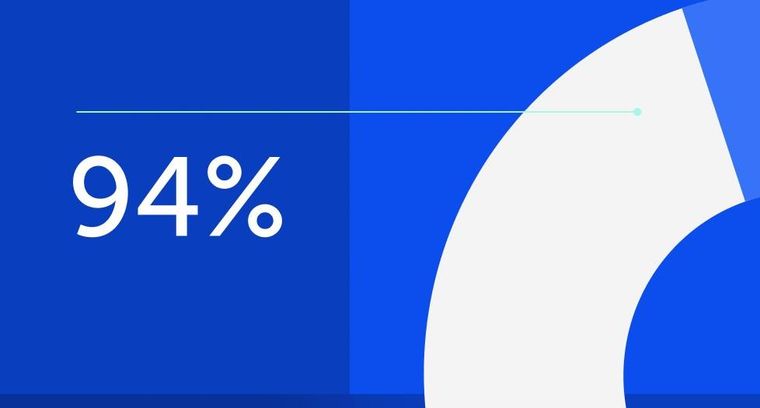
94% of researchers rate our articles as excellent or good
Learn more about the work of our research integrity team to safeguard the quality of each article we publish.
Find out more
REVIEW article
Front. Pharmacol., 29 November 2022
Sec. Cardiovascular and Smooth Muscle Pharmacology
Volume 13 - 2022 | https://doi.org/10.3389/fphar.2022.1045501
This article is part of the Research TopicCardiovascular RemodelingView all 17 articles
The prevalence and mortality rates of cardiovascular diseases are increasing, and new treatment strategies are urgently needed. From the perspective of basic pathogenesis, the occurrence and development of cardiovascular diseases are related to inflammation, apoptosis, fibrosis and autophagy of cardiomyocytes, endothelial cells and other related cells. The involvement of maternally expressed gene 3 (MEG3) in human disease processes has been increasingly reported. P53 and PI3K/Akt are important pathways by which MEG3 participates in regulating cell apoptosis. MEG3 directly or competitively binds with miRNA to participate in apoptosis, inflammation, oxidative stress, endoplasmic reticulum stress, EMT and other processes. LncRNA MEG3 is mainly involved in malignant tumors, metabolic diseases, immune system diseases, cardiovascular and cerebrovascular diseases, etc., LncRNA MEG3 has a variety of pathological effects in cardiomyocytes, fibroblasts and endothelial cells and has great clinical application potential in the prevention and treatment of AS, MIRI, hypertension and HF. This paper will review the research progress of MEG3 in the aspects of mechanism of action, other systemic diseases and cardiovascular diseases, and point out its great potential in the prevention and treatment of cardiovascular diseases. lncRNAs also play a role in endothelial cells. In addition, lncRNA MEG3 has shown biomarker value, prognostic value and therapeutic response measurement in tumor diseases. We boldly speculate that MEG3 will play a role in the emerging discipline of tumor heart disease.
The prevalence and mortality rates of cardiovascular diseases are on the rise, with an estimated 19 million people worldwide dying from cardiovascular diseases in 2020, an increase of 18.7% from 2010 (Tsao et al., 2022). 80% of deaths are related to CVD (Global, 2017). By 2030, 23.6 million people are expected to die from cardiovascular disease, including heart disease and stroke (Dehghan et al., 2017), (Yusuf et al., 2014). New treatment strategies are urgently needed. From the perspective of basic pathogenesis, the occurrence and development of cardiovascular diseases are related to inflammation, apoptosis, fibrosis and autophagy of cardiomyocytes, endothelial cells and other related cells (Hou et al., 2014; Zhang et al., 2019a; Lan et al., 2019; Shihabudeen Haider Ali et al., 2019). In recent years, precision medicine has been gradually applied to the cardiovascular field along with the development of gene medicine. Currently, the following diseases are considered: common cardiovascular diseases, such as hypertension (Sun et al., 2017a; Padmanabhan and Joe, 2017; Savoia et al., 2017); diagnosis and treatment of uncertain diseases, such as angina and coronary artery disease (Ladapo et al., 2017; Niccoli et al., 2017); high-mortality diseases and/or interventions are complex and expensive, such as dilated cardiomyopathy and cardiac resynchronization therapy (Halliday et al., 2017). It is worth emphasizing that these methods involve new molecular and genetic diagnostic methods (Padmanabhan and Joe, 2017; Savoia et al., 2017).
Maternally expressed gene 3 (MEG3) is an imprinted gene with maternal expression that encodes a noncoding RNA with a length of −1,600 nt (Tian et al., 2015) located at chromosome 14q32 (Zhang et al., 2010). The involvement in human disease processes is increasingly reported. For example, MEG3 functions as a competing endogenous RNA to regulate cancer progression, reduces mitochondrial-derived apoptosis (Wang et al., 2021a), participates in the regulation of tumor drug resistance (Yu et al., 2020), participates in glaucoma onset (Sun et al., 2018), etc., This noncoding RNA is not only involved in the pathogenesis of many diseases but also found in an increasing number of mechanisms. For instance, it participates in cell migration and proliferation, promotes cell apoptosis, inhibits cell autophagy activity, and inhibits inflammatory factors (Li et al., 2017; Han et al., 2020; Yang et al., 2022). Cardiovascular disease researchers are increasingly interested in MEG3. Hongchun Wu’s group reported that MEG3 exists in mouse myocardial cells and myocardial fibroblasts and mainly plays a role in the myocardial nucleus (Wu et al., 2018). Thum’s team reported that MEG3 plays a key role in promoting cardiac fibroblast fibrosis (Piccoli et al., 2017). The inhibition of Meg3 prevents cardiac fibrosis and diastolic dysfunction (Piccoli et al., 2017), and the downregulation of MEG3 protects myocardial cells against I/R-induced apoptosis through the miR-7-5p/PARP1 pathway (Zou et al., 2019) Chao He et al. found in their study that MEG3, as a ceRNA, inhibits miR-9, affects the phenotype of MEG3-mediated vascular endothelial cells (He et al., 2017).
Currently, studies on lncRNA MEG3 in cardiovascular diseases are relatively rare, and there is still a large space for exploration. This paper will review the research progress of MEG3 in the functional mechanism, other systemic diseases and cardiovascular diseases and find its great potential in the prevention and treatment of cardiovascular diseases.
This paper focuses on the role of lncRNA MEG3 in cardiovascular disease. Although so far, there have been few studies on the effects of lncMEG3 on cardiovascular diseases, most of which focus on tumor diseases, from the perspective of functional mechanism, the regulatory mechanisms involved in MEG3 are also common in cardiovascular diseases, suggesting that MEG3 may be involved in the occurrence and development of cardiovascular diseases through these pathways. Therefore, the second part of this paper reviews MEG3 from the functional mechanism.
The p53 protein is a transcription factor that activates cell cycle arrest, DNA repair and apoptosis in response to stress and DNA damage (Kruiswijk et al., 2015). The 27 known splicing variants of MEG3 contain variable intermediate exons with common exons entraining at the 50 (E1-E3) and 30 (E10-E12) ends, and their ability to stimulate the p53 pathway varies (Yu et al., 2020). Based on this, MEG3 can regulate cell physiology by regulating p53 and downstream signaling pathways and play a role in a variety of diseases. In vascular endothelial cells, the p53 signaling pathway is the most significantly regulated pathway after MEG3 knockout. Downregulation of MEG3 leads to phosphorylation of p53 at serine 15 and weakens its binding with multiple p53 target gene promoters, such as MDM2, resulting in accumulation of p53. Meanwhile, MEG3 loss leads to high expression of P21, induces p53 cell cycle arrest, reduces cell proliferation, promotes cell apoptosis, and aggravates cardiac dysfunction after pressure overload in mice. Early studies have shown that the loss of p53 in the vascular endothelium can reduce apoptosis of endothelial cells and protect cardiac dysfunction after pressure overload in mice (Deng et al., 1995; Gartel and Radhakrishnan, 2005; Gogiraju et al., 2015; Shihabudeen Haider Ali et al., 2019). In tumor cells, lncRNA MEG3 inhibits the proliferation and metastasis of breast, gastric, liver and pancreatic cancer cells by activating the p53 signaling pathway (Chen et al., 2016; Hu et al., 2016; Wei and Wang, 2017; Huang et al., 2022). Especially in hepatocellular carcinoma cells, MEG3 activates the NF-κB signaling pathway and then activates the p53 pathway and upregulates the expression of the ER stress-related protein GRP78, resulting in ER stress (Chen et al., 2016). Xueling Li’s group found that lncMEG3 was downregulated around the infarcted myocardial tissue, which directly acted on p53 and reduced its expression level. Meanwhile, the expression of ERS-related protein GRP78 decreased, which proved that lncRNA MEG3 in myocardial cells was awaiting ERS-mediated apoptosis through the p53 pathway (Li et al., 2019). On the other hand, inhibition of endogenous Meg3 in mouse fibroblasts reduced the expression of Mmp-2 at promoters by inhibiting p53 binding but had no effect on cell apoptosis or proliferation (Piccoli et al., 2017). Western blotting was used to detect the proteins in the pathway, and it was found that p53 was regulated by MeG3, which then regulated the apoptosis of adipose stem cells (adScs) through the Bcl-2/Bax pathway (Shi, 2020). In mouse neurons, overexpression of Meg3 induces p53 and enhances its transcriptional activity, leading to increased cell death (Yan et al., 2016) (Figure 1).
FIGURE 1. lncRNA MEG3 is frequently involved in endoplasmic reticulum stress, apoptosis, inflammation and epithelial-mesenchymal transformation via the P53 and PI3K/AKT pathways. The common downstream pathways are MDM2, NF-κB, Bcl-2/Bax, mTOR and other protein pathways. Regulation is also accomplished by competitively binding downstream protein pathways with miRNA.
The phosphatidylinositol 3-kinase (PI3K)/protein kinase B (PKB/AKT) signaling pathway is one of the core signaling pathways involved in regulating cell growth, proliferation, apoptosis and other processes (Yang et al., 2019a). Studies have shown that inhibition of the PI3K/AKT signaling pathway can inhibit cell proliferation and promote cell apoptosis (Okano et al., 2000; Oyama et al., 2007; Feng et al., 2016). LncRNA MEG3 has a negative regulatory effect on the PI3K/Akt signaling pathway. LncRNA MEG3 expression was significantly increased in the temporal cortex of rats with subarachnoid hemorrhage and in the cerebrospinal fluid of patients with subarachnoid hemorrhage, while the expression of the PI3K/Akt signaling pathway was decreased, which promoted neuronal apoptosis (Liang et al., 2018). At the same time, the upregulation of MEG3 can improve cognitive dysfunction by inhibiting the PI3K/Akt signaling pathway and then inhibiting the activation of hippocampal astrocytes (Yi et al., 2019). MEG3 overexpression of the inactivated PI3K/Akt signaling pathway can inhibit the growth of cervical cancer HeLa cells and breast cancer cells, thus achieving anticancer effects (Wang et al., 2017; Zhu et al., 2019).
PI3K/AKT can play a regulatory role alone and crosstalk with the mTOR signaling pathway to regulate various pathological processes (Rosenbloom et al., 2013). It is through this signaling axis that MEG3 inhibits the TNF-α-induced inflammatory response (Tang et al., 2021). In endometrial cancer cells, MEG3 regulates the PI3K/m-TOR signaling pathway to regulate cell cycle progression, thus regulating apoptosis (Sun et al., 2017b). MEG3 enhances adenosine-induced hepatocellular carcinoma cytotoxicity by downregulating ILF3 and activating this signaling axis (Pu et al., 2019). MEG3 also plays a positive role as a potential target for the treatment of epilepsy by activating this pathway to reduce proinflammatory factors, oxidative stress and apoptosis of hippocampal neurons in rats (Zhang et al., 2020). In rat and cell models of diabetic retinopathy, overexpression of MEG3 inhibits endothelium-stromal transformation by inhibiting the PI3K/Akt/mTOR signaling pathway (He et al., 2021).
The tumor suppressor PTEN, a central negative regulator of the PI3K/Akt signaling pathway, is often mutated in cancer and loses its tumor suppressor function (Leslie, 2012; Wang et al., 2018). It has been reported that both MEG3 and PTEN are downregulated in ovarian cancer cells, which promotes tumor cell proliferation and inhibits cell apoptosis. LncRNA MEG3 regulates PTEN/PI3K/AKT to play an anti-proliferation role in hemangioma cells and testicular germ cell tumor cells through sponge adsorption of miR-494 and miR-1297, respectively.
Experiments have proven that upregulation of MEG3 can sponge miR-27a-3p, upregulate IGF1 and activate the PI3K/Akt signaling pathway, thus promoting osteogenic differentiation (Liu et al., 2019a) (Figure 1).
Apoptosis is a programmed cell death that occurs after many stimuli, infections or injuries and plays a key role in normal physiological processes such as embryogenesis and adult tissue homeostasis (Morana et al., 2022). With the advancement of research on MEG3, it has been found that it has an obvious regulatory effect on the molecular mechanism of cell apoptosis, thus participating in a variety of diseases.
MEG3 down-regulation can regulate FOXO1 and FOXO4 to promote apoptosis through competitive binding of miR-361-5P and miR-23b-3p respectively (Tsao et al., 2022), (Wang et al., 2019a), (Wang et al., 2021b). H2O2 induces oxidative stress in adipose stem cells (adScs), thus increasing the apoptosis rate of adScs. MeG3 silencing can reduce H2O2-induced apoptosis, while MeG3 overexpression can aggravate apoptosis (Shi, 2020). MEG3 recovery can reduce β-catenin and cyclin D1 in melanoma cells, improve GSK-3 β levels in vitro, block Wnt signaling activity, inhibit cell proliferation, migration and invasion, and trigger cell apoptosis (Li et al., 2018a).
MEG3 functions as a ceRNA to regulate apoptosis by sponging different miRNAs (Moradi et al., 2019). Activation of Sema3A, a member of the Sema family, may disregulate a series of apoptosis-related regulatory factors, thus promoting apoptosis (Vadasz and Toubi, 2014; Cheng et al., 2019). MEG3 inhibition significantly increased the expression of miR-424-5p, decreased the expression of Sema3A, increased cell viability, and reduced cell apoptosis (Xiang et al., 2020). As an endogenous sponge, MEG3 inhibits miR-223 function, increases NLRP3 expression and promotes endothelial cell apoptosis through sequence complementation (Zhang et al., 2018). Overexpression of MEG3 inhibits miR-141-3p, activates RNA binding motif single stranded protein 3 (RBMS3), and promotes apoptosis of breast cancer cells (Dong et al., 2021a). Overexpression of MEG3 and miR-376B-3p inhibits the tumogenesis of PDFS cells and promotes cell apoptosis (Zhu et al., 2020). In the In vitro AP model, MEG3 promotes cell apoptosis through the MEG3/miR-195-5p/FGFR2 signaling axis (Chen and Song, 2021). MEG3 has been reported to regulate miR-21, target Caspase-8 to regulate proliferation and apoptosis of psoriatic epidermal cells, and inactivate the PI3K/Akt pathway to promote apoptosis in breast cancer cells (Jia et al., 2019; Zhu et al., 2019). Mir-361–5p. In the research of Shen B’s group, it is suggested that MEG3 regulates miR-361–5p and promotes cell apoptosis (Shen et al., 2019). In laryngeal cancer cells, overexpressed MEG3 specifically binds and negatively regulates miR-23a, activates apoptotic protease activator factor-1 (APAF-1), and thus activates Caspase-9 and Caspase-3, leading to apoptosis (Zhang et al., 2019b). MEG3 overexpression leads to reduced apoptosis of intestinal ganglion cells and increased cell viability, and the upregulation of the MEG3 downstream gene miR-211-5p will counteract this effect. Further studies found that the increased expression of GDNF reversed the upregulation effect of miR-211-5p. In summary, hypoxia induced intestinal ganglion cell apoptosis through the MEG3/miR-211-5p/GDNF axis (Xia et al., 2019). MEG3 was found to be highly expressed in the blood and placental villus tissues of gestational diabetes mellitus (GDM). MEG3 knockdown significantly increased the viability of human chorionic trophoblast HTR-8/SVneo cells and reduced cell apoptosis. Inhibition of miR-345-3p negates all observed physiological effects of MEG3 downregulation on HTR-8/SVneo cells (Zhang, 2019). In HCC cells, MEG3 overexpression can sponge out miR-9-5p and upregulate the expression of SOX11, and then observe apoptosis-related changes of Bcl-2 and caspase-3, suggesting that MEG3 can promote the apoptosis of HCC cells (Liu et al., 2019b). For thyroid cancer cells resistant to 131I, low MEG3 expression is not good news. Downregulated MEG3 can promote cell apoptosis by upregulating miR-182 expression and reducing the therapeutic effect of 131I in TC cells (Liu et al., 2018). miR-205-5p has been identified as the downstream gene of MEG3 and is negatively regulated by MEG3, promoting cell apoptosis (Tao et al., 2020). MEG3 inhibition negatively regulates miR-145-5p, resulting in a decreased apoptosis ability of macrophages (Sun et al., 2020). In summary, MEG3, as a ceRNA, mostly has a negative regulatory effect on miRNA and then positively regulates cell apoptosis through the downstream signaling pathway of miRNA.
MEG3 inhibition significantly increases the expression of miR-424-5p, reduces the expression of Sema3A, increases cell viability, reduces cell apoptosis, inhibits the function of miR-223, and increases the expression of NLRP3. Overexpression of MEG3 can inhibit miR-141-3p, activate RBMS3, and promote cell apoptosis. MEG3 promotes cell apoptosis through the MEG3/miR-195-5p/FGFR2 signaling axis. MEG3 can regulate miR-21, target Caspase-8 to regulate cell proliferation and apoptosis, and inactivate the PI3K/Akt pathway to promote cell apoptosis. Bcl-2 overexpression was detected in the siRNA-MEG3 and miR-361–5P inhibitor groups. MEG3 regulates miR-361–5p and promotes apoptosis. MEG3 overexpression and negative regulation of miR-23a activate APAF-1, thereby activating caspase-9 and caspase-3, leading to cell apoptosis. MEG3 gene knockout significantly increased the viability of HTR-8/SVneo cells and reduced cell apoptosis. MEG3 overexpression can sponge miR-9-5p and upregulate the expression of SOX11, and changes related to apoptosis of Bcl-2 and Caspase-3 were observed. Downregulation of MEG3 can promote cell apoptosis by upregulating the expression of miR-182 (Figure 2).
FIGURE 2. Downregulation of MEG3 can directly or through miR-361-5p mediate the downregulation of FOXO1, promote cell apoptosis, negatively regulate the expression level of miR-23b 3p, and negatively regulate the expression level of FOXO4 in the same family to promote cell apoptosis. MEG3 recovery can reduce β-catenin and CyclinD1, improve the level of GSK-3β in vitro culture, block the activity of the Wnt signaling pathway, and then inhibit cell proliferation, migration and invasion, triggering cell apoptosis.
Autophagy refers to the transport of intracellular components to lysosomal chambers for degradation and circulation. To date, autophagy has been defined as three types: macrophage, microphage and chaperone-mediated autophagy, which can be “body autophagy” with nonselective degradation of autophagosome content and “selective autophagy” with elimination of single cell components (Saftig et al., 2008; He and Klionsky, 2009; Galluzzi et al., 2017; Dikic and Elazar, 2018; Gohel et al., 2020). Autophagy is an important function for maintaining cell and body homeostasis, and the basal level of autophagy is important for maintaining normal cellular homeostasis. Therefore, strict regulation of autophagy to induce it when needed plays a crucial role in health and disease (Botti et al., 2006; Meijer and Codogno, 2006; Yorimitsu and Klionsky, 2007; Cheng, 2019). The regulation of LncRNA MEG3 on autophagy is mainly positive regulation, and this effect has been verified in a variety of diseases and multiple pathways. FOXO1 belongs to the forkhead box protein family, which can regulate a variety of biological characteristics including apoptosis, autophagy, proliferation (Kitamura et al., 2005; Kobayashi et al., 2012; Zhang et al., 2016; Li et al., 2021). Downregulation of MEG3 or FOXO1 can lead to decreased autophagy of INS-1 cells induced by high glucose.1During pulmonary fibrosis, downregulation of MEG3 activates the Hedgehog (Hh) signaling pathway and inhibits autophagy activity of A549 cells (Gao et al., 2022). Autophagy exists in tumor cells, and downregulation of MEG3 can partially inhibit autophagy in lung cancer cells and can also regulate ATG3 activity to inhibit autophagy in epithelial ovarian cancer cells. (Xia et al., 2018), (Xiu et al., 2017). MiR-7-5p was found to play an active role in autophagy in cardiomyocytes of patients with ventricular septal defects, the binding sequence of MEG3 was found in the 3′UTR of miR-7-5p, and the predicted binding site of miR-7-5p was also found in the 3′UTR of EGFR. Upregulated MEG3 reverses myocyte autophagy through the miR-7-5p/EGFR axis (Kitamura et al., 2005; Kobayashi et al., 2012; Zhang et al., 2016; Li et al., 2021). Low expression of lncRNA MEG3 upregulates miR-543 to regulate autophagy in the IDO signaling pathway (Wang et al., 2021c). continued downregulation of MEG3 leads to IFN-γ induction of autophagy in infected macrophages (Pawar et al., 2016). LncRNA MEG3 enhances TNF-α-induced autophagy by inhibiting the PI3K/AKT/mTOR signaling pathway while inhibiting autophagy through the Beclin-1 signaling pathway (Pu et al., 2019; Tang et al., 2021).
Inflammation is a double-edged sword with many functions that can be seen everywhere in the process of injury and the repair of disease. MEG3 regulates inflammation in a number of ways. KLF4, an important regulator of macrophage polarization, was decreased in M1 macrophages and significantly increased in M2 macrophages, and the expression of macrophage proinflammatory genes with KLF4 knockout was increased (Liao et al., 2011). Inhibition of MEG3 can regulate the secretion of microglia inflammatory cytokines by inhibiting M1 polarization and promoting M2 polarization through KLF4 (Li et al., 2020a). M2 macrophages themselves have certain anti-inflammatory activity. MEG3 can regulate the miR-20b-5p/CREB1 axis and induce M2 macrophage-derived extracellular vesicles (M2-EVs) to reduce the inflammatory response (Wang et al., 2021d). Upregulation of MEG3 in ulcerative colitis (UC) can enhance the protective effect of M2-macrophage-derived extracellular vesicles (M2-evs) against UC and reduce inflammation (Wang et al., 2021d). MEG3 upregulation in methylene blue (MB)-induced peripheral nerve axons alleviates pain and inflammation associated with obstructive sleep apnea by inhibiting P2X3 protein expression (Li et al., 2018b).
Inflammasomes are multimeric protein complexes that are assembled in the cytosol upon sensing pathogen-associated molecular patterns (PAMPs) and danger-associated molecular patterns (DAMPs) (Strowig et al., 2012). AIM2 is one of the key proteins that regulates the formation of the inflammasome. Overexpression of MEG3 regulates the action of AIM2 on caspase1 signaling to trigger an inflammatory response (Liang et al., 2020). After sensing multiple molecular patterns, the n-terminal pyrin fragment (PYD) of NLRP3 serves as a scaffold to nucleate apoptosis-associated spotted proteins and recruit caspase preproteins to the inflammasome (Cai et al., 2014; Lu et al., 2014). MEG3 increases NLRP3-induced inflammation by inhibiting miR-223 or miR-7A-5p (Zhang et al., 2018; Meng et al., 2021). Upregulated MEG3 increases the CXCL12/CXCR4/Rac1 axis and TLR4/NF-κB pathway by inhibiting miR130A-5P, aggravating neuroinflammation (Dong et al., 2021b). Under the condition that IL-1β induces chondrocytes to simulate OA, MEG3 regulates the miR-93/TGFBR2 axis to regulate the inflammatory response and delay the OA process (Chen et al., 2021). miR-181b is an important gene in many human diseases. Experiments verified that lncRNA MEG3 directly regulates the level of miR-181b by binding the 3′UTR of miR-181B and reduces the levels of TNF-α and IL-1B in the serum and cerebrospinal fluid of ICH rats (Xie et al., 2021). MEG3 upregulation inhibits the expression of miR-146a and LET-7I, and the inflammatory factors IL-1β, IL-6 and TNF-α decrease synchronously, alleviating the inflammatory response in ankylosing spondylitis (Li et al., 2020b), (Ma et al., 2020). Acute pancreatitis (AP) is marked by severe inflammation. In the In vitro model of AP, the expression levels of MEG3 and FGFR2 are decreased, while the expression levels of miR-195-5p are increased. MEG3 promotes inflammatory responses through the MEG3/miR-195-5p/FGFR2 signaling axis (Chen and Song, 2021). MEG3 overexpression can downregulate miR-34a, enhance SIRT1 protein expression, inhibit the secretion of the inflammatory factors IL-1β, IL-6 and TNF-α, and inhibit liver inflammation by targeting SIRT6 with EZH2 (Tong et al., 2019; Zou et al., 2022).
During a large amount of genetic and environmental damage, the er of the cell is hindered in its ability to properly fold and translate postmodified proteins, leading to misfolding of proteins in the organelles, known as “er stress”. When a large number of misfolded proteins are present, an intracellular signaling pathway called the “unfolded protein response” (UPR) performs transcription and translation functions, restoring homeostasis. Endoplasmic reticulum stress and UPR defects are increasingly becoming key factors in human diseases, and the regulatory role of MEG3 in this aspect has begun to emerge (Sevier and Kaiser, 2002; Tu and Weissman, 2004; Ron and Walter, 2007; Wang and Kaufman, 2012). After MEG3 is downregulated in rectal cancer cells and MEG3 is restored, UPR response-related proteins, including GRP78, ATF6 and CHOP, are highly induced, and the cell apoptosis rate increases. The above reactions can be alleviated by simulated administration of miR-103A-3p. It can be concluded that MEG3 induces ER stress to promote the apoptosis of rectal cancer cells, and the interaction between miR-103A-3p and MEG3 negatively regulates this process (Wang et al., 2021e). At the same time, MEG3 upregulation activates NF-κB signaling to initiate ERS (Bao et al., 2020). As also mentioned above, MEG3 is involved in ER stress in HCC cells and cardiomyocytes by activating the p53 signaling pathway.
Genomic deletion of QKI-5, a member of the RNA (STAR) signal transduction activator protein family, promotes proliferation and dedifferentiation of cancer cells (Fu and Feng, 2015; He et al., 2016). Overexpression of MEG3 inhibits the expression of miR-9-5p and then upregulates QKI-5 to inhibit the proliferation, migration and invasion of prostate cancer cells (Wu et al., 2019). MEG3 overexpression inhibits the proliferation, invasion and migration of pituitary tumor cells, which is achieved by negatively regulating miR-23b -3p and miR-23b -3p negatively regulating FOXO4 (Wang et al., 2021b). MEG3, miR-376B-3p and HMGA2 form a signaling axis to regulate the invasion of pituitary tumor cells (Zhu et al., 2020). MEG3 and miR-361-5p regulate FoxM1 in vitro and inhibit the proliferation, migration and invasion of osteosarcoma cells (Shen et al., 2019). In a study of gastric cancer, MEG3 overexpression inhibits the expression of miR-21 and inhibits cell proliferation and metastasis (Dan et al., 2018).
For thyroid cancer cells resistant to 131I, low expression of MEG3 means increased expression of miR-182 and promotion of cell proliferation, which is detrimental to the treatment effect of 131I for thyroid cancer (Liu et al., 2018).
EMT was originally defined as the developmental program of epithelial cells during gastrula formation or movement of various cell types from the neural crest to distant sites in the embryo (Nieto et al., 2016; Dongre and Weinberg, 2019). In adult epithelial tissue, EMT is involved in the physiological process of wound repair and can be activated under certain pathological conditions, such as fibrosis and cancer (Nieto et al., 2016). In principle, cell phenotypic transitions engineered by EMT are reversible, so that individual cells previously activated by the EMT program can and often do revert to an epithelial state through mesenchymal to epithelial transition (MET) (Lu and Kang, 2019). EMT programs are initiated in cells by paracrine signaling factors to which they are exposed, most notably transforming growth factor-β (TGFβ). In addition, there are various other signals, such as WNT proteins, cytokines, growth factors, and extracellular matrix (ECM)-integrin interactions (Lambert and Weinberg, 2021). In reports on U251 cells, MEG3 overexpression resulted in decreased EMT, decreased expression of n-cadherin, vimentin, snail-1 and β-catenin, and increased expression of e-cadherin in U87 and U251 cells (Gong and Huang, 2020). MEG3 silencing also promotes EMT by regulating the miR-377/PTEN axis (Wang et al., 2019b). Yang et al. reported that the expression of MEG3 induced more mesenchymal cell morphology and increased zeb1/2 expression. Notably, autophagy inhibition can inhibit MEG3-induced EMT (Gao et al., 2018).
Cardiac fibrosis is a pathologic process involved in almost all cardiac diseases. Cardiac fibroblasts (CFs) are the main coordinators of extracellular matrix (ECM) remodelling after left ventricular pressure overload and play a key role in maintaining cardiac ECM integrity and homeostasis. Upon injury, CFs are activated into myofibroblasts that produce matrix metalloproteinases (MMPs) (Eghbali et al., 1989; Spinale, 2007; Travers et al., 2016; Frangogiannis, 2021). As a member of the MMP family, the increase in MMP-2 leads to the aggravation of fibrosis. LncRNA MEG3 was significantly enriched in myocardial fibroblasts. MEG3 silencing inhibits transforming growth factor (TGF-βI), interferes with the binding of p53 to sites −843 and −825 on the MMP-2 promoter, inhibits the activity of MMP-2, and subsequently decreases the expression of the fibrosis marker Ctgf. Thus, lncRNA MEG3 is coupled with p53 and plays a role in myocardial fibrosis (Piccoli et al., 2017) (Figure 3A).
FIGURE 3. MEG3 plays a role in cardiovascular-related cell types: cardiomyocytes, cardiac fibroblasts and endothelial cells. Regulation of TGE-β1 and P53 in cardiac fibroblasts; It is involved in apoptosis and autophagy in cardiomyocytes. It is associated with autophagy and DNA damage in endothelial cells.
In the process of cardiac hypertrophy, the role of lncRNA MEG3 cannot be ignored. Jingchang Zhang et al. found that the expression of MEG3 was abundant in cardiomyocytes treated with 150 nM ANG-II, and SH-MEG3 reversed the increase in the surface area of cardiomyocytes induced by ANG-II. Meanwhile, the expression of atrial natriuretic factor (ANF), brain natriuretic peptide (BNP) and β-myosin heavy chain (β-MHC) were decreased. This result preliminarily indicates that MEG3 positively regulates cardiac hypertrophy. After further research, Jingchang Zhang et al. found the promoter of MEG3-STAT3 with a binding relationship at site 2. Activated by STAT3, MEG3 competitively binds and inhibits miR-361-5p, upregulates downstream HDAC9 protein, and has a negative effect on cardiac hypertrophy (Zhang et al., 2019a).
Several studies have demonstrated that multiple upstream signals can regulate mTOR activity by regulating the phospholipid inositol three kinase protein (PI3K)/kinase B (AKT) signaling pathway (Nishimoto, 2000; Sun et al., 2017b; Wang et al., 2018). Hou et al. (2014) reported that advanced glycation end products inhibited the PI3K/Akt/mTOR pathway to promote cardiac autophagy. miR-7-5p is an important regulator of autophagy in a variety of cells (Song et al., 2016; Cai et al., 2017; Gu et al., 2017). Yinyin Cao et al. reported that MEG3 directly targets miR-7-5p and regulates downstream mTOR signal activation, thus regulating cardiac autophagy (Cao et al., 2019).
Hongchun Wu et al. reported that in hypoxic cardiomyocytes, p53 affects the expression of Meg3 by directly binding upstream of the Meg3 gene. Meg3 is upregulated by P53 and promotes cell apoptosis by directly binding to FUS protein. At the same time, after MEG3 inhibition, the expression of the pro-apoptotic gene Caspase-3 was decreased, and the expression of the anti-apoptotic gene Bcl-2 was increased, thus inhibiting myocardial cell apoptosis.23Ya Zhao et al. demonstrated that MEG3 regulates the expression of Bcl-2 and Caspase-3 proteins through miR-325 3p mediation, negatively regulating the expression of TRPV4 and thus alleviating myocardial cell injury and apoptosis (Zhou et al., 2021). In the study of Bin Yang et al., Bax expression was downregulated in H9C2 cells after oxygen and glucose deprivation (OGD) induction, caspase-3 and Caspase-9 were cleaved, and apoptosis occurred. In this process, MEG3 expression is increased, MDM2 depletion is increased, and the production of p53 is promoted, while the phosphorylation level of AMPK is reduced (Yang et al., 2019b). In the study of Yiwei Chen et al., lncRNA MEG3 expression was significantly increased in AC16 cardiomyocytes induced by high glucose (HG). Meanwhile, downregulation of MEGers.
Three could restore the effects of decreased expression of Bcl-2/Bax and increased expression of Caspase-3, which had beneficial effects on cell viability and apoptosis. Further studies showed that miR-145 directly acted on MEG3 and targeted PDCD4 protein at the 3′UTR binding site, and inhibition of miR-145 would offset the beneficial effects of downregulated MEG3 on HG-treated AC16 cardiomyocytes (Chen et al., 2019). Through the study of H9C2 cardiomyocytes after I/R injury, it was confirmed that downregulation of MEG3 can directly and negatively regulate miR-7-5p, regulate the downstream PARP1 protein and caspase-3 protein of miR-7-5P, and save cell apoptosis (Zou et al., 2019).
ERS induced by myocardial ischemia can induce myocardial cell apoptosis (Wei et al., 2016). In vivo experimental studies have shown that inhibition of lncRNA MEG3 can reduce the upregulation of endoplasmic reticulum stress-related proteins after ischemia, including BIP/GRP78, ATF4, and C-EBP homologous proteins (CHOP) (Li et al., 2019). Decreased levels of reactive oxygen species (ROS) are downstream events of endoplasmic reticulum stress after ischemia, and lenti-Si lncRNA MEG3 pretreatment can reduce ROS levels. Superoxide dismutase (SOD), an important antioxidant enzyme, is significantly reduced in ischemic myocardium, and downregulation of the MEG3 gene can negatively regulate this effect (Li et al., 2019). Previous studies have shown that the PERK-eIF2α and Caspase 12 pathways play a dominant role in stress-mediated apoptosis (Groenendyk et al., 2013), and downregulation of lncRNA MEG3 can inhibit ER stress through this pathway. In conclusion, downregulation of MEG3 can inhibit endoplasmic reticulum stress in cardiomyocytes and protect cardiomyocytes (Li et al., 2019).
According to L.-Y. Zhao et al., lncRNA MEG3 can also promote the apoptosis of hypoxic cardiomyocytes through the FoxO1 signaling pathway. FoxO1 is activated in the form of phosphorylation, which affects cell stability, leads to cell disorder, and promotes apoptosis of cardiomyocytes (Liu et al., 2017a; Kinyua et al., 2018). However, MEG3 upregulation in hypoxic cardiomyocytes not only upregulates FoxO1 but also increases the expression of FoxO3a in the same family. The combination of the two can enhance autophagy of cardiomyocytes and aggravate apoptosis of cardiomyocytes (Zhao et al., 2019).
Jinwen Su et al. found that the survival and proliferation of cardiac progenitor cells (CPCs) were inhibited under hypoxia, and the level of MEG3 significantly increased. Moreover, the cell viability and proliferation potential of cardiomyocytes increased after MEG3 inhibition. With the inhibition of MEG3, the expression level of miR-22 is increased, the expression level of HMGB1 protein, the next level target of MEG3, is decreased, and cell viability and proliferation are restored. In summary, MEG3/miR-22/HMGB1 has been confirmed to play a role in the survival and proliferation of cardiomyocytes after hypoxia (Su et al., 2018) (Figure 3B).
Liu et al. showed that MEG3 silencing can negatively regulate the Notch pathway to promote endothelial cell proliferation and angiogenesis (Liu et al., 2017b). In line with this, Chao He et al. found in their study that MEG3, as a ceRNA, inhibits miR-9, affects the phenotype of MEG3-mediated vascular endothelial cells (VECs), and inhibits cell proliferation and in vitro angiogenesis. However, the restoration of miR-9 can only rescue part of the angiogenesis inhibition caused by MEG3, which means that other microRNAs are also involved in this process (He et al., 2017). The experiment of Liu, H. Z et al. reported that the interaction between MEG3 and miR-150-5p was also involved in the angiogenesis of endothelial progenitor cells (Liu et al., 2016), which confirmed this point. In a report on anisomycin inhibition of angiogenesis, the spongy action of lncRNA MEG3 was inhibited and angiogenesis was inhibited via the miR-421/PDGFRA axis (Ye et al., 2019).
DNA double-strand breaks (DSBs) are a serious form of DNA damage. Tail length and tail moment of DNA are positively correlated with DSB burden (Olive and Banáth, 2006; Ribas-Maynou et al., 2012). MEG3 is a DNA damage response gene mediated by the p53 pathway. 5According to a report, in endothelial cells, after Meg3 was knocked out by 10 nM GapmeRs (chemically modified antisense oligonucleotides), tail length and tail camber were increased by 1.9 times and 2.7 times, respectively. After Meg3 is reduced by 2 nM GapmeRs, tail length and tail camber are increased by 2.4 times and 2.9 times, respectively (Shihabudeen Haider Ali et al., 2019). Phosphorylation of histone H2AX at Ser-19, a marker of DSB damage and repair (Mah et al., 2010; Sharma et al., 2012; Turinetto and Giachino, 2015), also increases. These data suggest that MEG3 protects DNA from damage in endothelial cells. Further studies have found that knocking down PTBP3 induces the expression of the target gene p53, leading to cell apoptosis, while MEG3 can participate in the DSB process by regulating PTBP3’s action on p53 or directly acting on p53, thus protecting endothelial function (Shihabudeen Haider Ali et al., 2019).
LncRNA MEG3 also plays a role in endothelial cell senescence. It has been detected that the competitive adsorption of MEG3 in aging endothelial cells decreases, leading to the upregulation of miR-128 and the targeting of Girdin protein, reducing the expression of Girdin protein. In addition, these processes inhibit platelet phagocytosis of endothelial cells (Lan et al., 2019) (Figure 3C).
lnc1. The diseases involving lncRNA MEG3 mainly include osteosarcoma, laryngeal cancer, prostate cancer, lung cancer, liver cancer, breast cancer, endometrial cancer, oral squamous cell carcinoma, gastric cancer, pancreatic cancer, colorectal cancer, and other malignant tumors. Metabolic diseases such as diabetic retinopathy; rheumatoid arthritis, ankylosing spondylitis, systemic lupus erythematosus and other immune system diseases; heart failure, hypertension, stroke and other cardiovascular and cerebrovascular diseases. A comprehensive understanding of its mechanism is essential for its safety and efficacy in clinical application.
lncRNA MEG3 plays a preventive and/or therapeutic role in cardiovascular diseases by reducing inflammatory injury, apoptosis and endoplasmic reticulum stress. lncRNA MEG3 is closely related to various pathologic effects of cardiomyocytes, fibroblasts and endothelial cells, and has great clinical application potential in the prevention and treatment of AS, MIRI, hypertension and HFLnc. Although some knowledge has been gained about the benefits of lncRNA MEG3 for cardiovascular disease from experimental data, it is worth noting that its specific underlying mechanisms are still relatively unknown. Therefore, available clinical and pharmacological data are insufficient to evaluate its efficacy.
In addition, lncRNA MEG3 has a profound research foundation in tumor diseases, first showing biomarker value in straight-colon cancer (Wang et al., 2019c), gastric cancer (Ghaedi et al., 2019), breast cancer (Ali et al., 2020) and other cancers (Duan et al., 2016; Zhao et al., 2018; Ali et al., 2020). Further research and technology development are needed to improve its bioavailability and overcome the challenges of its clinical application. Second, MEG3 expression is associated with WHO grade of tumor, old age at diagnosis, Karnofsky performance score (KPS), wild-type isocitrate dehydrogenase (IDH), tumor recurrence and overall survival, which is of prognostic value (Gong and Huang, 2017; Zhao et al., 2018; Buccarelli et al., 2020). Third, enhanced MEG3 expression can increase chemotherapy sensitivity to cisplatin, while SI-RNA silencing of MEG3 can induce cisplatin resistance (Ma et al., 2017), which can be used to measure therapeutic response. At the same time, MEG3 has gradually gained attention in cardiovascular disease, a major human public health problem. It can be a worthy object of study. Based on the research basis of lncRNA MEG3 in cancer diseases, we boldly speculate that MEG3 will play a role in the emerging discipline of cancer heart disease (Momtazmanesh and Rezaei, 2021).
In this paper, ZL is the first author and JG is the co-first author. ZL was responsible for the compilation, drawing and submission of the article; JG was responsible for the design of the article; DS, QJ, JM, SL and Xu Fan were responsible for the collation of the literature; YL and WC were responsible for the proofreading; HL was the corresponding author.
This work was supported by the National Natural Science Foundation of China [NO. 81973682].
The authors declare that the research was conducted in the absence of any commercial or financial relationships that could be construed as a potential conflict of interest.
All claims expressed in this article are solely those of the authors and do not necessarily represent those of their affiliated organizations, or those of the publisher, the editors and the reviewers. Any product that may be evaluated in this article, or claim that may be made by its manufacturer, is not guaranteed or endorsed by the publisher.
Ali, M. A., Shaker, O. G., Alazrak, M., AbdelHafez, M. N., Khalefa, A. A., Hemeda, N. F., et al. (2020). Association analyses of a genetic variant in long non-coding RNA MEG3 with breast cancer susceptibility and serum MEG3 expression level in the Egyptian population. Cancer Biomark. 28, 49–63. doi:10.3233/CBM-191072
Bao, D., Yuan, R. X., and Zhang, Y. (2020). Effects of lncRNA MEG3 on proliferation and apoptosis of gallbladder cancer cells through regulating NF-κB signaling pathway. Eur. Rev. Med. Pharmacol. Sci. 24, 6632–6638. doi:10.26355/eurrev_202006_21649
Botti, J., Djavaheri-Mergny, M., Pilatte, Y., and Codogno, P. (2006). Autophagy signaling and the cogwheels of cancer. Autophagy 2, 67–73. doi:10.4161/auto.2.2.2458
Buccarelli, M., Lulli, V., Giuliani, A., Signore, M., Martini, M., D'Alessandris, Q. G., et al. (2020). Deregulated expression of the imprinted DLK1-DIO3 region in glioblastoma stemlike cells: Tumor suppressor role of lncRNA MEG3. Neuro. Oncol. 22, 1771–1784. doi:10.1093/neuonc/noaa127
Cai, S., Shi, G. S., Cheng, H. Y., Zeng, Y. N., Li, G., Zhang, M., et al. (2017). Exosomal miR-7 mediates bystander autophagy in lung after focal brain irradiation in mice. Int. J. Biol. Sci. 13, 1287–1296. doi:10.7150/ijbs.18890
Cai, X., Chen, J., Xu, H., Liu, S., Jiang, Q. X., Halfmann, R., et al. (2014). Prion-like polymerization underlies signal transduction in antiviral immune defense and inflammasome activation. Cell 156, 1207–1222. doi:10.1016/j.cell.2014.01.063
Cao, Y., Wen, J., Li, Y., Chen, W., Wu, Y., Li, J., et al. (2019). Uric acid and sphingomyelin enhance autophagy in iPS cell-originated cardiomyocytes through lncRNA MEG3/miR-7-5p/EGFR axis. Artif. Cells Nanomed. Biotechnol. 47, 3774–3785. doi:10.1080/21691401.2019.1667817
Chen, K., Zhu, H., Zheng, M. Q., and Dong, Q. R. (2021). LncRNA MEG3 inhibits the degradation of the extracellular matrix of chondrocytes in osteoarthritis via targeting miR-93/TGFBR2 Axis. Cartilage 13, 1274s–1284s. doi:10.1177/1947603519855759
Chen, R. P., Huang, Z. L., Liu, L. X., Xiang, M. Q., Li, G. P., Feng, J. L., et al. (2016). Involvement of endoplasmic reticulum stress and p53 in lncRNA MEG3-induced human hepatoma HepG2 cell apoptosis. Oncol. Rep. 36, 1649–1657. doi:10.3892/or.2016.4919
Chen, X., and Song, D. (2021). LncRNA MEG3 participates in caerulein-induced inflammatory injury in human pancreatic cells via regulating miR-195-5p/FGFR2 Axis and inactivating NF-κB pathway. Inflammation 44, 160–173. doi:10.1007/s10753-020-01318-6
Chen, Y., Zhang, Z., Zhu, D., Zhao, W., and Li, F. (2019). Long non-coding RNA MEG3 serves as a ceRNA for microRNA-145 to induce apoptosis of AC16 cardiomyocytes under high glucose condition. Biosci. Rep. 39, BSR20190444. doi:10.1042/BSR20190444
Cheng, H. Y., Wang, Y. S., Hsu, P. Y., Chen, C. Y., Liao, Y. C., and Juo, S. H. (2019). miR-195 has a potential to treat ischemic and hemorrhagic stroke through neurovascular protection and neurogenesis. Mol. Ther. Methods Clin. Dev. 13, 121–132. doi:10.1016/j.omtm.2018.11.011
Cheng, Z. (2019). The FoxO-autophagy Axis in health and disease. Trends Endocrinol. Metab. 30, 658–671. doi:10.1016/j.tem.2019.07.009
Dan, J., Wang, J., Wang, Y., Zhu, M., Yang, X., Peng, Z., et al. (2018). LncRNA-MEG3 inhibits proliferation and metastasis by regulating miRNA-21 in gastric cancer. Biomed. Pharmacother. = Biomedecine Pharmacother. 99, 931–938. doi:10.1016/j.biopha.2018.01.164
Dehghan, M., Mente, A., Zhang, X., Swaminathan, S., Li, W., Mohan, V., et al. (2017). Associations of fats and carbohydrate intake with cardiovascular disease and mortality in 18 countries from five continents (PURE): A prospective cohort study. Lancet (London, Engl. 390, 2050–2062. doi:10.1016/S0140-6736(17)32252-3
Deng, C., Zhang, P., Harper, J. W., Elledge, S. J., and Leder, P. (1995). Mice lacking p21CIP1/WAF1 undergo normal development, but are defective in G1 checkpoint control. Cell 82, 675–684. doi:10.1016/0092-8674(95)90039-x
Dikic, I., and Elazar, Z. (2018). Mechanism and medical implications of mammalian autophagy. Nat. Rev. Mol. Cell Biol. 19, 349–364. doi:10.1038/s41580-018-0003-4
Dong, J., Xia, R., Zhang, Z., and Xu, C. (2021). lncRNA MEG3 aggravated neuropathic pain and astrocyte overaction through mediating miR-130a-5p/CXCL12/CXCR4 axis. Aging 13, 23004–23019. doi:10.18632/aging.203592
Dong, S., Ma, M., Li, M., Guo, Y., Zuo, X., Gu, X., et al. (2021). LncRNA MEG3 regulates breast cancer proliferation and apoptosis through miR-141-3p/RBMS3 axis. Genomics 113, 1689–1704. doi:10.1016/j.ygeno.2021.04.015
Dongre, A., and Weinberg, R. A. (2019). New insights into the mechanisms of epithelial-mesenchymal transition and implications for cancer. Nat. Rev. Mol. Cell Biol. 20, 69–84. doi:10.1038/s41580-018-0080-4
Duan, W., Du, L., Jiang, X., Wang, R., Yan, S., Xie, Y., et al. (2016). Identification of a serum circulating lncRNA panel for the diagnosis and recurrence prediction of bladder cancer. Oncotarget 7, 78850–78858. doi:10.18632/oncotarget.12880
Eghbali, M., Blumenfeld, O. O., Seifter, S., Buttrick, P. M., Leinwand, L. A., Robinson, T. F., et al. (1989). Localization of types I, III and IV collagen mRNAs in rat heart cells by in situ hybridization. J. Mol. Cell. Cardiol. 21, 103–113. doi:10.1016/0022-2828(89)91498-3
Feng, L. X., Sun, P., Mi, T., Liu, M., Liu, W., Yao, S., et al. (2016). Agglutinin isolated from Arisema heterophyllum Blume induces apoptosis and autophagy in A549 cells through inhibiting PI3K/Akt pathway and inducing ER stress. Chin. J. Nat. Med. 14, 856–864. doi:10.1016/S1875-5364(16)30102-9
Frangogiannis, N. G. (2021). Cardiac fibrosis. Cardiovasc. Res. 117, 1450–1488. doi:10.1093/cvr/cvaa324
Fu, X., and Feng, Y. (2015). QKI-5 suppresses cyclin D1 expression and proliferation of oral squamous cell carcinoma cells via MAPK signalling pathway. Int. J. Oral Maxillofac. Surg. 44, 562–567. doi:10.1016/j.ijom.2014.10.001
Galluzzi, L., Buqué, A., Kepp, O., Zitvogel, L., and Kroemer, G. (2017). Immunogenic cell death in cancer and infectious disease. Nat. Rev. Immunol. 17, 97–111. doi:10.1038/nri.2016.107
Gao, Q., Chang, X., Yang, M., Zheng, J., Gong, X., Liu, H., et al. (2022). LncRNA MEG3 restrained pulmonary fibrosis induced by NiO NPs via regulating hedgehog signaling pathway-mediated autophagy. Environ. Toxicol. 37, 79–91. doi:10.1002/tox.23379
Gao, S., Zhao, Z. Y., Wu, R., Zhang, Y., and Zhang, Z. Y. (2018). Prognostic value of long noncoding RNAs in gastric cancer: A meta-analysis. Onco. Targets. Ther. 11, 4877–4891. doi:10.2147/OTT.S169823
Gartel, A. L., and Radhakrishnan, S. K. (2005). Lost in transcription: p21 repression, mechanisms, and consequences. Cancer Res. 65, 3980–3985. doi:10.1158/0008-5472.CAN-04-3995
Ghaedi, H., Mozaffari, M. A. N., Salehi, Z., Ghasemi, H., Zadian, S. S., Alipoor, S., et al. (2019). Co-expression profiling of plasma miRNAs and long noncoding RNAs in gastric cancer patients. Gene 687, 135–142. doi:10.1016/j.gene.2018.11.034
Global, regional (2017). Global, regional, and national age-sex specific mortality for 264 causes of death, 1980-2016: A systematic analysis for the global burden of disease study 2016. Lancet (London, Engl. 390, 1151–1210. doi:10.1016/S0140-6736(17)32152-9
Gogiraju, R., Xu, X., Bochenek, M. L., Steinbrecher, J. H., Lehnart, S. E., Wenzel, P., et al. (2015). Endothelial p53 deletion improves angiogenesis and prevents cardiac fibrosis and heart failure induced by pressure overload in mice. J. Am. Heart Assoc. 4, e001770. doi:10.1161/JAHA.115.001770
Gohel, R., Kournoutis, A., Petridi, S., and Nezis, I. P. (2020). Molecular mechanisms of selective autophagy in Drosophila. Int. Rev. Cell Mol. Biol. 354, 63–105. doi:10.1016/bs.ircmb.2019.08.003
Gong, X., and Huang, M. (2017). Long non-coding RNA MEG3 promotes the proliferation of glioma cells through targeting Wnt/β-catenin signal pathway. Cancer Gene Ther. 24, 381–385. doi:10.1038/cgt.2017.32
Gong, X., and Huang, M. Y. (2020). Tumor-suppressive function of lncRNA-MEG3 in glioma cells by regulating miR-6088/SMARCB1 Axis. Biomed. Res. Int. 2020, 4309161. doi:10.1155/2020/4309161
Groenendyk, J., Agellon, L. B., and Michalak, M. (2013). Coping with endoplasmic reticulum stress in the cardiovascular system. Annu. Rev. Physiol. 75, 49–67. doi:10.1146/annurev-physiol-030212-183707
Gu, D. N., Jiang, M. J., Mei, Z., Dai, J. J., Dai, C. Y., Fang, C., et al. (2017). microRNA-7 impairs autophagy-derived pools of glucose to suppress pancreatic cancer progression. Cancer Lett. 400, 69–78. doi:10.1016/j.canlet.2017.04.020
Halliday, B. P., Cleland, J. G. F., Goldberger, J. J., and Prasad, S. K. (2017). Personalizing risk stratification for sudden death in dilated cardiomyopathy: The past, present, and future. Circulation 136, 215–231. doi:10.1161/CIRCULATIONAHA.116.027134
Han, K., Wang, F. R., Yu, M. Q., and Xu, B. (2020). Expression of concern: LncRNA MEG3 inhibits proliferation and promotes apoptosis of synovial cells in rats with knee osteoarthritis by regulating PTEN. Eur. Rev. Med. Pharmacol. Sci. 24, 8622. doi:10.26355/eurrev_202009_22747
He, C., and Klionsky, D. J. (2009). Regulation mechanisms and signaling pathways of autophagy. Annu. Rev. Genet. 43, 67–93. doi:10.1146/annurev-genet-102808-114910
He, C., Yang, W., Yang, J., Ding, J., Li, S., Wu, H., et al. (2017). Long noncoding RNA MEG3 negatively regulates proliferation and angiogenesis in vascular endothelial cells. DNA Cell Biol. 36, 475–481. doi:10.1089/dna.2017.3682
He, Y., Dan, Y., Gao, X., Huang, L., Lv, H., and Chen, J. (2021). DNMT1-mediated lncRNA MEG3 methylation accelerates endothelial-mesenchymal transition in diabetic retinopathy through the PI3K/Akt/mTOR signaling pathway. Am. J. Physiol. Endocrinol. Metab. 320, E598–e608. doi:10.1152/ajpendo.00089.2020
He, Z., Yi, J., Liu, X., Chen, J., Han, S., Jin, L., et al. (2016). MiR-143-3p functions as a tumor suppressor by regulating cell proliferation, invasion and epithelial-mesenchymal transition by targeting QKI-5 in esophageal squamous cell carcinoma. Mol. Cancer 15, 51. doi:10.1186/s12943-016-0533-3
Hou, X., Hu, Z., Xu, H., Xu, J., Zhang, S., Zhong, Y., et al. (2014). Advanced glycation endproducts trigger autophagy in cadiomyocyte via RAGE/PI3K/AKT/mTOR pathway. Cardiovasc. Diabetol. 13, 78. doi:10.1186/1475-2840-13-78
Hu, D., Su, C., Jiang, M., Shen, Y., Shi, A., Zhao, F., et al. (2016). Fenofibrate inhibited pancreatic cancer cells proliferation via activation of p53 mediated by upregulation of LncRNA MEG3. Biochem. Biophys. Res. Commun. 471, 290–295. doi:10.1016/j.bbrc.2016.01.169
Huang, Z. F., Tang, Y. L., Shen, Z. L., Yang, K. Y., and GaoUXT, K. (2022). UXT, a novel DNMT3b-binding protein, promotes breast cancer progression via negatively modulating lncRNA MEG3/p53 axis. Mol. Ther. Oncolytics 24, 497–506. doi:10.1016/j.omto.2021.12.008
Jia, H. Y., Zhang, K., Lu, W. J., Xu, G. W., Zhang, J. F., and Tang, Z. L. (2019). LncRNA MEG3 influences the proliferation and apoptosis of psoriasis epidermal cells by targeting miR-21/caspase-8. BMC Mol. Cell Biol. 20, 46. doi:10.1186/s12860-019-0229-9
Kinyua, A. W., Ko, C. M., Doan, K. V., Yang, D. J., Huynh, M. K. Q., Moh, S. H., et al. (2018). 4-hydroxy-3-methoxycinnamic acid regulates orexigenic peptides and hepatic glucose homeostasis through phosphorylation of FoxO1. Exp. Mol. Med. 50, e437. doi:10.1038/emm.2017.253
Kitamura, Y. I., Kitamura, T., Kruse, J. P., Raum, J. C., Stein, R., Gu, W., et al. (2005). FoxO1 protects against pancreatic beta cell failure through NeuroD and MafA induction. Cell Metab. 2, 153–163. doi:10.1016/j.cmet.2005.08.004
Kobayashi, M., Kikuchi, O., Sasaki, T., Kim, H. J., Yokota-Hashimoto, H., Lee, Y. S., et al. (2012). FoxO1 as a double-edged sword in the pancreas: Analysis of pancreas- and β-cell-specific FoxO1 knockout mice. Am. J. Physiol. Endocrinol. Metab. 302, E603–E613. doi:10.1152/ajpendo.00469.2011
Kruiswijk, F., Labuschagne, C. F., and Vousden, K. H. (2015). p53 in survival, death and metabolic health: a lifeguard with a licence to kill. Nat. Rev. Mol. Cell Biol. 16, 393–405. doi:10.1038/nrm4007
Ladapo, J. A., Budoff, M., Sharp, D., Zapien, M., Huang, L., Maniet, B., et al. (2017). Clinical utility of a precision medicine test evaluating outpatients with suspected obstructive coronary artery disease. Am. J. Med. 130, 482.e411–482. doi:10.1016/j.amjmed.2016.11.021
Lambert, A. W., and Weinberg, R. A. (2021). Linking EMT programmes to normal and neoplastic epithelial stem cells. Nat. Rev. Cancer 21, 325–338. doi:10.1038/s41568-021-00332-6
Lan, Y., Li, Y. J., Li, D. J., Li, P., Wang, J. Y., Diao, Y. P., et al. (2019). Long noncoding RNA MEG3 prevents vascular endothelial cell senescence by impairing miR-128-dependent Girdin downregulation. Am. J. Physiol. Cell Physiol. 316, C830–c843. doi:10.1152/ajpcell.00262.2018
Leslie, N. R. (2012). Pten: An intercellular peacekeeper? Sci. Signal. 5, pe50. doi:10.1126/scisignal.2003685
Li, H., Li, B., Zhu, D., Xie, H., Du, C., Xia, Y., et al. (2017). Downregulation of lncRNA MEG3 and miR-770-5p inhibit cell migration and proliferation in Hirschsprung's disease. Oncotarget 8, 69722–69730. doi:10.18632/oncotarget.19207
Li, P., Gao, Y., Li, J., Zhou, Y., Yuan, J., Guan, H., et al. (2018). LncRNA MEG3 repressed malignant melanoma progression via inactivating Wnt signaling pathway. J. Cell. Biochem. 119, 7498–7505. doi:10.1002/jcb.27061
Li, T., Luo, Y., Zhang, P., Guo, S., Sun, H., Yan, D., et al. (2020)., 129. Bethesda, Md, 1460–1467. doi:10.1152/japplphysiol.00433.2020LncRNA MEG3 regulates microglial polarization through KLF4 to affect cerebral ischemia-reperfusion injuryJ. Appl. Physiol.
Li, X., Tang, C., Wang, J., Guo, P., Wang, C., Wang, Y., et al. (2018). Methylene blue relieves the development of osteoarthritis by upregulating lncRNA MEG3. Exp. Ther. Med. 15, 3856–3864. doi:10.3892/etm.2018.5918
Li, X., Zhao, J., Geng, J., Chen, F., Wei, Z., Liu, C., et al. (2019). Long non-coding RNA MEG3 knockdown attenuates endoplasmic reticulum stress-mediated apoptosis by targeting p53 following myocardial infarction. J. Cell. Mol. Med. 23, 8369–8380. doi:10.1111/jcmm.14714
Li, X. D., He, S. S., Wan, T. T., and Li, Y. B. (2021). Liraglutide protects palmitate-induced INS-1 cell injury by enhancing autophagy mediated via FoxO1. Mol. Med. Rep. 23, 147. doi:10.3892/mmr.2020.11786
Li, Y., Zhang, S., Zhang, C., and Wang, M. (2020). LncRNA MEG3 inhibits the inflammatory response of ankylosing spondylitis by targeting miR-146a. Mol. Cell. Biochem. 466, 17–24. doi:10.1007/s11010-019-03681-x
Liang, J., Wang, Q., Li, J. Q., Guo, T., and Yu, D. (2020). Long non-coding RNA MEG3 promotes cerebral ischemia-reperfusion injury through increasing pyroptosis by targeting miR-485/AIM2 axis. Exp. Neurol. 325, 113139. doi:10.1016/j.expneurol.2019.113139
Liang, Z., Chi, Y. J., Lin, G. Q., Xiao, L. F., Su, G. L., and Yang, L. M. (2018). LncRNA MEG3 participates in neuronal cell injury induced by subarachnoid hemorrhage via inhibiting the Pi3k/Akt pathway. Eur. Rev. Med. Pharmacol. Sci. 22, 2824–2831. doi:10.26355/eurrev_201805_14983
Liao, X., Sharma, N., Kapadia, F., Zhou, G., Lu, Y., Hong, H., et al. (2011). Krüppel-like factor 4 regulates macrophage polarization. J. Clin. Invest. 121, 2736–2749. doi:10.1172/JCI45444
Liu, H. Z., Wang, Q. Y., Zhang, Y., Qi, D. T., Li, M. W., Guo, W. Q., et al. (2016). Pioglitazone up-regulates long non-coding RNA MEG3 to protect endothelial progenitor cells via increasing HDAC7 expression in metabolic syndrome. Biomed. Pharmacother. = Biomedecine Pharmacother. 78, 101–109. doi:10.1016/j.biopha.2016.01.001
Liu, J., Li, Q., Zhang, K. S., Hu, B., Niu, X., Zhou, S. M., et al. (2017). Downregulation of the long non-coding RNA Meg3 promotes angiogenesis after ischemic brain injury by activating Notch signaling. Mol. Neurobiol. 54, 8179–8190. doi:10.1007/s12035-016-0270-z
Liu, T., Ma, L., Zheng, Z., Li, F., Liu, S., Xie, Y., et al. (2017). Resveratrol inhibits age-dependent spontaneous tumorigenesis by SIRT1-mediated post-translational modulations in the annual fish Nothobranchius guentheri. Oncotarget 8, 55422–55434. doi:10.18632/oncotarget.19268
Liu, Y., Liu, C., Zhang, A., Yin, S., Wang, T., Wang, Y., et al. (2019). Down-regulation of long non-coding RNA MEG3 suppresses osteogenic differentiation of periodontal ligament stem cells (PDLSCs) through miR-27a-3p/IGF1 axis in periodontitis. Aging 11, 5334–5350. doi:10.18632/aging.102105
Liu, Y., Yue, P., Zhou, T., Zhang, F., Wang, H., and Chen, X. (2018). LncRNA MEG3 enhances (131)I sensitivity in thyroid carcinoma via sponging miR-182. Biomed. Pharmacother. = Biomedecine Pharmacother. 105, 1232–1239. doi:10.1016/j.biopha.2018.06.087
Liu, Z., Chen, J. Y., Zhong, Y., Xie, L., and Li, J. S. (2019). lncRNA MEG3 inhibits the growth of hepatocellular carcinoma cells by sponging miR-9-5p to upregulate SOX11. Braz. J. Med. Biol. Res. = Revista brasileira de pesquisas medicas e Biol. 52, e8631. doi:10.1590/1414-431X20198631
Lu, A., Magupalli, V. G., Ruan, J., Yin, Q., Atianand, M. K., Vos, M. R., et al. (2014). Unified polymerization mechanism for the assembly of ASC-dependent inflammasomes. Cell 156, 1193–1206. doi:10.1016/j.cell.2014.02.008
Lu, W., and Kang, Y. (2019). Epithelial-mesenchymal plasticity in cancer progression and metastasis. Dev. Cell 49, 361–374. doi:10.1016/j.devcel.2019.04.010
Ma, B., Gao, Z., Lou, J., Zhang, H., Yuan, Z., Wu, Q., et al. (2017). Long non-coding RNA MEG3 contributes to cisplatin-induced apoptosis via inhibition of autophagy in human glioma cells. Mol. Med. Rep. 16, 2946–2952. doi:10.3892/mmr.2017.6897
Ma, J., Zhang, X., Zhang, H., and Chen, H. (2020). lncRNA MEG3 suppresses the progression of ankylosis spondylitis by regulating the let-7i/SOST Axis. Front. Mol. Biosci. 7, 173. doi:10.3389/fmolb.2020.00173
Mah, L. J., El-Osta, A., and Karagiannis, T. C. (2010). gammaH2AX: a sensitive molecular marker of DNA damage and repair. Leukemia 24, 679–686. doi:10.1038/leu.2010.6
Meijer, A. J., and Codogno, P. (2006). Signalling and autophagy regulation in health, aging and disease. Mol. Asp. Med. 27, 411–425. doi:10.1016/j.mam.2006.08.002
Meng, J., Ding, T., Chen, Y., Long, T., Xu, Q., Lian, W., et al. (2021). LncRNA-Meg3 promotes Nlrp3-mediated microglial inflammation by targeting miR-7a-5p. Int. Immunopharmacol. 90, 107141. doi:10.1016/j.intimp.2020.107141
Momtazmanesh, S., and Rezaei, N. (2021). Long non-coding RNAs in diagnosis, treatment, prognosis, and progression of glioma: A state-of-the-art review. Front. Oncol. 11, 712786. doi:10.3389/fonc.2021.712786
Moradi, M. T., Fallahi, H., and Rahimi, Z. (2019). Interaction of long noncoding RNA MEG3 with miRNAs: A reciprocal regulation. J. Cell. Biochem. 120, 3339–3352. doi:10.1002/jcb.27604
Morana, O., Wood, W., and Gregory, C. D. (2022). The apoptosis paradox in cancer. Int. J. Mol. Sci. 23, 1328. doi:10.3390/ijms23031328
Niccoli, G., Montone, R. A., Lanza, G. A., and Crea, F. (2017). Angina after percutaneous coronary intervention: The need for precision medicine. Int. J. Cardiol. 248, 14–19. doi:10.1016/j.ijcard.2017.07.105
Nieto, M. A., Huang, R. Y., Jackson, R. A., and Thiery, J. P. (2016). Emt: 2016. Cell 166, 21–45. doi:10.1016/j.cell.2016.06.028
Nishimoto, T. (2000). Upstream and downstream of ran GTPase. Biol. Chem. 381, 397–405. doi:10.1515/BC.2000.052
Okano, J., Gaslightwala, I., Birnbaum, M. J., Rustgi, A. K., and Nakagawa, H. (2000). Akt/protein kinase B isoforms are differentially regulated by epidermal growth factor stimulation. J. Biol. Chem. 275, 30934–30942. doi:10.1074/jbc.M004112200
Olive, P. L., and Banáth, J. P. (2006). The comet assay: A method to measure DNA damage in individual cells. Nat. Protoc. 1, 23–29. doi:10.1038/nprot.2006.5
Oyama, K., Okawa, T., Nakagawa, H., Takaoka, M., Andl, C. D., Kim, S. H., et al. (2007). AKT induces senescence in primary esophageal epithelial cells but is permissive for differentiation as revealed in organotypic culture. Oncogene 26, 2353–2364. doi:10.1038/sj.onc.1210025
Padmanabhan, S., and Joe, B. (2017). Towards precision medicine for hypertension: A review of genomic, epigenomic, and microbiomic effects on blood pressure in experimental rat models and humans. Physiol. Rev. 97, 1469–1528. doi:10.1152/physrev.00035.2016
Pawar, K., Hanisch, C., Palma Vera, S. E., Einspanier, R., and Sharbati, S. (2016). Down regulated lncRNA MEG3 eliminates mycobacteria in macrophages via autophagy. Sci. Rep. 6, 19416. doi:10.1038/srep19416
Piccoli, M. T., Gupta, S. K., Viereck, J., Foinquinos, A., Samolovac, S., Kramer, F. L., et al. (2017). Inhibition of the cardiac fibroblast-enriched lncRNA Meg3 prevents cardiac fibrosis and diastolic dysfunction. Circ. Res. 121, 575–583. doi:10.1161/CIRCRESAHA.117.310624
Pu, Z., Wu, L., Guo, Y., Li, G., Xiang, M., Liu, L., et al. (2019). LncRNA MEG3 contributes to adenosine-induced cytotoxicity in hepatoma HepG2 cells by downregulated ILF3 and autophagy inhibition via regulation PI3K-AKT-mTOR and beclin-1 signaling pathway. J. Cell. Biochem. 120, 18172–18185. doi:10.1002/jcb.29123
Ribas-Maynou, J., García-Peiró, A., Abad, C., Amengual, M. J., Navarro, J., Benet, J., et al. (2012). Alkaline and neutral Comet assay profiles of sperm DNA damage in clinical groups. Hum. Reprod. 27, 652–658. doi:10.1093/humrep/der461
Ron, D., and Walter, P. (2007). Signal integration in the endoplasmic reticulum unfolded protein response. Nat. Rev. Mol. Cell Biol. 8, 519–529. doi:10.1038/nrm2199
Rosenbloom, J., Mendoza, F. A., and Jimenez, S. A. (2013). Strategies for anti-fibrotic therapies. Biochim. Biophys. Acta 1832, 1088–1103. doi:10.1016/j.bbadis.2012.12.007
Saftig, P., Beertsen, W., and Eskelinen, E. L. (2008). LAMP-2: A control step for phagosome and autophagosome maturation. Autophagy 4, 510–512. doi:10.4161/auto.5724
Savoia, C., Volpe, M., Grassi, G., Borghi, C., Agabiti Rosei, E., and Touyz, R. M. (2017)., 131. London, England, 2671–2685. doi:10.1042/CS20160407Personalized medicine-a modern approach for the diagnosis and management of hypertensionClin. Sci.
Sevier, C. S., and Kaiser, C. A. (2002). Formation and transfer of disulphide bonds in living cells. Nat. Rev. Mol. Cell Biol. 3, 836–847. doi:10.1038/nrm954
Sharma, A., Singh, K., and Almasan, A. (2012). Histone H2AX phosphorylation: A marker for DNA damage. Methods Mol. Biol. 920, 613–626. doi:10.1007/978-1-61779-998-3_40
Shen, B., Zhou, N., Hu, T., Zhao, W., Wu, D., and Wang, S. (2019). LncRNA MEG3 negatively modified osteosarcoma development through regulation of miR-361-5p and FoxM1. J. Cell. Physiol. 234, 13464–13480. doi:10.1002/jcp.28026
Shi, Y. (2020). MEG3 regulates apoptosis of adipose-derived stem cells. Mol. Med. Rep. 21, 2435–2442. doi:10.3892/mmr.2020.11059
Shihabudeen Haider Ali, M. S., Cheng, X., Moran, M., Haemmig, S., Naldrett, M. J., Alvarez, S., et al. (2019). LncRNA Meg3 protects endothelial function by regulating the DNA damage response. Nucleic Acids Res. 47, 1505–1522. doi:10.1093/nar/gky1190
Song, M., Wang, Y., Shang, Z. F., Liu, X. D., Xie, D. F., Wang, Q., et al. (2016). Bystander autophagy mediated by radiation-induced exosomal miR-7-5p in non-targeted human bronchial epithelial cells. Sci. Rep. 6, 30165. doi:10.1038/srep30165
Spinale, F. G. (2007). Myocardial matrix remodeling and the matrix metalloproteinases: Influence on cardiac form and function. Physiol. Rev. 87, 1285–1342. doi:10.1152/physrev.00012.2007
Strowig, T., Henao-Mejia, J., Elinav, E., and Flavell, R. (2012). Inflammasomes in health and disease. Nature 481, 278–286. doi:10.1038/nature10759
Su, J., Fang, M., Tian, B., Luo, J., Jin, C., Wang, X., et al. (2018). Atorvastatin protects cardiac progenitor cells from hypoxia-induced cell growth inhibition via MEG3/miR-22/HMGB1 pathway. Acta Biochim. Biophys. Sin. 50, 1257–1265. doi:10.1093/abbs/gmy133
Sun, D., Liu, J., Xiao, L., Liu, Y., Wang, Z., Li, C., et al. (2017). Recent development of risk-prediction models for incident hypertension: An updated systematic review. PloS one 12, e0187240. doi:10.1371/journal.pone.0187240
Sun, K. X., Wu, D. D., Chen, S., Zhao, Y., and Zong, Z. H. (2017). LncRNA MEG3 inhibit endometrial carcinoma tumorigenesis and progression through PI3K pathway. Apoptosis 22, 1543–1552. doi:10.1007/s10495-017-1426-7
Sun, W., Li, Y. N., Ye, J. F., Guan, Y. Q., and Li, S. J. (2018). MEG3 is involved in the development of glaucoma through promoting the autophagy of retinal ganglion cells. Eur. Rev. Med. Pharmacol. Sci. 22, 2534–2540. doi:10.26355/eurrev_201805_14942
Sun, W., Lou, H., Cao, J., Wang, P., Sha, W., and Sun, Q. (2020). LncRNA MEG3 control Mycobacterium Tuberculosis infection via controlled MiR-145-5p expression and modulation of macrophages proliferation. Microb. Pathog. 149, 104550. doi:10.1016/j.micpath.2020.104550
Tang, Z. L., Zhang, K., Lv, S. C., Xu, G. W., Zhang, J. F., and Jia, H. Y. (2021). Corrigendum to "LncRNA MEG3 suppresses PI3K/AKT/mTOR signalling pathway to enhance autophagy and inhibit inflammation in TNF-α-treated keratinocytes and psoriatic mice" [Cytokine 148 (2021) 155657]. Cytokine 148, 155853. doi:10.1016/j.cyto.2022.155853
Tao, P., Yang, B., Zhang, H., Sun, L., Wang, Y., and Zheng, W. (2020). The overexpression of lncRNA MEG3 inhibits cell viability and invasion and promotes apoptosis in ovarian cancer by sponging miR-205-5p. Int. J. Clin. Exp. Pathol. 13, 869–879.
Tian, Z. Z., Guo, X. J., Zhao, Y. M., and Fang, Y. (2015). Decreased expression of long non-coding RNA MEG3 acts as a potential predictor biomarker in progression and poor prognosis of osteosarcoma. Int. J. Clin. Exp. Pathol. 8, 15138–15142.
Tong, P., Peng, Q. H., Gu, L. M., Xie, W. W., and Li, W. J. (2019). LncRNA-MEG3 alleviates high glucose induced inflammation and apoptosis of retina epithelial cells via regulating miR-34a/SIRT1 axis. Exp. Mol. Pathol. 107, 102–109. doi:10.1016/j.yexmp.2018.12.003
Travers, J. G., Kamal, F. A., Robbins, J., Yutzey, K. E., and Blaxall, B. C. (2016). Cardiac fibrosis: The fibroblast awakens. Circ. Res. 118, 1021–1040. doi:10.1161/CIRCRESAHA.115.306565
Tsao, C. W., Aday, A. W., Almarzooq, Z. I., Alonso, A., Beaton, A. Z., Bittencourt, M. S., et al. (2022). Heart disease and stroke statistics-2022 update: A report from the American heart association. Circulation 145, e153–e639. doi:10.1161/CIR.0000000000001052
Tu, B. P., and Weissman, J. S. (2004). Oxidative protein folding in eukaryotes: Mechanisms and consequences. J. Cell Biol. 164, 341–346. doi:10.1083/jcb.200311055
Turinetto, V., and Giachino, C. (2015). Multiple facets of histone variant H2AX: A DNA double-strand-break marker with several biological functions. Nucleic Acids Res. 43, 2489–2498. doi:10.1093/nar/gkv061
Vadasz, Z., and Toubi, E. (2014). Semaphorins: Their dual role in regulating immune-mediated diseases. Clin. Rev. Allergy Immunol. 47, 17–25. doi:10.1007/s12016-013-8360-4
Wang, A., Hu, N., Zhang, Y., Chen, Y., Su, C., Lv, Y., et al. (2019). MEG3 promotes proliferation and inhibits apoptosis in osteoarthritis chondrocytes by miR-361-5p/FOXO1 axis. BMC Med. Genomics 12, 201. doi:10.1186/s12920-019-0649-6
Wang, C., Tao, X., and Wei, J. (2021). Effects of LncRNA MEG3 on immunity and autophagy of non-small cell lung carcinoma through Ido signaling pathway. World J. Surg. Oncol. 19, 244. doi:10.1186/s12957-021-02346-8
Wang, D., Fu, C. W., and Fan, D. Q. (2019). Participation of tumor suppressors long non-coding RNA MEG3, microRNA-377 and PTEN in glioma cell invasion and migration. Pathol. Res. Pract. 215, 152558. doi:10.1016/j.prp.2019.152558
Wang, G., Ye, Q., Ning, S., Yang, Z., Chen, Y., Zhang, L., et al. (2021). LncRNA MEG3 promotes endoplasmic reticulum stress and suppresses proliferation and invasion of colorectal carcinoma cells through the MEG3/miR-103a-3p/PDHB ceRNA pathway. Neoplasma 68, 362–374. doi:10.4149/neo_2020_200813N858
Wang, J., Xu, W., He, Y., Xia, Q., and Liu, S. (2018). LncRNA MEG3 impacts proliferation, invasion, and migration of ovarian cancer cells through regulating PTEN. Inflamm. Res. 67, 927–936. doi:10.1007/s00011-018-1186-z
Wang, S., and Kaufman, R. J. (2012). The impact of the unfolded protein response on human disease. J. Cell Biol. 197, 857–867. doi:10.1083/jcb.201110131
Wang, W., Xie, Y., Chen, F., Liu, X., Zhong, L. L., Wang, H. Q., et al. (2019). LncRNA MEG3 acts a biomarker and regulates cell functions by targeting ADAR1 in colorectal cancer. World J. Gastroenterol. 25, 3972–3984. doi:10.3748/wjg.v25.i29.3972
Wang, X., Li, X., and Wang, Z. (2021). lncRNA MEG3 inhibits pituitary tumor development by participating in cell proliferation, apoptosis and EMT processes. Oncol. Rep. 45, 40. doi:10.3892/or.2021.7991
Wang, X., Wang, Z., Wang, J., Wang, Y., Liu, L., and Xu, X. (2017). LncRNA MEG3 has anti-activity effects of cervical cancer. Biomed. Pharmacother. = Biomedecine Pharmacother. 94, 636–643. doi:10.1016/j.biopha.2017.07.056
Wang, Y. X., Lin, C., Cui, L. J., Deng, T. Z., Li, Q. M., Chen, F. Y., et al. (2021). Mechanism of M2 macrophage-derived extracellular vesicles carrying lncRNA MEG3 in inflammatory responses in ulcerative colitis. Bioengineered 12, 12722–12739. doi:10.1080/21655979.2021.2010368
Wang, Z., Xia, P., Hu, J., Huang, Y., Zhang, F., Li, L., et al. (2021). LncRNA MEG3 alleviates diabetic cognitive impairments by reducing mitochondrial-derived apoptosis through promotion of FUNDC1-related mitophagy via rac1-ROS Axis. ACS Chem. Neurosci. 12, 2280–2307. doi:10.1021/acschemneuro.0c00682
Wei, C., Wang, Y., Li, M., Li, H., Lu, X., Shao, H., et al. (2016). Spermine inhibits endoplasmic reticulum stress-induced apoptosis: A new strategy to prevent cardiomyocyte apoptosis. Cell. Physiol. biochem. 38, 531–544. doi:10.1159/000438648
Wei, G. H., and Wang, X. (2017). lncRNA MEG3 inhibit proliferation and metastasis of gastric cancer via p53 signaling pathway. Eur. Rev. Med. Pharmacol. Sci. 21, 3850–3856.
Wu, H., Zhao, Z. A., Liu, J., Hao, K., Yu, Y., Han, X., et al. (2018). Long noncoding RNA Meg3 regulates cardiomyocyte apoptosis in myocardial infarction. Gene Ther. 25, 511–523. doi:10.1038/s41434-018-0045-4
Wu, M., Huang, Y., Chen, T., Wang, W., Yang, S., Ye, Z., et al. (2019). LncRNA MEG3 inhibits the progression of prostate cancer by modulating miR-9-5p/QKI-5 axis. J. Cell. Mol. Med. 23, 29–38. doi:10.1111/jcmm.13658
Xia, H., Qu, X. L., Liu, L. Y., Qian, D. H., and Jing, H. Y. (2018). LncRNA MEG3 promotes the sensitivity of vincristine by inhibiting autophagy in lung cancer chemotherapy. Eur. Rev. Med. Pharmacol. Sci. 22, 1020–1027. doi:10.26355/eurrev_201802_14384
Xia, Z., Ding, D., Zhang, N., Wang, J., Yang, H., and Zhang, D. (2019). LncRNA-MEG3 protects against ganglion cell dysplasia in congenital intestinal atresia through directly regulating miR-211-5p/GDNF axis. Biomed. Pharmacother. = Biomedecine Pharmacother. 111, 436–442. doi:10.1016/j.biopha.2018.11.089
Xiang, Y., Zhang, Y., Xia, Y., Zhao, H., Liu, A., and Chen, Y. (2020). LncRNA MEG3 targeting miR-424-5p via MAPK signaling pathway mediates neuronal apoptosis in ischemic stroke. Aging 12, 3156–3174. doi:10.18632/aging.102790
Xie, B., Qiao, M., and Xuan, J. (2021). lncRNA MEG3 downregulation relieves intracerebral hemorrhage by inhibiting oxidative stress and inflammation in an miR-181b-dependent manner. Med. Sci. Monit. 27, e929435. doi:10.12659/MSM.929435
Xiu, Y. L., Sun, K. X., Chen, X., Chen, S., Zhao, Y., Guo, Q. G., et al. (2017). Upregulation of the lncRNA Meg3 induces autophagy to inhibit tumorigenesis and progression of epithelial ovarian carcinoma by regulating activity of ATG3. Oncotarget 8, 31714–31725. doi:10.18632/oncotarget.15955
Yan, H., Yuan, J., Gao, L., Rao, J., and Hu, J. (2016). Long noncoding RNA MEG3 activation of p53 mediates ischemic neuronal death in stroke. Neuroscience 337, 191–199. doi:10.1016/j.neuroscience.2016.09.017
Yang, B., Zheng, C., Yu, H., Zhang, R., Zhao, C., and Cai, S. (2019). Cardio-protective effects of salvianolic acid B on oxygen and glucose deprivation (OGD)-treated H9c2 cells. Artif. Cells Nanomed. Biotechnol. 47, 2274–2281. doi:10.1080/21691401.2019.1621885
Yang, J., Nie, J., Ma, X., Wei, Y., Peng, Y., and Wei, X. (2019). Targeting PI3K in cancer: Mechanisms and advances in clinical trials. Mol. Cancer 18, 26. doi:10.1186/s12943-019-0954-x
Yang, M., Chang, X., Gao, Q., Gong, X., Zheng, J., Liu, H., et al. (2022). LncRNA MEG3 ameliorates NiO nanoparticles-induced pulmonary inflammatory damage via suppressing the p38 mitogen activated protein kinases pathway. Environ. Toxicol. 37, 1058–1070. doi:10.1002/tox.23464
Ye, W., Ni, Z., Yicheng, S., Pan, H., Huang, Y., Xiong, Y., et al. (2019). Anisomycin inhibits angiogenesis in ovarian cancer by attenuating the molecular sponge effect of the lncRNA-Meg3/miR-421/PDGFRA axis. Int. J. Oncol. 55, 1296–1312. doi:10.3892/ijo.2019.4887
Yi, J., Chen, B., Yao, X., Lei, Y., Ou, F., and Huang, F. (2019). Upregulation of the lncRNA MEG3 improves cognitive impairment, alleviates neuronal damage, and inhibits activation of astrocytes in hippocampus tissues in Alzheimer's disease through inactivating the PI3K/Akt signaling pathway. J. Cell. Biochem. 120, 18053–18065. doi:10.1002/jcb.29108
Yorimitsu, T., and Klionsky, D. J. (2007). Eating the endoplasmic reticulum: Quality control by autophagy. Trends Cell Biol. 17, 279–285. doi:10.1016/j.tcb.2007.04.005
Yu, Y., Kou, D., Liu, B., Huang, Y., Li, S., Qi, Y., et al. (2020). LncRNA MEG3 contributes to drug resistance in acute myeloid leukemia by positively regulating ALG9 through sponging miR-155. Int. J. Lab. Hematol. 42, 464–472. doi:10.1111/ijlh.13225
Yusuf, S., Rangarajan, S., Teo, K., Islam, S., Li, W., Liu, L., et al. (2014). Cardiovascular risk and events in 17 low-middle-and high-income countries. N. Engl. J. Med. 371, 818–827. doi:10.1056/NEJMoa1311890
Zhang, H., Tao, J., Zhang, S., and Lv, X. (2020). LncRNA MEG3 reduces hippocampal neuron apoptosis via the PI3K/AKT/mTOR pathway in a rat model of temporal lobe epilepsy. Neuropsychiatr. Dis. Treat. 16, 2519–2528. doi:10.2147/NDT.S270614
Zhang, H. (2019). Mechanism associated with aberrant lncRNA MEG3 expression in gestational diabetes mellitus. Exp. Ther. Med. 18, 3699–3706. doi:10.3892/etm.2019.8062
Zhang, J., Liang, Y., Huang, X., Guo, X., Liu, Y., Zhong, J., et al. (2019). STAT3-induced upregulation of lncRNA MEG3 regulates the growth of cardiac hypertrophy through miR-361-5p/HDAC9 axis. Sci. Rep. 9, 460. doi:10.1038/s41598-018-36369-1
Zhang, T., Kim, D. H., Xiao, X., Lee, S., Gong, Z., Muzumdar, R., et al. (2016). FoxO1 plays an important role in regulating β-cell compensation for insulin resistance in male mice. Endocrinology 157, 1055–1070. doi:10.1210/en.2015-1852
Zhang, X., Gejman, R., Mahta, A., Zhong, Y., Rice, K. A., Zhou, Y., et al. (2010). Maternally expressed gene 3, an imprinted noncoding RNA gene, is associated with meningioma pathogenesis and progression. Cancer Res. 70, 2350–2358. doi:10.1158/0008-5472.CAN-09-3885
Zhang, X., Wu, N., Wang, J., and Li, Z. (2019). LncRNA MEG3 inhibits cell proliferation and induces apoptosis in laryngeal cancer via miR-23a/APAF-1 axis. J. Cell. Mol. Med. 23, 6708–6719. doi:10.1111/jcmm.14549
Zhang, Y., Liu, X., Bai, X., Lin, Y., Li, Z., Fu, J., et al. (2018). Melatonin prevents endothelial cell pyroptosis via regulation of long noncoding RNA MEG3/miR-223/NLRP3 axis. J. Pineal Res. 64, e12449. doi:10.1111/jpi.12449
Zhao, H., Wang, X., Feng, X., Li, X., Pan, L., Liu, J., et al. (2018). Long non-coding RNA MEG3 regulates proliferation, apoptosis, and autophagy and is associated with prognosis in glioma. J. Neurooncol. 140, 281–288. doi:10.1007/s11060-018-2874-9
Zhao, L. Y., Li, X., Gao, L., and Xu, Y. (2019). LncRNA MEG3 accelerates apoptosis of hypoxic myocardial cells via FoxO1 signaling pathway. Eur. Rev. Med. Pharmacol. Sci. 23, 334–340. doi:10.26355/eurrev_201908_18665
Zhou, Y., Li, X., Zhao, D., Li, X., and Dai, J. (2021). Long non-coding RNA MEG3 knockdown alleviates hypoxia-induced injury in rat cardiomyocytes via the miR-325-3p/TRPV4 axis. Mol. Med. Rep. 23, 18. doi:10.3892/mmr.2020.11656
Zhu, D., Xiao, Z., Wang, Z., Hu, B., Duan, C., Zhu, Z., et al. (2020). MEG3/MIR-376B-3P/HMGA2 axis is involved in pituitary tumor invasiveness. J. Neurosurg., 1–13. doi:10.3171/2019.10.JNS191959
Zhu, M., Wang, X., Gu, Y., Wang, F., Li, L., and Qiu, X. (2019). MEG3 overexpression inhibits the tumorigenesis of breast cancer by downregulating miR-21 through the PI3K/Akt pathway. Arch. Biochem. Biophys. 661, 22–30. doi:10.1016/j.abb.2018.10.021
Zou, D., Liu, L., Zeng, Y., Wang, H., Dai, D., and Xu, M. (2022). LncRNA MEG3 up-regulates SIRT6 by ubiquitinating EZH2 and alleviates nonalcoholic fatty liver disease. Cell Death Discov. 8, 103. doi:10.1038/s41420-022-00889-7
Keywords: lncRNA MEG3, cardiovascular diseases, P53 signaling pathway, PI3K/akt signaling pathway, apoptosis, autophagy, inflammation, endoplasmic reticulum
Citation: Li Z, Gao J, Sun D, Jiao Q, Ma J, Cui W, Lou Y, Xu F, Li S and Li H (2022) LncRNA MEG3: Potential stock for precision treatment of cardiovascular diseases. Front. Pharmacol. 13:1045501. doi: 10.3389/fphar.2022.1045501
Received: 15 September 2022; Accepted: 11 November 2022;
Published: 29 November 2022.
Edited by:
Yan Yang, outhwestern Medical University, ChinaReviewed by:
Carlos Ernesto Fernández-García, Princess University Hospital, SpainCopyright © 2022 Li, Gao, Sun, Jiao, Ma, Cui, Lou, Xu, Li and Li. This is an open-access article distributed under the terms of the Creative Commons Attribution License (CC BY). The use, distribution or reproduction in other forums is permitted, provided the original author(s) and the copyright owner(s) are credited and that the original publication in this journal is cited, in accordance with accepted academic practice. No use, distribution or reproduction is permitted which does not comply with these terms.
*Correspondence: Haixia Li, MjI3MjIzNjA1NUBxcS5jb20=
†These authors have contributed equally to this work and share first authorship
Disclaimer: All claims expressed in this article are solely those of the authors and do not necessarily represent those of their affiliated organizations, or those of the publisher, the editors and the reviewers. Any product that may be evaluated in this article or claim that may be made by its manufacturer is not guaranteed or endorsed by the publisher.
Research integrity at Frontiers
Learn more about the work of our research integrity team to safeguard the quality of each article we publish.