- 1Key Laboratory of Basic Pharmacology of Ministry of Education and Joint International Research Laboratory of Ethnomedicine of Ministry of Education, Zunyi Medical University, Zunyi, China
- 2Key Laboratory of Basic Pharmacology of Guizhou Province and School of Pharmacy, Department of Pharmacology, Zunyi Medical University, Zunyi, China
Cardiovascular diseases (CVD) are a group of cardiac and vascular disorders including myocardial ischemia, congenital heart disease, heart failure, hypertension, atherosclerosis, peripheral artery disease, rheumatic heart disease, and cardiomyopathies. Despite considerable progress in prophylaxis and treatment options, CVDs remain a leading cause of morbidity and mortality and impose an extremely high socioeconomic burden. Oxidative stress (OS) caused by disequilibrium in the generation of reactive oxygen species plays a crucial role in the pathophysiology of CVDs. Nuclear erythroid 2-related factor 2 (Nrf2), a transcription factor of endogenous antioxidant defense systems against OS, is considered an ideal therapeutic target for management of CVDs. Increasingly, natural products have emerged as a potential source of Nrf2 activators with cardioprotective properties and may therefore provide a novel therapeutic tool for CVD. Here, we present an updated comprehensive summary of naturally occurring products with cardioprotective properties that exert their effects by suppression of OS through activation of Nrf2 signaling, with the aim of providing useful insights for the development of therapeutic strategies exploiting natural products.
Introduction
Cardiovascular diseases (CVD) are a group of cardiac and vascular disorders including hypertension (HTN), atherosclerosis (AS), myocardial ischemia (MI), heart failure (HF), peripheral artery disease, rheumatic heart disease, and cardiomyopathies (Kolluru et al., 2022). Despite considerable progress in the prophylaxis and treatment of CVDs, associated morbidity and mortality rates remain extremely high, accounting for >32% of all global deaths and presenting a significant socioeconomic burden (Tsao et al., 2022). Importantly, with an aging society worldwide (Liberale et al., 2022) and the recent outbreak of coronavirus disease 2019 (COVID-19), the risk of CVDs continues to increase (Y. Xie et al., 2022), resulting in persistently high incidence and mortality rates. In view of the lack of efficacious therapeutic regimens, the development of novel curative agents to combat CVDs remains an urgent clinical need (X. Li et al., 2021).
While the precise underlying mechanisms are yet to be established, emerging evidence strongly indicates that dysregulation of the redox equilibrium is a hallmark of cardiovascular disease (Xu et al., 2021; Krzemińska et al., 2022). Dysregulation of the redox equilibrium is generally triggered by oxidative stress (OS), defined as a shift in the equilibrium between generation of reactive oxygen species (ROS, such as hydrogen peroxide [H2O2] and superoxide [O2.-]) or reactive nitrogen species (RNS, such as nitric oxide [NO.]) and their elimination by antioxidants, thereby leading to cell injury (Griendling et al., 2021). ROS/RNS act as a double-edged sword during physiological and pathological processes. Under physiological conditions, ROS/RNS are involved in cellular signal transduction while in the presence of excess ROS/RNS, pathologies leading to CVD are triggered (Panda et al., 2022). Thus, comprehensive understanding of the mechanisms underlying CVDs is essential for the development of novel and efficient therapeutic options for prophylaxis and treatment.
Intriguingly, accumulating evidence suggests that cells generally offset the negative effects of ROS/RNS via activation of nuclear factor E2-related factor 2 (Nrf2; gene name Nfe2l2) and impaired activation of Nrf2 is associated with CVD progression (Gutiérrez-Cuevas et al., 2022). Nrf2 is a “Cap’n’Collar” transcription factor with a b-ZIP domain, which governs multiple genes encoding proteins with cytoprotective effects, such as nicotinamide adenine dinucleotide phosphate (NADPH) quinone dehydrogenase 1 (NQO1), heme oxygenase 1 (HO-1), isocitrate dehydrogenase 1 (IDH1), and glutathione (GSH) (Zhu et al., 2022). Under physiological conditions, Nrf2 is controlled by the negative regulator Kelch-like ECH-associated protein 1 (Keap1) and undergoes successive ubiquitination and proteasomal degradation (Torrente & DeNicola, 2022). However, in response to OS, Keap1 is oxidized and inactivated, promoting Nrf2 translocation from the cytoplasm to nucleus where it binds to the antioxidant response element (ARE) as a heterodimer and facilitates transcription of target genes (e.g., HO-1, NQO1, glutathione peroxidase [GSH-Px], superoxide dismutase [SOD], catalase [CAT]) (Huang et al., 2020). Bach-1 and sMaf proteins are dissociated, promoting dimerization of Nrf2 with sMaf and gene transcription (Q. Zhang L. M. et al., 2021) (Figure 1). Therefore, Nrf2 presents a potential target for the development of novel candidate agents that confer protective effects against CVDs through suppression of OS. Bioactive phytochemicals and other natural products are extensively used to prevent and treat multiple disorders owing to their high efficacy and safety profiles. Several natural products have achieved encouraging outcomes for CVDs and may serve as valuable resources for novel drug discovery (D. Yao et al., 2022).
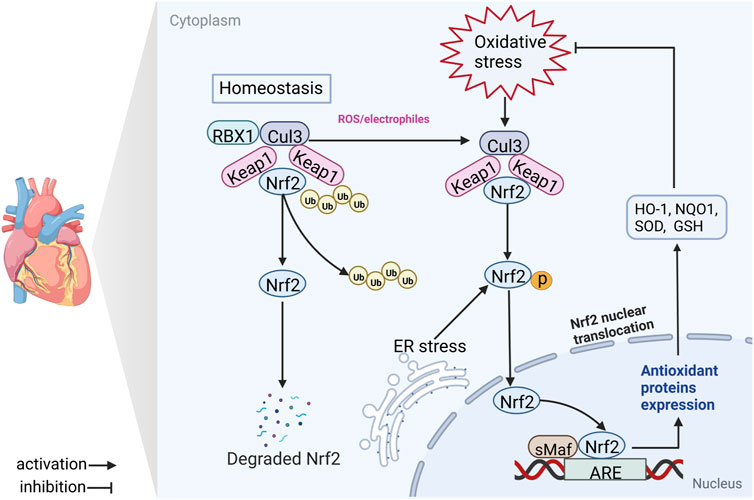
FIGURE 1. Schematic diagram of the Nrf2/ARE signaling axis. In the majority of conditions, Keap1 binds the Cul3 E3 ligase, forming a Keap1-Cul3-RBX1 E3 ligase complex, which promotes ubiquitination and degradation of Nrf2. Nrf2 is cleaved from the Keap1-Cul3-RBX1 complex and translocases to the nucleus where it heterodimerizes with sMaf and binds ARE in response to antioxidant stress (electrophilic, ROS or ER stress) damage. Nrf2, nuclear factor erythroid 2-related factor 2; Keap1, Kelch-like ECH-associated protein 1; RBX1, RING-box protein 1; Ub, ubiquitin; Cul3, cullin 3; ER stress, endoplasmic reticulum stress; sMaf, small musculoaponeurotic fibrosarcoma; ARE, antioxidant response element; GSH, glutathione; NQO1, NADPH quinone oxidoreductase 1; SOD, superoxide dismutase; HO-1, heme oxygenase 1.
In this review, we provide a comprehensive overview of the protective role of the Nrf2 signaling pathway in CVDs. The natural products or derivatives with beneficial properties against CVDs through activation of Nrf2 signaling are summarized, with the aim of providing a reference for subsequent research on CVD management.
Role of Nrf2 signaling in CVDs
Earlier studies have confirmed a vital role of OS in CVD progression and the protective effect of Nrf2 signaling (Andreadou et al., 2021). In view of the activity of Nrf2 as a critical regulator of antioxidant defense, the premise that Nrf2 protects against OS-related cardiovascular disease is feasible.
Nrf2 and arterial HTN
Earlier studies reported distinct physiological equilibrium between ROS/RNS and antioxidants necessary for multiple cellular functions. Overproduction of ROS/RNS impairs vascular cell function along with reduced availability and vasoconstriction of NO., in turn, eliciting HTN (Griendling et al., 2021; Krzemińska et al., 2022; Tanase et al., 2022). While the precise role of Nrf2 in HTN remains to be established, several associations among Nrf2 and ROS/RNS have been detected in HTN animal models (Tanase et al., 2022). Nrf2 is crucial in the regulation of blood pressure (BP) in multiple ways. One of the underlying mechanisms is overexpression of HO-1, which has been shown to decrease BP in a spontaneously hypertensive rat (SHR) animal model (Gao et al., 2017; X. Tan et al., 2021). HO-1 degrades heme to produce carbon monoxide (CO) (Liao et al., 2021), a new type of gaseous signal molecule that can regulate the vascular response through vasoconstrictor suppression via activation of soluble guanylate cyclase and generating cyclic guanosine monophosphate (cGMP) (Álvarez-Maestro et al., 2021). In addition, biliverdin, another product of HO-1 degradation, is transformed to the powerful antioxidant, bilirubin, and further stimulates HO-1 activity for maintaining vascular homeostasis (Cuadrado et al., 2019). In the SHR model, the levels of Nrf2 and its target antioxidant enzymes are reduced, which results in decreased antioxidant activity, increased OS and vascular disruption (Lopes et al., 2015; Ye et al., 2022). Conversely, activation of Nrf2 effectively sustains the redox equilibrium by reducing OS, increases endothelial-dependent vasodilation and decreases BP in the vasculature of the SHR model (Meephat et al., 2021). Similarly, HO-1 expression effectively reverses OS injury in mice with arterial HTN and vascular dysfunction caused by environmental stress, such as noise exposure (Bayo Jimenez et al., 2021). The Nrf2-induced vasoprotective effect on HTN may present another protective mechanism (Tanase et al., 2022). Selective Nrf2 gene deletion in the rostral ventrolateral medulla.
Interestingly, studies to date support a dual role of Nrf2 in diabetes-related HTN. In diabetic Akita mice, genetic deletion of Nrf2 or pharmacological inhibition of Nrf2 attenuated HTN through upregulation of intrarenal angiotensin converting enzyme 2 and angiotensin 1-7 receptors. The opposite effect of Nrf2 activation may be due to Nrf2-mediated stimulation of intrarenal renin-angiotensin system, by which chronic hyperglycemia induces HTN and renal injury in diabetes (S. Zhao et al., 2018). Although the precise role of Nrf2 in diabetes-related HTN remains controversial, numerous preclinical studies suggest that activation of Nrf2 signaling offers a promising strategy for amelioration of vascular OS and endothelial dysfunction during HTN.
Nrf2 and AS
AS is a primary contributor to CVDs, such as MI and HF (Liberale et al., 2021), predominantly through permeation and accumulation of low-density lipoproteins (LDL) into the arterial intima where they are subjected to oxidation (T. X. Zhao & Mallat, 2019; Roy et al., 2022). Oxidation of LDL (ox-LDL) promotes endothelial lesions and activation of foam cells, leading to the formation of fatty streaks in carotid atherosclerotic walls (Alonso-Piñeiro et al., 2021). OS modulated by ROS/RNS therefore plays a key role in the process of AS. At the blood vessel level, multiple endogenous enzymes are considered primary sources of ROS/RNS, including lipoxygenase, NADPH oxidase (NOX), myeloperoxidases, xanthine oxidase (XO) and uncoupled endothelial NO. synthase (eNOS) (Tejero et al., 2019). Enzymes of the NOX family convert O2 to O2.-, which reacts with NO., generating peroxynitrite (ONOO−), thereby inducing endothelial cell damage (Hahner et al., 2020; Ogboo et al., 2022). In addition, uncoupled eNOS contributes to the generation of O2.- and ONOO−, leading to impairment of endothelial function and initiation of AS (Das et al., 2021).
Various antioxidant systems, including SOD, CAT and GPx, within the carotid atherosclerotic wall play a crucial role in counteracting ROS/RNS generation (Alonso-Piñeiro et al., 2021). During this process, Nrf2 acts as a major modulator of antioxidant defense through not only increasing antioxidant enzyme expression but also mediating NADPH oxidase activity (Q. M. Chen, 2021). Nrf2 is closely associated with AS and reported to play an antagonistic role in its development. For instance, in high-fat high-cholesterol diet (HFD)-treated LDL receptor-deficient (LDLR−/−) mice, specific Nrf2 deficiency in bone marrow-derived cells exacerbated early AS (Ruotsalainen et al., 2013).
Recent studies have identified Nrf2 as a key regulator of endothelial miRNA expression under oxidized phospholipid stimulation, which contributes to decreased proliferation of injured vascular endothelial cells (Linna-Kuosmanen et al., 2021). In contrast to these protective effects, Nrf2 has also been shown to exert pro-atherogenic effects. For instance, Nrf2 deficiency protected against AS in ApoE−/− mice (Barajas et al., 2011; Ruotsalainen et al., 2019). Moreover, loss of Nrf2 resulted in increased uptake of modified LDL in thioglycollate-elicited peritoneal macrophages and attenuated the atherosclerotic plaque burden, proliferation, and migration of vascular smooth muscle cells (Caselli et al., 2021; H. Li et al., 2022) reported that in patients, higher plasma HO-1 levels are associated with lower cholesterol and a more diffuse but mainly non-obstructive coronary AS, confirming a potential role of the Nrf2/HO-1 pathway as a protective feedback mechanism.
Nrf2 and MI or reperfusion
The definition of MI is based on evidence of myocyte death on account of extended ischemia associated with occlusion of the coronary artery (Algoet et al., 2022). To rescue cardiac tissue from ischemic damage, reperfusion is accomplished by coronary artery bypass grafting surgery or non-surgical percutaneous coronary intervention (Q. M. Chen, 2021). Although these therapies are beneficial in ameliorating symptoms, reducing infarct size, and sustaining ventricle function, reperfusion triggers further myocyte death, with consequent augmentation of myocardial infarct size (Zeng et al., 2021). Accumulating evidence indicates that OS is a major cause of poor prognosis in MI or reperfusion, leading to myocardial cell death and reduced systolic-diastolic function (Rajesh et al., 2010; Lei et al., 2018). Interestingly, earlier experimental evidence supports the utility of Nrf2 as a lead target for drug development to further improve treatment outcomes for MI and reperfusion (Z. Jiang et al., 2020; Q. Zhang et al., 2022).
In H2O2-treated H9c2 cells, knockdown of Nrf2 was recently shown to induce a decrease in the levels of antioxidant enzymes (GSH, SOD, CAT) in association with increased OS (Z. Zhang et al., 2022). Similarly, in H9c2 cells exposed to hypoxia/reoxygenation (H/R)-induced injury, Nrf2 silencing promoted H/R-stimulated OS damage (W. Jiang et al., 2021; M. Zhang et al., 2021). Moreover, genetic and pharmacological activation of Nrf2 effectively protects against ischemic heart injury in animal models while Nrf2 deficiency exacerbates HF after myocardial infarction (Ashrafian et al., 2012; Cao et al., 2015). These findings collectively highlight the importance of Nrf2 in mitigating ischemic heart disease and support the application of effective Nrf2 stimulators as potential treatment agents for MI reperfusion injury (MIRI). In the mouse model, a key role of Nrf2 in protecting the heart has been widely demonstrated. Earlier studies indicated that cardiomyopathy is exacerbated in Nrf2 deficient (Nrf2−/−) mice after PM2.5 insult (Ge et al., 2020; J. Liu et al., 2022). Augmentation of MI, cardiac insufficiency and senescence in Nrf2−/− mice were induced by left coronary artery ligation (Luo et al., 2020). In cardiac-specific Nrf2 transgenic mice exposed to isoproterenol (ISO), enhanced Nrf2-dependent antioxidant defense suppresses OS and prevents pathological cardiac remodeling (Shanmugam et al., 2019). Induction of Nrf2 antioxidant signaling delivers protection against MIRI by suppressing mitochondrial ROS production and improving bioenergetics in vitro and in vivo (Duan et al., 2017; Y. Q. Li et al., 2022; H. Xu et al., 2022).
Nrf2 and HF
Patients recovering from MI or reperfusion are at considerably higher risk for progression of HF in subsequent years (Mauro et al., 2022; Salerno et al., 2022). An imbalance between oxygen radical synthesis and elimination by antioxidant defense mechanisms leads to macromolecular damage and disruption of cellular redox signaling, which affects cardiac structure and function (Leyane et al., 2022). Continuous OS contributes to myocyte death or cardiac dysfunction (X. Xu et al., 2022). Increasing evidence supports a crucial role of Nrf2 in the process of HF.
In an HF mouse model, Nrf2 was expressed at low levels in the rostral ventrolateral medulla and its overexpression led to significantly reduced sympathetic nerve activity and improved arterial function (Ma et al., 2019). Excessive OS in obesity and HF with preserved ejection fraction induced by a high-fructose/high-fat Western diet is associated with reduced phosphorylation of Nrf2 (Hayden & Bostick, 2019). Furthermore, Nrf2 depletion leads to increased cardiac damage and accelerated HF after ischemic injury (Strom & Chen, 2017; Gutiérrez-Cuevas et al., 2022). Recently, Wu et al. (2021) demonstrated that an antagonist of Nrf2 increased the ER OS response of cardiomyocytes in HF rats. Activation of Nrf2 successfully alleviated diastolic dysfunction and reduced NO in vivo (Yoon et al., 2021) and induced a significant increase in NQO1 expression, conferring beneficial antioxidant effects and reducing afterload in the myocardium of patients with HF (A. Zhang et al., 2018). The collective results suggest that activation of Nrf2 is effective in prevention of cardiomyocyte injury in HF.
Nrf2 and other CVDs
The transcription factor Nrf2 exerts beneficial effects against cardiovascular injury (for example, doxorubicin (DOX)-induced cardiotoxicity, diabetic cardiomyopathy (DCM) and cardiorenal syndrome (CRS)) by maintaining the redox equilibrium. Downregulation of Nrf2 and HO-1 expression was observed in an earlier mouse model of DOX-induced chronic cardiotoxicity (X. Li et al., 2022). More recent studies on cultured rat cardiomyocytes showed that overexpression of Nrf2 ameliorates DOX-induced myocardial oxidative damage, mitochondrial dysfunction and cardiac myocyte death via enhancing anti-oxidative activity (Y. Wang et al., 2022) whereas its knockdown exacerbates OS, mitochondrial dysfunction and cardiac myocyte death (W. B. Zhang et al., 2021; J. Li et al., 2022). DOX-induced cardiotoxicity was amplified in Nrf2−/− mice, along with increased myocardial OS and cardiomyocyte necrosis (W. B. Zhang et al., 2021). Accumulating evidence over the last few years has demonstrated a critical role of the Nrf2/ARE signaling axis in preventing hyperglycemia-induced OS in diabetic heart (Syed et al., 2021). In a diabetic mouse model, the Nrf2−/− phenotype was shown to induce cardiac remodeling and dysfunction (Gao et al., 2018; Fang et al., 2022). Moreover, silencing of Nrf2 increased MDA and decreased superoxide dismutase levels in glucolipotoxicity-induced H9c2 cells (L. Wang et al., 2022). Conversely, activation of the Nrf2 pathway significantly increased cardiomyocyte viability, reduced ROS formation and enhanced antioxidant enzyme activity in a Type 2 diabetic rat model (X. Wu et al., 2022). Diseases of the kidney and heart often occur in parallel, eventually leading to CRS, whereby acute or chronic dysfunction of one organ induces the same disorder in the other (Patel et al., 2022). In the 5/6 nephrectomy-induced CRS mouse model, the intensity of ROS expression was markedly increased and Nrf2 was significantly reduced in kidney and heart tissue (Yang et al., 2019; Wang et al., 2020). Similarly, the increase in OS due to Nrf2 depletion aggravated cardiac dysfunction in the CRS model (L. Xu et al., 2022). Additionally, aging is one of the most critical risk factors for the development of CVDs and their complications (Kloska et al., 2019). There are several studies demonstrate that Nrf2 exerts beneficial effect on vascular function in aging CVDs. In aged rhesus macaques (Macaca mulatta, age: ≥20 years) animal model, the level of ROS in carotid arteries was significantly increased in comparison with young macaques (age: ∼10 years); Whereas, age-related OS did not activate or induce Nrf2 and its downstream target genes (e.g. NQO1, GCLC, and HMOX1). Interestingly, in cultured vascular smooth muscle cells that derived from aged macaques or young macaques, H2O2 combined with high glucose-induced Nrf2 activity and Nrf2-driven gene expressions were blunted in vascular smooth muscle cells from aged macaques than those of young macaques (Ungvari et al., 2011a). Moreover, in 24-month-old aged mice, Nrf2 dysfunction can impair cellular stress recovery and increase OS, thereby accelerating vascular aging (Fulop et al., 2018). Nrf2 deficiency contributes to the increased OS in cardiac muscle and vasculature during aging (Ungvari et al., 2011b; Kubben et al., 2016). Of note, in Nrf2−/− mice, the effects of cardiovascular risk factors (e.g. obesity) were enhanced due to Nrf2 deficiency accelerates cellular senescence (R. Wang et al., 2017; Tarantini et al., 2018).
Protective effects of natural products on CVDs
Comprehensive studies have verified that natural products from herbal medicines exert excellent protective effects against CVDs through activation of the Nrf2 pathway. In the following sections, recent developments in studies on the beneficial effects of natural products against CVDs associated with Nrf2 are reviewed.
Natural plant extracts
In the ISO-induced MI model, Rhaponticum carthamoides (Willd.) Iljin and Myrrh essential oils induce upregulation of Nrf2 and HO-1 and protect the oxidative status of cardiac myocytes by increasing the activities of cardiac antioxidants, such as GSH, SOD and CAT (Younis & Mohamed, 2021; Zheng et al., 2022). In addition, an aqueous extract of Amauroderma rugosum (AR) has been shown to significantly promote Nrf2/HO-1 signaling and reduce OS, mitochondrial dysfunction and apoptosis in Dox-treated H9c2 cells or mice. Notably, knockdown of Nrf2 abolished the protective effect of AR in these cells (J. Li et al., 2022) (Table 1).
Flavonoids
Phytoconstituents, such as flavonoids, terpenoids and phenols with anti-OS abilities, have significant therapeutic potential against cardiovascular damage. Flavonoids possess highly beneficial anti-oxidant, anti-inflammatory, anti-viral, anti-diabetic, anti-cancer, neuroprotective, and cardioprotective properties (Naeem et al., 2022). In recent years, the potential of flavonoids against diseases related to AS, MI and HF has been clinically confirmed. Baicalin is a plant-derived flavonoid with cardioprotective effects that increases hypoxia-stimulated H9c2 activity and expression of Nrf2 and HO-1 proteins (H. Yu et al., 2019). In addition, baicalin reduces ROS and inflammation via Nrf2 activation in human umbilical vein vascular endothelial cells (HUVECs) stimulated with high glucose or mice with streptozotocin (STZ)-induced diabetes. However, reported that the protective effects of baicalin are almost abolished in HUVECs transduced with shRNA against Nrf2 (G. Chen et al., 2018).
Arthrinium sp., 2,3,4,6,8-pentahydroxy-1-methylxanthene and Arthon C are novel flavonoids from deep-sea fungi, which effectively scavenge ROS and enhance nuclear translocation of Nrf2 and its downstream antioxidant gene, HO-1. Knockdown of Nrf2 abolishes these effects and exacerbates ox LDL-induced oxidative damage in HUVECs (Hou et al., 2021). Anthocyanin in plants promotes Nrf2 activation and release in a concentration-dependent manner, leading to increased HO-1 and NQO1 expression in HUVECs under hyperoxia (Cimino et al., 2013; Herrera-Bravo et al., 2022). Cyanidin-3-O-glucoside, another type of anthocyanin, induces nuclear localization of Nrf2 and activation of cellular antioxidant and cytoprotective genes in palmitate or TNF-α-induced HUVECs (Speciale et al., 2013; Fratantonio et al., 2015). Additionally, irigenin is reported to elevate cell viability and balance the OS status of HUVECs after exposure to angiotensin II (Ang II) through activation of Nrf2 signaling and, conversely, Nrf2 deficiency abrogates the protective effects of irigenin (Q. Zhang M. et al., 2021). Moreover, hesperidin is a naturally occurring flavonone, which presents in citrus fruits and has been shown to protect against cardiac OS in aged rat through activates Nrf2 and then maintains the redox status (Elavarasan et al., 2012).
Diosmetin is a citrus flavonoid that improves endothelial dysfunction, suppresses over activity of sympathetic nerve-mediated vasoconstriction and upregulates Nrf2 and HO-1 proteins in Nω-nitro-l-arginine methyl ester l-NAME-induced HTN rats (Meephat et al., 2021). Recent study further indicates that diosmetin improves cardiomyocyte hypertrophy and inhibits cardiac OS through increasing protein expression of Nrf2 and Keap1 in aortic banding-induced pressure overload mouse models or phenylephrine (PE)-induced neonatal rat ventricular myocytes. However, blockade of Nrf2 activation offsets the diosmetin-mediated protective effects against PE in vitro (Guo et al., 2022). Moreover, xanthohumol, a major prenylated chalcone found in hops, exerts antioxidant effects to inhibit vascular calcification in rat induced by vitamin D3 plus nicotine through enhanced nuclear translocation of Nrf2 and HO-1 (Liou et al., 2020). Interestingly, however, in H9c2 cells treated with Fe-SP and RSL3 or ischemia/reperfusion (I/R)-induced rat heart, xanthohumol induced a decrease in the Nrf2 level to protect the myocardium against I/R injury-induced ferroptosis (Lin et al., 2022).
In an LAD-induced MI model, isoliquiritigenin (ISL) or icariin significantly reduced the myocardial infarction area, improved cardiac function, inhibited ROS and MDA production, suppressed SOD and GPx consumption through increased nuclear Nrf2 and cytosolic HO-1 levels and reduced OS after AMI (Sai et al., 2022; D. Yao et al., 2022). Furthermore, in mice or rats with STZ-induced diabetes mellitus or high glucose-cultured H9c2, ISL attenuated inflammation and OS and protected against aortic injury through upregulation of Nrf2 and HO-1 (X. Gu et al., 2020; Alzahrani et al., 2021).
Pinocembrin, a flavonoids compound from honey, propolis and Boesenbergia pandurata, exhibits diverse biological activities reported to enhance ROS uptake and activate Nrf2 in LAD-induced HF or DOX-induced cardiotoxicity (J. Gu et al., 2021). Interestingly, specific Nrf2 inhibitors significantly reversed the antioxidant effects of pinocembrin in vitro (X. Chen et al., 2021). Cardamonin, a flavone compound naturally residing in multiple herbs, such as Alpinia katsumadai, Ginkgo biloba, and Carya cathayensis Sarg, under conditions of LPS-induced HF or DOX-induced cardiotoxicity, effectively inhibited cardiac mechanical dysfunction and OS through promoting nuclear translocation of Nrf2 along with increasing HO-1 and antioxidant defense, which were reversed upon intervention with a Nrf2 inhibitor (Qi et al., 2020; Y. Tan et al., 2021). Kaempferol, another natural flavonoid widely distributed in plants, possesses multiple pharmacological properties, including anti-inflammatory, anti-oxidant and ant-apoptotic activities (Sharma et al., 2019). Protective cardiovascular effects of kaempferol through activation of the Nrf2 pathway have been demonstrated in numerous animal models, including ISO-induced HF in diabetic rats (L. Zhang et al., 2019), HFD-induced AS in APOE−/− mice (Feng et al., 2021), STZ-induced DCM in mice (X. Chen et al., 2018), and LAD induced MIRI (D. Wang et al., 2017) (Table 2).
Terpenoids
Terpenoids produced by plants are specific metabolites with significant biological activity that show considerable promise as therapeutic compounds. Terpenoids have been shown to exert protective effects against CVDs through activation of the Nrf2 pathway. For instance, kirenol, a terpenoid derived from Herba Siegesbeckia, attenuates benzo[a]pyrene-induced AS in HUVECs via activation of Nrf2. Notably, however, Nrf2 deficiency largely abolishes the protective effects of kirenol on HUVEC cells following OS challenge (Rajendran et al., 2021). Tanshinone I, a primary component of Salvia miltiorrhiza, confers a variety of favorable pharmacological activities, including cardiovascular protection. In MI/R animal model, tert-butyl hydroperoxide-stimulated H9c2 cells (Zhuo et al., 2021) or DOX-induced cardiotoxicity model (Q. Jiang et al., 2022), tanshinone I significantly attenuates OS through activation of Nrf2 to promote antioxidant-related protein expression. However, its protective effect was abolished in Nrf2−/− mice (Y. T. Wu et al., 2021). In addition, dihydrotanshinone-I (DT) has been shown to ameliorate MIRI and H/R injury with maintenance of redox homeostasis by activating Nrf2. However, DT fails to improve the final infarct size and subsequent cardiac remodeling in Nrf2−/− mice after MIRI. Moreover, DT-induced beneficial effects are abolished in Nrf2-depleted cardiomyocytes, further supporting a pivotal role of Nrf2 in therapeutic efficacy of DT (Zeng et al., 2021).
In mice with LAD-induced MI or diabetic cardiomyopathy, andrographolide effectively reduces OS and enhances antioxidant stress capacity through activation of Nrf2 (Liang et al., 2018). Conversely, a specific Nrf2 inhibitor or silencing of Nrf2 substantially counteracts the protective effects of andrographolide in H9c2 (S. Xie et al., 2020). Ruscogenin, a naturally occurring steroidal sapogenin, induces a significant decrease in infarct size and ameliorates biochemical indicators and cardiac pathological changes after MI in mice through activation of the Keap1/Nrf2/HO-1 signaling pathway (Fu et al., 2022). Nootkatone is a naturally occurring bioactive sesquiterpene shown to attenuate myocardial OS in ISO-induced MI in by activating the PI3K/Nrf2/Akt cascade (Meeran et al., 2021). Moreover, astragaloside IV has been shown to exert potent cardioprotective effects in various animal models, including abdominal aortic constriction (AB)-induced HF, LAD-induced HF and MIRI, through activation of Nrf2/HO-1 signaling (M. Jiang et al., 2019; Nie et al., 2019; Sui et al., 2020). Astragaloside IV additionally prevents ox-LDL-induced HUVEC injury through mediation of Nrf2/HO-1 signaling (Z. Zhu et al., 2019) (Table 3).
Alkaloids
Sulforaphane (SFN) is a natural plant compound found in many cruciferous vegetables such as broccoli, cabbage, cauliflower and kale. SFN attenuates OS and cell death by reducing ROS via activation of Nrf2 in several in vitro models, including Ang II-induced HUVEC injury (M. Zhang et al., 2020), TGF-β1-induced activation of rat cardiac fibroblasts (Fix et al., 2019) and DOX-induced cardiotoxicity in H9c2 cells (Li et al., 2015). Furthermore, SFN protects cardiomyocytes from OS by activating Nrf2 in mouse models in several in vivo animal models, including Ang II-induced cardiomyopathy in mice (Xin et al., 2018), HFD/STZ-induced diabetic cardiomyopathy in mice (J. Gu et al., 2017; Y. Sun et al., 2020; X. Wang et al., 2022) and intermittent hypoxia-induced cardiomyopathy in mice (Zhou et al., 2018). Moreover, SFN also prevents age-associated cardiac and muscular dysfunction through activation of Nrf2 (Angulo et al., 2019; Bose et al., 2020). Remarkably, there are currently more than 20 ongoing clinical trials of SFN for treating multiple disorders such as diabetes, autism, breast cancer, chronic obstructive pulmonary disease, asthma, non-alcoholic fatty acid. Notably, both the sprout extract and highly purified SFN show excellent safety profile in clinic (Cuadrado et al., 2019). Berbamine, a natural compound of berberis, alleviates LAD-induced MIRI by inhibiting OS through promoting Nrf2 nuclear translocation. A Nrf2-specific inhibitor has been shown to counteract the antioxidant effects of berbamine (C. Xu et al., 2022). Recent studies indicate that songorine protects cardiac function in LPS-treated mice and rescues cardiomyocytes from LPS-induced endotoxin insult through activation of Nrf2/ARE and Nrf1 signaling cascades (Y. Li et al., 2021). In addition, sinomenine ameliorates cardiac hypertrophy in ISO-induced HF through activating the Nrf2/ARE signaling pathway. However, Nrf2 inhibitors abolish the protective effect of sinomenine on HF (Yuan et al., 2021) (Table 4).
Glucosides
Sweroside, a secoiridoid glucoside extracted from Swertia pseudochinensis Hara, has a wide range of pharmacological properties, including antioxidant and anti-inflammatory effects. In H9c2 with H/R-induced injury and a rat model with Langendorff-induced MIRI, sweroside effectively improved cardiac function and reduced OS through promoting nuclear translocation of Nrf2. These beneficial effects were blocked by a Nrf2 inhibitor (J. Li et al., 2021). Pubescenoside A has been reported to exert cardioprotective effects with anti-OS properties in multiple experimental models, including LAD-induced MIRI, LPS/ATP-mediated RAW264.7 macrophages, OGD/R-induced H9c2 cells and primary cardiomyocytes, through activation of the Nrf2 pathway, which are almost offset in Nrf2−/− mice. Further mechanistic studies have revealed selective covalent binding of pubescenoside A to conserved cysteine residues, specifically, cysteine 77 in the BTB domain and cysteine 434 in the Kelch domain of Keap1, with consequent inhibition of Nrf2 ubiquitination and activation of antioxidant enzymes (Cheng et al., 2021) (Table 5).
Other products
In addition to the above compounds, numerous other natural products have been shown to exert protective effects on CVDs through activation of Nrf2 signaling. Withaferin A, a natural phytochemical derived from the plant Withania somnifera, time- and concentration-dependently induces HO-1 expression in HUVECs and EA.hy926 via upregulation and increased nuclear translocation of Nrf2 (Heyninck et al., 2016). Similarly, in endothelial cell line (EA.hy926) with tert-butyl hydroperoxide-induced injury originating from fusion of human umbilical vein endothelial and A549 human lung carcinoma cells, Z-ligustilide attenuates atherosclerotic plaque formation and enhances antioxidant enzyme activity through activation of Nrf2 signaling (Y. Zhu et al., 2019).
In LAD- and HFD-induced ApoE−/− or HFD-induced LDLR−/− mice, resveratrol and Z-ligustilide block the development of AS via inducing Nrf2 activity (Seo et al., 2019). Furthermore, resveratrol mitigates HTN in SHR through stimulation of Nrf2 activity (Javkhedkar et al., 2015; Y. Zhu et al., 2019). Of note, anti-inflammatory and antioxidant effects of resveratrol are ongoing in phase 3 clinical trial. Curcumin is a known natural compound with multiple bioactivities, including antitumor, antioxidant and anti-inflammatory activities. Study has shown that curcumin effectively not only improves the histological changes in the myocardial tissues of diabetic rats, but also reduces generation of ROS through activation of the Nrf2 (Xiang et al., 2020; Wei et al., 2022). Furthermore, by modifying the Cys151 residue of Keap1, curcumin inhibits the ability of the Cullin3-Rbx1 E3 ubiquitin ligase complex to ubiquitinate against Nrf2, thereby potentially altering the conformation of the complex, saturating the binding capacity of Keap1 to Nrf2 and facilitating the nuclear translocation of newly synthesized Nrf2 (Shin et al., 2020). Most importantly, supplementation with resveratrol or curcumin do not cause cardiovascular risk as evidenced by ongoing clinical trials (Vors et al., 2018). In mice with LAD-induced MIRI, histochrome, panaxatriol saponin or lithospermic acid exert cardioprotective effects through upregulation of Nrf2 and HO-1 proteins. However, the beneficial effects of these compounds are almost abolished under conditions of Nrf2 deficiency (Hwang et al., 2021; M. Zhang et al., 2021; H. Yao et al., 2022). Zingerone attenuates aortic banding-induced cardiac remodeling and cardiac hypertrophy, along with reducing OS through mediating Nrf2/ARE activation. Consistent with earlier findings, Nrf2 deficiency counteracts the cardioprotective effects of zingerone in vivo (C. Liu et al., 2019). Additionally, procyanidins effectively decrease ROS and MDA levels and increase SOD activity in PM2.5-induced rats through upregulation of protein expression of Nrf2 and its downstream antioxidant genes NQO1 and HO-1 (L. M. Zhang et al., 2021). Ellagic acid, a naturally occurring phenolic constituent, derives from fruits and nuts effectively improves hypochlorous acid-induced endothelial dysfunction in HAECs and protects against HFD-induced atherosclerosis in ApoE−/− mice through activation of Nrf2. Whereas, these protective effects of Ellagic acid are substantially offset in Nrf2−/− mice (Ding et al., 2014) (Table 6).
Molecular mechanisms of cardioprotective natural products targeting the Nrf2 signaling pathway
The cardioprotective role of natural products that target the Nrf2 pathway has been widely investigated. There are multiple mechanisms that are involved in activating Nrf2, including interaction with cysteine residues on Keap1, disruption of Nrf2/Keap1 interaction and activation of protein kinases (Figure 2). Keap1 is a cysteine rich adaptor protein, of which Cys151, Cys273 and Cys288 play essential roles in inhibiting the activation of Nrf2 signaling (Saito et al., 2016). Studies have revealed that SFN, rutin and curcumin can inhibit the ability of the Cullin3-Rbx1 E3 ubiquitin ligase complex via modifying Keap1 Cys151 residue, resulting in Nrf2 activation to protect against myocardial injury in mice (Takaya et al., 2012; Sthijns et al., 2017; Shin et al., 2020). Similarly, data of molecular docking indicates that the BTB domains in Keap1-Nrf2 complex directly bound the cyanidin-3-glucoside (−6.9 kcal/mol), malvidin-3-glucoside (−6.6 kcal/mol) and peonidin-3-glucoside (−6.6 kcal/mol), which are the major active compound of glycosylated anthocyanins (Herrera-Bravo et al., 2022). Apart from that, a number of bioactive compounds including withaferin A, xanthohumol and betanin can modify cysteine residues, specifically Cys151, Cys273 and Cys288, through interaction with their sulfhydryl groups, allowing the cleavage of the Keap1-Nrf2 interaction inducing antioxidant proteins and phase II detoxification enzymes (Yao et al., 2015; Heyninck et al., 2016; Silva et al., 2022). Emerging evidence demonstrates that several natural product compounds exert cardioprotective effects by disrupting the Nrf2/Keap1 interaction and promoting Nrf2 nuclear translocation. For instance, baicalein and myricetin directily bind Keap1-Nrf2 complex and reduces the steady-state level of Keap1 by increasing its ubiquitination and modification without dissociation from Nrf2, which leads to the disengagement of Nrf2 from ubiquitination (Qin et al., 2013). α-Linolenic acid has been found to protect against DOX-induced cardiotoxicity by promoting the degradation of Keap1 and thus facilitating nuclear translocation of Nrf2 via directly binds Keap1-Nrf2 complex (X. Yu et al., 2013). Moreover, protein kinases such as ERK, GSK3β, MAPK, and PI3K/AKT can mediate Nrf2 phosphorylation which enhance Nrf2 stability, thereby promoting nuclear Nrf2 translocation and transactivation activity. Recent studies have reported that Baicalein increased the phosphorylation level of MEK1/2, AKT and JNK1/2, and stabilized Nrf2 protein by inhibiting the ubiquitination and proteasomal turnover of Nrf2 (Qin et al., 2014). Genistein and baicalin can activate the Nrf2 pathway to alleviate oxidative injury via the activation of the ERK1/2 signaling pathways (Zhai et al., 2013; Shi et al., 2018). In addition, ginsenoside-Rb3 activated the NRF2 signaling pathway through PERK, which can protect cardiomyocytes against H/R injury (J. Sun et al., 2019). These findings suggest that natural products might be directly bind to Keap1-Nrf2 complex or indirect activation by facilitating or inhibiting other factors to confer the cardioprotection.
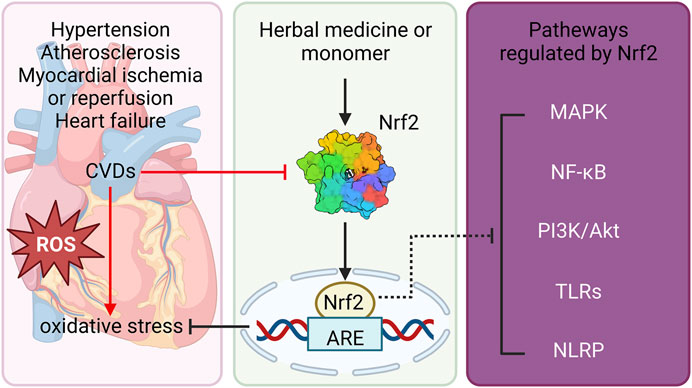
FIGURE 2. Schematic diagram of natural product-mediated activation of Nrf2 against CVDs. Natural products protect against CVDs including hypertension, atherosclerosis, myocardial ischemia, heart failure via reducing OS. Furthermore, the beneficial effects of natural products on CVDs, at least partly, through activation of Nrf2 and its downstream ARE, as well as modulation of other signaling pathways including MAPK, NF-κB, PI3K/Akt, TLRs and NLRP signalings. Nrf2, nuclear erythroid 2-related factor 2; CVDs, cardiovascular diseases; OS, oxidative stress; ARE, antioxidant response element; MAPK, mitogen-activated protein kinase; NF-κB, nuclear factor kappa-light-chain-enhancer of activated B cells; PI3K, phosphatidylinositol-3-kinase; AKT, protein kinase B; TLRs, toll-like receptors; NLRP, NOD-like receptors.
Challenges and considerations
Currently, there are still remain challenges and considerations, including pharmacodynamic evaluation and safety profile of Nrf2. Since Nrf2 possesses a very short half-life (15–30 min), the therapeutic benefit of optimal curative dosing regimen needs to be reflected by indirect indicators of its activation in the various damage organs. To solve this question, assessment of drug distribution and gene expression signatures of NRF2 in accessible tissues or cells with a view to that the Nrf2 transcriptomic signature in those cells predicts local engagement in other tissues. In addition, the levels of urinary and its metabolites have been utilized as indicators of pharmacodynamic effect modulated through Nrf2 to avoid exposing to hazardous environmental chemicals. Recent studies reveal that the pharmacodynamics of Nrf2 activators are observed for longer periods of time than the Nrf2 levels and they do not correspond to the concentrations of plasma drug. Therefore, TBE-31, a Nrf2 activator, has a half-life of 10 h in skin and plasma of mice; while, NQO1 is still evident in the murine skin for at day 3 after the last administration of Nrf2 activator. Thus, a model of “indirect pharmacodynamic response” might be a more reasonable approach for assessing the pharmacodynamics of Nrf2 activator towards clinical applications.
The concentration and distribution of phytochemical Nrf2 activators, as well as their solubility also play crucial roles in the extent of NRF2 activation, and eventually leading to the ideal long-lasting pharmacodynamic actions. Often, several phytochemicals have poor bioavailability (e.g. curcumin, resveratrol and baicalin), so much that the systemic concentrations reached are seldom close to the physiological concentrations required for scavenging free radicals. In fact, most studies have used supra-physiological concentrations of these compounds which is very difficult to achieve with normal diet consumption (Y. T. Wu et al., 2021; P. Chen et al., 2022). Thus, optimization of clinically promising drug exposure needs to analyze dose-dependent gene mediation by a putative Nrf2 activator. Moreover, advanced drug delivery systems that achieve maximal therapeutic efficacy and optimal drug dosage may be a logical approach to conquer poor bioavailability of phytochemicals.
Although Nrf2 activator confers cardioprotection against CVDs, it is important to investigate how significant is the risk posed by promoting levels of Nrf2 beyond a safe-threshold. Recent reports have been revealed that somatic mutations in NFE2L2 or Keap1 or collaborate with the associated oncogenic pathways elicit over activation of Nrf2 and accelerate carcinogenesis. Preclinical proof-of-concept studies demonstrated that due to determine the potential cancer risk, dosing of specific Nrf2 activators need to be extremely carefully monitored. Thus, pharmacological activation of Nrf2 to treat CVDs requires not beyond the safe therapeutic window.
Frontiers and hotspots
Keyword visualization analysis of the research literature in this area was conducted using CiteSpace software (Figure 3). In brief, the following search strategy was used: TS = “Nrf2” and “Cardiovascular Diseases”. Studies published from the inception of the database to 2022 were retrieved. All valid data were imported to CiteSpace 6.1.R2 Basic and de-duplicated for subsequent visual analysis. Factors used for previous research on the CiteSpace setup were applied as follows: look back years (=5), link retaining factor (=3), e for top N (e = 1), years per slice (1), time span (2014–2022), links selection criteria (g-index, k = 25; strength: cosine, scope: within slices) and pruning (pathfinder-pruning sliced networks) (Y. Zhang et al., 2022). Keywords with frequency >15 were labeled and those with the top 14 strongest bursts identified. Visualization of keywords additionally showed that research hotspots on Nrf2-mediated CVDs in recent years have mainly focused on OS, CVDs, Nrf2, NF-κB, endothelial dysfunction, inflammation, and apoptosis. A map based on the top 14 keywords with the strongest citation bursts was generated, which showed the shift in research hotspots over time. The significant keywords in the early stages (2014–2017) included NF-κB, antioxidant response, HO-1, and gene expression. Over recent years (2019–2022), ARE, ROS, particulate matter, obesity, vascular inflammation and mitochondrial dysfunction have been the main research hotspots. The collective findings validate the beneficial effects of Nrf2 against CVDs, supporting the development of naturally occurring Nrf2 agonists as promising therapeutic approach.
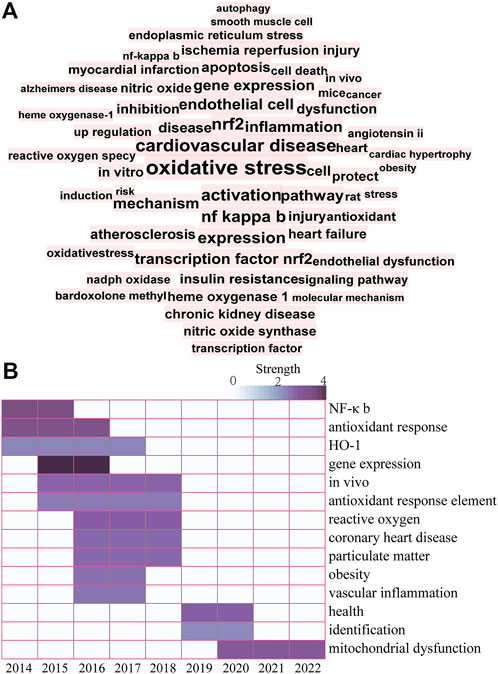
FIGURE 3. (A) Keyword co-occurrence knowledge map. A larger and more centered font corresponds to the number of times a keyword appears. (B) Top 14 keywords with the strongest citation bursts. The color depth represents the strength value of keywords with the strongest citation bursts. Darker color corresponds to a larger strength value.
Conclusion and perspectives
OS plays a vital role in CVD progression and thus presents a key therapeutic target. Cells possess inherent antioxidant systems to overcome the toxic effects of OS, such as the Nrf2 signal transduction pathway. Multiple preclinical experiments support targeting of Nrf2 as an option for OS management. Pharmacological regulators that stimulate the Nrf2 antioxidant defense system may thus offer an effective treatment strategy for OS-associated CVDs. Notwithstanding, in this review, we summarized and updated the potential naturally occurring products with cardioprotective properties on CVDs that exert their effects by inhibition of OS through activation of Nrf2, there are still several pending issues need to be addressed. Firstly, elaborately designed, large cohort clinical trials of naturally occurring Nrf2 activators to ascertain their adverse effects in humans are required in the future. Secondly, the detailed mechanisms of Nrf2 activators against CVDs should be explored in depth. In fact, the ongoing clinical trials will definitely offer momentous advances in answering these pending issues.
Author contributions
JG and QG conceived, designed and revised this review. The literature was collected and organized by XW, JW, and YY. The manuscript was drafted by XW. JG approved the final version of the manuscript on behalf of all the authors.
Funding
This work was supported by Natural Science Foundation of China (No.82160756), Science and Technology Support Plan of Guizhou Province (No. [2020]1Y010), The “hundred” level of high-level innovative talents in Guizhou Province (No. QKHRCPT 20165684), Collaborative Innovation Center of Chinese Ministry of Education (No. 2020-39), Science and technology planning project of Zunyi (No. [HZ 2021]187), National Natural Science Foundation of China (No. 32270848), Guizhou Province Science and Technology Project (No. Qian Ke He[2019]5406), Guizhou Province Science and Technology Project (No. Qian Ke He[2020]4Y192).
Conflict of interest
The authors declare that the research was conducted in the absence of any commercial or financial relationships that could be construed as a potential conflict of interest.
Publisher’s note
All claims expressed in this article are solely those of the authors and do not necessarily represent those of their affiliated organizations, or those of the publisher, the editors and the reviewers. Any product that may be evaluated in this article, or claim that may be made by its manufacturer, is not guaranteed or endorsed by the publisher.
References
Algoet, M., Janssens, S., Himmelreich, U., Gsell, W., Pusovnik, M., Van den Eynde, J., et al. (2022). Myocardial ischemia-reperfusion injury and the influence of inflammation. Trends cardiovasc. Med. doi:10.1016/j.tcm.2022.02.005
Alonso-Piñeiro, J. A., Gonzalez-Rovira, A., Sánchez-Gomar, I., Moreno, J. A., and Durán-Ruiz, M. C. (2021). Nrf2 and heme oxygenase-1 involvement in atherosclerosis related oxidative stress. Antioxidants (Basel) 10 (9), 1463. doi:10.3390/antiox10091463
Álvarez-Maestro, M., Eguibar, A., Chanca, P., Klett-Mingo, M., Gómez Rivas, J., Buño-Soto, A., et al. (2021). Androgen deprivation therapy in patients with prostate cancer increases serum levels of thromboxane A(2): Cardiovascular implications. Front. Cardiovasc. Med. 8, 653126. doi:10.3389/fcvm.2021.653126
Alzahrani, S., Said, E., Ajwah, S. M., Alsharif, S. Y., El-Bayoumi, K. S., Zaitone, S. A., et al. (2021). Isoliquiritigenin attenuates inflammation and modulates Nrf2/caspase-3 signalling in STZ-induced aortic injury. J. Pharm. Pharmacol. 73 (2), 193–205. doi:10.1093/jpp/rgaa056
Andreadou, I., Efentakis, P., Frenis, K., Daiber, A., and Schulz, R. (2021). Thiol-based redox-active proteins as cardioprotective therapeutic agents in cardiovascular diseases. Basic Res. Cardiol. 116 (1), 44. doi:10.1007/s00395-021-00885-5
Angulo, J., El Assar, M., Sevilleja-Ortiz, A., Fernández, A., Sánchez-Ferrer, A., Romero-Otero, J., et al. (2019). Short-term pharmacological activation of Nrf2 ameliorates vascular dysfunction in aged rats and in pathological human vasculature. A potential target for therapeutic intervention. Redox Biol. 26, 101271. doi:10.1016/j.redox.2019.101271
Ashrafian, H., Czibik, G., Bellahcene, M., Aksentijević, D., Smith, A. C., Mitchell, S. J., et al. (2012). Fumarate is cardioprotective via activation of the Nrf2 antioxidant pathway. Cell. Metab. 15 (3), 361–371. doi:10.1016/j.cmet.2012.01.017
Barajas, B., Che, N., Yin, F., Rowshanrad, A., Orozco, L. D., Gong, K. W., et al. (2011). NF-E2-related factor 2 promotes atherosclerosis by effects on plasma lipoproteins and cholesterol transport that overshadow antioxidant protection. Arterioscler. Thromb. Vasc. Biol. 31 (1), 58–66. doi:10.1161/ATVBAHA.110.210906
Bayo Jimenez, M. T., Frenis, K., Kröller-Schön, S., Kuntic, M., Stamm, P., Kvandová, M., et al. (2021). Noise-induced vascular dysfunction, oxidative stress, and inflammation are improved by pharmacological modulation of the NRF2/HO-1 Axis. Antioxidants (Basel) 10 (4), 625. doi:10.3390/antiox10040625
Bose, C., Alves, I., Singh, P., Palade, P. T., Carvalho, E., Børsheim, E., et al. (2020). Sulforaphane prevents age-associated cardiac and muscular dysfunction through Nrf2 signaling. Aging Cell. 19 (11), e13261. doi:10.1111/acel.13261
Cao, S., Chao, D., Zhou, H., Balboni, G., and Xia, Y. (2015). A novel mechanism for cytoprotection against hypoxic injury: δ-Opioid receptor-mediated increase in Nrf2 translocation. Br. J. Pharmacol. 172 (7), 1869–1881. doi:10.1111/bph.13031
Caselli, C., De Caterina, R., Ragusa, R., Liga, R., Gimelli, A., Scholte, A., et al. (2021). Association of circulating heme oxygenase-1, lipid profile and coronary disease phenotype in patients with chronic coronary syndrome. Antioxidants (Basel) 10 (12), 2002. doi:10.3390/antiox10122002
Chen, G., Chen, X., Niu, C., Huang, X., An, N., Sun, J., et al. (2018). Baicalin alleviates hyperglycemia-induced endothelial impairment via Nrf2. J. Endocrinol. 240, 81–98. doi:10.1530/JOE-18-0457
Chen, P., Pei, J., Wang, X., Tai, S., Tang, L., and Hu, X. (2022). Gut bacterial metabolite Urolithin A inhibits myocardial fibrosis through activation of Nrf2 pathway in vitro and in vivo. Mol. Med. 28 (1), 19. doi:10.1186/s10020-022-00444-1
Chen, Q. M. (2021). Nrf2 for cardiac protection: Pharmacological options against oxidative stress. Trends Pharmacol. Sci. 42 (9), 729–744. doi:10.1016/j.tips.2021.06.005
Chen, X., Qian, J., Wang, L., Li, J., Zhao, Y., Han, J., et al. (2018). Kaempferol attenuates hyperglycemia-induced cardiac injuries by inhibiting inflammatory responses and oxidative stress. Endocrine 60 (1), 83–94. doi:10.1007/s12020-018-1525-4
Chen, X., Wan, W., Guo, Y., Ye, T., Fo, Y., Sun, Y., et al. (2021). Pinocembrin ameliorates post-infarct heart failure through activation of Nrf2/HO-1 signaling pathway. Mol. Med. 27 (1), 100. doi:10.1186/s10020-021-00363-7
Cheng, Y., Cheng, L., Gao, X., Chen, S., Wu, P., Wang, C., et al. (2021). Covalent modification of Keap1 at Cys77 and Cys434 by pubescenoside a suppresses oxidative stress-induced NLRP3 inflammasome activation in myocardial ischemia-reperfusion injury. Theranostics 11 (2), 861–877. doi:10.7150/thno.48436
Cimino, F., Speciale, A., Anwar, S., Canali, R., Ricciardi, E., Virgili, F., et al. (2013). Anthocyanins protect human endothelial cells from mild hyperoxia damage through modulation of Nrf2 pathway. Genes. Nutr. 8 (4), 391–399. doi:10.1007/s12263-012-0324-4
Cuadrado, A., Rojo, A. I., Wells, G., Hayes, J. D., Cousin, S. P., Rumsey, W. L., et al. (2019). Therapeutic targeting of the NRF2 and KEAP1 partnership in chronic diseases. Nat. Rev. Drug Discov. 18 (4), 295–317. doi:10.1038/s41573-018-0008-x
Das, M., Devi, K. P., Belwal, T., Devkota, H. P., Tewari, D., Sahebnasagh, A., et al. (2021). Harnessing polyphenol power by targeting eNOS for vascular diseases. Crit. Rev. Food Sci. Nutr., 1–26. doi:10.1080/10408398.2021.1971153
Ding, Y., Zhang, B., Zhou, K., Chen, M., Wang, M., Jia, Y., et al. (2014). Dietary ellagic acid improves oxidant-induced endothelial dysfunction and atherosclerosis: Role of Nrf2 activation. Int. J. Cardiol. 175 (3), 508–514. doi:10.1016/j.ijcard.2014.06.045
Duan, J., Guan, Y., Mu, F., Guo, C., Zhang, E., Yin, Y., et al. (2017). Protective effect of butin against ischemia/reperfusion-induced myocardial injury in diabetic mice: Involvement of the AMPK/GSK-3β/Nrf2 signaling pathway. Sci. Rep. 7, 41491. doi:10.1038/srep41491
Elavarasan, J., Velusamy, P., Ganesan, T., Ramakrishnan, S. K., Rajasekaran, D., and Periandavan, K. (2012). Hesperidin-mediated expression of Nrf2 and upregulation of antioxidant status in senescent rat heart. J. Pharm. Pharmacol. 64 (10), 1472–1482. doi:10.1111/j.2042-7158.2012.01512.x
Fang, Q., Liu, X., Ding, J., Zhang, Z., Chen, G., Du, T., et al. (2022). Soluble epoxide hydrolase inhibition protected against diabetic cardiomyopathy through inducing autophagy and reducing apoptosis relying on Nrf2 upregulation and transcription activation. Oxid. Med. Cell. Longev. 2022, 3773415. doi:10.1155/2022/3773415
Feng, Z., Wang, C., Yue, Jin, Meng, Q., Wu, J., Sun, H., et al. (2021). Kaempferol-induced GPER upregulation attenuates atherosclerosis via the PI3K/AKT/Nrf2 pathway. Pharm. Biol. 59 (1), 1106–1116. doi:10.1080/13880209.2021.1961823
Fix, C., Carver-Molina, A., Chakrabarti, M., Azhar, M., and Carver, W. (2019). Effects of the isothiocyanate sulforaphane on TGF-β1-induced rat cardiac fibroblast activation and extracellular matrix interactions. J. Cell. Physiol. 234 (8), 13931–13941. doi:10.1002/jcp.28075
Fratantonio, D., Speciale, A., Ferrari, D., Cristani, M., Saija, A., Cimino, F., et al. (2015). Palmitate-induced endothelial dysfunction is attenuated by cyanidin-3-O-glucoside through modulation of Nrf2/Bach1 and NF-κB pathways. Toxicol. Lett. 239 (3), 152–160. doi:10.1016/j.toxlet.2015.09.020
Fu, F., Lai, Q., Hu, J., Zhang, L., Zhu, X., Kou, J., et al. (2022). Ruscogenin alleviates myocardial ischemia-induced ferroptosis through the activation of BCAT1/BCAT2. Antioxidants (Basel) 11 (3), 583. doi:10.3390/antiox11030583
Fulop, G. A., Kiss, T., Tarantini, S., Balasubramanian, P., Yabluchanskiy, A., Farkas, E., et al. (2018). Nrf2 deficiency in aged mice exacerbates cellular senescence promoting cerebrovascular inflammation. Geroscience 40 (5-6), 513–521. doi:10.1007/s11357-018-0047-6
Gao, L., Liu, Y., Guo, S., Xiao, L., Wu, L., Wang, Z., et al. (2018). Laz3 protects cardiac remodeling in diabetic cardiomyopathy via regulating miR-21/PPARa signaling. Biochim. Biophys. Acta. Mol. Basis Dis. 1864 (10), 3322–3338. doi:10.1016/j.bbadis.2018.07.019
Gao, L., Zimmerman, M. C., Biswal, S., and Zucker, I. H. (2017). Selective Nrf2 gene deletion in the rostral ventrolateral medulla evokes hypertension and sympathoexcitation in mice. Hypertension 69 (6), 1198–1206. doi:10.1161/HYPERTENSIONAHA.117.09123
Ge, C., Hu, L., Lou, D., Li, Q., Feng, J., Wu, Y., et al. (2020). Nrf2 deficiency aggravates PM(2.5)-induced cardiomyopathy by enhancing oxidative stress, fibrosis and inflammation via RIPK3-regulated mitochondrial disorder. Aging (Albany NY) 12 (6), 4836–4865. doi:10.18632/aging.102906
Griendling, K. K., Camargo, L. L., Rios, F. J., Alves-Lopes, R., Montezano, A. C., and Touyz, R. M. (2021). Oxidative stress and hypertension. Circ. Res. 128 (7), 993–1020. doi:10.1161/CIRCRESAHA.121.318063
Gu, J., Cheng, Y., Wu, H., Kong, L., Wang, S., Xu, Z., et al. (2017). Metallothionein is downstream of Nrf2 and partially mediates sulforaphane prevention of diabetic cardiomyopathy. Diabetes 66 (2), 529–542. doi:10.2337/db15-1274
Gu, J., Huang, H., Liu, C., Jiang, B., Li, M., Liu, L., et al. (2021). Pinocembrin inhibited cardiomyocyte pyroptosis against doxorubicin-induced cardiac dysfunction via regulating Nrf2/Sirt3 signaling pathway. Int. Immunopharmacol. 95, 107533. doi:10.1016/j.intimp.2021.107533
Gu, X., Shi, Y., Chen, X., Sun, Z., Luo, W., Hu, X., et al. (2020). Isoliquiritigenin attenuates diabetic cardiomyopathy via inhibition of hyperglycemia-induced inflammatory response and oxidative stress. Phytomedicine 78, 153319. doi:10.1016/j.phymed.2020.153319
Guo, Y., Li, D., Cen, X. F., Qiu, H. L., Ma, Y. L., Liu, Y., et al. (2022). Diosmetin protects against cardiac hypertrophy via p62/keap1/nrf2 signaling pathway. Oxid. Med. Cell. Longev. 2022, 8367997. doi:10.1155/2022/8367997
Gutiérrez-Cuevas, J., Galicia-Moreno, M., Monroy-Ramírez, H. C., Sandoval-Rodriguez, A., García-Bañuelos, J., Santos, A., et al. (2022). The role of NRF2 in obesity-associated cardiovascular risk factors. Antioxidants (Basel) 11 (2), 235. doi:10.3390/antiox11020235
Hahner, F., Moll, F., and Schröder, K. (2020). NADPH oxidases in the differentiation of endothelial cells. Cardiovasc. Res. 116 (2), 262–268. doi:10.1093/cvr/cvz213
Hayden, J., and Bostick, B. (2019). Western diet induced obesity increases oxidative stress in the heart by impairing the Nrf2 antioxidant response pathway. J. Am. Coll. Cardiol. 73 (9S1), 896. doi:10.1016/s0735-1097(19)31503-7
Herrera-Bravo, J., Beltrán, J. F., Huard, N., Saavedra, K., Saavedra, N., Alvear, M., et al. (2022). Anthocyanins found in pinot noir waste induce target genes related to the Nrf2 signalling in endothelial cells. Antioxidants (Basel) 11 (7), 1239. doi:10.3390/antiox11071239
Heyninck, K., Sabbe, L., Chirumamilla, C. S., Szarc Vel Szic, K., Vander Veken, P., Lemmens, K. J. A., et al. (2016). Withaferin A induces heme oxygenase (HO-1) expression in endothelial cells via activation of the Keap1/Nrf2 pathway. Biochem. Pharmacol. 109, 48–61. doi:10.1016/j.bcp.2016.03.026
Hou, J. R., Wang, Y. H., Zhong, Y. N., Che, T. T., Hu, Y., Bao, J., et al. (2021). Protective effect of flavonoids from a deep-sea-derived arthrinium sp. against ox-LDL-induced oxidative injury through activating the AKT/Nrf2/HO-1 pathway in vascular endothelial cells. Mar. Drugs 19 (12), 712. doi:10.3390/md19120712
Huang, C. Y., Deng, J. S., Huang, W. C., Jiang, W. P., and Huang, G. J. (2020). Attenuation of lipopolysaccharide-induced acute lung injury by hispolon in mice, through regulating the TLR4/PI3K/Akt/mTOR and keap1/nrf2/HO-1 pathways, and suppressing oxidative stress-mediated ER stress-induced apoptosis and autophagy. Nutrients 12 (6), 1742. doi:10.3390/nu12061742
Hwang, J. W., Park, J. H., Park, B. W., Kim, H., Kim, J. J., Sim, W. S., et al. (2021). Histochrome attenuates myocardial ischemia-reperfusion injury by inhibiting ferroptosis-induced cardiomyocyte death. Antioxidants (Basel) 10 (10), 1624. doi:10.3390/antiox10101624
Javkhedkar, A. A., Quiroz, Y., Rodriguez-Iturbe, B., Vaziri, N. D., Lokhandwala, M. F., and Banday, A. A. (2015). Resveratrol restored Nrf2 function, reduced renal inflammation, and mitigated hypertension in spontaneously hypertensive rats. Am. J. Physiol. Regul. Integr. Comp. Physiol. 308 (10), R840–R846. doi:10.1152/ajpregu.00308.2014
Jiang, M., Ni, J., Cao, Y., Xing, X., Wu, Q., and Fan, G. (2019). Astragaloside IV attenuates myocardial ischemia-reperfusion injury from oxidative stress by regulating succinate, lysophospholipid metabolism, and ROS scavenging system. Oxid. Med. Cell. Longev. 2019, 9137654. doi:10.1155/2019/9137654
Jiang, Q., Chen, X., Tian, X., Zhang, J., Xue, S., Jiang, Y., et al. (2022). Tanshinone I inhibits doxorubicin-induced cardiotoxicity by regulating Nrf2 signaling pathway. Phytomedicine. 106, 154439. doi:10.1016/j.phymed.2022.154439
Jiang, W., Song, J., Zhang, S., Ye, Y., Wang, J., and Zhang, Y. (2021). CTRP13 protects H9c2 cells against hypoxia/reoxygenation (H/R)-Induced injury via regulating the AMPK/Nrf2/ARE signaling pathway. Cell. Transpl. 30, 9636897211033275. doi:10.1177/09636897211033275
Jiang, Z., Wu, J., Ma, F., Jiang, J., Xu, L., Du, L., et al. (2020). MicroRNA-200a improves diabetic endothelial dysfunction by targeting KEAP1/NRF2. J. Endocrinol. 245 (1), 129–140. doi:10.1530/JOE-19-0414
Kloska, D., Kopacz, A., Piechota-Polanczyk, A., Nowak, W. N., Dulak, J., Jozkowicz, A., et al. (2019). Nrf2 in aging - focus on the cardiovascular system. Vasc. Pharmacol. 112, 42–53. doi:10.1016/j.vph.2018.08.009
Kolluru, G. K., Shackelford, R. E., Shen, X., Dominic, P., and Kevil, C. G. (2022). Sulfide regulation of cardiovascular function in health and disease. Nat. Rev. Cardiol., 1–17. doi:10.1038/s41569-022-00741-6
Krzemińska, J., Wronka, M., Młynarska, E., Franczyk, B., and Rysz, J. (2022). Arterial hypertension-oxidative stress and inflammation. Antioxidants (Basel) 11 (1), 172. doi:10.3390/antiox11010172
Kubben, N., Zhang, W., Wang, L., Voss, T. C., Yang, J., Qu, J., et al. (2016). Repression of the antioxidant NRF2 pathway in premature aging. Cell. 165 (6), 1361–1374. doi:10.1016/j.cell.2016.05.017
Lei, Q., Yi, T., and Chen, C. (2018). NF-κB-Gasdermin D (GSDMD) Axis couples oxidative stress and NACHT, LRR and PYD domains-containing protein 3 (NLRP3) inflammasome-mediated cardiomyocyte pyroptosis following myocardial infarction. Med. Sci. Monit. 24, 6044–6052. doi:10.12659/MSM.908529
Leyane, T. S., Jere, S. W., and Houreld, N. N. (2022). Oxidative stress in ageing and chronic degenerative pathologies: Molecular mechanisms involved in counteracting oxidative stress and chronic inflammation. Int. J. Mol. Sci. 23 (13), 7273. doi:10.3390/ijms23137273
Li, B., Kim, D. S., Yadav, R. K., Kim, H. R., and Chae, H. J. (2015). Sulforaphane prevents doxorubicin-induced oxidative stress and cell death in rat H9c2 cells. Int. J. Mol. Med. 36 (1), 53–64. doi:10.3892/ijmm.2015.2199
Li, H., Zhuang, W., Xiong, T., Park, W. S., Zhang, S., Zha, Y., et al. (2022). Nrf2 deficiency attenuates atherosclerosis by reducing LOX-1-mediated proliferation and migration of vascular smooth muscle cells. Atherosclerosis 347, 1–16. doi:10.1016/j.atherosclerosis.2022.02.025
Li, J., Cheng, Y., Li, R., Wu, X., Zheng, C., Shiu, P. H., et al. (2022). Protective effects of Amauroderma rugosum on doxorubicin-induced cardiotoxicity through suppressing oxidative stress, mitochondrial dysfunction, apoptosis, and activating akt/mTOR and Nrf2/HO-1 signaling pathways. Oxid. Med. Cell. Longev. 2022, 9266178. doi:10.1155/2022/9266178
Li, J., Zhao, C., Zhu, Q., Wang, Y., Li, G., Li, X., et al. (2021). Sweroside protects against myocardial ischemia-reperfusion injury by inhibiting oxidative stress and pyroptosis partially via modulation of the keap1/nrf2 Axis. Front. Cardiovasc. Med. 8, 650368. doi:10.3389/fcvm.2021.650368
Li, X., Liang, J., Qu, L., Liu, S., Qin, A., Liu, H., et al. (2022). Exploring the role of ferroptosis in the doxorubicin-induced chronic cardiotoxicity using a murine model. Chem. Biol. Interact. 363, 110008. doi:10.1016/j.cbi.2022.110008
Li, X., Zhang, Y., Ren, X., Wang, Y., Chen, D., Li, Q., et al. (2021). Ischemic microenvironment-responsive therapeutics for cardiovascular diseases. Adv. Mat. 33 (52), e2105348. doi:10.1002/adma.202105348
Li, Y., Feng, Y. F., Liu, X. T., Li, Y. C., Zhu, H. M., Sun, M. R., et al. (2021). Songorine promotes cardiac mitochondrial biogenesis via Nrf2 induction during sepsis. Redox Biol. 38, 101771. doi:10.1016/j.redox.2020.101771
Li, Y. Q., Jiao, Y., Liu, Y. N., Fu, J. Y., Sun, L. K., and Su, J. (2022). PGC-1α protects from myocardial ischaemia-reperfusion injury by regulating mitonuclear communication. J. Cell. Mol. Med. 26 (3), 593–600. doi:10.1111/jcmm.16236
Liang, E., Liu, X., Du, Z., Yang, R., and Zhao, Y. (2018). Andrographolide ameliorates diabetic cardiomyopathy in mice by blockage of oxidative damage and NF-κB-Mediated inflammation. Oxid. Med. Cell. Longev. 2018, 9086747. doi:10.1155/2018/9086747
Liao, W., Yang, W., Shen, Z., Ai, W., Pan, Q., Sun, Y., et al. (2021). Heme oxygenase-1 regulates ferrous iron and Foxo1 in control of hepatic gluconeogenesis. Diabetes 70 (3), 696–709. doi:10.2337/db20-0954
Liberale, L., Badimon, L., Montecucco, F., Lüscher, T. F., Libby, P., and Camici, G. G. (2022). Inflammation, aging, and cardiovascular disease: JACC review topic of the week. J. Am. Coll. Cardiol. 79 (8), 837–847. doi:10.1016/j.jacc.2021.12.017
Liberale, L., Ministrini, S., Carbone, F., Camici, G. G., and Montecucco, F. (2021). Cytokines as therapeutic targets for cardio- and cerebrovascular diseases. Basic Res. Cardiol. 116 (1), 23. doi:10.1007/s00395-021-00863-x
Lin, J. H., Yang, K. T., Lee, W. S., Ting, P. C., Luo, Y. P., Lin, D. J., et al. (2022). Xanthohumol protects the rat myocardium against ischemia/reperfusion injury-induced ferroptosis. Oxid. Med. Cell. Longev. 2022, 9523491. doi:10.1155/2022/9523491
Linna-Kuosmanen, S., Tomas Bosch, V., Moreau, P. R., Bouvy-Liivrand, M., Niskanen, H., Kansanen, E., et al. (2021). NRF2 is a key regulator of endothelial microRNA expression under proatherogenic stimuli. Cardiovasc. Res. 117 (5), 1339–1357. doi:10.1093/cvr/cvaa219
Liou, S. F., Nguyen, T. T. N., Hsu, J. H., Sulistyowati, E., Huang, S. E., Wu, B. N., et al. (2020). The preventive effects of xanthohumol on vascular calcification induced by vitamin D(3) plus nicotine. Antioxidants (Basel) 9 (10), 956. doi:10.3390/antiox9100956
Liu, C., Wu, Q. Q., Cai, Z. L., Xie, S. Y., Duan, M. X., Xie, Q. W., et al. (2019). Zingerone attenuates aortic banding-induced cardiac remodelling via activating the eNOS/Nrf2 pathway. J. Cell. Mol. Med. 23 (9), 6466–6478. doi:10.1111/jcmm.14540
Liu, J., Sun, Q., Sun, M., Lin, L., Ren, X., Li, T., et al. (2022). Melatonin alleviates PM(2.5)-triggered macrophage M1 polarization and atherosclerosis via regulating NOX2-mediated oxidative stress homeostasis. Free Radic. Biol. Med. 181, 166–179. doi:10.1016/j.freeradbiomed.2022.02.005
Lopes, R. A., Neves, K. B., Tostes, R. C., Montezano, A. C., and Touyz, R. M. (2015). Downregulation of nuclear factor erythroid 2-related factor and associated antioxidant genes contributes to redox-sensitive vascular dysfunction in hypertension. Hypertension 66 (6), 1240–1250. doi:10.1161/HYPERTENSIONAHA.115.06163
Luo, X., Zhou, J., Wang, Z., He, Y., Yu, L., Ma, S., et al. (2020). An inhibitor role of Nrf2 in the regulation of myocardial senescence and dysfunction after myocardial infarction. Life Sci. 259, 118199. doi:10.1016/j.lfs.2020.118199
Ma, A., Hong, J., Shanks, J., Rudebush, T., Yu, L., Hackfort, B. T., et al. (2019). Upregulating Nrf2 in the RVLM ameliorates sympatho-excitation in mice with chronic heart failure. Free Radic. Biol. Med. 141, 84–92. doi:10.1016/j.freeradbiomed.2019.06.002
Mauro, A. G., Mezzaroma, E., Toldo, S., Melendez, G., Franco, R. L., Lesnefsky, E. J., et al. (2022). NLRP3-mediated inflammation in cardio-oncology: Sterile yet harmful. Transl. Res. S1931-5244 (22), 00177–3. doi:10.1016/j.trsl.2022.08.004
Meephat, S., Prasatthong, P., Potue, P., Bunbupha, S., Pakdeechote, P., and Maneesai, P. (2021). Diosmetin ameliorates vascular dysfunction and remodeling by modulation of Nrf2/HO-1 and p-JNK/p-NF-κB expression in hypertensive rats. Antioxidants (Basel) 10 (9), 1487. doi:10.3390/antiox10091487
Meeran, M. F. N., Azimullah, S., Adeghate, E., and Ojha, S. (2021). Nootkatone attenuates myocardial oxidative damage, inflammation, and apoptosis in isoproterenol-induced myocardial infarction in rats. Phytomedicine. 84, 153405. doi:10.1016/j.phymed.2020.153405
Naeem, A., Ming, Y., Pengyi, H., Jie, K. Y., Yali, L., Haiyan, Z., et al. (2022). The fate of flavonoids after oral administration: A comprehensive overview of its bioavailability. Crit. Rev. Food Sci. Nutr. 62 (22), 6169–6186. doi:10.1080/10408398.2021.1898333
Nie, P., Meng, F., Zhang, J., Wei, X., and Shen, C. (2019). Astragaloside IV exerts a myocardial protective effect against cardiac hypertrophy in rats, partially via activating the Nrf2/HO-1 signaling pathway. Oxid. Med. Cell. Longev. 2019, 4625912. doi:10.1155/2019/4625912
Ogboo, B. C., Grabovyy, U. V., Maini, A., Scouten, S., van der Vliet, A., Mattevi, A., et al. (2022). Architecture of the NADPH oxidase family of enzymes. Redox Biol. 52, 102298. doi:10.1016/j.redox.2022.102298
Panda, P., Verma, H. K., Lakkakula, S., Merchant, N., Kadir, F., Rahman, S., et al. (2022). Biomarkers of oxidative stress tethered to cardiovascular diseases. Oxid. Med. Cell. Longev. 2022, 9154295. doi:10.1155/2022/9154295
Patel, K. P., Katsurada, K., and Zheng, H. (2022). Cardiorenal syndrome: The role of neural connections between the heart and the kidneys. Circ. Res. 130 (10), 1601–1617. doi:10.1161/CIRCRESAHA.122.319989
Qi, W., Boliang, W., Xiaoxi, T., Guoqiang, F., Jianbo, X., and Gang, W. (2020). Cardamonin protects against doxorubicin-induced cardiotoxicity in mice by restraining oxidative stress and inflammation associated with Nrf2 signaling. Biomed. Pharmacother. 122, 109547. doi:10.1016/j.biopha.2019.109547
Qin, S., Chen, J., Tanigawa, S., and Hou, D. X. (2013). Microarray and pathway analysis highlight Nrf2/ARE-mediated expression profiling by polyphenolic myricetin. Mol. Nutr. Food Res. 57 (3), 435–446. doi:10.1002/mnfr.201200563
Qin, S., Deng, F., Wu, W., Jiang, L., Yamashiro, T., Yano, S., et al. (2014). Baicalein modulates Nrf2/Keap1 system in both Keap1-dependent and Keap1-independent mechanisms. Arch. Biochem. Biophys. 559, 53–61. doi:10.1016/j.abb.2014.03.011
Rajendran, P., Alzahrani, A. M., Ahmed, E. A., and Veeraraghavan, V. P. (2021). Kirenol inhibits B[a]P-induced oxidative stress and apoptosis in endothelial cells via modulation of the Nrf2 signaling pathway. Oxid. Med. Cell. Longev. 2021, 5585303. doi:10.1155/2021/5585303
Rajesh, M., Mukhopadhyay, P., Bátkai, S., Patel, V., Saito, K., Matsumoto, S., et al. (2010). Cannabidiol attenuates cardiac dysfunction, oxidative stress, fibrosis, and inflammatory and cell death signaling pathways in diabetic cardiomyopathy. J. Am. Coll. Cardiol. 56 (25), 2115–2125. doi:10.1016/j.jacc.2010.07.033
Roy, P., Orecchioni, M., and Ley, K. (2022). How the immune system shapes atherosclerosis: Roles of innate and adaptive immunity. Nat. Rev. Immunol. 22 (4), 251–265. doi:10.1038/s41577-021-00584-1
Ruotsalainen, A. K., Inkala, M., Partanen, M. E., Lappalainen, J. P., Kansanen, E., Mäkinen, P. I., et al. (2013). The absence of macrophage Nrf2 promotes early atherogenesis. Cardiovasc. Res. 98 (1), 107–115. doi:10.1093/cvr/cvt008
Ruotsalainen, A. K., Lappalainen, J. P., Heiskanen, E., Merentie, M., Sihvola, V., Näpänkangas, J., et al. (2019). Nuclear factor E2-related factor 2 deficiency impairs atherosclerotic lesion development but promotes features of plaque instability in hypercholesterolaemic mice. Cardiovasc. Res. 115 (1), 243–254. doi:10.1093/cvr/cvy143
Sai, X., Li, Z., Deng, G., Wang, L., Xiaowu, W., Nasser, M. I., et al. (2022). Immunomodulatory effects of icariin in a myocardial infarction mouse model. Bioengineered 13 (5), 12504–12515. doi:10.1080/21655979.2022.2076453
Saito, R., Suzuki, T., Hiramoto, K., Asami, S., Naganuma, E., Suda, H., et al. (2016). Characterizations of three major cysteine sensors of Keap1 in stress response. Mol. Cell. Biol. 36 (2), 271–284. doi:10.1128/MCB.00868-15
Salerno, N., Salerno, L., Marino, F., Scalise, M., Chiefalo, A., Panuccio, G., et al. (2022). Myocardial regeneration protocols towards the routine clinical scenario: An unseemly path from bench to bedside. EClinicalMedicine 50, 101530. doi:10.1016/j.eclinm.2022.101530
Seo, Y., Park, J., Choi, W., Ju Son, D., Sung Kim, Y., Kim, M. K., et al. (2019). Antiatherogenic effect of resveratrol attributed to decreased expression of ICAM-1 (intercellular adhesion molecule-1). Arterioscler. Thromb. Vasc. Biol. 39 (4), 675–684. doi:10.1161/ATVBAHA.118.312201
Shanmugam, G., Challa, A. K., Litovsky, S. H., Devarajan, A., Wang, D., Jones, D. P., et al. (2019). Enhanced Keap1-Nrf2 signaling protects the myocardium from isoproterenol-induced pathological remodeling in mice. Redox Biol. 27, 101212. doi:10.1016/j.redox.2019.101212
Sharma, D., Gondaliya, P., Tiwari, V., and Kalia, K. (2019). Kaempferol attenuates diabetic nephropathy by inhibiting RhoA/Rho-kinase mediated inflammatory signalling. Biomed. Pharmacother. 109, 1610–1619. doi:10.1016/j.biopha.2018.10.195
Shi, L., Hao, Z., Zhang, S., Wei, M., Lu, B., Wang, Z., et al. (2018). Baicalein and baicalin alleviate acetaminophen-induced liver injury by activating Nrf2 antioxidative pathway: The involvement of ERK1/2 and PKC. Biochem. Pharmacol. 150, 9–23. doi:10.1016/j.bcp.2018.01.026
Shin, J. W., Chun, K. S., Kim, D. H., Kim, S. J., Kim, S. H., Cho, N. C., et al. (2020). Curcumin induces stabilization of Nrf2 protein through Keap1 cysteine modification. Biochem. Pharmacol. 173, 113820. doi:10.1016/j.bcp.2020.113820
Silva, D., Baião, D. D. S., Ferreira, V. F., and Paschoalin, V. M. F. (2022). Betanin as a multipath oxidative stress and inflammation modulator: A beetroot pigment with protective effects on cardiovascular disease pathogenesis. Crit. Rev. Food Sci. Nutr. 62 (2), 539–554. doi:10.1080/10408398.2020.1822277
Speciale, A., Anwar, S., Canali, R., Chirafisi, J., Saija, A., Virgili, F., et al. (2013). Cyanidin-3-O-glucoside counters the response to TNF-alpha of endothelial cells by activating Nrf2 pathway. Mol. Nutr. Food Res. 57 (11), 1979–1987. doi:10.1002/mnfr.201300102
Sthijns, M., Schiffers, P. M., Janssen, G. M., Lemmens, K. J. A., Ides, B., Vangrieken, P., et al. (2017). Rutin protects against H(2)O(2)-triggered impaired relaxation of placental arterioles and induces Nrf2-mediated adaptation in Human Umbilical Vein Endothelial Cells exposed to oxidative stress. Biochim. Biophys. Acta. Gen. Subj. 1861 (5), 1177–1189. doi:10.1016/j.bbagen.2017.03.004
Strom, J., and Chen, Q. M. (2017). Loss of Nrf2 promotes rapid progression to heart failure following myocardial infarction. Toxicol. Appl. Pharmacol. 327, 52–58. doi:10.1016/j.taap.2017.03.025
Sui, Y. B., Zhang, K. K., Ren, Y. K., Liu, L., and Liu, Y. (2020). The role of Nrf2 in astragaloside IV-mediated antioxidative protection on heart failure. Pharm. Biol. 58 (1), 1192–1198. doi:10.1080/13880209.2020.1849319
Sun, J., Yu, X., Huangpu, H., and Yao, F. (2019). Ginsenoside Rb3 protects cardiomyocytes against hypoxia/reoxygenation injury via activating the antioxidation signaling pathway of PERK/Nrf2/HMOX1. Biomed. Pharmacother. 109, 254–261. doi:10.1016/j.biopha.2018.09.002
Sun, Y., Zhou, S., Guo, H., Zhang, J., Ma, T., Zheng, Y., et al. (2020). Protective effects of sulforaphane on type 2 diabetes-induced cardiomyopathy via AMPK-mediated activation of lipid metabolic pathways and NRF2 function. Metabolism. 102, 154002. doi:10.1016/j.metabol.2019.154002
Syed, A. M., Ram, C., Murty, U. S., and Sahu, B. D. (2021). A review on herbal Nrf2 activators with preclinical evidence in cardiovascular diseases. Phytother. Res. 35 (9), 5068–5102. doi:10.1002/ptr.7137
Takaya, K., Suzuki, T., Motohashi, H., Onodera, K., Satomi, S., Kensler, T. W., et al. (2012). Validation of the multiple sensor mechanism of the Keap1-Nrf2 system. Free Radic. Biol. Med. 53 (4), 817–827. doi:10.1016/j.freeradbiomed.2012.06.023
Tan, X., Jiao, P. L., Sun, J. C., Wang, W., Ye, P., Wang, Y. K., et al. (2021). β-Arrestin1 reduces oxidative stress via Nrf2 activation in the rostral ventrolateral medulla in hypertension. Front. Neurosci. 15, 657825. doi:10.3389/fnins.2021.657825
Tan, Y., Wan, H. H., Sun, M. M., Zhang, W. J., Dong, M., Ge, W., et al. (2021). Cardamonin protects against lipopolysaccharide-induced myocardial contractile dysfunction in mice through Nrf2-regulated mechanism. Acta Pharmacol. Sin. 42 (3), 404–413. doi:10.1038/s41401-020-0397-3
Tanase, D. M., Apostol, A. G., Costea, C. F., Tarniceriu, C. C., Tudorancea, I., Maranduca, M. A., et al. (2022). Oxidative stress in arterial hypertension (HTN): The nuclear factor erythroid factor 2-related factor 2 (Nrf2) pathway, implications and future perspectives. Pharmaceutics 14 (3), 534. doi:10.3390/pharmaceutics14030534
Tarantini, S., Valcarcel-Ares, M. N., Yabluchanskiy, A., Tucsek, Z., Hertelendy, P., Kiss, T., et al. (2018). Nrf2 deficiency exacerbates obesity-induced oxidative stress, neurovascular dysfunction, blood-brain barrier disruption, neuroinflammation, amyloidogenic gene expression, and cognitive decline in mice, mimicking the aging phenotype. J. Gerontol. A Biol. Sci. Med. Sci. 73 (7), 853–863. doi:10.1093/gerona/glx177
Tejero, J., Shiva, S., and Gladwin, M. T. (2019). Sources of vascular nitric oxide and reactive oxygen species and their regulation. Physiol. Rev. 99 (1), 311–379. doi:10.1152/physrev.00036.2017
Torrente, L., and DeNicola, G. M. (2022). Targeting NRF2 and its downstream processes: Opportunities and challenges. Annu. Rev. Pharmacol. Toxicol. 62, 279–300. doi:10.1146/annurev-pharmtox-052220-104025
Tsao, C. W., Aday, A. W., Almarzooq, Z. I., Alonso, A., Beaton, A. Z., Bittencourt, M. S., et al. (2022). Heart disease and stroke statistics-2022 update: A report from the American heart association. Circulation 145 (8), e153–e639. doi:10.1161/CIR.0000000000001052
Ungvari, Z., Bailey-Downs, L., Gautam, T., Sosnowska, D., Wang, M., Monticone, R. E., et al. (2011a). Age-associated vascular oxidative stress, Nrf2 dysfunction, and NF-{kappa}B activation in the nonhuman primate Macaca mulatta. J. Gerontol. A Biol. Sci. Med. Sci. 66 (8), 866–875. doi:10.1093/gerona/glr092
Ungvari, Z., Bailey-Downs, L., Sosnowska, D., Gautam, T., Koncz, P., Losonczy, G., et al. (2011b). Vascular oxidative stress in aging: A homeostatic failure due to dysregulation of NRF2-mediated antioxidant response. Am. J. Physiol. Heart Circ. Physiol. 301 (2), H363–H372. doi:10.1152/ajpheart.01134.2010
Vors, C., Couillard, C., Paradis, M. E., Gigleux, I., Marin, J., Vohl, M. C., et al. (2018). Supplementation with resveratrol and curcumin does not affect the inflammatory response to a high-fat meal in older adults with abdominal obesity: A randomized, placebo-controlled crossover trial. J. Nutr. 148 (3), 379–388. doi:10.1093/jn/nxx072
Wang, D., Zhang, X., Li, D., Hao, W., Meng, F., Wang, B., et al. (2017). Kaempferide protects against myocardial ischemia/reperfusion injury through activation of the PI3K/Akt/GSK-3β pathway. Mediat. Inflamm. 2017, 5278218. doi:10.1155/2017/5278218
Wang, J., Toan, S., Li, R., and Zhou, H. (2020). Melatonin fine-tunes intracellular calcium signals and eliminates myocardial damage through the IP3R/MCU pathways in cardiorenal syndrome type 3. Biochem. Pharmacol. 174, 113832. doi:10.1016/j.bcp.2020.113832
Wang, L., Zeng, Y. Q., Gu, J. H., Song, R., Cang, P. H., Xu, Y. X., et al. (2022). Novel oral edaravone attenuates diastolic dysfunction of diabetic cardiomyopathy by activating the Nrf2 signaling pathway. Eur. J. Pharmacol. 920, 174846. doi:10.1016/j.ejphar.2022.174846
Wang, R., Yu, Z., Sunchu, B., Shoaf, J., Dang, I., Zhao, S., et al. (2017). Rapamycin inhibits the secretory phenotype of senescent cells by a Nrf2-independent mechanism. Aging Cell. 16 (3), 564–574. doi:10.1111/acel.12587
Wang, X., Chen, X., Zhou, W., Men, H., Bao, T., Sun, Y., et al. (2022). Ferroptosis is essential for diabetic cardiomyopathy and is prevented by sulforaphane via AMPK/NRF2 pathways. Acta Pharm. Sin. B 12 (2), 708–722. doi:10.1016/j.apsb.2021.10.005
Wang, Y., Yan, S., Liu, X., Deng, F., Wang, P., Yang, L., et al. (2022). PRMT4 promotes ferroptosis to aggravate doxorubicin-induced cardiomyopathy via inhibition of the Nrf2/GPX4 pathway. Cell. Death Differ. 29, 1982–1995. doi:10.1038/s41418-022-00990-5
Wei, Z., Shaohuan, Q., Pinfang, K., and Chao, S. (2022). Curcumin attenuates ferroptosis-induced myocardial injury in diabetic cardiomyopathy through the Nrf2 pathway. Cardiovasc. Ther. 2022, 3159717. doi:10.1155/2022/3159717
Wu, X., Zhou, X., Lai, S., Liu, J., and Qi, J. (2022). Curcumin activates Nrf2/HO-1 signaling to relieve diabetic cardiomyopathy injury by reducing ROS in vitro and in vivo. Faseb J. 36 (9), e22505. doi:10.1096/fj.202200543RRR
Wu, Y. T., Xie, L. P., Hua, Y., Xu, H. L., Chen, G. H., Han, X., et al. (2021). Tanshinone I inhibits oxidative stress-induced cardiomyocyte injury by modulating Nrf2 signaling. Front. Pharmacol. 12, 644116. doi:10.3389/fphar.2021.644116
Xiang, L., Zhang, Q., Chi, C., Wu, G., Lin, Z., Li, J., et al. (2020). Curcumin analog A13 alleviates oxidative stress by activating Nrf2/ARE pathway and ameliorates fibrosis in the myocardium of high-fat-diet and streptozotocin-induced diabetic rats. Diabetol. Metab. Syndr. 12, 1. doi:10.1186/s13098-019-0485-z
Xie, S., Deng, W., Chen, J., Wu, Q. Q., Li, H., Wang, J., et al. (2020). Andrographolide protects against adverse cardiac remodeling after myocardial infarction through enhancing Nrf2 signaling pathway. Int. J. Biol. Sci. 16 (1), 12–26. doi:10.7150/ijbs.37269
Xie, Y., Xu, E., Bowe, B., and Al-Aly, Z. (2022). Long-term cardiovascular outcomes of COVID-19. Nat. Med. 28 (3), 583–590. doi:10.1038/s41591-022-01689-3
Xin, Y., Bai, Y., Jiang, X., Zhou, S., Wang, Y., Wintergerst, K. A., et al. (2018). Sulforaphane prevents angiotensin II-induced cardiomyopathy by activation of Nrf2 via stimulating the Akt/GSK-3ß/Fyn pathway. Redox Biol. 15, 405–417. doi:10.1016/j.redox.2017.12.016
Xu, C., Liu, Y., Yang, J., Zhai, M., Fan, Z., Qiao, R., et al. (2022). Effects of berbamine against myocardial ischemia/reperfusion injury: Activation of the 5' adenosine monophosphate-activated protein kinase/nuclear factor erythroid 2-related factor pathway and changes in the mitochondrial state. Biofactors 48 (3), 651–664. doi:10.1002/biof.1820
Xu, H., Wan, X. D., Zhu, R. R., Liu, J. L., Liu, J. C., and Zhou, X. L. (2022). Keap-NRF2 signaling contributes to the Notch1 protected heart against ischemic reperfusion injury via regulating mitochondrial ROS generation and bioenergetics. Int. J. Biol. Sci. 18 (4), 1651–1662. doi:10.7150/ijbs.63297
Xu, L., Han, S., Chen, Z., Shen, C., Yao, Z., Wang, P., et al. (2022). Increase of oxidative stress by deficiency of the ALDH2/UCP2/nrf2 Axis exacerbates cardiac dysfunction in chronic kidney disease. Rev. Cardiovasc. Med. 23 (4), 127. doi:10.31083/j.rcm2304127
Xu, S., Ilyas, I., Little, P. J., Li, H., Kamato, D., Zheng, X., et al. (2021). Endothelial dysfunction in atherosclerotic cardiovascular diseases and beyond: From mechanism to pharmacotherapies. Pharmacol. Rev. 73 (3), 924–967. doi:10.1124/pharmrev.120.000096
Xu, X., Jin, K., Bais, A. S., Zhu, W., Yagi, H., Feinstein, T. N., et al. (2022). Uncompensated mitochondrial oxidative stress underlies heart failure in an iPSC-derived model of congenital heart disease. Cell. Stem Cell. 29 (5), 840–855.e7. e847. doi:10.1016/j.stem.2022.03.003
Yang, C. C., Chen, Y. T., Chen, C. H., Li, Y. C., Shao, P. L., Huang, T. H., et al. (2019). The therapeutic impact of entresto on protecting against cardiorenal syndrome-associated renal damage in rats on high protein diet. Biomed. Pharmacother. 116, 108954. doi:10.1016/j.biopha.2019.108954
Yao, D., Shi, B., Wang, S., Bao, L., Tan, M., Shen, H., et al. (2022). Isoliquiritigenin ameliorates ischemia-induced myocardial injury via modulating the Nrf2/HO-1 pathway in mice. Drug Des. devel. Ther. 16, 1273–1287. doi:10.2147/DDDT.S362754
Yao, H., Xie, Q., He, Q., Zeng, L., Long, J., Gong, Y., et al. (2022). Pretreatment with panaxatriol saponin attenuates mitochondrial apoptosis and oxidative stress to facilitate treatment of myocardial ischemia-reperfusion injury via the regulation of keap1/nrf2 activity. Oxid. Med. Cell. Longev. 2022, 9626703. doi:10.1155/2022/9626703
Yao, J., Zhang, B., Ge, C., Peng, S., and Fang, J. (2015). Xanthohumol, a polyphenol chalcone present in hops, activating Nrf2 enzymes to confer protection against oxidative damage in PC12 cells. J. Agric. Food Chem. 63 (5), 1521–1531. doi:10.1021/jf505075n
Ye, C., Geng, Z., Zhang, L. L., Zheng, F., Zhou, Y. B., Zhu, G. Q., et al. (2022). Chronic infusion of ELABELA alleviates vascular remodeling in spontaneously hypertensive rats via anti-inflammatory, anti-oxidative and anti-proliferative effects. Acta Pharmacol. Sin. 43, 2573–2584. doi:10.1038/s41401-022-00875-w
Yoon, S., Kim, M., Lee, H., Kang, G., Bedi, K., Margulies, K. B., et al. (2021). S-nitrosylation of histone deacetylase 2 by neuronal nitric oxide synthase as a mechanism of diastolic dysfunction. Circulation 143 (19), 1912–1925. doi:10.1161/CIRCULATIONAHA.119.043578
Younis, N. S., and Mohamed, M. E. (2021). Protective effects of myrrh essential oil on isoproterenol-induced myocardial infarction in rats through antioxidant, anti-inflammatory, Nrf2/HO-1 and apoptotic pathways. J. Ethnopharmacol. 270, 113793. doi:10.1016/j.jep.2021.113793
Yu, H., Chen, B., and Ren, Q. (2019). Baicalin relieves hypoxia-aroused H9c2 cell apoptosis by activating Nrf2/HO-1-mediated HIF1α/BNIP3 pathway. Artif. Cells Nanomed. Biotechnol. 47 (1), 3657–3663. doi:10.1080/21691401.2019.1657879
Yu, X., Cui, L., Zhang, Z., Zhao, Q., and Li, S. (2013). α-Linolenic acid attenuates doxorubicin-induced cardiotoxicity in rats through suppression of oxidative stress and apoptosis. Acta Biochim. Biophys. Sin. 45 (10), 817–826. doi:10.1093/abbs/gmt082
Yuan, M., Zhao, B., Jia, H., Zhang, C., and Zuo, X. (2021). Sinomenine ameliorates cardiac hypertrophy by activating Nrf2/ARE signaling pathway. Bioengineered 12 (2), 12778–12788. doi:10.1080/21655979.2021.2000195
Zeng, H., Wang, L., Zhang, J., Pan, T., Yu, Y., Lu, J., et al. (2021). Activated PKB/GSK-3β synergizes with PKC-δ signaling in attenuating myocardial ischemia/reperfusion injury via potentiation of NRF2 activity: Therapeutic efficacy of dihydrotanshinone-I. Acta Pharm. Sin. B 11 (1), 71–88. doi:10.1016/j.apsb.2020.09.006
Zhai, X., Lin, M., Zhang, F., Hu, Y., Xu, X., Li, Y., et al. (2013). Dietary flavonoid genistein induces Nrf2 and phase II detoxification gene expression via ERKs and PKC pathways and protects against oxidative stress in Caco-2 cells. Mol. Nutr. Food Res. 57 (2), 249–259. doi:10.1002/mnfr.201200536
Zhang, A., Tian, C., Gao, L., and Zucker, I. H. (2018). Bardoxolone activates cardiac Nrf2, increases antioxidant expression and lowers arterial pressure in rats with heart failure. FASEB J. 32, 903–911903. doi:10.1096/fasebj.2018.32.1_supplement.903.11
Zhang, L., Guo, Z., Wang, Y., Geng, J., and Han, S. (2019). The protective effect of kaempferol on heart via the regulation of Nrf2, NF-κβ, and PI3K/Akt/GSK-3β signaling pathways in isoproterenol-induced heart failure in diabetic rats. Drug Dev. Res. 80 (3), 294–309. doi:10.1002/ddr.21495
Zhang, L. M., Lv, S. S., Fu, S. R., Wang, J. Q., Liang, L. Y., Li, R. Q., et al. (2021a). Procyanidins inhibit fine particulate matter-induced vascular smooth muscle cells apoptosis via the activation of the Nrf2 signaling pathway. Ecotoxicol. Environ. Saf. 223, 112586. doi:10.1016/j.ecoenv.2021.112586
Zhang, M., Wei, L., Xie, S., Xing, Y., Shi, W., Zeng, X., et al. (2021b). Activation of Nrf2 by lithospermic acid ameliorates myocardial ischemia and reperfusion injury by promoting phosphorylation of AMP-activated protein kinase α (AMPKα). Front. Pharmacol. 12, 794982. doi:10.3389/fphar.2021.794982
Zhang, M., Xu, Y., and Jiang, L. (2020). Sulforaphane attenuates angiotensin II-induced human umbilical vein endothelial cell injury by modulating ROS-mediated mitochondrial signaling. Hum. Exp. Toxicol. 39 (5), 734–747. doi:10.1177/0960327119893414
Zhang, Q., Liu, J., Duan, H., Li, R., Peng, W., and Wu, C. (2021c). Activation of Nrf2/HO-1 signaling: An important molecular mechanism of herbal medicine in the treatment of atherosclerosis via the protection of vascular endothelial cells from oxidative stress. J. Adv. Res. 34, 43–63. doi:10.1016/j.jare.2021.06.023
Zhang, Q., Wang, L., Wang, S., Cheng, H., Xu, L., Pei, G., et al. (2022). Signaling pathways and targeted therapy for myocardial infarction. Signal Transduct. Target. Ther. 7 (1), 78. doi:10.1038/s41392-022-00925-z
Zhang, Q., Xue, T., Guan, J., Wang, W., Shi, J., Lu, J., et al. (2021d). Irigenin alleviates angiotensin II-induced oxidative stress and apoptosis in HUVEC cells by activating Nrf2 pathway. Drug Dev. Res. 82 (7), 999–1007.
Zhang, W. B., Lai, X., and Guo, X. F. (2021e). Activation of Nrf2 by miR-152 inhibits doxorubicin-induced cardiotoxicity via attenuation of oxidative stress, inflammation, and apoptosis. Oxid. Med. Cell. Longev. 2021, 8860883. doi:10.1155/2021/8860883
Zhang, Y., Huo, L., Wei, Z., Tang, Q., and Sui, H. (2022). Hotspots and Frontiers in inflammatory tumor microenvironment research: A scientometric and visualization analysis. Front. Pharmacol. 13, 862585. doi:10.3389/fphar.2022.862585
Zhang, Z., Yan, B., Li, Y., Yang, S., and Li, J. (2022c). Propofol inhibits oxidative stress injury through the glycogen synthase kinase 3 beta/nuclear factor erythroid 2-related factor 2/heme oxygenase-1 signaling pathway. Bioengineered 13 (1), 1612–1625. doi:10.1080/21655979.2021.2021062
Zhao, S., Ghosh, A., Lo, C. S., Chenier, I., Scholey, J. W., Filep, J. G., et al. (2018). Nrf2 deficiency upregulates intrarenal angiotensin-converting enzyme-2 and angiotensin 1-7 receptor expression and attenuates hypertension and nephropathy in diabetic mice. Endocrinology 159 (2), 836–852. doi:10.1210/en.2017-00752
Zhao, T. X., and Mallat, Z. (2019). Targeting the immune system in atherosclerosis: JACC state-of-the-art review. J. Am. Coll. Cardiol. 73 (13), 1691–1706. doi:10.1016/j.jacc.2018.12.083
Zheng, Z., Xian, Y., Jin, Z., Yao, F., Liu, Y., Deng, Y., et al. (2022). Rhaponticum carthamoides improved energy metabolism and oxidative stress through the SIRT6/Nrf2 pathway to ameliorate myocardial injury. Phytomedicine. 105, 154197. doi:10.1016/j.phymed.2022.154197
Zhou, S., Wang, J., Yin, X., Xin, Y., Zhang, Z., Cui, T., et al. (2018). Nrf2 expression and function, but not MT expression, is indispensable for sulforaphane-mediated protection against intermittent hypoxia-induced cardiomyopathy in mice. Redox Biol. 19, 11–21. doi:10.1016/j.redox.2018.07.014
Zhu, L., He, S., Huang, L., Ren, D., Nie, T., Tao, K., et al. (2022). Chaperone-mediated autophagy degrades Keap1 and promotes Nrf2-mediated antioxidative response. Aging Cell. 21 (6), e13616. doi:10.1111/acel.13616
Zhu, Y., Zhang, Y., Huang, X., Xie, Y., Qu, Y., Long, H., et al. (2019). Z-Ligustilide protects vascular endothelial cells from oxidative stress and rescues high fat diet-induced atherosclerosis by activating multiple NRF2 downstream genes. Atherosclerosis 284, 110–120. doi:10.1016/j.atherosclerosis.2019.02.010
Zhu, Z., Li, J., and Zhang, X. (2019). Astragaloside IV protects against oxidized low-density lipoprotein (ox-LDL)-Induced endothelial cell injury by reducing oxidative stress and inflammation. Med. Sci. Monit. 25, 2132–2140. doi:10.12659/MSM.912894
Keywords: Nrf2, natural products, cardiovascular diseases, oxidative stress, citespace
Citation: Wu X, Wei J, Yi Y, Gong Q and Gao J (2022) Activation of Nrf2 signaling: A key molecular mechanism of protection against cardiovascular diseases by natural products. Front. Pharmacol. 13:1057918. doi: 10.3389/fphar.2022.1057918
Received: 30 September 2022; Accepted: 24 November 2022;
Published: 08 December 2022.
Edited by:
Zeba Farooqui, University of Illinois at Chicago, United StatesReviewed by:
Naureen Fatima, University of Texas Health Science Center at Houston, United StatesRazia Mohammad, University of Houston, United States
Copyright © 2022 Wu, Wei, Yi, Gong and Gao. This is an open-access article distributed under the terms of the Creative Commons Attribution License (CC BY). The use, distribution or reproduction in other forums is permitted, provided the original author(s) and the copyright owner(s) are credited and that the original publication in this journal is cited, in accordance with accepted academic practice. No use, distribution or reproduction is permitted which does not comply with these terms.
*Correspondence: Jianmei Gao, Z2Fvamlhbm1laUB6bXUuZWR1LmNu