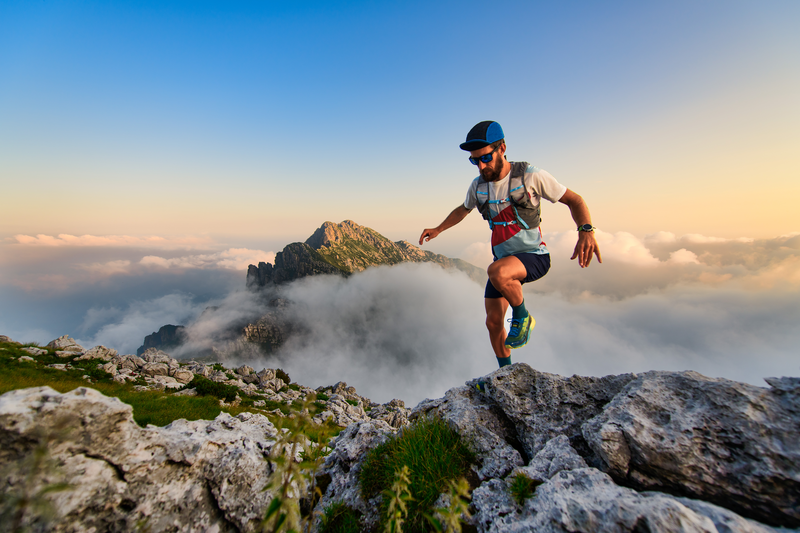
95% of researchers rate our articles as excellent or good
Learn more about the work of our research integrity team to safeguard the quality of each article we publish.
Find out more
ORIGINAL RESEARCH article
Front. Pharmacol. , 04 January 2023
Sec. Experimental Pharmacology and Drug Discovery
Volume 13 - 2022 | https://doi.org/10.3389/fphar.2022.1038812
This article is part of the Research Topic Brief Research Reports in Experimental Pharmacology and Drug Discovery: 2022 View all 7 articles
Purpose: Fermented camel milk from Xinjiang is rich in probiotics and has immunomodulatory effects as an important source of bioactive peptides. However, it is not clear whether it is the probiotic or the bioactive peptide that acts. The present study aimed to extract and identify bioactive peptides from fermented camel milk in Xinjiang and investigate their immunomodulatory effects and mechanisms based on network pharmacology and molecular docking.
Methods: Four probiotic bacteria were used to ferment the fresh camel milk and the bioactive peptides were extracted and isolated by ultrafiltration and column chromatography. Network pharmacology predicts targets and pathways of action. GeneCards and OMIM-GENE-MAP database were used in order to search disease target genes and screen common target genes. Then we used STRING web to construct a protein-protein interaction (PPI) interaction network of the common target protein. The key targets were analyzed by GO (Gene Ontology) and KEGG (Kyoto Encyclopedia of Genes and Genomes) analysis through the David database. The "drug (bioactive peptide)-disease-targets-pathway" network was established and molecular docking was used for prediction.
Results: Two fractions were obtained by UV spectrophotometer; whey acidic protein, α-lactalbumin, and peptidoglycan recognition protein 1 were the main protein-like components of Xinjiang fermented camel milk-derived bioactive peptides. The repeat sequence of peptidoglycan recognition protein 1 was selected and then seven bioactive peptides were obtained. Bioactive peptides had 222 gene targets, anti-inflammatory diseases had 2598 gene targets, and immune regulation had 866 gene targets, the intersection of which was 66 in common gene targets. Gene ontology and KEGG analysis reveals that bioactive peptides mainly play a vital role in the signaling pathways of lipid and atherosclerosis, pathways in cancer. The molecular docking results showed that the seven bioactive peptides bound well to the top four scoring proteins.
Conclusion: The immunomodulatory and anti-inflammatory effects and mechanisms of Xinjiang fermented camel milk-derived bioactive peptides were initially investigated by network pharmacology and molecular docking, providing a scientific basis for future studies.
The immune system is a critical defense system for the organism (Poles et al., 2021). Immunomodulatory abnormalities often lead to autoimmune tolerance disorders and autoimmune diseases, and understanding their underlying mechanisms could provide potential new targets for more effective therapies (Giat et al., 2017). It is estimated that 7.6%–9.4% of the world population has various types of autoimmune diseases (Cooper et al., 2009), which makes it the third most common chronic disease after cardiovascular diseases and cancer (Zhai and Yang, 2022). In recent years, the incidence of immunological diseases has been on the rise (Aaron et al., 2015), seriously affecting the quality of life of the workforce and patients, even threatening their lives.
Small molecule drugs and targeted biologic are the mainstays of treatment (Cheng and Li, 2021). The increasing number of patients with autoimmune diseases calls for new therapeutic approaches. These immune checkpoint molecules, such as CTLA-4 and PD-1, have been shown to be involved in the development of autoimmune diseases and could be targeted for treatment (Huang et al., 2019). Changes in immune function are also associated with intestinal flora, and in particular, intestinal immunity is linked to the development of many chronic inflammatory diseases, such as diabetes (Scheithauer et al., 2020), cardiovascular disease (Zhou et al., 2020) and tumors (Qiu et al., 2021). Probiotics and bioactive peptides also play an adjuvant therapeutic role in their treatment process (Wong et al., 2022; Wang et al., 2020a; Wang et al., 2020a). Probiotics have also been reported as an effective preventive and therapeutic strategy for the treatment of allergic/autoimmune diseases (Isolauri et al., 2012; Liu et al., 2018). At the same time, bioactive peptides are receiving increasing attention due to their involvement in various biological processes (Chakrabarti et al., 2018). Antimicrobial peptides, such as Cathelicidins, not only modulate inflammatory responses and B cell function but also attenuate autoimmune diseases (Sun et al., 2016; Sun et al., 2015). The production of bioactive peptides is caused by protein degradation, their lower molecular weight, easier absorption by the body and various biological properties such as hypotensive, antioxidant, antibacterial, anti-inflammatory, chelating, and other effects (Jakubczyk et al., 2020). Milk protein is considered an important source of bioactive natural peptides (Vargas-Bello-Pérez et al., 2019). Conventional fermented foods containing lactic acid bacteria have been shown to have beneficial effects on human health, some of which are associated with protein-derived products including bacteriocins and bioactive peptides (Venegas-Ortega et al., 2019).
Scholars have found that the prevalence of metabolic syndrome in the Kazakh population is lower than that of other ethnic groups in Xinjiang, China, which is closely related to their long-term daily habit of using contain bioactive peptides products such as traditional fermented cheese whey and fermented camel milk (Wang et al., 2010; Li et al., 2012; Yan et al., 2015). In our previous work, we found that traditional fermented cheese whey has anti-atherosclerotic and anti-inflammatory roles in rats (Nabi et al., 2007) and anti-atherosclerotic effects in rabbits (Nabi et al., 2016), and found that probiotic fermented camel milk has significant hypoglycemic potential in rats models of type 2 diabetes mellitus (Manaer et al., 2015). Also we found that the composite probiotics from fermented camel milk has anti-diabetic effect in db/db mice (Wang et al., 2020b). Fourteen probiotics were isolated from fermented camel milk in Xinjiang (Ma et al., 2010; Liu et al., 2017), and four of them were found to alleviate the immune deficiency in mice caused by cyclophosphamide (Yusufu et al., 2022) and to exert anti-tumor effects by adjusting the CD4+/CD8+ cell ratio and increasing the secretion of Th1-type cytokines (Xiao et al., 2017). We also found that bioactive peptides extracted from fermented camel milk from Xinjiang had anti-inflammatory and immunomodulatory functions (Zhang et al., 2015) and promoted the expression of IFN-γ (Wulazibieke et al., 2014).
In this study, we fermented fresh camel milk with four probiotic bacteria (Issatchenkia orientali, et al.) (Yusufu et al., 2022; Xiao et al., 2017). Bioactive peptides were extracted and isolated from the fermented camel milk and identified by HPLC-MS/MS. Through preliminary studies, it was shown that this bioactive peptide has immunomodulatory and anti-inflammatory effects, but the targets and pathways of action are not clear. The potential targets and signaling pathways of the bioactive peptide with immunomodulatory and anti-inflammatory effects were predicted by using the network pharmacology method combined with molecular docking, which provided a theoretical basis for further large data integration experiments. The procedure for this study is shown in Figure 1.
The fresh camel milk was centrifuged at 3,000 r/min for 30 min and repeated three times, the upper-fat layer was discarded and pasteurized. The fermented camel milk was centrifuged at 3,000 r/min for 15 min repeated three times, and collected whey. The whey was ultrafiltered using a nanofiltration membrane with a molecular retention value of 30 ku, and the filtrate was concentrated and freeze-dried for 24 h to obtain the crude lyophilized powder. The crude lyophilized powder was separated by Sephadex-50 gel column chromatography, and one tube was collected every 3 ml. The absorption value was detected at 214 nm (Baheti et al., 2014) using a UV spectrophotometer, and the eluates with the same peak time were combined and freeze-dried for 24 h. The bioactive peptides obtained were identified by the HPLC-MS\MS method, which was performed by Beijing Protein Innovation Co., Ltd.
The 2D structures of the identified bioactive peptides were drawn using MarvinSketch 22.11 software (Li et al., 2022) and the obtained bioactive peptides were subjected to target prediction through the Super PRED (https://prediction.charite.de/) database. Then the GeneCards (https://www.genecards.org/) and OMIM-GENE-MAP (https://omim.org/search/advanced/geneMap) databases were then screened using “immunomodulatory”" and “anti-inflammatory” as keywords to obtain disease-related targets (set Score > 10 in GeneCards for both keywords). Gene targets at the intersection of bioactive peptide immunomodulation and anti-inflammatory diseases were obtained through the online platform of Venn diagram mapping (http://bioinformatics.psb.ugent.be/webtools/Venn/).
The intersecting gene targets were entered into the String web (https://www.string-db.org), set to “"homo sapiens” species, and the network relationship data of the target protein interactions were obtained, and the PPI was mapped using Cytoscape 3.9.0 software. Network diagrams were created using Cytoscape 3.9.0 and key targets were screened using the Network Analyzer tool (Chen et al., 2022).
GO and KEGG enrichment analyses of key targets were performed through the David database (https://david.ncifcrf.gov/), set at p < .05, to obtain the functions and pathways of key targets.
The results of the intersection of bioactive peptide and disease key targets and KEGG pathway enrichment analysis were collected and imported into Cytoscape 3.9.0 software to create a “drug(bioactive peptide)-disease-target-pathway” network.
The experiments used molecular docking to validate interactions between bioactive peptides and predicted targets. The 2D structures of the bioactive peptides were converted to 3D structures using MarvinSketch 22.11. The 3D structures of key target proteins were obtained using the PDB (protein data bank) database (http:/www.rcsb.org/pdb). Then the operations such as removal of water molecules, hydrogen addition, and setting the small molecule ligand flexible bonds to rotatable were performed by AutoDockTool 1.5.7 (Zhu et al., 2021). Finally, the docking process was performed using AutoDock Vina 1.1.2 and molecular binding energies were calculated.
Based on the experimental results, the molecular dynamics simulation of bioactive peptide 2/STAT3 and bioactive peptide 5/CASP3 complex was performed using Desmond software (Liu et al., 2021). A reasonable number of Na+ and Cl− ions were added to keep the system neutral, and the final generation phase of 100 ns was achieved (Chen et al., 2020).
Fresh camel milk was fermented with a mixture of four probiotics to separate the whey, and the crude product with a molecular weight of less than 30 ku was obtained by ultrafiltration. The crude product was then separated on a Sephadex G-50 gel column and detected at 214 nm with a UV spectrophotometer to obtain two fractions (Figure 2).
FIGURE 2. The fractions obtained by gel chromatography. Column chromatography: one tube per 3 ml was collected, 60 tubes in total.
The two bioactive peptides were identified by HPLC-MS/MS and 30 proteins were identified, of which whey acidic protein, α-lactalbumin and peptidoglycan recognition protein 1 were the main protein-like components. It was shown that peptidoglycan recognition protein 1 has various biological activities such as bactericidal, antitumor, and immunomodulatory activities (Nylund et al., 2018). The sequences duplicated with peptidoglycan recognition protein 1 were selected, and a total of seven bioactive peptides were obtained after de-duplication (Table 1).
The seven bioactive peptides were retrieved from the Super PRED database and 222 potential targets were identified. The bioactive peptides and potential targets were imported into Cytoscape 3.9.0 to construct the “bioactive peptide-target” network (Figure 3). The GeneCards database and OMIM-GENE-MAP database were used to screen potential disease targets, and duplicate values were combined and removed, resulting in a total of 2,598 anti-inflammatory gene targets and 866 Immune regulation gene targets. The crossover of drug targets and disease targets was performed using the online platform of Venn diagram mapping, and a total of 66 crossover targets were obtained (Figure 4).
FIGURE 3. network of “drug (bioactive peptide)-target.” Red nodes drug; green nodes represent target and the line segments represent the relationship between the two.
The 66 gene targets obtained for the bioactive peptide intersecting with the disease were entered into the String database and mapped using Cytoscape 3.9.0 (Figure 5). Topological properties were analyzed by the Network Analyzer tool and those at the median degree and above were selected as key targets, as well as a total of 18 key targets were obtained and further identified by the UniProt (https://www.uniprot.org/) database (Table 2).
FIGURE 5. PPI network. The color and size of the nodes represent the size of the degree value, with darker red and larger nodes representing larger degree values; the inner circle represents degree values above the median.
The GO analysis included three components: biological process (BP), cellular component (CC) and molecular function (MF). A total of 144 biological processes or pathways were obtained by GO enrichment analysis, set at p < .05, of which 99 were associated with BP, 18 with CC and 27 with MF. BP mainly involves biological processes such as response to estradiol, apoptotic process, and positive regulation of nitric oxide biosynthetic process. CC mainly involves cytoplasm, macromolecular complex, cytosol and other cellular components. MF is mainly involved in identical protein binding, protein heterodimerization activity, scaffold protein binding and other functions. The top 10 GO entries were selected according to the order of p-value from smallest to largest and plotted in a bar chart (Figure 6).
A total of 117 pathways were obtained by KEGG analysis (p < .05) and the main pathways are Lipid and atherosclerosis, Pathways in cancer, Kaposi sarcoma-associated herpesvirus infection, etc. The top 20 pathways were filtered from smallest to largest p-value and plotted in a bar chart (Figure 7).
The results of the key targets and KEGG pathway enrichment analysis of the intersection of bioactive peptides and diseases (top 20) were imported into Cytoscape 3.9.0 and constructed the “drug (bioactive peptide)-disease-target-pathway” network diagram (Figure 8).
FIGURE 8. Network of “drug(bioactive peptide)-disease-target-pathway”. Red nodes represent diseases; yellow nodes represent bioactive peptides; green nodes represent key targets; blue nodes represent pathways and the line segments represent the relationship between the two.
The top four proteins STAT3, HSP90AA1, CASP3, and PIK3CA were selected as the receptors and bioactive peptides for molecular docking using Autodock vina 1.1.2 and visualized using Pymol 2.4.0 (Table3; Figure 9). Generally, affinity < −5.5 kcal/mol is considered to have better binding activity. The results showed that all molecules had affinity < −5.5 kcal/mol to the target, indicating that the bioactive peptide had better binding activity to the key target.
FIGURE 9. Diagram of the molecular docking pattern. (A) 1-STAT3; (B) 2-STAT3; (C) 4-STAT3; (D) 5-STAT3; (E) 6-STAT3; (F) 7-STAT3; (G) 1-HSP90AA1; (H) 3-HSP90AA1; (I) 4-HSP90AA1; (J) 5-HSP90AA1; (K) 6- HSP90AA1; (L) 7-HSP90AA1; (M) 1-CASP3; (N) 5-CASP3; (O) 6-CASP3; (P) 1-PIK3CA; (Q) 5-PIK3CA. Blue represents the protein; green represents the bioactive peptide binding site to the protein; red represents the active peptide.
The molecular docking results show that almost all of the seven bioactive peptides interact with STAT3, partly with CASP3, and the bioactive peptide 2/STAT3 and bioactive peptide 5/CASP3 have the best binding energy. Thus bioactive peptide 2/STAT3 and bioactive peptide 5/CASP3 are selected to perform molecular dynamics simulation.
The Root Mean Square Deviation (RMSD) can reflect the composite motion process, with large or small fluctuations representing strong or stable motion (Cao et al., 2022). The results of molecular dynamics simulation (Figure 10A) show that bioactive peptides and proteins fluctuate greatly in the early stage, move vigorously, and small fluctuations tend to stabilize in the later stage. Bioactive peptides fluctuate more than proteins and may be caused by the excessive flexibility of bioactive peptides. The root mean square fluctuation (RMSF) values can reflect the flexibility size of the protein (Chang et al., 2022). As shown in Figure 10B, the RMSFs of the two complexes are relatively low, and the RMSFs of most of the protein sequences are below 2 Å, indicating that the protein system is still relatively stable. Hydrogen Bonds (H-bonds) play a significant role in ligand binding (Cao et al., 2022). The results (Figure 11) showed that the number of hydrogen bonds in the bioactive peptide 2/STAT3 and bioactive peptide 5/CASP3 systems was more in the molecule dynamics stage.
FIGURE 10. Root mean square deviation (RMSD) (A) and root Mean Square Fluctuation (RMSF) (B) during molecular dynamics simulations.
FIGURE 11. Interaction of proteins and ligands during molecule dynamics. (A) bioactive peptide 2/STAT3; (B) bioactive peptide 5/CASP3. The stacked bar charts are normalized over the course of the trajectory: for example, a value of .7 suggests that 70% of the simulation time the specific interaction is maintained.
Bioactive peptides are considered to be a preferred alternative to drugs, being present mainly in natural foods and with low toxic effects (Shivanna and Nataraj, 2020). Moreover, dairy-derived bioactive peptides can be released during gastrointestinal digestion, food processing, or through enzymatic and bacterial fermentation and are thought to have various beneficial effects such as hypolipidemic, hypotensive, immunomodulatory, anti-inflammatory and antithrombotic effects (Marcone et al., 2017). Waqas N. Baba et al. (2021) showed that bioactive peptides from camel whey hydrolysate can assist in the treatment of hypercholesterolemia by inhibiting pancreatic lipase and cholesterol esterase. One of them, fermented camel milk from Xinjiang, is commonly consumed by Kazakhs, Mongolians, and other ethnic groups and contains unique microflora (Ghosh et al., 2019). Fermented camel milk is rich in probiotics (Rabab M.H.A. Madany, 2017), and many of probiotics’ health benefits and disease alleviation abilities are achieved through immune induction (Varsha et al., 2021). Research has shown that probiotics can enhance the innate immunity of the organism by upregulating the number of immunoglobulins and antimicrobial proteins in the body and increasing the activity of phagocytes and natural killer cells after normal host cell colonization; they can enhance the acquired immunity of the organism by increasing the function of antigen-presenting B cells and T lymphocytes (Di Pierro et al., 2014). In this work, we used network pharmacology and molecular docking approach to elucidate the molecular biological mechanisms of immune modulation by bioactive peptides.
In this study, the KEGG study shows lipid and atherosclerosis, as well as pathways in cancer, are the two most enriched pathways. Atherosclerosis is a chronic inflammatory disease that is prevented and treated through the interplay of lipid metabolism and inflammatory responses (Liu et al., 2014). The development of cancer is usually accompanied by inflammation and cancer development and metastasis can be inhibited by treating or controlling inflammation and blocking the production of related factors (e.g., TNF-α, IL-6, IL-17, etc.) (Landskron et al., 2014). The STAT3 signaling pathway is a major intrinsic pathway in cancer inflammation as it is frequently activated in malignant cells and can induce a large number of inflammations (Johnson et al., 2018). STAT3 plays a dual role in tumor inflammation and immunity by promoting the pro-tumor inflammatory pathway of nuclear factor-κb (NF-κb) and interleukin-6 (IL-6)-GP130-Janus kinase (JAK) and by inhibiting STAT1 and NF-κb-mediated Th1 antitumor immune responses (Yu et al., 2009; Gharibi et al., 2020). It has been shown that in the immune system, receptors for most cytokines expressed by lymphocytes such as IL-6, IL-10 and interferons (IFNs) can also activate STAT3 (Deenick et al., 2018). Heat shock proteins (HSP) have different effects on the immune system and they can stimulate or improve the immune response (Androvitsanea et al., 2021). Newly formed HSP90AA1 can be secreted into the extracellular environment and the nucleus, stimulating the formation of immune memory and participating in tumor formation (Guo et al., 2022). The apoptosis-associated gene CASP3 causes nuclear DNA breaks during apoptosis and CASP3 is also involved in MAPK signaling pathways and inflammatory responses (Ma et al., 2021). PIK3CA regulates cell growth and apoptosis by participating in the PI3K/AKT signaling pathway (Shi et al., 2021). GO and KEGG analyses have shown that the apoptotic process plays an important role. Aberrant apoptosis leads to excessive cell clearance or prolonged survival and is therefore involved in the pathogenesis of many immune diseases through the regulation of aberrant apoptosis (Sankari et al., 2015). According to the aforementioned findings, the bioactive peptides’ immunomodulatory and anti-inflammatory effects can be attributed to a variety of biological mechanisms and signaling pathways. The molecular docking results show that the seven bioactive peptides bind well to the first four key targets, demonstrating the reliability of the network pharmacology predictions. And the binding of bioactive peptides to proteins was also found to be stable after molecular dynamics simulations.
This study explored the immunomodulatory and anti-inflammatory mechanisms of bioactive peptides extracted from fermented camel milk in Xinjiang based on network pharmacology and molecular docking. By speculating on the targets and pathways of action of bioactive peptides extracted from fermented camel milk in Xinjiang, this paper provides theoretical support for future experiments to be conducted. It also provides some references for the future development of fermented camel milk in Xinjiang.
The datasets presented in this study can be found in online repositories. The names of the repository/repositories and accession number(s) can be found in the article/Supplementary Material.
YW performed the experiments and wrote the first draft of the manuscript. ZL completed part of the experiment. TM revised the paper. XN and WZ designed, conceived, guided and supported experiments, assessed the results and wrote the manuscript. FS and DJ analysed the data. All authors read and approved the final manuscript.
This project was supported by the fund from Key Projects of Natural Science Foundation of Xinjiang Uygur Autonomous Region in China (title of the key project: Mechanistic study of camel milk-derived bioactive peptides based on Treg cells to improve the microenvironment of colon cancer).
The authors declare that the research was conducted in the absence of any commercial or financial relationships that could be construed as a potential conflict of interest.
All claims expressed in this article are solely those of the authors and do not necessarily represent those of their affiliated organizations, or those of the publisher, the editors and the reviewers. Any product that may be evaluated in this article, or claim that may be made by its manufacturer, is not guaranteed or endorsed by the publisher.
Aaron, L., Patricia, J., and Torsten, M. (2015). The World Incidence and Prevalence of Autoimmune Diseases is Increasing. International Journal of Celiac Disease 3 (4), 151–155. doi:10.12691/ijcd-3-4-8
Androvitsanea, A., Stylianou, K., Drosataki, E., and Petrakis, I. (2021). The Pathophysiological Role of Heat Shock Response in Autoimmunity: A Literature Review. Cells 10 (10), 2626. doi:10.3390/cells10102626
Baba, W. N., Mudgil, P., Baby, B., Vijayan, R., Gan, C. Y., and Maqsood, S. (2021). New insights into the cholesterol esterase- and lipase-inhibiting potential of bioactive peptides from camel whey hydrolysates: Identification, characterization, and molecular interaction. J. Dairy Sci. 104 (7), 7393–7405. doi:10.3168/jds.2020-19868
Baheti, W., Liu, H., and Xunhua, N. (2014). Evaluation of immunomodulatory effects of bioactive peptides derived from fermented camel milk on splenocytes proliferation. China Dairy Industry 42 (04), 15–17.
Cao, J., Li, L., Xiong, L., Wang, C., Chen, Y., and Zhang, X. (2022). Research on the mechanism of berberine in the treatment of COVID-19 pneumonia pulmonary fibrosis using network pharmacology and molecular docking. Phytomed Plus 2 (2), 100252. doi:10.1016/j.phyplu.2022.100252
Chakrabarti, S., Guha, S., and Majumder, K. (2018). Food-Derived Bioactive Peptides in Human Health: Challenges and Opportunities. Nutrients 10 (11), 1738. doi:10.3390/nu10111738
Chang, J., Feng, T., Zhuang, H., Song, S., Sun, M., Yao, L., et al. (2022). Taste mechanism of kokumi peptides from yeast extracts revealed by molecular docking and molecular dynamics simulation. Journal of Future Foods 2 (4), 358–364. doi:10.1016/j.jfutfo.2022.08.007
Chen, Y., Zheng, Y., Fong, P., Mao, S., and Wang, Q. (2020). The application of the MM/GBSA method in the binding pose prediction of FGFR inhibitors. Phys. Chem. Chem. Phys. 22, 9656–9663. doi:10.1039/d0cp00831a
Chen, Z., Wang, X., Liu, H., Yang, C., Sun, L., Xie, C., et al. (2022). Research on Molecular Mechanism of Shenqi Compound in Treating Diabetic Kidney Disease Based on Network Pharmacology and Molecular Docking. Journal of Liaoning University of Traditional Chinese Medicine (09), 61–66. doi:10.13194/j.issn.1673-842x.2022.09.014
Cheng, X. Y., and Li, J. N. (2021). Biological agents and small molecules in the treatment of inflammatory bowel disease. Chinese Journal of Internal Medicine 60 (04), 376–379. doi:10.3760/cma.j.cn112138-20201229-01058
Cooper, G. S., Bynum, M. L., and Somers, E. C. (2009). Recent insights in the epidemiology of autoimmune diseases: improved prevalence estimates and understanding of clustering of diseases. J. Autoimmun. 33 (3-4), 197–207. doi:10.1016/j.jaut.2009.09.008
Deenick, E. K., Pelham, S. J., Kane, A., and Ma, C. S. (2018). Signal Transducer and Activator of Transcription 3 Control of Human T and B Cell Responses. Front. Immunol. 9, 168. doi:10.3389/fimmu.2018.00168
Di Pierro, F., Colombo, M., Zanvit, A., Risso, P., and Rottoli, A. S. (2014). Use of Streptococcus salivarius K12 in the prevention of streptococcal and viral pharyngotonsillitis in children. Drug Healthc. Patient. Saf. 6, 15–20. doi:10.2147/DHPS.S59665
Gharibi, T., Babaloo, Z., Hosseini, A., Abdollahpour-Alitappeh, M., Hashemi, V., Marofi, F., et al. (2020). Targeting STAT3 in cancer and autoimmune diseases. Eur. J. Pharmacol. 878, 173107. doi:10.1016/j.ejphar.2020.173107
Ghosh, T., Beniwal, A., Semwal, A., and Navani, N. K. (2019). Mechanistic Insights Into Probiotic Properties of Lactic Acid Bacteria Associated With Ethnic Fermented Dairy Products. Front. Microbiol. 10, 502. doi:10.3389/fmicb.2019.00502
Giat, E., Ehrenfeld, M., and Shoenfeld, Y. (2017). Cancer and autoimmune diseases. Autoimmun. Rev. 16 (10), 1049–1057. doi:10.1016/j.autrev.2017.07.022
Guo, W. L., Xu, Y. Q., Jin, X., and Shi, B. L. (2022). Moderating Role of Heat Shock Protein Under Inflammatory Response Oxidative Stress Caused by Cold Stress. Acta Veterinaria et Zootechnica Sinica 53 (06), 1668–1677.
Huang, C., Zhu, H. X., Yao, Y., Bian, Z. H., Zheng, Y. J., Li, L., et al. (2019). Immune checkpoint molecules. Possible future therapeutic implications in autoimmune diseases. J. Autoimmun. 104, 102333. doi:10.1016/j.jaut.2019.102333
Isolauri, E., Rautava, S., and Salminen, S. (2012). Probiotics in the development and treatment of allergic disease. Gastroenterol. Clin. North Am. 41 (4), 747–62. doi:10.1016/j.gtc.2012.08.007
Jakubczyk, A., Karaś, M., Rybczyńska-Tkaczyk, K., Zielińska, E., and Zieliński, D. (2020). Current Trends of Bioactive Peptides-New Sources and Therapeutic Effect. Foods 9 (7), 846. doi:10.3390/foods9070846
Johnson, D. E., O'Keefe, R. A., and Grandis, J. R. (2018). Targeting the IL-6/JAK/STAT3 signalling axis in cancer. Nat. Rev. Clin. Oncol. 15 (4), 234–248. doi:10.1038/nrclinonc.2018.8
Landskron, G., De la Fuente, M., Thuwajit, P., Thuwajit, C., and Hermoso, M. A. (2014). Chronic inflammation and cytokines in the tumor microenvironment. J. Immunol. Res. 2014, 149185. doi:10.1155/2014/149185
Li, N., Wang, H., Yan, Z., Yao, X., Hong, J., and Zhou, L. (2012). Ethnic disparities in the clustering of risk factors for cardiovascular disease among the Kazakh, Uygur, Mongolian and Han populations of Xinjiang: a cross-sectional study. BMC Public Health 12, 499. doi:10.1186/1471-2458-12-499
Li, J., Wang, X., Wei, Y., Lin, B., Liu, R., Wu, H., et al. (2022). The prevalence and clinical correlates of medical disorders comorbidities in patients with bipolar disorder. Food and Fermentation Industries 22 (15), 176–184. doi:10.1186/s12888-022-03819-0
Liu, H. L., Sun, Y., Lang, Y. S., and Wang, H. T. (2014). Role of monocyte/macrophage lipid metabolism and inflammation in atherosclerosis. Chinese Journal of Cellular and Molecular Immunology 30 (11), 1224–1227. doi:10.13423/j.cnki.cjcmi.007164
Liu, L., Dilidaxi, D., Wu, Y. C., Wang, J., Xiao, X. J., and Nabi, X. H. (2017). Isolation and antibiotic resistance of lactic acid bacteria in fermented dairy produced in Xinjiang. Chinese Journal of Microecology 29 (07), 745–749+753. doi:10.13381/j.cnki.cjm.201707001
Liu, Y., Alookaran, J. J., and Rhoads, J. M. (2018). Probiotics in Autoimmune and Inflammatory Disorders. Nutrients 10 (10), 1537. doi:10.3390/nu10101537
Liu, H., Zeng, M., He, J., and Ouyang, L. (2021). Identification of small molecule sirtuin-1 inhibitors for treating acute myeloid leukemia based on molecular docking, quantitative structure-activity relationships and molecular dynamics. Acta Pharmaceutica Sinica (02), 545–552. doi:10.16438/j.0513-4870.2020-1461
Ma, C. Y., Nabi, X., and Liu, H. (2010). Isolation and identification of zymocyte from the traditional Kazakh fermentation of cheese. China Dairy Industry 38 (04), 7–9+19.
Ma, X. Y., Zhang, M., Fang, G., Cheng, C. J., Wang, M. K., Han, Y. M., et al. (2021). Ursolic acid reduces hepatocellular apoptosis and alleviates alcohol-induced liver injury via irreversible inhibition of CASP3 in vivo. Acta Pharmacol. Sin. 42 (7), 1101–1110. doi:10.1038/s41401-020-00534-y
Madany, Rabab M.H.A. (2017). Fermented Foods and Enhancement of Immune System. International Journal of Fermented Foods 6 (2).
Manaer, T., Yu, L., Zhang, Y., Xiao, X. J., and Nabi, X. H. (2015). Anti-diabetic effects of shubat in type 2 diabetic rats induced by combination of high-glucose-fat diet and low-dose streptozotocin. J. Ethnopharmacol. 169, 269–74. doi:10.1016/j.jep.2015.04.032
Marcone, S., Belton, O., and Fitzgerald, D. J. (2017). Milk-derived bioactive peptides and their health promoting effects: a potential role in atherosclerosis. Br. J. Clin. Pharmacol. 83 (1), 152–162. doi:10.1111/bcp.13002
Nabi, X. H., Rehemu, N., Luo, L., Wang, X. W., and Kelimu, P. (2007). Effects of traditional fermented cheese whey on experimental atherosclerosis in rats. Chin. J. New Drugs 16, 1776–1779.
Nabi, X H., Ma, C. Y., Manaer, T., Heizati, M., Wulazibieke, B., and Aierken, L. (2016). Anti-atherosclerotic effect of traditional fermented cheese whey in atherosclerotic rabbits and identification of probiotics. BMC Complement. Altern. Med. 16 (1), 309. doi:10.1186/s12906-016-1285-8
Nylund, K. M., Ruokonen, H., Sorsa, T., Heikkinen, A. M., Meurman, J. H., Ortiz, F., et al. (2018). Association of the salivary triggering receptor expressed on myeloid cells/its ligand peptidoglycan recognition protein 1 axis with oral inflammation in kidney disease. J. Periodontol. 89 (1), 117–129. doi:10.1902/jop.2017.170218
Poles, J., Karhu, E., McGill, M., McDaniel, H. R., and Lewis, J. E. (2021). The effects of twenty-four nutrients and phytonutrients on immune system function and inflammation: A narrative review. J. Clin. Transl. Res. 7 (3), 333–376.
Qiu, Q., Lin, Y., Ma, Y., Li, X., Liang, J., Chen, Z., et al. (2021). Exploring the Emerging Role of the Gut Microbiota and Tumor Microenvironment in Cancer Immunotherapy. Front. Immunol. 11, 612202. doi:10.3389/fimmu.2020.612202
Sankari, S. L., Babu, N. A., Rajesh, E., and Kasthuri, M. (2015). Apoptosis in immune-mediated diseases. J. Pharm. Bioallied Sci. 7, S200–2. doi:10.4103/0975-7406.155902
Scheithauer, T. P. M., Rampanelli, E., Nieuwdorp, M., Vallance, B. A., Verchere, C. B., van Raalte, D. H., et al. (2020). Gut Microbiota as a Trigger for Metabolic Inflammation in Obesity and Type 2 Diabetes. Front. Immunol. 11, 571731. doi:10.3389/fimmu.2020.571731
Shi, X., Sun, Y., Zhang, Y., Wang, W., Xu, J., Guan, Y., et al. (2021). MEX3A promotes development and progression of breast cancer through regulation of PIK3CA. Exp. Cell Res. 404 (1), 112580. doi:10.1016/j.yexcr.2021.112580
Shivanna, S. K., and Nataraj, B. H. (2020). Revisiting Therapeutic and Toxicological Fingerprints of Milk-Derived Bioactive Peptides: An Overview. Food Bioscience 38, 100771. doi:10.1016/j.fbio.2020.100771
Sun, J., Furio, L., Mecheri, R., van der Does, A. M., Lundeberg, E., Saveanu, L., et al. (2015). Pancreatic β-Cells Limit Autoimmune Diabetes via an Immunoregulatory Antimicrobial Peptide Expressed under the Influence of the Gut Microbiota. Immunity 43 (2), 304–317. doi:10.1016/j.immuni.2015.07.013
Sun, J., Xu, M., Ortsäter, H., Lundeberg, E., Juntti-Berggren, L., Chen, Y. Q., et al. (2016). Cathelicidins positively regulate pancreatic β-cell functions. FASEB J 30 (2), 884–894. doi:10.1096/fj.15-275826
Vargas-Bello-Pérez, E., Márquez-Hernández, R. I., and Hernández-Castellano, L. E. (2019). Bioactive peptides from milk: animal determinants and their implications in human health. J. Dairy Res. 86 (2), 136–144. doi:10.1017/S0022029919000384
Varsha, K. K., Maheshwari, A. P., and Nampoothiri, K. M. (2021). Accomplishment of probiotics in human health pertaining to immunoregulation and disease control. Clin. Nutr. ESPEN 44, 26–37. doi:10.1016/j.clnesp.2021.06.020
Venegas-Ortega, M. G., Flores-Gallegos, A. C., Martínez-Hernández, J. L., Aguilar, C. N., and Nevárez-Moorillón, G. V. (2019). Production of Bioactive Peptides from Lactic Acid Bacteria: A Sustainable Approach for Healthier Foods. Compr. Rev. Food Sci. Food Saf. 18 (4), 1039–1051. doi:10.1111/1541-4337.12455
Wang, L., Tao, Y., Xie, Z., Ran, X., Zhang, M., Wang, Y., et al. (2010). Prevalence of metabolic syndrome, insulin resistance, impaired fasting blood glucose, and dyslipidemia in Uygur and Kazak populations. J. Clin. Hypertens. (Greenwich) 12 (9), 741–745. doi:10.1111/j.1751-7176.2010.00349.x
Wang, Y., Wu, Y., Sailike, J., Sun, X., Abuduwaili, N., Tuoliuhan, H., et al. (2020a). Fourteen composite probiotics alleviate type 2 diabetes through modulating gut microbiota and modifying M1/M2 phenotype macrophage in db/db mice. Pharmacol. Res. 161, 105150. doi:10.1016/j.phrs.2020.105150
Wang, Y., Dilidaxi, D., Wu, Y., Sailike, J., Sun, X., and Nabi, X. H. (2020b). Composite probiotics alleviate type 2 diabetes by regulating intestinal microbiota and inducing GLP-1 secretion in db/db mice. Biomed. Pharmacother. 125, 109914. doi:10.1016/j.biopha.2020.109914
Wong, C.K., Yusta, B., Koehler, J.A., Baggio, L.L., McLean, B.A., Matthews, D., et al. (2022). Divergent roles for the gut intraepithelial lymphocyte GLP-1R in control of metabolism, microbiota, and T cell-induced inflammation. Cell Metab 34 (22), 151400346–1531.e71. doi:10.1016/j.cmet.2022.08.003
Wulazibieke, B., Liu, H. M., and Nabi, X. H. (2014). Evaluation of immunomodulatory effects of bioactive peptides derived from fermented camel milk on splenocytes proliferation. China Dairy Industry 42 (04), 15–17.
Xiao, X. J., Zhang, Y., and Nabi, X. (2017). Immunoregulatory effects of four probiotics derived from Xinjiang fermented camel milk in mice. Science and Technology of Food Industry 38 (04), 337–341. doi:10.13386/j.issn1002-0306.2017.04.055
Yan, W. L., Li, X. S., Wang, Q., Huang, Y. D., Zhang, W. G., Zhai, X. H., et al. (2015). Overweight, high blood pressure and impaired fasting glucose in Uyghur, Han, and Kazakh Chinese children and adolescents. Ethn. Health 20 (4), 365–375. doi:10.1080/13557858.2014.921894
Yu, H., Pardoll, D., and Jove, R. (2009). STATs in cancer inflammation and immunity: a leading role for STAT3. Nat. Rev. Cancer 9 (11), 798–809. doi:10.1038/nrc2734
Yusufu, M., Nasiroula, A., Shen, F., Wang, Y. X., and Nabi, X. H. (2022). Antitumor Effect of Lactogenic Compound Probiotics on CT-26 Tumor-bearing Mice. China Dairy Industry 50 (02), 11–15+30. doi:10.19827/j.issn1001-2230.2022.02.002
Zhai, X., and Yang, C. (2022). Research advance of anti-CD22 monoclonal antibody in the treatment of autoimmune diseases. International Journal of Biologicals 45 (1), 56–60.
Zhang, Y., Yu, L., Xiao, X. J., Manaer, T., and Nabi, X. (2015). Isolation and identification of immune active peptide from Xinjiang fermented camel milk. China Dairy Industry 43 (11), 12–14.
Zhou, W., Cheng, Y., Zhu, P., Nasser, M. I., Zhang, X., and Zhao, M. (2020). Implication of Gut Microbiota in Cardiovascular Diseases. Oxid. Med. Cell. Longev. 2020, 5394096. doi:10.1155/2020/5394096
Keywords: fermented camel milk from Xinjiang, bioactive peptides, immune regulation, network pharmacology, molecular docking
Citation: Wang Y, Liang Z, Shen F, Zhou W, Manaer T, Jiaerken D and Nabi X (2023) Exploring the immunomodulatory effects and mechanisms of Xinjiang fermented camel milk-derived bioactive peptides based on network pharmacology and molecular docking. Front. Pharmacol. 13:1038812. doi: 10.3389/fphar.2022.1038812
Received: 07 September 2022; Accepted: 16 December 2022;
Published: 04 January 2023.
Edited by:
Naseer A. Kutchy, Children’s National Hospital, United StatesReviewed by:
Waseem Lone, University of Nebraska Medical Center, United StatesCopyright © 2023 Wang, Liang, Shen, Zhou, Manaer, Jiaerken and Nabi. This is an open-access article distributed under the terms of the Creative Commons Attribution License (CC BY). The use, distribution or reproduction in other forums is permitted, provided the original author(s) and the copyright owner(s) are credited and that the original publication in this journal is cited, in accordance with accepted academic practice. No use, distribution or reproduction is permitted which does not comply with these terms.
*Correspondence: Xinhua Nabi, MjQ3NjMyMDI4MEBxcS5jb20=
Disclaimer: All claims expressed in this article are solely those of the authors and do not necessarily represent those of their affiliated organizations, or those of the publisher, the editors and the reviewers. Any product that may be evaluated in this article or claim that may be made by its manufacturer is not guaranteed or endorsed by the publisher.
Research integrity at Frontiers
Learn more about the work of our research integrity team to safeguard the quality of each article we publish.