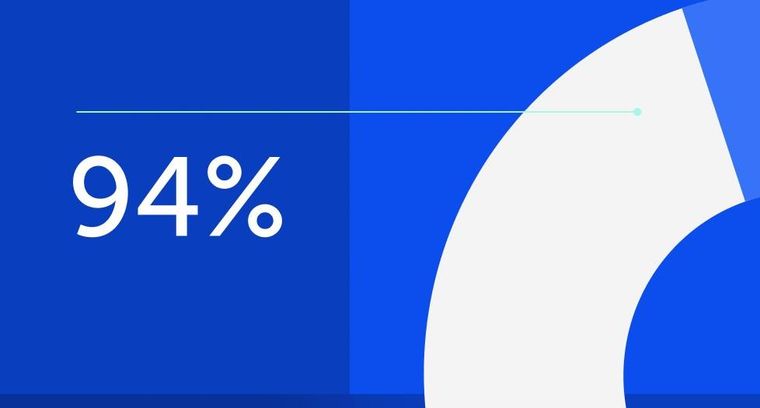
94% of researchers rate our articles as excellent or good
Learn more about the work of our research integrity team to safeguard the quality of each article we publish.
Find out more
ORIGINAL RESEARCH article
Front. Pharmacol., 07 November 2022
Sec. Inflammation Pharmacology
Volume 13 - 2022 | https://doi.org/10.3389/fphar.2022.1023450
This article is part of the Research TopicModulation of Oxidative Stress and Inflammation via the NRF2 Signaling PathwayView all 5 articles
Background and purpose: Neutrophil extracellular traps (NETs) are special web-like structures that can be generated in both infectious and noninfectious diseases. Previous studies showed that reactive oxygen species (ROS) were crucial in the formation of NETs (NETosis). The purpose of this study is to evaluate the effect of (+)-borneol, an antioxidant, on NETosis.
Methods: Human neutrophils were stimulated with phorbol-12-myristate-13-acetate (PMA) to induce NETosis in vitro. Neutrophils treated with (+)-borneol at three different time points (−30 min, 0, and 30 min) associated with PMA stimulation were used to examine the effect of (+)-borneol on the formation of NETs. The ROS generation of neutrophils was also measured to explore the potential mechanism of the inhibitory effect of (+)-borneol on NETosis.
Results: (+)-Borneol pretreatment inhibited NETosis induced by PMA. Immunofluorescence staining visualized and confirmed the inhibitory effect. (+)-Borneol inhibited the burst of ROS in neutrophils caused by PMA. Suppressing NADPH oxidase or protein kinase C (PKC) eliminated the effect of (+)-borneol on NETosis. Moreover, inhibiting Toll-like receptor 2 (TLR2) led to increased NETosis which can be inhibited by (+)-borneol.
Conclusion: (+)-Borneol decreases the ROS level in activated neutrophils and inhibits NETosis triggered by PMA stimulation in vitro. (+)-Borneol therapy may be effective in some NET-dependent conditions.
Neutrophils are essential phagocytes that play a crucial role in immune responses. They make up around 60% of white blood cells in normal conditions in humans (Bainton et al., 1971). As the first line of host defense against pathogens, they are activated and recruited to the infectious sites rapidly after pathogen entry (Mayadas et al., 2014). Activated neutrophils can not only phagocytose pathogens into the phagosome for intracellular killing but also release various proteases into the extracellular milieu to enhance their microbicidal function (Ley et al., 2018). In addition, neutrophils can capture and kill microorganisms by releasing special web-like structures called neutrophil extracellular traps (NETs) (Brinkmann et al., 2004). NETs are mainly composed of decondensed chromatin, with granular, cytoplasmic, and nuclear proteins attached (Brinkmann and Zychlinsky, 2007). The formation of NETs, known as NETosis, helps the immune system to eliminate bacteria (Brinkmann et al., 2004; Pilsczek et al., 2010), fungi (Urban et al., 2006), and viruses (Saitoh et al., 2012) more efficiently. Recently, an increasing body of evidence suggests that NETosis can be observed in noninfectious inflammation. In cardiovascular diseases, NETs can act as stimuli and scaffolds for thrombus formation (Fuchs et al., 2010; Döring et al., 2020). In autoimmune diseases, DNA and citrullinated peptides in NETs may be potential sources of autoantibody production (Hakkim et al., 2010; Sur Chowdhury et al., 2014; Grayson and Kaplan, 2016; Gupta and Kaplan, 2016). In cancers, NETs can promote tumor progression and metastasis (Olsson and Cedervall, 2016; Albrengues et al., 2018). Therefore, NETosis is a double-edged sword and should be inhibited in certain circumstances.
There are two main mechanisms of NETosis, vital NETosis and suicidal NETosis. Vital NETosis can be observed in infectious diseases. Neutrophils are still alive and can also crawl and phagocytose pathogens after vital NETosis (Yipp et al., 2012). Suicidal NETosis occurs mainly in noninfectious diseases. Phorbol-12-myristate-13-acetate (PMA) is a good stimulus to induce suicidal NETosis in vitro, which has been used originally and widely in studies (Brinkmann et al., 2004; Jorch and Kubes, 2017; Kenny et al., 2017). PMA stimulation initiates the activation of protein kinase C (PKC) and NADPH oxidase (Bianchi et al., 2009; Thiam et al., 2020) which causes reactive oxygen species (ROS) generation. Then, protein arginine deiminase 4 (PAD4), an enzyme that citrullinates histones and decondenses chromatin, is activated (Thiam et al., 2020). Finally, chromatin is released into the cytosol and expelled out of the cell. The purpose of this study is to explore methods to decrease suicidal NETosis.
Borneol, a traditional Chinese medicine, has anti-inflammatory, antioxidative, and analgesic effects (Liu et al., 2021). As one of the borneol products, (+)-borneol is extracted from fresh branches and leaves of Cinnamomum camphora (L.) Presl. It is found that (+)-borneol can enhance the activity of antioxidant enzymes such as superoxide dismutase and glutathione peroxidase (Huang et al., 2020). (+)-Borneol can also increase the expression of nuclear factor erythroid 2-related factor 2 (Nrf2) which can activate antioxidant enzymes to alleviate the effects of ROS (Li et al., 2021). Treating primary cultured cortical neurons with (+)-borneol results in decreased ROS generation (Liu et al., 2011). Furthermore, it is noteworthy that ROS are central to PMA-induced NETosis. Stimulating neutrophils with PMA leads to ROS generation (Papayannopoulos, 2018). ROS scavengers can inhibit PMA-induced NETosis (Fuchs et al., 2007; Kenny et al., 2017). Neutrophils isolated from chronic granulomatous disease patients have impaired NADPH oxidase function, and stimulating them with PMA fails to induce NETosis (Bianchi et al., 2009). Taking into consideration the crucial role of ROS in NETosis, we speculate that (+)-borneol reduces NETosis by regulating ROS generation.
In this study, we isolate neutrophils from whole human blood and stimulate them with PMA to generate NETs. Then, we demonstrate the inhibitory effects of (+)-borneol on NETosis, and the underlying mechanism is also explored.
(+)-Borneol was obtained from the Simcere Pharmaceutical Group. Polymorphprep was obtained from Axis–Shield. RPMI-1640 was obtained from Gibco. PMA and the NETosis assay kit were obtained from Cayman Chemical. HEPES buffer, enhanced cell counting kit-8 (CCK-8), and ROS assay kit were obtained from Beyotime Biotechnology. The Quant-iT PicoGreen dsDNA Assay Kit was obtained from Invitrogen. SYTOX Green nucleic acid stain and Calcein Blue AM were obtained from Maokang Biotechnology. Diphenyleneiodonium chloride (DPI) and Go6976 were obtained from Selleck. C29 and TAK-242 were obtained from MedChemExpress.
(+)-Borneol was dissolved in DMSO (80 mg/ml) and then diluted with RPMI-1640 to different concentrations (1.56, 6.25, 25, 100, and 400 μM). PMA (1 mg/ml in DMSO) was diluted with RPMI-1640 to 100 nM. The vehicle group had no (+)-borneol or inhibitor but the same PMA and DMSO level as the 400-μM (+)-borneol group. In the control group, neutrophils were treated without PMA.
This study was approved by the Medical Ethical Committee of Sir Run Run Shaw Hospital. Healthy volunteers above the age of 18 were recruited. People were excluded if they have a history of any chronic disorder or take any medication within 2 weeks. Human blood was collected according to the Declaration of Helsinki. Participants involved in the study provided written informed consent before participation.
Blood was collected using EDTA-K2 blood collection tubes. Neutrophils were isolated using Polymorphprep, according to the manufacturer’s instructions. Briefly, 5 ml of fresh blood was layered over 5 ml of Polymorphprep. After centrifugation at 600 g for 30 min, the lower band was collected and erythrocytes were removed using RBC lysis buffer (CWBIO). The neutrophils were washed and resuspended in RPMI-1640 supplemented with HEPES buffer.
Cell viability was evaluated using enhanced cell counting kit-8. The time points were determined according to the instruction. Briefly, 5 × 104/well neutrophils from three healthy volunteers were seeded in 96-well plates and treated with vehicle and different concentrations of (+)-borneol (1.56, 6.25, 25, 100, and 400 μM) for 4 h. A measure of 10 μl/well of CCK-8 solution was added and incubated for 1 h at 37°C. Wells containing no neutrophil but the same volume of RPMI-1640 and CCK-8 solutions were set as a negative control. The absorbance was then measured at 450 nm using a microplate reader (SpectraMax M5, Molecular Device). The results were normalized by dividing the value in the vehicle group and representing as percentages.
NETosis analysis was performed, as previously described (Schulz et al., 2020). Briefly, freshly collected neutrophils were plated in 24-well plates (1 × 106/well). Neutrophils were treated with or without inhibitors such as the NADPH oxidase inhibitor (DPI, 20 μM), PKC inhibitor (Go6976, 1 μM), TLR2 inhibitor (C29, 100 μM), or TLR4 inhibitor (TAK242, 100 μM) for 30 min at 37°C. Then, 400 μM (+)-borneol was added. After incubation for another 30 min, 100 nM PMA was added to each well to stimulate NETosis and incubated for 4 h. At the end of incubation, neutrophils were washed twice with 1 ml RPMI-1640 to remove unbound neutrophil elastase. Then, S7 nuclease (50 U/ml) was added to digest DNA and release NET-associated neutrophil elastase. Following incubation for 30 min, 15 μl EDTA (500 mM) was added to stop the effect of S7 nuclease. The supernatant was collected for neutrophil elastase activity analysis using N-methoxysuccinyl-Ala-Ala-Pro-Val p-nitroanilide. Cell-free DNA (cfDNA) was detected using the Quant-iT PicoGreen dsDNA Assay Kit, according to the instruction. To explore the effect of (+)-borneol, 400 μM of it was added at three different time points (−30 min, 0, and 30 min) associated with PMA stimulation. Relative fold expression of elastase and cfDNA was used in data analysis to make the results comparable.
Freshly isolated neutrophils were resuspended in RPMI-1640 and plated in 96-well plates (1 × 106/well) for 30 min. Then, neutrophils were treated with 400 μM (+)-borneol for another 30 min, followed by stimulation by 100 nM PMA for 4 h. For ROS assay, 100 μl of DCFH-DA (10 μM, diluted with RPMI-1640) was added to each well. After incubation for 30 min at 37°C, fluorescence was detected at an excitation wavelength of 480 nm and emission wavelength of 520 nm using a microplate reader (SpectraMax M5, Molecular Device).
For imaging, 1 × 104 neutrophils were plated in Φ 15-mm glass bottom dishes and incubated in the incubator for 30 min. Then, they were treated with or without 400 μM (+)-borneol for another 30 min. After that, 100 nM PMA was added to induce NETosis. After stimulation for 4 h, neutrophils were washed with 1 ml PBS. We used 5 μM Calcein Blue AM and 0.1 μM SYTOX Green nucleic acid stain to visualize live neutrophils and DNA in NETs, respectively. Images were acquired using a Nikon A1 confocal microscope.
Data from at least three independent experiments are presented as mean ± SEM. GraphPad Prism software (version 8.0) was used for data analysis. Student’s t-test and one-way ANOVA were used for two-group and multi-group comparisons, respectively. p < 0.05 was considered significant.
The chemical structure of (+)-borneol is shown in Figure 1A. To determine the effect of (+)-borneol on neutrophil viability, 5 × 104 neutrophils from healthy volunteers were treated with different concentrations of (+)-borneol (1.56, 6.25, 25, 100, and 400 μM) for 4 h. Then, the viability of neutrophils was measured using CCK-8 assay. As shown in Figure 1B, (+)-borneol did not affect neutrophil viability significantly.
FIGURE 1. (+)-Borneol has no effect on neutrophil viability. (A) Chemical structural formula of (+)-borneol. (B) 5 × 104 neutrophils from healthy volunteers were treated with different concentrations of (+)-borneol (1.56, 6.25, 25, 100, and 400 μM) for 4 h, and the cell viability was measured using a CCK-8 assay (n = 4).
Human neutrophils were isolated and stimulated with 100 nM PMA, an activator of protein kinase C (PKC) and NADPH oxidase. After stimulation for 4 h, the generation of NETs and dsDNA increased significantly compared to the control group (Figures 2A,B). In order to investigate the effect of (+)-borneol on NETosis, different concentrations of (+)-borneol were added to the medium at different time points. In groups where (+)-borneol were added 30 min before PMA stimulation, 400-μM (+)-borneol inhibited NETosis significantly (Figure 2C). Moreover, 400-μM (+)-borneol exhibited similar inhibitory effects in groups where (+)-borneol and PMA were added simultaneously (Figure 2D). However, (+)-borneol had no inhibitory effect when added 30 min after PMA stimulation (Figure 2E). The effect of (+)-borneol on the generation of dsDNA was also evaluated. (+)-Borneol decreased the generation of dsDNA significantly only when added 30 min before PMA stimulation (Figures 2F–H).
FIGURE 2. (+)-Borneol reduces PMA-induced NETosis. Neutrophils were stimulated with PMA for 4 h, (A) NETosis was measured by the NETosis assay kit, and (B) cfDNA was measured by the Quant-iT PicoGreen dsDNA Assay Kit. Neutrophils were treated with (+)-borneol (1.56, 6.25, 25, 100, and 400 μM) at three different time points related to PMA stimulation. NETosis (C–E) and cfDNA (F–H) were measured, respectively. *p < 0.05, **p < 0.01, and ***p < 0.001 compared with the control or vehicle groups (n = 3).
Immunofluorescence staining was used to visualize and confirm the inhibitory effect of (+)-borneol on NETosis. Neutrophils were pre-incubated with (+)-borneol for 30 min before PMA stimulation. Neutrophils that had no treatment or stimulation were set as control. We visualize live neutrophils and NETs with Calcein Blue AM (cell-permeable) and SYTOX Green nucleic acid stain (cell-impermeable), respectively.
After PMA stimulation, neutrophils were characterized by cell disruption, chromatin decondensation, and the subsequent massive release of NETs. Consistent with the aforementioned results, more neutrophils with morphological intact survived and NETosis was inhibited when preincubated with 400 μM (+)-borneol (Figure 3).
FIGURE 3. Immunostaining confirms the effect of (+)-borneol on NETosis. Neutrophils that had no treatment or stimulation were set as control. In the PMA group, neutrophils were stimulated with PMA for 4 h. In the (+)-borneol group, neutrophils were preincubated with 400-μM (+)-borneol for 30 min before 100-nM PMA stimulation. We visualized live neutrophils and NETs with Calcein Blue AM (blue) and SYTOX Green nucleic acid stain (green), respectively. Scale bars: 100 μm (n = 3).
Next, we examined the effect of (+)-borneol on the generation of ROS, which plays a crucial role in NETosis. PMA stimulation induced a burst of ROS in neutrophils. However, this PMA-stimulated ROS was inhibited significantly by (+)-borneol (Figure 4A). To explore the effect of (+)-borneol on ROS generation, we treated neutrophils with DPI. Consequently, DPI decreased NETosis induced by PMA, and (+)-borneol cannot enhance the effect of DPI (Figure 4B). Detection of cfDNA showed similar results (Figure 4C). Moreover, PMA-induced NETosis was inhibited by Go6976, a PKC inhibitor. Treating neutrophils with (+)-borneol did not change the inhibitory effect of Go6976 significantly (Figure 4D). Likewise, the cfDNA assay showed consistent results (Figure 4E).
FIGURE 4. (+)-Borneol inhibits PMA-stimulated ROS generation. (A) ROS generation by neutrophils. ROS generation was measured in the presence or absence of (+)-borneol before PMA stimulation. n = 4 per group. (B–E) NETs and cfDNA produced by neutrophils. n = 3 per group. (B,C) Neutrophils were preincubated with 20 μM DPI for 30 min, followed by being treated with or without (+)-borneol for another 30 min and then stimulated with PMA. (D,E) Neutrophils were pre-incubated with 1 μM Go6976 for 30 min, followed by being treated with or without (+)-borneol for another 30 min and then stimulated with PMA. *p < 0.05, **p < 0.01, and ***p < 0.001; ns = not significant.
To explore the role of TLR2/4 on PMA-induced NETosis, we pre-incubated neutrophils with C29 and TAK242 to inhibit TLR2/4. Then, neutrophils were stimulated with PMA for NETosis. It was worth noting that the inhibition of TLR2 increased the generation of NETs and cfDNA induced by PMA compared with PMA treatment alone (Figures 5A,B). However, TAK242 preincubation did not change the level of NETosis and cfDNA (Figures 5C,D). Compared with neutrophils preincubated with C29/TAK242 alone, neutrophils treated with (+)-borneol after preincubation of C29/TAK242 generated fewer NETs and cfDNA after PMA stimulation (Figures 5A–D). These results indicated that (+)-borneol suppressed NETosis in a way independent of TLR2/4.
FIGURE 5. (+)-Borneol suppressed NETosis in a way independent of TLR2/4. (A,B) Neutrophils pre-incubated with 100 μM C29 were treated with or without (+)-borneol and then stimulated with PMA. (A) NETosis and (B) cfDNA were detected after stimulation. (C,D) Neutrophils preincubated with 100 μM TAK242 were treated with or without (+)-borneol and then stimulated with PMA. (C) NETosis and (D) cfDNA were detected after stimulation. n = 3 per group; *p < 0.05 and **p < 0.01; ns = not significant.
NETs have been found to play increasingly important roles in many diseases. The effect of NETosis is a double-edged sword that should be taken seriously. In this study, we demonstrated for the first time that (+)-borneol inhibits ROS generation and NETosis of human neutrophils triggered by PMA stimulation in vitro.
The stimulus used for NETosis in this study is PMA. PMA stimulation initiates the activation of PKC and NADPH oxidase, which causes ROS generation (Bianchi et al., 2009; Thiam et al., 2020). Afterward, PAD4 is activated by ROS. Meanwhile, neutrophil elastase and myeloperoxidase are released into the cytosol from azurophilic granules. All these responses lead to histone hypercitrullination and chromatin decondensation, followed by NETosis (Jorch and Kubes, 2017). Previous studies have shown that ROS inhibitors block PMA-induced NETosis (Fuchs et al., 2007; Kenny et al., 2017). Neutrophils isolated from chronic granulomatous disease patients have impaired the NADPH oxidase function, and stimulating them with PMA fails to induce NETosis (Bianchi et al., 2009). Therefore, ROS are central to NETosis induced by PMA.
(+)-Borneol is a bicyclic terpenoid that has been shown to synergistically enhance the antitumor and neuroprotective effects of other drugs (Chen et al., 2014; Chen et al., 2015; Lai et al., 2020; Xu et al., 2021). It has been proven to inhibit Aβ-induced ROS generation in SH-SY5Y cells (Hur et al., 2013) and decrease neuronal ROS production caused by oxygen-glucose deprivation (Liu et al., 2011). (+)-Borneol can also increase the expression of Nrf2 (Hur et al., 2013), which can bind to antioxidant response element receptors and induce the expression of antioxidant enzymes to help resist ROS. Moreover, (+)-borneol exerts its antioxidative effect by increasing the superoxide dismutase and glutathione peroxidase activity (Yu et al., 2016). PMA can induce mitochondrial ROS production in neutrophils despite a slight effect on NETosis (Lood et al., 2016). A recent study shows that (+)-borneol reduces mitochondrial ROS generation in endothelial cells (Li et al., 2022).
Taking into consideration the important role of ROS in NETosis and the antioxidative effect of (+)-borneol, we evaluated the effect of (+)-borneol on NETosis. As shown previously, 400-μM (+)-borneol inhibited PMA-induced NETosis without affecting the neutrophil viability. We measured ROS generation and found that (+)-borneol can decrease PMA-stimulated ROS levels in neutrophils. DPI and Go6976 can inhibit NADPH oxidase and PKC, respectively. Treating neutrophils with them can result in a decrease in PMA-induced ROS generation and inhibition of NETosis (Fuchs et al., 2007; Hakkim et al., 2011; Kenny et al., 2017). In this study, we used DPI and Go6976 to inhibit ROS to explore the role of ROS in (+)-borneol’s effect on NETosis inhibition. As shown in Figure 4, the effects of (+)-borneol on NETosis were eliminated in neutrophils pre-treated with DPI or Go6976, which further confirmed the finding mentioned previously. However, it is noteworthy that the inhibitory effect of (+)-borneol on NETosis is time-dependent. A stronger effect was observed when (+)-borneol was added 30 min before PMA stimulation. Thus, (+)-borneol therapy targeting NETosis may be effective in some diseases, especially those with relatively slow neutrophil activation. In future experiments, in order to realize the effect of (+)-borneol in vivo, it should be administered before neutrophil activation.
Currently, DNase is the most frequently used inhibitor of NETs (Jorch and Kubes, 2017). It can eliminate DNA, which is the framework of NETs, and the proteins attached in NETs are then released. Thus, the detrimental effect of these proteins cannot be neutralized. As shown in our study, (+)-borneol inhibits ROS generation and NETosis of neutrophils triggered by PMA stimulation. However, the effect of (+)-borneol on NETosis in vivo and the specific mechanism await further study.
TLRs located on the cell surface and in endosomes are the first pattern-recognition receptors to be identified (Baral et al., 2014). They detect numerous damage-associated molecular patterns and mediate innate immune responses. TLR2 and TLR4 are two vital members of the neutrophil TLRs (Prince et al., 2011). NETosis can be induced by bacteria, parasites, or lipopolysaccharides (infectious stimuli) via TLR2 and/or TLR4 in a few minutes (Jorch and Kubes, 2017). However, the roles TLR2/4 played in the process of PMA-induced NETosis are not clear. In this study, we show that inhibiting TLR4 has no effect on PMA-induced NETosis, which is in line with the previous study (Khan et al., 2017). However, it is interesting that inhibiting TLR2 results in an elevated level of NETosis induced by PMA. Hitherto, TLR2 has not engaged much attention in the process of PMA-induced NETosis. This study indicates that the role of TLR2 in NETosis may be mysterious, and future studies should pay more attention to it because we have not found a reasonable explanation.
There are some limitations to our study. First, we showed that (+)-borneol can inhibit ROS generation of neutrophils, but the exact target of (+)-borneol in neutrophils during NETosis is still unclear. Second, NETs can be induced by many stimuli; we only stimulated neutrophils with PMA. Other stimuli such as lipopolysaccharide (LPS), A23187, and the immune complex can also induce NETosis, and the mechanisms involved are also different. The effect of (+)-borneol on NETosis induced by other stimuli should be studied in the future. However, PMA is a robust NET inducer that has been used originally and widely in studies about NETosis (Brinkmann et al., 2004; Jorch and Kubes, 2017; Kenny et al., 2017). PMA, (auto) antibodies and cholesterol crystals elicit similar pathways in NETosis (Jorch and Kubes, 2017). PMA stimulation can induce suicidal NETosis in vitro which can be found in many noninfectious diseases. The purpose of our study is to explore the effect of (+)-borneol on suicidal NETosis. Thus, we selected PMA as the stimulant. Third, according to the Chinese Pharmacopoeia, there are three borneol products: (+)-borneol, (−)-borneol, and (±) borneol. The functions of these products are similar but not identical. However, only the function of (+)-borneol was evaluated in this study. Therefore, our results may not be applicable to other borneol products.
In summary, our study shows for the first time that (+)-borneol inhibits ROS generation and NETosis of neutrophils triggered by PMA stimulation in vitro. This finding indicates that (+)-borneol therapy targeting NETosis may be effective in some diseases.
The original contributions presented in the study are included in the article/Supplementary Material; further inquiries can be directed to the corresponding authors.
The studies involving human participants were reviewed and approved by the Medical Ethical Committee of Sir Run Run Shaw Hospital. The patients/participants provided their written informed consent to participate in this study.
XH and LW designed the study. QT and LN collected the blood samples. HC, XX, QT, and LN performed the experiments. SC and YH analyzed the results. HC and XX wrote the manuscript. All the authors have read and approved the final manuscript.
The study was funded by the Chinese Stroke Association (No. 2020017) and the Natural Science Foundation of Zhejiang Province of China (LY19H090016).
The authors declare that the research was conducted in the absence of any commercial or financial relationships that could be construed as a potential conflict of interest.
All claims expressed in this article are solely those of the authors and do not necessarily represent those of their affiliated organizations, or those of the publisher, the editors, and the reviewers. Any product that may be evaluated in this article, or claim that may be made by its manufacturer, is not guaranteed or endorsed by the publisher.
Albrengues, J., Shields, M. A., Ng, D., Park, C. G., Ambrico, A., Poindexter, M. E., et al. (2018). Neutrophil extracellular traps produced during inflammation awaken dormant cancer cells in mice. Science 361 (6409), eaao4227. doi:10.1126/science.aao4227
Bainton, D. F., Ullyot, J. L., and Farquhar, M. G. (1971). The development of neutrophilic polymorphonuclear leukocytes in human bone marrow. J. Exp. Med. 134 (4), 907–934. doi:10.1084/jem.134.4.907
Baral, P., Batra, S., Zemans, R. L., Downey, G. P., and Jeyaseelan, S. (2014). Divergent functions of Toll-like receptors during bacterial lung infections. Am. J. Respir. Crit. Care Med. 190 (7), 722–732. doi:10.1164/rccm.201406-1101PP
Bianchi, M., Hakkim, A., Brinkmann, V., Siler, U., Seger, R. A., Zychlinsky, A., et al. (2009). Restoration of NET formation by gene therapy in CGD controls aspergillosis. Blood 114 (13), 2619–2622. doi:10.1182/blood-2009-05-221606
Brinkmann, V., Reichard, U., Goosmann, C., Fauler, B., Uhlemann, Y., Weiss, D. S., et al. (2004). Neutrophil extracellular traps kill bacteria. Science 303 (5663), 1532–1535. doi:10.1126/science.1092385
Brinkmann, V., and Zychlinsky, A. (2007). Beneficial suicide: Why neutrophils die to make NETs. Nat. Rev. Microbiol. 5 (8), 577–582. doi:10.1038/nrmicro1710
Chen, J., Li, L., Su, J., Li, B., Chen, T., and Wong, Y. S. (2014). Synergistic apoptosis-inducing effects on A375 human melanoma cells of natural borneol and curcumin. PLoS One 9 (6), e101277. doi:10.1371/journal.pone.0101277
Chen, J., Li, L., Su, J., Li, B., Zhang, X., and Chen, T. (2015). Proteomic analysis of G2/M arrest triggered by natural borneol/curcumin in HepG2 cells, the importance of the reactive oxygen species-p53 pathway. J. Agric. Food Chem. 63 (28), 6440–6449. doi:10.1021/acs.jafc.5b01773
Döring, Y., Libby, P., and Soehnlein, O. (2020). Neutrophil extracellular traps participate in cardiovascular diseases: Recent experimental and clinical insights. Circ. Res. 126 (9), 1228–1241. doi:10.1161/circresaha.120.315931
Fuchs, T. A., Abed, U., Goosmann, C., Hurwitz, R., Schulze, I., Wahn, V., et al. (2007). Novel cell death program leads to neutrophil extracellular traps. J. Cell Biol. 176 (2), 231–241. doi:10.1083/jcb.200606027
Fuchs, T. A., Brill, A., Duerschmied, D., Schatzberg, D., Monestier, M., Myers, D. D., et al. (2010). Extracellular DNA traps promote thrombosis. Proc. Natl. Acad. Sci. U. S. A. 107 (36), 15880–15885. doi:10.1073/pnas.1005743107
Grayson, P. C., and Kaplan, M. J. (2016). At the Bench: Neutrophil extracellular traps (NETs) highlight novel aspects of innate immune system involvement in autoimmune diseases. J. Leukoc. Biol. 99 (2), 253–264. doi:10.1189/jlb.5BT0615-247R
Gupta, S., and Kaplan, M. J. (2016). The role of neutrophils and NETosis in autoimmune and renal diseases. Nat. Rev. Nephrol. 12 (7), 402–413. doi:10.1038/nrneph.2016.71
Hakkim, A., Fuchs, T. A., Martinez, N. E., Hess, S., Prinz, H., Zychlinsky, A., et al. (2011). Activation of the Raf-MEK-ERK pathway is required for neutrophil extracellular trap formation. Nat. Chem. Biol. 7 (2), 75–77. doi:10.1038/nchembio.496
Hakkim, A., Fürnrohr, B. G., Amann, K., Laube, B., Abed, U. A., Brinkmann, V., et al. (2010). Impairment of neutrophil extracellular trap degradation is associated with lupus nephritis. Proc. Natl. Acad. Sci. U. S. A. 107 (21), 9813–9818. doi:10.1073/pnas.0909927107
Huang, J., Wang, Z., Wang, S., Guo, H., Chai, L., Hu, L., et al. (2020). Protection of active pharmaceutical ingredients in the Xingnaojing Injection against oxygen glucose deprivation/re - oxygenation - induced PC12 cells injury. Chin. J. Clin. Pharmacol. 36 (10), 1337–1340. doi:10.13699/j.cnki.1001-6821.2020.10.045
Hur, J., Pak, S. C., Koo, B. S., and Jeon, S. (2013). Borneol alleviates oxidative stress via upregulation of Nrf2 and Bcl-2 in SH-SY5Y cells. Pharm. Biol. 51 (1), 30–35. doi:10.3109/13880209.2012.700718
Jorch, S. K., and Kubes, P. (2017). An emerging role for neutrophil extracellular traps in noninfectious disease. Nat. Med. 23 (3), 279–287. doi:10.1038/nm.4294
Kenny, E. F., Herzig, A., Krüger, R., Muth, A., Mondal, S., Thompson, P. R., et al. (2017). Diverse stimuli engage different neutrophil extracellular trap pathways. Elife 6, e24437. doi:10.7554/eLife.24437
Khan, M. A., Farahvash, A., Douda, D. N., Licht, J. C., Grasemann, H., Sweezey, N., et al. (2017). JNK activation turns on LPS- and gram-negative bacteria-induced NADPH oxidase-dependent suicidal NETosis. Sci. Rep. 7 (1), 3409. doi:10.1038/s41598-017-03257-z
Lai, H., Liu, C., Hou, L., Lin, W., Chen, T., and Hong, A. (2020). TRPM8-regulated calcium mobilization plays a critical role in synergistic chemosensitization of Borneol on Doxorubicin. Theranostics 10 (22), 10154–10170. doi:10.7150/thno.45861
Ley, K., Hoffman, H. M., Kubes, P., Cassatella, M. A., Zychlinsky, A., Hedrick, C. C., et al. (2018). Neutrophils: New insights and open questions. Sci. Immunol. 3 (30), eaat4579. doi:10.1126/sciimmunol.aat4579
Li, Y. C., Li, Y., Zhang, Y. N., Zhao, Q., Zhang, P. L., Sun, M. R., et al. (2022). Muscone and (+)-Borneol cooperatively strengthen CREB induction of claudin 5 in IL-1β-induced endothelium injury. Antioxidants (Basel) 11 (8), 1455. doi:10.3390/antiox11081455
Li, Y., Ren, M., Wang, J., Ma, R., Chen, H., Xie, Q., et al. (2021). Progress in borneol intervention for ischemic Stroke: A systematic review. Front. Pharmacol. 12, 606682. doi:10.3389/fphar.2021.606682
Liu, R., Zhang, L., Lan, X., Li, L., Zhang, T. T., Sun, J. H., et al. (2011). Protection by borneol on cortical neurons against oxygen-glucose deprivation/reperfusion: Involvement of anti-oxidation and anti-inflammation through nuclear transcription factor κappaB signaling pathway. Neuroscience 176, 408–419. doi:10.1016/j.neuroscience.2010.11.029
Liu, S., Long, Y., Yu, S., Zhang, D., Yang, Q., Ci, Z., et al. (2021). Borneol in cardio-cerebrovascular diseases: Pharmacological actions, mechanisms, and therapeutics. Pharmacol. Res. 169, 105627. doi:10.1016/j.phrs.2021.105627
Lood, C., Blanco, L. P., Purmalek, M. M., Carmona-Rivera, C., De Ravin, S. S., Smith, C. K., et al. (2016). Neutrophil extracellular traps enriched in oxidized mitochondrial DNA are interferogenic and contribute to lupus-like disease. Nat. Med. 22 (2), 146–153. doi:10.1038/nm.4027
Mayadas, T. N., Cullere, X., and Lowell, C. A. (2014). The multifaceted functions of neutrophils. Annu. Rev. Pathol. 9, 181–218. doi:10.1146/annurev-pathol-020712-164023
Olsson, A. K., and Cedervall, J. (2016). NETosis in cancer - platelet-neutrophil crosstalk promotes tumor-associated pathology. Front. Immunol. 7, 373. doi:10.3389/fimmu.2016.00373
Papayannopoulos, V. (2018). Neutrophil extracellular traps in immunity and disease. Nat. Rev. Immunol. 18 (2), 134–147. doi:10.1038/nri.2017.105
Pilsczek, F. H., Salina, D., Poon, K. K., Fahey, C., Yipp, B. G., Sibley, C. D., et al. (2010). A novel mechanism of rapid nuclear neutrophil extracellular trap formation in response to Staphylococcus aureus. J. Immunol. 185 (12), 7413–7425. doi:10.4049/jimmunol.1000675
Prince, L. R., Whyte, M. K., Sabroe, I., and Parker, L. C. (2011). The role of TLRs in neutrophil activation. Curr. Opin. Pharmacol. 11 (4), 397–403. doi:10.1016/j.coph.2011.06.007
Saitoh, T., Komano, J., Saitoh, Y., Misawa, T., Takahama, M., Kozaki, T., et al. (2012). Neutrophil extracellular traps mediate a host defense response to human immunodeficiency virus-1. Cell Host Microbe 12 (1), 109–116. doi:10.1016/j.chom.2012.05.015
Schulz, A., Pagerols Raluy, L., Kolman, J. P., Königs, I., Trochimiuk, M., Appl, B., et al. (2020). The inhibitory effect of Curosurf(®) and Alveofact(®) on the formation of neutrophil extracellular traps. Front. Immunol. 11, 582895. doi:10.3389/fimmu.2020.582895
Sur Chowdhury, C., Giaglis, S., Walker, U. A., Buser, A., Hahn, S., and Hasler, P. (2014). Enhanced neutrophil extracellular trap generation in rheumatoid arthritis: Analysis of underlying signal transduction pathways and potential diagnostic utility. Arthritis Res. Ther. 16 (3), R122. doi:10.1186/ar4579
Thiam, H. R., Wong, S. L., Wagner, D. D., and Waterman, C. M. (2020). Cellular mechanisms of NETosis. Annu. Rev. Cell Dev. Biol. 36, 191–218. doi:10.1146/annurev-cellbio-020520-111016
Urban, C. F., Reichard, U., Brinkmann, V., and Zychlinsky, A. (2006). Neutrophil extracellular traps capture and kill Candida albicans yeast and hyphal forms. Cell. Microbiol. 8 (4), 668–676. doi:10.1111/j.1462-5822.2005.00659.x
Xu, J., Wang, A., Meng, X., Yalkun, G., Xu, A., Gao, Z., et al. (2021). Edaravone dexborneol versus edaravone alone for the treatment of acute ischemic Stroke: A phase III, randomized, double-blind, comparative trial. Stroke 52 (3), 772–780. doi:10.1161/strokeaha.120.031197
Yipp, B. G., Petri, B., Salina, D., Jenne, C. N., Scott, B. N., Zbytnuik, L. D., et al. (2012). Infection-induced NETosis is a dynamic process involving neutrophil multitasking in vivo. Nat. Med. 18 (9), 1386–1393. doi:10.1038/nm.2847
Keywords: neutrophil extracellular traps, borneol, reactive oxygen species, antioxidant, inflammation
Citation: Chen H, Xu X, Tang Q, Ni L, Cao S, Hao Y, Wang L and Hu X (2022) (+)-Borneol inhibits the generation of reactive oxygen species and neutrophil extracellular traps induced by phorbol-12-myristate-13-acetate. Front. Pharmacol. 13:1023450. doi: 10.3389/fphar.2022.1023450
Received: 07 September 2022; Accepted: 19 October 2022;
Published: 07 November 2022.
Edited by:
Barbara Rossi, University of Verona, ItalyReviewed by:
Lindsay Marshall, Humane Society of the United States, United StatesCopyright © 2022 Chen, Xu, Tang, Ni, Cao, Hao, Wang and Hu. This is an open-access article distributed under the terms of the Creative Commons Attribution License (CC BY). The use, distribution or reproduction in other forums is permitted, provided the original author(s) and the copyright owner(s) are credited and that the original publication in this journal is cited, in accordance with accepted academic practice. No use, distribution or reproduction is permitted which does not comply with these terms.
*Correspondence: Li Wang, d2FuZ2xpMTk4MEB6anUuZWR1LmNu; Xingyue Hu, aHV4aW5neXVlMjAwM0B6anUuZWR1LmNu
†These authors have contributed equally to this work
Disclaimer: All claims expressed in this article are solely those of the authors and do not necessarily represent those of their affiliated organizations, or those of the publisher, the editors and the reviewers. Any product that may be evaluated in this article or claim that may be made by its manufacturer is not guaranteed or endorsed by the publisher.
Research integrity at Frontiers
Learn more about the work of our research integrity team to safeguard the quality of each article we publish.