- 1Pancreas Center, The First Affiliated Hospital of Nanjing Medical University, Nanjing, China
- 2Gastroenterology Department, Jiangsu Province Hospital of Chinese Medicine, Affiliated Hospital of Nanjing University of Chinese Medicine, Nanjing, China
- 3Jiangsu Key Laboratory for Pharmacology and Safety Evaluation of Chinese Materia Medica, School of Pharmacy, Nanjing University of Chinese Medicine, Nanjing, China
- 4Department of Endocrinology, Jiangsu Province Hospital of Chinese Medicine, Affiliated Hospital of Nanjing University of Chinese Medicine, Nanjing, China
- 5Department of Oncology, The First Affiliated Hospital of Nanjing Medical University, Nanjing, China
Chronic pancreatitis (CP) is a precancerous illness linked to pancreatic ductal adenocarcinoma (PDAC), although the evolutionary mechanism is uncertain. CP is distinguished by severe fibrosis caused by the activation of pancreatic stellate cells (PSCs). The current clinical therapeutic protocol for CP lacks specific therapeutic medicines for the prevention and suppression of inflammation and fibrosis aggravating in CP. More research on specifically targeting PSCs would help facilitate the development of novel therapies for pancreatic fibrosis. Notably, using natural compounds from medicinal plants as new antifibrotic agents has become a focus of recent research and is widely employed as an alternative and complementary approach. Our goal was to shed light on the role of PSCs in the development of CP and provide a focused update on the new potential therapeutic strategies against PSCs in CP models. Future studies can refer to these possible strategies for drug design, bioavailability, pharmacokinetics, and other issues to obtain better clinical outcomes for treating CP.
Introduction
CP is characterized by inflammation, fibrosis, and loss of acinar and islet cells, which may present as abdominal pain, steatorrhea, pancreatic exocrine and endocrine insufficiency, and imaging-detectable pancreatic damage (Beyer et al., 2020). CP has a poorly known pathogenesis. Nonetheless, there appears to be a consistent sequence of an initial shock with damage, followed by recovery via fibrosis and regeneration. Recurrent oxidative stress or inflammation are fundamental causes of persistent pancreatic injury, causing irreversible changes in pancreatic structure and function and eventually leading to the onset of CP (Ren et al., 2020).
Pathogenesis of chronic pancreatitis differs based on underlying etiology, genetic background, and environmental exposures. Alcohol, the most prevalent cause of CP, may induce damage via toxic alcohol metabolites, overexpression of numerous genes related to cell death, direct stimulation of PSCs (resulting in fibrosis), and other mechanisms (Setiawan et al., 2017). Abnormal stromal/desmoplastic response is the hallmark histological feature of CP, which is attributed mainly to the activation of PSCs. Targeting PSCs may be a viable treatment strategy for CP (Kandikattu et al., 2020).
Currently, there are still few clinically available therapeutic agents for CP. Recently, some preclinical research into PSCs has been conducted, which will aid in creating innovative therapeutics for CP. In addition, natural chemicals derived from medicinal plants have great potential as antifibrotic drugs for the treatment of CP and deserve more in-depth studies in vivo and in vitro (Calfio et al., 2020).
This paper focuses on research targeting PSCs in CP models and reviews in detail the antifibrotic capabilities of several potential drugs for treating CP.
Pancreatic stellate cells
Nearly a century after Karl von Kupffer’s discovery of hepatic stellate cells (HSCs) in 1876, Wateri’s group first detected similar cells (later termed PSCs) in the pancreas (Watari et al., 1982). It was not until 1998 that two milestone studies detailed the isolation and culture procedures of PSCs in vitro, allowing researchers to examine them in health and disease (Apte et al., 1998; Bachem et al., 1998). Since then, a slew of new research has been conducted in this area, and PSCs have become widely recognized as the primary source of the stromal/desmoplastic response that is characteristic of pancreatic cancer and CP (Apte and Wilson, 2004; Apte and Wilson, 2012; Pang et al., 2017).
PSCs have two phenotypes: quiescent (qPSCs) and activated (aPSCs). Under normal physiological conditions, qPSCs can be found in the peri-acinar or interlobular areas and comprise around 4% of the pancreatic cells (Apte et al., 1998; Bachem et al., 1998). qPSCs play a role in vitamin A storage, immunity, and the preservation of the normal structure in the pancreas (Allam et al., 2017). qPSCs have many typical features, such as abundant perinuclear lipid droplets, molecular markers (cytoglobin and adipophilin), and a low capacity to proliferate, migrate and synthesize ECM (Apte et al., 2013; Nielsen et al., 2017). Several factors can contribute to the activation process of qPSCs, such as smoking, alcohol intake, oxidative stress, hypoxia, etc. (Fu et al., 2018). In diseases like PDAC or CP, qPSCs get continuously activated and transform into aPSCs (Apte et al., 1999; Bynigeri et al., 2017). aPSCs show a myofibroblast-like phenotype: positive staining of α-smooth muscle actin (αSMA), loss of lipid droplets, increased production of cytokines and ECM (collagens, hyaluronic acid, fibronectin, etc.), and elevated ability to migrate, proliferate (Apte et al., 1999; Bynigeri et al., 2017). We have previously given a detailed overview of PDAC-associated PSCs, and the following will focus on their role in CP and targeted strategies (Wu et al., 2020).
Pancreatic stellate cells in the development of chronic pancreatitis
PSCs are special pancreatic resident cells that provide critical functions in both normal and abnormal pancreata. PSCs activation is a crucial step in the fibrogenic process of the pancreas (Bynigeri et al., 2017; Beyer et al., 2020). Multiple stimuli (ethanol, hyperglycemia, oxidative stress, cytokines, chemokines, etc.) can activate PSCs, causing them to release an abundance of ECM and hence promote the severe interlobular and intralobular desmoplastic reaction. Increased pancreatic fibrosis can lead to impaired exocrine and endocrine function of the pancreas. In early CP, damaged acinar cells stimulate major inflammatory cells (macrophages, granulocytes, and lymphocytes) that subsequently release large amounts of cytokines, such as interleukin (IL)-1/-4/-6/-8, tumor necrosis factor-alpha (TNF-α), transforming growth factor-beta 1 (TGF-β1), platelet-derived growth factor (PDGF), etc. (Jin et al., 2020; Kandikattu et al., 2020; Zheng et al., 2021). These molecules then stimulate PSCs, while PSCs can also secrete cytokines and continue to activate themselves through an autocrine pathway. The continuous stimulation of PSCs disrupts the synthesis-degradation balance of the ECM, ultimately leading to ECM deposition and fibrosis in the pancreas (Jin et al., 2020; Kandikattu et al., 2020; Zheng et al., 2021). Figure 1 is a schematic representation of the activation of PSCs in CP.
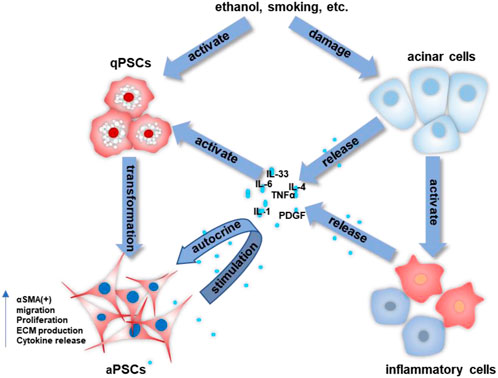
FIGURE 1. The activation process of PSCs in CP. The crosstalk among acinar cells, inflammatory cells, and stellate cells allows for the continued activation of PSCs and the progression of pancreatic fibrosis.
Several investigations on the mechanisms have been undertaken. Wu et al. indicated that TGF-β1 induced the activation of the nuclear factor kappa B (NF-κB) pathway in PSCs through modulating phospho-transforming growth factor beta-activated kinase 1 (p-TAK1), and this pathway might be a target for treating CP (Wu et al., 2021). Li et al. (2021a) showed that nicotine facilitated pancreatic fibrosis by inducing the activation of PSCs through Janus kinase 2/signal transducer and activator of transcription 3 (JAK2/STAT3) signaling pathway in rats, revealing the mechanism of smoking on CP. Lee et al. (2015) demonstrated that the cumulative effects of ethanol and cigarette components could activate PSCs in alcoholic smokers, accelerating the evolution of pancreatic fibrosis. Another study showed that the JAK/STAT pathway was essential for the proliferation and activation of PSCs and that inhibition of this pathway reduced caerulein-induced CP in vivo (Komar et al., 2017). Recently, Yang et al. indicated that very low-density lipoprotein receptor (VLDLR)-enhanced lipoprotein metabolism in PSCs increased fibrosis and that IL-33 played an essential role in this process in CP (Yang et al., 2022).
All these findings indicate that PSCs’ activation plays a crucial role in the development of CP, making PSCs a promising therapeutic target for CP.
Drugs for the treatment of chronic pancreatitis by targeting pancreatic stellate cells
Even though the area of PSCs is still in its infancy, scientists have made significant efforts and proposed different strategies to target PSCs for treating CP. Recent research has indicated that siRNAs and small molecule kinase inhibitors can treat CP by targeting PSCs (Pezzilli et al., 2011; Tan et al., 2019; Mohanavelu et al., 2021). In addition, studies have shown that natural compounds from plants are widely used as complementary and alternative medications to treat diseases and extend life spans (Lin et al., 2015). Such drugs have promising clinical applications in the prevention and treatment of CP. The following is a discussion of the current status of several potential drugs and their possible mechanisms in treating CP.
Since morphological, functional, and gene expression investigations revealed considerable similarities between PSCs and HSCs, research on HSCs will be very informative (Erkan et al., 2012; Paulo et al., 2013). In addition, considering that PSCs derived from PDAC and CP have much in common in causing pancreatic fibrosis, some drugs of potential research value will also be listed here. Table 1 provides a summary of the existing antifibrotic strategies.
siRNA
siRNAs represent a form of posttranscriptional gene silencing and have emerged as a fundamental and extensive regulator of gene expression. The most recent technology of high-throughput sequencing allows the analysis of siRNA abundance and expression profile in specific organs, tissues, and cells more and more precisely, allowing for the investigation of the roles of miRNAs and siRNAs (Fowler et al., 2018). mRNA transcripts of altered genes in genetic disorders and tumors are typical siRNA targets (Nambudiri and Widlund, 2013). Several studies have shown that the involvement of siRNAs in the expression of certain genes, which in turn influence the onset and progression of chronic pancreatitis, has potential therapeutic value (Charo et al., 2013). For instance, recent studies showed that the NACHT, LRR, and PYD domains-containing protein 3 (NLRP3) inflammatory bodies were directly involved in PSCs’ activation in vivo and in vitro. NLPR3 siRNA decreased the activation markers of PSCs (αSMA, collagen I, fibronectin) expression (Li et al., 2021b). Another study indicated that activated yes-associated protein (YAP) enhanced PSCs proliferation and knockdown of YAP by siRNA decreased the activation of PSCs and fibrosis in the CP animal model (Spanehl et al., 2022). However, these studies are still in the laboratory stage and must be further validated in clinical trials.
Artemisinin and its derivatives
Artemisinin was identified as an isolated phytochemical derived from the Artemisia annua in 1972 (White et al., 2015). Several chemical derivatives have been developed to increase artemisinin’s water and oil solubility without compromising its therapeutic effect. Artemisinin was first used by the ancient Chinese to treat “fever,” commonly thought of as malaria (Miller and Su, 2011). This substance and its derivatives are still vital in treating malaria today.
Recent studies have also identified these chemicals’ role in treating different diseases, including inflammation, infection, cancer, and fibrosis (Efferth, 2006; Lai et al., 2013; Kim et al., 2015; Shi et al., 2015). Our preliminary research found that dihydroartemisinin (DHA) reduced liver fibrosis via inducing ferroptosis in HSCs (Shen et al., 2022). However, artemisinin and its derivatives have not been reported in pancreatic fibrosis in CP, which is a potential hot spot for future research.
Vitamin A/D derivatives
In CP, vitamin A derivatives were reported to induce significant apoptosis, inhibit proliferation, and suppress ECM production of PSCs in vitro, indicating the potential of Vitamin A derivatives in reducing pancreatic fibrosis in CP (Xiao et al., 2015). However, the application of Vitamin A and its derivatives has been little studied in CP research. Therefore its use in targeting PSCs in PDAC is of a reference value. Previous research has demonstrated that Vitamin A analogs can inhibit the activation of PSCs in PDAC (Chronopoulos et al., 2016). ATRA (a Vitamin A analog) was found to reprise the quiescent state of PSCs in KPC mice (Froeling et al., 2011). All these studies show that Vitamin A analogs have potential research and clinical translational value in treating CP-related fibrosis.
A high prevalence of Vitamin D (VD) deficiency in CP patients is associated with the risk and prognosis of CP (Klapdor et al., 2012; Joker-Jensen et al., 2020). VD serves several biological roles in the body and has been extensively studied in inflammatory illnesses. VD and its derivatives have been found to inhibit PSCs’ activation and decrease ECM deposition, therefore relieving pancreatic fibrosis. Several investigations have shown that VD analogs may reprise the quiescence of PSCs and reduce fibrosis in CP and PDAC (Sherman et al., 2014; Wallbaum et al., 2018). These findings imply that VD might be an effective antifibrotic treatment for CP. More high-quality research and clinical trials are necessary to validate the anti-fibrosis function of VD in CP.
Small molecule kinase inhibitors
Kinases have been the subject of contemporary pharmacological study due to their crucial function in cellular signal transmission (Wu et al., 2015). Previous research has shown that fibromodulin (FMOD) is increased in CP and is an essential downstream mediator of oxidative stress. The extracellular signal-regulated kinases (ERK) and c-Jun N-terminal kinases (JNK) inhibitors can decrease FMOD, thereby decreasing PSCs’ activation (An et al., 2020). Dasatinib, an inhibitor of several tyrosine kinases (TKs), was shown to have possible anti-fibrosis effects on CP and decrease pancreatic fibrosis and macrophage infiltration (Zeng et al., 2019). Olaparib, a peroxisome proliferator-activated receptor gamma (PARP) inhibitor, was reported to have antifibrotic effects in cerulein-induced CP (El-Hamoly et al., 2021). Imatinib, an inhibitor of discoidin domain receptor 1 (DDR1) and DDR2, can reduce ECM deposition and PSCs’ activation while inhibiting the TGF-β1/Smad pathway (Bansod et al., 2021). Small molecule kinase inhibitors are one of the current research hotspots and have promising applications in the treatment of CP.
Resveratrol
Resveratrol is a polyphenolic stilbene in significant concentrations in grape, raspberry, blueberry, and peanut species (Rauf et al., 2018). Resveratrol has been shown to induce apoptosis of CP cells and alleviate fibrosis by promoting caspase-3 activation (Zhou et al., 2011). Previous studies have shown that resveratrol can suppress intracellular reactive oxygen species (ROS) and boost PSCs’ activation and glycolytic metabolism by suppressing miR-21 expression (Yan et al., 2018). Resveratrol inhibited ROS-induced activation of PSCs by decreasing miR-21 expression and raising the phosphatase and tensin homolog deleted on Chromosome 10 (PTEN) (Yan et al., 2018). Another study reported that resveratrol could inhibit the activation markers of PSCs (αSMA, collagen I, and fibronectin) by downregulating NF-κB signaling (Lin et al., 2015).
Rhein
Rhein, the active component of rhubarb, is used extensively in treatment because of its anti-inflammatory, anti-angiogenic and anticancer characteristics (Xian et al., 2020; Li et al., 2021c). Rhein was reported to attenuate PSCs’ activation and suppress sonic hedgehog (SHH)/glioma-associated oncogene homolog 1 (GLI1) signaling in pancreatic fibrosis (Tsang et al., 2013). Rhein was able to suppress αSMA, Fibronectin, and matrix metalloproteinases (MMPs) in cultured PSCs via modulating the SHH pathway (Tsang et al., 2013; Tsang and Bian, 2015). Studies have shown that down-regulation of NF-κB and STAT3 signaling pathways may be the potential mechanism of Rhein’s anti-fibrosis and anti-tumor effects (Lin et al., 2015).
Epigallocatechin gallate
Epigallocatechin gallate (EGCG), the main phenolic compound in green tea, was reported to have antioxidant, anti-inflammatory, and anticancer functions (Lee et al., 2014; Hosseini and Ghorbani, 2015). Polyphenols in green tea can prevent fibrosis by decreasing the activation of PSCs (Asaumi et al., 2006). Masamune et al. indicated that EGCG could reduce the ability of PSCs to proliferate and migrate (Masamune et al., 2005). In vitro experiments showed that EGCG pretreatment could inhibit the ethanol-induced activation of PSCs (Asaumi et al., 2007). Ethanol was known to increase the protein expression of αSMA, activate TGF-β1, and induce p38 mitogen-activated protein kinase (MAPK) phosphorylation. After EGCG treatment, p38 MAPK phosphorylation was eliminated, and the activation process of PSCs was inhibited (Asaumi et al., 2006).
Metformin
Metformin has gained widespread use in treating type II diabetes. (Bailey, 2017). Studies have shown that metformin exerts anticancer effects by regulating inflammatory responses (Vancura et al., 2018). Previous studies have indicated that metformin significantly reduces the expression of αSMA and collagen and inhibits PSCs proliferation by upregulating phosphorylation-AMP-activated protein kinase (p-AMPK) expression (Duan et al., 2017).
Emodin
Emodin, a natural anthraquinone derivative in some herbs, was reported to have anti-inflammation, anti-angiogenesis, anti-dyslipidemia, and anticancer functions (Gaman et al., 2018; Semwal et al., 2021). According to the literature, after 4 mmol/l emodin treatment, emodin downregulated the expression of several fibrosis markers (αSMA, fibronectin, and collagen I), thereby reducing the cell viability of primary PSCs (Lin et al., 2015). Table 1 lists the drugs that may be explored in CP treatment.
Discussion
Multiple investigations have established that PSCs play a critical role in CP. All the above treatments have been reported to prevent the activation of PSCs in CP and the resulting fibrosis. These studies have also revealed the molecular mechanisms of the various drugs.
We address several drugs with antifibrotic effects that may serve as innovative antifibrotic treatments for PSCs in CP. Notably, phytochemicals should be encouraged for further in-depth research due to their cheap, non-toxic nature and sound therapeutic effects on PSCs in CP. Based on preclinical studies, given their potential as new antifibrotic drugs, it is important to consider drug delivery and low bioavailability. Recently, applications of nanoparticle systems have emerged in clinical practice related to drug delivery (Poilil Surendran et al., 2017; Sharma et al., 2017). We are confident that there will be relevant studies to test and validate these hypotheses and find new solutions for treating CP in the future.
Apart from these, some other promising options, such as ferroptosis inducers, have not been studied in CP. In recent years, ferroptosis has become a new hotspot to describe the regulatory form of cell death. Ferroptosis is closely related to tumors, degenerative diseases, ischemia-reperfusion injury, and cardiovascular diseases (Wei et al., 2020). Previous research showed that tripartite motif-containing protein 26 (TRIM26), a ferroptosis inducer, promoted ferroptosis in HSCs to inhibit liver fibrosis, which indicated that ferroptosis inducers might be potential anti-fibrosis agents (Zhu et al., 2021). Ellagic acid (EA), a natural product, exerted its antifibrotic effects by inducing ferroportin (FPN)-dependent ferroptosis of HSCs by disrupting the formation of SNARE complexes in liver fibrosis (Li et al., 2022). Luo et al. indicated that celastrol demonstrated anti-fibrotic effects on activated HSCs via boosting ROS generation and triggering ferroptosis (Luo et al., 2022). Our published paper has also revealed that DHA alleviates liver fibrosis by inducing ferroptosis in HSCs. We uncovered the possible mechanism of DHA against hepatic fibrosis and demonstrated that ferroptosis might be a novel method to remove activated HSCs (Zhang et al., 2021). Although these medications have not been investigated for CP fibrosis, their scientific value and clinical application possibilities in CP are promising.
However, drug research in CP still faces many challenges. When testing new treatments, it is vital to choose models that replicate the realities of CP in humans as closely as possible. There is still a long way to go in the development of new CP models. Besides, it is essential to note that the treatment modalities in these experimental models were performed at the same time as or before the induction of CP, which does not fully reflect the actual clinical situation. Therefore, the next major step is to construct phase I and subsequent trials for human CP so that the laboratory outcomes may be transferred to the bedside.
Author contributions
Investigate and write the original paper: YW, CZ, and WH; review and edit: YQ, DX, and ML; Supervision: MG and PW; Manuscript revision: JS and RS; Project administration: ZZ and KJ. All authors have read and agreed to the published version of the manuscript. All authors contributed to the article and approved the submitted version.
Funding
The work was supported by the National Natural Science Foundation of China (Nos. 82000572, 81871980, 82203850), the Natural Science Foundation of Jiangsu Province (No. BK20200840), General Projects of the Natural Science Research of Jiangsu Higher Education Institutions (No. 20KJB310003), the Natural Science Foundation of Nanjing University of Chinese Medicine (No. NZY82000572).
Conflict of interest
The authors declare that the research was conducted in the absence of any commercial or financial relationships that could be construed as a potential conflict of interest.
Publisher’s note
All claims expressed in this article are solely those of the authors and do not necessarily represent those of their affiliated organizations, or those of the publisher, the editors and the reviewers. Any product that may be evaluated in this article, or claim that may be made by its manufacturer, is not guaranteed or endorsed by the publisher.
References
Allam, A., GothwalM., , Saha, D., Maurer, J., and Brunner, T. B. (2017). Pancreatic stellate cells in pancreatic cancer: In focus. Pancreatology 17 (4), 514–522. doi:10.1016/j.pan.2017.05.390
An, W., Zhu, J. W., Jiang, F., Jiang, H., Zhao, J. L., Liu, M. Y., et al. (2020). Fibromodulin is upregulated by oxidative stress through the MAPK/AP-1 pathway to promote pancreatic stellate cell activation. Pancreatology 20 (2), 278–287. doi:10.1016/j.pan.2019.09.011
Apte, M. V., Haber, P. S., Applegate, T. L., Norton, I. D., McCaughan, G. W., Korsten, M. A., et al. (1998). Periacinar stellate shaped cells in rat pancreas: Identification, isolation, and culture. Gut 43 (1), 128–133. doi:10.1136/gut.43.1.128
Apte, M. V., Haber, P. S., Darby, S. J., Rodgers, S. C., McCaughan, G. W., Korsten, M. A., et al. (1999). Pancreatic stellate cells are activated by proinflammatory cytokines: Implications for pancreatic fibrogenesis. Gut 44 (4), 534–541. doi:10.1136/gut.44.4.534
Apte, M. V., and Wilson, J. S. (2012). Dangerous liaisons: Pancreatic stellate cells and pancreatic cancer cells. J. Gastroenterol. Hepatol. 27, 69–74. doi:10.1111/j.1440-1746.2011.07000.x
Apte, M. V., Wilson, J. S., Lugea, A., and Pandol, S. J. (2013). A starring role for stellate cells in the pancreatic cancer microenvironment. Gastroenterology 144 (6), 1210–1219. doi:10.1053/j.gastro.2012.11.037
Apte, M. V., and Wilson, J. S. (2004). Mechanisms of pancreatic fibrosis. Dig. Dis. 22 (3), 273–279. doi:10.1159/000082799
Asaumi, H., Watanabe, S., Taguchi, M., Tashiro, M., and Otsuki, M. (2007). Externally applied pressure activates pancreatic stellate cells through the generation of intracellular reactive oxygen species. Am. J. Physiol. Gastrointest. Liver Physiol. 293 (5), G972–G978. doi:10.1152/ajpgi.00018.2007
Asaumi, H., Watanabe, S., TaguchiM., , TashiroM., , Nagashio, Y., NomiYama, Y., et al. (2006). Green tea polyphenol (-)-epigallocatechin-3-gallate inhibits ethanol-induced activation of pancreatic stellate cells. Eur. J. Clin. Invest. 36 (2), 113–122. doi:10.1111/j.1365-2362.2006.01599.x
Bachem, M. G., SchnEidEr, E., Gross, H., WeidenbacH, H., Schmid, R. M., Menke, A., et al. (1998). Identification, culture, and characterization of pancreatic stellate cells in rats and humans. Gastroenterology 115 (2), 421–432. doi:10.1016/s0016-5085(98)70209-4
Bailey, C. J. (2017). Metformin: Historical overview. Diabetologia 60 (9), 1566–1576. doi:10.1007/s00125-017-4318-z
Bansod, S., Saifi, M. A., and Godugu, C. (2021). Inhibition of discoidin domain receptors by imatinib prevented pancreatic fibrosis demonstrated in experimental chronic pancreatitis model. Sci. Rep. 11 (1), 12894. doi:10.1038/s41598-021-92461-z
Beyer, G., Habtezion, A., Werner, J., Lerch, M. M., and Mayerle, J. (2020). Chronic pancreatitis. Lancet 396 (10249), 499–512. doi:10.1016/S0140-6736(20)31318-0
Bynigeri, R. R., Jakkampudi, A., Jangala, R., Subramanyam, C., Sasikala, M., Rao, G. V., et al. (2017). Pancreatic stellate cell: Pandora's box for pancreatic disease biology. World J. Gastroenterol. 23 (3), 382–405. doi:10.3748/wjg.v23.i3.382
Calfio, C., Gonzalez, A., Singh, S. K., Rojo, L. E., and Maccioni, R. B. (2020). The emerging role of nutraceuticals and phytochemicals in the prevention and treatment of alzheimer's disease. J. Alzheimers Dis. 77 (1), 33–51. doi:10.3233/JAD-200443
Charo, C., Holla, V., Arumugam, T., Hwang, R., Yang, P., Dubois, R. N., et al. (2013). Prostaglandin E2 regulates pancreatic stellate cell activity via the EP4 receptor. Pancreas 42 (3), 467–474. doi:10.1097/MPA.0b013e318264d0f8
Chronopoulos, A., Robinson, B., Sarper, M., Cortes, E., Auernheimer, V., Lachowski, D., et al. (2016). ATRA mechanically reprograms pancreatic stellate cells to suppress matrix remodelling and inhibit cancer cell invasion. Nat. Commun. 7, 12630. doi:10.1038/ncomms12630
Cui, L. H., Li, C. X., Zhuo, Y. Z., Yang, L., Cui, N. Q., and Zhang, S. K. (2019). Saikosaponin d ameliorates pancreatic fibrosis by inhibiting autophagy of pancreatic stellate cells via PI3K/Akt/mTOR pathway. Chem. Biol. Interact. 300, 18–26. doi:10.1016/j.cbi.2019.01.005
Duan, W., Chen, K., Jiang, Z., Chen, X., Sun, L., Li, J., et al. (2017). Desmoplasia suppression by metformin-mediated AMPK activation inhibits pancreatic cancer progression. Cancer Lett. 385, 225–233. doi:10.1016/j.canlet.2016.10.019
Efferth, T. (2006). Molecular pharmacology and pharmacogenomics of artemisinin and its derivatives in cancer cells. Curr. Drug Targets 7 (4), 407–421. doi:10.2174/138945006776359412
El-Hamoly, T., Hajnady, Z., Nagy-Penzes, M., Bakondi, E., Regdon, Z., Demeny, M. A., et al. (2021). Poly(ADP-Ribose) polymerase 1 promotes inflammation and fibrosis in a mouse model of chronic pancreatitis. Int. J. Mol. Sci. 22 (7), 3593. doi:10.3390/ijms22073593
Erkan, M., Adler, G., Apte, M. V., Bachem, M. G., Buchholz, M., Detlefsen, S., et al. (2012). StellaTUM: Current consensus and discussion on pancreatic stellate cell research. Gut 61 (2), 172–178. doi:10.1136/gutjnl-2011-301220
Fowler, E. K., Mohorianu, I., Smith, D. T., Dalmay, T., and Chapman, T. (2018). Small RNA populations revealed by blocking rRNA fragments in Drosophila melanogaster reproductive tissues. PLoS One 13 (2), e0191966. doi:10.1371/journal.pone.0191966
Froeling, F. E., Feig, C., Chelala, C., Dobson, R., Mein, C. E., Tuveson, D. A., et al. (2011). Retinoic acid-induced pancreatic stellate cell quiescence reduces paracrine Wnt-beta-catenin signaling to slow tumor progression. Gastroenterology 141 (4), 1486–1497. doi:10.1053/j.gastro.2011.06.047
Fu, Y., Liu, S., Zeng, S., and Shen, H. (2018). The critical roles of activated stellate cells-mediated paracrine signaling, metabolism and onco-immunology in pancreatic ductal adenocarcinoma. Mol. Cancer 17 (1), 62. doi:10.1186/s12943-018-0815-z
Gaman, L., Dragos, D., Vlad, A., Robu, G. C., Radoi, M. R., Stroica, L., et al. (2018). Phytoceuticals in acute pancreatitis: Targeting the balance between apoptosis and necrosis. Evid. Based Complement. Altern. Med. 2018, 5264592. doi:10.1155/2018/5264592
Hosseini, A., and Ghorbani, A. (2015). Cancer therapy with phytochemicals: Evidence from clinical studies. Avicenna J. Phytomed. 5 (2), 84–97.
Jin, G., Hong, W., Guo, Y., Bai, Y., and Chen, B. (2020). Molecular mechanism of pancreatic stellate cells activation in chronic pancreatitis and pancreatic cancer. J. Cancer 11 (6), 1505–1515. doi:10.7150/jca.38616
Joker-Jensen, H., Mathiasen, A. S., Kohler, M., Rasmussen, H. H., Drewes, A. M., and Olesen, S. S. (2020). Micronutrient deficits in patients with chronic pancreatitis: Prevalence, risk factors and pitfalls. Eur. J. Gastroenterol. Hepatol. 32 (10), 1328–1334. doi:10.1097/MEG.0000000000001866
Kandikattu, H. K., Venkateshaiah, S. U., and Mishra, A. (2020). Chronic pancreatitis and the development of pancreatic cancer. Endocr. Metab. Immune Disord. Drug Targets 20 (8), 1182–1210. doi:10.2174/1871530320666200423095700
Kim, W. S., Choi, W. J., Lee, S., Lee, D. C., Sohn, U. D., Kim, W. J., et al. (2015). Anti-inflammatory, antioxidant and antimicrobial effects of artemisinin extracts from Artemisia annua L. Korean J. Physiol. Pharmacol. 19 (1), 21–27. doi:10.4196/kjpp.2015.19.1.21
Klapdor, S., Richter, E., and Klapdor, R. (2012). Vitamin D status and per-oral vitamin D supplementation in patients suffering from chronic pancreatitis and pancreatic cancer disease. Anticancer Res. 32 (5), 1991–1998.
Komar, H. M., Serpa, G., Kerscher, C., Schwoegl, E., Mace, T. A., Jin, M., et al. (2017). Inhibition of Jak/STAT signaling reduces the activation of pancreatic stellate cells in vitro and limits caerulein-induced chronic pancreatitis in vivo. Sci. Rep. 7 (1), 1787. doi:10.1038/s41598-017-01973-0
Lai, H. C., Singh, N. P., and Sasaki, T. (2013). Development of artemisinin compounds for cancer treatment. Invest. New Drugs 31 (1), 230–246. doi:10.1007/s10637-012-9873-z
Lee, A. T., Xu, Z., Pothula, S. P., Patel, M. B., Pirola, R. C., Wilson, J. S., et al. (2015). Alcohol and cigarette smoke components activate human pancreatic stellate cells: Implications for the progression of chronic pancreatitis. Alcohol. Clin. Exp. Res. 39 (11), 2123–2133. doi:10.1111/acer.12882
Lee, L. S., Kim, S. H., Kim, Y. B., and Kim, Y. C. (2014). Quantitative analysis of major constituents in green tea with different plucking periods and their antioxidant activity. Molecules 19 (7), 9173–9186. doi:10.3390/molecules19079173
Li, C. X., Cui, L. H., Zhang, L. Q., Yang, L., Zhuo, Y. Z., Cui, N. Q., et al. (2021). Role of NLR family pyrin domain-containing 3 inflammasome in the activation of pancreatic stellate cells. Exp. Cell Res. 404 (2), 112634. doi:10.1016/j.yexcr.2021.112634
Li, G. M., Chen, J. R., Zhang, H. Q., Cao, X. Y., Sun, C., Peng, F., et al. (2021). Update on pharmacological activities, security, and pharmacokinetics of rhein. Evid. Based. Complement. Altern. Med. 2021, 4582412. doi:10.1155/2021/4582412
Li, L., Wang, K., Jia, R., Xie, J., Ma, L., Hao, Z., et al. (2022). Ferroportin-dependent ferroptosis induced by ellagic acid retards liver fibrosis by impairing the SNARE complexes formation. Redox Biol. 56, 102435. doi:10.1016/j.redox.2022.102435
Li, Z., Lu, D., Jin, T., Liu, X., and Hao, J. (2021). Nicotine facilitates pancreatic fibrosis by promoting activation of pancreatic stellate cells via α7nAChR-mediated JAK2/STAT3 signaling pathway in rats. Toxicol. Lett. 349, 84–91. doi:10.1016/j.toxlet.2021.06.012
Lin, Z., Zheng, L. C., Zhang, H. J., Tsang, S. W., and Bian, Z. X. (2015). Anti-fibrotic effects of phenolic compounds on pancreatic stellate cells. BMC Complement. Altern. Med. 15, 259. doi:10.1186/s12906-015-0789-y
Luo, P., Liu, D., Zhang, Q., Yang, F., Wong, Y. K., Xia, F., et al. (2022). Celastrol induces ferroptosis in activated HSCs to ameliorate hepatic fibrosis via targeting peroxiredoxins and HO-1. Acta Pharm. Sin. B 12 (5), 2300–2314. doi:10.1016/j.apsb.2021.12.007
Masamune, A., Kikuta, K., Satoh, M., Suzuki, N., and Shimosegawa, T. (2005). Green tea polyphenol epigallocatechin-3-gallate blocks PDGF-induced proliferation and migration of rat pancreatic stellate cells. World J. Gastroenterol. 11 (22), 3368–3374. doi:10.3748/wjg.v11.i22.3368
Miller, L. H., and Su, X. (2011). Artemisinin: Discovery from the Chinese herbal garden. Cell 146 (6), 855–858. doi:10.1016/j.cell.2011.08.024
Mohanavelu, P., Mutnick, M., Mehra, N., White, B., Kudrimoti, S., Hernandez Kluesner, K., et al. (2021). Meta-analysis of gastrointestinal adverse events from tyrosine kinase inhibitors for chronic myeloid leukemia. Cancers (Basel) 13 (7), 1643. doi:10.3390/cancers13071643
Nambudiri, V. E., and Widlund, H. R. (2013). Small interfering RNA. J. Invest. Dermatol. 133 (12), 1–4. doi:10.1038/jid.2013.411
Nielsen, M. F. B., Mortensen, M. B., and Detlefsen, S. (2017). Identification of markers for quiescent pancreatic stellate cells in the normal human pancreas. Histochem. Cell Biol. 148 (4), 359–380. doi:10.1007/s00418-017-1581-5
Pang, T. C. Y., Wilson, J. S., and Apte, M. V. (2017). Pancreatic stellate cells: what's new? Curr. Opin. Gastroenterol. 33 (5), 366–373. doi:10.1097/MOG.0000000000000378
Paulo, J. A., Kadiyala, V., Banks, P. A., Conwell, D. L., and Steen, H. (2013). Mass spectrometry-based quantitative proteomic profiling of human pancreatic and hepatic stellate cell lines. Genomics Proteomics Bioinforma. 11 (2), 105–113. doi:10.1016/j.gpb.2013.01.009
Pezzilli, R., Fabbri, D., Imbrogno, A., and Corinaldesi, R. (2011). Tyrosine kinase inhibitors, pancreatic hyperenzymemia and acute pancreatitis: A review. Recent Pat. Inflamm. Allergy Drug Discov. 5 (2), 165–168. doi:10.2174/187221311795399255
Poilil Surendran, S., George Thomas, R., Moon, M. J., and Jeong, Y. Y. (2017). Nanoparticles for the treatment of liver fibrosis. Int. J. Nanomedicine 12, 6997–7006. doi:10.2147/IJN.S145951
Rauf, A., Imran, M., Butt, M. S., Nadeem, M., Peters, D. G., and Mubarak, M. S. (2018). Resveratrol as an anti-cancer agent: A review. Crit. Rev. Food Sci. Nutr. 58 (9), 1428–1447. doi:10.1080/10408398.2016.1263597
Ren, Y., Zhang, J., Wang, M., Bi, J., Wang, T., Qiu, M., et al. (2020). Identification of irisin as a therapeutic agent that inhibits oxidative stress and fibrosis in a murine model of chronic pancreatitis. Biomed. Pharmacother. 126, 110101. doi:10.1016/j.biopha.2020.110101
Semwal, R. B., Semwal, D. K., Combrinck, S., and Viljoen, A. (2021). Emodin - a natural anthraquinone derivative with diverse pharmacological activities. Phytochemistry 190, 112854. doi:10.1016/j.phytochem.2021.112854
Setiawan, V. W., Monroe, K., Lugea, A., Yadav, D., and Pandol, S. (2017). Uniting epidemiology and experimental disease models for alcohol-related pancreatic disease. Alcohol Res. 38 (2), 173–182.
Sharma, N., Bhandari, S., Deshmukh, R., Yadav, A. K., and Mishra, N. (2017). Development and characterization of embelin-loaded nanolipid carriers for brain targeting. Artif. Cells Nanomed. Biotechnol. 45 (3), 409–413. doi:10.3109/21691401.2016.1160407
Shen, M., Guo, M., Li, Y., Wang, Y., Qiu, Y., Shao, J., et al. (2022). m(6 A methylation is required for dihydroartemisinin to alleviate liver fibrosis by inducing ferroptosis in hepatic stellate cells. Free Radic. Biol. Med. 182, 246–259. doi:10.1016/j.freeradbiomed.2022.02.028
Sherman, M. H., Yu, R. T., Engle, D. D., Ding, N., Atkins, A. R., Tiriac, H., et al. (2014). Vitamin D receptor-mediated stromal reprogramming suppresses pancreatitis and enhances pancreatic cancer therapy. Cell 159 (1), 80–93. doi:10.1016/j.cell.2014.08.007
Shi, C., Li, H., Yang, Y., and Hou, L. (2015). Anti-inflammatory and immunoregulatory functions of artemisinin and its derivatives. Mediat. Inflamm. 2015, 435713. doi:10.1155/2015/435713
Spanehl, L., Revskij, D., Bannert, K., Ehlers, L., and Jaster, R. (2022). YAP activates pancreatic stellate cells and enhances pancreatic fibrosis. Hepatobiliary Pancreat. Dis. Int. doi:10.1016/j.hbpd.2022.06.004
Tan, P., Wang, A., Chen, H., Du, Y., Qian, B., Shi, H., et al. (2019). SPOP inhibits mice pancreatic stellate cell activation by promoting FADD degradation in cerulein-induced chronic pancreatitis. Exp. Cell Res. 384 (1), 111606. doi:10.1016/j.yexcr.2019.111606
Tsang, S. W., and Bian, Z. X. (2015). Anti-fibrotic and anti-tumorigenic effects of rhein, a natural anthraquinone derivative, in mammalian stellate and carcinoma cells. Phytother. Res. 29 (3), 407–414. doi:10.1002/ptr.5266
Tsang, S. W., Zhang, H., Lin, C., Xiao, H., Wong, M., Shang, H., et al. (2013). Rhein, a natural anthraquinone derivative, attenuates the activation of pancreatic stellate cells and ameliorates pancreatic fibrosis in mice with experimental chronic pancreatitis. PLoS One 8 (12), e82201. doi:10.1371/journal.pone.0082201
Vancura, A., Bu, P., Bhagwat, M., Zeng, J., and Vancurova, I. (2018). Metformin as an anticancer agent. Trends Pharmacol. Sci. 39 (10), 867–878. doi:10.1016/j.tips.2018.07.006
Wallbaum, P., Rohde, S., Ehlers, L., Lange, F., Hohn, A., Bergner, C., et al. (2018). Antifibrogenic effects of vitamin D derivatives on mouse pancreatic stellate cells. World J. Gastroenterol. 24 (2), 170–178. doi:10.3748/wjg.v24.i2.170
Wang, K., Wang, J., Song, M., Wang, H., Xia, N., and Zhang, Y. (2020). Angelica sinensis polysaccharide attenuates CCl4-induced liver fibrosis via the IL-22/STAT3 pathway. Int. J. Biol. Macromol. 162, 273–283. doi:10.1016/j.ijbiomac.2020.06.166
Wang, K., Yang, X., Wu, Z., Wang, H., Li, Q., Mei, H., et al. (2020). Dendrobium officinale polysaccharide protected CCl4-induced liver fibrosis through intestinal homeostasis and the LPS-TLR4-NF-κb signaling pathway. Front. Pharmacol. 11, 240. doi:10.3389/fphar.2020.00240
Watari, N., Hotta, Y., and Mabuchi, Y. (1982). Morphological studies on a vitamin A-storing cell and its complex with macrophage observed in mouse pancreatic tissues following excess vitamin A administration. Okajimas Folia Anat. Jpn. 58 (4-6), 837–858. doi:10.2535/ofaj1936.58.4-6_837
Wei, X., Yi, X., Zhu, X. H., and Jiang, D. S. (2020). Posttranslational modifications in ferroptosis. Oxid. Med. Cell. Longev. 2020, 8832043. doi:10.1155/2020/8832043
White, N. J., Hien, T. T., and Nosten, F. H. (2015). A brief history of qinghaosu. Trends Parasitol. 31 (12), 607–610. doi:10.1016/j.pt.2015.10.010
Wu, N., Xu, X. F., Xin, J. Q., Fan, J. W., Wei, Y. Y., Peng, Q. X., et al. (2021). The effects of nuclear factor-kappa B in pancreatic stellate cells on inflammation and fibrosis of chronic pancreatitis. J. Cell. Mol. Med. 25 (4), 2213–2227. doi:10.1111/jcmm.16213
Wu, P., Nielsen, T. E., and Clausen, M. H. (2015). FDA-approved small-molecule kinase inhibitors. Trends Pharmacol. Sci. 36 (7), 422–439. doi:10.1016/j.tips.2015.04.005
Wu, Y., Zhang, C., Jiang, K., Werner, J., Bazhin, A. V., and D'Haese, J. G. (2020). The role of stellate cells in pancreatic ductal adenocarcinoma: Targeting perspectives. Front. Oncol. 10, 621937. doi:10.3389/fonc.2020.621937
Xian, Z., Tian, J., Wang, L., Zhang, Y., Han, J., Deng, N., et al. (2020). Effects of rhein on bile acid homeostasis in rats. Biomed. Res. Int. 2020, 8827955. doi:10.1155/2020/8827955
Xiao, W., Jiang, W., Shen, J., Yin, G., Fan, Y., Wu, D., et al. (2015). Retinoic acid ameliorates pancreatic fibrosis and inhibits the activation of pancreatic stellate cells in mice with experimental chronic pancreatitis via suppressing the wnt/β-catenin signaling pathway. PLoS One 10 (11), e0141462. doi:10.1371/journal.pone.0141462
Yan, B., Cheng, L., Jiang, Z., Chen, K., Zhou, C., Sun, L., et al. (2018). Resveratrol inhibits ROS-promoted activation and glycolysis of pancreatic stellate cells via suppression of miR-21. Oxid. Med. Cell Longev. 2018, 1346958. doi:10.1155/2018/1346958
Yang, X., Chen, J., Wang, J., Ma, S., Feng, W., Wu, Z., et al. (2022). Very-low-density lipoprotein receptor-enhanced lipid metabolism in pancreatic stellate cells promotes pancreatic fibrosis. Immunity 55 (7), 1185–1199 e8. doi:10.1016/j.immuni.2022.06.001
Zeng, X. P., Wang, L. J., Guo, H. L., He, L., Bi, Y. W., Xu, Z. L., et al. (2019). Corrigendum to "Dasatinib ameliorates chronic pancreatitis induced by caerulein via anti-fibrotic and anti-inflammatory mechanism" [Pharmacol Res. 147 (2019) 104357]. Pharmacol. Res. 147, 104788. doi:10.1016/j.phrs.2020.104788
Zhang, Z., Wang, X., Wang, Z., Cao, Y., Wei, Z., Zhang, Z., et al. (2021). Dihydroartemisinin alleviates hepatic fibrosis through inducing ferroptosis in hepatic stellate cells. Biofactors 47 (5), 801–818. doi:10.1002/biof.1764
Zheng, M., Li, H., Sun, L., Brigstock, D. R., and Gao, R. (2021). Interleukin-6 participates in human pancreatic stellate cell activation and collagen I production via TGF-β1/Smad pathway. Cytokine 143, 155536. doi:10.1016/j.cyto.2021.155536
Zhou, J. H., Cheng, H. Y., Yu, Z. Q., He, D. W., Pan, Z., and Yang, D. T. (2011). Resveratrol induces apoptosis in pancreatic cancer cells. Chin. Med. J. 124 (11), 1695–1699.
Keywords: CP, PSCs, natural compounds, pancreatic fibrosis, anti-fibrotic drug
Citation: Wu Y, Zhang C, Guo M, Hu W, Qiu Y, Li M, Xu D, Wu P, Sun J, Shi R, Zhang Z and Jiang K (2022) Targeting pancreatic stellate cells in chronic pancreatitis: Focus on therapeutic drugs and natural compounds. Front. Pharmacol. 13:1042651. doi: 10.3389/fphar.2022.1042651
Received: 12 September 2022; Accepted: 10 October 2022;
Published: 19 October 2022.
Edited by:
Yan Huang, Anhui Medical University, ChinaReviewed by:
Zhaoguo Liu, Nantong University, ChinaFeixiang Liu, First Affiliated Hospital of Henan University of Traditional Chinese Medicine, China
Copyright © 2022 Wu, Zhang, Guo, Hu, Qiu, Li, Xu, Wu, Sun, Shi, Zhang and Jiang. This is an open-access article distributed under the terms of the Creative Commons Attribution License (CC BY). The use, distribution or reproduction in other forums is permitted, provided the original author(s) and the copyright owner(s) are credited and that the original publication in this journal is cited, in accordance with accepted academic practice. No use, distribution or reproduction is permitted which does not comply with these terms.
*Correspondence: Zili Zhang, zilizhang@njucm.edu.cn; Kuirong Jiang, jiangkuirong@njmu.edu.cn
†These authors have contributed equally to this work