- 1Department of Plastic and Reconstructive Surgery, The First Hospital of Jilin University, Changchun, Jilin, China
- 2Department of Thoracic Surgery, The First Hospital of Jilin University, Changchun, Jilin, China
Esophageal cancer (EC) is a common tumor of the gastrointestinal system and a major threat to human health. The etiology and incidence of EC vary depending on the type of pathology. Owing to the unique physiological structure of the esophagus and the poor biological behavior of EC, the treatment modalities available are limited, and the prognosis of patients is relatively poor. Curcumin is a type of natural phytochemical belonging to the class of phenolic compounds. It exerts favorable anticancer effects on various cancers. A growing body of evidence indicates that curcumin suppresses tumor development and progression by inhibiting tumor cell proliferation, invasion, and migration, thus inducing apoptosis, regulating microRNA expression, reversing multidrug resistance, and inducing sensitivity to the therapeutic effect of chemoradiotherapy. Multiple cellular molecules, growth factors, and genes encoding proteins participating in different signaling pathways interact with each other to contribute to the complex and orderly anticancer effect. The efficacy and safety of curcumin have been established in preclinical studies for EC and clinical trials for other cancers. However, the low bioavailability of curcumin limits its clinical application. Therefore, the modification of curcumin analogs, the combination of curcumin with other drugs or therapies, and the use of novel nanocarriers have been widely investigated to improve the clinical effects of curcumin in EC.
1 Introduction
The esophagus forms the upper segment of the gastrointestinal tract and is an important part of the gastrointestinal system. Esophageal cancer (EC) is one of the most common gastrointestinal system malignancies worldwide, with approximately 604,000 new cases and 544,000 deaths reported in 2020 according to global cancer statistics (Sung et al., 2021). EC accounts for the seventh-highest incidence of cancer and ranks as the sixth-leading cause of cancer death globally (Sung et al., 2021). EC comprises two primary histological types, esophageal adenocarcinoma (EAC) and esophageal squamous cell carcinoma (ESCC), which differ in etiology and geography. The risk factors of EAC primarily include obesity, gastroesophageal reflux disease (GERD), and Barrett’s esophagus, and the increase in these risk factors in populations of high-income countries contributes to the high prevalence of EAC, accounting for approximately two-thirds of EC cases (Blot et al., 2018; Uhlenhopp et al., 2020). Barrett’s esophagus is characterized by the presence of a precancerous lesion and the induction of columnar metaplasia in the normal squamous epithelium owing to injury resulting from GERD in the distal esophagus (Clermont and Falk, 2018). However, in countries with a lower income, ESCC accounts for approximately 90% of patients with EC. Smoking, nitrosamine intake, dietary habits such as preference for hot food, pickled vegetables, and high alcohol consumption are important factors leading to ESCC (Uhlenhopp et al., 2020). The pathogenesis of EC has not been completely elucidated, and the occurrence of EC may result from the long-term interaction between genetic factors and environmental factors. However, despite extensive progress in research on the diagnosis and treatment of EC, its morbidity and mortality rates are still on the rise, and the 5-year survival rate remains low (Uhlenhopp et al., 2020).
The symptoms of EC are insidious in the early stage of the disease. When the symptoms of dysphagia and other discomforts become apparent, often, the disease has already been locally advanced. Very limited treatment options are available owing to the late diagnosis and poor biological behavior of EC. Currently, the treatment of EC is based on different stages and primarily includes endoscopic therapy, surgery, chemotherapy, and radiotherapy, among which endoscopic therapy is applied more commonly for early mucosal disease. For locally advanced EC, the significance of combining chemotherapy and radiotherapy with surgery has been established. For example, a meta-analysis including 17 trials and seven studies reported that neoadjuvant chemoradiotherapy or chemotherapy had a significant survival benefit over surgery alone for patients with EC (Sjoquist et al., 2011). A more recent clinical trial also had shown that patients with EC who received neoadjuvant chemoradiotherapy had higher overall survival rates than those who received only surgery (Shapiro et al., 2015). The technology used for EC treatment has made great advances, but the survival rate has not improved significantly, and the overall efficacy remains unsatisfactory. In addition, these treatment modalities also have certain negative effects, such as the various complications arising from surgery, drug toxicity from chemotherapy, and tissue damage from radiation. New therapeutic strategies need to be complemented by suppressing side effects and enhancing sensitivity to chemoradiotherapy to improve the outcome.
In recent times, dietary phytochemicals have received extensive attention in anticancer research. Some safe and nontoxic molecules isolated from plant products have been identified as potential anticancer agents. Curcumin (diferuloylmethane), a natural phenolic compound, is derived from the plant Curcuma longa. The rhizomes of this plant have been used extensively as a flavor supplement in Asian cuisine for centuries. Curcumin or 1,7-bis-(4-hydroxy-3-methoxyphenyl)-1,6-heptadieno-3,5-dione is composed of two ferulic acid residues linked by a methylene bridge. It is insoluble in water but soluble in several organic solvents (Gupta et al., 2013). The safety of curcumin is confirmed by its dietary consumption for centuries and evidence reported in clinical trials. Curcumin was also shown to be well tolerated and efficiently eliminated in humans (Cheng et al., 2001). A growing body of evidence indicates that curcumin possesses multiple pharmacological properties such as anticancer, antioxidant, and anti-inflammatory properties (Menon and Sudheer, 2007; Tomeh et al., 2019). In addition, the cancer-preventive potential and anticancer effect of curcumin remain important topics in cancer research. To this end, the effects of curcumin have been investigated in various cancers, such as prostate, colon, lung, esophagus, and breast cancers, among others (Allegra et al., 2017).
In this review, we summarize the anticancer mechanism of action of curcumin with respect to the effects reported in vitro in cancer cells, animal models, and clinical trials in the treatment of EC. Besides, we also highlight the importance of new analogs of curcumin, combination therapies, and novel delivery systems in EC, to enhance the clinical efficacy of curcumin. The mechanism of action of curcumin and strategies for improvement of efficacy are shown in Figure 1.
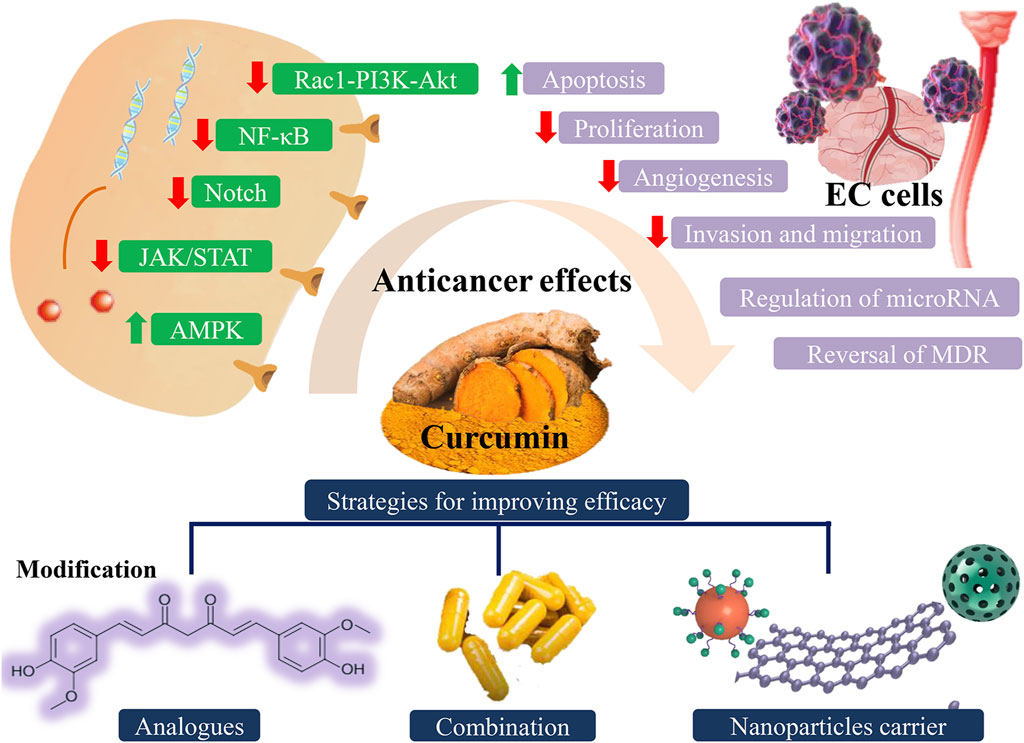
FIGURE 1. Different anticancer effects of curcumin involved in multiple signaling pathways and possible strategies for improving efficacy of curcumin. EC: esophageal cancer.
2 The anticancer mechanism of action of curcumin
The anti-tumor activity of curcumin can affect different types of cancer cells at multiple stages of tumorigenesis and cancer development. The anticancer mechanism of action of curcumin is complex and diverse and can be summarized from various aspects.
2.1 Growth inhibition and apoptosis induction
Cancer cells have the property of unlimited proliferation and induction of cell cycle arrest may be an effective way to suppress cell growth. A study reported that curcumin could trigger G2/M cell cycle arrest and cellular senescence in cervical cancer cell lines in a dose-dependent manner, leading to decreased cell survival (Wang et al., 2020). In addition to inhibiting cell growth, curcumin was also involved in the induction of cellular autophagy by promoting the accumulation of reactive oxygen species (ROS) (Wang et al., 2020). Excessive ROS levels can affect cell function and even cause cell death. Under the oxidative stress, autophagy is activated and cellular components are degraded.
Apoptosis, also known as programmed cell death, is often regulated by a highly ordered multigene process of active cell death. Abnormalities in apoptosis lead to uncontrolled cell proliferation and eventually to cancer development (Pistritto et al., 2016). Curcumin can induce the apoptosis of cancer cells and inhibit tumor growth through various mechanisms, thus achieving anti-tumor effects. Caspases play a critical role in apoptosis activation and execution. Apoptosis is a cascade reaction in which caspases are activated and perform their corresponding functions (Boice and Bouchier-Hayes, 2020). Curcumin was shown to induce apoptosis in EC cells by activating caspase 3 and continuously amplifying death signaling (Subramaniam et al., 2012). The promotion of apoptosis was characterized by the increased ratio of Bcl-2/Bax proteins. Reportedly, the mitochondria could be targeted by curcumin to induce apoptosis in cancer cells (Morin et al., 2001). Curcumin contributed to the management of the opening and closing of the permeability transition pore of the mitochondria. When curcumin induces cell apoptosis, it can increase the membrane permeability, leading to swelling of mitochondria. This causes the loss of the mitochondrial membrane potential and the blockade of ATP synthesis, thus triggering the death of cancer cells. Moreover, curcumin can activate apoptotic signaling by perturbing intracellular calcium homeostasis through the endoplasmic reticulum pathway in thyroid carcinoma cells (Zhang et al., 2018). The endoplasmic reticulum plays a key role in maintaining the stability of the cellular calcium content and the synthesis and processing of proteins. The increase in the cytosolic calcium content induced by curcumin can cause excessive endoplasmic reticulum stress, leading to the activation of the mitochondrial apoptotic pathway. Furthermore, the induction of a mitotic catastrophe may act as a distinct death mechanism, a type of non-apoptotic cell death (O'Sullivan-Coyne et al., 2009). These different targets of apoptosis induction may be promising therapeutic targets in the inhibition of cancer cells.
2.2 Inhibition of tumor cell invasion and migration
More patients with tumors are estimated to eventually die from the growth of metastatic tumors than from the primary tumor (Bergers and Fendt, 2021). The dispersal of tumor cells is the most dangerous process in tumor development. Epithelial-mesenchymal transition is characterized by an altered cell phenotype and enhanced cell migration and motility and is closely related to tumor metastasis (Yang and Weinberg, 2008). Tumor cells are dislodged from the primary site and bind to the extracellular matrix (ECM) via cell surface receptors and secrete proteases to promote cellular ECM breakdown to reach their metastatic sites (Seyfried and Huysentruyt, 2013). When tumor cells form clones in distant organs, they often cause serious damage to the organism.
Reduced intercellular adhesion is a morphological characteristic of malignancy and tumor metastasis and leads to cell detachment and destruction (Hirohashi and Kanai, 2003). Curcumin-treated esophageal cancer cells showed the promotion of cell-cell adhesion and cell-matrix adhesion, which indicated the antimetastatic effect of curcumin (Zheng et al., 2018). Ray et al. (2003) showed that the binding to ECM, the expression of cell receptors, and the activity of collagenase in melanoma cells can be reduced by treatment with curcumin. In addition, curcumin enhanced the expression of nonmetastatic gene 23 (Nm23), antimetastatic protein tissue inhibitor metalloproteinase (TIMP)-2, and E-cadherin, which inhibited the migration of tumor cells. In addition, Wroński et al. (2021) revealed a mechanism of bladder cancer progression, in which cancer cells activated matrix metalloproteinases (MMPs) and invaded the urothelial basement membrane. Curcumin can inhibit the aberrant activation of MMPs and maintain the integrity of the basement membrane, thus suppressing eventual cancer invasion into the bladder. The anti-metastatic effect of curcumin in the different phases of tumor invasion provides a therapeutic opportunity to prevent or reduce tumor metastasis.
2.3 Anti-angiogenesis
Angiogenesis is an important condition for tumor development and facilitates the entry of tumor cells into the circulation for metastasis and dissemination (Folkman, 2002). Multiple growth factors, such as tumor growth factor β (TGF-β), vascular endothelial growth factor (VEGF), and angiopoietin, among others, are involved in angiogenesis and exhibit mutual interaction.
Curcumin and its analogs could potentiate the anti-angiogenic effects in pancreatic cancer (PC) cell lines by inhibiting the secretion of VEGF, angiopoietin, and TGF-β via the suppression of nuclear factor kappa B (NF-κB) and hypoxia-induced factor-1α (HIF-1α) (Nagaraju et al., 2015). Similarly, a reduction in angiogenesis was also observed in colorectal cancer (CRC) cells treated with curcumin and its analogs (Rajitha et al., 2017). Cancer cells elicited the decreased secretion of HIF-1α and VEGF owing to the inhibition of NF-κB expression by curcumin. Hepatic stellate cells (HSCs) were capable of inducing angiogenesis and cell invasion in the progression of hepatocarcinoma (HCC) (Li et al., 2016). The downregulation of HIF-1α induced by curcumin could decrease tube formation and VEGF expression in HSC-induced HCC, thus reducing tumor angiogenesis and invasion (Shao et al., 2019). The authors further suggested that the anti-angiogenesis effect of curcumin was attributed to the expression of a downstream gene of HIF-1α, namely connective tissue growth factor (CTGF). These growth factors may be potential targets for the prevention of tumor angiogenesis and progression.
2.4 Regulation of microRNA (miRNAs)
MiRNAs are a class of evolutionarily conserved non-coding small molecule RNAs that range from 20 to 24 nucleotides in length and function as regulators in gene expression at the translational level (Bartel, 2004). MiRNAs play a role in cell proliferation, apoptosis, differentiation, cell cycle, and metabolism. Findings from numerous studies have shown that curcumin affects tumor development through the regulation of miRNAs.
MiRNA-21 (miR-21), known as a type of onco-miRNA, contributes to tumor growth and proliferation by targeting the PTEN/PI3K/Akt pathway (Zhang et al., 2012). Curcumin could induce apoptosis and suppress proliferation in gastric cancer (GC) cell lines by downregulating miR-21 (Qiang et al., 2019). Besides, the decreased expression of Akt and increased expression of PTEN indicated that the PTEN/PI3K/Akt pathway was negatively modulated by curcumin to inhibit tumor progression. In another study, curcumin and its analogs were shown to decrease cell viability and induce apoptosis in CRC cell lines by downregulating specificity protein family, which is dependent on activation by ROS (Gandhy et al., 2012). ZBTB10 and ZBTB4 acted as repressors of specificity protein family and were regulated by miR-27a, miR-20a, and miR-17-5p. Therefore, curcumin exert its anticancer effect by the regulation of these miRNAs.
Moreover, miRNAs play a role in cell migration and drug resistance. The loss of expression of the epithelial marker E-cadherin has been recognized as one of the most distinctive features of EMT (Perl et al., 1998). The overexpression of miR-200 resulted in the reduction of cell migration in HCC cell lines via the upregulation of E-cadherin and inhibition of EMT, with no effect on cell growth (Hung et al., 2013). Low levels of miR-200 expression were associated with a poor differentiation status of HCC cells, as determined based on the cell morphology and migratory capacity. Besides, an association exists between miRNA and drug resistance. Liang et al. (2013) compared the effect of curcumin on different HCC cell lines (HepJ5 and HepG2) and found that HepJ5 cells expressed less miR-200a/b and were more responsive to curcumin than HepG2 cells. The overexpression of miR-200a/b may make HCC cells more resistant to curcumin. In addition, an increase in apoptosis was observed in control HepJ5 cells compared with that in HepJ5 cells overexpressing miR200 a/b after curcumin treatment. MiR200 may be a helpful biomarker to assess the anti-apoptotic effect of curcumin. Furthermore, EMT-suppressive miRNAs (miR200b/c, miR-429, miR-141, and others) were activated by curcumin to induce EMT inhibition, which suppressed proliferation and triggered apoptosis in 5-fluorouracil (5-FU)-resistant CRC cells (Toden et al., 2015). The overexpression of miR-200c enhanced the sensitivity of curcumin and 5-FU through the suppression of EMT, thus promoting drug efficacy. The role of the miR200 family requires to be elucidated further, as it exhibits varied effects in different cell lines.
2.5 Reversal of multidrug resistance
Chemotherapy is an important modality in the clinical treatment of oncological diseases. The development of multidrug resistance (MDR) in tumor cells is one of the major causes of poor prognosis in patients owing to chemotherapy failure and an important challenge in clinical antitumor therapy. Therefore, the inhibition of MDR in cancer cells and improvement of the efficacy of chemotherapeutic drugs are urgent needs in the field. Besides exerting anticancer effects, curcumin also exhibits MDR reversal properties.
Tang et al. (2005) assessed the effect of curcumin on the MDR of vincristine (VCR)-resistant GC cell lines (Tang et al., 2005). Curcumin could enhance apoptosis induced by VCR in VCR-resistant cells, which indicates that it countered apoptotic resistance in these MDR cells. The MDR-reversing potential of curcumin was achieved via the downregulation of P-glycoprotein (P-gp) expression and activation of caspase-3. P-gp could transport unrelated substances, such as drugs, through plasma membranes to reduce the level of cytotoxicity. Curcumin was also shown to reverse MDR in PC cell lines by suppressing multi-drug resistance-associated protein 5 (MRP5) and thus reducing drug efflux (Li et al., 2011). Moreover, Shakibaei et al. (2014) revealed a novel mechanism of action of curcumin in reversing resistance to 5-FU in 5-FU-resistant CRC cell lines by inhibiting cancer stem cells (CSCs). Curcumin could sensitize cells to 5-FU and potentiate the anticancer effects of 5-FU in chemo-resistant cells. CRCs treated with both curcumin and 5-FU showed a significant reduction in the expression of CSC markers (CD133, CD44, and ALDH1) compared to CRCs treated with either, which suggests that curcumin exerts a strong chemosensitizing effect on CSCs. CSCs are subpopulations of the tumor cell population with the potential for renewal and contribute to tumor invasion (Huang et al., 2020). Conventional chemotherapeutic agents cannot destroy cancer cells owing to the drug resistance of CSCs. The functions of curcumin in drug resistance promote the clinical application of conventional chemotherapeutic drugs.
2.6 Sensitization of radiation therapy
Radiotherapy may be one of the preferred methods for patients with cancer who are not sensitive to chemotherapy. However, some patients are also resistant to radiotherapy. Novel radiosensitization approaches are needed to increase the responsiveness of patients to radiation. The application of curcumin as an adjuvant in radiotherapy has been shown to be effective.
Curcumin combined with gamma-radiation inhibited tumor growth in mice with a colon cancer xenograft through the downregulation of the NF-κB pathway (Kunnumakkara et al., 2008). The expression of the proliferation marker Ki-67, angiogenesis markers (CD31 and VEGF), and NF-κB-regulated genes decreased in tumor tissues, leading to the enhanced susceptibility of cancer cells to radiation and suppression of tumor growth. Liu et al. (2018a) also demonstrated that curcumin potentiated the anticancer effect of radiation in ESCC cell lines by inhibiting the NF-κB pathway. Treatment with curcumin in an irradiated environment promoted apoptosis induction in cancer cells and prolonged survival in xenograft mice. Moreover, Shehzad et al. (2013) revealed another mechanism by which curcumin improved the sensitivity of cancer cells to radiation therapy. Curcumin enhanced ionizing radiation-induced death in colon cancer cells by downregulating the pre-mRNA processing factor 4 kinase (Prp4K) and upregulating ROS. Prp4K is an antioxidant enzyme that protects cells against oxidative damage, and the overexpression of Prp4K conferred resistance to radiation in cancer cells.
3 Anticancer effect of curcumin in preclinical studies of EC and clinical trials of other cancers
3.1 In vitro studies
Preclinical in vitro studies have shown the efficacy of curcumin in the treatment of EC (Table 1). Accumulating evidence indicates that curcumin can affect different cellular and molecular signaling pathways involved in tumor development. Zhang et al. (2015) reported that curcumin could suppress cell growth and cause cell cycle arrest in the G2/M phase in esophageal Ec109 cells by activating the AMPK signaling pathway. Cancer cells can increase energy metabolism through glycolysis to promote their proliferation. AMPK plays an important role in cellular metabolic activities. The curcumin-mediated activation of AMPK was associated with a decrease in the expression of glycolytic enzymes, which reduced the energy demand of cancer cells and suppressed their proliferation. Another signaling pathway, the NF-κB signaling pathway, is involved in cancer cell proliferation, chemoresistance, and metastasis (Dolcet et al., 2005). NF-κB was shown to be a contributing factor in the progression of Barrett’s metaplasia to EAC (Hartojo et al., 2010). Barrett’s metaplasia samples showed lower expression of NF-κB and greater expression of the effector caspase compared to that in EAC. Besides, in esophageal Flo-1 and OE33 cell lines treated with curcumin, NF-κB activity was suppressed, and cancer cells were induced to increase apoptosis and promoted chemosensitivity to 5-FU and cisplatin (Hartojo et al., 2010). Consistent with this result, another study demonstrated that a reduction in cell viability in esophageal Eca109 and EC9706 cell lines treated with curcumin was associated with suppression of NF-κB (Tian et al., 2012a). Rawat et al. (2012) also showed that curcumin could inhibit bile acid-induced NF-κB activity and DNA damage in esophageal OE33 cell lines by inducing apoptosis. In addition, with the inhibition of the associated Rac1-PI3K-Akt signaling complex by curcumin treatment, the migration and invasion potential of SDF-1α-induced EC cells was attenuated (Lin et al., 2014). The PI3K/Akt pathway was demonstrated to induce oncogenic effects by activating Rac1 (Qiu et al., 1995). Curcumin-induced apoptosis in breast cancer cell lines was also promoted upon the suppression of the PI3K/Akt survival pathway (Kizhakkayil et al., 2010). Moreover, Liu et al. (2018b) reported that curcumin could suppress the JAK/STAT pathway to inhibit cell growth and induce apoptosis in ESCC. The authors further revealed the effect of curcumin on ESCC xenografts and showed that there was less tumor growth in these models. The JAK/STAT3 pathway was also related to cell cancer invasion and metastasis. Curcumin could enhance cell-cell adhesion and cell-matrix adhesion and reduce oxidative stress in esophageal Eca-109 cell lines by suppressing the JAK/STAT3 pathway, which helped reduce cell migration (Zheng et al., 2018). Furthermore, Notch signaling was suggested as a novel mechanism underlying the apoptosis-inducing effect of curcumin on EC cell lines (TE-7, TE-10, and ESO-1) (Subramaniam et al., 2012). The downregulation of Notch signaling reduced cell proliferation and colony formation and decreased the number of esophagospheres. The expression of Notch-1-specific microRNAs (miR-21 and miR-34a) was downregulated and that of tumor suppressor microRNA was upregulated in response to curcumin treatment. In conclusion, curcumin is an effective anticancer agent that induces the in vitro inhibition of EC cell lines by targeting different signaling pathways.
3.2 In vivo studies
In addition to evidence from in vitro studies, evidence from in vivo studies has also demonstrated the anticancer potential of curcumin (Table 2). Ushida et al. (2000) established a rat model of EC treated with N-nitrosomethylbenzylamine (NMBA) and evaluated the incidence of EC and moderate or severe epithelial dysplasia in the different groups. The group with the dietary feeding of curcumin showed a lower rate of EC and epithelial dysplasia, suggesting the inhibitory effect of curcumin on NMBA-induced esophageal tumorigenesis and cell proliferation. In an in vivo study conducted by Tian et al. (2012b), mice were treated with curcumin, p65 siRNA, and 5-FU after being implanted with esophageal EC9706 cells. The authors found that the size and weight of the tumors were significantly reduced in the curcumin+5-FU group and p65 siRNA+5-FU group compared to that in the group treated with 5-FU alone. Curcumin and p65 siRNA can potentiate the apoptotic effect of 5-FU in tumor tissues. In addition, the findings of this in vivo study revealed that the anti-cancer effect of curcumin was also achieved through the blockade of the NF-κB pathway. Furthermore, in a small pilot trial involving 33 patients with Barrett’s esophagus, 16 patients received oral curcumin tablets, and biopsy samples were collected from all patients (Rawat et al., 2012). An increased apoptotic frequency was observed in the epithelial cells of Barrett’s tissue from the group treated with curcumin, indicating that curcumin may confer beneficial effects on patients with Barrett’s esophagus.
3.3 Clinical trials
Clinical trials have been conducted on the therapeutic effects of curcumin in different cancers. Unfortunately, no clinical trial has investigated the effectiveness of curcumin alone in EC.
A clinical randomized study assessed the anticancer effect of curcumin on CRC cells in 126 patients (He et al., 2011). Half of the patients received oral curcumin capsules at a dose of 360 mg/day before surgery, and these patients showed an increased body weight and decreased serum levels of tumor necrosis factor-α (TNF-α) in response to curcumin treatment. TNF-α is an inflammatory cytokine released by cancer cells, which contributes to malignant progression (Balkwill, 2006). Besides, a greater number of apoptotic cells, DNA fragmentation, and p53 expression were observed in CRC tissues in the curcumin-treated group compared to that in the control group. P53 acts as an apoptosis inducer and tumor suppressor by modulating apoptotic pathways (Teodoro et al., 2006). Curcumin induced cancer cell apoptosis by enhancing p53 expression. Therefore, curcumin supplementation may be safe and effective for patients with CRC. A phase II clinical trial enrolling 25 patients with advanced PC demonstrated that curcumin was well tolerated and exhibited no toxic effect when administered at 8 g/day until disease progression (Dhillon et al., 2008). The expression of some markers associated with tumor development (NF-κB, cyclooxygenase-2, and pSTAT3) was reduced in peripheral blood samples from patients compared to that in samples collected from healthy participants. In addition, limited biological activity of curcumin and its potential anticancer effect were observed in two patients. This evidence showed the potential therapeutic role of curcumin in the treatment of PC. Data from a recent prospective phase II trial suggested that patients receiving gemcitabine supplemented with the phytosome complex of curcumin for advanced PC had an overall survival of 10.2 months, which was greater than that reported in previous studies on patients treated with gemcitabine alone (Pastorelli et al., 2018). The curcumin complex can enhance the chemotherapeutic efficacy of gemcitabine in advanced PC and is safe and beneficial.
4 Improvement of the anticancer efficacy of curcumin
4.1 Analogs of curcumin
Despite the promising outcomes of curcumin against cancer, the clinical utility of curcumin is limited owing to its low solubility in water and poor absorption. New analogs are being developed by structural modification for better efficacy, which can help preserve the primary activity of curcumin and promote its stability and bioavailability. The conjugates of curcumin with some amino acids show greater solubility, promote cellular uptake, and lower metabolic degradation, which leads to stronger anticancer activity (Dubey et al., 2008). The carrier proteins of amino acids can carry additional curcumin molecules into the cell, thus enhancing the accumulation of curcumin. Alibeiki et al. (2017) synthesized 2,6-bis benzylidine cyclohexanone analogs of curcumin and investigated the anticancer mechanism of action of these analogs in GC and EC cell lines. These analogs caused the upregulation of apoptosis-related factors (Bax and caspase-3) and downregulation of anti-apoptotic factors, indicating that the analogs triggered the apoptotic pathway. Compared to curcumin, the novel synthesized analogs showed greater potency in the inhibition of cancer cell proliferation, apoptosis induction, and cell cycle arrest. In an in vitro study by Tung et al. (2018), eight newly synthesized curcumin analogs exhibited better anti-tumor effect in EC cells than curcumin. The authors further developed tumor xenograft models in mice, and analog SSC-5 was selected for the subsequent in vivo study because it had the strongest ability to induce apoptosis and cause cell cycle arrest. The tumor size and risk of tumor invasion were reduced in xenograft mice treated with SSC-5. Moreover, the anticancer effect on and molecular mechanisms of action of 2-pyridyl cyclohexanone analogs of curcumin in esophageal Eca109 and EC9706 cells were also explored (Wang et al., 2018). These analogs could inhibit cell growth and induce cell apoptosis by downregulating STAT3 phosphorylation and Bcl-2 expression.
4.2 Combination of curcumin with other drugs or therapeutic agents
A new formulation of curcumin, named Theracurmin, was demonstrated to exhibit stronger inhibitory effect on the proliferation of EC cells and the growth of xenografted tumors than curcumin, owing to its high bioavailability (Mizumoto et al., 2019). Curcumin is present in the form of colloidal submicron particles in Theracurmin. Besides, combined treatment with Theracurmin and an NAD(P)H quinone dehydrogenase (NQO1) inhibitor showed better anticancer effectiveness by regulating the NRF2-NMRAL2P-NQO1 pathway. NQO1 protected cells from oxidative stress and functioned as an antioxidant in Theracurmin -mediated cytotoxicity. NQO1 inhibitor can increase oxidative stress and enhance the sensitivity of cells to Theracurmin, thus promoting the anticancer effect. Moreover, Theracurmin was shown to inhibit the proliferation of EC cells but exerted no effect on normal esophageal cells, which indicates the safety of Theracurmin (Milano et al., 2013). Dendritic cell (DC)-based immunotherapy seems to be a promising treatment for cancer. DC loaded with tumor antigens was prepared ex vivo and delivered to patients to activate their immune system (Milano et al., 2007). The authors suggested that the combination of DC immunotherapy with Theracurmin is effective for the treatment of EC (Milano et al., 2013). Theracurmin can enhance the anticancer response of the immune system by promoting the function of DCs and T cells and sensitizing cancer cells to cytotoxicity. Statins and (-)-epigallocatechin-3-gallate (EGCG) were shown to suppress tumorigenesis in esophageal cancer (Li et al., 2002; Ogunwobi and Beales, 2008). Ye et al. (2012) applied curcumin, lovastatin, and EGCG and investigated their combined effects in EC cell lines and mouse xenografts. The authors found that cell viability and tumor growth were inhibited upon the combined treatment via the downregulation of Erk1/2, c-Jun, and cyclooxygenase-2 expression. However, possibly owing to the low water solubility of curcumin, it exerted no effect on tumor formation and growth in mice treated with the highest dose of curcumin alone. Therefore, the application of combination therapy is useful for improving the synergistic effects of drugs for better therapeutic efficacy. The interaction between curcumin and other drugs warrants additional investigation.
4.3 Application of novel nanocarriers
The use of novel delivery systems formulated using different types of biopolymers is of great interest for the application of curcumin via nanocarrier particles. Biopolymeric carriers can enhance the stability and bioavailability of biopolymer molecules through the targeted and sustained release of their components. A new nanoparticle carrier of recombinant HGFI and curcumin was designed and exhibited higher solubility and stability in aqueous solution for up to 12 h (Niu et al., 2020). Besides, the nanoparticles promoted the release of curcumin and enhanced cytotoxicity against EC cells compared to free curcumin; this effect was attributed to the amphiphilic property and self-assembly characteristics of HGFI. Hydrophobin HGFI is an amphiphilic protein, capable of forming amphipathic membranes through self-assembly at hydrophilic-hydrophobic interfaces (Szilvay et al., 2007). The authors also suggested that HGFI coating was an effective method for preparing nanoparticles. Martin et al. (2015) developed a biopolymeric carrier of a PLGA-b-PEG copolymer delivered with gold nanorods and curcumin and investigated its effect on Barrett’s esophagus and EC. The results showed a significant decrease in cell viability in Barrett’s esophagus and cancer cell lines but no significant changes in benign esophageal cell lines. In vivo investigation revealed that the destruction of the mucosa in Barrett’s esophagus was eliminated in response to the synergistic toxic effect of gold nanorods and curcumin. Gold nanorods can cause damage to cancer cells by inducing hyperthermia through the photothermal effect after laser light exposure (Chen et al., 2007). This novel biopolymeric carrier could deliver gold nanorods and curcumin to the target tumor site to exert a synergistic anticancer effect without influencing the adjacent normal tissue. Stimuli-responsive nanoparticles can control drug release by responding to the differences between normal and tumor tissues. The pH-responsive nanoparticle polymers can sense the change in the acidity of the tumor microenvironment and selectively release the contained drug at the tumor site, thus eliciting effective anticancer activity (Xie et al., 2018). Similarly, Deng et al. (2020) designed a pH-responsive nanoparticle carrier that improved the anticancer effects of docetaxel and curcumin in EC. In addition, this nanoparticle carrier was modified with a T7 peptide and promoted the permeability and retention of curcumin and increased the cellular uptake efficiency.
Nanoparticle structure modification constitutes a new direction in this field. For instance, cell membrane wrapping is a new strategy for the modification of nanoparticles that exhibit different properties owing to the presence of different types of cell membranes. Nanoparticles wrapped with the tumor cell membrane can achieve the homologous recognition of tumor cells and are more precisely targeted to tumors (Zhen et al., 2019). MDR in tumors is a major obstacle to clinical chemotherapy and severely affects the effectiveness of chemotherapy. MDR is characterized by the resistance of tumor cells to multiple drugs with different structures and mechanisms of action, which makes it difficult for anticancer drugs to reach effective intracellular concentrations. Gao et al. (2021) used esophageal TE10 cell membrane to encapsulate PLGA-nanoparticles loaded with curcumin and doxorubicin and investigated the therapeutic efficacy of this combination in the treatment of drug-resistant EC. TE10 cell lines were established to elicit resistance to doxorubicin and were then used in a mouse model. The results confirmed that this new nanoparticle carrier showed controlled drug release, targeted intracellular uptake, and high biological safety, thus achieving efficient drug delivery and enhancing the anticancer efficacy in MDR cancer cells.
Different types and structures of biopolymers can affect the stability and bioavailability of curcumin, leading to varied drug efficacy. The designing of new nanoparticle carriers of curcumin combined with multiple drugs integrating the functions of controlled drug release and targeted action on tumor location can broaden the scope of curcumin application in tumor treatment. Furthermore, the modification of the nanoparticle structure and encapsulation of curcumin also show promise in clinical application.
5 Discussion
The anticancer effect of phytochemicals such as curcumin has been demonstrated in the treatment of various cancers, especially in cancers of the gastrointestinal system. The esophagus, which is connected to the pharynx and the cardia of the stomach, is the necessary passage for food digestion and absorption. When various conditions and diseases develop in the esophagus, the patient experiences severe pain, and treatment is complicated because of its physiological location, structure, and functions. Additionally, the incidence of EC and precancerous conditions such as Barrett’s esophagus is increasing, which poses a major threat to human health. Owing to the low bioavailability of orally administered curcumin, EC seems to be more suitable for curcumin treatment than other non-gastrointestinal cancers. In fact, the application of curcumin has promising outcomes in the treatment of EC.
The specific anticancer mechanism of action of curcumin is not fully understood. Curcumin inhibits tumor cell growth, migration, invasion, and angiogenesis and promotes apoptosis through the regulation of various cellular molecules, growth factors, miRNAs, and genes involved in different signaling pathways. Besides, curcumin can sensitize chemoresistant and radioresistant cancer cells, reversing their resistance and making them more susceptible to inhibition or death. Curcumin exhibits both direct and indirect anti-EC activity in different cell lines and animal models, which indicates its potential as a phytochemical agent in the prevention and treatment of EC. To better increase the bioavailability and efficacy of curcumin, strategies such as the modification of curcumin analogs, combination with other drugs or therapies, and use of novel nanocarriers have been explored extensively.
The effect and bioavailability of curcumin is determined by its treatment period, concentration, and the type of cells. A limitation in the application of curcumin is its low bioavailability. The synthesis of new curcumin analogs has recently garnered significant interest in research. The new analogs not only retain the original properties of curcumin but also enhance its potency and stability owing to the modification of different side chain groups.
Radiotherapy and chemotherapy are important modalities in the treatment of EC, whereas resistant cancer cells may contribute to the failure of treatment. The combination of traditional chemical agents or radiation with natural phytochemicals is an important strategy in this field, where curcumin exhibits superior sensitizing effects. The sensitizing potential of curcumin can be used to achieve superior therapeutic effects and reduce the adverse effects of treatment by aiding the minimization of the dose of chemotherapeutic drugs or radiation. Besides, curcumin in combination with other anticancer agents may improve the synergistic effect of drugs and enhance their efficacy.
Nanocarriers can be efficiently loaded with multiple drugs simultaneously, and their surfaces can be functionally modified. Different modifications can lead to a longer in vivo circulation time of the carrier, lower drug toxicity, and greater bioavailability of the drug. The nanocarrier surface can also be linked to tumor-specific ligands to enhance the targeting of drugs and reduce the damage to normal tissues.
The above findings give us a glimpse of the benefits of curcumin in the treatment of cancer, or EC in particular. Although curcumin is safe, it is unknown whether its long-term use can cause any side effects. However, the anticancer effects of curcumin have been observed more frequently in cellular and animal models, and more convincing clinical trials need to be conducted to confirm its effectiveness. For these results to be used in clinical antitumor therapy, further studies on the effects, mechanisms, absorption, and metabolic characteristics of curcumin and its analogs, combined therapies, and new nanocarrier forms in humans are required. Therefore, we have reason to believe that curcumin, an ancient phytochemical, will play a prominent role in the prevention and treatment of EC in the future.
Author contributions
SW drafted the review. XG, JL, SW, YS, and YY generated the graph and guided the construction of the manuscript. DZ and MT edited the review. All the authors contributed to the article and approved the submitted version.
Acknowledgments
We thank KetengEdit (www.ketengedit.com) for linguistic assistance during the preparation of this study.
Conflict of interest
The authors declare that the research was conducted in the absence of any commercial or financial relationships that could be construed as a potential conflict of interest.
Publisher’s note
All claims expressed in this article are solely those of the authors and do not necessarily represent those of their affiliated organizations, or those of the publisher, the editors and the reviewers. Any product that may be evaluated in this article, or claim that may be made by its manufacturer, is not guaranteed or endorsed by the publisher.
References
Alibeiki, F., Jafari, N., Karimi, M., and Peeri Dogaheh, H. (2017). Potent anti-cancer effects of less polar curcumin analogues on gastric adenocarcinoma and esophageal squamous cell carcinoma cells. Sci. Rep. 7 (1), 2559. Epub 2017/06/02. doi:10.1038/s41598-017-02666-4
Allegra, A., Innao, V., Russo, S., Gerace, D., Alonci, A., and Musolino, C. (2017). Anticancer activity of curcumin and its analogues: Preclinical and clinical studies. Cancer Invest. 35 (1), 1–22. Epub 2016/12/21. doi:10.1080/07357907.2016.1247166
Balkwill, F. (2006). Tnf-alpha in promotion and progression of cancer. Cancer Metastasis Rev. 25 (3), 409–416. Epub 2006/09/05. doi:10.1007/s10555-006-9005-3
Bartel, D. P. (2004). Micrornas: Genomics, biogenesis, mechanism, and function. Cell 116 (2), 281–297. Epub 2004/01/28. doi:10.1016/s0092-8674(04)00045-5
Bergers, G., and Fendt, S. M. (2021). The metabolism of cancer cells during metastasis. Nat. Rev. Cancer 21 (3), 162–180. Epub 2021/01/20. doi:10.1038/s41568-020-00320-2
Blot, W., and Tarone, R. (2018). “Esophageal cancer,” in Schottenfeld and fraumeni cancer epidemiology and prevention. Editors Mj Thun, Linet Ms, Cerhan, C. Haiman, and D. Schottenfeld (New York, NY: Oxford University Press).
Boice, A., and Bouchier-Hayes, L. (2020). Targeting apoptotic caspases in cancer. Biochim. Biophys. Acta. Mol. Cell Res. 1867 (6), 118688. Epub 2020/02/23. doi:10.1016/j.bbamcr.2020.118688
Chen, J., Wang, D., Xi, J., Au, L., Siekkinen, A., Warsen, A., et al. (2007). Immuno gold nanocages with tailored optical properties for targeted photothermal destruction of cancer cells. Nano Lett. 7 (5), 1318–1322. Epub 2007/04/14. doi:10.1021/nl070345g
Cheng, A. L., Hsu, C. H., Lin, J. K., Hsu, M. M., Ho, Y. F., Shen, T. S., et al. (2001). Phase I clinical trial of curcumin, a chemopreventive agent, in patients with high-risk or pre-malignant lesions. Anticancer Res. 21 (4), 2895–2900. Epub 2001/11/20.
Clermont, M., and Falk, G. W. (2018). Clinical guidelines update on the diagnosis and management of Barrett's esophagus. Dig. Dis. Sci. 63 (8), 2122–2128. Epub 2018/04/20. doi:10.1007/s10620-018-5070-z
Deng, L., Zhu, X., Yu, Z., Li, Y., Qin, L., Liu, Z., et al. (2020). Novel T7-modified ph-responsive targeted nanosystem for Co-delivery of docetaxel and curcumin in the treatment of esophageal cancer. Int. J. Nanomedicine 15, 7745–7762. Epub 2020/10/30. doi:10.2147/ijn.s257312
Dhillon, N., Aggarwal, B. B., Newman, R. A., Wolff, R. A., Kunnumakkara, A. B., Abbruzzese, J. L., et al. (2008). Phase ii trial of curcumin in patients with advanced pancreatic cancer. Clin. Cancer Res. 14 (14), 4491–4499. Epub 2008/07/17. doi:10.1158/1078-0432.ccr-08-0024
Dolcet, X., Llobet, D., Pallares, J., and Matias-Guiu, X. (2005). Nf-kb in development and progression of human cancer. Virchows Arch. 446 (5), 475–482. Epub 2005/04/28. doi:10.1007/s00428-005-1264-9
Dubey, S. K., Sharma, A. K., Narain, U., Misra, K., and Pati, U. (2008). Design, synthesis and characterization of some bioactive conjugates of curcumin with Glycine, glutamic acid, valine and demethylenated piperic acid and study of their antimicrobial and antiproliferative properties. Eur. J. Med. Chem. 43 (9), 1837–1846. Epub 2008/01/19. doi:10.1016/j.ejmech.2007.11.027
Folkman, J. (2002). Role of angiogenesis in tumor growth and metastasis. Semin. Oncol. 29 (6), 15–18. Epub 2003/01/08. doi:10.1053/sonc.2002.37263
Gandhy, S. U., Kim, K., Larsen, L., Rosengren, R. J., and Safe, S. (2012). Curcumin and synthetic analogs induce reactive oxygen species and decreases specificity protein (sp) transcription factors by targeting micrornas. BMC Cancer 12, 564. Epub 2012/12/01. doi:10.1186/1471-2407-12-564
Gao, Y., Zhu, Y., Xu, X., Wang, F., Shen, W., Leng, X., et al. (2021). Surface pegylated cancer cell membrane-coated nanoparticles for codelivery of curcumin and doxorubicin for the treatment of multidrug resistant esophageal carcinoma. Front. Cell Dev. Biol. 9, 688070. Epub 2021/08/14. doi:10.3389/fcell.2021.688070
Gupta, S. C., Kismali, G., and Aggarwal, B. B. (2013). Curcumin, a component of turmeric: From farm to pharmacy. Biofactors 39 (1), 2–13. Epub 2013/01/23. doi:10.1002/biof.1079
Hartojo, W., Silvers, A. L., Thomas, D. G., Seder, C. W., Lin, L., Rao, H., et al. (2010). Curcumin promotes apoptosis, increases chemosensitivity, and inhibits nuclear factor kappab in esophageal adenocarcinoma. Transl. Oncol. 3 (2), 99–108. Epub 2010/04/03. doi:10.1593/tlo.09235
He, Z. Y., Shi, C. B., Wen, H., Li, F. L., Wang, B. L., and Wang, J. (2011). Upregulation of P53 expression in patients with colorectal cancer by administration of curcumin. Cancer Invest. 29 (3), 208–213. Epub 2011/02/15. doi:10.3109/07357907.2010.550592
Hirohashi, S., and Kanai, Y. (2003). Cell adhesion system and human cancer morphogenesis. Cancer Sci. 94 (7), 575–581. Epub 2003/07/05. doi:10.1111/j.1349-7006.2003.tb01485.x
Huang, T., Song, X., Xu, D., Tiek, D., Goenka, A., Wu, B., et al. (2020). Stem cell programs in cancer initiation, progression, and therapy resistance. Theranostics 10 (19), 8721–8743. Epub 2020/08/06. doi:10.7150/thno.41648
Hung, C. S., Liu, H. H., Liu, J. J., Yeh, C. T., Chang, T. C., Wu, C. H., et al. (2013). Microrna-200a and -200b mediated hepatocellular carcinoma cell migration through the epithelial to mesenchymal transition markers. Ann. Surg. Oncol. 20 (3), S360–S368. Epub 2012/08/08. doi:10.1245/s10434-012-2482-4
Kizhakkayil, J., Thayyullathil, F., Chathoth, S., Hago, A., Patel, M., and Galadari, S. (2010). Modulation of curcumin-induced Akt phosphorylation and apoptosis by Pi3k inhibitor in mcf-7 cells. Biochem. Biophys. Res. Commun. 394 (3), 476–481. Epub 2010/02/09. doi:10.1016/j.bbrc.2010.01.132
Kunnumakkara, A. B., Diagaradjane, P., Guha, S., Deorukhkar, A., Shentu, S., Aggarwal, B. B., et al. (2008). Curcumin sensitizes human colorectal cancer xenografts in nude mice to gamma-radiation by targeting nuclear factor-kappab-regulated gene products. Clin. Cancer Res. 14 (7), 2128–2136. Epub 2008/04/03. doi:10.1158/1078-0432.ccr-07-4722
Li, W., Miao, S., Miao, M., Li, R., Cao, X., Zhang, K., et al. (2016). Hedgehog signaling activation in hepatic stellate cells promotes angiogenesis and vascular mimicry in hepatocellular carcinoma. Cancer Invest. 34 (9), 424–430. Epub 2016/10/22. doi:10.1080/07357907.2016.1227442
Li, Y., Revalde, J. L., Reid, G., and Paxton, J. W. (2011). Modulatory effects of curcumin on multi-drug resistance-associated protein 5 in pancreatic cancer cells. Cancer Chemother. Pharmacol. 68 (3), 603–610. Epub 2010/12/01. doi:10.1007/s00280-010-1515-6
Li, Z. G., Shimada, Y., Sato, F., Maeda, M., Itami, A., Kaganoi, J., et al. (2002). Inhibitory effects of epigallocatechin-3-gallate on N-Nitrosomethylbenzylamine-Induced esophageal tumorigenesis in F344 rats. Int. J. Oncol. 21 (6), 1275–1283. Epub 2002/11/14. doi:10.3892/ijo.21.6.1275
Liang, H. H., Wei, P. L., Hung, C. S., Wu, C. T., Wang, W., Huang, M. T., et al. (2013). Microrna-200a/B influenced the therapeutic effects of curcumin in hepatocellular carcinoma (hcc) cells. Tumour Biol. 34 (5), 3209–3218. Epub 2013/06/14. doi:10.1007/s13277-013-0891-z
Lin, M. L., Lu, Y. C., Chen, H. Y., Lee, C. C., Chung, J. G., and Chen, S. S. (2014). Suppressing the formation of lipid raft-associated rac1/pi3k/akt signaling complexes by curcumin inhibits sdf-1α-induced invasion of human esophageal carcinoma cells. Mol. Carcinog. 53 (5), 360–379. Epub 2012/11/30. doi:10.1002/mc.21984
Liu, G., Wang, Y., and Li, M. (2018). Curcumin sensitized the antitumour effects of irradiation in promoting apoptosis of oesophageal squamous-cell carcinoma through nf-?b signalling pathway. J. Pharm. Pharmacol. 70 (10), 1340–1348. Epub 2018/07/20. doi:10.1111/jphp.12981
Liu, Y., Wang, X., Zeng, S., Zhang, X., Zhao, J., Zhang, X., et al. (2018). The natural polyphenol curcumin induces apoptosis by suppressing Stat3 signaling in esophageal squamous cell carcinoma. J. Exp. Clin. Cancer Res. 37 (1), 303. Epub 2018/12/07. doi:10.1186/s13046-018-0959-0
Martin, R. C., Locatelli, E., Li, Y., Zhang, W., Li, S., Monaco, I., et al. (2015). Gold nanorods and curcumin-loaded nanomicelles for efficient in vivo photothermal therapy of Barrett's esophagus. Nanomedicine Lond. Engl. 10 (11), 1723–1733. Epub 2015/02/24. doi:10.2217/nnm.15.25
Menon, V. P., and Sudheer, A. R. (2007). Antioxidant and anti-inflammatory properties of curcumin. Adv. Exp. Med. Biol. 595, 105–125. Epub 2007/06/16. doi:10.1007/978-0-387-46401-5_3
Milano, F., Mari, L., van de Luijtgaarden, W., Parikh, K., Calpe, S., and Krishnadath, K. K. (2013). Nano-curcumin inhibits proliferation of esophageal adenocarcinoma cells and enhances the T cell mediated immune response. Front. Oncol. 3, 137. Epub 2013/06/12. doi:10.3389/fonc.2013.00137
Milano, F., Rygiel, A. M., Buttar, N., Bergman, J. J. G. H. M., Sondermeijer, C., van Baal, J. W. P. M., et al. (2007). An ex vivo readout for evaluation of dendritic cell-induced autologous cytotoxic T lymphocyte responses against esophageal cancer. Cancer Immunol. Immunother. 56 (12), 1967–1977. doi:10.1007/s00262-007-0341-0
Mizumoto, A., Ohashi, S., Kamada, M., Saito, T., Nakai, Y., Baba, K., et al. (2019). Combination treatment with highly bioavailable curcumin and Nqo1 inhibitor exhibits potent antitumor effects on esophageal squamous cell carcinoma. J. Gastroenterol. 54 (8), 687–698. Epub 2019/02/10. doi:10.1007/s00535-019-01549-x
Morin, D., Barthélémy, S., Zini, R., Labidalle, S., and Tillement, J. P. (2001). Curcumin induces the mitochondrial permeability transition pore mediated by membrane protein thiol oxidation. FEBS Lett. 495 (1-2), 131–136. Epub 2001/04/27. doi:10.1016/s0014-5793(01)02376-6
Nagaraju, G. P., Zhu, S., Ko, J. E., Ashritha, N., Kandimalla, R., Snyder, J. P., et al. (2015). Antiangiogenic effects of a novel synthetic curcumin analogue in pancreatic cancer. Cancer Lett. 357 (2), 557–565. Epub 2014/12/17. doi:10.1016/j.canlet.2014.12.007
Niu, B., Li, M., Jia, J., Zhang, C., Fan, Y. Y., and Li, W. (2020). Hydrophobin-enhanced stability, dispersions and release of curcumin nanoparticles in water. J. Biomater. Sci. Polym. Ed. 31 (14), 1793–1805. Epub 2020/06/09. doi:10.1080/09205063.2020.1775761
O'Sullivan-Coyne, G., O'Sullivan, G. C., O'Donovan, T. R., Piwocka, K., and McKenna, S. L. (2009). Curcumin induces apoptosis-independent death in oesophageal cancer cells. Br. J. Cancer 101 (9), 1585–1595. Epub 2009/10/08. doi:10.1038/sj.bjc.6605308
Ogunwobi, O. O., and Beales, I. L. (2008). Statins inhibit proliferation and induce apoptosis in Barrett's esophageal adenocarcinoma cells. Am. J. Gastroenterol. 103 (4), 825–837. Epub 2008/03/29. doi:10.1111/j.1572-0241.2007.01773.x
Pastorelli, D., Fabricio, A. S. C., Giovanis, P., D'Ippolito, S., Fiduccia, P., Soldà, C., et al. (2018). Phytosome complex of curcumin as complementary therapy of advanced pancreatic cancer improves safety and efficacy of gemcitabine: Results of a prospective phase ii trial. Pharmacol. Res. 132, 72–79. Epub 2018/04/04. doi:10.1016/j.phrs.2018.03.013
Perl, A. K., Wilgenbus, P., Dahl, U., Semb, H., and Christofori, G. (1998). A causal role for E-cadherin in the transition from adenoma to carcinoma. Nature 392 (6672), 190–193. Epub 1998/03/27. doi:10.1038/32433
Pistritto, G., Trisciuoglio, D., Ceci, C., Garufi, A., and D'Orazi, G. (2016). Apoptosis as anticancer mechanism: Function and dysfunction of its modulators and targeted therapeutic strategies. Aging (Albany NY) 8 (4), 603–619. Epub 2016/03/29. doi:10.18632/aging.100934
Qiang, Z., Meng, L., Yi, C., Yu, L., Chen, W., and Sha, W. (2019). Curcumin regulates the mir-21/pten/akt pathway and acts in synergy with Pd98059 to induce apoptosis of human gastric cancer mgc-803 cells. J. Int. Med. Res. 47 (3), 1288–1297. Epub 2019/02/08. doi:10.1177/0300060518822213
Qiu, R. G., Chen, J., Kirn, D., McCormick, F., and Symons, M. (1995). An essential role for rac in ras transformation. Nature 374 (6521), 457–459. Epub 1995/03/30. doi:10.1038/374457a0
Rajitha, B., Nagaraju, G. P., Shaib, W. L., Alese, O. B., Snyder, J. P., Shoji, M., et al. (2017). Novel synthetic curcumin analogs as potent antiangiogenic agents in colorectal cancer. Mol. Carcinog. 56 (1), 288–299. Epub 2016/04/30. doi:10.1002/mc.22492
Rawat, N., Alhamdani, A., McAdam, E., Cronin, J., Eltahir, Z., Lewis, P., et al. (2012). Curcumin abrogates bile-induced nf-?b activity and DNA damage in vitro and suppresses nf-?b activity whilst promoting apoptosis in vivo, suggesting chemopreventative potential in Barrett's oesophagus. Clin. Transl. Oncol. 14 (4), 302–311. Epub 2012/04/10. doi:10.1007/s12094-012-0799-x
Ray, S., Chattopadhyay, N., Mitra, A., Siddiqi, M., and Chatterjee, A. (2003). Curcumin exhibits antimetastatic properties by modulating integrin receptors, collagenase activity, and expression of Nm23 and E-cadherin. J. Environ. Pathol. Toxicol. Oncol. 22 (1), 47–56. Epub 2003/04/08. doi:10.1615/jenvpathtoxoncol.v22.i1.50
Seyfried, T. N., and Huysentruyt, L. C. (2013). On the origin of cancer metastasis. Crit. Rev. Oncog. 18 (1-2), 43–73. Epub 2012/12/15. doi:10.1615/critrevoncog.v18.i1-2.40
Shakibaei, M., Buhrmann, C., Kraehe, P., Shayan, P., Lueders, C., and Goel, A. (2014). Curcumin chemosensitizes 5-fluorouracil resistant mmr-deficient human colon cancer cells in high density cultures. PloS one 9 (1), e85397. Epub 2014/01/10. doi:10.1371/journal.pone.0085397
Shao, S., Duan, W., Xu, Q., Li, X., Han, L., Li, W., et al. (2019). Curcumin suppresses hepatic stellate cell-induced hepatocarcinoma angiogenesis and invasion through downregulating ctgf. Oxid. Med. Cell. Longev. 2019, 8148510. Epub 2019/02/26. doi:10.1155/2019/8148510
Shapiro, J., van Lanschot Jjb, , Hulshof, M., van Hagen, P., van Berge Henegouwen, M. I., Wijnhoven, B. P. L., et al. (2015). Neoadjuvant chemoradiotherapy plus surgery versus surgery alone for oesophageal or junctional cancer (cross): Long-term results of a randomised controlled trial. Lancet. Oncol. 16 (9), 1090–1098. Epub 2015/08/10. doi:10.1016/s1470-2045(15)00040-6
Shehzad, A., Park, J. W., Lee, J., and Lee, Y. S. (2013). Curcumin induces radiosensitivity of in vitro and in vivo cancer models by modulating pre-mrna processing factor 4 (Prp4). Chem. Biol. Interact. 206 (2), 394–402. Epub 2013/10/23. doi:10.1016/j.cbi.2013.10.007
Sjoquist, K. M., Burmeister, B. H., Smithers, B. M., Zalcberg, J. R., Simes, R. J., Barbour, A., et al. (2011). Survival after neoadjuvant chemotherapy or chemoradiotherapy for resectable oesophageal carcinoma: An updated meta-analysis. Lancet. Oncol. 12 (7), 681–692. Epub 2011/06/21. doi:10.1016/s1470-2045(11)70142-5
Subramaniam, D., Ponnurangam, S., Ramamoorthy, P., Standing, D., Battafarano, R. J., Anant, S., et al. (2012). Curcumin induces cell death in esophageal cancer cells through modulating Notch signaling. PloS one 7 (2), e30590. Epub 2012/03/01. doi:10.1371/journal.pone.0030590
Sung, H., Ferlay, J., Siegel, R. L., Laversanne, M., Soerjomataram, I., Jemal, A., et al. (2021). Global cancer statistics 2020: Globocan estimates of incidence and mortality worldwide for 36 cancers in 185 countries. Ca. Cancer J. Clin. 71 (3), 209–249. Epub 2021/02/05. doi:10.3322/caac.21660
Szilvay, G. R., Paananen, A., Laurikainen, K., Vuorimaa, E., Lemmetyinen, H., Peltonen, J., et al. (2007). Self-assembled hydrophobin protein films at the air-water interface: Structural analysis and molecular engineering. Biochemistry 46 (9), 2345–2354. Epub 2007/02/15. doi:10.1021/bi602358h
Tang, X. Q., Bi, H., Feng, J. Q., and Cao, J. G. (2005). Effect of curcumin on multidrug resistance in resistant human gastric carcinoma cell line sgc7901/vcr. Acta Pharmacol. Sin. 26 (8), 1009–1016. Epub 2005/07/26. doi:10.1111/j.1745-7254.2005.00149.x
Teodoro, J. G., Parker, A. E., Zhu, X., and Green, M. R. (2006). P53-Mediated inhibition of angiogenesis through up-regulation of a collagen prolyl hydroxylase. Science 313 (5789), 968–971. Epub 2006/08/19. doi:10.1126/science.1126391
Tian, F., Fan, T., Zhang, Y., Jiang, Y., and Zhang, X. (2012). Curcumin potentiates the antitumor effects of 5-fu in treatment of esophageal squamous carcinoma cells through downregulating the activation of nf-?b signaling pathway in vitro and in vivo. Acta Biochim. Biophys. Sin. 44 (10), 847–855. Epub 2012/09/29. doi:10.1093/abbs/gms074
Tian, F., Zhang, C., Tian, W., Jiang, Y., and Zhang, X. (2012). Comparison of the effect of P65 sirna and curcumin in promoting apoptosis in esophageal squamous cell carcinoma cells and in nude mice. Oncol. Rep. 28 (1), 232–240. Epub 2012/05/04. doi:10.3892/or.2012.1777
Toden, S., Okugawa, Y., Jascur, T., Wodarz, D., Komarova, N. L., Buhrmann, C., et al. (2015). Curcumin mediates chemosensitization to 5-fluorouracil through mirna-induced suppression of epithelial-to-mesenchymal transition in chemoresistant colorectal cancer. Carcinogenesis 36 (3), 355–367. Epub 2015/02/06. doi:10.1093/carcin/bgv006
Tomeh, M. A., Hadianamrei, R., and Zhao, X. (2019). A review of curcumin and its derivatives as anticancer agents. Int. J. Mol. Sci. 20 (5), E1033. Epub 2019/03/02. doi:10.3390/ijms20051033
Tung, L. N., Song, S., Chan, K. T., Choi, M. Y., Lam, H. Y., Chan, C. M., et al. (2018). Preclinical study of novel curcumin analogue ssc-5 using orthotopic tumor xenograft model for esophageal squamous cell carcinoma. Cancer Res. Treat. 50 (4), 1362–1377. Epub 2018/01/25. doi:10.4143/crt.2017.353
Uhlenhopp, D. J., Then, E. O., Sunkara, T., and Gaduputi, V. (2020). Epidemiology of esophageal cancer: Update in global trends, etiology and risk factors. Clin. J. Gastroenterol. 13 (6), 1010–1021. Epub 2020/09/24. doi:10.1007/s12328-020-01237-x
Ushida, J., Sugie, S., Kawabata, K., Pham, Q. V., Tanaka, T., Fujii, K., et al. (2000). Chemopreventive effect of curcumin on N-Nitrosomethylbenzylamine-Induced esophageal carcinogenesis in rats. Jpn. J. Cancer Res. 91 (9), 893–898. Epub 2000/09/30. doi:10.1111/j.1349-7006.2000.tb01031.x
Wang, T., Wu, X., Al Rudaisat, M., Song, Y., and Cheng, H. (2020). Curcumin induces G2/M arrest and triggers autophagy, ros generation and cell senescence in cervical cancer cells. J. Cancer 11 (22), 6704–6715. Epub 2020/10/14. doi:10.7150/jca.45176
Wang, Y., Zhou, P., Qin, S., Xu, D., Liu, Y., Fu, W., et al. (2018). The curcumin analogs 2-pyridyl cyclohexanone induce apoptosis via inhibition of the jak2-stat3 pathway in human esophageal squamous cell carcinoma cells. Front. Pharmacol. 9, 820. Epub 2018/09/07. doi:10.3389/fphar.2018.00820
Wroński, P., Wroński, S., Kurant, M., Malinowski, B., and Wiciński, M. (2021). Curcumin may prevent basement membrane disassembly by matrix metalloproteinases and progression of the bladder cancer. Nutrients 14 (1), 32. Epub 2022/01/12. doi:10.3390/nu14010032
Xie, J., Fan, Z., Li, Y., Zhang, Y., Yu, F., Su, G., et al. (2018). Design of ph-sensitive methotrexate prodrug-targeted curcumin nanoparticles for efficient dual-drug delivery and combination cancer therapy. Int. J. Nanomedicine 13, 1381–1398. Epub 2018/03/23. doi:10.2147/ijn.s152312
Yang, J., and Weinberg, R. A. (2008). Epithelial-mesenchymal transition: At the crossroads of development and tumor metastasis. Dev. Cell 14 (6), 818–829. Epub 2008/06/10. doi:10.1016/j.devcel.2008.05.009
Ye, F., Zhang, G. H., Guan, B. X., and Xu, X. C. (2012). Suppression of esophageal cancer cell growth using curcumin, (-)-Epigallocatechin-3-Gallate and lovastatin. World J. Gastroenterol. 18 (2), 126–135. Epub 2012/01/19. doi:10.3748/wjg.v18.i2.126
Zhang, B. G., Li, J. F., Yu, B. Q., Zhu, Z. G., Liu, B. Y., and Yan, M. (2012). Microrna-21 promotes tumor proliferation and invasion in gastric cancer by targeting pten. Oncol. Rep. 27 (4), 1019–1026. Epub 2012/01/24. doi:10.3892/or.2012.1645
Zhang, F. J., Zhang, H. S., Liu, Y., and Huang, Y. H. (2015). Curcumin inhibits Ec109 cell growth via an ampk-mediated metabolic switch. Life Sci. 134, 49–55. Epub 2015/06/04. doi:10.1016/j.lfs.2015.05.016
Zhang, L., Cheng, X., Xu, S., Bao, J., and Yu, H. (2018). Curcumin induces endoplasmic reticulum stress-associated apoptosis in human papillary thyroid carcinoma bcpap cells via disruption of intracellular calcium homeostasis. Med. Baltim. 97 (24), e11095. Epub 2018/06/15. doi:10.1097/md.0000000000011095
Zhen, X., Cheng, P., and Pu, K. (2019). Recent advances in cell membrane-camouflaged nanoparticles for cancer phototherapy. Small (Weinheim der Bergstrasse, Ger. 15 (1), e1804105. Epub 2018/11/21. doi:10.1002/smll.201804105
Keywords: curcumin, esophageal cancer, anticancer effect, bioavailability, gastrointenstinal
Citation: Wang S, Gao X, Li J, Wei S, Shao Y, Yin Y, Zhang D and Tang M (2022) The anticancer effects of curcumin and clinical research progress on its effects on esophageal cancer. Front. Pharmacol. 13:1058070. doi: 10.3389/fphar.2022.1058070
Received: 30 September 2022; Accepted: 17 October 2022;
Published: 28 October 2022.
Edited by:
Yulin Dai, Changchun University of Chinese Medicine, ChinaReviewed by:
Menghua Xue, Tangdu Hospital, ChinaMingbo Wang, Fourth Hospital of Hebei Medical University, China
Copyright © 2022 Wang, Gao, Li, Wei, Shao, Yin, Zhang and Tang. This is an open-access article distributed under the terms of the Creative Commons Attribution License (CC BY). The use, distribution or reproduction in other forums is permitted, provided the original author(s) and the copyright owner(s) are credited and that the original publication in this journal is cited, in accordance with accepted academic practice. No use, distribution or reproduction is permitted which does not comply with these terms.
*Correspondence: Duo Zhang, emhhbmdkdW9Aamx1LmVkdS5jbg==; Mingbo Tang, bWJ0YW5nQGpsdS5lZHUuY24=