- 1Histology Department, Faculty of Veterinary Medicine, Benha University, Benha, Egypt
- 2Cytology and Histology Department, Faculty of Veterinary Medicine, Suez Canal University, Ismailia, Egypt
- 3Pathology Department, Collage of Veterinary Medicine, King Faisal University, Al-Hofuf, Saudi Arabia
- 4Clinical Pharmacology Department, Faculty of Medicine, Benha University, Benha, Egypt
- 5Clinical Pathology Department, Faculty of Veterinary Medicine, Benha University, Benha, Egypt
Lead toxicity is a common occupational and environmental health hazard that exerts many toxic effects on animals and humans, including immunotoxicity. Curcumin (CUR) and cinnamon (CIN) are common medicinal herbs with immunostimulatory and antioxidant properties. Therefore, this study investigated the protective effect of curcumin and cinnamon against lead acetate (LA)-induced splenotoxicity in rats via hemato-biochemical, immunological, oxidative stress marker, CYP-2E1 expression, histological, and immunohistological evaluations. Four groups of seven rats each were used: the control group received corn oil as a vehicle; the lead acetate group received (100 mg/kg), the CUR + LA group received curcumin (400 mg/kg) plus lead acetate, and the CIN + LA group received cinnamon (200 mg/kg) plus lead acetate orally for 1 month. LA exposure induced macrocytic hypochromic anemia, leukocytosis, neutrophilia, monocytosis, and lymphopenia. Additionally, significant elevations in serum iron, ferritin levels, and transferrin saturation percentage with significant decline of total and unsaturated iron binding capacities (TIBC and UIBC), transferrin, and immunoglobulin G and M levels were recorded. In addition, lead acetate significantly upregulated splenic CYP-2E1 expression, that was evident by significant depletion of reduced glutathione (GSH) activity and elevation of malondihyde (MDA), nitric oxide (NO), and protein carbonyl (PC) concentrations in the spleen. Histologically, hyperplasia of lymphoid follicles, hemosiderin deposition, and disturbance of CD3 and CD68 immuno-expressions were evident in the spleen from the lead acetate group. However, curcumin and cinnamon administration restored the hemato-biochemical, immunological, and oxidative stress parameters as well as histological and immunohistological pictures toward normalcy. In conclusion, curcumin and cinnamon can partially ameliorate LA-induced oxidative damage in the spleen, possibly through their antioxidant, immunomodulatory, and gene-regulating activities.
1 Introduction
The spleen contains about one-fourth of the lymphocytes in the mammalian body, so it is considered the largest secondary immune organ (Cesta, 2006). Histologically, splenic parenchyma is formed of red and white pulps. The former is a blood filter and storage site for iron, erythrocytes, and platelets. However, lymphocytes of the white pulp initiate immune responses to blood-borne antigens (Lewis et al., 2019).
Lead is a common occupational and environmental pollutant that has many toxic effects on animals and humans, including immunotoxicity (Aldahmash and El-Nager, 2016). Lead is a widely used heavy metal in various industrial activities, like the production of paints, batteries, ceramics, motor and electric vehicles, electronic technologies, pipes, tobacco smoke, and printing of books (Mishra et al., 2003).
Contaminated drinking water, food, and air are considered the main sources for lead poisoning (Ferreyra et al., 2009). About 30%–40% of inhaled lead is directly absorbed into the bloodstream, while absorption of ingested lead varies depending on age and nutritional status (Patrick, 2006). About 95% of circulating lead is present in erythrocytes, interfering with their proper functions (Chwalba et al., 2018).
In spite of the exact mechanism by which lead causes immunotoxicity still remaining unknown, some studies have reported its adverse effects.
The target immune cells for lead are T cells (Mishra, 2009) and macrophages (Chen et al., 1997). Exposure to lead impairs the lymphocyte’s function (Dyatlov and Lawrence, 2002) and production of cytokines (Chen et al., 2004) and immunoglobulins (Dietert and Piepenbrink, 2006). Generally, lead induces cellular injury and oxidative stress. It causes an imbalance between free radical generation and the antioxidant defense system (Flora et al., 2007), leading to generation of reactive oxygen species (ROS), including hydrogen peroxide and singlet oxygen, resulting in damage to DNA and membrane lipids (Alhusaini et al., 2019) and damage of mitochondria and lysosomes (Zarei et al., 2017). Lead also interrupts enzyme activation, sulfhydryl protein synthesis, and calcium homeostasis, in addition to decreasing trace mineral absorption and antioxidant reserves in the body (Lynpatrick, 2006).
Recently, significant attention has been paid to the use of herbal products rather than synthetic ones in attenuating the toxic impact of pollutants (Alkhedaide, 2016; Farouk et al., 2021a; 2021b). Among these herbs are curcumin (CUR) and cinnamon (CIN).
CUR is a food spice and coloring agent that has been used in traditional herbal medicines for many centuries (Yadollahi and Zargaran, 2019). Its therapeutic properties are owing to its anti-oxidant, anti-inflammatory, anti-cancer, anti-fibrotic, anti-diabetic, and anti-neurotoxic effects (El-Maddawy and El- Sayed 2018; Emam and El-Shafey 2018; Abdelhamid et al., 2020). CUR reduces oxidative stress via scavengers of ROS, reduction of lipid peroxidation, upregulation of antioxidant protein biosynthesis, and inhibition of inflammatory cytokines (Hosseini and Hosseinzadeh, 2018). Additionally, it can chelate oxidative metal ions, including lead, which consequently protects and repairs the cells (Soliman et al., 2015). Although several works of literature have described the protective role of CUR against LA-induced toxicity of the liver (García-Niño and Pedraza-Chaverri, 2014; Soliman et al., 2015; Abdelhamid et al., 2020), kidney (Soliman et al., 2015), and testis (Sudjarwo et al., 2017; Abdelhamid et al., 2020), there is a shortage of studies on the spleen.
On the other hand, CIN is an herbal medicinal plant that is a famous condiment in many foods. Cinnamaldehyde is the main active principle of CIN, which has anti-inflammatory, anti-oxidant, and anti-diabetic effects (Subash-Babu et al., 2014; Lee et al., 2018). In addition, it can be used as an antidote against natural or chemical toxicity (Dorri et al., 2018). CIN is considered a stimulator for antioxidant enzyme activities (Kim et al., 2006), along with acting as an ROS scavenger, metal chelator, and enzyme modulator (Rice-Evans et al., 1997). To our knowledge, the protective effect of CIN against LA-induced testicular toxicity was proven (Elgawish and Abdelrazek, 2014). However, the effect of CIN on LA-induced splenotoxicity has not been studied.
Depending on this background, our study was designed to evaluate the potential protective impact of CUR and CIN against splenic toxicity induced by LA exposure. Hematological, serum iron and immunoglobulin parameter, oxidative stress marker, CYP-2E1 expression, splenic histology, and CD3 and CD68 immunohistochemical expressions were evaluated.
2 Materials and methods
2.1 Chemicals
LA and CUR were obtained from El-Gomhoria Company, Egypt. CIN barks were purchased from a local market, El-Azhar, Cairo, Egypt. All these materials were freshly prepared before use by dissolving LA and CIN in distilled water (Morgan et al., 2014; Abd Elrasoul et al., 2020), while CUR, a bright yellow powder, was dissolved in corn oil (Sudjarwo et al., 2017) due to its insolubility in water. Diagnostic kits for estimation of malondihyde (MDA), nitric oxide (NO), and reduced glutathione (GSH) were obtained from Biodiagnostic Company (Cairo, Egypt). Protein carbonyl (PC), immunoglobulin G (IgG), and immunoglobulin M (IgM) were purchased from MyBioSource Company (Giza, Egypt). Meanwhile, iron, ferritin, and total iron binding capacity were obtained from Spectrum Company (Germany).
2.2 Preparation of CIN aqueous extract
CIN barks were identified by the Faculty of Agriculture, Benha University, Egypt. It was prepared as described by Morgan et al. (2014). The dried CIN was ground into a fine powder and then soaked in distilled water (10 gm/100 ml) for 2 h at 90°C. Next, the solution was filtered, and the filtrate was dehydrated in a hot-air oven at 80°C overnight to obtain a dark reddish brown dry extract. The yield concentration of CIN aqueous solution was about 20% (w/w).
2.3 Animals
Twenty-eight mature male Albino rats (weighing 180–200 g) were obtained from the animal house, Faculty of Veterinary Medicine, Benha University, Egypt. The rats were kept under an appropriate environment of temperature (25 ± 2 C), humidity (60%–70%), light (12-h dark/light cycles), and with free access to water and a commercial pellet diet.
2.4 Experimental design
Both experimental design and animal handling were ethically approved by the Committee of Research Ethics Board at the Faculty of Veterinary Medicine, Benha University, Egypt (Approval No. BUFVTM 17–03-22). After a 2-week acclimatization period, the rats were randomly divided into four groups (7 rats in each group) in separate cages. The first group served as control animals and received 0.5 ml corn oil, the vehicle of CUR; the second group received LA at a dose of 100 mg/kg (Abd Elrasoul et al., 2020); the third group received LA + CUR where CUR was given at a dose of 400 mg/kg (Sudjarwo et al., 2017) plus LA as in the second group; and the fourth group received LA + CIN where CIN was given at a dose of 200 mg/kg (Morgan et al., 2014) plus LA as in the second group. The experiment was extended for a month, and all animals received their treatments orally once daily using a gavage tube.
2.4.1 Blood collection, serum, and tissue preparations
After 24 h from the last treatment, blood samples were collected from the retro-orbital venus plexus of each animal and divided into two parts. The first part was collected into clean dry tubes containing EDTA for both erythrogram and leukogram including red blood cell count (RBC), hemoglobin concentration (Hb), packed corpuscular volume (PCV), mean corpuscular volume (MCV), mean corpuscular hemoglobin (MCH), mean corpuscular hemoglobin concentration (MCHC), total white blood cell count (WBC), and differential leukocytic count. Meanwhile, the second part of the blood was collected into plain tubes for serum separation, and blood was allowed to coagulate at room temperature for 20 min and then centrifuged at 5,000 rpm for 10 min. The separated sera were carefully collected and stored in deep freeze (−20°C) for biochemical assays.
The rats were killed by cervical decapitation under light anesthesia (ketamine–xylazine mixture, .15 ml/100 g BW/IP). A midline abdominal incision was performed to expose their viscera. The spleen of each rat was excised, cleaned from their surrounding tissues, washed with normal saline, and then prepared for oxidative stress parameter, detection of lead concentration, gene expression of CYP-2E1, and histological and immunohistochemical assessments. Small cross-sections of the spleen were fixed in 10% neutral buffered formalin for histological and immunohistochemical examinations; however, the rest of the spleen was wiped dry with a filter paper and quickly fragmented into small specimens. The portion was homogenized in potassium phosphate buffers (5 ml buffer of PH 7.4/0.5 g tissue), centrifuged at 5,000 rpm for 10 min at 4°C, and then the collected supernatant was stored at −20°C until further assessment of oxidative stress parameters. Another small spleen sample was preserved for gene expression of CYP-2E1 at −80°C. Other spleen specimens were used for detection of lead concentration.
2.5 Hematological analyses
The hematological analyses, including erythrogram and leukogram, were determined using an automatic cell counter (CLINDIAG-HA-VET, Belgium) according to Thrall et al. (2012).
2.6 Assessment of iron parameters
Serum samples were used for detection of iron parameters, including iron, total iron binding capacity (TIBC) (Fairbanks and Klee, 1987), and ferritin (Blackmore et al., 2008). Other iron parameters, including unsaturated iron binding capacity (UIBC), serum transferrin (Tf), and serum transferrin saturation % (TS%), were calculated as described by Burits and Burns (2014) and Fairbanks and Klee (1987).
2.7 Measurement of serum immunoglobulin
Serum samples were used for measurement of IgG and IgM according to the manufacturer’s instructions.
2.8 Evaluation of oxidative stress/antioxidant parameters
MDA, NO, PC, and GSH were measured in splenic tissue homogenates according to Ohkawa et al. (1979), Green et al. (1982), Levine et al. (1990), and Beutler et al. (1963), respectively.
2.9 Determination of lead content in spleen tissue
Lead concentration in splenic tissues was analyzed using the flame atomic absorption spectrophotometer as the method reported by Szkoda and Zmudzki (2005).
2.10 Quantitative analysis of gene expression of CYP-2E1 by real-time PCR (qRT-PCR)
Total cellular RNA was extracted from splenic tissue using an RNeasy Mini kit (Qiagen, Valencia, CA, United States) according to the manufacturer’s instructions. The concentration and purity of RNA were measured by a nanodrop spectrophotometer.
The extracted RNA was reverse-transcribed into complementary DNA (cDNA) using the QuantiTect Reverse Transcription Kit (Applied Biosystem) following the manufacturer’s instructions.
Real-time qPCR amplification and analysis were performed using Applied Biosystem real-time PCR system software to measure the expression of mRNAs of the target gene in the spleen, with B-actin as an internal reference (housekeeping gene). The isolated cDNA was amplified using the SYBR Green Master Mix (Applied Biosystems) following the manufacturer’s protocol. The primers used in the amplification are shown in Table 1 based on NBCI rat sequences. The result was calculated using the comparative cycle threshold (Ct) method. All values were normalized to ß-actin and reported as fold change over background levels detected in the treated groups. All these steps were performed according to the methods of Livak and Schmittgen (2001).
2.11 Histological evaluation
After fixation in 10% neutral buffered formalin for 48 h, the splenic tissue specimens were dehydrated in increasing concentrations of ethyl alcohol, cleaned using xylene, blocked in paraffin wax, sectioned at 5-μm thicknesses, and stained with hematoxylin and eosin (H&E) according to Bancroft and Gamble (2008).
2.12 Immunohistochemical evaluation
For detection of CD3 and CD68, paraffin sections were mounted on positively charged slides and exposed to the antigen retrieval process in citrate buffer (pH 6.0) using a microwave for 10 min. Subsequently, endogenous peroxidase was blocked using H2O2 for 30 min. The slides were incubated with mouse monoclonal anti-CD3 and anti-CD68 at a dilution of 1:250 (catalog numbers sc-20047 and sc-20060, respectively, Santa Cruz Biotechnology Inc., CA, United States) overnight at 4 °C. After that, the sections were treated with secondary antibodies associated with the streptavidin–biotin–peroxidase complex. Diamino-benzidine (DAB) was used as a chromogen. All tissue sections were counter-stained with hematoxylin.
2.13 Histological and immunohistochemical scoring methods
According to Meyerholz and Beck (2019), the ordinal scoring method was used for grading the histological changes in splenic tissues among the different groups. The scores were from 0 to 4, where 0 was normal, 1 was <25% affection, 2 was 25%–50% affection, 3 was 50%–75% affection, and 4 was >75% affection. However, quantitative immunohistochemical analysis of CD3 and CD68 expressions in all the examined splenic tissues was scored using ImageJ 1.47 software (National Institutes of Health, Bethesda, United States) as outlined by Smolen (1990), where the brown color intensities were expressed as the relative optical density of the DAB reaction.
Five random images per slide (n=3) at 400X were checked blindly for histological and immunohistochemical scoring using the Leica DM3000 microscope.
2.14 Statistical analysis
The statistical analysis was performed using SPSS (SPSS Inc., Chicago, Illinois, USA). All data points were represented as mean values ±SE. Differences between the groups were analyzed using one-way ANOVA (analysis of variance) and post hoc Tukey’s tests. p values <.05 were regarded as significant.
3 Results
3.1 Hematological analyses
In regard to the hemogram, there were significant reductions in RBC count, Hb concentration, and hematocrit percentage in the LA-treated group compared to the control group. Meanwhile, those parameters revealed significant elevations in the LA + CUR and LA + CIN groups compared to the LA-treated group. Concerning red blood cell indices, MCV showed a significant increase with significant decreases in MCH and MCHC in the LA-treated group compared to the control one. However, the LA + CUR and LA + CIN groups exhibited a significant decrease in MCV along with significant increases in MCH and MCHC compared to the LA-treated group (Figure 1).
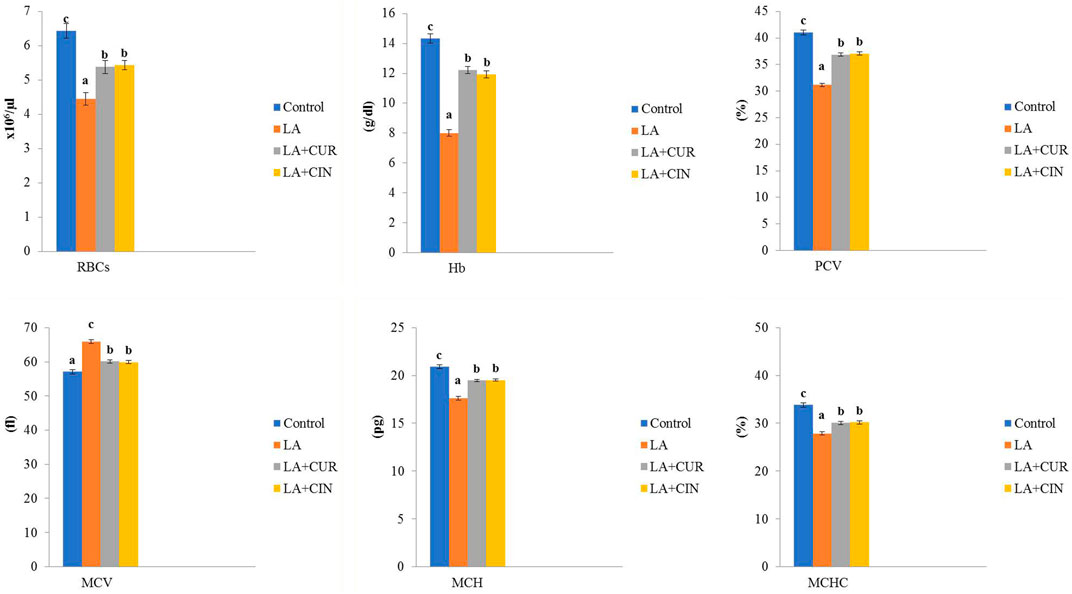
FIGURE 1. Changes of erythrogram in different treatment groups compared to the control (mean ± SE). Different superscript letters in each figure indicate significant differences at p < .05.
As shown in Figure 2, leukogram results revealed significant elevations in WBC, neutrophil, and monocyte counts and a significant reduction in lymphocyte count in the LA-treated group compared to the control group. In contrast, the LA + CUR and LA + CIN groups showed significant decreases in WBC, neutrophil, and monocyte counts with a significant increase in lymphocyte count compared to the LA-treated group.
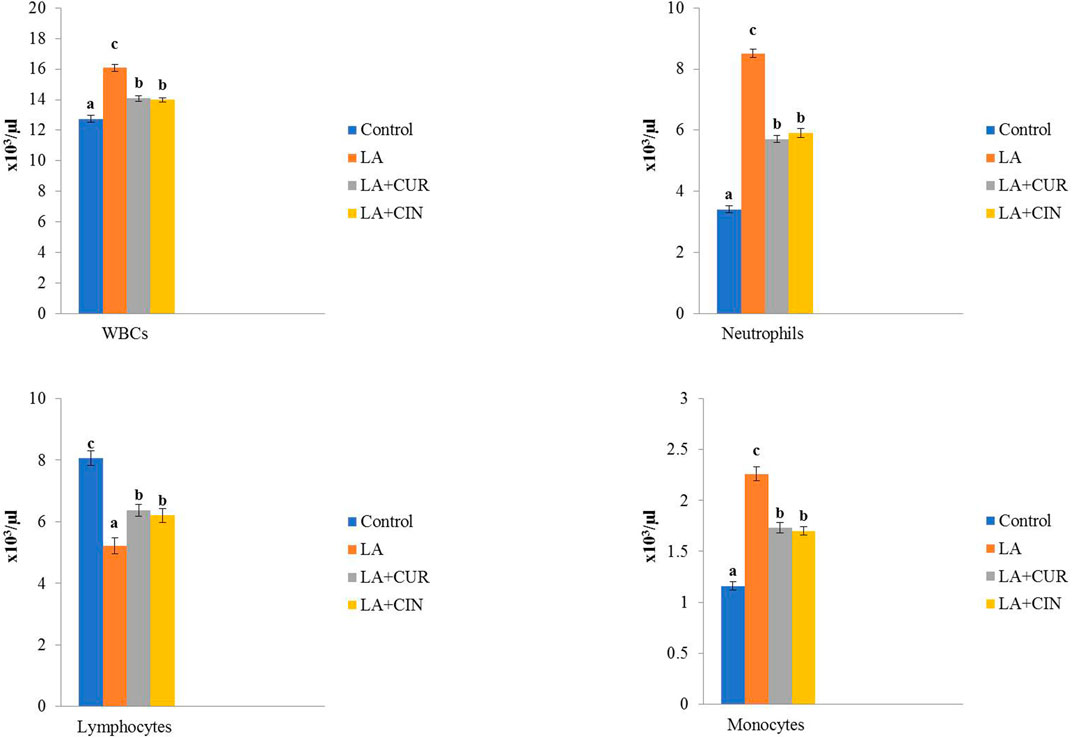
FIGURE 2. Changes of leukogram in different treatment groups compared to the control (mean ± SE). Different superscript letters in each figure indicate significant differences at p < .05.
From these results, we concluded that LA induced macrocytic hypochromic anemia with leukocytosis, neutrophilia, monocytosis, and lymphopenia. On the other hand, groups treated by CIN and CUR reversed the bad effect of LA on erythrogram and leukogram.
3.2 Iron parameters
Significant elevations in serum iron and ferritin levels, as well as transferrin saturation percentage, along with significant decreases in TIBC, UIBC, and transferrin levels were recorded in the LA-treated group compared to the control group. On the other hand, the LA + CUR and LA + CIN groups revealed an observable decline in serum iron, ferritin, and transferrin saturation percentage besides significant elevations in TIBC, UIBC, and transferrin levels compared to the LA-treated group (Figure 3). It was concluded that LA caused a disturbance in iron parameters which returned toward normal in the CIN- and CUR-treated groups.
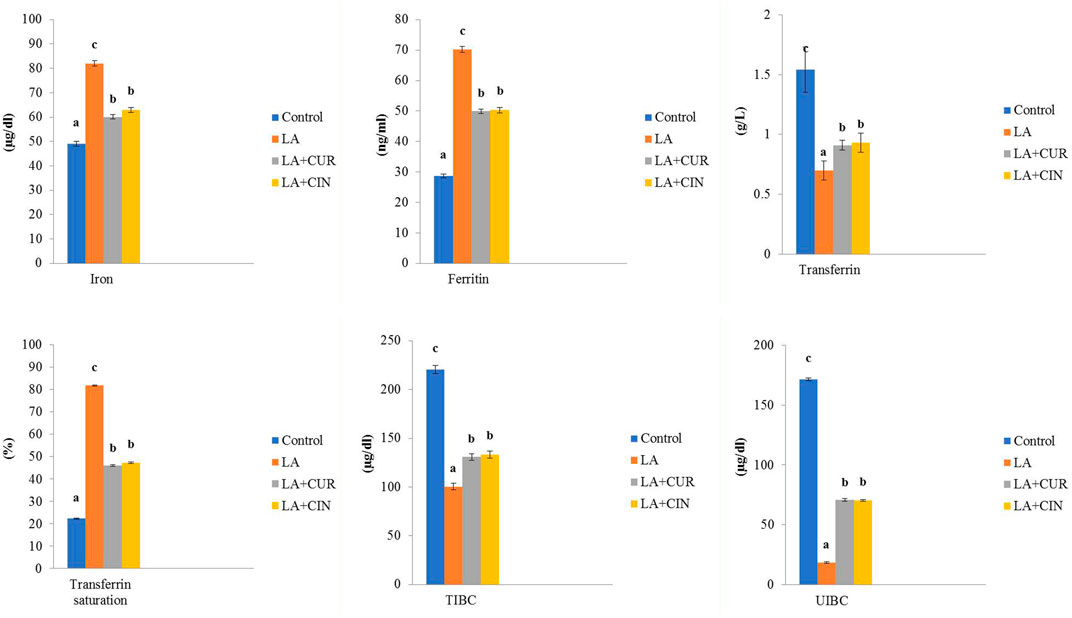
FIGURE 3. Changes of iron parameters in different treatment groups compared to the control (mean ± SE). Different superscript letters in each figure indicate significant differences at p < .05.
3.3 Serum immunoglobulins
In comparison to the control group, LA-treated rats exhibited a significant decline in both IgG and IgM levels. Meanwhile, their levels revealed significant increases in the LA + CUR and LA + CIN groups compared to the LA-treated group (Figure 4).
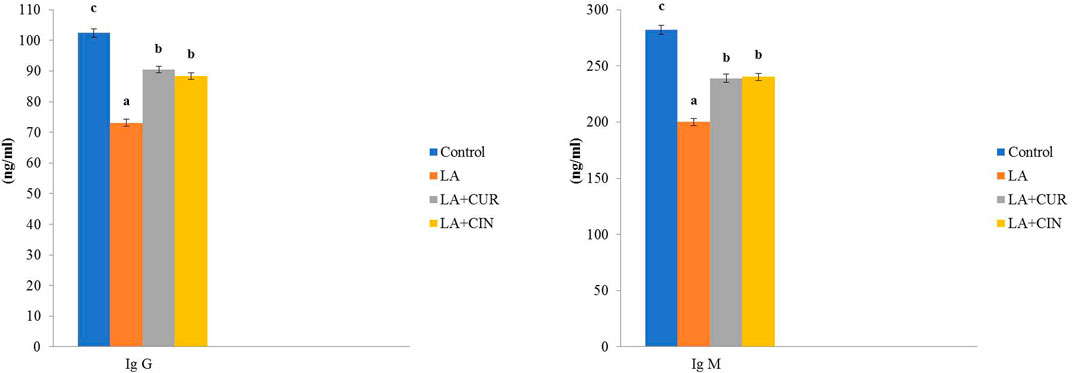
FIGURE 4. Changes of immunoglobulins in different treatment groups compared to the control (mean ± SE). Different superscript letters in each figure indicate significant differences at p < .05.
3.4 Splenic oxidative/antioxidant parameters
Rats exposed to LA showed significant increases in MDA, NO, and PC, along with a significant reduction in GSH activity compared to the control group. Meanwhile, the LA + CUR and LA + CIN groups exhibited significant declines in MDA, NO, and PC levels as well as a significant elevation in GSH activity compared to the LA-treated group (Figure 5). LA induces oxidative stress and depletion of antioxidants within splenic tissue, but CIN and CUR ameliorated these effects.
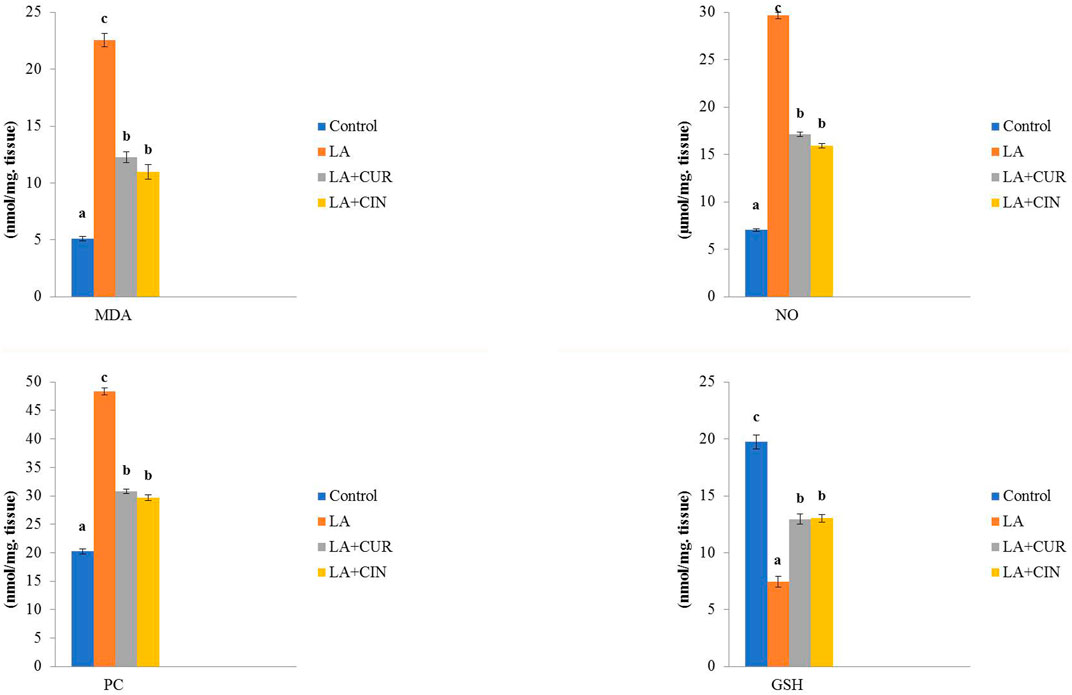
FIGURE 5. Changes of oxidative stress/antioxidant parameters in different treatment groups compared to the control (mean ± SE). Different superscript letters in each figure indicate significant differences at p < .05.
3.5 Lead content in splenic tissues
Splenic tissues from the LA-treated group exhibited a significantly high lead content compared to those from the control group. Meanwhile, groups supplemented with CUR and CIN revealed significantly lower splenic content of lead (Figure 6) compared to the LA-treated group.
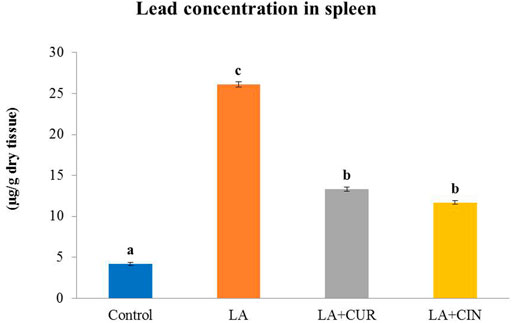
FIGURE 6. Lead concentration in the splenic tissue of different treatment groups compared with the control (mean ± SE). Different superscripts (A–C) in each figure indicate significant differences at p < .05.
3.6 Splenic expression of CYP-2E1
The splenic expression of CYP-2E1 was upregulated in the LA-treated group compared with the control group. On the contrary, the CUR- and CIN-treated groups revealed significant decreases in the expression of CYP-2E1 compared with the LA-treated group (Figure 7).
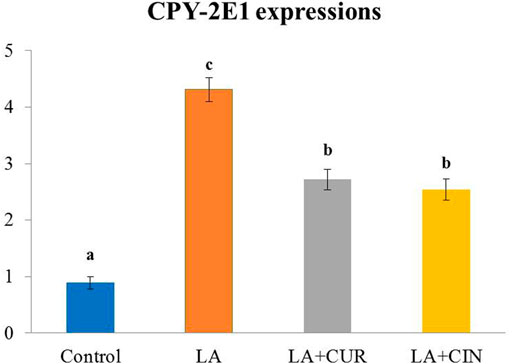
FIGURE 7. Splenic expression of CYP 2E1 in different treatment groups compared with ß-actin (mean ± SE). Different superscripts (A–C) in each figure indicate significant differences at p < .05.
3.7 Histological findings
Splenic tissue sections obtained from the control group revealed normal histo-architecture of red and white pulps (Figure 8A). The splenic red pulp was formed of branching splenic cords and vascular sinusoids. Meanwhile, the white pulp was formed of lymphoid follicles containing closely packed lymphocytes with an eccentrically located arteriole (Figure 8B).
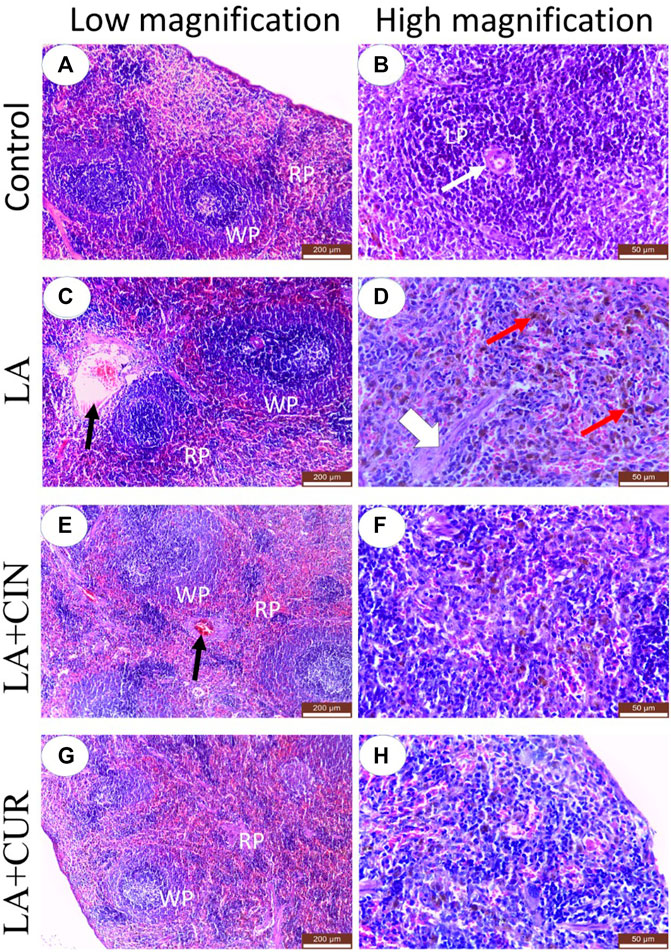
FIGURE 8. H&E-stained sections of the spleen from adult Albino rats, (A) control, (C) LA, (E) LA + CIN, and (G) LA + CUR groups. (B), (D), (F), and (H) were higher magnification of control, LA, LA + CIN, and (G) LA + CUR groups, respectively. Red pulp (RP), white pulp (WP), lymphoid follicle (LP), central arteriole (thin white arrows), blood vessels (black arrows), septa (broad white arrow), and hemosiderin pigments (red arrows). H&E stain.
In the LA group, marked histological alterations were detected within the splenic tissue. Significant enlargement of splenic white pulp due to lymphatic follicle proliferation was observed in addition to dilatation of central arterioles (Figure 8C). The splenic red pulp revealed a loss of architecture with significant congestion of blood sinusoids and dilated blood vessels (Figure 8C). Significant cytoplasmic depositions of hemosiderin pigments in many cells of red pulp, in addition to thickened splenic septa, were noticed (Figure 8D).
Both the LA + CIN- and LA + CUR-treated groups revealed a significant improvement of splenic tissue architectures with partially normal white and red pulps compared to the LA group (Figures 8E,G), where prominent red pulps were detected, indicating a reduction of the hyperplastic lymphoid reaction (Figures 8E,G). In comparison to the LA group, the splenic blood vessels showed significantly slight congestion in the CIN + LA group (Figure 8E), and no congestion in the LA + CUR group (Figure 8G) along with significantly slight dilatation of sinusoids with scanty hemosiderin pigment deposition were noticed (Figures 8F,H). A summary of the scoring of histological alteration in splenic tissues among different groups is recorded in Table 2.
3.8 Immunohistochemical findings
The positive immunohistochemical reactions for CD3 and CD68 appeared as brown cytoplasmic staining within splenic tissue cells (Figure 9). A summary of the scoring of splenic CD3 and CD68 expressions among different groups is recorded (Figure 10).
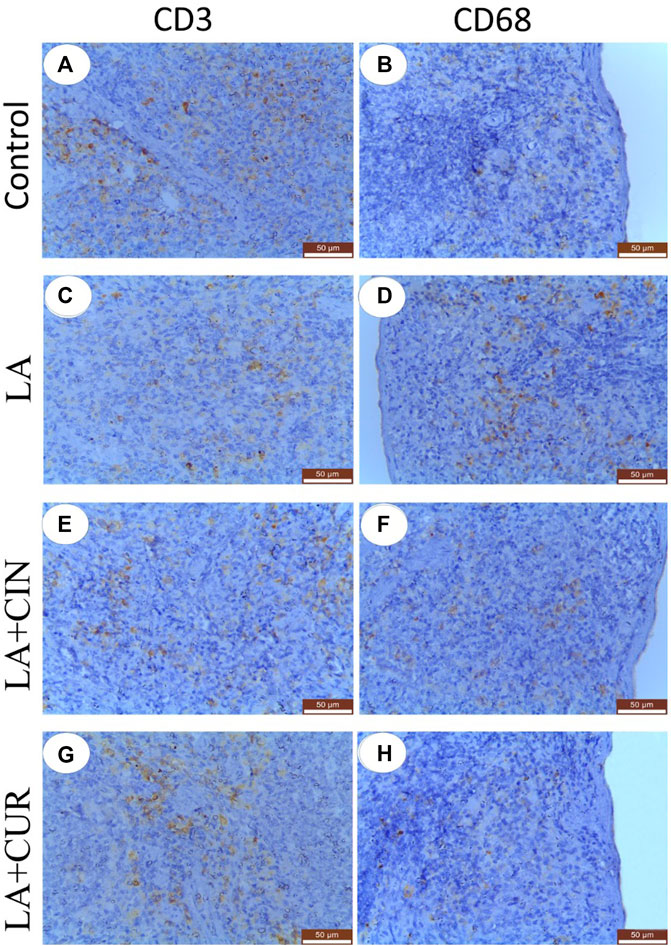
FIGURE 9. Immunohistochemically stained sections of the spleen with CD3 (A,C,E, and G) and CD68 (B,D,F, and, H) from different groups. (A) control group showed strong CD3 immunostaining, (C) LA group showed weak CD3 immunostaining, e and g; LA + CIN, and LA + CUR groups showed moderate CD3 immunostaining, (B) and (H); control and LA + CUR groups showed weak CD68, (D); LA group showed strong CD68 immunostaining, and (F); LA + CIN group showed moderate CD68 immunostaining.
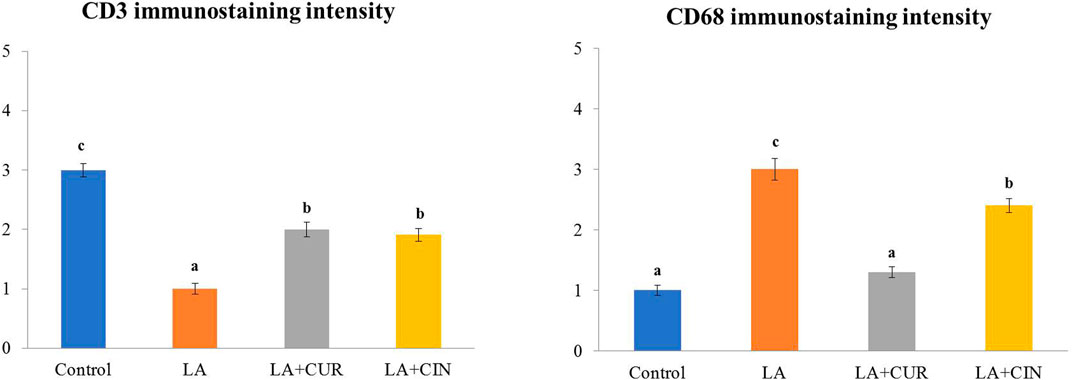
FIGURE 10. Immunohistochemical intensities of CD3 and CD68 in the spleen of all experimental groups.
3.8.1 CD3
Splenic sections from the control group revealed significantly strong immunostaining for CD3 (Figure 9A) compared to other groups. Otherwise, a significantly weak CD3 immunostaining was noticed in splenic tissues of the LA group (Figure 9C) compared to control rats. However, significantly moderate immunostaining of CD3 was apparently demonstrated in spleens of both the LA + CIN and LA + CUR groups compared to the LA group (Figures 9E,G).
3.8.2 CD68
A significantly weak CD68 immunostaining in the splenic tissues of both control and LA + CUR groups was noticed (Figures 9B,H). Meanwhile, significantly strong CD68 immunostaining was detected in the spleen of the LA group (Figure 9D) compared to other groups, whereas moderate CD68 immunostaining was seen in the LA + CIN group (Figure 9F).
4 Discussion
Lead is one of the most widespread heavy metals in the environment; hence, lead poisoning is a common case. Nowadays, attention has been paid to using herbal medicines instead of synthetic ones due to their low cost, availability, and fewer side effects. Therefore, the current study aims to investigate the potential efficacy of CIN and CUR, as herbal medicine, on hemato-biochemical, immunological, and oxidative stress markers, along with splenic histology and immunohistochemistry after exposure to LA.
Generally, heavy metal exposure can cause damage to the hematopoietic system, resulting in a disturbance in the hematological picture of both animals and humans (WHO, 1995). In the current study and after exposure to LA, rats exhibited significant reductions in RBC count, Hb concentration, PCV percentage, MCH, and MCHC, besides a significant increase in MCV compared to the control group, revealing macrocytic hypochromic anemia. Similar results were reported by Ekanem et al. (2015) and Ismail (2017). Additionally, Hossain et al. (2015) reported that LA induces hemolytic anemia.
LA-induced anemia could be attributed to binding lead to proteins in RBCs (García-Niño and Pedraza-Chaverri, 2014) as well as to shortening of RBCs’ life-span via depletion of the antioxidant system within the RBCs (Patra and Swarup, 2000; Lavicoli et al., 2003), inhibition of some Hb synthesizing enzymes (Dongre et al., 2011), and induction of RBC hemolysis via Hb oxidation (Flora et al., 2012).
All the previous erythrogram parameters showed an improvement in the LA + CUR and LA + CIN groups compared to the LA-treated group. The improvement of the erythrogram by CUR was in harmony with Ahmad et al. (2015) and El-Maddawy and El-Sayed (2018), where CUR could enhance erythropoiesis and stabilize cell membranes, preventing cellular damage (Banji et al., 2011). CUR may also protect Hb from oxidation (Unnikrishnan and Rao, 1992), and it could increase the intracellular glutathione, which interacts with ROS and free radicals, converting them into an oxidized form Al-Attar, (2022), besides the ability of CUR to improve the absorption of iron from the gut or by facilitating the reduction of oxidized iron to its reduced form (Wardlaw, 1990).
Leukogram results revealed significant elevations in WBC, neutrophil, and monocyte counts and a significant reduction in lymphocyte count in the LA-treated group compared to the control group. This was in agreement with Mugahi et al. (2003). Also, Oyem et al. (2021) founded that LA induces leukocytosis and neutrophilia. Leukocytosis might be considered a response of the phagocytic cells of the blood (neutrophils and monocytes), which engulf the denatured Hb and damaged RBCs (Ekanem et al., 2015) and/or might be due to inflammation induced by LA (Alwaleedi, 2015). Concerning lymphopenia, it was in accordance with Ismail (2017), that may be attributed to immunosuppression induced by LA (Ercal et al., 2001), splenotoxicity (Rocha et al., 2016), or damage of mitochondria and lysosomes in circulating lymphocytes (Zarei et al., 2017).
In contrast, LA + CUR and LA + CIN groups showed significant decreases in WBC, neutrophil, and monocyte counts with a significant increase in lymphocyte count compared to the LA-treated group. This might be attributed to the ability of CUR to modulate the activity of neutrophils and restoration of WBCs (Banji et al., 2011). Furthermore, the increase in lymphocytes might be due to activation of the immune system, stimulating lymphopoiesis (Cetin et al., 2010).
Regarding the ameliorative effect of CIN on LA-induced blood picture disturbance, Ghonim et al. (2017) recorded a similar role of CIN on both erythrogram and leukogram, owing to the antioxidant properties of CIN polyphenols and flavonoids that act as scavengers for reactive oxygen and nitrogen species, besides its effects on chelation of metal and modulation of enzymes (Azab et al., 2011).
Concerning the serum iron parameters, there were significant elevations in serum iron and ferritin levels as well as transferrin saturation percentage, along with significant decreases in TIBC, UIBC, and transferrin levels in the LA-treated group compared to the control group. Our results partially were in harmony with those of Chen et al. (2019a), who reported decreased levels of transferrin and TIBC in lead workers compared to unexposed ones. Elevated serum iron, ferritin, and transferrin saturation percentage might be due to hemolytic anemia induced by LA, where large quantities of iron are released from destroyed RBCs, consequently increasing ferritin level and transferrin saturation percentage. The high serum iron level, accompanied by high ferritin level and low transferrin level, confirms the inverse relationship between ferritin and transferrin levels (Majoni et al., 2017). The LA + CUR and LA + CIN groups revealed an observable decline in serum iron, ferritin, and transferrin saturation percentage, besides significant elevations in TIBC, UIBC, and transferrin levels compared to the LA-treated group. This might be owed to the antioxidant and chelator effects of both CUR and CIN to free iron (Jiao et al., 2006; Azab et al., 2011), where CUR contains chemical groups such as the ß-diketonate group (Bernabe-Pineda et al., 2004).
Regarding oxidative stress, ROS, including singlet oxygen (O−), hydroxyl radical (OH+), and hydrogen peroxide (H2O2), are generated naturally in various body cells during normal cellular respiration (Le Bras et al., 2005; Small et al., 2012).
These ROS are cytotoxic molecules even during normal cellular respiration, so they should be naturally neutralized by an endogenous antioxidant defense system, including GSH, MDA, and NO (Le Bras et al., 2005; Avery, 2011; Small et al., 2012). If there is an imbalance between ROS generation and antioxidants, severe oxidative stress-induced cellular damage occurs. The excess ROS causes cell membrane and DNA damage, lipid peroxidation, and protein oxidation, leading to various forms of cellular toxicity (Small et al., 2012; Farouk et al., 2021a; b). In the current study, LA-induced oxidative damage involves first ROS generation and then the destruction of the body’s antioxidant defense system (Barbouti et al., 2020). One of the reasons for increasing levels of MDA in the splenic tissue was the increased ROS traversing the cell membrane and destroying neighboring cells, leading to cellular damage of the spleen (Rocha et al., 2016). Lead also renders the cellular GSH inactive, so it becomes unable to restore the GSH supply. Moreover, lead inactivates δ-aminolevulinic acid dehydratase (ALAD), glutathione reductase (GR), glutathione peroxidase (GPX), and glutathione-S-transferase, which depresses the glutathione levels (Carocci et al., 2016).
PC is a carbonyl product formed as a result of protein oxidation, where ROS could attack amino acid residues (Harishekar and Kiran, 2011). Owing to the chemical stability of PC, it is easily detectable, and its increase takes place earlier than MDA from lipid peroxidation (Del Rio et al., 2005; Erdogan et al., 2006). Levine et al. (1990) reported that PC derivative formation is associated with some pathological conditions, including rheumatoid arthritis, atherosclerosis, neurological disorders, cataractogenesis, and ischemia. In the case of LA exposure, protein oxidation is predominant, so PC is increased (Rouach et al., 1997).
Rats exposed to LA showed significant increases in MDA, NO, and PC, along with a significant reduction in GSH activity compared to the control group. These results agreed with those of El-Sherbini et al. (2016), El-Tantawy (2016), Ismail (2017), and Oyem et al. (2021). Lead inhibits the cellular thiol antioxidant capacity (Ercal et al., 1996; Gürer et al., 1998). In addition, lead might cause oxidative stress by inhibiting d-aminolevulinic acid dehydratase (ALAD), leading to auto-oxidation of ALAD, forming H2O2 (Gürer et al., 1998), resulting in oxidative stress and depletion of the antioxidant system. On the other hand, the LA + CUR and LA + CIN groups exhibited significant declines in MDA, NO, and PC levels as well as a significant elevation in GSH activity compared to the LA-treated group. The protective effect of CUR against LA intoxication was a result of its attenuation to antioxidant depletion (Del Rio et al., 2005), scavenging ROS, and chelating LA (García-Niño and Pedraza-Chaverri, 2014; Hismiogullari et al., 2015), that increases the intracellular glutathione concentration, consequently protects lipid peroxidation, and reduces oxidative tissue stress (Okada et al., 2001) and inflammatory damages (Ali et al., 2018). CUR also enhances detoxifying enzyme activity (Abdou and Hassan, 2014).
Regarding the protective effect of CIN, our result was in harmony with that of Elshopakey and Elazab (2021), who reported that CIN can reduce MDA and NO and elevate GSH levels against oxidative stress induced by diclofenac sodium and oxytetracycline. The protective activity of CIN may be attributed to its polyphenol and flavonoid content that acts as ROS scavengers, metal chelators, and enzyme modulators (Azab et al., 2011). Moreover, the active components of CIN such as cinnamaldehyde, cinnamic acid, and eugenol are the principles of its antioxidant and free radical scavenging properties (Gülçin 2011; Jayaprakasha and Rao 2011). Additionally, the reduction in NO level by CIN might be owed to its anti-inflammatory effect associated with its various active components, including cinnamic aldehyde, cinnamyl aldehyde, and tannins, inhibiting the production of NO (Lin et al., 2008).
Regarding the immunoglobulin levels, the LA-treated group revealed a significant reduction in IgM and IgG levels, which was in accordance with Soliman et al. (2015) and Ismail (2017), that might be owed to the effect of LA-induced oxidative stress on B cell function (Hsiao et al., 2011). Such oxidative stress causes Th cell disturbance and alters the availability of Th1 and Th2 cytokines (Hsiao et al., 2011). Meanwhile, IgG and IgM levels revealed significant increases in the LA + CUR and LA + CIN groups compared to the LA-treated group. Similarly, Ciftci (2011) reported that CUR enhances IgG and IgM levels, referring to its immuno-stimulant impact via activation of macrophages, neutrophils, natural killer cells, and dendritic cells (Jagetia and Aggarwal, 2007), besides its stimulatory property on humoral immunity (Kim et al., 2013). On the other hand, CIN revealed an improvement in immunoglobulin levels, especially IgG, which agreed with Elshopakey and Elazab (2021). Also, Niphade et al. (2009) reported that CIN has a humoral immunity stimulatory effect via elevation of serum immunoglobulins levels through its cinnamaldehyde, benzaldehyde, cuminaldehyde, and terpenes.
Lead concentration in the splenic tissue of LA-exposed rats was significantly high. However, rats supplemented by CUR and CIN revealed lower splenic lead concentration than those in the LA group, and this might be attributed to the metal-chelating property of CUR and CIN (Azab et al., 2011; Soliman et al., 2015).
CYP-2E1 enzyme is one of the cytochrome P450 involved in oxidative stress and lipid peroxidation (Martikainen et al., 2012). CYP-2E1 can metabolize toxic substrates, producing excess ROS (Danielson, 2002), which degrades the CYP hemeprotein releasing iron, potentiating lipid peroxidation (Caro and Cederbaum, 2004). In the present study, LA-induced overexpression of the splenic CYP-2E1 gene agreed with Chen et al. (2019b). On the other hand, administration of CUR and CIN was able to inhibit the upregulation of CYP-2E1 expression in the spleen of treated rats. Also, Eassawy et al. (2021) reported that CUR reduces the expression of CYP-2E1 in the liver of acetaminophen-intoxicated and gamma-irradiated rats.
The hemato-biochemical, immunological, oxidative stress, and gene expression results were confirmed by the histological examination of splenic tissues. The spleen of the control group revealed normal histo-architecture of both white and red pulps, as mentioned by Alkhedaide, (2016). LA exposure induced marked distorted splenic architecture, which was represented by significant hyperplasia of lymphoid follicles and its diffusion into the red pulp as well as depositions of hemosiderin pigment in many cells, which matched with Ekanem et al. (2015), Aldahmash and El-Nager (2016), and Hasan et al. (2016). Hemosiderin pigment might be a result of the engulfment of RBCs by macrophages, indicating LA-induced hemolytic anemia and chronic congestion. Spleen macrophage plays a critical role in iron metabolism via iron recycling from damaged or senescent erythrocytes (Wang et al., 2010). Although these histological changes were detected in all treated groups, it was mild and less prominent in both the LA + CIN and LA + CUR groups, confirming the findings of Elgawish and Abdelrazek (2014), who recorded a protective effect of CIN against LA-induced dysfunction of the testes.
Immunohistochemical findings of the current study revealed that LA induced a significant reduction in CD3 and a significant increase of CD68 expressions in the splenic tissues, confirming the immunosuppressive effect of lead (Hsiao et al., 2011; Rocha et al., 2016) and its stimulatory effect on phagocytic cells (Mugahi et al., 2003), respectively. Otherwise, the LA + CIN and LA + CUR groups revealed an increase of CD3 and a reduction of CD68 expressions in splenic tissues compared to the LA group, indicating an immuno-enhancement effect of both CUR and CIN (Banji et al., 2011; Lee et al., 2013; Elshopakey and Elazab, 2021).
In conclusion, the current study figured out the potential ameliorative effect of CIN and CUR upon LA-induced changes in hemato-biochemical parameters and oxidative stress markers, as well as the histology of the spleen and CD3 and CD68 expressions. These results indicate antioxidant, immunomodulatory, and gene-regulating effects of CUR and CIN against LA-induced splenotoxicty in rat models.
Data availability statement
The original contributions presented in the study are included in the article/Supplementary Material; further inquiries can be directed to the corresponding author.
Ethics statement
The animal study was reviewed and approved by the Committee of Research Ethics Board at the Faculty of Veterinary Medicine, Benha University, Egypt (Approval No. BUFVTM 17-03-22).
Author contributions
ME: Conceptualization, Data curation, Investigation, Methodology, Visualization, Writing original draft, Writing review & editing. SF: Conceptualization, Data curation, Investigation, Methodology, Visualization, Writing original draft, Writing review & editing. AA: Data curation, Writing review & editing, Funding the work. AA: Conceptualization, Data curation, Formal analysis, Methodology, Writing original draft, Writing review & editing. AE: Conceptualization, Data curation, Formal analysis, Methodology, Writing original draft, Writing review & editing. FG: Conceptualization, Data curation, Formal analysis, Investigation, Methodology, Writing original draft, Writing review & editing.
Conflict of interest
The authors declare that the research was conducted in the absence of any commercial or financial relationships that could be construed as a potential conflict of interest.
Publisher’s note
All claims expressed in this article are solely those of the authors and do not necessarily represent those of their affiliated organizations, or those of the publisher, the editors, and the reviewers. Any product that may be evaluated in this article, or claim that may be made by its manufacturer, is not guaranteed or endorsed by the publisher.
References
A-Attar, A. M. (2022). Hematological and biochemical investigations on the effect of curcumin and Thymoquinone in male mice exposed to Thioacetamide. Saudi J. Biol. Sci. 29, 660–665. doi:10.1016/j.sjbs.2021.10.037
Abd Elrasoul, A. S., Mousa, A. A., Orabi, S. H., Mohamed, M. A. E. G., Gad-Allah, S. M., Almeer, R., et al. (2020). Antioxidant, anti-inflammatory, and anti-apoptotic effects of Azolla pinnata ethanolic extract against lead-induced hepatotoxicity in rats. Antioxidants 9, 1014. doi:10.3390/antiox9101014
Abdelhamid, F. M., Mahgoub, H. A., and Ateya, A. I. (2020). Ameliorative effect of curcumin against lead acetate–induced hemato-biochemical alterations, hepatotoxicity, and testicular oxidative damage in rats. Environ. Sci. Pollut. Res. 27, 10950–10965. doi:10.1007/s11356-020-07718-3
Abdou, H. M., and Hassan, M. A. (2014). Protective role of omega-3 polyunsaturated fatty acid against lead acetate-induced toxicity in liver and kidney of female rats. Biomed. Res. Int. 435857, 435857. doi:10.1155/2014/435857
Ahmad, I., Raina, R., Verma, P. K., and Pandita, S. (2015). Protective effect of curcumin on hematological alterations induced by repeated administration of fluoride and λ-cyhalothrin alone and in combination in Wistar rats. World J. Pharm. Res. 4 (3), 1231–1238.
Aldahmash, B. A., and El-Nager, D. M. (2016). Antioxidant effects of captopril against lead acetate-induced hepatic and splenic tissue toxicity in Swiss Albino mice. Saudi J. Biol. Sci. 23, 667–673. doi:10.1016/j.sjbs.2016.05.005
Alhusaini, A. M., Faddah, L. M., Hasan, I. H., Jarallah, S. J., Alghamdi, S. H., Alhadab, N. M., et al. (2019). Vitamin C and turmeric attenuate Bax and Bcl-2 proteins’ expressions and DNA damage in lead acetate-induced liver injury. Dose-Response 17 (4), 1559325819885782. doi:10.1177/1559325819885782
Ali, B. H., Al-Salam, S., Al Suleimani, Y., Al Kalbani, J., Al Bahlani, S., Ashique, M., et al. (2018). Curcumin ameliorates kidney function and oxidative stress in experimental chronic kidney disease. Basic Clin. Pharmacol. Toxicol. 122, 65–73. doi:10.1111/bcpt.12817
Alkhedaide, A., et al. (2016). Ameliorative role of grape seed extract on cadmium induced splenic toxicity in Albino rats. Imperial J. Interdiscip. Res. 2 (10), 1655–16642016.
Alwaleedi, S. A. (2015). Haemato-biochemical changes induced by lead intoxication in male and female Albino mice. Intl. J. Rec. Sci. Res. 6 (4), 3999–4004.
Avery, S. V. (2011). Molecular targets of oxidative stress. Biochem. J. 434, 201–210. doi:10.1042/BJ20101695
Azab, K. S., Mostafa, A. H. A., Ali, E. M., and Abdel-Aziz, M. A. (2011). Cinnamon extract ameliorates ionizing radiation-induced cellular injury in rats. Ecotoxicol. Environ. Saf. 74, 2324–2329. doi:10.1016/j.ecoenv.2011.06.016
Bancroft, J. D., and Gamble, M. (2008). Theory and practice of histological techniques. China: Churchill Livingstone, Elsevier.
Banji, D., Pinnapureddy, J., Banji, O. J. V., Kumar, A. R., and Reddy, K. N. (2011). Evaluation of the concomitant use of methotrexate and curcumin on Freund's complete adjuvant-induced arthritis and hematological indices in rats. Indian J. Pharmacol. 43 (5), 546–550. doi:10.4103/0253-7613.84970
Barbouti, A., Vasileiou, P. V. S., Evangelou, K., Vlasis, K. G., Papoudou-Bai, A., Gorgoulis, V. G., et al. (2020). Implications of oxidative stress and cellular senescence in age-related thymus involution. Oxidative Med. Cell. Longev. 2020, 7986071. doi:10.1155/2020/7986071
Bernabe-Pineda, M., Ramirez-Silva, M. T., Romero-Romo, M. A., Gonzalez-Vergara, E., and Rojas-Hernandez, A. (2004). Spectrophotometric and electrochemical determination of the formation constants of the complexes Curcumin-Fe(III)-water and Curcumin-Fe(II)-water. Spectrochim. Acta. A. Mol. Biomol. Spectrosc. 60, 1105–1113. doi:10.1016/S1386-1425(03)00344-5
Beutler, E., Duron, O., and Kelly, B. M. (1963). Improved method for the determination of blood glutathione. J. Laboratory Clin. Med. 61, 882–888.
Blackmore, S., Hamilton, M., Lee, A., Worwood, M., Brierley, M., Heath, A., et al. (2008). Automated immunoassay methods for ferritin: Recovery studies to assess traceability to an international standard. Clin. Chem. Lab. Med. 46 (10), 1450–1457. doi:10.1515/CCLM.2008.304
Burits, C. A., and Burns, D. E. (2014). Tietz fundamentals of clinical chemistry and molecular diagnostics. 1st ed. Elsevier Health Sciences.
Caro, A. A., and Cederbaum, A. I. (2004). Oxidative stress, toxicology, and pharmacology of CYP2E1. Annu. Rev. Pharmacol. Toxicol. 44, 27–42. doi:10.1146/annurev.pharmtox.44.101802.121704
Carocci, A., Catalano, A., Lauria, G., Sinicropi, M. S., and Genchi, G. (2016). Lead toxicity, antioxidant defense and environment. Rev. Environ. Contam. Toxicol. 238, 45–67. doi:10.1007/398_2015_5003
Cesta, M. F. (2006). Normal structure, function, and histology of the spleen. Toxicol. Pathol. 34, 455–465. doi:10.1080/01926230600867743
Cetin, E., Kanbur, M., Silici, S., and Eraslan, G. (2010). Propetamphos induced changes in haematological and biochemical parameters of female rats: Protective role of propolis. Food Chem. Toxicol. 48 (7), 1806–1810. doi:10.1016/j.fct.2010.04.010
Chen, C., Lin, B., Qi, S., He, J., and Zheng, H. (2019a). Protective effects of salidroside on lead acetate-induced oxidative stress and hepatotoxicity in sprague-dawley rats. Biol. Trace Elem. Res. 191, 426–434. doi:10.1007/s12011-019-1635-8
Chen, C., Wang, C. L., Ho, C. K., Chuang, H. Y., and Yu, H. S. (2019b). Interactive effects between chronic lead exposure and the homeostatic iron regulator transport HFE polymorphism on the human red blood cell mean corpuscular volume (MCV). Int. J. Environ. Res. Public Health 16 (3), 354. doi:10.3390/ijerph16030354
Chen, S., Golemboski, K. A., Piepenbrink, M., and Dietert, R. R. (2004). Developmental immunotoxicity of lead in the rat: Influence of maternal diet. J. Toxicol. Environ. Health Part A 67, 495–511. doi:10.1080/15287390490276520
Chen, S., Miller, T. E., Golemboski, K. A., and Dietert, R. R. (1997). Suppression of macrophage metabolite production by lead glutamate in vitro is reversed by meso 2, 3-dimercaptosuccinic acid (DMSA). Vitro Toxicol. 10, 351–358.
Chwalba, A., Maksym, B., Machoń-Grecka, A., Cisowska-Babraj, M., Orłowska, J., and Kasperczyk, A. (2018). The influence of lead on hematopoesis - contemporary views. Med. Środowiskowa - Environ. Med. 21 (4), 39–43.
Ciftci, O. (2011). Curcumin prevents toxic effects of 2, 3, 7, 8 tetrachlorodibenzo-p-dioxin (TCDD) on humoral immunity in rats. Food Agric. Immunol. 22 (1), 31–38. doi:10.1080/09540105.2010.517308
Danielson, P. B. (2002). The cytochrome P450 superfamily: Biochemistry, evolution and drug metabolism in humans. Curr. Drug Metab. 3, 561–597. doi:10.2174/1389200023337054
Del Rio, D., Stewart, A. J., and Pellegrini, N. (2005). A review of recent studies on malondialdehyde as toxic molecule and biological marker of oxidative stress. Nutr. Metab. Cardiovasc. Dis. 15, 316–328. doi:10.1016/j.numecd.2005.05.003
Dietert, R. R., and Piepenbrink, M. S. (2006). Lead and immune function. Crit. Rev. Toxicol. 36, 359–385. doi:10.1080/10408440500534297
Dongre, N. N., Suryakar, A. N., Patil, A. J., Ambekar, J. G., and Rathi, D. B. (2011). Biochemical effects of lead exposure on systolic and diastolic blood pressure, heme biosynthesis and hematological parameters in automobile workers of north Karnataka (India). Indian J. Clin. biochem. 26 (4), 400–406. doi:10.1007/s12291-011-0159-6
Dorri, M., Hashemitabar, S., and Hosseinzadeh, H. (2018). Cinnamon (cinnamomum zeylanicum) as an antidote or a protective agent against natural or chemical toxicities: A review. Drug Chem. Toxicol. 41, 338–351. doi:10.1080/01480545.2017.1417995
Dyatlov, V. A., and Lawrence, D. A. (2002). Neonatal lead exposure potentiates sickness behavior induced by Listeria monocytogenes infection of mice. Brain Behav. Immun. 16, 477–492. doi:10.1006/brbi.2001.0641
Eassawy, M. M., Salem, A. A., and Ismail, A. F. M. (2021). Biochemical study on the protective effect of curcumin on acetaminophen and gamma-irradiation induced hepatic toxicity in rats. Environ. Toxicol. 36 (5), 748–763. doi:10.1002/tox.23077
Ekanem, A. U., Kwari, H. D., Garba, S. H., and Salami, H. A. (2015). Effect of lead acetate on spleen and blood parameters in Albino rats. IOSR J. Dent. Med. Sci. 14 (3), 43–49.
El-Maddawy, Z. K., and El-Sayed, Y. S. (2018). Comparative analysis of the protective effects of curcumin and N-acetyl cysteine against paracetamol-induced hepatic, renal, and testicular toxicity in Wistar rats. Environ. Sci. Pollut. Res. Int. 25, 3468–3479. doi:10.1007/s11356-017-0750-3
El-Sherbini, E., et al. (2016). Ameliorative effects of L-carnitine on rats raised on a diet supplemented with lead acetate. Saudi J. Biol. Sci. 24 (6), 1410–1417.
El-Tantawy, W. H. (2016). Antioxidant effects of Spirulina supplement against lead acetate-induced hepatic injury in rats. J. Tradit. Complement. Med. 6, 327–331. doi:10.1016/j.jtcme.2015.02.001
Elgawish, R. A., and Abdelrazek, H. M. A. (2014). Effects of lead acetate on testicular function and caspase-3 expression with respect to the protective effect of cinnamon in Albino rats. Toxicol. Rep. 1, 795–801. doi:10.1016/j.toxrep.2014.10.010
Elshopakey, G. E., and Elazab, S. T. (2021). Cinnamon aqueous extract attenuates diclofenac sodium and oxytetracycline mediated hepato-renal toxicity and modulates oxidative stress, cell apoptosis, and inflammation in male Albino rats. Vet. Sci. 8 (9), 9–19. doi:10.3390/vetsci8010009
Emam, M. A., and El-Shafey, A. A. (2018). Curcumin protects against the adverse effect of long term administration of lithium on cerebral and cerebellar cortices in rats: Histological and immunohistochemical study. J. Veterinary Anantomy 11 (1), 1–13.
Ercal, N., Gurer-Orhan, H., and Aykin-Burns, N. (2001). Toxic metals and oxidative stress part I: Mechanisms involved in metal-induced oxidative damage. Curr. Top. Med. Chem. 1, 529–539. doi:10.2174/1568026013394831
Ercal, N., TreeratPhan, P., Hammond, T. C., Matthews, R. H., Grannemann, N. H., and Spitz, D. R. (1996). In vivo indices of oxidative stress in lead-exposed C57BL/6 mice are reduced by treatment with meso-2, 3-dimercaptosuccinic acid or N-acetylcysteine. Free Radic. Biol. Med. 21, 157–161. doi:10.1016/0891-5849(96)00020-2
Erdogan, H., Fadillioglu, E., Yagmurca, M., Uçar, M., and Irmak, M. K. Protein oxidation and lipid peroxidation after renal ischemia-reperfusion injury: Protective effects of erdosteine and N-acetylcysteine. Urol. Res. 34(1), 41–46. doi:10.1007/s00240-005-0031-32006).
Fairbanks, V., and Klee, G. (1987). in Biochemical aspect of hematology, Fundamentals of clinical chemistry. Editor N. W. Tietz (Philadelphia: Saunders), 803–804.
Farouk, S. M., Gad, F. A., Almeer, R., Abdel-Daim, M. M., and Emam, M. A. (2021a). Exploring the possible neuroprotective and antioxidant potency of lycopene against acrylamide-induced neurotoxicity in rats’ brain. Biomed. Pharmacother. 138, 111458. doi:10.1016/j.biopha.2021.111458
Farouk, S. M., Gad, F. A., and Emam, M. A. (2021b). Comparative immuno-modulatory effects of basil and sesame seed oils against diazinon-induced toxicity in rats; a focus on TNF-α immunolocalization. Environ. Sci. Pollut. Res. 28, 5332–5346. doi:10.1007/s11356-020-10840-x
Ferreyra, H., Romano, M., and Uhart, M. (2009). Recent and chronic exposure of wild ducks to lead in human-modified wetlands in Santa Fe Province, Argentina. J. Wildl. Dis. 45, 823–827. doi:10.7589/0090-3558-45.3.823
Flora, G., Gupta, D., and Tiwari, A. (2012). Toxicity of lead: A review with recent updates. Interdiscp. Toxicol. 5 (2), 47–58. doi:10.2478/v10102-012-0009-2
Flora, S. J. S., Saxena, G., and Mehta, A. (2007). Reversal of lead-induced neuronal apoptosis by chelation treatment in rats: Role of reactive oxygen species and intracellular Ca2 +. J. Pharmacol. Exp. Ther. 322, 108–116. doi:10.1124/jpet.107.121996
García-Niño, W. R., and Pedraza-Chaverri, J. (2014). Protective effect of curcumin against heavy metals-induced liver damage. Food Chem. toxico. 69, 182–201. doi:10.1016/j.fct.2014.04.016
Ghonim, A., El-Shawarby, R., Abdel-Aleem, N., El-Shewy, E., Abdo, M., et al. (2017). Protective effect of cinnamon against cadmium-induced hepatorenal oxidative damage in rats. Int. J. Pharmacol. Toxicol. 5 (1), 17–22. doi:10.14419/ijpt.v5i1.7119
Green, L. C., Wagner, D. A., Glogowski, J., Skipper, P. L., Wishnok, J. S., and Tannenbaum, S. R. (1982). Analysis of nitrate, nitrite, and [15N] nitrate in biological fluids. Anal. Biochem. 126, 131–138. doi:10.1016/0003-2697(82)90118-x
Gülçin, I. (2011). Antioxidant activity of eugenol: A structure–activity relationship study. J. Med. Food. 14, 975–985. doi:10.1089/jmf.2010.0197
Gürer, H., Ozgünes, H., Neal, R., Spitz, D. R., and Erçal, N. (1998). Antioxidant effects of N-acetylcysteine and succimer in red blood cells from lead-exposed rats. Toxicology 128, 181–189. doi:10.1016/s0300-483x(98)00074-2
Harishekar, M. B., and Kiran, B. (2011). Effect of lead, alcohol and vitamin E on protein carbonyl content in rats. J. Appl. Pharm. Sci. 1 (9), 154–156.
Hasan, S. M. H., Abou-Rawash, A. A., and Bekheet, M. S. (2016). Protective role of an aqueous extract of punica granatum (pomegranate) peel on lead-induced anemia in rats. Alexandria J. Veterinary Sci. 50 (1), 99–108. doi:10.5455/ajvs.228349
Hismiogullari, A. A., Hismiogullari, S. E., Karaca, O., Sunay, F. B., Paksoy, S., Can, M., et al. (2015). The protective effect of curcumin administration on carbon tetrachloride (CCl4)-induced nephrotoxicity in rats. Pharmacol. Rep. 67, 410–416. doi:10.1016/j.pharep.2014.10.021
Hossain, S. h., Bhowmick, S., Islam, S., Rozario, L., Jahan, S., Hassan, M., et al. (2015). Oral administration of ganoderma lucidum to lead-exposed rats protects erythrocytes against hemolysis: Implicates to anti-anemia. Evid. Based Complement. Altern. Med. 2015, 463703. doi:10.1155/2015/463703
Hosseini, A., and Hosseinzadeh, H. (2018). Antidotal or protective effects of curcuma longa (turmeric) and its active ingredient, curcumin, against natural and chemical toxicities: A review. Biomed. Pharmacother. 99, 411–421. doi:10.1016/j.biopha.2018.01.072
Hsiao, C. L., Wu, K. H., and Wan, K. S. (2011). Effects of environmental lead exposure on T-helper cell-specific cytokines in children. J. Immunotoxicol. 8, 284–287. doi:10.3109/1547691X.2011.592162
Ismail, S. A. A. (2017). Ameliorative potential of spirulina platensis against lead acetate induced immuno-suppression and kidney apoptosis in rats. Ann. Clin. Pathol. 5 (5), 1120.
Jagetia, G. C., and Aggarwal, B. B. (2007). Spicing up” of the immune system by curcumin. J. Clin. Immunol. 27 (1), 19–35. doi:10.1007/s10875-006-9066-7
Jiao, Y., Wilkinson, J., ChristinE PiEtsch, E., Buss, J. L., Wang, W., Planalp, R., et al. (2006). Iron chelation in the biological activity of curcumin. Free Radic. Biol. Med. 40, 1152–1160. doi:10.1016/j.freeradbiomed.2005.11.003
Kim, D. K., Lillehoj, H. S., Lee, S. H., Jang, S. I., Lillehoj, E. P., and Bravo, D. (2013). Dietary Curcuma longa enhances resistance against Eimeria maxima and Eimeria tenella infections in chickens. Poult. Sci. 92 (10), 2635–2643. doi:10.3382/ps.2013-03095
Kim, S. H., Hyun, S. H., and Choung, S. Y. (2006). Antioxidative effects of Cinnamomi cassiae and Rhodiola rosea extracts in liver of diabetic mice. Biofactor 26, 209–219. doi:10.1002/biof.5520260306
Lavicoli, I., Carelli, G., Stanek, E. J., CastelliNoN., , and Calabrese, E. J. (2003). Effects of low doses of dietary lead on red blood cell production in male and female mice. Toxicol. Lett. 137, 193–199. doi:10.1016/s0378-4274(02)00404-6
Le Bras, M., Clément, M. V., Pervaiz, S., and Brenner, C. (2005). Reactive oxygen species and the mitochondrial signaling pathway of cell death. Histol. Histopathol. 20, 205–219. doi:10.14670/HH-20.205
Lee, S. C., Wang, S. Y., Li, C. C., and Liu, C. T. (2018). Anti-inflammatory effect of cinnamaldehyde and linalool from the leaf essential oil of Cinnamomum osmophloeum Kanehira in endotoxin-induced mice. J. Food Drug Analysis 26, 211–220. doi:10.1016/j.jfda.2017.03.006
Levine, R., Garland, D., Oliver, C., Amici, A., ClIment, I., Lenz, A. G., et al. (1990). Determination of carbonyl content in oxidatively modified proteins. Methods Enzym. 186, 464–478. doi:10.1016/0076-6879(90)86141-h
Lewis, S. M., Williams, A., and Eisenbarth, S. C. (2019). Structure and function of the immune system in the spleen. Sci. Immunol. 4 (33), eaau6085. doi:10.1126/sciimmunol.aau6085
Lin, C. T., Chen, C. J., Lin, T. Y., Tung, J. C., and Wang, S. Y. (2008). Anti inflammation activity of fruit essential oil from Cinnamomum insularimontanum Hayata. Bioresour. Technol. 99, 8783–8787. doi:10.1016/j.biortech.2008.04.041
Lynpatrick, N. (2006). Lead toxicity part-II: The role of free radical damage and the use of antioxidants in the pathology and treatment of lead toxicity. Altern. Med. Rev. 11 (2), 114–127.
Majoni, S. W., Lawton, P. D., Barzi, F., Cass, A., and Hughes, J. T. (2017). Assessing the association between serum ferritin, transferrin saturation, and C-reactive protein in northern territory indigenous Australian patients with high serum ferritin on maintenance haemodialysis. Int. J. Nephrol. 2017, 5490963. doi:10.1155/2017/5490963
Martikainen, L. E., Rahnasto-Rilla, M., Neshybova, S., Lahtela- Kakkonen, M., Raunio, H., and Juvonen, R. O. (2012). Interactions of inhibitor molecules with the human CYP2E1 enzyme active site. Eur. J. Pharm. Sci. 47 (5), 996–1005. doi:10.1016/j.ejps.2012.09.018
Meyerholz, D. K., and Beck, A. P. (2019). Fundamental concepts for semiquantitative tissue scoring in translational research. ILAR J. 59 (1), 13–17. doi:10.1093/ilar/ily025
Mishra, K. (2009). Lead exposure and its impact on immune system: A review. Toxicol. Vitro 23, 969–972. doi:10.1016/j.tiv.2009.06.014
Mishra, K. P., Singh, V. K., Rani, R., Yadav, V. S., Chandran, V., Srivastava, S. P., et al. (2003). Effect of lead exposure on the immune response of some occupationally exposed individuals. Toxicology 188, 251–259. doi:10.1016/s0300-483x(03)00091-x
Morgan, A. M., El-Ballal, S. S., El-Bialy, B. E., and El-Boraie, N. B. (2014). Studies on the potential protective effect of cinnamon against bi-sphenol A- and octylphenol-induced oxidative stress in male Albino rats. Toxicol. Rep. 1, 92–101. doi:10.1016/j.toxrep.2014.04.003
Mugahi, M. H. N., Heidari, Z., Sagheb, H. M., and Barbarestani, M. (2003). Effects of chronic lead acetate intoxication on blood indices of male adult rat. Daru.Pharm. J. 11 (4), 147–151.
Niphade, S. R., Asad, M., Chandrakala, G. K., Toppo, E., and Deshmukh, P. (2009). Immunomodulatory activity of Cinnamomum zeylanicum bark. Pharm. Biol. 47, 1168–1173. doi:10.3109/13880200903019234
Ohkawa, H., Oohishi, N., and Yagi, K. (1979). Assay for lipid peroxides in animal tissues by thiobarbituric acid reaction. Ann. Biochem. 95, 351–358. doi:10.1016/0003-2697(79)90738-3
Okada, K., Wangpoengtrakul, C., Tanaka, T., Toyokuni, S., Uchida, K., and Osawa, T. (2001). Curcumin and especially tetrahydrocurcumin ameliorate oxidative stress-induced renal injury in mice. J. Nutr. 131, 2090–2095. doi:10.1093/jn/131.8.2090
Oyem, J. C., Chris-Ozoko, L. E., Enaohwo, M. T., Otabor, F. O., Okudayo, V. A., and Udi, O. A. (2021). Antioxidative properties of Ocimum gratissimum alters Lead acetate induced oxidative damage in lymphoid tissues and hematological parameters of adult Wistar rats. Toxicol. Rep. 8, 215–222. doi:10.1016/j.toxrep.2021.01.003
Patra, R. C., and Swarup, D. (2000). Effect of lead on erythrocytic antioxidant defence, lipid peroxide level and thiol groups in calves. Res. Vet. Sci. 68, 71–74. doi:10.1053/rvsc.1999.0340
Patrick, L. (2006). Lead toxicity, a review of the literature. Part 1: Exposure, evaluation, and treatment. Altern. Med. Rev. 11 (1), 2–22.
Rice-Evans, C. A., Miller, N. J., and Papanga, G. (1997). Antioxidant properties of phenolic compounds. Trends Plant Sci. 2, 152–159. doi:10.1016/s1360-1385(97)01018-2
Rocha, C., Cavalcante, A. L. M., Hauser-Davis, R. A., Lopes, R. M., and Mattos, R. D. C. O. D. C. (2016). Effects of sub-lethal and chronic lead concentrations on blood and liver ALA-D activity and hematological parameters in Nile tilapia. Ecotoxicol. Environ. Saf. 129, 250–256. doi:10.1016/j.ecoenv.2016.03.028
Rouach, H., Fataccioli, V., GentilM., , French, S. W., MoriMotoM., , and NoRdmann, R. (1997). Effect of chronic ethanol feeding on lipid peroxidation and protein oxidation in relation to liver pathology. Hepatology 25 (2), 351–355. doi:10.1002/hep.510250216
Small, D. M., Coombes, J. S., Bennett, N., Johnson, D. W., and Gobe, G. C. (2012). Oxidative stress, anti-oxidant therapies and chronic kidney disease. Nephrology 17, 311–321. doi:10.1111/j.1440-1797.2012.01572.x
Smolen, A. J. (1990). “Image analytic techniques for quantification of immunocytochemical staining in the nervous system,” in Methods in neurosciences. Editor P. M. Conn (San Diego: Academic Press).
Soliman, M. M., Baiomy, A. A., and Yassin, M. H. (2015). Molecular and histopathological study on the ameliorative effects of curcumin against lead acetate-induced hepatotoxicity and nephrototoxicity in Wistar rats. Biol. Trace Elem. Res. 167, 91–102. doi:10.1007/s12011-015-0280-0
Subash-Babu, P., Alshatwi, A. A., and Ignacimuthu, S. (2014). Beneficial antioxidative and antiperoxidative effect of cinnamaldehyde protect streptozotocin-induced pancreatic -cells damage in wistar rats. Biomol. Ther. 22, 47–54. doi:10.4062/biomolther.2013.100
Sudjarwo, S. A., Sudjarwo, G. W., and Koerniasari, (2017). Protective effect of curcumin on lead acetate-induced testicular toxicity in Wistar rats. Res. Pharm. Sci. 12, 381–390. doi:10.4103/1735-5362.213983
Szkoda, J., and Zmudzki, J. (2005). Determination of lead and cadmium in biologicalmaterial by graphite furnace atomic absorption spectrometry method. Bull. Vet. Inst. Pulawy 49, 89–92.
Thrall, M. A., Weiser, G., Allison, R., and Campbell, J. M. (2012). Veterinary hematology and clinical chemistry. John Wiley and Sons.
Unnikrishnan, M. K., and Rao, M. N. (1992). Curcumin inhibits nitrite induced met hemoglobin formation. FEBS Lett. 301, 195–196. doi:10.1016/0014-5793(92)81246-i
Wang, Y., Juan, L. V., Ma, X., Wang, D., Ma, H., Chang, Y., et al. (2010). Specific Hemosiderin deposition in spleen induced by a low dose of cisplatin: Altered iron metabolism and its implication as an acute hemosiderin formation model. Curr. Drug Metab. 11 (6), 507–515. doi:10.2174/138920010791636149
Who, (1995). Environmental health criteria 165: Inorganic lead. Geneva, Switzerland: World Health Organization.
Yadollahi, A., and Zargaran, B. (2019). The beneficial effects of curcumin on cardiovascular diseases and their risk factors. Rev. Clin. Med. 6, 12–19.
Keywords: lead, cinnamon, curcumin, splenotoxicity, oxidative stress
Citation: Emam MA, Farouk SM, Aljazzar A, Abdelhameed AA, Eldeeb AA and Gad FA (2023) Curcumin and cinnamon mitigates lead acetate-induced oxidative damage in the spleen of rats. Front. Pharmacol. 13:1072760. doi: 10.3389/fphar.2022.1072760
Received: 17 October 2022; Accepted: 28 December 2022;
Published: 16 January 2023.
Edited by:
Aziza Abdel-Salam El-Nekeety, National Research Centre (Egypt), EgyptReviewed by:
Sekena Hassanien Abdel-Aziem, National Research Centre (Egypt), EgyptBasma Hamed Marghani, Department of Physiology Faculty of Veterinary Medicine Mansoura University Egypt, Egypt
Copyright © 2023 Emam, Farouk, Aljazzar, Abdelhameed, Eldeeb and Gad. This is an open-access article distributed under the terms of the Creative Commons Attribution License (CC BY). The use, distribution or reproduction in other forums is permitted, provided the original author(s) and the copyright owner(s) are credited and that the original publication in this journal is cited, in accordance with accepted academic practice. No use, distribution or reproduction is permitted which does not comply with these terms.
*Correspondence: Mahmoud Abdelghaffar Emam, bWFobW91ZC5odXNzZWluQGZ2dG0uYnUuZWR1LmVn