- School of Pharmacy, Nantong University, Nantong, Jiangsu, China
Platinum compounds-based drugs are used widely in the clinic for the treatment of many types of cancer. However, serious undesirable side effects and intrinsic or acquired resistance limit their successful clinic use. Nanocarrier-based combination chemotherapy is considered to be an effective strategy to resolve these challenges. This review introduces the recent advance in nanocarriers containing platinum compounds for combination cancer chemotherapy, including liposomes, polymer nanoparticles, polymer micelles, mesoporous silica nanoparticles, carbon nanohors, polymer-caged nanobins, carbon nanotube, nanostructured lipid carriers, solid lipid nanoparticles, and multilayered fiber mats in detail.
Introduction
Cancer is a primary cause of death and a significant obstacle to prolonging life spans around the world. According to GLOBOCAN 2020, the estimated number of new cases and deaths from cancer was 19.3 and 10 million, respectively (Sung et al., 2021). Platinum compounds-based drugs are used widely in the clinic for the treatment of various types of cancer, such as lung, testicular, gastric, bladder, ovary, and colorectal (Dilruba and Kalayda, 2016). Cisplatin, the first platinum compound, was prepared by Italian chemist Michel Peyrone in 1844 (Kauffman et al., 2010). Over one hundred years later, Rosenberg and his group found that the electrolyte of platinum electrode had an inhibitory effect on the cell division of Escherichia Coli (Rosenberg et al., 1965). It is inferred that platinum compounds can inhibit the growth of bacteria. Then, they discovered the anticancer effect of cisplatin in mice bearing sarcoma 180 and leukemia L1210 in 1969 (Rosenberg et al., 1969). Cisplatin was approved for the treatment of testicular and ovarian cancer by the US FDA in 1978 (Ghosh 2019). Cisplatin represents a success story in inorganic medicinal chemistry. It initiates the innovation in the development of platinum-based anticancer drugs until today. Currently, seven platinum-based anticancer drugs are approved for clinic use, including cisplatin, carboplatin, oxaliplatin, nedaplatin, lobaplatin, heptaplatin, and miriplatin (Johnstone et al., 2016) (Figure 1A).
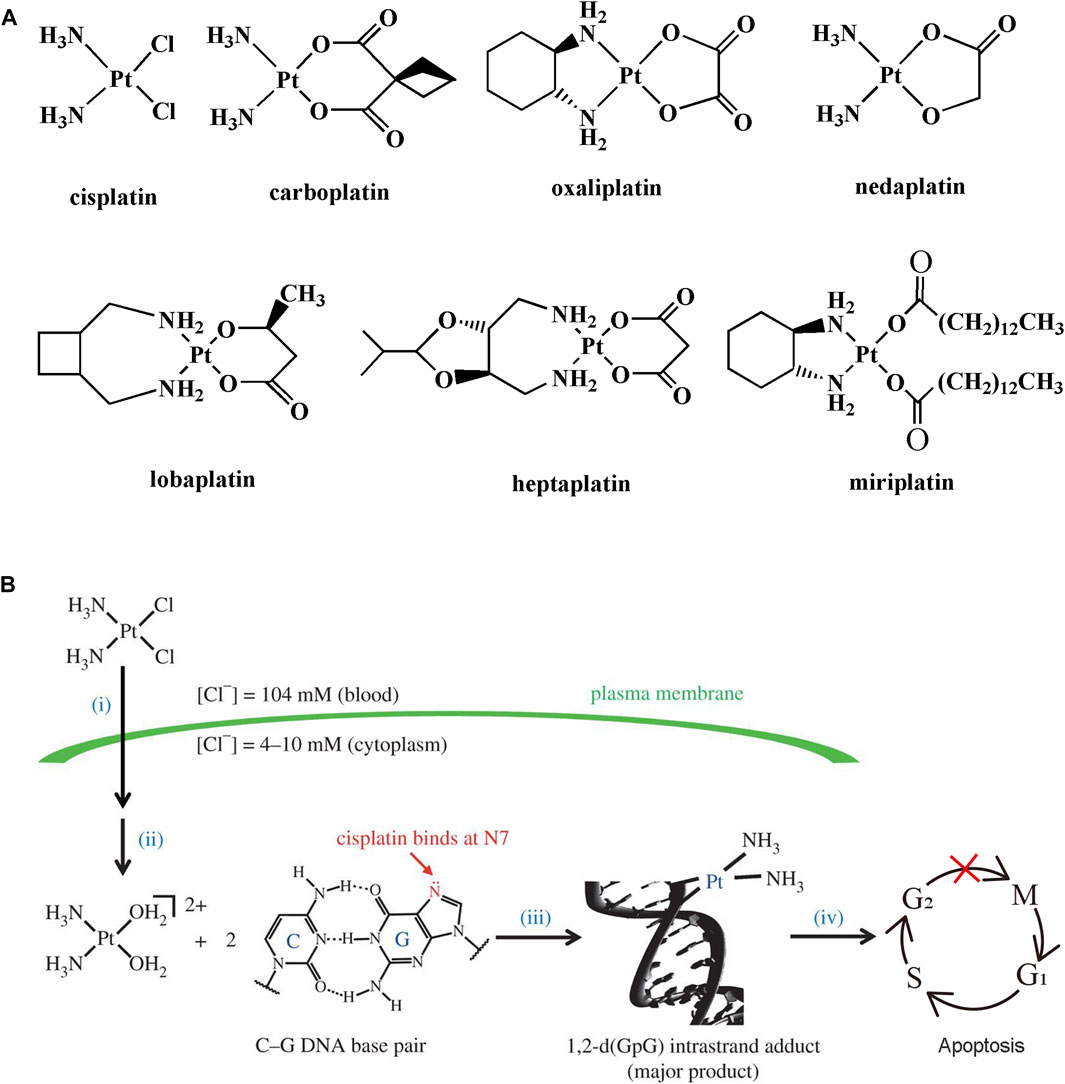
FIGURE 1. (A) Chemical structures of clinically used platinum-based anticancer drugs. (B) Mechanism action of cisplatin. (i) Cellular uptake, (ii) activation, (iii) DNA binding, and (iv) DNA damage trigger cell apoptosis. Reproduced from reference (Johnstone et al., 2015) with permission.
The mechanism of action of cisplatin and related platinum compounds has been elucidated over the past few decades (Wang and Lippard, 2005; Johnstone et al., 2015) (Figure 1B). The generally accepted mechanism contains the following four steps: 1) the dissolved cisplatin travels through the bloodstream to the extracellular matrix. The cellar uptake of cisplatin is mediated by passive diffusion or active transport relied on the membrane protein; 2) the concentration of chloride ions is uneven distribution inside and outside of the cells. The intracellular chloride ion concentration (4–10 mM) is much lower than that of the extracellular matrix (104 mM), which is beneficial for transformation of cisplatin to its cation hydrate such as cis-[Pt(NH3)2(OH2)2]2+; 3) platinum hydrate with strong electrophilic tendency preferentially binds to the N7 position of guanosine on DNA. The adjacent DNA bases are crosslinked by platinum hydrate; 4) finally, the DNA damage triggers cell cycle arrest at the S/G2 stage and induces cell death by apoptosis if the damage cannot be repaired.
Although platinum-based drugs have been widely used in the clinic to treat several types of cancers, many serious undesirable side effects and intrinsic or acquired resistance limit the further clinic use of platinum-based drugs (Markman 2003; Oun et al., 2018; Zhou et al., 2020). Common side effects include nephrotoxicity, myelosuppression, neurotoxicity, anaphylaxis, cytopenias, hepatotoxicity, ototoxicity, cardiotoxicity, and so on (Wong and Giandomenico, 1999; Mjos and Orvig, 2014; Oun et al., 2018). The various side effects prevented the administration of higher drug dosage. To relieve the side effects, patients need to lower the administration dosage. Moreover, patients need careful monitoring of their functional and biochemical markers during treatment and taking other non-chemotherapeutic drugs to combat side effects, such as antiemetics, propafenone, and antioxidants (Oun et al., 2018). The resistance mechanism of platinum-based drugs is badly complicated. It mainly includes decreased intracellular drug concentration, DNA damage and repair, evading apoptosis and autophagy (Ohmichi et al., 2005; Zhou et al., 2020). Acquired resistance to platinum drugs is mainly associated with a dramatic reduction of drug accumulation in the cell. Current studies have indicated that in addition to passive diffusion, the cellular uptake of platinum drugs was also medicated by multiple transporters, such as organic cation transporters (OCTs), copper transporters (CPTs) and multidrug resistance protein (MRP) (Ohmichi et al., 2005; Yokoo et al., 2007; Howell et al., 2010). Disease conditions can affect transporter expression, and thereby altering the cellular uptake of platinum drugs and affecting the therapeutic efficacy. Moreover, the enhanced DNA repair process exerted a tremendous influence on drug resistance (Howell et al., 2010). The mechanisms of DNA damage repair are complex. A nucleotide excision repair system could excise the damaged nucleotides in the majority of intrastrand cross-links and reconstruct the gene integrity (Yokoo et al., 2007). The function of many components played nonnegligible roles in the DNA repair process, such as breast cancer susceptibility genes and excision repair cross-complementing members (Oberoi et al., 2013; Zhang et al., 2022). Furthermore, evading apoptosis is a universal phenomenon of cancer cells (Parhi et al., 2012). To evade apoptosis, platinum-resistant cancer cells often overexpress anti-apoptotic proteins (e.g., Bcl-2) (Qin et al., 2018). It was also reported that pro-survival signal pathway (e.g., PI3K/AKT/mTOR pathway), epigenetic change, and tumor microenvironment also contributed to the development of drug resistance (Hu et al., 2016; Prihantono and Faruk, 2021; Pomeroy et al., 2022). In addition, drug-induced autophagy increased in the platinum-resistant cells after treatment with platinum drugs, and the addition of autophagy inhibitors could effectively reduce drug resistance (Lei et al., 2019). However, some scientists have come to the opposite conclusion (Li et al., 2014; Mokhtari et al., 2017). Therefore, further studies on the relationship between autophagy and platinum resistance are warranted.
Currently, numerous strategies have been explored to reduce the side effects and resistance of platinum drugs, such as chemical modification of the platinum drugs with a targeting moiety, delivery of platinum drugs using nanomaterials or hydrogels, combination chemotherapy, preparation of platinum nanoclusters and glutathione-scavenging platinum drugs (Oberoi et al., 2013; Oun et al., 2018; Zhang et al., 2022). Among them, combination chemotherapy and nanotechnology have received a lot of attention (Parhi et al., 2012). Combination chemotherapy has been widely used in clinics to solve the problems caused by single cancer chemotherapy. Combination chemotherapy involves the application of two or more drugs or simultaneous use of different therapeutic methods such as chemotherapy, ultrasound therapy, electrotherapy, and radiotherapy (Hu et al., 2016; Qin et al., 2018; Pomeroy et al., 2022). In these methods, the most common combination therapeutic modality is the co-administration of different therapeutic drugs. Combination chemotherapy can allow each drug to exert anticancer effects at a lower dosage by synergistically targeting different signal pathways or molecular targets, which could overcome resistance mechanism and reduce the side effects associated with the high dose of a single-drug, or retard the cancer adaptation through regulating the genetic barriers (Mokhtari et al., 2017; Lei et al., 2019; Prihantono and Faruk, 2021). Furthermore, combination chemotherapy can allow drugs synergistically target the same cellular signal pathways or molecular targets, improving the therapeutic effect (Li et al., 2014; Mokhtari et al., 2017). Dasanu et al. reported that the combined administration of carboplatin and gemcitabine was a safe and effective therapeutic method for metastatic ovarian cancer with reduced hematological toxicity (Dasanu et al., 2009).
Although combination administration has made some extent progress in the treatment of cancer, the huge achievement is impeded by poor water solubility, insufficient drug accumulation in the tumor tissue, rapid drug elimination, and improper in vivo distribution (Greco and Vicent, 2009). To overcome these limitations aforementioned, it is urgent to develop suitable drug delivery systems that could optimize the in vitro and in vivo characteristics of drugs and reduce the toxic and side effects. Nanotechnology has been proposed as an effective approach to improving the therapeutic effect of various cancers. Nanocarriers could increase the water solubility of poorly soluble drugs, prolong blood circulation time, and endow drugs with tumor targetability, which enhances the availability of drugs in the tumor cell while reducing the toxic and side effects associated with conventional chemotherapy (Bertrand and Leroux, 2012; Moradi Kashkooli et al., 2020). Several nanocarriers for cisplatin or cisplatin combination therapy have entered clinical trials. Lipoplatin, a liposomal cisplatin formulation with a particle size of about 110 nm, has passed phase III clinical trials, which exhibited excellent anticancer efficacy in several tumors, such as lung, colon, gastric, and prostate cancers (Li et al., 2015). In addition, Poly(glutamic acid) (PGlu) has been used widely in drug delivery systems. NC-6004 was a PGlu-based polymeric micelles containing cisplatin. It reduced nephrotoxicity compared with cisplatin alone in the clinical trials for the treatment of head and neck tumors, and pancreatic cancer (Song et al., 2014; Shen et al., 2017). Then, NC-6004/gemcitabine combination increased the median overall survival rate in a phase II study for the treatment of pancreatic cancer (He et al., 2015). These clinical trials demonstrated that nanocarriers containing platinum drugs have a promising prospect for the treatment of cancers. In this review, we provide a focused review on the use of nanocarriers to synergistically deliver platinum compounds and others for cancer therapy.
Nanocarriers containing platinum compounds for combination chemotherapy
Nanocarriers have been demonstrated to be effective drug carriers that can deliver two or more drugs with different physicochemical properties and pharmacological action and mechanisms for the treatment of cancer. The application of nanocarriers to encapsulate platinum compounds and other drugs for combination chemotherapy has been extensively studied and the details are summarized in Table 1. Cisplatin is the earliest and most widely used platinum compound for the treatment of various cancers in the clinic, such as ovaries, testes, head and neck, and lung cancer. However, unwanted side effects and drug resistance limited its more successful clinical application. Combination chemotherapy is a relatively effective method to reduce the unwanted side effects and drug resistance of cisplatin (Yang et al., 2017). Cisplatin-based combination chemotherapy has obtained some progress in the clinic. For example, cisplatin combined with etoposide or irinotecan for lung cancer; cisplatin combined with 5-fluorouracil, or gemcitabine, or paclitaxel for head and neck cancer (Gerweck, 2000; Chou, 2006; Liu et al., 2017; Yu et al., 2020). However, highly effective combination chemotherapy is not easy to design due to its different physicochemical and biological properties. Nanocarriers offered a better opportunity to address this problem. Among the combination chemotherapy of nanocarriers containing platinum compounds and other drugs, the combination of cisplatin and paclitaxel has received the most attention. Various nanocarriers have been prepared for the co-delivery of cisplatin and paclitaxel including mPEG-b-P(LA-co-MCC/Cis Pt(IV)) and mPEG P(LA-co-MCC/paclitaxel) self-assemble micelles (Xiao et al., 2012), telodendrimer micelles (Cai et al., 2015), mPEG-b-PAGE/MPA-b-PLA micelles (Li et al., 2015), mPEG-b-P(Glu)-b-P(Phe) micelles (Song et al., 2014), Pt(IV)-conjugated copolymer (mPEG-PLGA) micelles (Shen et al., 2017), Folic acid-modified PEG-PLGA nanoparticles and nanostructured lipid carriers (He et al., 2015; Yang et al., 2017), Trans-activating transcriptional activator modified solid lipid nanoparticles (Liu et al., 2017). Xuesi Chen’s Group prepared mPEG-b-P(Glu)-b-P(Phe) triblock copolymer self-assembled micelles and explored its co-delivery of cisplatin and paclitaxel for the treatment of lung cancer (Song et al., 2014). The formed core-shell-corona micelles had three specific zones: the inner hydrophobic P(Phe) core for loading paclitaxel, the anionic P(Glu) middle shell for cisplatin complexation, and PEG outer shell as protection corona. In vitro release experiments showed that paclitaxel released faster than cisplatin, which was due to the physical encapsulation of PTX compared to the complexation of Pt(II). Moreover, cisplatin exhibited fast-release behavior at pH 5.5 compared to that at pH 7.4, which was attributed to the weakened complexation of Pt(II) caused by the protonation of carboxylic groups of P(Glu) in an acidic medium. The fast-release behavior is beneficial for anti-tumor therapy because of the acidic microenvironment in tumor tissue (Gerweck, 2000). The cytotoxicity test showed that the proliferation inhibition effect of the double-drug loaded micelles was greater than that of the single-drug-loaded micelles only at 72 h, which was ascribed to the retarded drug release by double-drug loaded micelles. With the extension of time, the combined effect became more obvious. The combination index (CI) was often used to evaluate the synergistic effect (Chou, 2006). The double-drug loaded micelles showed a high synergistic effect on A549 cells in the range of drug effect levels (CI<1), while the free drug combination showed an obvious antagonistic effect (CI>1). Finally, the in vivo anti-tumor efficacy and system toxicity were evaluated in Balb/C nude mice bearing xenograft A549 human lung tumor. Dual-drug-loaded micelles showed a better tumor suppression rate (83.1%) than that of single paclitaxel groups (58.1% for paclitaxel solution and 67.8% for paclitaxel micelles) and free drug combination group (73.8%). In addition, the dual-drug-loaded micelles did not cause serious weight loss during treatment compared to the single paclitaxel group and free drug combination group. Overall, dual-drug-loaded micelles offer a better therapeutic efficacy in comparison with single-drug-loaded micelles and free drug combination groups.
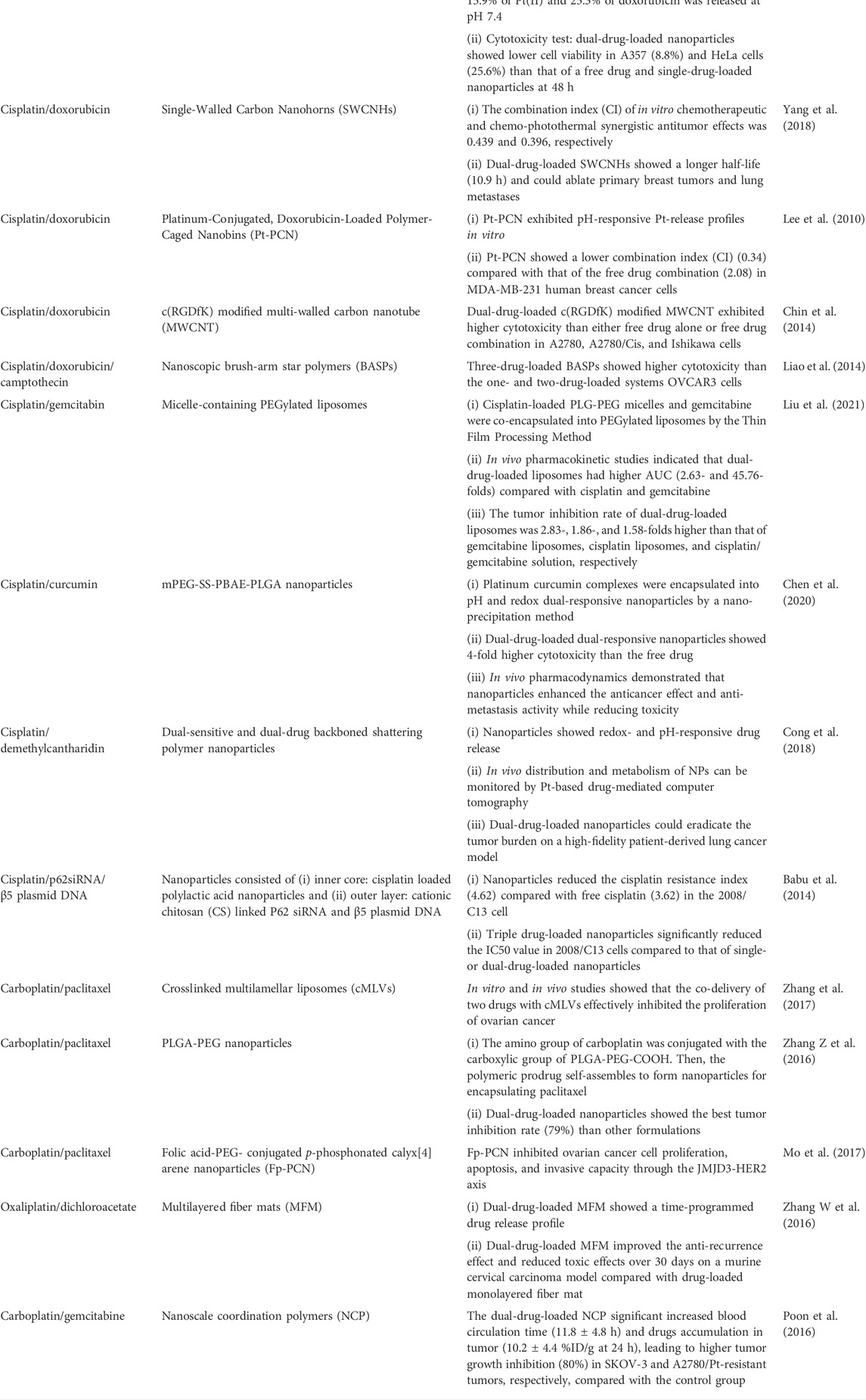
TABLE 1. Recent advances in nanocarriers containing platinum compounds and other drugs for combination chemotherapy.
Apart from the aforementioned co-delivery nanosystems for cisplatin and paclitaxel, co-delivery of cisplatin and doxorubicin by hyaluronic acid micelles, poly(acrylic acid) modified mesoporous silica nanoparticles, single-walled carbon nanohorns, polymer-caged nanobins, c(RGDfK) modified multi-walled carbon nanotube, and nanoscopic brush-arm star polymers has made significant progress in the treatment of cancers (Lee et al., 2010; Chin et al., 2014; Liao et al., 2014; Li et al., 2016; Yang et al., 2018; Yu et al., 2020). In addition to paclitaxel and doxorubicin, designed nanocarriers for the co-delivery of platinum drugs and gemcitabin, curcumin, demethylcantharidin, dichloroacetate, and gene drugs have also been extensively investigated (Babu et al., 2014; Poon et al., 2016; Zhang W et al., 2016; Cong et al., 2018; Chen et al., 2020; Liu et al., 2021). Therefore, nanocarriers containing platinum drugs and other drugs are a promising strategy for combination chemotherapy, which can prolong blood circulation time, increase drug accumulation in tumor cells, as well as enhance therapeutic efficacy, overcome intrinsic or acquired resistance and alleviate the toxic and side effects.
Conclusion and perspectives
Platinum drugs have been used commonly for the treatment of cancers in clinics. However, harmful side effects and drug resistance limited it to yielding better therapeutic efficacy. Considerable efforts have been made to solve these thorny issues until the advent of nanotechnology, which brought much confidence to scientists. The application of nanocarriers for the co-delivery of platinum compounds and other drugs for combination chemotherapy has achieved quite a few progresses owing to its advantages in overcoming intrinsic or acquired resistance and reducing side effects caused by the treatment with platinum agents. To date, just a few nanocarriers containing platinum drugs have entered clinical trials for combination chemotherapy. Therefore, more innovative studies are needed to facilitate the translation of nanocarriers containing platinum compounds and other drugs to the clinical product. First, the physicochemical properties, action mechanisms, ratio, and dosage of multiple-drug can affect the in vitro release behavior, cell uptake, and biodistribution, so to obtain a better synergistic anticancer effect, the desired combined drugs, ratio, and dosage in nanocarriers need to be optimized through multiple tests in vitro and in vivo. Furthermore, the constructed nanocarriers should ensure the release of drugs in tumor tissues and cells, such as tumor-targeting nanocarriers and stimulus-responsive nanocarriers, thereby improving therapeutic efficacy and reducing toxic side effects. Despite the design of nanocarriers for combination chemotherapy is complicated, building more intelligent nanocarriers based on the pharmacological purposes of different anticancer drugs will make our dream come true.
Author contributions
GF wrote the manuscript; AZ, LZ, and QW: performed the literature search of the databases; FS and BT: designed this work of review and revised the manuscript. All authors approved the paper for publication.
Funding
This work was supported by the Natural Science Foundation of Jiangsu Province (BK20170445), Jiangsu Planned Projects for Postdoctoral Research Funds (2021K271B).
Conflict of interest
The authors declare that the research was conducted in the absence of any commercial or financial relationships that could be construed as a potential conflict of interest.
Publisher’s note
All claims expressed in this article are solely those of the authors and do not necessarily represent those of their affiliated organizations, or those of the publisher, the editors and the reviewers. Any product that may be evaluated in this article, or claim that may be made by its manufacturer, is not guaranteed or endorsed by the publisher.
References
Babu, A., Wang, Q., Muralidharan, R., Shanker, M., Munshi, A., and Ramesh, R. (2014). Chitosan coated polylactic acid nanoparticle-mediated combinatorial delivery of cisplatin and siRNA/plasmid DNA chemosensitizes cisplatin-resistant human ovarian cancer cells. Mol. Pharm. 11, 2720–2733. doi:10.1021/mp500259e
Bertrand, N., and Leroux, J-C. (2012). The journey of a drug-carrier in the body: An anatomo-physiological perspective. J. Control. Release 161, 152–163. doi:10.1016/j.jconrel.2011.09.098
Cai, L., Xu, G., Shi, C., Guo, D., Wang, X., and Luo, J. (2015). Telodendrimer nanocarrier for co-delivery of paclitaxel and cisplatin: A synergistic combination nanotherapy for ovarian cancer treatment. Biomaterials 37, 456–468. doi:10.1016/j.biomaterials.2014.10.044
Chen, Y., Chen, C., Zhang, X., He, C., Zhao, P., Li, M., et al. (2020). Platinum complexes of curcumin delivered by dual-responsive polymeric nanoparticles improve chemotherapeutic efficacy based on the enhanced anti-metastasis activity and reduce side effects. Acta Pharm. Sin. B 10, 1106–1121. doi:10.1016/j.apsb.2019.10.011
Chin, C. F., Yap, S. Q., Li, J., Pastorin, G., and Ang, W. H. (2014). Ratiometric delivery of cisplatin and doxorubicin using tumour-targeting carbon-nanotubes entrapping platinum(iv) prodrugs. Chem. Sci. 5, 2265–2270. doi:10.1039/c3sc53106f
Chou, T-C. (2006). Theoretical basis, experimental design, and computerized simulation of synergism and antagonism in drug combination studies. Pharmacol. Rev. 58, 621–681. doi:10.1124/pr.58.3.10
Cong, Y., Xiao, H., Xiong, H., Wang, Z., Ding, J., Li, C., et al. (2018). Dual drug backboned shattering polymeric theranostic nanomedicine for synergistic eradication of patient-derived lung cancer. Adv. Mat. 30, 1706220. doi:10.1002/adma.201706220
Dasanu, C. A., Herzog, T. J., and Alexandrescu, D. T. (2009). Carboplatin—gemcitabine in the therapy of advanced ovarian cancer: Dose reduction consideration. J. Oncol. Pharm. Pract. 16, 63–66. doi:10.1177/1078155209105396
Dilruba, S., and Kalayda, G. V. (2016). Platinum-based drugs: Past, present and future. Cancer Chemother. Pharmacol. 77, 1103–1124. doi:10.1007/s00280-016-2976-z
Gerweck, L. E. (2000). The pH difference between tumor and normal tissue offers a tumor specific target for the treatment of cancer. Drug resist. updat. 3, 49–50. doi:10.1054/drup.2000.0122
Ghosh, S. (2019). Cisplatin: The first metal based anticancer drug. Bioorg. Chem. 88, 102925. doi:10.1016/j.bioorg.2019.102925
Greco, F., and Vicent, M. J. (2009). Combination therapy: Opportunities and challenges for polymer–drug conjugates as anticancer nanomedicines. Adv. Drug Deliv. Rev. 61, 1203–1213. doi:10.1016/j.addr.2009.05.006
He, Z., Huang, J., Xu, Y., Zhang, X., Teng, Y., Huang, C., et al. (2015). Co-delivery of cisplatin and paclitaxel by folic acid conjugated amphiphilic PEG-PLGA copolymer nanoparticles for the treatment of non-small lung cancer. Oncotarget 6, 42150–42168. doi:10.18632/oncotarget.6243
Howell, S. B., Safaei, R., Larson, C. A., and Sailor, M. J. (2010). Copper transporters and the cellular Pharmacology of the platinum-containing cancer drugs. Mol. Pharmacol. 77, 887–894. doi:10.1124/mol.109.063172
Hu, Q., Sun, W., Wang, C., and Gu, Z. (2016). Recent advances of cocktail chemotherapy by combination drug delivery systems. Adv. Drug Deliv. Rev. 98, 19–34. doi:10.1016/j.addr.2015.10.022
Johnstone, T. C., Suntharalingam, K., and Lippard, S. J. (2016). The next generation of platinum drugs: Targeted Pt(II) agents, nanoparticle delivery, and Pt(IV) prodrugs. Chem. Rev. 116, 3436–3486. doi:10.1021/acs.chemrev.5b00597
Johnstone, T. C., Suntharalingam, K., and Lippard, S. J. (2015). Third row transition metals for the treatment of cancer. Philos. Trans. A Math. Phys. Eng. Sci. 373, 20140185. doi:10.1098/rsta.2014.0185
Kauffman, G. B., Pentimalli, R., Doldi, S., and Hall, M. D. (2010). <I>Michele Peyrone (1813-1883), discoverer of Cisplatin</I>. platin. Met. Rev. 54, 250–256. doi:10.1595/147106710x534326
Lee, S-M., O’Halloran, T. V., and Nguyen, S. T. (2010). Polymer-caged nanobins for synergistic Cisplatin−Doxorubicin combination chemotherapy. J. Am. Chem. Soc. 132, 17130–17138. doi:10.1021/ja107333g
Lei, F., Xi, X., Batra, S. K., and Bronich, T. K. (2019). Combination therapies and drug delivery platforms in combating pancreatic cancer. J. Pharmacol. Exp. Ther. 370, 682–694. doi:10.1124/jpet.118.255786
Li, F., Zhao, C., and Wang, L. (2014). Molecular-targeted agents combination therapy for cancer: Developments and potentials. Int. J. Cancer 134, 1257–1269. doi:10.1002/ijc.28261
Li, H., Yu, H., Zhu, C., Hu, J., Du, M., Zhang, F., et al. (2016). Cisplatin and doxorubicin dual-loaded mesoporous silica nanoparticles for controlled drug delivery. RSC Adv. 6, 94160–94169. doi:10.1039/c6ra17213j
Li, J., Li, Z., Li, M., Zhang, H., and Xie, Z. (2015). Synergistic effect and drug-resistance relief of paclitaxel and cisplatin caused by Co-delivery using polymeric micelles. J. Appl. Polym. Sci. 132. doi:10.1002/app.41440
Liao, L., Liu, J., Dreaden, E. C., Morton, S. W., Shopsowitz, K. E., Hammond, P. T., et al. (2014). A convergent synthetic platform for single-nanoparticle combination cancer therapy: Ratiometric loading and controlled release of cisplatin, doxorubicin, and camptothecin. J. Am. Chem. Soc. 136, 5896–5899. doi:10.1021/ja502011g
Liu, B., Han, L., Liu, J., Han, S., Chen, Z., and Jiang, L. (2017). Co-delivery of paclitaxel and TOS-cisplatin via TAT-targeted solid lipid nanoparticles with synergistic antitumor activity against cervical cancer. Int. J. Nanomedicine 12, 955–968. doi:10.2147/IJN.S115136
Liu, Z., Chu, W., Sun, Q., Zhao, L., Tan, X., Zhang, Y., et al. (2021). Micelle-contained and PEGylated hybrid liposomes of combined gemcitabine and cisplatin delivery for enhancing antitumor activity. Int. J. Pharm. 602, 120619. doi:10.1016/j.ijpharm.2021.120619
Markman, M. (2003). Toxicities of the platinum antineoplastic agents. Expert Opin. Drug Saf. 2, 597–607. doi:10.1517/14740338.2.6.597
Mjos, K. D., and Orvig, C. (2014). Metallodrugs in medicinal inorganic chemistry. Chem. Rev. 114, 4540–4563. doi:10.1021/cr400460s
Mo, J., Wang, L., Huang, X., Lu, B., Zou, C., Wei, L., et al. (2017). Multifunctional nanoparticles for co-delivery of paclitaxel and carboplatin against ovarian cancer by inactivating the JMJD3-HER2 axis. Nanoscale 9, 13142–13152. doi:10.1039/c7nr04473a
Mokhtari, R. B., Homayouni, T. S., Baluch, N., Morgatskaya, E., Kumar, S., Das, B., et al. (2017). Combination therapy in combating cancer. Oncotarget 8, 38022–38043. doi:10.18632/oncotarget.16723
Moradi Kashkooli, F., Soltani, M., and Souri, M. (2020). Controlled anti-cancer drug release through advanced nano-drug delivery systems: Static and dynamic targeting strategies. J. Control. Release 327, 316–349. doi:10.1016/j.jconrel.2020.08.012
Oberoi, H. S., Nukolova, N. V., Kabanov, A. V., and Bronich, T. K. (2013). Nanocarriers for delivery of platinum anticancer drugs. Adv. Drug Deliv. Rev. 65, 1667–1685. doi:10.1016/j.addr.2013.09.014
Ohmichi, M., Hayakawa, J., Tasaka, K., Kurachi, H., and Murata, Y. (2005). Mechanisms of platinum drug resistance. Trends Pharmacol. Sci. 26, 113–116. doi:10.1016/j.tips.2005.01.002
Oun, R., Moussa, Y. E., and Wheate, N. J. (2018). The side effects of platinum-based chemotherapy drugs: A review for chemists. Dalton Trans. 47, 6645–6653. doi:10.1039/c8dt00838h
Parhi, P., Mohanty, C., and Sahoo, S. K. (2012). Nanotechnology-based combinational drug delivery: An emerging approach for cancer therapy. Drug Discov. Today 17, 1044–1052. doi:10.1016/j.drudis.2012.05.010
Pomeroy, A. E., Schmidt, E. V., Sorger, P. K., and Palmer, A. C. (2022). Drug independence and the curability of cancer by combination chemotherapy. Trends Cancer 8, 915–929. doi:10.1016/j.trecan.2022.06.009
Poon, C., Duan, X., Chan, C., Han, W., and Lin, W. (2016). Nanoscale coordination polymers codeliver carboplatin and gemcitabine for highly effective treatment of platinum-resistant ovarian cancer. Mol. Pharm. 13, 3665–3675. doi:10.1021/acs.molpharmaceut.6b00466
Prihantono, , and Faruk, M. (2021). Breast cancer resistance to chemotherapy: When should we suspect it and how can we prevent it? Ann. Med. Surg. 70, 102793. doi:10.1016/j.amsu.2021.102793
Qin, S-Y., Cheng, Y-J., Lei, Q., Zhang, A-Q., and Zhang, X-Z. (2018). Combinational strategy for high-performance cancer chemotherapy. Biomaterials 171, 178–197. doi:10.1016/j.biomaterials.2018.04.027
Rosenberg, B., Van Camp, L., and Krigas, T. (1965). Inhibition of cell division in Escherichia coli by electrolysis products from a platinum electrode. Nature 205, 698–699. doi:10.1038/205698a0
Rosenberg, B., Vancamp, L., Trosko, J. E., and Mansour, V. H. (1969). Platinum compounds: A new class of potent antitumour agents. Nature 222, 385–386. doi:10.1038/222385a0
Shen, W., Chen, X., Luan, J., Wang, D., Yu, L., and Ding, J. (2017). Sustained codelivery of cisplatin and paclitaxel via an injectable prodrug hydrogel for ovarian cancer treatment. ACS Appl. Mat. Interfaces 9, 40031–40046. doi:10.1021/acsami.7b11998
Song, W., Tang, Z., Li, M., Lv, S., Sun, H., Deng, M., et al. (2014). Polypeptide-based combination of paclitaxel and cisplatin for enhanced chemotherapy efficacy and reduced side-effects. Acta Biomater. 10, 1392–1402. doi:10.1016/j.actbio.2013.11.026
Sung, H., Ferlay, J., Siegel, R. L., Laversanne, M., Soerjomataram, I., Jemal, A., et al. (2021). Global cancer statistics 2020: GLOBOCAN estimates of incidence and mortality worldwide for 36 cancers in 185 countries. Ca. Cancer J. Clin. 71, 209–249. doi:10.3322/caac.21660
Wang, D., and Lippard, S. J. (2005). Cellular processing of platinum anticancer drugs. Nat. Rev. Drug Discov. 4, 307–320. doi:10.1038/nrd1691
Wong, E., and Giandomenico, C. M. (1999). Current status of platinum-based antitumor drugs. Chem. Rev. 99, 2451–2466. doi:10.1021/cr980420v
Xiao, H., Song, H., Yang, Q., Cai, H., Qi, R., Yan, L., et al. (2012). A prodrug strategy to deliver cisplatin(IV) and paclitaxel in nanomicelles to improve efficacy and tolerance. Biomaterials 33, 6507–6519. doi:10.1016/j.biomaterials.2012.05.049
Yang, J., Ju, Z., and Dong, S. (2017). Cisplatin and paclitaxel co-delivered by folate-decorated lipid carriers for the treatment of head and neck cancer. Drug Deliv. 24, 792–799. doi:10.1080/10717544.2016.1236849
Yang, J., Su, H., Sun, W., Cai, J., Liu, S., Chai, Y., et al. (2018). Dual chemodrug-loaded single-walled carbon nanohorns for multimodal imaging-guided chemo-photothermal therapy of tumors and lung metastases. Theranostics 8, 1966–1984. doi:10.7150/thno.23848
Yokoo, S., Yonezawa, A., Masuda, S., Fukatsu, A., Katsura, T., and Inui, K-I. (2007). Differential contribution of organic cation transporters, OCT2 and MATE1, in platinum agent-induced nephrotoxicity. Biochem. Pharmacol. 74, 477–487. doi:10.1016/j.bcp.2007.03.004
Yu, T., Li, Y., Gu, X., and Li, Q. (2020). Development of a hyaluronic acid-based nanocarrier incorporating doxorubicin and cisplatin as a pH-sensitive and CD44-targeted anti-breast cancer drug delivery system. Front. Pharmacol. 11, 532457. doi:10.3389/fphar.2020.532457
Zhang, C., Xu, C., Gao, X., and Yao, Q. (2022). Platinum-based drugs for cancer therapy and anti-tumor strategies. Theranostics 12, 2115–2132. doi:10.7150/thno.69424
Zhang, W., Li, C., Shen, C., Liu, Y., Zhao, X., Liu, Y., et al. (2016). Prodrug-based nano-drug delivery system for co-encapsulate paclitaxel and carboplatin for lung cancer treatment. Drug Deliv. 23, 2575–2580. doi:10.3109/10717544.2015.1035466
Zhang, X., Liu, Y., Kim, Y. J., Mac, J., Zhuang, R., and Wang, P. (2017). Co-delivery of carboplatin and paclitaxel via cross-linked multilamellar liposomes for ovarian cancer treatment. RSC Adv. 7, 19685–19693. doi:10.1039/c7ra01100h
Zhang, Z., Liu, S., Qi, Y., Zhou, D., Xie, Z., Jing, X., et al. (2016). Time-programmed DCA and oxaliplatin release by multilayered nanofiber mats in prevention of local cancer recurrence following surgery. J. Control. Release 235, 125–133. doi:10.1016/j.jconrel.2016.05.046
Keywords: platinum compounds, side effects, resistance, nanocarriers, combination chemotherapy
Citation: Fang G, Zhang A, Zhu L, Wang Q, Sun F and Tang B (2022) Nanocarriers containing platinum compounds for combination chemotherapy. Front. Pharmacol. 13:1050928. doi: 10.3389/fphar.2022.1050928
Received: 22 September 2022; Accepted: 25 October 2022;
Published: 08 November 2022.
Edited by:
Yi Gou, Guilin Medical University, ChinaReviewed by:
Haowen Jiang, Shanghai Institute of Materia Medica (CAS), ChinaMing Jiang, Guangxi Science and Technology Normal University, China
Copyright © 2022 Fang, Zhang, Zhu, Wang, Sun and Tang. This is an open-access article distributed under the terms of the Creative Commons Attribution License (CC BY). The use, distribution or reproduction in other forums is permitted, provided the original author(s) and the copyright owner(s) are credited and that the original publication in this journal is cited, in accordance with accepted academic practice. No use, distribution or reproduction is permitted which does not comply with these terms.
*Correspondence: Feilong Sun, ZnN1bkBudHUuZWR1LmNu; Bo Tang, dGFuZ2JvQG50dS5lZHUuY24=