- Hubei Engineering Technology Research Center of Chinese Materia Medica Processing, College of Pharmacy, Hubei University of Chinese Medicine, Wuhan, China
Osteoarthritis (OA) is a common degenerative disease of the joints. It is primarily caused by age, obesity, mechanical damage, genetics, and other factors, leading to cartilage degradation, synovial inflammation, and subchondral sclerosis with osteophyte formation. Many recent studies have reported that glycolysis disorders are related lead to OA. There is a close relationship between glycolysis and OA. Because of their hypoxic environment, chondrocytes are highly dependent on glycolysis, their primary energy source for chondrocytes. Glycolysis plays a vital role in OA development. In this paper, we comprehensively summarized the abnormal expression of related glycolytic enzymes in OA, including Hexokinase 2 (HK2), Pyruvate kinase 2 (PKM2), Phosphofructokinase-2/fructose-2, 6-Bisphosphatase 3 (PFKFB3), lactate dehydrogenase A (LDHA), and discussed the potential application of glycolysis in treating OA. Finally, the natural products that can regulate the glycolytic pathway were summarized. Targeting glucose transporters and rate-limiting enzymes to glycolysis may play an essential role in treating OA.
Introduction
Osteoarthritis (OA) is a degenerative joint disease characterized by articular cartilage destruction and synovial inflammation (Shen et al., 2012). OA mostly develops in the knee, followed by the hand and hip joints (Xu et al., 2021a). OA affects the entire joint structure, including articular cartilage, subchondral bone, meniscus, synovium, ligament, and sub-patellar fat pad (Pereira et al., 2015). It is characterized by pathological changes in joint structure, including cartilage degradation, synovial inflammation, and subchondral sclerosis with osteophyte formation (Zheng et al., 2021). It is featured by joint pain and stiffness, which reduces the quality of life (Yunus et al., 2020). Recent reports have shown that about 240 million people suffer from OA worldwide, and the incidence of OA among women is about 1.5 times that of men (Neogi & Zhang, 2013). Age, obesity, mechanical injury, and heredity are essential factors promoting OA development. However, the pathogenesis of OA remains unclear (Johnson and Hunter, 2014). Studies have shown that the incidence of the disease is high in the age group of 50–54 years, and the prevalence of men and women over 60 years old is 10% and 18%, respectively (Neogi and Zhang, 2013). Obesity can lead to joint overload and promote OA development. In addition, mechanical damage to articular cartilage and subchondral bone promotes OA development (Balahura et al., 2020; Gao et al., 2021). Part of OA is attributable to inheritance, with hereditary OA due to mutations in collagen type II, IX, or XI (Kannu et al., 2010).
Modern treatment of OA primarily includes surgical and non-surgical treatments (Tejero et al., 2021). Surgical treatments include peri-knee osteotomy and joint replacement (Madry, 2022). It can relieve pain, correct joint deformity, and restore joint function. However, problems exist, including high operation costs, high risk, and many other complications such as joint loss and infection. Non-surgical treatment includes standard treatment and medication, including rational exercise, weight control, and physical therapy. Drug therapy includes oral and topical administration and intra-articular injection (Rodriguez-Merchan, 2018). Oral non-steroidal anti-inflammatory drugs (NSAIDs) and opioids can relieve OA pain effectively (Paterson and Gates, 2019). However, long-term use could cause stomach and cardiovascular problems and kidney and liver damage. Topical application of NSAIDs or Chinese herbal medicines can provide anti-inflammatory and pain-relieving effects, although there are occasional adverse reactions such as local skin rash, burning sensation and itching. For patients with poor response to oral and topical drug treatment, intra-articular irrigation and injection therapy can be used, and the drug can act directly on the lesion site to relieve pain and improve joint function (Hameed and Ihm, 2012). However, repeated treatment can cause damage to the tendon and ligament of the articular cartilage. Therefore, developing alternative drugs with good efficacy and fewer side effects for treating OA is vital.
Modern studies have shown that metabolic disorders contribute to OA development (Sellam and Berenbaum, 2013). Regulating metabolism plays a key role in the treatment of OA (Mobasheri et al., 2017). There is a close relationship between OA and lipid metabolism (Cao et al., 2022), and massive lipid deposition is observed in osteoarthritic chondrocytes (Lippiello et al., 1991; Gkretsi et al., 2011). Some adipokines can directly affect joint health and regulate inflammation to promote OA progression (Wang & He, 2018). Amino acid metabolism is thought to be involved in the pathogenesis of OA (Li et al., 2016b), and a variety of amino acids are abnormally expressed in OA chondrocytes (Mickiewicz et al., 2015; Zheng et al., 2017). Unlike most tissues, articular cartilage has no blood vessels, nerves, or lymphatic vessels and is composed mainly of extracellular matrix (ECM) and chondrocytes (Wang et al., 2020b). ECM mainly consists of water, collagen, and proteoglycans (Sophia Fox et al., 2009). Studies have shown that the primary energy source of chondrocytes is glycolysis (Lane et al., 2015), and disorders of glycolytic metabolism can lead to chondrocyte hypertrophy and extracellular matrix degradation, promoting OA development (Kudelko et al., 2016). Generally, in healthy joints, chondrocytes are in metabolic balance (Wang et al., 2020b). In OA joints or inflammatory environments, chondrocytes undergo metabolic reprogramming, enhancing the glycolytic pathway. Glucose is transported into chondrocytes by the glucose transporter 1 (GLUT1), the first rate-limiting step in glycolysis (Peansukmanee et al., 2009). Glycolysis involves a variety of enzymes and continuous enzymatic reactions. HK2, PKM2, PFKFB3, and LDHA are abnormally expressed in OA chondrocytes (Errea et al., 2016; Qu et al., 2016; Wang et al., 2020c). These enzymes may serve as potential targets for OA treatment. Lactate, a metabolite of glycolysis, can block metabolic reprogramming and pro-inflammatory signaling pathways that play a pro-inflammatory role (Wang et al., 2020a). In addition, histone modifications reduce M1 macrophage activation and promote M2 macrophage polarization (Zhang et al., 2019; Ivashkiv, 2020). It regulates inflammation through a variety of mechanisms. Accumulating evidence suggests that the metabolic shift to glycolysis enables cells to gain energy and is vital for activating immune responses and inflammatory pathways in OA (Loftus and Finlay, 2016). Therefore, targeting the glycolytic switch, especially the glucose transporters and rate-limiting enzymes involved in this metabolic reprogramming, could be a massive breakthrough in OA therapy. Studies have found that natural products could regulate glycolysis (Duya et al., 2022; Kooshki et al., 2022), interfere with chondrocyte metabolic reprogramming, improve chondrocyte viability, and inhibit OA development. Moreover, natural products have the advantages of low toxicity, low cost and multiple targets (Gao et al., 2021).
This paper discussed the rate-limiting enzymes and metabolites involved in chondrocyte glycolysis that may have significant breakthroughs in OA treatment. In addition, we systematically summarized the natural products that can regulate glycolysis. The aim was to develop alternative drugs to effectively treat OA with fewer side effects.
Glycolytic metabolism
During glycolysis, glucose molecules are taken up through the GLUT1. One glucose molecule is converted into two pyruvate molecules. These two pyruvate molecules relate to other metabolic pathways. They can enter mitochondria under aerobic conditions, where they are converted into acetyl CoA by pyruvate dehydrogenase complex and then completely metabolized into CO2 by the TCA cycle, generating NADH and reducing FADH2 to oxidative phosphorylation (OXPHOS) (Li et al., 2022b). Under anaerobic conditions, pyruvate is converted to lactate in the cytoplasm to regenerate NAD+, called anaerobic glycolysis (Zheng et al., 2021). Lactate formation from pyruvate under normoxic conditions is known as aerobic glycolysis or the Warburg phenomenon (Li et al., 2022a). High-throughput glycolysis occurs primarily in the cytoplasm, and lactate production from pyruvate is driven by lactate dehydrogenase (LDH) (Sotelo-Hitschfeld et al., 2012). LDH functions as a tetramer of LDHA and LDHB subunits. LDHB facilitates the conversion of lactate to pyruvate. LDHA is a rate-limiting enzyme with a high affinity for pyruvate and catalyzes lactate production from pyruvate (Feng et al., 2018). Since ATP production by glycolysis is much less efficient than OXPHOS, ATP production and lactate accumulation are reduced when OXPHOS is inhibited (Pucino et al., 2017).
Osteoarthritis and glycolytic metabolic disorders
OA is the most common joint disease. OA has long been considered a degenerative disease caused by daily wear and tear of joints, primarily due to mechanical factors (Ahmad et al., 2020). However, recent evidence has shown that OA is a multifactorial disease. The development of OA involves mechanical, genetic, metabolic and inflammatory processes (Chen et al., 2017). During OA, inflammation is primarily driven by damage-associated molecular patterns (DAMPs). DAMPs are released in extracellular mediators after cell stress or injury and interact with pathogen-recognition receptors (PRRs), including Toll-like receptors (TLRs) (Ahmad et al., 2020; Lambert et al., 2020) and the receptor for advanced glycation end products (RAGE) to stimulate macrophage and chondrocyte activation (Xie et al., 2013; Lambert et al., 2020; Suzuki et al., 2022). Moreover, they produce inflammatory cytokines and chemokines (Rigoglou and Papavassiliou, 2013; Choi et al., 2019; Rai et al., 2022). The generated inflammatory mediators stimulate cartilage-degrading enzyme production and the recruitment of inflammatory cells (Berenbaum and Walker, 2020; Molnar et al., 2021), inhibit the synthesis of proteoglycan and collagen, regulate chondrocyte apoptosis, and promote joint degradation (Ridnour et al., 2007; Abramson, 2008). The degree of inflammation in OA is relatively low, but inflammation is considered an essential component of OA pathology and plays the driving role in joint destruction and OA progression (Siebuhr et al., 2016). OA is now regarded as a metabolically related disease. The establishment and OA development are associated with inflammation and metabolic changes (Berenbaum et al., 2017). Metabolism plays a vital role in OA development.
In OA, low-grade inflammation causes chondrocytes to be hypoxic, and energy metabolism changes from a resting regulated state to a metabolically active state (Kim et al., 2015). At this time, glycolysis levels increase to meet the energy requirements of chondrocytes (Maneiro et al., 2003). The primary energy source of OA chondrocytes is glycolysis (Li et al., 2020c). Articular cartilage, unlike most tissues, has no blood, nerve, or lymphatic vessels. The nutrients are provided by synovial fluid in the joints. Compared to plasma, oxygen and glucose are less available (Mobasheri et al., 2008). Its environment is relatively anoxic, and the energy produced by oxidative phosphorylation (OXPHOS) is low (Zhai, 2019). Glycolysis is a rapid process of ATP production (Vazquez et al., 2010). Therefore, chondrocytes are heavily dependent on glycolysis, and disorders of glycolytic metabolism can lead to chondrocyte hypertrophy and ECM degradation. This cellular metabolism is essential for energy balance and may also be critical for cell function and signaling changes (Kim, 2018). Increasing evidence indicates that metabolic disorders are the cause of OA (Sellam and Berenbaum, 2013). Therefore, regulating cell metabolism is crucial to prevent and treating OA.
Targeted regulation of glycolytic metabolism to relieve osteoarthritis
Despite ongoing efforts to understand the pathogenesis and treatment of OA, to date, there is no cure for OA because cartilage is difficult to recover after being damaged (Xu et al., 2021b). The main objectives of modern OA treatment are to reduce pain, improve or maintain joint function, increase joint strength, and prevent further OA development (Xu et al., 2019). Clinically, approaches to treating OA have limitations in efficacy and long-term safety (Messina et al., 2019). Therefore, there is an urgent need to develop alternative drugs with few side effects that can effectively stop the progression of OA. Glycolysis plays a crucial role in OA. Its process includes a variety of enzymes and continuous enzymatic reactions, among which glucose import, hexokinase, PFKFB3, lactate export, and other enzymes play essential roles in glycolysis (Eisenberg et al., 2020). These enzymes and processes may also be involved in OA pathogenesis. Figure 1 summarizes the glycolytic metabolic pathways of OA. Therefore, targeting glycolysis, particularly glucose transporters and regulatory enzymes involved in regulating OA metabolism, might lead to significant breakthroughs in OA therapy. Table 1 summarizes the critical targets of OA pathogenesis in glycolysis. Recent studies have shown that some natural products regulate glycolysis (Li et al., 2022b). Intervention in the glycolytic process is an accurate and feasible way to prevent and treat OA (Dong et al., 2022), and Table 2 lists natural products that could interfere with glycolysis. These natural products might be potential drugs to inhibit OA development.
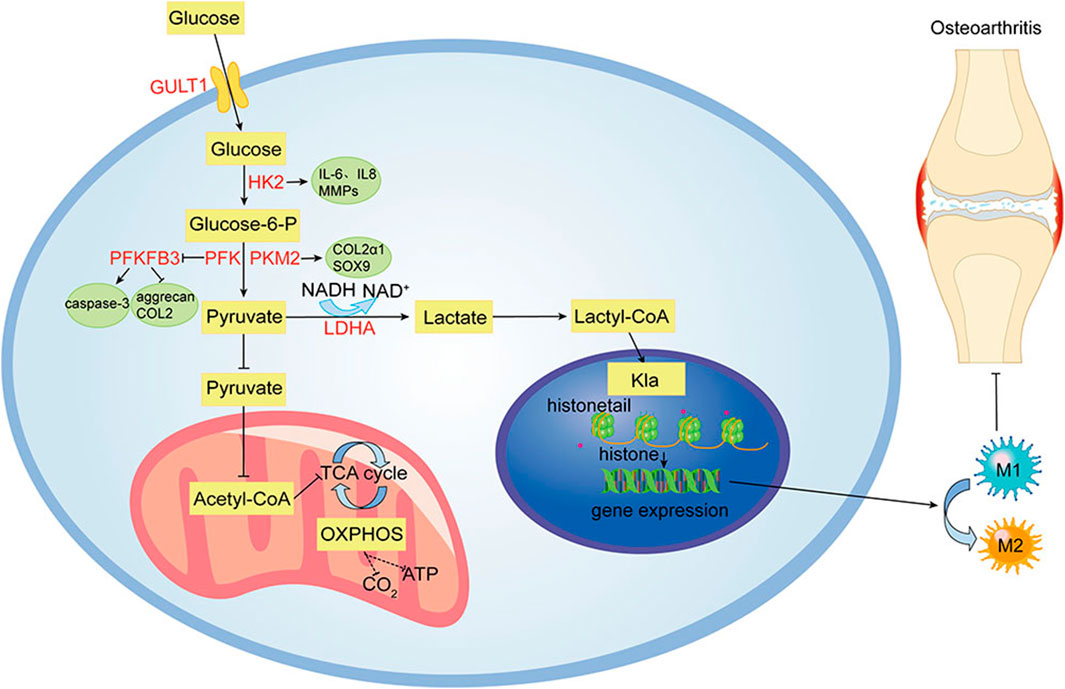
FIGURE 1. Glycolytic metabolism pathways of OA. Under hypoxic and inflammatory conditions in OA joints, cellular glycolysis is enhanced for rapid energy production. Many enzymes involved in glycolysis, including HK2, PKM2, PFKFB3, and LDHA, are abnormally expressed in OA chondrocytes. Lactate, a metabolite of glycolysis, reduces M1 macrophage activation and promotes M2 macrophage polarization by modifying histones.
GLUT1
Glucose is the main energy substrate for chondrocytes and the main precursor for glycosaminoglycan synthesis. When glucose levels rise, chondrocytes, if unable to adjust, absorb more glucose and produce more reactive oxygen species. Increased extracellular glucose levels also lead to increased production of AGEs, leading to cartilage damage. Glucose import is the first rate-limiting step in chondrocyte glycolysis (Mobasheri et al., 2002). Glucose is transported into chondrocytes by GLUT1, which is upregulated in hypoxia and glucose deprivation and decreased in high-glucose environments. GLUT1 improves the ability to take up glucose under hypoxic conditions and balances glucose levels in cells (Peansukmanee et al., 2009). Articular cartilage lacking the GLUT1 gene shows cytopenia and proteoglycan loss, which can seriously aggravate OA injury. Consistently elevated GLUT1 expression degrades cartilage by increasing glucose uptake and producing excessive AGEs (Rasheed et al., 2011). It might play a potential role in OA pathogenesis.
HK2
HK2 is the first rate-limiting enzyme in glycolysis, which can catalyze the conversion of glucose to glucose-6-phosphate (G-6-P) and participate in the main glycolysis pathway (Bao et al., 2022). A subtype of HKs, HK2 is a key regulator of glucose metabolism, promoting the conversion of glucose metabolism from oxidative phosphorylation to aerobic glycolysis (Feng et al., 2020). The expression level of HK2 in OA synovial tissue (FLS) is higher than that in the normal group. Overexpression of HK2 results in increased RNA expression levels of pro-inflammatory cytokines, including IL-6, IL-8, and MMPs in OA FLS. This suggests that HK2 has a potential therapeutic effect on OA (Bustamante et al., 2018). Identifying metabolic targets in the development of OA therapy is of great significance.
PKM2
PKM2 is a rate-limiting enzyme in glycolysis. It has been reported that PKM2 is upregulated, and ATP production is decreased in OA chondrocytes. Inhibition of PKM2 can prevent the proliferation of OA chondrocytes, promote cell apoptosis, and reduce the expression levels of COL2α1 and SOX9. When PKM2 is overexpressed in OA chondrocytes, it leads to lactate accumulation and the formation of an acidic microenvironment (Yang et al., 2018a). The acidic microenvironment inhibits matrix synthesis in chondrocytes and may promote cartilage degeneration in OA (High et al., 2019), suggesting PKM2 might be a therapeutic target for OA (Yang et al., 2018a).
PFKFB3
PFKFB3 is a crucial stimulator of glycolysis. PFKFB3 is down-regulated in human OA cartilage tissues, and human chondrocytes are stimulated with TNF-α or IL-1β. PFKFB3 overexpression ameliorates the damaged glycolytic process in OA cartilage. In addition, PFKFB3 can improve the cell viability of chondrocytes, reduce the activation of caspase-3, and promote the expression of aggrecan and type II collagen, which is a potential target for OA prevention and treatment (Qu et al., 2016).
LDHA
LDHA is closely related to aerobic glycolysis. It is essential for lactate production (Errea et al., 2016; Kaushik et al., 2019). Overexpression of LDHA disrupts metabolic homeostasis in synovial tissue, which directly upregulates lactate secretion. Extracellular lactate can stimulate histone lactation. Hypoxia enhances glycolysis, increases intracellular lactate levels, and increases histone lysine lactylation (Kla) levels (Pan et al., 2022). It has been found that lactate reduces M1 macrophage activation and promotes M2 macrophage polarization by modifying histones in the inflammatory response (Zhang et al., 2019; Ivashkiv, 2020). Further studies on histone lactation may lead to new therapeutic targets for OA treatment and strategies for resolving inflammation (Zhou et al., 2022). The activity and expression of LDH are significantly increased in primary chondrocytes treated with IL-1β. The mRNA sequencing results showed that IL-1β induction significantly increased the expression of genes involved in glycolysis, including PKM2, LDHA, and HK2 (Arra et al., 2020). In addition, the levels of LDHA and lactate in synovial fluid of TMJOA patients are significantly higher than those of controls (Li et al., 2020a). LDHA can promote ROS formation in chondrocytes in an inflammatory state. Inhibition of LDHA activity is an effective therapeutic target for OA.
Conclusion
In conclusion, improving the metabolic environment is conducive to treating OA, primarily by regulating glycolysis. Articular cartilage lacking GLUT1 exhibits cytopenia and proteoglycan loss. Inhibition of PKM2 could prevent the proliferation of OA chondrocytes and reduce the expression levels of COL2α1 and SOX9. PFKFB3 can improve the cell viability of chondrocytes and promote the expression of aggrecan and collagen type II. LDHA is closely related to glycolysis and plays a vital role in chondrocyte proliferation and apoptosis. Lactate, a byproduct of glycolysis, is a vital signal determining inflammation. Lactate can inhibit inflammatory responses by blocking pro-inflammatory signaling pathways. In addition, lactate exerts anti-inflammatory effects by promoting the polarization of M2 macrophages after modification of histone lactylation. Further studies of lactate may identify new therapeutic targets for treating OA and resolving inflammation.
Many researchers are interested in the role of glycolysis in OA pathogenesis. However, comprehensive details of its specific regulatory mechanisms and signaling pathways are yet to be studied. In addition, many natural products have been shown to regulate glycolysis, but there are few reports that natural products inhibit OA via glycolysis, which could provide a new strategy for OA treatment. Targeting glucose transporter proteins and rate-limiting enzymes in glycolysis may have a tremendous breakthrough in OA treatment. By discussing glycolysis and OA, we believe that targeting glycolysis is an important strategy to treat OA. And natural products have great advantages for improving OA.
Author contributions
CT conceived and wrote the outline of the manuscript. LL and JH collected and screened the literature. XL and KX directed and improved the content of this manuscript. All authors have read and approved the final manuscript.
Funding
This work was supported by Hubei Provincial Central Government Guided Local Science and Technology Development Special Project “Traditional Chinese Herbal Medicine Properties and Quality Evaluation Platform” (2020ZYYD030).
Conflict of interest
The authors declare that the research was conducted in the absence of any commercial or financial relationships that could be construed as a potential conflict of interest.
Publisher’s note
All claims expressed in this article are solely those of the authors and do not necessarily represent those of their affiliated organizations, or those of the publisher, the editors and the reviewers. Any product that may be evaluated in this article, or claim that may be made by its manufacturer, is not guaranteed or endorsed by the publisher.
References
Abramson, S. B. (2008). Osteoarthritis and nitric oxide. Osteoarthr. Cartil. 16 (2), S15–S20. doi:10.1016/s1063-4584(08)60008-4
Ahmad, N., Ansari, M. Y., and Haqqi, T. M. (2020). Role of iNOS in osteoarthritis: Pathological and therapeutic aspects. J. Cell. Physiol. 235, 6366–6376. doi:10.1002/jcp.29607
Arra, M., Swarnkar, G., Ke, K., Otero, J. E., Ying, J., Duan, X., et al. (2020). LDHA-mediated ROS generation in chondrocytes is a potential therapeutic target for osteoarthritis. Nat. Commun. 11, 3427. doi:10.1038/s41467-020-17242-0
Balahura, L. R., Selaru, A., Dinescu, S., and Costache, M. (2020). Inflammation and inflammasomes: Pros and cons in tumorigenesis. J. Immunol. Res. 2020, 2549763. doi:10.1155/2020/2549763
Ban, D., Hua, S., Zhang, W., Shen, C., Miao, X., and Liu, W. (2019). Costunolide reduces glycolysis-associated activation of hepatic stellate cells via inhibition of hexokinase-2. Cell. Mol. Biol. Lett. 24, 52. doi:10.1186/s11658-019-0179-4
Bao, C., Zhu, S., Song, K., and He, C. (2022). HK2: A potential regulator of osteoarthritis via glycolytic and non-glycolytic pathways. Cell Commun. Signal. 20, 132. doi:10.1186/s12964-022-00943-y
Berenbaum, F., Griffin, T. M., and Liu-Bryan, R. (2017). Review: Metabolic regulation of inflammation in osteoarthritis. Arthritis Rheumatol. 69, 9–21. doi:10.1002/art.39842
Berenbaum, F., and Walker, C. (2020). Osteoarthritis and inflammation: A serious disease with overlapping phenotypic patterns. Postgrad. Med. 132, 377–384. doi:10.1080/00325481.2020.1730669
Bustamante, M. F., Oliveira, P. G., Garcia-Carbonell, R., Croft, A. P., Smith, J. M., Serrano, R. L., et al. (2018). Hexokinase 2 as a novel selective metabolic target for rheumatoid arthritis. Ann. Rheum. Dis. 77, 1636–1643. doi:10.1136/annrheumdis-2018-213103
Cao, X., Cui, Z., Ding, Z., Chen, Y., Wu, S., Wang, X., et al. (2022). An osteoarthritis subtype characterized by synovial lipid metabolism disorder and fibroblast-like synoviocyte dysfunction. J. Orthop. Transl. 33, 142–152. doi:10.1016/j.jot.2022.02.007
Chen, D., Shen, J., Zhao, W., Wang, T., Han, L., Hamilton, J. L., et al. (2017). Osteoarthritis: Toward a comprehensive understanding of pathological mechanism. Bone Res. 5, 16044. doi:10.1038/boneres.2016.44
Chen, M., Liu, M., Luo, Y., Cao, J., Zeng, F., Yang, L., et al. (2022). Celastrol protects against cerebral ischemia/reperfusion injury in mice by inhibiting glycolysis through targeting HIF-1α/PDK1 Axis. Oxid. Med. Cell. Longev. 2022, 7420507. doi:10.1155/2022/7420507
Choi, M. C., Jo, J., Park, J., Kang, H. K., and Park, Y. (2019). NF-κB signaling pathways in osteoarthritic cartilage destruction. Cells 8. doi:doi:10.3390/cells8070734
Dai, W., Wang, F., Lu, J., Xia, Y., He, L., Chen, K., et al. (2015). By reducing hexokinase 2, resveratrol induces apoptosis in HCC cells addicted to aerobic glycolysis and inhibits tumor growth in mice. Oncotarget 6, 13703–13717. doi:10.18632/oncotarget.3800
Dai, Y., Liu, Y., Li, J., Jin, M., Yang, H., and Huang, G. (2022). Shikonin inhibited glycolysis and sensitized cisplatin treatment in non-small cell lung cancer cells via the exosomal pyruvate kinase M2 pathway. Bioengineered 13, 13906–13918. doi:10.1080/21655979.2022.2086378
Dong, X.-R., Hu, M.-J., Pan, H.-X., Li, K.-F., and Cui, Y.-L. (2022). Chondrogenic differentiation of rat bone marrow mesenchymal stem cells induced by puerarin and tetrandrine. Acupunct. Herb. Med. 2, 130–138. doi:10.1097/hm9.0000000000000031
Duya, P. A., Chen, Y., Bai, L., Li, Z., Li, J., Chai, R., et al. (2022). Nature products of traditional Chinese medicine provide new ideas in γδT cell for tumor immunotherapy. Acupunct. Herb. Med. 2, 78–83. doi:10.1097/hm9.0000000000000032
Eisenberg, L., Eisenberg-Bord, M., Eisenberg-Lerner, A., and Sagi-Eisenberg, R. (2020). Metabolic alterations in the tumor microenvironment and their role in oncogenesis. Cancer Lett. 484, 65–71. doi:10.1016/j.canlet.2020.04.016
Errea, A., Cayet, D., Marchetti, P., Tang, C., Kluza, J., Offermanns, S., et al. (2016). Lactate inhibits the pro-inflammatory response and metabolic reprogramming in murine macrophages in a GPR81-independent manner. PloS one 11, e0163694. doi:10.1371/journal.pone.0163694
Feng, J., Li, J., Wu, L., Yu, Q., Ji, J., Wu, J., et al. (2020). Emerging roles and the regulation of aerobic glycolysis in hepatocellular carcinoma. J. Exp. Clin. Cancer Res. 39, 126. doi:10.1186/s13046-020-01629-4
Feng, Y., Xiong, Y., Qiao, T., Li, X., Jia, L., and Han, Y. (2018). Lactate dehydrogenase A: A key player in carcinogenesis and potential target in cancer therapy. Cancer Med. 7, 6124–6136. doi:10.1002/cam4.1820
Gao, F., Li, M., Liu, W. B., Zhou, Z. S., Zhang, R., Li, J. L., et al. (2015). Epigallocatechin gallate inhibits human tongue carcinoma cells via HK2-mediated glycolysis. Oncol. Rep. 33, 1533–1539. doi:10.3892/or.2015.3727
Gao, M., Chen, C., Zhang, Q., Bian, J., Qin, L., and Bao, L. (2021). Research progress on the antiosteoarthritic mechanism of action of natural products. Evid. Based. Complement. Altern. Med. 2021, 7714533. doi:10.1155/2021/7714533
Gkretsi, V., Simopoulou, T., and Tsezou, A. (2011). Lipid metabolism and osteoarthritis: Lessons from atherosclerosis. Prog. Lipid Res. 50, 133–140. doi:10.1016/j.plipres.2010.11.001
Hameed, F., and Ihm, J. (2012). Injectable medications for osteoarthritis. PM R J. Inj. Funct. rehabilitation 4, S75–S81. doi:10.1016/j.pmrj.2012.02.010
Han, J. H., Kim, M., Kim, H. J., Jang, S. B., Bae, S. J., Lee, I. K., et al. (2021). Targeting lactate dehydrogenase A with catechin resensitizes SNU620/5FU gastric cancer cells to 5-fluorouracil. Int. J. Mol. Sci. 22, 5406. doi:10.3390/ijms22105406
Han, S., Yang, S., Cai, Z., Pan, D., Li, Z., Huang, Z., et al. (2015). Anti-Warburg effect of rosmarinic acid via miR-155 in gastric cancer cells. Drug Des. devel. Ther. 9, 2695–2703. doi:10.2147/dddt.S82342
High, R. A., Ji, Y., Ma, Y. J., Tang, Q., Murphy, M. E., Du, J., et al. (2019). In vivo assessment of extracellular pH of joint tissues using acidoCEST-UTE MRI. Quant. Imaging Med. Surg. 9, 1664–1673. doi:10.21037/qims.2019.08.11
Huang, B., Wang, Q., Jiang, L., Lu, S., Li, C., Xu, C., et al. (2022). Shikonin ameliorated mice colitis by inhibiting dimerization and tetramerization of PKM2 in macrophages. Front. Pharmacol. 13, 926945. doi:10.3389/fphar.2022.926945
Ivashkiv, L. B. (2020). The hypoxia-lactate axis tempers inflammation. Nat. Rev. Immunol. 20, 85–86. doi:10.1038/s41577-019-0259-8
Jia, L., Huang, S., Yin, X., Zan, Y., Guo, Y., and Han, L. (2018). Quercetin suppresses the mobility of breast cancer by suppressing glycolysis through Akt-mTOR pathway mediated autophagy induction. Life Sci. 208, 123–130. doi:10.1016/j.lfs.2018.07.027
Johnson, V. L., and Hunter, D. J. (2014). The epidemiology of osteoarthritis. Best. Pract. Res. Clin. Rheumatol. 28, 5–15. doi:10.1016/j.berh.2014.01.004
Kannu, P., Bateman, J. F., Randle, S., Cowie, S., Du Sart, D., Mcgrath, S., et al. (2010). Premature arthritis is a distinct type II collagen phenotype. Arthritis Rheum. 62, 1421–1430. doi:10.1002/art.27354
Kaushik, D. K., Bhattacharya, A., Mirzaei, R., Rawji, K. S., Ahn, Y., Rho, J. M., et al. (2019). Enhanced glycolytic metabolism supports transmigration of brain-infiltrating macrophages in multiple sclerosis. J. Clin. Invest. 129, 3277–3292. doi:10.1172/jci124012
Kim, H., Kang, D., Cho, Y., and Kim, J. H. (2015). Epigenetic regulation of chondrocyte catabolism and anabolism in osteoarthritis. Mol. Cells 38, 677–684. doi:10.14348/molcells.2015.0200
Kim, J. (2018). Regulation of immune cell functions by metabolic reprogramming. J. Immunol. Res. 2018, 8605471. doi:10.1155/2018/8605471
Kim, Y. J., Lee, S., Jin, J., Woo, H., Choi, Y. K., and Park, K. G. (2022). Cassiaside C inhibits M1 polarization of macrophages by downregulating glycolysis. Int. J. Mol. Sci. 23, 1696. doi:10.3390/ijms23031696
Kooshki, L., Mahdavi, P., Fakhri, S., Akkol, E. K., and Khan, H. (2022). Targeting lactate metabolism and glycolytic pathways in the tumor microenvironment by natural products: A promising strategy in combating cancer. BioFactors Oxf. Engl. 48, 359–383. doi:10.1002/biof.1799
Kudelko, M., Chan, C. W., Sharma, R., Yao, Q., Lau, E., Chu, I. K., et al. (2016). Label-free quantitative proteomics reveals survival mechanisms developed by hypertrophic chondrocytes under ER stress. J. Proteome Res. 15, 86–99. doi:10.1021/acs.jproteome.5b00537
Lambert, C., Zappia, J., Sanchez, C., Florin, A., Dubuc, J. E., and Henrotin, Y. (2020). The damage-associated molecular patterns (DAMPs) as potential targets to treat osteoarthritis: Perspectives from a review of the literature. Front. Med. 7, 607186. doi:10.3389/fmed.2020.607186
Lane, R. S., Fu, Y., Matsuzaki, S., Kinter, M., Humphries, K. M., and Griffin, T. M. (2015). Mitochondrial respiration and redox coupling in articular chondrocytes. Arthritis Res. Ther. 17, 54. doi:10.1186/s13075-015-0566-9
Li, H. M., Guo, H. L., Xu, C., Liu, L., Hu, S. Y., Hu, Z. H., et al. (2020a). Inhibition of glycolysis by targeting lactate dehydrogenase A facilitates hyaluronan synthase 2 synthesis in synovial fibroblasts of temporomandibular joint osteoarthritis. Bone 141, 115584. doi:10.1016/j.bone.2020.115584
Li, J., Pang, J., Liu, Z., Ge, X., Zhen, Y., Jiang, C. C., et al. (2021). Shikonin induces programmed death of fibroblast synovial cells in rheumatoid arthritis by inhibiting energy pathways. Sci. Rep. 11, 18263. doi:10.1038/s41598-021-97713-6
Li, L., Wang, X., Xu, H., Liu, X., and Xu, K. (2022a). Perspectives and mechanisms for targeting ferroptosis in the treatment of hepatocellular carcinoma. Front. Mol. Biosci. 9, 947208. doi:10.3389/fmolb.2022.947208
Li, L., Xu, H., Qu, L., Xu, K., and Liu, X. (2022b). Daidzin inhibits hepatocellular carcinoma survival by interfering with the glycolytic/gluconeogenic pathway through downregulation of TPI1. BioFactors Oxf. Engl. 48, 883–896. doi:10.1002/biof.1826
Li, M., Gao, F., Zhao, Q., Zuo, H., Liu, W., and Li, W. (2020b). Tanshinone IIA inhibits oral squamous cell carcinoma via reducing Akt-c-Myc signaling-mediated aerobic glycolysis. Cell Death Dis. 11, 381. doi:10.1038/s41419-020-2579-9
Li, W., Ma, X., Li, N., Liu, H., Dong, Q., Zhang, J., et al. (2016a). Resveratrol inhibits Hexokinases II mediated glycolysis in non-small cell lung cancer via targeting Akt signaling pathway. Exp. Cell Res. 349, 320–327. doi:10.1016/j.yexcr.2016.11.002
Li, W., Qiu, Y., Hao, J., Zhao, C., Deng, X., and Shu, G. (2018). Dauricine upregulates the chemosensitivity of hepatocellular carcinoma cells: Role of repressing glycolysis via miR-199a:HK2/PKM2 modulation. Food Chem. Toxicol. 121, 156–165. doi:10.1016/j.fct.2018.08.030
Li, X., Sun, J., Xu, Q., Duan, W., Yang, L., Wu, X., et al. (2020c). Oxymatrine inhibits colorectal cancer metastasis via attenuating PKM2-mediated aerobic glycolysis. Cancer Manag. Res. 12, 9503–9513. doi:10.2147/cmar.S267686
Li, Y., Xiao, W., Luo, W., Zeng, C., Deng, Z., Ren, W., et al. (2016b). Alterations of amino acid metabolism in osteoarthritis: Its implications for nutrition and health. Amino acids 48, 907–914. doi:10.1007/s00726-015-2168-x
Lippiello, L., Walsh, T., and Fienhold, M. (1991). The association of lipid abnormalities with tissue pathology in human osteoarthritic articular cartilage. Metabolism. 40, 571–576. doi:10.1016/0026-0495(91)90046-y
Liu, W., Yu, X., Zhou, L., Li, J., Li, M., Li, W., et al. (2020). Sinomenine inhibits non-small cell lung cancer via downregulation of hexokinases II-mediated aerobic glycolysis. Onco. Targets. Ther. 13, 3209–3221. doi:10.2147/ott.S243212
Loftus, R. M., and Finlay, D. K. (2016). Immunometabolism: Cellular metabolism turns immune regulator. J. Biol. Chem. 291, 1–10. doi:10.1074/jbc.R115.693903
Long, N., Peng, S., Chu, L., Jia, J., Dong, M., and Liu, J. (2020). Paclitaxel inhibits the migration of CD133+ U251 malignant glioma cells by reducing the expression of glycolytic enzymes. Exp. Ther. Med. 20, 72. doi:10.3892/etm.2020.9200
Madry, H. (2022). Surgical therapy in osteoarthritis. Osteoarthr. Cartil. 30, 1019–1034. doi:10.1016/j.joca.2022.01.012
Maneiro, E., Martín, M. A., De Andres, M. C., López-Armada, M. J., Fernández-Sueiro, J. L., Del Hoyo, P., et al. (2003). Mitochondrial respiratory activity is altered in osteoarthritic human articular chondrocytes. Arthritis Rheum. 48, 700–708. doi:10.1002/art.10837
Messina, O. D., Vidal Wilman, M., and Vidal Neira, L. F. (2019). Nutrition, osteoarthritis and cartilage metabolism. Aging Clin. Exp. Res. 31, 807–813. doi:10.1007/s40520-019-01191-w
Mickiewicz, B., Kelly, J. J., Ludwig, T. E., Weljie, A. M., Wiley, J. P., Schmidt, T. A., et al. (2015).,Metabolic analysis of knee synovial fluid as a potential diagnostic approach for osteoarthritis, J. Orthop. Res. 33, 1631–1638. doi:10.1002/jor.22949
Mobasheri, A., Bondy, C. A., Moley, K., Mendes, A. F., Rosa, S. C., Richardson, S. M., et al. (2008). Facilitative glucose transporters in articular chondrocytes. Expression, distribution and functional regulation of GLUT isoforms by hypoxia, hypoxia mimetics, growth factors and pro-inflammatory cytokines. Adv. Anat. Embryol. Cell Biol. 1, 1-84
Mobasheri, A., Rayman, M. P., Gualillo, O., Sellam, J., Van Der Kraan, P., and Fearon, U. (2017). The role of metabolism in the pathogenesis of osteoarthritis. Nat. Rev. Rheumatol. 13, 302–311. doi:10.1038/nrrheum.2017.50
Mobasheri, A., Vannucci, S. J., Bondy, C. A., Carter, S. D., Innes, J. F., Arteaga, M. F., et al. (2002). Glucose transport and metabolism in chondrocytes: A key to understanding chondrogenesis, skeletal development and cartilage degradation in osteoarthritis. Histol. Histopathol. 17, 1239–1267. doi:10.14670/hh-17.1239
Molnar, V., Matišić, V., Kodvanj, I., Bjelica, R., Jeleč, Ž., Hudetz, D., et al. (2021). Cytokines and chemokines involved in osteoarthritis pathogenesis. Int. J. Mol. Sci. 22, 9208. doi:10.3390/ijms22179208
Neogi, T., and Zhang, Y. (2013). Epidemiology of osteoarthritis. Rheum. Dis. Clin. North Am. 39, 1–19. doi:10.1016/j.rdc.2012.10.004
Pan, L., Feng, F., Wu, J., Fan, S., Han, J., Wang, S., et al. (2022). Demethylzeylasteral targets lactate by inhibiting histone lactylation to suppress the tumorigenicity of liver cancer stem cells. Pharmacol. Res. 181, 106270. doi:10.1016/j.phrs.2022.106270
Paterson, K. L., and Gates, L. (2019). Clinical assessment and management of foot and ankle osteoarthritis: A review of current evidence and focus on pharmacological treatment. Drugs Aging 36, 203–211. doi:10.1007/s40266-019-00639-y
Peansukmanee, S., Vaughan-Thomas, A., Carter, S. D., Clegg, P. D., Taylor, S., Redmond, C., et al. (2009).Effects of hypoxia on glucose transport in primary equine chondrocytes in vitro and evidence of reduced GLUT1 gene expression in pathologic cartilage in vivo, J. Orthop. Res., 27. , 529–535. doi:10.1002/jor.20772
Pei, L., Le, Y., Chen, H., Feng, J., Liu, Z., Zhu, J., et al. (2021). Cynaroside prevents macrophage polarization into pro-inflammatory phenotype and alleviates cecal ligation and puncture-induced liver injury by targeting PKM2/HIF-1α axis. Fitoterapia 152, 104922. doi:10.1016/j.fitote.2021.104922
Pereira, D., Ramos, E., and Branco, J. (2015). Osteoarthritis. Acta Med. Port. 28, 99–106. doi:10.20344/amp.5477
Pucino, V., Bombardieri, M., Pitzalis, C., and Mauro, C. (2017). Lactate at the crossroads of metabolism, inflammation, and autoimmunity. Eur. J. Immunol. 47, 14–21. doi:10.1002/eji.201646477
Qu, J., Lu, D., Guo, H., Miao, W., Wu, G., and Zhou, M. (2016). PFKFB3 modulates glycolytic metabolism and alleviates endoplasmic reticulum stress in human osteoarthritis cartilage. Clin. Exp. Pharmacol. Physiol. 43, 312–318. doi:10.1111/1440-1681.12537
Qu, L., Lin, X., Liu, C., Ke, C., Zhou, Z., Xu, K., et al. (2021). Atractylodin attenuates dextran sulfate sodium-induced colitis by alleviating gut microbiota dysbiosis and inhibiting inflammatory response through the MAPK pathway. Front. Pharmacol. 12, 665376. doi:10.3389/fphar.2021.665376
Rai, V., Dilisio, M. F., Samadi, F., and Agrawal, D. K. (2022). Counteractive effects of IL-33 and IL-37 on inflammation in osteoarthritis. Int. J. Environ. Res. Public Health 19, 5690. doi:10.3390/ijerph19095690
Rasheed, Z., Akhtar, N., and Haqqi, T. M. (2011). Advanced glycation end products induce the expression of interleukin-6 and interleukin-8 by receptor for advanced glycation end product-mediated activation of mitogen-activated protein kinases and nuclear factor-κB in human osteoarthritis chondrocytes. Rheumatol. Oxf. Engl. 50, 838–851. doi:10.1093/rheumatology/keq380
Ridnour, L. A., Windhausen, A. N., Isenberg, J. S., Yeung, N., Thomas, D. D., Vitek, M. P., et al. (2007). Nitric oxide regulates matrix metalloproteinase-9 activity by guanylyl-cyclase-dependent and -independent pathways. Proc. Natl. Acad. Sci. U. S. A. 104, 16898–16903. doi:10.1073/pnas.0702761104
Rigoglou, S., and Papavassiliou, A. G. (2013). The NF-κB signalling pathway in osteoarthritis. Int. J. Biochem. Cell Biol. 45, 2580–2584. doi:10.1016/j.biocel.2013.08.018
Rodriguez-Merchan, E. C. (2018). Topical therapies for knee osteoarthritis. Postgrad. Med. 130, 607–612. doi:10.1080/00325481.2018.1505182
Sellam, J., and Berenbaum, F. (2013). Is osteoarthritis a metabolic disease? Jt. bone spine 80, 568–573. doi:10.1016/j.jbspin.2013.09.007
Shen, C. L., Smith, B. J., Lo, D. F., Chyu, M. C., Dunn, D. M., Chen, C. H., et al. (2012). Dietary polyphenols and mechanisms of osteoarthritis. J. Nutr. Biochem. 23, 1367–1377. doi:10.1016/j.jnutbio.2012.04.001
Siebuhr, A. S., Bay-Jensen, A. C., Jordan, J. M., Kjelgaard-Petersen, C. F., Christiansen, C., Abramson, S. B., et al. (2016). Inflammation (or synovitis)-driven osteoarthritis: An opportunity for personalizing prognosis and treatment? Scand. J. Rheumatol. 45, 87–98. doi:10.3109/03009742.2015.1060259
Sophia Fox, A. J., Bedi, A., and Rodeo, S. A. (2009). The basic science of articular cartilage: Structure, composition, and function. Sports health 1, 461–468. doi:10.1177/1941738109350438
Sotelo-Hitschfeld, T., Fernández-Moncada, I., and Barros, L. F. (2012). Acute feedback control of astrocytic glycolysis by lactate. Glia 60, 674–680. doi:10.1002/glia.22304
Suzuki, A., Yabu, A., and Nakamura, H. (2022). Advanced glycation end products in musculoskeletal system and disorders. Methods (San Diego, Calif.) 203, 179–186. doi:10.1016/j.ymeth.2020.09.012
Tejero, S., Prada-Chamorro, E., González-Martín, D., García-Guirao, A., Galhoum, A., Valderrabano, V., et al. (2021). Conservative treatment of ankle osteoarthritis. J. Clin. Med. 10, 4561. doi:10.3390/jcm10194561
Vališ, K., Talacko, P., Grobárová, V., Černý, J., and Novák, P. (2016). Shikonin regulates C-MYC and GLUT1 expression through the MST1-YAP1-TEAD1 axis. Exp. Cell Res. 349, 273–281. doi:10.1016/j.yexcr.2016.10.018
Vazquez, A., Liu, J., Zhou, Y., and Oltvai, Z. N. (2010). Catabolic efficiency of aerobic glycolysis: The Warburg effect revisited. BMC Syst. Biol. 4, 58. doi:10.1186/1752-0509-4-58
Wang, C., Gao, Y., Zhang, Z., Chen, C., Chi, Q., Xu, K., et al. (2020a). Ursolic acid protects chondrocytes, exhibits anti-inflammatory properties via regulation of the NF-κB/NLRP3 inflammasome pathway and ameliorates osteoarthritis. Biomed. Pharmacother. = Biomedecine Pharmacother. 130, 110568. doi:10.1016/j.biopha.2020.110568
Wang, C., Sha, Y., Wang, S., Chi, Q., Sung, K. L. P., Xu, K., et al. (2020b). Lysyl oxidase suppresses the inflammatory response in anterior cruciate ligament fibroblasts and promotes tissue regeneration by targeting myotrophin via the nuclear factor-kappa B pathway. J. Tissue Eng. Regen. Med. 14, 1063–1076. doi:10.1002/term.3077
Wang, P., Xiong, X., Zhang, J., Qin, S., Wang, W., and Liu, Z. (2020c). Icariin increases chondrocyte vitality by promoting hypoxia-inducible factor-1α expression and anaerobic glycolysis. Knee 27, 18–25. doi:10.1016/j.knee.2019.09.012
Wang, T., and He, C. (2018). Pro-inflammatory cytokines: The link between obesity and osteoarthritis. Cytokine Growth Factor Rev. 44, 38–50. doi:10.1016/j.cytogfr.2018.10.002
Wang, Y., Ma, J., Yan, X., Chen, X., Si, L., Liu, Y., et al. (2016). Isoliquiritigenin inhibits proliferation and induces apoptosis via alleviating hypoxia and reducing glycolysis in mouse melanoma B16F10 cells. Recent Pat. anticancer. Drug Discov. 11, 215–227. doi:10.2174/1573406412666160307151904
Wu, H., Du, J., Li, C., Li, H., Guo, H., and Li, Z. (2022). Kaempferol can reverse the 5-fu resistance of colorectal cancer cells by inhibiting PKM2-mediated glycolysis. Int. J. Mol. Sci. 23, 3544. doi:10.3390/ijms23073544
Wu, H., He, L., Shi, J., Hou, X., Zhang, H., Zhang, X., et al. (2018a). Resveratrol inhibits VEGF-induced angiogenesis in human endothelial cells associated with suppression of aerobic glycolysis via modulation of PKM2 nuclear translocation. Clin. Exp. Pharmacol. Physiol. 45, 1265–1273. doi:10.1111/1440-1681.13017
Wu, H., Pan, L., Gao, C., Xu, H., Li, Y., Zhang, L., et al. (2019). Quercetin inhibits the proliferation of glycolysis-addicted HCC cells by reducing hexokinase 2 and akt-mTOR pathway, Mol. (Basel, Switz., 24. doi:10.3390/molecules24101993
Wu, J., Zhang, X., Wang, Y., Sun, Q., Chen, M., Liu, S., et al. (2018b). Licochalcone A suppresses hexokinase 2-mediated tumor glycolysis in gastric cancer via downregulation of the Akt signaling pathway. Oncol. Rep. 39, 1181–1190. doi:10.3892/or.2017.6155
Xie, J., Méndez, J. D., Méndez-Valenzuela, V., and Aguilar-Hernández, M. M. (2013). Cellular signalling of the receptor for advanced glycation end products (RAGE). Cell. Signal. 25, 2185–2197. doi:10.1016/j.cellsig.2013.06.013
Xu, H., Li, L., Tan, C., Han, J., Qu, L., Tu, J., et al. (2022). Quality assessment of processed Eucommiae Cortex based on the color and tensile force. Med. Nov. Technol. Devices 16, 100167. doi:10.1016/j.medntd.2022.100167
Xu, K., Gao, Y., Yang, L., Liu, Y., and Wang, C. (2021a). Magnolin exhibits anti-inflammatory effects on chondrocytes via the NF-κB pathway for attenuating anterior cruciate ligament transection-induced osteoarthritis. Connect. Tissue Res. 62, 475–484. doi:10.1080/03008207.2020.1778679
Xu, K., Sha, Y., Wang, S., Chi, Q., Liu, Y., Wang, C., et al. (2019). Effects of Bakuchiol on chondrocyte proliferation via the PI3K-Akt and ERK1/2 pathways mediated by the estrogen receptor for promotion of the regeneration of knee articular cartilage defects. Cell Prolif. 52, e12666. doi:10.1111/cpr.12666
Xu, K., Shao, Y., Xia, Y., Qian, Y., Jiang, N., Liu, X., et al. (2021b). Tenascin-C regulates migration of SOX10 tendon stem cells via integrin-α9 for promoting patellar tendon remodeling. BioFactors Oxf. Engl. 47, 768–777. doi:10.1002/biof.1759
Yang, X., Chen, W., Zhao, X., Chen, L., Li, W., Ran, J., et al. (2018a). Pyruvate kinase M2 modulates the glycolysis of chondrocyte and extracellular matrix in osteoarthritis. DNA Cell Biol. 37, 271–277. doi:10.1089/dna.2017.4048
Yang, Y., Cao, Y., Chen, L., Liu, F., Qi, Z., Cheng, X., et al. (2018b). Cryptotanshinone suppresses cell proliferation and glucose metabolism via STAT3/SIRT3 signaling pathway in ovarian cancer cells. Cancer Med. 7, 4610–4618. doi:10.1002/cam4.1691
Yao, H., Wu, Z., Xu, Y., Xu, H., Lou, G., Jiang, Q., et al. (2019). Andrographolide attenuates imbalance of gastric vascular homeostasis induced by ethanol through glycolysis pathway. Sci. Rep. 9, 4968. doi:10.1038/s41598-019-41417-5
Yao, Q., Jing, G., Zhang, X., Li, M., Yao, Q., and Wang, L. (2021). Cinnamic acid inhibits cell viability, invasion, and glycolysis in primary endometrial stromal cells by suppressing NF-κB-induced transcription of PKM2. Biosci. Rep., BSR20211828. doi:10.1042/bsr20211828
Ying, Z. H., Li, H. M., Yu, W. Y., and Yu, C. H. (2021). Iridin prevented against lipopolysaccharide-induced inflammatory responses of macrophages via inactivation of PKM2-mediated glycolytic pathways. J. Inflamm. Res. 14, 341–354. doi:10.2147/jir.S292244
Yuan, J., Peng, G., Xiao, G., Yang, Z., Huang, J., Liu, Q., et al. (2020). Xanthohumol suppresses glioblastoma via modulation of Hexokinase 2 -mediated glycolysis. J. Cancer 11, 4047–4058. doi:10.7150/jca.33045
Yunus, M. H. M., Nordin, A., and Kamal, H. (2020). Pathophysiological perspective of osteoarthritis, Med. Kaunas. Lith., 56, 110614. doi:10.3390/medicina56110614
Zhai, G. (2019). Alteration of metabolic pathways in osteoarthritis. Metabolites 9, E11. doi:10.3390/metabo9010011
Zhang, C., Cai, T., Zeng, X., Cai, D., Chen, Y., Huang, X., et al. (2018). Astragaloside IV reverses MNNG-induced precancerous lesions of gastric carcinoma in rats: Regulation on glycolysis through miRNA-34a/LDHA pathway. Phytother. Res. 32, 1364–1372. doi:10.1002/ptr.6070
Zhang, D., Tang, Z., Huang, H., Zhou, G., Cui, C., Weng, Y., et al. (2019). Metabolic regulation of gene expression by histone lactylation. Nature 574, 575–580. doi:10.1038/s41586-019-1678-1
Zhang, Q., Liu, Q., Zheng, S., Liu, T., Yang, L., Han, X., et al. (2021). Shikonin inhibits tumor growth of ESCC by suppressing PKM2 mediated aerobic glycolysis and STAT3 phosphorylation. J. Cancer 12, 4830–4840. doi:10.7150/jca.58494
Zhang, W. H., Qiu, M. H., Wang, X. J., Sun, K., Zheng, Y., and Jing, Z. C. (2014). Up-regulation of hexokinase1 in the right ventricle of monocrotaline induced pulmonary hypertension. Respir. Res. 15, 119. doi:10.1186/s12931-014-0119-9
Zhao, X., Zhu, Y., Hu, J., Jiang, L., Li, L., Jia, S., et al. (2018). Shikonin inhibits tumor growth in mice by suppressing pyruvate kinase M2-mediated aerobic glycolysis. Sci. Rep. 8, 14517. doi:10.1038/s41598-018-31615-y
Zhao, Y., Huang, H., Jia, C. H., Fan, K., Xie, T., Zhu, Z. Y., et al. (2021). Apigenin increases radiosensitivity of glioma stem cells by attenuating HIF-1α-mediated glycolysis. Med. Oncol. 38, 131. doi:10.1007/s12032-021-01586-8
Zheng, K., Shen, N., Chen, H., Ni, S., Zhang, T., Hu, M., et al. (2017).,Global and targeted metabolomics of synovial fluid discovers special osteoarthritis metabolites, J. Orthop. Res. 35. , 1973–1981. doi:10.1002/jor.23482
Zheng, L., Zhang, Z., Sheng, P., and Mobasheri, A. (2021). The role of metabolism in chondrocyte dysfunction and the progression of osteoarthritis. Ageing Res. Rev. 66, 101249. doi:10.1016/j.arr.2020.101249
Zhou, J., Su, C. M., Chen, H. A., Du, S., Li, C. W., Wu, H., et al. (2020). Cryptanshinone inhibits the glycolysis and inhibits cell migration through PKM2/β-catenin Axis in breast cancer. Onco. Targets. Ther. 13, 8629–8639. doi:10.2147/ott.S239134
Keywords: osteoarthritis, glycolysis, enzymes, natural products, metabolic
Citation: Tan C, Li L, Han J, Xu K and Liu X (2022) A new strategy for osteoarthritis therapy: Inhibition of glycolysis. Front. Pharmacol. 13:1057229. doi: 10.3389/fphar.2022.1057229
Received: 29 September 2022; Accepted: 27 October 2022;
Published: 10 November 2022.
Edited by:
Ujjal Bhawal, Nihon University, JapanCopyright © 2022 Tan, Li, Han, Xu and Liu. This is an open-access article distributed under the terms of the Creative Commons Attribution License (CC BY). The use, distribution or reproduction in other forums is permitted, provided the original author(s) and the copyright owner(s) are credited and that the original publication in this journal is cited, in accordance with accepted academic practice. No use, distribution or reproduction is permitted which does not comply with these terms.
*Correspondence: Kang Xu, kangxu05@hbtcm.edu.cn; Xianqiong Liu, 1424@hbtcm.edu.cn
†These authors have contributed equally to this work