpoint regulation. Proc. Natl. Acad. Sci. U. S. A. 118 (19), e2012692118. doi:10.1073/pnas.2012692118
Kim, D. W., Gazourian, L., Quadri, S. A., Romieu-Mourez, R., Sherr, D. H., and Sonenshein, G. E. (2000). The RelA NF-kappaB subunit and the aryl hydrocarbon receptor (AhR) cooperate to transactivate the c-myc promoter in mammary cells. Oncogene 19 (48), 5498–5506. doi:10.1038/sj.onc.1203945
Kim, E. Y., Choi, B., Kim, J. E., Park, S. O., Kim, S. M., and Chang, E. J. (2020). Interleukin-22 mediates the chemotactic migration of breast cancer cells and macrophage infiltration of the bone microenvironment by potentiating S1P/SIPR signaling. Cells 9 (1), 131. doi:10.3390/cells9010131
Kim, K., Kim, G., Kim, J. Y., Yun, H. J., Lim, S. C., and Choi, H. S. (2014). Interleukin-22 promotes epithelial cell transformation and breast tumorigenesis via MAP3K8 activation. Carcinogenesis 35 (6), 1352–1361. doi:10.1093/carcin/bgu044
Kingsley, S. L., Eliot, M. N., Whitsel, E. A., Wang, Y., Coull, B. A., Hou, L., et al. (2015). Residential proximity to major roadways and incident hypertension in post-menopausal women. Environ. Res. 142, 522–528. doi:10.1016/j.envres.2015.08.002
Kirwa, K., Eliot, M. N., Wang, Y., Adams, M. A., Morgan, C. G., Kerr, J., et al. (2014). Residential proximity to major roadways and prevalent hypertension among postmenopausal women: Results from the women's health initiative san diego cohort. J. Am. Heart Assoc. 3 (5), e000727. doi:10.1161/JAHA.113.000727
Kolluri, S. K., Jin, U. H., and Safe, S. (2017). Role of the aryl hydrocarbon receptor in carcinogenesis and potential as an anti-cancer drug target. Arch. Toxicol. 91 (7), 2497–2513. doi:10.1007/s00204-017-1981-2
Koual, M., Tomkiewicz, C., Guerrera, I. C., Sherr, D., Barouki, R., and Coumoul, X. (2021). Aggressiveness and metastatic potential of breast cancer cells Co-cultured with preadipocytes and exposed to an environmental pollutant dioxin: An in vitro and in vivo zebrafish study. Environ. Health Perspect. 129 (3), 37002. doi:10.1289/EHP7102
Kubli, S. P., Bassi, C., Roux, C., Wakeham, A., Göbl, C., Zhou, W., et al. (2019). AhR controls redox homeostasis and shapes the tumor microenvironment in BRCA1-associated breast cancer. Proc. Natl. Acad. Sci. U. S. A. 116 (9), 3604–3613. doi:10.1073/pnas.1815126116
Kubli, S. P., Bassi, C., Roux, C., Wakeham, A., Gobl, C., Zhou, W., et al. (2018). Residential proximity to major roadways and risk of incident ischemic stroke in NOMAS (the northern manhattan study). Stroke 49 (4), 835–841. doi:10.1161/STROKEAHA.117.019580
La Merrill, M., Harper, R., Birnbaum, L. S., Cardiff, R. D., and Threadgill, D. W. (2010). Maternal dioxin exposure combined with a diet high in fat increases mammary cancer incidence in mice. Environ. Health Perspect. 118 (5), 596–601. doi:10.1289/ehp.0901047
Lagunas-Rangel, F. A., Liu, W., and Schiöth, H. B. (2022). Can exposure to environmental pollutants Be associated with less effective chemotherapy in cancer patients? Int. J. Environ. Res. Public Health 19 (4), 2064. doi:10.3390/ijerph19042064
Large, C., and Wei, Y. (2017). Geographic variations in female breast cancer incidence in relation to ambient air emissions of polycyclic aromatic hydrocarbons. Environ. Sci. Pollut. Res. Int. 24 (21), 17874–17880. doi:10.1007/s11356-017-9395-5
Lazennec, G., and Lam, P. Y. (2016). Recent discoveries concerning the tumor - mesenchymal stem cell interactions. Biochim. Biophys. Acta 1866 (2), 290–299. doi:10.1016/j.bbcan.2016.10.004
Lazennec, G., and Richmond, A. (2010). Chemokines and chemokine receptors: New insights into cancer-related inflammation. Trends Mol. Med. 16 (3), 133–144. doi:10.1016/j.molmed.2010.01.003
Lee, D. G., Burstyn, I., Lai, A. S., Grundy, A., Friesen, M. C., Aronson, K. J., et al. (2019). Women's occupational exposure to polycyclic aromatic hydrocarbons and risk of breast cancer. Occup. Environ. Med. 76 (1), 22–29. doi:10.1136/oemed-2018-105261
Leek, R. D., Lewis, C. E., Whitehouse, R., Greenall, M., Clarke, J., and Harris, A. L. (1996). Association of macrophage infiltration with angiogenesis and prognosis in invasive breast carcinoma. Cancer Res. 56, 4625–4629.
Lew, B. J., Manickam, R., and Lawrence, B. P. (2011). Activation of the aryl hydrocarbon receptor during pregnancy in the mouse alters mammary development through direct effects on stromal and epithelial tissues. Biol. Reprod. 84, 1094–1102. doi:10.1095/biolreprod.110.087544
Li, X., Lewis, M. T., Huang, J., Gutierrez, C., Osborne, C. K., Wu, M. F., et al. (2008). Intrinsic resistance of tumorigenic breast cancer cells to chemotherapy. J. Natl. Cancer Inst. 100 (9), 672–679. doi:10.1093/jnci/djn123
Li, Z. D., Wang, K., Yang, X. W., Zhuang, Z. G., Wang, J. J., and Tong, X. W. (2014). Expression of aryl hydrocarbon receptor in relation to p53 status and clinicopathological parameters in breast cancer. Int. J. Clin. Exp. Pathol. 7 (11), 7931–7937.
Lichtiger, L., Rivera, J., Sahay, D., and Miller, R. L. (2021). Polycyclic aromatic hydrocarbons and mammary cancer risk: Does obesity matter too? J. Cancer Immunol. 3 (3), 154–162.
Lue, S. H., Wellenius, G. A., Wilker, E. H., Mostofsky, E., and Mittleman, M. A. (2013). Residential proximity to major roadways and renal function. J. Epidemiol. Community Health 67 (8), 629–634. doi:10.1136/jech-2012-202307
Mahmoud, S. M., Lee, A. H., Paish, E. C., Macmillan, R. D., Ellis, I. O., and Green, A. R. (2012). Tumour-infiltrating macrophages and clinical outcome in breast cancer. J. Clin. Pathol. 65, 159–163. doi:10.1136/jclinpath-2011-200355
Mancini, S. J. C., Balabanian, K., Corre, I., Gavard, J., Lazennec, G., Le Bousse-Kerdiles, M. C., et al. (2021). Deciphering tumor niches: Lessons from solid and hematological malignancies. Front. Immunol. 12, 766275. doi:10.3389/fimmu.2021.766275
Masih, M., Agarwal, S., Kaur, R., and Gautam, P. K. (2022). Role of chemokines in breast cancer. Cytokine 155, 155909. doi:10.1016/j.cyto.2022.155909
McKay, Z. P., Brown, M. C., and Gromeier, M. (2021). Aryl hydrocarbon receptor signaling controls CD155 expression on macrophages and mediates tumor immunosuppression. J. Immunol. 206 (6), 1385–1394. doi:10.4049/jimmunol.2000792
Minn, A. J., Gupta, G. P., Siegel, P. M., Bos, P. D., Shu, W., Giri, D. D., et al. (2005). Genes that mediate breast cancer metastasis to lung. Nature 436 (7050), 518–524. doi:10.1038/nature03799
Miyazaki, T., Chung, S., Sakai, H., Ohata, H., Obata, Y., Shiokawa, D., et al. (2022). Stemness and immune evasion conferred by the TDO2-AHR pathway are associated with liver metastasis of colon cancer. Cancer Sci. 113 (1), 170–181. doi:10.1111/cas.15182
Mohamed, H. T., Gadalla, R., El-Husseiny, N., Hassan, H., Wang, Z., Ibrahim, S. A., et al. (2018). Inflammatory breast cancer: Activation of the aryl hydrocarbon receptor and its target CYP1B1 correlates closely with Wnt5a/b-β-catenin signalling, the stem cell phenotype and disease progression. J. Adv. Res. 16, 75–86. doi:10.1016/j.jare.2018.11.006
Mordukhovich, I., Beyea, J., Herring, A. H., Hatch, M., Stellman, S. D., Teitelbaum, S. L., et al. (2016). Vehicular traffic-related polycyclic aromatic hydrocarbon exposure and breast cancer incidence: The long island breast cancer study project (LIBCSP). Environ. Health Perspect. 124 (1), 30–38. doi:10.1289/ehp.1307736
Morello-Frosch, R., and Lopez, R. (2006). The riskscape and the color line: Examining the role of segregation in environmental health disparities. Environ. Res. 102 (2), 181–196. doi:10.1016/j.envres.2006.05.007
Morello-Frosch, R., and Shenassa, E. D. (2006). The environmental "riskscape" and social inequality: Implications for explaining maternal and child health disparities. Environ. Health Perspect. 114 (8), 1150–1153. doi:10.1289/ehp.8930
Movahedi, K., Laoui, D., Gysemans, C., Baeten, M., Stangé, G., Van den Bossche, J., et al. (2010). Different tumor microenvironments contain functionally distinct subsets of macrophages derived from Ly6C(high) monocytes. Cancer Res. 70 (14), 5728–5739. doi:10.1158/0008-5472.CAN-09-4672
Mukhtar, R. A., Moore, A. P., Nseyo, O., Baehner, F. L., Au, A., et al. (2011a). Elevated PCNA+ tumor-associated macrophages in breast cancer are associated with early recurrence and non-Caucasian ethnicity. Breast Cancer Res. Treat. 130, 635–644. doi:10.1007/s10549-011-1646-4
Mukhtar, R. A., Nseyo, O., Campbell, M. J., and Esserman, L. J. (2011b). Tumor-associated macrophages in breast cancer as potential biomarkers for new treatments and diagnostics. Expert Rev. Mol. diagn. 11, 91–100. doi:10.1586/erm.10.97
Muller, A., Homey, B., Soto, H., Ge, N., Catron, D., Buchanan, M. E., et al. (2001). Involvement of chemokine receptors in breast cancer metastasis. Nature 410 (6824), 50–56. doi:10.1038/35065016
Murray, I. A., Patterson, A. D., and Perdew, G. H. (2014). Aryl hydrocarbon receptor ligands in cancer: Friend and foe. Nat. Rev. Cancer 14 (12), 801–814. doi:10.1038/nrc3846
Narasimhan, S., Stanford Zulick, E., Novikov, O., Parks, A. J., Schlezinger, J. J., Wang, Z., et al. (2018). Towards resolving the pro- and anti-tumor effects of the aryl hydrocarbon receptor. Int. J. Mol. Sci. 19 (5), 1388. doi:10.3390/ijms19051388
Narita, D., Seclaman, E., Anghel, A., Ilina, R., Cireap, N., Negru, S., et al. (2016). Altered levels of plasma chemokines in breast cancer and their association with clinical and pathological characteristics. Neoplasma 63 (1), 141–149. doi:10.4149/neo_2016_017
Neamah, W. H., Singh, N. P., Alghetaa, H., Abdulla, O. A., Chatterjee, S., Busbee, P. B., et al. (2019). AhR activation leads to massive mobilization of myeloid-derived suppressor cells with immunosuppressive activity through regulation of CXCR2 and MicroRNA miR-150-5p and miR-543-3p that target anti-inflammatory genes. J. Immunol. 203 (7), 1830–1844. doi:10.4049/jimmunol.1900291
Nie, J., Beyea, J., Bonner, M. R., Han, D., Vena, J. E., Rogerson, P., et al. (2007). Exposure to traffic emissions throughout life and risk of breast cancer: The western New York exposures and breast cancer (WEB) study. Cancer Causes Control 18 (9), 947–955. doi:10.1007/s10552-007-9036-2
Niehoff, N. M., Keil, A. P., Jones, R. R., Fan, S., Gierach, G. L., and White, A. J. (2020). Outdoor air pollution and terminal duct lobular involution of the normal breast. Breast Cancer Res. 22 (1), 100. doi:10.1186/s13058-020-01339-x
Nothdurft, S., Thumser-Henner, C., Breitenbücher, F., Okimoto, R. A., Dorsch, M., Opitz, C. A., et al. (2020). Functional screening identifies aryl hydrocarbon receptor as suppressor of lung cancer metastasis. Oncogenesis 9 (11), 102. doi:10.1038/s41389-020-00286-8
O'Donnell, E. F., Jang, H. S., Liefwalker, D. F., Kerkvliet, N. I., and Kolluri, S. K. (2021). Discovery and mechanistic characterization of a Select modulator of AhR-regulated transcription (SMAhRT) with anti-cancer effects. Apoptosis. 26 (5-6), 307–322. doi:10.1007/s10495-021-01666-0
O'Driscoll, C. A., Gallo, M. E., Fechner, J. H., Schauer, J. J., and Mezrich, J. D. (2019). Real-world PM extracts differentially enhance Th17 differentiation and activate the aryl hydrocarbon receptor (AHR). Toxicology 414, 14–26. doi:10.1016/j.tox.2019.01.002
O'Driscoll, C. A., and Mezrich, J. D. (2018). The aryl hydrocarbon receptor as an immune-modulator of atmospheric particulate matter-mediated autoimmunity. Front. Immunol. 9, 2833. doi:10.3389/fimmu.2018.02833
Orimo, A., Gupta, P. B., Sgroi, D. C., Arenzana-Seisdedos, F., Delaunay, T., Naeem, R., et al. (2005). Stromal fibroblasts present in invasive human breast carcinomas promote tumor growth and angiogenesis through elevated SDF-1/CXCL12 secretion. Cell 121 (3), 335–348. doi:10.1016/j.cell.2005.02.034
Ostrand-Rosenberg, S. (2016). Immune suppressive myeloid-derived suppressor cells in cancer. Encycl. Immunol. 4, 512–525.
Ouyang, L., Yan, B., Liu, Y., Mao, C., Wang, M., Liu, N., et al. (2020). The deubiquitylase UCHL3 maintains cancer stem-like properties by stabilizing the aryl hydrocarbon receptor. Signal Transduct. Target. Ther. 5 (1), 78. doi:10.1038/s41392-020-0181-3
Phillips, J. L., Löhr, C. V., Nguyen, B. D., Buermeyer, A. B., and Kolluri, S. K. (2022). Loss of the aryl hydrocarbon receptor increases tumorigenesis in p53-deficient mice. Toxicol. Appl. Pharmacol. 454, 116191. doi:10.1016/j.taap.2022.116191
Place, A. E., Jin Huh, S., and Polyak, K. (2011). The microenvironment in breast cancer progression: Biology and implications for treatment. Breast Cancer Res. 13 (6), 227. doi:10.1186/bcr2912
Prud'homme, G. J., Glinka, Y., Toulina, A., Ace, O., Subramaniam, V., and Jothy, S. (2010). Breast cancer stem-like cells are inhibited by a non-toxic aryl hydrocarbon receptor agonist. PLoS One 5 (11), e13831. doi:10.1371/journal.pone.0013831
Puga, A., Barnes, S. J., Dalton, T. P., Chang, C. Y., Knudsen, E. S., and Maier, M. A. (2000). Aromatic hydrocarbon receptor interaction with the retinoblastoma protein potentiates repression of E2F-dependent transcription and cell cycle arrest. J. Biol. Chem. 275 (4), 2943–2950. doi:10.1074/jbc.275.4.2943
Qiu, S. Q., Waaijer, S., Zwager, M. C., de Vries, E. G., van der Vegt, B., and Schröder, C. P. (2018). Tumor-associated macrophages in breast cancer: Innocent bystander or important player? Cancer Treat. Rev. 70, 178–189. doi:10.1016/j.ctrv.2018.08.010
Razmkhah, M., Jaberipour, M., Hosseini, A., Safaei, A., Khalatbari, B., and Ghaderi, A. (2010). Expression profile of IL-8 and growth factors in breast cancer cells and adipose-derived stem cells (ASCs) isolated from breast carcinoma. Cell. Immunol. 265 (1), 80–85. doi:10.1016/j.cellimm.2010.07.006
Romaniuk, А., Lyndin, M., Sikora, V., Lyndina, Y., Romaniuk, S., and Sikora, K. (2017). Heavy metals effect on breast cancer progression. J. Occup. Med. Toxicol. 12, 32. doi:10.1186/s12995-017-0178-1
Rowland, L. K., Campbell, P. S., Mavingire, N., Wooten, J. V., McLean, L., Zylstra, D., et al. (2019). Putative tumor suppressor cytoglobin promotes aryl hydrocarbon receptor ligand-mediated triple negative breast cancer cell death. J. Cell. Biochem. 120 (4), 6004–6014. doi:10.1002/jcb.27887
Rückerl, R., Greven, S., Ljungman, P., Aalto, P., Antoniades, C., Bellander, T., et al. AIRGENE Study Group (2007). Air pollution and inflammation (interleukin-6, C-reactive protein, fibrinogen) in myocardial infarction survivors. Environ. Health Perspect. 115 (7), 1072–1080. doi:10.1289/ehp.10021
Safarzadeh, E., Hashemzadeh, S., Duijf, P. H. G., Mansoori, B., Khaze, V., Mohammadi, A., et al. (2019). Circulating myeloid-derived suppressor cells: An independent prognostic factor in patients with breast cancer. J. Cell. Physiol. 234 (4), 3515–3525. doi:10.1002/jcp.26896
Safe, S., Cheng, Y., and Jin, U. H. (2017). The aryl hydrocarbon receptor (AhR) as a drug target for cancer chemotherapy. Curr. Opin. Toxicol. 2, 24–29. doi:10.1016/j.cotox.2017.01.012
Saito, N., Kanno, Y., Yamashita, N., Degawa, M., Yoshinari, K., and Nemoto, K. (2021). The differential selectivity of aryl hydrocarbon receptor (AHR) agonists towards AHR-dependent suppression of mammosphere formation and gene transcription in human breast cancer cells. Biol. Pharm. Bull. 44 (4), 571–578. doi:10.1248/bpb.b20-00961
Sarić, N., Selby, M., Ramaswamy, V., Kool, M., Stockinger, B., Hogstrand, C., et al. (2020). The AHR pathway represses TGFβ-SMAD3 signalling and has a potent tumour suppressive role in SHH medulloblastoma. Sci. Rep. 10 (1), 148. doi:10.1038/s41598-019-56876-z
Shan, N. L., Shin, Y., Yang, G., Furmanski, P., and Suh, N. (2021). Breast cancer stem cells: A review of their characteristics and the agents that affect them. Mol. Carcinog. 60 (2), 73–100. doi:10.1002/mc.23277
Shen, J., Liao, Y., Hopper, J. L., Goldberg, M., Santella, R. M., and Terry, M. B. (2017). Dependence of cancer risk from environmental exposures on underlying genetic susceptibility: An illustration with polycyclic aromatic hydrocarbons and breast cancer. Br. J. Cancer 116 (9), 1229–1233. doi:10.1038/bjc.2017.81
Simones, T., and Shepherd, D. M. (2011). Consequences of AhR activation in steady-state dendritic cells. Toxicol. Sci. 119 (2), 293–307. doi:10.1093/toxsci/kfq354
Song, R., and Struhl, K. (2021). S100A8/S100A9 cytokine acts as a transcriptional coactivator during breast cellular transformation. Sci. Adv. 7 (1), eabe5357. doi:10.1126/sciadv.abe5357
Soria, G., and Ben-Baruch, A. (2008). The inflammatory chemokines CCL2 and CCL5 in breast cancer. Cancer Lett. 267 (2), 271–285. doi:10.1016/j.canlet.2008.03.018
Stanford, E. A., Wang, Z., Novikov, O., Mulas, F., Landesman-Bollag, E., Monti, S., et al. (2016). The role of the aryl hydrocarbon receptor in the development of cells with the molecular and functional characteristics of cancer stem-like cells. BMC Biol. 14, 20. doi:10.1186/s12915-016-0240-y
Stuart, A. L., Mudhasakul, S., and Sriwatanapongse, W. (2009). The social distribution of neighborhood-scale air pollution and monitoring protection. J. Air Waste Manag. Assoc. 59 (5), 591–602. doi:10.3155/1047-3289.59.5.591
Swope, C. B., Hernández, D., and Cushing, L. J. (2022). The relationship of historical redlining with present-day neighborhood environmental and health outcomes: A scoping review and conceptual model. J. Urban Health, 1–25. doi:10.1007/s11524-022-00665-z
Takenaka, M. C., Gabriely, G., Rothhammer, V., Mascanfroni, I. D., Wheeler, M. A., Chao, C. C., et al. (2019). Control of tumor-associated macrophages and T cells in glioblastoma via AHR and CD39. Nat. Neurosci. 22 (5), 729–740. doi:10.1038/s41593-019-0370-y
Tan, K. P., Wang, B., Yang, M., Boutros, P. C., Macaulay, J., Xu, H., et al. (2010). Aryl hydrocarbon receptor is a transcriptional activator of the human breast cancer resistance protein (BCRP/ABCG2). Mol. Pharmacol. 78 (2), 175–185. doi:10.1124/mol.110.065078
Therachiyil, L., Hussein, O. J., Uddin, S., and Korashy, H. M. (2022). Regulation of the aryl hydrocarbon receptor in cancer and cancer stem cells of gynecological malignancies: An update on signaling pathways. Semin. Cancer Biol. 86 (3), 1186–1202. doi:10.1016/j.semcancer.2022.10.003
Timaxian, C., Vogel, C. F. A., Orcel, C., Vetter, D., Durochat, C., Chinal, C., et al. (2021). Pivotal role for Cxcr2 in regulating tumor-associated neutrophil in breast cancer. Cancers (Basel) 13 (11), 2584. doi:10.3390/cancers13112584
Timperi, E., Gueguen, P., Molgora, M., Magagna, I., Kieffer, Y., Lopez-Lastra, S., et al. (2022). Lipid-associated macrophages are induced by cancer-associated fibroblasts and mediate immune suppression in breast cancer. Cancer Res. 82 (18), 3291–3306. doi:10.1158/0008-5472.CAN-22-1427
Trombino, A. F., Near, R. I., Matulka, R. A., Yang, S., Hafer, L. J., Toselli, P. A., et al. (2000). Expression of the aryl hydrocarbon receptor/transcription factor (AhR) and AhR-regulated CYP1 gene transcripts in a rat model of mammary tumorigenesis. Breast Cancer Res. Treat. 63 (2), 117–131. doi:10.1023/a:1006443104670
Ugel, S., De Sanctis, F., Mandruzzato, S., and Bronte, V. (2015). Tumor-induced myeloid deviation: When myeloid-derived suppressor cells meet tumor-associated macrophages. J. Clin. Invest. 125, 3365–3376. doi:10.1172/JCI80006
Vacher, S., Castagnet, P., Chemlali, W., Lallemand, F., Meseure, D., Pocard, M., et al. (2018). High AHR expression in breast tumors correlates with expression of genes from several signaling pathways namely inflammation and endogenous tryptophan metabolism. PLoS One 13 (1), e0190619. doi:10.1371/journal.pone.0190619
Vogel, C., and Abel, J. (1995). Effect of 2, 3, 7, 8-tetrachlorodibenzo-p-dioxin on growth factor expression in the human breast cancer cell line MCF-7. Arch. Toxicol. 69 (4), 259–265. doi:10.1007/s002040050168
Vogel, C., Boerboom, A. M., Baechle, C., El-Bahay, C., Kahl, R., Degen, G. H., et al. (2000). Regulation of prostaglandin endoperoxide H synthase-2 induction by dioxin in rat hepatocytes: Possible c-Src-mediated pathway. Carcinogenesis 21 (12), 2267–2274. doi:10.1093/carcin/21.12.2267
Vogel, C. F., Chang, W. L., Kado, S., McCulloh, K., Vogel, H., Wu, D., et al. (2016). Transgenic overexpression of aryl hydrocarbon receptor repressor (AhRR) and AhR-mediated induction of CYP1A1, cytokines, and acute toxicity. Environ. Health Perspect. 124 (7), 1071–1083. doi:10.1289/ehp.1510194
Vogel, C. F., Goth, S. R., Dong, B., Pessah, I. N., and Matsumura, F. (2008). Aryl hydrocarbon receptor signaling mediates expression of indoleamine 2, 3-dioxygenase. Biochem. Biophys. Res. Commun. 375 (3), 331–335. doi:10.1016/j.bbrc.2008.07.156
Vogel, C. F., Khan, E. M., Leung, P. S., Gershwin, M. E., Chang, W. L., Wu, D., et al. (2014). Cross-talk between aryl hydrocarbon receptor and the inflammatory response: A role for nuclear factor-κB. J. Biol. Chem. 289 (3), 1866–1875. doi:10.1074/jbc.M113.505578
Vogel, C. F., Li, W., Wu, D., Miller, J. K., Sweeney, C., Lazennec, G., et al. (2011). Interaction of aryl hydrocarbon receptor and NF-κB subunit RelB in breast cancer is associated with interleukin-8 overexpression. Arch. Biochem. Biophys. 512 (1), 78–86. doi:10.1016/j.abb.2011.05.011
Vogel, C. F., Sciullo, E., Park, S., Liedtke, C., Trautwein, C., and Matsumura, F. (2004). Dioxin increases C/EBPbeta transcription by activating cAMP/protein kinase A. J. Biol. Chem. 279 (10), 8886–8894. doi:10.1074/jbc.M310190200
Vogel, C. F., Sciullo, E., Li, W., Wong, P., Lazennec, G., and Matsumura, F. (2007). RelB, a new partner of aryl hydrocarbon receptor-mediated transcription. Mol. Endocrinol. 21 (12), 2941–2955. doi:10.1210/me.2007-0211
Vogel, C. F., Wu, D., Goth, S. R., Baek, J., Lollies, A., Domhardt, R., et al. (2013). Aryl hydrocarbon receptor signaling regulates NF-κB RelB activation during dendritic-cell differentiation. Immunol. Cell Biol. 91 (9), 568–575. doi:10.1038/icb.2013.43
Vogel, C. F. A., Lazennec, G., Kado, S. Y., Dahlem, C., He, Y., Castaneda, A., et al. (2021). Targeting the aryl hydrocarbon receptor signaling pathway in breast cancer development. Front. Immunol. 12, 625346. doi:10.3389/fimmu.2021.625346
Vogel, C. F. A., Van Winkle, L. S., Esser, C., and Haarmann-Stemmann, T. (2020). The aryl hydrocarbon receptor as a target of environmental stressors - implications for pollution mediated stress and inflammatory responses. Redox Biol. 34, 101530. doi:10.1016/j.redox.2020.101530
Voigt, C., May, P., Gottschlich, A., Markota, A., Wenk, D., Gerlach, I., et al. (2017). Cancer cells induce interleukin-22 production from memory CD4+ T cells via interleukin-1 to promote tumor growth. Proc. Natl. Acad. Sci. U. S. A. 114 (49), 12994–12999. doi:10.1073/pnas.1705165114
Vorderstrasse, B. A., Fenton, S. E., Bohn, A. A., Cundiff, J. A., and Lawrence, B. P. (2004). A novel effect of dioxin: Exposure during pregnancy severely impairs mammary gland differentiation. Toxicol. Sci. 78, 782248–782257. doi:10.1093/toxsci/kfh062
Wang, S., Yao, Y., Yao, M., Fu, P., and Wang, W. (2018). Interleukin-22 promotes triple negative breast cancer cells migration and paclitaxel resistance through JAK-STAT3/MAPKs/AKT signaling pathways. Biochem. Biophys. Res. Commun. 503 (3), 1605–1609. doi:10.1016/j.bbrc.2018.07.088
Wang, Z., Monti, S., and Sherr, D. (2017). The diverse and important contributions of the AHR to cancer and cancer immunity. Curr. Opin. Toxicol. 2, 93–102. doi:10.1016/j.cotox.2017.01.008
Wang, Z., Snyder, M., Kenison, J. E., Yang, K., Lara, B., Lydell, E., et al. (2020). How the AHR became important in cancer: The role of chronically active AHR in cancer aggression. Int. J. Mol. Sci. 22 (1), 387. doi:10.3390/ijms22010387
Warner, M., Eskenazi, B., Mocarelli, P., Gerthoux, P. M., Samuels, S., Needham, L., et al. (2002). Serum dioxin concentrations and breast cancer risk in the Seveso Women's Health Study. Environ. Health Perspect. 110 (7), 625–628. doi:10.1289/ehp.02110625
Wong, P. S., Li, W., Vogel, C. F., and Matsumura, F. (2009). Characterization of MCF mammary epithelial cells overexpressing the Arylhydrocarbon receptor (AhR). BMC Cancer 9, 234. doi:10.1186/1471-2407-9-234
Wu, W., Muller, R., Berhane, K., Fruin, S., Liu, F., Jaspers, I., et al. (2014). Inflammatory response of monocytes to ambient particles varies by highway proximity. Am. J. Respir. Cell Mol. Biol. 51 (6), 802–809. doi:10.1165/rcmb.2013-0265OC
Wu, Z., Yan, L., Lin, J., Ke, K., and Yang, W. (2021). Constitutive TDO2 expression promotes liver cancer progression by an autocrine IL-6 signaling pathway. Cancer Cell Int. 21 (1), 538. doi:10.1186/s12935-021-02228-9
Xue, P., Fu, J., and Zhou, Y. (2018). The aryl hydrocarbon receptor and tumor immunity. Front. Immunol. 9, 286. doi:10.3389/fimmu.2018.00286
Yamashita, N., Taga, C., Ozawa, M., Kanno, Y., Sanada, N., and Kizu, R. (2022). Camalexin, an indole phytoalexin, inhibits cell proliferation, migration, and mammosphere formation in breast cancer cells via the aryl hydrocarbon receptor. J. Nat. Med. 76 (1), 110–118. doi:10.1007/s11418-021-01560-8
Yamashita, N., Yoshizuka, A., Kase, A., Ozawa, M., Taga, C., Sanada, N., et al. (2021). Activation of the aryl hydrocarbon receptor by 3-methylcholanthrene, but not by indirubin, suppresses mammosphere formation via downregulation of CDC20 expression in breast cancer cells. Biochem. Biophys. Res. Commun. 570, 131–136. doi:10.1016/j.bbrc.2021.07.047
Yan, B., Liu, S., Shi, Y., Liu, N., Chen, L., Wang, X., et al. (2018). Activation of AhR with nuclear IKKα regulates cancer stem-like properties in the occurrence of radioresistance. Cell Death Dis. 9 (5), 490. doi:10.1038/s41419-018-0542-9
Yu, J., Du, W., Yan, F., Wang, Y., Li, H., Cao, S., et al. (2013). Myeloid-derived suppressor cells suppress antitumor immune responses through Ido expression and correlate with lymph node metastasis in patients with breast cancer. J. Immunol. 190 (7), 3783–3797. doi:10.4049/jimmunol.1201449
Yu, J., Wang, Y., Yan, F., Zhang, P., Li, H., Zhao, H., et al. (2014). Noncanonical NF-κB activation mediates STAT3-stimulated Ido upregulation in myeloid-derived suppressor cells in breast cancer. J. Immunol. 193 (5), 2574–2586. doi:10.4049/jimmunol.1400833
Yuan, W., Fulgar, C. C., Sun, X., Vogel, C. F. A., Wu, C. W., Zhang, Q., et al. (2020). In vivo and in vitro inflammatory responses to fine particulate matter (PM2.5) from China and California. Toxicol. Lett. 328, 52–60. doi:10.1016/j.toxlet.2020.04.010
Zattoni, I. F., Delabio, L. C., Dutra, J. P., Kita, D. H., Scheiffer, G., Hembecker, M., et al. (2022). Targeting breast cancer resistance protein (BCRP/ABCG2): Functional inhibitors and expression modulators. Eur. J. Med. Chem. 237, 114346. doi:10.1016/j.ejmech.2022.114346
Zhang, Q., Qin, J., Zhong, L., Gong, L., Zhang, B., Zhang, Y., et al. (2015). CCL5-Mediated Th2 immune polarization promotes metastasis in luminal breast cancer. Cancer Res. 75 (20), 4312–4321. doi:10.1158/0008-5472.CAN-14-3590
Zhang, Q. W., Liu, L., Gong, C. Y., Shi, H. S., Zeng, Y. H., Wang, X. Z., et al. (2012). Prognostic significance of tumor-associated macrophages in solid tumor: A meta-analysis of the literature. PLoS ONE 7, e50946. doi:10.1371/journal.pone.0050946
Zhao, F., Hoechst, B., Duffy, A., Gamrekelashvili, J., Fioravanti, S., Manns, M. P., et al. (2012). S100A9 a new marker for monocytic human myeloid-derived suppressor cells. Immunology 136 (2), 176–183. doi:10.1111/j.1365-2567.2012.03566.x
Zhao, S., Kanno, Y., Nakayama, M., Makimura, M., Ohara, S., and Inouye, Y. (2012). Activation of the aryl hydrocarbon receptor represses mammosphere formation in MCF-7 cells. Cancer Lett. 317 (2), 192–198. doi:10.1016/j.canlet.2011.11.025
Zhao, S., Ohara, S., Kanno, Y., Midorikawa, Y., Nakayama, M., Makimura, M., et al. (2013). HER2 overexpression-mediated inflammatory signaling enhances mammosphere formation through up-regulation of aryl hydrocarbon receptor transcription. Cancer Lett. 330 (1), 41–48. doi:10.1016/j.canlet.2012.11.021
Zhao, X., Qu, J., Sun, Y., Wang, J., Liu, X., Wang, F., et al. (2017). Prognostic significance of tumor-associated macrophages in breast cancer: A meta-analysis of the literature. Oncotarget 8, 30576–30586. doi:10.18632/oncotarget.15736
Zhou, S., Schuetz, J. D., Bunting, K. D., Colapietro, A. M., Sampath, J., Morris, J. J., et al. (2001). The ABC transporter Bcrp1/ABCG2 is expressed in a wide variety of stem cells and is a molecular determinant of the side-population phenotype. Nat. Med. 7 (9), 1028–1034. doi:10.1038/nm0901-1028
Zou, E., and Matsumura, F. (2003). Long-term exposure to beta-hexachlorocyclohexane (beta-HCH) promotes transformation and invasiveness of MCF-7 human breast cancer cells. Biochem. Pharmacol. 66 (5), 831–840. doi:10.1016/s0006-2952(03)00394-0
Keywords: AhR, breast cancer, air pollution, particulate matter, environmental injustice, macrophages, tumor microenvironment, tumor promotion
Citation: Sweeney C, Lazennec G and Vogel CFA (2022) Environmental exposure and the role of AhR in the tumor microenvironment of breast cancer. Front. Pharmacol. 13:1095289. doi: 10.3389/fphar.2022.1095289
Received: 11 November 2022; Accepted: 05 December 2022;
Published: 15 December 2022.
Edited by:
Amir Shadboorestan, Tarbiat Modares University, IranReviewed by:
Rridha Oueslati, University Carthage—Sciences Faculty of Bizerte, TunisiaDavid H. Sherr, Boston University, United States
Copyright © 2022 Sweeney, Lazennec and Vogel. This is an open-access article distributed under the terms of the Creative Commons Attribution License (CC BY). The use, distribution or reproduction in other forums is permitted, provided the original author(s) and the copyright owner(s) are credited and that the original publication in this journal is cited, in accordance with accepted academic practice. No use, distribution or reproduction is permitted which does not comply with these terms.
*Correspondence: Christoph F. A. Vogel, Y2Z2b2dlbEB1Y2RhdmlzLmVkdQ==