- Department of General Surgery, The Second Affiliated Hospital of Nanchang University, Nanchang, Jiangxi, China
Background: Nano drug delivery system (NDDS) can significantly improve the delivery and efficacy of drugs against pancreatic cancer (PC) in many ways. The purpose of this study is to explore the related research fields of NDDS for PC from the perspective of bibliometrics.
Methods: Articles and reviews on NDDS for PC published between 2003 and 2022 were obtained from the Web of Science Core Collection. CiteSpace, VOSviewer, R-bibliometrix, and Microsoft Excel were comprehensively used for bibliometric and visual analysis.
Results: A total of 1329 papers on NDDS for PC were included. The number of papers showed an upward trend over the past 20 years. The United States contributed the most papers, followed by China, and India. Also, the United States had the highest number of total citations and H-index. The institution with the most papers was Chinese Acad Sci, which was also the most important in international institutional cooperation. Professors Couvreur P and Kazuoka K made great achievements in this field. JOURNAL OF CONTROLLED RELEASE published the most papers and was cited the most. The topics related to the tumor microenvironment such as “tumor microenvironment”, “tumor penetration”, “hypoxia”, “exosome”, and “autophagy”, PC treatment-related topics such as “immunotherapy”, “combination therapy”, “alternating magnetic field/magnetic hyperthermia”, and “ultrasound”, and gene therapy dominated by “siRNA” and “miRNA” were the research hotspots in the field of NDDS for PC.
Conclusion: This study systematically uncovered a holistic picture of the performance of NDDS for PC-related literature over the past 20 years. We provided scholars to understand key information in this field with the perspective of bibliometrics, which we believe may greatly facilitate future research in this field.
Introduction
As an extremely malignant digestive system tumor, pancreatic cancer (PC) has atypical clinical symptoms (Strobel et al., 2019), progresses rapidly, and lacks sensitive biomarkers for early diagnosis (Siegel et al., 2019), resulting in more than 80% of PC patients being in advanced stage at the initial diagnosis and missing the opportunity of surgical resection (Xie et al., 2018). At present, the efficacy of commonly used chemotherapy schemes, such as FOLFIRINOX and gemcitabine plus nab-paclitaxel, is still limited (Kielaite-Gulla et al., 2022). The main reasons that hinder these chemotherapy drugs from significantly improving the prognosis of the patients are the biological complexity and heterogeneity of PC (Fan et al., 2017), the poor specificity of chemotherapy drugs, uneven distribution in the body, and side effects on normal tissues and organs (Peng et al., 2017), and chemotherapy resistance of PC (Stott et al., 2022). The clinical application of immunotherapy also faces challenges: first, the human immune system is difficult to accurately distinguish tumor cells from normal cells, resulting in “targeted extra tumor” toxicity (Yilmaz et al., 2020); Secondly, the complex immunosuppressive microenvironment of solid tumors prevents intravenous infusion of immune cells or cytokines from reaching the tumor site (Lu et al., 2021).
Therefore, finding an ideal biocompatible targeted drug delivery system to overcome the above difficulties has become a research hotspot (Lammers et al., 2012). Nanomaterial has a small volume, large surface area (Rai et al., 2021), high permeability, can be effectively combined with a variety of biological materials to improve their biocompatibility, and can effectively control drug release (Chen et al., 2016). Therefore, a nano-drug delivery system (NDDS) can protect encapsulated drugs from blood circulation degradation, target drug delivery, reduce systemic toxicity, improve drug solubility, and improve drug pharmacokinetics and therapeutic efficacy (Kaushik et al., 2022). NDDS works best in areas where many limitations exist with molecular targeted therapy, and a need exists to push treatment boundaries for PC (Kokkinos et al., 2020).
Currently, bibliometrics has become a very important methodology for scholars to effectively identify the latest progress in a certain research field, predict research hotspots, and evaluate the development trend of this field. In recent years, bibliometrics has been applied to the research field related to nanomaterials (Huang et al., 2015; Zhu et al., 2021; Huang et al., 2022). However, there is still a lack of bibliometric analysis of the application of nanomaterials in oncology in the face of the research trend of oncology, materials science, pharmacology, and other disciplines gradually interpenetrating. As far as we know, there was no bibliometric analysis of NDDS for PC at present. In this study, based on the Web of Science Core Collection (WOSCC) database, we used CiteSpace, VOSviewer, and R-Bibliometrix to conduct bibliometric and visual analysis on the number of publications, citations, and research trends of countries/regions, institutions, authors, and keywords in NDDS for PC related literature, and sorted out the research hotspots and predicted the development trends in this field.
Methods
Data sources and search strategies
We comprehensively searched publications related to NDDS for PC in the WOSCC database from 2003 to 2022 (as of September 8).Reasonable use of wildcards makes retrieval strategies more organized and scientific (Cheng et al., 2022a; Cheng et al., 2022b). The retrieval strategy of this study was as follows: [TS=((Nanoparticle* OR Nanocrystalline Material* OR Nanocrystal* OR Nano Particle*) AND (Drug Delivery System* OR Drug Targeting* OR Drug Delivery))] AND [TS=((Pancreas OR Pancreatic) NEAR/1 (cancer* OR tumo$r* OR neoplasm* OR carcinoma* OR oncology))]. Only English language publications were included; the data category was limited to “article” and “review”. After excluding the publications that meet the language and article type requirements, further, we evaluated the title and abstract articles to determine whether the literature meets the theme of NDDs for PC. For the uncertain literature, the full text was downloaded and evaluated in more detail. Figure 1 showed the research flow chart.
Bibliometric analysis
CiteSpace (6.1.R3) was used to analyze the included literature, including co-citation analysis performed on countries/regions and institutions, dual-map overlay of citations, timeline view, co-cited references analysis, and references with the strongest citation burst.
VOSviewer (1.6.16) was used to visualize the co-citation network of authors and journals, and the co-occurrence of keywords. In the visual map, different nodes represented authors, journals, or keywords, etc; The node size indicated numbers or frequency; The thickness of the line represented the strength of the link; The colors of nodes represented different clusters or times.
R-Bibliometrix was used to analyze the theme evolution based on keywords over time, visualize the cooperation network between countries, and make a descriptive analysis of the publishing characteristics of journals. In addition, we used R software and R-Bibliometrix to generate the distribution map of high-frequency keywords over time.
Moreover, the scientometric online platform (https://bibliometric.com/) was used to conduct international cooperation networks between countries.
Results
Annual publications and trend
A total of 1329 publications regarding NDDS for PC were included. As shown in Figure 2, in general, since 2008, the annual number of publications has exceeded 10 and increased over time. The number of publications of NDDS for PC was the highest in 2021, although there was a slight decline in volatility in some years (2011 and 2018). 1329 publications had a total of 57,431 citations, with an average of 43.21 citations per paper, and an H-index of 102.
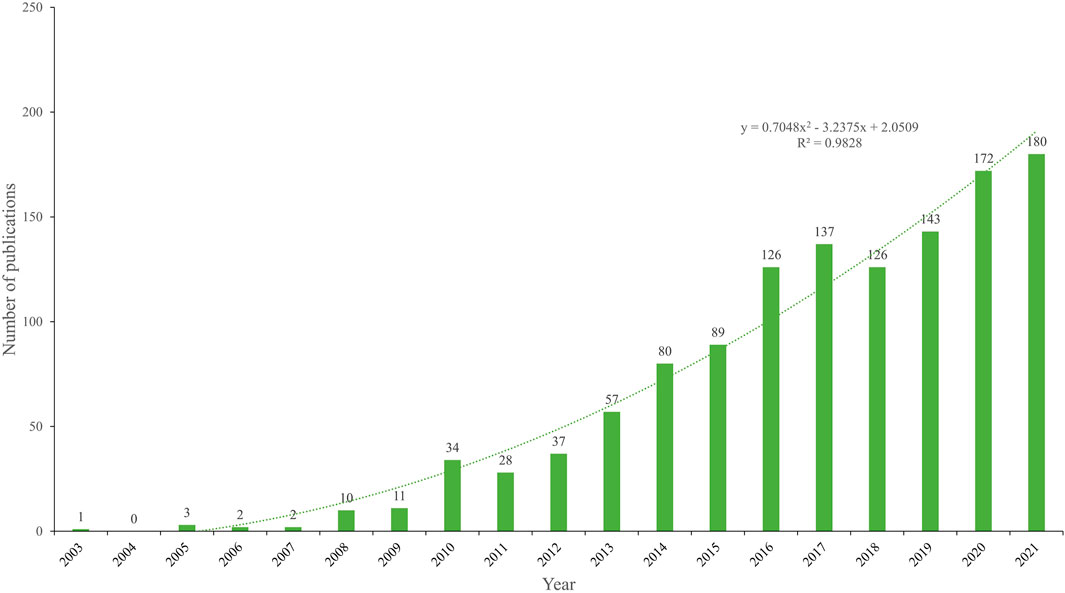
FIGURE 2. Number of annual publications regarding NDDS for PC from 2003 to 2021. Each bar shows the number of publications per year. The dotted line represents the curve fitting for this trend. Abbreviation: NDDS, Nano-drug Delivery System; PC, pancreatic cancer.
Countries/regions
A total of 71 countries/regions contributed to the publications included. The United States had the largest number of publications (n = 456, accounting for 34.312% of the total; 25,009 citations, with an average of 54.84 citations per paper, and an H-index of 79), followed by China (n = 446, accounting for 33.559%; 15,117 citations, with an average of 33.89 citations per paper, and an H-index of 62), and India (n = 108, accounting for 8.126%; 4,071 citations, with an average of 37.69 citations per paper, and an H-index of 37) (Figure 3A). Although the total number of publications in France ranked fourth, its total number of citations was second only to the United States and China, and its average number of citations was higher than that of the United States. Figure 3B summarized the annual output trend of the top 3 productive countries from 2003 to 2021. Figures 3C,D showed international cooperation among countries. Figure 3C was generated by CiteSpace: The thickness of the lines between countries showed the strength of cooperation. Among the top 20 countries with the most papers, the United States and China had the closest cooperation with other countries/regions; The United States, European countries (e.g., Italy and France), and Asian countries (e.g., China, India, and Saudi Arabia) were still the most important part of the national cooperation map, and extensive cooperative relations have been established between them, but the cooperation of other developing countries was still weak. In addition, cooperation in neighboring regions (e.g., the United States and Canada; China and Japan, South Korea), intra-continental cooperation (e.g., Germany and Spain, England, and Italy), and inter-continental cooperation (e.g., China and the United States) were also observed.
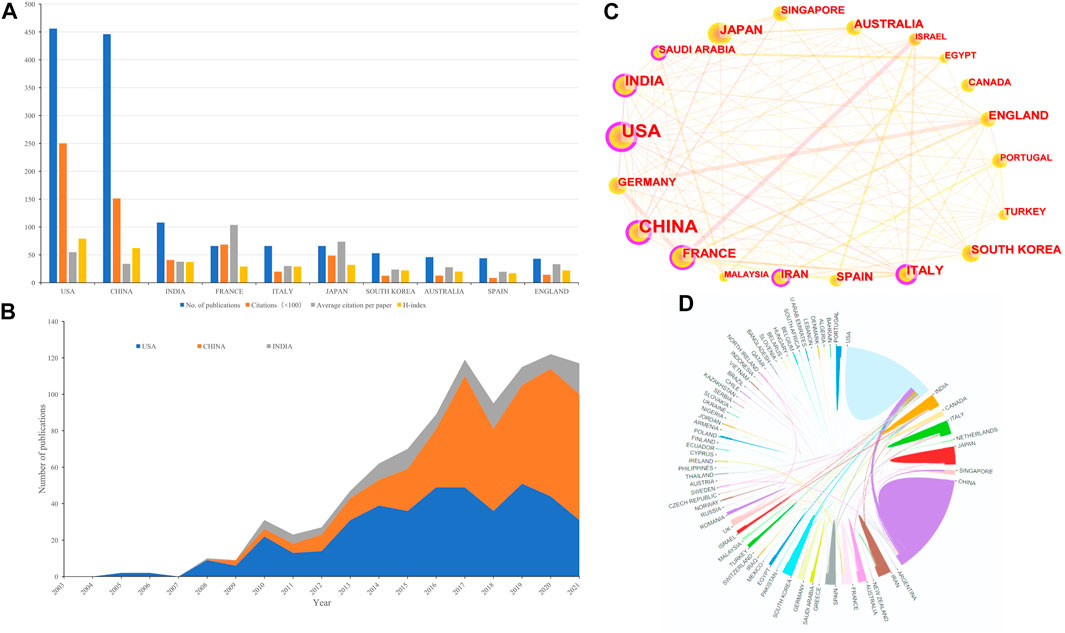
FIGURE 3. (A) The number of publications, total citations (×100), average citation per paper, and H-index of the 10 most productive countries/regions. (B) Annual output trend of the top 3 productive countries. (C) The country cooperation network generated by Citespace. Each node reprent a country, and the size of each node is proportional to the number of publications. The links between countries reflect co-occurrence relationships, while line thickness reflects the strength of cooperation. (D) The international cooperation networks between countries. Line thickness between countries reflects the intensity of the closeness.
Institutions
A total of 1,600 institutions worldwide contributed to the 1329 publications related to NDDS for PC. CiteSpace generated a cooperation network of institutions, as shown in Figure 4. The top 5 institutions with the most papers were Chinese Acad Sci, Fudan Univ, Zhejiang Univ, Shanghai Jiao Tong Univ, and Univ Chinese Acad Sci. The betweenness centrality (BC) value was an index to evaluate the importance of nodes in a collaborative network, and a BC value >0.1 was considered a vital node (Cheng et al., 2022b). Among the top 22 institutions with the most publications, institutions with BC values greater than 0.1 included Chinese Acad Sci (0.27), Harvard Med Sch (0.11), and Univ Sci and Technol China (0.11). Among these 22 institutions, China had 10 institutions, and the United States had 8 institutions (Table 1).
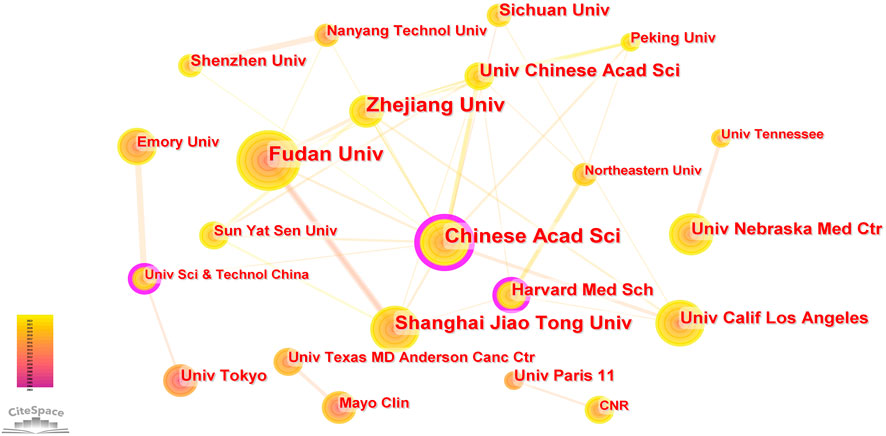
FIGURE 4. The cooperation network of institutions generated by Citespace. In the visualization map, one node represents an institution, and its size is proportional to the number of publications. The links between nodes represent the strength of cooperation.
Authors and co-cited authors
In terms of an author analysis, the total citations (×100), average citation per paper (×10), and H-index of the top five most prolific authors were shown in Figure 5A. Couvreur P contributed the most publications, followed by Kataoka K and Yong KT. The publications of Couvreur P had the highest total and average citations (4976 and 248.8, respectively), and the H-index is 14; The total and average citations of publications by Kataoka K ranked second (3784 and 189.2 respectively), with the highest H-index (n = 19); Although the number of publications by Meng H ranked fifth, the total and average number of citations of which ranked third (1918 and 137 respectively). Figure 5B showed the number of publications by the top five most prolific authors in different years and the total citations per year: It could be seen that the total citations of the six papers by Couvreur P in 2013 exceeded 1000 (n = 4,306), the total citations of two papers by Kataoka K in 2011 exceeded 1000 (n = 1,870), and one article by Meng H in 2009 was cited 687 times in total. Figure 5C showed the cluster density map of author co-authorship analysis. Only 158 authors with more than 5 papers were included, forming a total of 9 author clusters. By analyzing the co-citation network of authors, 85 authors who have been cited more than 45 times were defined as key researchers (Figure 5D): The connection represented the cooperation between authors, and the size of the circle represented the number of citations. Total link strength (TLS) indicated the impact of authors’ published papers on other authors involved in the studies. Meng H had the greatest TLS (n = 2134), followed by Von Hof DD (n = 1597), and Maeda H (n = 1582).
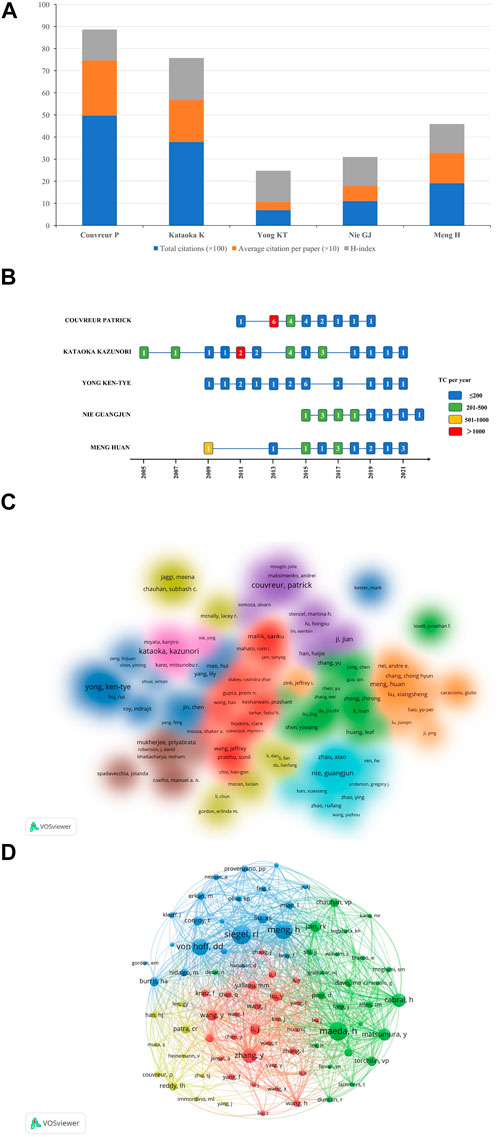
FIGURE 5. (A) The total citations (×100), average citation per paper (×10), and H-index of the top five most prolific authors. (B) The number of publications by the top five most prolific authors in different years and the total citations per year. Different color of each cell reflects different number of TC per year, and the number in the cell represents the number of publications each year. (C) Author co-authorship analysis by VOSviewer. One color reprents a cluster, and authors with close relationship are allocated to the same cluster. (D) Author co-citation analysis by VOSviewer. One node represents an author, and the lines between nodes represent the co-citation relationship. Abbreviation: TC, total citations.
Journals
329 journals published 1329 publications regarding NDDS for PC, and 66 journals published more than 5 publications. Among the top 16 journals in terms of the number of papers, JOURNAL OF CONTROLLED RELEASE ranked first (58 papers), followed by BIOMATERIALS and INTERNATIONAL JOURNAL OF NANOMEDICINE (52 and 44 papers, respectively) (Table 2; Figure 6A). Among these 16 journals, 5 were published in the Netherlands, 5 were published in England, and 3 in the United States. The papers of ACS NANO had the highest total number of citations (n = 4,110), followed by BIOMATERIALS (n = 3,294), and JOURNAL OF CONTROLLED RELEASE (n = 2,577). According to the latest JCR division in 2021, 13 journals were in JCR Q1 and 3 journals were in JCR Q2, and ACS NANO was the journal with the highest impact factor. In addition, we also conducted the map of annual occurrences of the top 16 journals, to more specifically understand the changing trend of the number of publications of these journals in different years (Figure 6B). As shown in Figure 6C, the network visualization diagram of journal co-citation analysis was created by VOSviewer. Only visually cite journals at least 300 times. Among the 59 journals that met the standard, the top 5 journals commonly cited were JOURNAL OF CONTROLLED RELEASE, BIOMATERIALS, ACS NANO, CANCER RESEARCH, and ADVANCED DRUG DELIVERY REVIEWS.
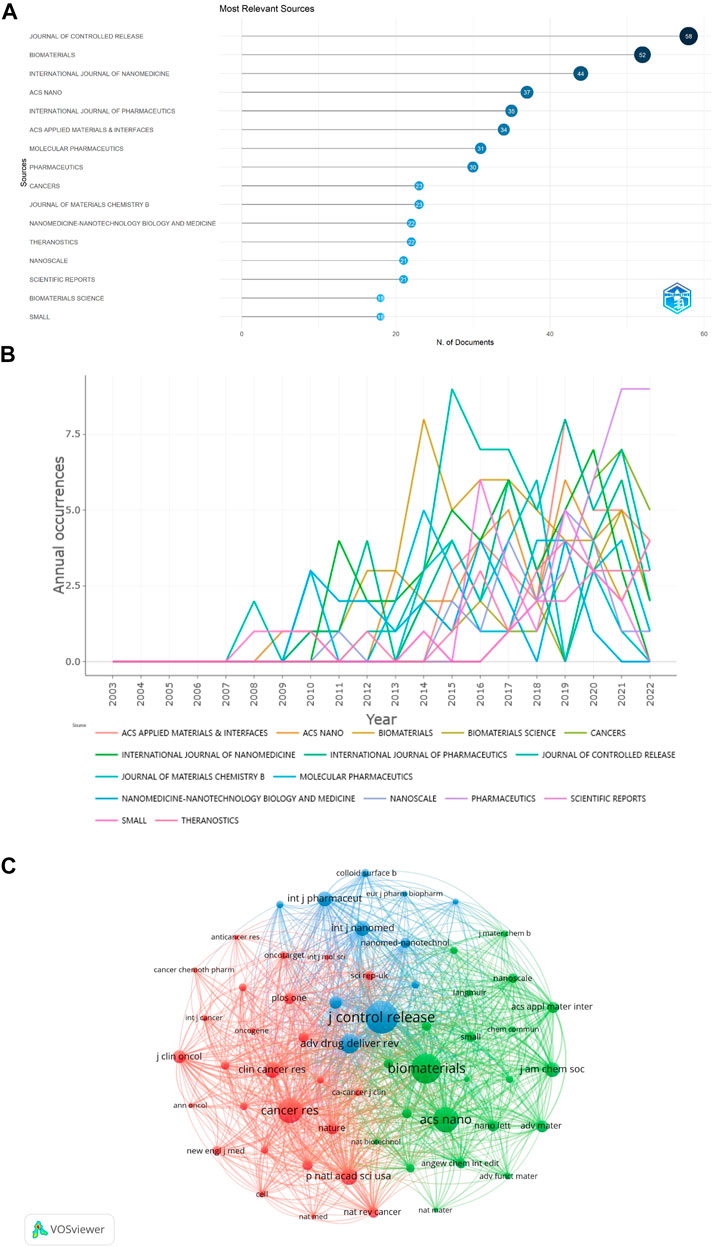
FIGURE 6. (A) The top 16 journals with the most publications generated by R-Bibliometrix. (B) Annual occurrences of the top 16 journals with the most publications generated by R-Bibliometrix. (C) The network visualization diagram of journal co-citation analysis generated by VOSviewer. One node represents one journal, and the area means the citation frequency. Sizes of the nodes are reflected with co-citations.
Dual-map overlays of nano drug delivery system for pancreatic cancer
The superposition of dual-map overlays revealed the overall scientific contribution. The left side was the citing journal, the right side was the cited journal, and the colored line path represented the citation relationship, indicating the citation trajectory and knowledge flow of knowledge (Chen and Leydesdorff, 2014). The result indicated that the citing papers regarding NDDS for PC were mainly focused on journals in the field of molecular, biology, immunology, and physics, materials, chemistry, whereas most of the cited articles were published in journals in the field of molecular, biology, genetics, and chemistry, materials, physics (Figure 7).
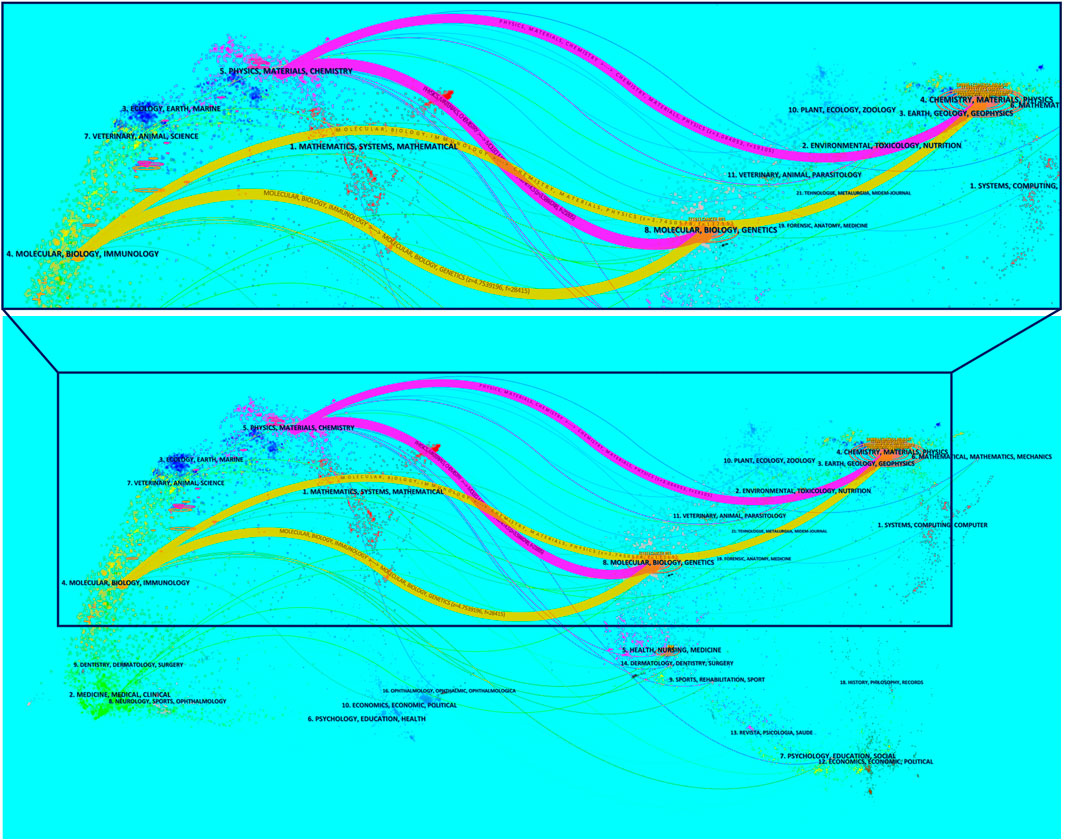
FIGURE 7. The dual-map overlay of journals contributed to publications regarding NDDS for PC from 2003 to 2022. The left side was the citing journal, the right side was the cited journal, and the colored line path represented the citation relationship. In order to display the local area more precisely, the local area is magnified (the area enclosed by the box). Abbreviation: NDDS, Nano-drug Delivery System; PC, pancreatic cancer.
Co-cited references and the strongest citation burst
Table 3 summarized the top 10 highly co-cited references of NDDS for PC research. The visualization network of co-cited references by CiteSpace were shown in Figure 8A. In the visualization network of co-cited references, all nodes representing the references were clustered into 17 specific clusters with the highest K values, including “#0 tumor penetration”, “#1 gemcitabine”, “#2 exosome”, and “#3 pancreatic stellate cells”, and so on. The timeline view could help us understand the evolution track of this field (Liu et al., 2021b). Visualized timeline for these 17 clusters was further performed (Figure 8B), and we found that “half generation PAMAM dendrimer” and “lipid polymer hybrid nanoparticle” were earlier fields on NDDS for PC. In addition, we also applied CiteSpace to identify the top 30 references with the strongest citation burst. References citation burst refers to references that have been widely cited by other studies over a period of time, which meant that they have received special attention in a certain period (Min et al., 2022). As shown in Figure 8C, since 2008, the strongest citation burst came from the paper of Von HOFFDD et al. (Von Hoff et al., 2013) in 2013, followed by the article of Bray F et al. on CA-CANCER J CLIN in 2018 (Bray et al., 2018), and the article of Siegel RL et al. on CA-CANCER J CLIN in 2015 (Siegel et al., 2015).
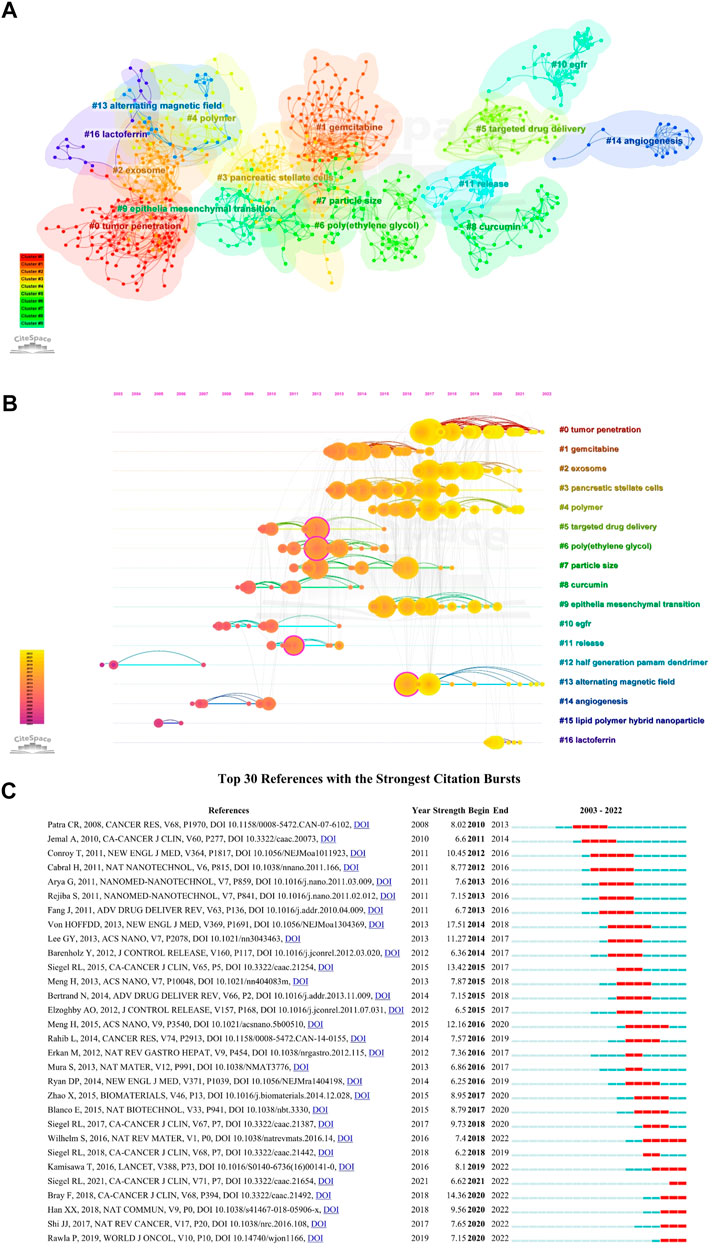
FIGURE 8. (A) The visualization network of co-cited references generated by Citespace. Each circle represents a reference, and circles with the same color represent a cluster with the same topic. (B) The timeline view map of references co-cited analysis generated by CiteSpace; This map implies the differences in the appearance time point of 17 clusters (2003–2022). The position of each circle on the horizontal axis indicates the time point of the first appearance, the size of the circle represents the total number of it was cited, and the circle on the same line represents a cluster with the same topic; The lines connecting the nodes represent co-cited relationships. (C) Top 30 references with the strongest citation bursts. The strength value represents the strength of citation bursts. The red bars indicate the durations of the bursts.
Keywords
Figures 9A,B show the network visualization of keywords generated by VOSviewer. Among 2713 keywords, the frequency of occurrence was set to at least 8, and finally, 82 keywords were included in the analysis. Figure 9B showed the overlay visualization of author keywords. Earlier keywords were displayed in blue, while orange represented the most recent keywords. For example, keywords such as “angiogenesis”, “EGFR”, and “controlled release” were the main topics in the early stage, and the keywords of “exosome”, “hypoxia”, “autophagy”, “tumor penetration”, and “immunotherapy” were hot topics in recent years. In addition, the distribution map of 35 high-frequency keywords over time was conducted by R software (Figure 10A), in which each cell represented the occurrence frequency of a keyword in a year, and the corresponding value was formed after standardizing these occurrences frequencies (0–1). The value of the black cell was the smallest, which represented the lowest occurrence frequency of the keyword this year, with the change of color, the value of the yellow cell was the biggest, and its corresponding keywords appeared the most frequently this year. For example, from 2002 to 2022 (as of September 8), “Magnetic resonance imaging” appeared more frequently in 2017, while in 2020 and 2021, the frequency decreased for two consecutive years.
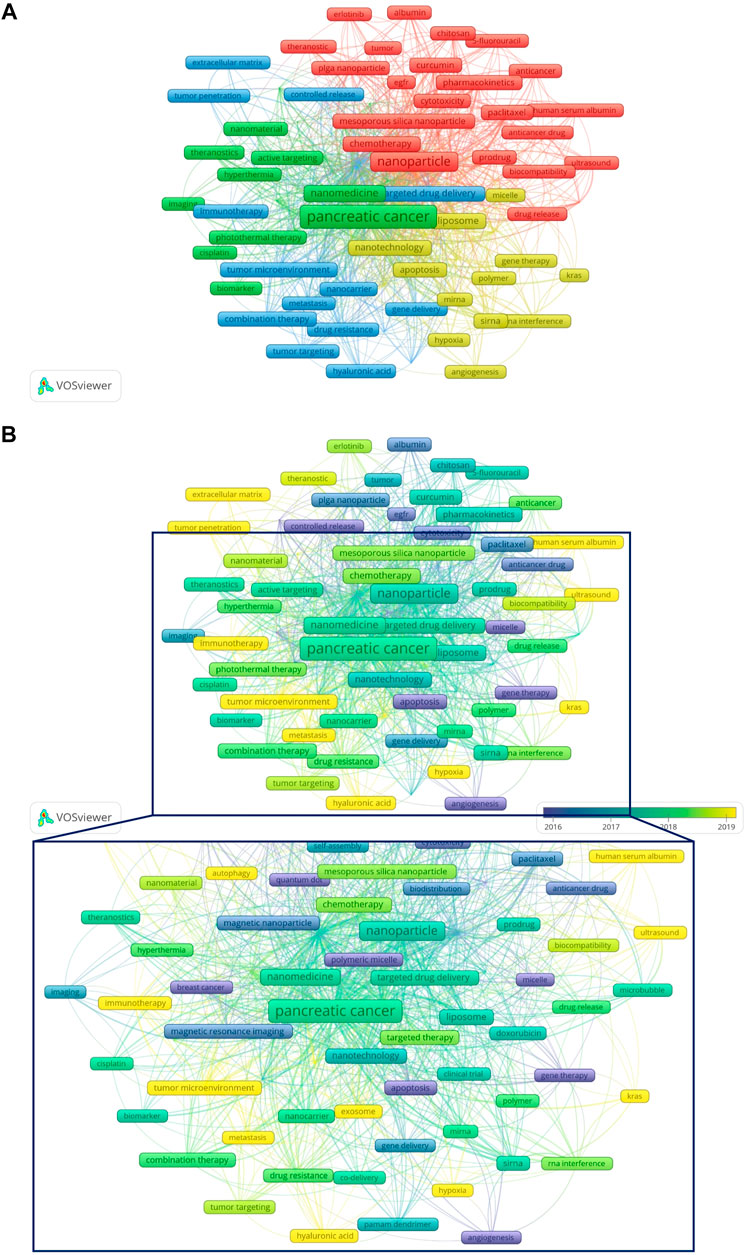
FIGURE 9. Keywords regarding NDDS for PC. (A) Network visualization of keywords generated by VOSviewer. (B) Overlay visualization of keywords generated by VOSviewer. Earlier keywords were displayed in blue, while orange represented the most recent keywords. In order to display the local area more precisely, the local area is magnified (the area enclosed by the box). Abbreviation: NDDS, Nano-drug Delivery System; PC, pancreatic cancer.
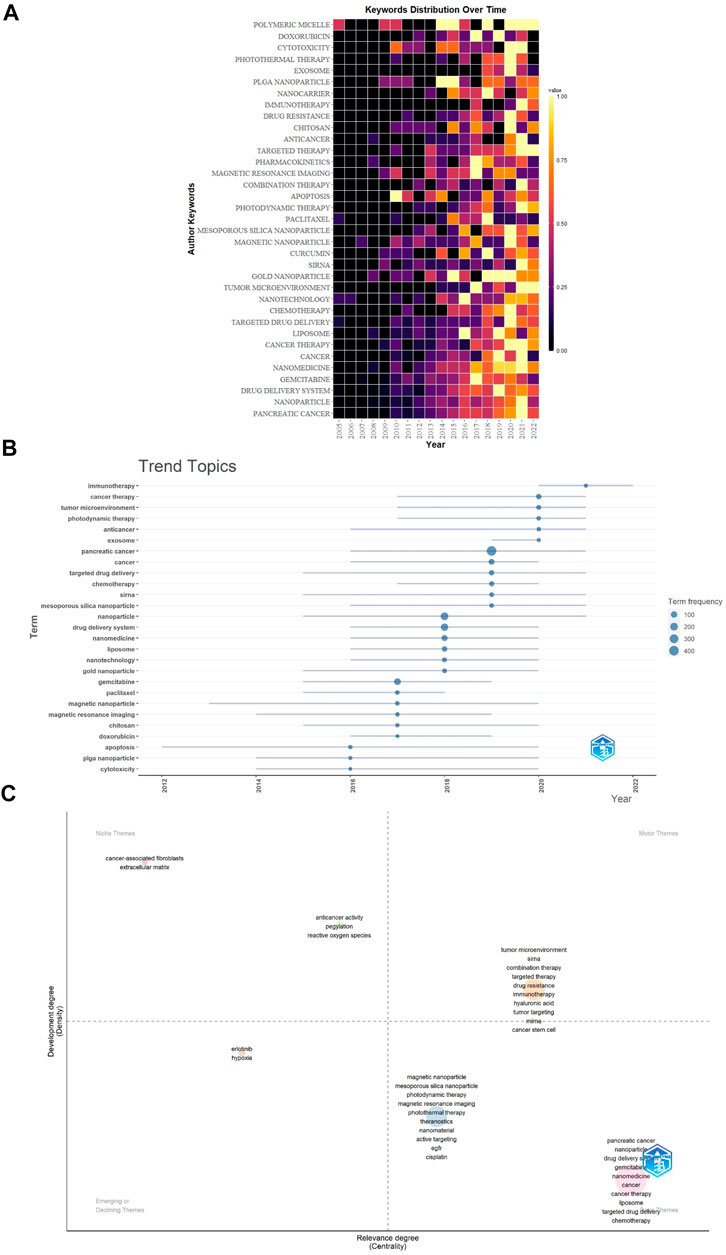
FIGURE 10. (A) The distribution map of 35 high-frequency keywords over time generated by R software. Each cell represented the occurrence frequency of a keyword in a year, and the corresponding value was formed after standardizing these occurrences frequencies (0–1). (B) Trend topics. The X-axis represents the year, while the Y-axis is the cumulate occurrences of the keywords. (C) The keywords thematic map generated by R-Bibliometrix. The X-axis represents the centrality indicating the importance of a theme; The Y-axis symbolizes the densityindicating the development of a theme.
The trend topic analysis was an important mapping tool that helped to portray the seed of trend integration rooted in the previous stream (Dai et al., 2022). The trend topics map was generated by R-Bibliometrix based on the occurrence frequency of author keywords and set word minimum frequency = 15 and the number of words per year = 6 (Figure 10B). The results showed that the duration of “apoptosis” was the longest (8 years), followed by “magnetic nanoparticle” (7 years). “Immunotherapy” began to appear in the field of NDDS for PC in 2020, and “exosome” began to appear in the field in 2019. “Immunotherapy” had the highest frequency in 2021, while “exosome”, “tumor microenvironment”, and “photodynamic therapy” had the highest frequency in 2020.
Finally, the keywords thematic map was generated by R-Bibliometrix (Figure 10C), and a total of 260 keywords were examined where a minimum cluster frequency was 6 and the number of labels for each cluster was 10. The upper right quadrant (motor theme), which was characterized by a high density and centrality, showed probably the well-developed and important themes for the structuring of the NDDS for PC research field. The cluster included “tumor microenvironment”, “siRNA”, “combination therapy”, “targeted therapy”, “drug resistance”, “immunotherapy”, “hyaluronic acid”, “tumor targeting”, “miRNA”, and “cancer stem cell”. The upper-left quadrant (niche theme) contained two clusters, cluster one included “cancer-associated fibroblasts” and “extracellular matrix”; cluster two included “anticancer activity”, “pegylation”, and “reactive oxygen species”. The cluster in the third quadrant (emerging or declining theme) was characterized by low centrality and density, which means that it was weakly developed and marginal, including “erlotinib” and “hypoxia” as the major themes. The fourth quadrant (basic themes) contained two clusters, cluster one included “pancreatic cancer”, “nanoparticle”, “drug delivery system”, “gemcitabine”, “nanomedicine”, “cancer”, “cancer therapy”, “liposome”, “targeted drug delivery”, and “chemotherapy”; cluster two included “magnetic nanoparticle”, “mesoporous silica nanoparticle”, “photodynamic therapy”, “magnetic resonance imaging”, “photothermal therapy”, “theranostics”, “nanomaterial”, “active targeting”, “EGFR”, and “cisplatin”. They concern general topics that were transversal to different research areas of the field.
Discussion
In this study, we used CiteSpace, VOSviewer, and R-Bibliometrix to conduct a bibliometric and visual analysis of 1329 publications related to NDDS for PC published between 2003 and 2022 (as of September 8), to sort out the research status of global publications related to this field, summarize research hotspots and predict future research trends.
The change in the number of academic publications is an important indicator of the development trend of a field (Cheng et al., 2022a). Over the past 20 years, publications related to NDDS for PC have shown a significant growth trend (Figure 2). From 2002 to 2007, the number of publications related to NDDS for PC was still small (no more than 10) and the trend was unstable; From 2008 to 2011, although there were more than 10 publications per year, the trend was still unstable, and the number of publications decreased slightly in 2011; Since 2012, the number of the publications increased steadily, and the annual number of publications has exceeded 100 since 2016, although there was a slight decline in 2018. 86.3% of the papers (n = 1,174) were published in the past 10 years (2012–2021). These results showed that in the past 10 years, the research on NDDS for PC has begun to develop rapidly.
The number of publications in a country is an important indicator of a country’s output. Figure 3A showed that the United States, four Asian countries (China, India, Japan, and South Korea), and European countries (France, Italy, Spain, and England) almost accounted for the top 10 contributions to NDDS for PC. The United States had the highest total number of citations and H-index. These results reflected that the United States had made great contributions and established its leading position in the field of NDDS for PC. In addition to the research topic of NDDS for PC, similar results were also obtained in the bibliometric analysis of other topics such as “pancreatic cancer” (Wang and Herr, 2022), “pancreatic stellate cells” (Yang et al., 2022b), and “inhalable nanosystems” (Huang et al., 2021). As for the cooperation among countries, it could be seen from Figure 3C that among the 20 countries with the most publications, the United States and China had the closest cooperation with other countries/regions; The United States, European countries (e.g., Italy and France), and Asian countries (e.g., China, India, and Saudi Arabia) were still the most important parts of the national cooperation landscape, and extensive cooperation had been established between them, but the cooperation of other developing countries was still relatively weak, and these countries needed to further cooperate to promote the development of NDDS for PC research field worldwide.
Among the top 22 institutions with the most publications, Chinese Acad SCI, Harvard Med Sch, and Univ Sci and Technol China had BC values greater than 0.1 (0.27, 0.11, and 0.11, respectively), indicating that these institutions were at the core of international cooperation in the field of NDDS for PC. It could be seen from Figure 4 that although some institutions had cooperation with other national institutions, such as Chinese Acad SCI’s cooperation with Univ Calif Los Angeles of the United States, it mostly cooperated with Zhejiang Univ, Fudan Univ, Shanghai Jiao Tong Univ, Sun Yat Sen Univ and other domestic institutions of China, which revealed a phenomenon, that was, in the field of NDDS for PC research, there was relatively little cooperation and exchange of results between institutions in different countries, and most of the cooperation institutions were limited to the domestic. Considering that nanomedicine was more and more widely used in oncology and other medical fields, and the research was gradually in-depth, this situation has greatly hindered the development of related research fields. Therefore, it is imperative to strengthen the cooperation between international institutions and jointly promote the development of research in this field.
Among the top 5 authors who published the most papers, Couvreur P of Paris-Saclay University contributed the most papers, with the highest total and average number of citations (4,976 and 248.8, respectively); Although the total and average number of citations of the publications by Kazuoka K ranked second (3,784 and 189.2, respectively), the H-index was the highest (n = 19); Although Meng H’s number of publications ranked fifth, the whose total and average number of citations ranked third (1,918 and 137, respectively). As could be seen from Figure 5B, among the top 5 authors, Kataoka K carried out research in this field first, starting in 2005, with the largest annual number of publications in 2014 (n = 4), and the highest total citations per year in 2011 (n = 1,870); Among the 2 articles published by Kataoka K in 2011, the total number of citations for one article was as high as 1,727, the authors compared the accumulation and effectiveness of different sizes of long-circulating, drug-loaded polymeric micelles (with diameters of 30, 50, 70 and 100 nm) in both highly and poorly permeable tumors. All the polymer micelles penetrated highly permeable tumors in mice, but only the 30 nm micelles could penetrate poorly permeable tumors to achieve an antitumor effect, and they found that the penetration and efficacy of the larger micelles could be enhanced by using a transforming growth factor-beta inhibitor to increase the permeability of PC (Cabral et al., 2011a). Meng H carried out research in this field first, starting in 2009, with the largest annual number of articles in 2017 and 2021 (n = 3), and the highest total citations per year in 2009 (n = 687): This highly cited paper showed that polyethyleneimine (PEI) polymers could enhance the cellular uptake of mesoporous silica nanoparticles (NPs) and improved the delivery of the hydrophobic anticancer drug paclitaxel to pancreatic cancer cells (Xia et al., 2009). Couvreur P started research in this field in 2011. The author’s annual output of articles (n = 6) and total citations per year (n = 4,306) reached the highest in 2013, and one of the six articles had high scientific influence in the field of NDDS for PC research: In the review (Mura et al., 2013), the authors discussed recent advances in the design of nanoscale stimuli-responsive systems that were able to control drug biodistribution in response to specific stimuli, either exogenous (variations in temperature, magnetic field, ultrasound intensity, light or electric pulses) or endogenous (changes in pH, enzyme concentration or redox gradients). However, from 2020 to 2021, Couvreur P did not publish any research on NDDS for PC. Kataoka K made contributions to nano polymer micelles, nano gene delivery targeting PC, and nano mediated sonodynamic therapy, etc, and Meng H mainly contributed to silicasome nanocarrier delivery system of chemotherapeutic drugs for PC, nano-enabled PC immunotherapy, and the targeting strategies for the carcinogenic molecular mechanism of PC, etc, while Couvreur P made contributions to squalene-based nanosystems for controlled drug release, and stimuli-responsive nanocarriers for drug delivery, etc.
As for the author co-citation analysis, 85 authors cited at least 45 times were included. As shown in Figure 5D, the top three authors with the largest TLS were Meng H, Von Hof DD, and Maeda H. At the same time, it was worth noting that although only 7 papers on NDDS for PC were published, Cabral H from Univ Tokyo still occupied an important position in the co-citation map, which might be related to several highly cited papers they have published, especially the article published on Nature Nanotechnology in 2011 contributed as the first author, which was cited 1,727 times (Cabral et al., 2011b). This result showed that the number of publications was not the only indicator of the author’s academic influence in this field.
Analyzing the characteristics of international peer-reviewed journals is helpful to understand the current trend, which is directly reflected in helping scholars understand the important journals related to the field of NDDS for PC, and select the most appropriate published journals for their research. In this study, the 16 journals with the highest productivity were all JCR Q1 or Q2 journals, among which JOURNAL OF CONTROLLED RELEASE, BIOMATERIALS, and INTERNATIONAL JOURNAL OF NANOMEDICINE were the three journals with the highest productivity in the field of NDDS for PC. It could be seen from Figure 6B that since 2017, the annual publications on NDDS for PC published by PHARMACEUTICS increased significantly, and PHARMACEUTICS published the most papers among the 16 journals in 2021 and 2022 (as of September 8), which showed that the attention of this journal to the related research of NDDS for PC was increasing or the attention of scholars of this field to PHARMACEUTICS was increasing. The top five journals commonly co-cited were JOURNAL OF CONTROLLED RELEASE, BIOMATERIALS, ACS NANO, CANCER RESEARCH, and ADVANCED DRUG DELIVERY REVIEWS. Therefore, the research results related to NDDS for PC published in these journals may be easier to be cited and receive more attention. Moreover, it is necessary to pay attention to the published papers in these journals to obtain the latest progress in the field of NDDS for PC.
Figure 8C listed the top 30 references with strong citation burst. Since 2008, the strongest citation burst came from the article of Von HOFFDD et al. (Von Hoff et al., 2013) in 2013, followed by the article of Bray F et al. on CA-CANCER J CLIN in 2018 (Bray et al., 2018), and the article of Siegel RL et al. on CA-CANCER J CLIN in 2015 (Siegel et al., 2015). Considering the strength and time of burst, four articles were worthy of attention: In addition to an article by Cabral et al. (2011c), an article by Meng H et al. has maintained a continuous burst from 2016 (Meng et al., 2015), they developed a mesoporous silica NPs vector for PC, which cooperatively delivered gemcitabine/paclitaxel combinations. Cell experiments found that it could inhibit the expression of cytidine deaminase and induce oxidative stress; In vivo experiments showed that the growth of PC was significantly inhibited and metastasis was eliminated. Another clinical trial investigated the efficacy of FOLFIRINOX in metastatic PC (Conroy et al., 2011). In addition, Wilhelm et al. analyzed the reasons for the poor efficiency of NPs delivered to solid tumors from the perspective of tumor biology and competing organs and proposed effective strategies to address this limitation (Wilhelm et al., 2016).
The references co-citation and keyword co-occurrence analysis can help to reveal the main research directions, hot spots, and evolution process in this field (Wang et al., 2021).
Figure 8B showed the trajectory of reference clusters over time. The results indicated that the current research hotspot has shifted to “#0 tumor penetration”, “#2 exosome”, “#4 polymer”, and “#13 alternating magnetic field”.
Figure 9B showed that the keywords “exosome”, “hypoxia”, “autophagy”, “tumor penetration”, “extracellular matrix”, “tumor microenvironment”, “immunotherapy”, “ultrasound”, and “hyaluronic acid”, etc. were hot topics in recent years. In addition, we also conducted a trend topic analysis based on author keywords (Figure 10B), and the results showed that “immunotherapy” began to appear in the field of NDDS for PC in 2020, and “exosome” began to appear in the field in 2019. “Immunotherapy” had the highest frequency in 2021, while “exosome”, “tumor microenvironment”, and “photodynamic therapy” had the highest frequency in 2020. The results based on the trend topic analysis showed that in 2020 and 2021, researchers gradually paid more attention to these keywords. We also used R software to conduct a distribution map of the top 35 high-frequency keywords over time (Figure 10A), and the results showed that the two core keywords “pancreatic cancer” and “nanoparticle” had the highest frequency in 2021, and “drug delivery system” had a higher frequency in 2021, which indicated that research related to NDDS for PC was still hot; Besides, “nanomedicine”, “cancer therapy”, “nanotechnology”, “tumor microenvironment”, “siRNA”, “photodynamic therapy”, “combination therapy”, “targeted therapy”, “immunotherapy”, “cytotoxicity”, and “polymetric micelle” also had the highest frequency in 2021, and the frequency of some of these keywords fluctuated significantly: For example, “tumor microenvironment” had a relatively higher frequency in 2017 and 2021, but the frequency was lower in 2018 and 2020. Similar phenomena also occurred in “polymetric micelle”. Different software and research methods have their advantages and can complement each other. In this study, we used VOSviewer, R software, and R-Bibliometrix to analyze keywords, using keyword co-occurrence network and overlay visualization maps, trend topics map, and distribution map of high-frequency keywords over time to display the topic evolution and hotspots of keywords as broadly and objectively as possible. Keywords’ thematic map can analyze topics that may have long-term development in the future. The keywords that appear in the motor themes are important and mature. Figure 10C showed that the motor theme contained one cluster, which included “tumor microenvironment”, “siRNA”, “combination therapy”, “targeted therapy”, “drug resistance”, “immunotherapy”, “hyaluronic acid”, “tumor targeting”, “miRNA”, and “cancer stem cell”.
The above results showed that the current research hotspots of PC NDDs mainly focused on the tumor microenvironment and its molecular mechanisms, as well as topics related to tumor penetration, such as “tumor microenvironment”, “extracellular matrix”, “tumor penetration”, “hypoxia”, “exosome”, and “autophagy”; Treatment related topics, such as “immunotherapy”, “combination therapy”, “alternating magnetic field/magnetic hyperthermia”, and “ultrasound”; And gene therapy dominated by “siRNA” and “miRNA”.
An important feature of PC is the extensive deposition of extracellular matrix (ECM) components in the tumor microenvironment (TME) and the activation of cancer-associated fibroblasts (CAFs). These changes can reduce vascular patency, lead to hypoxia (Jain et al., 2014), hinder the effective delivery of drugs (Jia et al., 2021), and change the anti-tumor immune response (Hosein et al., 2020). As key participants in TME, CAFs can promote ECM deposition by producing fibrotic compounds such as collagen, hyaluronic acid, and fibronectin (Tian et al., 2019), and also have complex crosstalk with cancer and immune cells (Kota et al., 2017). In addition, CAFs can secrete chemokines, cytokines, growth factors, microRNAs (miRNAs), and extracellular vesicles to communicate with cancer cells and other TME participants to promote tumor progression (Vennin et al., 2018). Therefore, blocking the activation and proliferation of CAFs or targeting them for drug delivery is a new therapeutic strategy for PC. Study shows that the absorption of NPs by CAFs is more than 10% higher than that of tumor cells (Alhussan et al., 2021), so drugs targeting CAFs can give full play to the advantages of NDDS. Although CAFs-targeting NPs can lead to significant inactivation of CAFs, and further reduce ECM production (Feng et al., 2020a), however, blocking the activation of CAFs alone may not be effective in killing PC. Therefore, the use of nanocarriers combined with drugs that block CAFs from producing matrix and chemotherapeutic drugs (such as paclitaxel, etc.) has become a direction worthy of in-depth research (Zhao et al., 2018; Yu et al., 2020; Zang et al., 2022). In addition, targeted therapy against the over-expressed enzymes on the membrane of CAFs can also inhibit the function of CAFs, which can effectively combine with NDDS and significantly enhance drug accumulation at tumor sites (Yu et al., 2020).
The low tumor penetration dominated by TME is the core factor hindering the clinical efficacy of NDDS, mainly because of the complex arrangement and distribution of blood vessels in tumors (Jain and Stylianopoulos, 2010; Matsumoto et al., 2016), which is mainly manifested in, on the one hand, the rapid diffusion of tumor cells leads to the lack of oxygen and nutrition, which leads to vascular abnormalities and heterogeneity (Chauhan et al., 2011), and ultimately inhibits the penetration of NPs (Jain, 2003); The uneven distribution of blood vessels from the periphery to the center of the tumor further hinders the deep penetration of NPs into the tumor (Munir, 2022). On the other hand, the ECM has very narrow pores, which hinders the delivery of NPs by electrostatic interactions and steric restriction (Stylianopoulos et al., 2010; Sriraman et al., 2014). Therefore, destroying tumor ECM and complex blood vessel distribution becomes one of the main methods to improve the penetration of NPs (Munir, 2022). However, forcefully destroying the matrix barrier and the blood vessels in the tumor may lead to the imbalance of signal transduction and dependence in TME, and uncontrolled PC growth and metastasis (Chen et al., 2022). Researches show that photothermal therapy (PTT) can promote NPs to penetrate deep in sites away from vasculature exposed to the near-infrared laser (He et al., 2015; Chen et al., 2017; Yu et al., 2017), and enhance the accumulation and efficacy of chemotherapy drugs in PC (Yu et al., 2020; Zhang et al., 2021). Combined ultrasound microbubble technology can also enhance the permeability of NPs and inhibit the growth of PC (Xing et al., 2016; Xu et al., 202b). In addition, the transcellular drug transport mediated by tumor penetrating peptide iRGD has been proved to enhance the effective penetration of NPs in PC tissues (Liu et al., 2017a; Liu et al., 2017b; Ruoslahti, 2017; Hurtado de Mendoza et al., 2021), which shows the potential of iRGD as a tumor-specific enhancer. However, due to the complexity of the biological barrier, the penetration depth of NPs still needs to be determined by in-depth research. The combination of photodynamic therapy (PDT) and PTT-mediated NPs and checkpoint blocking immunotherapy (Liu et al., 2019b; Sun et al., 2021; Qiu et al., 2022a; Xu et al., 2022a; Yun et al., 2022), as well as the combination of NPs and small interacting RNA (siRNA) targeted to checkpoints (Barshidi et al., 2022; Won et al., 2022), is expected to effectively activate the immune system, cause tumor degeneration, and get rid of the technical difficulties of tumor penetration.
However, due to the abnormal vascular network in TME and other reasons, the drugs delivered by traditional nano delivery systems show reduced biocompatibility, low permeability, retention effect, and high toxicity (Zhou et al., 2020). The targeted stimulus-responsive NDDS is a very promising solution, which shows high stability, biocompatibility, enhanced permeability, reduced toxicity, and retention effect, and can improve the efficacy of delivered drugs and significantly reduce side effects (Masood, 2016; Mahato, 2017; Narayanaswamy and Torchilin, 2019). Currently, a large number of studies on NDDS targeting different stimuli-responsive elements have been carried out, including temperature (Yang et al., 2018; Lu and Ten Hagen, 2020; Zhang et al., 2022b), magnetic field (Chen et al., 2019; Sun et al., 2020), light (Sun et al., 2019; Ge et al., 2021; Feng et al., 2022), pH (Li et al., 2016a; Qu et al., 2017; Latorre et al., 2019), ATP (Lai et al., 2015; Zhang et al., 2016), enzyme (Cai et al., 2020; Deng et al., 2022b), redox-potential (Raza et al., 2018; Kumar et al., 2019), and hypoxia (Kulkarni et al., 2018; Im et al., 2019; Zhen et al., 2019; Zhu et al., 2019). However, there are still few studies on PC-targeted stimuli-responsive NDDS. It can be predicted that, unlike other solid tumors, TME of PC is more specific and complex, which is a difficult problem to overcome in the further study of targeted stimuli-responsive NDDS.
Exosomes, saucer-shaped extracellular vesicles of approximately 30–100 nm diameter that are delimited by a lipid bilayer, contain a large amount of biologically active molecules, such as lipids, enzymes, metabolites, and various non-coding RNAs (miRNAs, long noncoding RNAs, and circular RNAs) (Yu et al., 2021), and play important roles in cell-cell communications in TME (Aguilar-Cazares et al., 2022; Ghosh and Ghosh, 2022). In addition, due to the excellent biosafety, low immunogenicity, carrier properties, nanoscale penetration effect, longer half-life, and no microvascular embolism (Ghosh et al., 2020), numerous studies have shown that as a carrier of conventional chemotherapy drugs (Zhao et al., 2021; Premnath et al., 2022), targeted therapy (Deng et al., 2021; Xu et al., 2021), or mediate photodynamic therapy and immunotherapy (Jang et al., 2021), exosomes have shown potential as a new NDDS for the treatment of PC. However, the isolation and preparation of a large number of engineered exosomes is still an important test for PC treatment, which requires the support of materials science, engineering, and other disciplines; In addition, when exosomes are coupled with NPs or encapsulated with drugs, the pharmacokinetics of exosomes in vivo is still not completely clear, and a large number of retention effects or drug off-target phenomena may occur, affecting the efficacy; In addition, a lot of work is needed to confirm the biosafety, targeted efficacy, and adverse reactions of exosomes before clinical use.
As an extremely complex bidirectional regulatory mechanism in the development of PC, autophagy can inhibit the transformation from precancerous lesions to PC in the initial stage, and also contribute to PC progression and chemotherapy resistance (Ma et al., 2021), which is closely related to TME. Nanomaterials have been designed to inhibit the progression of PC or induce the death of PC cells by autophagy or as autophagy inhibitor carriers (Raju et al., 2018; Li et al., 2019; Thomas et al., 2020; Chen et al., 2022). However, it is also noteworthy that autophagy has also been considered an important mechanism of nanomaterial-induced toxicity (Feng et al., 2020b). Studies have reported organ damage related to nanomaterial-driven autophagy, including hepatotoxicity (Li et al., 2015; Zhang et al., 2017; Zhu et al., 2017), pulmonary toxicity (Park et al., 2015; Jiang et al., 2018b), nephrotoxicity (Lin et al., 2016; Jiang et al., 2018a), neurotoxicity (Gao et al., 2015; Shang et al., 2021), cardiovascular toxicity (Guo et al., 2016; Zhang et al., 2019b), and these organ damage processes include nanomaterial mediated autophagy triggered mitochondrial damage (Yuan et al., 2017), lysosomal dysfunction (Wang et al., 2017), endoplasmic reticulum damage (Wei et al., 2017), cytoskeleton damage (Liu et al., 2019a), Golgi body injury (Huang et al., 2018), and DNA damage (Sadhu et al., 2018), etc. Therefore, the rational use of nanomaterials to mediate autophagy in the treatment of PC still needs further research, such as the bidirectional regulation mechanism of autophagy, avoiding organ damage, and so on.
Immune checkpoint inhibitors targeting PD-1/PD-L1 have shown good efficacy in many solid tumors (Zhang et al., 202a). However, most PCs are resistant to PD-(L)1 treatment (Feng et al., 2017), and immunosuppressive TME is the intrinsic core of PC treatment resistance (Amrutkar and Gladhaug, 2017; Karamitopoulou, 2020; Zhang et al., 2022a; Sally et al., 2022). The dense fibrous matrix and deficient vascular system in PC TME can hinder the entry of effector T cells (Sahin et al., 2017; Gong et al., 2018). Moreover, the unique PC TME results in a physical barrier preventing the infiltration of chimeric antigen receptor T (CAR-T) cells. TME immune cells secrete and express molecules that suppress T cell activation, limiting CAR-T cells’ antitumor response (Bailey et al., 2016). Therefore, it can be predicted that TME will be the main mechanism that hinders the efficacy of immunotherapy for PC for a long time. However, some NDDS has shown the potential to overcome the poor immunotherapeutic efficacy caused by TME: such as NDDS to promote pyroptosis (Xiao et al., 2021; Qiu et al., 2022b), intelligent stimulus response NDDS (Bu et al., 2021; Tan et al., 2021; Wang et al., 2022c; Mao et al., 2022), NDDS with high efficiency of transcytosis (Wang et al., 2022b; Wu et al., 2022); vaccines based on NDDS (Banerjee et al., 2019; Affandi et al., 2020; Liu et al., 2021a; Tang et al., 2022); NDDS of siRNAs targeted immune (Ghasemi-Chaleshtari et al., 2020; Deng et al., 2022a; Biber et al., 2022; Li et al., 2022; Yoo et al., 2022). The development of multifunctional NDDS with diverse and complementary functions is expected to enhance the efficacy of PC immunotherapy.
Magnetic hyperthermia (MH) refers to a new therapeutic method for cancer by converting electromagnetic energy into local heat by magnetic NPs via hysteresis or Néel/Brownian relaxation exposed to the alternating magnetic field (AMF) (Chiu-Lam and Rinaldi, 2016; Song et al., 2020). Compared with PTT, magnetic hyperthermia has little damage to normal tissues (Tamura et al., 2022). Moreover, magnetic NPs in AMF have been proved to be able to efficiently deliver chemotherapeutic drugs and other molecular drugs, and show remarkable efficacy against various tumors including PC (Wang et al., 2015; Sanhaji et al., 2019; Zuo et al., 2021; Demin et al., 2022; Wang et al., 2022d). However, for magnetic NPs used for drug delivery, it is necessary to optimize the size and shape to achieve safe blood circulation, prolong the circulation time, and reduce the release of unsafe degradation products into the blood circulation. It is worth mentioning that stealthy design has initially shown the potential to address the above limitations (Salmaso and Caliceti, 2013; Friedl et al., 2021). The magnetic coil used for delivering the magnetic field of magnetic NPs also has technical challenges and needs to solve the precise solutions of MH combined with chemotherapy, such as duration and frequency (Palzer et al., 2021).
A recent bibliometric study showed that the combination of NDDS and ultrasound microbubbles has become a hot spot to improve tumor efficacy (Wu et al., 2021). Ultrasound microbubbles can improve membrane permeability, making therapeutic drugs easy to pass through biological barriers such as vascular endothelium and cell membrane (Meng et al., 2021; Snipstad et al., 2021). Compared with higher-frequency ultrasound, low-frequency ultrasound microbubbles are more effective in promoting the accumulation of NPs in PC tissues (Lin et al., 2018). The latest research by Meng et al. reported that the optimized NPs with virus-mimic surface topology combined with low-frequency ultrasound microbubbles significantly enhanced the permeability of the biological barrier and enhanced the distribution of NPs in solid tumors (Meng et al., 2021). In recent years, the combination of NDDS and ultrasound microbubbles has shown great potential in improving gene transfection efficiency in gene therapy (Tay and Xu, 2017; Wang et al., 2022a; Kida et al., 2022), and improving the efficacy of chemotherapy drugs on a variety of tumors including PC (Gao et al., 2019; Lee et al., 2020; Liu et al., 2020; Yang et al., 2022a; Ngamcherdtrakul et al., 2022; Schoen et al., 2022). However, it should be noted that the size and concentration of microbubbles and the total amount of gas contained in the liquid are important factors affecting NDDS mediated by ultrasound microbubbles (Kida et al., 2022).
In addition to the use of chemotherapy drugs and immunotherapy, drugs such as siRNA and miRNA targeting different targets are also important cancer therapies. Various methods have been proven to be able to achieve targeted inhibition of specific miRNAs (Sun et al., 2015; Liang et al., 2016; Van Roosbroeck et al., 2017; De Cola et al., 2018). However, susceptibility to degradation by nucleases, low intracellular absorption rate due to an inherent negative charge and hydrophilic structure, and potential off-target effects limit the application of miRNAs (Kara et al., 2022). MiRNA nanocarriers based on polymers, inorganic materials, and lipids have demonstrated their potential in the treatment of PC (Arora et al., 2014; Gurbuz and Ozpolat, 2019; Gokita et al., 2020; Wu et al., 2020; Borchardt et al., 2022). However, it is important to note that therapeutic miRNAs can also accumulate in healthy tissues due to interstitial fluid pressure and shearing stress promoting NPs extravasation, which may lead to their degradation (Zhang et al., 2019a), and cause toxic and other adverse reactions (Kara et al., 2022). Effective delivery and cell uptake of siRNA are major challenges in therapeutic applications (Wang et al., 2016; Arnold et al., 2017; Tan et al., 2022). PEGylated ligand-targeted liposomes or micelles avoid nonspecific clearance of siRNA by the reticuloendothelial system and protect siRNA from the degradation of nuclease (Charbe et al., 2020), which makes it possible to address these major obstacles. Although several studies have shown that the NDDS can effectively deliver siRNA and synergize with chemotherapeutic drugs to inhibit the growth of PC and the expression of related genes (Li et al., 2016b; Strand et al., 2019; Wang et al., 2020; Jung et al., 2021; Luo et al., 2021), however, the biosafety and biodegradability of siRNA delivery vehicles, the targeting specificity and endosomal escape capability of nanocarriers to tumor tissues, toxicity and immune stimulation need to be addressed by further studies (Aghamiri et al., 2021; Huang and Xiao, 2022).
Dual-map overlays showed that NDDS for PC research mainly focuses on molecular, biology, immunology, and physics, materials, chemistry (Figure 7). Fortunately, these researches related to NDDS for PC has reflected the effective penetration of multiple disciplines and the result of deepening cooperation, which is also the development trend of various disciplines at present, especially in nanomaterials and medicine.
Limitations
There were several limitations. First, this study only included the publications from 2003 to 2022 (as of September 8), and it was possible to miss some important and landmark studies before 2002; Secondly, because the literature of 2022 was incomplete and some unpublished hot spots may not be included, and some results of this study may change after being included in the data for the whole year of 2022, so what is necessary to carry out follow-up research to evaluate the results more objectively; Finally, although we used various software to analyze the content of countries, institutions, authors, keywords, and references, the analysis still could not provide a comprehensive overview of NDDS for PC-related literature. However, we believe that the current literature-based bibliometric studies may, to the greatest extent, allow scholars to understand the research hotspots and development trends of NDDS for PC.
Conclusion
In the past 20 years, the number of publications related to NDDS for PC has increased, indicating that the interest of scholars in this field is increasing. The United States is absolutely in the leading position in the field of NDDS for PC research. In terms of publication volume and global institutional cooperation, Chinese Acad SCI has made the greatest contribution in this field. Professors Couvreur P and Kazuoka K made great achievements in this field. JOURNAL OF CONTROLLED RELEASE is at the core of the publishing of NDDS for PC research. The topics related to the TME such as “tumor microenvironment”, “tumor penetration”, “hypoxia”, “exosome”, and “autophagy”, PC treatment-related topics such as “immunotherapy”, “combination therapy”, “alternating magnetic field/magnetic hyperthermia”, and “ultrasound”, and gene therapy dominated by “siRNA” and “miRNA” are the research hotspots and trends in the field of NDDS for PC.
Data availability statement
The original contributions presented in the study are included in the article/Supplementary Material, further inquiries can be directed to the corresponding author.
Author contributions
J-FZa and R-FY contributed to the study conception design. J-FZa, J-FZu, F-LZ, CH, and F-QB contributed to the acquisition, analysis, and interpretation of data. Manuscript draft and revision: all authors. All authors read and approved the final manuscript.
Funding
The study was supported by the National Natural Science Foundation of China (Grant No.: 81960436), Project of the Jiangxi Provincial Department of Science and Technology (Grant No.: 20202BBGL73037), and Double Thousand Talents Project of Jiangxi Province (Grant No.: jxsq2019201100).
Conflict of interest
The authors declare that the research was conducted in the absence of any commercial or financial relationships that could be construed as a potential conflict of interest.
Publisher’s note
All claims expressed in this article are solely those of the authors and do not necessarily represent those of their affiliated organizations, or those of the publisher, the editors and the reviewers. Any product that may be evaluated in this article, or claim that may be made by its manufacturer, is not guaranteed or endorsed by the publisher.
References
Affandi, A. J., Grabowska, J., Olesek, K., Lopez Venegas, M., Barbaria, A., Rodríguez, E., et al. (2020). Selective tumor antigen vaccine delivery to human CD169(+) antigen-presenting cells using ganglioside-liposomes. Proc. Natl. Acad. Sci. U. S. A. 117 (44), 27528–27539. doi:10.1073/pnas.2006186117
Aghamiri, S., Raee, P., Talaei, S., Mohammadi-Yeganeh, S., Bayat, S., Rezaee, D., et al. (2021). Nonviral siRNA delivery systems for pancreatic cancer therapy. Biotechnol. Bioeng. 118 (10), 3669–3690. doi:10.1002/bit.27869
Aguilar-Cazares, D., Chavez-Dominguez, R., Marroquin-Muciño, M., Perez-Medina, M., Benito-Lopez, J. J., Camarena, A., et al. (2022). The systemic-level repercussions of cancer-associated inflammation mediators produced in the tumor microenvironment. Front. Endocrinol. 13, 929572. doi:10.3389/fendo.2022.929572
Alhussan, A., Bromma, K., Bozdogan, E. P. D., Metcalfe, A., Karasinska, J., Beckham, W., et al. (2021). Investigation of nano-bio interactions within a pancreatic tumor microenvironment for the advancement of nanomedicine in cancer treatment. Curr. Oncol. 28 (3), 1962–1979. doi:10.3390/curroncol28030183
Amrutkar, M., and Gladhaug, I. P. (2017). Pancreatic cancer chemoresistance to gemcitabine. Cancers (Basel) 9 (11), E157. doi:10.3390/cancers9110157
Arnold, A. E., Czupiel, P., and Shoichet, M. (2017). Engineered polymeric nanoparticles to guide the cellular internalization and trafficking of small interfering ribonucleic acids. J. Control. Release 259, 3–15. doi:10.1016/j.jconrel.2017.02.019
Arora, S., Swaminathan, S. K., Kirtane, A., Srivastava, S. K., Bhardwaj, A., Singh, S., et al. (2014). Synthesis, characterization, and evaluation of poly (D, L-lactide-co-glycolide)-based nanoformulation of miRNA-150: Potential implications for pancreatic cancer therapy. Int. J. Nanomedicine 9, 2933–2942. doi:10.2147/ijn.S61949
Bailey, P., Chang, D. K., Forget, M. A., Lucas, F. A., Alvarez, H. A., Haymaker, C., et al. (2016). Exploiting the neoantigen landscape for immunotherapy of pancreatic ductal adenocarcinoma. Sci. Rep. 6, 35848. doi:10.1038/srep35848
Banerjee, K., Gautam, S. K., Kshirsagar, P., Ross, K. A., Spagnol, G., Sorgen, P., et al. (2019). Amphiphilic polyanhydride-based recombinant MUC4β-nanovaccine activates dendritic cells. Genes. Cancer 10 (3-4), 52–62. doi:10.18632/genesandcancer.189
Barshidi, A., Karpisheh, V., Noukabadi, F. K., Kiani, F. K., Mohammadi, M., Afsharimanesh, N., et al. (2022). Dual blockade of PD-1 and LAG3 immune checkpoints increases dendritic cell vaccine mediated T cell responses in breast cancer model. Pharm. Res. 39 (8), 1851–1866. doi:10.1007/s11095-022-03297-9
Biber, G., Sabag, B., Raiff, A., Ben-Shmuel, A., Puthenveetil, A., Benichou, J. I. C., et al. (2022). Modulation of intrinsic inhibitory checkpoints using nano-carriers to unleash NK cell activity. EMBO Mol. Med. 14 (1), e14073. doi:10.15252/emmm.202114073
Borchardt, H., Kogel, A., Kalwa, H., Weirauch, U., and Aigner, A. (2022). Therapeutic miR-506-3p replacement in pancreatic carcinoma leads to multiple effects including autophagy, apoptosis, senescence, and mitochondrial alterations in vitro and in vivo. Biomedicines 10 (7), 1692. doi:10.3390/biomedicines10071692
Bray, F., Ferlay, J., Soerjomataram, I., Siegel, R. L., Torre, L. A., and Jemal, A. (2018). Global cancer statistics 2018: GLOBOCAN estimates of incidence and mortality worldwide for 36 cancers in 185 countries. Ca. Cancer J. Clin. 68 (6), 394–424. doi:10.3322/caac.21492
Bu, L. L., Wang, H. Q., Pan, Y., Chen, L., Wu, H., Wu, X., et al. (2021). Gelatinase-sensitive nanoparticles loaded with photosensitizer and STAT3 inhibitor for cancer photothermal therapy and immunotherapy. J. Nanobiotechnology 19 (1), 379. doi:10.1186/s12951-021-01125-7
Cabral, H., Matsumoto, Y., Mizuno, K., Chen, Q., Murakami, M., Kimura, M., et al. (2011a). Accumulation of sub-100 nm polymeric micelles in poorly permeable tumours depends on size. Nat. Nanotechnol. 6 (12), 815–823. doi:10.1038/NNANO.2011.166
Cabral, H., Matsumoto, Y., Mizuno, K., Chen, Q., Murakami, M., Kimura, M., et al. (2011b). Accumulation of sub-100 nm polymeric micelles in poorly permeable tumours depends on size. Nat. Nanotechnol. 6 (12), 815–823. doi:10.1038/nnano.2011.166
Cabral, H., Matsumoto, Y., Mizuno, K., Chen, Q., Murakami, M., Kimura, M., et al. (2011c). Accumulation of sub-100 nm polymeric micelles in poorly permeable tumours depends on size. Nat. Nanotechnol. 6 (12), 815–823. doi:10.1038/nnano.2011.166
Cai, D., Han, C., Liu, C., Ma, X., Qian, J., Zhou, J., et al. (2020). Chitosan-capped enzyme-responsive hollow mesoporous silica nanoplatforms for colon-specific drug delivery. Nanoscale Res. Lett. 15 (1), 123. doi:10.1186/s11671-020-03351-8
Charbe, N. B., Amnerkar, N. D., Ramesh, B., Tambuwala, M. M., Bakshi, H. A., Aljabali, A. A. A., et al. (2020). Small interfering RNA for cancer treatment: Overcoming hurdles in delivery. Acta Pharm. Sin. B 10 (11), 2075–2109. doi:10.1016/j.apsb.2020.10.005
Chauhan, V. P., Stylianopoulos, T., Boucher, Y., and Jain, R. K. (2011). Delivery of molecular and nanoscale medicine to tumors: Transport barriers and strategies. Annu. Rev. Chem. Biomol. Eng. 2, 281–298. doi:10.1146/annurev-chembioeng-061010-114300
Chen, C., and Leydesdorff, L. (2014). Patterns of connections and movements in dual-map overlays: A new method of publication portfolio analysis. J. Assoc. Inf. Sci. Technol. 65 (2), 334–351. doi:10.1002/asi.22968
Chen, C., Wang, S., Li, L., Wang, P., Chen, C., Sun, Z., et al. (2016). Bacterial magnetic nanoparticles for photothermal therapy of cancer under the guidance of MRI. Biomaterials 104, 352–360. doi:10.1016/j.biomaterials.2016.07.030
Chen, H., Guo, Q., Chu, Y., Li, C., Zhang, Y., Liu, P., et al. (2022). Smart hypoxia-responsive transformable and charge-reversible nanoparticles for the deep penetration and tumor microenvironment modulation of pancreatic cancer. Biomaterials 287, 121599. doi:10.1016/j.biomaterials.2022.121599
Chen, W., Cheng, C. A., and Zink, J. I. (2019). Spatial, temporal, and dose control of drug delivery using noninvasive magnetic stimulation. ACS Nano 13 (2), 1292–1308. doi:10.1021/acsnano.8b06655
Chen, Y., Li, H., Deng, Y., Sun, H., Ke, X., and Ci, T. (2017). Near-infrared light triggered drug delivery system for higher efficacy of combined chemo-photothermal treatment. Acta Biomater. 51, 374–392. doi:10.1016/j.actbio.2016.12.004
Cheng, K., Guo, Q., Shen, Z., Yang, W., Wang, Y., Sun, Z., et al. (2022a). Bibliometric analysis of global research on cancer photodynamic therapy: Focus on nano-related research. Front. Pharmacol. 13, 927219. doi:10.3389/fphar.2022.927219
Cheng, K., Guo, Q., Yang, W., Wang, Y., Sun, Z., and Wu, H. (2022b). Mapping knowledge landscapes and emerging trends of the links between bone metabolism and diabetes mellitus: A bibliometric analysis from 2000 to 2021. Front. Public Health 10, 918483. doi:10.3389/fpubh.2022.918483
Chiu-Lam, A., and Rinaldi, C. (2016). Nanoscale thermal phenomena in the vicinity of magnetic nanoparticles in alternating magnetic fields. Adv. Funct. Mat. 26 (22), 3933–3941. doi:10.1002/adfm.201505256
Conroy, T., Desseigne, F., Ychou, M., Bouché, O., Guimbaud, R., Bécouarn, Y., et al. (2011). FOLFIRINOX versus gemcitabine for metastatic pancreatic cancer. N. Engl. J. Med. 364 (19), 1817–1825. doi:10.1056/NEJMoa1011923
Dai, Z., Xu, S., Wu, X., Hu, R., Li, H., He, H., et al. (2022). Knowledge mapping of multicriteria decision analysis in healthcare: A bibliometric analysis. Front. Public Health 10, 895552. doi:10.3389/fpubh.2022.895552
De Cola, A., Lamolinara, A., Lanuti, P., Rossi, C., Iezzi, M., Marchisio, M., et al. (2018). MiR-205-5p inhibition by locked nucleic acids impairs metastatic potential of breast cancer cells. Cell. Death Dis. 9 (8), 821. doi:10.1038/s41419-018-0854-9
Demin, A. M., Vakhrushev, A. V., Pershina, A. G., Valova, M. S., Efimova, L. V., Syomchina, A. A., et al. (2022). Magnetic-responsive doxorubicin-containing materials based on Fe(3)O(4) nanoparticles with a SiO(2)/PEG shell and study of their effects on cancer cell lines. Int. J. Mol. Sci. 23 (16), 9093. doi:10.3390/ijms23169093
Deng, K., Yang, D., and Zhou, Y. (2022a). Nanotechnology-based siRNA delivery systems to overcome tumor immune evasion in cancer immunotherapy. Pharmaceutics 14 (7), 1344. doi:10.3390/pharmaceutics14071344
Deng, L., Zhang, H., Zhang, Y., Luo, S., Du, Z., Lin, Q., et al. (2021). An exosome-mimicking membrane hybrid nanoplatform for targeted treatment toward Kras-mutant pancreatic carcinoma. Biomater. Sci. 9 (16), 5599–5611. doi:10.1039/d1bm00446h
Deng, Y., Tan, Y., Zhang, Y., Zhang, L., Zhang, C., Ke, Y., et al. (2022b). Design of uracil-modified DNA nanotubes for targeted drug release via DNA-modifying enzyme reactions. ACS Appl. Mat. Interfaces 14, 34470–34479. doi:10.1021/acsami.2c09488
Fan, W., Yung, B., Huang, P., and Chen, X. (2017). Nanotechnology for multimodal synergistic cancer therapy. Chem. Rev. 117 (22), 13566–13638. doi:10.1021/acs.chemrev.7b00258
Feng, J., Xu, M., Wang, J., Zhou, S., Liu, Y., Liu, S., et al. (2020a). Sequential delivery of nanoformulated alpha-mangostin and triptolide overcomes permeation obstacles and improves therapeutic effects in pancreatic cancer. Biomaterials 241, 119907. doi:10.1016/j.biomaterials.2020.119907
Feng, M., Xiong, G., Cao, Z., Yang, G., Zheng, S., Song, X., et al. (2017). PD-1/PD-L1 and immunotherapy for pancreatic cancer. Cancer Lett. 407, 57–65. doi:10.1016/j.canlet.2017.08.006
Feng, X., Li, M., Wang, J., Zou, X., Wang, H., Wang, D., et al. (2022). MXene quantum dot/zeolitic imidazolate framework nanocarriers for dual stimulus triggered tumor chemo-phototherapy. Mater. (Basel) 15 (13), 4543. doi:10.3390/ma15134543
Feng, X., Zhang, Y., Zhang, C., Lai, X., Zhang, Y., Wu, J., et al. (2020b). Nanomaterial-mediated autophagy: Coexisting hazard and health benefits in biomedicine. Part. Fibre Toxicol. 17 (1), 53. doi:10.1186/s12989-020-00372-0
Friedl, J. D., Nele, V., De Rosa, G., and Bernkop-Schnürch, A. (2021). Bioinert, stealth or interactive: How surface chemistry of nanocarriers determines their fate in vivo. Adv. Funct. Mat. 31 (34), 2103347. doi:10.1002/adfm.202103347
Gao, F., Wu, J., Niu, S., Sun, T., Li, F., Bai, Y., et al. (2019). Biodegradable, pH-sensitive hollow mesoporous organosilica nanoparticle (HMON) with controlled release of pirfenidone and ultrasound-target-microbubble-destruction (UTMD) for pancreatic cancer treatment. Theranostics 9 (20), 6002–6018. doi:10.7150/thno.36135
Gao, J., Zhang, X., Yu, M., Ren, G., and Yang, Z. (2015). Cognitive deficits induced by multi-walled carbon nanotubes via the autophagic pathway. Toxicology 337, 21–29. doi:10.1016/j.tox.2015.08.011
Ge, L., Qiao, C., Tang, Y., Zhang, X., and Jiang, X. (2021). Light-activated hypoxia-sensitive covalent organic framework for tandem-responsive drug delivery. Nano Lett. 21 (7), 3218–3224. doi:10.1021/acs.nanolett.1c00488
Ghasemi-Chaleshtari, M., Kiaie, S. H., Irandoust, M., Karami, H., Nabi Afjadi, M., Ghani, S., et al. (2020). Concomitant blockade of A2AR and CTLA-4 by siRNA-loaded polyethylene glycol-chitosan-alginate nanoparticles synergistically enhances antitumor T-cell responses. J. Cell. Physiol. 235 (12), 10068–10080. doi:10.1002/jcp.29822
Ghosh, S., Garg, S., and Ghosh, S. (2020). Cell-derived exosome therapy: A novel approach to treat post-traumatic brain injury mediated neural injury. ACS Chem. Neurosci. 11 (14), 2045–2047. doi:10.1021/acschemneuro.0c00368
Ghosh, S., and Ghosh, S. (2022). Exosome: The "Off-the-Shelf" cellular nanocomponent as a potential pathogenic agent, a disease biomarker, and neurotherapeutics. Front. Pharmacol. 13, 878058. doi:10.3389/fphar.2022.878058
Gokita, K., Inoue, J., Ishihara, H., Kojima, K., and Inazawa, J. (2020). Therapeutic potential of LNP-mediated delivery of miR-634 for cancer therapy. Mol. Ther. Nucleic Acids 19, 330–338. doi:10.1016/j.omtn.2019.10.045
Gong, J., Hendifar, A., Tuli, R., Chuang, J., Cho, M., Chung, V., et al. (2018). Combination systemic therapies with immune checkpoint inhibitors in pancreatic cancer: Overcoming resistance to single-agent checkpoint blockade. Clin. Transl. Med. 7 (1), 32. doi:10.1186/s40169-018-0210-9
Guo, C., Yang, M., Jing, L., Wang, J., Yu, Y., Li, Y., et al. (2016). Amorphous silica nanoparticles trigger vascular endothelial cell injury through apoptosis and autophagy via reactive oxygen species-mediated MAPK/Bcl-2 and PI3K/Akt/mTOR signaling. Int. J. Nanomedicine 11, 5257–5276. doi:10.2147/ijn.S112030
Gurbuz, N., and Ozpolat, B. (2019). MicroRNA-based targeted therapeutics in pancreatic cancer. Anticancer Res. 39 (2), 529–532. doi:10.21873/anticanres.13144
He, X., Bao, X., Cao, H., Zhang, Z., Yin, Q., Gu, W., et al. (2015). Tumor-penetrating nanotherapeutics loading a near-infrared probe inhibit growth and metastasis of breast cancer. Adv. Funct. Mat. 25 (19), 2831–2839. doi:10.1002/adfm.201500772
Hosein, A. N., Brekken, R. A., and Maitra, A. (2020). Pancreatic cancer stroma: An update on therapeutic targeting strategies. Nat. Rev. Gastroenterol. Hepatol. 17 (8), 487–505. doi:10.1038/s41575-020-0300-1
Huang, G., Liu, Z., He, L., Luk, K. H., Cheung, S. T., Wong, K. H., et al. (2018). Autophagy is an important action mode for functionalized selenium nanoparticles to exhibit anti-colorectal cancer activity. Biomater. Sci. 6 (9), 2508–2517. doi:10.1039/c8bm00670a
Huang, J., and Xiao, K. (2022). Nanoparticles-based strategies to improve the delivery of therapeutic small interfering RNA in precision oncology. Pharmaceutics 14 (8), 1586. doi:10.3390/pharmaceutics14081586
Huang, Y., Ma, J., Porter, A. L., Kwon, S., and Zhu, D. H. (2015). Analyzing collaboration networks and developmental patterns of nano-enabled drug delivery (NEDD) for brain cancer. Beilstein J. Nanotechnol. 6, 1666–1676. doi:10.3762/bjnano.6.169
Huang, Z., Wu, L., Wang, W., Zhou, Y., Zhang, X., Huang, Y., et al. (2021). Unraveling the publication trends in inhalable nano-systems. J. Nanopart. Res. 24 (1), 10. doi:10.1007/s11051-021-05384-1
Huang, Z. W., Wu, L. J., Wang, W. H., Zhou, Y., Zhang, X. J., Huang, Y., et al. (2022). Unraveling the publication trends in inhalable nano-systems. J. Nanopart. Res. 24 (1), 10. doi:10.1007/s11051-021-05384-1
Hurtado de Mendoza, T., Mose, E. S., Botta, G. P., Braun, G. B., Kotamraju, V. R., French, R. P., et al. (2021). Tumor-penetrating therapy for β5 integrin-rich pancreas cancer. Nat. Commun. 12 (1), 1541. doi:10.1038/s41467-021-21858-1
Im, S., Lee, J., Park, D., Park, A., Kim, Y. M., and Kim, W. J. (2019). Hypoxia-triggered transforming immunomodulator for cancer immunotherapy via photodynamically enhanced antigen presentation of dendritic cell. ACS Nano 13 (1), 476–488. doi:10.1021/acsnano.8b07045
Jain, R. K., Martin, J. D., and Stylianopoulos, T. (2014). The role of mechanical forces in tumor growth and therapy. Annu. Rev. Biomed. Eng. 16, 321–346. doi:10.1146/annurev-bioeng-071813-105259
Jain, R. K. (2003). Molecular regulation of vessel maturation. Nat. Med. 9 (6), 685–693. doi:10.1038/nm0603-685
Jain, R. K., and Stylianopoulos, T. (2010). Delivering nanomedicine to solid tumors. Nat. Rev. Clin. Oncol. 7 (11), 653–664. doi:10.1038/nrclinonc.2010.139
Jang, Y., Kim, H., Yoon, S., Lee, H., Hwang, J., Jung, J., et al. (2021). Exosome-based photoacoustic imaging guided photodynamic and immunotherapy for the treatment of pancreatic cancer. J. Control. Release 330, 293–304. doi:10.1016/j.jconrel.2020.12.039
Jia, M., Zhang, D., Zhang, C., and Li, C. (2021). Nanoparticle-based delivery systems modulate the tumor microenvironment in pancreatic cancer for enhanced therapy. J. Nanobiotechnology 19 (1), 384. doi:10.1186/s12951-021-01134-6
Jiang, S., Lin, Y., Yao, H., Yang, C., Zhang, L., Luo, B., et al. (2018a). The role of unfolded protein response and ER-phagy in quantum dots-induced nephrotoxicity: An in vitro and in vivo study. Arch. Toxicol. 92 (4), 1421–1434. doi:10.1007/s00204-018-2169-0
Jiang, X., Tang, Q., Zhang, J., Wang, H., Bai, L., Meng, P., et al. (2018b). Autophagy-dependent release of zinc ions is critical for acute lung injury triggered by zinc oxide nanoparticles. Nanotoxicology 12 (9), 1068–1091. doi:10.1080/17435390.2018.1513094
Jung, J. Y., Ryu, H. J., Lee, S. H., Kim, D. Y., Kim, M. J., Lee, E. J., et al. (2021). siRNA nanoparticle targeting PD-L1 activates tumor immunity and abrogates pancreatic cancer growth in humanized preclinical model. Cells 10 (10), 2734. doi:10.3390/cells10102734
Kara, G., Arun, B., Calin, G. A., and Ozpolat, B. (2022). miRacle of microRNA-Driven Cancer Nanotherapeutics. Cancers (Basel) 14 (15), 3818. doi:10.3390/cancers14153818
Karamitopoulou, E. (2020). The tumor microenvironment of pancreatic cancer. Cancers (Basel) 12 (10), E3076. doi:10.3390/cancers12103076
Kaushik, N., Borkar, S. B., Nandanwar, S. K., Panda, P. K., Choi, E. H., and Kaushik, N. K. (2022). Nanocarrier cancer therapeutics with functional stimuli-responsive mechanisms. J. Nanobiotechnology 20 (1), 152. doi:10.1186/s12951-022-01364-2
Kida, H., Feril, L. B., Irie, Y., Endo, H., Itaka, K., and Tachibana, K. (2022). Influence of nanobubble size distribution on ultrasound-mediated plasmid DNA and messenger RNA gene delivery. Front. Pharmacol. 13, 855495. doi:10.3389/fphar.2022.855495
Kielaite-Gulla, A., Andriusaityte, U., Zdanys, G. T., Babonaite, E., Strupas, K., and Kelly, H. (2022). The impact of epithelial-mesenchymal transition and metformin on pancreatic cancer chemoresistance: A pathway towards individualized therapy. Med. Kaunas. 58 (4), 467. doi:10.3390/medicina58040467
Kokkinos, J., Ignacio, R. M. C., Sharbeen, G., Boyer, C., Gonzales-Aloy, E., Goldstein, D., et al. (2020). Targeting the undruggable in pancreatic cancer using nano-based gene silencing drugs. Biomaterials 240, 119742. doi:10.1016/j.biomaterials.2019.119742
Kota, J., Hancock, J., Kwon, J., and Korc, M. (2017). Pancreatic cancer: Stroma and its current and emerging targeted therapies. Cancer Lett. 391, 38–49. doi:10.1016/j.canlet.2016.12.035
Kulkarni, P., Haldar, M. K., Karandish, F., Confeld, M., Hossain, R., Borowicz, P., et al. (2018). Tissue-penetrating, hypoxia-responsive echogenic polymersomes for drug delivery to solid tumors. Chemistry 24 (48), 12490–12494. doi:10.1002/chem.201802229
Kumar, P., Liu, B., and Behl, G. (2019). A comprehensive outlook of synthetic strategies and applications of redox-responsive nanogels in drug delivery. Macromol. Biosci. 19 (8), e1900071. doi:10.1002/mabi.201900071
Lai, J., Shah, B. P., Zhang, Y., Yang, L., and Lee, K. B. (2015). Real-time monitoring of ATP-responsive drug release using mesoporous-silica-coated multicolor upconversion nanoparticles. ACS Nano 9 (5), 5234–5245. doi:10.1021/acsnano.5b00641
Lammers, T., Kiessling, F., Hennink, W. E., and Storm, G. (2012). Drug targeting to tumors: Principles, pitfalls and (pre-) clinical progress. J. Control. Release 161 (2), 175–187. doi:10.1016/j.jconrel.2011.09.063
Latorre, A., Latorre, A., Castellanos, M., Rodriguez Diaz, C., Lazaro-Carrillo, A., Aguado, T., et al. (2019). Multifunctional albumin-stabilized gold nanoclusters for the reduction of cancer stem cells. Cancers (Basel) 11 (7), E969. doi:10.3390/cancers11070969
Lee, S., Kim, J. H., Moon, H., Lee, H. J., and Han, J. K. (2020). Combined treatment of sorafenib and doxorubicin-loaded microbubble-albumin nanoparticle complex for hepatocellular carcinoma: A feasibility study. PLoS One 15 (12), e0243815. doi:10.1371/journal.pone.0243815
Li, H. J., Du, J. Z., Du, X. J., Xu, C. F., Sun, C. Y., Wang, H. X., et al. (2016a). Stimuli-responsive clustered nanoparticles for improved tumor penetration and therapeutic efficacy. Proc. Natl. Acad. Sci. U. S. A. 113 (15), 4164–4169. doi:10.1073/pnas.1522080113
Li, J., Chen, Y., Zeng, L., Lian, G., Chen, S., Li, Y., et al. (2016b). A nanoparticle carrier for Co-delivery of gemcitabine and small interfering RNA in pancreatic cancer therapy. J. Biomed. Nanotechnol. 12 (8), 1654–1666. doi:10.1166/jbn.2016.2269
Li, X., Ma, S., Deng, Y., Yi, P., and Yu, J. (2022). Targeting the RNA m(6)A modification for cancer immunotherapy. Mol. Cancer 21 (1), 76. doi:10.1186/s12943-022-01558-0
Li, X., Xu, H., Li, C., Qiao, G., Farooqi, A. A., Gedanken, A., et al. (2019). Zinc-doped copper oxide nanocomposites inhibit the growth of pancreatic cancer by inducing autophagy through AMPK/mTOR pathway. Front. Pharmacol. 10, 319. doi:10.3389/fphar.2019.00319
Li, Y., Zeng, X., Wang, S., Sun, Y., Wang, Z., Fan, J., et al. (2015). Inhibition of autophagy protects against PAMAM dendrimers-induced hepatotoxicity. Nanotoxicology 9 (3), 344–355. doi:10.3109/17435390.2014.930533
Liang, A. L., Zhang, T. T., Zhou, N., Wu, C. Y., Lin, M. H., and Liu, Y. J. (2016). MiRNA-10b sponge: An anti-breast cancer study in vitro. Oncol. Rep. 35 (4), 1950–1958. doi:10.3892/or.2016.4596
Lin, L., Fan, Y., Gao, F., Jin, L., Li, D., Sun, W., et al. (2018). UTMD-promoted Co-delivery of gemcitabine and miR-21 inhibitor by dendrimer-entrapped gold nanoparticles for pancreatic cancer therapy. Theranostics 8 (7), 1923–1939. doi:10.7150/thno.22834
Lin, Y. F., Chiu, I. J., Cheng, F. Y., Lee, Y. H., Wang, Y. J., Hsu, Y. H., et al. (2016). The role of hypoxia-inducible factor-1α in zinc oxide nanoparticle-induced nephrotoxicity in vitro and in vivo. Part. Fibre Toxicol. 13 (1), 52. doi:10.1186/s12989-016-0163-3
Liu, J., Kang, Y., Yin, S., Chen, A., Wu, J., Liang, H., et al. (2019a). Key role of microtubule and its acetylation in a zinc oxide nanoparticle-mediated lysosome-autophagy system. Small 15 (25), e1901073. doi:10.1002/smll.201901073
Liu, L., Kshirsagar, P., Christiansen, J., Gautam, S. K., Aithal, A., Gulati, M., et al. (2021a). Polyanhydride nanoparticles stabilize pancreatic cancer antigen MUC4β. J. Biomed. Mat. Res. A 109 (6), 893–902. doi:10.1002/jbm.a.37080
Liu, T., Yang, L., Mao, H., Ma, F., Wang, Y., and Zhan, Y. (2021b). Knowledge domain and emerging trends in podocyte injury research from 1994 to 2021: A bibliometric and visualized analysis. Front. Pharmacol. 12, 772386. doi:10.3389/fphar.2021.772386
Liu, X., Jiang, J., Nel, A. E., and Meng, H. (2017a). Major effect of transcytosis on nano drug delivery to pancreatic cancer. Mol. Cell. Oncol. 4 (4), e1335273. doi:10.1080/23723556.2017.1335273
Liu, X., Lin, P., Perrett, I., Lin, J., Liao, Y. P., Chang, C. H., et al. (2017b). Tumor-penetrating peptide enhances transcytosis of silicasome-based chemotherapy for pancreatic cancer. J. Clin. Investig. 127 (5), 2007–2018. doi:10.1172/jci92284
Liu, Y., Long, T., Zhang, N., Qiao, B., Yang, Q., Luo, Y., et al. (2020). Ultrasound-mediated long-circulating nanopolymer delivery of therapeutic siRNA and antisense MicroRNAs leads to enhanced paclitaxel sensitivity in epithelial ovarian cancer chemotherapy. ACS Biomater. Sci. Eng. 6 (7), 4036–4050. doi:10.1021/acsbiomaterials.0c00330
Liu, Y., Pan, Y., Cao, W., Xia, F., Liu, B., Niu, J., et al. (2019b). A tumor microenvironment responsive biodegradable CaCO(3)/MnO(2)- based nanoplatform for the enhanced photodynamic therapy and improved PD-L1 immunotherapy. Theranostics 9 (23), 6867–6884. doi:10.7150/thno.37586
Lu, T., and Ten Hagen, T. L. M. (2020). A novel kinetic model to describe the ultra-fast triggered release of thermosensitive liposomal drug delivery systems. J. Control. Release 324, 669–678. doi:10.1016/j.jconrel.2020.05.047
Lu, Y., He, W., Huang, X., He, Y., Gou, X., Liu, X., et al. (2021). Strategies to package recombinant Adeno-Associated Virus expressing the N-terminal gasdermin domain for tumor treatment. Nat. Commun. 12 (1), 7155. doi:10.1038/s41467-021-27407-0
Luo, D., Xu, X., Iqbal, M. Z., Zhao, Q., Zhao, R., Farheen, J., et al. (2021). siRNA-loaded hydroxyapatite nanoparticles for KRAS gene silencing in anti-pancreatic cancer therapy. Pharmaceutics 13 (9), 1428. doi:10.3390/pharmaceutics13091428
Ma, J., Xue, H., He, L. H., Wang, L. Y., Wang, X. J., Li, X., et al. (2021). The role and mechanism of autophagy in pancreatic cancer: An update review. Cancer Manag. Res. 13, 8231–8240. doi:10.2147/CMAR.S328786
Mahato, R. (2017). Nanoemulsion as targeted drug delivery system for cancer therapeutics. J. Pharm. Sci. Pharmacol. 3, 83–97. doi:10.1166/jpsp.2017.1082
Mao, C., Yeh, S., Fu, J., Porosnicu, M., Thomas, A., Kucera, G. L., et al. (2022). Delivery of an ectonucleotidase inhibitor with ROS-responsive nanoparticles overcomes adenosine-mediated cancer immunosuppression. Sci. Transl. Med. 14 (648), eabh1261. doi:10.1126/scitranslmed.abh1261
Masood, F. (2016). Polymeric nanoparticles for targeted drug delivery system for cancer therapy. Mat. Sci. Eng. C Mat. Biol. Appl. 60, 569–578. doi:10.1016/j.msec.2015.11.067
Matsumoto, Y., Nichols, J. W., Toh, K., Nomoto, T., Cabral, H., Miura, Y., et al. (2016). Vascular bursts enhance permeability of tumour blood vessels and improve nanoparticle delivery. Nat. Nanotechnol. 11 (6), 533–538. doi:10.1038/nnano.2015.342
Meng, H., Wang, M., Liu, H., Liu, X., Situ, A., Wu, B., et al. (2015). Use of a lipid-coated mesoporous silica nanoparticle platform for synergistic gemcitabine and paclitaxel delivery to human pancreatic cancer in mice. ACS Nano 9 (4), 3540–3557. doi:10.1021/acsnano.5b00510
Meng, Z., Zhang, Y., Shen, E., Li, W., Wang, Y., Sathiyamoorthy, K., et al. (2021). Marriage of virus-mimic surface topology and microbubble-assisted ultrasound for enhanced intratumor accumulation and improved cancer theranostics. Adv. Sci. 8 (13), 2004670. doi:10.1002/advs.202004670
Min, K., Wu, Y., Wang, S., Yang, H., Deng, H., Wei, J., et al. (2022). Developmental trends and research hotspots in bronchoscopy anesthesia: A bibliometric study. Front. Med. 9, 837389. doi:10.3389/fmed.2022.837389
Munir, M. U. (2022). Nanomedicine penetration to tumor: Challenges, and advanced strategies to tackle this issue. Cancers (Basel) 14 (12), 2904. doi:10.3390/cancers14122904
Mura, S., Nicolas, J., and Couvreur, P. (2013). Stimuli-responsive nanocarriers for drug delivery. Nat. Mat. 12 (11), 991–1003. doi:10.1038/nmat3776
Narayanaswamy, R., and Torchilin, V. P. (2019). Hydrogels and their applications in targeted drug delivery. Molecules 24 (3), E603. doi:10.3390/molecules24030603
Ngamcherdtrakul, W., Bejan, D. S., Cruz-Muñoz, W., Reda, M., Zaidan, H. Y., Siriwon, N., et al. (2022). Targeted nanoparticle for Co-delivery of HER2 siRNA and a taxane to mirror the standard treatment of HER2+ breast cancer: Efficacy in breast tumor and brain metastasis. Small 18 (11), e2107550. doi:10.1002/smll.202107550
Palzer, J., Eckstein, L., Slabu, I., Reisen, O., Neumann, U. P., and Roeth, A. A. (2021). Iron oxide nanoparticle-based hyperthermia as a treatment option in various gastrointestinal malignancies. Nanomater. (Basel) 11 (11), 3013. doi:10.3390/nano11113013
Park, E. J., Lee, G. H., Han, B. S., Lee, B. S., Lee, S., Cho, M. H., et al. (2015). Toxic response of graphene nanoplatelets in vivo and in vitro. Arch. Toxicol. 89 (9), 1557–1568. doi:10.1007/s00204-014-1303-x
Peng, M., Darko, K. O., Tao, T., Huang, Y., Su, Q., He, C., et al. (2017). Combination of metformin with chemotherapeutic drugs via different molecular mechanisms. Cancer Treat. Rev. 54, 24–33. doi:10.1016/j.ctrv.2017.01.005
Premnath, A., Benny, S., Presanna, A. T., and Mangalathillam, S. (2022). Promising role of natural exosomal nanoparticles in cancer chemoimmunotherapy. Curr. Drug Metab. 23. doi:10.2174/1389200223666220627103213
Qiu, W., Su, W., Xu, J., Liang, M., Ma, X., Xue, P., et al. (2022a). Immunomodulatory-photodynamic nano-stimulators for invoking pyroptosis to augment tumor immunotherapy. Adv. Healthc. Mat., e2201233. doi:10.1002/adhm.202201233
Qiu, W., Su, W., Xu, J., Liang, M., Ma, X., Xue, P., et al. (2022b). Immunomodulatory-photodynamic nanostimulators for invoking pyroptosis to augment tumor immunotherapy. Adv. Healthc. Mat., e2201233. doi:10.1002/adhm.202201233
Qu, J. B., Chapman, R., Chen, F., Lu, H., and Stenzel, M. H. (2017). Swollen micelles for the preparation of gated, squeezable, pH-responsive drug carriers. ACS Appl. Mat. Interfaces 9 (16), 13865–13874. doi:10.1021/acsami.7b01120
Rai, A., Noor, S., Ahmad, S. I., Alajmi, M. F., Hussain, A., Abbas, H., et al. (2021). Recent advances and implication of bioengineered nanomaterials in cancer theranostics. Med. Kaunas. 57 (2), 91. doi:10.3390/medicina57020091
Raju, G. S. R., Pavitra, E., Merchant, N., Lee, H., Prasad, G. L. V., Nagaraju, G. P., et al. (2018). Targeting autophagy in gastrointestinal malignancy by using nanomaterials as drug delivery systems. Cancer Lett. 419, 222–232. doi:10.1016/j.canlet.2018.01.044
Raza, A., Hayat, U., Rasheed, T., Bilal, M., and Iqbal, H. M. N. (2018). Redox-responsive nano-carriers as tumor-targeted drug delivery systems. Eur. J. Med. Chem. 157, 705–715. doi:10.1016/j.ejmech.2018.08.034
Ruoslahti, E. (2017). Tumor penetrating peptides for improved drug delivery. Adv. Drug Deliv. Rev. 110-111, 3–12. doi:10.1016/j.addr.2016.03.008
Sadhu, A., Ghosh, I., Moriyasu, Y., Mukherjee, A., and Bandyopadhyay, M. (2018). Role of cerium oxide nanoparticle-induced autophagy as a safeguard to exogenous H2O2-mediated DNA damage in tobacco BY-2 cells. Mutagenesis 33 (2), 161–177. doi:10.1093/mutage/gey004
Sahin, I. H., Askan, G., Hu, Z. I., and O'Reilly, E. M. (2017). Immunotherapy in pancreatic ductal adenocarcinoma: An emerging entity? Ann. Oncol. 28 (12), 2950–2961. doi:10.1093/annonc/mdx503
Sally, A., McGowan, R., Finn, K., and Moran, B. M. (2022). Current and future therapies for pancreatic ductal adenocarcinoma. Cancers (Basel) 14 (10), 2417. doi:10.3390/cancers14102417
Salmaso, S., and Caliceti, P. (2013). Stealth properties to improve therapeutic efficacy of drug nanocarriers. J. Drug Deliv. 2013, 374252. doi:10.1155/2013/374252
Sanhaji, M., Göring, J., Couleaud, P., Aires, A., Cortajarena, A. L., Courty, J., et al. (2019). The phenotype of target pancreatic cancer cells influences cell death by magnetic hyperthermia with nanoparticles carrying gemicitabine and the pseudo-peptide NucAnt. Nanomedicine 20, 101983. doi:10.1016/j.nano.2018.12.019
Schoen, S., Kilinc, M. S., Lee, H., Guo, Y., Degertekin, F. L., Woodworth, G. F., et al. (2022). Towards controlled drug delivery in brain tumors with microbubble-enhanced focused ultrasound. Adv. Drug Deliv. Rev. 180, 114043. doi:10.1016/j.addr.2021.114043
Shang, M., Chang, X., Niu, S., Li, J., Zhang, W., Wu, T., et al. (2021). The key role of autophagy in silver nanoparticle-induced BV2 cells inflammation and polarization. Food Chem. Toxicol. 154, 112324. doi:10.1016/j.fct.2021.112324
Siegel, R. L., Miller, K. D., and Jemal, A. (2015). Cancer statistics, 2015. Ca. Cancer J. Clin. 65 (1), 5–29. doi:10.3322/caac.21254
Siegel, R. L., Miller, K. D., and Jemal, A. (2019). Cancer statistics, 2019. Ca. Cancer J. Clin. 69 (1), 7–34. doi:10.3322/caac.21551
Snipstad, S., Hanstad, S., Bjørkøy, A., Mørch, Ý., and de Lange Davies, C. (2021). Sonoporation using nanoparticle-loaded microbubbles increases cellular uptake of nanoparticles compared to Co-incubation of nanoparticles and microbubbles. Pharmaceutics 13 (5), 640. doi:10.3390/pharmaceutics13050640
Song, Y., Li, D., Lu, Y., Jiang, K., Yang, Y., Xu, Y., et al. (2020). Ferrimagnetic mPEG-b-PHEP copolymer micelles loaded with iron oxide nanocubes and emodin for enhanced magnetic hyperthermia-chemotherapy. Natl. Sci. Rev. 7 (4), 723–736. doi:10.1093/nsr/nwz201
Sriraman, S. K., Aryasomayajula, B., and Torchilin, V. P. (2014). Barriers to drug delivery in solid tumors. Tissue Barriers 2, e29528. doi:10.4161/tisb.29528
Stott, M. C., Oldfield, L., Hale, J., Costello, E., and Halloran, C. M. (2022). Recent advances in understanding pancreatic cancer. Fac. Rev. 11, 9. doi:10.12703/r/11-9
Strand, M. S., Krasnick, B. A., Pan, H., Zhang, X., Bi, Y., Brooks, C., et al. (2019). Precision delivery of RAS-inhibiting siRNA to KRAS driven cancer via peptide-based nanoparticles. Oncotarget 10 (46), 4761–4775. doi:10.18632/oncotarget.27109
Strobel, O., Neoptolemos, J., Jager, D., and Buchler, M. W. (2019). Optimizing the outcomes of pancreatic cancer surgery. Nat. Rev. Clin. Oncol. 16 (1), 11–26. doi:10.1038/s41571-018-0112-1
Stylianopoulos, T., Poh, M. Z., Insin, N., Bawendi, M. G., Fukumura, D., Munn, L. L., et al. (2010). Diffusion of particles in the extracellular matrix: The effect of repulsive electrostatic interactions. Biophys. J. 99 (5), 1342–1349. doi:10.1016/j.bpj.2010.06.016
Sun, B., Yang, N., Jiang, Y., Zhang, H., Hou, C., Ji, C., et al. (2015). Antagomir-1290 suppresses CD133⁺ cells in non-small cell lung cancer by targeting fyn-related Src family tyrosine kinase. Tumour Biol. 36 (8), 6223–6230. doi:10.1007/s13277-015-3307-4
Sun, F., Zhu, Q., Li, T., Saeed, M., Xu, Z., Zhong, F., et al. (2021). Regulating glucose metabolism with prodrug nanoparticles for promoting photoimmunotherapy of pancreatic cancer. Adv. Sci. 8 (4), 2002746. doi:10.1002/advs.202002746
Sun, L., Li, Q., Zhang, L., Chai, H., Yu, L., Xu, Z., et al. (2019). Stimuli responsive PEGylated bismuth selenide hollow nanocapsules for fluorescence/CT imaging and light-driven multimodal tumor therapy. Biomater. Sci. 7 (7), 3025–3040. doi:10.1039/c9bm00351g
Sun, X., Alcaraz, N., Qiao, R., Hawley, A., Tan, A., and Boyd, B. J. (2020). Magnetically-stimulated transformations in nanostructure of lipid mesophases: Effect of structure of iron oxide nanoparticles. Colloids Surf. B Biointerfaces 191, 110965. doi:10.1016/j.colsurfb.2020.110965
Tamura, Y., Ito, A., Wakamatsu, K., Kamiya, T., Torigoe, T., Honda, H., et al. (2022). Immunomodulation of melanoma by chemo-thermo-immunotherapy using conjugates of melanogenesis substrate NPrCAP and magnetite nanoparticles: A review. Int. J. Mol. Sci. 23 (12), 6457. doi:10.3390/ijms23126457
Tan, S., Chen, Z., Mironchik, Y., Mori, N., Penet, M. F., Si, G., et al. (2022). VEGF overexpression significantly increases nanoparticle-mediated siRNA delivery and target-gene downregulation. Pharmaceutics 14 (6), 1260. doi:10.3390/pharmaceutics14061260
Tan, Y. N., Li, Y. P., Huang, J. D., Luo, M., Li, S. S., Lee, A. W., et al. (2021). Thermal-sensitive lipid nanoparticles potentiate anti-PD therapy through enhancing drug penetration and T lymphocytes infiltration in metastatic tumor. Cancer Lett. 522, 238–254. doi:10.1016/j.canlet.2021.09.031
Tang, S., Zhou, L., He, H., Cui, L., Ren, Z., Tai, Y., et al. (2022). MnO(2)-melittin nanoparticles serve as an effective anti-tumor immunotherapy by enhancing systemic immune response. Biomaterials 288, 121706. doi:10.1016/j.biomaterials.2022.121706
Tay, L. M., and Xu, C. (2017). Coating microbubbles with nanoparticles for medical imaging and drug delivery. Nanomedicine (Lond) 12 (2), 91–94. doi:10.2217/nnm-2016-0362
Thomas, A., Samykutty, A., Gomez-Gutierrez, J. G., Yin, W., Egger, M. E., McNally, M., et al. (2020). Actively targeted nanodelivery of echinomycin induces autophagy-mediated death in chemoresistant pancreatic cancer in vivo. Cancers (Basel) 12 (8), E2279. doi:10.3390/cancers12082279
Tian, C., Clauser, K. R., Ohlund, D., Rickelt, S., Huang, Y., Gupta, M., et al. (2019). Proteomic analyses of ECM during pancreatic ductal adenocarcinoma progression reveal different contributions by tumor and stromal cells. Proc. Natl. Acad. Sci. U. S. A. 116 (39), 19609–19618. doi:10.1073/pnas.1908626116
Van Roosbroeck, K., Fanini, F., Setoyama, T., Ivan, C., Rodriguez-Aguayo, C., Fuentes-Mattei, E., et al. (2017). Combining anti-mir-155 with chemotherapy for the treatment of lung cancers. Clin. Cancer Res. 23 (11), 2891–2904. doi:10.1158/1078-0432.Ccr-16-1025
Vennin, C., Murphy, K. J., Morton, J. P., Cox, T. R., Pajic, M., and Timpson, P. (2018). Reshaping the tumor stroma for treatment of pancreatic cancer. Gastroenterology 154 (4), 820–838. doi:10.1053/j.gastro.2017.11.280
Von Hoff, D. D., Ervin, T., Arena, F. P., Chiorean, E. G., Infante, J., Moore, M., et al. (2013). Increased survival in pancreatic cancer with nab-paclitaxel plus gemcitabine. N. Engl. J. Med. 369 (18), 1691–1703. doi:10.1056/NEJMoa1304369
Wang, H., Hu, Z., Sukumar, U. K., Bose, R. J., Telichko, A., Dahl, J. J., et al. (2022a). Ultrasound-Guided microbubble-mediated locoregional delivery of multiple MicroRNAs improves chemotherapy in hepatocellular carcinoma. Nanotheranostics 6 (1), 62–78. doi:10.7150/ntno.63320
Wang, J., Yu, Y., Lu, K., Yang, M., Li, Y., Zhou, X., et al. (2017). Silica nanoparticles induce autophagy dysfunction via lysosomal impairment and inhibition of autophagosome degradation in hepatocytes. Int. J. Nanomedicine 12, 809–825. doi:10.2147/IJN.S123596
Wang, K., and Herr, I. (2022). Machine-learning-based bibliometric analysis of pancreatic cancer research over the past 25 years. Front. Oncol. 12, 832385. doi:10.3389/fonc.2022.832385
Wang, K., Kievit, F. M., and Zhang, M. (2016). Nanoparticles for cancer gene therapy: Recent advances, challenges, and strategies. Pharmacol. Res. 114, 56–66. doi:10.1016/j.phrs.2016.10.016
Wang, L., An, Y., Yuan, C., Zhang, H., Liang, C., Ding, F., et al. (2015). GEM-loaded magnetic albumin nanospheres modified with cetuximab for simultaneous targeting, magnetic resonance imaging, and double-targeted thermochemotherapy of pancreatic cancer cells. Int. J. Nanomedicine 10, 2507–2519. doi:10.2147/ijn.S77642
Wang, L., Jiang, W., Su, Y., Zhan, M., Peng, S., Liu, H., et al. (2022b). Self-splittable transcytosis nanoraspberry for NIR-II photo-immunometabolic cancer therapy in deep tumor tissue. Adv. Sci. (Weinh)., e2204067. doi:10.1002/advs.202204067
Wang, R., Xu, X., Li, D., Zhang, W., Shi, X., Xu, H., et al. (2022c). Smart pH-responsive polyhydralazine/bortezomib nanoparticles for remodeling tumor microenvironment and enhancing chemotherapy. Biomaterials 288, 121737. doi:10.1016/j.biomaterials.2022.121737
Wang, X., Li, D., Huang, X., Luo, Q., Li, X., Zhang, X., et al. (2021). A bibliometric analysis and visualization of photothermal therapy on cancer. Transl. Cancer Res. 10 (3), 1204–1215. doi:10.21037/tcr-20-2961
Wang, Y., Boero, G., Zhang, X., and Brugger, J. (2022d). Nanopore generation in biodegradable silk/magnetic nanoparticle membranes by an external magnetic field for implantable drug delivery. ACS Appl. Mat. Interfaces 14 (35), 40418–40426. doi:10.1021/acsami.2c10603
Wang, Y., Gao, Z., Du, X., Chen, S., Zhang, W., Wang, J., et al. (2020). Co-inhibition of the TGF-β pathway and the PD-L1 checkpoint by pH-responsive clustered nanoparticles for pancreatic cancer microenvironment regulation and anti-tumor immunotherapy. Biomater. Sci. 8 (18), 5121–5132. doi:10.1039/d0bm00916d
Wei, F., Wang, Y., Luo, Z., Li, Y., and Duan, Y. (2017). New findings of silica nanoparticles induced ER autophagy in human colon cancer cell. Sci. Rep. 7, 42591. doi:10.1038/srep42591
Wilhelm, S., Tavares, A. J., Dai, Q., Ohta, S., Chan, W. C. W., Dvorak, H. F., et al. (2016). Analysis of nanoparticle delivery to tumours. Nat. Rev. Mat. 1 (5), 16014. doi:10.1038/natrevmats.2016.14
Won, J. E., Byeon, Y., Wi, T. I., Lee, C. M., Lee, J. H., Kang, T. H., et al. (2022). Immune checkpoint silencing using RNAi-incorporated nanoparticles enhances antitumor immunity and therapeutic efficacy compared with antibody-based approaches. J. Immunother. Cancer 10 (2), e003928. doi:10.1136/jitc-2021-003928
Wu, H., Tong, L., Wang, Y., Yan, H., and Sun, Z. (2021). Bibliometric analysis of global research trends on ultrasound microbubble: A quickly developing field. Front. Pharmacol. 12, 646626. doi:10.3389/fphar.2021.646626
Wu, J., Guo, Z., Ni, W., Feng, Y., Guo, X., Meng, M., et al. (2022). Novel cocktail therapy based on a nanocarrier with an efficient transcytosis property reverses the dynamically deteriorating tumor microenvironment for enhanced immunotherapy. Nano Lett. 22 (17), 7220–7229. doi:10.1021/acs.nanolett.2c02724
Wu, Y., Tang, Y., Xie, S., Zheng, X., Zhang, S., Mao, J., et al. (2020). Chimeric peptide supramolecular nanoparticles for plectin-1 targeted miRNA-9 delivery in pancreatic cancer. Theranostics 10 (3), 1151–1165. doi:10.7150/thno.38327
Xia, T. A., Kovochich, M., Liong, M., Meng, H., Kabehie, S., George, S., et al. (2009). Polyethyleneimine coating enhances the cellular uptake of mesoporous silica nanoparticles and allows safe delivery of siRNA and DNA constructs. ACS Nano 3 (10), 3273–3286. doi:10.1021/nn900918w
Xiao, Y., Zhang, T., Ma, X., Yang, Q. C., Yang, L. L., Yang, S. C., et al. (2021). Microenvironment-responsive prodrug-induced pyroptosis boosts cancer immunotherapy. Adv. Sci. 8 (24), e2101840. doi:10.1002/advs.202101840
Xie, C., Xu, X., Wang, X., Wei, S., Shao, L., Chen, J., et al. (2018). Cyclooxygenase-2 induces angiogenesis in pancreatic cancer mediated by prostaglandin E2. Oncol. Lett. 16 (1), 940–948. doi:10.3892/ol.2018.8786
Xing, L., Shi, Q., Zheng, K., Shen, M., Ma, J., Li, F., et al. (2016). Ultrasound-mediated microbubble destruction (UMMD) facilitates the delivery of CA19-9 targeted and paclitaxel loaded mPEG-PLGA-PLL nanoparticles in pancreatic cancer. Theranostics 6 (10), 1573–1587. doi:10.7150/thno.15164
Xu, L., Faruqu, F. N., Lim, Y. M., Lim, K. Y., Liam-Or, R., Walters, A. A., et al. (2021). Exosome-mediated RNAi of PAK4 prolongs survival of pancreatic cancer mouse model after loco-regional treatment. Biomaterials 264, 120369. doi:10.1016/j.biomaterials.2020.120369
Xu, W., Pang, C., Song, C., Qian, J., Feola, S., Cerullo, V., et al. (2022a). Black porous silicon as a photothermal agent and immunoadjuvant for efficient antitumor immunotherapy. Acta Biomater. doi:10.1016/j.actbio.2022.08.073
Xu, Y., Liu, R., Yang, H., Qu, S., Qian, L., and Dai, Z. (2022b). Enhancing photodynamic therapy efficacy against cancer metastasis by ultrasound-mediated oxygen microbubble destruction to boost tumor-targeted delivery of oxygen and renal-clearable photosensitizer micelles. ACS Appl. Mat. Interfaces 14 (22), 25197–25208. doi:10.1021/acsami.2c06655
Yang, H. K., Kim, J. H., Lee, H. J., Moon, H., Ryu, H., and Han, J. K. (2022a). Early response evaluation of doxorubicin-nanoparticle-microbubble therapy in orthotopic hepatocellular carcinoma rat model using contrast-enhanced ultrasound and intravoxel incoherent motion-diffusion MRI. Ultrasonography 41 (1), 150–163. doi:10.14366/usg.21036
Yang, Z., Cheng, R., Zhao, C., Sun, N., Luo, H., Chen, Y., et al. (2018). Thermo- and pH-dual responsive polymeric micelles with upper critical solution temperature behavior for photoacoustic imaging-guided synergistic chemo-photothermal therapy against subcutaneous and metastatic breast tumors. Theranostics 8 (15), 4097–4115. doi:10.7150/thno.26195
Yang, Z., Xie, Z., Wan, J., Yi, B., Xu, T., Shu, X., et al. (2022b). Current trends and research hotspots in pancreatic stellate cells: A bibliometric study. Front. Oncol. 12, 896679. doi:10.3389/fonc.2022.896679
Yilmaz, A., Cui, H., Caligiuri, M. A., and Yu, J. (2020). Chimeric antigen receptor-engineered natural killer cells for cancer immunotherapy. J. Hematol. Oncol. 13 (1), 168. doi:10.1186/s13045-020-00998-9
Yoo, Y. J., Lee, C. H., Park, S. H., and Lim, Y. T. (2022). Nanoparticle-based delivery strategies of multifaceted immunomodulatory RNA for cancer immunotherapy. J. Control. Release 343, 564–583. doi:10.1016/j.jconrel.2022.01.047
Yu, Q., Qiu, Y., Li, J., Tang, X., Wang, X., Cun, X., et al. (2020). Targeting cancer-associated fibroblasts by dual-responsive lipid-albumin nanoparticles to enhance drug perfusion for pancreatic tumor therapy. J. Control. Release 321, 564–575. doi:10.1016/j.jconrel.2020.02.040
Yu, W., Hurley, J., Roberts, D., Chakrabortty, S. K., Enderle, D., Noerholm, M., et al. (2021). Exosome-based liquid biopsies in cancer: Opportunities and challenges. Ann. Oncol. 32 (4), 466–477. doi:10.1016/j.annonc.2021.01.074
Yu, Y., Zhang, Z., Wang, Y., Zhu, H., Li, F., Shen, Y., et al. (2017). A new NIR-triggered doxorubicin and photosensitizer indocyanine green co-delivery system for enhanced multidrug resistant cancer treatment through simultaneous chemo/photothermal/photodynamic therapy. Acta Biomater. 59, 170–180. doi:10.1016/j.actbio.2017.06.026
Yuan, Y. G., Wang, Y. H., Xing, H. H., and Gurunathan, S. (2017). Quercetin-mediated synthesis of graphene oxide-silver nanoparticle nanocomposites: A suitable alternative nanotherapy for neuroblastoma. Int. J. Nanomedicine 12, 5819–5839. doi:10.2147/IJN.S140605
Yun, W. S., Park, J. H., Lim, D. K., Ahn, C. H., Sun, I. C., and Kim, K. (2022). How did conventional nanoparticle-mediated photothermal therapy become "hot" in combination with cancer immunotherapy? Cancers (Basel) 14 (8), 2044. doi:10.3390/cancers14082044
Zang, S., Huang, K., Li, J., Ren, K., Li, T., He, X., et al. (2022). Metabolic reprogramming by dual-targeting biomimetic nanoparticles for enhanced tumor chemo-immunotherapy. Acta Biomater. 148, 181–193. doi:10.1016/j.actbio.2022.05.045
Zhang, H., Duan, X. R., Xing, L. Y., Jia, Y. X., Zhou, J. Y., and Ma, J. J. (2022a). Exploring novel systemic therapies for pancreatic cancer: A review of emerging anti-PD-1/PD-L1 combination therapy. Neoplasma, 220306N246. doi:10.4149/neo_2022_220306N246
Zhang, J. Q., Zhou, W., Zhu, S. S., Lin, J., Wei, P. F., Li, F. F., et al. (2017). Persistency of enlarged autolysosomes underscores nanoparticle-induced autophagy in hepatocytes. Small 13 (7), 1602876. doi:10.1002/smll.201602876
Zhang, L., Liao, Y., and Tang, L. (2019a). MicroRNA-34 family: A potential tumor suppressor and therapeutic candidate in cancer. J. Exp. Clin. Cancer Res. 38 (1), 53. doi:10.1186/s13046-019-1059-5
Zhang, P., Wang, C., Zhao, J., Xiao, A., Shen, Q., Li, L., et al. (2016). Near infrared-guided smart nanocarriers for MicroRNA-controlled release of doxorubicin/siRNA with intracellular ATP as fuel. ACS Nano 10 (3), 3637–3647. doi:10.1021/acsnano.5b08145
Zhang, Q., Liu, Z., Du, J., Qin, W., Lu, M., Cui, H., et al. (2019b). Dermal exposure to nano-TiO2 induced cardiovascular toxicity through oxidative stress, inflammation and apoptosis. J. Toxicol. Sci. 44 (1), 35–45. doi:10.2131/jts.44.35
Zhang, W., Chen, L., Cui, M., Xie, L., Xi, Z., Wang, Y., et al. (2021). Successively triggered Rod-shaped protocells for enhanced tumor Chemo-Photothermal therapy. Eur. J. Pharm. Biopharm. 169, 1–11. doi:10.1016/j.ejpb.2021.08.012
Zhang, Z., Wang, Y., Rizk, M. M. I., Liang, R., Wells, C. J. R., Gurnani, P., et al. (2022b). Thermo-responsive nano-in-micro particles for MRI-guided chemotherapy. Biomater. Adv. 134, 112716. doi:10.1016/j.msec.2022.112716
Zhao, J., Wang, H., Hsiao, C. H., Chow, D. S., Koay, E. J., Kang, Y., et al. (2018). Simultaneous inhibition of hedgehog signaling and tumor proliferation remodels stroma and enhances pancreatic cancer therapy. Biomaterials 159, 215–228. doi:10.1016/j.biomaterials.2018.01.014
Zhao, Y., Zheng, Y., Zhu, Y., Zhang, Y., Zhu, H., and Liu, T. (2021). M1 macrophage-derived exosomes loaded with gemcitabine and deferasirox against chemoresistant pancreatic cancer. Pharmaceutics 13 (9), 1493. doi:10.3390/pharmaceutics13091493
Zhen, J., Tian, S., Liu, Q., Zheng, C., Zhang, Z., Ding, Y., et al. (2019). Nanocarriers responsive to a hypoxia gradient facilitate enhanced tumor penetration and improved anti-tumor efficacy. Biomater. Sci. 7 (7), 2986–2995. doi:10.1039/c9bm00461k
Zhou, Y., Chen, X., Cao, J., and Gao, H. (2020). Overcoming the biological barriers in the tumor microenvironment for improving drug delivery and efficacy. J. Mat. Chem. B 8 (31), 6765–6781. doi:10.1039/d0tb00649a
Zhu, R., He, H., Liu, Y., Cao, D., Yan, J., Duan, S., et al. (2019). Cancer-selective bioreductive chemotherapy mediated by dual hypoxia-responsive nanomedicine upon photodynamic therapy-induced hypoxia aggravation. Biomacromolecules 20 (7), 2649–2656. doi:10.1021/acs.biomac.9b00428
Zhu, S., Meng, H., Gu, Z. J., and Zhao, Y. L. (2021). Research trend of nanoscience and nanotechnology - a bibliometric analysis of Nano Today. Nano TODAY 39, 101233. doi:10.1016/j.nantod.2021.101233
Zhu, S., Zhang, J., Zhang, L., Ma, W., Man, N., Liu, Y., et al. (2017). Inhibition of kupffer cell autophagy abrogates nanoparticle-induced liver injury. Adv. Healthc. Mat. 6 (9), 1601252. doi:10.1002/adhm.201601252
Keywords: pancreatic cancer, nano-drug delivery system, bibliometric analysis, CiteSpace, R-bibliometrix, VOSviewer
Citation: Zhao J-F, Zou F-L, Zhu J-F, Huang C, Bu F-Q, Zhu Z-M and Yuan R-F (2022) Nano-drug delivery system for pancreatic cancer: A visualization and bibliometric analysis. Front. Pharmacol. 13:1025618. doi: 10.3389/fphar.2022.1025618
Received: 23 August 2022; Accepted: 22 September 2022;
Published: 18 October 2022.
Edited by:
Peisheng Xu, University of South Carolina, United StatesReviewed by:
Haiyang Wu, Tianjin Medical University, ChinaHuali Chen, Chongqing Medical University, China
Copyright © 2022 Zhao, Zou, Zhu, Huang, Bu, Zhu and Yuan. This is an open-access article distributed under the terms of the Creative Commons Attribution License (CC BY). The use, distribution or reproduction in other forums is permitted, provided the original author(s) and the copyright owner(s) are credited and that the original publication in this journal is cited, in accordance with accepted academic practice. No use, distribution or reproduction is permitted which does not comply with these terms.
*Correspondence: Rong-Fa Yuan, eXVhbnJmNzc4OEAxNjMuY29t