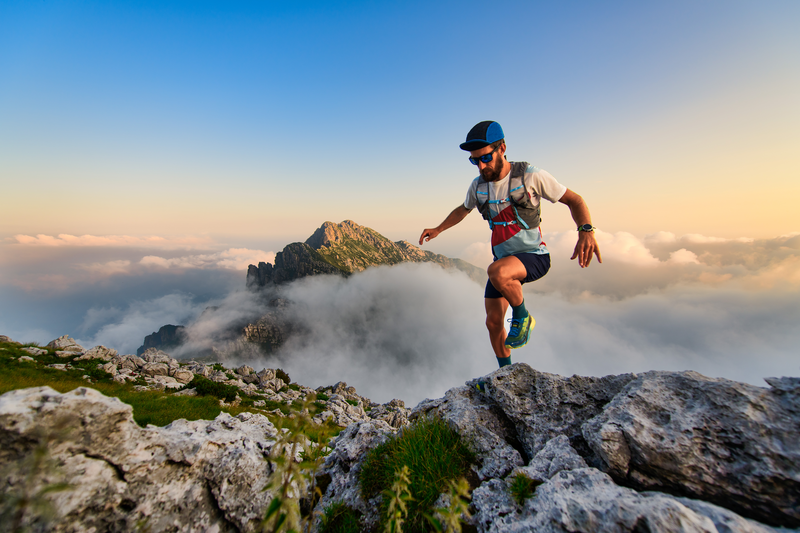
94% of researchers rate our articles as excellent or good
Learn more about the work of our research integrity team to safeguard the quality of each article we publish.
Find out more
MINI REVIEW article
Front. Pharmacol. , 12 October 2022
Sec. Pharmacology of Anti-Cancer Drugs
Volume 13 - 2022 | https://doi.org/10.3389/fphar.2022.1034129
This article is part of the Research Topic The Roles of Immune Cell Homeostasis in Cancer Research and Therapeutic Response View all 12 articles
Metastasis is responsible for 90% of deaths in cancer patients. Most patients diagnosed with metastatic cancer will die within 5 years. PA is good for health and has become an emerging adjuvant therapy for cancer survivors. Regular moderate exercise substantially lowers the incidence and recurrence of several cancers, alleviates cancer-related adverse events, enhances the efficacy of anti-cancer treatments, and improves the quality of life of cancer patients. Revealing the mechanisms of PA inhibiting tumor metastasis could upgrade our understanding of cancer biology and help researchers explore new therapeutic strategies to improve survival in cancer patients. However, it remains poorly understood how physical activity prevents metastasis by modulating tumor behavior. The immune system is involved in each step of tumor metastasis. From invasion to colonization, immune cells interact with tumor cells to secret cytokines and proteases to remodel the tumor microenvironment. Substantial studies demonstrated the ability of physical activity to induce antitumor effects of immune cells. This provides the possibility that physical activity can modulate immune cells behavior to attenuate tumor metastasis. The purpose of this review is to discuss and summarize the critical link between immune function and exercise in metastasis prevention.
Cancer metastasis is an important cause of death in cancer patients, with up to 90% of solid tumor patients dying from metastasis (Steeg, 2016). Most patients diagnosed with metastatic cancer will die within 5 years. The majority of current treatments concentrate on resection or elimination of primary tumor. Moreover, some clinical treatment strategies such as surgery have been demonstrated to aggravate cancer metastasis (Ma et al., 2019). Finding a safe and effective therapy for metastasis remains urgent.
Physical activity (PA) is good for improving physical and mental health. Nowadays, PA has become an important adjuvant therapy for cancer patients and has a remarkable influence on reinforcing conventional cancer therapies (Schmitz et al., 2019). Compared to other cancer treatments, PA has almost no toxic side effects, shows significant safety, and reduces treatment-related adverse events. According to the World Health Organization (WHO), cancer survivors should undertake at least 150–300 min of moderate intensity physical activity, or 75 min of vigorous intensity physical activity per week (Bull et al., 2020). Recently, PA has been shown to reduce the incidence of various cancers and improve the survival of cancer patients. A previous prospective cohort study reported that PA was negatively correlated with the incidence of post-menopausal breast cancer (Bellocco et al., 2016). Besides breast cancer, compelling evidence revealed that PA reduced the risk of additional cancer types, including colon, kidney, endometrial, bladder, esophageal and stomach cancers (Rock et al., 2020). Some prospective observational studies found that PA after cancer diagnosis may decrease cancer mortality, especially in colon (Meyerhardt et al., 2006), breast (Rock et al., 2020) and endometrial (Friedenreich et al., 2020) cancers. In addition, PA has been shown to improve the fatigue and quality of life (QoL) of cancer survivors, relieving anxiety and depression (Schmidt et al., 2015). However, whether PA has beneficial effects on metastasis is more attractive. Revealing the mechanisms of PA inhibiting tumor metastasis could upgrade our understanding of cancer biology and help researchers explore new therapeutic strategies to improve survival in cancer patients. In order to explore the potential mechanism linking PA with metastasis, some preclinical studies established various exercising animal models, especially running and swimming.
The immune system can effectively prevent the occurrence, development and metastasis of primary tumors through immune surveillance. Immune cells can recognize tumor-specific antigens and destroy cancer cells. Recently, some studies suggested that the modulation of the immune system through PA can significantly affect the exercise-dependent prevention of tumor metastasis (Lucia and Ramírez, 2016; Febbraio, 2017). Therefore, the aim of this review was to discuss and summarize recent findings that highlight the critical link between immune function and exercise in metastasis prevention.
Tumor metastasis is a tangled and complicated process that can be categorized into five stages: invasion, intravasation, circulation, extravasation, and colonization. The cells were isolated from the primary tumor and acquired an invasive mesenchymal phenotype. In turn, invasive tumor cells infiltrate the blood vessels, a process closely related to vascular permeability and the interaction between tumor cells and endothelial cells. Once in circulation, invasive tumor cells are called circulating tumor cells (CTCs), and they confront challenges such as shear stress, oxidative stress, and immune surveillance. A few surviving CTCs invade blood vessels and colonize distant tissues, forming metastases. Emerging evidence suggests that physical exercise inhibits not only the invasion of tumor cells, but also the survival and distant colonization of circulating tumor cells. A schematic illustration of the association of exercise and metastasis is shown in Figure 1.
FIGURE 1. PA and the metastatic cascade. Firstly, PA reduced the invasion of tumor cells by inhibiting EMT. Next, PA reduced vascular permeability, inhibitd the interaction between endothelial cells and tumor cells, and suppressed intravasation. Exercise can also inhibit the survival of circulating tumor cells (CTCs) by increasing vascular shear force, recruiting macrophages, NK cells, and CD8+T cells, regulating metabolism and inducing anoikis. Exercise inhibitd platelet-tumor cells aggregates and the capacity of tumor cells to adhere to endothelial cells, which suppress extravasation. The figure was created with BioRender.com.
PA has been confirmed to attenuate the invasion of tumor cells via inhibiting epithelial-mesenchymal transition (EMT). A study showed that voluntary exercise led to an intratumor increase in E-cadherin levels and an intratumor decrease in the nuclear levels of β-catenin in ApcMin/+ mice (Ju et al., 2008). As is known to all, decreased expression of E-cadherin and increased expression of vimentin are the main characteristics of EMT. PA regulates multiple pathways to attenuate EMT. Moderate swimming could suppress EMT induced by TGF-β in transplanted hepatocellular carcinoma cells via promoting dopamine receptor 2 (DR2) activity (Zhang et al., 2016a). High-performance sports and resistance training can induce skeletal muscle to release myokine irisin. Irisin could inhibit EMT and invasion of tumor cells via the PI3K/Akt/Snail pathway has been demonstrated (Shao et al., 2017). Another study reported that irisin could be relevant to the activation of AMPK (Tang et al., 2016). Irisin downregulated the mTOR pathway and inhibited EMT of human pancreatic cancer cells via activating the AMPK (Liu et al., 2018). Moreover, irisin reversed the IL-6 induced EMT and downregulated the expression of MMP-2 by suppressing the STAT3/Snail signaling pathway (Kong et al., 2017).
Physical exercise could influence intra-tumor angiogenesis by altering vascular epithelial growth factor (VEGF) in serum and tumor tissue. In prostate cancer, exercise induced the upregulation of HIF-1α and VEGF via activating MEK/MAPK and PI3K/mTOR signaling pathway, which is associated with a shift to tumor vascular normalization and inhibition of tumor metastasis (Jones et al., 2012). Data from ultrasonographic and thermographic also indicated higher vascularization of mammary tumors in exercised rats (Faustino-Rocha et al., 2017). The hypoxia and high permeability of the intratumoral vasculature also promote the intravasation of tumor cells. Physical exercise enhances tumor perfusion, diminishes hypoxia and transforms an aggressive tumor phenotype with abnormal leaky tumor vasculature to a weakly invasive tumor phenotype with normalized and mature vasculature (McCullough et al., 2014). Previous studies have demonstrated that physical exercise increases microvessel density and vessel maturity (Jones et al., 2012). In Ewing sarcoma, exercise modulated S1PR1 and S1PR2 expression, remodelling vasculature to reduce vessel hyperpermeability.
CTCs exposed to the circulation need to face various physical and biological stressors such as shear force, immune system surveillance, anoikis, and so on. Only a small portion (0.1%) of CTCs survive, and they have a relatively short half-life of about 1.0–2.4 h in circulation. Many studies have shown that exercise reduced CTCs in cancer patients. For instance, using a microfluidic antibody-mediated capture device to quantify CTCs inside venous blood of stage I-III colon cancer patients, researchers found that exercise led to a significant decrease in CTCs (Brown et al., 2018a). Exercise leads to an obvious increase in vascular shear force. During moderate-intensity exercise, the hemodynamic shear force can increase to 60 dyn/cm2 in human arteries and 5.2–6.2 dyn/cm2 in human veins (Tanaka et al., 2006). A previous study investigated the impact of hemodynamic shear force on the CTCs survival. The result revealed that high shear stress of 60 dyn/cm2 at intensive exercise killed more than 90% of CTCs within the first 4 h of circulation, contrasted with low shear stress of 15 dyn/cm2 at the resting state only killed 48% of CTCs (Regmi et al., 2017). Anoikis resistance played an important role in maintaining the survival of CTCs within circulation. HIF-1α protected CTCs from anoikis cell death by keeping an EMT state of CTCs (Majidpoor and Mortezaee, 2021). In untrained humans, acute exercise induced a transient increase of HIF-1α levels, while regular endurance exercise steadily reduced HIF-1α (Lundby et al., 2006; Wu et al., 2020a). Hippo signaling pathway has also been reported to be correlated with anoikis resistance. In metastatic breast cancer, up-regulated expression of zinc finger protein 367 (ZNF367) inhibited Hippo signaling pathway, giving rise to anoikis resistance and increased CTCs in circulation (Wu et al., 2020b). Exercise-conditioned sera could activate the Hippo signaling pathway and increase the inactivation of YAP (Baldelli et al., 2021). Exercise-induced epinephrine and norepinephrine also activated the tumor suppressor Hippo signaling pathway and promoted the phosphorylation of YAP. The phosphorylation then contributed to the sequestration of YAP in cytoplasm, which deterring the induction of tumor cell proliferation and survival by target genes (Dethlefsen et al., 2017). The effects of exercise on immune surveillance will be described in detail later.
Surviving CTCs must arrest in the circulation and then start extravasation. In a previous study, long-term exercise led to a consistent lower retention of tumor cells in the pulmonary capillary bed compared with sedentary mice (MacNeil and Hoffman-Goetz, 1993a). Similarly, another study detected the radioactivity of 51Cr labelled CIRAS 1 tumor cells in lungs, liver, spleen and kidney. Researchers found that exercising mice showed a lower retention of radioactivity in secondary organs after tumor cells were injected into a tail vein (Hoffmann-Goetz et al., 1994).
Exercise may change the microenvironment of the major sites of metastases to inhibit the process of colonization. Recently, a study reported that exercise suppressed ovarian cancer colonization in the peritoneal cavity (Morrisson et al., 2021). The secretion of CCL2 and IL-15 had a significant increase in the peritoneal fluid of exercised mice. CCL2 can recruit macrophages and enhance their cytotoxicity. IL-15 can increase the reactivity of NK cells and CD8+Tcells in the peritoneal environment. Exercise also decreased the level of CCL22, VEGF, and CCL12 in peritoneal fluid. These cytokines lead to an immunosuppressive microenvironment by recruiting MDSC and Treg cells. Lung is a common metastasis site in malignant tumors, and it is also dramatically modulated by exercise. A further mechanism exploration found that exercise elevated antitumor cytotoxicity of alveolar macrophages by increasing the levels of tumor necrosis factor or reactive nitrogen intermediates (Davis et al., 1998). In order to successfully colonize the bone, tumor cells must escape the dormancy and keep proliferation. Tumor–osteoblast interactions have been proved to promote the dormancy of tumor cells. Physical exercise activated Cx43 hemichannels, and mechanically stimulated osteocytes to secrete Wnt and OPN (Fan et al., 2020), enhancing osteoblast activity and promoting the dormancy. Simultaneously, exercise increased the release of ATP from osteocytes (Genetos et al., 2007). The ATP-rich tumor microenvironment has been reported to suppress the proliferation of various tumor cells.
The immune system can be divided into natural and adaptive immunity, and these two immune responses work synergistically to protect the organism (Wang et al., 2020). Natural immunity, also called innate immunity, is a semi-specific and extensive form of immunity. Natural immunity includes multiple immune cells and soluble factors and plays important roles in battling against pathogens. For example, neutrophils, macrophages, dendritic cells (DCs), natural killer (NK) cells, complement proteins and antimicrobial peptides (Janeway and Medzhitov, 2002). Adaptive immunity, also called acquired immunity or specific immunity, is a type of immune response that is generated by contact with a specific pathogen that can be recognized and initiated against the specific pathogen (Bonilla and Oettgen, 2010). Adaptive immunity consists mainly of T and B lymphocytes. T cells mediate cellular immune responses, while B cells are closely associated with the humoral immune response.
In the process of tumor metastasis, most cytotoxic innate and adaptive immune cells can synergistically control tumor behavior. A large number of cytotoxic immune cells such as NK and CD8+ T cells infiltrated around the primary tumor to eliminate many immunogenic cancer cells (Pagès et al., 2010). Natural killer (NK) cells can mediate tumor cells apoptosis via releasing granzyme B- and perforin. Cytotoxic CD8+ T cells kill tumor cells by secreting TNF-α and IFN-γ, while CD4+ T cells produce multiple cytokines to boost anti-tumor immune responses (Swann and Smyth, 2007; Ostrand-Rosenberg, 2008). The high levels of NK cells and cytotoxic T cells infiltration around the tumor are associated with better prognosis in cancer survivors (Nelson, 2008). CTCs are particularly sensitive to circulating immune cells. Circulating immune cells can directly and indirectly affect the viability of CTCs to control cancer metastasis (Dianat-Moghadam et al., 2021). The recruitment of cytotoxic M1 macrophages and N1 neutrophils, NK cells and mature DCs can all contribute to the elimination of CTCs.
However, some immunosuppressive cells such as myeloid-derived suppressor cells (MDSC) and regulatory T cells (Tregs), can secrete multiple cytokines and proteases to reshape the tumor microenvironment and promote immune escape, thereby promoting tumor metastasis (Smith and Kang, 2013). As tumors progress, cancer cells can secret multiple cytokines such as IL-4 and IL-13 to induce polarization of M2 macrophages and N2 neutrophils, which contributes to angiogenesis, extracellular matrix (ECM) remodeling and immune evasion. In addition, immature DCs also play important roles in facilitating tumor metastasis (Gonzalez et al., 2018).
PA has a positive effect on the human immune system, especially the innate immune system. During PA, cytotoxic immune cells are mobilized into the circulation through stress-induced shear stress and adrenergic signaling (Idorn and Hojman, 2016). This mobilization is not to induce the body to produce a new generation of immune cells, but to recruit the existing storage of immune cells (Walsh et al., 2011). According to numerous studies, chronic and acute physical exercise show significant responses in terms of immune cells redistribution, activity and function in cancer patients. The intensity and duration of exercise also affects the redistribution of immune cells to the circulation (Robson et al., 1999; Freidenreich and Volek, 2012; Bigley and Simpson, 2015). In some tumor-bearing animal models, exercise led to an increase in the number and function of effector cells and a decrease in immunosuppressive cells (Thompson et al., 2010; Hagar et al., 2019). Here we discuss the effect of exercise on multiple immune cells in the process of metastasis. A schematic illustration of the association of exercise and immune cells is shown in Figure 2.
FIGURE 2. Modulation of immune cells during exercise. During physical activity, the numbers and antitumor effects of NK cells, dendritic cells, T cells and B cells were increased, the polarization of M2 macrophages and N2 neutrophils are inhibited, and the recruitment of MDSC and Treg was suppressed. Moreover, PA inhibited the formation of platelet-CTCs aggregates and reduced the adhesion of platelets to endothelial cells. The figure was created with BioRender.com.
As the first-line defenders against pathogens, natural immune cells are hot topics to exercise immunology.
Among natural immune cells, NK cells are the most responsive to exercise, showing exercise-dependent acute mobilization. The number of NK cells can be increased to more than six-fold during a brief stair climb, without immediate functional decrease after rest (Millard et al., 2013). This rapid mobilization of NK cells is mainly related to the exercise intensity-dependent changes in catecholamine concentrations (Kappel et al., 1991). NK cells have the most abundant β-adrenergic receptors in all immune cells (Landmann, 1992). Systemic administration of epinephrine mimics the exercise-induced increase in circulating NK cell infiltration, while nonselective and selective β1-and β2-blockers can block this mobilization effect from exercise (Murray et al., 1992). During acute PA, muscle-derived cytokines such as IL-15, IL-7, and IL-6 are also involved in NK cells activation (Benatti and Pedersen, 2015). However, after long-term exercise, the number of circulating NK cells was reduced, which may due to tissue migration or re-marginalization (Timmons and Cieslak, 2008). PA not only increases the number of circulating NK cells, but also enhances their antitumor activity. A previous study has demonstrated that exercise enhanced splenic NK cells activity in tumor-bearing mice (MacNeil and Hoffman-Goetz, 1993b). Another study has found that mice randomly assigned to the voluntary wheel had an obvious increase in NK cells infiltration in various tumor models (melanoma, Lewis lung cancer and liver cancer), leading to reductions in tumor incidence, growth and metastases. Exercise recruits NK cells via β-adrenergic signaling and induces muscle-derived IL-6 to redistribute and activate NK cells. Moreover, the expression levels of NK cell-related activating receptor ligands (NKG2D, MULT1, H60a, and Clr-b) also had an increase in the tumors of running mice, revealing that exercise worked on the mobilization of NK cells and the formation of NK cell activated tumor microenvironment (Pedersen et al., 2016).
Macrophages also play a pivotal role in controlling tumor metastasis. M1 macrophages have the capacity to diminish a large number of CTCs, while M2 macrophages are related to the promotion of tumor metastasis. Exercise can enhance antitumor macrophage cytotoxicity and suppress the polarization of macrophages to the M2 (Davis et al., 1998; Goh et al., 2012). Short-term moderate-exercise training led to an increase in macrophages antitumor cytotoxicity and decreased the lung tumor metastases of injected B16 melanoma cells (Murphy et al., 2004). Another recent study using a triple-negative breast cancer mouse model reported that exercise reduced M2 macrophage polarization by inhibiting the JAK-STAT signaling pathway, thus decreasing lung cancer metastasis (Kim et al., 2020). M2 macrophages secreted chemokine CCL22, which attracted CCR4-expressing Tregs in circulation toward the CCL22 gradient, thus facilitating the recruitment of Tregs. Exercise contributed to a significant decrease in CCL22 mRNA expression in M2 macrophages and resulted in a reduction in Treg recruitment, which delayed invasive breast cancer progression and metastasis in polyoma middle T oncoprotein (PyMT) transgenic mouse (Goh et al., 2013). Similarly, in an ApcMin/+ mouse model, the mRNA expression of M2 related macrophage markers such as CD206, CCL22 and Arg consistently decreased in exercise mice (McClellan et al., 2014).
Some recent studies revealed that neutrophils promoted the metastasis potential of cancer cells. In circulation, neutrophils induced the aggregation of tumor cells to improve the survival rate of CTCs (Szczerba et al., 2019). Neutrophil extracellular traps (NETs) released by neutrophils was also demonstrated to enhance the tumor metastasis. Some studies have shown that exercise can inhibit NETs formation (Shi et al., 2020). The accumulation of exercise-induced lactic acid decreases the release of NETs in serum (Shi et al., 2019). A recent study reported that exercise mitigated liver ischemia-reperfusion injury derived inflammatory responses and metastasis via inhibiting neutrophil recruitment and diminishing NETs release in the mouse model of colorectal adenocarcinoma (Yazdani et al., 2021). Nevertheless, tumor-associated neutrophils (TANs) had a two-sided effect in the progression of tumor (Uribe-Querol and Rosales, 2015). Some previous reports demonstrated that neutrophils directly destroyed tumor cells both in vitro and in vivo (Uribe-Querol and Rosales, 2015). In metastatic breast cancer and renal carcinoma, the tumor cells produced CCL2 and IL-8 that induced neutrophil recruitment to inhibit lung metastasis, respectively (Granot et al., 2011; López-Lago et al., 2013).
Similar to the M1 and M2 phenotypes of macrophages, neutrophils also have N1 and N2 polarization states. N1 neutrophils have anti-tumor function by secreting type I interferon and inducing NK cells to secret IL-18. N2 neutrophils secrete multiple molecules such as arginase and peroxidase to inhibit T cells and NK cells functions, which promote tumor metastasis. TGF-β derived from tumor microenvironment can induce the activation of N2 neutrophils (Fridlender et al., 2009). PA has been demonstrated to inhibit the expression of TGF-β in tumor tissue (da Silva Alves et al., 2020), which attenuates the polarization of N2 neutrophils.
DCs play a key role in eliminating and controlling tumor progression. In human exercise studies, PA can increase the number of DCs in the peripheral blood circulation (Ho et al., 2001; LaVoy et al., 2015). Further study showed that exercise upregulated the expression of MHC II and IL-12 on DCs in animal models (Liao et al., 2006; Chiang et al., 2007). A previous study investigated the composition of DCs subpopulations mobilized in response to acute aerobic exercise. The findings showed that exercise preferentially mobilized plasmacytoid DCs into peripheral blood to enhance immune surveillance (Brown et al., 2018b). However, there are few studies investigating the effects of exercise on DCs in cancer patients, and more research is needed in the future.
MDSCs are effective inhibitory factors of immune function and contribute to the immune escape. Augmented ROS produced by MDSCs induced the upregulation of Notch1 in CTCs through the ROS-NRF2-ARE axis, thus enhancing CTCs metastatic traits (Sprouse et al., 2019). Recently, a preclinical study found that PA reduced the tumor-induced accumulation of MDSCs and delayed the tumor growth in a mouse model of triple negative breast cancer (Wennerberg et al., 2020). In 4T1 tumor-bearing mice, voluntary wheel running potently relayed the accumulation of IMCs/MDSCs in the spleen, blood, and tumor. Moreover, these effects led to a reduction in the number of metastatic lung nodules in exercising mice (Garritson et al., 2020). Another previous study also showed that the combination of PA and energy restriction decreased MDSC accumulation by restraining myelopoiesis and/or the mobilization and transportation of MDSCs to secondary sites (Turbitt et al., 2019). In a mouse model of pancreatic cancer, PA diminished MDSC via downregulating the expression levels of Cxcr2 and Csf3r on myeloid cells (Kurz et al., 2022). These findings suggested that PA was beneficial to inhibiting tumor progression and metastases via suppressing MDSCs accumulation.
Platelets activation plays an essential role in elevating the survival rate of the CTCs. Activated platelets adhere to CTCs to protect the tumor cells from various stressors in circulation. Moreover, aggregates of platelets and CTCs have been shown to inhibit NK cells antitumor cytotoxicity in vitro-model. Some reports have found that PA affected the clearance of CTCs by modulating platelets activity. Compared with sedentary mice with breast cancer, exercising mice had a lower number of circulating platelets (Smeda et al., 2017). In patients with nasopharyngeal carcinoma, moderate-intensity exercise decreased the formation of platelets-CTCs aggregates and minimized the risk of metastasis (Wang et al., 2007). Another study by the same team found that warm-up exercise before severe exercise reduced platelet-impeded cytotoxicity of NK cells to nasopharyngeal carcinoma cells (Wang et al., 2009).
The activation of platelets is also critical for CTCs to extravasate. Adhesion molecules on activated platelets can gather CTCs to securely adhere to the activated vascular endothelial cells. PA might be related with the downregulation of adhesion molecules on platelets and endothelial cells (ECs), such as P-selectin and epithelial cell adhesion molecule-1 (EPCAM-1) (Wang et al., 2005; Souza et al., 2017). In P-selectin-deficient mice, lung metastasis was significantly reduced post injection of tumor cells (Borsig, 2004). Nevertheless, the positive effects of exercise might be limited by intensity. Some investigations have found that strenuous exercise promoted platelets aggregation and the capacity of CTCs to adhere to ECs for sedentary healthy humans (Chen et al., 2009), yet moderate exercise inhibited platelets aggregation and adhesiveness (Wang and Liao, 2004; Wang et al., 2005; Wang, 2006).
Adaptive immune cells consist mainly of T and B lymphocytes. In general, exercise-induced lymphocytosis is proportional to the duration and intensity of exercise.
Cytotoxic T cells recognize and diminish CTCs by specifically identifying mutation-induced neoantigens. A study using breast cancer mice found that acute exercise caused a transient increase in CD8+ T cells. Exercise-induced decrease in tumor growth was contingent on the levels of CD8+ T cell in circulation. And key metabolites that muscles released into the blood during exercise, including lactate, made CD8+ T cells more effective. Moreover, these super-effective CD8+ T cells extracted from exercising mice showed better antitumor efficacy when transferred to sedentary mice (Rundqvist et al., 2020). Recently, a preclinical study also suggested that PA can increase the infiltration and effector function of CD8 T cells in breast tumors. Further investigation showed that CXCL9/11-CXCR3 pathway is required for the CD8+ T cell-mediated antitumor effect of PA (Gomes-Santos et al., 2021). In a mouse model of pancreatic cancer, PA activated IL-15/ IL15Rα pathway to promotes activation of CD8+ T cells (Kurz et al., 2022). Notable, exercise-induced IL-15Rα CD8+ T cells selectively upregulate checkpoint PD-1, which contributes to increase sensitivity to chemotherapy.
CD4+ T cells also play a central role in antitumor immune response. Similar to CD8+ T cells, a temporary increase in CD4+ T cells was detected after resistance exercise (Natale et al., 2003). In a mouse model of hepatocellular carcinoma, exercise enhanced immunity by raising CD4+ T lymphocytes in peripheral blood (Zhang et al., 2016b).
Tregs effectually inhibit the activation and proliferation of CD8+ T cells, which are considered to be the important barriers to impede the effect of anti-tumor immunity. An increased number of Tregs indicated a higher CTCs-positive rate and contributed to a poorer clinical outcome in cancer patients (Xue et al., 2018). A previous study demonstrated that endurance exercise suppressed the recruitment of Tregs and relayed the tumor growth in breast cancer. Exercise led to a greater tumor immune response by increasing the ratio of CD8/Tregs (Hagar et al., 2019). PA induced the downregulation of chemokines such as CCL5, CCL20 and CCL25, which were closely associated with the recruitment of Tregs (Turbitt et al., 2019).
The role of B cells in cancer progression is much less understood than that of T cells. Growing evidence suggested that tumor-infiltrating B cells may exert both tumor suppressive and tumor promoting effects (Gu et al., 2019; Xu et al., 2022). During exercise, circulating B-cell counts increased mildly immediately and in proportion to exercise duration and intensity (Ronsen et al., 2001). However, there are few studies elucidating the effects of exercise on B cells immune function in cancer patients.
Physical exercise improves blood perfusion and hypoxia, which also affect immune function. Hypoxia induces overexpression of connexin 43 in tumor cells, leading to degradation of NK cell immune synapses and impairing NK cell killing activity (Tittarelli et al., 2015). Improving intra-tumor hypoxia can indirectly increase the cytotoxicity of tumor-infiltrating NK cells. Moreover, PA promotes normalization of intratumoral vessels and blood perfusion, which can increase the accessibility of immune cells and delivery of antitumor drugs.
Existing preclinical and clinical studies have demonstrated that PA, particularly regular moderate exercise, plays a beneficial role in tumor metastasis. The immune system is highly responsive to exercise, which may lead to beneficial effects on tumor metastasis. During exercise, a large number of cytotoxic immune cells with antitumor functions are mobilized into circulation to kill CTCs. To be sure, the mechanisms of exercise modulating immune cells are extensive and diverse. However, the exploration of the potential mechanisms underlying the beneficial effect of exercise on immune cells is still in its early stages. The review analyzed that PA can control metastasis by regulating immune function. As the understanding of the mechanisms by which PA effects tumor metastasis continues to improve, new therapeutic strategies will be identified and validated, potentially contributing to improve survival in cancer patients.
AZ wrote original draft and drew the figures. JY, XY, TZ, XW, and XM corrected the draft. LZ revised most of the manuscript. All authors have read and agreed to the published version of the manuscript.
We acknowledge the editors and the reviewers for insightful suggestions on this work.
The authors declare that the research was conducted in the absence of any commercial or financial relationships that could be construed as a potential conflict of interest.
All claims expressed in this article are solely those of the authors and do not necessarily represent those of their affiliated organizations, or those of the publisher, the editors and the reviewers. Any product that may be evaluated in this article, or claim that may be made by its manufacturer, is not guaranteed or endorsed by the publisher.
Baldelli, G., De Santi, M., Gervasi, M., Annibalini, G., Sisti, D., Højman, P., et al. (2021). The effects of human sera conditioned by high-intensity exercise sessions and training on the tumorigenic potential of cancer cells. Clin. Transl. Oncol. 23 (1), 22–34. Epub 2020/05/25. doi:10.1007/s12094-020-02388-6
Bellocco, R., Marrone, G., Ye, W., Nyrén, O., Adami, H. O., Mariosa, D., et al. (2016). A prospective cohort study of the combined effects of physical activity and anthropometric measures on the risk of post-menopausal breast cancer. Eur. J. Epidemiol. 31 (4), 395–404. Epub 2015/07/02. doi:10.1007/s10654-015-0064-z
Benatti, F. B., and Pedersen, B. K. (2015). Exercise as an anti-inflammatory therapy for rheumatic diseases-myokine regulation. Nat. Rev. Rheumatol. 11 (2), 86–97. Epub 2014/11/26. doi:10.1038/nrrheum.2014.193
Bigley, A. B., and Simpson, R. J. (2015). Nk cells and exercise: Implications for cancer immunotherapy and survivorship. Discov. Med. 19 (107), 433–445. Epub 2015/07/16.
Bonilla, F. A., and Oettgen, H. C. (2010). Adaptive immunity. J. Allergy Clin. Immunol. 125 (2), S33–S40. Epub 2010/01/12. doi:10.1016/j.jaci.2009.09.017
Borsig, L. (2004). Selectins facilitate carcinoma metastasis and heparin can prevent them. News Physiol. Sci. 19, 16–21. Epub 2004/01/24. doi:10.1152/nips.01450.2003
Brown, J. C., Rhim, A. D., Manning, S. L., Brennan, L., Mansour, A. I., Rustgi, A. K., et al. (2018). Effects of exercise on circulating tumor cells among patients with resected stage I-iii colon cancer. PLoS One 13 (10), e0204875. Epub 2018/10/18. doi:10.1371/journal.pone.0204875
Brown, F. F., Campbell, J. P., Wadley, A. J., Fisher, J. P., Aldred, S., and Turner, J. E. (2018). Acute aerobic exercise induces a preferential mobilisation of plasmacytoid dendritic cells into the peripheral blood in man. Physiol. Behav. 194, 191–198. Epub 2018/05/16. doi:10.1016/j.physbeh.2018.05.012
Bull, F. C., Al-Ansari, S. S., Biddle, S., Borodulin, K., Buman, M. P., Cardon, G., et al. (2020). World health organization 2020 guidelines on physical activity and sedentary behaviour. Br. J. Sports Med. 54 (24), 1451–1462. Epub 2020/11/27. doi:10.1136/bjsports-2020-102955
Chen, Y. W., Chen, J. K., and Wang, J. S. (2009). Exercise affects platelet-promoted tumor cell adhesion and invasion to endothelium. Eur. J. Appl. Physiol. 105 (3), 393–401. Epub 2008/11/11. doi:10.1007/s00421-008-0916-2
Chiang, L. M., Chen, Y. J., Chiang, J., Lai, L. Y., Chen, Y. Y., and Liao, H. F. (2007). Modulation of dendritic cells by endurance training. Int. J. Sports Med. 28 (9), 798–803. Epub 2007/04/17. doi:10.1055/s-2007-964914
da Silva Alves, R., Abdalla, D. R., Iunes, D. H., Mariano, K. O. P., Borges, J. B. C., Murta, E. F. C., et al. (2020). Influence of an exergaming training program on reducing the expression of il-10 and tgf-Β in cancer patients. Games Health J. 9 (6), 446–452. Epub 2020/06/06. doi:10.1089/g4h.2020.0022
Davis, J. M., Kohut, M. L., Jackson, D. A., Colbert, L. H., Mayer, E. P., and Ghaffar, A. (1998). Exercise effects on lung tumor metastases and in vitro alveolar macrophage antitumor cytotoxicity. Am. J. Physiol. 274 (5), R1454–R1459. Epub 1998/06/05. doi:10.1152/ajpregu.1998.274.5.R1454
Dethlefsen, C., Hansen, L. S., Lillelund, C., Andersen, C., Gehl, J., Christensen, J. F., et al. (2017). Exercise-induced catecholamines activate the Hippo tumor suppressor pathway to reduce risks of breast cancer development. Cancer Res. 77 (18), 4894–4904. Epub 2017/09/10. doi:10.1158/0008-5472.Can-16-3125
Dianat-Moghadam, H., Mahari, A., Heidarifard, M., Parnianfard, N., Pourmousavi-Kh, L., Rahbarghazi, R., et al. (2021). Nk cells-directed therapies target circulating tumor cells and metastasis. Cancer Lett. 497, 41–53. Epub 2020/09/29. doi:10.1016/j.canlet.2020.09.021
Fan, Y., Jalali, A., Chen, A., Zhao, X., Liu, S., Teli, M., et al. (2020). Skeletal loading regulates breast cancer-associated osteolysis in a loading intensity-dependent fashion. Bone Res. 8, 9. Epub 2020/03/05. doi:10.1038/s41413-020-0083-6
Faustino-Rocha, A. I., Gama, A., Oliveira, P. A., Vanderperren, K., Saunders, J. H., Pires, M. J., et al. (2017). A contrast-enhanced ultrasonographic study about the impact of long-term exercise training on mammary tumor vascularization. J. Ultrasound Med. 36 (12), 2459–2466. Epub 2017/06/25. doi:10.1002/jum.14287
Febbraio, M. A. (2017). Exercise metabolism in 2016: Health benefits of exercise - more than meets the eye. Nat. Rev. Endocrinol. 13 (2), 72–74. Epub 2017/01/05. doi:10.1038/nrendo.2016.218
Freidenreich, D. J., and Volek, J. S. (2012). Immune responses to resistance exercise. Exerc. Immunol. Rev. 18, 8–41. Epub 2012/08/11.
Fridlender, Z. G., Sun, J., Kim, S., Kapoor, V., Cheng, G., Ling, L., et al. (2009). Polarization of tumor-associated neutrophil phenotype by tgf-beta: "N1" versus "N2" tan. Cancer Cell 16 (3), 183–194. Epub 2009/09/08. doi:10.1016/j.ccr.2009.06.017
Friedenreich, C. M., Cook, L. S., Wang, Q., Kokts-Porietis, R. L., McNeil, J., Ryder-Burbidge, C., et al. (2020). Prospective cohort study of pre- and postdiagnosis physical activity and endometrial cancer survival. J. Clin. Oncol. 38 (34), 4107–4117. Epub 2020/10/08. doi:10.1200/jco.20.01336
Garritson, J., Krynski, L., Haverbeck, L., Haughian, J. M., Pullen, N. A., and Hayward, R. (2020). Physical activity delays accumulation of immunosuppressive myeloid-derived suppressor cells. PLoS One 15 (6), e0234548. Epub 2020/06/17. doi:10.1371/journal.pone.0234548
Genetos, D. C., Kephart, C. J., Zhang, Y., Yellowley, C. E., and Donahue, H. J. (2007). Oscillating fluid flow activation of gap junction hemichannels induces atp release from mlo-Y4 osteocytes. J. Cell. Physiol. 212 (1), 207–214. Epub 2007/02/16. doi:10.1002/jcp.21021
Goh, J., Kirk, E. A., Lee, S. X., and Ladiges, W. C. (2012). Exercise, physical activity and breast cancer: The role of tumor-associated macrophages. Exerc. Immunol. Rev. 18, 158–176. Epub 2012/08/11.
Goh, J., Tsai, J., Bammler, T. K., Farin, F. M., Endicott, E., and Ladiges, W. C. (2013). Exercise training in transgenic mice is associated with attenuation of early breast cancer growth in a dose-dependent manner. PLoS One 8 (11), e80123. Epub 2013/12/07. doi:10.1371/journal.pone.0080123
Gomes-Santos, I. L., Amoozgar, Z., Kumar, A. S., Ho, W. W., Roh, K., Talele, N. P., et al. (2021). Exercise training improves tumor control by increasing Cd8(+) T-cell infiltration via Cxcr3 signaling and sensitizes breast cancer to immune checkpoint blockade. Cancer Immunol. Res. 9 (7), 765–778. Epub 2021/04/12. doi:10.1158/2326-6066.Cir-20-0499
Gonzalez, H., Hagerling, C., and Werb, Z. (2018). Roles of the immune system in cancer: From tumor initiation to metastatic progression. Genes Dev. 32 (19-20), 1267–1284. Epub 2018/10/03. doi:10.1101/gad.314617.118
Granot, Z., Henke, E., Comen, E. A., King, T. A., Norton, L., and Benezra, R. (2011). Tumor entrained neutrophils inhibit seeding in the premetastatic lung. Cancer Cell 20 (3), 300–314. Epub 2011/09/13. doi:10.1016/j.ccr.2011.08.012
Gu, Y., Liu, Y., Fu, L., Zhai, L., Zhu, J., Han, Y., et al. (2019). Tumor-educated B cells selectively promote breast cancer lymph node metastasis by hspa4-targeting igg. Nat. Med. 25 (2), 312–322. Epub 2019/01/16. doi:10.1038/s41591-018-0309-y
Hagar, A., Wang, Z., Koyama, S., Serrano, J. A., Melo, L., Vargas, S., et al. (2019). Endurance training slows breast tumor growth in mice by suppressing Treg cells recruitment to tumors. BMC Cancer 19 (1), 536. Epub 2019/06/06. doi:10.1186/s12885-019-5745-7
Ho, C. S., López, J. A., Vuckovic, S., Pyke, C. M., Hockey, R. L., and Hart, D. N. (2001). Surgical and physical stress increases circulating blood dendritic cell counts independently of monocyte counts. Blood 98 (1), 140–145. Epub 2001/06/22. doi:10.1182/blood.v98.1.140
Hoffmann-Goetz, L., MacNeil, B., and Arumugam, Y. (1994). Tissue distribution of radiolabelled tumor cells in wheel exercised and sedentary mice. Int. J. Sports Med. 15 (5), 249–253. Epub 1994/07/01. doi:10.1055/s-2007-1021055
Idorn, M., and Hojman, P. (2016). Exercise-dependent regulation of nk cells in cancer protection. Trends Mol. Med. 22 (7), 565–577. Epub 2016/06/06. doi:10.1016/j.molmed.2016.05.007
Janeway, C. A., and Medzhitov, R. (2002). Innate immune recognition. Annu. Rev. Immunol. 20, 197–216. Epub 2002/02/28. doi:10.1146/annurev.immunol.20.083001.084359
Jones, L. W., Antonelli, J., Masko, E. M., Broadwater, G., Lascola, C. D., Fels, D., et al. (2012). Exercise modulation of the host-tumor interaction in an orthotopic model of murine prostate cancer. J. Appl. Physiol. 113 (2), 263–272. Epub 2012/05/19. doi:10.1152/japplphysiol.01575.2011
Ju, J., Nolan, B., Cheh, M., Bose, M., Lin, Y., Wagner, G. C., et al. (2008). Voluntary exercise inhibits intestinal tumorigenesis in apc(min/+) mice and azoxymethane/dextran sulfate sodium-treated mice. BMC Cancer 8, 316. Epub 2008/11/04. doi:10.1186/1471-2407-8-316
Kappel, M., Tvede, N., Galbo, H., Haahr, P. M., Kjaer, M., Linstow, M., et al. (1991). Evidence that the effect of physical exercise on nk cell activity is mediated by epinephrine. J. Appl. Physiol. 70 (6), 2530–2534. Epub 1991/06/01. doi:10.1152/jappl.1991.70.6.2530
Kim, M. K., Kim, Y., Park, S., Kim, E., Kim, Y., Kim, Y., et al. (2020). Effects of steady low-intensity exercise on high-fat diet stimulated breast cancer progression via the alteration of macrophage polarization. Integr. Cancer Ther. 19, 1534735420949678. Epub 2020/09/11. doi:10.1177/1534735420949678
Kong, G., Jiang, Y., Sun, X., Cao, Z., Zhang, G., Zhao, Z., et al. (2017). Irisin reverses the il-6 induced epithelial-mesenchymal transition in osteosarcoma cell migration and invasion through the stat3/snail signaling pathway. Oncol. Rep. 38 (5), 2647–2656. Epub 2017/10/20. doi:10.3892/or.2017.5973
Kurz, E., Hirsch, C. A., Dalton, T., Shadaloey, S. A., Khodadadi-Jamayran, A., Miller, G., et al. (2022). Exercise-induced engagement of the il-15/il-15rα Axis promotes anti-tumor immunity in pancreatic cancer. Cancer Cell 40 (7), 720–737. Epub 2022/06/07. doi:10.1016/j.ccell.2022.05.006
Landmann, R. (1992). Beta-adrenergic receptors in human leukocyte subpopulations. Eur. J. Clin. Invest. 22, 30–36. Epub 1992/10/01.
LaVoy, E. C., Bollard, C. M., Hanley, P. J., O'Connor, D. P., Lowder, T. W., Bosch, J. A., et al. (2015). A single bout of dynamic exercise by healthy adults enhances the generation of monocyte-derived-dendritic cells. Cell. Immunol. 295 (1), 52–59. Epub 2015/03/10. doi:10.1016/j.cellimm.2015.02.007
Liao, H. F., Chiang, L. M., Yen, C. C., Chen, Y. Y., Zhuang, R. R., Lai, L. Y., et al. (2006). Effect of a periodized exercise training and active recovery program on antitumor activity and development of dendritic cells. J. Sports Med. Phys. Fit. 46 (2), 307–314. Epub 2006/07/11.
Liu, J., Song, N., Huang, Y., and Chen, Y. (2018). Irisin inhibits pancreatic cancer cell growth via the ampk-mtor pathway. Sci. Rep. 8 (1), 15247. Epub 2018/10/17. doi:10.1038/s41598-018-33229-w
López-Lago, M. A., Posner, S., Thodima, V. J., Molina, A. M., Motzer, R. J., and Chaganti, R. S. (2013). Neutrophil chemokines secreted by tumor cells mount a lung antimetastatic response during renal cell carcinoma progression. Oncogene 32 (14), 1752–1760. Epub 2012/06/06. doi:10.1038/onc.2012.201
Lucia, A., and Ramírez, M. (2016). Muscling in on cancer. N. Engl. J. Med. 375 (9), 892–894. Epub 2016/09/01. doi:10.1056/NEJMcibr1606456
Lundby, C., Gassmann, M., and Pilegaard, H. (2006). Regular endurance training reduces the exercise induced hif-1alpha and hif-2alpha mrna expression in human skeletal muscle in normoxic conditions. Eur. J. Appl. Physiol. 96 (4), 363–369. Epub 2005/11/15. doi:10.1007/s00421-005-0085-5
Ma, X., Wang, M., Yin, T., Zhao, Y., and Wei, X. (2019). Myeloid-derived suppressor cells promote metastasis in breast cancer after the stress of operative removal of the primary cancer. Front. Oncol. 9, 855. Epub 2019/09/26. doi:10.3389/fonc.2019.00855
MacNeil, B., and Hoffman-Goetz, L. (1993). Chronic exercise enhances in vivo and in vitro cytotoxic mechanisms of natural immunity in mice. J. Appl. Physiol. 74 (1), 388–395. Epub 1993/01/01. doi:10.1152/jappl.1993.74.1.388
MacNeil, B., and Hoffman-Goetz, L. (1993). Effect of exercise on natural cytotoxicity and pulmonary tumor metastases in mice. Med. Sci. Sports Exerc. 25 (8), 922–928. Epub 1993/08/01. doi:10.1249/00005768-199308000-00007
Majidpoor, J., and Mortezaee, K. (2021). Steps in metastasis: An updated review. Med. Oncol. 38 (1), 3. Epub 2021/01/05. doi:10.1007/s12032-020-01447-w
McClellan, J. L., Steiner, J. L., Day, S. D., Enos, R. T., Davis, M. J., Singh, U. P., et al. (2014). Exercise effects on polyp burden and immune markers in the Apcmin/+ mouse model of intestinal tumorigenesis. Int. J. Oncol. 45 (2), 861–868. Epub 2014/05/27. doi:10.3892/ijo.2014.2457
McCullough, D. J., Stabley, J. N., Siemann, D. W., and Behnke, B. J. (2014). Modulation of blood flow, hypoxia, and vascular function in orthotopic prostate tumors during exercise. J. Natl. Cancer Inst. 106 (4), dju036. Epub 2014/03/15. doi:10.1093/jnci/dju036
Meyerhardt, J. A., Heseltine, D., Niedzwiecki, D., Hollis, D., Saltz, L. B., Mayer, R. J., et al. (2006). Impact of physical activity on cancer recurrence and survival in patients with stage iii colon cancer: Findings from calgb 89803. J. Clin. Oncol. 24 (22), 3535–3541. Epub 2006/07/11. doi:10.1200/jco.2006.06.0863
Millard, A. L., Valli, P. V., Stussi, G., Mueller, N. J., Yung, G. P., and Seebach, J. D. (2013). Brief exercise increases peripheral blood nk cell counts without immediate functional changes, but impairs their responses to ex vivo stimulation. Front. Immunol. 4, 125. Epub 2013/06/12. doi:10.3389/fimmu.2013.00125
Morrisson, M. J., Bi, F., Yang, K., Cady, S. L., Hartwich, T. M., Cerchia, A. P., et al. (2021). Effect of exercise on peritoneal microenvironment and progression of ovarian cancer. Am. J. Cancer Res. 11 (10), 5045–5062. Epub 2021/11/13.
Murphy, E. A., Davis, J. M., Brown, A. S., Carmichael, M. D., Mayer, E. P., and Ghaffar, A. (2004). Effects of moderate exercise and oat beta-glucan on lung tumor metastases and macrophage antitumor cytotoxicity. J. Appl. Physiol. 97 (3), 955–959. Epub 2004/05/18. doi:10.1152/japplphysiol.00252.2004
Murray, D. R., Irwin, M., Rearden, C. A., Ziegler, M., Motulsky, H., and Maisel, A. S. (1992). Sympathetic and immune interactions during dynamic exercise. Mediation via a beta 2-adrenergic-dependent mechanism. Circulation 86 (1), 203–213. Epub 1992/07/01. doi:10.1161/01.cir.86.1.203
Natale, V. M., Brenner, I. K., Moldoveanu, A. I., Vasiliou, P., Shek, P., and Shephard, R. J. (2003). Effects of three different types of exercise on blood leukocyte count during and following exercise. Sao Paulo Med. J. 121 (1), 9–14. Epub 2003/05/20. doi:10.1590/s1516-31802003000100003
Nelson, B. H. (2008). The impact of T-cell immunity on ovarian cancer outcomes. Immunol. Rev. 222, 101–116. Epub 2008/03/28. doi:10.1111/j.1600-065X.2008.00614.x
Ostrand-Rosenberg, S. (2008). Immune surveillance: A balance between protumor and antitumor immunity. Curr. Opin. Genet. Dev. 18 (1), 11–18. Epub 2008/03/01. doi:10.1016/j.gde.2007.12.007
Pagès, F., Galon, J., Dieu-Nosjean, M. C., Tartour, E., Sautès-Fridman, C., and Fridman, W. H. (2010). Immune infiltration in human tumors: A prognostic factor that should not Be ignored. Oncogene 29 (8), 1093–1102. Epub 2009/12/01. doi:10.1038/onc.2009.416
Pedersen, L., Idorn, M., Olofsson, G. H., Lauenborg, B., Nookaew, I., Hansen, R. H., et al. (2016). Voluntary running suppresses tumor growth through epinephrine- and il-6-dependent nk cell mobilization and redistribution. Cell Metab. 23 (3), 554–562. Epub 2016/02/21. doi:10.1016/j.cmet.2016.01.011
Regmi, S., Fu, A., and Luo, K. Q. (2017). High shear stresses under exercise condition destroy circulating tumor cells in a microfluidic system. Sci. Rep. 7, 39975. Epub 2017/01/06. doi:10.1038/srep39975
Robson, P. J., Blannin, A. K., Walsh, N. P., Castell, L. M., and Gleeson, M. (1999). Effects of exercise intensity, duration and recovery on in vitro neutrophil function in male athletes. Int. J. Sports Med. 20 (2), 128–135. Epub 1999/04/06. doi:10.1055/s-2007-971106
Rock, C. L., Thomson, C., Gansler, T., Gapstur, S. M., McCullough, M. L., Patel, A. V., et al. (2020). American cancer society guideline for diet and physical activity for cancer prevention. Ca. Cancer J. Clin. 70 (4), 245–271. Epub 2020/06/10. doi:10.3322/caac.21591
Ronsen, O., Pedersen, B. K., Øritsland, T. R., Bahr, R., and Kjeldsen-Kragh, J. (2001). Leukocyte counts and lymphocyte responsiveness associated with repeated bouts of strenuous endurance exercise. J. Appl. Physiol. 91 (1), 425–434. Epub 2001/06/16. doi:10.1152/jappl.2001.91.1.425
Rundqvist, H., Veliça, P., Barbieri, L., Gameiro, P. A., Bargiela, D., Gojkovic, M., et al. (2020). Cytotoxic T-cells mediate exercise-induced reductions in tumor growth. Elife 9, e59996. Epub 2020/10/24. doi:10.7554/eLife.59996
Schmidt, M. E., Wiskemann, J., Armbrust, P., Schneeweiss, A., Ulrich, C. M., and Steindorf, K. (2015). Effects of resistance exercise on fatigue and quality of life in breast cancer patients undergoing adjuvant chemotherapy: A randomized controlled trial. Int. J. Cancer 137 (2), 471–480. Epub 2014/12/09. doi:10.1002/ijc.29383
Schmitz, K. H., Campbell, A. M., Stuiver, M. M., Pinto, B. M., Schwartz, A. L., Morris, G. S., et al. (2019). Exercise is medicine in oncology: Engaging clinicians to help patients move through cancer. Ca. Cancer J. Clin. 69 (6), 468–484. Epub 2019/10/17. doi:10.3322/caac.21579
Shao, L., Li, H., Chen, J., Song, H., Zhang, Y., Wu, F., et al. (2017). Irisin suppresses the migration, proliferation, and invasion of lung cancer cells via inhibition of epithelial-to-mesenchymal transition. Biochem. Biophys. Res. Commun. 485 (3), 598–605. Epub 2016/12/18. doi:10.1016/j.bbrc.2016.12.084
Shi, Y., Shi, H., Nieman, D. C., Hu, Q., Yang, L., Liu, T., et al. (2019). Lactic acid accumulation during exhaustive exercise impairs release of neutrophil extracellular traps in mice. Front. Physiol. 10, 709. Epub 2019/07/03. doi:10.3389/fphys.2019.00709
Shi, Y., Liu, T., Nieman, D. C., Cui, Y., Li, F., Yang, L., et al. (2020). Aerobic exercise attenuates acute lung injury through net inhibition. Front. Immunol. 11, 409. Epub 2020/04/09. doi:10.3389/fimmu.2020.00409
Smeda, M., Przyborowski, K., Proniewski, B., Zakrzewska, A., Kaczor, D., Stojak, M., et al. (2017). Breast cancer pulmonary metastasis is increased in mice undertaking spontaneous physical training in the running wheel; a call for revising beneficial effects of exercise on cancer progression. Am. J. Cancer Res. 7 (9), 1926–1936. Epub 2017/10/06.
Smith, H. A., and Kang, Y. (2013). The metastasis-promoting roles of tumor-associated immune cells. J. Mol. Med. 91 (4), 411–429. Epub 2013/03/22. doi:10.1007/s00109-013-1021-5
Souza, P. S., Gonçalves, E. D., Pedroso, G. S., Farias, H. R., Junqueira, S. C., Marcon, R., et al. (2017). Physical exercise attenuates experimental autoimmune encephalomyelitis by inhibiting peripheral immune response and blood-brain barrier disruption. Mol. Neurobiol. 54 (6), 4723–4737. Epub 2016/07/23. doi:10.1007/s12035-016-0014-0
Sprouse, M. L., Welte, T., Boral, D., Liu, H. N., Yin, W., Vishnoi, M., et al. (2019). Pmn-mdscs enhance ctc metastatic properties through reciprocal interactions via ros/notch/nodal signaling. Int. J. Mol. Sci. 20 (8), E1916. Epub 2019/04/21. doi:10.3390/ijms20081916
Steeg, P. S. (2016). Targeting metastasis. Nat. Rev. Cancer 16 (4), 201–218. Epub 2016/03/25. doi:10.1038/nrc.2016.25
Swann, J. B., and Smyth, M. J. (2007). Immune surveillance of tumors. J. Clin. Invest. 117 (5), 1137–1146. Epub 2007/05/04. doi:10.1172/jci31405
Szczerba, B. M., Castro-Giner, F., Vetter, M., Krol, I., Gkountela, S., Landin, J., et al. (2019). Neutrophils escort circulating tumour cells to enable cell cycle progression. Nature 566 (7745), 553–557. Epub 2019/02/08. doi:10.1038/s41586-019-0915-y
Tanaka, H., Shimizu, S., Ohmori, F., Muraoka, Y., Kumagai, M., Yoshizawa, M., et al. (2006). Increases in blood flow and shear stress to nonworking limbs during incremental exercise. Med. Sci. Sports Exerc. 38 (1), 81–85. Epub 2006/01/06. doi:10.1249/01.mss.0000191166.81789.de
Tang, H., Yu, R., Liu, S., Huwatibieke, B., Li, Z., and Zhang, W. (2016). Irisin inhibits hepatic cholesterol synthesis via ampk-srebp2 signaling. EBioMedicine 6, 139–148. Epub 2016/05/24. doi:10.1016/j.ebiom.2016.02.041
Thompson, H. J., Wolfe, P., McTiernan, A., Jiang, W., and Zhu, Z. (2010). Wheel running-induced changes in plasma biomarkers and carcinogenic response in the 1-methyl-1-nitrosourea-induced rat model for breast cancer. Cancer Prev. Res. 3 (11), 1484–1492. Epub 2010/09/30. doi:10.1158/1940-6207.Capr-10-0078
Timmons, B. W., and Cieslak, T. (2008). Human natural killer cell subsets and acute exercise: A brief review. Exerc. Immunol. Rev. 14, 8–23. Epub 2009/02/11.
Tittarelli, A., Janji, B., Van Moer, K., Noman, M. Z., and Chouaib, S. (2015). The selective degradation of synaptic connexin 43 protein by hypoxia-induced autophagy impairs natural killer cell-mediated tumor cell killing. J. Biol. Chem. 290 (39), 23670–23679. Epub 2015/07/30. doi:10.1074/jbc.M115.651547
Turbitt, W. J., Xu, Y., Sosnoski, D. M., Collins, S. D., Meng, H., Mastro, A. M., et al. (2019). Physical activity plus energy restriction prevents 4t1.2 mammary tumor progression, mdsc accumulation, and an immunosuppressive tumor microenvironment. Cancer Prev. Res. 12 (8), 493–506. Epub 2019/07/04. doi:10.1158/1940-6207.Capr-17-0233
Uribe-Querol, E., and Rosales, C. (2015). Neutrophils in cancer: Two sides of the same coin. J. Immunol. Res. 2015, 983698. Epub 2016/01/29. doi:10.1155/2015/983698
Walsh, N. P., Gleeson, M., Shephard, R. J., Gleeson, M., Woods, J. A., Bishop, N. C., et al. (2011). Position statement. Part One: Immune function and exercise. Exerc. Immunol. Rev. 17, 6–63. Epub 2011/03/31.
Wang, J. S., and Liao, C. H. (2004). Moderate-intensity exercise suppresses platelet activation and polymorphonuclear leukocyte interaction with surface-adherent platelets under shear flow in men. Thromb. Haemost. 91 (3), 587–594. Epub 2004/02/26. doi:10.1160/th03-10-0644
Wang, J. S., Li, Y. S., Chen, J. C., and Chen, Y. W. (2005). Effects of exercise training and deconditioning on platelet aggregation induced by alternating shear stress in men. Arterioscler. Thromb. Vasc. Biol. 25 (2), 454–460. Epub 2004/12/01. doi:10.1161/01.Atv.0000151987.04607.24
Wang, J. S., Chang, C. Y., Chow, S. E., Chen, Y. W., and Yang, C. M. (2007). Exercise modulates platelet-nasopharyngeal carcinoma cell aggregation and subsequent tissue factor and matrix metalloproteinase activities. J. Appl. Physiol. 103 (3), 763–770. Epub 2007/05/15. doi:10.1152/japplphysiol.00165.2007
Wang, J. S., Chung, Y., and Chow, S. E. (2009). Exercise affects platelet-impeded antitumor cytotoxicity of natural killer cell. Med. Sci. Sports Exerc. 41 (1), 115–122. Epub 2008/12/19. doi:10.1249/MSS.0b013e3181831f27
Wang, J., Liu, S., Li, G., and Xiao, J. (2020). Exercise regulates the immune system. Adv. Exp. Med. Biol. 1228, 395–408. Epub 2020/04/29. doi:10.1007/978-981-15-1792-1_27
Wang, J. S. (2006). Exercise prescription and thrombogenesis. J. Biomed. Sci. 13 (6), 753–761. Epub 2006/08/26. doi:10.1007/s11373-006-9105-7
Wennerberg, E., Lhuillier, C., Rybstein, M. D., Dannenberg, K., Rudqvist, N. P., Koelwyn, G. J., et al. (2020). Exercise reduces immune suppression and breast cancer progression in a preclinical model. Oncotarget 11 (4), 452–461. Epub 2020/02/18. doi:10.18632/oncotarget.27464
Wu, D., Cao, W., Xiang, D., Hu, Y. P., Luo, B., and Chen, P. (2020). Exercise induces tissue hypoxia and hif-1α redistribution in the small intestine. J. Sport Health Sci. 9 (1), 82–89. Epub 2020/01/11. doi:10.1016/j.jshs.2019.05.002
Wu, X., Zhang, X., Yu, L., Zhang, C., Ye, L., Ren, D., et al. (2020). Zinc finger protein 367 promotes metastasis by inhibiting the Hippo pathway in breast cancer. Oncogene 39 (12), 2568–2582. Epub 2020/01/29. doi:10.1038/s41388-020-1166-y
Xu, Y., Wei, Z., Feng, M., Zhu, D., Mei, S., Wu, Z., et al. (2022). Tumor-infiltrated activated B cells suppress liver metastasis of colorectal cancers. Cell Rep. 40 (9), 111295. Epub 2022/09/01. doi:10.1016/j.celrep.2022.111295
Xue, D., Xia, T., Wang, J., Chong, M., Wang, S., and Zhang, C. (2018). Role of regulatory T cells and Cd8(+) T lymphocytes in the dissemination of circulating tumor cells in primary invasive breast cancer. Oncol. Lett. 16 (3), 3045–3053. Epub 2018/08/22. doi:10.3892/ol.2018.8993
Yazdani, H. O., Kaltenmeier, C., Morder, K., Moon, J., Traczek, M., Loughran, P., et al. (2021). Exercise training decreases hepatic injury and metastases through changes in immune response to liver ischemia/reperfusion in mice. Hepatology 73 (6), 2494–2509. Epub 2020/09/15. doi:10.1002/hep.31552
Zhang, Q. B., Zhang, B. H., Zhang, K. Z., Meng, X. T., Jia, Q. A., Zhang, Q. B., et al. (2016). Moderate swimming suppressed the growth and metastasis of the transplanted liver cancer in mice model: With reference to nervous system. Oncogene 35 (31), 4122–4131. Epub 2015/12/22. doi:10.1038/onc.2015.484
Keywords: physical activity, tumor metastasis, microenvironment, immune function, immune cells
Citation: Zheng A, Zhang L, Yang J, Yin X, Zhang T, Wu X and Ma X (2022) Physical activity prevents tumor metastasis through modulation of immune function. Front. Pharmacol. 13:1034129. doi: 10.3389/fphar.2022.1034129
Received: 01 September 2022; Accepted: 20 September 2022;
Published: 12 October 2022.
Edited by:
Zhijie Xu, Central South University, ChinaCopyright © 2022 Zheng, Zhang, Yang, Yin, Zhang, Wu and Ma. This is an open-access article distributed under the terms of the Creative Commons Attribution License (CC BY). The use, distribution or reproduction in other forums is permitted, provided the original author(s) and the copyright owner(s) are credited and that the original publication in this journal is cited, in accordance with accepted academic practice. No use, distribution or reproduction is permitted which does not comply with these terms.
*Correspondence: Xin Wu, d3V4aW5kb2N0b3JAcXEuY29t; Xuelei Ma, ZHJtYXh1ZWxlaUBnbWFpbC5jb20=
†These authors share first authorship
Disclaimer: All claims expressed in this article are solely those of the authors and do not necessarily represent those of their affiliated organizations, or those of the publisher, the editors and the reviewers. Any product that may be evaluated in this article or claim that may be made by its manufacturer is not guaranteed or endorsed by the publisher.
Research integrity at Frontiers
Learn more about the work of our research integrity team to safeguard the quality of each article we publish.