- 1Infectious Disease Pharmacokinetics Lab, Emerging Pathogens Institute, University of Florida, Gainesville, FL, United States
- 2Department of Pharmacotherapy and Translational Research, College of Pharmacy, University of Florida, Gainesville, FL, United States
- 3Department of Medicine, Division of Internal Medicine, Duke University, Durham, NC, United States
- 4National Center for Tuberculosis and Lung Diseases, Tbilisi, Georgia
- 5David Tvildiani Medical University, Tbilisi, Georgia
- 6Department of Medicine, Division of Infectious Diseases, Emory University, Atlanta, GA, United States
Background: Tuberculosis meningitis (TBM) is the most lethal form of TB. It is difficult to treat in part due to poor or uncertain drug penetration into the central nervous system (CNS). To help fill this knowledge gap, we evaluated the cerebrospinal fluid (CSF) concentrations of fluoroquinolones and carbapenems in patients being treated for TBM.
Methods: Serial serum and CSF samples were collected from hospitalized patients being treated for TBM. CSF was collected from routine lumbar punctures between alternating timepoints of 2 and 6 h after drug administration to capture early and late CSF penetration. Rich serum sampling was collected after drug administration on day 28 for non-compartmental analysis.
Results: Among 22 patients treated for TBM (8 with confirmed disease), there was high use of fluoroquinolones (levofloxacin, 21; moxifloxacin, 10; ofloxacin, 6) and carbapenems (imipenem, 11; meropenem, 6). Median CSF total concentrations of levofloxacin at 2 and 6 h were 1.34 mg/L and 3.36 mg/L with adjusted CSF/serum ratios of 0.41 and 0.63, respectively. For moxifloxacin, the median CSF total concentrations at 2 and 6 h were 0.78 mg/L and 1.02 mg/L with adjusted CSF/serum ratios of 0.44 and 0.62. Serum and CSF concentrations of moxifloxacin were not affected by rifampin use. Among the 76 CSF samples measured for carbapenem concentrations, 79% were undetectable or below the limit of detection.
Conclusion: Fluoroquinolones demonstrated high CSF penetration indicating their potential usefulness for the treatment of TBM. Carbapenems had lower than expected CSF concentrations.
Introduction
Tuberculosis (TB) is an infectious disease caused by Mycobacterium tuberculosis (Mtb) and remains a major cause of mortality globally. In 2020, approximately 10 million people developed active disease and there were 1.5 million deaths, representing the first annual increase in deaths since 2005 (World Health Organization, 2021). While the lungs are the major site of TB infection, extrapulmonary disease can affect virtually all other body sites. Extrapulmonary TB accounts for approximately 15%–20% of all cases and the most severe manifestation is tuberculosis meningitis (TBM) (Sharma et al., 2021). TBM can be devastating, leading to substantial neurological impairments, paralysis, seizures, and high mortality (Bartzatt, 2011). Mortality rates during treatment for TBM are 20%–69%, with higher rates seen in cohorts of patients with drug resistant TB (Christensen et al., 2011; Dheda et al., 2017).
A major challenge in the treatment of TBM is drug delivery to the site of disease. Central nervous system (CNS) penetration of first-line TB medications including rifampin and ethambutol can be suboptimal at standard dosages, seldom exceeding minimal inhibitory concentrations (MIC) (Donald, 2010). This is especially a concern following resolution of meningeal inflammation (Thwaites et al., 2013; Roos, 2000). Second-line medications including fluoroquinolones appear to have improved penetration across the blood brain barrier, making them appealing antibiotics for the treatment of TBM (Litjens et al., 2020). Fluoroquinolones, such as levofloxacin and moxifloxacin, may be implemented into both short term and long-term regimens (Ghimire et al., 2019). Among the fluoroquinolones, moxifloxacin appears to have the highest in vitro activity against Mtb. However, concentrations may be most susceptible to reduction if administered with rifampin, compromising treatment efficacy (Nijland et al., 2007; Ramachandran et al., 2012). While penetration of fluoroquinolones appears to be adequate in bacterial CNS infections, additional data regarding penetration specifically in the treatment of TBM is needed.
Carbapenems are extended-spectrum beta-lactams with broad antimicrobial activity, including activity against Mtb (Jaganath et al., 2016). They are utilized for both multidrug-resistant and extensively drug-resistant TB, even though published data supporting their use is limited. To improve activity against Mtb, carbapenems are often administered with clavulanic acid, which blocks class A beta-lactamases (van Rijn et al., 2019). Data regarding imipenem and meropenem penetration into the CSF suggests adequate exposure to eradicate most pathogens, especially in the presence of CNS inflammation (Zhanel et al., 2007; Craig, 1997). However, data is limited regarding the CNS penetration of carbapenems in TBM (Jaganath et al., 2016).
To further understand the exposure of fluoroquinolones and carbapenems in TBM, our objective was to describe and compare serum and CSF concentrations of fluoroquinolones (levofloxacin, moxifloxacin, and ofloxacin) and carbapenems (imipenem and meropenem) among persons treated for TBM.
Materials and methods
Setting and participants
This prospective study enrolled participants at the National Center for Tuberculosis and Lung Diseases (NCTLD) in Tbilisi, Georgia. The NCTLD includes an inpatient ward for patients with TBM and directly observed therapy clinics for ambulatory care. Study participants were selected from a larger prospective cohort evaluating clinical outcomes among persons treated for TBM (Smith et al., 2021). Written formal consent was obtained from all study participants. The study was approved by the institutional review boards of the NCTLD (IRB# IORG0009467), the University of Florida, Gainesville, FL, United States, and Emory University, Atlanta, GA, United States.
All patients underwent a lumbar puncture and CSF diagnostic testing, including acid-fast bacilli (AFB) staining, molecular testing with Xpert® MTB/RIF assay (Cepheid, United States) and AFB culture on a Lowenstein-Jensen solid and Mycobacterial Growth Indicator Tube liquid media. To identify drug resistance to rifampin and isoniazid, a Genotype® MTBDRplus (Hain-Lifescience, Germany) assay was performed on positive cultures. Serial lumbar punctures were performed at 7, 14, 28 days, and then monthly after treatment initiation per standard of care. With regards to treatment and dosing, WHO guidance was followed and treatment regimens were based on the Georgian National TB Program 2015 guidelines, as outlined previously (Smith et al., 2021). Both levofloxacin and moxifloxacin were given by mouth (levofloxacin dose was either 750 mg or 1000mg, moxifloxacin 400 mg or 1000 mg). Ofloxacin was administered as 800 mg intravenously every 12 h. Meropenem and imipenem were administered intravenously as 1000 mg every 12 h. Multiple participants received more than one study drug of interest from the same class, but not concurrently. All participants received multidrug regimens for TBM, although only fluoroquinolones and carbapenems were analyzed in the present study (Smith et al., 2021). For MDR-TBM, regimens were chosen based upon Georgian National TB guidelines, and patients received regimens including carbapenems, injectable agents (such as amikacin), linezolid, and novel/repurposed anti-TBM agents such as clofazimine, delamanid, and bedaquiline (Smith et al., 2021). Additionally, all participants received adjunctive dexamethasone therapy for 6–8 weeks. Participants were hospitalized for treatment until clinical improvement such that they could be treated in an outpatient setting (Smith et al., 2021; Kempker et al., 2022).
Drug sample collection and quantification
Participants enrolled in the pharmacokinetic (PK) study had both serum and CSF samples collected at baseline and at approximately 7, 14, and 28 days following initiation of treatment. Monthly samples were subsequently collected during hospitalization for up to 112 days. Participants had two- and 6-h samples collected following drug administration from both serum and CSF. The CSF sampling was alternated between two- and 6-h concentrations to assess for early and delayed penetration. Rich serum sampling for non-compartmental analysis (NCA) was performed at 28 days, and the collection timepoints were 0, 2, 4, 8, 12, and 24 h after drug administration with slight variations for persons receiving bedaquiline (0, 2, 6, 12, 24, 48, 72 h) and delamanid (0, 1, 2, 4, 6,8 and 12 h). Following serum and CSF collection, samples were centrifuged then stored at −80°C at the NCTLD until shipping to the Infectious Disease Pharmacokinetic Laboratory (IDPL) at the University of Florida, with the cold chain remaining intact throughout. Total drug concentrations were quantified using validated liquid chromatography tandem mass spectrometry assays. Serum curve was used for the serum samples, while artificial CSF curve was used for CSF samples to match CSF matrix. The plasma and CSF detection ranges for levofloxacin, moxifloxacin, and ofloxacin were 0.2–15 mg/L and 2–100 mg/L for meropenem and imipenem. Intra- and inter-batch precision and accuracy were <10%. Samples below the limit of quantification (BLQ) were assigned a value of “0” for analysis.
Data management
NCA was performed using Phoenix WinNonlin (Certara, v8.3) to determine maximum serum concentration (Cmax), time to maximum concentration (Tmax), elimination rate constant (Ke), half-life (t1/2) and area under the concentration-time curve over 24 h (AUC0-24). Serum protein binding rates for levofloxacin (31%), moxifloxacin (40%), ofloxacin (32%), imipenem (20%), and meropenem (2%) were obtained from previously published literature and used to estimate free serum drug concentrations (Craig, 1997), (Lamp et al., 1992; Janssen Pharmaceuticals and Inc, 1996; Pickerill et al., 2000; Ortho-McNeil-Janssen Pharmaceuticals and Inc, 2008; Bolon, 2009; Salmon-Rousseau et al., 2020). We assumed there was no protein binding in the CSF (i.e., 100% free drug) (Bonati et al., 1982; de Lange, 2013). To assess CSF penetration, a ratio of CSF to serum concentrations was calculated for matching time points for participants. Adjusted CSF to serum ratios were calculated to account for serum protein binding. Samples BLQ or zero were excluded in the ratio calculations.
Continuous data were presented as median and interquartile range, and categorical data as count and percentage. Student t-tests were used to assess for differences in drug concentrations at 2 and 6 h, differences in the CSF to serum ratios between 2 and 6 h, and compare CSF concentrations from baseline over the duration of therapy to the final drawn sample for each drug at two and 6-h timepoints. JMP Pro v16.1 (JMP, Cary, NC, United States) was used for statistical analysis.
Results
Twenty-two participants were enrolled in the PK study, all of whom received a fluoroquinolone and/or carbapenem. Over half (55%) of enrolled patients were female and the median (IQR) age was 38 (29.3–47.8) years. Additional information regarding baseline demographics, comorbidities, and relevant clinical measures including CSF composition are included in Tables 1, 2. Of note, CSF white blood cell count declined over the first 28 days of treatment (Table 2). Trends in fluoroquinolone and carbapenem CSF and serum concentrations after diagnosis of TBM can be visualized in (Figure 1).
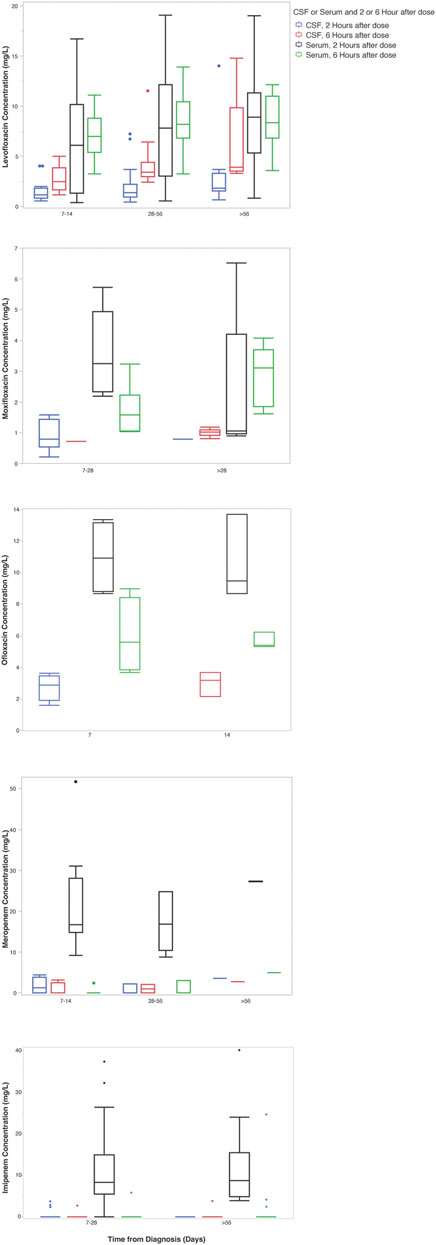
FIGURE 1. Carbapenem and fluoroquinolone CSF and serum concentrations over time from diagnosis. *The x-axis indicates the time in days from the start of any anti-tuberculosis treatment. Boxes indicate the median and interquartile range of the samples.
Fluoroquinolones
A total of 21 participants received levofloxacin during their treatment, resulting in 45 matching CSF and serum samples at 2 h and 38 at 6 h (Table 3). Median (IQR) total serum concentrations for levofloxacin at 2 and 6 h were 7.36 mg/L (2.83–11.21) and 7.68 mg/L (5.75–10.11), while total CSF concentrations at 2 and 6 h were 1.34 mg/L (0.91–1.98) and 3.36 mg/L (2.43–4.03). Cmax concentrations through NCA were 11.62 mg/L (8.64–13.12), with a Tmax of 4 h (Table 4). Median CSF to serum ratios adjusted for protein binding were higher at six versus 2 h (0.63 vs 0.41, p = 0.05). Following TBM diagnosis, levofloxacin CSF concentrations increased significantly from baseline at both 2 h (p = 0.02) and 6 h (p = 0.002) (Table 5).
Ten participants received moxifloxacin during treatment, 6 (60%) following a change in therapy from levofloxacin. There were 10 and six matching CSF and serum samples at 2 and 6 h, respectively. Median (IQR) total serum concentrations at 2 and 6 h were 2.74 mg/L (1.65–4.84) and 1.91 mg/L (1.56–3.28), while median total CSF concentrations at 2 and 6 h were 0.78 mg/L (0.63–1.40) and 1.02 mg/L (0.78–1.06) (Table 3). Cmax concentrations through NCA were 4.04 mg/L (2.78–5.34), with a Tmax of 2 h (Table 4). Median CSF to serum concentrations adjusted for protein binding increased from 2 to 6 h (0.44 vs 0.62, p = 0.05). Following TBM diagnosis and treatment initiation, moxifloxacin in CSF trended towards increased concentrations, but this was not statistically significant Among the participants receiving moxifloxacin, seven received concomitant rifampin, yet no difference in moxifloxacin serum AUC0-24 were seen between patients receiving rifampin versus those not receiving rifampin (32.86 vs 33.38 Hr*mg/L, p = 1.00). Additionally, CSF concentrations were not significantly different between patients receiving rifampin versus not receiving rifampin at 2 h (0.78 vs. 0.67 mg/L, p = 0.78) or 6 h (1.02 vs 0.72 mg/L, p = 0.72).
Five participants received ofloxacin, with four matched CSF to serum samples at 2 h and three at 6 h. Median (IQR) total serum concentrations at 2 and 6 h were 9.47 mg/L (8.67–13.36) and 5.40 mg/L (4.43–6.75), while total CSF concentrations at 2 and 6 h were 2.22 mg/L (0.93–3.11) and 3.20 mg/L (2.17–3.69) (Table 3). Median Cmax concentrations through NCA were 9.47 mg/L (8.67–13.36), with a Tmax 2 h (Table 4). Median CSF to serum concentrations adjusted for protein binding were higher at 6 h versus 2 h (0.76 vs 0.37, p = 0.03) (Table 3). Ofloxacin CSF concentrations at 2 h increased significantly from baseline (p = 0.04) whereas concentrations at the 6-h time-point did not (p = 0.28) (Table 5).
Carbapenems
There were 11 participants who received imipenem resulting in three matched CSF to serum samples at 2 h and two at 6 h. For meropenem, six participants with six matched CSF to serum samples at 2 h and one matched sample at 6 h were available. With the exception of 2-h serum samples, a substantial number of samples were undetectable or BLQ (<2 mg/L) for both serum and CSF (Table 6). 12/15 (80%) of meropenem total serum concentrations at 6 h were undetectable or BLQ in addition to 6/12 (50%) and 5/9 (56%) meropenem total CSF concentrations at 2 and 6 h 39/43 (91%) imipenem total serum concentrations at 6 h were undetectable or BLQ in addition to 28/32 (88%) and 21/23 (91%) total CSF concentrations at 2 and 6 h, respectively. Meropenem had more detectable levels in the CSF than imipenem.
Discussion
Our findings demonstrate a high CSF penetration of fluoroquinolone antibiotics and provide support for their consideration in the treatment of TBM. In particular, the CSF concentrations of levofloxacin were high and above the Mtb susceptibility cutoff in most samples tested, supporting its ability to achieve effective concentrations in TBM. Our study also provides novel data on the CSF concentrations of antibiotics over time which unexpectedly trended higher with the fluoroquinolones despite decreasing CSF inflammation, providing data that continued moderate-high CSF concentrations can be obtained during treatment. While current clinical trial data have not shown an ability of fluoroquinolones to improve outcomes among patients treated for drug-susceptible TBM, our study provides key pharmacokinetic data for their continued study in clinical trials and use in drug-resistant TBM (Heemskerk et al., 2016; Huynh et al., 2022). To the contrary, our study revealed that the carbapenems did not achieve adequate concentrations in our patient cohort, questioning their utility for patients with TBM.
All fluoroquinolones in our study had median serum Cmax values within normal ranges (8–12 mg/L for levofloxacin and ofloxacin, 3–5 mg/L for moxifloxacin) indicating typical systemic exposure (Alsultan and Peloquin, 2014). Our finding of increasing CSF concentrations from 2 to 6 h demonstrates delayed penetration which has important implications. In the case of levofloxacin, this delay may have been in part from delayed oral absorption as median serum concentrations increased from 2 to 6 h. These findings suggest clinical sampling of fluoroquinolone serum and CSF concentrations may be optimized by including both early and late samples. Due to their moderate lipophilic and protein bound nature in conjunction with relatively low molecular mass (Nau et al., 2010; Nau et al., 1994), fluoroquinolones enter CSF more completely than other antibiotics, with an estimated CSF concentration of approximately 20–80% of peak serum levels (Andes and Craig, 1999; Wilkinson et al., 2017). Our data support these published estimates, with median levofloxacin CSF concentrations ∼41% of total serum concentrations at 2 h and −63% at 6 h, and ofloxacin reaching −37% and −75% of total serum concentrations at 2 and 6 h post dose, respectively. Fluoroquinolone bacterial killing depends on concentration (both Cmax and AUC), with a proposed Cmax/MIC ratio target of >8–10 in TB (Turnidge, 1999; Berning, 2001; Levison and Levison, 2009). Although we were unable to calculate CSF Cmax/MIC or AUC0-24/MIC for this population, fluoroquinolones in our study achieved concentrations within expected ranges, suggesting potential attainment of targets. Assuming susceptible MICs of 1 mg/L for ofloxacin and 0.5 mg/L for levofloxacin and moxifloxacin, levofloxacin may have the best potential to achieve PK/PD targets given our findings (Angeby et al., 2010). In addition, we found that levofloxacin CSF concentrations increased from baseline at both two and 6-h timepoints, supporting previous research that fluoroquinolones may adequately penetrate into the CNS regardless of inflammation (Andes and Craig, 1999; Wilkinson et al., 2017). The increase in CSF concentrations over time may be the result of drug accumulation and changes in the inflammatory state/blood brain barrier. These findings improve confidence that patients receiving fluoroquinolones for TBM can continue to have CSF drug exposure throughout therapy. Interestingly, while coadministration of moxifloxacin with rifampin has been associated with a reduction of moxifloxacin concentrations by approximately 26%–32% due to rifampin’s induction of cytochrome P450 enzymes, the present study did not find any statistically significant differences in moxifloxacin exposure (Nijland et al., 2007).
Although carbapenems have shown efficacy in bacterial meningitis and against Mtb in vitro and in vivo animal models (Chambers et al., 1995; Hugonnet et al., 2009; Veziris et al., 2011; Kaushik et al., 2015), this study found lower than expected CSF concentrations. While meropenem and imipenem had similar PK profiles to previous literature, median serum Cmax concentrations were below previously reported ranges after a 1-g dose (60–70 mg/L for imipenem and 50–60 mg/L for meropenem) (Zhanel et al., 2007). CSF penetration of imipenem and meropenem in previously published literature ranged from −16 to 41% of serum for imipenem and −11% for meropenem (Andes and Craig, 1999). In the present study, the majority of imipenem and meropenem CSF concentrations were either undetectable or BLQ. One potential contributor to our unexpected low CSF concentrations is that beta lactams may diffuse from the central nervous compartments to the blood during meningitis due to an increase in CSF pH, facilitating excretion of beta lactams (Nau et al., 2010; Thea and Barza, 1989). However, data suggests that brain tissue concentrations of meropenem may be sufficient, even in non-inflamed brain tissue (Hosmann et al., 2021). Techniques such as cerebral microdialysis or innovative molecular imaging methods are needed for a better understanding of the CNS penetration of carbapenems in the setting of infections such as TBM (Tucker et al., 2018), (Brunner et al., 2004).
While increased penetration of antimicrobials into the CNS is desired for treatment of TBM, this may be associated with increased risk of CNS-related adverse effects. Previous literature has described adverse CNS effects for fluoroquinolones and carbapenems, potentially due to interferences with gamma-aminobutyric acid receptors (Akahane et al., 1989; Wong et al., 1991; Hikida et al., 1993; Domagala, 1994; Sunagawa et al., 1995; Walton et al., 1997; Kushner et al., 2001; Owens and Ambrose, 2005; Mehlhorn and Brown, 2007; Kalita et al., 2016). Notably, while rate of seizures in patients receiving intravenous imipenem for bacterial meningitis has been reported as high as 33% (Wong et al., 1991), the safety margin is considered to be higher for meropenem (Norrby, 1996). It is promising that in this study, none of the participants experienced seizures.
There are limitations to this study, including a relatively small sample size of 22 patients. Also, due to the descriptive nature of the study, the impact of co-administered medications, renal function, and hepatic function were not controlled for in the analysis. However, expected impact is minimal. While we assumed the CSF samples with undetectable imipenem and meropenem concentrations were due to poor CSF penetration, we could not rule out other causes. The effect of carbapenem instability both before and after sample collection could not be assessed and may have contributed to the low CSF and serum concentrations, even though samples were rapidly stored at −80°C to help minimize degradation. For example, imipenem tested in conjunction with cilastatin only remained 90% stable for 2 h at 37°C or 3 h at 25°C, demonstrating its unstable nature prior to sample freezing (Viaene et al., 2002). With storage at temperatures below −20°C, the data on carbapenem stability is inconclusive (Gravallese et al., 1984; Myers and Blumer, 1984; Garcia-Capdevila et al., 1997). Previous literature suggests that meropenem undergoes degradation, even at −20°C, so it is possible unintentional degradation may have occurred during our sample collection, storage, or shipping processes (Gijsen et al., 2021). In addition to these limitations, CSF to serum ratios and PK/PD targets that can improve clinical outcomes in patients with TBM were not ascertained in this study. While these targets are not known, nor their effects on clinical outcomes, it is promising that 21/22 (95%) of participants in our study were able to complete therapy and only 1/22 (5%) had treatment failure. Also, the impact of co-administered medications, renal function, and hepatic function on drug concentrations was not assessed. Finally, the protein content and drug protein binding in CSF are not well established and the assumption of 0% protein binding in the CSF is based on limited data (Bonati et al., 1982; Nau et al., 2010).
In summary, we described fluoroquinolone and carbapenem penetration into the CSF in a cohort of patients treated for TBM. Our study is unique in that serial CSF concentrations were collected at 2 and 6 h over the first few months of TBM treatment, allowing for assessment of delayed penetration. We found that fluoroquinolones showed CSF penetration peaking after serum concentrations, potentially increasing over the duration of treatment. Carbapenems had lower than expected CSF concentrations, with the majority of samples undetectable or BLQ in the CSF while detectable in serum. These findings warrant further exploration with techniques such as cerebral microdialysis to see how carbapenem brain tissue concentrations compare to plasma and CSF concentrations.
Data availability statement
The raw data supporting the conclusions of this article will be made available by the authors, without undue reservation.
Ethics statement
The studies involving human participants were reviewed and approved by National Center for Tuberculosis and Lung Disease, University of Florida, Emory University. The patients/participants provided their written informed consent to participate in this study.
Author contributions
NM performed statistical analysis and led manuscript writing. MA helped with data interpretation and table/figure generation. AS managed/cleaned the dataset prior to analysis. TA and MG participated in data collection and cleaning. TB and SS provided direct care for patients with TB at the National Center for Tuberculosis and Lung Diseases. ZA assisted with study implementation. MK led project implementation and assisted with data interpretation and logistics. CP oversaw the effort to perform drug concentrations for study patients. RK designed the study, provided input for data collection, and contributed to manuscript writing. All authors reviewed manuscript drafts.
Funding
This work was supported in part by the National Institutes of Health (NIH) including the Fogarty International Center (D43 TW007124) and National Institute of Allergy and Infectious Diseases (R03 AI139871, K23AI103044, TL1TR002382, UL1TR002378, and P30AI168386).
Acknowledgments
The authors are thankful for study participants with tuberculosis meningitis who were willing to participate in the study to contribute meaningful data that may help future patients with the same illness.
Conflict of interest
The authors declare that the research was conducted in the absence of any commercial or financial relationships that could be construed as a potential conflict of interest.
Publisher’s note
All claims expressed in this article are solely those of the authors and do not necessarily represent those of their affiliated organizations, or those of the publisher, the editors and the reviewers. Any product that may be evaluated in this article, or claim that may be made by its manufacturer, is not guaranteed or endorsed by the publisher.
References
Akahane, K., Sekiguchi, M., Une, T., and Osada, Y. (1989). Structure-epileptogenicity relationship of quinolones with special reference to their interaction with gamma-aminobutyric acid receptor sites. Antimicrob. Agents Chemother. 33 (10), 1704–1708. doi:10.1128/AAC.33.10.1704
Alsultan, A., and Peloquin, C. A. (2014). Therapeutic drug monitoring in the treatment of tuberculosis: An update. Drugs 74 (8), 839–854. doi:10.1007/s40265-014-0222-8
Andes, D. R., and Craig, W. A. (1999). Pharmacokinetics and pharmacodynamics of antibiotics in meningitis. Infect. Dis. Clin. North Am. 13 (3), 595–618. doi:10.1016/s0891-5520(05)70096-9
Angeby, K. A., Jureen, P., Giske, C. G., Chryssanthou, E., SturEgard, E., NordvallM., , et al. (2010). Wild-type MIC distributions of four fluoroquinolones active against Mycobacterium tuberculosis in relation to current critical concentrations and available pharmacokinetic and pharmacodynamic data. J. Antimicrob. Chemother. 65 (5), 946–952. doi:10.1093/jac/dkq091
Bartzatt, R. (2011). Tuberculosis infections of the central nervous system. Cent. Nerv. Syst. Agents Med. Chem. 11 (4), 321–327. doi:10.2174/1871524911106040321
Berning, S. E. (2001). The role of fluoroquinolones in tuberculosis today. Drugs 61 (1), 9–18. doi:10.2165/00003495-200161010-00002
Bolon, M. K. (2009). The newer fluoroquinolones. Infect. Dis. Clin. North Am. 23 (4), 1027–1051. x. doi:10.1016/j.idc.2009.06.003
Bonati, M., Kanto, J., and Tognoni, G. (1982). Clinical pharmacokinetics of cerebrospinal fluid. Clin. Pharmacokinet. 7 (4), 312–335. doi:10.2165/00003088-198207040-00003
Brunner, M., Langer, O., Dobrozemsky, G., Muller, U., Zeitlinger, M., Mitterhauser, M., et al. (2004). [18F]Ciprofloxacin, a new positron emission tomography tracer for noninvasive assessment of the tissue distribution and pharmacokinetics of ciprofloxacin in humans. Antimicrob. Agents Chemother. 48 (10), 3850–3857. doi:10.1128/AAC.48.10.3850-3857.2004
Chambers, H. F., Moreau, D., Yajko, D., MiiCk, C., Wagner, C., HaCkbarth, C., et al. (1995). Can penicillins and other beta-lactam antibiotics be used to treat tuberculosis? Antimicrob. Agents Chemother. 39 (12), 2620–2624. doi:10.1128/AAC.39.12.2620
Christensen, A. S. H., Roed, C., Omland, L. H., Andersen, P. H., Obel, N., and Andersen, Å. B. (2011). Long-term mortality in patients with tuberculous meningitis: A Danish nationwide cohort study. PLoS One 6 (11), e27900. doi:10.1371/journal.pone.0027900
Craig, W. A. (1997). The pharmacology of meropenem, a new carbapenem antibiotic. Clin. Infect. Dis. 24, S266–S275. doi:10.1093/clinids/24.supplement_2.s266
de Lange, E. C. M. (2013). Utility of CSF in translational neuroscience. J. Pharmacokinet. Pharmacodyn. 40 (3), 315–326. doi:10.1007/s10928-013-9301-9
Dheda, K., Gumbo, T., Maartens, G., Dooley, K. E., McNerney, R., Murray, M., et al. (2017). The epidemiology, pathogenesis, transmission, diagnosis, and management of multidrug-resistant, extensively drug-resistant, and incurable tuberculosis. Lancet Respir. Med. 15S2213-2600 (17), 291–360. doi:10.1016/S2213-2600(17)30079-6
Domagala, J. M. (1994). Structure-activity and structure-side-effect relationships for the quinolone antibacterials. J. Antimicrob. Chemother. 33 (4), 685–706. doi:10.1093/jac/33.4.685
Donald, P. R. (2010). Cerebrospinal fluid concentrations of antituberculosis agents in adults and children. Tuberculosis 90 (5), 279–292. doi:10.1016/j.tube.2010.07.002
Garcia-Capdevila, L., López-Calull, C., Arroyo, C., Moral, M. A., Mangues, M. A., and Bonal, J. (1997). Determination of imipenem in plasma by high-performance liquid chromatography for pharmacokinetic studies in patients. J. Chromatogr. B Biomed. Sci. Appl. 692 (1), 127–132. doi:10.1016/s0378-4347(96)00498-7
Ghimire, S., Maharjan, B., Jongedijk, E. M., Kosterink, J. G. W., Ghimire, G. R., Touw, D. J., et al. (2019). Levofloxacin pharmacokinetics, pharmacodynamics and outcome in multidrug-resistant tuberculosis patients. Eur. Respir. J. 53 (4), 1802107. doi:10.1183/13993003.02107-2018
Gijsen, M., Filtjens, B., Annaert, P., Armoudjian, Y., Debaveye, Y., Wauters, J., et al. (2021). Meropenem stability in human plasma at -20 °C: Detailed assessment of degradation. Antibiot. (Basel) 10 (4), 449. doi:10.3390/antibiotics10040449
Gravallese, D. A., Musson, D. G., Pauliukonis, L. T., and Bayne, W. F. (1984). Determination of imipenem (N-formimidoyl thienamycin) in human plasma and urine by high-performance liquid chromatography, comparison with microbiological methodology and stability. J. Chromatogr. 310 (1), 71–84. doi:10.1016/0378-4347(84)80069-9
Heemskerk, A. D., Bang, N. D., Mai, N. T. H., Chau, T. T. H., Phu, N. H., Loc, P. P., et al. (2016). Intensified antituberculosis therapy in adults with tuberculous meningitis. N. Engl. J. Med. 374 (2), 124–134. doi:10.1056/NEJMoa1507062
Hikida, M., Masukawa, Y., Nishiki, K., and Inomata, N. (1993). Low neurotoxicity of LJC 10, 627, a novel 1 beta-methyl carbapenem antibiotic: Inhibition of gamma-aminobutyric acidA, benzodiazepine, and glycine receptor binding in relation to lack of central nervous system toxicity in rats. Antimicrob. Agents Chemother. 37 (2), 199–202. doi:10.1128/AAC.37.2.199
Hosmann, A., Ritscher, L., Burgmann, H., Al Jalali, V., Wulkersdorfer, B., Wolfl-Duchek, M., et al. (2021). Meropenem concentrations in brain tissue of neurointensive care patients exceed CSF levels. J. Antimicrob. Chemother. 76 (11), 2914–2922. doi:10.1093/jac/dkab286
Hugonnet, J. E., Tremblay, L. W., Boshoff, H. I., Barry, C. E., and Blanchard, J. S. (2009). Meropenem-clavulanate is effective against extensively drug-resistant Mycobacterium tuberculosis. Science 323 (5918), 1215–1218. doi:10.1126/science.1167498
Huynh, J., Donovan, J., Phu, N. H., Nghia, H. D. T., Thuong, N. T. T., and Thwaites, G. E. (2022). Tuberculous meningitis: Progress and remaining questions. Lancet. Neurol. 21 (5), 450–464. doi:10.1016/S1474-4422(21)00435-X
Jaganath, D., Lamichhane, G., and Shah, M. (2016). Carbapenems against Mycobacterium tuberculosis: A review of the evidence. Int. J. Tuberc. Lung Dis. 20 (11), 1436–1447. doi:10.5588/ijtld.16.0498
Janssen Pharmaceuticals, Inc (1996). Levaquin [package insert]. Titusville, NJ: Janssen Pharmaceuticals, Inc.
Kalita, J., Bhoi, S. K., Betai, S., and Misra, U. K. (2016). Safety and efficacy of additional levofloxacin in tuberculous meningitis: A randomized controlled pilot study. Tuberc. (Edinb) 98, 1–6. doi:10.1016/j.tube.2016.01.004
Kaushik, A., Makkar, N., Pandey, P., Parrish, N., Singh, U., and Lamichhane, G. (2015). Carbapenems and rifampin exhibit synergy against Mycobacterium tuberculosis and Mycobacterium abscessus. Antimicrob. Agents Chemother. 59 (10), 6561–6567. doi:10.1128/AAC.01158-15
Kempker, R. R., Smith, A. G. C., Avaliani, T., Gujabidze, M., Bakuradze, T., Sabanadze, S., et al. (2022). Cycloserine and linezolid for tuberculosis meningitis: Pharmacokinetic evidence of potential usefulness. Clin. Infect. Dis. 29, 682ciab992–689. doi:10.1093/cid/ciab992
Kushner, J. M., Peckman, H. J., and Snyder, C. R. (2001). Seizures associated with fluoroquinolones. Ann. Pharmacother. 35 (10), 1194–1198. doi:10.1345/aph.10359
Lamp, K. C., Bailey, E. M., and Rybak, M. J. (1992). Ofloxacin clinical pharmacokinetics. Clin. Pharmacokinet. 22 (1), 32–46. doi:10.2165/00003088-199222010-00004
Levison, M. E., and Levison, J. H. (2009). Pharmacokinetics and pharmacodynamics of antibacterial agents. Infect. Dis. Clin. North Am. 23 (4), 791–815. vii. doi:10.1016/j.idc.2009.06.008
Litjens, C. H. C., Aarnoutse, R. E., and Te Brake, L. H. M. (2020). Preclinical models to optimize treatment of tuberculous meningitis - a systematic review. Tuberculosis 122, 101924. doi:10.1016/j.tube.2020.101924
Mehlhorn, A. J., and Brown, D. A. (2007). Safety concerns with fluoroquinolones. Ann. Pharmacother. 41 (11), 1859–1866. doi:10.1345/aph.1K347
Myers, C. M., and Blumer, J. L. (1984). Determination of imipenem and cilastatin in serum by high-pressure liquid chromatography. Antimicrob. Agents Chemother. 26 (1), 78–81. doi:10.1128/AAC.26.1.78
Nau, R., Kinzig, M., Dreyhaupt, T., Kolenda, H., Sörgel, F., and Prange, H. W. (1994). Kinetics of ofloxacin and its metabolites in cerebrospinal fluid after a single intravenous infusion of 400 milligrams of ofloxacin. Antimicrob. Agents Chemother. 38 (8), 1849–1853. doi:10.1128/AAC.38.8.1849
Nau, R., Sörgel, F., and Eiffert, H. (2010). Penetration of drugs through the blood-cerebrospinal fluid/blood-brain barrier for treatment of central nervous system infections. Clin. Microbiol. Rev. 23 (4), 858–883. doi:10.1128/CMR.00007-10
Nijland, H. M. J., Ruslami, R., Suroto, A. J., Burger, D. M., AlisjahBana, B., van Crevel, R., et al. (2007). Rifampicin reduces plasma concentrations of moxifloxacin in patients with tuberculosis. Clin. Infect. Dis. 45 (8), 1001–1007. doi:10.1086/521894
Norrby, S. R. (1996). Neurotoxicity of carbapenem antibacterials. Drug Saf. 15 (2), 87–90. doi:10.2165/00002018-199615020-00001
Ortho-McNeil-Janssen Pharmaceuticals, Inc (2008). Ofloxacin [package insert]. Raritan, NJ: Ortho-McNeil-Janssen Pharmaceuticals, Inc.
Owens, R. C., and Ambrose, P. G. (2005). Antimicrobial safety: Focus on fluoroquinolones. Clin. Infect. Dis. 41, S144–S157. doi:10.1086/428055
Pickerill, K. E., Paladino, J. A., and Schentag, J. J. (2000). Comparison of the fluoroquinolones based on pharmacokinetic and pharmacodynamic parameters. Pharmacotherapy 20 (4), 417–428. doi:10.1592/phco.20.5.417.35062
Ramachandran, G., Hemanth Kumar, A. K., Srinivasan, R., GeethArAni, A., Sugirda, P., Nandhakumar, B., et al. (2012). Effect of rifampicin & isoniazid on the steady state pharmacokinetics of moxifloxacin. Indian J. Med. Res. 136 (6), 979–984.
Roos, K. L. (2000). Mycobacterium tuberculosis meningitis and other etiologies of the aseptic meningitis syndrome. Semin. Neurol. 20 (3), 329–335. doi:10.1055/s-2000-9428
Salmon-Rousseau, A., Martins, C., Blot, M., Mahy, S., Chavanet, P., Piroth, L., et al. (2020). Comparative review of imipenem/cilastatin versus meropenem. Med. Mal. Infect. 50 (4), 316–322. doi:10.1016/j.medmal.2020.01.001
Sharma, S. K., Mohan, A., and Kohli, M. (2021). Extrapulmonary tuberculosis. Expert Rev. Respir. Med. 15 (7), 931–948. doi:10.1080/17476348.2021.1927718
Smith, A. G. C., Gujabidze, M., Avaliani, T., Blumberg, H. M., Collins, J. M., Sabanadze, S., et al. (2021). Clinical outcomes among patients with tuberculous meningitis receiving intensified treatment regimens. Int. J. Tuberc. Lung Dis. 25 (8), 632–639. doi:10.5588/ijtld.21.0159
Sunagawa, M., Matsumura, H., Sumita, Y., and Nouda, H. (1995). Structural features resulting in convulsive activity of carbapenem compounds: Effect of C-2 side chain. J. Antibiot. 48 (5), 408–416. doi:10.7164/antibiotics.48.408
Thea, D., and Barza, M. (1989). Use of antibacterial agents in infections of the central nervous system. Infect. Dis. Clin. North Am. 3 (3), 553–570. doi:10.1016/s0891-5520(20)30289-0
Thwaites, G. E., van Toorn, R., and Schoeman, J. (2013). Tuberculous meningitis: More questions, still too few answers. Lancet. Neurol. 12 (10), 999–1010. doi:10.1016/S1474-4422(13)70168-6
Tucker, E. W., Guglieri-Lopez, B., Ordonez, A. A., Ritchie, B., Klunk, M. H., Sharma, R., et al. (2018). Noninvasive 11C-rifampin positron emission tomography reveals drug biodistribution in tuberculous meningitis. Sci. Transl. Med. 10 (470), eaau0965. doi:10.1126/scitranslmed.aau0965
Turnidge, J. (1999). Pharmacokinetics and pharmacodynamics of fluoroquinolones. Drugs 58, 29–36. doi:10.2165/00003495-199958002-00006
van Rijn, S. P., Zuur, M. A., Anthony, R., Wilffert, B., van Altena, R., Akkerman, O. W., et al. (2019). Evaluation of carbapenems for treatment of multi- and extensively drug-resistant Mycobacterium tuberculosis. Antimicrob. Agents Chemother. 63 (2), E01489-18. doi:10.1128/AAC.01489-18
Veziris, N., Truffot, C., Mainardi, J. L., and Jarlier, V. (2011). Activity of carbapenems combined with clavulanate against murine tuberculosis. Antimicrob. Agents Chemother. 55 (6), 2597–2600. doi:10.1128/AAC.01824-10
Viaene, E., Chanteux, H., Servais, H., Mingeot-Leclercq, M. P., and Tulkens, P. M. (2002). Comparative stability studies of antipseudomonal beta-lactams for potential administration through portable elastomeric pumps (home therapy for cystic fibrosis patients) and motor-operated syringes (intensive care units). Antimicrob. Agents Chemother. 46 (8), 2327–2332. doi:10.1128/AAC.46.8.2327-2332.2002
Walton, G. D., Hon, J. K., and Mulpur, T. G. (1997). Ofloxacin-induced seizure. Ann. Pharmacother. 31 (12), 1475–1477. doi:10.1177/106002809703101206
Wilkinson, R. J., Rohlwink, U., Misra, U. K., van Crevel, R., Mai, N. T. H., Dooley, K. E., et al. (2017). Tuberculous meningitis. Nat. Rev. Neurol. 13 (10), 581–598. doi:10.1038/nrneurol.2017.120
Wong, V. K., Wright, H. T., Ross, L. A., Mason, W. H., Inderlied, C. B., and Kim, K. S. (1991). Imipenem/cilastatin treatment of bacterial meningitis in children. Pediatr. Infect. Dis. J. 10 (2), 122–125. doi:10.1097/00006454-199102000-00009
World Health Organization (2021). Global tuberculosis report 2021. Geneva: World Health Organization. (Accessed June 29, 2022).
Keywords: tuberculosis meningitis, fluoroquinolones, carbapenems, pharmacokinetics, cerebrospinal fluid, antitubercular agents
Citation: Maranchick NF, Alshaer MH, Smith AGC, Avaliani T, Gujabidze M, Bakuradze T, Sabanadze S, Avaliani Z, Kipiani M, Peloquin CA and Kempker RR (2022) Cerebrospinal fluid concentrations of fluoroquinolones and carbapenems in tuberculosis meningitis. Front. Pharmacol. 13:1048653. doi: 10.3389/fphar.2022.1048653
Received: 19 September 2022; Accepted: 01 December 2022;
Published: 12 December 2022.
Edited by:
Shashikant Srivastava, University of Texas at Tyler, United StatesReviewed by:
Dipti Agarwal, Dr Ram Manohar Lohia Institute of Medical Sciences, IndiaHung-Ling Huang, Kaohsiung Medical University Hospital, Taiwan
Copyright © 2022 Maranchick, Alshaer, Smith, Avaliani, Gujabidze, Bakuradze, Sabanadze, Avaliani, Kipiani, Peloquin and Kempker. This is an open-access article distributed under the terms of the Creative Commons Attribution License (CC BY). The use, distribution or reproduction in other forums is permitted, provided the original author(s) and the copyright owner(s) are credited and that the original publication in this journal is cited, in accordance with accepted academic practice. No use, distribution or reproduction is permitted which does not comply with these terms.
*Correspondence: Russell R. Kempker, cmtlbXBrZUBlbW9yeS5lZHU=