- 1Department of Gastrointestinal Surgery, The Second Affiliated Hospital of Dalian Medical University, Dalian, China
- 2Affiliated Foshan Maternity and Child Healthcare Hospital, Southern Medical University, Foshan, China
- 3School of Pharmaceutical Sciences, Southern Medical University, Guangzhou, China
The human gut microbiota is associated with the development and progression of colorectal cancer, and manipulation of the gut microbiota is a novel strategy for the prevention and treatment of colorectal cancer. Some bacteria have antitumor activity against colorectal cancer, where specific bacteria can improve the tumor microenvironment, activate immune cells including dendritic cells, helper T cells, natural killer cells, and cytotoxic T cells, and upregulate the secretion of pro-tumor immune cytokines such as interleukin-2 and interferon. In this paper, we summarize some bacteria with potential benefits in colorectal cancer and describe their roles in the tumor microenvironment, demonstrate the application of gut microbes in combination with immunosuppressive agents, and provide suggestions for further experimental studies and clinical practice applications.
Introduction
The occurrence and development of colorectal cancer (CRC) are closely related to the intestinal flora, with some microorganisms exerting a tumor-promoting effect and others exerting an inhibitory effect. Intestinal microbes colonize near tumors and participate in constituting and influencing the tumor microenvironment (TME). Some beneficial gut microbes can influence host immunity and affect immune cells and cytokines in the TME, thus exerting anti-tumor immune effects. With the application and development of tumor immunotherapy, the role of gut microbiota in tumor immunity has been emerging. We have studied some beneficial microorganisms and outlined their essential roles in tumor immunity.
The relationship between microorganisms and CRC
The human microbiota colonizes the gastrointestinal tract, oropharyngeal cavity, skin, and genitourinary urogenital tract. There are 3.8×1013 bacteria in the human body, more than the number of cells in the human body (Sender, et al., 2016). The gut microbiota is composed of different species of bacteria, archaea, fungi, protozoa, and viruses (El-Sayed, et al., 2021). The colon is a favorable environment for bacterial growth because of its high pH value, low antimicrobial content, and low bile acid concentration (Donaldson, et al., 2016). At the same time, the oxygen content in the colon is low; intestinal bacteria are mainly anaerobic. Most of the bacteria are Bacteroidetes and Firmicutes. There are also a few other bacteria, such as Actinobacteria and Proteobacteria (Kwon, et al., 2021). The gut microbiota is involved in host physiological processes, such as nutrition, metabolism, and immunity. Dysbiosis of the gut microbiota is associated with CRC (Kong and Cai, 2019). In CRC, the microbiome generally has increased bacteria such as Bacteroides, Parabacteroides, Alistipes, Akkermansia spp., Porphyromonadaceae, Corynebacteriaceae, Staphylococcaceae, and Methanobacteriales, whereas other bacteria such as Bifidobacterium, Lactobacillus, Ruminococcus, Faecalibacterium spp., Roseburia, and Treponema are consistently reduced (Cheng, et al., 2020; Huo, et al., 2022).
Microbes in the prevention and treatment of CRC
The intestinal microbiome plays an integral role in the development and progression of CRC, and manipulation of the microbiome such as the use of probiotics can prevent or treat CRC. The International Scientific Association for Probiotics and Prebiotics panel recommends that the term probiotics be used only in products that have the appropriate number of live microorganisms and that clearly define that the strains can provide reasonably expected benefits to the health of the host (Gibson, et al., 2017). Some commensal microorganisms also have anticancer activity, some of which are considered next-generation probiotics. The mechanisms of action of these beneficial bacteria include regulation of the intestinal microbiota, enhancement of the intestinal epithelial barrier, improvement of intestinal physicochemical conditions, production of beneficial metabolites, and suppression of intestinal inflammation (Molska and Reguła, 2019).
Bifidobacterium
Bifidobacterium is a major member of the gut microbiome in early life, and Bifidobacteria belong to the phylum Actinobacteria, mainly including Bifidobacterium infantile, Bifidobacterium longata, and Bifidobacterium breve (Saturio, et al., 2021). Bifidobacterium metabolizes and produces lactate and acetic acid, which decrease the pH in the gut and thus affect the gut microbiota.
In mice with a point mutation in the adenomatous polyposis coli gene, Bifidobacterium colonization reduced polyps by 41% (Liao, et al., 2021). Administration of Bifidobacterium CGMCC 15068 attenuated tumorigenesis in a mouse model of inflammation-associated colitis-associated CRC (CAC) (Wang, et al., 2020a). Bifidobacterium longum administration caused a decrease in the number of aberrant crypt foci lesions in CRC mice (Fahmy, et al., 2019). Bifidobacterium has “protective” anticancer properties comparable with those of cetuximab and trastuzumab and can simultaneously downregulate epidermal growth factor receptor, human epidermal growth factor receptor 2, and prostaglandin-endoperoxide synthase 2, significantly improve the disease activity index, restore colon length, inhibit increased tumor incidence, and prevent tumor progression (Asadollahi, et al., 2020). Bahmani et al. found that cell-free supernatant of B. bifidum can inhibit the growth of colon cancer cells (Bahmani, et al., 2019). Consumption of yogurt containing Bifidobacterium BB536-year and fructo-oligosaccharides prevented CRC in healthy subjects, and short-chain fatty acids were produced because of increased intake of Bifidobacterium BB536-year and fructo-oligosaccharides (Ohara and Suzutani, 2018). The Bifidobacterium CGMCC 15068 pretreatment increased the relative abundance of Akkermansia, Desulfovibrionaceae, Romboutsia, Turicibacter, Verrucomicrobiaceae, Ruminococcaceae_UCG_013, Lachnospiraceae_UCG_004, and Lactobacillus (Wang, et al., 2020b).
Lactobacillus
Lactobacillus is a common probiotic belonging to facultative anaerobes that are widely found in the human gut and have many beneficial properties, including immunomodulatory, anti-inflammatory, antioxidant, and antiproliferative activities. Some Lactobacillus mixtures can inhibit tumor growth (Ghanavati, et al., 2020a; Ghanavati, et al., 2020b). Lactobacillus rhamnosus GG (LGG) colonization early in life promotes intestinal development, increases tight junction formation, reduces low-grade inflammation, and improves intestinal microbiota composition. In addition, LGG colonization regulates the Wingless/Integrated pathway and promotes tumor cell apoptosis, thereby inhibiting tumor formation (Liu, et al., 2022). LGG reduces tumor load in a mouse model of intestinal cancer by initiating an anti-tumor immune response (Owens, et al., 2021). Lactobacillus fermentum YL-11 exopolysaccharide inhibited the growth of HT-29 cells in tumor-bearing mice (Li, et al., 2022). Lactobacillus acidophilus has potential prophylactic effects in a population with a family history of CRC (Zinatizadeh, et al., 2018). Companilactobacillus crustorum MN047 can partially inhibit CAC by regulating the intestinal microbiota, reducing inflammation, and enhancing intestinal barrier integrity (Wang, et al., 2021a). Lactobacillus coryniformis MXJ32 can inhibit CAC by regulating the intestinal microenvironment and alleviating inflammation and intestinal barrier damage (Wang, et al., 2022).
Enterococcus faecalis
The role of Enterococcus faecalis is controversial. On the one hand, the harmful effect of E. faecalis is thought to be mainly related to oxidative stress (Léger, et al., 2019); on the other hand, early colonization of E. faecalis in infants contributes to the development of intestinal immunity.
Metabolites produced by the respiration of E. faecalis have anti-proliferative activity against the colon cancer cell line HT-29 (Jiao, et al., 2022). E. faecalis in the azoxymethane (AOM)/dextran sodium sulfate (DSS) mouse model ameliorates the severity of intestinal inflammation and prevents CAC (Chung, et al., 2019).
Escherichia coli
Escherichia coli Nissle (EcN) was isolated in 1917 by Professor Alfred Nissle of Freiburg, Germany, from a young soldier. The soldier did not have infectious diarrhea when he was stationed in southeast Europe, where Shigella was endemic. The strain was designated as EcN 1917 (Scaldaferri, et al., 2016).
EcN has an important role in apoptosis in colon cancer HT-29 cells through the upregulation of phosphatase and tensin homolog and B-cell lymphoma 2-associated X protein and downregulation of protein kinase B alpha and B-cell lymphoma-extra-large genes (Alizadeh, et al., 2020). Furthermore, EcN 1917 is a transformable bacterial vector with probiotic properties for the production and delivery of anticancer agents in microscopic living therapies, including 5-amino acetyl propionate (Chen, et al., 2021a), butyrate (Chiang and Huang, 2021), and the small microcytotoxic protein (Chiang and Hong, 2021).
Bacteroides fragilis
Bacteroides fragilis is a Gram-negative, obligate anaerobic bacterium in which enterotoxigenic B. fragilis is considered an oncogenic bacterium, whereas non-virulent B. fragilis strains may have probiotic properties.
B. fragilis has the potential to prevent inflammatory diseases in the gut. B. fragilis plays a protective role in a mouse model of Clostridium difficile infection by regulating intestinal microbiota and alleviating barrier disruption, thereby relieving the epithelial stress caused by C. difficile and pathogenic colitis (Deng, et al., 2018). Oral treatment with the B. fragilis ZY-312 strain improves the symptoms of antibiotic-associated diarrhea by increasing the abundance of a specific symbiotic microbiota. These changes were consistent with the restoration of intestinal barrier function and enterocyte regeneration in antibiotic-associated diarrhea rats. In addition, polysaccharide A in B. fragilis ameliorates abnormal voriconazole metabolism by inhibiting toll-like receptor 4-mediated nuclear factor kappa-B (NF-κB) transcription and regulating the expression of drug metabolism enzymes and transporters, which therefore can be used for the clinical adjuvant treatment (Wang, et al., 2021b).
Streptococcus thermophilus
Streptococcus thermophilus is a Gram-positive bacterium that is widely used as a starter in the dairy industry as well as in many traditionally fermented products.
Transoral gavage of S. thermophilus significantly reduced tumor formation in mice with a point mutation in the adenomatous polyposis coli gene and mice injected with AOM. The proliferation of CRC cells was inhibited when cocultured with S. thermophilus or their conditioned medium. β-Galactosidase is a key protein produced by S. thermophilus. It inhibits cell proliferation, reduces colony formation, induces cell cycle arrest, promotes apoptosis of CRC cells, and delays the growth of CRC xenografts while increasing the intestinal abundance of probiotics, including Bifidobacterium and Lactobacillus (Li, et al., 2021a). Two S. thermophilus strains, M17PTZA496 and TH982, have in vitro probiotic properties as well as anticancer activity, simultaneously producing folate and inhibiting in HT-29 cells (Tarrah, et al., 2018).
Clostridium butyricum
The Clostridium butyricum (CB) cell-free supernatant and Bacillus subtilis inhibited the development of dimethylhydrazine-induced CRC in vivo. CB inhibits the progression of CRC, improves inflammation in AOM/DSS mice, changes intestinal microbiota composition, and regulates the expression of MyD88 and NF-κB (Zhou, et al., 2022). CB reduces Firmicutes/Bacteroidetes ratio, increases the relative abundance of probiotics, reduces colitis, reduces CRC incidence and tumor size, increases apoptosis of tumor cells, reduces cytokines including tumor necrosis factor-alpha (TNF-α) and interleukin (IL)-6, reduces cyclooxygenase-2, reduces phosphorylation of NF-κB, reduces B-cell lymphoma two protein, and increases B-cell lymphoma 2-associated X protein expression (Liu, et al., 2020). CB inhibits enterotoxigenic B. fragilis growth in planktonic culture and exhibits anti-biofilm effects by inhibiting biofilm development, breaking down preformed biofilms, and reducing the metabolic activity of cells in biofilms and therefore can serve as a biotherapeutic agent (Shin, et al., 2020).
Faecalibacterium prausnitzii
Faecalibacterium prausnitzii significantly reduced the frequency and formation of abnormal colonic crypt foci in rat AOM-induced CRC. Furthermore, the application of F. prausnitzii reduced the level of lipid peroxidation in colonic tissues. Cell-free supernatant of F. prausnitzii inhibited HCT116 cell growth in a dose-dependent manner. Meanwhile, F. prausnitzii regulated the rat gut microbiota and increased diversity (Dikeocha, et al., 2022).
Microbes and the tumor microenvironment
CRC is a tumor infiltrated by effector memory lymphocytes, and the TME is the key to cancer immunotherapy. The components of TME in CRC include tumor cells, blood vessels, extracellular matrix, fibroblasts, lymphocytes, bone marrow-derived suppressor cells, and signaling molecules (Chen, et al., 2021b). Some bacteria proliferate in the TME and alter it to promote tumor progression (Kasper, et al., 2020); accordingly, some bacteria contribute to improving the TME and thus exert anti-cancer activity. Currently, immunotherapies for CRC such as programmed cell death protein 1 (PD-1) or cytotoxic T lymphocyte-associated antigen-4 (CTLA-4) blockers act mainly through T cells (Makaremi, et al., 2021), while beneficial bacteria act synergistically with immune checkpoint blockade (ICB) by activating immune cells and regulating cytokine secretion.
Immunocytes
Immune cell analysis of spleen and tumor tissues showed that short Bifidobacterium strains alone enhanced antitumor immunity by the increasing cluster of differentiation (CD)8+ T-cell and effector CD8+ T-cell numbers and by increasing CD8+ regulatory T cells (Treg) and effector CD8+/Treg ratios (Table 1) (Yoon, et al., 2021). Some Bifidobacterium spp. cause the reduction of proinflammatory factor IL-6 (Table 1) (Figure 1) and the high accumulation of mature DCs, helper T cells (Th), and cytotoxic T cells (CTLs) at tumor sites under IL-6-deficient conditions (Table 1) (Singh, et al., 2020; Chen, et al., 2021c; Cui, et al., 2022). IL-6 promotes metastatic colonization of CRC cells by modulating the tumor immune microenvironment, and in primary tumors, CRC patients with low IL-6 expression exhibit prolonged disease-free survival (Toyoshima, et al., 2019). In addition, the Bifidobacterium strain Bifidobacterium breve JCM92 regulates the recruitment of immune cells in the TME to increase antitumor immunity, enhancing the antitumor effect of oxaliplatin (Yoon, et al., 2021).
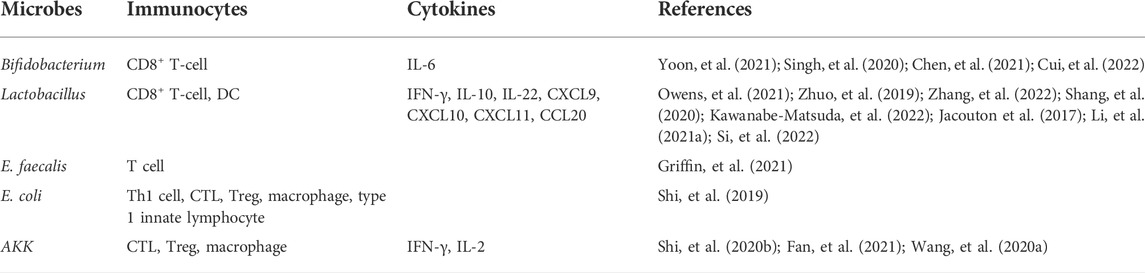
TABLE 1. Influence of intestinal microorganisms on the immune microenvironment of colorectal tumors.
Increased colonic CD8+ T-cell responses were detected in LGG-supplemented mice, induction of CD8+ T cells was dependent on toll-like receptor 2-mediated activation, and LGG reduced tumor burden in a mouse intestinal cancer model in a CD8+ T cell-dependent manner (Table 1) (Owens, et al., 2021). Meanwhile, L. acidophilus lysates had immunomodulatory effects by inhibiting the IL-10 expression levels in M2 polarization and Lipopolysaccharide-activated Raw264.7 macrophages (Table 1) (Zhuo, et al., 2019). L. paracasei sh2020–triggered antitumor immune response is dependent on CD8+ T cells. In vitro and in vivo studies have shown that the L. paracasei sh2020 enhanced CD8+ T-cell recruitment, with increased T-cell infiltration, and improved the poorly infiltrated TME, thus promoting immunotherapy (Table 1) (Zhang, et al., 2022). A probiotic mixture containing B. longum, B. bifidum, L. acidophilus, L. plantarum, and other components inhibits the invasion, migration, and proliferation of CT26 cells and may exert anti-tumor effects by inducing CD8+ T cell immune responses (Table 1) (Shang, et al., 2020).
E. faecalis CECT7121 can stimulate local mucosal immunity and adhere to intestinal epithelial cells, which can stimulate the mucosal immune system and increase the number of IgA + cells in the lamina propria, without inducing an epithelial inflammatory response (Castro et al., 2016). Immunoreactive muropeptides are prevalent throughout human-associated enterococcal species. Enterococci with unique NlpC/p60 peptidoglycan hydrolase activity produce nucleotide-binding oligomerization domain two active muropeptides and modulate the effects of ICB immunotherapy in vivo, and tumors treated with active muropeptide-L, D isoforms show a significant increase in the proportion of intra-tumor T cells (Table 1) (Griffin, et al., 2021). Pretreatment with E. faecalis significantly attenuated nucleotide-binding oligomerization domain-like receptor protein 3 (NLRP3) inflammasome activation in macrophages induced by intestinal commensal microorganisms and attenuated colitis and CAC (Chung, et al., 2019).
Oral administration of EcN alone inhibited tumor growth, which may be due to increased tumor-specific effector T-cell infiltration and improved tumor immunosuppression. Combination treatment of transforming growth factor-β blocker with EcN appeared to restore tumor-infiltrating CTL-disrupted cancer cells and increased the proportion of tumor-infiltrating CD3+CD8+IFN-γ+CTL (Table 1) (Shi, et al., 2019). EcN 541–15 implantation affected the TME, with a decrease in myeloid infiltration, including tumor-associated macrophages, mononuclear myeloid-derived suppressor cells, and polymorphonuclear myeloid-derived suppressor cells, and a decrease in Tregs in tumors of EcN 541–15 implanted mice. Changes in lymphocyte recruitment to tumors were found in 541–15 colonized mice, with an increase in Th1 cells, CTLs, and type 1 innate lymphocytes (Table 1) (Zegarra Ruiz, et al., 2022). Furthermore, B. fragilis can alter the Treg/Th-17 balance by counteracting the lipopolysaccharide-induced inflammatory responses, thereby exerting immunomodulatory effects (Chang, et al., 2017).
Oral Akkermansia muciniphila (AKK) triggers antitumor immune responses, induces tumor shrinkage, and prolongs median survival in tumor-bearing mice, and While AKK treatment is successful in lowering the proportion of Treg cells in the TME, AKK alone attracts a higher proportion of CTL than the preadministration of IL-2 combined with AKK. In addition to significantly lowering the proportion of CD133+ cells in tumor tissue and diminishing tumor stem cell-like potency, the combination of IL-2 and AKK is more effective than monotherapy at controlling Treg levels (Table 1) (Shi, et al., 2020a). Supplementation with AKK inhibits colon tumorigenesis in mice with a point mutation in the adenomatous polyposis coli gene and the growth of implanted HCT116 or CT26 tumors in nude mice. AKK promotes the enrichment of M1-like macrophages in vivo and in vitro in an NLRP3-dependent manner (Table 1) (Fan, et al., 2021). A protein extracted from AKK named Amuc_1100 significantly increased the percentage of CTL in mesenteric lymph nodes and the colon, thereby exacerbating apoptosis in tumor cells (Table 1) (Wang, et al., 2020a).
Cytokines
Lactobacillus paracasei sh2020 promotes the expression of T cell chemokines such as chemokine (C-X-C motif) ligand (CXCL)9, CXCL10, and CXCL11. In an in vitro tumor cell culture model, stimulation of L. paracasei sh2020 may lead to increased production of CXCL10, a T helper type 1 chemokine that controls the entry of major anti-tumor immune cells into tumor bed (Table 1) (Figure 1) (Zhang, et al., 2022). Lactobacillus-derived exopolysaccharide-R1-induced T cells can infiltrate CCL20-producing tumors and produce IFN-γ, enhancing the effect of ICB therapy in producing CCL20 tumors in mice (Table 1) (Figure 1) (Kawanabe-Matsuda, et al., 2022). L. casei BL23 mediated immunomodulatory effects through the downregulation of IL-22 cytokines (Table 1) (Figure 1) (Jacouton et al., 2017). In the in situ ligated intestine loop model, stimulation of B. breve triggered upregulation of DC-associated chemokine CCL20 expression and increased DCs recruitment in the intestinal villi, enhancing DC-derived IL-12 secretion on the antitumor effect of B. breve (Figure 1) (Li, et al., 2021b).
In the AOM/DSS-induced CAC mouse model, B. fragilis acted in a polysaccharide A-dependent manner, with a significant reduction in the number and size of tumors in the colon of the B. fragilis-treated mice, and reduced expression of chemokine receptor (CCR) five was observed in the colon tissue of B. fragilis-treated mice (Lee, et al., 2018).
Combined administration of AKK and IL-2 has a better tumor suppressive effect than single administration, altering the TME, except affecting immune cells, and inducing the production of pro-inflammatory cytokines, significantly increasing IFN-γ and IL-2 levels in tumor tissues (Table 1) (Figure 1) (Shi, et al., 2020b).
Combination of gut microbes and immune checkpoint inhibitors
Bifidobacteria promote local anti-CD47 to tumor immunotherapy by accumulating in the TME, which effectively stimulates the stimulator of interferon genes signaling and increases the cross-initiation of dendritic cells (DCs) after anti-CD47 treatment. Type I interferon (IFN) signaling in DCs is critical for the therapeutic outcome of Bifidobacterium-promoted CD47 blockade. After CD47 blockade, type I IFN can be upregulated in bone marrow-derived DCs co-cultured with tumor cells and Bifidobacterium (Figure 1) (Shi, et al., 2020a). Intratumor cytokine expression showed that mice treated with PD-1 blocker and B. breve JCM92 had higher IFN-γ and IL-2 expression compared to mice treated with PD-1 blocker alone (Figure 1) (Yoon, et al., 2021).
In addition, antigenic mimicry of gut microbes affects T-cell immunity and contributes to cross-reactive antitumor responses. T cells targeting an epitope called SVYRYYGL expressed in the commensal bacterium B. breve cross-react with the model neoantigen SIYRYYGL. In a mouse melanoma model, SVYRYYGL-reactive T cells were reduced in mice lacking B. breve, and SVYRYYGL -specific T cells recognized in vivo melanomas expressing SIYRYYGL (Bessell, et al., 2020). Bifidobacterium pseudolongum isolated from ICB-treated CRC tumors promotes conventional DCs-dependent Th1 cell circuits, thereby greatly enhancing the effects of ICB therapy in mouse models of intestinal and epithelial tumors, and modulates the enhanced immunotherapeutic response through the production of the metabolite inosine (Mager, et al., 2020).
Oral live LGG enhances the antitumor activity of anti-PD-1 immunotherapy by increasing tumor-infiltrating DCs and T cells. In addition, LGG combined with PD-1 treatment shifted the gut microbial community towards Lactobacillus murinus and Bacteroides uniformis enrichment, increasing DCs activation and CD8+ tumor recruitment (Table 1) (Si, et al., 2022). Dietary intake of Lactobacillus-derived exopolysaccharide induces CCR6+CD8+ T cells in the Peyer’s patches, enhancing the antitumor effect of anti-CTLA-4 or anti-PD-1 monoclonal antibodies on CCL20-expressing tumors (Kawanabe-Matsuda, et al., 2022). L. acidophilus lysates enhanced the antitumor activity of CTLA-4 monoclonal antibodies in a mouse model. In the TME, CD8+ T cells were increased, effector memory T cells (CD44+CD8+CD62L+) were increased, and Treg (CD4+CD25+FoxP3+) and M2 macrophages (F4/80+CD206+) were decreased (Table 1) (Zhuo, et al., 2019). In mice and patients, the T-cell response of Bacteroides thetaiotaomicron or B. fragilis was correlated with the efficacy of CTLA-4 blockade. Tumors from antibiotic-treated mice or germ-free mice did not respond to CTLA-4 blockade. This absence of response can be improved by the use of B. fragilis for gavage, polysaccharide application, or adoptive transfer of B. fragilis. Fecal microbial transplantation from humans to mice confirmed that the treatment of melanoma patients with antibodies directed against CTLA-4 favored the growth of B. fragilis with anticancer properties (Vétizou, et al., 2015).
Conclusion
The intestinal microbiota is closely associated with the development and progression of CRC, and the prevention and treatment of CRC can be facilitated by the intake of specific intestinal bacteria, including probiotics. These bacteria promote apoptosis, inhibit tumor cell proliferation, and play an important role in the TME, either by themselves or by producing beneficial metabolites. In addition to tumor cells, the TME also includes immune cells, and intestinal microorganisms are also members of the microenvironment. The TME is closely related to the development and progression of tumors.
We review some beneficial bacteria that act on DCs, NK cells, cytotoxic T cells, and helper T cells to promote the secretion of the pro-tumor cytokines IFN-1, IFN-γ, and IL-2 and down-regulate the secretion of TNF-α, IL-6, IL-10, and IL-22, thus exerting anti-tumor immune effects. Targeting and manipulating the cells and factors in the TME can actively treat tumors. Focusing on the active role of the gut microbiota in the TME and combining the gut microbiota with immunotherapy is conducive to improving current immunosuppressive therapies, demonstrating their promise, and facilitating the progress of tumor immunotherapy with the use of the gut microbiota in clinical practice. Meanwhile, microbiota associated with the TME is bound to be further developed, however, the positive effects of these gut microbes on the TME and the therapeutic effects of beneficial combinations of gut microbes and immunosuppressive agents on tumors need to be demonstrated in more animal and clinical experiments.
Author contributions
All authors contributed to the study’s conception and design. CX contributed to the study’s conception, design, review, and edit the manuscript. CX and SR reviewed the manuscript. CX performed the literature search. The first draft of the manuscript was written by CX. CX, YC, and CX drew Figures. All authors commented on previous versions of the manuscript. All authors read and approved the final manuscript.
Funding
This work was supported by grants from the Science and Technology Bureau of Foshan (No. FS0AA-KJ218-1301-0008 and no. FS0AA-KJ819-4,901-0082).
Conflict of interest
The authors declare that the research was conducted in the absence of any commercial or financial relationships that could be construed as a potential conflict of interest.
Publisher’s note
All claims expressed in this article are solely those of the authors and do not necessarily represent those of their affiliated organizations, or those of the publisher, the editors and the reviewers. Any product that may be evaluated in this article, or claim that may be made by its manufacturer, is not guaranteed or endorsed by the publisher.
Abbreviations
AKK, Akkermansia muciniphila; AOM, Azoxymethane; CAC, Colitis-associated CRC; CB, Clostridium butyricum; CCR, Chemokine receptor; CD, Cluster of differentiation of differentiation; CRC, Colorectal cancer; CTLA-4, Cytotoxic T lymphocyte-associated antigen 4; CXCL, Chemokine (C-X-C motif) ligand; DC, Dendritic cell; DSS, Dextran sodium sulfate; EcN, Escherichia coli Nissle; IFN, Interferon; IL, Interleukin; LGG, Lactobacillus rhamnosus GG;NF-κB, Nuclear factor kappa-B; NLRP3, Nucleotide-binding oligomerization domain-like receptor protein 3; PD-1, Programmed cell death protein 1; Th, Helper T cell; TME, Tumor microenvironment; TNF-α, Tumor necrosis factor-alpha; Treg, Regulatory T cell.
References
Alizadeh, S., Esmaeili, A., and Omidi, Y. (2020). Anti-cancer properties of Escherichia coli Nissle 1917 against HT-29 colon cancer cells through regulation of Bax/Bcl-xL and AKT/PTEN signaling pathways. Iran. J. Basic Med. Sci. 23, 886–893. doi:10.22038/ijbms.2020.43016.10115
Asadollahi, P., Ghanavati, R., Rohani, M., Razavi, S., Esghaei, M., and Talebi, M. (2020). Anti-cancer effects of Bifidobacterium species in colon cancer cells and a mouse model of carcinogenesis. PLoS ONE 15, e0232930. doi:10.1371/journal.pone.0232930
Bahmani, S., Azarpira, N., and Moazamian, E. (2019). Anti-colon cancer activity of Bifidobacterium metabolites on colon cancer cell line SW742. Turk. J. Gastroenterol. 30, 835–842. doi:10.5152/tjg.2019.18451
Bessell, C. A., Isser, A., Havel, J. J., Lee, S., Bell, D. R., Hickey, J. W., et al. (2020). Commensal bacteria stimulate antitumor responses via T cell cross-reactivity. JCI Insight 5, 135597. doi:10.1172/jci.insight.135597
Chang, Y. C., Ching, Y. H., Chiu, C. C., Liu, J. Y., Hung, S. W., Huang, W. C., et al. (2017). TLR2 and interleukin-10 are involved in Bacteroides fragilis-mediated prevention of DSS-induced colitis in gnotobiotic mice. PLoS ONE 12, e0180025. doi:10.1371/journal.pone.0180025
Chen, J., Li, X., Liu, Y., Su, T., Lin, C., Shao, L., et al. (2021a). Engineering a probiotic strain of Escherichia coli to induce the regression of colorectal cancer through production of 5-aminolevulinic acid. Microb. Biotechnol. 14, 2130–2139. doi:10.1111/1751-7915.13894
Chen, Y., Chen, H., Ding, J., Stanton, C., Ross, R. P., Zhao, J., et al. (2021b). Bifidobacterium longum ameliorates dextran sulfate sodium-induced colitis by producing conjugated linoleic acid, protecting intestinal mechanical barrier, restoring unbalanced gut microbiota, and regulating the toll-like receptor-4/nuclear factor-κb signaling pathway. J. Agric. Food Chem. 69, 14593–14608. doi:10.1021/acs.jafc.1c06176
Chen, Y., Zheng, X., and Wu, C. (2021c). The role of the tumor microenvironment and treatment strategies in colorectal cancer. Front. Immunol. 12, 792691. doi:10.3389/fimmu.2021.792691
Cheng, Y., Ling, Z., and Li, L. (2020). The intestinal microbiota and colorectal cancer. Front. Immunol. 11, 615056. doi:10.3389/fimmu.2020.615056
Chiang, C. J., and Hong, Y. H. (2021). In situ delivery of biobutyrate by probiotic Escherichia coli for cancer therapy. Sci. Rep. 11, 18172. doi:10.1038/s41598-021-97457-3
Chiang, C. J., and Huang, P. H. (2021). Metabolic engineering of probiotic Escherichia coli for cytolytic therapy of tumors. Sci. Rep. 11, 5853. doi:10.1038/s41598-021-85372-6
Chung, I. C., OuYang, C. N., Yuan, S. N., Lin, H. C., Huang, K. Y., Wu, P. S., et al. (2019). Pretreatment with a heat-killed probiotic modulates the NLRP3 inflammasome and attenuates colitis-associated colorectal cancer in mice. Nutrients 11, E516. doi:10.3390/nu11030516
Cui, Q. Y., Tian, X. Y., Liang, X., Zhang, Z., Wang, R., Zhou, Y., et al. (2022). Bifidobacterium bifidum relieved DSS-induced colitis in mice potentially by activating the aryl hydrocarbon receptor. Food Funct. 13, 5115–5123. doi:10.1039/d1fo04219j
Deng, H., Yang, S., Zhang, Y., Qian, K., Zhang, Z., Liu, Y., et al. (2018). Bacteroides fragilis prevents Clostridium difficile infection in a mouse model by restoring gut barrier and microbiome regulation. Front. Microbiol. 9, 2976. doi:10.3389/fmicb.2018.02976
Dikeocha, I. J., Al-Kabsi, A. M., Chiu, H. T., and Alshawsh, M. A. (2022). Faecalibacterium prausnitzii ameliorates colorectal tumorigenesis and suppresses proliferation of HCT116 colorectal cancer cells. Biomedicines 10, 1128. doi:10.3390/biomedicines10051128
Donaldson, G. P., Lee, S. M., and Mazmanian, S. K. (2016). Gut biogeography of the bacterial microbiota. Nat. Rev. Microbiol. 14, 20–32. doi:10.1038/nrmicro3552
El-Sayed, A., Aleya, L., and Kamel, M. (2021). The link among microbiota, epigenetics, and disease development. Environ. Sci. Pollut. Res. Int. 28, 28926–28964. doi:10.1007/s11356-021-13862-1
Fahmy, C. A., Gamal-Eldeen, A. M., El-Hussieny, E. A., Raafat, B. M., Mehanna, N. S., Talaat, R. M., et al. (2019). Bifidobacterium longum suppresses murine colorectal cancer through the modulation of oncomiRs and tumor suppressor miRNAs. Nutr. Cancer 71, 688–700. doi:10.1080/01635581.2019.1577984
Fan, L., Xu, C., Ge, Q., Lin, Y., Wong, C. C., Qi, Y., et al. (2021). A. Muciniphila suppresses colorectal tumorigenesis by inducing TLR2/NLRP3-mediated M1-like TAMs. Cancer Immunol. Res. 9, 1111–1124. doi:10.1158/2326-6066.CIR-20-1019
Ghanavati, R., Akbari, A., Mohammadi, F., Asadollahi, P., Javadi, A., Talebi, M., et al. (2020a). Lactobacillus species inhibitory effect on colorectal cancer progression through modulating the Wnt/β-catenin signaling pathway. Mol. Cell. Biochem. 470, 1–13. doi:10.1007/s11010-020-03740-8
Ghanavati, R., Asadollahi, P., Shapourabadi, M. B., Razavi, S., Talebi, M., and Rohani, M. (2020b). Inhibitory effects of Lactobacilli cocktail on HT-29 colon carcinoma cells growth and modulation of the Notch and Wnt/β-catenin signaling pathways. Microb. Pathog. 139, 103829. doi:10.1016/j.micpath.2019.103829
Gibson, G. R., Hutkins, R., Sanders, M. E., Prescott, S. L., Reimer, R. A., Salminen, S. J., et al. (2017). Expert consensus document: The International Scientific Association for Probiotics and Prebiotics (ISAPP) consensus statement on the definition and scope of prebiotics. Nat. Rev. Gastroenterol. Hepatol. 14, 491–502. doi:10.1038/nrgastro.2017.75
Griffin, M. E., Espinosa, J., Becker, J. L., Luo, J. D., Carroll, T. S., Jha, J. K., et al. (2021). Enterococcus peptidoglycan remodeling promotes checkpoint inhibitor cancer immunotherapy. Science 373, 1040–1046. doi:10.1126/science.abc9113
Huo, R. X., Wang, Y. J., Hou, S. B., Wang, W., Zhang, C. Z., and Wan, X. H. (2022). Gut mucosal microbiota profiles linked to colorectal cancer recurrence. World J. Gastroenterol. 28, 1946–1964. doi:10.3748/wjg.v28.i18.1946
Jiao, Y., Yang, H., Shigwedha, N., Zhang, S., Liu, F., and Zhang, L. (2022). Probiotic effects and metabolic products of Enterococcus faecalis LD33 with respiration capacity. Foods 11, 606. doi:10.3390/foods11040606
Kasper, S. H., Morell-Perez, C., Wyche, T. P., Sana, T. R., Lieberman, L. A., and Hett, E. C. (2020). Colorectal cancer-associated anaerobic bacteria proliferate in tumor spheroids and alter the microenvironment. Sci. Rep. 10, 5321. doi:10.1038/s41598-020-62139-z
Kawanabe-Matsuda, H., Takeda, K., Nakamura, M., Makino, S., Karasaki, T., Kakimi, K., et al. (2022). Dietary lactobacillus-derived exopolysaccharide enhances immune-checkpoint blockade therapy. Cancer Discov. 12, 1336–1355. doi:10.1158/2159-8290.CD-21-0929
Kong, F., and Cai, Y. (2019). Study insights into gastrointestinal cancer through the gut microbiota. Biomed. Res. Int. 2019, 8721503. doi:10.1155/2019/8721503
Kwon, Y. J., Kwak, H. J., Lee, H. K., Lim, H. C., and Jung, D. H. (2021). Comparison of bacterial community profiles from large intestine specimens, rectal swabs, and stool samples. Appl. Microbiol. Biotechnol. 105, 9273–9284. doi:10.1007/s00253-021-11650-y
Lee, Y. K., Mehrabian, P., Boyajian, S., Wu, W. L., Selicha, J., Vonderfecht, S., et al. (2018). The protective role of Bacteroides fragilis in a murine model of colitis-associated colorectal cancer. mSphere 3 (6), e00587–18. doi:10.1128/mSphere.00587-18
Léger, L., Budin-Verneuil, A., Cacaci, M., Benachour, A., Hartke, A., and Verneuil, N. (2019). β-Lactam exposure triggers reactive oxygen species formation in Enterococcus faecalis via the respiratory chain component DMK. Cell Rep. 29, 2184–2191. doi:10.1016/j.celrep.2019.10.080
Li, F., Jiao, X., Zhao, J., Liao, X., Wei, Y., and Li, Q. (2022). Antitumor mechanisms of an exopolysaccharide from Lactobacillus fermentum on HT-29 cells and HT-29 tumor-bearing mice. Int. J. Biol. Macromol. 209, 552–562. doi:10.1016/j.ijbiomac.2022.04.023
Li, Q., Hu, W., Liu, W. X., Zhao, L. Y., Huang, D., Liu, X. D., et al. (2021a). Streptococcus thermophilus inhibits colorectal tumorigenesis through secreting β-galactosidase. Gastroenterology 160, 1179–1193.e14. doi:10.1053/j.gastro.2020.09.003
Li, Q., Li, Y., Wang, Y., Xu, L., Guo, Y., Wang, Y., et al. (2021b). Oral administration of Bifidobacterium breve promotes antitumor efficacy via dendritic cells-derived interleukin 12. Oncoimmunology 10, 1868122. doi:10.1080/2162402X.2020.1868122
Liao, W., Khan, I., Huang, G., Chen, S., Liu, L., Leong, W. K., et al. (2021). Bifidobacterium animalis: The missing link for the cancer-preventive effect of gynostemma pentaphyllum. Gut Microbes 13 (1), 1847629. doi:10.1080/19490976.2020.1847629
Liu, M., Xie, W., Wan, X., and Deng, T. (2020). Clostridium butyricum modulates gut microbiota and reduces colitis associated colon cancer in mice. Int. Immunopharmacol. 88, 106862. doi:10.1016/j.intimp.2020.106862
Liu, X., Jin, G., Tang, Q., Huang, S., Zhang, Y., Sun, Y., et al. (2022). Early life Lactobacillus rhamnosus GG colonisation inhibits intestinal tumour formation. Br. J. Cancer 126, 1421–1431. doi:10.1038/s41416-021-01562-z
Mager, L. F., Burkhard, R., Pett, N., Cooke, N., Brown, K., Ramay, H., et al. (2020). Microbiome-derived inosine modulates response to checkpoint inhibitor immunotherapy. Science 369, 1481–1489. doi:10.1126/science.abc3421
Makaremi, S., Asadzadeh, Z., Hemmat, N., Baghbanzadeh, A., Sgambato, A., Ghorbaninezhad, F., et al. (2021). Immune checkpoint inhibitors in colorectal cancer: Challenges and future prospects. Biomedicines 9, 1075. doi:10.3390/biomedicines9091075
Molska, M., and Reguła, J. (2019). Potential mechanisms of probiotics action in the prevention and treatment of colorectal cancer. Nutrients 11, E2453. doi:10.3390/nu11102453
Ohara, T., and Suzutani, T. (2018). Intake of Bifidobacterium longum and fructo-oligosaccharides prevents colorectal carcinogenesis. Euroasian J. Hepatogastroenterol. 8, 11–17. doi:10.5005/jp-journals-10018-1251
Owens, J. A., Saeedi, B. J., Naudin, C. R., Hunter-Chang, S., Barbian, M. E., Eboka, R. U., et al. (2021). Lactobacillus rhamnosus GG orchestrates an antitumor immune response. Cell. Mol. Gastroenterol. Hepatol. 12, 1311–1327. doi:10.1016/j.jcmgh.2021.06.001
Saturio, S., Nogacka, A. M., Suárez, M., Fernández, N., Mantecón, L., Mancabelli, L., et al. (2021). Early-life development of the bifidobacterial community in the infant gut. Int. J. Mol. Sci. 22, 3382. doi:10.3390/ijms22073382
Scaldaferri, F., Gerardi, V., Mangiola, F., Lopetuso, L. R., Pizzoferrato, M., Petito, V., et al. (2016). Role and mechanisms of action of Escherichia coli Nissle 1917 in the maintenance of remission in ulcerative colitis patients: An update. World J. Gastroenterol. 22, 5505–5511. doi:10.3748/wjg.v22.i24.5505
Sender, R., Fuchs, S., and Milo, R. (2016). Revised estimates for the number of human and bacteria cells in the body. PLoS Biol. 14, e1002533. doi:10.1371/journal.pbio.1002533
Shang, F., Jiang, X., Wang, H., Chen, S., Wang, X., Liu, Y., et al. (2020). The inhibitory effects of probiotics on colon cancer cells: In vitro and in vivo studies. J. Gastrointest. Oncol. 11, 1224–1232. doi:10.21037/jgo-20-573
Shi, L., Sheng, J., Chen, G., Zhu, P., Shi, C., Li, B., et al. (2020a). Combining IL-2-based immunotherapy with commensal probiotics produces enhanced antitumor immune response and tumor clearance. J. Immunother. Cancer 8, e000973. doi:10.1136/jitc-2020-000973
Shi, L., Sheng, J., Wang, M., Luo, H., Zhu, J., Zhang, B., et al. (2019). Combination therapy of TGF-β blockade and commensal-derived probiotics provides enhanced antitumor immune response and tumor suppression. Theranostics 9, 4115–4129. doi:10.7150/thno.35131
Shi, Y., Zheng, W., Yang, K., Harris, K. G., Ni, K., Xue, L., et al. (2020b). Intratumoral accumulation of gut microbiota facilitates CD47-based immunotherapy via STING signaling. J. Exp. Med. 217, e20192282. doi:10.1084/jem.20192282
Shin, D. S., Rhee, K. J., and Eom, Y. B. (2020). Effect of probiotic Clostridium butyricum NCTC 7423 supernatant on biofilm formation and gene expression of Bacteroides fragilis. J. Microbiol. Biotechnol. 30, 368–377. doi:10.4014/jmb.2001.01027
Si, W., Liang, H., Bugno, J., Xu, Q., Ding, X., Yang, K., et al. (2022). Lactobacillus rhamnosus GG induces cGAS/STING- dependent type I interferon and improves response to immune checkpoint blockade. Gut 71, 521–533. doi:10.1136/gutjnl-2020-323426
Singh, S., Bhatia, R., Khare, P., Sharma, S., Rajarammohan, S., Bishnoi, M., et al. (2020). Anti-inflammatory Bifidobacterium strains prevent dextran sodium sulfate induced colitis and associated gut microbial dysbiosis in mice. Sci. Rep. 10, 18597. doi:10.1038/s41598-020-75702-5
Tarrah, A., de Castilhos, J., Rossi, R. C., Duarte, V., Ziegler, D. R., Corich, V., et al. (2018). In vitro probiotic potential and anti-cancer activity of newly isolated folate-producing Streptococcus thermophilus strains. Front. Microbiol. 9, 2214. doi:10.3389/fmicb.2018.02214
Toyoshima, Y., Kitamura, H., Xiang, H., Ohno, Y., Homma, S., Kawamura, H., et al. (2019). IL6 modulates the immune status of the tumor microenvironment to facilitate metastatic colonization of colorectal cancer cells. Cancer Immunol. Res. 7, 1944–1957. doi:10.1158/2326-6066.CIR-18-0766
Vétizou, M., Pitt, J. M., Daillère, R., Lepage, P., Waldschmitt, N., Flament, C., et al. (2015). Anticancer immunotherapy by CTLA-4 blockade relies on the gut microbiota. Science 350, 1079–1084. doi:10.1126/science.aad1329
Wang, L., Tang, L., Feng, Y., Zhao, S., Han, M., Zhang, C., et al. (2020a). A purified membrane protein from Akkermansia muciniphila or the pasteurised bacterium blunts colitis associated tumourigenesis by modulation of CD8(+) T cells in mice. Gut 69, 1988–1997. doi:10.1136/gutjnl-2019-320105
Wang, Q., Wang, K., Wu, W., Lv, L., Bian, X., Yang, L., et al. (2020b). Administration of Bifidobacterium bifidum CGMCC 15068 modulates gut microbiota and metabolome in azoxymethane (AOM)/dextran sulphate sodium (DSS)-induced colitis-associated colon cancer (CAC) in mice. Appl. Microbiol. Biotechnol. 104, 5915–5928. doi:10.1007/s00253-020-10621-z
Wang, T., Wang, P., Ge, W., Shi, C., Xiao, G., Wang, X., et al. (2021a). The probiotic Companilactobacillus crustorum MN047 alleviates colitis-associated tumorigenesis via modulating the intestinal microenvironment. Food Funct. 12, 11331–11342. doi:10.1039/d1fo01531a
Wang, T., Zhang, L., Wang, P., Liu, Y., Wang, G., Shan, Y., et al. (2022). Lactobacillus coryniformis MXJ32 administration ameliorates azoxymethane/dextran sulfate sodium-induced colitis-associated colorectal cancer via reshaping intestinal microenvironment and alleviating inflammatory response. Eur. J. Nutr. 61, 85–99. doi:10.1007/s00394-021-02627-8
Wang, X., Ye, C., Xun, T., Mo, L., Tong, Y., Ni, W., et al. (2021b). Bacteroides fragilis polysaccharide A ameliorates abnormal voriconazole metabolism accompanied with the inhibition of TLR4/NF-κB pathway. Front. Pharmacol. 12, 663325. doi:10.3389/fphar.2021.663325
Yoon, Y., Kim, G., Jeon, B. N., Fang, S., and Park, H. (2021). Bifidobacterium strain-specific enhances the efficacy of cancer therapeutics in tumor-bearing mice. Cancers (Basel) 13, 957. doi:10.3390/cancers13050957
Zhang, S. L., Han, B., Mao, Y. Q., Zhang, Z. Y., Li, Z. M., Kong, C. Y., et al. (2022). Lacticaseibacillus paracasei sh2020 induced antitumor immunity and synergized with anti-programmed cell death 1 to reduce tumor burden in mice. Gut Microbes 14, 2046246. doi:10.1080/19490976.2022.2046246
Zhou, M., Yuan, W., Yang, B., Pei, W., Ma, J., and Feng, Q. (2022). Clostridium butyricum inhibits the progression of colorectal cancer and alleviates intestinal inflammation via the myeloid differentiation factor 88 (MyD88)-nuclear factor-kappa B (NF-κB) signaling pathway. Ann. Transl. Med. 10, 478. doi:10.21037/atm-22-1670
Zhuo, Q., Yu, B., Zhou, J., Zhang, J., Zhang, R., Xie, J., et al. (2019). Lysates of Lactobacillus acidophilus combined with CTLA-4-blocking antibodies enhance antitumor immunity in a mouse colon cancer model. Sci. Rep. 9, 20128. doi:10.1038/s41598-019-56661-y
Keywords: tumor microenvironment, immunocyte, microbioma, cytokin, colorectal cancer
Citation: Xia C, Cai Y, Ren S and Xia C (2022) Role of microbes in colorectal cancer therapy: Cross-talk between the microbiome and tumor microenvironment. Front. Pharmacol. 13:1051330. doi: 10.3389/fphar.2022.1051330
Received: 22 September 2022; Accepted: 24 October 2022;
Published: 08 November 2022.
Edited by:
Dake Cai, Guangdong Second Hospital of Traditional Chinese Medicine, ChinaReviewed by:
Fei Yu, Hebei Agricultural University, ChinaChangliang Shan, Nankai University, China
Li Qin, Hunan University of Chinese Medicine, China
Copyright © 2022 Xia, Cai, Ren and Xia. This is an open-access article distributed under the terms of the Creative Commons Attribution License (CC BY). The use, distribution or reproduction in other forums is permitted, provided the original author(s) and the copyright owner(s) are credited and that the original publication in this journal is cited, in accordance with accepted academic practice. No use, distribution or reproduction is permitted which does not comply with these terms.
*Correspondence: Chenglai Xia, xiachenglai@smu.edu.cn; Shuangyi Ren, renshuangyidl@163.com