- 1School of Pharmacy, Shahid Beheshti University of Medical Sciences, Tehran, Iran
- 2Student Research Committee, School of Medicine, Mazandaran University of Medical Sciences, Sari, Iran
- 3School of Medicine, Tehran University of Medical Sciences, Tehran, Iran
- 4School of Medicine, Zanjan University of Medical Sciences, Zanjan, Iran
- 5Student Research Committee, School of Medicine, Shahid Beheshti University of Medical Sciences, Tehran, Iran
- 6School of Medicine, Shahid Beheshti University of Medical Sciences, Tehran, Iran
- 7School of Pharmacy, Zanjan University of Medical Sciences, Zanjan, Iran
- 8Drug and Food Control Department, Faculty of Pharmacy, Tehran University of Medical Sciences, Tehran, Iran
- 9Amol Faculty of Paramedical Sciences, Mazandaran University of Medical Sciences, Sari, Iran
- 10Student Research Committee, Faculty of Medicine, Guilan University of Medical Sciences, Rasht, Iran
- 11Faculty of Pharmacy, Cyprus International University, Nicosia, Cyprus
- 12Student Research Committee, Faculty of Pharmacy, Mazandaran University of Medical Sciences, Sari, Iran
- 13Research Center for Prevention of Cardiovascular Disease, Institute of Endocrinology and Metabolism, Iran University of Medical Science, Tehran, Iran
- 14Student Research Committee, School of Medicine, Tehran Medical Sciences, Islamic Azad University, Tehran, Iran
- 15Universal Scientific Education and Research Network (USERN), Tehran, Iran
- 16Department of Cardiovascular Disease, Cardiovascular Research Center, Mazandaran University of Medical Sciences, Sari, Iran
Flavonoids are found in natural health products and plant-based foods. The flavonoid molecules contain a 15-carbon skeleton with the particular structural construction of subclasses. The most flavonoid’s critical subclasses with improved health properties are the catechins or flavonols (e.g., epigallocatechin 3-gallate from green tea), the flavones (e.g., apigenin from celery), the flavanones (e.g., naringenin from citrus), the flavanols (e.g., quercetin glycosides from berries, onion, and apples), the isoflavones (e.g., genistein from soya beans) and the anthocyanins (e.g., cyanidin-3-O-glucoside from berries). Scientific data conclusively demonstrates that frequent intake of efficient amounts of dietary flavonoids decreases chronic inflammation and the chance of oxidative stress expressing the pathogenesis of human diseases like cardiovascular diseases (CVDs). The endoplasmic reticulum (ER) is a critical organelle that plays a role in protein folding, post-transcriptional conversion, and transportation, which plays a critical part in maintaining cell homeostasis. Various stimuli can lead to the creation of unfolded or misfolded proteins in the endoplasmic reticulum and then arise in endoplasmic reticulum stress. Constant endoplasmic reticulum stress triggers unfolded protein response (UPR), which ultimately causes apoptosis. Research has shown that endoplasmic reticulum stress plays a critical part in the pathogenesis of several cardiovascular diseases, including diabetic cardiomyopathy, ischemic heart disease, heart failure, aortic aneurysm, and hypertension. Endoplasmic reticulum stress could be one of the crucial points in treating multiple cardiovascular diseases. In this review, we summarized findings on flavonoids’ effects on the endoplasmic reticulum and their role in the prevention and treatment of cardiovascular diseases.
1 Introduction
Flavonoids are present in all photosynthesizing cells, thus occurring commonly in the plant and they describe as the largest class of polyphenols. They have been discovered in flowers, stems, nuts, vegetables, seeds, and fruit in addition to honey, tea, propolis, and wine, representing an ordinary human food component (Cushnie and Lamb, 2005). These combinations are comprised of 15 carbon atoms in their chemical structure. The main structural part of flavonoid combinations is the flavone nucleus consisting of two benzene rings conjoined via a heterocyclic pyrene ring (Serafini et al., 2010). Flavonoids are divided into flavans, anthocyanidins, flavonols, flavanones, isoflavones, and flavones (Ponte et al., 2021). Flavonoids are being used as epigenetic modifiers and anti-cancer agents in breast cancer (Mahmoud et al., 2019). Moreover, they are common products of plant derivatives that are reportedly documented to be therapeutically effective phytochemicals against considerable illnesses including oxidative stress, inflammatory disorders (cardiovascular diseases, neurodegenerative disorder), and malignancies. One of the diseases that has been proven to affect flavonoids is cardiovascular disease. For instance, cardiomyopathy, vasoconstriction, hypertension, heart failure, arrhythmia, thrombosis, inflammation, and Atherosclerosis are cardiovascular diseases affecting flavonoids (Mahmoud et al., 2019). The endoplasmic reticulum (ER) plays an important role in the metabolism of lipids, transmembrane proteins, maturation, folding, and synthesis of transmembrane and secretory proteins in most eukaryotic cells (Giordano et al., 2014). ER disorders, particularly ER stress, is a pathophysiological reaction concerned with lipid metabolism and cardiovascular injury. Thus, suppression of ER stress may refine lipid metabolic disease and decrease cardiovascular risk (Li et al., 2018a). Human and animal samples of atherosclerosis lesions have provided specific proof that ER stress exists in atherosclerotic plaques, especially at the advanced level (Liu et al., 2016). This narrative review may open avenues for investigation of the flavonoids’ properties in the decrease of ER stress in treating cardiovascular diseases.
2 Methods
In this study, we searched various online databases including Google Scholar, PubMed, Web of Science, and EMBASE to find all articles related to ER as a target in cardiovascular diseases and flavonoids’ role in ER stress, published from January 2003 until March 2020. No limitation has been applied to the language of the articles. The search strategies used for each database were independently designed and MeSH terms were also included. The keywords were:
#1 Flavonols #2 Quercetin #3 Kaempferol #4 Fisetin #5 Flavanones #6 Naringenin #7 Isoflavones #8 Genistein #9 Flavones #10 Apigenin #11 Baicalein #12 Luteolin #13 Wogonin #14 Nobiletin #15 Total flavonoids #16 Thrombosis #17 Myocardial infarction #18 Myocardial ischemia/reperfusion #19 Autoimmune myocarditis #20 Cardiomyocyte apoptosis #21 Cardiac inflammation #22 Arrhythmia #23 Diabetic cardiomyopathy #24 Hypertrophic cardiomyopathy #25 Atherosclerosis #26 Hypertensive heart disease #27 Cardioprotective #28 Endoplasmic reticulum stress.
We selected relevant studies by screening the title and abstracts of the articles. Afterward, the reference lists of included articles were also surveyed to identify any further studies.
3 Role of ER in cardiovascular diseases
Increasing physiological and pathological damage, including changes in intracellular calcium, genetic or environmental damage, oxidative stress, and glycosylation, could disturb ER’s steady state leading to the ER lumen not folding and misfolded proteins. This condition is known as ER stress (Paschen and Frandsen, 2001; Schröder and Kaufman, 2005; Zhang et al., 2015). ER stress is associated with autophagy and the immune response to pathogens. Targeting ER stress and the unfolded protein response (UPR) with small molecules is considered a promising therapy for treating various diseases such as neurodegeneration, cancer, metabolic diseases, stroke, and heart diseases (Valenzuela et al., 2018). Interestingly, in some diseases (e.g., pancreatic β cells in diabetes mellitus), it may be beneficial to suppress ER stress and/or block UPR, whereas in other diseases (e.g., viral infections), inducing ER stress and/or increase the UPR may be necessary for a therapeutic effect (Lin et al., 2008).
Cardiomyocyte hypoxia in addition to chronic and acute inflammatory reactions, which are present in heart failure, might cause ER stress (Dickhout et al., 2011). ER stress and the UPR will result in cardiac hypertrophy and heart failure by increasing PERK and EIF2A–ATF4–CCAAT–CHOP. Cardiovascular stress prompts ER stress and activates the UPR response to restore ER homeostasis. As an adjustable mechanism, the PERK–PI3K–AKT cascade inhibits apoptosis. The PERK activates autophagy and interrupts the generation of reactive oxygen species (ROS) which can prevent ROS-mediated cardiomyocyte injury; it also induces autophagy by activating eukaryotic initiation factor 2A (EIF2A) and prompts the activation of activating transcription factor 4 (ATF4), which leads to initiation of various types of autophagy (Ren et al., 2021). ATF4 triggers C/EBP homologous protein (CHOP) to induce various biological responses such as apoptosis and inflammation. Inositol-requiring protein 1α (IRE1α) also activates several cell signaling systems which lead to the inhibition of apoptosis and reduction of ROS levels. If the adaptive UPR fails to stabilize ER homeostasis, it results in cardiomyocyte apoptosis (Ren et al., 2021). The ER is an organelle providing multiple functions, including lipid biosynthesis, calcium storage, and protein folding and processing. Due to its various functions, ER stress has been highlighted as an effective regulator of cardiovascular diseases (Hong et al., 2017). Herein, we review ER stress in known cardiovascular disease.
3.1 ER stress in ischemic heart diseases
Zhang et al. (2017a) demonstrated that the key ERS molecules (ATF4 and CHOP) are stimulated in ischemic heart disease, and CHOP can induce the expression of proapoptotic proteins during ERS, accelerating the process of disease. A study by Xin et al. (2011a) showed that the PERK pathway was also activated due to the expression of phosphorylated eIF2α, and also ATF4 proteins were increased. Additionally, GRP78, the central regulator of ER function and UPR network modulator was also induced in the ischemic myocardium. Another study by Belmont et al. (2010) showed that Derl3 which encodes a protein involved in ERAD (Endoplasmic-reticulum-associated protein degradation), was induced by ATF6. Derl3 was also induced in the infarct border zone in a mouse model of myocardial infarction, and by simulated ischemia in cultured cardiac myocytes. It was found that overexpressing Derl3 reduces long-term ER stress response signaling and cell death.
3.2 ER stress in heart failure
Heart failure is a progressive disorder of the myocardial remodeling (Chin et al., 2011). Sigma-1 receptor (Sig-1R) is a chaperone receptor located at ER that acts as a dynamic pluripotent modulator regulating cellular pathophysiological processes. Sig-1R inhibition promotes autophagy in cardiomyocytes under oxidative stress conditions causing heart failure (Ortíz-Rentería et al., 2018). GRP78 protein and ER stress-related apoptotic pathways, CHOP, caspase-12, and JNK1 significantly increased in animal models of heart failure (Xin et al., 2011b). It has been suggested that TRB3 acts as an important role in cell death in relation to CHOP where CHOP is also induced by other stress signals; Under prolonged ER stress, induced TRB could lead to cell death and heart failure (Ohoka et al., 2005). SERCA3f is also upregulated in heart failure, and overexpression of the Ca2+ pump, increases the XBP1 splicing and Grp78 expression; This demonstrated that SERCA3f is probably a UPR effector and participates in the ER stress in human heart failure (Safiedeen et al., 2017).
3.3 ER stress in myocardial hypertrophy
Nogo-B is a conserved ER protein that is a player in maintaining the ER structure (Park et al., 2017; Weng et al., 2018). By activating the PERK/ATF4 signaling pathway, Nogo-β is prevented, resulting in myocardial hypertrophy (Li et al., 2018b). Evidence reveals that the expression of Nogo-β and ERS markers (such as p-PERK, ATF4, p-IRE1, and XBP1s) are significantly upregulated in transverse aortic constriction (TAC)-induced hypertrophic myocardium. In addition, MFN2 is known for its role in mitochondrial fusion. It is localized at ER and mitochondrial membranes and forms a hetero- or homo-dimer with mitofusin-1 (MFN1) or another MFN2 in the outer mitochondrial membrane (Choi et al., 2006; Eura et al., 2006). Downregulation of MFN2 has been found in rat models of cardiac hypertrophy (Sun et al., 2019).
3.4 ER stress in arrhythmias
Under prolonged ER stress, the ER could increase Ca2+ release, which activates cell apoptosis and also induce triggered activity that can cause cardiac arrhythmias (Safiedeen et al., 2017). A study by Liu et al. (2014) showed that ER stress is involved in the occurrence of ventricular arrhythmias in primary cardiomyocytes. ER stress markers including GRP78 and p-PERK were significantly elevated in the diabetic cardiomyopathy. Moreover, GRP78 expression and PERK phosphorylation elevations were found in diabetic myocardial infarcted hearts, indicating that the hyper-activation of ER stress-mediated PERK signaling was along with post-myocardial infarction ventricular arrhythmias in diabetes ones. It was reported that ER stress was hyperactivated through the RAGE (receptor for advanced glycation end products)/ROS pathway in myocardial infarcted hearts exposed to advanced glycation end products (Liu et al., 2021).
3.5 ER stress in atherosclerosis
Atherosclerosis is a chronic inflammatory disease in which inflammatory signaling pathways are involved (Ross, 1999; Libby et al., 2002; Tuttolomondo et al., 2012). Three ER stress sensors, PERK, IRE1, and ATF6, could all induce specific inflammatory responses through the UPR, especially in macrophages and ECs (Yang et al., 2020). PERK pathway results in phosphorylation of the inhibitor of nuclear factor-κB (NF-κB), IκB, in which IκB kinase is involved. This leads to the activation of genes involved in downstream pathways of inflammation, such as those that encode the cytokines TNF-α and interleukin (IL-1). The ATF6 pathway also triggers the NF-κB pathway (Deng et al., 2004; Yamazaki et al., 2009; Hotamisligil, 2010). In addition, the IRE1 pathway induces the elevation of thioredoxin-interacting protein (TXNIP), which activates the NLRP3 inflammasome (Lerner et al., 2012). The activated form of NLRP3 promotes the secretion of IL-1β and IL-18 and leads to an inflammatory response (Martinon et al., 2002; Martinon and Tschopp, 2007; Takahashi, 2014). CHOP is also upregulated by the UPR in the ER, along with the progression of atherosclerosis in the aorta (Joo et al., 2015). Moreover, ER stress significantly stimulates macrophage lipid accumulation (Huang et al., 2018). The PERK signal activates GSK-3α expression. A study by Martin et al. (2005) showed that inhibition of GSK-3α remarkably reduces ER stress-induced free cholesterol levels and inhibits the expression of genes that regulate lipid and cholesterol metabolism, including fatty acid synthase, sterol element-binding protein-1 C (SREBP-1C), etc. Therefore, ER stress and PERK signal could trigger lipid metabolism which leads to atherosclerosis.
4 Current drug targets of ER for prevention or amelioration of cardiovascular diseases
ER stress significantly facilitates the development and occurrence of cardiovascular diseases. Various medications, including antihypertensive drugs, anti-glycemic drugs, lipid-lowering drugs, hormones, chemicals, herbal medicines, and other drugs, are used to target ER stress, which leads to preventing or alleviating cardiovascular diseases. Previous research has shown that Angiotensin II can cause ER stress (Wang et al., 2015). When ER stress arises, the UPR, a complex cellular signaling network, may be triggered, activating reparative signaling that includes PERK, eIF2, ATF3, and ATF6. For instance, Enalapril controls hypertension by blocking the PERK/eIF2α/ATF3/ATF6 pathway (Zhou et al., 2015a).
It is known that ER stress contributes to cardiotoxicity and heart conditions that could eventually lead to heart failure. Several molecular markers of ER stress, including GRP78, PERK, eIF2a, and CHOP, have been identified in the research to be expressed. Comparing the phosphorylated levels of eIF2a and PERK in patients with heart failure to controls, a considerable activation of the PERK to the eIF2a arm of the stress response was discovered. Metoprolol and propranolol as b-AR blockers suppress ER stress in cardiac hypertrophy and heart failure by blocking the GRP78/CHOP/XBP-1 pathway (George et al., 2011; Ni et al., 2011).
ER stress has a significant role in the initiation and progression of atherosclerosis (Tabas, 2010). ER stress indicators were found in atherosclerosis-prone portions of arterial regions susceptible or resistant to atherosclerosis separated from non-atherosclerotic pigs (Civelek et al., 2011). Atorvastatin affects atherosclerosis by inhibiting BIP/PERK/eIF2α/CHOP pathway (Song et al., 2011; Jia et al., 2012; Ivanova and Orekhov, 2016). Additionally, studies suggest that by inhibiting the PERK/eIF2/caspase-3 pathway, Atorvastatin may prevent apoptosis and, reduce cell damage (Yang and Hu, 2015).
Reports indicate that excessive glucose, improper fat acid metabolism, lipid buildup, and ROS increase cause diabetic cardiomyopathy by ER stress (Frustaci et al., 2016). Adding Metformin can prevent systolic and diastolic blood pressure by inhibiting ER stress markers including p-eIF2, GRP78, and XBP1s. Through the upregulation of p-eIF2, protein disulfide isomerase, and XBP1 mRNA expression, AMPK2 deletion causes ER stress progression. However, metformin lowers blood pressure via activating AMPK and inhibiting angiotensin-induced ER stress. Therefore, by boosting the phosphorylation of phosphoprotein, which was thought to be a key mechanism in the treatment of hypertension, metformin-activated AMPK2 may reduce ER stress (Hundal et al., 2000; Duan et al., 2017). Another anti-glycemic medication with cardioprotective effects is Empagliflozin. The Effects of Empagliflozin on diabetes and cardiomyopathy are via preventing the GRP78/CHOP/ATF4/caspase-12 signaling pathway (Zhou and Wu, 2017). Studies have demonstrated that empagliflozin enhanced cardiac functioning, decreased cardiomyocyte apoptosis, and decreased the expression of ER stress indicators such as Grp78, CHOP, and cleaved caspase-12 in proteins from the cardiomyocyte (Campeau and Leask, 2022).
One of the anti-anginal medications that are used commonly, is nicorandil. In addition to its vasodilator activity, it has been shown that it boosts fibrinolytic ability, protects the myocardium against thrombus formation, and promotes angiogenesis. Recent research has linked the development of atherosclerosis to ER stress (Thorp et al., 2009). A long-term nicorandil treatment significantly reduced the increased expression of the ER stress markers in atherosclerotic lesions. These findings imply that nicorandil’s beneficial effects are mediated by decreased ER stress. Following nicorandil treatment, the extent of the atherosclerotic lesion and plaque necrosis were significantly reduced. In atherosclerotic lesions, nicorandil significantly decreased the expression of the endoplasmic reticulum stress markers CHOP and GRP78. In cultured THP-1 macrophages, nicorandil significantly reduced tunicamycin-induced CHOP upregulation (Izumiya et al., 2011).
The vitamin D receptor (VDR), primarily expressed in the cardiovascular system, can control cardiovascular function. According to studies, VDR activation prevents ER stress-induced apoptosis, which protects against myocardial damage. Additionally, it has been shown that myocardial ischemia/reperfusion injury upregulates the expression of VDR. Curiously, calcitriol and paricalcitol, among other natural and synthetic agonists, activate VDR, significantly reducing infarct size and enhancing cardiac function. By decreasing the expression of CHOP and activating caspase-12, it has been shown that activating VDR limits the expression of ERS-relative protein, thereby alleviating myocardial ischemia/reperfusion injury (Pu et al., 2013; Yao et al., 2015; Haas et al., 2016). Through the inhibition of ER stress, Melatonin inhibits the evolution of cardiomyopathy. The pineal gland is the primary organ that secretes the endogenous chemical melatonin. Inhibiting ER stress-induced apoptosis is how Melatonin protects the myocardium. According to studies, Melatonin can reduce the expression of ER stress indicators in cardiac tissue, including CHOP, GRP78, PERK, and ATF6. It can also lower the expression of apoptosis-related markers like Bcl-2 and reverse the production of caspase-3 and Bcl-2 (Xiong et al., 2018).
Besides the drugs mentioned above, some herbal medicines that target ERS, have cardioprotective effects. Hypertension is linked to hyperhomocysteinemia or high homocysteine levels. Homocysteine induces ER stress in endothelial cells (Sutton-Tyrrell et al., 1997). Thus, ER stress plays a significant role in vascular dysfunction during hypertension. Consumption of Black tea is associated with reduced expressions of ER stress markers such as phosphorylated elF2α at ATF3 and cleaved ATF6, induced by hyperhomocysteinemia (San Cheang et al., 2015). Salidroside is another herbal remedy that protects endothelium cells from homocysteine-induced damage. Salidroside, an active ingredient in Rhodiola Rosea, has been shown to possess antioxidant properties. The underlying mechanisms of inhibition of Hcy-induced endothelial cells regulate the ER-stress pathway by down-regulating phosphorylation of PERK or IRE1, as well as the binding protein (Bip) and CHOP (Zhu et al., 2017a). Paeonia suffruticosa has been widely utilized in traditional oriental medicine for thousands of years and has a number of medicinal uses. Paeonia Suffruticosa’s root bark’s primary component is Paeonol (Zhang et al., 2019a). In a study, the vascular protective effects of chronic treatment with Paeonol have been explored. They found that using Paeonol preserved endothelial function and normalized blood pressure by inhibiting ER stress markers including GRP78, ATF6, and p-eIF2α (Choy et al., 2017; Chen et al., 2018).
Another chemical compounds that can affect ER stress are Flavonoids (Arumugam et al., 2012; Zhang et al., 2013a; Qin et al., 2016; Mahmoud et al., 2019). Flavonoids are used widely for this purpose, and this review aims to summarize the effects of this pharmaceutical category as a therapeutic agent in cardiovascular diseases. Table 1 summarizes the data of the studies on the effect of flavonoids on ERS inhibition in treating cardiovascular diseases.
5 Cardiomyopathy
5.1 Quercetin
In an animal study, Cai et al. (2015) supported the role of quercetin in preserving rat hearts with post-myocarditis dilated cardiomyopathy. They had given the intervention group of rats quercetin at a dose of 10 mg/kg. Interestingly, they found reduced myocardial levels of fibrosis and ER stress in the intervention group compared to the vehicle-treated dilated cardiomyopathy rats (control group). Furthermore, the cardiac function and myocardial size were notably protected in the rats treated with quercetin in contrast to the control group. Surprisingly, the quercetin-treated rates showed considerable suppression of the myocardial endothelin-1 in addition to mitogen-activated protein kinases. In addition, Chang et al. (2021) conducted an in vitro study to evaluate the effects of quercetin on oxidative stress induced by hypoxia in human cardiomyocyte cell cultures. They found that quercetin could inhibit endoplasmic reticulum stress (ERS) by regulating silent information regulator protein 1 (SIRT1), which governs the ERS, apoptosis, oxidative stress, and also through regulating transmembrane BAX Inhibitor Motif-including protein 6 (TMBIM6), which has a protective function against cell death.
5.2 Nobiletin
Zhang et al. (2017b) conducted an in vivo and in vitro study on mice who underwent aortic banding (AB) operation to induce cardiac hypertrophy. The study group was remedied with 50 mg/kg of Nobiletin for 4 weeks. Based on the study results, nobiletin can prevent cardiac hypertrophy by reducing NADPH oxidase (NOX) 2 and NOX4 expression. Moreover, nobiletin could alleviate ER stress by inhibiting NOX2 in neonatal rat cardiomyocytes (NRCMs).
6 Autoimmune myocarditis
6.1 Quercetin
In a study by Arumugam et al. (2012), the effects of quercetin on the advancement of practical autoimmune myocarditis were evaluated. They found suppressed ER stress and oxidative stress through endothelin-1/MAPK signaling. Moreover, they showed that treatment with quercetin can suppress both the mitogen-active protein kinases (MAPK) and the myocardial endothelin-1. Consequently, therapy against the advancement of experimental autoimmune myocarditis (EAM) to dilated cardiomyopathy (DCM) affects the modulation of the MAPK signaling cascade. To investigate this hypothesis (Martinet and Kockx, 2001), an oral regime of quercetin (10 mg/kg/day) in rats with EAM induced by porcine heart myosin was conducted. It was found that the post-myocarditis rats travailed from increased ER stress and myocardial fibrosis. Quercetin showed considerable suppression of myocardial expressions of growth arrest, glucose-regulated protein 78 (GRP78) levels, and DNA damage-inducible factor 153 (GADD153). In addition, quercetin reduced the cytosolic level of cytochrome C which elevates apoptosis and therefore plays its protective role against adverse cardiac remodeling due to prolonged ER stress during DCM. Furthermore, quercetin significantly suppressed myocardial endothelin-1 (ET-1) and MAPK which causes EAM progression. All in all, quercetin plays its cardioprotective role against EMA through the modulating MAPK signaling cascade (Arumugam et al., 2012).
7 Thrombosis
7.1 Quercetin
Quercetin-3-O-rutinoside, abundant in common foods, inhibits protein disulfide isomerase (PDI) and prevents thrombus formation in vivo and in vitro. Quercetin-3-O-rutinoside inhibits platelet aggregation in vitro and prevents injury-caused fibrin construction on endothelial cell monolayers (Figure 1) (Jasuja et al., 2012). In a laser-caused mouse model of thrombus formation, quercetin-3-O-rutinoside prevented fibrin formation and platelet accumulation at concentrations of .5 mg/kg (Jasuja et al., 2012). Quercetin-3-O-rutinoside inhibited thrombus formation in a ferric chloride-caused thrombus formation model. Intravenously quercetin-3-O-rutinoside and also oral compounds were antithrombotic in vivo. Notably, these combinations were antithrombotic at concentrations less than those used by individuals receiving over-the-counter formulations of quercetin-3-O-rutinoside. Quercetin-3-O-rutinoside inhibited purified PDI with an IC50 of ∼6 μM and bound PDI underflow with a Kd of ∼3 μM (Jasuja et al., 2012). They showed that just flavonoids with a 3-O-glycosidic link in the 3 positions of the C ring blocked PDI action. The fact that just glycosylated quercetins are active against PDI is critical when evaluating the possible toxicity of these combinations. This glycoside decreases the cell permeability of quercetin. Therefore, the exact chemical moiety that causes a quercetin analog active against PDI, inhibits its entrance into cells, which may be the cause of the low toxicity (Flaumenhaft, 2013; Chtourou et al., 2015; Bekendam and Flaumenhaft, 2016).
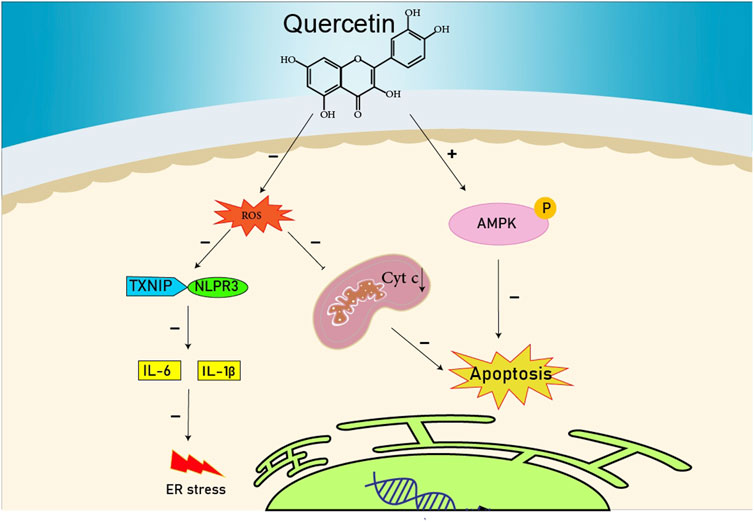
FIGURE 1. The reduction of ROS production by quercetin decreases TXNIP and NLPR3, which in turn results in a reduction of endoplasmic reticulum stress by reducing IL1B and IL6. On the other hand, the reduction of ROS reduces the production of Cyt c in the mitochondria, which ultimately reduces apoptosis. The compound also increases AMPK phosphorylation in the endothelial cells, causing an increase in NO production and eventually a decrease in apoptosis.
8 Myocardial cell death
8.1 Naringenin
Tang et al. (2017) showed that naringenin reduces H9c2 myocardial cell death due to ER apoptosis in an in vitro study. In this study, they found that naringenin reduces ER-caused apoptosis in H9c2 myocardial cells in several ways. Naringenin enhanced the expression of an anti-apoptotic protein called Bcl-2 and reduced the caspase-3 activity, Cytochrome c, and Bax expression as a pro-apoptotic protein (Kiraz et al., 2016; Qin et al., 2016). In addition, naringenin reduced the expression of ER stress-related proteins containing cleaved caspase-12, glucose-regulated protein 78, and C/EBP homologous protein (Miyazaki et al., 2011; Tao et al., 2011; Zhang et al., 2013a). Finally, activating transcription phospho-extracellular regulated protein kinases, inositol-requiring enzyme-1a, and factor 6, which are the three signaling pathways related to ER stress, were inhibited by naringenin (Ron and Walter, 2007; Wu et al., 2015; Yu et al., 2016). All these results showed that naringenin improves hypoxia/reoxygenation-induced apoptosis and cytotoxicity by moderating signaling pathways associated with ER stress.
8.2 Icariin
Qian et al. (2021) assessed the protection activity of Icariin against cardiomyocyte apoptosis due to hypertension-induced damage in an in vivo study. In this study, spontaneously hypertensive rats were selected and orally administered Icariin at the concentration of 10, 20, and 40 mg/kg per day in the study group and water in the control group for 2 weeks. Treatments with Icariin showed enhanced left ventricular function and increased stroke output and ejection fraction. The hypertrophy of left ventricular cardiomyocytes declined and the expression of ERS-induced proteins such as ATF-6, ATF-4, GRP78, PERK, CHOP, Caspase 12, DR5, c-JUN, and ASK-1 decreased. Consequently, Icariin prevented ERS-induced apoptosis (Qian et al., 2021). Moreover, Zhang et al. assessed in vitro effects of Icariin on the apoptosis induced by ERS. In this study, H9c2 rat cardiomyoblast cells were exposed to tunicamycin (ER stressor) and they were pretreated with Icariin (2.5, 5, 10, and 20 μm) for 30 min in advance. They reported that Icariin reduced the formation of reactive oxygen species (ROS) and caspase-3 activity. Moreover, ER markers’ levels and mitochondrial membrane potential decreased. In conclusion, this study showed the protective efficacy of Icariin in the apoptosis (Zhang et al., 2013b).
8.3 Baicalin
Shen et al. (2014) determined the effects of Baicalin on cardiomyocyte apoptosis induced by ER stress in an in vitro study. The result of this study indicated that Baicalin possesses anti-oxidant and anti-apoptotic properties and it can reduce ER stress-induced cardiomyocyte apoptosis through the CHOP/Enos/NO pathway, due to decreasing the expression of CHOP, caspase-3, and Enos, JNK.
9 Myocardial ischemia-reperfusion injury
9.1 Naringenin
Yu et al. (2019a) investigated the effects of naringenin on myocardial ischemia-reperfusion damage and cyclic guanosine monophosphate-PKGI alpha. They found that the naringenin-treated group showed antioxidant enzyme expressions and decreased myocardial oxidative stress levels during myocardial ischemia-reperfusion injury. Previous studies showed that naringenin had antioxidative impacts and developed cardiac functional recovery after the injury. These data also demonstrated that naringenin, by its antioxidant impacts, may protect against ischemic heart disease. Previously, it was discovered that cGMP-PKG signaling affected myocardial ER function and decreased ER stress levels in stress situations (Han et al., 2012; Ramprasath et al., 2014; Subburaman et al., 2014; Chtourou et al., 2015; Da Pozzo et al., 2017). In conclusion, both in vivo and in vitro studies showed that naringenin increased myocardial cGMP content and upregulated PKGIα expression.
9.2 Kaempferol
In a study by Kim et al. (2008), in vitro effect of Kaempferol treatment on H9c2 cardiac muscle cells was assessed. The study group was perfused with a 10 µM concentration of Kaempferol during the pre-ischemia period for 20 min followed by the post-ischemia period for 50 min. They found that Kaempferol, as a treatment, could lead to a considerable increase in Bcl-2 (anti-apoptotic protein) expression and a decrease in Bax (apoptotic protein) expression. In addition, the downregulation of proteins involved in ER stress was observed in both in vivo and ex vivo experiments. The comparison between the study and control group (vehicle) in the ex vivo-Langendorff experiment showed that kaempferol could improve the post-ischemic left ventricular end-diastolic pressure and also left ventricular diastolic pressure noticeably, followed by 20, 30, 40, and 50 min of reperfusion (Figure 2).
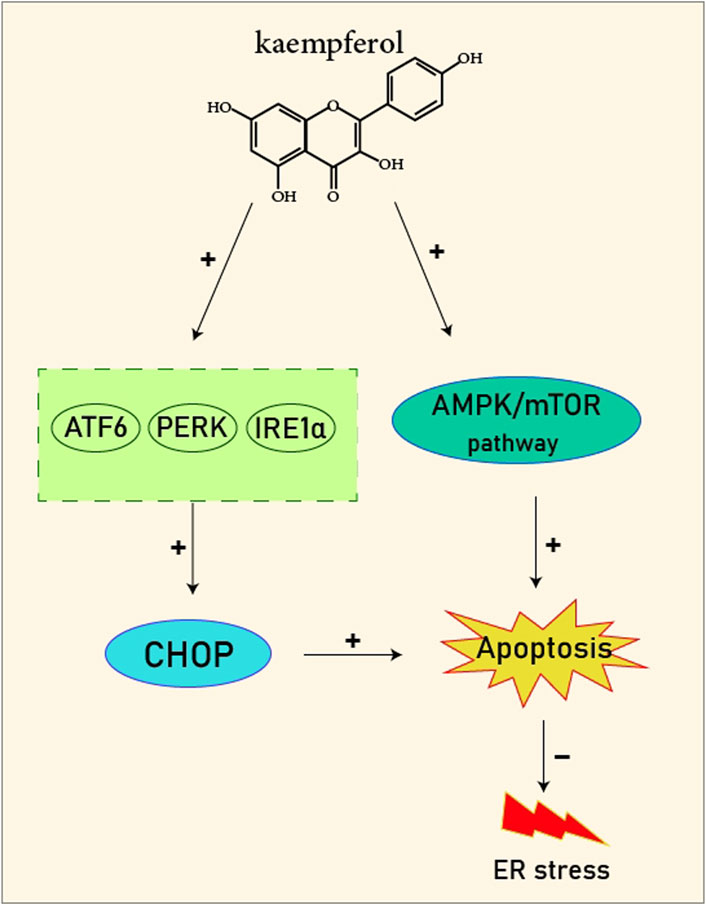
FIGURE 2. Kaempferol induces CHOP through IRE1a, PERK, ATF6 and also induces AMPK/mTOR, which induce apoptosis and as result reduce ER stress.
9.3 Nobiletin
Zhang et al. (2019b) assessed the effects of Nobiletin on reperfusion and myocardial injury. In this study, rats were treated with Nobiletin (15, 30, and 45 mg/kg) followed by the induction of myocardial ischemia and reperfusion injury. The study represented that mRNA and protein levels of ER stress-related signal molecules including GRP78, caspase-12, and CHOP are downregulated by Nobiletin. In contrast, the levels of p-PI3K and p-AKT are increased. Finally, the attenuation of myocardial apoptosis caused by PI3K/AKT-mediated ER stress was observed.
9.4 Apigenin
Feng et al. (2018) studied the Apigenin-7-O-β-D-(-6''-p-coumaroyl)-glucopyranoside (APG) cardioprotective mechanism and the role of ER stress in myocardial ischemia/reperfusion in rat hearts. They concluded that pretreatment with Apigenin enhances cardiac performance and reduces ER stress by stimulating the AMPK signaling pathway. This signaling pathway has several beneficial activities such as reducing cell apoptosis, enhancing cardiac performance, and improving cell viability.
9.5 Dihydroquercetin
Shu et al. (2019) evaluated the impact of Dihydroquercetin (DHQ) on ER stress-induced apoptosis in rat cardiomyocyte cell cultures. Their study showed that DHQ inhibits the apoptotic pathways by decreasing the expression levels of pro-apoptotic proteins such as C/EBP homologous protein (CHOP), Caspase-12, and c-junk-terminal kinase (p-JNK). DHQ can also postpone the onset of ER stress by decreasing the expression levels of Phosphorylated eukaryotic translation initiation factor 2 (P-EIF2), protein kinase-like Endoplasmic reticulum kinase (PERK), and Glucose-regulated protein 78 (GRP78), and stimulating the expression of Heme Oxygenase-1 (HO-1) which is a cytoprotective enzyme, and increasing nuclear factor-erythroid factor 2-related factor 2 (Nrf2) binding to antioxidant response elements which play a main role in the expression of HO-1. In total, it can be concluded that DHQ can significantly decrease ER stress and its consequent apoptosis.
9.6 Flavonoids
Kim et al. (2010) investigated the protective effect of flavonoids on reperfusion/ischemia-cause cardiac impairment in an in vitro study. They found that Flavonoids, as a treatment, notably raised the expression of an anti-apoptotic protein (Bcl-2) but reduced the proapoptotic protein called Bax. The flavonoids decreased the expression levels of ER stress proteins, glucose-regulated protein-78, triggering transcription factor 6α, inositol-needing protein-1, X-box binding protein 1, C/EBP-homologous protein, and phosphor-eukaryotic initiation factor 2α. This study indicated that cardiac reperfusion/ischemia was associated with the ER stress reaction, in which mitochondrial Bcl-2 proteins were affected too.
9.7 Luteolin
Zhu et al. (2017b) used a p38 mitogen-activated protein kinase inhibitor (SB203580) and luteolin to determine whether SERCA2a is involved and impacts the negative cardiac role caused by p38 MAPK during myocardial I/R injury. This study showed that luteolin increases SERCA2a activity to enhance systolic/diastolic role during Ischemia/Reperfusion in rat hearts and cardiomyocytes by decreasing the inhibitive results of the p38 pathway (Zhu et al., 2017b).
10 Atherosclerosis
10.1 Silymarin
A study by Badawy Khair and Mohammed (2021) compared the neuroprotective effects of silymarin and Co Q10 on sixty adult male albino rats suffering from atherosclerosis. They divided rats into four classes: Group one (control group), Group two (atherosclerotic group), Group three (atherosclerotic Co Q10 treated group), and Group four (atherosclerotic Silymarin treated group). Three months later they processed cerebellar cortices that demonstrated widened cisternae of rough ER and disruption and loss of the Purkinje layer with pyknotic nuclei. Thus, Silymarin supplementation had a neurological protecting function in atherosclerosis. Silymarin is perhaps the perfect neuroprotective mechanism and an unexplored method for decreasing many neurological disorders in high-risk people (Badawy Khair and Mohammed, 2021).
11 Cardiomyocyte contraction
11.1 Genistein
Liew et al. (2003) conducted a study on a guinea pig and showed that genistein increases cardiac excitation-contraction coupling. The most important result of this study is the stimulation of myocyte contraction in the guinea pig by genistein while inhibiting the primary stimulus of cell contraction namely the L-type Ca2+ current (Liew et al., 2003). Their examinations with caffeine-induced contractions showed that genistein enhances the sarcoplasmic reticulum (SR) Ca2+ load. The following mechanisms are involved: Genistein causes a transient increase in Ca2+ through Na+/Ca2+ exchanger dysfunction, resulting in increased calcium reabsorption by the SR (Bers and Bridge, 1989; Bers and Berlin, 1995). Furthermore, it is conceivable that genistein influences the phosphorylation of phospholamban, raising the Ca2+ affinity of the SR Ca2+ pump. Moreover, genistein can speed up SR release by affecting the ryanodine receptor. Altogether, their findings support the effect of genistein on the cardiovascular system as a positive cardiac inotrope.
11.2 Catechin
In a study conducted by Feng et al. (2012) nanomolar concentrations of epigallocatechin 3 gallate (EGCG) notably increased the contractility of unchanged murine myocytes by raising SR Ca2+ content, electrically stimulated Ca2+ transients, and ryanodine receptor type 2 channel opening possibility. Voltage clamp experimentations showed that epigallocatechin-3-gallate notably inhibits the Na+/Ca2+ exchanger. However, other Na+ and Ca2+ handling proteins like Ca2+-ATPase, Na+-K+ ATPase, and Na+-H+ exchanger, were not affected by EGCG. Therefore, nanomolar EGCG rises contractility in unchanged myocytes. These findings should be further evaluated and may supply a new therapeutic approach to enhancing the heart’s contractility.
12 Cardiomyocyte relaxation
Hamaguchi et al. (2021) examined the lusitropic effects of quercetin on isolated ventricular myocardia from normal and Streptozotocin-caused diabetic mice. In this study, they showed that quercetin can speed up myocardium relaxation and detected the carrier involved by selective Sarco–endoplasmic reticulum Ca2+ pump (SERCA) and Na+–Ca2+ exchanger (NCX) blockers. The SERCA is the main carrier to absorb Ca2+ from the cytoplasm to the SR (Choi et al., 2002; Belke and Dillmann, 2004), while cyclopiazonic acid (CPA) is a SERCA inhibitor (Takahashi et al., 1995). These results indicated that quercetin has positive lusitropic results on the myocardium. The action was blocked by CPA, which indicates that it is done by activating SERCA. Quercetin decreased the contractile strength and tended to speed up the contraction rate. Quercetin was reported to block the ryanodine receptor (Carrasco-Pozo et al., 2012) and the L-type Ca2+ channel (Castillo et al., 2018; Liang et al., 2020). Its blockage by quercetin may reduce the Ca2+ concentration and the moment to achieve it. Altogether, the quercetin effect on the myocardium is diverse from agonists of β-adrenoceptor, which present both positive lusitropic and inotropic effects (Namekata et al., 2018). This suggests that quercetin is less likely to enhance the myocardial need for O2, which may be a benefit in the cure of failing myocardium. Quercetin caused a little reduction in the contractile strength and the contraction duration and speeded up the relaxation, which depends on concentration. The quercetin-caused speed of relaxation was completely blocked by CPA.
13 Viral myocarditis
13.1 Flavonoids
The study by Zhou et al. (2016) attempted to show the relationship between the pathophysiology of viral myocarditis and total flavonoids of Astragalus and identify the possible cardioprotective effects of total flavonoids of Astragalus (TFA). CoxsackievirusB3 (CVB3) infection caused the cardiac malfunction and histopathological estimation demonstrated myocyte degeneration. Intraperitoneal injections of total flavonoids of Astragalus with CVB3 rescued functional and also morphological parameters. In vitro studies in HL-1 cells showed acute treatment with high doses of TFA develops SERCA2 and calumenin association, the association that was reduced during CVB3 infected cells. The protective effect of TFA was evaluated by treating animals simultaneously with TFA at a dose of 20 mg/kg and CVB3. Medium doses of TFA prevented the loss of calumenin, as mRNA levels were higher in animals that were treated by TFA and CVB3 compared to animals infected with CVB3 alone. This effect was protein specific as neither CVB3 infection nor TFA treatment changed the protein levels of the ER Ca2+-ATPase (SERCA2), showing that the cardio-protective effect of TFA in a mouse model of viral myocarditis is through mediating the translation and transcription of proteins that are applied in ER stress and the UPR pathway rather than regulating of calcium mobilizing proteins. Interestingly, high dosages of TFA treatment did not rescue the deficit in calumenin mRNA levels, which is emphasizing the limited sufficient dose range or therapeutic window of this flavonoid. In conclusion, the study stressed the therapeutic potential of TFA in treating viral myocarditis, suggesting that this compound conserves pathogen-caused cardiomyopathy by regulating those elements involved in ER stress and calcium homeostasis ER stress in cardiomyocytes. For the first time, TFA was shown to prevent loss of mRNA and protein levels of calumenin and also maintained the connection of this protein with the Sarco-endoplasmic reticulum Ca2+-ATPase (SERCA2) (Zhou et al., 2016). Xuan et al. (2016) evaluated the impact of whole flavonoids on the expression of ER connexin 43, calumenin, and chaperone in suckling mouse myocardium heart muscle inflammation induced by CVB3. They randomly divided the initial culture of mouse myocardium cells into total flavonoids, CVB3 infected group, and control group. CVB3 infection perhaps induces ER stress of myocardium cells by decreasing the expression of CX43 and calumenin and raising the expression of GRP78. The results of this investigation may be closely linked to the results of anti-arrhythmia with virus-related myocarditis yielded by CVB3 (Xuan et al., 2016).
14 Cardioprotection
14.1 Kaempferol
Kaempferol (3,4/,5,1-tetrahydroxyflavoune) is a yellow flavonoid compound that is seen in different fruits and vegetables like broccoli, cabbage, tea, etc., (Georgiev et al., 2014). Kaempferol is often administrated in traditional medicine because of its multiple therapeutic and biological activities including antioxidants (Du et al., 2018; Wu et al., 2018), anti-obesity (Torres-Villarreal et al., 2019), and cardiovascular protection (Zhou et al., 2015b; Suchal et al., 2016; Xiao et al., 2016; Suchal et al., 2017). Targeting ER stress pathways seems to be useful in related diseases. Meanwhile, among the traditional herb medicine, kaempferol as a flavonoid appears to be hopeful to adjust ER stress and autophagy and display protective impacts on cells. Some reports are showing the ability of kaempferol in influencing ER stress and autophagy. Briefly, kaempferol adjusts autophagy in non-cancerous cells to protect cells against dysfunction.
Kaempferol can exert a modulatory effect on ER stress. Upon ER stress, protein kinase RNA-like endoplasmic reticulum kinase (PERK), inositol-requiring enzyme 1 (IRE1α), and activating transcription factor 6 (ATF6) undergo activation to decrease a load of stress, and kaempferol also prompt these pathways. In addition, kaempferol produces CHOP through IRE1α, PERK, and ATF6 to trigger apoptotic cell death and reduce ER stress. Another key pathway for ER stress blockage by kaempferol is the prompting of 78-kDa glucose-regulated protein (GRP78) (Ashrafizadeh et al., 2020). Markedly, during stress, autophagy is activated to reduce the load of stress and loss of autophagy in performing this target causes the induction of apoptosis. Following this strategy, kaempferol positively impacts the AMPK/mTOR (mammalian target of rapamycin) signaling pathway to yield autophagy, leading to ER stress inhibition. Although, if the induced autophagy could not reduce the stress, kaempferol activates CHOP via pathways leading to apoptosis (Ashrafizadeh et al., 2020). In a study by Kim et al. (2008), cardioprotective effects of a 10 μm dosage of Kaempferol on the H9c2 cardiac muscle cells were observed. Major outcomes were protection against ischemic/reperfusion damage by down-regulation of ER stress-related markers like ATF6, GRP78, ATF6, x-box binding protein 2 (XBP-2), CHOP, and IRE1. To the best of our knowledge, ATF6, IRE1α, and PERK are considered the three main upstream regulators of ER stress (Ghobadi et al., 2017; Najafi et al., 2017; Rezapoor et al., 2017). It was recommended that kaempferol is a naturally occurring compound that has inhibitory and stimulatory function on ER stress (Ashrafizadeh et al., 2020).
14.2 Wogonin
Qu et al. (2015) reported that wogonin prevented the increase of Ca2+ initiated by KCl (60 mm) after exhausting calcium stored in sarcoplasmic and endoplasmic reticula with thapsigargin (1 μm) or by ATP (100 μm) in VSMCs. Wogonin is a flavone and has a mixture of cardiovascular defensive effects. This report showed that wogonin has therapeutic potential for cerebrovascular and cardiovascular diseases. Although, it was informed that little wogonin was uncovered in rat plasma after an intragastric regime of wogonin (5 mg/kg).
14.3 Naringenin and naringin
Different in vitro and in vivo studies have verified the positive effects of naringenin on the prevention of atherosclerosis progression. In an experiment, favorable effects of naringenin and naringin as powerful anti-atherogenic compounds were detected in rabbits fed a high-cholesterol regime. It has been discovered that the anti-atherogenic result is mainly correlated to a reduced hepatic acyl-coenzyme A, cholesterol acyltransferase activity, downregulation of monocyte chemotactic protein-1 (MCP-1), and VCAM-1 genes (Lee et al., 2001). The impacts of the naringenin cure against cardiac hypertrophy may be related to reducing angiotensin-converting enzyme 1 (ACE1) and angiotensin II expression in cardiac tissues (INVALID CITATION). Naringenin provided conservation against ischemia/reperfusion damage by stimulating mitochondrial biogenesis and protecting mitochondrial function by the AMP-activated protein kinase-sirtuin 3 signaling pathway (Yu et al., 2019b). Contrary to lipid accumulation, naringin protects the myocardium (Rajadurai and Mainzen Prince, 2006). In a confirmatory study, naringenin stimulated myocardial cGMP peroxisome proliferator-activated receptor gamma coactivator 1-alpha signaling in vivo and in vitro which was conducted to reduce oxidative stress and ER stress levels, in addition to inhibited myocardial apoptosis during Ischemia/reperfusion state (Yu et al., 2019a). Naringenin defended the heart via the activation of mitochondrial large-conductance calcium-activated potassium channel (mitoBK) and could establish cardioprotection in senescent H9c2 cardiomyoblasts (Testai et al., 2017). Naringenin has a potent defensive impact on the myocardial cells against age-related harm by modulating the ROS levels, the estrogen-associated pathway, and mitochondrial potassium channels (Da Pozzo et al., 2017). Xu et al. (2019) discovered the impacts and effects of naringenin on cardiovascular diseases and lipoprotein profiles in vitro and in vivo. The results indicated that naringenin decreased ER stress in atherosclerotic stations and the ER stress-ATF 6 paths were involved with the naringenin-inhibited lipid accumulation and foam cell-building.
15 Others
15.1 Modulating ER stress in cardiovascular disease
15.1.1 Quercetin
Quercetin has been shown to reduce the creation of ROS impeding pyrin domain containing-3 (NLRP3), the NOD-like receptor family, and thioredoxin-interacting protein (TXNIP) inflammasome activation caused by palmitate. TXNIP and NLRP3 stimulate the induction of IL-6 and IL-1 β, which can cause ER stress. In addition, quercetin can promote basal AMPK activity by improving the phosphorylation of AMPK increasing nitric oxide (NO) production in endothelial cells, and preventing cell apoptosis through changing δψm (Wu et al., 2014). The inhibitory effects of 30, 40-dihydroxyflavonol (DHF) or 5,7-dideoxyquercetin on ER stress caused by tunicamycin were evaluated in the C57BLK/6 J mice’s aorta. DHF decreased the expression of GRP78 and CHOP, caspase-3 cleaved structure, and eif2α phosphorylation. Moreover, DHF decreased ROS generation and increased NO in aortic rings. An in vitro study showed that DHF reduced eIF2 α phosphorylation and GRP78 expression induced a rise in XBP1 splicing and induced apoptosis (Lau et al., 2018). Oral administration of quercetin (10 mg/kg/day) was found to prevent the exacerbation of experimental autoimmune myocarditis to dilated cardiomyopathy. Quercetin reduced the myocardial expression of GRP78 and GADD153. In addition, it suppressed the endothelin-1 (ET-1) and myocardial MAPK yielding the progression of experimental autoimmune myocarditis. Quercetin reduced the expression of mouse polyclonal antiosteopontin and TGF-ß 1 that emerge in fibrosis. It even decreased the cytosolic cytochrome C amount which was increased in the apoptosis (Arumugam et al., 2012). Therefore, based on these investigations, quercetin can be effective to decrease the impact of ER stress signaling ways and preserving against cardiovascular disease (Eisvand et al., 2022).
15.2 Blood pressure and left ventricular remodeling
15.2.1 Baicalin
An in vivo study conducted by Dai et al. (2017) assessed how baicalin affects left ventricular remodeling and also changes blood pressure in renal hypertension rats. The control group administered .9% NaCl solution, while the study group received baicalin at the concentration of 100 mg/kg with .9% NaCl solution once a day for 4 weeks. The result of the study showed significant positive effects on left ventricular remodeling and a noticeable decline in the expression of fibrosis-related factors and caspase-3. Moreover, the expression of glucose-regulated proteins including GRP78 and GRP94, and also pro-apoptotic factors including CHOP and caspase-3 decreased in the study group receiving baicalin.
15.3 Insulin resistance and hyperlipidemia
15.3.1 Apigenin
Wu et al. (2021) evaluated the role of apigenin in insulin resistance in mice. They found that apigenin could lower the levels of stearyl-CoA desaturase 1, sterol regulatory element-binding protein 2 (SREBP-2), sterol regulatory element-binding protein 1c (SREBP-1c), 3-hydroxy-3-methyl-glutaric CoA reductase, fatty acid synthase, and accumulation of lipid in the cells of the specimens, and thus leading to body weight loss. In addition, apigenin can reduce insulin resistance by decreasing ER stress. They concluded that apigenin can reduce hyperlipidemia, insulin resistance, body fat, and consequently diabetes and cardiovascular diseases.
15.4 Cardiac dysfunction
15.4.1 Fisetin
A study by Ge et al. (2019) showed how fisetin and metformin remarkably suppressed metabolic stress-induced cardiomyopathy. Fisetin is amongst the most significant edible flavonoids with conceivably useful properties for human health (Khan et al., 2013; Shi et al., 2018; Yousefzadeh et al., 2018). Mice were selected at random to be fed an HFD (high-fat diet) and were given 4-PBA (4-phenyl butyric acid), fisetin, and metformin solution by gavage daily; this continued for 16 weeks. The therapeutic effects of fisetin and metformin on metabolic stress-induced heart injury were confirmed in HFD-challenged mice in vivo. Fisetin or metformin treatment noticeably reduced HFD-induced heart weight and heart weight to tibia length ratio. Furthermore, fisetin or metformin decreased IL-1β, IL-4, F4/80, IL-6, TNF-α, and CCL2 mRNA levels in cardiac samples from HFD-fed mice. Fisetin or metformin significantly recovered superoxide dismutase activity and lowered malondialdehyde levels in HFD-induced heart samples. The in vitro study confirmed that fisetin or metformin exposure exhibited an important role in easing PAL-caused oxidative stress, ER stress, inflammation, and dyslipidemia in cardiomyocytes and macrophages. At last, western blot analysis demonstrated that fisetin or metformin treatment supposedly prevents the expression of p-PERKT980, p-eif2α S51, pire1s724, XBP1, and CHOP in heart tissues from HFD-challenged mice. In conclusion, the results suggested that fisetin or metformin could reduce metabolic stress-caused cardiac inflammation, abnormal function, oxidative stress, and ER stress (Ge et al., 2019).
15.5 Hypertensive heart disease
15.5.1 Icariside II
A study by Wu et al. (2019) aimed to discover whether Icariside II can inhibit ER stress-caused cardiomyocyte apoptosis through the PERK/ATF-4/CHOP signaling pathway. Hypertensive rats were separated into model groups and Icariside II groups at random. The rats in the Icariside II groups were intragastrically managed with Icariside II 4, 8, and 16 mg/kg from 14 to 26 weeks-age. At the end of the 26th week, cardiomyocyte apoptosis was analyzed and the levels of GRP78, PERK, ATF-4, and CHOP gene and protein were detected. In vivo study highlighted that the activation capacity of protein kinase RNA-like ER kinase was increased, and the expression levels of ATF-4 and CHOP protein were upregulated in spontaneously hypertensive rats, demonstrating that cardiomyocyte apoptosis caused by ER stress was through activating PERK. Icariside II weakened the phosphorylation capacity of protein kinase RNA-like ER kinase and reduced the expression of ATF-4 and CHOP, signifying its inhibitory effect on the PERK signaling pathway. To conclude, Icariside II prevents hypertensive heart disease by alleviating ER stress-induced cardiomyocyte apoptosis, and its mechanism is associated with the inhibition of the PERK/ATF-4/CHOP signaling pathway.
15.6 Myocardial injury
15.6.1 Vitexin
The study by Ashokkumar et al. (2018) focused on the improvement of ER function via suppression of the extra ROS production with the raw flavonoid Vitexin. This study was designed to determine the impact of isoproterenol agonist b-AR moderated stimulus of ER stress and its joint activation of hippo signaling in the heart in the time of post-myocardial infarction. In brief, it showed the therapeutic ability of Vitexin against post-myocardial infarction and may pavSe the way for expanding a natural drug against post-myocardial infarction individuals as a non-invasive treatment (Ashokkumar et al., 2018).
16 Conclusion
Amongst all the groups of Flavonoids that have been reviewed in this study, Flavonols, Flavanones, and Flavones have been the most preferred groups; which include familiar cardioprotective compounds such as Quercetin, Kaempferol, Naringenin, Nobiletin, and Apigenin. Quercetin which might be the most known member of the Flavonol group and even Flavonoids in general could be found in red onion, kale, and many other vegetables. Studies showed that quercetin can decrease ER stress, either protecting against oxidative stress injuries or mitigating ER stress directly and the related apoptosis. Quercetin was protective in myocarditis by preventing mitochondria dysfunction and caspase activity inhibition in H9c2 cardiomyoblasts. Moreover, it could have been able to downregulate important ER stress markers such as GADD153, GRP78, and cytosolic Cytochrome C levels. Kaempferol is another subtype of flavonol and natural anti-oxidant that has been proven to have an inhibitory effect on ER stress proteins, such as GRP78, ATF-6alpha, and CHOP and an anti-apoptotic effect by regulating B-cl2 and Bax proteins, which can explain its impressive role in protection against ischemic heart disease. Naringenin, a Flavanone derived from grapefruit and herbs, also has been reported frequently to have anti-oxidant effects. It reduces ER stress levels by activating the cGMP-PKGIα signaling pathway. Since ER stress-associated apoptosis can be a consequence of myocardial ischemia/reperfusion injury, further in vivo-in vitro studies may demonstrate the pharmacological effects of this compound in myocardial ischemia/reperfusion patients. Nobiletin and Apigenin are both members of the Flavone group. In vivo experiments about cardiac hypertrophy have displayed inhibiting effects for Nobiletin on NOX4 and NOX2 expression, which can suppress ER stress. It can also attenuate the apoptosis that is mediated by ER stress by regulating PI3K/AKT pathway in myocardial ischemia/reperfusion injury. Apigenin is also beneficial in myocardial ischemia/reperfusion injuries and in modifying ER stress via the AMPK pathway. Some outstanding questions in the context of flavonoid intake in cardiovascular diseases remain for further studies. For instance, is there any place for flavonoids to show synergistic effects in combination with other cardiovascular medications? Does the consumption of flavonoids have significant side effects? Do any nano-formulated flavonoids exist? If yes, what are their advantages rather to conventional formulations? More pre-clinical investigations and further RCTs could be helpful to confirm Flavonoids’ therapeutic effect in clinical settings.
Author contributions
Data acquisition: KK, DA, NK, FA, NN, PS, MK, AR, MA. Manuscript drafting: KK, FA, AK, AR, DA, MK, PS, NK, NN, MP, MA, ME, SH. Manuscript revision: AMK, EA, AFK, ARA, AMA. Conception, design, and supervision: ND.
Acknowledgments
The authors would like to thank the researchers whose works were included in this study.
Conflict of interest
The authors declare that the research was conducted in the absence of any commercial or financial relationships that could be construed as a potential conflict of interest.
Publisher’s note
All claims expressed in this article are solely those of the authors and do not necessarily represent those of their affiliated organizations, or those of the publisher, the editors and the reviewers. Any product that may be evaluated in this article, or claim that may be made by its manufacturer, is not guaranteed or endorsed by the publisher.
References
Arumugam, S., Thandavarayan, R. A., Arozal, W., Sari, F. R., Giridharan, V. V., Soetikno, V., et al. (2012). Quercetin offers cardioprotection against progression of experimental autoimmune myocarditis by suppression of oxidative and endoplasmic reticulum stress via endothelin-1/MAPK signalling. Free Radic. Res. 46 (2), 154–163. doi:10.3109/10715762.2011.647010
Ashokkumar, R., Jamuna, S., Sadullah, M. S., and Devaraj, S. N. (2018). Vitexin protects isoproterenol induced post myocardial injury by modulating hipposignaling and ER stress responses. Biochem. biophysical Res. Commun. 496 (2), 731–737. doi:10.1016/j.bbrc.2018.01.104
Ashrafizadeh, M., Tavakol, S., Ahmadi, Z., Roomiani, S., Mohammadinejad, R., and Samarghandian, S. (2020). Therapeutic effects of kaempferol affecting autophagy and endoplasmic reticulum stress. Phytother. Res. 34 (5), 911–923. doi:10.1002/ptr.6577
Badawy Khair, N. S., and Mohammed, S. A. J. E. J. (2021). A comparative study on the protective role of silymarin and coenzyme-Q10 on the cerebellar cortex of experimentally induced atherosclerosis in adult male albino rats: A histological. Immunohistochem. Biochem. study 44 (2), 322–338. doi:10.21608/EJH.2020.28009.1276
Bekendam, R. H., and Flaumenhaft, R. (2016). Inhibition of protein disulfide isomerase in thrombosis. Basic & Clin. Pharmacol. Toxicol. 119, 42–48. doi:10.1111/bcpt.12573
Belke, D. D., and Dillmann, W. H. (2004). Altered cardiac calcium handling in diabetes. Curr. Hypertens. Rep. 6 (6), 424–429. doi:10.1007/s11906-004-0035-3
Belmont, P. J., Chen, W. J., San Pedro, M. N., Thuerauf, D. J., Gellings Lowe, N., Gude, N., et al. (2010). Roles for endoplasmic reticulum-associated degradation and the novel endoplasmic reticulum stress response gene Derlin-3 in the ischemic heart. Circ. Res. 106 (2), 307–316. doi:10.1161/CIRCRESAHA.109.203901
Bers, D. M., and Berlin, J. R. (1995). Kinetics of [Ca] i decline in cardiac myocytes depend on peak [Ca] i. Am. J. Physiology-Cell Physiology 268 (1), C271–C277. doi:10.1152/ajpcell.1995.268.1.C271
Bers, D. M., and Bridge, J. (1989). Relaxation of rabbit ventricular muscle by Na-Ca exchange and sarcoplasmic reticulum calcium pump. Ryanodine and voltage sensitivity. Circulation Res. 65 (2), 334–342. doi:10.1161/01.res.65.2.334
Cai, X., Bao, L., Dai, X., Ding, Y., Zhang, Z., and Li, Y. (2015). Quercetin protects RAW264.7 macrophages from glucosamine-induced apoptosis and lipid accumulation via the endoplasmic reticulum stress pathway. Mol. Med. Rep. 12 (5), 7545–7553. doi:10.3892/mmr.2015.4340
Campeau, M. A., and Leask, R. L. (2022). Empagliflozin mitigates endothelial inflammation and attenuates endoplasmic reticulum stress signaling caused by sustained glycocalyx disruption. Sci. Rep. 12 (1), 12681. doi:10.1038/s41598-022-16763-6
Carrasco-Pozo, C., Pastene, E., Vergara, C., Zapata, M., Sandoval, C., and Gotteland, M. (2012). Stimulation of cytosolic and mitochondrial calcium mobilization by indomethacin in caco-2 cells: Modulation by the polyphenols quercetin, resveratrol and rutin. Biochimica Biophysica Acta (BBA)-General Subj. 1820 (12), 2052–2061. doi:10.1016/j.bbagen.2012.09.015
Castillo, R. L., Herrera, E. A., Gonzalez-Candia, A., Reyes-Farias, M., de la Jara, N., Peña, J. P., et al. (2018). Quercetin prevents diastolic dysfunction induced by a high-cholesterol diet: Role of oxidative stress and bioenergetics in hyperglycemic rats. Oxidative Med. Cell. Longev. 2018, 7239123. doi:10.1155/2018/7239123
Chang, X., Zhang, T., Meng, Q., Yan, P., Wang, X., Luo, D., et al. (2021). Quercetin improves cardiomyocyte vulnerability to hypoxia by regulating SIRT1/TMBIM6-related mitophagy and endoplasmic reticulum stress. Oxidative Med. Cell. Longev. 2021, 5529913. doi:10.1155/2021/5529913
Chen, J., Zhang, M., Zhu, M., Gu, J., Song, J., Cui, L., et al. (2018). Paeoniflorin prevents endoplasmic reticulum stress-associated inflammation in lipopolysaccharide-stimulated human umbilical vein endothelial cells via the IRE1α/NF-κB signaling pathway. Food Funct. 9 (4), 2386–2397. doi:10.1039/c7fo01406f
Chin, K. T., Kang, G., Qu, J., Gardner, L. B., Coetzee, W. A., Zito, E., et al. (2011). The sarcoplasmic reticulum luminal thiol oxidase ERO1 regulates cardiomyocyte excitation-coupled calcium release and response to hemodynamic load. Faseb J. 25 (8), 2583–2591. doi:10.1096/fj.11-184622
Choi, K. M., Zhong, Y., Hoit, B. D., Grupp, I. L., Hahn, H., Dilly, K. W., et al. (2002). Defective intracellular Ca2+ signaling contributes to cardiomyopathy in Type 1 diabetic rats. Am. J. Physiology-Heart Circulatory Physiology 283 (4), H1398–H1408. doi:10.1152/ajpheart.00313.2002
Choi, S. Y., Huang, P., Jenkins, G. M., Chan, D. C., Schiller, J., and Frohman, M. A. (2006). A common lipid links Mfn-mediated mitochondrial fusion and SNARE-regulated exocytosis. Nat. Cell Biol. 8 (11), 1255–1262. doi:10.1038/ncb1487
Choy, K. W., Lau, Y. S., Murugan, D., and Mustafa, M. R. (2017). Chronic treatment with paeonol improves endothelial function in mice through inhibition of endoplasmic reticulum stress-mediated oxidative stress. PLoS One 12 (5), e0178365. doi:10.1371/journal.pone.0178365
Chtourou, Y., Slima, A. B., Makni, M., Gdoura, R., and Fetoui, H. (2015). Naringenin protects cardiac hypercholesterolemia-induced oxidative stress and subsequent necroptosis in rats. Pharmacol. Rep. 67 (6), 1090–1097. doi:10.1016/j.pharep.2015.04.002
Civelek, M., Manduchi, E., Riley, R. J., Stoeckert, C. J., and Davies, P. F. (2011). Coronary artery endothelial transcriptome in vivo: Identification of endoplasmic reticulum stress and enhanced reactive oxygen species by gene connectivity network analysis. Circ. Cardiovasc Genet. 4 (3), 243–252. doi:10.1161/CIRCGENETICS.110.958926
Cushnie, T. P., and Lamb, A. J. (2005). Antimicrobial activity of flavonoids. Int. J. Antimicrob. Agents 26 (5), 343–356. doi:10.1016/j.ijantimicag.2005.09.002
Da Pozzo, E., Costa, B., Cavallini, C., Testai, L., Martelli, A., Calderone, V., et al. (2017). The citrus flavanone naringenin protects myocardial cells against age-associated damage. Oxidative Med. Cell. Longev. 2017, 9536148. doi:10.1155/2017/9536148
Dai, H., Zhang, X., Yang, Z., Li, J., and Zheng, J. (2017). Effects of baicalin on blood pressure and left ventricular remodeling in rats with renovascular hypertension. Med. Sci. Monit. Int. Med. J. Exp. Clin. Res. 23, 2939–2948. doi:10.12659/msm.902536
Deng, J., Lu, P. D., Zhang, Y., Scheuner, D., Kaufman, R. J., Sonenberg, N., et al. (2004). Translational repression mediates activation of nuclear factor kappa B by phosphorylated translation initiation factor 2. Mol. Cell Biol. 24 (23), 10161–10168. doi:10.1128/MCB.24.23.10161-10168.2004
Dickhout, J. G., Carlisle, R. E., and Austin, R. C. (2011). Interrelationship between cardiac hypertrophy, heart failure, and chronic kidney disease: Endoplasmic reticulum stress as a mediator of pathogenesis. Circulation Res. 108 (5), 629–642. doi:10.1161/CIRCRESAHA.110.226803
Du, W., An, Y., He, X., Zhang, D., and He, W. (2018). Protection of kaempferol on oxidative stress-induced retinal pigment epithelial cell damage. Oxid. Med. Cell Longev. 2018, 1610751. doi:10.1155/2018/1610751
Duan, Q., Song, P., Ding, Y., and Zou, M. H. (2017). Activation of AMP-activated protein kinase by metformin ablates angiotensin II-induced endoplasmic reticulum stress and hypertension in mice in vivo. Br. J. Pharmacol. 174 (13), 2140–2151. doi:10.1111/bph.13833
Eisvand, F., Tajbakhsh, A., Seidel, V., Zirak, M. R., Tabeshpour, J., and Shakeri, A. (2022). Quercetin and its role in modulating endoplasmic reticulum stress: A review. Phytotherapy Res. 36 (1), 73–84. doi:10.1002/ptr.7283
Eura, Y., Ishihara, N., Oka, T., and Mihara, K. (2006). Identification of a novel protein that regulates mitochondrial fusion by modulating mitofusin (Mfn) protein function. J. Cell Sci. 119, 4913–4925. doi:10.1242/jcs.03253
Feng, W., Hwang, H. S., Kryshtal, D. O., Yang, T., Padilla, I. T., Tiwary, A. K., et al. (2012). Coordinated regulation of murine cardiomyocyte contractility by nanomolar (-)-epigallocatechin-3-gallate, the major green tea catechin. Mol. Pharmacol. 82 (5), 993–1000. doi:10.1124/mol.112.079707
Feng, Y., Lu, Y., Liu, D., Zhang, W., Liu, J., Tang, H., et al. (2018). Apigenin-7-O-β-d-(-6 ″-p-coumaroyl)-glucopyranoside pretreatment attenuates myocardial ischemia/reperfusion injury via activating AMPK signaling. Life Sci. 203, 246–254. doi:10.1016/j.lfs.2018.04.048
Flaumenhaft, R. (2013). Protein disulfide isomerase as an antithrombotic target. Trends Cardiovasc. Med. 23 (7), 264–268. doi:10.1016/j.tcm.2013.03.001
Frustaci, A., Ciccosanti, F., Chimenti, C., Nardacci, R., Corazzari, M., Verardo, R., et al. (2016). Histological and proteomic profile of diabetic versus non-diabetic dilated cardiomyopathy. Int. J. Cardiol. 203, 282–289. doi:10.1016/j.ijcard.2015.10.119
Gao, Y., Wang, Z., Zhang, Y., Liu, Y., Wang, S., Sun, W., et al. (2018). Naringenin inhibits NG-nitro-L-arginine methyl ester-induced hypertensive left ventricular hypertrophy by decreasing angiotensin-converting enzyme 1 expression. Exp. Ther. Med. 16 (2), 867–873. doi:10.3892/etm.2018.6258
Ge, C. X., Xu, M. X., Qin, Y. T., Gu, T. T., Lou, D. S., Li, Q., et al. (2019). Endoplasmic reticulum stress-induced iRhom2 up-regulation promotes macrophage-regulated cardiac inflammation and lipid deposition in high fat diet (HFD)-challenged mice: Intervention of fisetin and metformin. Free Radic. Biol. Med. 141, 67–83. doi:10.1016/j.freeradbiomed.2019.05.031
George, I., Sabbah, H. N., Xu, K., Wang, N., and Wang, J. (2011). β-adrenergic receptor blockade reduces endoplasmic reticulum stress and normalizes calcium handling in a coronary embolization model of heart failure in canines. Cardiovasc Res. 91 (3), 447–455. doi:10.1093/cvr/cvr106
Georgiev, V., Ananga, A., and Tsolova, V. (2014). Recent advances and uses of grape flavonoids as nutraceuticals. Nutrients 6 (1), 391–415. doi:10.3390/nu6010391
Ghobadi, A., Shirazi, A., Najafi, M., Kahkesh, M. H., and Rezapoor, S. (2017). Melatonin ameliorates radiation-induced oxidative stress at targeted and nontargeted lung tissue. J. Med. Phys. 42 (4), 241–244. doi:10.4103/jmp.JMP_60_17
Giordano, E., Davalos, A., Nicod, N., and Visioli, F. (2014). Hydroxytyrosol attenuates tunicamycin-induced endoplasmic reticulum stress in human hepatocarcinoma cells. Mol. Nutr. Food Res. 58 (5), 954–962. doi:10.1002/mnfr.201300465
Haas, M. J., Jafri, M., Wehmeier, K. R., Onstead-Haas, L. M., and Mooradian, A. D. (2016). Inhibition of endoplasmic reticulum stress and oxidative stress by vitamin D in endothelial cells. Free Radic. Biol. Med. 99, 1–10. doi:10.1016/j.freeradbiomed.2016.07.020
Hamaguchi, S., Abe, K., Komatsu, M., Kainuma, J., Namekata, I., and Tanaka, H. (2021). Positive lusitropic effect of quercetin on isolated ventricular myocardia from normal and streptozotocin-induced diabetic mice. Biol. Pharm. Bull. 44 (12), 1894–1897. doi:10.1248/bpb.b21-00580
Han, X., Gao, S., Cheng, Y., Sun, Y., Liu, W., Tang, L., et al. (2012). Protective effect of naringenin-7-O-glucoside against oxidative stress induced by doxorubicin in H9c2 cardiomyocytes. Biosci. Trends 6 (1), 19–25. doi:10.5582/bst.2012.v6.1.19
Hong, J., Kim, K., Kim, J. H., and Park, Y. (2017). The role of endoplasmic reticulum stress in cardiovascular disease and exercise. Int. J. Vasc. Med. 2017, 2049217. doi:10.1155/2017/2049217
Hotamisligil, G. S. (2010). Endoplasmic reticulum stress and the inflammatory basis of metabolic disease. Cell 140 (6), 900–917. doi:10.1016/j.cell.2010.02.034
Huang, A., Patel, S., McAlpine, C. S., and Werstuck, G. H. (2018). The role of endoplasmic reticulum stress-glycogen synthase kinase-3 signaling in atherogenesis. Int. J. Mol. Sci. 19 (6), 1607. doi:10.3390/ijms19061607
Hundal, R. S., Krssak, M., Dufour, S., Laurent, D., Lebon, V., Chandramouli, V., et al. (2000). Mechanism by which metformin reduces glucose production in type 2 diabetes. Diabetes 49 (12), 2063–2069. doi:10.2337/diabetes.49.12.2063
Ivanova, E. A., and Orekhov, A. N. (2016). The role of endoplasmic reticulum stress and unfolded protein response in atherosclerosis. Int. J. Mol. Sci. 17 (2), 193. doi:10.3390/ijms17020193
Izumiya, Y., Kojima, S., Kojima, S., Araki, S., Usuku, H., Matsubara, J., et al. (2011). Long-term use of oral nicorandil stabilizes coronary plaque in patients with stable angina pectoris. Atherosclerosis 214 (2), 415–421. doi:10.1016/j.atherosclerosis.2010.11.032
Jasuja, R., Passam, F. H., Kennedy, D. R., Kim, S. H., van Hessem, L., Lin, L., et al. (2012). Protein disulfide isomerase inhibitors constitute a new class of antithrombotic agents. J. Clin. investigation 122 (6), 2104–2113. doi:10.1172/JCI61228
Jia, F., Wu, C., Chen, Z., and Lu, G. (2012). Atorvastatin inhibits homocysteine-induced endoplasmic reticulum stress through activation of AMP-activated protein kinase. Cardiovasc Ther. 30 (6), 317–325. doi:10.1111/j.1755-5922.2011.00287.x
Joo, J. H., Ueda, E., Bortner, C. D., Yang, X. P., Liao, G., and Jetten, A. M. (2015). Farnesol activates the intrinsic pathway of apoptosis and the ATF4-ATF3-CHOP cascade of ER stress in human T lymphoblastic leukemia Molt4 cells. Biochem. Pharmacol. 97 (3), 256–268. doi:10.1016/j.bcp.2015.08.086
Khan, N., Syed, D. N., Ahmad, N., and Mukhtar, H. (2013). Fisetin: A dietary antioxidant for health promotion. Antioxid. Redox Signal 19 (2), 151–162. doi:10.1089/ars.2012.4901
Kim, D-S., Ha, K-C., Kwon, D-Y., Kim, M-S., Kim, H-R., Chae, S-W., et al. (2008). Kaempferol protects ischemia/reperfusion-induced cardiac damage through the regulation of endoplasmic reticulum stress. Immunopharmacol. Immunotoxicol. 30 (2), 257–270. doi:10.1080/08923970701812530
Kim, D. S., Kwon, D. Y., Kim, M. S., Kim, H. K., Lee, Y. C., Park, S. J., et al. (2010). The involvement of endoplasmic reticulum stress in flavonoid-induced protection on cardiac cell death caused by ischaemia/reperfusion. J. Pharm. Pharmacol. 62 (2), 197–204. doi:10.1211/jpp.62.02.0007
Kiraz, Y., Adan, A., Kartal Yandim, M., and Baran, Y. (2016). Major apoptotic mechanisms and genes involved in apoptosis. Tumor Biol. 37 (7), 8471–8486. doi:10.1007/s13277-016-5035-9
Lau, Y. S., Mustafa, M. R., Choy, K. W., Chan, S. M. H., Potocnik, S., Herbert, T. P., et al. (2018). 3′, 4′-dihydroxyflavonol ameliorates endoplasmic reticulum stress-induced apoptosis and endothelial dysfunction in mice. Sci. Rep. 8 (1), 1818. doi:10.1038/s41598-018-19584-8
Lee, C-H., Jeong, T-S., Choi, Y-K., Hyun, B-H., Oh, G-T., Kim, E-H., et al. (2001). Anti-atherogenic effect of citrus flavonoids, naringin and naringenin, associated with hepatic ACAT and aortic VCAM-1 and MCP-1 in high cholesterol-fed rabbits. Biochem. Biophysical Res. Commun. 284 (3), 681–688. doi:10.1006/bbrc.2001.5001
Lerner, A. G., Upton, J. P., Praveen, P. V., Ghosh, R., Nakagawa, Y., Igbaria, A., et al. (2012). IRE1α induces thioredoxin-interacting protein to activate the NLRP3 inflammasome and promote programmed cell death under irremediable ER stress. Cell Metab. 16 (2), 250–264. doi:10.1016/j.cmet.2012.07.007
Li, J., Wu, W., Xin, Y., Zhao, M., and Liu, X. (2018). Inhibition of Nogo-B promotes cardiac hypertrophy via endoplasmic reticulum stress. Biomed. Pharmacother. 104, 193–203. doi:10.1016/j.biopha.2018.05.039
Li, T., Jiang, S., Lu, C., Hu, W., Ji, T., Han, M., et al. (2018). Snapshots: Endoplasmic reticulum stress in lipid metabolism and cardiovascular disease. Curr. issues Mol. Biol. 28, 14–28. doi:10.21775/cimb.028.014
Liang, Y., Zhang, Y., Liu, M., Han, X., Zhang, J., Zhang, X., et al. (2020). Protective effect of quercetin against myocardial ischemia as a Ca2+ channel inhibitor: Involvement of inhibiting contractility and Ca2+ influx via L-type Ca2+ channels. Archives Pharmacal Res. 43 (8), 808–820. doi:10.1007/s12272-020-01261-y
Libby, P., Ridker, P. M., and Maseri, A. (2002). Inflammation and atherosclerosis. Circulation 105 (9), 1135–1143. doi:10.1161/hc0902.104353
Liew, R., Macleod, K. T., and Collins, P. (2003). Novel stimulatory actions of the phytoestrogen genistein: Effects on the gain of cardiac excitation-contraction coupling. Faseb J. 17 (10), 1307–1309. doi:10.1096/fj.02-0760fje
Lin, J. H., Walter, P., and Yen, T. S. (2008). Endoplasmic reticulum stress in disease pathogenesis. Annu. Rev. Pathol. 3, 399–425. doi:10.1146/annurev.pathmechdis.3.121806.151434
Liu, M., Chen, Z., and Chen, L. (2016). Endoplasmic reticulum stress: A novel mechanism and therapeutic target for cardiovascular diseases. Acta Pharmacol. Sin. 37 (4), 425–443. doi:10.1038/aps.2015.145
Liu, Z., Cai, H., Zhu, H., Toque, H., Zhao, N., Qiu, C., et al. (2014). Protein kinase RNA-like endoplasmic reticulum kinase (PERK)/calcineurin signaling is a novel pathway regulating intracellular calcium accumulation which might be involved in ventricular arrhythmias in diabetic cardiomyopathy. Cell. Signal. 26 (12), 2591–2600. doi:10.1016/j.cellsig.2014.08.015
Liu, Z., Zhang, Y., Pan, S., Qiu, C., Jia, H., Wang, Y., et al. (2021). Activation of RAGE-dependent endoplasmic reticulum stress associates with exacerbated postmyocardial infarction ventricular arrhythmias in diabetes. Am. J. Physiol. Endocrinol. Metab. 320 (3), E539–E550. doi:10.1152/ajpendo.00450.2020
Mahmoud, A. M., Hernández Bautista, R. J., Sandhu, M. A., and Hussein, O. E. (2019). Beneficial effects of citrus flavonoids on cardiovascular and metabolic health. Oxid. Med. Cell Longev. 2019, 5484138. doi:10.1155/2019/5484138
Martin, M., Rehani, K., Jope, R. S., and Michalek, S. M. (2005). Toll-like receptor–mediated cytokine production is differentially regulated by glycogen synthase kinase 3. Nat. Immunol. 6 (8), 777–784. doi:10.1038/ni1221
Martinet, W., and Kockx, M. M. (2001). Apoptosis in atherosclerosis: Focus on oxidized lipids and inflammation. Curr. Opin. Lipidol. 12 (5), 535–541. doi:10.1097/00041433-200110000-00009
Martinon, F., Burns, K., and Tschopp, J. (2002). The inflammasome: A molecular platform triggering activation of inflammatory caspases and processing of proIL-beta. Mol. Cell 10 (2), 417–426. doi:10.1016/s1097-2765(02)00599-3
Martinon, F., and Tschopp, J. (2007). Inflammatory caspases and inflammasomes: Master switches of inflammation. Cell Death Differ. 14 (1), 10–22. doi:10.1038/sj.cdd.4402038
Miyazaki, Y., Kaikita, K., Endo, M., Horio, E., Miura, M., Tsujita, K., et al. (2011). C/EBP homologous protein deficiency attenuates myocardial reperfusion injury by inhibiting myocardial apoptosis and inflammation. Arterioscler. Thromb. Vasc. Biol. 31 (5), 1124–1132. doi:10.1161/ATVBAHA.111.224519
Najafi, M., Shirazi, A., Motevaseli, E., Geraily, G., Norouzi, F., Heidari, M., et al. (2017). The melatonin immunomodulatory actions in radiotherapy. Biophys. Rev. 9 (2), 139–148. doi:10.1007/s12551-017-0256-8
Namekata, I., Hiiro, H., Kanae, H., Yoshino, A., Kazama, A., Hamaguchi, S., et al. (2018). Acceleratory effect of ellagic acid on sarcoplasmic reticulum Ca<sup>2+</sup> uptake and myocardial relaxation. Int. J. Hum. Cult. Stud. 2018 (28), 701–707. doi:10.9748/hcs.2018.701
Ni, L., Zhou, C., Duan, Q., Lv, J., Fu, X., Xia, Y., et al. (2011). β-AR blockers suppresses ER stress in cardiac hypertrophy and heart failure. PLoS One 6 (11), e27294. doi:10.1371/journal.pone.0027294
Ohoka, N., Yoshii, S., Hattori, T., Onozaki, K., and Hayashi, H. (2005). TRB3, a novel ER stress-inducible gene, is induced via ATF4-CHOP pathway and is involved in cell death. Embo J. 24 (6), 1243–1255. doi:10.1038/sj.emboj.7600596
Ortíz-Rentería, M., Juárez-Contreras, R., González-Ramírez, R., Islas, L. D., Sierra-Ramírez, F., Llorente, I., et al. (2018). TRPV1 channels and the progesterone receptor Sig-1R interact to regulate pain. Proc. Natl. Acad. Sci. U. S. A. 115 (7), E1657–e66. doi:10.1073/pnas.1715972115
Park, J. K., Shao, M., Kim, M. Y., Baik, S. K., Cho, M. Y., Utsumi, T., et al. (2017). An endoplasmic reticulum protein, Nogo-B, facilitates alcoholic liver disease through regulation of kupffer cell polarization. Hepatology 65 (5), 1720–1734. doi:10.1002/hep.29051
Paschen, W., and Frandsen, A. (2001). Endoplasmic reticulum dysfunction–a common denominator for cell injury in acute and degenerative diseases of the brain? J. Neurochem. 79 (4), 719–725. doi:10.1046/j.1471-4159.2001.00623.x
Ponte, L. G. S., Pavan, I. C. B., Mancini, M. C. S., da Silva, L. G. S., Morelli, A. P., Severino, M. B., et al. (2021). The hallmarks of flavonoids in cancer. Mol. (Basel, Switz. 26 (7), 2029. doi:10.3390/molecules26072029
Pu, J., Yuan, A., Shan, P., Gao, E., Wang, X., Wang, Y., et al. (2013). Cardiomyocyte-expressed farnesoid-X-receptor is a novel apoptosis mediator and contributes to myocardial ischaemia/reperfusion injury. Eur. Heart J. 34 (24), 1834–1845. doi:10.1093/eurheartj/ehs011
Qian, Z., Zhu, L., Li, Y., Li, Y., Wu, Y., Fu, S., et al. (2021). Icarrin prevents cardiomyocyte apoptosis in spontaneously hypertensive rats by inhibiting endoplasmic reticulum stress pathways. J. Pharm. Pharmacol. 73 (8), 1023–1032. doi:10.1093/jpp/rgaa016
Qin, W., Ren, B., Wang, S., Liang, S., He, B., Shi, X., et al. (2016). Apigenin and naringenin ameliorate PKCβII-associated endothelial dysfunction via regulating ROS/caspase-3 and NO pathway in endothelial cells exposed to high glucose. Vasc. Pharmacol. 85, 39–49. doi:10.1016/j.vph.2016.07.006
Qu, J-T., Zhang, D-X., Liu, F., Mao, H-P., Ma, Y-K., Yang, Y., et al. (2015). Vasodilatory effect of wogonin on the rat aorta and its mechanism study. Biol. Pharm. Bull. 38 (12), 1873–1878. doi:10.1248/bpb.b15-00444
Rajadurai, M., and Mainzen Prince, P. S. (2006). Preventive effect of naringin on lipids, lipoproteins and lipid metabolic enzymes in isoproterenol-induced myocardial infarction in wistar rats. J. Biochem. Mol. Toxicol. 20 (4), 191–197. doi:10.1002/jbt.20136
Ramprasath, T., Senthamizharasi, M., Vasudevan, V., Sasikumar, S., Yuvaraj, S., and Selvam, G. S. (2014). Naringenin confers protection against oxidative stress through upregulation of Nrf2 target genes in cardiomyoblast cells. J. physiology Biochem. 70 (2), 407–415. doi:10.1007/s13105-014-0318-3
Ren, J., Bi, Y., Sowers, J. R., Hetz, C., and Zhang, Y. (2021). Endoplasmic reticulum stress and unfolded protein response in cardiovascular diseases. Nat. Rev. Cardiol. 18 (7), 499–521. doi:10.1038/s41569-021-00511-w
Rezapoor, S., Shirazi, A., Abbasi, S., Bazzaz, J. T., Izadi, P., Rezaeejam, H., et al. (2017). Modulation of radiation-induced base excision repair pathway gene expression by melatonin. J. Med. Phys. 42 (4), 245–250. doi:10.4103/jmp.JMP_9_17
Ron, D., and Walter, P. (2007). Signal integration in the endoplasmic reticulum unfolded protein response. Nat. Rev. Mol. Cell Biol. 8 (7), 519–529. doi:10.1038/nrm2199
Ross, R. (1999). Atherosclerosis--an inflammatory disease. N. Engl. J. Med. 340 (2), 115–126. doi:10.1056/NEJM199901143400207
Safiedeen, Z., Rodríguez-Gómez, I., Vergori, L., Soleti, R., Vaithilingam, D., Douma, I., et al. (2017). Temporal cross talk between endoplasmic reticulum and mitochondria regulates oxidative stress and mediates microparticle-induced endothelial dysfunction. Antioxid. Redox Signal 26 (1), 15–27. doi:10.1089/ars.2016.6771
San Cheang, W., Yuen Ngai, C., Yen Tam, Y., Yu Tian, X., Tak Wong, W., Zhang, Y., et al. (2015). Black tea protects against hypertension-associated endothelial dysfunction through alleviation of endoplasmic reticulum stress. Sci. Rep. 5, 10340. doi:10.1038/srep10340
Schröder, M., and Kaufman, R. J. (2005). ER stress and the unfolded protein response. Mutat. Research/Fundamental Mol. Mech. Mutagen. 569 (1-2), 29–63. doi:10.1016/j.mrfmmm.2004.06.056
Serafini, M., Peluso, I., and Raguzzini, A. (2010). Flavonoids as anti-inflammatory agents. Proc. Nutr. Soc. 69 (3), 273–278. doi:10.1017/S002966511000162X
Shen, M., Wang, L., Yang, G., Gao, L., Wang, B., Guo, X., et al. (2014). Baicalin protects the cardiomyocytes from ER stress-induced apoptosis: Inhibition of CHOP through induction of endothelial nitric oxide synthase. PLoS One 9 (2), e88389. doi:10.1371/journal.pone.0088389
Shi, Y. S., Li, C. B., Li, X. Y., Wu, J., Li, Y., Fu, X., et al. (2018). Fisetin attenuates metabolic dysfunction in mice challenged with a high-fructose diet. J. Agric. Food Chem. 66 (31), 8291–8298. doi:10.1021/acs.jafc.8b02140
Shu, Z., Yang, Y., Yang, L., Jiang, H., Yu, X., and Wang, Y. (2019). Cardioprotective effects of dihydroquercetin against ischemia reperfusion injury by inhibiting oxidative stress and endoplasmic reticulum stress-induced apoptosis via the PI3K/Akt pathway. Food & Funct. 10 (1), 203–215. doi:10.1039/c8fo01256c
Song, X. J., Yang, C. Y., Liu, B., Wei, Q., Korkor, M. T., Liu, J. Y., et al. (2011). Atorvastatin inhibits myocardial cell apoptosis in a rat model with post-myocardial infarction heart failure by downregulating ER stress response. Int. J. Med. Sci. 8 (7), 564–572. doi:10.7150/ijms.8.564
Subburaman, S., Ganesan, K., and Ramachandran, M. (2014). Protective role of naringenin against doxorubicin-induced cardiotoxicity in a rat model: Histopathology and mRNA expression profile studies. J. Environ. Pathology, Toxicol. Oncol. 33, 363–376. doi:10.1615/jenvironpatholtoxicoloncol.2014010625
Suchal, K., Malik, S., Gamad, N., Malhotra, R. K., Goyal, S. N., Chaudhary, U., et al. (2016). Corrigendum to "kaempferol attenuates myocardial ischemic injury via inhibition of MAPK signaling pathway in experimental model of myocardial ischemia-reperfusion injury" Oxid. Med. Cell Longev. 2016, 4530278. doi:10.1155/2020/4530278
Suchal, K., Malik, S., Khan, S. I., Malhotra, R. K., Goyal, S. N., Bhatia, J., et al. (2017). Molecular pathways involved in the amelioration of myocardial injury in diabetic rats by kaempferol. Int. J. Mol. Sci. 18 (5), 1001. doi:10.3390/ijms18051001
Sun, D., Li, C., Liu, J., Wang, Z., Liu, Y., Luo, C., et al. (2019). Expression profile of microRNAs in hypertrophic cardiomyopathy and effects of microRNA-20 in inducing cardiomyocyte hypertrophy through regulating gene MFN2. DNA Cell Biol. 38 (8), 796–807. doi:10.1089/dna.2019.4731
Sutton-Tyrrell, K., Bostom, A., Selhub, J., and Zeigler-Johnson, C. (1997). High homocysteine levels are independently related to isolated systolic hypertension in older adults. Circulation 96 (6), 1745–1749. doi:10.1161/01.cir.96.6.1745
Tabas, I. (2010). The role of endoplasmic reticulum stress in the progression of atherosclerosis. Circ. Res. 107 (7), 839–850. doi:10.1161/CIRCRESAHA.110.224766
Takahashi, M. (2014). NLRP3 inflammasome as a novel player in myocardial infarction. Int. Heart J. 55 (2), 101–105. doi:10.1536/ihj.13-388
Takahashi, S., Kato, Y., Adachi, M., Agata, N., Tanaka, H., and Shigenobu, K. (1995). Effects of cyclopiazonic acid on rat myocardium: Inhibition of calcium uptake into sarcoplasmic reticulum. J. Pharmacol. Exp. Ther. 272 (3), 1095–1100.
Tang, J-Y., Jin, P., He, Q., Lu, L-H., Ma, J-P., Gao, W-L., et al. (2017). Naringenin ameliorates hypoxia/reoxygenation-induced endoplasmic reticulum stress-mediated apoptosis in H9c2 myocardial cells: Involvement in ATF6, IRE1α and PERK signaling activation. Mol. Cell. Biochem. 424 (1), 111–122. doi:10.1007/s11010-016-2848-1
Tao, J., Zhu, W., Li, Y., Xin, P., Li, J., Liu, M., et al. (2011). Apelin-13 protects the heart against ischemia-reperfusion injury through inhibition of ER-dependent apoptotic pathways in a time-dependent fashion. Am. J. Physiol. Heart Circ. Physiol. 301 (4), H1471–H1486. doi:10.1152/ajpheart.00097.2011
Testai, L., Da Pozzo, E., Piano, I., Pistelli, L., Gargini, C., Breschi, M. C., et al. (2017). The citrus flavanone naringenin produces cardioprotective effects in hearts from 1 year old rat, through activation of mitoBK channels. Front. Pharmacol. 8, 71. doi:10.3389/fphar.2017.00071
Thorp, E., Li, G., Seimon, T. A., Kuriakose, G., Ron, D., and Tabas, I. (2009). Reduced apoptosis and plaque necrosis in advanced atherosclerotic lesions of Apoe-/- and Ldlr-/- mice lacking CHOP. Cell Metab. 9 (5), 474–481. doi:10.1016/j.cmet.2009.03.003
Torres-Villarreal, D., Camacho, A., Castro, H., Ortiz-Lopez, R., and de la Garza, A. L. (2019). Anti-obesity effects of kaempferol by inhibiting adipogenesis and increasing lipolysis in 3T3-L1 cells. J. Physiol. Biochem. 75 (1), 83–88. doi:10.1007/s13105-018-0659-4
Tuttolomondo, A., Di Raimondo, D., Pecoraro, R., Arnao, V., Pinto, A., and Licata, G. (2012). Atherosclerosis as an inflammatory disease. Curr. Pharm. Des. 18 (28), 4266–4288. doi:10.2174/138161212802481237
Valenzuela, V., Jackson, K. L., Sardi, S. P., and Hetz, C. (2018). Gene therapy strategies to restore ER proteostasis in disease. Mol. Ther. 26 (6), 1404–1413. doi:10.1016/j.ymthe.2018.04.004
Wang, J., Wen, Y., Lv, L. L., Liu, H., Tang, R. N., Ma, K. L., et al. (2015). Involvement of endoplasmic reticulum stress in angiotensin II-induced NLRP3 inflammasome activation in human renal proximal tubular cells in vitro. Acta Pharmacol. Sin. 36 (7), 821–830. doi:10.1038/aps.2015.21
Weng, L., Jia, S., Xu, C., Ye, J., Cao, Y., Liu, Y., et al. (2018). Nogo-C regulates post myocardial infarction fibrosis through the interaction with ER Ca(2+) leakage channel Sec61α in mouse hearts. Cell Death Dis. 9 (6), 612. doi:10.1038/s41419-018-0598-6
Wu, J., Xu, X., Li, Y., Kou, J., Huang, F., Liu, B., et al. (2014). Quercetin, luteolin and epigallocatechin gallate alleviate TXNIP and NLRP3-mediated inflammation and apoptosis with regulation of AMPK in endothelial cells. Eur. J. Pharmacol. 745, 59–68. doi:10.1016/j.ejphar.2014.09.046
Wu, L., Guo, T., Deng, R., Liu, L., and Yu, Y. (2021). Apigenin ameliorates insulin resistance and lipid accumulation by endoplasmic reticulum stress and SREBP-1c/SREBP-2 pathway in palmitate-induced HepG2 cells and high-fat diet–fed mice. J. Pharmacol. Exp. Ther. 377 (1), 146–156. doi:10.1124/jpet.120.000162
Wu, N., Zhang, X., Jia, P., and Jia, D. (2015). Hypercholesterolemia aggravates myocardial ischemia reperfusion injury via activating endoplasmic reticulum stress-mediated apoptosis. Exp. Mol. Pathol. 99 (3), 449–454. doi:10.1016/j.yexmp.2015.08.010
Wu, P., Meng, X., Zheng, H., Zeng, Q., Chen, T., Wang, W., et al. (2018). Kaempferol attenuates ROS-induced hemolysis and the molecular mechanism of its induction of apoptosis on bladder cancer Bladder Cancer. Mol. 23, 2592. doi:10.3390/molecules23102592
Wu, Y., Yue, Y., Fu, S., Li, Y., Wu, D., Lv, J., et al. (2019). Icariside II prevents hypertensive heart disease by alleviating endoplasmic reticulum stress via the PERK/ATF-4/CHOP signalling pathway in spontaneously hypertensive rats. J. Pharm. Pharmacol. 71 (3), 400–407. doi:10.1111/jphp.13041
Xiao, H. B., Lu, X. Y., Liu, Z. K., and Luo, Z. F. (2016). Kaempferol inhibits the production of ROS to modulate OPN-αvβ3 integrin pathway in HUVECs. J. Physiol. Biochem. 72 (2), 303–313. doi:10.1007/s13105-016-0479-3
Xin, W., Li, X., Lu, X., Niu, K., and Cai, J. (2011). Involvement of endoplasmic reticulum stress-associated apoptosis in a heart failure model induced by chronic myocardial ischemia. Int. J. Mol. Med. 27 (4), 503–509. doi:10.3892/ijmm.2011.612
Xin, W., Lu, X., Li, X., Niu, K., and Cai, J. (2011). Attenuation of endoplasmic reticulum stress-related myocardial apoptosis by SERCA2a gene delivery in ischemic heart disease. Mol. Med. 17 (3-4), 201–210. doi:10.2119/molmed.2010.00197
Xiong, F. Y., Tang, S. T., Su, H., Tang, H. Q., Jiang, P., Zhou, Q., et al. (2018). Melatonin ameliorates myocardial apoptosis by suppressing endoplasmic reticulum stress in rats with long-term diabetic cardiomyopathy. Mol. Med. Rep. 17 (1), 374–381. doi:10.3892/mmr.2017.7841
Xu, X., Lei, T., Li, W., and Ou, H. (2019). Enhanced cellular cholesterol efflux by naringenin is mediated through inhibiting endoplasmic reticulum stress-ATF6 activity in macrophages. Biochimica Biophysica Acta (BBA)-Molecular Cell Biol. Lipids 1864 (10), 1472–1482. doi:10.1016/j.bbalip.2019.06.005
Xuan, L. Y., Tao, X. X., Zhao, Y. J., Ge, H. Y., Bao, L. H., Wang, D. P., et al. (2016). [Effect of total flavonoids of astragalus on endoplasmic reticulum chaperone, calumenin and connecxin 43 in suckling mouse myocardium with myocarditis caused by coxsackievirus B3]. Zhongguo ying yong sheng li xue za zhi = Zhongguo yingyong shenglixue zazhi = Chin. J. Appl. physiology 32 (1), 51–54.
Yamazaki, H., Hiramatsu, N., Hayakawa, K., Tagawa, Y., Okamura, M., Ogata, R., et al. (2009). Activation of the Akt-NF-kappaB pathway by subtilase cytotoxin through the ATF6 branch of the unfolded protein response. J. Immunol. 183 (2), 1480–1487. doi:10.4049/jimmunol.0900017
Yang, J. W., and Hu, Z. P. (2015). Neuroprotective effects of atorvastatin against cerebral ischemia/reperfusion injury through the inhibition of endoplasmic reticulum stress. Neural Regen. Res. 10 (8), 1239–1244. doi:10.4103/1673-5374.162755
Yang, S., Wu, M., Li, X., Zhao, R., Zhao, Y., Liu, L., et al. (2020). Role of endoplasmic reticulum stress in atherosclerosis and its potential as a therapeutic target. Oxid. Med. Cell Longev. 2020, 9270107. doi:10.1155/2020/9270107
Yao, T., Ying, X., Zhao, Y., Yuan, A., He, Q., Tong, H., et al. (2015). Vitamin D receptor activation protects against myocardial reperfusion injury through inhibition of apoptosis and modulation of autophagy. Antioxid. Redox Signal 22 (8), 633–650. doi:10.1089/ars.2014.5887
Yousefzadeh, M. J., Zhu, Y., McGowan, S. J., Angelini, L., Fuhrmann-Stroissnigg, H., Xu, M., et al. (2018). Fisetin is a senotherapeutic that extends health and lifespan. EBioMedicine 36, 18–28. doi:10.1016/j.ebiom.2018.09.015
Yu, L., Li, B., Zhang, M., Jin, Z., Duan, W., Zhao, G., et al. (2016). Melatonin reduces PERK-eIF2α-ATF4-mediated endoplasmic reticulum stress during myocardial ischemia–reperfusion injury: Role of RISK and SAFE pathways interaction. Apoptosis 21 (7), 809–824. doi:10.1007/s10495-016-1246-1
Yu, L-M., Dong, X., Xue, X-D., Zhang, J., Li, Z., Wu, H-J., et al. (2019). Naringenin improves mitochondrial function and reduces cardiac damage following ischemia-reperfusion injury: The role of the AMPK-SIRT3 signaling pathway. Food & Funct. 10 (5), 2752–2765. doi:10.1039/c9fo00001a
Yu, L-M., Dong, X., Zhang, J., Li, Z., Xue, X-D., Wu, H-J., et al. (2019). Naringenin attenuates myocardial ischemia-reperfusion injury via cGMP-PKGIα signaling and in vivo and in vitro studies. Oxidative Med. Cell. Longev. 2019, 7670854. doi:10.1155/2019/7670854
Zhang, B., Jiang, H., Chen, J., Guo, X., Li, Y., Hu, Q., et al. (2019). Nobiletin ameliorates myocardial ischemia and reperfusion injury by attenuating endoplasmic reticulum stress-associated apoptosis through regulation of the PI3K/AKT signal pathway. Int. Immunopharmacol. 73, 98–107. doi:10.1016/j.intimp.2019.04.060
Zhang, C., Tang, Y., Li, Y., Xie, L., Zhuang, W., Liu, J., et al. (2017). Unfolded protein response plays a critical role in heart damage after myocardial ischemia/reperfusion in rats. PLoS One 12 (6), e0179042. doi:10.1371/journal.pone.0179042
Zhang, G. G., Cai, H. Q., Li, Y. H., Sui, Y. B., Zhang, J. S., Chang, J. R., et al. (2013). Ghrelin protects heart against ERS-induced injury and apoptosis by activating AMP-activated protein kinase. Peptides 48, 156–165. doi:10.1016/j.peptides.2013.08.015
Zhang, H-Y., Wang, Z., Lu, X-H., Kong, X-X., Wu, F-Z., Lin, L., et al. (2015). Endoplasmic reticulum stress: Relevance and therapeutics in central nervous system diseases. Mol. Neurobiol. 51 (3), 1343–1352. doi:10.1007/s12035-014-8813-7
Zhang, L., Li, D. C., and Liu, L. F. (2019). Paeonol: Pharmacological effects and mechanisms of action. Int. Immunopharmacol. 72, 413–421. doi:10.1016/j.intimp.2019.04.033
Zhang, N., Wei, W-Y., Yang, Z., Che, Y., Jin, Y-G., Liao, H-H., et al. (2017). Nobiletin, a polymethoxy flavonoid, protects against cardiac hypertrophy induced by pressure-overload via inhibition of NAPDH oxidases and endoplasmic reticulum stress. Cell. Physiology Biochem. 42 (4), 1313–1325. doi:10.1159/000478960
Zhang, Q., Li, H., Wang, S., Liu, M., Feng, Y., and Wang, X. (2013). Icariin protects rat cardiac H9c2 cells from apoptosis by inhibiting endoplasmic reticulum stress. Int. J. Mol. Sci. 14 (9), 17845–17860. doi:10.3390/ijms140917845
Zhou, M., Ren, H., Han, J., Wang, W., Zheng, Q., and Wang, D. (2015). Protective effects of kaempferol against myocardial ischemia/reperfusion injury in isolated rat heart via antioxidant activity and inhibition of glycogen synthase kinase-3β. Oxid. Med. Cell Longev. 2015, 481405. doi:10.1155/2015/481405
Zhou, X., Xin, Q., Wang, Y., Zhao, Y., Chai, H., Huang, X., et al. (2016). Total flavonoids of Astragalus plays a cardioprotective role in viral myocarditis. Acta Cardiol. Sin. 32 (1), 81–88. doi:10.6515/acs20150424h
Zhou, Y., and Wu, W. (2017). The sodium-glucose Co-transporter 2 inhibitor, empagliflozin, protects against diabetic cardiomyopathy by inhibition of the endoplasmic reticulum stress pathway. Cell Physiol. Biochem. 41 (6), 2503–2512. doi:10.1159/000475942
Zhou, Y., Zhao, L., Zhang, Z., and Lu, X. (2015). Protective effect of Enalapril against methionine-enriched diet-induced hypertension: Role of endoplasmic reticulum and oxidative stress. Biomed. Res. Int. 2015, 724876. doi:10.1155/2015/724876
Zhu, L., Jia, F., Wei, J., Yu, Y., Yu, T., Wang, Y., et al. (2017). Salidroside protects against homocysteine-induced injury in human umbilical vein endothelial cells via the regulation of endoplasmic reticulum stress. Cardiovasc Ther. 35 (1), 33–39. doi:10.1111/1755-5922.12234
Keywords: endoplasmic reticulum stress, flavonoids, quercetin, kaempferol, cardiovascular diseases
Citation: Keylani K, Arbab Mojeni F, Khalaji A, Rasouli A, Aminzade D, Karimi MA, Sanaye PM, Khajevand N, Nemayandeh N, Poudineh M, Azizabadi Farahani M, Esfandiari MA, Haghshoar S, Kheirandish A, Amouei E, Abdi A, Azizinezhad A, Khani A and Deravi N (2023) Endoplasmic reticulum as a target in cardiovascular diseases: Is there a role for flavonoids?. Front. Pharmacol. 13:1027633. doi: 10.3389/fphar.2022.1027633
Received: 21 September 2022; Accepted: 19 December 2022;
Published: 10 January 2023.
Edited by:
Francesco Visioli, University of Padua, ItalyReviewed by:
Ramoji Kosuru, Versiti Blood Research Institute, United StatesMithun Rudrapal, Rasiklal M. Dhariwal Institute of Pharmaceutical Education and Research, India
Copyright © 2023 Keylani, Arbab Mojeni, Khalaji, Rasouli, Aminzade, Karimi, Sanaye, Khajevand, Nemayandeh, Poudineh, Azizabadi Farahani, Esfandiari, Haghshoar, Kheirandish, Amouei, Abdi, Azizinezhad, Khani and Deravi. This is an open-access article distributed under the terms of the Creative Commons Attribution License (CC BY). The use, distribution or reproduction in other forums is permitted, provided the original author(s) and the copyright owner(s) are credited and that the original publication in this journal is cited, in accordance with accepted academic practice. No use, distribution or reproduction is permitted which does not comply with these terms.
*Correspondence: Niloofar Deravi, bmlsb29mYXJkZXJhdmlAc2JtdS5hYy5pcg==
†These authors have contributed equally to this work and share first authorship