- Institute of Chinese Material Medica, China Academy of Chinese Medical Sciences, Beijing, China
Danlu tongdu tablets (DLTD) is a listed Chinese patent medicine collected in the Pharmacopoeia of the People’s Republic of China (version 2020). This prescription has been applied in clinics in China for lumbar spinal stenosis and lumbosacral disc herniations. The wide application of Danlu tongdu in therapy has raised some clinical adverse reactions, such as significant elevation of alanine transaminase (ALT) and aspartate transaminase (AST) in individual patients after use. The present study aimed to investigate the safety of Danlu tongdu and analyze its adverse effects on the liver. The maximum feasible dose (MFD) was used to carry out the acute toxicity tests. Mortality, adverse effects, body weight and food consumption were recorded for up to 14 days post treatment. In the 6-month chronic toxicity test, sprague-dawley rats were randomly divided into four groups according body weight, the experimental groups were administrated to rats at the concentrations of 1.67, 3.34 and 6.67 g/kg/day, whereas the control group was received the ultrapure water (vehicle) only, 10 ml/kg, once a day. The animal’s body weight, food consumption was monitored weekly. In addition, their hematological and biochemical parameters, body and organ weights and histopathology, were all measured at specific observation time points. Additionally, we further explored the adverse effects mechanism of Danlu tongdu on the liver through transcriptome analysis. No deaths or substance-relative toxicity were observed in the acute toxicity study or the 6-month chronic toxicity study with doses of 1.67 g/kg and 3.34 g/kg, respectively. We found that mild hypertrophy and hyperplasia of hepatic interlobular bile ducts were detected in some rats with doses of 6.67 g/kg after repeated oral administration of Danlu tongdu for 13 and 26 weeks, but the above changes in liver were reversible. The results of transcriptome sequencing showed that Danlu tongdu had a significant effect on cytochrome P450 enzymes in rat liver, especially cytochrome P450 1 (CYP1) subtype. Therefore, the toxic target organ of Danlu tongdu is the liver and the mechanism of mild liver injury is closely related to the up-regulation of cytochrome P450 1A1 (CYP1A1) and cytochrome P450 1A2 (CYP1A2) expression.
1 Introduction
Danlu tongdu tablets (DLTD), a listed Chinese patent medicine consisting of five traditional Chinese drugs, include Salviae miltiorrhizae, Astragali radix, Eucommiae cortex, Corydalis rhizoma and Cervi cornus colla (Li et al., 2013; Li and Zhao, 2013; Commission, 2020). This prescription has the functions of promoting blood circulation, removing obstruction in channels, tonifying kidney and supplementing qi, which is used in clinical treatment of lumbar spinal stenosis with pattern of qi deficiency and blood stasis, lumbosacral disc herniations, intermittent claudication, pain in waist and leg, limitation of movement, acid distension in lower limbs, etc. The clinical effect is remarkable(Pan, 2010; Guo, 2011a; Guo, 2011b; Huang, 2012; Liu et al., 2012; Zhou, 2012; Chen, 2015; Duo, 2015; Yan et al., 2019; Wei, 2022).
Cervi cornus colla in DLTD has the function of enriching blood and nourishing essence. Pharmacological experiments have proved that it has many functions, such as anti-inflammation and analgesia, tonifying blood and promoting blood circulation, anti-osteoporosis, promoting cartilage and osteoblast proliferation (Lu et al., 2021). Salviae miltiorrhizae in DLTD has the function of promoting blood circulation and resolving stasis and the chemical components were mainly classified as lipid-soluble compounds tanshinones and water-soluble compounds salvianolic acids. The pharmacological studies, including anti-platelet aggregation, anti-thrombosis, improve hemorheology, improve microcirculation, anti-inflammatory, lipid metabolism, have been widely performed (Fan et al., 2010; Wu et al., 2018). The main pharmacodynamic material base of Corydalis rhizoma is the total alkaloid. The pharmacological activity studies showed that Corydalis rhizoma has good sedative, analgesic, antiarrhythmic and antihypertensive effects (Feng et al., 2018). The chemical components of Eucommiae cortex mainly included lignans, iridoids, phenylpropanoids, flavonoids and other active ingredients. Modern pharmacological actions included regulating bone metabolism, tonifying kidney, enhancing immunity function, and so on (Wang et al., 2017). The Astragali radix is mainly composed of polysaccharides, triterpenes and flavonoids. It has pharmacological effects such as enhancing and regulating body immunity, anti-aging and anti-fatigue (Zhang et al., 2012). The whole prescription changes the microcirculation of the lesion site, protects the compressed spinal cord from damage, and promotes the functional recovery of the injured nerve.
With the widespread use of DLTD, 4 cases of drug-induced liver injury caused by DLTD have been reported, including 1 case in 2011 (Chen, 2011), 1 case in 2017 (Zhao and Wang, 2017) and 2 cases in 2018 (Zhang and Leng, 2018). All patients had nausea, general fatigue, pathological increase of liver function indexes such as alanine aminotransferase (ALT), aspartate transaminase (AST), alkaline phosphatase (ALP), total bilirubin (TBIL) and direct bilirubin (DBIL), and all indexes of liver function recovered after drug withdrawal. In the drug description, the adverse reaction only indicates that “rash occurs in individual patients”, and does not indicate that long-term application may have potential liver function damage. In addition, there are no reports on the preclinical safety evaluation and hepatotoxicity of DLTD. In order to further determine the safety of DLTD in clinical use, especially whether it has adverse effects on the liver, we evaluated DLTD from three aspects: liver damage marker detection, histopathological examination and transcriptomic analysis, hoping to obtain more information and provide certain data for the safety of clinical use.
2 Materials and methods
2.1 Test substances
Danlu tongdu tablets are the listed Chinese patent medicine collected in the Pharmacopoeia of the People’s Republic of China (version 2020, volume 1, page 717–718). In order to facilitate the preparation of the drug liquid, the test substances were the powder of Danlu tongdu tablets without magnesium stearate and other auxiliary materials. The powder of Danlu tongdu tablets (batch No. 200303–04) was supplied by Henan Lingrui Pharmaceutical Co., Ltd. (Xinyang, Henan, China) and prepared as follows: 1,500 kg of Astragali radix [Leguminosae; Astragalus membranaceus (Fisch.) Bge.var.mongholicus (Bge.) Hsiao; Astragalus membranaceus (Fisch.) Bge.] and 1,500 kg of Eucommiae cortex (Eucommiaceae; Eucommia ulmoides Oliv.) were boiled with water for 3 times, 1 h each time, combined the water decoction, filtered, filtered liquid concentrated to the right amount; 501 kg of Cervi cornus colla (a gelatin made of deer antlers by boiling and concentrating process) melted by heat; 1,500 kg of Salviae miltiorrhizae (Labiatae; Salvia miltiorrhiza Bge.) and 999 kg of Corydalis rhizome (Papaveraceae; Corydalis yanhusuo W.T.Wang) were refluxed with 70% ethanol for 3 times, 1 h each time, the extracting solution was merged, filtered, and the filtrate was concentrated to an appropriate amount under reduced pressure; finally, the above-mentioned ointment was combined, dried and crushed. The relative share of Astragali radix, Eucommiae cortex, Salviae miltiorrhizae, Corydalis rhizome, Cervi cornus colla are 3:3:3:2:1. The drug extract ratio was 24.2% and per Gram of powder was equivalent to 4.11 g of crude drug. The moisture content of the powder was 3.3%. Thin-layer chromatography was used for differentiating the Salviae miltiorrhizae, Astragali radix and Corydalis rhizome in the prescription. The content of astragaloside per Gram of powder was 0.91 mg.
2.2 Experimental animals
40 specific pathogen-free (SPF) ICR mice (half of each sex) were obtained from Beijing Vital River Laboratory Animal Technology Co., Ltd. (Beijing, China) with the confirmation number SCXK (Jing) 2016–0006. Mice were housed in polypropylene cages at an ambient temperature of 23°C (±3°C) and 40–70% relative humidity, with a 12:12 h light/dark cycle. Animals were provided with commercial food pellets and water ad libitum unless stated otherwise. The animals were allowed 3 days of acclimatization before the commencement of experimental procedures.
200 SPF rats (100 male and 100 female) were obtained from Beijing Vital River Laboratory Animal Technology Co., Ltd. (Beijing, China) with the confirmation number SCXK (Jing) 2016–0006. Rats were housed in polypropylene cages at an ambient temperature of 23°C (±3°C) and 40–70% relative humidity, with a 12:12 h light/dark cycle. Animals were provided with commercial food pellets and water ad libitum unless stated otherwise. The animals were allowed 7 days of acclimatization before the commencement of experimental procedures.
The animal experiments were conducted at Traditional Chinese Medicine Safety Evaluation Center, Institute of Chinese Materia Medica China Academy of Chinese medical science (Beijing, China), according to good laboratory practice (GLP) guidelines, and were authorized by Committee of Welfare and Ethics for Experimental Animals. The approval numbers for acute toxicity study and chronic toxicity studies were 2020A023 of and 2020A028, respectively.
2.3 Safety evaluation of Danlu tongdu tablets
2.3.1 Acute toxicity study
For the acute toxicity study, we followed the technical guidelines for single dose toxicity study of pharmaceuticals published by the National Medical Products Administration (NMPA, 13 May 2014). The maximum feasible dose (MFD) was used to carry out the acute toxicity tests. The concentration of DLTD solution was 0.89 g/ml, which is the maximum concentration that could be given to mice by gavage. The dose volume was 40 ml/kg, once within 24 h. Therefore, the dose of DLTD given to each mice was 35.60 g/kg, and was equivalent to 427 times of the proposed clinical dose. 40 mice were randomly divided into two groups of 20 (equally divided by sex): control group (treated with ultrapure water) and DLTD treated group (35.60 g/kg). All mice were fasted overnight prior to the intragastric administration. The mice were closely monitored for 2 h after the dose administration, both in the morning and afternoon. The day when the mice were exposed to the test substance was recorded as D0. General health observations (daily), symptoms of poisoning (daily) and mortality (daily) were monitored and registered for 2 weeks. Body weights and food consumption were measured after administration on days 1, 2, 3, 5, 7, 10 and 14. The observation period of 2 week’s post treatment was recorded as D1 to D14. The mice were sacrificed by cervical dislocation and examined for histopathological changes at D14.
2.3.2 6-Month chronic toxicity study
The 6-month chronic toxicity of DLTD was evaluated according to the technical guidelines for repeated dose toxicity study of pharmaceuticals (NMPA, 13 May 2014). 200 rats were randomly divided into four groups of 50 (half of each sex): control group (treated with ultrapure water), low-dose group (treated with 1.67 g/kg DLTD), medium-dose group (treated with 3.34 g/kg DLTD) and high-dose group (treated with 6.67 g/kg DLTD). Rats were given the above dose of liquid medicine by gavage every morning for 6 months and the corresponding period was recorded as D1-D183. In addition, there was a 4-week observation period after the final administration which was recorded as rD1 to rD29. General health observations were conducted daily, and the weights and food consumption for each survival rat were recorded twice per week for the first 2 weeks, and then once per week. The volume of DLTD was adjusted according to the weights of the rats.
In the early stages of administration period (D28), 10 rats (half of each sex) among each group were fasted for overnight, and their urine samples were collected. On the next day (D29), 40 rats were subjected general anesthesia by intramuscular injection of zoletil®50 at a dose of 40 mg/kg in male rats or 30 mg/kg in female rats. After anesthesia, blood was collected from the abdominal aorta until the animals died. And the collected blood samples were applied to hematologic analysis (EDTA-K2 tubes), biochemistry analysis (dry tubes) and coagulation tests (sodium citrate tubes). Vital organs, including the heart, liver, kidney and so on, were excised, weighed and fixed in 10% formaldehyde solution for histopathological examination after euthanasia. The femur marrow was used for the bone marrow smear. Similarly, rats were sacrificed and examined at the middle of the administration period (D92, 20 male and 20 female), at the end of the administration period (D182, 40 male; D183, 40 female) and the end of the recovery period (rD29, 20 male and 20 female), respectively.
2.3.2.1 Hematologic analysis
For the hematologic analysis, the samples were collected in EDTA-K2 tubes and analyzed on the day of preparation. The red blood cell count (RBC), hemoglobin (HGB), hematocrit level (HCT), mean corpuscular volume (MCV), mean corpuscular hemoglobin (MCH), mean corpuscular hemoglobin concentration (MCHC), white blood cell count (WBC), neutrophil ratio (NEUT%), lymphocyte ratio (LYM%), monocyte ratio (MONO%), eosinophil ratio (EOS%), basophil ratio (BASO%), platelet count (PLT), and reticulocyte ratio (RET%) were examined using an sysmex XN-1000 automated hematology analyzer (Sysmex, Japan). Samples collected with sodium citrate-containing tubes were used for the coagulation test. The prothrombin time (PT) and activated partial thromboplastin time (APTT) were measured using a ACL TOP 500 automatic coagulation analyzer (Werfen, Spain).
2.3.2.2 Blood biochemistry analysis
For the blood biochemistry analysis, the blood samples collected with dry tubes were rested for 30 min at room temperature. Then the samples were centrifuged at 3,000 rpm for 15 min and the supernatant was carefully transferred to another cleaning tube. Creatine kinase (CK), creatine kinase MB isoenzyme (CK-MB), lactic de-hydrogenase (LDH), alanine transaminase (ALT), aspartate transaminase (AST), alkaline phosphatase (ALP), γ-glutamyltransferase (GGT), total bilirubin (TBIL), total protein (TP), albumin (ALB), creatinine (CRE), urea nitrogen (UREA), glucose (GLU), total cholesterol (CHOL) and triglycerides (TG) were detected using a toshiba TBA-120FR analyzer (Toshiba, Japan), while potassium (K+), sodium (Na+), chloride (Cl−) were detected using a medica Easylyte analyzer (Medica, United States).
2.3.3.3 Histological analysis
The fixed organs were dehydrated in gradient ethanol, vitrified, and embedded in paraffin. Sections (3 μm thick) were cut with an RM2135 rotary microtome sinica (Leica, Germany) and stained with hematoxylin and eosin. Histological observations were performed using olympus BX51 microscope (Olympus, Japan) at 200 magnification to evaluate the degree of pathological alteration. Pathological evaluation of the tissue sections was performed by an experienced pathologist.
2.4 RNA sequencing assay
At the end of administration, four male rats were selected from the control group and the high-dose group respectively for transcriptome analysis. The selection criteria were random selection from the control group, the four rats selected from the high-dose group showed pathological changes in the liver. For the whole transcriptome analysis, total RNA were extracted from the liver isolated from the control group or high-dose group rats at the end of the administration period. Each group of the RNA-sequencing assay used four samples. The RNA-sequencing assay included the cDNA library construction, library purification, transcriptome sequencing and GO and KEGG enrichment analyses. All experiments were finished according to the protocols of the Tianjin Novogene Bioinformatic Technology Company’s Instructions. GO and KEGG enrichment analyses revealed the functional roles of differentially expressed genes (DEGs). The threshold was 0.05 to test the hypergeometric distribution of the default enrichment results.
2.5 Quantitative real-time PCR
At the end of the administration, 10 male rats in the control group and 10 male rats in the high dose group were used for quantitative real-time PCR and western blot detection below, and the sample numbers were set as control 1–10 and DLTD 11–20. The total RNA of the liver was extracted with trizol reagent. The revert aid first strand cDNA synthesis kit (Thermo) was used to reverse-transcribe RNA to cDNA. quantitative real-time PCR was performed with the GoTaq® qPCR master mix (Promega). The beta-actin value was used as the normalized expression control. The sequences of primers are shown in Table 1.
2.6 Western blot
Proteins were obtained from tissue lysates with the RIPA buffer. The bicinchoninic acid (BCA) assay kit was used to determine the concentration of the protein. The total lysates were loaded and separated via Tris-SDS PAGE and then transferred to polyvinylidene difluoride (PVDF) membranes. After blocking with 5% fat-free milk powder, specific primary antibodies were incubated with PVDF membranes overnight. Then, HRP-conjugated secondary antibody was added, and the expression of the protein was examined with the ECL plus reagents.
2.7 Statistical analysis
The experimental data were analyzed using SPSS 20.0 statistical software, and the data were expressed as mean ± standard deviation (SD). The weekly body weight and food consumption were analyzed by repeated measure one-way analysis of variance (ANOVA). The hematological indicators, serum biochemical indicators, visceral body ratio coefficient and other indicators were assessed by ANOVA, according to the results of homogeneity test of variance, Dunnett method is used for homogeneous variance, and Dunnett’s T3 method is used for uneven variance. p-values less than 0.05 were considered to be significant.
3 Results
3.1 Acute toxicity study of Danlu tongdu tablets
After 14-day observation period, no deaths occurred in mice and the major adverse reaction was transient diarrhea with the DLTD treatment, the incidence of diarrhea was 70% (♂9/10♀5/10). Body weight and food consumption was measured at D1, D2, D3, D5, D7, D10 and D14 after administration. Only on the first day after administration, the body weight and food consumption of female animals decreased significantly, and there was no statistically significant difference between the DLTD-treated and non-treated groups at other time points (Figures 1A–E). This transient change may be related to the thicker and larger volume of liquid medicine. At the end of the acute administration evaluation, pathological examination showed no obvious lesions or abnormalities in the volume, color, or texture of isolated organs due to the treatment with DLTD (data not shown).
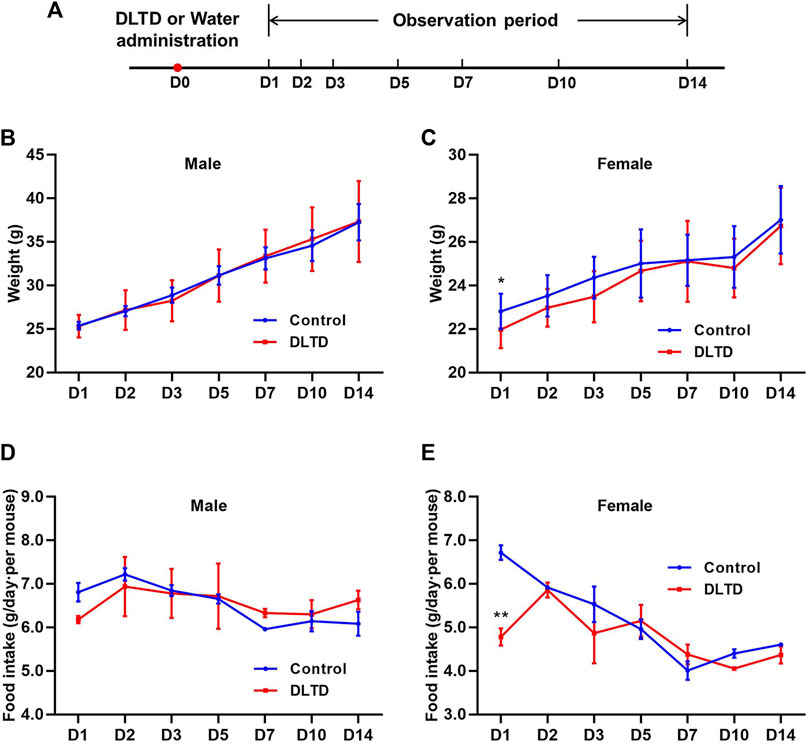
FIGURE 1. Body weight and food consumption of mice in acute toxicity studies. (A) Timeline of the acute toxicity study. Weights of male mice (B) and female mice (C) in the acute toxicity study. Food consumption of male mice (D) and female mice (E) in the acute toxicity study. Statistical analysis was performed using the t-test. *p < 0.05, **p < 0.01 versus the control.
3.2 Chronic toxicity study of Danlu tongdu tablets
We performed ophthalmology and urine examinations on rats at the mid-term administration period (D91), the end of the administration period (D181 and D182) and the recovery period (rD28), and the hematology, blood biochemical assay and histopathological examinations were performed at D29, D92, D182, D183, and rD29 (Figure 2A). From the chronic toxicity testing, there was no abnormal clinical signs or deaths occurred in rats with the DLTD treatment.
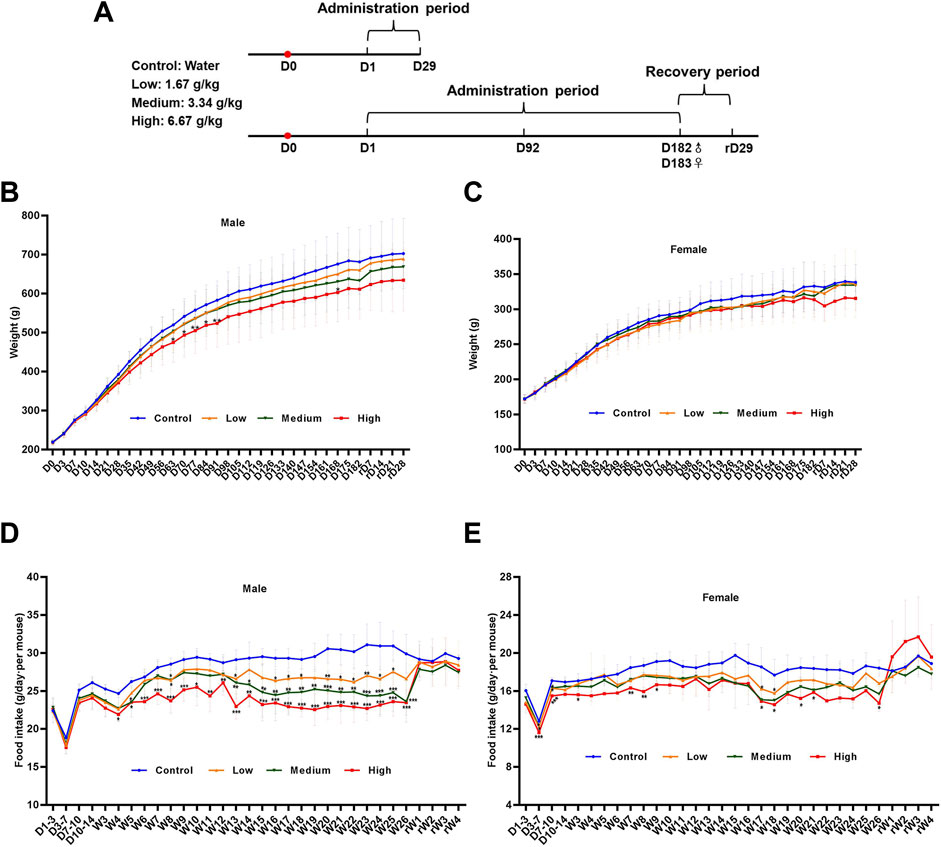
FIGURE 2. Body weight and food consumption of rats in chronic toxicity studies. (A) Timeline of the 6-month chronic toxicity study. Weights of male rats (B) and female rats (C) in the 6-month chronic toxicity study. Food consumption of male rats (D) and female rats (E) in the 6-month chronic toxicity study. Statistical analysis was performed using the one-way ANOVA. *p < 0.05, **p < 0.01, ***p < 0.001 versus the control.
3.2.1 Body weight and food consumption
As expected, the rats gained weight with time during the administration and recovery period. In the male rats, body weights decreased significantly in the high-dose group on days 63, 70, 77, 84, 91 and 168 compared with that in the control group. At the same time, body weights showed no significant differences in the low- and mid-dose group. In the female rats, there was no significant difference between the low, medium, high-dose group and the control group. Body weight curves for male and female rats are shown in Figures 2B,C. Although statistically significant intergroup differences in mean food consumption were seen in different time, which could be considered as a normal change because rats were administered 15 ml/kg liquid medicine twice within a day (Figures 2D,E).
3.2.2 Hematology
Hematological changes were evaluated five times: on Day 29, Day 92, Day 182 and Day 183 of the study and D29 after recovery. The effect of oral administration DLTD on hematological parameters compared with control group is presented in Table 2. In hematological parameters, a significant increase in platelet count was observed in low-dose (p < 0.05), in mid-dose (p < 0.05) and in high-dose males (p < 0.01) on Day 29. At the same time, reticulocyte ratio were significantly increased in high-dose group (p < 0.01). On Day 92 significant increase were observed in hematocrit level in low-dose (p < 0.05), in mid-dose (p < 0.05) and in high-dose males (p < 0.01), meanwhile, in hemoglobin in low-dose (p < 0.01) and in mid-dose males (p < 0.05). Red blood cell count was also elevated in mid-dose (p < 0.05) and high-dose males (p < 0.01) on Day 182. In high-dose females, hemoglobin were significantly increased after 4-week recovery (p < 0.05). There was no abnormality in hematological parameters of male rats in each dose group in the recovery period.
In addition, significant decreases were observed in neutrophil ratio in the low-dose females (p < 0.05) on Day 29, and in mid-dose (p < 0.05) and high-dose(p < 0.05) females on Day 183. There was no significant difference in other hematological parameters between the DLTD-treated and the control groups. Above parameters remained within the physiological range throughout the 30-week experimental period. There is no toxicological significance in the clinical hematology in both sexes from the each dose group.
3.2.3 Blood chemistry
The effect of oral administration of DLTD on blood chemistry is presented in Table 3. The serum glucose were significantly decreased in low-dose (p < 0.05) and mid-dose males (p < 0.05), and the serum triglycerides were significantly decreased in mid-dose males (p < 0.05) on Day 92. On Day 182 significant decrease were observed in serum urea nitrogen in low-dose males (p < 0.01), and in serum potassium in low-dose males (p < 0.05). In high-dose females, total bilirubin were significantly decreased, while serum glucose were significantly increased on day 183. There was no significant difference in other biochemistry parameters between the DLTD-treated and the control groups. Above parameters remained within the physiological range and there was neither dose response relationship nor time response relationship throughout the 30-week experimental period. There is no toxicological significance in the clinical blood chemistry in both sexes from the each dose group.
3.2.4 Organ weight and histopathological examination
Relative organ weights (organ weight/brain weight) of the 30-week treated rats are shown in Table 4. At the mid of the treatment with DLTD, the relative kidney weights in high-dose males were significantly increased in comparison to those in control males. The relative weight change of kidney was not considered of toxicological significance since this change had neither dose response and time response relationship nor consistent histopathological changes of corresponding organs, but was strongly associated with low fasting weight. At the end of the treatment and recovery period, no significance changes were observed in the relative organ weights in males and females.
After repeated oral administration of DLTD for 13 and 26 weeks, mild hypertrophy and hyperplasia of hepatic interlobular bile ducts were detected (3/10 on 13 weeks, 9/20 on 26 weeks, respectively) in some rats at high dose, but no significant changes were observed in liver at low dose and medium dose. At the end of the recovery period, the above changes in liver were eliminated and the tissue structure returned to normal Figure 3. DLTD had no obvious toxic damage to other organs and tissues.
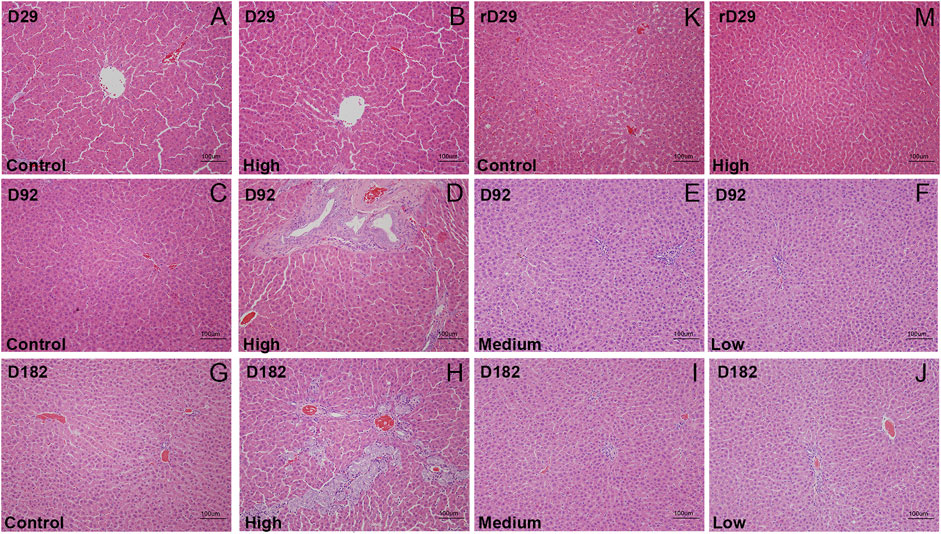
FIGURE 3. The histopathological analysis (H&E staining × 200) of liver of the control group and high-dose group were performed at D29 (A and B), D92 (C and D), D182(G and H) and rD29(K and M), of the midium-dose group and low-dose group were performed at D92 (E and F) and D182 (I and J), respectively.
3.3 Differential gene expression, GO and KEGG analysis
To go further and explore the possible roles of DLTD on the rat liver, an RNA-seq analysis was conducted. We analyzed the gene expression profiles of the control group and high-dose group rats at the end of the administration period. Six hundred and fifty-six differentially expressed genes (DEG) were found according to the criteria of |log2 fold changes| ≥ 0 and p-value ≤ 0.05. Among the 656 DEGs, DLTD upregulated 328 genes and downregulated 328 genes in the liver tissue (Figure 4A). Figure 4B showed the heatmaps of the distributions of the DEGs. To characterize the potential functional pathways altered by DLTD, GO and KEGG pathway enrichment analysis were performed. The GO analysis identified 21 GO terms that were significantly enriched (p-value < 0.05, Figure 4C), including terms related to the biological process, cellular components and molecular function. The biological process analysis showed that the DEGs were related to protein activation cascade, adaptive immune response, antigen processing and presentation of peptide, xenobiotic metabolic process, immunoglobulin mediated immune response, B cell mediated immunity. The cellular components analysis showed that the DEGs were related to secretory granule, secretory granule membrane, presynaptic cytosol, region of cytosol, postsynaptic cytosol. The molecular function analysis showed that the DEGs were related to heme binding, tetrapyrrole binding, peptidase regulator activity, oxidoreductase activity, steroid hydroxylase activity, peptidase inhibitor activity, aromatase activity, cofactor binding, endopeptidase regulator activity, endopeptidase inhibitor activity. There were 15 pathways with a p-value < 0.05 figured out by the KEGG pathway analysis of the DEGs shown in Figure 4D. The significantly enriched pathways were related to complement and coagulation cascades, chemical carcinogenesis, retinol metabolism, steroid hormone biosynthesis, metabolism of xenobiotics by cytochrome P450, staphylococcus aureus infection, drug metabolism-cytochrome P450, enzymes drug metabolism-other enzymes, pentose and glucuronate interconversions, pertussis, coronavirus disease-COVID-19, porphyrin and chlorophyll metabolism, carbon metabolism, phagosome, fat digestion and absorption. In conclusion, we focused our research on the effect of DLTD on hepatic drug enzymes.
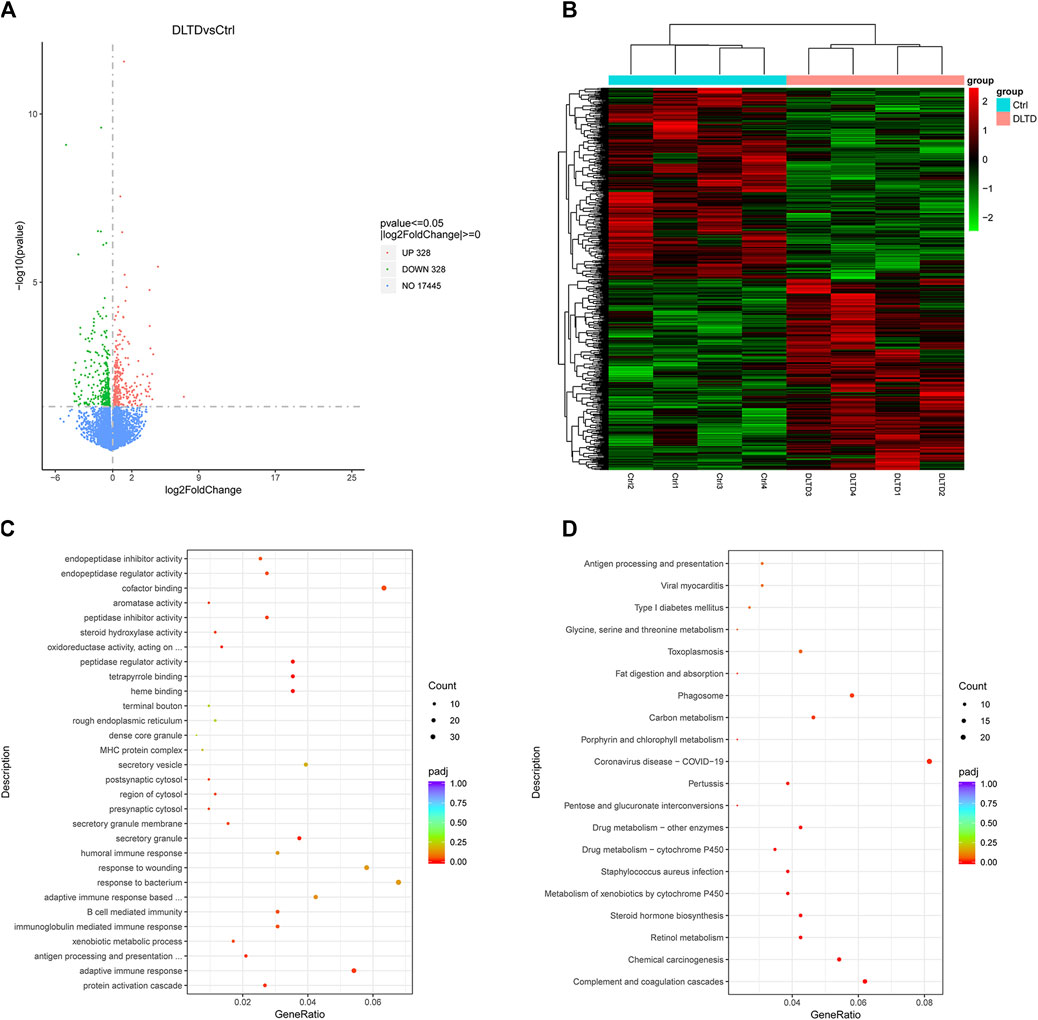
FIGURE 4. Differential gene expression, GO and KEGG Analysis of liver between the control group and DLTD group. (A) The number of genes upregulated and downregulated by DLTD. (B) Heatmap of differentially expressed genes between the control group and DLTD group. (C) Gene ontology (GO) enrichment and (D) Kyoto Encyclopedia of Genes and Genomes (KEGG) pathway enrichment analysis of differentially expressed genes between the control group and DLTD group.
3.4 Validation of RNA-sequencing results by quantitative real-time PCR and western blot
RNA-sequencing results showed that cytochrome P450 enzymes (CYPs) including CYP1A1, CYP2B1, CYP1A2, CYP4A3, CYP3A9 and CYP3A18 were up-regulated in the high-dose group, and Log2 fold change values are 3.97, 1.57, 0.92, 0.51, 0.50 and 0.48 respectively. To confirm the results of the RNA-seq, we performed quantitative real-time PCR and western blot assays. Consistent with RNA-seq data, the mRNA expressions of CYP1A1 (Control 1–5 vs. DLTD 11–15, p = 0.013; Control 6–10 vs. DLTD 16–20, p = 0.11, Figure 5 A and B) and CYP1A2 (Control 1–5 vs. DLTD 11–15, p = 0.054; Control 6–10 vs. DLTD 16–20, p = 0.006, Figure 5A,B); the protein level of CYP1A1 (Control 1–5 vs. DLTD 11–15, p = 0.002; Control 6–10 vs. DLTD 16–20, p = 0.99, Figure 5C) and CYP1A2 (Control 1–5 vs. DLTD 11–15, p = 0.049; Control 6–10 vs. DLTD 16–20, p = 0.056, Figure 5D) were upregulated significantly in liver tissue of high dose group compared to control group (Figure 5).
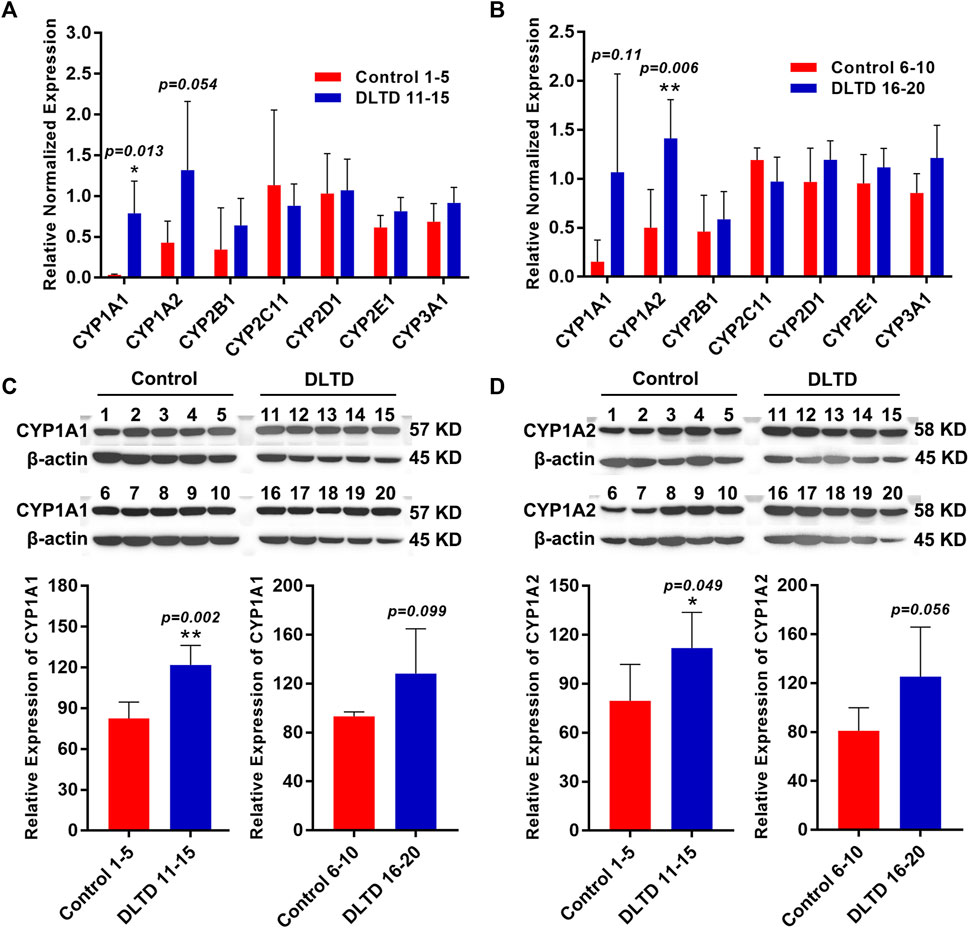
FIGURE 5. Validation the differential expression of CYP450 enzyme in the control group and DLTD group. (A) The mRNA expressions of CYP1A1, CYP1A2, CYP2B1, CYP2C11, CYP2D1, CYP2E1, CYP3A1 was detected in Control 1–5 and DLTD 11–15 by quantitative real-time PCR. (B) The mRNA expressions of CYP1A1, CYP1A2, CYP2B1, CYP2C11, CYP2D1, CYP2E1, CYP3A1 was detected in Control 6–10 and DLTD 16–20 by quantitative real-time PCR. (C) The protein level of CYP1A1 was detected in Control 1–5 and DLTD 11–15 or in Control 6–10 and DLTD 16–20 by western blot. (D) The protein level of CYP1A2 was detected in Control 1–5 and DLTD 11–15 or in Control 6–10 and DLTD 16–20 by western blot. The statistical significance of differences was calculated by t-test. *p < 0.05, **p < 0.01 versus the control.
4 Discussion
There are few information and studies regarding the toxicity of DLTD. The present study aimed to assess the toxicity and explore the mechanism of toxicity of the DLTD prior to clinical trials. The acute toxicity study demonstrated that there were no mortality or meaningful adverse changes in mice following DLTD administration. Meaningful toxicity changes in chronic toxicity studies were mild hypertrophy and hyperplasia of hepatic interlobular bile ducts were detected in some rats at high dose, and the above changes in liver were eliminated and the tissue structure returned to normal at the end of the recovery period. Based on the chronic toxicity study and three reports of drug-induced liver injury caused by DLTD, we further focused on the impact of DLTD on the liver. Four male rats were selected from the control group and the high-dose group respectively, and their liver tissues were analyzed by transcriptome. The results of transcriptome sequencing showed that DLTD had a significant effect on CYPs in rat liver, especially CYP1 subtype.
In human body, CYPs as a terminal oxygenase, are responsible for the oxidation-reduction reaction process of endogenous substrates, exogenous compounds and 90% of common drugs, and plays an important role in ensuring drug efficacy and controlling drug toxicity. So far, fifty-seven human genes coding for various CYPs have been identified. Based on the similarity of amino acid sequences, CYPs genes can be mainly divided into CYP1, CYP2 and CYP3 subfamilies(Stipp and Acco, 2021). CYP1 family comprises three members, namely CYP1A1, CYP1A2, and CYP1B1. CYP1A1 can catalyze the biotransformation of polycyclic aromatic hydrocarbons, aromatic amines, and polychlorinated biphenyls into polar compounds, so that can be conjugated to soluble compounds suitable for excretion by urine or bile. As an important metabolic enzyme in the body, CYP1A1 participates in a variety of physiological processes, such as immune response, oxidative stress, mutation induction and so on; and related to a variety of diseases, including various tumors, acute and chronic inflammation, lung injury, atherosclerosis, pathogen infection, etc(Gideon et al., 2012; Hussain et al., 2014; Gdula-Argasińska et al., 2016; Schiering et al., 2017). CYP1A2 is one of the major CYPs in human liver (∼13%–15%) and metabolizes of more than 20 drugs, such as caffeine, theophylline, warfarin, clozapine, phenacetin, tacrine, propranolol, and mexiletine(Zhou et al., 2009). CYP1A2 also plays an essential role in the metabolism of endogenous substrates and environmental toxins, as well as the activation of some precarcinogens. CYP1A2 enzyme activity varies significantly from individual to individual and can be affected by smoking, drugs, genes, and other factors(Guo et al., 2021).
Liu et al. found that doxycycline and azithromycin and their combination could cause drug-induced oxidative liver injury by increasing the expression levels of CYP1A1 and CYP1B1 (Liu et al., 2019). Hussain’s reserch confirmed that induction of CYP1A1, CYP1A2 and CYP1B1 increased oxidative stress and inflammation in the lung and liver tissues of rats exposed to incense smoke (Hussain et al., 2014). The metabolic enzyme system of CYPs were also the essential place for the metabolism of most components of traditional Chinese medicine to exert pharmacological and toxicological effects. When the activity of CYPs is inhibited or induced, drug interactions and adverse reactions are probably occur, which will lead to hepatotoxicity (Liao and Chen, 2012). Xanthii Fructus induced the activity of CYP1A2 and CYP3A4 enzymes, after processing, it could reduce the induction of CYP1A2 and CYP3A4 enzymes, and at the same time reduce liver damage (Huang et al., 2022). Emodin could induce intracellular oxidative stress and endoplasmic reticulum stress through AHR-CYP1A1 pathway, and then mediated apoptosis pathway leading to hepatocyte injury (Wang, 2018). The Polygoni Multiflori Radix and gallic acid caused significant damage to the liver function of mice by inducing the expression of CYP1A1, CYP3A4 mRNA and protein, and thereby activating the expression of the apoptosis factor Caspase-3 (Wang, 2020). Rhizoma dioscoreae bulbiferae could cause liver injury by upregulating the expression of CYP1A2 and CYP2E1 proteins in rat liver tissue (Liu, 2014).
There are many reports on drug-induced hepatobiliary hyperplasia. Drugs can cause biliary tract irritation or injury through bile excretion or the action of its metabolites (Hickling et al., 2010). Bile duct hyperplasia usually begins with bile duct epithelial injury and inflammation, and finally appears bile duct hyperplasia. At this time, inflammation, fibroblasts and bile duct hyperplasia appear in the portal duct area, and sometimes the proliferated bile duct extends to the liver lobular parenchyma, and may also be accompanied by oval cell proliferation (Gopinath et al., 1987). It has been confirmed that DLTD could cause mild hypertrophy and hyperplasia of interlobular bile ducts, and that DLTD could significantly up-regulate of CYP1A1 and CYP1A2 expression. Therefore, we speculate that the causes of mild liver injury caused by DLTD may include two aspects: on the one hand, the increased expression of CYP1A1 and CYP1A2 will accelerate the metabolism of drugs in the liver, produce more active intermediate substances to stimulate the bile duct system, and cause bile duct hyperplasia; on the other hand, the up-regulation of CYP1A1 and CYP1A2 expression can aggravate the oxidative stress and inflammatory stimulation of the hepatobiliary system, and then cause the damage of the hepatobiliary system. In addition, given the genetic polymorphisms of CYP1A1 and CYP1A2 (Vibhuti et al., 2010), high attention to the specific constitution, close observation to understand the condition, to the elderly, infants, gestating period, and liver function decline in patients with drugs, should be monitored regularly, ensure that the incidence of serious adverse reactions was reduced and drug safety.
Data availability statement
The datasets presented in this study can be found in online repositories. The names of the repository/repositories and accession number(s) can be found below: GEO, the series record number is GSE212036.
Ethics statement
The animal study was reviewed and approved by Committee of Welfare and Ethics for Experimental Animals, Institute of Chinese Materia Medica China Academy of Chinese Medical Science.
Author contributions
All authors listed have made a substantial, direct, and intellectual contribution to the work and approved it for publication.
Funding
This work was supported by the Fundamental Research Funds for the Central public welfare research institutes (ZXKT20030 and ZZ15-YQ-037).
Conflict of interest
The authors declare that the research was conducted in the absence of any commercial or financial relationships that could be construed as a potential conflict of interest.
Publisher’s note
All claims expressed in this article are solely those of the authors and do not necessarily represent those of their affiliated organizations, or those of the publisher, the editors and the reviewers. Any product that may be evaluated in this article, or claim that may be made by its manufacturer, is not guaranteed or endorsed by the publisher.
References
Botwin, K. P., and Gruber, R. D. (2003)., 14. v, 1–15. doi:10.1016/s1047-9651(02)00063-3Lumbar spinal stenosis: Anatomy and pathogenesisPhys. Med. Rehabil. Clin. N. Am.
Chen, L. (2011). Drug-induced liver damage caused by Danlu tongdu tablet: A case report. Chin. J. Integr. Traditional West. Med. Liver Dis. 21, 54. doi:10.3969/j.issn.1005-0264.2011.01.021
Chen, X. Y. (2015). Clinical effect of Danlu Tongdu tablet in the treatment of lumbar disc herniation. China Mod. Med. 22, 111–113.
Commission, C. P. (2020). Pharmacopeia of the people's Republic of China. Beijing: The Medicine Science and Technology Press of China.
Duo, J. (2015). Observation on the curative effect of Danlu Tongdu Tablet in the treatment of lumbar disc herniation. Chin. J. Mod. drug Appl. 9, 241–242. doi:10.14164/j.cnki.cn11-5581/r.2015.12.163
Fan, H. Y., Fu, F. H., Yang, M. Y., Xu, H., Zhang, A. H., and Liu, K. (2010). Antiplatelet and antithrombotic activities of salvianolic acid A. Thromb. Res. 126, e17–e22. doi:10.1016/j.thromres.2010.04.006
Feng, Z. L., Zhao, Z. D., and Liu, J. X. (2018). Research progress on chemical components and pharmacological effects of Corydalis yanhusuo. Chengdu: Natural product research and development.
Gdula-Argasińska, J., Czepiel, J., Totoń-Żurańska, J., Jurczyszyn, A., Wołkow, P., Librowski, T., et al. (2016). Resolvin D1 down-regulates CYP1A1 and PTGS2 gene in the HUVEC cells treated with benzo(a)pyrene. Pharmacol. Rep. 68, 939–944. doi:10.1016/j.pharep.2016.05.005
Gideon, D. A., Kumari, R., Lynn, A. M., and Manoj, K. M. (2012). What is the functional role of N-terminal transmembrane helices in the metabolism mediated by liver microsomal cytochrome P450 and its reductase? Cell. biochem. Biophys. 63, 35–45. doi:10.1007/s12013-012-9339-0
Gopinath, C., Prentice, D. E., and Lewis, D. J. (1987). “The liver: Atlas of experimental toxicological pathology,” in Atlas of experimental toxicological pathology. Editors C. GOPINATH, D. E. PRENTICE, and D. J. LEWIS (Dordrecht: Springer Netherlands).
Guo, C. Q. (2011a). Clinical application of Danlu tongdu tablet. Chin. Med. Mod. distance Educ. china 9, 81–82. doi:10.3969/j.issn.1672-2779.2011.20.054
Guo, C. Q. (2011b). Treatment of 128 cases of lumbar spinal stenosis with Danlu Tongdu Tablet. Chin. Med. Mod. distance Educ. china 9, 72. doi:10.3969/j.issn.1672-2779.2011.21.040
Guo, J., Zhu, X., Badawy, S., Ihsan, A., Liu, Z., Xie, C., et al. (2021). Metabolism and mechanism of human cytochrome P450 enzyme 1A2. Curr. Drug Metab. 22, 40–49. doi:10.2174/1389200221999210101233135
Hickling, K. C., Hitchcock, J. M., Chipman, J. K., Hammond, T. G., and Evans, J. G. (2010). Induction and progression of cholangiofibrosis in rat liver injured by oral administration of furan. Toxicol. Pathol. 38, 213–229. doi:10.1177/0192623309357945
Huang, C., Zhou, R. J., Huang, B. S., Han, Y. Q., Peng, T. Y., Jin, C. S., et al. (2022). Study on the attenuation mechanism of Cang'erzi (Xanthii Fructus) processing based on CYP1A2 and CYP3A4 enzyme activity. Guid. J. traditional Chin. Med. Pharm. 28, 79–83.
Huang, W. G. (2012). Clinical observation of Danlu Tongdu in the treatment of lumbago and leg pain. Beijing: China health case & nutrition, 2149.
Hussain, T., Al-Attas, O. S., Al-Daghri, N. M., Mohammed, A. A., de Rosas, E., IBRAHIM, S, et al. (2014). Induction of CYP1A1, CYP1A2, CYP1B1, increased oxidative stress and inflammation in the lung and liver tissues of rats exposed to incense smoke. Mol. Cell. Biochem. 391, 127–136. doi:10.1007/s11010-014-1995-5
Li, Z. H., Zhao, X. Y., and Qiu, S. (2013). Study on quality standard of Danlu Tongdu tablet. China Pharm. 22, 43–45. doi:10.3969/j.issn.1006-4931.2013.15.021
Liao, N.S., and Chen, W. L. (2012). Progress in cytochrome P450 enzyme in toxicity of traditional Chinese medicines. Chin. J. Pharmacol. Toxicol. 26, 402–405. doi:10.3867/j.issn.1000-3002.2012.03.028
Liu, A. M., Pan, Y. H., Chen, D. M., Tao, Y. F., Cheng, G. Y., Liu, Z. L., et al. (2019). “Role of CYP1A1 and CYP1B1 in oxidative stress injury induced by azithromycin and doxycycline in L02 cells,” in The 15th symposium of the branch of veterinary Pharmacology and toxicology (Lanzhou, Gansu, China: Chinese Society of Animal Husbandry and Veterinary Medicine).
Liu, J. (2014). “Study on oxidative stress and CYP1A2 and CYP2E1 protein expression in liver of rats by Dioscorea bulbifera L. compatibility with Glycyrrhiza uralensis Fisch,”. Master degree of Medicine (China, Fujian, Fuzhou: Fujian University of Traditional Chinese Medicine).
Liu, Y. H., Feng, T., Ran, C., and Jiao, Z. Q. (2012). Clinical observation of 130 cases of lumbar spinal stenosis treated with Danlu Tongdu Tablet. Chin. Med. Mod. distance Educ. china 10. doi:10.3969/j.issn.1672-2779.2012.08.096
Lu, B. W., Wang, N., and He, Q. H. (2021). The latest research progress of antler gum. Guangming J. Chin. medcine 36, 2881–2884. doi:10.3969/j.issn.1003-8914.2021.17.018
Pan, C. R. (2010). Clinical observation of oral Danlu Tongdu tablet in the treatment of lumbago and leg pain. Chin. J. Mod. drug Appl. 4, 135–136. doi:10.14164/j.cnki.cn11-5581/r.2010.18.012
Schiering, C., Wincent, E., Metidji, A., Iseppon, A., Li, Y., Potocnik, A. J., et al. (2017). Feedback control of AHR signalling regulates intestinal immunity. Nature 542, 242–245. doi:10.1038/nature21080
Stipp, M. C., and Acco, A. (2021). Involvement of cytochrome P450 enzymes in inflammation and cancer: A review. Cancer Chemother. Pharmacol. 87, 295–309. doi:10.1007/s00280-020-04181-2
Vibhuti, A., Arif, E., Mishra, A., Deepak, D., Singh, B., Rahman, I., et al. (2010). CYP1A1, CYP1A2 and CYBA gene polymorphisms associated with oxidative stress in COPD. Clin. Chim. Acta. 411, 474–480. doi:10.1016/j.cca.2009.12.018
Wang, J. J., Qin, X. M., Gao, X. X., Zhang, B., Wang, P. Y., Hao, J. Q., et al. (2017). Research progress on chemical compounds, pharmacological action, and quality status of Eucommia ulmoides. Chin. traditional Herb. drugs 48, 3228–3237. doi:10.7501/j.issn.0253-2670.2017.15.031
Wang, L. P. (2020). “Study on the material basis of liver injury induced by Radix Polygoni mutiflori and its effect on the expression of CYP1A1 and CYP3A4,”. Master degree of Chinese medicine (Xianyang, Shaanxi, China: Shaanxi University of Chinese Medicine).
Wang, M. X. (2018). Study on the mechanisms of emodin increasing liver injury in polygonum multiflorum thunb through activating CYP1A1. Guangzhou: Guangdong Pharmaceutical University.
Wei, Z. Y. (2022). Clinical efficacy of Danlu Tongdu tablet in the treatment of lumbar disc herniation and its effect on pain and lipid peroxidation. Chin. J. Clin. Ration. Drug Use 15, 120–123. doi:10.15887/j.cnki.13-1389/r.2022.15.038
Wu, D. M., Wang, Y. J., Han, X. R., Wen, X., Li, L., Xu, L., et al. (2018). Tanshinone IIA prevents left ventricular remodelling via the TLR4/MyD88/NF-κB signalling pathway in rats with myocardial infarction. J. Cell. Mol. Med. 22, 3058–3072. doi:10.1111/jcmm.13557
Yan, B. H., Niu, Q., Fu, Z. J., Yang, F., Yu, D. R., and Niu, K. (2019). Efficacy and mechanism of Danlu tongdu tablet on lumbar disc herniation with kidney deficiency and stasis syndrome. Chin. J. Exp. Traditional Med. Formulae 25, 66–71. doi:10.13422/j.cnki.syfjx.20191634
Zhang, Q., Gao, W. Y., and Man, S. L. (2012). Chemical composition and pharmacological activities of Astragali Radix. China J. Chin. materia medica 37, 3203–3207. doi:10.4268/cjcmm20122109
Zhang, Q., and Leng, G. (2018). Two cases of severe drug-induced liver injury caused by Danlu Tongdu tablet. Chin. Remedies Clin. 18, 133–134. doi:10.11655/zgywylc2018.Suppl.075
Zhao, X. H., and Wang, W. H. (2017). A case of severe drug-induced liver damage caused by Danlutongdu tablet. China Pharm. 26, 96. doi:10.3969/j.issn.1006-4931.2017.07.030
Zhou, L. Y. (2012). Treatment of 150 cases of lumbar intervertebral disc herniation with Danlu Tongdu Tablet. Chin. J. Mod. drug Appl. 6, 78–79. doi:10.14164/j.cnki.cn11-5581/r.2012.04.005
Keywords: Danlu tongdu tablets, acute toxicity, chronic toxicity, hepatotoxicity, transcriptome analysis, cytochrome P450 enzyme
Citation: Zhang H, Yang Y, Guo F, He R, Gao S, Cao C, Zhao C, Xia B, Xu Q, Gong P, Wang L, Su P and Liu T (2022) Danlu tongdu tablets: Preclinical safety evaluation and mechanism of hepatotoxicity. Front. Pharmacol. 13:1023379. doi: 10.3389/fphar.2022.1023379
Received: 19 August 2022; Accepted: 17 October 2022;
Published: 03 November 2022.
Edited by:
Rolf Teschke, Hospital Hanau, GermanyReviewed by:
Mubarak Hussaini Ahmad, Ahmadu Bello University, NigeriaVikram Patial, Institute of Himalayan Bioresource Technology (CSIR), India
Copyright © 2022 Zhang, Yang, Guo, He, Gao, Cao, Zhao, Xia, Xu, Gong, Wang, Su and Liu. This is an open-access article distributed under the terms of the Creative Commons Attribution License (CC BY). The use, distribution or reproduction in other forums is permitted, provided the original author(s) and the copyright owner(s) are credited and that the original publication in this journal is cited, in accordance with accepted academic practice. No use, distribution or reproduction is permitted which does not comply with these terms.
*Correspondence: Ping Su, c3VwaW5nMTIyM0AxMjYuY29t; Ting Liu, dGxpdUBpY21tLmFjLmNu
†These authors have contributed equally to this work and share first authorship