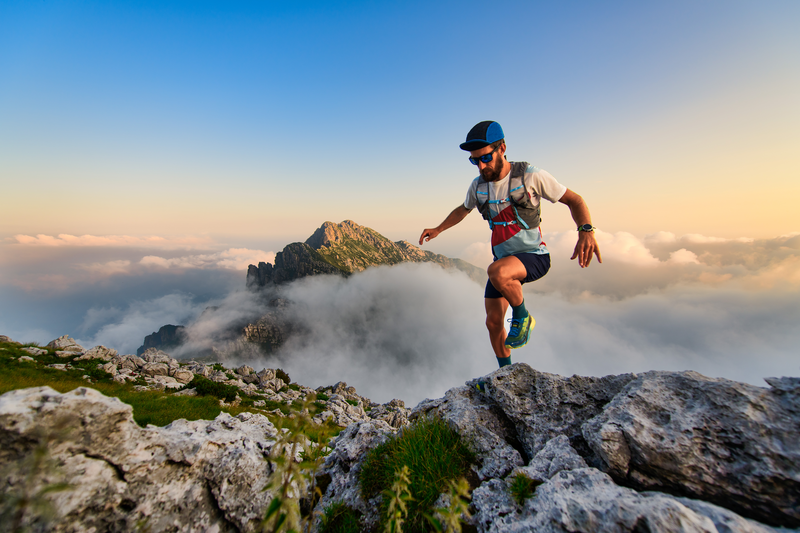
95% of researchers rate our articles as excellent or good
Learn more about the work of our research integrity team to safeguard the quality of each article we publish.
Find out more
MINI REVIEW article
Front. Pharmacol. , 05 October 2022
Sec. Experimental Pharmacology and Drug Discovery
Volume 13 - 2022 | https://doi.org/10.3389/fphar.2022.1030895
This article is part of the Research Topic Purinergic Pharmacology, Volume II View all 14 articles
Adenosine receptors (ARs) have been identified as promising therapeutic targets for countless pathological conditions, spanning from inflammatory diseases to central nervous system disorders, from cancer to metabolic diseases, from cardiovascular pathologies to respiratory diseases, and beyond. This extraordinary therapeutic potential is mainly due to the plurality of pathophysiological actions of adenosine and the ubiquitous expression of its receptors. This is, however, a double-edged sword that makes the clinical development of effective ligands with tolerable side effects difficult. Evidence of this is the low number of AR agonists or antagonists that have reached the market. An alternative approach is to target allosteric sites via allosteric modulators, compounds endowed with several advantages over orthosteric ligands. In addition to the typical advantages of allosteric modulators, those acting on ARs could benefit from the fact that adenosine levels are elevated in pathological tissues, thus potentially having negligible effects on normal tissues where adenosine levels are maintained low. Several A1 and various A3AR allosteric modulators have been identified so far, and some of them have been validated in different preclinical settings, achieving promising results. Less fruitful, instead, has been the discovery of A2A and A2BAR allosteric modulators, although the results obtained up to now are encouraging. Collectively, data in the literature suggests that allosteric modulators of ARs could represent valuable pharmacological tools, potentially able to overcome the limitations of orthosteric ligands.
Adenosine is a fundamental component of human physiology. It is a major constituent of nucleic acids, of life’s “energy currency” and signaling molecule adenosine triphosphate (ATP), as well as a ubiquitous cell function modulator itself. Adenosine acts as an autocrine/paracrine mediator with a short half-life whose low extracellular levels in healthy tissues are maintained mostly by rapid cellular uptake and cytosolic metabolism by adenosine deaminase or adenosine kinase (Haskó et al., 2008). However, following tissue injury, cells release large amounts of ATP, which is then converted to adenosine by ecto-nucleotidases. Generally, the resulting increased concentration of adenosine has largely beneficial effects in acute pathological conditions by restoring tissue homeostasis (Borea et al., 2016), while its chronic overproduction can be detrimental and cause inflammation, fibrosis, and organ damage (Borea et al., 2017). Adenosine triggers its effects through the interaction with four G-protein coupled receptors (GPCRs), named A1, A2A, A2B, and A3 adenosine receptors (ARs). Some of the biological functions of adenosine include, but are not limited to, regulation of neurotransmitter release, neuronal excitability, heart rate and contractility, blood flow, platelet aggregation, inflammation and immune system responses, wound healing, and metabolic processes (Borea et al., 2018). In addition to the several physiological effects of adenosine, its receptor-mediated signaling has many documented effects on the progression of countless pathological states (Karmouty-Quintana et al., 2013). Among the main ones, modulation of adenosine receptors has been indicated as a promising therapeutic strategy in pathological states such as cancer (Vijayan et al., 2017; Allard et al., 2020), cardiovascular diseases (Reiss et al., 2019), pain (Vincenzi et al., 2020a), neurological/neurodegenerative diseases (Blum et al., 2018; Sebastião et al., 2018; Jenner et al., 2021; Merighi et al., 2021), neuropsychiatric disorders (Pasquini et al., 2022), inflammatory diseases (Pasquini et al., 2021; Antonioli et al., 2022), respiratory diseases (Caruso et al., 2013), ocular diseases (Spinozzi et al., 2021), diabetes, and other metabolic disorders (Antonioli et al., 2015; Sanni and Terre’Blanche, 2021). Despite this encouraging profusion of experimental evidence, relatively few adenosinergic system-based drugs have so far achieved clinical approval. When looking for accountability for this lack of finalization, this cannot be attributed to the lack of highly affine and selective ligands, as the search for new ligands has been quite productive (Jacobson et al., 2021; IJzerman et al., 2022), but rather the redundancy of adenosine signaling, the agonist-dependent receptor desensitization, and the broad expression of ARs provide the biggest challenges (Peleli et al., 2017). As a result of these drawbacks, most attempts to test orthosteric AR ligands in clinical trials have failed due to inefficiency or serious and unfavorable side effects. Different strategies were explored to overcome the above-mentioned obstacles, including partial agonists (Greene et al., 2016; Voors et al., 2019), indirect receptor targeting (Kutryb-Zajac et al., 2020; Wang et al., 2021), prodrugs (Suresh et al., 2020), multi-target drugs (Huang et al., 2011), but one of the most promising seems to be allosteric modulation. By affecting endogenous agonist affinity and/or efficacy, a positive allosteric modulator (PAM) is an allosteric ligand that enhances an agonist-mediated receptor response, while a negative allosteric modulator (NAM) attenuates activity (Gentry et al., 2015). Other classes include neutral allosteric ligands (NAL) that bind at the allosteric site without affecting receptor or orthosteric ligand activity and allosteric agonists, ligands that directly activate the receptors from the allosteric site even in the absence of an orthosteric agonist. Traditionally, GPCRs have been targeted using compounds that bind to the orthosteric site. Allosteric ligands, binding at sites that are topologically distinct from the orthosteric sites, have expanded the ways to manipulate GPCR functionality, providing several pharmacological advantages and potential therapeutic benefits (Wootten et al., 2013). Due to the reduced evolutionary pressure that would ordinarily be necessary to maintain an orthosteric binding pocket capable of accepting the endogenous ligand, allosteric sites are less conserved among related receptor subtypes (Wild et al., 2014). Furthermore, since allosteric modulators may cause a variety of conformational changes in GPCR structures, they can be rationally tailored to create a strong biased signaling response from a GPCR triggered by an otherwise non-biased orthosteric ligand (Wold and Zhou, 2018). By imparting biased modulation upon orthosteric agonists, these allosteric modulators have the ability to only enhance therapeutically relevant signaling while preventing on-target side effects (Gao et al., 2011; Slosky et al., 2021). Apart from allosteric agonists, allosteric modulators such as PAMs and NAMs only have an effect in the presence of orthosteric ligands and can enhance or decrease receptor activation induced by endogenous agonists. Therefore, they act more physiologically and are predicted to have fewer adverse effects and tolerance-inducing consequences than orthosteric ligands. A particularly crucial element in the case of the short-lived molecule adenosine is the ability of PAMs and NAMs to finely tune its activity by following the spatiotemporal distribution of its extracellular concentration. Another important advantage is the reciprocal communication with the orthosteric domain: the allosteric modulator exerts an effect on the binding of the endogenous ligand, but the latter can also affect the binding of the modulator. This mechanism supports the selectivity of allosteric ligands, especially under conditions where there is a pathology-dependent alteration in the concentration of the endogenous agonist at a particular site (Draper-Joyce et al., 2021). This review summarizes the advances in the development of ARs allosteric modulators (Table 1) that may provide support for their use as new therapeutic options.
A1ARs are widespread and implicated in many physiological mechanisms, therefore they are regarded as a prominent drug target for different diseases. Adenosine through A1ARs exerts sedative, anticonvulsant, anxiolytic, and locomotor depressant effects (Varani et al., 2017). Furthermore, the heart rate and rhythm, the conduction speed in the atrioventricular node, and cardiac muscle contraction are negatively controlled by A1ARs (Deb et al., 2019; Jacobson et al., 2019). In particular, A1AR agonists mediate cardioprotection through the inhibition of norepinephrine release (Dinh et al., 2017). An important role of A1ARs is in nociception, due to their location in peripheral sensory nerve terminals in the spinal cord dorsal horn and in supraspinal pain-processing structures (Sawynok, 2016; Vincenzi et al., 2020a). Many studies have been conducted to exploit the therapeutic potential of these receptors, but the development of orthosteric agonists has been hampered by several drawbacks, the main ones being cardiac side effects and receptor desensitization. An alternative strategy to exploit the positive effects of A1AR stimulation is allosteric modulation. Much research effort in recent decades has been devoted to the synthesis and in vitro and in vivo evaluation of A1AR PAMs (Romagnoli et al., 2015b; Jacobson and Gao, 2016). The first and most extensively studied class of compounds synthesized are the benzoylthiophene derivatives, the prototype of which is PD 81,723 (Bruns et al., 1990). Different studies revealed a potential application of PD 81,723 in ischemic injury (Meno et al., 2003; Park et al., 2012). Another extensively studied compound belonging to this class of modulators is T62 (Baraldi et al., 2000). It was effective in reducing nociception and hypersensitivity in animal models of neuropathic pain (Pan et al., 2001; Li et al., 2002, 2003; Obata et al., 2004). It was also used in a phase II clinical trial for postherpetic neuropathic pain. However, the study was abandoned due to a lack of efficacy and the presence of transient high levels of liver transaminase in some patients (Giorgi and Nieri, 2013). Subsequently, numerous other derivatives were discovered, endowed with greater allosteric activity (Romagnoli et al., 2008, 2012, 2013, 2014; 2015a). Of these, TRR469 was selected for in vivo studies. TRR469 has been reported to have an analgesic effect comparable to that of morphine in animal models of both acute and neuropathic pain without showing the side effects typical of orthosteric A1AR agonists such as locomotor disturbances or sedation (Vincenzi et al., 2014). This compound also proved effective as an anxiolytic in several mouse models of anxiety with an effect comparable to that of diazepam but without the locomotor side effects typical of benzodiazepines (Vincenzi et al., 2016). Also noteworthy is the protective effect of TRR469 found in an in vitro model of glutamate-induced cytotoxicity in neuronal cells (Vincenzi et al., 2020b). Another series of 2-amino-3-benzoylthiophene A1AR PAMs were synthesized (Aurelio et al., 2009), including VCP171, whose in vivo analgesic effect in a model of neuropathic pain proved weaker than that of the orthosteric A1AR agonist, but which nevertheless has greater therapeutic potential due to fewer side effects, particularly in tissues with higher adenosine concentrations or A1AR tone (Imlach et al., 2015). Instead, VCP333 has been shown to improve cardiac function and reduce cardiomyocyte death following cardiac ischemia (Butcher et al., 2013). The most recently synthesized is MIPS521, an A1AR PAM that has shown analgesic effects in models of neuropathic pain by being able to modulate the high concentrations of adenosine present. Interestingly, a new binding pocket was identified by studying the structure of the A1AR bound to adenosine, MIPS521, and the Gi protein. This lead to hypothesize that the modulator also exerts its effects by stabilizing the adenosine-receptor-G protein complex (Draper-Joyce et al., 2021). Recently, a multisite binding model for A1AR allosteric modulation has been proposed. It predicts that there are several extracellular sites capable of binding the modulator, not just a distinct pocket generally located on the second extracellular loop (Deganutti et al., 2021).
Adenosine, mainly through the activation of A2AARs expressed in peripheral immune cells, represents a potent inflammatory self-limiting factor (Antonioli et al., 2022). Depending on the pathology, this can have both positive and negative impacts. On the positive side, A2AAR activation is potentially useful for the treatment of autoimmune and inflammatory diseases (Vincenzi et al., 2013), as evidenced by the fact that the anti-inflammatory and immunosuppressive effects of methotrexate, a gold standard for the treatment of rheumatoid arthritis, as well as some of the anti-inflammatory effects of sulfasalazine, are mediated by adenosine (Cronstein and Sitkovsky, 2017). Although A2AAR agonists may be effective in the treatment of inflammatory illnesses, they are likely to have too many adverse effects to be tolerated, mainly owing to their significant hypotensive effect. One alternative approach that could potentially circumvent the agonist-related side effects while enhancing the potent anti-inflammatory action of adenosine is represented by A2AAR PAMs. On the negative side of adenosine-mediated inflammation suppression, many solid tumors escape immune response by increasing the concentration of adenosine in the tumor microenvironment. Both animal studies and clinical trials have shown that blocking A2AAR can induce tumor regression (Sun et al., 2022). Although not yet tested, one can speculate that A2AAR NAM could potentially counteract in a spatial-selective manner the tumor-increased adenosine immunosuppressive action. In the CNS, blockade of A2AARs is indicated, with varying degrees of preclinical and clinical evidence, as a promising therapeutic strategy for Parkinson’s disease, supported by the recent approval of the antagonist istradefylline as add-on therapy (Chen and Cunha, 2020), but also for Alzheimer’s disease (Merighi et al., 2022), acute brain dysfunction (Cunha, 2016), and some neuropsychiatric disorders such as fragile X syndrome, depression, and anxiety (Domenici et al., 2019).
Unfortunately, only a small number of A2AAR allosteric modulators have been reported so far. Some N6-1,3-diphenylurea derivatives of 2-phenyl-9-benzyladenines and 8-azaadenines have been suggested to act as allosteric modulators at the A2AARs (Giorgi et al., 2008). Later, using a fragment screening technique, some PAMs and NAMs of ARs were identified. In particular, ZB1854 potentiated the action of the A2AAR agonist CGS 21680, thereby behaving as a PAM (Chen et al., 2012). A compound denoted as AEA061 increased adenosine’s anti-inflammatory properties by allosterically enhancing its activity at A2AARs in the lipopolysaccharide (LPS)-induced mouse model of inflammation (Welihinda and Amento, 2014). In a subsequent work, AEA061 was also shown to enhance inosine-mediated A2AAR activation and consequent inhibition of pro-inflammatory cytokine and chemokine production in splenic monocytes (Welihinda et al., 2018). Very recently, AEA061 also reduced clinical scores and cytokine expression in two different models of psoriasis-like dermatitis induced by imoquimod or IL-23 (Welihinda et al., 2022). Another A2AAR PAM, named A2AR PAM-1, increased the total amount of slow wave sleep, from which individuals with insomnia might benefit, without affecting blood pressure, heart rhythm, and body temperature as the agonist CGS21680 did (Korkutata et al., 2017, 2019).
The A2BAR is widely expressed in organs such as the bladder, intestine, and lung, as well as in various cell types such as fibroblasts, smooth muscle, endothelial, immune, and alveolar epithelial cells (Borea et al., 2018). Of all the ARs, the A2B subtype is the least characterized from a pharmacological point of view. It has been proposed as a potential target in acute lung injury, as its activation with the agonist BAY 60–6,583 led to a reduction in inflammation and pulmonary edema, and an increase in alveolar fluid clearance (Eckle et al., 2013; Hoegl et al., 2015; Wang et al., 2020). Recently, Gnad and others found that activation of A2BARs restores muscle and brown fat function in elderly and obese mice to that of young, lean animals, establishing its anti-aging and anti–obesity potential (Gnad et al., 2022). In addition, it has been suggested that A2BARs have therapeutic potential in bone diseases, as their activation appears to promote osteoblast differentiation and bone formation (Carroll et al., 2012; Corciulo et al., 2016).
The first class of allosteric modulators for the A2BAR, a series of 1-benzyl-3-ketoindoles, was serendipitously discovered (starting from a scaffold previously used to develop benzodiazepine receptor ligands) and consisted of three PAM and four NAM (Taliani et al., 2013; Trincavelli et al., 2014b). Subsequently, one of these A2BAR PAMs, denoted as KI-7, was shown to enhance the effects of adenosine and synthetic A2BAR agonists in the differentiation of mesenchymal stem cells (MSC) to osteoblasts while also increasing differentiated osteoblast viability (Trincavelli et al., 2014a). More recently, a series of novel derivatives chemically related to those previously synthesized has been reported. One of these compounds, a benzofurane derivative that was confirmed to behave as A2BAR PAM, stimulated matrix mineralization in MSC, making it a lead structure for the synthesis of new compounds with anti-osteoporosis properties (Barresi et al., 2021a; 2021b).
Since A2BAR blockade may represent a promising approach for the treatment of some diseases, such as in cancer immunotherapy (Gao and Jacobson, 2019), A2BAR NAM could also result in a valuable pharmacological resource. Interestingly, the well-known selective A2BAR antagonist PSB 603 was recently suggested to act as a NAM, at least in A2B-mediated cAMP accumulation in HEK 293 cells (Goulding et al., 2018).
A3AR is expressed in the brain, heart, testis, lung, placenta, uterus, kidneys, spleen, liver, bladder, and proximal colon, but, while low expression is found in normal cells, this receptor subtype is overexpressed in immune and cancer cells (Gessi et al., 2008). The activation of A3AR mediates anti-inflammatory, antitumor, and anti-ischemic beneficial effects, showing a therapeutic potential for the treatment of inflammatory diseases, such as rheumatoid arthritis and psoriasis, hepatitis, cancer, glaucoma, cardiovascular diseases, and cerebral ischemia (Borea et al., 2015).
In addition to some selective A3AR agonists, several series of allosteric modulators, mainly PAMs, have also been developed, representing an alternative approach for the treatment of those aforementioned diseases in which A3AR activation appears to be a promising therapeutic strategy (Gao et al., 2001, 2002; Göblyös et al., 2006; Heitman et al., 2009; Kim et al., 2009). However, as opposed to orthosteric agonists, A3AR PAMs have the benefit of being able to target regions where adenosine levels are elevated, such as tumor and inflammatory sites, with low or no effects on normal tissues where adenosine levels are low.
Among the most well-known A3AR PAMS are the LUF6000 and LUF6096. LUF6000 is an imidazoquinolinamine PAM at the A3AR that showed anti-inflammatory effects in a rat adjuvant-induced arthritis model, inhibited monoiodoacetate-induced osteoarthritis development, and exhibited protective effects in a liver inflammation model of acute hepatitis in mice. At the molecular level, LUF6000 administration resulted in a marked deregulation of the NF-κB signaling pathway (Cohen et al., 2014). Itzhak and co-workers evaluated the effect of LUF6000 (also known as CF602) on resolving erectile dysfunction (ED) in a diabetic ED rat model. CF602 increased intracavernosal pressure, endothelial nitric oxide synthase (eNOS), and vascular endothelial growth factor (VEGF) levels, improving erectile function (Itzhak et al., 2022). This compound may thus provide an alternative treatment for phosphodiesterase 5 (PDE5) inhibitors, which are usually employed in ED therapy, considering that half of the patients with diabetes do not respond to PDE5 inhibitors.
In addition, LUF6096, which is structurally similar to LUF6000, reduced infarct size in a barbital-anesthetized dog model of myocardial ischemia/reperfusion injury. The infarct size reduction was equally evident when LUF6096 was administered in two doses before coronary artery occlusion and immediately before reperfusion or a single dose immediately before reperfusion (Du et al., 2012).
Studies conducted by Lane and others suggest that the endocannabinoid two- arachidonylglycerol (2-AG) acts as a NAM at the A3AR. This evidence may be especially important in certain pathological conditions like cerebral ischemia when levels of 2-AG are elevated and could interact with A3AR expressed in astrocytes and microglia (Lane et al., 2010).
GPCR allosteric modulators are promising therapeutic agents. By altering the receptor conformation, they potentiate or attenuate the effect of the endogenous agonist, acting more physiologically than orthosteric ligands and offering spatiotemporal selectivity. The adenosinergic system, making use of a short-lived autocrine/paracrine mediator, represents an ideal situation to take advantage of the benefits of allosteric modulation. The available preclinical results are encouraging, and there is hope for an acceleration that may lead to the clinical use of allosteric modulators of ARs. Nevertheless, no allosteric modulator has entered clinical trials to date, underlining the challenges in the discovery and development of this class of compounds. Allosteric sites generally have a shallow structure-activity relationship and are often unknown or difficult to discover as they are only accessible in specific protein conformations. The fact that allosteric sites are less evolutionarily conserved than orthosteric ones can lead to species differences that can hamper their validation. Furthermore, allosteric modulators have a high propensity for molecular switching and can show complex in vivo pharmacology. Despite these challenges to identifying, validating, and developing allosteric modulators for GPCRs, they have the potential to become one of the most highly effective and minimally toxic pharmacological agents.
SP, PB, KV, and FV conceived the work and wrote the manuscript. CC and MC contributed to writing and editing. All authors contributed to the article and approved the submitted version.
The authors declare that the research was conducted in the absence of any commercial or financial relationships that could be construed as a potential conflict of interest.
All claims expressed in this article are solely those of the authors and do not necessarily represent those of their affiliated organizations, or those of the publisher, the editors and the reviewers. Any product that may be evaluated in this article, or claim that may be made by its manufacturer, is not guaranteed or endorsed by the publisher.
Allard, B., Allard, D., Buisseret, L., and Stagg, J. (2020). The adenosine pathway in immuno-oncology. Nat. Rev. Clin. Oncol. 17, 611–629. doi:10.1038/s41571-020-0382-2
Antonioli, L., Blandizzi, C., Csóka, B., Pacher, P., and Haskó, G. (2015). Adenosine signalling in diabetes mellitus--pathophysiology and therapeutic considerations. Nat. Rev. Endocrinol. 11, 228–241. doi:10.1038/nrendo.2015.10
Antonioli, L., Pacher, P., and Haskó, G. (2022). Adenosine and inflammation: it’s time to (re)solve the problem. Trends Pharmacol. Sci. 43, 43–55. doi:10.1016/j.tips.2021.10.010
Aurelio, L., Valant, C., Flynn, B. L., Sexton, P. M., Christopoulos, A., and Scammells, P. J. (2009). Allosteric modulators of the adenosine A1 receptor: Synthesis and pharmacological evaluation of 4-substituted 2-Amino-3-benzoylthiophenes. J. Med. Chem. 52, 4543–4547. doi:10.1021/jm9002582
Baraldi, P. G., Zaid, A. N., Lampronti, I., Fruttarolo, F., Pavani, M. G., Tabrizi, M. A., et al. (2000). Synthesis and biological effects of a new series of 2-amino-3-benzoylthiophenes as allosteric enhancers of A1-adenosine receptor. Bioorg. Med. Chem. Lett. 10, 1953–1957. doi:10.1016/S0960-894X(00)00379-6
Barresi, E., Giacomelli, C., Marchetti, L., Baglini, E., Salerno, S., Greco, G., et al. (2021a). Novel positive allosteric modulators of A2B adenosine receptor acting as bone mineralisation promoters. J. Enzyme Inhib. Med. Chem. 36, 286–294. doi:10.1080/14756366.2020.1862103
Barresi, E., Martini, C., Da Settimo, F., Greco, G., Taliani, S., Giacomelli, C., et al. (2021b). Allosterism vs. Orthosterism: Recent findings and future perspectives on A2B AR physio-pathological implications. Front. Pharmacol. 12, 652121. doi:10.3389/fphar.2021.652121
Blum, D., Chern, Y., Domenici, M. R., Buée, L., Lin, C.-Y., Rea, W., et al. (2018). The role of adenosine tone and adenosine receptors in huntington’s disease. J. Caffeine Adenosine Res. 8, 43–58. doi:10.1089/caff.2018.0006
Borea, P. A., Gessi, S., Merighi, S., and Varani, K. (2016). Adenosine as a multi-signalling guardian angel in human diseases: When, where and how does it exert its protective effects? Trends Pharmacol. Sci. 37, 419–434. doi:10.1016/j.tips.2016.02.006
Borea, P. A., Gessi, S., Merighi, S., Vincenzi, F., and Varani, K. (2017). Pathological overproduction: The bad side of adenosine. Br. J. Pharmacol. 174, 1945–1960. doi:10.1111/bph.13763
Borea, P. A., Gessi, S., Merighi, S., Vincenzi, F., and Varani, K. (2018). Pharmacology of adenosine receptors: The state of the art. Physiol. Rev. 98, 1591–1625. doi:10.1152/physrev.00049.2017
Borea, P. A., Varani, K., Vincenzi, F., Baraldi, P. G., Tabrizi, M. A., Merighi, S., et al. (2015). The A3 adenosine receptor: History and perspectives. Pharmacol. Rev. 67, 74–102. doi:10.1124/pr.113.008540
Bruns, R. F., Fergus, J. H., Coughenour, L. L., Courtland, G. G., Pugsley, T. A., Dodd, J. H., et al. (1990). Structure-activity relationships for enhancement of adenosine A1 receptor binding by 2-amino-3-benzoylthiophenes. Mol. Pharmacol. 38, 950–958.
Butcher, A., Scammells, P. J., White, P. J., Devine, S. M., and Rose’meyer, R. B. (2013). An allosteric modulator of the adenosine A1 receptor improves cardiac function following ischaemia in murine isolated hearts. Pharm. (Basel) 6, 546–556. doi:10.3390/ph6040546
Carroll, S. H., Wigner, N. A., Kulkarni, N., Johnston-Cox, H., Gerstenfeld, L. C., and Ravid, K. (2012). A2B adenosine receptor promotes mesenchymal stem cell differentiation to osteoblasts and bone formation in vivo. J. Biol. Chem. 287, 15718–15727. doi:10.1074/jbc.M112.344994
Caruso, M., Alamo, A., Crisafulli, E., Raciti, C., Fisichella, A., and Polosa, R. (2013). Adenosine signaling pathways as potential therapeutic targets in respiratory disease. Expert Opin. Ther. Targets 17, 761–772. doi:10.1517/14728222.2013.795220
Chen, D., Errey, J. C., Heitman, L. H., Marshall, F. H., Ijzerman, A. P., and Siegal, G. (2012). Fragment screening of GPCRs using biophysical methods: Identification of ligands of the adenosine A2A receptor with novel biological activity. ACS Chem. Biol. 7, 2064–2073. doi:10.1021/cb300436c
Chen, J.-F., and Cunha, R. A. (2020). The belated US FDA approval of the adenosine A2A receptor antagonist istradefylline for treatment of Parkinson’s disease. Purinergic Signal. 16, 167–174. doi:10.1007/s11302-020-09694-2
Cohen, S., Barer, F., Bar-Yehuda, S., Ijzerman, A. P., Jacobson, K. A., and Fishman, P. (2014). A₃ adenosine receptor allosteric modulator induces an anti-inflammatory effect: In vivo studies and molecular mechanism of action. Mediat. Inflamm. 2014, 708746. doi:10.1155/2014/708746
Corciulo, C., Wilder, T., and Cronstein, B. N. (2016). Adenosine A2B receptors play an important role in bone homeostasis. Purinergic Signal. 12, 537–547. doi:10.1007/s11302-016-9519-2
Cronstein, B. N., and Sitkovsky, M. (2017). Adenosine and adenosine receptors in the pathogenesis and treatment of rheumatic diseases. Nat. Rev. Rheumatol. 13, 41–51. doi:10.1038/nrrheum.2016.178
Cunha, R. A. (2016). How does adenosine control neuronal dysfunction and neurodegeneration? J. Neurochem. 139, 1019–1055. doi:10.1111/jnc.13724
Deb, P. K., Deka, S., Borah, P., Abed, S. N., and Klotz, K.-N. (2019). Medicinal chemistry and therapeutic potential of agonists, antagonists and allosteric modulators of A1 adenosine receptor: Current status and perspectives. Curr. Pharm. Des. 25, 2697–2715. doi:10.2174/1381612825666190716100509
Deganutti, G., Barkan, K., Ladds, G., and Reynolds, C. A. (2021). Multisite model of allosterism for the adenosine A1 receptor. J. Chem. Inf. Model. 61, 2001–2015. doi:10.1021/acs.jcim.0c01331
Dinh, W., Albrecht-Küpper, B., Gheorghiade, M., Voors, A. A., van der Laan, M., and Sabbah, H. N. (2017). Partial adenosine A1 agonist in heart failure. Handb. Exp. Pharmacol. 243, 177–203. doi:10.1007/164_2016_83
Domenici, M. R., Ferrante, A., Martire, A., Chiodi, V., Pepponi, R., Tebano, M. T., et al. (2019). Adenosine A2A receptor as potential therapeutic target in neuropsychiatric disorders. Pharmacol. Res. 147, 104338. doi:10.1016/j.phrs.2019.104338
Draper-Joyce, C. J., Bhola, R., Wang, J., Bhattarai, A., Nguyen, A. T. N., Cowie-Kent, I., et al. (2021). Positive allosteric mechanisms of adenosine A1 receptor-mediated analgesia. Nature 597, 571–576. doi:10.1038/s41586-021-03897-2
Du, L., Gao, Z.-G., Nithipatikom, K., Ijzerman, A. P., Veldhoven, J. P. D. van, Jacobson, K. A., et al. (2012). Protection from myocardial ischemia/reperfusion injury by a positive allosteric modulator of the A₃ adenosine receptor. J. Pharmacol. Exp. Ther. 340, 210–217. doi:10.1124/jpet.111.187559
Eckle, T., Hughes, K., Ehrentraut, H., Brodsky, K. S., Rosenberger, P., Choi, D.-S., et al. (2013). Crosstalk between the equilibrative nucleoside transporter ENT2 and alveolar Adora2b adenosine receptors dampens acute lung injury. FASEB J. 27, 3078–3089. doi:10.1096/fj.13-228551
Gao, Z.-G., and Jacobson, K. A. (2019). A2B adenosine receptor and cancer. Int. J. Mol. Sci. 20, 5139. doi:10.3390/ijms20205139
Gao, Z.-G., Kim, S. G., Soltysiak, K. A., Melman, N., Ijzerman, A. P., and Jacobson, K. A. (2002). Selective allosteric enhancement of agonist binding and function at human A3 adenosine receptors by a series of imidazoquinoline derivatives. Mol. Pharmacol. 62, 81–89. doi:10.1124/mol.62.1.81
Gao, Z.-G., Verzijl, D., Zweemer, A., Ye, K., Göblyös, A., Ijzerman, A. P., et al. (2011). Functionally biased modulation of A(3) adenosine receptor agonist efficacy and potency by imidazoquinolinamine allosteric enhancers. Biochem. Pharmacol. 82, 658–668. doi:10.1016/j.bcp.2011.06.017
Gao, Z. G., Van Muijlwijk-Koezen, J. E., Chen, A., Müller, C. E., Ijzerman, A. P., and Jacobson, K. A. (2001). Allosteric modulation of A(3) adenosine receptors by a series of 3-(2-pyridinyl)isoquinoline derivatives. Mol. Pharmacol. 60, 1057–1063. doi:10.1124/mol.60.5.1057
Gentry, P. R., Sexton, P. M., and Christopoulos, A. (2015). Novel allosteric modulators of G protein-coupled receptors. J. Biol. Chem. 290, 19478–19488. doi:10.1074/jbc.R115.662759
Gessi, S., Merighi, S., Varani, K., Leung, E., Mac Lennan, S., and Borea, P. A. (2008). The A3 adenosine receptor: An enigmatic player in cell biology. Pharmacol. Ther. 117, 123–140. doi:10.1016/j.pharmthera.2007.09.002
Giorgi, I., Biagi, G., Bianucci, A. M., Borghini, A., Livi, O., Leonardi, M., et al. (2008). N6-1, 3-diphenylurea derivatives of 2-phenyl-9-benzyladenines and 8-azaadenines: Synthesis and biological evaluation as allosteric modulators of A2A adenosine receptors. Eur. J. Med. Chem. 43, 1639–1647. doi:10.1016/j.ejmech.2007.10.021
Giorgi, I., and Nieri, P. (2013). Adenosine A1 modulators: A patent update (2008 to present). Expert Opin. Ther. Pat. 23, 1109–1121. doi:10.1517/13543776.2013.799142
Gnad, T., Navarro, G., Lahesmaa, M., Reverte-Salisa, L., Copperi, F., Cordomi, A., et al. (2022). Adenosine/A2B receptor signaling ameliorates the effects of aging and counteracts obesity. Cell Metab. 34, 56–70. doi:10.1016/j.cmet.2020.06.006
Göblyös, A., Gao, Z.-G., Brussee, J., Connestari, R., Santiago, S. N., Ye, K., et al. (2006). Structure-activity relationships of new 1H-imidazo[4, 5-c]quinolin-4-amine derivatives as allosteric enhancers of the A3 adenosine receptor. J. Med. Chem. 49, 3354–3361. doi:10.1021/jm060086s
Goulding, J., May, L. T., and Hill, S. J. (2018). Characterisation of endogenous A2A and A2B receptor-mediated cyclic AMP responses in HEK 293 cells using the GloSensorTM biosensor: Evidence for an allosteric mechanism of action for the A2B-selective antagonist PSB 603. Biochem. Pharmacol. 147, 55–66. doi:10.1016/j.bcp.2017.10.013
Greene, S. J., Sabbah, H. N., Butler, J., Voors, A. A., Albrecht-Küpper, B. E., Düngen, H.-D., et al. (2016). Partial adenosine A1 receptor agonism: A potential new therapeutic strategy for heart failure. Heart fail. Rev. 21, 95–102. doi:10.1007/s10741-015-9522-7
Haskó, G., Linden, J., Cronstein, B., and Pacher, P. (2008). Adenosine receptors: Therapeutic aspects for inflammatory and immune diseases. Nat. Rev. Drug Discov. 7, 759–770. doi:10.1038/nrd2638
Heitman, L. H., Göblyös, A., Zweemer, A. M., Bakker, R., Mulder-Krieger, T., van Veldhoven, J. P. D., et al. (2009). A series of 2, 4-disubstituted quinolines as a new class of allosteric enhancers of the adenosine A3 receptor. J. Med. Chem. 52, 926–931. doi:10.1021/jm8014052
Hoegl, S., Brodsky, K. S., Blackburn, M. R., Karmouty-Quintana, H., Zwissler, B., and Eltzschig, H. K. (2015). Alveolar epithelial A2B adenosine receptors in pulmonary protection during acute lung injury. J. Immunol. 195, 1815–1824. doi:10.4049/jimmunol.1401957
Huang, N.-K., Lin, J.-H., Lin, J.-T., Lin, C.-I., Liu, E. M., Lin, C.-J., et al. (2011). A new drug design targeting the adenosinergic system for huntington’s disease. PLOS ONE 6, e20934. doi:10.1371/journal.pone.0020934
Ijzerman, A. P., Jacobson, K. A., Müller, C. E., Cronstein, B. N., and Cunha, R. A. (2022). International union of basic and clinical Pharmacology. CXII: Adenosine receptors: A further update. Pharmacol. Rev. 74, 340–372. doi:10.1124/pharmrev.121.000445
Imlach, W. L., Bhola, R. F., May, L. T., Christopoulos, A., and Christie, M. J. (2015). A positive allosteric modulator of the adenosine A1 receptor selectively inhibits primary afferent synaptic transmission in a neuropathic pain model. Mol. Pharmacol. 88, 460–468. doi:10.1124/mol.115.099499
Itzhak, I., Cohen, S., Fishman, S., and Fishman, P. (2022). A3 adenosine receptor allosteric modulator CF602 reverses erectile dysfunction in a diabetic rat model. Andrologia 54, e14498. doi:10.1111/and.14498
Jacobson, K. A., and Gao, Z.-G. (2016). CHAPTER 11:allosteric modulators of adenosine, P2Y and P2X receptors. Allosterism in Drug Discovery, 247–270. doi:10.1039/9781782629276-00247
Jacobson, K. A., Ijzerman, A. P., and Müller, C. E. (2021). Medicinal chemistry of P2 and adenosine receptors: Common scaffolds adapted for multiple targets. Biochem. Pharmacol. 187, 114311. doi:10.1016/j.bcp.2020.114311
Jacobson, K. A., Tosh, D. K., Jain, S., and Gao, Z.-G. (2019). Historical and current adenosine receptor agonists in preclinical and clinical development. Front. Cell. Neurosci. 13, 124. doi:10.3389/fncel.2019.00124
Jenner, P., Mori, A., Aradi, S. D., and Hauser, R. A. (2021). Istradefylline - a first generation adenosine A2A antagonist for the treatment of Parkinson’s disease. Expert Rev. Neurother. 21, 317–333. doi:10.1080/14737175.2021.1880896
Karmouty-Quintana, H., Xia, Y., and Blackburn, M. R. (2013). Adenosine signaling during acute and chronic disease states. J. Mol. Med. 91, 173–181. doi:10.1007/s00109-013-0997-1
Kim, Y., de Castro, S., Gao, Z.-G., Ijzerman, A. P., and Jacobson, K. A. (2009). Novel 2- and 4-substituted 1H-imidazo[4, 5-c]quinolin-4-amine derivatives as allosteric modulators of the A3 adenosine receptor. J. Med. Chem. 52, 2098–2108. doi:10.1021/jm801659w
Korkutata, M., Saitoh, T., Cherasse, Y., Ioka, S., Duo, F., Qin, R., et al. (2019). Enhancing endogenous adenosine A2A receptor signaling induces slow-wave sleep without affecting body temperature and cardiovascular function. Neuropharmacology 144, 122–132. doi:10.1016/j.neuropharm.2018.10.022
Korkutata, M., Saitoh, T., Feng, D., Murakoshi, N., Sugiyama, F., Cherasse, Y., et al. (2017). Allosteric modulation of adenosine A2A receptors in mice induces slow-wave sleep without cardiovascular effects. Sleep. Med. 40, e181. doi:10.1016/j.sleep.2017.11.530
Kutryb-Zajac, B., Mierzejewska, P., Slominska, E. M., and Smolenski, R. T. (2020). Therapeutic perspectives of adenosine deaminase inhibition in cardiovascular diseases. Molecules 25, E4652. doi:10.3390/molecules25204652
Lane, J. R., Beukers, M. W., Mulder-Krieger, T., and Ijzerman, A. P. (2010). The endocannabinoid 2-arachidonylglycerol is a negative allosteric modulator of the human A3 adenosine receptor. Biochem. Pharmacol. 79, 48–56. doi:10.1016/j.bcp.2009.07.024
Li, X., Conklin, D., Ma, W., Zhu, X., and Eisenach, J. C. (2002). Spinal noradrenergic activation mediates allodynia reduction from an allosteric adenosine modulator in a rat model of neuropathic pain. Pain 97, 117–125. doi:10.1016/s0304-3959(02)00011-8
Li, X., Conklin, D., Pan, H.-L., and Eisenach, J. C. (2003). Allosteric adenosine receptor modulation reduces hypersensitivity following peripheral inflammation by a central mechanism. J. Pharmacol. Exp. Ther. 305, 950–955. doi:10.1124/jpet.102.047951
Meno, J. R., Higashi, H., Cambray, A. J., Zhou, J., D’Ambrosio, R., and Winn, H. R. (2003). Hippocampal injury and neurobehavioral deficits are improved by PD 81, 723 following hyperglycemic cerebral ischemia. Exp. Neurol. 183, 188–196. doi:10.1016/s0014-4886(03)00162-6
Merighi, S., Borea, P. A., Varani, K., Vincenzi, F., Travagli, A., Nigro, M., et al. (2022). Pathophysiological role and medicinal chemistry of A2A adenosine receptor antagonists in Alzheimer’s disease. Molecules 27, 2680. doi:10.3390/molecules27092680
Merighi, S., Poloni, T. E., Pelloni, L., Pasquini, S., Varani, K., Vincenzi, F., et al. (2021). An open question: Is the A2A adenosine receptor a novel target for Alzheimer’s disease treatment? Front. Pharmacol. 12, 652455. doi:10.3389/fphar.2021.652455
Obata, H., Li, X., and Eisenach, J. C. (2004). Spinal adenosine receptor activation reduces hypersensitivity after surgery by a different mechanism than after nerve injury. Anesthesiology 100, 1258–1262. doi:10.1097/00000542-200405000-00030
Pan, H. L., Xu, Z., Leung, E., and Eisenach, J. C. (2001). Allosteric adenosine modulation to reduce allodynia. Anesthesiology 95, 416–420. doi:10.1097/00000542-200108000-00025
Park, S. W., Kim, J. Y., Ham, A., Brown, K. M., Kim, M., D’Agati, V. D., et al. (2012). A1 adenosine receptor allosteric enhancer PD-81723 protects against renal ischemia-reperfusion injury. Am. J. Physiol. Ren. Physiol. 303, F721–F732. doi:10.1152/ajprenal.00157.2012
Pasquini, S., Contri, C., Borea, P. A., Vincenzi, F., and Varani, K. (2021). Adenosine and inflammation: Here, there and everywhere. Int. J. Mol. Sci. 22, 7685. doi:10.3390/ijms22147685
Pasquini, S., Contri, C., Merighi, S., Gessi, S., Borea, P. A., Varani, K., et al. (2022). Adenosine receptors in neuropsychiatric disorders: Fine regulators of neurotransmission and potential therapeutic targets. Int. J. Mol. Sci. 23, 1219. doi:10.3390/ijms23031219
Peleli, M., Fredholm, B. B., Sobrevia, L., and Carlström, M. (2017). Pharmacological targeting of adenosine receptor signaling. Mol. Asp. Med. 55, 4–8. doi:10.1016/j.mam.2016.12.002
Reiss, A. B., Grossfeld, D., Kasselman, L. J., Renna, H. A., Vernice, N. A., Drewes, W., et al. (2019). Adenosine and the cardiovascular system. Am. J. Cardiovasc. Drugs 19, 449–464. doi:10.1007/s40256-019-00345-5
Romagnoli, R., Baraldi, P. G., Carrion, M. D., Cara, C. L., Cruz-Lopez, O., Iaconinoto, M. A., et al. (2008). Synthesis and biological evaluation of 2-amino-3-(4-chlorobenzoyl)-4-[N-(Substituted) piperazin-1-yl]Thiophenes as potent allosteric enhancers of the A1 adenosine receptor. J. Med. Chem. 51, 5875–5879. doi:10.1021/jm800586p
Romagnoli, R., Baraldi, P. G., Carrion, M. D., Cara, C. L., Cruz-Lopez, O., Salvador, M. K., et al. (2012). Synthesis and biological evaluation of 2-amino-3-(4-chlorobenzoyl)-4-[(4-arylpiperazin-1-yl)methyl]-5-substituted-thiophenes. effect of the 5-modification on allosteric enhancer activity at the A1 adenosine receptor. J. Med. Chem. 55, 7719–7735. doi:10.1021/jm3007504
Romagnoli, R., Baraldi, P. G., Carrion, M. D., Lopez Cara, C., Kimatrai Salvador, M., Preti, D., et al. (2013). Synthesis and biological effects of novel 2-amino-3-(4-chlorobenzoyl)-4-substituted thiophenes as allosteric enhancers of the A1 adenosine receptor. Eur. J. Med. Chem. 67, 409–427. doi:10.1016/j.ejmech.2013.07.002
Romagnoli, R., Baraldi, P. G., Ijzerman, A. P., Massink, A., Cruz-Lopez, O., Lopez-Cara, L. C., et al. (2014). Synthesis and biological evaluation of novel allosteric enhancers of the A1 adenosine receptor based on 2-amino-3-(4′-chlorobenzoyl)-4-substituted-5-arylethynyl thiophene. J. Med. Chem. 57, 7673–7686. doi:10.1021/jm5008853
Romagnoli, R., Baraldi, P. G., Lopez-Cara, C., Cruz-Lopez, O., Moorman, A. R., Massink, A., et al. (2015a). Synthesis and biological evaluation of a new series of 2-amino-3-aroyl thiophene derivatives as agonist allosteric modulators of the A1 adenosine receptor. A position-dependent effect study. Eur. J. Med. Chem. 101, 185–204. doi:10.1016/j.ejmech.2015.06.041
Romagnoli, R., Baraldi, P. G., Moorman, A. R., Borea, P. A., and Varani, K. (2015b). Current status of A1 adenosine receptor allosteric enhancers. Future Med. Chem. 7, 1247–1259. doi:10.4155/fmc.15.65
Sanni, O., and Terre’Blanche, G. (2021). Therapeutic potentials of agonist and antagonist of adenosine receptors in type 2 diabetes. Rev. Endocr. Metab. Disord. 22, 1073–1090. doi:10.1007/s11154-021-09668-8
Sawynok, J. (2016). Adenosine receptor targets for pain. Neuroscience 338, 1–18. doi:10.1016/j.neuroscience.2015.10.031
Sebastião, A. M., Rei, N., and Ribeiro, J. A. (2018). Amyotrophic lateral sclerosis (ALS) and adenosine receptors. Front. Pharmacol. 9, 267. doi:10.3389/fphar.2018.00267
Slosky, L. M., Caron, M. G., and Barak, L. S. (2021). Biased allosteric modulators: New Frontiers in GPCR drug discovery. Trends Pharmacol. Sci. 42, 283–299. doi:10.1016/j.tips.2020.12.005
Spinozzi, E., Baldassarri, C., Acquaticci, L., Del Bello, F., Grifantini, M., Cappellacci, L., et al. (2021). Adenosine receptors as promising targets for the management of ocular diseases. Med. Chem. Res. 30, 353–370. doi:10.1007/s00044-021-02704-x
Sun, C., Wang, B., and Hao, S. (2022). Adenosine-A2A receptor pathway in cancer immunotherapy. Front. Immunol. 13. Available at: https://www.frontiersin.org/articles/10.3389/fimmu.2022.837230 (Accessed August 11, 2022).
Suresh, R. R., Jain, S., Chen, Z., Tosh, D. K., Ma, Y., Podszun, M. C., et al. (2020). Design and in vivo activity of A3 adenosine receptor agonist prodrugs. Purinergic Signal. 16, 367–377. doi:10.1007/s11302-020-09715-0
Taliani, S., Trincavelli, M. L., Cosimelli, B., Laneri, S., Severi, E., Barresi, E., et al. (2013). Modulation of A2B adenosine receptor by 1-Benzyl-3-ketoindole derivatives. Eur. J. Med. Chem. 69, 331–337. doi:10.1016/j.ejmech.2013.09.001
Trincavelli, M. L., Daniele, S., Giacomelli, C., Taliani, S., Da Settimo, F., Cosimelli, B., et al. (2014a). Osteoblast differentiation and survival: A role for A2B adenosine receptor allosteric modulators. Biochim. Biophys. Acta 1843, 2957–2966. doi:10.1016/j.bbamcr.2014.09.013
Trincavelli, M. L., Giacomelli, C., Daniele, S., Taliani, S., Cosimelli, B., Laneri, S., et al. (2014b). Allosteric modulators of human A2B adenosine receptor. Biochim. Biophys. Acta 1840, 1194–1203. doi:10.1016/j.bbagen.2013.12.021
Varani, K., Vincenzi, F., Merighi, S., Gessi, S., and Borea, P. A. (2017). Biochemical and pharmacological role of A1 adenosine receptors and their modulation as novel therapeutic strategy. Adv. Exp. Med. Biol. 1051, 193–232. doi:10.1007/5584_2017_61
Vijayan, D., Young, A., Teng, M. W. L., and Smyth, M. J. (2017). Targeting immunosuppressive adenosine in cancer. Nat. Rev. Cancer 17, 709–724. doi:10.1038/nrc.2017.86
Vincenzi, F., Padovan, M., Targa, M., Corciulo, C., Giacuzzo, S., Merighi, S., et al. (2013). A(2A) adenosine receptors are differentially modulated by pharmacological treatments in rheumatoid arthritis patients and their stimulation ameliorates adjuvant-induced arthritis in rats. PLoS One 8, e54195. doi:10.1371/journal.pone.0054195
Vincenzi, F., Pasquini, S., Borea, P. A., and Varani, K. (2020a). Targeting adenosine receptors: A potential pharmacological avenue for acute and chronic pain. Int. J. Mol. Sci. 21, E8710. doi:10.3390/ijms21228710
Vincenzi, F., Pasquini, S., Gessi, S., Merighi, S., Romagnoli, R., Borea, P. A., et al. (2020b). The detrimental action of adenosine on glutamate-induced cytotoxicity in PC12 cells can Be shifted towards a neuroprotective role through A1AR positive allosteric modulation. Cells 9. doi:10.3390/cells9051242
Vincenzi, F., Ravani, A., Pasquini, S., Merighi, S., Gessi, S., Romagnoli, R., et al. (2016). Positive allosteric modulation of A1 adenosine receptors as a novel and promising therapeutic strategy for anxiety. Neuropharmacology 111, 283–292. doi:10.1016/j.neuropharm.2016.09.015
Vincenzi, F., Targa, M., Romagnoli, R., Merighi, S., Gessi, S., Baraldi, P. G., et al. (2014). TRR469, a potent A1 adenosine receptor allosteric modulator, exhibits anti-nociceptive properties in acute and neuropathic pain models in mice. Neuropharmacology 81, 6–14. doi:10.1016/j.neuropharm.2014.01.028
Voors, A. A., Bax, J. J., Hernandez, A. F., Wirtz, A. B., Pap, A. F., Ferreira, A. C., et al. (2019). Safety and efficacy of the partial adenosine A1 receptor agonist neladenoson bialanate in patients with chronic heart failure with reduced ejection fraction: A phase IIb, randomized, double-blind, placebo-controlled trial. Eur. J. Heart Fail. 21, 1426–1433. doi:10.1002/ejhf.1591
Wang, L., Londono, L. M., Cowell, J., Saatci, O., Aras, M., Ersan, P. G., et al. (2021). Targeting adenosine with adenosine deaminase 2 to inhibit growth of solid tumors. Cancer Res. 81, 3319–3332. doi:10.1158/0008-5472.CAN-21-0340
Wang, M., Guo, X., Zhao, H., Lv, J., Wang, H., and An, Y. (2020). Adenosine A2B receptor activation stimulates alveolar fluid clearance through alveolar epithelial sodium channel via cAMP pathway in endotoxin-induced lung injury. Am. J. Physiol. Lung Cell. Mol. Physiol. 318, L787–L800. doi:10.1152/ajplung.00195.2019
Welihinda, A. A., and Amento, E. P. (2014). Positive allosteric modulation of the adenosine A2a receptor attenuates inflammation. J. Inflamm. 11, 37. doi:10.1186/s12950-014-0037-0
Welihinda, A. A., Kaur, M., Raveendran, K. S., and Amento, E. P. (2018). Enhancement of inosine-mediated A2AR signaling through positive allosteric modulation. Cell. Signal. 42, 227–235. doi:10.1016/j.cellsig.2017.11.002
Welihinda, A., Ravikumar, P., Kaur, M., Mechanic, J., Yadav, S., Kang, G. J., et al. (2022). Positive allosteric modulation of A2AR alters immune cell responses and ameliorates psoriasis-like dermatitis in mice. J. Invest. Dermatol. 142, 624–632. e6. doi:10.1016/j.jid.2021.07.174
Wild, C., Cunningham, K. A., and Zhou, J. (2014). Allosteric modulation of G protein-coupled receptors: An emerging approach of drug discovery. Austin J. Pharmacol. Ther. 2, 1101.
Wold, E. A., and Zhou, J. (2018). GPCR allosteric modulators: Mechanistic advantages and therapeutic applications. Curr. Top. Med. Chem. 18, 2002–2006. doi:10.2174/1568026619999190101151837
Keywords: adenosine, allosteric modulators, GPCRs, adenosine receptors, drug development
Citation: Pasquini S, Contri C, Cappello M, Borea PA, Varani K and Vincenzi F (2022) Update on the recent development of allosteric modulators for adenosine receptors and their therapeutic applications. Front. Pharmacol. 13:1030895. doi: 10.3389/fphar.2022.1030895
Received: 29 August 2022; Accepted: 20 September 2022;
Published: 05 October 2022.
Edited by:
Kenneth A. Jacobson, National Institutes of Health (NIH), United StatesReviewed by:
Celine Valant, Monash University, AustraliaCopyright © 2022 Pasquini, Contri, Cappello, Borea, Varani and Vincenzi. This is an open-access article distributed under the terms of the Creative Commons Attribution License (CC BY). The use, distribution or reproduction in other forums is permitted, provided the original author(s) and the copyright owner(s) are credited and that the original publication in this journal is cited, in accordance with accepted academic practice. No use, distribution or reproduction is permitted which does not comply with these terms.
*Correspondence: Katia Varani, dnJrQHVuaWZlLml0
Disclaimer: All claims expressed in this article are solely those of the authors and do not necessarily represent those of their affiliated organizations, or those of the publisher, the editors and the reviewers. Any product that may be evaluated in this article or claim that may be made by its manufacturer is not guaranteed or endorsed by the publisher.
Research integrity at Frontiers
Learn more about the work of our research integrity team to safeguard the quality of each article we publish.