- 1Department of Surgical Sciences, Functional Pharmacology and Neuroscience, Uppsala University, Uppsala, Sweden
- 2Advanced Molecular Technologies LLC., Moscow, Russia
The phosphodiesterase (PDE) enzymes, key regulator of the cyclic nucleotide signal transduction system, are long-established as attractive therapeutic targets. During investigation of trends within clinical trials, we have identified a particularly high number of clinical trials involving PDE inhibitors, prompting us to further evaluate the current status of this class of therapeutic agents. In total, we have identified 87 agents with PDE-inhibiting capacity, of which 85 interact with PDE enzymes as primary target. We provide an overview of the clinical drug development with focus on the current clinical uses, novel molecules and indications, highlighting relevant clinical studies. We found that the bulk of current clinical uses for this class of therapeutic agents are chronic obstructive pulmonary disease (COPD), vascular and cardiovascular disorders and inflammatory skin conditions. In COPD, particularly, PDE inhibitors are characterised by the compliance-limiting adverse reactions. We discuss efforts directed to appropriately adjusting the dose regimens and conducting structure-activity relationship studies to determine the effect of structural features on safety profile. The ongoing development predominantly concentrates on central nervous system diseases, such as schizophrenia, Alzheimer’s disease, Parkinson’s disease and fragile X syndrome; notable advancements are being also made in mycobacterial infections, HIV and Duchenne muscular dystrophy. Our analysis predicts the diversification of PDE inhibitors’ will continue to grow thanks to the molecules in preclinical development and the ongoing research involving drugs in clinical development.
Introduction
The cyclic nucleotide signal transduction system, collectively encompassing the 3′-5′cyclic adenosine and guanosine monophosphate (cAMP and cGMP, respectively) signaling systems, have long been a subject to intensive research for its important biological implications and associated therapeutic potential. A part of a diverse group of non-protein signaling compounds called second messengers, the cAMP and cGMP signaling systems are ultimately involved in relaying the signal produced via receptor-ligand interactions at the cell surface to effector proteins (Newton et al., 2016). These effector proteins, such as the cAMP and cGMP-activated protein kinases, subsequently elicit a wide variety of biological responses.
The second messengers’ intracellular concentration is maintained by complex homeostatic mechanisms. Within the cyclic nucleotide system, these include the adenylyl/guanylyl cyclase enzymes and the cyclic nucleotide Phosphodiesterase (PDE); the former is activated in response to the receptor-ligand interaction, catalyzing the conversion of AMP/GMP into 3′-5′- cAMP/cGMP, while the latter catalyzes the cyclic nucleotides’ deactivation (Newton et al., 2016). The potential of this system, especially the PDE enzymes, from the drug development point of view had been recognized in as early as of late 20th century (Amer, 1977; Pang, 1988) and there has been a continuously growing interest towards further advancement in a variety of clinical indications; according to the ClinicalTrials.gov database, there are at least 1825 recorded clinical studies involving PDE inhibitors as of 2022. However, only a relatively small number of PDE inhibitors have entered market, while several initially promising therapeutic agents proved unsuccessful (Baillie et al., 2019).
During our investigation of trends within clinical trials (Rask-Andersen et al., 2011; Rask-Andersen et al., 2014; Attwood et al., 2018), we have noticed an especially large number of studies involving both the investigational and approved agents of the PDE inhibitors class, prompting us to conduct our own detailed investigation. The aim of present analysis is to provide a comprehensive overview of the most recent trends in clinical research regarding PDE inhibitors and to speculate regarding the future of this therapeutically important enzyme family.
The cyclic nucleotide phosphodiesterase superfamily—Overview
The cyclic nucleotide PDEs is a diverse superfamily of 11 families, encoded by 21 genes and responsible for catalyzing the 3′-cyclic phosphate bond hydrolysis in the cyclic nucleotide molecules, resulting in inactive compounds (Francis et al., 2011a). Structurally, the PDE enzymes are characterized by the conserved C-terminal catalytic domain and diverse N-terminal regions, comprised of several subdomains; the family designation is based on the C-terminal catalytic domain homology (Francis et al., 2011a). Each of the individual PDE families is characterized by varying specificities towards the cyclic nucleotide substrate: the families 1, 2, 3, 10, and 11 hydrolyze both cAMP and cGMP with comparable affinity; the families 4, 7, and 8 exhibit higher affinities towards cAMP, while the families 5, 6, and 9 are more specific towards cGMP (Francis et al., 2011a). The expression pattern among different PDE families is typically broad and characterized by predominantly cytosolic intracellular localization (Bender and Beavo, 2006; Conti and Beavo, 2007). The Figure 1 summarizes the known evidence regarding the structural and functional features of each family, while Table 1 illustrates the isoforms and expression patterns.
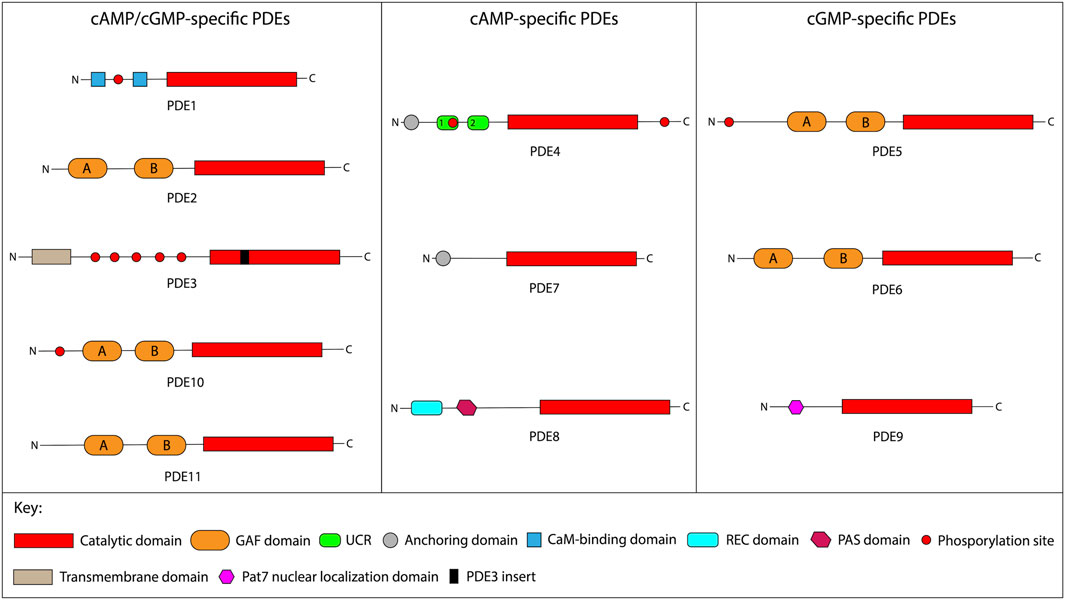
FIGURE 1. Schematic structure of PDE enzymes. This figure schematically presents principle structural features of the individual PDE isoforms. The PDE families are grouped according to the cyclic nucleotide affinities. The structural features and approximate isoform sizes of each PDE family are summarized as per (Baillie et al., 2019; Conti and Beavo, 2007; Francis et al., 2011; Maurice et al., 2014). Abbreviations: cAMP, cyclic adenosine monophosphate; cGMP, cyclic guanosine monophosphate; PDE, phosphodiesterase; GAF, cGMP-binding PDEs, Anabaena adenylyl cyclase, and Escherichia coli FhlA; UCR, upstream conserved region; CaM; calmodulin; REC, signal regulatory domain; PAS, Per-ARNT-Sim; Pat7, 7-residue nuclear localization signal.
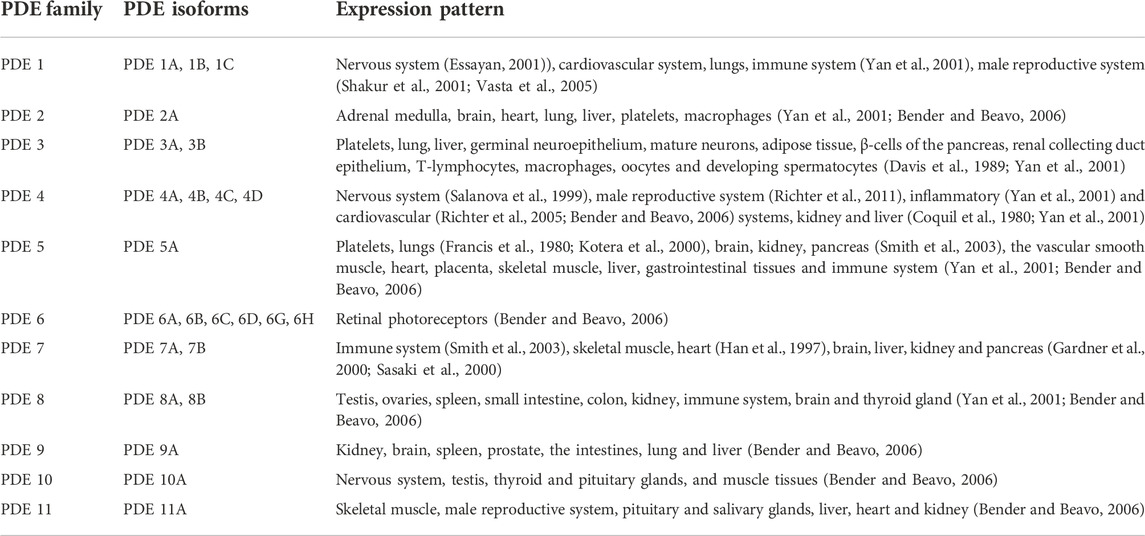
TABLE 1. PDE isoforms and expression pattern. This table provides a summary on the PDE enzymes’ isoforms and their expression pattern.
Biological functions of the individual families are diverse, including involvement in such processes, as myocytes contractility, male and female sex cells development and functioning, inflammatory cells activation, steroidogenesis or neuronal signaling (Bender and Beavo, 2006; Tsai and Beavo, 2011; Maurice et al., 2014). Such a variety of physiological involvement inevitably suggests involvement in human pathology as well. Indeed, numerous reviews have showed evidence on PDE-mediated implications in such diseases, as cancer (Peng et al., 2018), neurologic (Halene and Siegel, 2007; Nthenge-Ngumbau and Mohanakumar, 2018; Gurney, 2019), inflammatory (Page and Spina, 2011), pulmonary (Fan Chung, 2006; Mokry et al., 2018; Joskova et al., 2020; Kawamatawong, 2021; Mokra and Mokry, 2021), pediatric (Mokra et al., 2018), and cardiovascular disorders (Ravipati et al., 2007), among others. Additionally, the PDE enzymes are characterized by several factors, including a high degree of isoform variation, a high degree of isoform specificity towards substrates, distinct tissue expression and subcellular localization patterns among different isoforms, and the generally favorable intracellular cyclic nucleotides’ concentration, that further highlight their potential as drug targets (Bender and Beavo, 2006).
The global efforts to exploit the PDE enzymes therapeutically have so far produced a large number of molecules with varying selectivity and therapeutic applications, as well as a growing number of agents under development (Baillie et al., 2019). In the next section, we will provide an introduction to the PDE inhibitors as a class of therapeutic agents, as well as discuss a brief methodology of the dataset, used in our study.
Phosphodiesterase inhibitors overview and dataset
Chemically, the PDE inhibitors predominantly belong to a broad range of nitrogen-containing classes (Weinryb et al., 1972; Weishaar et al., 1985; Dyke and Montana, 2002; Andersson, 2018; Zagorska, 2020). At least one non-nitrogenous compound, an isoflavone derivative genistein, has also been identified to affect the PDE enzymes (Nichols and Morimoto, 2000). The majority of marketed PDE inhibitors are characterized by non-selective action; however, the PDE 3 and 5-selective inhibitors represent a notable portion of the PDE inhibitors market (Baillie et al., 2019).
The earliest identified class of PDE inhibitors is the class of xanthine derivatives, which provided important tools for the further development of this therapeutic class; acting as competitive inhibitors, these compounds are characterized by structural features that resemble the purine moiety of cyclic nucleotides: the heterocyclic ring which is comprised of a six-membered pyrimidine ring, conjoined with a five-membered ring containing nitrogen atoms (Francis et al., 2011b). The earliest such compounds, caffeine and theophylline, were both characterized by non-selective and relatively weak inhibitory activity (Choi et al., 1988); however, alkylxanthine compounds, such as 1-methyl-3-isobutylxanthine, were shown to be up to 15-times more potent as compared to theophylline (Beavo et al., 1970). These discoveries, subsequently, have resulted in the emergence of an ever-growing range of improved molecules, including the PDE 5-selective sildenafil and related compounds.
In our dataset, we have included 87 unique PDE inhibitors. The Figure 2 maps the approved and investigational inhibitors to individual PDE isoforms, as well as provides the phylogenetic relationship between PDE proteins. The data was extracted from updated versions of our previously published analyses on drug-target interactions of both (The US Food and Drug Administration TUS, 2018) approved agents as well as agents in clinical development (Rask-Andersen et al., 2011; Rask-Andersen et al., 2014; Attwood et al., 2018). These studies were originally based on information from the Drugs in Clinical Trials Database (discontinued) from CenterWatch1 and the DrugBank database (Wishart et al., 2018) and spans from 1983 to 2019. The targets, mechanisms of action, and indications were manually assessed using published studies and public databases including Drugs@FDA2, EMA (European Medicines Agency. 2019a) Medicines3 and NICE (The National Institute of Health. 2022) Technology appraisal guidance4 databases. US clinical trial information was obtained from the National Institute of Health Clinical Trials resource5. Where applicable, EU clinical trial information, identified through the EMA EU Clinical Trials Register (European Medicines Agency, 2019b)6, was additionally included. To ensure our review is as comprehensive as possible in regards of the recent advances, we additionally reference reviews on PDE inhibitors in clinical trials and/or selected diseases published throughout the past 10 years (i.e., 2010–2020) (Page and Spina, 2011; Matera et al., 2014; Tobin, 2015; Drobnis and Nangia, 2017; Geerts et al., 2017; Knott et al., 2017; Peng et al., 2018; Giorgi et al., 2020; Tzoumas et al., 2020).
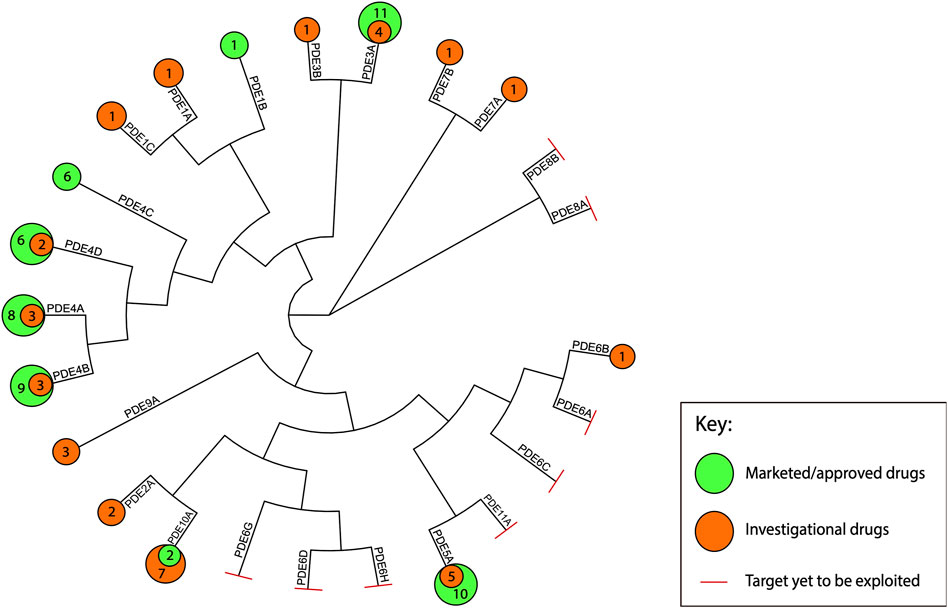
FIGURE 2. Marketed and investigational PDE inhibitors mapped to the human PDE proteins. This figure illustrates the phylogenetic relationship between the human PDE isoforms and maps the identified marketed and investigational agents to their targets. The amino acid sequences of each for each of the human PDE proteins were obtained from UniProt (UniProtKB database) (UniProt Consortium, 2021). Multiple sequence alignment was performed using Clustal Omega (RRID:SCR_001591). The phylogenetic tree was visualized in R Studio (Paradis and Schliep, 2019; Yu, 2020) and annotated in Adobe Illustrator CC 22.1.
In sections below, we will discuss various aspects of the PDE inhibitors’ clinical uses and research. For the approved agents, we will briefly discuss the rationale behind employing PDE inhibitors in the relevant disorders and discuss the efficacy and safety profiles of the included drugs. For the investigational agents, we will provide a summary on some of the currently available clinical and/or pre-clinical data, and will comment on the future of this important class of therapeutic agents, highlighting its currently evident strengths and weaknesses.
Phosphodiesterase inhibitors in clinics—Current status
As of 2022, we identified 35 agents that have been approved and authorized for marketing by either FDA or any other drug regulatory authority. The approval statuses and relevant trade names were determined through the DrugBank, Drugs@FDA and EMA Medicines databases records, Google search engine, or cited as per (Baillie et al., 2019). The majority of marketed indications include respiratory and cardiovascular diseases, as well as several inflammation-mediated pathologies of skin and joints. Central and peripheral nervous system disorders are also represented. Tables 2–Tables 4 summarize the drugs, their chemical structures, PDE isoform selectivity and indications.
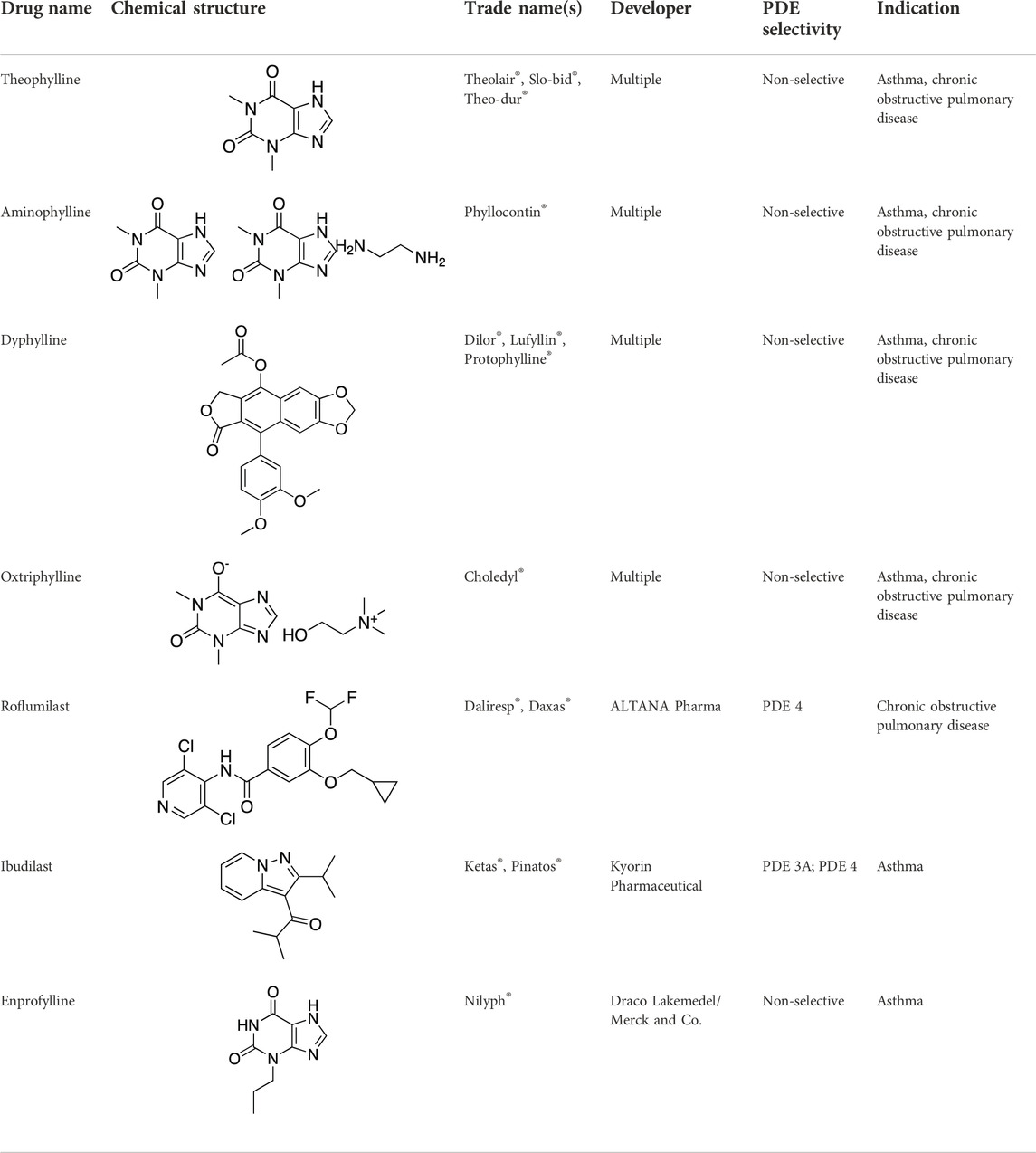
TABLE 2. Marketed PDE inhibitors in pulmonary diseases. This table provides a summary on the available clinical evidence for marketed PDE inhibitors in lung diseases. Trade names, developer name and the targeted PDE isozymes are listed according to the PubChem, DrugBank and Drugs@FDA database records. Chemical structures are derived from the PubChem database (Kim et al., 2021).
Phosphodiesterase inhibitors in respiratory diseases
Obstructive respiratory diseases (OPD), such as asthma or the chronic obstructive pulmonary disease (COPD), have been one of the earliest marketed indications, associated with the PDE inhibitors. Asthma and COPD are common heterogeneous, typically inflammation-mediated diseases of the airways, characterized by the airflow limitation (Rabe and Watz, 2017; Papi et al., 2018). Non-selective theophylline and its derivatives aminophylline, dyphylline and oxtriphylline had been widely used in managing bronchospasm in asthma and COPD under multiple trade names (Table 2); xanthines are characterized by multiple mechanisms of action, including adenosine receptor antagonism, phosphoinositide-3-kinase inhibition and histone deacetylase activation (Spina and Page, 2017). Other marketed PDE inhibitors in respiratory disorders include PDE 4-selective roflumilast, approved by the FDA (Daliresp®, 2011) and EMA (Daxas®, 2010) in COPD; PDE 4-selective ibudilast, marketed in Japan and several other Asian markets for the use in asthma as Ketas® and Pinatos®, and non-selective enprofylline, marketed in asthma as Nilyph®.
The rationale behind targeting PDEs to manage the airway diseases is based on the involvement of several families in the inflammatory and structural cells within the respiratory system. Among the individual PDE families, PDE 3, PDE 4, PDE 5, PDE 7, PDE 8, and PDE 9 have been described to hold the highest degree of relevance (Ntontsi et al., 2019). Inhibiting these enzymes result in a variety of cellular responses, including the airway smooth muscle relaxation, bronchodilation and inhibition of inflammatory pathways. Particularly, PDEs 4 and 5 inhibition has also been described to affect the process of airway wall remodeling (Fan Chung, 2006).
In the clinical setting, the evidence is scarce and limited to PDE 4 inhibitors in COPD, showing a benefit in improving lung function and reducing the likelihood of exacerbations, but without a significant impact on the quality-of-life, based on the results of a systematic review of clinical trials involving cilomilast, roflumilast, and tetomilast (Janjua et al., 2020). A 1-year study of roflumilast in severe COPD has specifically shown a post-bronchodilator forced expiratory flow increasing by 39 ml versus placebo without significant changes in the COPD exacerbation rate (mean values 0.86 vs. 0.92 exacerbations per patient with roflumilast vs. placebo) (Calverley et al., 2007). However, a subset of patients in stage IV disease has shown a greater decrease in the rate (1.01 vs. 1.59 exacerbations per patient). The common adverse drug reactions (ADRs) in PDE 4 inhibitors include diarrhea, nausea, vomiting, dyspepsia, and headache (Janjua et al., 2020). Roflumilast, in particular, is described to be additionally associated with insomnia, weight loss and depressed mood. In asthma, the sole published evidence on ibudilast has shown an improvement in airway hypersensitivity and reduction in asthma attack severity (Kawasaki et al., 1992).
To summarize, PDE inhibitors are currently recognized as an add-on therapy in patients with COPD showing persistent symptoms or exacerbations (Janjua et al., 2020). The major obstacles in their wider clinical use and development are safety profile negatively affecting compliance in patients with COPD and lack of clinical data in patients with severe asthma (Rabe and Watz, 2017). A currently Recruiting Phase 1 trial of roflumilast in patients with severe asthma (NCT04108377) could provide further insights on the clinical applicability of PDE 4 in asthma. Additionally, two novel PDE inhibitors, ensifentrine, and tanimilast, are currently in clinical trials for COPD and asthma; ClinicalTrials.gov records mention two Recruiting Phase 3 studies of tanimilast in COPD (NCT04636814, NCT04636801), while ensifentrine is in two Ongoing Phase 3 trials in COPD and a Phase 2 study in asthma that has shown a dose-dependent bronchodilation similar to salbutamol, but without impacting potassium levels and having a less significant impact on heart rate and pulse rate (Bjermer et al., 2019).
Phosphodiesterase inhibitors in cardiovascular diseases
Some of the currently most widely marketed PDE inhibitors fall under the diverse category of cardiovascular drugs, primarily including cardiotonics, vasodilatory agents, as well as antiaggregants. Cardiovascular diseases (CVD) are a heterogeneous group of disorders affecting heart and blood vessels; the (World Health Organization, 2022) includes coronary heart disease, cerebrovascular disease, peripheral arterial disease, rheumatic heart disease, congenital heart diseases and venous thromboembolism into the term. The approved indications in this category include erectile dysfunction (ED), congestive heart failure (CHF), intermittent claudication, hypertension, and thrombosis-related complications (Table 3).
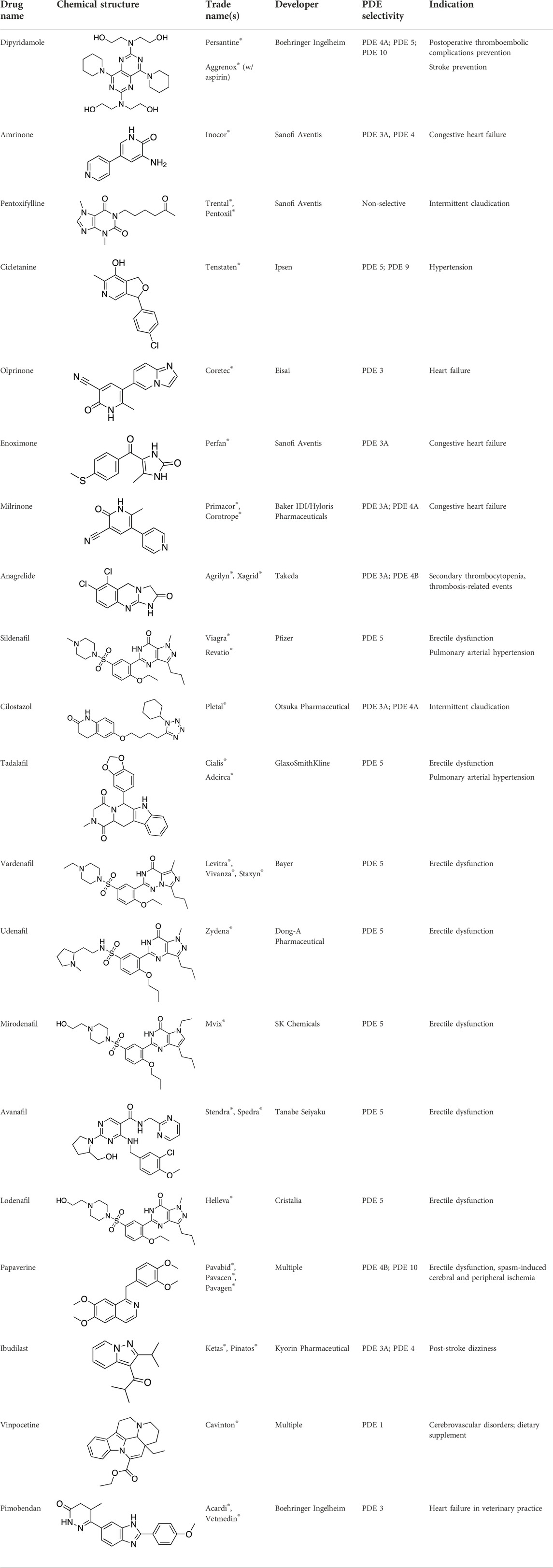
TABLE 3. Marketed PDE inhibitors in cardiovascular diseases. This table provides a summary on the available clinical evidence for marketed PDE inhibitors in cardiovascular diseases. Trade names, developer name and the targeted PDE isozymes are listed according to the PubChem and DrugBank database records. Chemical structures are derived from the PubChem database (Kim et al., 2021).
The earliest FDA-approved7 cardiovascular PDE inhibitors are PDE 3-selective dipyridamole (Persantine®, 1961), amrinone (Inocor®, 1984) and milrinone (Primacor®, 1987), and non-selective pentoxifylline (Trental®, 1984). Dipyridamole is approved as an adjunct to coumarin anticoagulants in the prevention of postoperative thromboembolic complications of cardiac valve replacement; in combination with aspirin (Aggrenox®, 1999), it is indicated for the stroke risk reduction. Amrinone and milrinone are both marketed in CHF, while pentoxifylline is approved in intermittent claudication. Other identified FDA approvals within this category include PDE 3-selective anagrelide (Agrylin®, 1997; also authorized as Xagrid® by the EMA in 2004), PDE 5-selective sildenafil (Viagra®, 1998) and its derivatives avanafil (Stendra®, 2012; also authorized as Spedra® by the EMA in 2013), tadalafil (Cialis®, 2003) and vardenafil (Levitra®, 2003 and Staxyn®, 2010; also authorized as Vivanza® by the EMA in 2003), and PDE 3-selective cilostazol (Pletal®, 1999). Anagrelide is approved for treating thrombocythemia, secondary to myeloproliferative malignancies, as well as to reduce risks of thrombosis-related events. Sildenafil, along with avanafil, tadalafil, and vardenafil, are all marketed in ED; sildenafil and tadalafil are also marketed in pulmonary arterial hypertension as Revatio® (2005) and Adcirca® (2009), respectively. Cilostazol is approved in intermittent claudication. Additionally, a number of PDE inhibitors, such as cGMP-PDE-specific cicletanine, PDE 3-selective enoximone and olprinone, non-selective papaverine, ibudilast, and PDE 5-specific mirodenafil, udenafil, and lodenafil, have received marketing authorization in other regions. Cicletanine is authorized in France for the treatment of hypertension (Tenstaten®, 1986). Enoximone is marketed across Europe for the treatment of CHF, initially authorized in France (Perfan®, 1987); olprinone is authorized for the same indication in Japan (Coretec®, 1986). Papaverine is another PDE inhibitor, used in ED, as well as vascular spasm-associated cerebral and peripheral ischemia; it is marketed worldwide under various trade names (Table 2). Ibudilast is marketed in post-stroke dizziness (Ketas® and Pinatos®). Mirodenafil (Mvix®, 2007) and udenafil (Zydena®, 2005) are both approved in the Asian markets for the treatment of ED. Lodenafil (Helleva®) is marketed for ED in Brazil. PDE 1-specific vinpocetine (Cavinton®) is marketed as a dietary supplement in managing cerebral vascular disorders and cognitive impairment. At least one cardiovascular PDE inhibitor is also used in veterinary practice; PDE 3-specific pimobendan is marketed under various trade names, including Acardi® (1997) for treatment of CHF in dogs.
The cardiac PDE families include PDEs 1, 2, 3, 4, 5, 8, 9, and 10; dysregulation in their expression patterns, activation and subcellular localization is often associated with CVDs (Chen and Yan, 2021). The bulk of currently marketed agents target PDEs 3 and 5, with PDEs 1, 4, 9, and 10 being additional targets. The PDE 3 inhibition was initially found to regulate the contractile function of the heart; global PDE 3A knockout increases cardiac contractility and relaxation through cAMP-dependent elevations of Ca2+ transient amplitudes and Ca2+ contents in the sarcoplasmic reticulum (Sun et al., 2007), which subsequently became the theoretical basis for its usage in congestive heart failure. Another important effect of PDE 3 is on the cardiomyocyte survival; chronic inhibition of its activity or reduction of PDE 3A expression induces apoptosis associated with a persistent induction of inducible cAMP early repressor (ICER), while preventing PDE 3A reduction is able to disrupt the PDE 3A-ICER feedback loop and protect cardiomyocytes from apoptosis (Ding et al., 2005). Furthermore, PDE 3 inhibition stimulates vascular relaxation, reducing peripheral and pulmonary vascular resistance and enhancing coronary blood flow (Chen and Yan, 2021). The PDE 5 inhibition has been shown to exert cardioprotective effects; a study involving sildenafil and vardenafil on a rabbit model of ischemia has shown a mitochondrial ATP-sensitive K channel opening-dependent protective effect against reperfusion injury (Ockaili et al., 2002). Additionally, studies involving sildenafil have showed a RhoA/Rho-kinase pathway inhibition-mediated attenuation of the left ventricular dysfunction (Chau et al., 2011) and the inhibition of the hypertrophy progression, fibrosis, and chamber remodeling, also improving basal and β-stimulated contractility and relaxation via protein kinase G activity, as well as Ca2+ handling (Nagyama et al., 2009).
Clinically, the bulk of published evidence is on the PDE 5 inhibitors. In ED, these agents are the first-line treatment in patients with primary disease with efficacy relating to placebo ranging from 0.47 in sildenafil to 0.26 in mirodenafil, based on the results of a trade-off network meta-analysis of 82 trials (n = 47,626 patients) (Chen et al., 2015). Vardenafil and lodenafil both have the relative efficacy of 0.35, udenafil and tadalafil—0.33 and avanafil—0.29. The most common ADRs, according to the analysis of 72 trials (n = 20,325) (Chen et al., 2015), are headache, flushing, dyspepsia, and nasal congestion; the frequency at therapeutic doses is highest in sildenafil (18.42%) and lowest in mirodenafil (10.23%). A recent meta-analysis of 44 trials (n = 3,853 patients) (Mykoniatis et al., 2021) has shown that combining PDE 5 inhibitors with other interventions, such as antioxidants, daily tadalafil, shockwaves or a vacuum device, improves the outcome, especially in the refractory and/or hard-to-treat disease. In pulmonary hypertension, a systematic review of 36 trials (n = 2,999 patients) (Barnes et al., 2019) has shown that treatment with PDE 5 inhibitors is associated with an improvement in WHO functional class and 6-min walking distance (up to 48 m), as well as reduced mortality, in patients with Group 1 pulmonary arterial hypertension.
PDE 3 inhibitors are another class of CVD-active inhibitors that has been explored clinically. In CHF, milrinone is described as an important option to treat patients with refractory disease (Chen and Yan, 2021); it functions by improving cardiac contractility and relaxation, induces vasodilation and has the overall effect of increased cardiac output, improvement of left ventricle-arterial coupling and enhanced cardiac mechanical efficiency (Ayres and Maani, 2022). ADRs associated with milrinone, such as an increased risk of arrhythmias and hypotension, are often dose-limiting, however (Chong et al., 2018). In intermittent claudication, according to the results of a systematic review of 16 trials (n = 3,972 patients) (Brown et al., 2021), the use of cilostazol is associated with improvements in functional status (mean initial claudication distance (ICD) of 26.49 m higher in cilostazol group vs. placebo group) and quality-of-life; comparison with pentoxifylline did not show any differences in functional status (mean ICD of 20 m higher in cilostazol group vs. pentoxifylline group). In cilostazol, the common ADRs are headache, diarrhea, dizziness, pain, and palpitations, headache being the most common (Brown et al., 2021). In thrombocytopenia, anagrelide is used in essential thrombocytopenia typically as a second-line therapy, preferentially in younger patients (Birgegård, 2016). A recently published longitudinal study (n = 150 patients) (Mazzucconi et al., 2020) have showed a response rate of 85.4%; ADRs included palpitations, peripheral vasodilation, anemia, diarrhea, gastric distress and thrombotic events, suggesting the importance of assessing thrombotic risk and monitoring cardiac function. The drug was also found to be characterized by nephrotoxicity, increasing the risk of renal function impairment, according to a recent retrospective study (Kwiatkowski et al., 2021).
Here, PDE inhibitors have been shown to be valuable tools to improve the functional status in patients with vascular and CVDs. It is suggested that developing isoform-specific inhibitors and/or activators could improve the clinical value of PDE modulators in CVDs, while modulating isoform-specific protein-protein interactions within specific signalosome could provide a strategy to improve the molecules’ specificity (Chen and Yan, 2021). In HF, an interesting novel approach is PDE 1 inhibition, investigated with ITI-214 (lenrispodun); it was shown to induce inodilator effects after a single oral dose, increase mean left ventricular power index and cardiac output, and reduce systemic vascular resistance (Gilotra et al., 2021).
Phosphodiesterase inhibitors in inflammatory disorders
Several PDE inhibitors have been marketed in inflammatory disorders affecting the skin and joints (Table 4). The FDA approvals in this category include PDE 4-specific amlexanox (Aphthasol®, 1996), apremilast (Otezla®, 2014), and crisaborole (Eucrisa®, 2016). Amlexanox was originally approved for the treatment of recurrent aphthous ulcerations in the oral mucosa; however, current FDA records list the drug as discontinued, presumably due to termination of licensing agreement between the drug’s developer, ULURU Inc., and Discus Dental Inc., the company that held exclusive rights for sales and marketing in the United States. Apremilast is approved for the treatment of active psoriatic arthritis and moderate-to-severe plague psoriasis, and crisaborole is approved in mild-to-moderate atopic dermatitis. Ibudilast, in addition to its previously mentioned uses in asthma and post-stroke dizziness, is marketed for allergic conjunctivitis as Ketas® and Eyevinal®. Recently, difumilast was marketed in Japan as Moizerto® (2021).
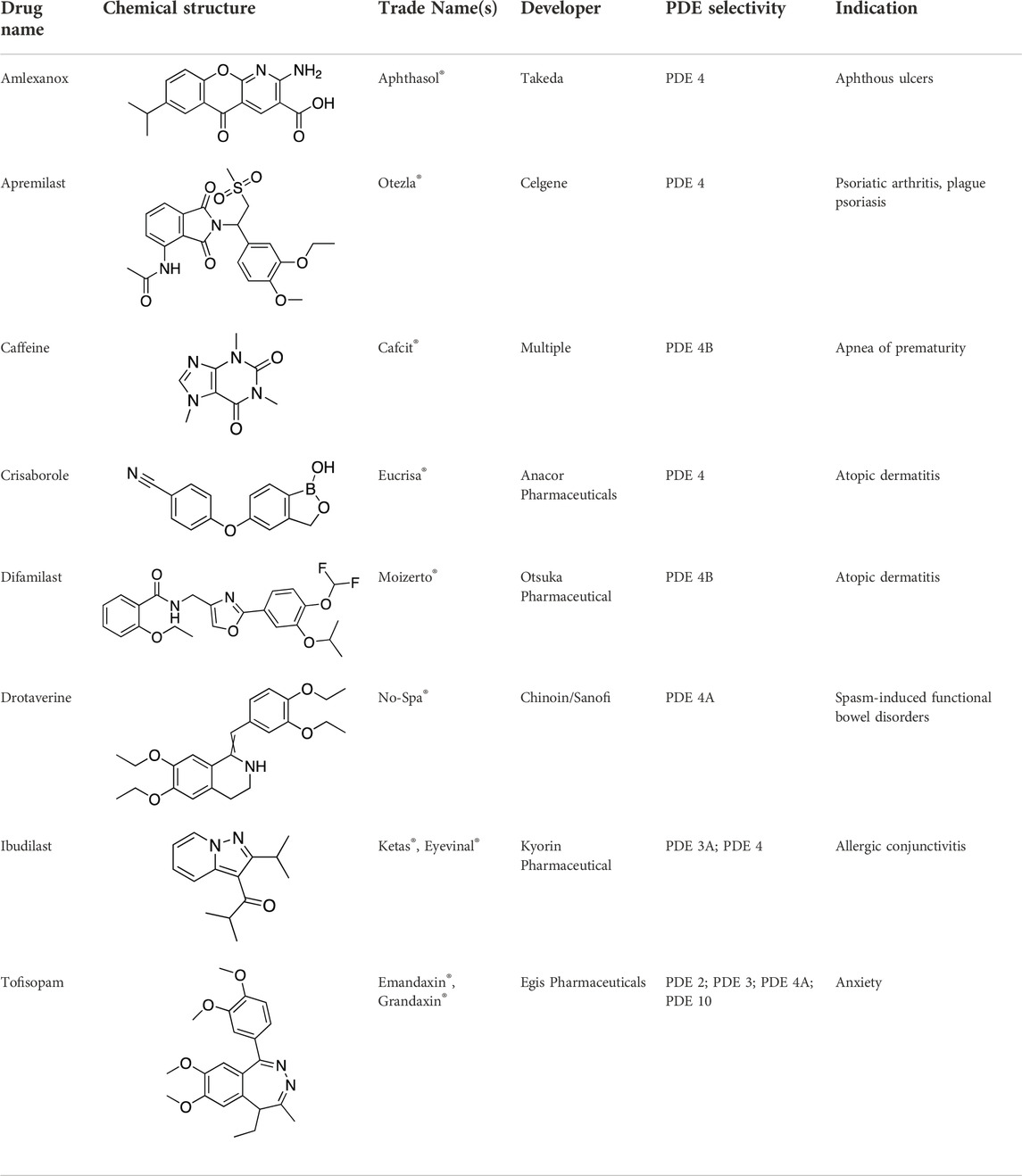
TABLE 4. Marketed PDE inhibitors in inflammatory and nervous system disorders. This table provides a summary on the available evidence for marketed PDE inhibitors in inflammatory diseases and some neurologic pathologies. Trade names, developer and the targeted PDE isozymes are listed according to the PubChem and DrugBank database records. Chemical structures are derived from the PubChem database (Kim et al., 2021).
Recurrent aphthous ulcerations is a common chronic disease in the oral mucosa, characterized by solitary or multiple, recurrent, small ulcers with erythematous halos and yellow or gray pseudomembranes (Lau and Smith, 2022). Psoriasis is an immune-mediated genetic skin disease that can also manifest in joints; chronic plague psoriasis represents the majority of cases (Boehncke and Schön, 2015). Atopic dermatitis is a common inflammatory skin disorder of multifactorial etiology characterized by recurring and intensely pruritic lesions (Tollefson and Bruckner, 2014). In the skin, the PDE 4 enzymes have been shown to be primarily expressed in keratinocytes, neutrophils, Langerhans cells, and T-cells (Li et al., 2018). Additionally, peripheral blood mononuclear cells demonstrate higher PDE 4B and PDE 4D mRNA levels in psoriatic patients (Schafer et al., 2016). Earlier studies have also noted potential disturbances in adenylyl cyclase signalling, associated with psoriasis (Wright et al., 1973). In atopic dermatitis, overexpression and overactivity of PDE 4 enzymes leads to the production of inflammatory cytokines and imbalance of T-cell activity and polarization, causing skin inflammation and disease exacerbation (Li et al., 2018).
Clinically, amlexanox, apremilast, crisaborole, and difumilast have all showed positive safety and efficacy. Amlexanox, originally formulated as a 5% topical oral paste, was shown to accelerate complete ulcer healing and the time to resolution of pain, and reduce the ulcer size (Bell, 2005). Treatment with apremilast, according to the most recent Phase 3 trial in patients with recurrent psoriatic arthritis (n = 505 patients) (Edwards et al., 2016), resulted in 40% achieving 20% functional improvement (as compared to 18% taking placebo) and 41% achieving 50% reduction in skin involvement (vs. 24% taking placebo). As an orally active drug, its use can be associated with systemic ADRs, such as diarrhea, nausea, headache, upper respiratory tract infection, vomiting, nasopharyngitis, and abdominal pain (Cada et al., 2014). Additionally, its use is associated with a risk of depression and weight loss. Crisaborole, based on the results of two Phase 3 trials in atopic dermatitis (n1 = 759 patients, n2 = 763 patients) (Paller et al., 2016), has showed an improvement in skin involvement (3 2-grade improvement; 32.8% and 31.4% vs. 25.4% and 18.0% taking the vehicle-control), as well as a pruritus relieve. The only ADR associated with the treatment was application site pain (Paller et al., 2016). Difamilast, based on the results of a Phase 3 long-term study in the Japanese adult and pediatric patients (nadult = 166 patients, npediatric = 200 patients) (Saeki et al., 2022), has shown a cumulative success EASI-75 (Eczema Area and Severity index) rates of 55.4% and 73.5%. The most common ADRs included dermatitis, acne and pigmentation disorder.
Here, PDE inhibitors are considered interesting additions to the established treatment strategies. Amlexanox is a first-line treatment option in apthous stomatitis (Lau and Smith, 2022). Apremilast is active in both psoriatic arthritis and cutaneous manifestations of the disease; however, its overall efficacy is low compared to other available options (Boehncke and Schön, 2015). Crisaborole is an option to treat mild-to-moderate disease, characterized by low systemic toxicity; it is reported, nevertheless, that it is not yet clear if it is characterized by lower toxicity than the other available topical therapeutic options (Li et al., 2018). Difamilast has showed attractive results in the Japanese population, more evidence is needed on patients of other ethnicities to discuss its wider use.
Phosphodiesterase inhibitors in nervous system disorders
Three PDE inhibitors have been marketed in central and peripheral nervous system disorders (Table 4): drotaverine (No-Spa®), tofisopam (Grandaxin®) and caffeine (Cafcit®). Drotaverine is marketed in functional bowel disorders due to the smooth muscle spasm, while tofisopam is marketed in anxiety. Additionally, caffeine is approved by the FDA in 1999 for use in the apnea of prematurity.
Drotaverine, a structural analogue of papaverine, acts as a direct smooth muscle relaxant through PDE inhibition and Ca2+ channel blocking (Patai et al., 2016). Caffeine is a centrally acting methylxanthine with a complex mechanism of action; however, it is believed that the principal mechanism involves adenosine receptors antagonism, due to PDE inhibition requiring very high concentrations (Atik et al., 2017). Tofisopam is described as an atypical benzodiazepine that doesn’t interact with γ-aminobutyric acid receptors, but instead acts as a selective PDE inhibitor with the highest affinities to PDE 4A1 (0.42 μM) and PDE 10A1 (0.92 μM) (Rundfeldt et al., 2010).
The published clinical evidence on these drugs is generally scarce. Drotaverine had been studied as a spasmolytic in irritable bowel syndrome (IBS) and labor augmentation. In IBS, drotaverine was shown to improve abdominal symptoms, including pain frequency and severity, and stool frequency, based on the results of a double-blind placebo-controlled study (Rai et al., 2014). In labor augmentation, it reduced the duration of first and second stages of labor, but with a limited impact on pain (Singh et al., 2004). In apnea of prematurity, caffeine reduces the incidence of bronchopulmonary dysplasia and improves the rate of survival without neurodevelopmental disability in long-term use, in addition to reducing the frequency of apnea of prematurity and the need for mechanical ventilation during the first 7 days of therapy (Schmidt et al., 2006; Schmidt et al., 2007). On tofisopam, literature sources state that it is potent in alleviating vegetative symptoms accompanying anxiety disorders as an anxiolytic without sedative-hypnotic, muscle relaxant and anticonvulsive properties (Szegó et al., 1993).
To summarize, the nervous system disorders currently represent a niche use for PDE inhibitors. Drotaverine and tofisopam lack clinical evidence to discuss its wider applicability. Caffeine is being considered as the mainstay of apnea of prematurity pharmacotherapy; however, it is not considered to act primarily as a PDE inhibitor.
Phosphodiesterase inhibitors in clinical research
In our dataset, we identified 52 PDE inhibitors which are either under ongoing investigation in clinical or pre-clinical studies, or which have been previously investigated but are either discontinued or have an unknown development status as of 2022. Among the agents in development (Tables 5, 7), indications are diverse, notably including CNS disorders, solid malignancies, metabolic, as well as inflammatory or immune-mediated disorders. Among the failed agents and/or agents in an unknown status (Table 6), the majority of indications are inflammatory disorders, CNS disorders, hematological and solid malignancies, and vascular disorders.
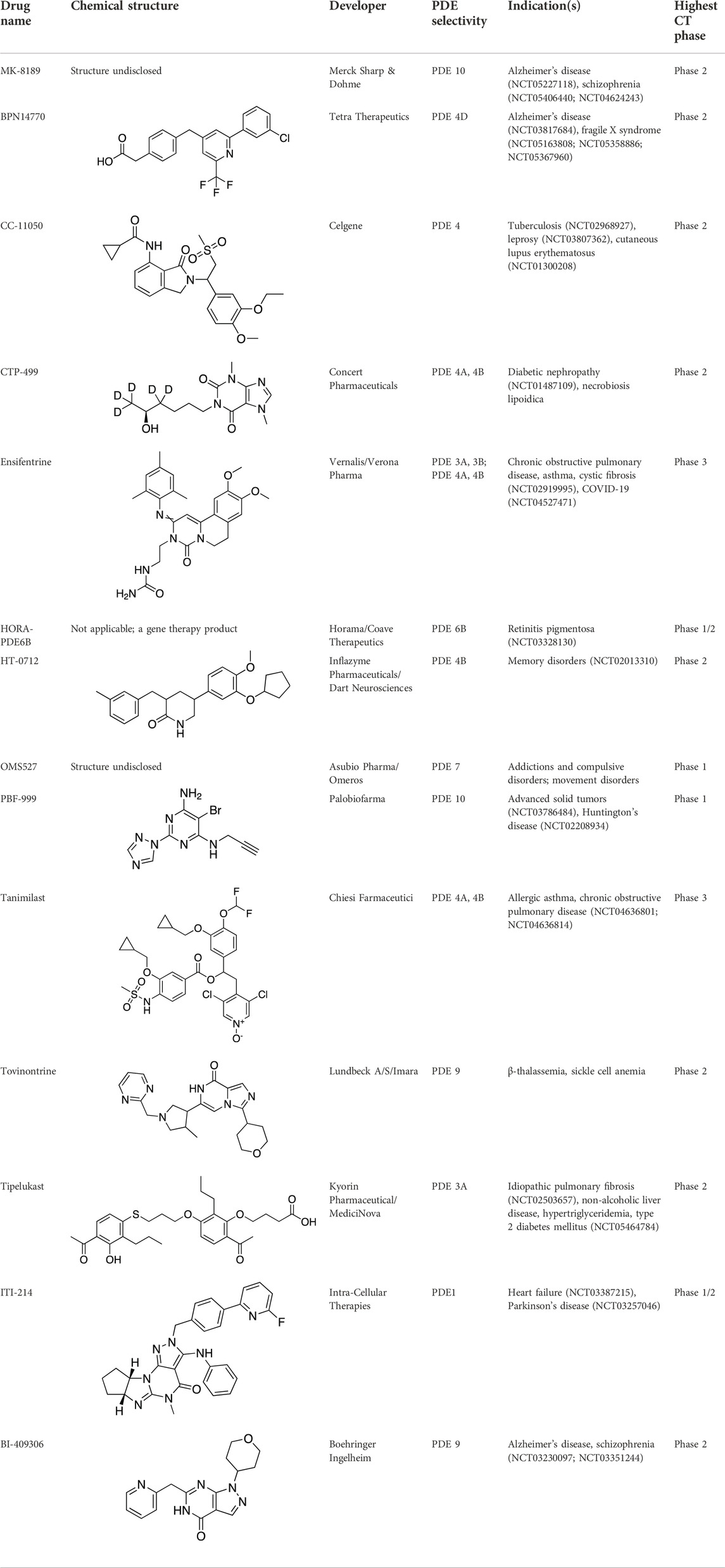
TABLE 5. Investigational PDE inhibitors in an ongoing clinical development. This table provides a summary on the novel PDE inhibitors that are currently investigated in clinical trials. The aggregated data on indications and clinical trials is listed as per studies identified on ClinicalTrials.gov, the EU Clinical Trials register and/or the developers’ websites. Chemical structures are derived from the PubChem database (Kim et al., 2021). Developer names are listed according to the relevant studies. Development status is listed according to the AdisInsight database., 2022 records11.
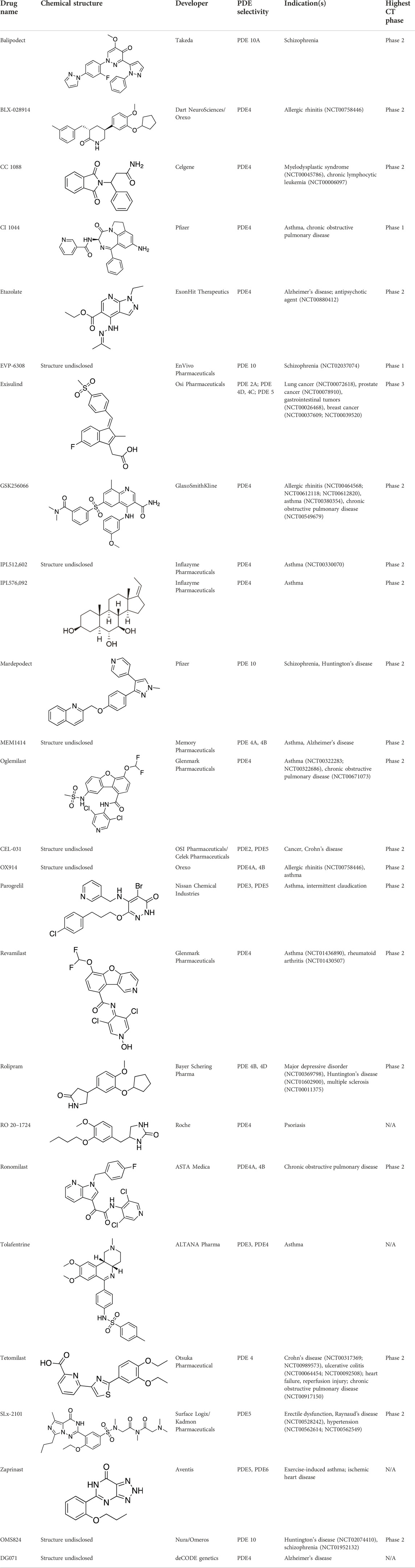
TABLE 6. Investigational PDE inhibitors in discontinued or unknown clinical development. This table provides a summary on the investigational PDE inhibitors that have been evaluated clinically, but whose development is either discontinued as of 2022 or is otherwise unknown. The aggregated data on clinical trials is listed as per the ClinicalTrials.gov, the EU Clinical Trials register and PubMed databases and/or the developers’ websites. Chemical structures are derived from the PubChem database (Kim et al., 2021). Developer names are listed according to the relevant studies. Development status is listed according to the AdisInsight database records.
Investigational phosphodiesterase inhibitors
Among the investigational CNS disorders, neurodegenerative and psychiatric conditions, such as Alzheimer’s disease (AD) and schizophrenia, are particularly prevalent. In schizophrenia, PDE 10A inhibition is reported as a plausible approach due to the expression pattern of PDE 10A in striatal medium spiny neurons and functional similarity of its inhibition to the dopamine D2 receptor antagonism (Snyder and Vanover, 2017). These agents include MK-8189, TAK-063 (also known as balipodect), FRM 6308 (EVP-6308), MP-10 (mardepodect) and OMS 824. Out of all these agents, published evidence on clinical studies exists for MK-8189 and TAK-063; both agents have showed acceptable tolerability, but the efficacy was reported to be low (Krogmann et al., 2019). As of 2022, only MK-8189 appears to be in the ongoing development, with a Phase 1 multiple ascending dose study of safety, tolerability, pharmacokinetics and the effect on QTc currently in the Recruiting stage (NCT05406440). In addition to PDE 10A, at least one PDE 9A inhibitor is undergoing development in psychiatric and neurodegenerative disorders, BI-409306 (osoresnontrine). The drug is currently in an Ongoing Phase 1 trial of the effect on ketamine-induced cognitive deficit in healthy male subjects (NCT04602221). It was previously investigated in cognitive impairment due to schizophrenia (Brown et al., 2019) and prodromal-to-mild AD (Frölich et al., 2019), but failed to demonstrate efficacy.
In AD, PDE4 inhibitors represent the bulk of investigational approaches (Klyucherev et al., 2022). The rationale here stems from the effect of cAMP on memory formation and cognition; an intracellular increase in cerebral cAMP activates protein kinase A associated with cAMP response element binding protein which is vital for synaptic plasticity (Tibbo et al., 2019). The drugs include BPN14770 (putative generic name zatolmilast), HT-0712, EHT 202 (etazolate), MEM1414, and DG071. BPN14770 has so far been investigated in three Phase 1 trials (NCT02648672, NCT02840279, and NCT03030105) in AD and was found to be associated with an improvement in working memory in healthy elderly subjects at low-to-mid doses, as reported in the developer’s press release (Tetra Discovery, 2016)8. Its ongoing development, however, goes predominantly in the direction of fragile X syndrome, a genetic neurodevelopmental disorder, characterized by intellectual disability, hyperactivity, sensory hypersensitivity, autistic-like behavior and susceptibility to seizures (Gurney et al., 2019). According to the results of a placebo-controlled Phase 2 study, BPN14770 resulted in a significant cognitive improvement related to language and improvements in caregiver scales rating language and daily functioning (Berry-Kravis et al., 2021). Additionally, there is one Recruiting Phase 2/3 study in male adolescents (NCT05163808), a Phase 3 Not yet recruiting study in male adults (NCT05358886) and an open-label extension Phase 3 Not yet recruiting study to assess the long-term safety and tolerability in subjects of the former studies. HT-0712 was reported to improve long-term memory in mice (Bourtchouladze et al., 2003; Peters et al., 2014) and facilitate behavioral recovery and cortical reorganization after an ischemic insult (MacDonald et al., 2007); however, no results from a clinical study could be identified. EHT 202 was investigated in a Phase 2 study as an adjunct to an acetylcholinesterase inhibitor in patients with mild-to-moderate AD (NCT00880412); no results had been published. Another CNS indication under ongoing investigation is Parkinson’s disease (PD); a previously mentioned PDE 1 inhibitor ITI-214 has shown reduction in neuroinflammation and enhancement of memory performance and cognition in preclinical models (Snyder et al., 2016; Pekcec et al., 2018; O’Brien et al., 2020). Clinically, it was reported to improve the motor functions, according to a Phase 1/2 trial in patients with mild to moderate PD (Davis et al., 2019).
Another notable investigational indication are neoplasms which is developing rapidly across numerous targets (reviewed in Attwood et al., 2021; Sokolov et al., 2021). In cancer, the PDE overexpression is observed in numerous types of cancer and its inhibition has shown positive effects on preclinical models (Peng et al., 2018). Antineoplastic PDE inhibitors in clinical development include PBF-999, exisulind, CEL-031 and CC-1088. PBF-999, a dual adenosine A2a receptor antagonist/PDE 10 inhibitor, is investigated in a Phase 1 Recruiting trial (NCT03786484) in patients with immunotherapy-naïve and pretreated advanced solid tumors. According to the developer’s website (PaloBioPharma Pipeline, 2022)9, colon cancer appears to be the primary indication. Exisulind is the sulfone derivative of sulindac, a selective apoptotic antineoplastic drug (Exisulind: Aptosyn, 2004). It had been evaluated in a wide range of tumors, the most recent being prostate (Weight et al., 2012), lung (Govindan et al., 2009), and melanoma (Curiel-Lewandrowski et al., 2012); however, no further development could be identified, possibly due to insufficient efficacy. CEL-031 is another apoptotic antineoplastic drug, developed for bladder cancer10; lack in its recent development could be due to poor oral bioavailability. CC-1088, a thalidomide analogue, was originally developed for myelodysplastic syndromes (Dredge, 2005). According to an in vitro study on myeloma cells (Molostvov et al., 2004), CC- 1088 acts by inhibiting production of vascular endothelial growth factor and interleukin (IL)- 6, and by inducing apoptosis11.
Among the other investigational indications of note are blood disorders, retinitis pigmentosa, necrobiosis lipoidica, autoimmune diseases, mycobacterial diseases, the novel coronavirus disease (COVID-19) and metabolic diseases. In blood disorders, tovinontrine is evaluated for sickle cell anemia in a Phase 2a Ongoing study to evaluate the long-term safety, tolerability and pharmacodynamics (NCT04053803). A gene therapy using an adeno-associated virus vector AAV2/5-hPDE6 is in a Phase 1/2 Ongoing trial (NCT03328130) in patients with retinitis pigmentosa. CC-11050 is currently evaluated in a Phase 2 Recruiting study in patients with leprosy and erythema nodosum leprosum (NCT03807362). The drug has also been studied in cutaneous lupus erythematosus, tuberculosis (TB) and HIV infection; published evidence in TB suggests that PDE inhibition can enhance the lung function recovery (Subbian et al., 2016; Wallis et al., 2021) and improve responsiveness to the antibacterial therapy (Subbian et al., 2016). In HIV, CC-11050 was shown to have no impact on CD4 counts or plasma viremia, but led to a decrease in natural killer cells and plasma IL-8 level, as well as to an increase in IL-6 (Boulougura et al., 2019). In COVID-19, ensifentrine had been investigated in a Phase 2 study to evaluate the efficacy and safety in the recovery of patients hospitalized with the disease (NCT04527471); the study is in an Unknown status since 2021, however. Nevertheless, tanimilast, another PDE 4 inhibitor, was found to exert a modulatory effect on the SARS-CoV-2 genomic ssRNA-induced pro-inflammatory and Th1- polarising potential of dendritic cells (Nguyen et al., 2022). MN-001 (tipelukast) was previously investigated in idiopathic pulmonary fibrosis (Phase 2, no results published) and currently is in a Not yet recruiting Phase 2 study in patients with non-alcoholic fatty liver disease, type 2 diabetes mellitus (T2DM), and hypertriglyceridemia (NCT05464784).
Experimental phosphodiesterase inhibitors
In addition to the PDE inhibitors in clinical development, mentioned above, we have identified several experimental drugs. Among the novel indications here is Duchenne muscular dystrophy, potentially manageable using a PDE 4 and PDE 5 inhibitor combination therapy. According to an in vivo study of a novel PDE 4 RP 73401 (piclamilast) in the mdx mice, it reduces the mRNA level of profibrotic genes in the gastrocnemius and diaphragm of the animal, and significantly reduced the percentage affected by fibrosis (Nio et al., 2017).
Many of experimental agents are PDE 10 inhibitors. JNJ-42314415, MR 1916, SEP-39, and ASP9436 are novel PDE 10 inhibitors with an antipsychotic activity; characterization of JNJ- 42314415, in particular, have showed that the effects of PDE 10A inhibition against dopaminergic stimulants and on catalepsy were potentiated by a D1 antagonist, providing a potential strategy to improve safety profile of PDE 10A inhibitors through reducing dopamine D2 and concomitantly potentiating dopamine D1 receptor-mediated neurotransmission (Megens et al., 2014). In addition, MR-1916, when combined with risperidone, resulted in a significant enhancement of the conditioned avoidance response in rats without affecting extrapyramidal side effects (Arakawa and Maehara, 2020).
Several agents target novel PDE targets. BRL-50481, a PDE 7 inhibitor, have showed neuroprotector (Chen et al., 2020) and anti-inflammatory properties (Kim et al., 2022) on animal models. Lu AF33241, a dual PDE 2A/PDE 10A inhibitor, was found to attenuate sub-chronic phencyclidine-induced deficits in novel object recognition and displayed antipsychotic-like activity (Redrobe et al., 2015). EHNA, a PDE 2 inhibitor, was recently shown to inhibit proliferation and stimulate migration of human osteosarcoma cells (Murata et al., 2019) and to inhibit growth and invasion of human malignant melanoma cells (Hiramoto et al., 2014). Interestingly, a PDE 3/4 inhibitor zardaverine was recently shown to act as a potent cytotoxic agent in embryonal rhabdomyosarcoma and cervical carcinoma cell lines (Cartledge et al., 2017), as well as in hepatocellular carcinoma (Sun et al., 2014); the mechanism, however, is independent of PDE inhibition.
One more agent to discuss is resveratrol, a naturally occurring polyphenol marketed as a dietary supplement due to its antioxidant properties (Salehi et al., 2018). Several preclinical studies have showed that some of its biological properties are mediated via PDE inhibition. Resveratrol was found to impart neuroprotection in brain ischemia by inhibiting PDEs and regulating the cAMP/AMPK/SIRT1 pathway, which reduces ATP energy consumption (Wan et al., 2016). Additionally, it was found to enhance glucose-stimulated insulin secretion by pancreatic β-cells (Rouse et al., 2014) and ameliorate aging-associated metabolic disorders by multiple mechanisms, including an increase in mitochondrial biogenesis and protection against diet-induced obesity and glucose intolerance (Park et al., 2012).
Several studies involving resveratrol were done on human subjects as well, such as AD, Gulf War syndrome, T2DM, postmenopausal osteoporosis and COVID-19. In AD, it was found to alter the Aβ40 levels and the brain volume loss increase (Turner et al., 2015), decrease MMP9 levels, modulate neuro-inflammation and induce adaptive immunity (Moussa et al., 2017); the mechanism involved may not be related to the PDE inhibition, however. In Gulf War syndrome, a chronic multi-symptom disorder of an unknown etiology that affects veterans of the Gulf War, it reduced the symptom severity (Hodgin et al., 2021). In T2DM, a systematic review of three trials has reported a neutral impact on glycosylated hemoglobin A1c (mean difference 0.1% vs. placebo), fasting blood glucose levels (2 mg/dl difference vs. placebo), and insulin resistance (−0.35 difference vs. placebo) (Jeyaraman et al., 2020). In postmenopausal osteoporosis, resveratrol has showed the potential to slow bone loss in the lumbar spine and femoral neck (Wong et al., 2020). In COVID-19, resveratrol was shown to reduce angiotensin-converting enzyme 2 expression in the adipose tissue, a potential SARS-CoV-2 reservoir contributing to massive viral spread in COVID-19 patients with obesity (de Ligt et al., 2021). The direction and status of overall clinical development for this compound is uncertain, however; among the major barriers reported for its development is poor bioavailability and solubility (Sun et al., 2014).
Discussion
Here, we provide a comprehensive study of the clinical trials and FDA approved drugs in relationship to their therapeutic targets. As of 2022, the majority of commercially available PDE inhibitors are marketed in cardiovascular disorders and inflammatory diseases, offering valuable therapeutic options. Particularly, PDE 5 inhibitor are the first-line option in ED, while PDE 4 inhibitors are actively explored in inflammatory conditions of the skin and COPD, showing variable, but promising results. The ongoing clinical research moves towards diversification with CNS disorders being particularly prevalent, however, specifically schizophrenia and AD. Other interesting directions are mycobacterial infectious diseases, such as tuberculosis and leprosy, and potential applicability in COVID-19. The clinical evidence on efficacy is currently limited and often conflicting, however. Many of the investigational PDE inhibitors, particularly drugs investigated in asthma or COPD as an indication are either discontinued, suspended or are in an otherwise unknown status. A common reason seems to be lack of efficacy and/or unsatisfactory ADR profile; however, there are gaps in published evidence on most agents, making it difficult to assess the issue.
Among the marketed agents, current issues include compliance-limiting ADRs and often insufficient clinical efficacy. A potential solution to the lack of efficacy could be directing drug development efforts to evaluate combinatory regimens, as suggested for PDE 5 inhibitors in ED; combining PDE 5 inhibitors with other therapeutic approaches, such as antioxidants, showed improved outcomes without increasing ADRs (Mykoniatis et al., 2021). A similar strategy is suggested for PDE 4 inhibitors in inflammatory diseases (Li et al., 2018). The issue of compliance-limiting ADRs could be amended by adjusting prescribing regimens and/or via rational drug design based on evidence of the effect of structural features on safety. Additionally, increasing the isoform specificity and/or designing allosteric modulators is mentioned in the literature as a plausible strategy to improve the ADR profile and efficacy (Li et al., 2018; Chen and Yan, 2021).
PDE enzymes are a long-established pharmacological target and the extensive research involving these proteins contributes to a continuous interest towards further expansion of the PDE inhibitors’ clinical use. The future development continues to diversify, particularly thanks to the experimental agents and/or agents in the preclinical development (Table 7). We expect this trend to continue in future. Among the particularly interesting and promising novel indications is tuberculosis, given how challenging the treatment can be and the prevalence of the disease. CNS disorders, such as schizophrenia and AD, are another interesting direction; however, there are recognized gaps in understanding the molecular mechanisms of psychiatric (Menniti et al., 2021) and neurodegenerative disorders (Tibbo et al., 2019) that need to be filled in order to maximize the CNS drug development efforts. Neoplasms are another important direction; several PDE inhibitors have shown antitumor activity, but there is a continuous need for better understanding of PDE molecular biology (Peng et al., 2018) for this direction to gain traction.
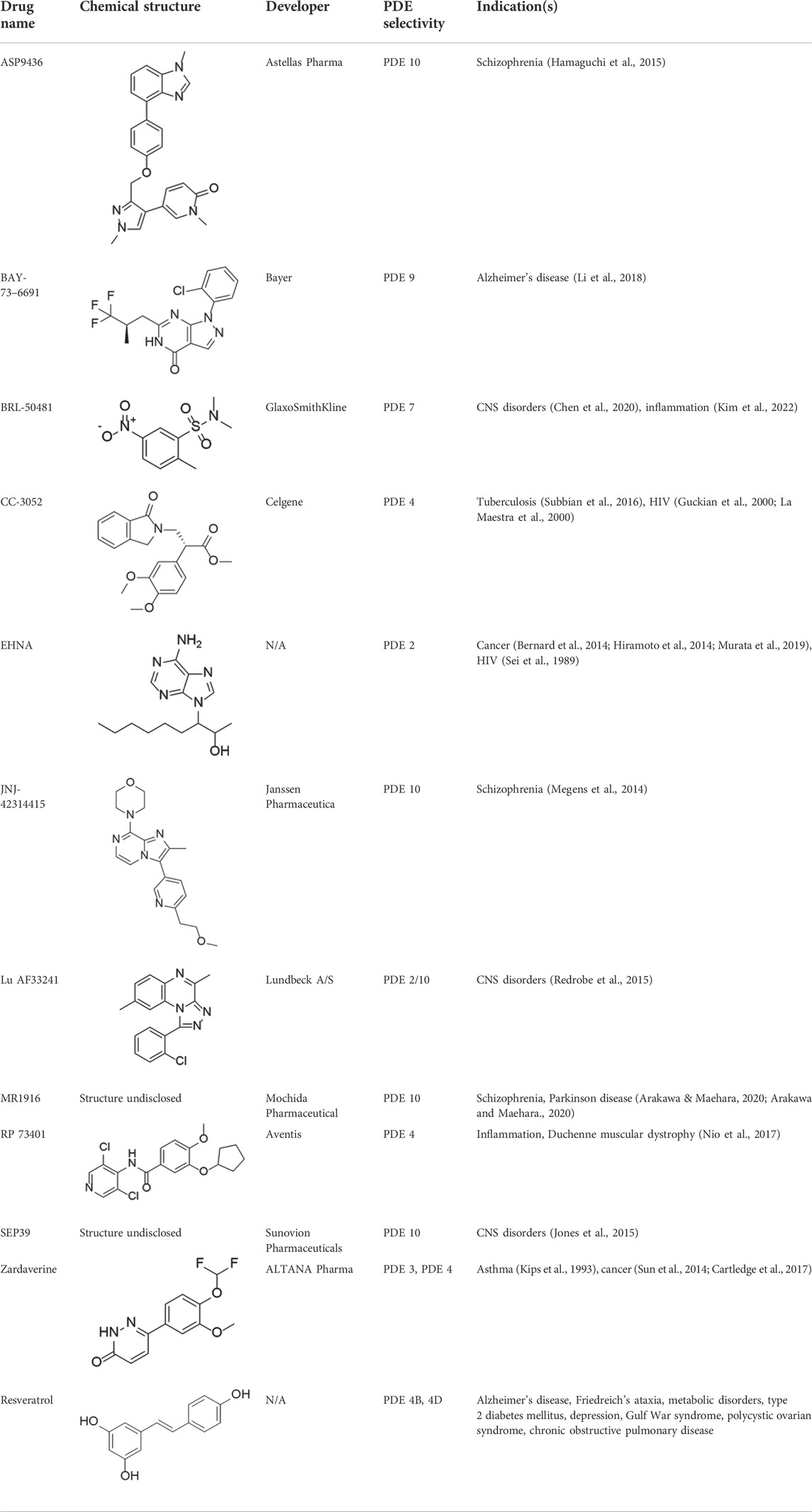
TABLE 7. Selected experimental PDE inhibitors. This table provides a summary on some of the experimental PDE inhibitors in pre-clinical studies. The aggregated data on studies is listed as per the PubMed database. Chemical structures are derived from the PubChem database (Kim et al., 2021). Developer names are listed according to the relevant studies.
The global market of PDE inhibitors is currently driven predominantly by the PDE 5 inhibitors and the unmet clinical needs and the increase in ED prevalence is also considered to contribute (Transparency Market Research, 2021). It is expected to grow by USD 2.86 billion throughout 2022–2026 period (Technavio, 2022), with the compound annual growth rate (CAGR) of 5.81%. During the 2022–2027 forecast period the market CAGR is expected to be 6.2% (Market Data Forecast, 2022). The ongoing COVID-19 pandemic has impacted the market, at least partially due to the clinical uses of PDE inhibitors in diseases that exacerbate the novel SARS-CoV-2 infection, such as CVDs and obstructive pulmonary diseases (Market Data Forecast, 2022). One of the largest challenges to the market’s growth can be considered the negative ADR profile that contributes to the lack of FDA and EMA approvals (Market Data Forecast, 2022).
Conclusion
Here, we provide a comprehensive overview of PDE inhibitors with focus on novel agents and indications. The current clinical uses of PDE inhibitors include COPD, vascular and cardiovascular disorders and skin inflammatory disorders. There is a trend for diversification of clinical uses for PDE inhibitors with numerous novel agents being in clinical and preclinical development. While a significant portion of drugs under development have not been successful, the scientific community recognizes some of the present gaps in knowledge that had been slowing the development. The continuous efforts to develop and evaluate both the approved and novel agents will undoubtedly expand our understanding of the PDE pharmacology and will likely contribute to the further growth.
Author contributions
Conceptualisation: AB, MA, JJ, VC, VT, WL, HS; Analysis: AB, MA, JJ, VC, VT, WL, HS; Funding acquisition: HS; Investigation: AB, MA, JJ, WL,; Methodology: AB, MA, VC, VT, HS; Project administration: HS; Supervision: HS; Validation: AB, MA, JJ, VC, VT, WL, HS; Visualisation: AB, MA, JJ, WL; Writing—original draft: AB, MA, JJ, VC, VT, WL, HS; Writing—review and editing: AB, MA, JJ, VC, VT, WL, HS.
Funding
HS is supported by the Novo Nordisk Foundation.
Conflict of interest
Authors VC and VT were employed by Advanced Molecular Technologies LLC.
The remaining authors declare that the research was conducted in the absence of any commercial or financial relationships that could be construed as a potential conflict of interest.
Publisher’s note
All claims expressed in this article are solely those of the authors and do not necessarily represent those of their affiliated organizations, or those of the publisher, the editors and the reviewers. Any product that may be evaluated in this article, or claim that may be made by its manufacturer, is not guaranteed or endorsed by the publisher.
Footnotes
2https://www.accessdata.fda.gov/scripts/cder/daf/index.cfm
3https://www.ema.europa.eu/en/medicines
4https://www.nice.org.uk/guidance/published?ngt=Technology%20appraisal%20guidance&ndt=Guidance
5https://clinicaltrials.gov/ct2/home
6https://www.clinicaltrialsregister.eu/ctr-search/search
7https://www.who.int/news-room/fact-sheets/detail/cardiovascular-diseases-(cvds)
8https://tetratherapeutics.com/wp-content/uploads/2016/11/FINAL-Tetra-Phase-1-121616-FINAL.pdf
9https://www.palobiofarma.com/pipeline-2/
10https://www.businesswire.com/news/home/20111222005044/en/Celek-Pharmaceuticals-Receives-National-Cancer-Institute-Award-to-Develop-CEL-031-for-the-Treatment-of-Bladder-Cancer
11https://adisinsight.springer.com
References
AdisInsight Database (2022). AdisInsight database (2022). Available at: https://adisinsight.springer.com. [Accessed on August, 2022].
Amer, M. S. (1977). Cyclic nucleotides as targets for drug design. Adv. Drug Res. 12, 1–38. doi:10.1016/b978-0-12-013312-3.50004-2
Andersson, K. E. (2018). PDE5 inhibitors - pharmacology and clinical applications 20 years after sildenafil discovery. Br. J. Pharmacol. 175 (13), 2554–2565. doi:10.1111/bph.14205
Arakawa, K., and Maehara, S. (2020). Combination of the phosphodiesterase 10A inhibitor, MR1916 with risperidone shows additive antipsychotic-like effects without affecting cognitive enhancement and cataleptic effects in rats. Neuropsychopharmacol. Rep. 40 (2), 190–195. doi:10.1002/npr2.12108
Atik, A., Harding, R., De Matteo, R., Kondos-Devcic, D., Cheong, J., Doyle, L. W., et al. (2017). Caffeine for apnea of prematurity: Effects on the developing brain. Neurotoxicology 58, 94–102. doi:10.1016/j.neuro.2016.11.012
Attwood, M. M., Fabbro, D., Sokolov, A. V., Knapp, S., and Schiöth, H. B. (2021). Trends in kinase drug discovery: Targets, indications and inhibitor design. Nat. Rev. Drug Discov. 20 (11), 839–861. doi:10.1038/s41573-021-00252-y
Attwood, M. M., Rask-Andersen, M., and Schiöth, H. B. (2018). Orphan drugs and their impact on pharmaceutical development. Trends Pharmacol. Sci. 39 (6), 525–535. doi:10.1016/j.tips.2018.03.003
Ayres, J. K., and Maani, C. V. (2022). “Milrinone,” in StatPearls (Florida, United States: StatPearls Publishing).
Baillie, G. S., Tejeda, G. S., and Kelly, M. P. (2019). Therapeutic targeting of 3', 5'-cyclic nucleotide phosphodiesterases: Inhibition and beyond. Nat. Rev. Drug Discov. 18 (10), 770–796. doi:10.1038/s41573-019-0033-4
Barnes, H., Brown, Z., Burns, A., and Williams, T. (2019). Phosphodiesterase 5 inhibitors for pulmonary hypertension. Cochrane Database Syst. Rev. 1 (1), CD012621. doi:10.1002/14651858.CD012621.pub2
Beavo, J. A., Rogers, N. L., Crofford, O. B., Hardman, J. G., Sutherland, E. W., and Newman, E. V. (1970). Effects of xanthine derivatives on lipolysis and on adenosine 3', 5'-monophosphate phosphodiesterase activity. Mol. Pharmacol. 6 (6), 597–603.
Bell, J. (2005). Amlexanox for the treatment of recurrent aphthous ulcers. Clin. Drug Investig. 25 (9), 555–566. doi:10.2165/00044011-200525090-00001
Bender, A. T., and Beavo, J. A. (2006). Cyclic nucleotide phosphodiesterases: Molecular regulation to clinical use. Pharmacol. Rev. 58 (3), 488–520. doi:10.1124/pr.58.3.5
Bernard, J. J., Lou, Y. R., Peng, Q. Y., Li, T., and Lu, Y. P. (2014). PDE2 is a novel target for attenuating tumor formation in a mouse model of UVB-induced skin carcinogenesis. PLoS One 9 (10), e109862. doi:10.1371/journal.pone.0109862
Berry-Kravis, E. M., Harnett, M. D., Reines, S. A., Reese, M. A., Ethridge, L. E., Outterson, A. H., et al. (2021). Inhibition of phosphodiesterase-4D in adults with fragile X syndrome: A randomized, placebo-controlled, phase 2 clinical trial. Nat. Med. 27 (5), 862–870. doi:10.1038/s41591-021-01321-w
Birgegård, G. (2016). The use of anagrelide in myeloproliferative neoplasms, with focus on essential thrombocythemia. Curr. Hematol. Malig. Rep. 11 (5), 348–355. doi:10.1007/s11899-016-0335-0
Bjermer, L., Abbott-Banner, K., and Newman, K. (2019). Efficacy and safety of a first-in-class inhaled PDE3/4 inhibitor (ensifentrine) vs salbutamol in asthma. Pulm. Pharmacol. Ther. 58, 101814. doi:10.1016/j.pupt.2019.101814
Boehncke, W. H., and Schön, M. P. (2015). Psoriasis. Lancet 386 (9997), 983–994. doi:10.1016/S0140-6736(14)61909-7
Boulougoura, A., Gabriel, E., Laidlaw, E., Khetani, V., Arakawa, K., Higgins, J., et al. (2019). A phase I, randomized, controlled clinical study of CC-11050 in people living with HIV with suppressed plasma viremia on antiretroviral therapy (APHRODITE). Open Forum Infect. Dis. 6 (6), ofz246. doi:10.1093/ofid/ofz246
Bourtchouladze, R., Lidge, R., Catapano, R., Stanley, J., Gossweiler, S., Romashko, D., et al. (2003). A mouse model of rubinstein-taybi syndrome: Defective long-term memory is ameliorated by inhibitors of phosphodiesterase 4. Proc. Natl. Acad. Sci. U. S. A. 100 (18), 10518–10522. doi:10.1073/pnas.1834280100
Brown, D., Nakagome, K., Cordes, J., Brenner, R., Grunder, G., Keefe, R. S. E., et al. (2019). Evaluation of the efficacy, safety, and tolerability of BI 409306, a novel phosphodiesterase 9 inhibitor, in cognitive impairment in schizophrenia: A randomized, double-blind, placebo-controlled, phase II trial. Schizophr. Bull. 45 (2), 350–359. doi:10.1093/schbul/sby049
Brown, T., Forster, R. B., Cleanthis, M., Mikhailidis, D. P., Stansby, G., and Stewart, M. (2021). Cilostazol for intermittent claudication. Cochrane Database Syst. Rev. 6 (6), CD003748. doi:10.1002/14651858.CD003748.pub5
Cada, D. J., Ingram, K., and Baker, D. E. (2014). Hosp. Pharm. 49 (8), 752–762. doi:10.1310/hpj4908-752
Calverley, P. M., Sanchez-Toril, F., McIvor, A., Teichmann, P., Bredenbroeker, D., and Fabbri, L. M. (2007). Effect of 1-year treatment with roflumilast in severe chronic obstructive pulmonary disease. Am. J. Respir. Crit. Care Med. 176 (2), 154–161. doi:10.1164/rccm.200610-1563OC
Cartledge, D. M., Robbins, K. M., Drake, K. M., Sternberg, R., Stabley, D. L., Gripp, K. W., et al. (2017). Cytotoxicity of zardaverine in embryonal rhabdomyosarcoma from a costello syndrome patient. Front. Oncol. 7, 42. doi:10.3389/fonc.2017.00042
Chau, V. Q., Salloum, F. N., Hoke, N. N., Abbate, A., and Kukreja, R. C. (2011). Mitigation of the progression of heart failure with sildenafil involves inhibition of RhoA/Rho-kinase pathway. Am. J. Physiol. Heart Circ. Physiol. 300 (6), H2272–H2279. doi:10.1152/ajpheart.00654.2010
Chen, L., Staubli, S. E., Schneider, M. P., Kessels, A. G., Ivic, S., Bachmann, L. M., et al. (2015). Phosphodiesterase 5 inhibitors for the treatment of erectile dysfunction: A trade-off network meta-analysis. Eur. Urol. 68 (4), 674–680. doi:10.1016/j.eururo.2015.03.031
Chen, S., and Yan, C. (2021). An update of cyclic nucleotide phosphodiesterase as a target for cardiac diseases. Expert Opin. Drug Discov. 16 (2), 183–196. doi:10.1080/17460441.2020.1821643
Chen, Y., Li, S., Zhong, X., Kang, Z., and Chen, R. (2020). PDE-7 inhibitor BRL-50481 reduces neurodegeneration and long-term memory deficits in mice following sevoflurane exposure. ACS Chem. Neurosci. 11 (9), 1353–1358. doi:10.1021/acschemneuro.0c00106
Choi, O. H., Shamim, M. T., Padgett, W. L., and Daly, J. W. (1988). Caffeine and theophylline analogues: Correlation of behavioral effects with activity as adenosine receptor antagonists and as phosphodiesterase inhibitors. Life Sci. 43 (5), 387–398. doi:10.1016/0024-3205(88)90517-6
Chong, L. Y. Z., Satya, K., Kim, B., and Berkowitz, R. (2018). Milrinone dosing and a culture of caution in clinical practice. Cardiol. Rev. 26 (1), 35–42. doi:10.1097/CRD.0000000000000165
Conti, M., and Beavo, J. (2007). Biochemistry and physiology of cyclic nucleotide phosphodiesterases: Essential components in cyclic nucleotide signaling. Annu. Rev. Biochem. 76, 481–511. doi:10.1146/annurev.biochem.76.060305.150444
Coquil, J. F., Franks, D. J., Wells, J. N., Dupuis, M., and Hamet, P. (1980). Characteristics of a new binding protein distinct from the kinase for guanosine 3':5'-monophosphate in rat platelets. Biochim. Biophys. Acta 631 (1), 148–165. doi:10.1016/0304-4165(80)90063-x
Curiel-Lewandrowski, C., Swetter, S. M., Einspahr, J. G., Hsu, C. H., Nagle, R., Sagerman, P., et al. (2012). Randomized, double-blind, placebo-controlled trial of sulindac in individuals at risk for melanoma: Evaluation of potential chemopreventive activity. Cancer 118 (23), 5848–5856. doi:10.1002/cncr.27540
Davis, R. L., Takayasu, H., Eberwine, M., and Myres, J. (1989). Cloning and characterization of mammalian homologs of the Drosophila dunce+ gene. Proc. Natl. Acad. Sci. U. S. A. 86 (10), 3604–3608. doi:10.1073/pnas.86.10.3604
Davis, R., Riesenberg, R., Laskowitz, D., Guptill, J., Sanders, L., Cooney, J., et al. (2019). ITI-214, A novel phosphodiesterase I inhibitor for the treatment of Parkinson’s disease: Results from A phase I/II clinical study (P2.8-051). Neurology 92 (15 Supplement), P2.8–051.
de Ligt, M., Hesselink, M. K. C., Jorgensen, J., Hoebers, N., Blaak, E. E., and Goossens, G. H. (2021). Resveratrol supplementation reduces ACE2 expression in human adipose tissue. Adipocyte 10 (1), 408–411. doi:10.1080/21623945.2021.1965315
Ding, B., Abe, J. I., Wei, H., Huang, Q., Walsh, R. A., Molina, C. A., et al. (2005). Functional role of phosphodiesterase 3 in cardiomyocyte apoptosis: Implication in heart failure. Circulation 111 (19), 2469–2476. doi:10.1161/01.CIR.0000165128.39715.87
Drobnis, E. Z., and Nangia, A. K. (2017). Phosphodiesterase inhibitors (PDE inhibitors) and male reproduction. Adv. Exp. Med. Biol. 1034, 29–38. doi:10.1007/978-3-319-69535-8_5
Dyke, H. J., and Montana, J. G. (2002). Update on the therapeutic potential of PDE4 inhibitors. Expert Opin. Investig. Drugs 11 (1), 1–13. doi:10.1517/13543784.11.1.1
Edwards, C. J., Blanco, F. J., Crowley, J., Birbara, C. A., Jaworski, J., Aelion, J., et al. (2016). Apremilast, an oral phosphodiesterase 4 inhibitor, in patients with psoriatic arthritis and current skin involvement: A phase III, randomised, controlled trial (PALACE 3). Ann. Rheum. Dis. 75 (6), 1065–1073. doi:10.1136/annrheumdis-2015-207963
Essayan, D. M. (2001). Cyclic nucleotide phosphodiesterases. J. Allergy Clin. Immunol. 108 (5), 671–680. doi:10.1067/mai.2001.119555
European Medicines Agency. EMA Medicines database. (2019a). Available at: https://www.ema.europa.eu/en/medicines. [Accessed on February, 2020].
European Medicines Agency. E.U. Clinical trials register. (2019b). Available at: https://www.clinicaltrialsregister.eu/ctr-search/search. [Accessed on February, 2020].
Exisulind: Aptosyn (2004). Exisulind: Aptosyn, FGN 1, prevatac, sulindac sulfone. Drugs R. D. 5 (4), 220–226. doi:10.2165/00126839-200405040-00007
Fan Chung, K. (2006). Phosphodiesterase inhibitors in airways disease. Eur. J. Pharmacol. 533 (1-3), 110–117. doi:10.1016/j.ejphar.2005.12.059
Francis, S. H., Blount, M. A., and Corbin, J. D. (2011a). Mammalian cyclic nucleotide phosphodiesterases: Molecular mechanisms and physiological functions. Physiol. Rev. 91 (2), 651–690. doi:10.1152/physrev.00030.2010
Francis, S. H., Lincoln, T. M., and Corbin, J. D. (1980). Characterization of a novel cGMP binding protein from rat lung. J. Biol. Chem. 255 (2), 620–626. doi:10.1016/s0021-9258(19)86221-x
Francis, S. H., Sekhar, K. R., Ke, H., and Corbin, J. D. (2011b). Inhibition of cyclic nucleotide phosphodiesterases by methylxanthines and related compounds. Handb. Exp. Pharmacol. 200, 93–133. doi:10.1007/978-3-642-13443-2_4
Frölich, L., Wunderlich, G., Thamer, C., Roehrle, M., Garcia, M., and Dubois, B. (2019). Evaluation of the efficacy, safety and tolerability of orally administered BI 409306, a novel phosphodiesterase type 9 inhibitor, in two randomised controlled phase II studies in patients with prodromal and mild Alzheimer's disease. Alzheimers Res. Ther. 11 (1), 18. doi:10.1186/s13195-019-0467-2
Gardner, C., Robas, N., Cawkill, D., and Fidock, M. (2000). Cloning and characterization of the human and mouse PDE7B, a novel cAMP-specific cyclic nucleotide phosphodiesterase. Biochem. Biophys. Res. Commun. 272 (1), 186–192. doi:10.1006/bbrc.2000.2743
Geerts, H., Spiros, A., and Roberts, P. (2017). Phosphodiesterase 10 inhibitors in clinical development for CNS disorders. Expert Rev. Neurother. 17 (6), 553–560. doi:10.1080/14737175.2017.1268531
Gilotra, N. A., DeVore, A. D., Povsic, T. J., Hays, A. G., Hahn, V. S., Agunbiade, T. A., et al. (2021). Acute hemodynamic effects and tolerability of phosphodiesterase-1 inhibition with ITI-214 in human systolic heart failure. Circ. Heart Fail. 14 (9), e008236. doi:10.1161/CIRCHEARTFAILURE.120.008236
Giorgi, M., Cardarelli, S., Ragusa, F., Saliola, M., Biagioni, S., Poiana, G., et al. (2020). Phosphodiesterase inhibitors: Could they Be beneficial for the treatment of COVID-19? Int. J. Mol. Sci. 21 (15), 5338. doi:10.3390/ijms21155338
Govindan, R., Wang, X., Baggstrom, M. Q., Burdette-Radoux, S., Hodgson, L., Vokes, E. E., et al. (2009). A phase II study of carboplatin, etoposide, and exisulind in patients with extensive small cell lung cancer: Calgb 30104. J. Thorac. Oncol. 4 (2), 220–226. doi:10.1097/JTO.0b013e3181951eb0
Guckian, M., Dransfield, I., Hay, P., and Dalgleish, A. G. (2000). Thalidomide analogue CC-3052 reduces HIV+ neutrophil apoptosis in vitro. Clin. Exp. Immunol. 121 (3), 472–479. doi:10.1046/j.1365-2249.2000.01332.x
Gurney, M. E. (2019). Genetic association of phosphodiesterases with human cognitive performance. Front. Mol. Neurosci. 12, 22. doi:10.3389/fnmol.2019.00022
Gurney, M. E., Nugent, R. A., Mo, X., Sindac, J. A., Hagen, T. J., Fox, D., et al. (2019). Design and synthesis of selective phosphodiesterase 4D (PDE4D) allosteric inhibitors for the treatment of fragile X syndrome and other brain disorders. J. Med. Chem. 62 (10), 4884–4901. doi:10.1021/acs.jmedchem.9b00193
Halene, T. B., and Siegel, S. J. (2007). PDE inhibitors in psychiatry--future options for dementia, depression and schizophrenia? Drug Discov. Today 12 (19-20), 870–878. doi:10.1016/j.drudis.2007.07.023
Hamaguchi, W., Masuda, N., Miyamoto, S., Kikuchi, S., Narazaki, F., Shiina, Y., et al. (2015). Addressing phototoxicity observed in a novel series of biaryl derivatives: Discovery of potent, selective and orally active phosphodiesterase 10A inhibitor ASP9436. Bioorg. Med. Chem. 23 (13), 3351–3367. doi:10.1016/j.bmc.2015.04.052
Han, P., Zhu, X., and Michaeli, T. (1997). Alternative splicing of the high affinity cAMP-specific phosphodiesterase (PDE7A) mRNA in human skeletal muscle and heart. J. Biol. Chem. 272 (26), 16152–16157. doi:10.1074/jbc.272.26.16152
Hiramoto, K., Murata, T., Shimizu, K., Morita, H., Inui, M., Manganiello, V. C., et al. (2014). Role of phosphodiesterase 2 in growth and invasion of human malignant melanoma cells. Cell. Signal. 26 (9), 1807–1817. doi:10.1016/j.cellsig.2014.03.031
Hodgin, K. S., Donovan, E. K., Kekes-Szabo, S., Lin, J. C., Feick, J., Massey, R. L., et al. (2021). A placebo-controlled, pseudo- randomized, crossover trial of botanical agents for Gulf war illness: Resveratrol (polygonum cuspidatum), luteolin, and fisetin (rhus succedanea). Int. J. Environ. Res. Public Health 18 (5), 2483. doi:10.3390/ijerph18052483
Janjua, S., Fortescue, R., and Poole, P. (2020). Phosphodiesterase-4 inhibitors for chronic obstructive pulmonary disease. Cochrane Database Syst. Rev. 5 (5), CD002309. doi:10.1002/14651858.CD002309.pub6
Jeyaraman, M. M., Al-Yousif, N. S. H., Singh Mann, A., Dolinsky, V. W., Rabbani, R., Zarychanski, R., et al. (2020). Resveratrol for adults with type 2 diabetes mellitus. Cochrane Database Syst. Rev. 1 (1), CD011919. doi:10.1002/14651858.CD011919.pub2
Jones, P. G., Hewitt, M. C., Campbell, J. E., Quinton, M. S., Engel, S., Lew, R., et al. (2015). Pharmacological evaluation of a novel phosphodiesterase 10A inhibitor in models of antipsychotic activity and cognition. Pharmacol. Biochem. Behav. 135, 46–52. doi:10.1016/j.pbb.2015.04.017
Joskova, M., Mokry, J., and Franova, S. (2020). Respiratory cilia as a therapeutic target of phosphodiesterase inhibitors. Front. Pharmacol. 11, 609. doi:10.3389/fphar.2020.00609
Kawamatawong, T. (2021). Phosphodiesterase-4 inhibitors for non-COPD respiratory diseases. Front. Pharmacol. 12, 518345. doi:10.3389/fphar.2021.518345
Kawasaki, A., Hoshino, K., Osaki, R., Mizushima, Y., and Yano, S. (1992). Effect of ibudilast: A novel antiasthmatic agent, on airway hypersensitivity in bronchial asthma. J. Asthma 29 (4), 245–252. doi:10.3109/02770909209048938
Kim, H. J., Song, J. Y., Park, T. I., Choi, W. S., Kim, J. H., Kwon, O. S., et al. (2022). The effects of BRL-50481 on ovalbumin-induced asthmatic lung inflammation exacerbated by co-exposure to Asian sand dust in the murine model. Arch. Pharm. Res. 45 (1), 51–62. doi:10.1007/s12272-021-01367-x
Kim, S., Chen, J., Cheng, T., Gindulyte, A., He, J., He, S., et al. (2021). PubChem in 2021: New data content and improved web interfaces. Nucleic Acids Res. 49 (D1), D1388–D1395. doi:10.1093/nar/gkaa971
Kips, J. C., Joos, G. F., Peleman, R. A., and Pauwels, R. A. (1993). The effect of zardaverine, an inhibitor of phosphodiesterase isoenzymes III and IV, on endotoxin-induced airway changes in rats. Clin. Exp. Allergy 23 (6), 518–523. doi:10.1111/j.1365-2222.1993.tb03240.x
Klyucherev, T. O., Olszewski, P., Shalimova, A. A., Chubarev, V. N., Tarasov, V. V., Attwood, M. M., et al. (2022). Advances in the development of new biomarkers for Alzheimer's disease. Transl. Neurodegener. 11 (1), 25. doi:10.1186/s40035-022-00296-z
Knott, E. P., Assi, M., Rao, S. N., Ghosh, M., and Pearse, D. D. (2017). Phosphodiesterase inhibitors as a therapeutic approach to neuroprotection and repair. Int. J. Mol. Sci. 18 (4), 696. doi:10.3390/ijms18040696
Kotera, J., Fujishige, K., and Omori, K. (2000). Immunohistochemical localization of cGMP-binding cGMP-specific phosphodiesterase (PDE5) in rat tissues. J. Histochem. Cytochem. 48 (5), 685–693. doi:10.1177/002215540004800512
Krogmann, A., Peters, L., von Hardenberg, L., Bödeker, K., Nöhles, V. B., and Correll, C. U. (2019). Keeping up with the therapeutic advances in schizophrenia: A review of novel and emerging pharmacological entities. CNS Spectr. 24 (S1), 38–69. doi:10.1017/S109285291900124X
Kwiatkowski, J., Kuliszkiewicz-Janus, M., Rymer, W., Jaźwiec, B., and Małecki, R. (2021). Treatment of essential thrombocythemia with anagrelide is associated with an increased risk of worsened kidney function. Pharmacology 106 (5-6), 316–322. doi:10.1159/000513377
La Maestra, L., Zaninoni, A., Marriott, J. B., Lazzarin, A., Dalgleish, A. G., and Barcellini, W. (2000). The thalidomide analogue CC-3052 inhibits HIV-1 and tumour necrosis factor-alpha (TNF-alpha) expression in acutely and chronically infected cells in vitro. Clin. Exp. Immunol. 119 (1), 123–129. doi:10.1046/j.1365-2249.2000.01117.x
Lau, C. B., and Smith, G. P. (2022). Recurrent aphthous stomatitis: A comprehensive review and recommendations on therapeutic options. Dermatol. Ther. 35 (6), e15500. doi:10.1111/dth.15500
Li, H., Zuo, J., and Tang, W. (2018). Phosphodiesterase-4 inhibitors for the treatment of inflammatory diseases. Front. Pharmacol. 9, 1048. doi:10.3389/fphar.2018.01048
MacDonald, E., Van der Lee, H., Pocock, D., Cole, C., Thomas, N., VandenBerg, P. M., et al. (2007). A novel phosphodiesterase type 4 inhibitor, HT-0712, enhances rehabilitation-dependent motor recovery and cortical reorganization after focal cortical ischemia. Neurorehabil. Neural Repair 21 (6), 486–496. doi:10.1177/1545968307305521
Market Data Forecast. (2022). Global PDE inhibitors market size, share, trends, COVID-19 impact and growth analysis report – segmented by application, Drug Class and Region – Industry Forecast (2022 to 2027). Available at: https://www.marketdataforecast.com/market-reports/pde-inhibitors-market-revenue. [Accessed on August, 2022].
Matera, M. G., Page, C., and Cazzola, M. (2014). PDE inhibitors currently in early clinical trials for the treatment of asthma. Expert Opin. Investig. Drugs 23 (9), 1267–1275. doi:10.1517/13543784.2014.921157
Maurice, D. H., Ke, H., Ahmad, F., Wang, Y., Chung, J., and Manganiello, V. C. (2014). Advances in targeting cyclic nucleotide phosphodiesterases. Nat. Rev. Drug Discov. 13 (4), 290–314. doi:10.1038/nrd4228
Mazzucconi, M. G., Baldacci, E., Latagliata, R., Breccia, M., Paoloni, F., Di Veroli, A., et al. (2020). Anagrelide in essential thrombocythemia (ET): Results from 150 patients over 25 years by the "Ph1-negative myeloproliferative neoplasms latium group. Eur. J. Haematol. 105 (3), 335–343. doi:10.1111/ejh.13454
Megens, A. A., Hendrickx, H. M., Hens, K. A., Fonteyn, I., Langlois, X., Lenaerts, I., et al. (2014). Pharmacology of JNJ-42314415, a centrally active phosphodiesterase 10A (PDE10A) inhibitor: A comparison of PDE10A inhibitors with D2 receptor blockers as potential antipsychotic drugs. J. Pharmacol. Exp. Ther. 349 (1), 138–154. doi:10.1124/jpet.113.211904
Menniti, F. S., Chappie, T. A., and Schmidt, C. J. (2021). PDE10A inhibitors-clinical failure or window into antipsychotic drug action? Front. Neurosci. 14, 600178. doi:10.3389/fnins.2020.600178
Mokra, D., Mokry, J., and Matasova, K. (2018). Phosphodiesterase inhibitors: Potential role in the respiratory distress of neonates. Pediatr. Pulmonol. 53 (9), 1318–1325. doi:10.1002/ppul.24082
Mokra, D., and Mokry, J. (2021). Phosphodiesterase inhibitors in acute lung injury: What are the perspectives? Int. J. Mol. Sci. 22 (4), 1929. doi:10.3390/ijms22041929
Mokry, J., Urbanova, A., Kertys, M., and Mokra, D. (2018). Inhibitors of phosphodiesterases in the treatment of cough. Respir. Physiol. Neurobiol. 257, 107–114. doi:10.1016/j.resp.2018.01.008
Molostvov, G., Morris, A., Rose, P., Basu, S., and Muller, G. (2004). The effects of selective cytokine inhibitory drugs (CC-10004 and CC-1088) on VEGF and IL-6 expression and apoptosis in myeloma and endothelial cell co-cultures. Br. J. Haematol. 124 (3), 366–375. doi:10.1046/j.1365-2141.2003.04777.x
Moussa, C., Hebron, M., Huang, X., Ahn, J., Rissman, R. A., Aisen, P. S., et al. (2017). Resveratrol regulates neuro-inflammation and induces adaptive immunity in Alzheimer's disease. J. Neuroinflammation 14 (1), 1. doi:10.1186/s12974-016-0779-0
Murata, T., Shimizu, K., Kurohara, K., Tomeoku, A., Koizumi, G., and Arai, N. (2019). Role of Phosphodiesterase2A in proliferation and migration of human osteosarcoma cells. Anticancer Res. 39 (11), 6057–6062. doi:10.21873/anticanres.13812
Mykoniatis, I., Pyrgidis, N., Sokolakis, I., Ouranidis, A., Sountoulides, P., Haidich, A. B., et al. (2021). Assessment of combination therapies vs monotherapy for erectile dysfunction: A systematic review and meta-analysis. JAMA Netw. Open 4 (2), e2036337. doi:10.1001/jamanetworkopen.2020.36337
Nagayama, T., Hsu, S., Zhang, M., Koitabashi, N., Bedja, D., Gabrielson, K. L., et al. (2009). Sildenafil stops progressive chamber, cellular, and molecular remodeling and improves calcium handling and function in hearts with pre-existing advanced hypertrophy caused by pressure overload. J. Am. Coll. Cardiol. 53 (2), 207–215. doi:10.1016/j.jacc.2008.08.069
Newton, A. C., Bootman, M. D., and Scott, J. D. (2016). Second messengers. Cold Spring Harb. Perspect. Biol. 8 (8), a005926. doi:10.1101/cshperspect.a005926
Nguyen, H. O., Schioppa, T., Tiberio, L., Facchinetti, F., Villetti, G., Civelli, M., et al. (2022). The PDE4 inhibitor tanimilast blunts proinflammatory dendritic cell activation by SARS-CoV-2 ssRNAs. Front. Immunol. 12, 797390. doi:10.3389/fimmu.2021.797390
Nichols, M. R., and Morimoto, B. H. (2000). Differential inhibition of multiple cAMP phosphodiesterase isozymes by isoflavones and tyrphostins. Mol. Pharmacol. 57 (4), 738–745. doi:10.1124/mol.57.4.738
Nio, Y., Tanaka, M., Hirozane, Y., Muraki, Y., Okawara, M., Hazama, M., et al. (2017). Phosphodiesterase 4 inhibitor and phosphodiesterase 5 inhibitor combination therapy has antifibrotic and anti-inflammatory effects in mdx mice with Duchenne muscular dystrophy. FASEB J. 31 (12), 5307–5320. doi:10.1096/fj.201700249R
Nthenge-Ngumbau, D. N., and Mohanakumar, K. P. (2018). Can cyclic nucleotide phosphodiesterase inhibitors Be drugs for Parkinson's disease? Mol. Neurobiol. 55 (1), 822–834. doi:10.1007/s12035-016-0355-8
Ntontsi, P., Detta, A., Bakakos, P., Loukides, S., and Hillas, G. (2019). Experimental and investigational phosphodiesterase inhibitors in development for asthma. Expert Opin. Investig. Drugs 28 (3), 261–266. doi:10.1080/13543784.2019.1571582
O'Brien, J. J., O'Callaghan, J. P., Miller, D. B., Chalgeri, S., Wennogle, L. P., Davis, R. E., et al. (2020). Inhibition of calcium-calmodulin-dependent phosphodiesterase (PDE1) suppresses inflammatory responses. Mol. Cell. Neurosci. 102, 103449. doi:10.1016/j.mcn.2019.103449
Ockaili, R., Salloum, F., Hawkins, J., and Kukreja, R. C. (2002). Sildenafil (Viagra) induces powerful cardioprotective effect via opening of mitochondrial K(ATP) channels in rabbits. Am. J. Physiol. Heart Circ. Physiol. 283 (3), H1263–H1269. doi:10.1152/ajpheart.00324.2002
Page, C. P., and Spina, D. (2011). Phosphodiesterase inhibitors in the treatment of inflammatory diseases. Handb. Exp. Pharmacol. 2011(204), 391–414. doi:10.1007/978-3-642-17969-3_17
Paller, A. S., Tom, W. L., Lebwohl, M. G., Blumenthal, R. L., Boguniewicz, M., Call, R. S., et al. (2016). Efficacy and safety of crisaborole ointment, a novel, nonsteroidal phosphodiesterase 4 (PDE4) inhibitor for the topical treatment of atopic dermatitis (AD) in children and adults. J. Am. Acad. Dermatol. 75 (43), 777494–777503. e6. doi:10.1016/j.jaad.2016.05.046
PaloBioPharma Pipeline (2022). PaloBioPharma pipeline. Available at: https://www.palobiofarma.com/pipeline-2/. [Accessed on August 21, 2022].
Pang, D. C. (1988). Cyclic AMP and cyclic GMP phosphodiesterases: Target for drug development. Drug Dev. Res. 12, 85–92. doi:10.1002/ddr.430120203
Papi, A., Brightling, C., Pedersen, S. E., and Reddel, H. K. (2018). Asthma. Lancet 391 (10122), 783–800. doi:10.1016/S0140-6736(17)33311-1
Paradis, E., and Schliep, K. (2019). Ape 5.0: an environment for modern phylogenetics and evolutionary analyses in R. Bioinformatics 35 (3), 526–528. doi:10.1093/bioinformatics/bty633
Park, S. J., Ahmad, F., Philp, A., Baar, K., Williams, T., Luo, H., et al. (2012). Resveratrol ameliorates aging-related metabolic phenotypes by inhibiting cAMP phosphodiesterases. Cell 148 (3), 421–433. doi:10.1016/j.cell.2012.01.017
Patai, Z., Guttman, A., and Mikus, E. G. (2016). Potential L-type voltage-operated calcium channel blocking effect of drotaverine on functional models. J. Pharmacol. Exp. Ther. 359 (3), 442–451. doi:10.1124/jpet.116.237271
Pekcec, A., Schülert, N., Stierstorfer, B., Deiana, S., Dorner-Ciossek, C., and Rosenbrock, H. (2018). Targeting the dopamine D1 receptor or its downstream signalling by inhibiting phosphodiesterase-1 improves cognitive performance. Br. J. Pharmacol. 175 (14), 3021–3033. doi:10.1111/bph.14350
Peng, T., Gong, J., Jin, Y., Zhou, Y., Tong, R., Wei, X., et al. (2018). Inhibitors of phosphodiesterase as cancer therapeutics. Eur. J. Med. Chem. 150, 742–756. doi:10.1016/j.ejmech.2018.03.046
Peters, M., Bletsch, M., Stanley, J., Wheeler, D., Scott, R., and Tully, T. (2014). The PDE4 inhibitor HT- 0712 improves hippocampus-dependent memory in aged mice. Neuropsychopharmacology 39 (13), 2938–2948. doi:10.1038/npp.2014.154
Rabe, K. F., and Watz, H. (2017). Chronic obstructive pulmonary disease. Lancet 389 (10082), 1931–1940. doi:10.1016/S0140-6736(17)31222-9
Rai, R. R., Dwivedi, M., and Kumar, N. (2014). Efficacy and safety of drotaverine hydrochloride in irritable bowel syndrome: A randomized double-blind placebo-controlled study. Saudi J. Gastroenterol. 20 (6), 378–382. doi:10.4103/1319-3767.145331
Rask-Andersen, M., Almén, M. S., and Schiöth, H. B. (2011). Trends in the exploitation of novel drug targets. Nat. Rev. Drug Discov. 10 (8), 579–590. doi:10.1038/nrd3478
Rask-Andersen, M., Masuram, S., and Schiöth, H. B. (2014). The druggable genome: Evaluation of drug targets in clinical trials suggests major shifts in molecular class and indication. Annu. Rev. Pharmacol. Toxicol. 54, 9–26. doi:10.1146/annurev-pharmtox-011613-135943
Ravipati, G., McClung, J. A., Aronow, W. S., Peterson, S. J., and Frishman, W. H. (2007). Type 5 phosphodiesterase inhibitors in the treatment of erectile dysfunction and cardiovascular disease. Cardiol. Rev. 15 (2), 76–86. doi:10.1097/01.crd.0000233904.77128.49
Redrobe, J. P., Rasmussen, L. K., Christoffersen, C. T., Bundgaard, C., and Jørgensen, M. (2015). Characterisation of Lu AF33241: A novel, brain-penetrant, dual inhibitor of phosphodiesterase (PDE) 2A and PDE10A. Eur. J. Pharmacol. 761, 79–85. doi:10.1016/j.ejphar.2015.04.040
Richter, W., Jin, S. L., and Conti, M. (2005). Splice variants of the cyclic nucleotide phosphodiesterase PDE4D are differentially expressed and regulated in rat tissue. Biochem. J. 388, 803–811. doi:10.1042/BJ20050030
Richter, W., Xie, M., Scheitrum, C., Krall, J., Movsesian, M. A., and Conti, M. (2011). Conserved expression and functions of PDE4 in rodent and human heart. Basic Res. Cardiol. 106 (2), 249–262. doi:10.1007/s00395-010-0138-8
Rouse, M., Younès, A., and Egan, J. M. (2014). Resveratrol and curcumin enhance pancreatic β-cell function by inhibiting phosphodiesterase activity. J. Endocrinol. 223 (2), 107–117. doi:10.1530/JOE-14-0335
Rundfeldt, C., Socała, K., and Wlaź, P. (2010). The atypical anxiolytic drug, tofisopam, selectively blocks phosphodiesterase isoenzymes and is active in the mouse model of negative symptoms of psychosis. J. Neural Transm. 117 (11), 1319–1325. doi:10.1007/s00702-010-0507-3
Saeki, H., Imamura, T., Yokota, D., and Tsubouchi, H. (2022). Difamilast ointment in Japanese adult and pediatric patients with atopic dermatitis: A phase III, long-term, open-label study. Dermatol. Ther. 12 (7), 1589–1601. doi:10.1007/s13555-022-00751-9
Salanova, M., Chun, S. Y., Iona, S., Puri, C., Stefanini, M., and Conti, M. (1999). Type 4 cyclic adenosine monophosphate-specific phosphodiesterases are expressed in discrete subcellular compartments during rat spermiogenesis. Endocrinology 140 (5), 2297–2306. doi:10.1210/endo.140.5.6686
Salehi, B., Mishra, A. P., Nigam, M., Sener, B., Kilic, M., Sharifi-Rad, M., et al. (2018). Resveratrol: A double-edged sword in Health benefits. Biomedicines 6 (3), 91. doi:10.3390/biomedicines6030091
Sasaki, T., Kotera, J., Yuasa, K., and Omori, K. (2000). Identification of human PDE7B, a cAMP-specific phosphodiesterase. Biochem. Biophys. Res. Commun. 271 (3), 575–583. doi:10.1006/bbrc.2000.2661
Schafer, P. H., Truzzi, F., Parton, A., Wu, L., Kosek, J., Zhang, L. H., et al. (2016). Phosphodiesterase 4 in inflammatory diseases: Effects of apremilast in psoriatic blood and in dermal myofibroblasts through the PDE4/CD271 complex. Cell. Signal. 28 (7), 753–763. doi:10.1016/j.cellsig.2016.01.007
Schmidt, B., Roberts, R. S., Davis, P., Doyle, L. W., Barrington, K. J., Ohlsson, A., et al. (2006). Caffeine therapy for apnea of prematurity. N. Engl. J. Med. 354 (20), 2112–2121. doi:10.1056/NEJMoa054065
Schmidt, B., Roberts, R. S., Davis, P., Doyle, L. W., Barrington, K. J., Ohlsson, A., et al. (2007). Long-term effects of caffeine therapy for apnea of prematurity. N. Engl. J. Med. 357 (19), 1893–1902. doi:10.1056/NEJMoa073679
Sei, Y., Inoue, M., Tsuboi, I., Yokoyama, M. M., and Arora, P. K. (1989). Evaluation of the effects of erythro-9(2-hydroxy-3-nonyl) adenine (EHNA) on HIV-1 production in vitro. Biochem. Biophys. Res. Commun. 164 (1), 345–350. doi:10.1016/0006-291x(89)91724-5
Shakur, Y., Holst, L. S., Landstrom, T. R., Movsesian, M., Degerman, E., and Manganiello, V. (2001). Regulation and function of the cyclic nucleotide phosphodiesterase (PDE3) gene family. Prog. Nucleic Acid. Res. Mol. Biol. 66, 241–277. doi:10.1016/s0079-6603(00)66031-2
Singh, K. C., Jain, P., Goel, N., and Saxena, A. (2004). Drotaverine hydrochloride for augmentation of labor. Int. J. Gynaecol. Obstet. 84 (1), 17–22. doi:10.1016/s0020-7292(03)00276-5
Smith, S. J., Brookes-Fazakerley, S., Donnelly, L. E., Barnes, P. J., Barnette, M. S., and Giembycz, M. A. (2003). Ubiquitous expression of phosphodiesterase 7A in human proinflammatory and immune cells. Am. J. Physiol. Lung Cell. Mol. Physiol. 284 (2), L279–L289. doi:10.1152/ajplung.00170.2002
Snyder, G. L., Prickaerts, J., Wadenberg, M. L., Zhang, L., Zheng, H., Yao, W., et al. (2016). Preclinical profile of ITI-214, an inhibitor of phosphodiesterase 1, for enhancement of memory performance in rats. Psychopharmacol. Berl. 233 (17), 3113–3124. doi:10.1007/s00213-016-4346-2
Snyder, G. L., and Vanover, K. E. (2017). PDE inhibitors for the treatment of schizophrenia. Adv. Neurobiol. 17, 385–409. doi:10.1007/978-3-319-58811-7_14
Sokolov, A. V., Dostdar, S. A., Attwood, M. M., Krasilnikova, A. A., Ilina, A. A., Nabieva, A. S., et al. (2021). Brain cancer drug discovery: Clinical trials, drug classes, targets, and combinatorial therapies. Pharmacol. Rev. 73 (4), 1–32. doi:10.1124/pharmrev.121.000317
Spina, D., and Page, C. P. (2017). Xanthines and phosphodiesterase inhibitors. Handb. Exp. Pharmacol. 237, 63–91. doi:10.1007/164_2016_71
Subbian, S., Koo, M. S., Tsenova, L., Khetani, V., Zeldis, J. B., Fallows, D., et al. (2016). Pharmacologic inhibition of host phosphodiesterase-4 improves isoniazid-mediated clearance of Mycobacterium tuberculosis. Front. Immunol. 7, 238. doi:10.3389/fimmu.2016.00238
Subbian, S., Tsenova, L., Holloway, J., Peixoto, B., O'Brien, P., Dartois, V., et al. (2016). Adjunctive phosphodiesterase-4 inhibitor therapy improves antibiotic response to pulmonary tuberculosis in a rabbit model. EBioMedicine 4, 104–114. doi:10.1016/j.ebiom.2016.01.015
Sun, B., Li, H., Shakur, Y., Hensley, J., Hockman, S., Kambayashi, J., et al. (2007). Role of phosphodiesterase type 3A and 3B in regulating platelet and cardiac function using subtype-selective knockout mice. Cell. Signal. 19 (8), 1765–1771. doi:10.1016/j.cellsig.2007.03.012
Sun, L., Quan, H., Xie, C., Wang, L., Hu, Y., and Lou, L. (2014). Phosphodiesterase 3/4 inhibitor zardaverine exhibits potent and selective antitumor activity against hepatocellular carcinoma both in vitro and in vivo independently of phosphodiesterase inhibition. PLoS One 9 (3), e90627. doi:10.1371/journal.pone.0090627
Szegó, J., Somogyi, M., and Papp, E. (1993). Excerpts from the clinical-pharmacologic and clinical studies of Grandaxin. Acta Pharm. hung. 63 (2), 91–98.
Technavio. (2022). Global phosphodiesterase (PDE) inhibitors market 2022-2026. Available at: https://www.giiresearch.com/report/infi1087367-global-phosphodiesterase-pde-inhibitors- market.html. [Accessed on August, 2022].
Tetra Discovery (2016). Tetra discovery partners announces positive results from phase 1 studies of cognition drug candidate. Available at: http://tetradiscovery.com/wp-content/uploads/2016/11/FINAL-Tetra-Phase-1-121616-FINAL.pdf (Accessed on August, 2022).BPN14770
The National Institute of Health. (2022). The national Institute of Health. Available at: https://clinicaltrials.gov/ct2/home. [Accessed on January, 2022].
The US Food and Drug Administration TUS. Drugs@FDA: FDA approved drug products. (2018). Available at: https://www.accessdata.fda.gov/scripts/cder/daf/index.cfm. [Accessed on August, 2022].
Tibbo, A. J., Tejeda, G. S., and Baillie, G. S. (2019). Understanding PDE4's function in Alzheimer's disease; a target for novel therapeutic approaches. Biochem. Soc. Trans. 47 (5), 1557–1565. doi:10.1042/BST20190763
Tobin, D. M. (2015). Host-directed therapies for tuberculosis. Cold Spring Harb. Perspect. Med. 5 (10), a021196. doi:10.1101/cshperspect.a021196
Tollefson, M. M., and Bruckner, A. L. (2014). Atopic dermatitis: Skin-directed management. Pediatrics 134 (6), e1735–e1744. doi:10.1542/peds.2014-2812
Transparency Market Research. (2021). PDE inhibitors market - global industry analysis, size, share, growth, trends, and forecast, 2021–2031. Available at: https://www.transparencymarketresearch.com/pde-inhibitors-market.html. [Accessed on August, 2022].
Tsai, L. C., and Beavo, J. A. (2011). The roles of cyclic nucleotide phosphodiesterases (PDEs) in steroidogenesis. Curr. Opin. Pharmacol. 11 (6), 670–675. doi:10.1016/j.coph.2011.09.003
Turner, R. S., Thomas, R. G., Craft, S., van Dyck, C. H., Mintzer, J., Reynolds, B. A., et al. (2015). A randomized, double-blind, placebo-controlled trial of resveratrol for Alzheimer disease. Neurology 85 (16), 1383–1391. doi:10.1212/WNL.0000000000002035
Tzoumas, N., Farrah, T. E., Dhaun, N., and Webb, D. J. (2020). Established and emerging therapeutic uses of PDE type 5 inhibitors in cardiovascular disease. Br. J. Pharmacol. 177 (24), 5467–5488. doi:10.1111/bph.14920
UniProt Consortium (2021). UniProt: The universal protein knowledgebase in 2021. Nucleic Acids Res. 49 (D1), D480–D489. doi:10.1093/nar/gkaa1100
Vasta, V., Sonnenburg, W. K., Yan, C., Soderling, S. H., Shimizu-Albergine, M., and Beavo, J. A. (2005). Identification of a new variant of PDE1A calmodulin-stimulated cyclic nucleotide phosphodiesterase expressed in mouse sperm. Biol. Reprod. 73 (4), 598–609. doi:10.1095/biolreprod.104.039180
Wallis, R. S., Ginindza, S., Beattie, T., Arjun, N., Likoti, M., Edward, V. A., et al. (2021). Adjunctive host-directed therapies for pulmonary tuberculosis: A prospective, open-label, phase 2, randomised controlled trial. Lancet Respir. Med. 9 (8), e55897–e55908. doi:10.1016/S2213-2600(20)30448-3
Wan, D., Zhou, Y., Wang, K., Hou, Y., Hou, R., and Ye, X. (2016). Resveratrol provides neuroprotection by inhibiting phosphodiesterases and regulating the cAMP/AMPK/SIRT1 pathway after stroke in rats. Brain Res. Bull. 121, 255–262. doi:10.1016/j.brainresbull.2016.02.011
Weight, C. J., Kim, S. P., Karnes, R. J., Bergstralh, E. J., Cheville, J. C., and Leibovich, B. C. (2012). A prospective, controlled phase II study of neoadjuvant exisulind therapy before radical prostatectomy: Effect on apoptosis. Urology 80 (2), 484.e17–e22. doi:10.1016/j.urology.2012.02.027
Weinryb, I., Chasin, M., Free, C. A., Harris, D. N., Goldenberg, H., Michel, I. M., et al. (1972). Effects of therapeutic agents on cyclic AMP metabolism in vitro. J. Pharm. Sci. 61 (10), 1556–1567. doi:10.1002/jps.2600611003
Weishaar, R. E., Cain, M. H., and Bristol, J. A. (1985). A new generation of phosphodiesterase inhibitors: Multiple molecular forms of phosphodiesterase and the potential for drug selectivity. J. Med. Chem. 28 (5), 537–545. doi:10.1021/jm50001a001
Wishart, D. S., Feunang, Y. D., Guo, A. C., Lo, E. J., Marcu, A., Grant, J. R., et al. (2018). DrugBank 5.0: A major update to the DrugBank database for 2018. Nucleic Acids Res. 46, D1074–D1082. doi:10.1093/nar/gkx1037
Wong, R. H., Thaung Zaw, J. J., Xian, C. J., and Howe, P. R. (2020). Regular supplementation with resveratrol improves bone mineral density in postmenopausal women: A randomized, placebo-controlled trial. J. Bone Min. Res. 35 (11), 2121–2131. doi:10.1002/jbmr.4115
World Health Organization. (2022). Cardiovascular diseases (CVDs). Available at: https://www.who.int/news-room/fact-sheets/detail/cardiovascular-diseases-(cvds) [Accessed on August 18, 2022].
Wright, R. K., Mandy, S. H., Halprin, K. M., and Hsia, S. L. (1973). Defects and deficiency of adenyl cyclase in psoriatic skin. Arch. Dermatol. 107 (1), 47–53. doi:10.1001/archderm.107.1.47
Yan, C., Zhao, A. Z., Sonnenburg, W. K., and Beavo, J. A. (2001). Stage and cell-specific expression of calmodulin-dependent phosphodiesterases in mouse testis. Biol. Reprod. 64 (6), 1746–1754. doi:10.1095/biolreprod64.6.1746
Yu, G. (2020). Using ggtree to visualize data on tree-like structures. Curr. Protoc. Bioinforma. 69 (1), e96. doi:10.1002/cpbi.96
Keywords: PDE inhibition, cyclic nucleotides, second messengers, sildenafil, roflumilast, apremilast, ibudilast
Citation: Bondarev AD, Attwood MM, Jonsson J, Chubarev VN, Tarasov VV, Liu W and Schiöth HB (2022) Recent developments of phosphodiesterase inhibitors: Clinical trials, emerging indications and novel molecules. Front. Pharmacol. 13:1057083. doi: 10.3389/fphar.2022.1057083
Received: 29 September 2022; Accepted: 04 November 2022;
Published: 24 November 2022.
Edited by:
Lei Xi, Virginia Commonwealth University, United StatesReviewed by:
Juraj Mokry, Comenius University, SlovakiaHengming Ke, University of North Carolina at Chapel Hill, United States
Copyright © 2022 Bondarev, Attwood, Jonsson, Chubarev, Tarasov, Liu and Schiöth. This is an open-access article distributed under the terms of the Creative Commons Attribution License (CC BY). The use, distribution or reproduction in other forums is permitted, provided the original author(s) and the copyright owner(s) are credited and that the original publication in this journal is cited, in accordance with accepted academic practice. No use, distribution or reproduction is permitted which does not comply with these terms.
*Correspondence: Helgi B. Schiöth, aGVsZ2kuc2NoaW90aEBuZXVyby51dS5zZQ==