- 1Institute of Interdisciplinary Integrative Medicine Research, Shanghai University of Traditional Chinese Medicine, Shanghai, China
- 2Shuguang Hospital Affiliated to Shanghai University of Traditional Chinese Medicine, Shanghai, China
- 3Experiment Center for Science and Technology, Shanghai University of Traditional Chinese Medicine, Shanghai, China
- 4Qinghai Province Key Laboratory of Tibetan Medicine Pharmacology and Safety Evaluation, Northwest Institute of Plateau Biology, Chinese Academy of Sciences, Shanghai, China
Hypertrophic scar (HS) is a typical pathological response during skin injury, which can lead to pain, itching, and contracture in patients and even affect their physical and mental health. The complexity of the wound healing process leads to the formation of HS affected by many factors. Several treatments are available for HS, whereas some have more adverse reactions and can even cause new injuries with exacerbated scarring. Traditional Chinese Medicine (TCM) has a rich source, and most botanical drugs have few side effects, providing new ideas and methods for treating HS. This paper reviews the formation process of HS, the therapeutic strategy for HS, the research progress of TCM with its relevant mechanisms in the treatment of HS, and the related new drug delivery system of TCM, aiming to provide ideas for further research of botanical compounds in the treatment of HS, to promote the discovery of more efficient botanical candidates for the clinical treatment of HS, to accelerate the development of the new drug delivery system and the final clinical application, and at the same time, to promote the research on the anti-HS mechanism of multiherbal preparations (Fufang), to continuously improve the quality control and safety and effectiveness of anti-HS botanical drugs in clinical application.
1 Introduction
The scar is the abnormal product of wound healing. Its formation and development are closely related to the tissue repair process and involve many regulatory pathways. The formation of a hypertrophic scar (HS) affects the appearance and function of patients’ skin and brings severe physiological and psychological problems to patients (Li W. et al., 2021; Li et al., 2021c). More than 100 million new cases of scarring occur every year in developing countries (Zhang et al., 2018). The overall incidence of HS after skin trauma is 40%–70%. The incidence of HS after burn is as high as 80% (Wei et al., 2021), and the market for anti-scar drugs is more than $12 billion annually (Sen et al., 2009). The treatment of HS is a challenging topic in the field of burn, plastic surgery, and rehabilitation (Mousavizadeh et al., 2021). Wound healing can be divided into four stages: hemostasis, inflammation, proliferation, and remodeling. The mechanisms involved in scar formation in each stage are also different (Bowers and Franco, 2020). At present, there are surgical treatment, physical therapy (Zhang et al., 2019), laser therapy (Tan et al., 2021), cryotherapy (Tunca et al., 2019), and drug therapy (Bi et al., 2019) for HS. Each treatment has its advantages and disadvantages (Elsaie, 2021). However, effective prevention and treatment measures were still limited. As a kind of drug therapy, the botanical compounds extracted from TCM, with a rich source, showed excellent potential for scar treatment. So far, the TCM commercial drugs for the treatment of HS, including the asiaticoside tablets and asiaticoside cream ointment (Shanghai Shyndec Pharmaceutical Co., Ltd., license No. Z31020564 and Z20054146), external scar antipruritic softening ointment (Chengdu Dongyang Baixin Pharmaceutical Co., Ltd., license No. Z20050438 ), and moist burn ointment (Shantou Meibao Pharmaceutical Co., Ltd., license No. Z20000004 ), are commonly used in the clinic. The formation process of HS, the therapeutic methods for HS, the effects of TCM on HS, the HS inhibitory mechanism of TCM, and the new drug delivery system of TCM for HS were reviewed in the paper, aiming to give insight into the research on the anti-HS mechanism of botanical drugs, to discover new anti-HS drug from TCM, to promote the development of new drug delivery system of anti-HS botanical drugs, to assist in developing effective experimental and clinical strategies for the treatment of HS using TCM, and to eventually optimize the clinical application of anti-HS botanical drugs based on the safety, efficacy, and rationality. So far, there are few comprehensive reviews on TCM for treating HS.
2 The formation process of HS
Each wound must pass through the wound-healing process to heal appropriately. The wound-healing process comprises four overlapping stages: hemostasis, inflammation, proliferation, and remodeling (as shown in Figure 1) (Veith et al., 2019; Orlowski et al., 2020). ①. Immediately after injury, hemostasis begins, where clotting pathways are triggered, a temporary fibrin matrix is formed, and associated cells migrate to the injury site (Subramaniam et al., 2021). ②. The healing process is initiated when platelets aggregate and release plenty of soluble mediators. Inflammatory responses begin shortly thereafter. When local penetration of neutrophils, lymphocytes, and monocytes increases, blood vessels dilate, capillary permeability increases, and macrophages accumulate and migrate to the wound to promote phagocytosis of the bacteria and damage tissues. The hemostatic and inflammatory phases usually take 3 days to end (Zhang et al., 2020). If this phase is prolonged, too many activated cells are recruited to the injury site, which can harm the wound healing process. ③. The proliferative stage begins within a few days of injury. It is the primary wound healing process and is coordinated by successive but overlapping events such as angiogenesis, collagen deposition, granulation tissue formation, and epithelialization (Pignet et al., 2021). During this stage, fibroblasts synthesize collagen and matrix materials (proteoglycan and fibronectin) to support the formation of new cells and fresh granulation tissue and repair epidermal defects. When fibroblasts are activated and differentiated into myofibroblasts, the excessive myofibroblasts form scars with the occurrence of angiogenesis at this time. Epidermal stem cells migrate linearly to the wound center following epithelialization, and epithelial cells migrate into granulation tissue to close the epidermal deficiency (Yang Z. et al., 2021; Guillamat-Prats, 2021; Monika et al., 2021; Qiang et al., 2021). ④. The remodeling is the last stage. During this stage, collagen type III is replaced by collagen type I to maximize strength in the wound. The increase in tensile strength may last up to a year (Han et al., 2017; Wang P. H. et al., 2018; Lee and Jang, 2018).
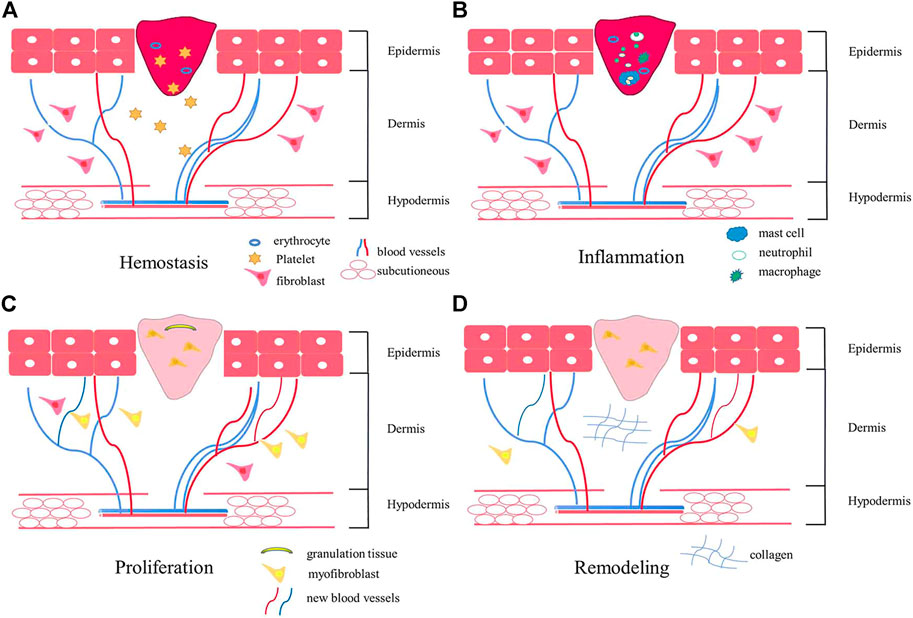
FIGURE 1. The schematic diagram of the wound healing process. Wound healing includes four stages: hemostasis (A), inflammation (B), proliferation (C), and remodeling (D). After the injury, platelets aggregate and release soluble media to initiate hemostasis. Shortly thereafter, inflammation begins, and macrophages accumulate and migrate to the wound, promoting phagocytosis of bacteria and injuring tissues. Angiogenesis occurs at the proliferation stage. In the final remodeling stage, wound tensile strength increases.
Typically, the body is efficient in repairing the skin after injury, yet wound healing is impaired when the normal repair response is out of whack. When a wound fails to heal, tissue recovery is out of kilter, leading to scarring. When the normal repair response goes awry, there are two primary outcomes: ulcerative skin defect (chronic wound) or hyperscarring (HS or keloid) (Eming et al., 2014). Chronic wounds are defined as wounds that cannot be repaired in an orderly and timely manner to achieve anatomic and functional integrity (Atkin, 2019). HS occurs after surgery, trauma, and especially burns. It is usually a hard and red raised scar (Han et al., 2017; Le and Wu, 2021). HS are fibrous scars caused by abnormal skin wound healing processes and are characterized by abnormal proliferation of fibroblasts and excessive deposition of collagen (Wang X. et al., 2018). HS and keloid are the same fibrous hyperplasia skin disease but differ in the intensity and duration of inflammation (Ogawa et al., 2016).
3 Therapeutic methods for HS
Currently, the clinical treatment methods for HS include physical therapy, laser therapy, blocking therapy, radiotherapy, surgery, drug therapy, etc. However, physical therapy, laser therapy, glucocorticoid injection, surgical resection, and other treatments are treaty-dependent, with many adverse reactions and even new injuries. Drug therapy has the effect of improving scars. However, the side effects of skin atrophy, capillary dilatation, and local necrosis cannot be ignored when common drugs such as 5-fluorouracil and corticosteroids are used. The current treatment methods for HS are summarized in Table 1.
3.1 Pressure therapy
Pressure therapy is a non-surgical method to prevent and control HS after the burn (Li et al., 2018). Pressure therapy is a method to suppress scar hyperplasia and promote scar maturation by continuous compression of wound healing parts. Reasonable pressure can reduce the thickness and increase the softness of the scar (DeBruler et al., 2020). PI3K/Akt signaling pathway was found to be an essential regulatory pathway during pressure treatment of HS(Liu et al., 2018). In the study of pressure therapy on the porcine HS model, it was observed that the percentage of elastin continuously increased after pressure treatment during 126 days, while no similar increase was observed in sham scars without pressure treatment. The increased number of elastin is associated with the increased flexibility of scars after stress therapy (Carney et al., 2017). However, the pressure and time in stress therapy still depend on experience (Van den Kerckhove et al., 2005). The lack of standardized applications reduces the effectiveness of stress therapy (Kim et al., 2015). When using a pressure suit for treatment, the design of the pressure suit will affect the contact pressure on the scar (Engrav et al., 2010). The pressure exerted by the pressure clothing on the HS directly affects the therapeutic effect. Therefore, in the research of scar pressure treatment, the precise manufacture of pressure available for specific treatment pressure still deserves further investigation (Leung et al., 2010).
3.2 Laser therapy
Laser therapy is a minimally invasive and low-risk treatment that can reduce the neuropathic pain caused by scar and improve the scar function (pigmentation, flexibility, texture, heat sensitivity, and contracture) to improve the overall quality of life of patients (Klifto et al., 2020). CO2 laser treatment is one of the comprehensive treatment methods for HS. The pigmentation, blood vessels, flexibility, and scar height were relieved with the decrease of the pain and itching after CO2 laser treatment (Zhang et al., 2021). Clinical studies verified the efficacy of the CO2 laser treatment. The elasticity and thickness of hypertrophic burn scar were mitigated, and these improvements were stable at least 6 months after the CO2 laser treatment (Miletta et al., 2021). Another study of 102 patients with keloid and HS found that 1064 nm Nd:YAG laser in treating HS was significantly better than keloid (Koike et al., 2014). After the treatment with 2940 nm Er:YAG laser, the scars were improved considerably in viscoelastic deformity, elastic deformity, and skin roughness. Such changes were not observed in the untreated scars (Madni et al., 2019). However, laser treatment could also bring side effects such as pigmentation, hypopigmentation, blister formation, and postoperative purpura (Alster, 2003; Chan et al., 2004).
3.3 Cryotherapy
Cryotherapy is to destroy scar tissue cells and blood microcirculation at extremely low temperatures to make them necrotic and fall off. Meanwhile, it can lead to scar tissue edema, increased cell space, and decreased scar density (van Leeuwen et al., 2014; Salem et al., 2021). The liquid nitrogen was imported into the freezing probe, and the freezing needle was inserted into the long axis of the HS to freeze the HS. After the HS was completely frozen, the thawed freezing probe was taken out, and the scar volume was reduced by an average of 51.4% after cryosurgery. Cryotherapy could significantly relieve scar symptoms (Har-Shai et al., 2003). However, the incidence of complications during cryotherapy could not be ignored (Meymandi et al., 2016).
3.4 Drug therapy
The efficacy of corticosteroid injection in treating HS has been well recognized, yet triamcinolone acetonide has side effects such as capillary dilatation and pigmentation (Waibel et al., 2019). Most HS’s color, thickness, softness, and vascular distribution were mitigated after intralesional injection of triamcinolone acetonide (Song et al., 2019). 5-Fluorouracil (5-FU), a nucleotide analog and a chemotherapeutic drug, can replace uracil with DNA and inhibit DNA synthesis, especially in rapidly proliferating cells. A study reported a patient with obvious HS and keloids in the nasolabial fold, the chin’s front edge, and the mandible’s lateral lower edge. After 2.5 months of treatment with local silica gel tablets and methylprednisolone acetate (40 mg/ml) in acne, the color and size of the lesions were slightly improved without an improvement in the scar fiber structure, the pain, and itching symptoms. After 7 months of 5-FU injection, the scar size, color, and texture were significantly attenuated with complete elimination of the pain and itching (Goldan et al., 2008). However, injection of 5-FU has adverse effects on normal cells with a high proliferative rate, such as digestive epithelial cells, and may also cause leukopenia and thrombocytopenia (Haurani et al., 2009; Yang B. et al., 2021). Other side effects such as pain, burning sensation, ulcer, pigmentation, and skin atrophy frequently occur (Tawfik et al., 2019). Researchers applied tacrolimus to HS to explore whether tacrolimus could promote cell apoptosis and inhibit fibroblast activity. Tacrolimus (0.1%) had a potent inhibitory effect on smooth muscle actin and can reduce the density of collagen fibers scars with the alleviation of scar inflammation even at the concertation of 0.03% tacrolimus. Tacrolimus could effectively inhibit TGF-β Smooth muscle actin, reduce mucin, and enhance collagen fiber quality and elastic fiber density (Menezes et al., 2021).
Many studies have proved that combination therapies have better efficacy than monotherapy. Compared with corticosteroids alone, corticosteroids combined with botulinum toxin A were more effective in treating keloids and HS(Tawfik et al., 2019). Among YAG laser alone, YAG laser combined with intralesional botulinum toxin type A, and YAG laser combined with intralesional steroid injection in the treatment of HS, YAG laser combined with intralesional steroid injection had the highest efficacy and safety in HS patients (Rahman et al., 2021). The HS intense pulsed light combined with lattice CO2 laser was also a successful therapeutic combination for HS with significant improvements in the color and texture of scars (Daoud et al., 2019). In summary, compared with monotherapy, the combination therapy makes the treatment more effective with less trauma, fewer side effects, a low recurrence rate, and a short course of treatment.
4 Effects of TCM on HS
In recent years, more and more botanical compounds exacted from TCM are discovered and verified as drug candidates for treating HS. The botanical sources of TCM included Alpinia officinarum Hance, Centella asiatica (L.) Urb., Rheum palmatum L., Panax ginseng C.A.Mey., Scutellaria baicalensis Georgi, Ginkgo biloba L., Conioselinum anthriscoides ‘Chuanxiong’, Salvia miltiorrhiza Bunge, Taxus wallichiana Zucc., Stephania tetrandra S. Moore, and Kaempferia galanga L.
Galangin (3,5,7-trihydroxyflavone) is a botanical compound extracted from the root of Alpinia officinarum Hance. In-vivo studies have shown that it had a potent anti-inflammatory effect using rabbit ear HS model. Galangin inhibited HS formation through the TGF-β/Smad signaling pathway (Ru et al., 2021) and ALK5/Smad2/3 signaling pathway (Zhang Y. et al., 2016). These results suggest that galangal is a potential drug candidate for treating HS.
Asiaticoside is extracted from Centella asiatica (L.) Urban. It has been used to treat skin, venous, and microvascular diseases for many years. Asiaticoside increased the mobility of skin cells and enhanced the adhesion of initial skin cells in the wound suture seeding model. The diffusion and migration of skin cells were the main determinants of wound healing, suggesting that asiaticoside could be used as a potential promoter of wound healing (Lee et al., 2012). Oral administration of asiaticoside at 24 mg/kg/d to rabbits remarkably attenuated wound healing, and reduced scar thickness, thereby inhibiting HS(Huang et al., 2021). Asiaticoside inhibited the increase of Smad7 expression by interrupting TGF-β signal transmission through negative feedback and significantly alleviated HS in rabbit ears (Ju-Lin et al., 2009).
As one of the main components of Rheum palmatum L., emodin is widely used in treating inflammatory and non-inflammatory diseases (such as cancer). The potential therapeutic effects of emodin in HS have been elucidated (Li W. et al., 2021; Jiang et al., 2021). The researchers used the mouse HS model induced by mechanical stress to study the effect of emodin on HS. The results indicated that emodin (40 mg/ml) had a therapeutic effect on the formation of HS, which was potentially related to the inhibition of the PI3K/Akt signal pathway (Liu, 2015). Further in vitro experiments verified that emodin modulated the polarization of macrophages M1 and M2 to reduce the formation and fibrosis of HS through the Notch and TGF-β pathways (Xia et al., 2021).
Ginsenoside Rb1 is extracted from the Panax ginseng C.A.Mey. It promoted angiogenesis to improve burn wound healing in the process of skin wound repair in mice (Kimura et al., 2006). Ginsenoside Rb1 also inhibited MMP2, TIMP1, α-SMA, and TGF-β1 to alleviate HS in rabbits (Tark et al., 2015). Besides, ginsenoside Rg3, with two optical isomers of 20 (R)—Rg3 and 20 (S)-Rg3, showed the highest HS inhibitory efficacy among ginsenosides. Studies revealed that 20 (R)-Rg3 could inhibit HS in in vitro model (HS specimens from patients) through the TGF-β/Smad and ERK1/2 signal pathways (Tang et al., 2018), inferring that 20 (S)-Rg3 could be potentially used as an early intervention to reduce the formation of HS.
Baicalin is a flavonoid compound extracted from the roots of Scutellaria baicalensis Georgi (Li et al., 2019). After treatment with baicalin, the formation of HS in mice model induced by mechanical load was remarkably reduced. The mechanism of the inhibitory effect of baicalin on HS was related to the TGF-β/Smad2/3 signaling pathways (Zhang Y. F. et al., 2016). Also, baicalin regulates the miR-9/IGF-1 axis to inhibit cell proliferation and collagen production through NF-κB and Wnt/β-catenin signaling pathways (Yang L. et al., 2019).
Flavonoid quercetin is a heterocyclic polyphenol. Quercetin exists in Ginkgo biloba L. and various fruits and vegetables. It has antiviral, anti-inflammatory, and antibacterial activities (Song J. Y. et al., 2018). In-vitro studies testified that quercetin suppressed scar formation by inhibiting proliferation and contraction of excessive scar-derived fibroblasts (Phan et al., 2003).
Moreover, the essential oil from Conioselinum anthriscoides ‘Chuanxiong' (Wu et al., 2011), cryptotanshinone from Salvia miltiorrhiza Bunge (Li Y. et al., 2016), paclitaxel from Taxus wallichiana Zucc.(Huang L. P. et al., 2015), tetrandrine from Stephania tetrandra S. Moore (Ning et al., 2016), and kaempferol from Kaempferia galanga L.(Li H. et al., 2016) could mitigate the formation of HS (Table 2).
5 The HS inhibitory mechanism of TCM
In recent years, in vivo and in vitro studies have found that the mechanism of TCM inhibiting HS can be divided into reducing the inflammatory response, inhibiting fibroblast proliferation, inducing fibroblast apoptosis and autophagy, promoting the degradation of extracellular matrix, reducing angiogenesis, and inhibiting cutaneous nerve system.
5.1 Reduction of inflammation
The potential mechanism of wound healing is complex. So far, inflammation is one of the admitted decisive factors. Inflammation occurs after skin injury due to tissue damage and microbial invasion. HS is a pathological scar caused by abnormal wound healing and is characterized by persistent local inflammation and excessive collagen deposition.
Quercetin could reduce the number of macrophages and myofibroblasts during wound healing in rabbits, thereby reducing HS formation (Song J. Y. et al., 2018). Arctigenin was used in a bleomycin-induced mouse model of skin fibrosis. The results showed that the expression levels of IL-1β, IL-4, IL-6, TNF-α, and monocyte chemoattractant protein decreased significantly after arctigenin treatment, inferring that arctigenin attenuated HS potentially by reducing inflammatory response (Jiang et al., 2020). In the mouse model of HS induced by mechanical stretching (Shan et al., 2017), naringenin effectively suppressed the infiltration of inflammatory cells and the production of inflammatory cytokines (TNF-α, IL-1β, and IL-6), thereby exerting its anti-inflammatory effect to alleviate HS (Figure 2).
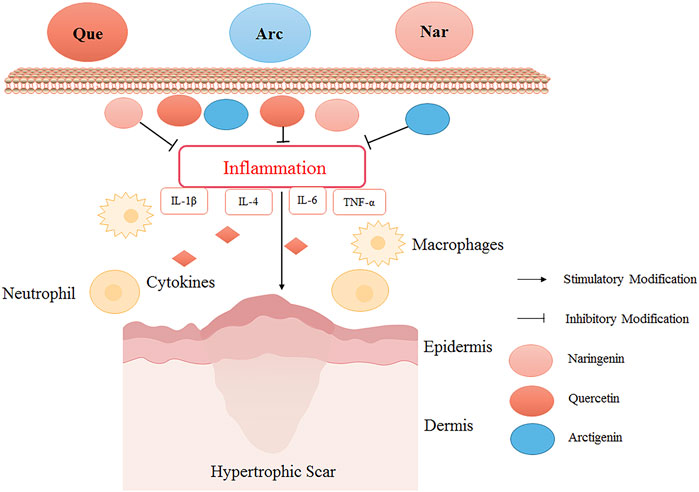
FIGURE 2. The HS inhibitory mechanism of TCM (quercetin, arctigenin, and naringenin) by reducing inflammation. Quercetin (Que), arctigenin (Arc), and naringenin (Nar) could inhibit the infiltration of inflammatory cells and the production of inflammatory cytokines to alleviate HS. Quercetin reduced the number of macrophages in the skin wound healing process, relieved inflammation, and reduced HS formation. Arctigenin alleviated HS by reducing inflammatory factors IL-1β, IL-4, IL-6, and TNF-α. Naringin down-regulated inflammatory cytokines to exert its anti-inflammatory effect to alleviate HS.
5.2 Inhibition of fibroblast proliferation and induction of fibroblast apoptosis and autophagy
In-vitro studies primarily focus on fibroblasts in the scar due to they have more active proliferation and invasion ability than fibroblasts in the steady-state environment. The activity of myofibroblasts in the scar is much higher than that in the normal state.
In-vitro studies have found that oxymatrine reduced the activity and collagen metabolism of human scar fibroblasts, increased cell apoptosis, and reduced the scar area and thickness (Deng et al., 2021). Tetramethylpyrazine, the primary chemical component of Conioselinum anthriscoides “Chuanxiong” down-regulated fibrosis-related molecules (type I collagen, type III collagen, and α-SMA) to inhibit the proliferation of scar fibroblasts and activated the expression of Bax and Cleaved Caspase-3, which finally promoted the repair of HS(Wu et al., 2020). Besides, resveratrol inhibited HS formation by activating autophagy in HS fibroblasts via the miR-4654/Rheb axis (Pang et al., 2020). As shown in Figure 3, some botanical drugs can inhibit fibroblast proliferation and induce fibroblasts’ apoptosis and autophagy to treat HS.
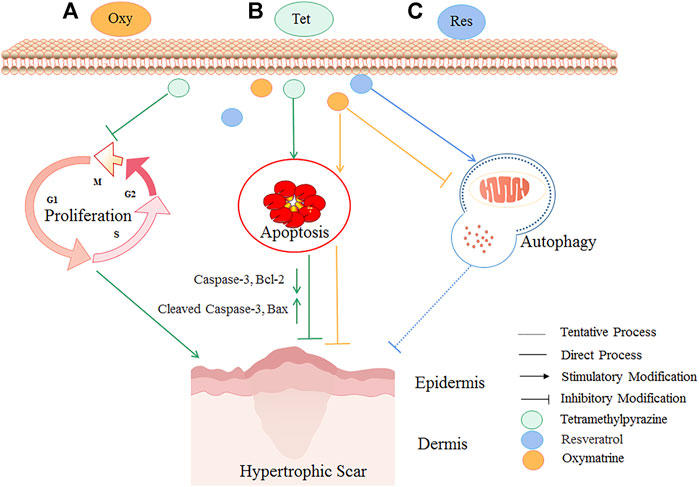
FIGURE 3. The HS inhibitory mechanism of TCM (oxymatrine, tetramethylpyrazine, and resveratrol) by inhibiting fibroblast proliferation and inducing fibroblasts’ apoptosis and autophagy. Autophagy is a conserved catabolic pathway that maintains cell metabolism and homeostasis. However, excessively activated autophagy can lead to cell death. Oxymatrine (A) could reduce scar area and epidermal-dermal thickness potentially by inhibiting autophagy and inducing apoptosis. Tetramethylpyrazine (B) could attenuate HS by inhibiting the proliferation of scar fibroblasts, activating the expression of proapoptotic proteins Bax and Cleaved Caspase-3, and reducing Caspase-3 and Bcl-2. Resveratrol (C) could potentially treat HS by activating autophagy. The yellow lines are the cellular processes modulated by oxymatrine, the green lines are the cellular processes modulated by tetramethylpyrazine, and the blue lines are the cellular processes modulated by resveratrol.
5.3 Degradation of extracellular matrix
In the remodeling stage of wound healing, the imbalance of matrix-degradation enzymes will cause excessive collagen synthesis and abnormal collagen conversion, resulting in exceeding wound healing, that is, scar formation. Therefore, promoting the extracellular matrix’s degradation can reduce the scar’s occurrence.
Panax notoginseng saponins (PNS) inhibited the extracellular matrix synthesis, stimulated cell apoptosis, and regulated the PI3K/AKT signaling pathway by changing the expression of TRPM7, thus hindering scar formation (Zhi et al., 2021). Corilagin (Cor) is a kind of ellagic tannin that exists in Phyllanthus Emblica L. and Geranium robertianum L. It has been found that in the rabbit ear scar model, corilagin could affect the protein levels of MMPs (matrix metalloproteinases) and TIMP1 (tissue inhibitor of metalloproteinases), inhibit the protein expression of TGF-β1, and reduce the level of p-Smad2/3, thereby inhibiting the deposition of extracellular matrix and various functions of fibroblasts. It is a potential drug for the treatment of HS(Li et al., 2021b). Baicalein (BAI) also effectively inhibited cell proliferation by inhibiting TGF-β1-induced accumulation of total soluble collagen, collagen I, and α-SMA through up-regulating miR29 (Yang X. et al., 2019) (As shown in Figure 4).
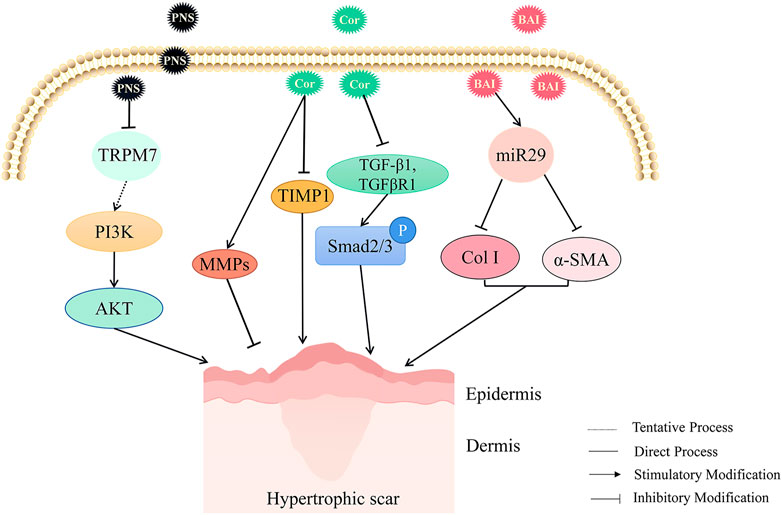
FIGURE 4. The HS inhibitory mechanism of TCM (Panax notoginseng saponins, corilagin, and baicalein) by promoting extracellular matrix degradation. Panax notoginseng saponins (PNS), corilagin (Cor), and baicalein (BAI) could promote the degradation of the extracellular matrix to reduce HS. PNS inhibited HS formation potentially by inhibiting TRPM7 and regulating PI3K/AKT signaling pathway to inhibit extracellular matrix deposition. Cor inhibited TGF-β1, reduced the expression of p-Smad2/3, affected the levels of MMPs and TIMP1 proteins, inhibited the deposition of extracellular matrix, and thus attenuated HS. Up-regulation of miR-29 by BAI could inhibit TGFβ1-induced accumulation of total soluble collagen, collagen I, and α-SMA to alleviate HS.
5.4 Inhibition of angiogenesis
Angiogenesis plays a vital role in wound healing, which involves the proliferation, migration, and formation of endothelial cells. Studies have shown that the number of microvessels in HS increases (Zheng et al., 2014; Molina et al., 2022).
Usnic acid has a variety of biological activities, such as antiviral, anti-microbial, anti-inflammatory, and anti-proliferation. Usnic acid could significantly inhibit the formation of HS and considerably reduce the thickness and color of the scar in the rabbit ear model. It has been proved that usnic acid remarkably inhibited scar angiogenesis using immunohistochemical analysis of CD31 expression. It also inhibited the proliferation of human umbilical vein endothelial cells and scar fibroblasts. These results proved that the therapeutic effect of usnic acid on HS formation in rabbit ears was due to its inhibition of scar angiogenesis (Song Y. et al., 2018). Another study revealed that a biflavonoid compound amentoflavone extracted from Selaginella tamariscina (P.Beauv.) spring also significantly inhibited the viability, migration, and angiogenesis of endothelial cells related to angiogenesis to treat HS(Zhang et al., 2014).
5.5 Inhibition of cutaneous nerve system
Inflammation and the cutaneous nerve system play essential roles in wound healing and HS formation (Yang et al., 2014; Li et al., 2015; Zhang et al., 2022). The evidence that cutaneous neurogenic inflammation could stimulate fibroblasts during scar formation has been confirmed (Zhang et al., 2022). Neurogenic inflammation is an inflammatory process due to acute injury with the release of neuropeptides, especially neuropeptide substance p (SP), from sensory nerves (Black, 2002). It has been proved that the concentration of SP was significantly increased in HS(Scott et al., 2007). Thus, the SP with its related signaling pathways may be a novel therapeutic target for HS.
SP and IL-33 released by nerves could significantly increase the secretion and gene expression of pro-inflammatory cytokine IL-1β by activating their corresponding receptors NK-1 and ST2 on human mast cells. Natural flavonoid methoxy luteolin could effectively reverse this trend and attenuate the inflammatory response, providing new therapeutic targets for treating inflammatory diseases (Taracanova et al., 2018). Puerarin, a single flavonoid glycoside, prevented paclitaxel-induced peripheral neuropathic pain in rats by inhibiting the upregulation of SP, TRPV1, and calcitonin gene-related peptide (Kong et al., 2015; Wu et al., 2019), indicating the inhibitory effects and potential of flavonoids on SP. Besides, many researches have proven the potential of flavonoids in the treatment of HS, including kaempferol (Li H. et al., 2016), quercetin (Song J. Y. et al., 2018), and dihydromyricetin (Ye et al., 2019). Can flavonoids alleviate HS by inhibiting cutaneous neurogenic inflammation via SP as the therapeutic target? Experimental evidence is needed. However, this provides a new idea for the mechanism study of flavonoids in treating HS.
6 New drug delivery system of TCM for HS
Many botanical drugs extracted from TCM have an anti-HS effect. However, the poor solubility and absorption of botanical drugs and the skin’s natural barrier hindered the penetration of chemical components and reduced their therapeutic effect (Ning et al., 2021). Long-term sustained-release and targeted preparations of TCM with appropriate carriers provide a new idea for the application of TCM in scar treatment. More and more new TCM delivery systems are developed, including hydrogel, microneedle, nano, liposome, etc.
6.1 Hydrogel
Hydrogels with different gelation mechanisms and compositions are one of the most widely used biomaterials (Sultan et al., 2019). Their advantages include good biocompatibility, ease to use, low toxicity, and excellent stability (Matiasek et al., 2018).
Researchers have prepared a new hydrogel composed of poly-γ-PGA, chit oligosaccharide, and papain. This hydrogel had a predominant porous three-dimensional network structure, good water absorption performance, and mechanical properties. This hydrogel promoted cell adhesion and inhibited the excessive proliferation of fibroblasts, which had the potential for in vivo application. It has been testified that this hydrogel could effectively inhibit excessive collagen deposition and HS formation during wound healing (Xue et al., 2021). The drug-loaded hydrogel using anti-HS botanical drugs may be a feasible preparation to increase the efficacy of the treatment of HS.
6.2 Microneedle
Microneedles can penetrate the cuticle barrier of the skin and quickly establish a large number of micro-dermal channels to make the drug penetrate and absorb accurately, which not only avoids the first pass effect of the liver but also has the advantages of no damage and no pain (McCrudden et al., 2015).
The schematic diagram of the microneedle is shown in Figure 5. The researchers mixed shikonin with hyaluronic acid to produce microneedles with enough mechanical strength to penetrate the skin and controllable dosage during preparation. The shikonin-soluble hyaluronic acid microneedle remarkably attenuated the viability and proliferation of fibroblasts and down-regulated fibrosis-related genes (TGF-β1, FAP-α, and COL1A1) to enhance the local therapeutic effect, which was conducive to the treatment of HS(Ning et al., 2021). In addition, other researchers have developed an active targeting drug delivery system for the local treatment of HS. The metal-organic framework of diphenyl carbide crosslinked cyclodextrin containing 26% quercetin was coated on the fibroblast membrane of HS. Then, the Bletilla striata polysaccharide was dispersed to prepare soluble microneedles. This microneedle showed remarkable anti-HS efficacy in rabbits (Wu et al., 2021).
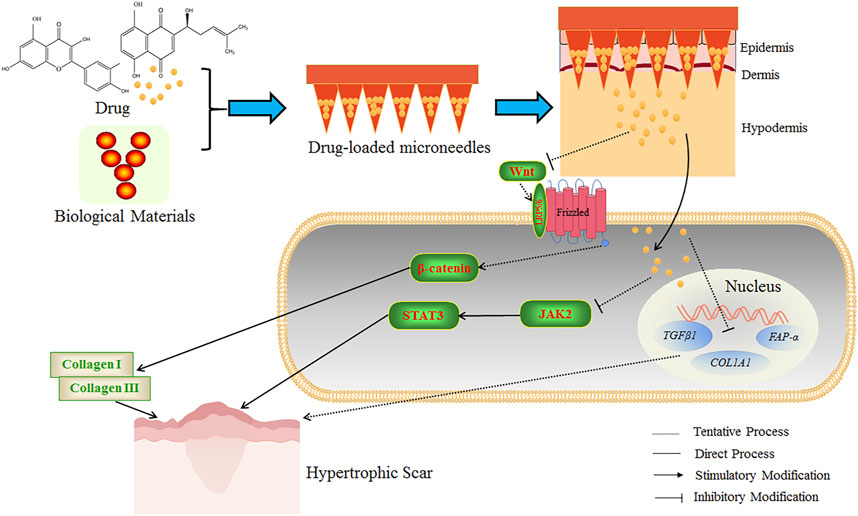
FIGURE 5. The schematic diagram of drug-loaded microneedles for the treatment of HS. Microneedles were prepared using biological materials and botanical drugs, which could establish many micro-dermal channels to accurately promote the penetration of drugs into the dermis and hypodermis. The Wnt ligand is a secreted glycoprotein that binds to Frizzled receptors, leading to the formation of a cell surface complex (Wnt-bound Frizzled combined with coreceptor LRP5/6), and β-catenin is subsequently activated and stabilized. The activated β-catenin can stimulate the transcription of Wnt target genes and the expression of fibrosis-related genes and promote the formation of collagen Ⅰ and Ⅲ to increase the HS formation. JAK2/STAT3 signaling pathway is essential in inhibiting apoptosis and tissue fibrosis. STAT3 is highly expressed in HS tissues. The application of Shikonin soluble hyaluronic acid microneedle could enhance the therapeutic effect of HS by down-regulating fibrosis-related genes to reduce the viability and proliferation of fibroblasts. The quercetin-loaded microneedle complex could improve the therapeutic effect on HS potentially by inhibiting the Wnt/β-catenin signaling pathway, reducing STAT3 expression, and inhibiting the JAK2/STAT3 signaling pathway. The above microneedles could improve the anti-HS efficacy of the botanical drugs.
6.3 Nano
Nano TCM delivery system can effectively improve the solubility and stability of effective components of TCM, improve bioavailability, and reduce the adverse reactions caused by drugs entering the systemic circulation (Huang Y. et al., 2015) (Figure 6).
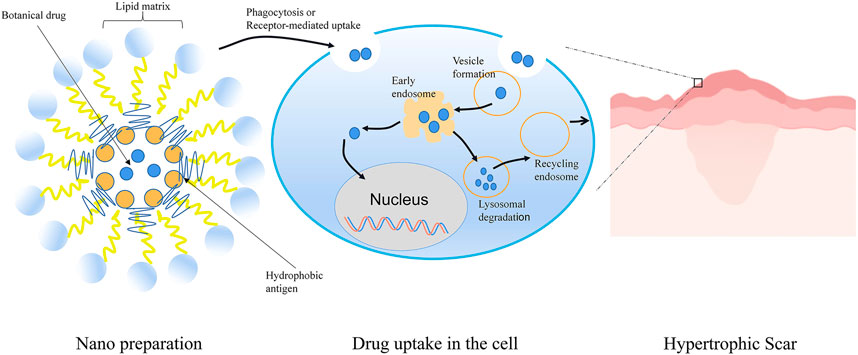
FIGURE 6. Schematic representation of Nano TCM delivery system to treat HS. Botanical drug-loaded nanoparticles were prepared by the self-assembling approach. The nanoparticles were absorbed into dermal cells by phagocytosis or receptor-mediated uptake. Subsequently, the free drug was released into the cell after the process of endosome and lysosome. Nano TCM delivery system could effectively improve the solubility and permeability of active ingredients in TCM. Spiral spray paeonol nanogel showed good transdermal performance and dermal retention. Asiaticoside nanoparticles, nanoemulsion, and nanoemulsion gel effectively overcame the skin barrier and had high drug permeability to enhance the therapeutic effect of HS.
Preparation of paeonol nanogel by spiral spray had good transdermal performance and dermal retention without stimulating the skin, providing a potential treatment strategy for HS(Guo et al., 2019). Asiaticoside is a triterpenoid pentacyclic saponin that has a pronounced therapeutic effect on human HS. However, its high molecular weight, low water solubility, and poor lipophilicity are not conducive to diffusion through the stratum corneum. The researchers designed asiaticoside nanoemulsion and nanoemulsion gel and found that these nano delivery systems showed high drug permeability through the transdermal pathway without toxicity (Li et al., 2020).
6.4 Other TCM delivery systems
It has been reported that TCM-loaded liposomes could promote drug permeability into heterogeneous scar epidermis and enhance dermal drug retention. Cell-penetrating peptide-modified salvianolic acid B liposomes successfully inhibited the proliferation, migration, and invasion of fibroblasts in a concentration- and time-dependent manner, providing a promising therapeutic strategy for transdermal drug delivery (Shi et al., 2020). Besides, microemulsion, a thermodynamic and kinetic stable system, improved the solubility of drugs and had strong transdermal permeable properties. Furthermore, it was proved that microemulsion and oxymatrine-phospholipid complex could enormously enhance the inhibitory effect of oxymatrine on scar fibroblasts (Cao et al., 2011). So far, there are more and more new TCM delivery systems are developed on the way.
7 Conclusion
HS is a fibrous tissue proliferative skin disease caused by abnormal proliferation of fibroblasts and excessive collagen deposition. The mechanisms of botanical compounds/extracts from TCM in the treatment of HS, including reducing inflammation, inhibiting fibroblast proliferation, regulating fibroblast activation and migration, inducing fibroblast apoptosis and autophagy, promoting extracellular matrix (ECM) degradation, reducing angiogenesis, and inhibiting cutaneous nerve system, are reviewed in the paper, and the new drug delivery systems of anti-HS botanical compounds are also reviewed, aiming to provide ideas for further research of TCM in the treatment of HS and finally to promote more efficient anti-HS botanical candidates for clinical application. With the updated knowledge on the mechanism of HS and the fast progress of TCM research, the botanical drugs extracted from TCM have made outstanding achievements in preventing and treating HS. However, the limitations should not be ignored. The phytopharmacology research of HS primarily focused on limited pathways without deep mechanism elucidation and new signaling pathways and targets. Interdisciplinary and larger datasets-driven research has been frequently used in pharmacology research, and more and more state-of-the-art sophisticated techniques are arising. The phytopharmacology research level is still far from pharmacology research level. Moreover, the metabolism and stability study and the transdermal kinetic study of botanical compounds in the treatment of HS are rare. Still, many multiherbal preparations (Fufang) in the treatment of HS have been clinically used in China, yet the related studies are limited. Most phytopharmacology studies of HS used a single botanical compound in animal models or cell models, which could not stand for TCM and was hard to extrapolate and lacked clinical relevance. Also, due to the complexity of the effective components in TCM, it still lacks a sufficient theoretical basis to design a reasonable dosage with TCM in clinical application. Thus, the updated analysis technology (such as High-resolution Mass Spectrometry and Ion Mobility Mass Spectrometry) combined with the bioinformatics method, as a priority, should be used to reveal the chemical components in botanical extracts, which will facilitate the pharmacology study and discovery of new candidates from TCM in the treatment of HS. The deep and exact phytopharmacological mechanism study and the new drug delivery system study of the botanical drugs in the treatment of HS based on interdisciplinary techniques should be focused on in the future. The combination of pharmacokinetics and pharmacodynamics, even the deep learning (such as BP/Elman Neural Network), should be also necessary for the phytopharmacology research of HS.
Author contributions
DC and QL contributed to writing the paper; CL and HW were responsible for the idea, fund, and paper revision. All authors contributed to the article and approved the submitted version.
Funding
This work was supported by grants from the National Natural Science Foundation of China (Grant Nos. 81974570 and 82205125), the Research on New Carrier Technology Development of Skin Barrier Repair Nursing Agent—Horizontal Project (Zhang Huimin), the Budgeted Fund of Shanghai University of Traditional Chinese Medicine (Natural Science) (Grant No. 2021LK037), and the Open Project of Qinghai Province Key Laboratory of Tibetan Medicine Pharmacology and Safety Evaluation (Grant No. 2021-ZY-03).
Conflict of interest
The authors declare that the research was conducted in the absence of any commercial or financial relationships that could be construed as a potential conflict of interest.
Publisher’s note
All claims expressed in this article are solely those of the authors and do not necessarily represent those of their affiliated organizations, or those of the publisher, the editors and the reviewers. Any product that may be evaluated in this article, or claim that may be made by its manufacturer, is not guaranteed or endorsed by the publisher.
References
Alster, T. (2003). Laser scar revision: Comparison study of 585-nm pulsed dye laser with and without intralesional corticosteroids. Dermatol. Surg. 29 (1), 25–29. doi:10.1046/j.1524-4725.2003.29024.x
Atkin, L. (2019). Chronic wounds: The challenges of appropriate management. Br. J. Community Nurs. 24, S26–S32. doi:10.12968/bjcn.2019.24.Sup9.S26
Bi, M., Sun, P., Li, D., Dong, Z., and Chen, Z. (2019). Intralesional injection of botulinum toxin type A compared with intralesional injection of corticosteroid for the treatment of hypertrophic scar and keloid: A systematic review and meta-analysis. Med. Sci. Monit. 25, 2950–2958. doi:10.12659/msm.916305
Black, P. H. (2002). Stress and the inflammatory response: A review of neurogenic inflammation. Brain Behav. Immun. 16 (6), 622–653. doi:10.1016/s0889-1591(02)00021-1
Bowers, S., and Franco, E. (2020). Chronic wounds: Evaluation and management. Am. Fam. Physician 101 (3), 159–166.
Cao, F. H., OuYang, W. Q., Wang, Y. P., Yue, P. F., and Li, S. P. (2011). A combination of a microemulsion and a phospholipid complex for topical delivery of oxymatrine. Arch. Pharm. Res. 34 (4), 551–562. doi:10.1007/s12272-011-0405-8
Carney, B. C., Liu, Z., Alkhalil, A., Travis, T. E., Ramella-Roman, J., Moffatt, L. T., et al. (2017). Elastin is differentially regulated by pressure therapy in a porcine model of hypertrophic scar. J. Burn Care Res. 38 (1), 28–35. doi:10.1097/bcr.0000000000000413
Chan, H. H., Wong, D. S., Ho, W. S., Lam, L. K., and Wei, W. (2004). The use of pulsed dye laser for the prevention and treatment of hypertrophic scars in Chinese persons. Dermatol. Surg. 30 (7), 987–994. doi:10.1111/j.1524-4725.2004.30303.x
Daoud, A. A., Gianatasio, C., Rudnick, A., Michael, M., and Waibel, J. (2019). Efficacy of combined intense pulsed light (ipl) with fractional CO(2) -laser ablation in the treatment of large hypertrophic scars: A prospective, randomized control trial. Lasers Surg. Med. 51 (8), 678–685. doi:10.1002/lsm.23092
DeBruler, D. M., Baumann, M. E., Zbinden, J. C., Blackstone, B. N., Bailey, J. K., Supp, D. M., et al. (2020). Improved scar outcomes with increased daily duration of pressure garment therapy. Adv. Wound Care 9 (8), 453–461. doi:10.1089/wound.2020.1161
Deng, X., Zhao, F., Zhao, D., Zhang, Q., Zhu, Y., Chen, Q., et al. (2021). Oxymatrine promotes hypertrophic scar repair through reduced human scar fibroblast viability, collagen and induced apoptosis via autophagy inhibition. Int. Wound J. 19, 1221–1231. doi:10.1111/iwj.13717
Elsaie, M. L. (2021). Update on management of keloid and hypertrophic scars: A systemic review. J. Cosmet. Dermatol. 20 (9), 2729–2738. doi:10.1111/jocd.14310
Eming, S. A., Martin, P., and Tomic-Canic, M. (2014). Wound repair and regeneration: Mechanisms, signaling, and translation. Sci. Transl. Med. 6 (265), 265sr6. doi:10.1126/scitranslmed.3009337
Engrav, L. H., Heimbach, D. M., Rivara, F. P., Moore, M. L., Wang, J., Carrougher, G. J., et al. (2010). 12-Year within-wound study of the effectiveness of custom pressure garment therapy. Burns 36 (7), 975–983. doi:10.1016/j.burns.2010.04.014
Goldan, O., Weissman, O., Regev, E., Haik, J., and Winkler, E. (2008). Treatment of postdermabrasion facial hypertrophic and keloid scars with intralesional 5-Fluorouracil injections. Aesthetic Plast. Surg. 32 (2), 389–392. doi:10.1007/s00266-007-9109-3
Guillamat-Prats, R. (2021). The role of MSC in wound healing, scarring and regeneration. Cells 10 (7), 1729. doi:10.3390/cells10071729
Guo, S. Y., Chen, G. T., Shi, J., Wu, Y. T., Lai, J. H., and Xu, X. Q. (2019). Study on physicochemical properties of paeonol-Helix aspersa muller nanogel and its inhibitory effects on hypertrophic scar tissue in rabbit ear. Zhongguo Zhong Yao Za Zhi 44 (22), 4857–4863. doi:10.19540/j.cnki.cjcmm.20190901.301
Han, T., Lin, D. F., and Jiang, H. (2017). Wound natural healing in treatment of tumor-like hypertrophic scar. An. Bras. Dermatol. 92 (4), 474–477. doi:10.1590/abd1806-4841.20176656
Har-Shai, Y., Amar, M., and Sabo, E. (2003). Intralesional cryotherapy for enhancing the involution of hypertrophic scars and keloids. Plast. Reconstr. Surg. 111 (6), 1841–1852. doi:10.1097/01.Prs.0000056868.42679.05
Haurani, M. J., Foreman, K., Yang, J. J., and Siddiqui, A. (2009). 5-Fluorouracil treatment of problematic scars. Plast. Reconstr. Surg. 123 (1), 139–148. doi:10.1097/PRS.0b013e3181904d1b
Huang, J., Zhou, X., Xia, L., Liu, W., Guo, F., Liu, J., et al. (2021). Inhibition of hypertrophic scar formation with oral asiaticoside treatment in a rabbit ear scar model. Int. Wound J. 18 (5), 598–607. doi:10.1111/iwj.13561
Huang, L. P., Wang, G. Q., Jia, Z. S., Chen, J. W., Wang, G., and Wang, X. L. (2015a). Paclitaxel reduces formation of hypertrophic scars in the rabbit ear model. Ther. Clin. Risk Manag. 11, 1089–1095. doi:10.2147/tcrm.S82961
Huang, Y., Zhao, Y., Liu, F., and Liu, S. (2015b). Nano traditional Chinese medicine: Current progresses and future challenges. Curr. Drug Targets 16 (13), 1548–1562. doi:10.2174/1389450116666150309122334
Jiang, L., Deng, Y., Li, W., and Lu, Y. (2020). Arctigenin suppresses fibroblast activity and extracellular matrix deposition in hypertrophic scarring by reducing inflammation and oxidative stress. Mol. Med. Rep. 22 (6), 4783–4791. doi:10.3892/mmr.2020.11539
Jiang, N., Li, Z., Luo, Y., Jiang, L., Zhang, G., Yang, Q., et al. (2021). Emodin ameliorates acute pancreatitis-induced lung injury by suppressing NLRP3 inflammasome-mediated neutrophil recruitment. Exp. Ther. Med. 22 (2), 857. doi:10.3892/etm.2021.10289
Ju-Lin, X., Shao-Hai, Q., Tian-Zeng, L., Bin, H., Jing-Ming, T., Ying-Bin, X., et al. (2009). Effect of asiaticoside on hypertrophic scar in the rabbit ear model. J. Cutan. Pathol. 36 (2), 234–239. doi:10.1111/j.1600-0560.2008.01015.x
Kim, J. Y., Willard, J. J., Supp, D. M., Roy, S., Gordillo, G. M., Sen, C. K., et al. (2015). Burn scar biomechanics after pressure garment therapy. Plast. Reconstr. Surg. 136 (3), 572–581. doi:10.1097/prs.0000000000001507
Kimura, Y., Sumiyoshi, M., Kawahira, K., and Sakanaka, M. (2006). Effects of ginseng saponins isolated from Red Ginseng roots on burn wound healing in mice. Br. J. Pharmacol. 148 (6), 860–870. doi:10.1038/sj.bjp.0706794
Klifto, K. M., Asif, M., and Hultman, C. S. (2020). Laser management of hypertrophic burn scars: A comprehensive review. Burns Trauma 8, tkz002. tkz002. doi:10.1093/burnst/tkz002
Koike, S., Akaishi, S., Nagashima, Y., Dohi, T., Hyakusoku, H., and Ogawa, R. (2014). Nd:YAG laser treatment for keloids and hypertrophic scars: An analysis of 102 cases. Plast. Reconstr. Surg. Glob. Open 2 (12), e272. doi:10.1097/gox.0000000000000231
Kong, L., Liu, J., Wang, J., Luo, Q., Zhang, H., Liu, B., et al. (2015). Icariin inhibits TNF-α/IFN-γ induced inflammatory response via inhibition of the substance P and p38-MAPK signaling pathway in human keratinocytes. Int. Immunopharmacol. 29 (2), 401–407. doi:10.1016/j.intimp.2015.10.023
Le, X., and Wu, W. W. (2021). The therapeutic effect of Interleukin-18 on hypertrophic scar through inducing Fas ligand expression. Burns. 47 (2), 430–438. doi:10.1016/j.burns.2020.07.008
Lee, H. J., and Jang, Y. J. (2018). Recent understandings of biology, prophylaxis and treatment strategies for hypertrophic scars and keloids. Int. J. Mol. Sci. 19 (3), E711. doi:10.3390/ijms19030711
Lee, J. H., Kim, H. L., Lee, M. H., You, K. E., Kwon, B. J., Seo, H. J., et al. (2012). Asiaticoside enhances normal human skin cell migration, attachment and growth in vitro wound healing model. Phytomedicine 19 (13), 1223–1227. doi:10.1016/j.phymed.2012.08.002
Leung, W. Y., Yuen, D. W., Ng, S. P., and Shi, S. Q. (2010). Pressure prediction model for compression garment design. J. Burn Care Res. 31 (5), 716–727. doi:10.1097/BCR.0b013e3181eebea0
Li, D., Shi, G., Wang, J., Zhang, D., Pan, Y., Dou, H., et al. (2019). Baicalein ameliorates pristane-induced lupus nephritis via activating Nrf2/HO-1 in myeloid-derived suppressor cells. Arthritis Res. Ther. 21 (1), 105. doi:10.1186/s13075-019-1876-0
Li, H., Peng, Q., Guo, Y., Wang, X., and Zhang, L. (2020). Preparation and in vitro and in vivo study of asiaticoside-loaded nanoemulsions and nanoemulsions-based gels for transdermal delivery. Int. J. Nanomedicine 15, 3123–3136. doi:10.2147/ijn.S241923
Li, H., Yang, L., Zhang, Y., and Gao, Z. (2016a). Kaempferol inhibits fibroblast collagen synthesis, proliferation and activation in hypertrophic scar via targeting TGF-β receptor type I. Biomed. Pharmacother. 83, 967–974. doi:10.1016/j.biopha.2016.08.011
Li, P., Li-Tsang, C. W. P., Deng, X., Wang, X., Wang, H., Zhang, Y., et al. (2018). The recovery of post-burn hypertrophic scar in a monitored pressure therapy intervention programme and the timing of intervention. Burns 44 (6), 1451–1467. doi:10.1016/j.burns.2018.01.008
Li, S. H., Yang, H. L., Xiao, H., Wang, Y. B., Wang, D. C., and Huo, R. (2015). Inflammation and cutaneous nervous system involvement in hypertrophic scarring. Neural Regen. Res. 10 (10), 1678–1682. doi:10.4103/1673-5374.167769
Li, W., Wang, D., Li, M., and Li, B. (2021a). Emodin inhibits the proliferation of papillary thyroid carcinoma by activating AMPK. Exp. Ther. Med. 22 (4), 1075. doi:10.3892/etm.2021.10509
Li, Y., Shi, S., Gao, J., Han, S., Wu, X., Jia, Y., et al. (2016b). Cryptotanshinone downregulates the profibrotic activities of hypertrophic scar fibroblasts and accelerates wound healing: A potential therapy for the reduction of skin scarring. Biomed. Pharmacother. 80, 80–86. doi:10.1016/j.biopha.2016.03.006
Li, Y., Yu, Z., Zhao, D., and Han, D. (2021b). Corilagin alleviates hypertrophic scars via inhibiting the transforming growth factor (TGF)-β/Smad signal pathway. Life Sci. 277, 119483. doi:10.1016/j.lfs.2021.119483
Li, Y., Zhang, J., Shi, J., Liu, K., Wang, X., Jia, Y., et al. (2021c). Exosomes derived from human adipose mesenchymal stem cells attenuate hypertrophic scar fibrosis by miR-192-5p/IL-17RA/Smad axis. Stem Cell Res. Ther. 12 (1), 221. doi:10.1186/s13287-021-02290-0
Liu, B., Liu, Y., Wang, L., Hou, C., and An, M. (2018). RNA-seq-based analysis of the hypertrophic scarring with and without pressure therapy in a Bama minipig model. Sci. Rep. 8 (1), 11831. doi:10.1038/s41598-018-29840-6
Liu, C. (2015). Inhibition of mechanical stress-induced hypertrophic scar inflammation by emodin. Mol. Med. Rep. 11 (6), 4087–4092. doi:10.3892/mmr.2015.3265
Madni, T. D., Lu, K., Nakonezny, P. A., Imran, J. B., Cunningham, H. B., Clark, A. T., et al. (2019). Treating hypertrophic burn scar with 2940-nm Er:YAG laser fractional ablation improves scar characteristics as measured by noninvasive technology. J. Burn Care Res. 40 (4), 416–421. doi:10.1093/jbcr/irz056
Matiasek, J., Kienzl, P., Unger, L. W., Grill, C., Koller, R., and Turk, B. R. (2018). An intra-individual surgical wound comparison shows that octenidine-based hydrogel wound dressing ameliorates scar appearance following abdominoplasty. Int. Wound J. 15 (6), 914–920. doi:10.1111/iwj.12944
McCrudden, M. T., McAlister, E., Courtenay, A. J., González-Vázquez, P., Singh, T. R., and Donnelly, R. F. (2015). Microneedle applications in improving skin appearance. Exp. Dermatol. 24 (8), 561–566. doi:10.1111/exd.12723
Menezes, M. C. S., Buzelin, M., Nunes, C. B., and Alberti, L. R. (2021). Tacrolimus action pathways in an ointment base for hypertrophic scar prevention in a rabbit ear model. An. Bras. Dermatol. 96 (4), 429–435. doi:10.1016/j.abd.2020.08.019
Meymandi, S. S., Moosazadeh, M., and Rezazadeh, A. (2016). Comparing two methods of cryotherapy and intense pulsed light with triamcinolone injection in the treatment of keloid and hypertrophic scars: A clinical trial. Osong Public Health Res. Perspect. 7 (5), 313–319. doi:10.1016/j.phrp.2016.08.005
Miletta, N., Siwy, K., Hivnor, C., Clark, J., Shofner, J., Zurakowski, D., et al. (2021). Fractional ablative laser therapy is an effective treatment for hypertrophic burn scars: A prospective study of objective and subjective outcomes. Ann. Surg. 274 (6), e574–e580. doi:10.1097/sla.0000000000003576
Molina, E. A., Hartmann, B., Oliver, M. A., Kirkpatrick, L. D., Keyloun, J. W., Moffatt, L. T., et al. (2022). Angiogenic gene characterization and vessel permeability of dermal microvascular endothelial cells isolated from burn hypertrophic scar. Sci. Rep. 12 (1), 12222. doi:10.1038/s41598-022-16376-z
Monika, P., Waiker, P. V., Chandraprabha, M. N., Rangarajan, A., and Murthy, K. N. C. (2021). Myofibroblast progeny in wound biology and wound healing studies. Wound Repair Regen. 29 (4), 531–547. doi:10.1111/wrr.12937
Mousavizadeh, S. M., Torbati, P. M., and Daryani, A. (2021). The effects of kiwifruit dressing on hypertrophic scars in a rabbit ear model. J. Wound Care 30, XVi–XVvii. doi:10.12968/jowc.2021.30.Sup9a.XV
Ning, P., Peng, Y., Liu, D. W., Hu, Y. H., Liu, Y., and Liu, D. M. (2016). Tetrandrine induces microRNA differential expression in human hypertrophic scar fibroblasts in vitro. Genet. Mol. Res. 15 (1). doi:10.4238/gmr.15017288
Ning, X., Wiraja, C., Chew, W. T. S., Fan, C., and Xu, C. (2021). Transdermal delivery of Chinese herbal medicine extract using dissolvable microneedles for hypertrophic scar treatment. Acta Pharm. Sin. B 11 (9), 2937–2944. doi:10.1016/j.apsb.2021.03.016
Ogawa, R., Akaishi, S., Kuribayashi, S., and Miyashita, T. (2016). Keloids and hypertrophic scars can now Be cured completely: Recent progress in our understanding of the pathogenesis of keloids and hypertrophic scars and the most promising current therapeutic strategy. J. Nippon. Med. Sch. 83 (2), 46–53. doi:10.1272/jnms.83.46
Orlowski, P., Zmigrodzka, M., Tomaszewska, E., Ranoszek-Soliwoda, K., Pajak, B., Slonska, A., et al. (2020). Polyphenol-conjugated bimetallic Au@AgNPs for improved wound healing. Int. J. Nanomedicine 15, 4969–4990. doi:10.2147/ijn.S252027
Pang, K., Li, B., Tang, Z., Yang, W., Hao, L., Shi, Z., et al. (2020). Resveratrol inhibits hypertrophic scars formation by activating autophagy via the miR-4654/Rheb axis. Mol. Med. Rep. 22 (4), 3440–3452. doi:10.3892/mmr.2020.11407
Phan, T. T., Sun, L., Bay, B. H., Chan, S. Y., and Lee, S. T. (2003). Dietary compounds inhibit proliferation and contraction of keloid and hypertrophic scar-derived fibroblasts in vitro: Therapeutic implication for excessive scarring. J. Trauma 54 (6), 1212–1224. doi:10.1097/01.Ta.0000030630.72836.32
Pignet, A. L., Schellnegger, M., Hecker, A., Kohlhauser, M., Kotzbeck, P., and Kamolz, L. P. (2021). Resveratrol-induced signal transduction in wound healing. Int. J. Mol. Sci. 22 (23), 12614. doi:10.3390/ijms222312614
Qiang, L., Yang, S., Cui, Y. H., and He, Y. Y. (2021). Keratinocyte autophagy enables the activation of keratinocytes and fibroblastsand facilitates wound healing. Autophagy 17 (9), 2128–2143. doi:10.1080/15548627.2020.1816342
Rahman, S. H. A., Mohamed, M. S., and Hamed, A. M. (2021). Efficacy and safety of Nd:YAG laser alone compared with combined Nd:YAG laser with intralesional steroid or botulinum toxin A in the treatment of hypertrophic scars. Lasers Med. Sci. 36 (4), 837–842. doi:10.1007/s10103-020-03120-0
Ru, Z., Hu, Y., Huang, S., Bai, L., Zhang, K., and Li, Y. (2021). Bioflavonoid galangin suppresses hypertrophic scar formation by the TGF-β/smad signaling pathway. Evid. Based. Complement. Altern. Med. 2021, 2444839. doi:10.1155/2021/2444839
Salem, S. A. M., Abdel Hameed, S. M., and Mostafa, A. E. (2021). Intense pulsed light versus cryotherapy in the treatment of hypertrophic scars: A clinical and histopathological study. J. Cosmet. Dermatol. 20 (9), 2775–2784. doi:10.1111/jocd.13971
Scott, J. R., Muangman, P., and Gibran, N. S. (2007). Making sense of hypertrophic scar: A role for nerves. Wound Repair Regen. 15, S27–S31. doi:10.1111/j.1524-475X.2007.00222.x
Sen, C. K., Gordillo, G. M., Roy, S., Kirsner, R., Lambert, L., Hunt, T. K., et al. (2009). Human skin wounds: A major and snowballing threat to public health and the economy. Wound Repair Regen. 17 (6), 763–771. doi:10.1111/j.1524-475X.2009.00543.x
Shan, S., Zhang, Y., Wu, M., Yi, B., Wang, J., and Li, Q. (2017). Naringenin attenuates fibroblast activation and inflammatory response in a mechanical stretch-induced hypertrophic scar mouse model. Mol. Med. Rep. 16 (4), 4643–4649. doi:10.3892/mmr.2017.7209
Shi, J., Guo, S., Wu, Y., Chen, G., Lai, J., and Xu, X. (2020). Behaviour of cell penetrating peptide TAT-modified liposomes loaded with salvianolic acid B on the migration, proliferation, and survival of human skin fibroblasts. J. Liposome Res. 30 (1), 93–106. doi:10.1080/08982104.2019.1593451
Song, H., Tan, J., Fu, Q., Huang, L., and Ao, M. (2019). Comparative efficacy of intralesional triamcinolone acetonide injection during early and static stage of pathological scarring. J. Cosmet. Dermatol. 18 (3), 874–878. doi:10.1111/jocd.12690
Song, J. Y., Truong, D. V., and Yang, B. S. (2018a). Quercetin shows the pharmacological activity to simultaneously downregulate the inflammatory and fibrotic responses to tissue injury in association with its ability to target multi-kinases. Pharmacology 102 (3-4), 142–153. doi:10.1159/000490417
Song, Y., Yu, Z., Song, B., Guo, S., Lei, L., Ma, X., et al. (2018b). Usnic acid inhibits hypertrophic scarring in a rabbit ear model by suppressing scar tissue angiogenesis. Biomed. Pharmacother. 108, 524–530. doi:10.1016/j.biopha.2018.06.176
Subramaniam, T., Fauzi, M. B., Lokanathan, Y., and Law, J. X. (2021). The role of calcium in wound healing. Int. J. Mol. Sci. 22 (12), 6486. doi:10.3390/ijms22126486
Sultan, A. S., Vila, T., Hefni, E., Karlsson, A. J., and Jabra-Rizk, M. A. (2019). Evaluation of the antifungal and wound-healing properties of a novel peptide-based bioadhesive hydrogel formulation. Antimicrob. Agents Chemother. 63 (10), e008888-19. doi:10.1128/aac.00888-19
Tan, J., Zhou, J., Huang, L., Fu, Q., Ao, M., Yuan, L., et al. (2021). Hypertrophic scar improvement by early intervention with ablative fractional carbon dioxide laser treatment. Lasers Surg. Med. 53 (4), 450–457. doi:10.1002/lsm.23301
Tang, M., Wang, W., Cheng, L., Jin, R., Zhang, L., Bian, W., et al. (2018). The inhibitory effects of 20(R)-ginsenoside Rg3 on the proliferation, angiogenesis, and collagen synthesis of hypertrophic scar derived fibroblasts in vitro. Iran. J. Basic Med. Sci. 21 (3), 309–317. doi:10.22038/ijbms.2018.19451.5153
Taracanova, A., Tsilioni, I., Conti, P., Norwitz, E. R., Leeman, S. E., and Theoharides, T. C. (2018). Substance P and IL-33 administered together stimulate a marked secretion of IL-1β from human mast cells, inhibited by methoxyluteolin. Proc. Natl. Acad. Sci. U. S. A. 115 (40), 9381–9390. doi:10.1073/pnas.1810133115
Tark, K. C., Lee, D. W., Lew, D. H., Kang, E. H., Roh, H., and Lee, M. C. (2015). Effects of ginsenoside Rb1 on hypertrophic scar remodeling in rabbit model. Eur. J. Pharmacol. 750, 151–159. doi:10.1016/j.ejphar.2015.01.011
Tawfik, A. A., Fathy, M., Badawi, A., Abdallah, N., and Shokeir, H. (2019). Topical 5 fluorouracil cream vs combined 5 fluorouracil and fractional erbium YAG laser for treatment of severe hypertrophic scars. Clin. Cosmet. Investig. Dermatol. 12, 173–180. doi:10.2147/ccid.S191137
Tunca, M., Gamsızkan, M., Yürekli, A., Alp Göksel, B., Fuat Çiçek, A., and Çalışkan, E. (2019). Cryosurgery to remove perichondrium for the rabbit ear hypertrophic scar model: A simplified method. Acta dermatovenerol. Alp. Pannonica Adriat. 28 (2), 57–59. doi:10.15570/actaapa.2019.15
Van den Kerckhove, E., Stappaerts, K., Fieuws, S., Laperre, J., Massage, P., Flour, M., et al. (2005). The assessment of erythema and thickness on burn related scars during pressure garment therapy as a preventive measure for hypertrophic scarring. Burns 31 (6), 696–702. doi:10.1016/j.burns.2005.04.014
van Leeuwen, M. C., Bulstra, A. E., van Leeuwen, P. A., and Niessen, F. B. (2014). A new argon gas-based device for the treatment of keloid scars with the use of intralesional cryotherapy. J. Plast. Reconstr. Aesthet. Surg. 67 (12), 1703–1710. doi:10.1016/j.bjps.2014.08.046
Veith, A. P., Henderson, K., Spencer, A., Sligar, A. D., and Baker, A. B. (2019). Therapeutic strategies for enhancing angiogenesis in wound healing. Adv. Drug Deliv. Rev. 146, 97–125. doi:10.1016/j.addr.2018.09.010
Waibel, J. S., Wulkan, A. J., Rudnick, A., and Daoud, A. (2019). Treatment of hypertrophic scars using laser-assisted corticosteroid versus laser-assisted 5-fluorouracil delivery. Dermatol. Surg. 45 (3), 423–430. doi:10.1097/dss.0000000000001678
Wang, P. H., Huang, B. S., Horng, H. C., Yeh, C. C., and Chen, Y. J. (2018a). Wound healing. J. Chin. Med. Assoc. 81 (2), 94–101. doi:10.1016/j.jcma.2017.11.002
Wang, X., Liu, K., Ruan, M., Yang, J., and Gao, Z. (2018b). Gallic acid inhibits fibroblast growth and migration in keloids through the AKT/ERK signaling pathway. Acta Biochim. Biophys. Sin. 50 (11), 1114–1120. doi:10.1093/abbs/gmy115
Wei, J., Wang, Z., Zhong, C., Ding, H., Wang, X., and Lu, S. (2021). LncRNA MIR503HG promotes hypertrophic scar progression via miR-143-3p-mediated Smad3 expression. Wound Repair Regen. 29 (5), 792–800. doi:10.1111/wrr.12913
Wu, J. G., Wei, Y. J., Ran, X., Zhang, H., Nian, H., and Qin, L. P. (2011). Inhibitory effects of essential oil from rhizomes of Ligusticum chuanxiong on hypertrophic scarring in the rabbit ear model. Pharm. Biol. 49 (7), 764–769. doi:10.3109/13880209.2010.542761
Wu, T., Hou, X., Li, J., Ruan, H., Pei, L., Guo, T., et al. (2021). Microneedle-mediated biomimetic cyclodextrin metal organic frameworks for active targeting and treatment of hypertrophic scars. ACS Nano 15 (12), 20087–20104. doi:10.1021/acsnano.1c07829
Wu, X., Wang, Z., Wu, G., Xu, X., Zhang, J., Li, Y., et al. (2020). Tetramethylpyrazine induces apoptosis and inhibits proliferation of hypertrophic scar-derived fibroblasts via inhibiting the phosphorylation of AKT. Front. Pharmacol. 11, 602. doi:10.3389/fphar.2020.00602
Wu, Y., Chen, J., and Wang, R. (2019). Puerarin suppresses TRPV1, calcitonin gene-related peptide and substance P to prevent paclitaxel-induced peripheral neuropathic pain in rats. Neuroreport 30 (4), 288–294. doi:10.1097/wnr.0000000000001199
Xia, Z., Wang, J., Yang, S., Liu, C., Qin, S., Li, W., et al. (2021). Emodin alleviates hypertrophic scar formation by suppressing macrophage polarization and inhibiting the Notch and TGF-β pathways in macrophages. Braz J. Med. Biol. Res. 54 (8), e11184. doi:10.1590/1414-431X2021e11184
Xue, Y., Qi, C., Dong, Y., Zhang, L., Liu, X., Liu, Y., et al. (2021). Poly (γ-glutamic acid)/chitooligo-saccharide/papain hydrogel prevents hypertrophic scar during skin wound healing. J. Biomed. Mat. Res. B Appl. Biomater. 109 (11), 1724–1734. doi:10.1002/jbm.b.34830
Yang, B., Dong, Y., Shen, Y., Hou, A., Quan, G., Pan, X., et al. (2021a). Bilayer dissolving microneedle array containing 5-fluorouracil and triamcinolone with biphasic release profile for hypertrophic scar therapy. Bioact. Mat. 6 (8), 2400–2411. doi:10.1016/j.bioactmat.2021.01.014
Yang, L., Di, G., Qi, X., Qu, M., Wang, Y., Duan, H., et al. (2014). Substance P promotes diabetic corneal epithelial wound healing through molecular mechanisms mediated via the neurokinin-1 receptor. Diabetes 63 (12), 4262–4274. doi:10.2337/db14-0163
Yang, L., Li, X., Zhang, S., Song, J., and Zhu, T. (2019a). Baicalein inhibits proliferation and collagen synthesis of mice fibroblast cell line NIH/3T3 by regulation of miR-9/insulin-like growth factor-1 axis. Artif. Cells Nanomed. Biotechnol. 47 (1), 3202–3211. doi:10.1080/21691401.2019.1645150
Yang, X., Zhang, C., Jiang, J., and Li, Y. (2019b). Baicalein retards proliferation and collagen deposition by activating p38MAPK-JNK via microRNA-29. J. Cell. Biochem. 120 (9), 15625–15634. doi:10.1002/jcb.28829
Yang, Z., Hu, X., Zhou, L., He, Y., Zhang, X., Yang, J., et al. (2021b). Photodynamic therapy accelerates skin wound healing through promoting re-epithelialization. Burns Trauma 9, tkab008. doi:10.1093/burnst/tkab008
Ye, X., Pang, Z., and Zhu, N. (2019). Dihydromyricetin attenuates hypertrophic scar formation by targeting activin receptor-like kinase 5. Eur. J. Pharmacol. 852, 58–67. doi:10.1016/j.ejphar.2019.02.039
Zhang, C., Yin, K., and Shen, Y. M. (2021). Efficacy of fractional carbon dioxide laser therapy for burn scars: A meta-analysis. J. Dermatol. Treat. 32 (7), 845–850. doi:10.1080/09546634.2019.1704679
Zhang, H., Wang, H. Y., Wang, D. L., and Zhang, X. D. (2019). Effect of pressure therapy for treatment of hypertrophic scar. Med. Baltim. 98 (26), e16263. doi:10.1097/md.0000000000016263
Zhang, J., Liu, Z., Cao, W., Chen, L., Xiong, X., Qin, S., et al. (2014). Amentoflavone inhibits angiogenesis of endothelial cells and stimulates apoptosis in hypertrophic scar fibroblasts. Burns 40 (5), 922–929. doi:10.1016/j.burns.2013.10.012
Zhang, L., Qin, H., Wu, Z., Chen, W., and Zhang, G. (2018). Identification of the potential targets for keloid and hypertrophic scar prevention. J. Dermatol. Treat. 29 (6), 600–605. doi:10.1080/09546634.2017.1421309
Zhang, S., Li, K., Yu, Z., Chai, J., Zhang, Z., Zhang, Y., et al. (2022). Dramatic effect of botulinum toxin type A on hypertrophic scar: A promising therapeutic drug and its mechanism through the SP-NK1R pathway in cutaneous neurogenic inflammation. Front. Med. 9, 820817. doi:10.3389/fmed.2022.820817
Zhang, X., Shu, W., Yu, Q., Qu, W., Wang, Y., and Li, R. (2020). Functional biomaterials for treatment of chronic wound. Front. Bioeng. Biotechnol. 8, 516. doi:10.3389/fbioe.2020.00516
Zhang, Y. F., Zhou, S. Z., Cheng, X. Y., Yi, B., Shan, S. Z., Wang, J., et al. (2016b). Baicalein attenuates hypertrophic scar formation via inhibition of the transforming growth factor-β/Smad2/3 signalling pathway. Br. J. Dermatol. 174 (1), 120–130. doi:10.1111/bjd.14108
Zhang, Y., Shan, S., Wang, J., Cheng, X., Yi, B., Zhou, J., et al. (2016a). Galangin inhibits hypertrophic scar formation via ALK5/Smad2/3 signaling pathway. Mol. Cell. Biochem. 413 (1-2), 109–118. doi:10.1007/s11010-015-2644-3
Zheng, J., Song, F., Lu, S. L., and Wang, X. Q. (2014). Dynamic hypoxia in scar tissue during human hypertrophic scar progression. Dermatol. Surg. 40 (5), 511–518. doi:10.1111/dsu.12474
Keywords: hypertrophic scar (HS), traditional Chinese medicine (TCM), anti-HS mechanism, new drug delivery system, therapeutic strategy
Citation: Chen D, Li Q, Zhang H, Kou F, Li Q, Lyu C and Wei H (2022) Traditional Chinese medicine for hypertrophic scars—A review of the therapeutic methods and potential effects. Front. Pharmacol. 13:1025602. doi: 10.3389/fphar.2022.1025602
Received: 23 August 2022; Accepted: 23 September 2022;
Published: 10 October 2022.
Edited by:
Somasundaram Arumugam, National Institute of Pharmaceutical Education and Research, IndiaReviewed by:
Muhammad Ali, COMSATS University Islamabad, PakistanZili Xie, Washington University in St. Louis, United States
Copyright © 2022 Chen, Li, Zhang, Kou, Li, Lyu and Wei. This is an open-access article distributed under the terms of the Creative Commons Attribution License (CC BY). The use, distribution or reproduction in other forums is permitted, provided the original author(s) and the copyright owner(s) are credited and that the original publication in this journal is cited, in accordance with accepted academic practice. No use, distribution or reproduction is permitted which does not comply with these terms.
*Correspondence: Chunming Lyu, chunming83g@126.com; Hai Wei, wei_hai@hotmail.com
†These authors have contributed equally to this work