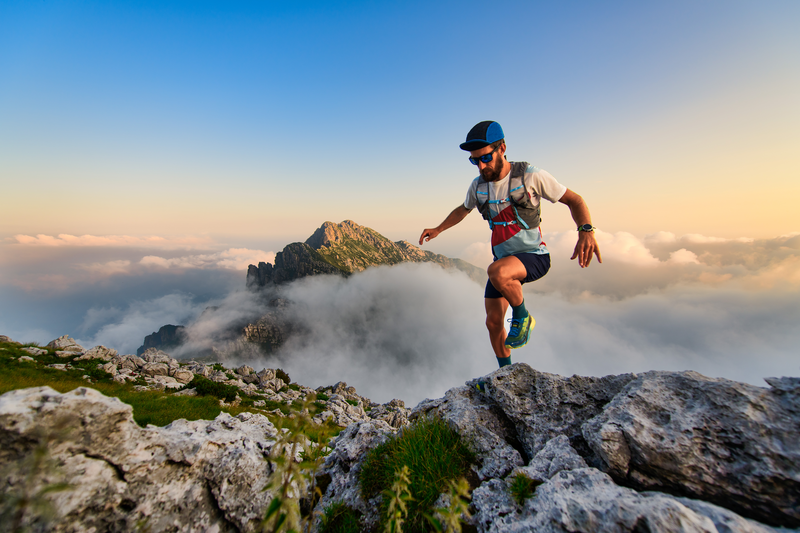
94% of researchers rate our articles as excellent or good
Learn more about the work of our research integrity team to safeguard the quality of each article we publish.
Find out more
REVIEW article
Front. Pharmacol. , 01 December 2022
Sec. Gastrointestinal and Hepatic Pharmacology
Volume 13 - 2022 | https://doi.org/10.3389/fphar.2022.1069310
This article is part of the Research Topic Natural Product Extracts in the Treatment of Gastrointestinal Cancer. View all 4 articles
Aquaporins (AQPs) are a family of transmembrane proteins expressed in various organ systems. Many studies have shown that the abnormal expression of AQPs is associated with gastrointestinal, skin, liver, kidneys, edema, cancer, and other diseases. The majority of AQPs are expressed in the digestive system and have important implications for the physiopathology of the gastrointestinal tract as well as other tissues and organs. AQP regulators can prevent and treat most gastrointestinal-related diseases, such as colorectal cancer, gastric ulcer, and gastric cancer. Although recent studies have proposed clinically relevant AQP-targeted therapies, such as the development of AQP inhibitors, clinical trials are still lacking and there are many difficulties. Traditional Chinese medicine (TCM) has been used in China for thousands of years to prevent, treat and diagnose diseases, and is under the guidance of Chinese medicine (CM) theory. Herein, we review the latest research on the regulation of AQPs by TCMs and their active components, including Rhei Radix et Rhizoma, Atractylodis macrocephalae Rhizoma, Salviae miltiorrhizae Radix et Rhizoma, Poria, Astragali radix, and another 26 TCMs, as well as active components, which include the active components include anthraquinones, saponins, polysaccharides, and flavonoid glycosides. Through our review and discussion of numerous studies, we attempt to explore the regulatory effects of TCMs and their active components on AQP expression in the corresponding parts of the body in terms of the Triple Energizer concept in Chinese medicine defined as “upper energizer, middle energizer, and lower energizer,”so as to offer unique opportunities for the development of AQP-related therapeutic drugs for digestive system diseases.
Aquaporins (AQPs) are a family of water channels/proteins that play an important role in water transport and metabolism in the body. The first AQP was discovered on human erythrocyte membranes in the 1980s (Benga, 2003, 2006). In the 1990s, other AQPs were discovered in plants, microorganisms, various animals, and humans (Benga, 2009). AQPs are widely distributed in numerous organs (e.g., the gastrointestinal tract, liver, eye lens, brain, kidneys, and lungs) and tissues of the human body. AQPs are involved in water absorption and secretion in the body, maintaining an equilibrium in the amount of intra- and extracellular water; in addition, they are involved in other important physiological activities, such as cell proliferation, migration and angiogenesis (Nagaraju et al., 2016; Liu et al., 2021).
The term “triple energizer” originates from the Yellow Emperor’s Inner Classic, which is the earliest traditional Chinese medical classic. And research proposes that the triple energizer organ is the largest tissue system linking all organs of the human body with a special tissue structure, and plays the role of a channel for stem cell reserve, water and fluid metabolism, immune regulation, and signal transmission (An et al., 2018). Many Chinese medical practitioners believe that above the diaphragm is the upper energizer, including the heart and lungs; below the diaphragm to the umbilicus is the middle energizer, including the spleen, stomach, liver, and gallbladder; and below the umbilicus up to the genitalia is the lower energizer, including the kidneys, large and small intestines, bladder, and woman’s uterus (Li et al., 2019a) (Figure 1). Water metabolism is the process of water production, transport, distribution and excretion. Chinese medicine (CM) theory suggests that water metabolism in the body involves a series of physiological functions of the viscera, particularly the coordination and cooperation of the “triple energizer” the lungs, spleen, kidneys, intestine, and bladder (Zhang et al., 2017b). Modern medicine believes that water metabolism requires AQPs, which are widely expressed in the spleen, lungs, kidneys, and digestive system and are closely related to water metabolism.
FIGURE 1. Division of the human internal organs according to the Triple Energizer concept of Traditional Chinese medicine.
Currently, at least 11 AQPs have been found in the stomach, small and large intestines (Lv et al., 2022) (Table 1). AQP1 is abundantly distributed in the endothelial cells of the gastrointestinal tract. AQP3 and AQP4 are mainly distributed in the basolateral membrane of gastric and intestinal epithelial cells. AQP7, AQP8, AQP10, and AQP11 are distributed in the apical of enterocytes in the small and large intestines (Zhu et al., 2016). According to CM, digestive system diseases are caused by exogenous evil-qi, internal injuries, diet, emotions, and viscera dysfunction. The application of CM theory to TCMs and their compounds suggests that most TCMs and TCM compounds can treat digestive system diseases by reducing pathogenic bacteria, improving the intestinal mucosal barrier, and inhibiting intestinal and systemic inflammation, thus safeguarding people’s digestive health (Zhang et al., 2022). Therefore, studies investigating the regulatory mechanism of TCM and its components on AQPs will have a significant impact on the development of aquaporin-related therapeutic drugs.
Research on the structure of the AQP family has been conducted, and many studies have reported on the various functions of AQPs in human health and disease (Ahmed and Ghafoor, 2021). The expression of AQPs is related to various diseases or pathological factors. It has been confirmed that AQP expression is involved in various pathological processes, such as intestinal, kidney, skin, and respiratory diseases and tumors. In the field of TCM, many scholars have conducted a series of studies on the correlation between AQPs and water metabolism. However, few studies have investigated the regulatory effects of TCMs and their main components on AQPs. Through their active components (e.g., anthraquinones, saponins, polysaccharides, and flavonoids), Rhei Radix et Rhizoma, Atractylodes macrocephala, Salviae miltiorrhizae Radix et Rhizoma, Poria, Scutellariae Radix, and Glycyrrhizae Radix et Rhizoma exert certain effects on diseases caused by water and electrolyte disorders by regulating the expression of AQPs. This review article summarizes the TCMs and their components that exert regulatory effects on AQPs. In addition, it provides a basis for further research on the treatment of AQP expression-related diseases through TCMs.
AQPs constitute a small and complete family of membrane proteins that promote water transport through the plasma membrane in response to osmotic gradients (Verkman et al., 2014). Each AQP has a structure comprising six transmembrane helices. According to the “hourglass model,” AQP monomers are arranged as tetramers in the plasma membrane: the four subunits are arranged in parallel, forming a fifth pore in the center of the tetramer (Kruse et al., 2006; Abir-Awan et al., 2019). Currently, 13 types of AQPs (AQP0–AQP12) have been described, which share the same characteristic amino acid sequence composed of the highly conserved motif asparagine-proline-alanine (Wittekindt and Dietl, 2019). AQPs are divided into three categories according to their functional characteristics. First, AQP0, AQP1, AQP2, AQP4, AQP5, and AQP8 are water-specific channels. Second, AQP3, AQP6, AQP7, AQP9, and AQP10 are permeable to water, urea, and glycerol. Finally, AQP11 and AQP12 are termed super AQPs because they share <15% structural homology with other AQPs (Cao et al., 2018; Xuan et al., 2021) (Table 2).
AQP1 was first discovered in erythrocytes and renal proximal tubules; it has been associated with pathological factors, such as cancer, inability to concentrate urine, and altered pain perception (Murata et al., 2000; Zou et al., 2013; Nave et al., 2016; Nakano et al., 2018; Cao et al., 2018). The expression of AQP1 in certain tumor types is associated with increased angiogenesis, invasion, metastasis, and proliferation (Verkman et al., 2014; Nave et al., 2016). Studies revealed that AQP1 is expressed in peripheral vascular endothelial cells and is involved in tumor angiogenesis. It is upregulated by angiogenic factors during hypoxia and is required for endothelial cell migration and angiogenesis (Nave et al., 2016; De Ieso and Yool, 2018). There is also evidence that AQP1 is expressed in choroid plexus epithelial cells and is closely related to the formation of cerebrospinal fluid (Oshio et al., 2005). Therefore, effective regulation of AQP1 plays an important role in the treatment of cancers.
AQP2 plays an important role in regulating the balance of water and other solutes in the body and is also a key protein that controls the permeability of the renal collecting duct (Kwon et al., 2013; Vukićević et al., 2016). Following an increase in plasma osmolality, the neurohypophysial hormone arginine vasopressin is secreted and binds to arginine vasopressin receptor 2 (AVPR2) in the principal cells of the renal collecting duct. This leads to the insertion of AQP2 in the apical plasma membrane, which is responsible for water reabsorption (Hj and Th, 2016; Ouyang et al., 2020). Dysregulation of AQP2 is closely associated with several clinical disorders characterized by disturbances in body water balance, including congenital nephrogenic diabetes insipidus (Li Q. et al., 2021), lithium-induced nephrogenic diabetes insipidus, electrolyte disturbances, acute and chronic renal function failure, ureteral obstruction, nephrotic syndrome, congestive heart failure, and liver cirrhosis (Kwon et al., 2013).
AQP3 is the most abundant aqua-glycerol-porin in the skin. Boury-Jamot et al. confirmed that AQP3 is expressed on the plasma membrane of human keratinocytes (Boury-Jamot et al., 2006). Studies have found that the overexpression of AQP3 is associated with skin malignancies, such as melanoma (Hara-Chikuma and Verkman, 2008). Hara-Chikuma et al. demonstrated that H2O2 is imported through AQP3 and participates in nuclear factor-κB (NF-κB) signal transduction in keratinocytes (Hara-Chikuma et al., 2015). Furthermore, AQP3 is involved in the pathogenesis of psoriasis. Recent experimental findings on whether systemic AQP3 deficiency causes vitiligo suggest that both systemic and localized AQP3 deficiency exists in vitiligo and correlates with disease severity and oxidative stress (Chandrashekar et al., 2021). In general, numerous studies have shown that AQP3 is closely related to human skin diseases and is of great significance for treatment.
Compared with other AQPs, AQP4 is characterized by relatively high single-channel water permeability (Yang et al., 1997). Similar to AQP1, AQP4 is the major water channel in the central nervous system and is considered a major regulator of cerebral fluid homeostasis in both healthy and pathophysiological conditions (Trillo-Contreras et al., 2019). It is mainly expressed in astrocytes (Nagelhus and Ottersen, 2013). AQP4 plays an important role in the pathology of brain diseases. Analysis of AQP4 knockout mice showed that the AQP4 gene is widely involved in brain water balance, neural excitation, glial scarring, neuroinflammation, neurodegeneration, and neurological mental illness (Verkman et al., 2017). Lan et al. confirmed that AQP4 may affect astrocyte calcium signaling and potassium homeostasis in the pathogenesis of Alzheimer’s disease (Lan et al., 2017). Moreover, AQP4 is related to the removal of interstitial β-amyloid and glutamate neurotransmission. Thus, an in-depth study of AQP4 is necessary to provide a reference for the treatment of various diseases.
AQP5 is present in airway epithelial cells, type I alveolar epithelial cells, and lung submucosal acinar cells (Verkman et al., 2000). It plays a role in many secretory glands (e.g., salivary, lacrimal, and sweat) (Day et al., 2014; Wang et al., 2020a), and is key in water transport. The high expression of AQP5 in breast cancer cells affects their invasive ability, proliferation, and metastatic potential (Jung et al., 2011). Downregulation of AQP5 expression can inhibit the proliferation and migration of ectopic endometrial epithelial cells, as well as the formation of endometrial cell nodules in nude mice (Xin et al., 2015). This evidence also suggests that the alteration of AQP5 expression is linked to tumor progression.
Originally, AQP0 was considered to be a membrane endogenous protein specifically expressed in lens fibers. It is the main endogenous protein of the lens termed MIP. Mutations and knockouts of AQP0 can lead to the development of lens cataracts. AQP0 functions as a water channel and adhesion molecule in lens fibers, resulting in a narrowing of the intercellular space of lens fibers, which is required for lens transparency and accommodation (Chepelinsky, 2009). AQP6 is selectively localized to the ganglion cell layer and outer plexiform layer in the rat retina (Jang et al., 2016); additionally, it is highly expressed in benign ovarian tumors (Ma et al., 2016). AQP7 is the most representative glycerol channel protein expressed in pancreatic β cells. It is involved in insulin secretion, triacylglycerol synthesis, and the proliferation of endocrine cells (Méndez-Giménez et al., 2018; Arsenijevic et al., 2019). Iena and Lebeck, 2018 found that AQP7 deficiency was associated with increased glycerol kinase activity and triglyceride accumulation in adipose tissue, leading to the secondary development of obesity and insulin resistance.
AQP8 is expressed in a variety of cancers (e.g., colorectal, breast, lung, and prostate) (Wu et al., 2018). AQP9 is a major factor in the pathway for glycerol uptake by hepatocytes (Jelen et al., 2011; Calamita et al., 2012). AQP9 is found in astrocytic processes and cell bodies and pathophysiologic conditions may regulate AQP9 expression (Badaut et al., 2002). Laforenza et al. showed that AQP10 is another glycerol channel expressed on the plasma membrane of human adipocytes (Laforenza et al., 2013). AQP11 is expressed in the testes, thymus, kidneys, liver, and intestines (Koike et al., 2016). Studies have shown that mice lacking AQP11 can develop polycystic kidney disease (Tanaka et al., 2016). The expression of AQP12 is associated with lower levels of pro-inflammatory cytokines, higher levels of anti-inflammatory cytokines, and the promotion of insulin secretion (da Silva et al., 2020).
Medicinals with the main efficacy of promoting defecation by purgation are known as purgative medicinals (Zhang and Ye, 2020).
Rhei Radix et Rhizoma (State Pharmacopoeia Commission., 2020; Zhang and Ye, 2020) is the main purgative medicine, which acts on the spleen, stomach, large intestine, and liver meridians and exerts various pharmacological effects (e.g., purgative, antipyretic, antibacterial, diuretic, and choleretic). It also can act on upper energizer, middle energizer, and lower energizer. The major purgative site of Rhei Radix et Rhizoma is the colon (LI et al., 2008). Bao et al. (2021) established a model of diarrhea in mice by gavage of Rhei Radix et Rhizoma. The results showed that Rhei Radix et Rhizoma can lead to the downregulation of AQP3 and AQP4 expression, reduce the absorption of water in the colon, and increase the water content of the intestinal tract. Wang et al. (2021) analyzed the metabolites of rhubarb anthraquinone and its chemical marker sennoside A in a Rhei Radix et Rhizoma diarrhea model. The results showed that rhubarb anthraquinone could downregulate the expression of various AQPs in the colon, kidneys, and liver of rats with diarrhea. Sennoside A, a component in Rhei Radix et Rhizoma, can reduce the expression of AQP3 in the colon, thereby inhibiting the transport of water from the intestinal lumen to the vascular side and exerting a laxative effect (Kon et al., 2014). Peng et al. (2017) showed that emodin can reduce the release of inflammatory factors, downregulate the expression of AQP4, reduce edema around the brain tissue after intracerebral hemorrhage, reduce the apoptosis of neurons, and promote the repair of nerve function. Kang et al. (2017) have found that Dahuang-Fuzi-Tang could ameliorate pulmonary and intestinal edema and injury induced by severe acute pancreatitis by up-regulating different AQPs in the lungs and intestines.
Cao et al. prepared senna leaf extract (SE), sennoside, and sennoside A, and used them to establish a rat model of diarrhea (Cao et al., 2018). Using quantitative reverse-transcription polymerase chain reaction (RT-PCR), they determined and analyzed the expression profiles of AQP in six organs, namely the colon, kidneys, liver, spleen, lungs, and stomach. It was found that the expression of AQPs in the kidneys and liver of rats in the SE group was upregulated, whereas that of AQPs in the colon of the rats in the sennoside group was significantly downregulated. These findings indicated that SE and sennoside exert important effects on the regulation of AQPs.
Esculentoside A is the most abundant triterpenoid saponin in Phytolaccae Radix (Cui et al., 2014), and has immunomodulatory, anti-inflammatory, anti-glomerular sclerosis, and diuretic pharmacological effects. Li et al. (2015) investigated the regulatory effect of esculentoside A on the expression of AQP2 and AQP4 in the kidneys of rats treated with water loading. The results showed that esculentoside A reduced the reabsorption of water by downregulating the expression of AQP2, AQP4, and their mRNA levels in the kidneys of rats to achieve a diuretic effect.
Medicinals with the major efficacies of supplementing the deficiency, reinforcing weakness, supplementing qi, blood, yin, and yang are known as supplementation medicinals (Zhang and Ye, 2020).
Studies using slow transit constipation (STC) rats as an animal model found that the expression of AQP3 in the colon of STC rats was reduced and AQP4 was increased compared with that of normal rats. These results suggested that the treatment of Atractylodis macrocephalae Rhizoma on STC may be associated with the inhibition of AQP4 expression and the increase of AQP3 expression (Chen et al., 2019). Atractylenolide I, the main component of Atractylodis macrocephalae Rhizoma, can regulate the expression of AQP3 and AQP4 in the colon of STC rats and reduce intestinal water absorption (Jiang, 2017). Zhang concluded that the mechanism underlying the effects of Atractylodis macrocephalae Rhizoma in the treatment of spleen deficiency and diarrhea may be the regulation of AQP3 expression (Zhang, 2012). Through this effect, the Atractylodis macrocephalae Rhizoma improves the function of intestinal water metabolism. Xia et al. (2022) found that the volatile oil of Atractylodis macrocephalae Rhizoma and Ginseng Radix et Rhizoma could up-regulate the expression of AQP3 and AQP4 to treat chronic atrophic gastritis in rats.
Astragali radix mainly contains astragalus polysaccharides, saponins, and flavonoids, as well as other active components (Lv et al., 2021). Astragali radix mainly acts on upper energizer and middle energizer. Astragali radix is a classic drug mainly used for the treatment of digestive tract-related inflammation and tumors (Sun R.-L. et al., 2021). Mao et al. (2010) prepared a diabetic rat model by intraperitoneal injection of streptozotocin. The results demonstrated that astragalus polysaccharide can downregulate the overexpression of AQP2 in the renal medulla, relieve water and sodium retention in diabetes mellitus, and protect the kidneys. Zhao, 2009 used immunohistochemistry and western blotting to examine the changes in AQP1 and AQP5 expression in the lungs of acute lung injury (ALI) rats induced by bacterial endotoxin. Alphaside upregulated the expression of AQP1 and AQP5 in the lungs of ALI rats. Li et al. (2016) established an oxygen-glucose deprivation and reoxygenation model and pointed out that astragaloside I may affect the expression of AQP4 by downregulating the expression of tumor necrosis factor-α (TNF-α), thereby affecting astrocyte edema.
Ginseng Radix et Rhizoma contains the main components ginsenosides, ginseng polysaccharides, ginseng volatile oil and other trace elements, proteins, amino acids, vitamins, and other types of compounds. Modern medical research has shown that Ginseng Radix et Rhizoma can improve human immunity and cardiovascular disease, and exerts anti-aging, anti-tumor, hypoglycemic, and other effects (Wang, 2019). Studies found that ginsenoside Rg3 can reduce the levels of inflammatory mediators in the peripheral blood of rectal polyp model rats, as well as increase the expression of AQP3 and AQP4 in rectal polyp tissue (Xie et al., 2019a; 2019b). The use of panaxadiol saponins in severe acute pancreatitis can inhibit the expression of TNF-α and interleukin-6 (IL-6), reduce blood concentration, improve microcirculation, and upregulate the expression of AQP1 in lung tissue, thereby reducing pulmonary edema and lung injury (Zhao et al., 2009). Ginsenoside Rb1 can reduce body weight and improve glucose and lipid metabolism by upregulating the peroxisome proliferator-activated receptor gamma (PPARγ) and AQP7 protein levels (Guo et al., 2020). It can also relieve spinal cord edema and improve neurological function by increasing the expression of AQP4 (Li Y.-N. et al., 2019).
The main components of Glycyrrhizae Radix et Rhizoma include flavonoids, triterpenes, and alkaloids; these components include the pentacyclic triterpenoid 18β-glycyrrhetinic acid. Liu et al., 2020 established a model of allergic rhinitis (AR) in rats by sensitization with ovalbumin. A therapeutic effect against AR in rats may be achieved by changing the content of inflammatory factors, increasing the expression of AQP5 in the nasal mucosa, and reducing the expression of mucin 5AC, oligomeric mucus/gel-forming.
Angelicae Sinensis Radix, a traditional medicinal plant, is mainly used for nourishing blood, relieving pain, moistening the intestines, and treating irregular menstruation and amenorrhea in women (Wei et al., 2016). N-butylidenephthalide is a bioactive compound in Angelicae Sinensis Radix. Liao et al. have found that N-butylidenephthalide caused gastric cancer cell death through the activation of the mitochondria-intrinsic pathway and induced the REDD1 expression leading to mTOR signal pathway inhibition in gastric cancer cells (Liao et al., 2018). Du et al. explored the mechanism of action of Angelicae Sinensis Radix and laxative through a mouse model of blood deficiency and constipation (Du et al., 2018a, 2017; 2018b). They concluded that three doses of Angelicae Sinensis Radix decoction can significantly downregulate the expression of AQP4 and AQP8 in the colon. The mechanism underlying this effect may be related to the regulation of the colon phospholipase-C-inositol 1,4,5-trisphosphate-calmodulin (PLC-IP3-CaM) signaling pathway and the adenylate cyclase/cyclic adenosine monophosphate/protein kinase A (AC-cAMP-PKA) signaling pathway by Angelicae Sinensis Radix, thereby reducing the reabsorption of colonic water. Angelicae polysaccharide and angelica volatile oil may be important components of Angelicae Sinensis Radix for exerting its “laxative” effect (Du et al., 2018c). Researchers established a mouse model of asthma and concluded that Angelicae Sinensis Radix can promote the expression of AQP1 and its gene in lung tissue, thereby exerting a certain therapeutic effect on Yin deficiency asthma (Wang et al., 2016).
Modern pharmacological studies have found that total glucosides of Paeoniae Radix Alba, the active component of Paeoniae Radix Alba, can enhance the amplitude of colon contraction and prolong the contraction time (Yang et al., 2002). Fang and Gao, 2017 explored the laxative effect and mechanism of Paeoniae Radix Alba by establishing an STC mouse model. The results showed that high and medium doses of Paeoniae Radix Alba could significantly reduce the expression of AQP4 in the colon of STC mice and enhance the intestinal water to achieve a laxative effect.
Cistanches Herba mainly acts on lower energizer. Tao et al., 2015 suggested that phenylethanoid glycosides from Cistanche can reduce the water content of brain tissue and the expression of AQP4 mRNA in the brain tissue of rats presenting high-altitude cerebral edema. These findings indicated that phenylethanoid glycosides from Cistanche can prevent the occurrence of high-altitude cerebral edema, and this effect may be related to the inhibition of AQP4 expression. Cistanches Herba can simultaneously regulate AQP1 in the lungs and kidneys and enhance the regulatory effect on water metabolism (Zhang et al., 2012).
Researchers have established a model of Sjögren’s syndrome (SS) mice to investigate the mechanism through which Ophiopogon japonicus polysaccharide can regulate the inflammatory microenvironment of the submandibular gland in SS. Using this model, Ophiopogon japonicus polysaccharide could significantly increase the expression levels of AQP5, thereby regulating the submandibular gland inflammatory microenvironment and improving SS (Tao et al., 2014). Sun et al. showed that the effective components of Ophiopogonis Radix (polysaccharides and saponins) could upregulate the expression of AQP1 and AQP5 in the lung tissue of mice with Mycoplasma pneumonia, reduce the pathological changes in lung tissue, and play a role in moisturizing the lungs and producing fluid (Sun Y. et al., 2021).
Medicinals with the main functions of regulating water passages, as well as draining water and dampness are called damp-draining diuretic medicinals (Zhang and Ye, 2020).
The main active components of Poria (i.e., polysaccharides and triterpenes) are commonly used clinically to treat dysuria, edema, diarrhea due to spleen deficiency, loose stools, palpitations, and insomnia (Li et al., 2022). Li et al. 2021a analyzed the dampness effect of Poria from different origins on kidney-Yang deficiency and lower energizer edema in rats. The levels of AQP1 and AQP2 in the renal medulla of the rats in each administration group were decreased; the serum anti-diuretic hormone levels were also significantly decreased. These results indicated that Poria may regulate AQP1- and anti-diuretic hormone-AQP2-related pathways to achieve its diuretic and osmotic effects. Zhang established a model of diarrhea due to spleen deficiency in rats and studied the regulatory effect of Poria on water metabolism by measuring the expression levels of AQP3 and AQP4 in the jejunum and colon mucosa (Zhang, 2012). The results indicated that Poria may enhance the reabsorption of water in colonic mucosa by upregulating the expression of AQP3 in colonic mucosa.
Alismatis Rhizoma facilitates urination and clears dampness and heat, and mainly acts on lower energizer. Wu et al. (2010) observed the relative expression of AQP2 mRNA in the renal medulla using RT-PCR after administration of the water extract of Alismatis Rhizoma in rats. The results showed that the AQP2 mRNA expression could be significantly reduced after eight consecutive administrations of Alismatis Rhizoma water extract to rats, indicating that the diuretic activity of Alismatis Rhizoma may be produced by regulating the reduction of AQP2 expression in the renal medulla. Ding et al. have found that Qutanhuoxue Decoction could upregulate AQP7 expression in adipocytes and downregulate AQP9 expression in hepatocytes to treat Nonalcoholic fatty liver disease (Zheng et al., 2019). And the composition of Qutanhuoxue Decoction inclouding Poria, Alismatis Rhizoma, Astragali radix, Coicis Semen, Bupleuri Radix, and another 6 TCMs.
Zhang et al. confirmed that the water extract of Polyporus sclerotiorum and Polyporus polysaccharide exerts obvious inhibitory effects on bladder cancer (Zhang et al., 2011). Researchers used Polyporus, combining N-butyl-N-(4-hydroxybutyl)-nitrosamine with saccharin, to establish a bladder cancer model in Fisher-344 rats (Zhang and Zhang, 2013). The expression of AQP1 and AQP3 in bladder cancer tissue was measured by immunohistochemistry and RT-PCR. The results showed that Polyporus could significantly downregulate the relative expression of AQP1 and AQP3 mRNA in bladder cancer tissue of rats, indicating that the anti-bladder cancer effect may be produced by regulating AQP1 and AQP3 in bladder cancer tissue.
Coixenopolysaccharide is the most abundant functional component in Coicis Semen. Wang Y. et al., 2018 explored the mechanism of Coixenopolysaccharide underlying the regulation of water metabolism in rats with dampness stagnancy due to spleen deficiency by replicating the rat model of spleen deficiency. The results showed that Coixenopolysaccharide upregulated the expression of AQP3 in colon tissue. The regulatory effect of Coixenopolysaccharide on AQP3 may be one of the mechanisms involved in the treatment of spleen deficiency and dampness in rats.
Yan et al. investigated the diuretic activity of Plantaginis Semen and reduced the expression of AQP2 and AQP1 in the renal medulla through a model of rats treated with water loading (Yan et al., 2014). Wang et al. used Sennae Folium to replicate the model of diarrhea in rats and studied the effect of Plantaginis Semen on the expression of AQP1 mRNA and protein in the small intestine of rats with diarrhea (Wang et al., 2020c). The results showed that the mechanism of Plantaginis Semen involved in relieving diarrhea is related to the upregulation of AQP1 expression, an increase in the absorption of water in the small intestine, and the promotion of water metabolism. Wang et al. showed that the mechanism may be related to the inhibition of the inflammatory response, repair of pathological damage to the colonic mucosa, and upregulation of AQP4 expression (Wang et al., 2020b).
Medicinals that can stop various forms of bleeding, internal or external, are called blood-stanching medicinals (Zhang and Ye, 2020).
Panax notoginseng saponins (PNS) are the main pharmacologically active components of Notoginseng Radix et Rhizoma. Li et al. found that PNS could inhibit the expression of caspase 1 (CASP1) and CASP3 proteins after cerebral ischemia-reperfusion, thereby playing a role in the protection of the brain (Li et al., 2006). Meng et al. measured the expression of AQP4 protein and mRNA in rats with cerebral hemorrhage by immunohistochemical staining and RT-PCR, respectively (Meng et al., 2007). It is hypothesized that PNS may reduce the formation of cerebral edema after intracerebral hemorrhage by inhibiting the expression of AQP4.
Medicinals with the main efficacy of facilitating blood vessels, promoting blood circulation and removing blood stasis are called blood-invigorating and stasis-dissolving medicinals (Zhang and Ye, 2020).
Salviae miltiorrhizae Radix et Rhizoma is commonly used in TCM to promote blood circulation and remove blood stasis. It eliminates blood stasis and improves microcirculation by dilating blood vessels and accelerating blood flow (Wang et al., 2015). Hu et al. reported that it can significantly reduce the expression of AQP2 in the kidney tissue of diabetic rats and increase the expression of AQP1 in the lungs (Hu et al., 2011). It is hypothesized that the protective effect of Salviae miltiorrhizae Radix et Rhizoma on the kidneys of diabetic rats may be related to the regulation of AQP1 and AQP2. Other studies demonstrated that tanshinone alleviated cerebral edema caused by brain injury through the inhibition of AQP4 induction by inflammatory factors (Huang Y. et al., 2020; Tang et al., 2022). In ALI and chronic lung injury, Salviae Miltiorrhizae Radix et Rhizoma can increase the expression of AQP1 in pulmonary capillaries, improve blood rheology, alleviate pathological changes in lung tissue, and alleviate the occurrence and development of lung injury to a certain extent (Li et al., 2009).
The main active components of Curcumae Longae Rhizoma are curcumin and volatile oil, which have anti-inflammatory, hypolipidemic, and anti-tumor properties (Ghandadi and Sahebkar, 2017). Lin established a model of focal cerebral ischemia-reperfusion in rats. Treatment with curcumin downregulated the expression of vascular endothelial growth factor (VEGF) and AQP4 (Lin, 2015). These findings indicated that the protective effect of curcumin on the cerebral ischemia-reperfusion injury was related to the downregulation of VEGF and AQP4 expression and alteration of the permeability of the blood-brain barrier (BBB).
Medicinals that can disperse exterior pathogens are known as exterior-releasing medicinals (Zhang and Ye, 2020).
Puerarin is a flavonoid glycoside isolated from the root of the TCM Puerariae Lobatae Radix. Many researchers found that puerarin has a protective effect on ischemia-reperfusion injury of the heart, brain, spinal cord, lung, intestine, and other organs (Gao et al., 2022). Studies have shown that puerarin may reduce the water channel function of AQP4 by regulating the cAMP/PKA/AQP4 pathway, thereby reducing subarachnoid hydrops (Ying and Liu, 2020). It may also inhibit the release of TNF-α and the phosphorylation of NF-κB and mitogen-activated protein kinase (MAPK) pathways, thereby inhibiting the upregulation of AQP4 expression during hypobaric hypoxia (Wang C. et al., 2018). In addition, puerarin affects the water transport of esophageal cancer cells by upregulating the expression of AQP1 mRNA (Jiang et al., 2011).
Saikosaponin a (SSa) is the main active component of Bupleurum Radix, exerting extensive pharmacological effects (e.g., anti-inflammatory, immunomodulatory, and antibacterial). Zhu et al. used the modified Allen heavy blow method to create a model of acute spinal cord injury in rats and explored the possible impact mechanism of SSa on the early stage of the injury (Zhu et al., 2017a). The results indicated that SSa may inhibit the NF-κB signaling pathway and the expression of AQP4. Moreover, it can reduce the inflammatory response and tissue edema after acute spinal cord injury, thus protecting the nerves.
The main component of Asari Radix et Rhizoma volatile oil is methyl eugenol. Wu et al. (2019) . established a model of AR in rats to observe the effect of methyl eugenol on the expression of AQP in the nasal mucosa. The results showed that methyl eugenol could upregulate the expression of AQP5 in the nasal mucosa of rats, thereby regulating the secretions by nasal mucosa glands in rats with AR and relieving their nasal symptoms.
Medicinals with the main function of clearing interior heat are called heat-clearing medicinals, which are usually to treat interior heat syndrome (Zhang and Ye, 2020).
The main effects of Cassiae semen are to clear the liver fire, improve eyesight, and moisten the intestine to promote defecation. Modern pharmacological studies have shown that it also has antihypertensive, lipid-lowering, liver-protecting, and anti-oxidative effects (Dong et al., 2021). Liu et al. (2015) established a mouse model of STC and explored the effects of Cassiae semen on colon electromyography activity and the expression of AQP3 in the colonic mucosa of mice with STC. The results showed that Cassia semen can reduce the expression of AQP3 in colonic mucosa and effectively improve colonic motor function in mice. Studies on Cassiae semen have shown that anthraquinones are the main components exhibiting laxative and lipid-lowering effects (Luo et al., 2011; Yu et al., 2022).
Baicalin is a flavonoid glycoside and the main active component of Scutellariae Radix. Zheng et al. (2022). recently reported the mechanism underlying the effects of baicalin in the treatment of cerebral ischemia-reperfusion injury and cerebral edema from the perspective of inhibiting astrocyte swelling. It was shown that the expression of glial fibrillary acidic protein (GFAP), transient receptor potential vanilloid 4 (TRPV4), and AQP4 in the cerebral cortex of rats was significantly reduced after treatment with low-dose baicalin. It is hypothesized that baicalin can effectively improve the cerebral edema caused by cerebral ischemia-reperfusion injury in rats. Thus, the effect may be related to the inhibition of astrocyte swelling and the opening of TRPV4 and AQP4 channels. Tu et al. showed that baicalin can inhibit the expression of TNF-α and AQP4 in the brain tissue of rats with ischemic brain injury, reduce the inflammatory response, repair the damaged BBB, and exert a protective effect on ischemic brain injury in rats (Tu et al., 2013).
Picrorhizae Rhizoma protects the liver, gallbladder, and neurons, and exerts anti-inflammatory and antiviral effects. Picroside II is its main component, exhibiting the highest levels. Studies established a rat model of forebrain ischemia by bilateral common carotid artery ligation to explore the effect of picroside II on the expression of AQP4 in the brain tissue of rats with ischemic injury (Jing et al., 2013; Chen et al., 2016). The results showed that the expression of AQP4 was significantly reduced in the brain tissue of the treatment group. It is hypothesized that picroside II can reduce the damage caused by cerebral ischemia to the BBB and nerve cells by downregulating the expression of AQP4.
With dispelling or dispersing phlegm and relieving or alleviating cough and panting as major efficacies, the drugs which are often used to treat phlegm, cough and panting syndromes are called phlegm-dissolving and cough- and panting-relieving medicinals (Zhang and Ye, 2020).
It has been found that Pinellia extract (EP) or compound Pinellia decoction regulates the expression of AQP in lung tissue (Deng et al., 2009). Zhou et al. explored the pharmacological mechanism of EP in the treatment of ALI (Zhou et al., 2015). The results showed that EP could reduce pulmonary edema by upregulating the expression of AQP5. This mechanism of action may be activated to inhibit the inflammatory factor TNF-α. A new study has also shown that the polysaccharide in aqueous decoction of Pinelliae Rhizoma can reduce the expression of AQP5 mRNA in colon tissue of rats in an asthma model, thus interfering with the asthma process (Huang et al., 2020a) (Figures 2, 3).
FIGURE 2. Therapeutic or pharmacological effects of TCM on diseases of the corresponding aspects of the “Triple Energizer” concept.
This review article summarizes the active components of TCMs that can regulate AQPs. They are mainly used to treat intestinal disease, diabetes, various types of edema, cerebral ischemia, esophageal cancer, bladder cancer, and other diseases. The therapeutic effects are achieved by upregulating or downregulating the expression of AQPs in related tissues and organs; moreover, these drugs tend to regulate the expression of AQP1-5, especially that of AQP4 (Table 3 and 4). The treatment of TCMs mainly concentrated on the middle energizer and lower energizer. Most AQPs are expressed in the gastrointestinal tract and are involved in the progresses of gastric cancer, colon cancer, diarrhea, constipation, inflammatory bowel disease, and other diseases. Therefore, it is of practical significance to review the current research on the regulation of AQPs to treat digestive system diseases by TCMs and their components.
First, abundant studies have proved that the expression of AQPs is related to digestive system tumors, such as gastric cancer, colorectal cancer, esophageal cancer, liver cancer, and pancreatic cancer. There is also evidence that TCM can treat gastrointestinal tumor disease by regulating AQP expression, such as Puerariae Lobatae Radix and its components can treat esophageal cancer. However, the treatment of AQP-related diseases by TCMs and their components mentioned in our review have only been assessed in preliminary pharmacological studies, and there are not yet adequate data on the mechanisms of TCM regulating AQPs to treat digestive system diseases. Second, there is limited evidence regarding the pharmacokinetics, toxicity, and clinical efficacy of these reported TCMs or their components. At present, research on the regulation of AQPs is focused more on the combination of TCMs, rather than on treatment with a single TCM. Therefore, despite continuous progress in this field, additional studies are needed to develop new drugs from these medicines for the treatment or prevention of diseases. In summary, the specific mechanisms involved in the regulation of AQPs by TCMs and their components warrant further investigation. This may make TCMs or AQP regulators become a new treatment for digestive diseases. We hope this review may provide directions for the development of new drugs from TCMs for the treatment of diseases caused by abnormal water metabolism.
Writing—original draft preparation, YH and EH; Writing—review and editing, YH, EH, and SY; Discussion, ZS, LX, JX, and FZ. All authors have read and agreed to the published version of the manuscript.
This work is supported by the National Natural Science Foundation of China (Grant No: 81960730), Guangxi Innovation-Driven Development Project (Grant No: GUIKEAA18242040), Guangxi Innovation-Driven Development project (Grant No: GUIKEAA19254033).
The authors declare that the research was conducted in the absence of any commercial or financial relationships that could be construed as a potential conflict of interest.
All claims expressed in this article are solely those of the authors and do not necessarily represent those of their affiliated organizations, or those of the publisher, the editors and the reviewers. Any product that may be evaluated in this article, or claim that may be made by its manufacturer, is not guaranteed or endorsed by the publisher.
ALI, Acute lung injury; AQP, Aquaporin; BBB, Blood-brain barrier; BBN, N-butyl-N-(4-hydroxybutyl)-nitrosamine; DM, Diabetes mellitus; EP, Pinellia extract; PNS, Panax notoginseng saponins; RT-PCR, Reverse transcription polymerase chain reaction; SS, Sjögren’s syndrome; SSa, Saikosaponin a; STC, Slow transit constipation; TCM, Traditional Chinese medicine; CM, Chinese medicine; TRPV4, Transient receptor potential vanilloid four; VEGF, Vascular endothelial growth factor.
Abir-Awan, M., Kitchen, P., Salman, M. M., Conner, M. T., Conner, A. C., and Bill, R. M. (2019). Inhibitors of mammalian aquaporin water channels. Int. J. Mol. Sci. 20, E1589. doi:10.3390/ijms20071589
Ahmed, T., and Ghafoor, S. (2021). Aquaporins; systemic, functional and therapeutic correlations in health and disease. J. Pak. Med. Assoc. 71, 1228–1233. doi:10.47391/JPMA.01-063
An, X., Chen, Y., Li, N., Fan, J., Zhu, R., and Zhao, C. (2018). Modern cell biology research interprets the medical essence of the triple energizer/mesenchymal tissue system. Basic Clin. Med. 38, 1599–1605. doi:10.3969/j.issn.1001-6325.2018.11.021
Arsenijevic, T., Perret, J., Van Laethem, J.-L., and Delporte, C. (2019). Aquaporins involvement in pancreas physiology and in pancreatic diseases. Int. J. Mol. Sci. 20, E5052. doi:10.3390/ijms20205052
Badaut, J., Lasbennes, F., Magistretti, P. J., and Regli, L. (2002). Aquaporins in brain: Distribution, physiology, and pathophysiology. J. Cereb. Blood Flow. Metab. 22, 367–378. doi:10.1097/00004647-200204000-00001
Bao, J., Zhao, W., Lu, Y., He, X., and Liu, H. (2021). Effects of rheum palmatum L.on aquaporin 3/4 and vasoactive intestinal peptide on mice. Prog. Veterinary Med. 42, 54–58. doi:10.3969/j.issn.1007-5038.2021.03.011
Benga, G. (2003). Birth of water channel proteins-the aquaporins. Cell Biol. Int. 27, 701–709. doi:10.1016/s1065-6995(03)00171-9
Benga, G. (2009). Water channel proteins (later called aquaporins) and relatives: Past, present, and future. IUBMB Life 61, 112–133. doi:10.1002/iub.156
Benga, G. (2006). Water channel proteins: From their discovery in 1985 in cluj-napoca, Romania, to the 2003 nobel prize in chemistry. Cell. Mol. Biol. 52, 10–19.
Boury-Jamot, M., Sougrat, R., Tailhardat, M., Le Varlet, B., Bonté, F., Dumas, M., et al. (2006). Expression and function of aquaporins in human skin: Is aquaporin-3 just a glycerol transporter? Biochim. Biophys. Acta 1758, 1034–1042. doi:10.1016/j.bbamem.2006.06.013
Calamita, G., Gena, P., Ferri, D., Rosito, A., Rojek, A., Nielsen, S., et al. (2012). Biophysical assessment of aquaporin-9 as principal facilitative pathway in mouse liver import of glucogenetic glycerol. Biol. Cell 104, 342–351. doi:10.1111/boc.201100061
Camilleri, M., Carlson, P., Chedid, V., Vijayvargiya, P., Burton, D., and Busciglio, I. (2019). Aquaporin expression in colonic mucosal biopsies from irritable bowel syndrome with diarrhea. Clin. Transl. Gastroenterol. 10, e00019. doi:10.14309/ctg.0000000000000019
Cao, Y., He, Y., Wei, C., Jing, L., Qu, L., Zhang, H., et al. (2018). Aquaporins alteration profiles revealed different actions of Senna, sennosides, and sennoside A in diarrhea-rats. Int. J. Mol. Sci. 19, E3210. doi:10.3390/ijms19103210
Chandrashekar, L., Rajappa, M., Rajendiran, K. S., and Munisamy, M. (2021). Is vitiligo associated with systemic aquaporin-3 deficiency? Postepy Dermatol. Alergol. 38, 156–158. doi:10.5114/ada.2021.104291
Chen, H., Liu, Y., and Zhao, L. (2016). Protective effect of picroside Ⅱ on cerebral ischemia reperfusion injury in rats and the expression of MMP-9 and AQP4. J. Emerg. Traditional Chin. Med. 25, 60–63. doi:10.3969/j.issn.1004-745X.2016.01.021
Chen, S., Guan, L., Pang, J., Chen, T., and Tang, X. (2019). Rhizoma Atractylodis macrocephalaee has effect on expression of aquaporins in the colon of model rats with slow transit constipation. J. New Chin. Med. 51, 12–16. doi:10.13457/j.cnki.jncm.2019.06.004
Chepelinsky, A. B. (2009). Structural function of MIP/aquaporin 0 in the eye lens; genetic defects lead to congenital inherited cataracts. Handb. Exp. Pharmacol. 2009. 265–297. doi:10.1007/978-3-540-79885-9_14
Cui, N., Meng, X., Ma, J., Li, K., and Zhang, S. (2014). Acute toxicity test and diuresis effect of esculentoside A. Her. Med. 33, 981–984. doi:10.3870/yydb.2014.08.001
da Silva, I. V., Cardoso, C., Méndez-Giménez, L., Camoes, S. P., Frühbeck, G., Rodríguez, A., et al. (2020). Aquaporin-7 and aquaporin-12 modulate the inflammatory phenotype of endocrine pancreatic beta-cells. Arch. Biochem. Biophys. 691, 108481. doi:10.1016/j.abb.2020.108481
Day, R. E., Kitchen, P., Owen, D. S., Bland, C., Marshall, L., Conner, A. C., et al. (2014). Human aquaporins: Regulators of transcellular water flow. Biochim. Biophys. Acta 1840, 1492–1506. doi:10.1016/j.bbagen.2013.09.033
De Ieso, M. L., and Yool, A. J. (2018). Mechanisms of aquaporin-facilitated cancer invasion and metastasis. Front. Chem. 6, 135. doi:10.3389/fchem.2018.00135
Deng, Q., Zhou, J., Guo, Z., and Zhang, P. (2009). Airway mucus hypersecretion in rats is inhibited by the extracts of Pinellia. Chin. J. Respir. Crit. Care Med. 8, 477–481. doi:10.3969/j.issn.1671-6205.2009.05.016
Deng, Z., Zhao, Y., Ma, Z., Zhang, M., Wang, H., Yi, Z., et al. (2021). Pathophysiological role of ion channels and transporters in gastrointestinal mucosal diseases. Cell. Mol. Life Sci. 78, 8109–8125. doi:10.1007/s00018-021-04011-5
Dong, Y., Jiang, Y., Liu, Y., Chen, J., Ge, X., Tian, C., et al. (2021). Research progress on chemical composition and pharmacological effects of Cassiae Semen and predictive analysis on quality markers. Chin. Traditional Herb. Drugs 52, 2719–2732. doi:10.7501/j.issn.0253-2670.2021.09.014
Du, L., Luo, J., Wu, G., Niu, T., Li, Y., and Yuan, J. (2018a). Effects of angelica sinensis radix on expressions of AQP8 in colon of blood-deficiency constipation in mice. Chin. J. Inf. Traditional Chin. Med. 25, 44–48. doi:10.3969/j.issn.1005-5304.2018.07.011
Du, L., Luo, J., Wu, G., Niu, T., Shao, J., and Wang, R. (2017). The impact of Angelica sinensis on the expression of AQP4 in colon of Xuexu constipation in mice. Pharmacol. Clin. Chin. Materia Medica 33, 103–107. doi:10.13412/j.cnki.zyyl.2017.05.027
Du, L., Ren, Y., Wu, G., Niu, T., Wang, Z., and Shao, J. (2018b). Effect of Angelica sinensis radix on colonic morphology and mucus secretion of experimental XuexuBianmi model mice. Chin. J. Appl. Physiology 34, 367–370. doi:10.12047/j.cjap.5616.2018.084
Du, L., Wu, G., Niu, T., Wang, Z., Shao, J., and Ren, Y. (2018c). Experimental study on the medicinal material base of Angelica sinensis in nourishing bowel to relieve constipation. J. Gansu Univ. Chin. Med. 35, 11–16. doi:10.16841/j.issn1003-8450.2018.03.03
Duan, H., Hao, C., Fan, Y., Wang, H., Liu, Y., Hao, J., et al. (2013). The role of neuropeptide Y and aquaporin 4 in the pathogenesis of intestinal dysfunction caused by traumatic brain injury. J. Surg. Res. 184, 1006–1012. doi:10.1016/j.jss.2013.03.096
Fang, Y., and Gao, J. (2017). Cathartic effect of baishao and its effect on AQP4 and VIP in mice with constipation. Shandong J. Traditional Chin. Med. 36, 62–65. doi:10.16295/j.cnki.0257-358x.2017.01.022
Gao, M., Zhang, Z., Lai, K., Deng, Y., Zhao, C., Lu, Z., et al. (2022). Puerarin: A protective drug against ischemia-reperfusion injury. Front. Pharmacol. 13, 927611. doi:10.3389/fphar.2022.927611
Ghandadi, M., and Sahebkar, A. (2017). Curcumin: An effective inhibitor of interleukin-6. Curr. Pharm. Des. 23, 921–931. doi:10.2174/1381612822666161006151605
Guo, R., Wang, L., Zeng, X., Liu, M., Zhou, P., Lu, H., et al. (2020). Aquaporin 7 involved in GINSENOSIDE-RB1-mediated anti-obesity via peroxisome proliferator-activated receptor gamma pathway. Nutr. Metab. 17, 69. doi:10.1186/s12986-020-00490-8
Hara-Chikuma, M., Satooka, H., Watanabe, S., Honda, T., Miyachi, Y., Watanabe, T., et al. (2015). Aquaporin-3-mediated hydrogen peroxide transport is required for NF-κB signalling in keratinocytes and development of psoriasis. Nat. Commun. 6, 7454. doi:10.1038/ncomms8454
Hara-Chikuma, M., and Verkman, A. S. (2008). Prevention of skin tumorigenesis and impairment of epidermal cell proliferation by targeted aquaporin-3 gene disruption. Mol. Cell. Biol. 28, 326–332. doi:10.1128/MCB.01482-07
Hj, J., and Th, K. (2016). Molecular mechanisms regulating aquaporin-2 in kidney collecting duct. Am. J. Physiol. Ren. Physiol. 311, F1318. doi:10.1152/ajprenal.00485.2016
Hu, B., Fan, H., Bao, J., Wang, Y., Chen, B., Wang, Z., et al. (2011). Effects of Salviae miltiorrhizae Radix et Rhizoma on water metabolism and aquaporin regulation in diabetic rats with renal injury. Lishizhen Med. Materia Medica Res. 22, 356–358. doi:10.3969/j.issn.1008-0805.2011.02.041
Huang, C., Peng, W., Wei, S., Mou, M., Wei, D., Gao, Y., et al. (2020a). Effect of Pinelliae rhizoma praeparatum cum alumine polysaccharides on colonic water and liquid metabolism in asthma model rats based on “ exterior-interior relationship between lung and large intestine. Chin. Archives Traditional Chin. Med. 38, 104. doi:10.13193/j.issn.1673-7717.2020.11.027
Huang, P., Hansen, J. S., Saba, K. H., Bergman, A., Negoita, F., Gourdon, P., et al. (2022). Aquaglyceroporins and orthodox aquaporins in human adipocytes. Biochim. Biophys. Acta. Biomembr. 1864, 183795. doi:10.1016/j.bbamem.2021.183795
Huang, Y., Long, X., Tang, J., Li, X., Zhang, X., Luo, C., et al. (2020b). The attenuation of traumatic brain injury via inhibition of oxidative stress and apoptosis by tanshinone IIA. Oxid. Med. Cell. Longev. 2020, 4170156. doi:10.1155/2020/4170156
Iena, F. M., and Lebeck, J. (2018). Implications of aquaglyceroporin 7 in energy metabolism. Int. J. Mol. Sci. 19, E154. doi:10.3390/ijms19010154
Jang, S. Y., Lee, E. S., Ohn, Y.-H., and Park, T. K. (2016). Expression of aquaporin-6 in rat retinal ganglion cells. Cell. Mol. Neurobiol. 36, 965–970. doi:10.1007/s10571-015-0283-6
Jelen, S., Wacker, S., Aponte-Santamaría, C., Skott, M., Rojek, A., Johanson, U., et al. (2011). Aquaporin-9 protein is the primary route of hepatocyte glycerol uptake for glycerol gluconeogenesis in mice. J. Biol. Chem. 286, 44319–44325. doi:10.1074/jbc.M111.297002
Jiang, X., Li, H., Li, X., Chen, Y., Shi, H., Ma, L., et al. (2011). Effect of puerarin on AQP1 mRNA expression level in esophageal cancer cell line TE-1 and ECA109. J. Xinjiang Med. Univ. 34, 258–260. doi:10.3969/j.issn.1009-5551.2011.03.007
Jiang, Y. (2017). Regulatory effects and mechanism of atractylenolide I on aquaporin 3, 4 of rats with slow transit constipation. China: Qingdao University.
Jing, N., Li, X., Zhao, L., and Chen, H. (2013). The influence of picroside -II on the expression of aquaporin 4 following cerebral ischemic injury in rats. Chin. J. Clin. Neurosci. 21, 631–636.
Jung, H. J., Park, J.-Y., Jeon, H.-S., and Kwon, T.-H. (2011). Aquaporin-5: A marker protein for proliferation and migration of human breast cancer cells. PLoS One 6, e28492. doi:10.1371/journal.pone.0028492
Kang, X., Lu, X.-G., Zhan, L.-B., Liang, Z.-K., Guo, W.-X., Ma, Q., et al. (2017). Dai-Huang-Fu-Zi-Tang alleviates pulmonary and intestinal injury with severe acute pancreatitis via regulating aquaporins in rats. BMC Complement. Altern. Med. 17, 288. doi:10.1186/s12906-017-1789-x
Koike, S., Tanaka, Y., Matsuzaki, T., Morishita, Y., and Ishibashi, K. (2016). Aquaporin-11 (AQP11) expression in the mouse brain. Int. J. Mol. Sci. 17, E861. doi:10.3390/ijms17060861
Kon, R., Ikarashi, N., Nagoya, C., Takayama, T., Kusunoki, Y., Ishii, M., et al. (2014). Rheinanthrone, a metabolite of sennoside A, triggers macrophage activation to decrease aquaporin-3 expression in the colon, causing the laxative effect of rhubarb extract. J. Ethnopharmacol. 152, 190–200. doi:10.1016/j.jep.2013.12.055
Kruse, E., Uehlein, N., and Kaldenhoff, R. (2006). The aquaporins. Genome Biol. 7, 206. doi:10.1186/gb-2006-7-2-206
Kwon, T.-H., Frøkiær, J., and Nielsen, S. (2013). Regulation of aquaporin-2 in the kidney: A molecular mechanism of body-water homeostasis. Kidney Res. Clin. Pract. 32, 96–102. doi:10.1016/j.krcp.2013.07.005
Laforenza, U., Scaffino, M. F., and Gastaldi, G. (2013). Aquaporin-10 represents an alternative pathway for glycerol efflux from human adipocytes. PLoS One 8, e54474. doi:10.1371/journal.pone.0054474
Laforenza, U. (2012). Water channel proteins in the gastrointestinal tract. Mol. Asp. Med. 33, 642–650. doi:10.1016/j.mam.2012.03.001
Lan, Y.-L., Chen, J.-J., Hu, G., Xu, J., Xiao, M., and Li, S. (2017). Aquaporin 4 in astrocytes is a target for therapy in Alzheimer’s disease. Curr. Pharm. Des. 23, 4948–4957. doi:10.2174/1381612823666170714144844
Li, F., Wang, S., Wang, X., Ren, Q., Wang, W., Shang, G., et al. (2008). Novel exploration of cathartic pharmacology induced by rhubarb. China J. Chin. Materia Medica 33, 481–484. doi:10.3321/j.issn:1001-5302.2008.04.042
Li, H., Deng, C., Chen, B., Chen, R., Zhang, S., and Liang, Y. (2006). Effects of Panax Notoginseng Saponins on expression of Caspase after focal cerebral ischemia-reperfusion in rats. Chin. Pharmacol. Bull. 2006, 189–193. doi:10.3321/j.issn:1001-1978.2006.02.014
Li, H., Guo, S., Wang, T., You, P., Zhang, D., Luo, X., et al. (2022). Study on the screening and mechanism of effective components of Poriain the treatment of damp-heat in lower Jiao. Chin. J. Hosp. Pharm. 42, 519–524. doi:10.13286/j.1001-5213.2022.05.10
Li, H., Wang, T., You, P., Xia, H., Zhang, D., and Han, S. (2021a). Clearing damp and promoting diuresis effect of Poria cocos from different origins on lower energizer edema of rat with kidney-yang deficiency. Traditional Chin. Drug Res. Clin. Pharmacol. 32, 632–638. doi:10.19378/j.issn.1003-9783.2021.05.006
Li, J., Hou, Y., Liu, C., Zhang, M., Chen, J., and Chen, J. (2019a). Brief analysis of the importance of triple energizer. J. Traditional Chin. Med. 60, 1981–1984. doi:10.13288/j.11-2166/r.2019.23.001
Li, K., Cui, N., Meng, X., Ma, J., and Zhang, S. (2015). [Effect of esculentoside A on expression of AQP2 and AQP4 protein of kidney in water-loaded rats]. Zhong Yao Cai 38, 1685–1689. doi:10.13863/j.issn1001-4454.2015.08.031
Li, M., Wan, Z., Zhao, J., Chen, X., Ma, Q., and Liu, G. (2016). The influence of astragaloside Ⅳ intervention on the expression of TNF-α and AQP-4 in astrocytes after OGD/R. Chin. J. Neuroanat. 32, 732–738. doi:10.16557/j.cnki.1000-7547.2016.06.0010
Li, M., Yang, M., and Liu, Y. (2009). Protective effect of salvia miltiorrhiza and its decoction on model of lung injury in rats. J. Emerg. Traditional Chin. Med. 18, 90–92. doi:10.3969/j.issn.1004-745X.2009.01.050
Li, N., Xu, X., Yang, H., Wang, H., Ouyang, Y., Zhou, Y., et al. (2021b). Activation of Aquaporin 5 by carcinogenic Helicobacter pylori infection promotes epithelial-mesenchymal transition via the MEK/ERK pathway. Helicobacter 26, e12842. doi:10.1111/hel.12842
Li, Q., Tian, D., Cen, J., Duan, L., and Xia, W. (2021c). Novel AQP2 mutations and clinical characteristics in seven Chinese families with congenital nephrogenic diabetes insipidus. Front. Endocrinol. 12, 686818. doi:10.3389/fendo.2021.686818
Li, Y.-N., Gao, Z.-W., Li, R., Zhang, Y.-F., Zhu, Q.-S., and Huang, F. (2019b). Aquaporin 4 regulation by ginsenoside Rb1 intervenes with oxygen-glucose deprivation/reoxygenation-induced astrocyte injury. Med. Baltim. 98, e17591. doi:10.1097/MD.0000000000017591
Liao, K.-F., Chiu, T.-L., Huang, S.-Y., Hsieh, T.-F., Chang, S.-F., Ruan, J.-W., et al. (2018). Anti-cancer effects of radix angelica sinensis (danggui) and N-butylidenephthalide on gastric cancer: Implications for REDD1 activation and mTOR inhibition. Cell. Physiol. biochem. 48, 2231–2246. doi:10.1159/000492641
Lin, G. (2015). Effects of curcumin on VEGF and AQP-4 of rats with focal cerebral ischemia-reperfusion injury. Chin. J. Clin. Pharmacol. Ther. 20, 621–624.
Liu, C., Wei, P., Hu, J., Li, R., Guan, X. J., and Fu, Y. P. (2020). p33ING1b regulates acetylation of p53 in oral squamous cell carcinoma via SIR2. Cancer Cell Int. 27, 398–403. doi:10.1186/s12935-020-01489-0
Liu, J., Chen, M., Chen, X., and Chen, M. (2021). Interpretation of modern scientific connotation of “kidney governing water” from aquaporins. Shandong J. Traditional Chin. Med. 40, 884–889. doi:10.16295/j.cnki.0257-358x.2021.08.021
Liu, X., Du, A., Jiang, H., Chen, Y., Zhang, X., Yang, S., et al. (2015). Effects of Semen Cassiae on colonic myoelectrical activity and AQP3 in mice. Chin. J. Gerontology 35, 2145–2147. doi:10.3969/j.issn.1005-9202.2015.08.066
Luo, X., Xu, X., Huang, C., Wu, X., Liu, J., Lan, B., et al. (2011). Experiment study of total anthraquinone in cassiae semen on lipid peroxidation and PPAR-gamma expression in liver tissues of rats with alcoholic fatty liver. Zhongguo Zhong Yao Za Zhi 36, 1654–1659.
Lv, H., Li, Y., Xue, C., Dong, N., Bi, C., and Shan, A. (2022). Aquaporin: Targets for dietary nutrients to regulate intestinal health. J. Anim. Physiol. Anim. Nutr. 106, 167–180. doi:10.1111/jpn.13539
Lv, Q., Zhao, W., Kong, X., Zhang, Y., Wang, Y., and Wang, W. (2021). Research progress on pharmacological mechanism of diuretic effect of Astragali radix. Chin. Tradit. Pat. Med. 43, 729–732. doi:10.3969/j.issn.1001-1528.2021.03.030
Ma, J., Zhou, C., Yang, J., Ding, X., Zhu, Y., and Chen, X. (2016). Expression of AQP6 and AQP8 in epithelial ovarian tumor. J. Mol. Histol. 47, 129–134. doi:10.1007/s10735-016-9657-4
Magouliotis, D. E., Tasiopoulou, V. S., Baloyiannis, I., Mamaloudis, I., and Tzovaras, G. (2020). Transcriptomic analysis of the aquaporin gene family and associated interactors in rectal cancer. Microrna 9, 153–166. doi:10.2174/2211536608666190917153332
Mao, S., Li, C., Li, J., and Kang, B. (2010). Effects of astragalus polysaccharides on the renal injury of diabetes rats and renal expression of aquaporin-2. Chin. J. Gerontology 30, 2301–2303. doi:10.3969/j.issn.1005-9202.2010.16.025
Méndez-Giménez, L., Ezquerro, S., da Silva, I. V., Soveral, G., Frühbeck, G., and Rodríguez, A. (2018). Pancreatic aquaporin-7: A novel target for anti-diabetic drugs? Front. Chem. 6, 99. doi:10.3389/fchem.2018.00099
Meng, L., Huang, R., Zhu, C., Wei, S., Li, X., and Huang, J. (2007). The effect of PNS on expression of AQP4 and brain edema after intracerebral hemorrhage in rats. Chin. J. Geriatric Heart Brain Vessel Dis. 2007, 53–56. doi:10.3969/j.issn.1009-0126.2007.01.018
Murata, K., Mitsuoka, K., Hirai, T., Walz, T., Agre, P., Heymann, J. B., et al. (2000). Structural determinants of water permeation through aquaporin-1. Nature 407, 599–605. doi:10.1038/35036519
Nagaraju, G. P., Basha, R., Rajitha, B., Alese, O. B., Alam, A., Pattnaik, S., et al. (2016). Aquaporins: Their role in gastrointestinal malignancies. Cancer Lett. 373, 12–18. doi:10.1016/j.canlet.2016.01.003
Nagelhus, E. A., and Ottersen, O. P. (2013). Physiological roles of aquaporin-4 in brain. Physiol. Rev. 93, 1543–1562. doi:10.1152/physrev.00011.2013
Nakano, T., Nishigami, C., Irie, K., Shigemori, Y., Sano, K., Yamashita, Y., et al. (2018). Goreisan prevents brain edema after cerebral ischemic stroke by inhibiting aquaporin 4 upregulation in mice. J. Stroke Cerebrovasc. Dis. 27, 758–763. doi:10.1016/j.jstrokecerebrovasdis.2017.10.010
Nave, M., Castro, R. E., Rodrigues, C. M., Casini, A., Soveral, G., and Gaspar, M. M. (2016). Nanoformulations of a potent copper-based aquaporin inhibitor with cytotoxic effect against cancer cells. Nanomedicine (Lond) 11, 1817–1830. doi:10.2217/nnm-2016-0086
Oshio, K., Watanabe, H., Song, Y., Verkman, A. S., and Manley, G. T. (2005). Reduced cerebrospinal fluid production and intracranial pressure in mice lacking choroid plexus water channel Aquaporin-1. FASEB J. 19, 76–78. doi:10.1096/fj.04-1711fje
Ouyang, S., Chen, W., Zeng, G., and Lei, C. (2020). Aquaporin-2 expression in the kidney and urine is elevated in rats with monocrotaline-induced pulmonary heart disease. J. Int. Med. Res. 48, 300060519894448. doi:10.1177/0300060519894448
Peng, S., Liu, J., Cui, J., Zhou, D., Zhou, G., and Wang, C. (2017). Effect of emodin on AQP-4 expression in cerebral hemorrhage rats. Acta Chin. Med. Pharmacol. 45, 67–71. doi:10.3969/j.issn.1002-2392.2017.05.017
Ricanek, P., Lunde, L. K., Frye, S. A., Støen, M., Nygård, S., Morth, J. P., et al. (2015). Reduced expression of aquaporins in human intestinal mucosa in early stage inflammatory bowel disease. Clin. Exp. Gastroenterol. 8, 49–67. doi:10.2147/CEG.S70119
Shiozaki, A., Marunaka, Y., and Otsuji, E. (2021). Roles of ion and water channels in the cell death and survival of upper gastrointestinal tract cancers. Front. Cell Dev. Biol. 9, 616933. doi:10.3389/fcell.2021.616933
State Pharmacopoeia Commission (2020). Pharmacopoeia of the people’s Republic of China. Part 1. Beijing: China Medical Science Press.
Sun, R.-L., Tang, D.-C., and Gu, J.-F. (2021a). [Study on intervention effect of Astragali Radix-Curcumae Rhizoma on growth and metastasis of colon cancer in orthotopic transplantation mice model of colon cancer]. Zhongguo Zhong Yao Za Zhi 46, 2267–2275. doi:10.19540/j.cnki.cjcmm.20201207.402
Sun, Y., Wang, J., Hou, Z., Wu, T., Wang, W., and Fei, Q. (2021b). Effects of effective fractions of maidong (ophiopogon Aeruginosa)on expressions of aquaporins 1 and aquaporins 5 in lung tissue of mice infected with Mycoplasma. Chin. J. Traditional Med. Sci. Technol. 28, 893–896.
Tanaka, Y., Watari, M., Saito, T., Morishita, Y., and Ishibashi, K. (2016). Enhanced autophagy in polycystic kidneys of AQP11 null mice. Int. J. Mol. Sci. 17, E1993. doi:10.3390/ijms17121993
Tang, Z., Yang, G., Liao, Z., Chen, F., Chen, S., Wang, W., et al. (2022). Tanshinone IIA reduces AQP4 expression and astrocyte swelling after OGD/R by inhibiting the HMGB1/RAGE/NF-κB/IL-6 pro-inflammatory axis. Sci. Rep. 12, 14110. doi:10.1038/s41598-022-17491-7
Tao, Y., Dong, X., Xu, Y., Jin, C., and Tu, P. (2015). Preventive effect of phenylethanoid glycosides from Cistanche tubulosa on rats with high altitude cerebral edema. Mod. Chin. Med. 17, 302–306. doi:10.13313/j.issn.1673-4890.2015.4.002
Tao, Y., Li, C., Wang, Y., Sun, L., Yan, T., and Wu, S. (2014). Effects of Ophiopogon japonicus on expression of NOD mice submandibular gland AQP5 and VIPmRNA. Lishizhen Med. Materia Medica Res. 25, 2072–2074. doi:10.3969/j.issn.1008-0805.2014.09.009
Tricarico, P. M., Mentino, D., De Marco, A., Del Vecchio, C., Garra, S., Cazzato, G., et al. (2022). Aquaporins are one of the critical factors in the disruption of the skin barrier in inflammatory skin diseases. Int. J. Mol. Sci. 23, 4020. doi:10.3390/ijms23074020
Trillo-Contreras, J. L., Toledo-Aral, J. J., Echevarría, M., and Villadiego, J. (2019). AQP1 and AQP4 contribution to cerebrospinal fluid homeostasis. Cells 8, E197. doi:10.3390/cells8020197
Tu, X., Yang, W., Shi, S., Chen, C., Chen, J., and Wang, C. (2013). Inhibitory effect of baicalin on the expression of TNF-α and AQP-4 and the brain damage following cerebral ischemia in rats. Chin. Pharmacol. Bull. 29, 1222–1225. doi:10.3969/j.issn.1001-1978.2013.09.010
Verkman, A. S., Anderson, M. O., and Papadopoulos, M. C. (2014). Aquaporins: Important but elusive drug targets. Nat. Rev. Drug Discov. 13, 259–277. doi:10.1038/nrd4226
Verkman, A. S., Matthay, M. A., and Song, Y. (2000). Aquaporin water channels and lung physiology. Am. J. Physiol. Lung Cell. Mol. Physiol. 278, L867–L879. doi:10.1152/ajplung.2000.278.5.L867
Verkman, A. S., Smith, A. J., Phuan, P.-W., Tradtrantip, L., and Anderson, M. O. (2017). The aquaporin-4 water channel as a potential drug target in neurological disorders. Expert Opin. Ther. Targets 21, 1161–1170. doi:10.1080/14728222.2017.1398236
Vukićević, T., Schulz, M., Faust, D., and Klussmann, E. (2016). The trafficking of the water channel aquaporin-2 in renal principal cells-a potential target for pharmacological intervention in cardiovascular diseases. Front. Pharmacol. 7, 23. doi:10.3389/fphar.2016.00023
Wang, C., Yan, M., Jiang, H., Wang, Q., He, S., Chen, J., et al. (2018a). Mechanism of aquaporin 4 (AQP 4) up-regulation in rat cerebral edema under hypobaric hypoxia and the preventative effect of puerarin. Life Sci. 193, 270–281. doi:10.1016/j.lfs.2017.10.021
Wang, L., Tang, H., Wang, C., Hu, Y., Wang, S., and Shen, L. (2019). Aquaporin 4 deficiency alleviates experimental colitis in mice. FASEB J. 33, 8935–8944. doi:10.1096/fj.201802769RR
Wang, P., Zhao, S., Wang, Q., and Kuang, H. (2015). Relationship between efficacy exertion of diuretic traditional Chinese medicines and aquaporin. Zhongguo Zhong Yao Za Zhi 40, 2272–2277. doi:10.4268/cjcmm20151202
Wang, R., Li, Q., Niu, L., Yang, B., Liu, G., and Zuo, H. (2019). Fracture toughening of peritubular microstructure in biological porous dentine. J. Mech. Behav. Biomed. Mat. 16, 194–203. doi:10.1016/j.jmbbm.2019.02.004
Wang, W.-R., Liu, H.-Y., Ren, Z.-X., Chen, L., Liu, S., Shi, L.-C., et al. (2020a). Effect of bushen qingre yuyin decoction on salivary secretion, spleen index, submandibular gland index, submandibular gland histomorphology, and aqp5 expression in the nonobese diabetic mouse model. World J. Tradit. Chin. Med. 6, 508–514. doi:10.4103/wjtcm.wjtcm_48_20
Wang, Y.-Z., Zhang, X.-T., Li, S.-J., Zhang, Y., Li, F.-Y., Zhang, C.-N., et al. (2018c). Expression of AQP2, AQP4 and AQP 8 in mouse intestine induced by unprocessed and processed Euphorbia lathyris. Pak. J. Pharm. Sci. 31, 1229–1235.
Wang, Y., Biao, Y., Chu, X., Zhang, N., Zhang, M., Wang, Q., et al. (2020b). Effect of Plantaginis Semen on inflammatory cytokines and mRNA and protein expressions of AQP4 in colon tissue of diarrhea rats. Chin. J. Exp. Traditional Med. Formulae 26, 52–58. doi:10.16370/j.cnki.13-1214/r.2020.02.002
Wang, Y., Biao, Y., Zhang, N., Han, X., Hu, W., Zhang, Y., et al. (2020c). Effect of cheqianzi on AQP1 expression in small intestinal mucosa of diarrhea rats college of pharmacy. J. Hebei Traditional Chin. Med. Pharmacol. 35, 5–8. doi:10.16370/j.cnki.13-1214/r.2020.02.002
Wang, Y., Ji, X., Han, X., and Wu, J. (2018b). Effect of different components of coix seed polysaccharides on the VIPR1 and AQP3 expression in the colon of rats with TCM syndrome of dampness stagnancy due to spleen deficiency. Pharmacol. Clin. Chin. Materia Medica 34, 43–47. doi:10.13412/j.cnki.zyyl.2018.02.012
Wang, Z., Cheng, Y., Su, W., Zhang, H., Li, C., Routledge, M. N., et al. (2021). Organ specific differences in alteration of aquaporin expression in rats treated with sennoside A, Senna anthraquinones and rhubarb anthraquinones. Int. J. Mol. Sci. 22, 8026. doi:10.3390/ijms22158026
Wang, Z., Liu, X., Tuo, H., Ren, Y., Li, R., and Li, H. (2016). Effects of Angelica sinensis radix on expression of aquaporin-1 in lung tissue of asthmatic mice with Yin deficiency. Pharmacol. Clin. Chin. Materia Medica 32, 95–98. doi:10.13412/j.cnki.zyyl.2016.01.027
Wei, W.-L., Zeng, R., Gu, C.-M., Qu, Y., and Huang, L.-F. (2016). Angelica sinensis in China-A review of botanical profile, ethnopharmacology, phytochemistry and chemical analysis. J. Ethnopharmacol. 190, 116–141. doi:10.1016/j.jep.2016.05.023
Wittekindt, O. H., and Dietl, P. (2019). Aquaporins in the lung. Pflugers Arch. 471, 519–532. doi:10.1007/s00424-018-2232-y
Wu, D. Q., Yang, Z. F., Wang, K. J., Feng, X. Y., Lv, Z. J., Li, Y., et al. (2018). AQP8 inhibits colorectal cancer growth and metastasis by down-regulating PI3K/AKT signaling and PCDH7 expression. Am. J. Cancer Res. 8, 266–279.
Wu, N., Zhang, X. L., Hou, Y., Lin, L. X., and Zhang, X. B. (2019). Effect of methyl eugenol on nasal mucosal aquaporin 5 in rats with allergic rhinitis. Beijing Da Xue Xue Bao Yi Xue Ban. 51, 1036–1041. doi:10.19723/j.issn.1671-167X.2019.06.010
Wu, T., Li, K., Lyu, Y., Yi, D., Zhao, D., Wang, L., et al. (2020). Trilactic glyceride regulates lipid metabolism and improves gut function in piglets. Front. Biosci. 25, 1324–1336. doi:10.2741/4858
Wu, X., Chen, Z., and Zhang, G. (2010). Effects of aqueous extract of rhizoma alismastis on diuretic activity and kidney medulla AQP2 expression in normal rats. J. Clin. Med. Pract. 14, 5–7+10. doi:10.3969/j.issn.1672-2353.2010.21.002
Xia, J., Yang, Y., Zhang, S., and Wan, Y. (2022). Comparative study on the regulation of the volatile oil of Rhizoma Atractylodes Macrocephalae on on AQP3 and AQP4 in rats with chronic Atrophic gastritis before and after compatibility with Ginseng Radix et Rhizoma. Nat. Prod. Res. Dev. 34, 33–41. doi:10.16333/j.1001-6880.2022.1.005
Xie, J., Luo, S., Mi, H., Du, Y., Bao, G., Zhou, J., et al. (2019a). Intake consumption of ginsenoside Rg3, profiling of selected cytokines, and development of rectal polyps. Cancer Manag. Res. 11, 4059–4064. doi:10.2147/CMAR.S197097
Xie, J., Tan, Y., Luo, L., Xu, S., Bao, G., and Li, C. (2019b). Effects of ginsenoside Rg3 on the expression of aquaporin 3 and aquaporin 4 in rat rectal polyps and its mechanism. J. Chin. Med. Mater. 42, 1164–1168. doi:10.13863/j.issn1001-4454.2019.05.042
Xie, Y., Zhan, X., Tu, J., Xu, K., Sun, X., Liu, C., et al. (2021). Atractylodes oil alleviates diarrhea-predominant irritable bowel syndrome by regulating intestinal inflammation and intestinal barrier via SCF/c-kit and MLCK/MLC2 pathways. J. Ethnopharmacol. 272, 113925. doi:10.1016/j.jep.2021.113925
Xin, L., Jiang, X., Ye, X., Wu, R., Xu, K., Ma, J., et al. (2015). AQP5 gene silencing inhibits proliferation and migration of ectopic endometrial glandular epithelial cells in endometriosis. J. Zhejiang Univ. Sci. 44, 285–292. doi:10.3785/j.issn.1008-9292.2015.05.08
Xuan, Y., Bian, Q., Li, Y., and Li, S. (2021). Experimental research progress of traditional Chinese medicine interventing aquaporin in the treatment of pulmonary inflammatory disease. Pharmacol. Clin. Chin. Materia Medica 37, 221–227. doi:10.13412/j.cnki.zyyl.20210120.012
Yan, S., Zeng, J., Bi, Y., Wei, J., Wang, X., Luo, G., et al. (2014). Effects of Plantago asiatica L.seeds on diuretic activity, aquaporins and ion channels in normal rats. Chin. J. Hosp. Pharm. 34, 968–971. doi:10.13286/j.cnki.chinhosppharmacyj.2014.12.03
Yang, B., van Hoek, A. N., and Verkman, A. S. (1997). Very high single channel water permeability of aquaporin-4 in baculovirus-infected insect cells and liposomes reconstituted with purified aquaporin-4. Biochemistry 36, 7625–7632. doi:10.1021/bi970231r
Yang, X., Li, J., XuanYuan, Q., and Wei, M. (2002). Proven remedy. Chin. J. Integr. Traditional West. Med. Dig. 8, 151–153. doi:10.1007/bf02934447
Yde, J., Keely, S. J., and Moeller, H. B. (2021). Expression, regulation and function of Aquaporin-3 in colonic epithelial cells. Biochim. Biophys. Acta. Biomembr. 1863, 183619. doi:10.1016/j.bbamem.2021.183619
Ying, Y., Liu, H., Gong, B. F., Wang, Y., Wei, H., Lin, D., et al. (2020). Efficacy of arsenic trioxide combined with ATRA and chemotherapy for relapsed acute promyelocytic leukemia patients. Zhongguo Shi Yan Xue Ye Xue Za Zhi 52, 1–6. doi:10.19746/j.cnki.issn.1009-2137.2020.01.001
Yu, L., Hao, H., Ren, S., Liu, Z., Huang, J., Li, N., et al. (2022). Clinical study on semen cassiae torae and buckwheat tea in the treatment of functional constipation. Guangming J. Chin. Med. 37, 3321–3325. doi:10.3969/j.issn.1003-8914.2022.18.019
Zhang, B., Liu, K., Yang, H., Jin, Z., Ding, Q., and Zhao, L. (2022). Gut microbiota: The potential key target of TCM’s therapeutic effect of treating different diseases using the same method-UC and T2DM as examples. Front. Cell. Infect. Microbiol. 12, 855075. doi:10.3389/fcimb.2022.855075
Zhang, D., Yang, L., Su, W., Zhao, Y., Ma, X., Zhou, H., et al. (2017a). Aquaporin-4 is downregulated in the basolateral membrane of ileum epithelial cells during enterotoxigenic Escherichia coli-induced diarrhea in mice. Front. Microbiol. 8, 2655. doi:10.3389/fmicb.2017.02655
Zhang, G., Zeng, X., Li, C., Li, J., Huang, Y., Han, L., et al. (2011). Inhibition of urinary bladder carcinogenesis by aqueous extract of sclerotia of Polyporus umbellatus fries and polyporus polysaccharide. Am. J. Chin. Med. 39, 135–144. doi:10.1142/S0192415X11008701
Zhang, N., Liu, S., Yu, D., Wang, N., Li, Z., and Yu, H. (2017b). Exploration on the relationship between aquaporin and water-liquid metabolism in traditional Chinese Medicine. Lishizhen Med. Materia Medica Res. 28, 1170–1172. doi:10.3969/j.issn.1008-0805.2017.05.055
Zhang, X. (2012). Study of the effect on gastrointestinal form and water metabolism in animal model of spleen deficiency diarrhea by using Bai Zhu Fu Ling and Fu Ling Bai Zhu Tang. China: Chengdu University of TCM.
Zhang, X., Wu, F., Lin, R., Zhang, X., Liu, N., Li, J., et al. (2012). Changes of lung, spleen and kidney aquaporin-1 in rats with Kidney Yang Deficiency:the “water metabolism theory” in traditional Chinese medicine. J. South. Med. Univ. 32, 1507–1510. doi:10.3969/j.issn.1673-4254.2012.10.028
Zhang, X., and Zhang, G. (2013). The regulation of sclerotia of P.umbellate aqueous extract with bladder AQP1, AQP3 in urinary bladder cancer. J. Yunnan Univ. Traditional Chin. Med. 36, 1–3. doi:10.3969/j.issn.1000-2723.2013.03.001
Zhao, H., Chen, G., Wang, G., Sun, D., Zhang, Y., and Zhao, X. (2009). Effects of panaxadiols on the expression of aquaporin in severe pancreatitis with acute lung injury. Chin. J. Gerontology 29, 561–562. doi:10.3969/j.issn.1005-9202.2009.05.020
Zhao, J. (2009). The influence of Astragaloside on aquaporin I and V in rats with part I acute lung injury [D]. ZunYi Med. Coll. doi:10.7666/d.y1581810
Zheng, D., Peng, M., Zhu, X., and Wang, J. (2019). Effects of Qutanhuoxue decoction on AQP7 and AQP9 expression in nonalcoholic fatty liver model rats. Evid. Based. Complement. Altern. Med. 2019, 5709626. doi:10.1155/2019/5709626
Zheng, X., Song, W., Zhang, T., Cao, H., and Liu, J. (2022). Baicalin treats cerebral ischemia reperfusion-induced brain edema in rats by inhibiting TRPV4 and AQP4 of astrocytes. China J. Chin. Materia Medica 47, 1031–1038. doi:10.19540/j.cnki.cjcmm.20211116.701
Zhou, J., Deng, Q., and Liang, J. (2015). Effect on extracts of pinellia (EP) on expression of aquaporin 5 in mice. J. Changchun Univ. Chin. Med. 31, 229–231. doi:10.13463/j.cnki.cczyy.2015.02.004
Zhu, C., Chen, Z., and Jiang, Z. (2016). Expression, distribution and role of aquaporin water channels in human and animal stomach and intestines. Int. J. Mol. Sci. 17, E1399. doi:10.3390/ijms17091399
Zhu, S., Duan, H., Liu, Y., Li, G., Liu, Y., Huang, M., et al. (2017a). [Neuroprotective effects and mechanism of saikosaponin A on acute spinal cord injury in rats]. Zhongguo Xiu Fu Chong Jian Wai Ke Za Zhi 31, 825–829. doi:10.7507/1002-1892.201702106
Zhu, S., Ran, J., Yang, B., and Mei, Z. (2017b). Aquaporins in digestive system. Adv. Exp. Med. Biol. 969, 123–130. doi:10.1007/978-94-024-1057-0_8
Keywords: aquaporin, gastrointestinal diseases, traditional Chinese medicine, water metabolism, triple energizer
Citation: Huang Y, Yan S, Su Z, Xia L, Xie J, Zhang F, Du Z, Hou X, Deng J and Hao E (2022) Aquaporins: A new target for traditional Chinese medicine in the treatment of digestive system diseases. Front. Pharmacol. 13:1069310. doi: 10.3389/fphar.2022.1069310
Received: 13 October 2022; Accepted: 21 November 2022;
Published: 01 December 2022.
Edited by:
Yulin Dai, Changchun University of Chinese Medicine, ChinaReviewed by:
Maria Pilar Lostao, University of Navarra, SpainCopyright © 2022 Huang, Yan, Su, Xia, Xie, Zhang, Du, Hou, Deng and Hao. This is an open-access article distributed under the terms of the Creative Commons Attribution License (CC BY). The use, distribution or reproduction in other forums is permitted, provided the original author(s) and the copyright owner(s) are credited and that the original publication in this journal is cited, in accordance with accepted academic practice. No use, distribution or reproduction is permitted which does not comply with these terms.
*Correspondence: Erwei Hao, aGFvZXdAZ3h0Y211LmVkdS5jbg==
Disclaimer: All claims expressed in this article are solely those of the authors and do not necessarily represent those of their affiliated organizations, or those of the publisher, the editors and the reviewers. Any product that may be evaluated in this article or claim that may be made by its manufacturer is not guaranteed or endorsed by the publisher.
Research integrity at Frontiers
Learn more about the work of our research integrity team to safeguard the quality of each article we publish.