- Neurobiology Research Laboratory, Department of Healthcare Biotechnology, Atta Ur Rahman School of Applied Biosciences (ASAB), National University of Sciences and Technology (NUST), Islamabad, Pakistan
Alzheimer’s disease (AD) is a multifaceted neurodegenerative disorder characterized by substantial neuronal damage which manifests in the form of deficits in memory and cognition. In spite of the debilitating nature of Alzheimer’s disease (AD), a dearth of treatment strategies calls for the need to develop therapeutic agents that stimulate neurogenesis and alleviate the associated cognitive deficits. The present study investigates the therapeutic potential of two major phytochemicals, rosmarinic acid (RA) and ursolic acid (UA) in an amyloid beta1–42 (Aβ1–42)-induced model of AD. UA, a natural pentacyclic triterpenoid and RA, a phenolic ester are major bioactive constituents of Rosmarinus officinalis, which is a medicinal herb belonging to family Lamiaceae and exhibiting significant biological properties including neuroprotection. Donepezil, a second generation cholinesterase inhibitor approved for the treatment of mild, moderate and severe Alzheimer’s disease (AD) is used as control. Out of eight groups of male BALB/c mice, stereotaxic surgery was performed on four groups (n = 6 each) to introduce Aβ1–42 in the hippocampus followed by treatment with vehicle (phosphate-buffered saline (PBS)), donepezil, UA or RA. The other four groups were given vehicle, donepezil, UA and RA only. Behavior analysis for social interaction was performed which constitutes the social affiliation and the social novelty preference test. Presence of Aβ plaques and expression of neurogenesis markers i.e., doublecortin (DCX) and Ki-67 were also assessed. Results revealed the neuroprotective effect of UA and RA observed through substantial reduction in Aβ plaques as compared to the Aβ1-42- and donepezil-treated groups. The neuronal density was also restored as evident via DCX and Ki-67 immunoreactivity in Aβ1–42 + RA and Aβ1–42+UA-treated groups in comparison to Aβ1–42-treated and Aβ1–42+donepezil-treated groups. The social affiliation was reestablished in the Aβ1–42 administered groups treated with UA and RA. Molecular docking studies further validated the comparable binding of UA and RA with Ki-67 and DCX to that of donepezil. Our findings suggest that UA and RA are potential neuroprotective compounds that reverses the histological hallmarks of AD and ameliorate impaired social memory and hippocampal neurogenesis.
Introduction
Alzheimer’s disease (AD) is the most common form of dementia accounting for more than 80% of the cases diagnosed. With a prevalence that continues to grow as the world population ages, it has emerged as a leading health problem. The debilitating disorder affects nearly 50 million people worldwide (Crous-Bou et al., 2017). It is characterized by the formation of neurofibrillary tangles (NFTs) of hyperphosphorylated tau protein and amyloid beta (Aβ) plaques which manifests as deficits in cognition and memory (Lopez et al., 2019). Aβ is a major contributing factor in neurotoxicity and neural function and the deposition of amyloid plaques in the hippocampus, cerebral cortex and amygdala can lead to stimulation of astrocytes and microglia, axonal and dendritic damage and synaptic loss which manifest as cognitive impairments (Armstrong, 2009; Chen et al., 2017). The plaque formation constitutes the primary pathological process associated with AD while NFT formation and the subsequent neurodegeneration are downstream processes (Lane et al., 2018).
Adult hippocampal neurogenesis, is a unique phenomenon hosted by the hippocampus, which confers significant levels of plasticity to the hippocampal circuitry improving pattern separation and spatial memory (Anacker et al., 2018). AD leads to a sharp decline in adult hippocampal neurogenesis in comparison to neurologically healthy subjects (Moreno-Jiménez et al., 2019). Impaired neurogenesis is thereby considered as a relevant mechanism that leads to cognitive deficit associated with AD.
Among the various markers of neurogenesis, Doublecortin (DCX) is a brain-specific protein associated with the microtubules which regulates neuronal migration through the polymerization and stabilization of microtubules in migrating neuroblasts (Sadeghi et al., 2018). DCX is vital for the proper initiation and maintenance of differentiation as well as migration during neurogenesis. Neural cells with reduced expression of DCX exhibit impaired migration, differentiation and neurite formation (Shahsavani et al., 2018). Ki-67 another widely acclaimed marker of cell proliferation and neurogenesis is expressed in dividing cells during mitosis except the G0 phase (Sun and Kaufman, 2018).
Currently, only two classes of drugs have been approved for the treatment of AD. Cholinesterase enzyme inhibitors and N-methyl d-aspartate (NMDA) antagonists function mainly by treating the symptoms of AD and do not possess preventive or curative effects (Breijyeh and Karaman, 2020). Although a huge amount of research on AD has been directed towards the development of disease-modifying therapy in the last decade, however there is still a dearth of therapeutic agents which will alter the course of disease rather than providing symptomatic treatment alone. Lack of disease modifying drugs even after decades of studies indicates the challenges associated with the development of therapeutic agents with curative potential against AD (Salomone et al., 2012).
In the recent times, natural compounds have garnered significant interest due to their pharmacologically significant activities. These natural products, including herbs and spices, possess various phytochemicals which serve as potential sources of natural antioxidants and neuroprotectants and are devoid of the potentially life-threatening side effects characteristic of the existing approved drugs (Twilley et al., 2020; Karthika et al., 2022). Rosmarinic acid (RA), a phenolic ester is abundantly present in the herbs belonging to the family Labiatae and exhibits antioxidant, antimutagenic, antiapoptotic and several other pharmacological activities (Amoah et al., 2016). It also plays a beneficial role against AD through the suppression of Aβ aggregation (Hase et al., 2019). Ursolic acid (UA), a natural pentacyclic triterpenoid also exerts health benefits against inflammation, oxidative stress and fibrosis (Wang X. T. et al., 2018; Xu et al., 2018). Also, RA and UA substantially improve the deficits in cognition as well synaptic dysregulation and the associated neurodegeneration in AD model of Aβ1–42 -induced neurotoxicity suggesting their therapeutic significance against AD (Mirza et al., 2021). Furthermore, an in silico study also presents the therapeutic potential of these compounds based on drug-likeness, pharmacokinetic properties and binding affinity with AD-associated proteins (Mirza et al., 2022).
In the current investigation we evaluated the neuroprotective effects of RA and UA against impaired neurogenesis and social memory deficits produced by Aβ1–42. The effects were assessed in comparison to donepezil, commonly prescribed for AD (Eskandary et al., 2019). It is routinely used as a standard drug in studies investigating the therapeutic potential of different agents against AD (Adlimoghaddam et al., 2018). Moreover computer-aided molecular docking assessment also elucidated the interaction of RA and UA with the markers of neurogenesis. The data obtained for the current study may provide further insights into molecular mechanisms and clinical intervention options for AD and its associated consequences.
Materials and methods
Experimental animals
Male BALB/c mice were maintained in the animal facility of Atta-ur-Rahman School of Applied Biosciences (ASAB) National University of Sciences and Technology (NUST). Mice were raised under 12 h natural light-dark cycles and had free access to food and water ad libitum. All animal experiments were conducted in conformity with the standards of the Institute of Laboratory Animal Research, Division on Earth and Life Sciences, National Institutes of Health, United States. The internal review board of ASAB, NUST approved the study design (IRB # 64).
Animal model induction
BALB/c mice (male, age 10–12 weeks; 35–45 g) were used for experimentation. A previously described method was used to establish the animal model (Mirza et al., 2021). The mice were administered 50 mg/ml ketamine (80 µL) and 5 mg/ml Diazepam (60 µL) per 40 g of animal weight prior to microinjection of Aβ1–42 (ab120301, Abcam, Cambridge, MA, United States; dilution 1 µg per µL) into the hippocampus (CA1 region) from bregma: anteroposterior, 2.3 mm; mediolateral, 1.8 mm; dorsoventral, 2.0 mm, using a stereotaxic apparatus using a Hamilton syringe. Similarly, the control mice were administered phosphate-buffered saline (PBS). The mice were kept in individual cages until further use.
Animal grouping
The male BALB/c mice were segregated into eight groups (n = 6 each). The groups 1–4 were pre-treated with Aβ1–42. The groups 2, 3 & 4 received donepezil (15 mg/kg) (Ahmed et al., 2017), RA (16 mg/kg; ab141450, Abcam, United Kingdom) (Farr et al., 2016), or UA (40 mg/kg; ab141113, Abcam, United Kingdom) (Liang et al., 2016), respectively for 15 days post Aβ1–42 administration. Group 5 received vehicle (PBS) while groups 6, 7, 8 received the similar dose of donepezil, RA and UA, respectively for similar duration. All the groups were orally administered with normal feed and water. The purity of UA and RA was >99% and >95% respectively while donepezil was taken as a positive control. After the end of treatment period the mice were subjected to behavioral tests and subsequently brain tissue harvesting.
Social interaction behavior
The procedure was performed as reported previously (Rizwan et al., 2016). The apparatus comprised of a glass box having dimensions 40 × 40 × 40 cm with two similar cages placed inside the box. The mice placed in the cages were characterized as mouse A and B while the treated mice were referred to as test subject. Mice A and B were never encountered before with the test subject and were of the same background in terms of age, weight and gender.
Session I: Social affiliation test
After habituating the test subjects (5 min) inside the box, they were introduced back into the box with a cage having mouse A on one side and an empty cage on the other side. The test subject was then left in the box for 10 min and allowed to move in both the cages. The discrimination index (DI) or the interaction time or with the empty cage and mouse A was evaluated and the difference between the time spent interacting with the mouse A or empty cage and the total time was scored.
Session II: Social novelty preference test
The mouse B was released in the empty cage while the mouse A remained unaltered. The test subject was again allowed an exploration time of 10 min. DI or interaction time was measured as the difference between the time spent interacting with mouse A or mouse B and the total time spent with mouse B.
Histology and immunohistochemistry
Histology was performed according to the protocol used by Amber et al., 2020. The tissue was dewaxed and rehydrated in ethanol and double distilled water (ddH2O). Congo red stain (1%) was applied to the tissue for 25 min after which it was thoroughly rinsed with ddH2O, followed by counter Haematoxylin and eosin staining. The sections were rinsed again with ddH2O, dried and incubated for 20–25 min before clearing in xylene solution.
Immunostaining was performed in accordance with the protocol previously described by Mirza et al., 2021. Hippocampal tissue sections (5 μm) were deparaffinized after antigen retrieval. H2O2 (1%) was applied to quench the peroxidase activity and blocked with BSA (5%) in PBS for 1 h. After an overnight incubation with primary antibodies at 4°C the sections were incubated for 1 h with a secondary antibody followed by washing thrice with TBST and visualised with 0.025% 3, 3′ diaminobenzidine (DAB Kit, ab50185, Abcam, MA, United States). Ki-67 (Leica Biosystems, PA0230) and DCX (Abcam, ab207175) diluted in the block solution 1:200 and 1:100 respectively, were used as primary antibodies while anti-rabbit IgG-HRP conjugated (ab97051, Abcam, MA, United States) diluted in block solution 1:100 was used as secondary antibody. The images were visualized using B-150, OPTIKA microscope (Italy) at 4× and 10× resolution. The images were captured using Optika Vision Lite 2.1 image analysis software. Quantitative analysis was performed for cell count in an area of 10,000 μm2 from three randomly selected areas and the average values were calculated and plotted.
Molecular docking
PatchDock server was used for docking using cluster RMSD at default value of 4.0 to identify the interaction among RA and UA with target proteins DCX and Ki-67. The interaction was compared with donepezil. Patchdock algorithm produces potential complexes based on the criteria of shape complementarity (Schneidman-Duhovny et al., 2005). The 3D structures of the target proteins Ki-67 and DCX were acquired from RCSB Protein data bank (PDB) (https://www.rcsb.org/). The PDB IDs of Ki-67 and DCX were 5J28 and 2BQQ, respectively. The 3D structures of RA, UA and donepezil were taken from PubChem database (https://pubchem.ncbi.nlm.nih.gov/). The automated docking models generated were further assessed using FireDock (Mashiach et al., 2008) and visualized through BIOVIA Discovery Studio (Systèmes, 2016).
Statistical analysis
Data are presented as mean ± SEM and statistical significance was set at 95% confidence level and a p-value <.05. Behavioural and biochemical data was analysed by one-way analysis of variance (ANOVA) with Bonferroni’s multiple comparisons as post hoc test using GraphPad Prism 5.0.
Results
Rosmarinic and ursolic acid improve social affiliation and social novelty preference in Aβ1–42 treated mice
To evaluate the effects of RA and on sociability in mice, social interaction test was performed. Aβ1–42 treated group (2.798 ± 0.7145; p < 0.0001) exhibited a significant reduction in interaction with mouse A, a conspecific placed in one of the cages, in comparison to the control (22.66 ± 1.726; p < 0.0001) and other experimental groups exhibiting a significant decrease in social affiliation. The Aβ1–42 + RA (20.25 ± 3.779; p < 0.001) and Aβ1–42+ UA-treated groups (23.74 ± 3.434; p = 0.0003) performed significantly better than the diseased group and comparable to the Aβ1–42+donepezil-treated group (22.91 ± 5.629; p = 0.0051). Aβ1–42+ UA-treated group exhibited improved social affiliation in comparison to all of the other groups (Figure 1).
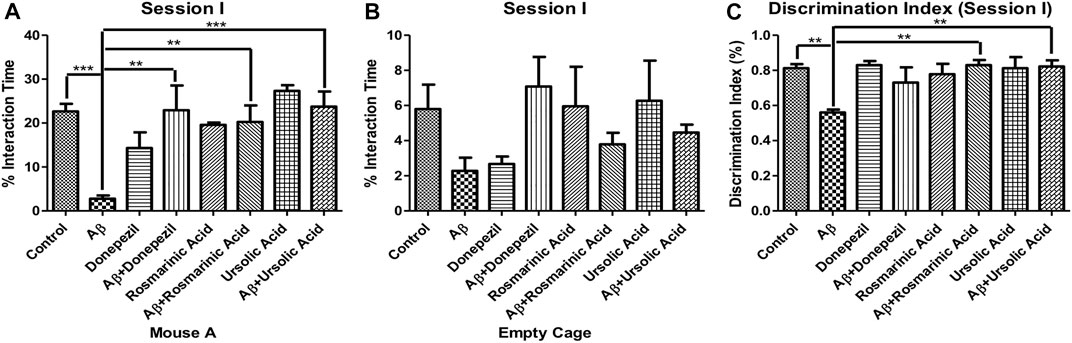
FIGURE 1. Effect of RA and UA on Social Affiliation behavior. (A) Interaction time with the Mouse A. (B) Interaction time with the empty cage. (C) Discrimination index during session (I). Significance was analyzed by one-way ANOVA followed by Bonferroni comparison test (mean ± SEM) using Graphpad Prism. *p < 0.05, **p < 0.01, ***p < 0.001.
Interactions with the empty cage also show least amount of interaction by the Aβ1–42 -treated group. The decrease social affiliation pattern observed during session I for Aβ1–42 -treated group is inferred from the same length of time spent with the mouse A (2.798 ± 0.7145; p < 0.0001) as well as empty cage (2.276 ± 0.7505; p = .0664). Conversely, the control and experimental groups interacted more with the mouse A in comparison to the empty cage (Figure 1).
The Aβ1–42-treated group (0.5600 ± 0.01703; p < 0.0001) exhibited significantly low DI in comparison to the control (0.8117 ± 0.02400; p < 0.0001) and the treated groups. A significant improvement in DI was observed in Aβ1–42 + RA (0.8300 ± 0.02887; p < 0.0001) and Aβ1–42+ UA-treated (0.8220 ± 0.03541; p = .0002) groups depicting restoration of social affiliation (Figure 1).
In the session II, the mice were assessed for novelty preference and social memory. The mice treated with Aβ1–42 showed diminished levels (6.572 ± 2.116; p = .0144) of interaction with an unfamiliar mouse B in comparison to the control group (21.38 ± 4.261). The treated groups did not show preference with any mouse cage.
The DI for session II revealed a significantly low value for the Aβ1–42 -treated group (0.3820 ± 0.02107; p < 0.0001) relative to the control (0.7880 ± 0.05054; p < 0.0001) and the other experimental groups, thus indicating alterations in social memory. A significant improvement in DI was evident in Aβ1–42 + RA (0.7300 ± 0.06258 p = .0007) and Aβ1–42+ UA-treated (0.6867 ± 0.03783 p < 0.0001) groups in comparison to the control. The DI in RA- and UA-treated groups was comparable to that of the standard drug donepezil (0.6850 ± 0.01893; p < 0.0001) demonstrating their protective effects against social memory deficit in AD (Figure 2).
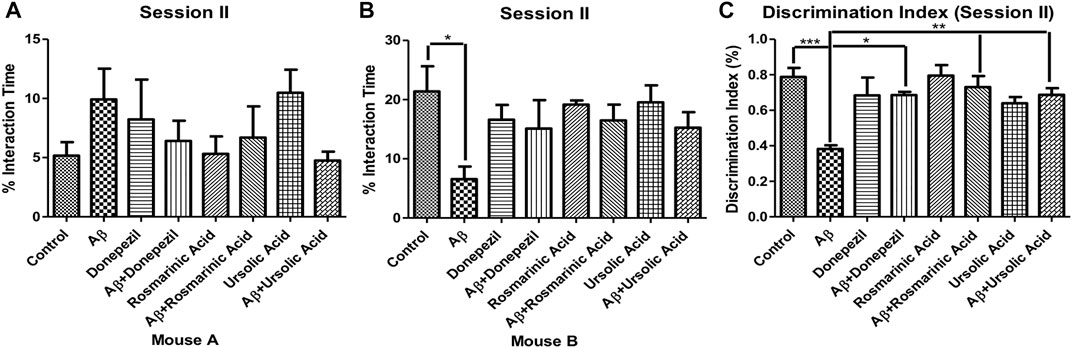
FIGURE 2. Effect of RA and UA on social novelty preference behavior. (A) Interaction time with the Mouse A. (B) Interaction time with the Mouse B. (C) Discrimination index during session II. Significance was analyzed by one-way ANOVA followed by Bonferroni comparison test (mean ± SEM) using Graphpad Prism. *p < 0.05, **p < 0.01, ***p < 0.001.
Improvement in neurogenesis by RA and UA
A considerable reduction in neuronal proliferation was significantly apparent in the group treated with Aβ1–42 relative to the control mice. An improvement in the density of Ki-67-positive neurons was observed with treatment with RA (22.87 ± 0.4667; p= .0111) and UA (25.33 ± 0.8819; p = 0.0027) post Aβ1–42 administration as compared to the mice treated Aβ1–42 only (19.50 ± 0.6455). It was found that RA and UA have greater improvement activity than donepezil (21.30 ± 1.825; p = 0.34), evident through the restoration of the Aβ1–42 deteriorated neurons. No obvious neuronal loss was encountered by the control mice (27.65 ± 1.525), donepezil, RA and UA in Aβ1–42 untreated groups (Figure 3).
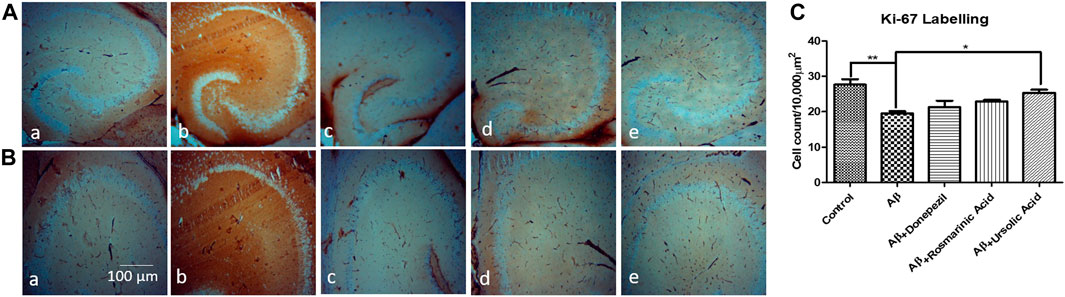
FIGURE 3. Neuron specific Ki-67 Labelling of mice hippocampal tissue: (a) Control (b) Aβ1–42 -treated group (c) Aβ1–42 + donepezil-treated group (d) Aβ1–42 +RA-treated group (e) Aβ1–42 + UA-treated groups at magnification (A) 4X (B) 10X. (C) Graph showing cell count/10000 µm2 of Ki-67 labelled cells in mice hippocampus in experimental groups respectively. Significance was analyzed by One-way ANOVA followed by Bonferroni comparison test (mean ± SEM) using Graphpad Prism. *p < 0.05 **p < 0.01.
A notable decrease in the hippocampal proliferating cells of group treated with Aβ1–42 (14.30 ± 0.4813; p = 0.0003) relative to control (29.33 ± 1.856) indicated the intensity of neurotoxic effects of Aβ. RA (24.53 ± 1.619; p= 0.0009) and UA (28.13 ± 2.551; p= 0.0015) treatment post Aβ1–42 administration showed improved immunoreactivity with DCX with increase DCX positive cells relative to control and donepezil-treated group (16.07 ± 0.7881; p= .0984) (Figure 4).
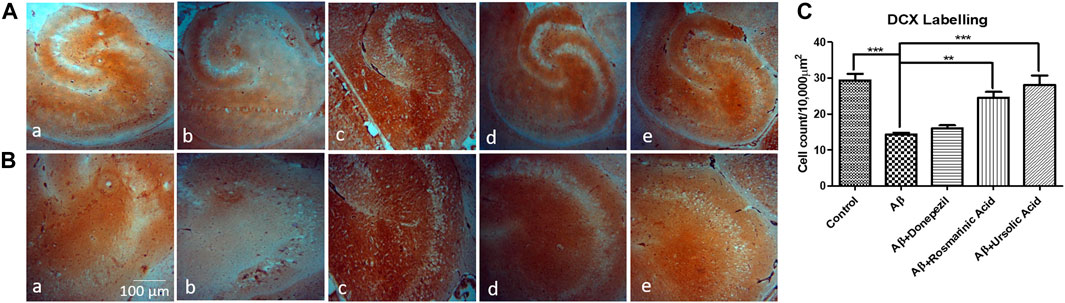
FIGURE 4. Neuron-specific DCX Labeling of mice hippocampal tissue: (a) Control (b) Aβ1–42 -treated group (c) Aβ1–42 + donepezil-treated group (d) Aβ1-42 + RA-treated group (e) Aβ1–42 +UA-treated groups at magnification (A) 4X (B) 10X (C) Graph showing cell count/10000 µm2 of DCX labelled cells in mice hippocampus in experimental groups respectively. Significance was analyzed by One-way ANOVA followed by Bonferroni comparison test (mean ± SEM) using GraphPad Prism. **p < 0.01, ***p < 0.001.
RA and UA treatment reduces the accumulated amyloid beta burden
The presence of congophilic amyloid plaques in the mice administered with Aβ1–42 was substantially decreased with the treatment of RA and UA indicating a reversal of the plaque formation. RA and UA treatment significantly rescued the cellular density and morphology in Aβ1–42 -treated groups and comparatively have greater neuronal restoration than donepezil. The control littermates showed a normal neuronal pattern (Figure 5).
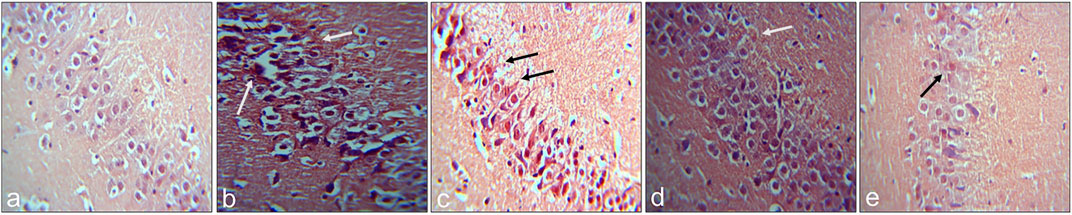
FIGURE 5. Congo red-stained sections of hippocampus for visualization of amyloid plaques: (A) Control (B) Aβ1–42 -treated group (C) Aβ1–42 + donepezil-treated group (D) Aβ1–42 + RA-treated group (E) Aβ1–42 + UA-treated group. Original Magnification 40X.
Molecular docking studies of Ki-67 and DCX with RA, UA and donepezil
Molecular docking studies were used to predict the receptor-ligand interaction geometrics of RA, UA and donepezil with the neurogenesis markers Ki-67 and DCX. All the compounds successfully docked against the target proteins. Lowest atomic contact energy (ACE) is depictive of higher binding affinity, therefore, the ligand molecules that had the lowest ACE were considered better ligands to Ki-67 and DCX. The docking scores of the complexes are shown in Table 1. The docking results were further refined using FireDock and the results obtained are stated in Table 2.
UA exhibits ACE comparable to donepezil in binding interactions with Ki-67 and DCX
Binding interaction of UA, RA, and donepezil with Ki-67 revealed that amongst the other compounds, UA with an ACE of −290.71 had better affinity than RA (−203.58) which was comparable to that of donepezil (−293.37). Interactions with DCX also demonstrated a comparable binding energy of UA (−154.2) and donepezil (−157.92). These binding energy values suggest an interaction of UA with Ki-67 and DCX validating the present in vivo results (Table 1).
UA shows global energy comparable to donepezil in binding interactions with Ki-67 and DCX
Global energy depicts the binding energy of the complex while the ACE is based on its contribution to global energy. Low values of ACE correspond to more stable complexes. UA exhibited an ACE of −10.96 in its interaction with Ki-67 which is comparable to that of donepezil (−10.92). UA (−10.06) also showed a comparable ACE to that of donepezil (−11.84) in its interaction with DCX. Attractive and repulsive vdw (Van der waal forces) are a quantification of the input of van der waal’s forces to the global binding energy. The values are tabulated in Table 2 and the interacting residues are shown in Figure 6.
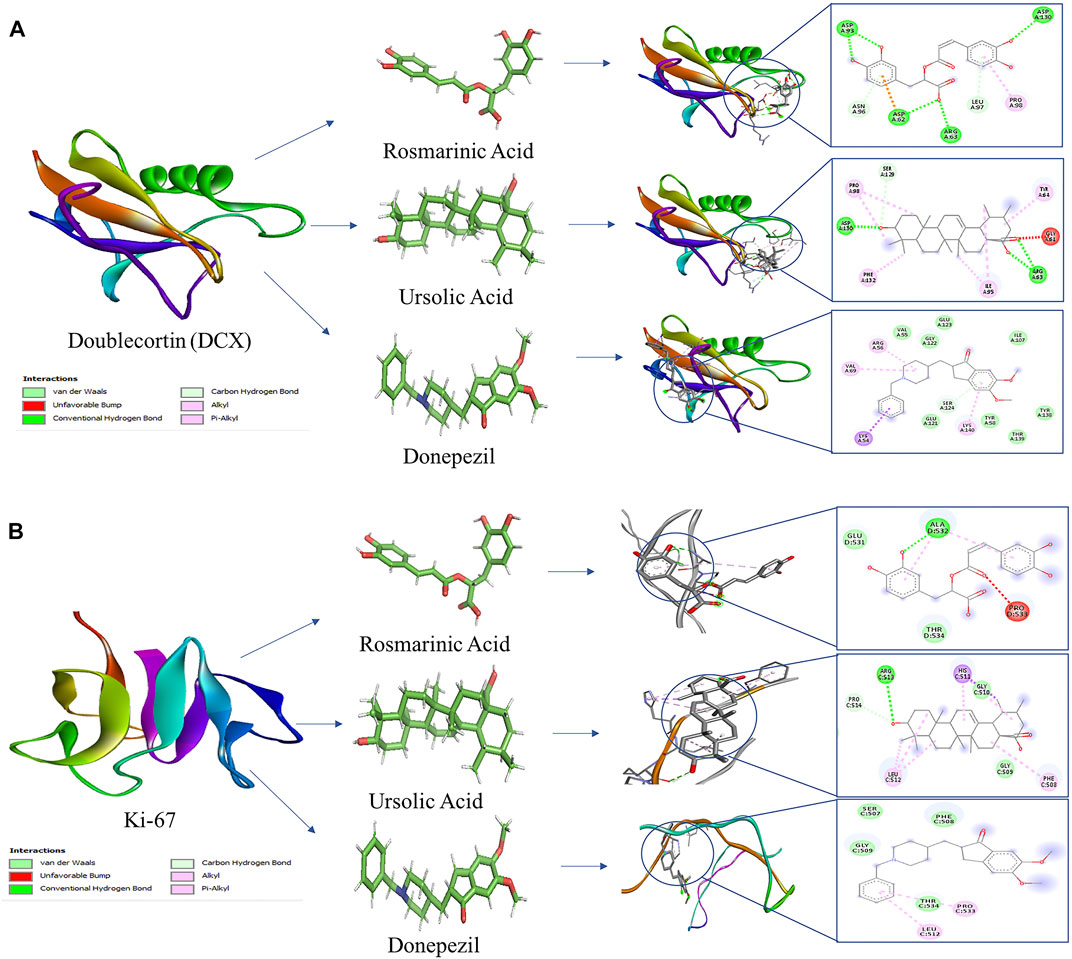
FIGURE 6. Molecular docking interaction models of RA, UA, and donepezil with DCX and Ki-67. 2D structures of the compounds are shown by line and stick models with the surrounding amino acids of (A) DCX and (B) Ki-67. The interactions are denoted by the following colors: hydrogen bonding interactions (green), alkyl bonds (pink) and bumps (red).
Discussion
Our study elucidated the potential effects of the bioactive compounds of R. officinalis, RA and UA on Aβ1–42 -induced neurotoxicity in comparison to donepezil. The data revealed the restoration capability of RA and UA of altered social memory in AD mouse models. Social engagement with the surrounding environment is associated with an improved angiogenesis, synaptogenesis, and neurogenesis which are crucial in delaying the progression of AD (Fratiglioni et al., 2004).
Notably, RA and UA are previously known to exert anxiolytic and antidepressant effects in various models of neurotoxicity (Colla et al., 2015; Ramos-Hryb et al., 2019; Lataliza et al., 2021). The present study showed that RA and UA treatment post Aβ1–42 administration exhibited significantly greater sociability levels relative to the diseased group. RA and UA treatment post Aβ1–42 administration also displayed significant improvement in social novelty preference in mice. The social interaction behavior of mice with RA and UA treatment was comparable to that of the standard drug donepezil which is indicative of their comparable protective effects to restore social memory. These data suggest the potential of RA and UA in alleviating the behavioral deficits in social affiliation and novelty preference induced by Aβ1–42.
Altered social behavior is demonstrated by a decrease in sociability, avoidance of novel social stimuli and exacerbation of aggression associated with amyloid pathology (Kosel et al., 2021). Impaired social interaction memory also results due to mitochondrial damage contributed to increased oxidative stress and deficits in hippocampus and medial pre-frontal cortex activity (Misrani et al., 2021). According to Okada et al., the removal of cholinergic cell groups in the basal forebrain cause an impairment of social behavior which was significantly reinstated by cholinesterase inhibitors, suggesting the critical role of cholinergic dysfunction in sociability deficits corelated with psychological and behavioral symptoms of dementia in AD (Okada et al., 2021). Interestingly, modulation of matrix metallopeptidase 9 (MMP9) caused an improvement in sociability and social recognition memory, along with a reduction in anxiety in AD mouse model, supporting the notion that targeting MMP9 could serve as a therapeutic strategy in restoring the neurobehavioral damage in AD (Ringland et al., 2021).
Although the effect of RA and UA on social memory has not been reported previously but their effect on the restoration of spatial memory and object recognition memory has been documented. A mechanistic study on RA describes its role in the inhibition of cognitive decline through the suppression of tau phosphorylation (Yamamoto et al., 2021) while UA ameliorates oxidative stress and inflammation to improve cognitive deficits in an Aβ-induced mouse model (Liang et al., 2016). UA also exerts radioprotective effects improving radiation induced deficits in memory and learning in BALB/c mice (Tang et al., 2017) whereas also exhibit a potent anti-dementia effect observed in olfactory bulbectomized mice (Nguyen et al., 2022). Improvement of spatial memory and amelioration of Aβ25–35 accumulation by UA has also been demonstrated (Li et al., 2020).
We further studied the effects of RA and UA on altered hippocampal neurogenesis induced by Aβ1-42. Several studies indicate the relevance of Aβ1–42 induction in the impairment of adult hippocampal neurogenesis eliciting neurodegeneration associated loss of memory and cognition. The intra-cerebrovascular injection of Aβ1–42 in male kunming mice caused mitochondrial damage, inflammation, loss of memory (Qi et al., 2019) and altered adult hippocampal neurogenesis observed in balb/c mice (Amber et al., 2020). In addition, the interneuronal accumulation of phosphorylated tau protein is also crucial for AD progression and impairs adult hippocampal neurogenesis through the suppression of GABAergic transmission (Zheng et al., 2020). Contrarily, promotion of neurogenesis reconstructs the degenerated neural circuits in AD hindering the associated cognitive decline (Choi et al., 2018). Aβ1–42 -induced neurotoxicity causes significant deterioration of Ki-67 and DCX expression levels in the hippocampal tissue (Amber et al., 2020). Our results indicated a reduction in the neuronal proliferation induced by Aβ1–42 evident through a significant decline in the immunoreactivity of DCX and Ki-67 positive cells in the mice treated with Aβ1–42, however it was considerably restored upon treatment with UA and RA.
The results of behavioral and immunohistochemical analysis indicated the neurogenic potential of UA and RA. Based on these results, in silico analysis was further conducted to determine the binding interactions of the compounds with the neurogenesis markers, Ki-67 and DCX in comparison to donepezil. Molecular docking analysis showed comparable binding energy values of UA to that of donepezil. UA had an ACE value comparable to that of donepezil in its interaction with Ki-67 and DCX. Previously, we reported the effect of UA and RA in normalizing the mRNA expression levels of neurogenesis markers, Ki-67, DCX and NeuN. Interestingly, UA exhibit significant restoration of the expression levels of these markers in comparison to RA and donepezil (Mirza et al., 2021). It also enhanced neurogenesis and repressed inflammation in temporal lobe epilepsy and cerebral ischemia models (Wang Y. et al., 2018; Liu et al., 2022). Its role in neurite outgrowth and neuronal survival mediated by nerve growth factor has also been reported (Theis et al., 2018). These results reiterate the neurogenic potential of UA through interaction with Ki-67 and DCX indicating its therapeutic potential against neurodegeneration associated with AD.
The reduction in the plaque formation by RA and UA post Aβ1–42 administration also suggests their role in the suppression of AD progression. RA has been previously found to be effective against copper (II)-induced neurotoxicity through the formation of an original ternary association between Aβ and Cu (II) (Kola et al., 2020). Also, the prevention of fibrillization and assembly of β sheets in tau protein suggest its therapeutic potential against AD (Cornejo et al., 2017). Interestingly, RA also reduces the formation of Aβ and ameliorated tissue structure in an AD-like dementia model induced by scopolamine (Deveci et al., 2021). It also exerts anti-apoptotic effect that results in the alleviation of inflammation and oxidative stress associated neurodegeneration as observed in a model for Parkinson’s disease (Lv et al., 2020). Consistently UA also hindered the deposition of Aβ and lowered the levels of its oligomers and monomers in an Aβ-induced Caenorhabditis elegans transgenic model (Wang et al., 2022). These results are indicative of the potential of UA and RA in the reduction of Aβ plaques which constitute a hallmark feature of AD.
Conclusion
This study suggests the pro-neurogenic potential and neuroprotective effects of UA and RA on neurotoxicity induced by Aβ1–42 that represents pathological hallmarks of AD. Our findings revealed that UA and RA can rescue the AD like alterations characterized by accumulated amyloid plaques, impaired social memory and neurogenesis induced by Aβ, thereby reiterating their potential as promising therapeutic agents against AD.
Data availability statement
The original contributions presented in the study are included in the article/supplementary material, further inquiries can be directed to the corresponding author.
Ethics statement
The animal study was reviewed and approved by Internal Review Board, Atta ur Rahman School of Applied Biosciences-NUST.
Author contributions
SZ, substantial contribution to conception and design of the study and finalization of the manuscript; FM, all experimental work, data analysis, interpretation, and drafting the article.
Funding
This work was supported by National University of Sciences and Technology (NUST), Islamabad, Pakistan. The work was partially supported through Research grant number 5974 awarded to SZ under National Research Grants Program for Universities, Higher Education Commission, Pakistan and through the research funding provided to FM under the Indigenous 5000 PhD Fellowship Program, Higher Education Commission, Pakistan. The funding sources had no involvement in study design; in the collection, analysis and interpretation of data; in the writing of the report; and in the decision to submit the article for publication.
Conflict of interest
The authors declare that the research was conducted in the absence of any commercial or financial relationships that could be construed as a potential conflict of interest.
Publisher’s note
All claims expressed in this article are solely those of the authors and do not necessarily represent those of their affiliated organizations, or those of the publisher, the editors and the reviewers. Any product that may be evaluated in this article, or claim that may be made by its manufacturer, is not guaranteed or endorsed by the publisher.
References
Adlimoghaddam, A., Neuendorff, M., Roy, B., and Albensi, B. C. (2018). A review of clinical treatment considerations of donepezil in severe Alzheimer's disease. CNS neurosci. ther. 24 (10), 876–888. doi:10.1111/cns.13035
Ahmed, S., Mahmood, Z., Javed, A., Hashmi, S. N., Zerr, I., Zafar, S., et al. (2017). Effect of metformin on adult hippocampal neurogenesis: comparison with donepezil and links to cognition. J. Mol. Neurosci. 62 (1), 88–98. doi:10.1007/s12031-017-0915-z
Amber, S., Mirza, F. J., Asif, M., Hassan, D., Ahmed, T., Zahid, S., et al. (2020). Amyloid-beta induced neurotoxicity impairs cognition and adult hippocampal neurogenesis in a mouse model for Alzheimer’s disease. Curr. Alzheimer Res. 17 (11), 1033–1042. doi:10.2174/1567205017666201224162730
Amoah, S. K., Sandjo, L. P., Kratz, J. M., and Biavatti, M. W. (2016). Rosmarinic acid–pharmaceutical and clinical aspects. Planta Med. 82 (05), 388–406. doi:10.1055/s-0035-1568274
Anacker, C., Luna, V. M., Stevens, G. S., Millette, A., Shores, R., Jimenez, J. C., et al. (2018). Hippocampal neurogenesis confers stress resilience by inhibiting the ventral dentate gyrus. Nature 559 (7712), 98–102. doi:10.1038/s41586-018-0262-4
Armstrong, R. A. (2009). The molecular biology of senile plaques and neurofibrillary tangles in Alzheimer’s disease. Folia Neuropathol. 47 (4), 289–299.
Breijyeh, Z., and Karaman, R. (2020). Comprehensive review on Alzheimer’s disease: Causes and treatment. Molecules 25 (24), 5789. doi:10.3390/molecules25245789
Chen, G. F., Xu, T. H., Yan, Y., Zhou, Y. R., Jiang, Y., Melcher, K., et al. (2017). Amyloid beta: structure, biology and structure-based therapeutic development. Acta Pharmacol. Sin. 38 (9), 1205–1235. doi:10.1038/aps.2017.28
Choi, S. H., Bylykbashi, E., Chatila, Z. K., Lee, S. W., Pulli, B., Clemenson, G. D., et al. (2018). Combined adult neurogenesis and BDNF mimic exercise effects on cognition in an Alzheimer’s mouse model. Science 361 (6406), eaan8821. doi:10.1126/science.aan8821
Colla, A. R., Rosa, J. M., Cunha, M. P., and Rodrigues, A. L. S. (2015). Anxiolytic-like effects of ursolic acid in mice. Eur. J. Pharmacol. 758, 171–176. doi:10.1016/j.ejphar.2015.03.077
Cornejo, A., Aguilar Sandoval, F., Caballero, L., Machuca, L., Muñoz, P., Caballero, J., et al. (2017). Rosmarinic acid prevents fibrillization and diminishes vibrational modes associated to β sheet in tau protein linked to Alzheimer’s disease. J. enzyme Inhib. Med. Chem. 32 (1), 945–953. doi:10.1080/14756366.2017.1347783
Crous-Bou, M., Minguillón, C., Gramunt, N., and Molinuevo, J. L. (2017). Alzheimer’s disease prevention: from risk factors to early intervention. Alzheimer's Res. Ther. 9 (1), 71–79. doi:10.1186/s13195-017-0297-z
Deveci, E., Özkorkmaz, E. G., Aşır, F., Şahin, F., Ermiş, I. S., Ertaş, A., et al. (2021). A histopathological comparison of prophylactic effects of Rosmarinic Acid and Oleanolic Acid isolated from Salvia species (sage) in scopolamine induced dementia model. J. Drug Deliv. Ther. 11 (6-S), 37–42. doi:10.22270/jddt.v11i6-S.5129
Eskandary, A., Moazedi, A. A., Zade, H. N., and Akhond, M. R. (2019). Effects of donepezil hydrochloride on neuronal response of pyramidal neurons of the CA1 hippocampus in rat model of Alzheimer’s disease. Basic Clin. Neurosci. 10 (2), 109–117. doi:10.32598/bcn.9.10.305
Farr, S. A., Niehoff, M. L., Ceddia, M. A., Herrlinger, K. A., Lewis, B. J., Feng, S., et al. (2016). Effect of botanical extracts containing carnosic acid or rosmarinic acid on learning and memory in SAMP8 mice. Physiol. Behav. 165, 328–338. doi:10.1016/j.physbeh.2016.08.013
Fratiglioni, L., Paillard-Borg, S., and Winblad, B. (2004). An active and socially integrated lifestyle in late life might protect against dementia. Lancet Neurol 3 (6), 343–353. doi:10.1016/S1474-4422(04)00767-7
Hase, T., Shishido, S., Yamamoto, S., Yamashita, R., Nukima, H., Taira, S., et al. (2019). Rosmarinic acid suppresses Alzheimer’s disease development by reducing amyloid β aggregation by increasing monoamine secretion. Sci. Rep. 9 (1), 8711–8713. doi:10.1038/s41598-019-45168-1
Karthika, C., Appu, A. P., Akter, R., Rahman, M., Tagde, P., Ashraf, G. M., et al. (2022). Potential innovation against Alzheimer’s disorder: A tricomponent combination of natural antioxidants (vitamin E, quercetin, and basil oil) and the development of its intranasal delivery. Environ. Sci. Pollut. Res. Int., 1–16. doi:10.1007/s11356-021-17830-7
Kola, A., Hecel, A., Lamponi, S., and Valensin, D. (2020). Novel perspective on Alzheimer’s disease treatment: Rosmarinic acid molecular interplay with copper (II) and amyloid β. Life 10 (7), 118. doi:10.3390/life10070118
Kosel, F., Hamilton, J. S., Harrison, S. L., Godin, V., and Franklin, T. B. (2021). Reduced social investigation and increased injurious behavior in transgenic 5xFAD mice. J. Neurosci. Res. 99 (1), 209–222. doi:10.1002/jnr.24578
Lane, C. A., Hardy, J., and Schott, J. M. (2018). Alzheimer's disease. Eur. J. neurology 25, 59–70. doi:10.1111/ene.13439
Lataliza, A. A. B., de Assis, P. M., da Rocha Laurindo, L., Gonçalves, E. C. D., Raposo, N. R. B., and Dutra, R. C. (2021). Antidepressant-like effect of rosmarinic acid during LPS-induced neuroinflammatory model: The potential role of cannabinoid receptors/PPAR-γ signaling pathway. Phytother. Res. 35 (12), 6974–6989. doi:10.1002/ptr.7318
Li, S. J., Liu, Q., He, X. B., Liu, J. P., Liu, X. L., Hu, J., et al. (2020). Pyrola incarnata demonstrates neuroprotective effects against β-amyloid-induced memory impairment in mice. Bioorg. Med. Chem. Lett. 30 (2), 126858. doi:10.1016/j.bmcl.2019.126858
Liang, W., Zhao, X., Feng, J., Song, F., and Pan, Y. (2016). Ursolic acid attenuates beta-amyloid-induced memory impairment in mice. Arq. neuro-psiquiatr 74, 482–488. doi:10.1590/0004-282X20160065
Liu, K. M., Huang, Y., Wan, P. P., Lu, Y. H., Zhou, N., Li, J. J., et al. (2022). Ursolic acid protects neurons in temporal lobe epilepsy and cognitive impairment by repressing inflammation and oxidation. Front. Pharmacol. 13, 877898. doi:10.3389/fphar.2022.877898
Lopez, J. S., Gonzalez, H. M., and Léger, G. (2019). Alzheimer disease. Handb. Clin. Neurol. 167, 231–255. doi:10.1016/B978-0-12-804766-8.00013-3
Lv, R., Du, L., Zhou, F., Yuan, X., Liu, X., and Zhang, L. (2020). Rosmarinic acid alleviates inflammation, apoptosis, and oxidative stress through regulating miR-155-5p in a mice model of Parkinson’s disease. ACS Chem. Neurosci. 11 (20), 3259–3266. doi:10.1021/acschemneuro.0c00375
Mashiach, E., Schneidman-Duhovny, D., Andrusier, N., Nussinov, R., and Wolfson, H. J. (2008). FireDock: a web server for fast interaction refinement in molecular docking. Nucleic acids Res. 36, W229–W232. doi:10.1093/nar/gkn186
Mirza, F. J., Amber, S., Hassan, D., Ahmed, T., and Zahid, S. (2021). Rosmarinic acid and ursolic acid alleviate deficits in cognition, synaptic regulation and adult hippocampal neurogenesis in an Aβ1-42-induced mouse model of Alzheimer's disease. Phytomedicine 83, 153490. doi:10.1016/j.phymed.2021.153490
Mirza, F. J., Zahid, S., Amber, S., Jabeen, H., Asim, N., Ali Shah, S. A., et al. (2022). Multitargeted molecular docking and dynamic simulation studies of bioactive compounds from Rosmarinus officinalis against Alzheimer’s disease. Molecules 27 (21), 7241. doi:10.3390/molecules27217241
Misrani, A., Tabassum, S., Huo, Q., Tabassum, S., Jiang, J., Ahmed, A., et al. (2021). Mitochondrial deficits with neural and social damage in early-stage Alzheimer’s disease model mice. Front. aging Neurosci. 13, 748388. doi:10.3389/fnagi.2021.748388
Moreno-Jiménez, E. P., Flor-García, M., Terreros-Roncal, J., Rábano, A., Cafini, F., Pallas-Bazarra, N., et al. (2019). Adult hippocampal neurogenesis is abundant in neurologically healthy subjects and drops sharply in patients with Alzheimer’s disease. Nat. Med. 25 (4), 554–560. doi:10.1038/s41591-019-0375-9
Nguyen, H. T., Le, X. T., Van Nguyen, T., Phung, H. N., Pham, H. T. N., Nguyen, K. M., et al. (2022). Ursolic acid and its isomer oleanolic acid are responsible for the anti-dementia effects of Ocimum sanctum in olfactory bulbectomized mice. J. Nat. Med. 76 (3), 621–633. doi:10.1007/s11418-022-01609-2
Okada, K., Nishizawa, K., Kobayashi, T., Sakata, S., Hashimoto, K., and Kobayashi, K. (2021). Distinct roles of basal forebrain cholinergic neurons in spatial and object recognition memory. Sci. Rep. 11 (1), 13158–13212. doi:10.1038/srep13158
Qi, Y., Shang, L., Liao, Z., Su, H., Jing, H., Wu, B., et al. (2019). Intracerebroventricular injection of resveratrol ameliorated Aβ-induced learning and cognitive decline in mice. Metab. Brain Dis. 34 (1), 257–266. doi:10.1007/s11011-018-0348-6
Ramos-Hryb, A. B., Platt, N., Freitas, A. E., Heinrich, I. A., López, M. G., Leal, R. B., et al. (2019). Protective effects of ursolic acid against cytotoxicity induced by corticosterone: role of protein kinases. Neurochem. Res. 44 (12), 2843–2855. doi:10.1007/s11064-019-02906-1
Ringland, C., Schweig, J. E., Eisenbaum, M., Paris, D., Ait-Ghezala, G., Mullan, M., et al. (2021). MMP9 modulation improves specific neurobehavioral deficits in a mouse model of Alzheimer’s disease. BMC Neurosci. 22 (1), 39–25. doi:10.1186/s12868-021-00643-2
Rizwan, S., Idrees, A., Ashraf, M., and Ahmed, T. (2016). Memory-enhancing effect of aspirin is mediated through opioid system modulation in an AlCl3-induced neurotoxicity mouse model. Exp. Ther. Med. 11 (5), 1961–1970. doi:10.3892/etm.2016.3147
Sadeghi, A., Esfandiary, E., Hami, J., Khanahmad, H., Hejazi, Z., Mardani, M., et al. (2018). The effects of maternal diabetes and insulin treatment on neurogenesis in the developing hippocampus of male rats. J. Chem. Neuroanat. 91, 27–34. doi:10.1016/j.jchemneu.2018.03.005
Salomone, S., Caraci, F., Leggio, G. M., Fedotova, J., and Drago, F. (2012). New pharmacological strategies for treatment of Alzheimer’s disease: focus on disease modifying drugs. Br. J. Clin. Pharmacol. 73 (4), 504–517. doi:10.1111/j.1365-2125.2011.04134.x
Schneidman-Duhovny, D., Inbar, Y., Nussinov, R., and Wolfson, H. J. (2005). PatchDock and SymmDock: servers for rigid and symmetric docking. Nucleic acids Res. 33, W363–W367. doi:10.1093/nar/gki481
Shahsavani, M., Pronk, R. J., Falk, R., Lam, M., Moslem, M., Linker, S. B., et al. (2018). An in vitro model of lissencephaly: expanding the role of DCX during neurogenesis. Mol. psychiatry 23 (7), 1674–1684. doi:10.1038/mp.2017.175
Sun, X., and Kaufman, P. D. (2018). Ki-67: more than a proliferation marker. Chromosoma 127 (2), 175–186. doi:10.1007/s00412-018-0659-8
Systèmes, D. (2016). Biovia, discovery studio modeling environment. San Diego, CA, USA: Dassault Systèmes Biovia.
Tang, F. R., Loke, W. K., Wong, P., and Khoo, B. C. (2017). Radioprotective effect of ursolic acid in radiation-induced impairment of neurogenesis, learning and memory in adolescent BALB/c mouse. Physiol. Behav. 175, 37–46. doi:10.1016/j.physbeh.2017.03.027
Theis, T., Johal, A. S., Kabat, M., Basak, S., and Schachner, M. (2018). Enhanced neuronal survival and neurite outgrowth triggered by novel small organic compounds mimicking the LewisX glycan. Mol. Neurobiol. 55 (10), 8203–8215. doi:10.1007/s12035-018-0953-8
Twilley, D., Rademan, S., and Lall, N. (2020). A review on traditionally used South African medicinal plants, their secondary metabolites and their potential development into anticancer agents. J. ethnopharmacol. 261, 113101. doi:10.1016/j.jep.2020.113101
Wang, N., Wang, E., Wang, R., Muhammad, F., Li, T., Yue, J., et al. (2022). Ursolic acid ameliorates amyloid β-induced pathological symptoms in Caenorhabditis elegans by activating the proteasome. NeuroToxicology 88, 231–240. doi:10.1016/j.neuro.2021.12.004
Wang, X. T., Gong, Y., Zhou, B., Yang, J. J., Cheng, Y., Zhao, J. G., et al. (2018). Ursolic acid ameliorates oxidative stress, inflammation and fibrosis in diabetic cardiomyopathy rats. Biomed. Pharmacother. 97, 1461–1467. doi:10.1016/j.biopha.2017.11.032
Wang, Y., Li, L., Deng, S., Liu, F., and He, Z. (2018). Ursolic acid ameliorates inflammation in cerebral ischemia and reperfusion injury possibly via high mobility group box 1/Toll-like receptor 4/NFκB pathway. Front. Neurol. 9, 253. doi:10.3389/fneur.2018.00253
Xu, H. L., Wang, X. T., Cheng, Y., Zhao, J. G., Zhou, Y. J., Yang, J. J., et al. (2018). Ursolic acid improves diabetic nephropathy via suppression of oxidative stress and inflammation in streptozotocin-induced rats. Biomed. Pharmacother. 105, 915–921. doi:10.1016/j.biopha.2018.06.055
Yamamoto, S., Kayama, T., Noguchi-Shinohara, M., Hamaguchi, T., Yamada, M., Abe, K., et al. (2021). Rosmarinic acid suppresses tau phosphorylation and cognitive decline by downregulating the JNK signaling pathway. NPJ Sci. food 5 (1), 1–11. doi:10.1038/s41538-021-00084-5
Keywords: Alzheimer’s disease, neurodegeneration, adult hippocampal neurogenesis, rosmarinic acid, ursolic acid, DCX, Ki-67
Citation: Mirza FJ and Zahid S (2022) Ursolic acid and rosmarinic acid ameliorate alterations in hippocampal neurogenesis and social memory induced by amyloid beta in mouse model of Alzheimer’s disease. Front. Pharmacol. 13:1058358. doi: 10.3389/fphar.2022.1058358
Received: 30 September 2022; Accepted: 12 December 2022;
Published: 22 December 2022.
Edited by:
Syed Shams ul Hassan, Shanghai Jiao Tong University, ChinaReviewed by:
Muhammad Ayaz, University of Malakand, PakistanAjmal Khan, University of Peshawar, Pakistan
Copyright © 2022 Mirza and Zahid. This is an open-access article distributed under the terms of the Creative Commons Attribution License (CC BY). The use, distribution or reproduction in other forums is permitted, provided the original author(s) and the copyright owner(s) are credited and that the original publication in this journal is cited, in accordance with accepted academic practice. No use, distribution or reproduction is permitted which does not comply with these terms.
*Correspondence: Saadia Zahid, saadia.zahid@asab.nust.edu.pk