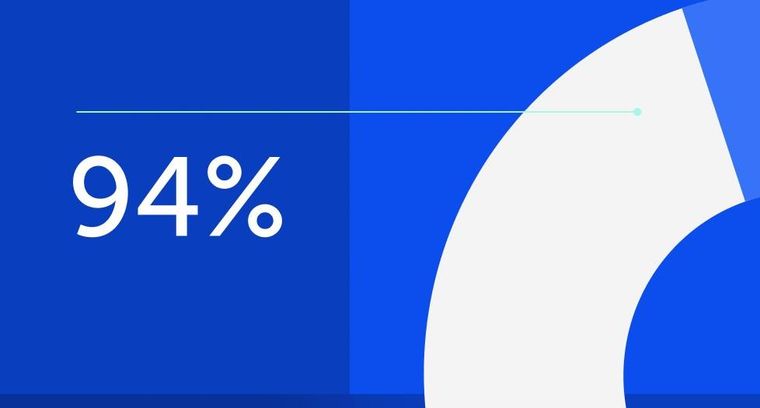
94% of researchers rate our articles as excellent or good
Learn more about the work of our research integrity team to safeguard the quality of each article we publish.
Find out more
ORIGINAL RESEARCH article
Front. Pharmacol., 14 December 2022
Sec. Neuropharmacology
Volume 13 - 2022 | https://doi.org/10.3389/fphar.2022.1022863
This article is part of the Research TopicSubstance Use Disorder: Above and Beyond AddictionView all 32 articles
Drug overdose deaths involving cocaine have skyrocketed, an outcome attributable in part to the lack of FDA-approved medications for the treatment of cocaine use disorder (CUD), highlighting the need to identify new pharmacotherapeutic targets. Vulnerability to cocaine-associated environmental contexts and stimuli serves as a risk factor for relapse in CUD recovery, with individual differences evident in the motivational aspects of these cues. The medial prefrontal cortex (mPFC) provides top-down control of striatal circuitry to regulate the incentive-motivational properties of cocaine-associated stimuli. Clinical and preclinical studies have identified genetic variations that impact the degree of executive restraint over drug-motivated behaviors, and we designed the present study to employ next-generation sequencing to identify specific genes associated with heightened cue-evoked cocaine-seeking in the mPFC of male, outbred rats. Rats were trained to stably self-administer cocaine, and baseline cue-reinforced cocaine-seeking was established. Rats were phenotyped as either high cue (HC) or low cue (LC) responders based upon lever pressing for previously associated cocaine cues and allowed 10 days of abstinence in their home cages prior to mPFC collection for RNA-sequencing. The expression of 309 genes in the mPFC was significantly different in HC vs. LC rats. Functional gene enrichment analyses identified ten biological processes that were overrepresented in the mPFC of HC vs. LC rats. The present study identifies distinctions in mPFC mRNA transcripts that characterizes individual differences in relapse-like behavior and provides prioritized candidates for future pharmacotherapeutics aimed to help maintain abstinence in CUD. In particular the Htr2c gene, which encodes the serotonin 5-HT2C receptor (5-HT2CR), is expressed to a lower extent in HC rats, relative to LC rats. These findings build on a plethora of previous studies that also point to the 5-HT2CR as an attractive target for the treatment of CUD.
Recent sharp rises in cocaine-related overdoses (Ahmad et al., 2022) associate with a history of cocaine use disorder (CUD) in some individuals (Yoon et al., 2014; John and Wu, 2017; Yule et al., 2018). Recidivism rates are high even among CUD individuals who have undergone rehabilitation (Brandon et al., 2007), and the field currently lacks approved pharmacotherapy to aid in sustaining recovery. Abstinence is challenged by sensitivity to stress, withdrawal symptoms, and cocaine-associated stimuli (e.g., cocaine-associated cues) which can trigger craving and relapse (Hendershot et al., 2011). Functional top-down control of the medial prefrontal cortex (mPFC) over striatal circuitry titrates the incentive-motivational properties of cocaine-associated stimuli (McLaughlin and See, 2003) and mPFC neuroadaptations resulting from chronic cocaine use associate with increased relapse vulnerability (for reviews) (Kalivas, 2005; Van den Oever et al., 2010; Jasinska et al., 2014; Cunningham et al., 2020). Preclinical analyses have illustrated persistent changes in mPFC gene transcripts resulting from cocaine exposure and withdrawal (Freeman et al., 2008; Walker et al., 2018). However, variation within the mPFC transcriptome contributory to individual differences in vulnerability to cocaine-seeking remains underexplored. Genes associated with heightened cue-evoked cocaine-seeking could serve as prioritized targets for neuropharmacological intervention to support abstinence and mitigate relapse in CUD patients particularly vulnerable to cocaine cue-evoked relapse.
The present study quantified cocaine-seeking following a 10-days period of abstinence from cocaine self-administration in outbred, male Sprague-Dawley rats to detect “low cue” (LC) and “high cue” (HC) phenotypes. Rats were categorized into phenotypes based on the degree of lever-presses for cocaine-associated cues. Next-generation sequencing (NGS) and bioinformatics analyses were utilized to identify neurobiological signatures within the mPFC transcriptome that correlate with heightened sensitivity to cocaine-associated cues (Geschwind and Konopka, 2009; Wang et al., 2009; Volkow et al., 2015). We established a unique mPFC transcriptomic landscape in the of HC vs. LC rats which validates known, and identifies, new molecular mechanisms to be interrogated in CUD relapse vulnerability.
Male, Sprague-Dawley rats (n = 12; Envigo, Houston, TX) weighing 250–275 g upon arrival were housed two per cage and maintained on a 12-h light-dark cycle with monitored and controlled temperature (21°C–23°C) and humidity (45%–50%). Rats were acclimated to the colony room for 7 days prior to handling and experimentation and were allowed ad libitum access to food and water except during daily operant sessions. Rats were 11 weeks old at the start of operant training. Experiments were carried out in accordance with the National Institutes of Health Guide for the Care and Use of Laboratory Animals (2011) and with the approval of the University of Texas Medical Branch Institutional Animal Care and Use Committee.
Rats (n = 12) were anesthetized (8.6 mg/kg of xylazine, 1.5 mg/kg of acepromazine, 43 mg/kg of ketamine in bacteriostatic saline) prior to surgical implantation of indwelling jugular catheters with back mounts; rats were allotted 7 days to permit postoperative recovery (Cunningham et al., 2011; Cunningham et al., 2013; Anastasio et al., 2014a; Anastasio et al., 2014b). Rats received a 0.1 ml infusion of a bacteriostatic saline solution containing heparin sodium (10 µ/ml; American Pharmaceutical Partners, East Schaumburg, IL), streptokinase (0.67 mg/ml; Sigma Chemical, St. Louis, MO), and ticarcillin disodium (66.67 mg/ml; Research Products International, Mt. Prospect, IL) into the catheter immediately following daily self-administration sessions to ensure catheter patency during experimentation.
The cocaine self-administration assay utilized standard operant conditioning chambers (Med Associates, Inc., St. Albans, VT) housed within sound-attenuated, ventilated cubicles equipped with fans (Med Associates, Inc.). Operant chambers were fitted with two retractable response levers, a stimulus light above each response lever, a houselight on the wall opposite the response levers, and an external pellet dispenser. In 180-min sessions, rats were trained to perform a lever press response reinforced by infusions (0.75 mg/kg/0./1 ml) of (-)-cocaine HCl (NIDA Drug Supply Program, Bethesda, MD) dissolved in 0.9% NaCl and delivered through syringes loaded daily into infusion pumps (Med Associates, Inc.) (Cunningham et al., 2011; Anastasio et al., 2014a; Anastasio et al., 2014b; Swinford-Jackson et al., 2016). The infusion pumps were connected to liquid swivels (Instech, Plymouth Meeting, PA) fastened to catheters via polyethylene 20 tubing encased inside a metal spring leash (Plastics One, Roanoke, VA).
Scheduled responses on the active lever resulted in the delivery of a cocaine infusion over a 6-s period; each infusion was simultaneously paired with the illumination of the house and stimulus lights and activation of the infusion pump (i.e., discrete cue complex paired with cocaine delivery). Inactive lever presses were recorded but had no scheduled consequences. The stimulus light and infusion pump were inactivated following the delivery of cocaine. The house light remained on to signal a timeout period (20 s); lever presses committed during the timeout period had no scheduled consequences. Rats were trained on a fixed ratio (FR) 1 schedule of reinforcement and progressed to a FR5 schedule until stability as assessed by achieving <10% variation in the number of infusions and lever presses for a minimum of three consecutive sessions (within-subjects (phenotype), repeated measures (day), two-way ANOVA; GraphPad Prism 9.2.0) (Cunningham et al., 2013; Anastasio et al., 2014a; Anastasio et al., 2014b; Sholler et al., 2019; Anastasio et al., 2020).
Once stable cocaine self-administration was achieved, individual levels of cue-reinforced cocaine-seeking were assessed in a 60-min session (see experimental timeline, Figure 1A). Lever presses on the previously active lever were reinforced by the cocaine-associated discrete cue complex (stimulus light illuminated, infusion pump activated) in the absence of cocaine, on a FR1 schedule. Inactive lever presses were recorded with no scheduled consequences. Rats were stratified as HC or LC rats based on a median split analysis of the total number of cue-reinforced lever presses on session 12 (unpaired, one-tailed Student’s t-test) (Anastasio et al., 2014a; Anastasio et al., 2014b; Fink et al., 2015; Sholler et al., 2020).
FIGURE 1. High cue (HC, red) and low cue (LC, blue) rats are stratified by levels of cue-reinforced lever presses in the self-administration (SA) model prior to transcriptomic analyses. (A) The experimental timeline is shown. (B) The time course of self-administration sessions is presented as the total presses (mean ± SEM) on the active (black circles; left Y-axis) or inactive lever (white circles; left Y-axis), and total number of cocaine infusions obtained in the 180-min session (purple circles; right Y-axis). (C) Total lifetime cocaine intake (mg/kg; mean ± SEM) is presented for HC (red) and LC (blue) rats. An unpaired, one-tailed Student’s t-test revealed HC rats (n = 5) self-administered a significantly higher amount of cocaine (568.31 ± 56.12 mg/kg) relative to LC rats (436.34 ± 33.49 mg/kg) (t8 = 3.546, p = 0.0076) (unpaired, two-tailed, Student’s t-test). (D) Total cue-reinforced lever and inactive lever responding during the first cue test on Day 12 (mean ± SEM) are presented for HC (red) and LC (blue). An unpaired, one-tailed Student’s t-test revealed HC rats (n = 5) displayed significantly higher lever presses (119.8 ± 6.21) for cocaine-associated cues relative to LC rats (n = 5; 87.6 ± 4.57) (t8 = 4.178, *p = 0.0015). An unpaired, one-tailed Student’s t-test revealed inactive lever presses did not differ between HC (16.0 ± 3.49) and LC rats (n = 5; 11.8 ± 2.73) (t8 = 0.9477, p = 0.1855).
Self-administration resumed following the cue probe and continued daily for an additional five maintenance sessions, followed by 10 days of abstinence in home cages and daily handling (Figure 1A). Half of the rats from each phenotype (n = 3/phenotype) were randomly selected and a second cue-reinforced cocaine-seeking test was conducted to interrogate maintenance of HC and LC phenotypes on session 27 (Pearson’s correlation). Total lifetime cocaine consumption [daily intake (mg/kg) = infusions per session * cocaine infusion dose (mg/ml) * infusion volume (0.1 ml/infusion) * body weight (kg)-1] for HC and LC rats was also determined (unpaired, two-tailed Student’s t-test).
Rats (n = 12) were anesthetized with chloral hydrate (400 mg/kg) on experimental day 27, and brains were dissected. The mPFC, comprised of infralimbic, prelimbic, and anterior cingulate cortex regions (from Bregma: 3.2–1.2 mm anteroposterior, ±1.2 mm mediolateral, −5.2 mm dorsoventral) (Paxinos and Watson, 2005), was microdissected immediately over ice, flash-frozen in liquid nitrogen, and stored at −80°C for subsequent RNA isolation as previously described (Anastasio et al., 2014a; Anastasio et al., 2014b; Fink et al., 2015; Sholler et al., 2020). The mPFC was homogenized in 10 X W/V extraction buffer (20 mM HEPES, 200 mM NaCl, 1 mM EDTA, 1 mM DTT, 10 μl/ml protease inhibitor cocktail, 10 μl/ml phosphatase inhibitor cocktails 2 and 3 (Sigma-Aldrich, St Louis, MO), and 5 μl/ml RNaseOUT™ Recombinant Ribonuclease Inhibitor (Thermo Fisher Scientific, Waltham, MA). Immediately following homogenization, the sample was transferred to 500 μl of TRI Reagent (Life Technologies, Grand Island, NY), and isolated RNA was purified using RNeasy Mini Kit (Qiagen, Germantown, MD). The RNA concentration and quality were analyzed using Cytation 5 Cell Imaging Multi-Mode Reader (BioTek Instruments, Winooski, VT) and preserved at −80°C until assayed.
Total RNA-sequencing was performed at the University of California Los Angeles Technology Center for Genomics and Bioinformatics. Libraries were prepared using Kapa Stranded RNA-Seq Kit (Kapa Biosystems, Wilmington, MA). During the library preparation step, samples underwent enrichment, cDNA conversion, end repair, A-tailing, multiplexing, and amplification. Next, libraries were sequenced as single-end, 50 base-pair reads using the Illumina Hiseq3000 instrument generating between 16 and 39 million reads per sample. Two samples (n = 1/phenotype) did not meet quality control standards and were excluded from all analyses, and our final sample size was ten (n = 5/phenotype). The literature supports a minimum of five biological replicates per experimental group for the conduct of transcriptomics analyses in vitro and ex vivo (Ching et al., 2014). CLC Genomics Workbench v21 (Qiagen Bioinformatics) (Sholler et al., 2020) was used for sequencing quality control and mapping of the RNA-Seq data to the Rattus norvegicus reference genome and the collection of the coding sequences (Sholler et al., 2020). Sequencing data were initially trimmed using CLC’s “Trim Reads” module. Reads containing nucleotides below the quality threshold of 0.05 (using the modified Richard Mott algorithm), those with two or more unknown nucleotides or sequencing adapters were trimmed from the dataset. Filtered sequencing reads were then processed using the “RNA-Seq Analysis” module by mapping using a global alignment strategy against the Rat Rnot_6.0.97 annotated reference genome with 95% length fraction and 80% similarity fraction scores. Differential gene expression analysis was performed as part of the “RNA-Seq Analysis” module. The gene expression count was modeled by a separate Generalized Linear Model (GLM), and statistical significance was evaluated using the Wald Test. A volcano plot of differentially expressed genes was rendered using the Volcano Plot tool in Galaxy Version 0.0.5 (Afgan et al., 2018). Functional enrichment analysis was performed separately using Ingenuity Pathway Analysis (IPA) (QIAGEN, Venlo, NL) to determine the biological functions of significantly differentially expressed genes. The most significant functional pathways [−log10 (p-value) ≥ 1.3] were then evaluated. The genes associated with behavioral pathways by IPA were extracted and further evaluated using the Cytoscape (Shannon et al., 2003) application ClueGo (Bindea et al., 2009). As a result, we remapped the significant, differentially expressed genes to a more common open source using Gene Ontology accession numbers (Ashburner et al., 2000; Gene Ontology, 2021) and further dissected into more specific and relevant biological processes specifically related to behavior. The Search Tool for the Retrieval of Interacting Genes/Proteins (STRING) database (Version 11.5; ELIXER, Cambridgeshire, United Kingdom) (Szklarczyk et al., 2019; Szklarczyk et al., 2021) was utilized to determine protein–protein interactions which are predicted based on published experimental data (e.g., co-expression, protein homology) and other bioinformatics databases [(e.g., Kyoto Encyclopedia of Genes and Genomes (KEGG)], Reactome, etc.) to generate the protein-protein interaction (PPI) network [reference genome: Rattus norvegicus, confidence score = 0.4 (default).
Outbred rats trained to self-administer cocaine (0.75 mg/kg/infusion) acquired stable self-administration by experimental session 11 (Figure 1B). Across the last three sessions, a two-way ANOVA (factors: phenotype, between-subjects and day, within-subjects) revealed a main effect of phenotype on infusions earned (F1,8 = 38.01, p = 0.0003), but no main effect of day (F2,16 = 0.9057, p = 0.4240) or significant phenotype X day interaction (F2,16 = 0.8262, p = 0.4555). These results indicate differences in cocaine-taking between phenotype, but not across the days preceding the cue test indicating stable self-administration. Total cocaine intake (Figure 1C; mean ± SEM) was higher in HC rats (568.31 ± 56.12 mg/kg) relative to LC rats (436.34 ± 33.49 mg/kg) (t8 = 3.546, p = 0.0076) (unpaired, two-tailed, Student’s t-test).
Rats were stratified as exhibiting greater than (HC rats) or less than (LC rats) the median (102.5) of total cue-reinforced lever presses on the first cue assessment on session 12, respectively (n = 5/phenotype) (Figure 1D) (Anastasio et al., 2014a; Anastasio et al., 2014b; Fink et al., 2015; Sholler et al., 2020). An unpaired, one-tailed Student’s t-test revealed total cue-reinforced lever presses (mean ± SEM) were significantly higher in the HC (119.8 ± 6.21) relative to the LC (87.6 ± 4.57) phenotype (t8 = 4.178, p = 0.0015). Total inactive lever presses (mean ± SEM) were not different between HC (16.0 ± 3.49) and LC (11.8 ± 2.73) rats (t8 = 0.9477, p = 0.1855) (unpaired, two-tailed Student’s t-test). On experimental day 27, cue-reinforced lever presses were reassessed in a subset of randomly selected rats (n = 6) and a positive correlation was observed between their two assessments (R2 = 0.75, p = 0.013), indicating stability of the HC and LC phenotype (Pearson’s correlation analysis).
Genes that were significantly increased or decreased in the mPFC of HC rat relative to LC rats was determined by analyzing gene expression count with a Generalized Linear Model (GLM) followed by the Wald Test (CLC Genomics Workbench v21, Qiagen Bioinformatics) (Sholler et al., 2020). A volcano plot of differentially expressed genes was rendered using the Volcano Plot tool in Galaxy Version 0.0.5 (Afgan et al., 2018). The expression of 309 genes in the mPFC significantly differed between HC vs. LC rats [-Log10 p-value ≥1.3 (y-axis) and |Log2 Fold Change| ≥ 0.58 (x-axis)]. The volcano plot (Figure 2) displays genes with lower (purple symbols; n = 227) and higher (orange symbols; n = 82) expression in the mPFC of HC vs. LC rats.
FIGURE 2. The volcano plot displays the results of mPFC RNA-seq results (CLC Genomics Workbench v21, Qiagen Bioinformatics) (Sholler et al., 2020) for HC and LC rats (generated by the Volcano Plot tool in Galaxy Version 0.0.5) (Afgan et al., 2018). Purple symbols (n = 227) indicate genes with lower expression in the mPFC of HC vs. LC rats. Orange symbols (n = 82) indicate genes with higher expression in mPFC of HC vs. LC rats. Significance criteria include -Log10 p-value ≥1.3 (y-axis) and Log2 fold change ≤ −0.58 or ≥ +0.58 (x-axis) (see Methods).
We utilized a combined gene set enrichment analysis approach with Ingenuity Pathway Analysis (IPA) (QIAGEN, Venlo, NL) and ClueGO (Cytoscape plug-in) to provide biological context to the registry of 309 differentially expressed genes (Bindea et al., 2009). A cluster of ten biological processes associated with behavior was found to be overrepresented in the HC phenotype (p < 0.05) (Table 1, top left column). Eighteen differentially expressed genes were found to populate these biological processes (Table 1). For example, adenosine receptor subtype 2A (Adora2a), cell cycle exit and neuronal differentiation 1 (Cend1), dopamine D2 receptor (Drd2), dopamine D3 receptor (Drd3), fibroblast growth factor 14 (Fgf14), serotonin 5-HT2C receptor (Htr2c), proenkephalin (Penk), protein phosphatase 1 regulatory inhibitor subunit 1B (Ppp1r1b), and synaptosome associated protein 25 (Snap25), and thyroid stimulating hormone receptor (Tshr) associate with locomotor behavior (GO:0007626). The computed false discovery rate (FDR)-adjusted p-values for behavior-related genes suggest a possibility of type I error, however the literature supports the involvement of these genes in CUD (see Discussion). A STRING network analysis (Version 11.5; ELIXER, Cambridgeshire, United Kingdom) (Szklarczyk et al., 2019; Szklarczyk et al., 2021) reveals predicted PPIs between nine of the 18 highlighted targets (e.g., Adora2a-Drd2, Figure 3). Network nodes (proteins) (Aci-SeChe et al., 2014) are shown with one or more connections (edges) to representing each line of evidence that predict an interaction between two nodes (e.g., protein homology; see Figure legend).
TABLE 1. Functional gene enrichment (IPA plus Cytoscape ClueGO) reveals behavior-related biological processes (top, left) that are overrepresented in the mPFC of HC vs. LC rats, significance and associated genes noted in upper middle and right columns, respectively. Full gene names for highlighted genes are noted below. Fold change values (bottom, right) for associated genes (bottom, left) that populate these biological process pathways are presented with significance noted (bottom, middle). Significance criteria include fold change >1.0, p-value <0.05 (see methods).
FIGURE 3. STRING bioinformatics analysis identified a network of predicted interactions in a subset of the selected genes. The specific color of network edges corresponds to the source of evidence for each known or predicted interaction (source: teal, curated database; magenta, experimentally determined; green, text mining; black, co-expression; lavender, protein homology). Confidence threshold based upon an interaction score of 0.4 minimum (see Methods).
We provide an unbiased evaluation of gene regulation in the mPFC associated with individual differences in cocaine-seeking during abstinence in male, outbred rats. Our interrogation isolated several candidate genes associated with heightened cue-evoked cocaine-seeking. Intriguingly, out of the 309 differentially expressed genes, the majority (∼73%) were decreased in the mPFC of HC rats. It is plausible this translated to a loss of fine-tuned, top-down regulation of downstream striatal circuitry implicated in drug-seeking behavior. Gene enrichment analyses narrowed our focus to genes that contribute to behavior-related biological processes (n = 18). We identified overarching themes (e.g., hyperactivity, heightened stress) connecting several biological processes overrepresented in the HC phenotype. These global themes might have been expected as hyperactivity and heightened stress states are often comorbid in individuals diagnosed with CUD, especially in the preoccupation/anticipation stage modeled here (Koob and Kreek, 2007). Here, we specifically highlight genes implicated in several biological processes (e.g., Htr2c) or predicted to interact at the protein level (e.g., Adora2a, Drd2). Our laboratory (Anastasio et al., 2013; Felsing et al., 2018; Price et al., 2019; Soto et al., 2019) and others (Tao et al., 2017; Hámor et al., 2018; Hasbi et al., 2020) have identified biologically meaningful PPIs which promise to enrich our understanding of SUD mechanisms and perhaps serve as targets for study in the therapeutic space.
The Htr2c gene encodes the serotonin (5-HT) G-protein coupled receptor (GPCR) 5-HT2C receptor (5-HT2CR) and our transcriptomic analyses identified lower Htr2c mRNA expression in the mPFC of abstinent HC vs. LC rats (FC: 1.510), aligning with lower 5-HT2CR protein expression previously observed in the mPFC during abstinence (Anastasio et al., 2014b; Swinford-Jackson et al., 2016). An investigational 5-HT2CR agonist evinced efficacy, but reduced potency, to inhibit cocaine-seeking during abstinence (Swinford-Jackson et al., 2016), supporting the concept that 5-HT2CR agonists (Fletcher et al., 2004; Cunningham et al., 2011; Harvey-Lewis et al., 2016; Anastasio et al., 2020), may ameliorate hypofunctional 5-HT2CR neurotransmission as a core mediator of relapse vulnerability (for reviews) (Howell and Cunningham, 2015; Cunningham et al., 2020). However, while the 5-HT2CR agonist lorcaserin reduced craving in non-treatment seeking CUD participants (Pirtle et al., 2019; Johns et al., 2021), this medication may facilitate continued abstinence in treatment-seeking CUD participants (NCT03007394). While these outcomes may reflect translational challenges in medication development for CUD, future studies are required to define specific molecular strategies to enhance performance of cortical 5-HT2CR function to mitigate relapse in CUD patients. For example, a 5-HT2CR positive allosteric modulator is sufficient to suppress cocaine-seeking in male rats (Wild et al., 2019), perhaps due to its superior selectivity for the 5-HT2CR over the other 5-HT2 receptors (Wold et al., 2019) relative to lorcaserin (Thomsen et al., 2008).
Eight Bardet-Biedl Syndrome (BBS) proteins form the BBsome complex, a stable complex involved in signaling receptor trafficking to and from ciliary membranes of neurons (Nachury et al., 2007; Scheidecker et al., 2014). The Bbip1 gene encodes one of these proteins (BBSome interacting protein 1) (Loktev et al., 2008) which facilitates vesicular trafficking of GPCRs from cytosolic compartments to the plasma membrane in neurons (Domire et al., 2011; Guo et al., 2016; Shinde et al., 2020). Our transcriptomic analysis identified the Bbip1 gene as expressed in the mPFC to a lower degree in HC rats vs. LC rats (FC: 1.642). Interestingly, genetic disruption of the BBsome complex stalled 5-HT2CR trafficking in late endosomal compartments, resulting in decreased 5-HT2CR ciliary membrane expression in neurons (Guo et al., 2019). This induced a biologically significant impact such that the anorectic effect of the 5-HT2CR agonist lorcaserin was blunted in a rodent model of obesity (Guo et al., 2019). It is possible that reduced Bbip1 expression led to diminished BBsome-mediated receptor trafficking and further disruption of 5-HT2CR function in HC rats. In line with this hypothesis, we demonstrated that the ratio of membrane to cytoplasmic 5-HT2CR protein expression in the mPFC is inversely correlated with cocaine-seeking (Swinford-Jackson et al., 2016). Future studies are required to determine the exact impact of Bbip1 loss and BBsome complex disruption on 5-HT2CR trafficking in key neuronal circuitry involved in cocaine-motivated behaviors.
A core network of predicted PPIs generated by the STRING biological database shows several layers of association (edges) between nine of the eighteen genes of interest. Genes coding for the adenosine A2A receptor (Adora2a; FC: 2.531), dopamine receptor D2 (Drd2; FC: 1.843), G protein subunit gamma 7 (Gng7; FC: 1.389), and protein phosphatase 1 regulatory inhibitor subunit 1B (Ppp1r1b; FC: 1.356) were the most interconnected nodes each with edges reaching to five other genes in the network. In contrast to the current findings, a separate study found Adora2a, Drd2, and Ppp1r1b to be increased in the mPFC of “vulnerable” mice that exhibit heightened cocaine-motivated behavior, relative to “resilient” mice; phenotypes were assigned to each animal through multi-factor analysis of operant behavior observed during cocaine self-administration sessions (e.g., cocaine intake) (Navandar et al., 2021). While both studies implicate these genes to be important regulators of cocaine-motivated behaviors, differential gene expression patterns could be expected to be dependent on the given stage of CUD being modeled (active drug consumption vs. abstinence). The adenosine 2A (A2A) and dopamine D2 (D2) receptors, predicted to physically interact in the current PPI analysis, form heterodimers within the striatum (Prasad et al., 2021; Valle-Leon et al., 2021). Activation of the A2A receptor blocks D2 receptor-mediated inhibition of striatal neurons when in complex, while A2A receptor antagonists enhance dopaminergic signaling (Azdad et al., 2009). The A2A-D2 receptor heteromer is under investigation as a therapeutic target for disorders associated with hypodopaminergic tone (i.e., CUD) (Parsons et al., 1991; Moeller et al., 2012). Most of this research is focused within the striatum; the functional impact of this association within cortical neurons remains underexplored.
Our experimental design allowed for the identification of 309 genes differentially expressed in the mPFC within male rats displaying differential responsiveness to cues previously associated cocaine intake. Intriguingly, but perhaps not surprisingly, we observed that total lifetime cocaine intake associated with the highest level of cue-evoked cocaine-seeking. This is consistent with previous observations that demonstrate greater levels of cocaine exposure associate with heightened CUD-like behavior (e.g., cocaine-primed cocaine-seeking) in rodents (Deroche-Gamonet et al., 2004). Conceivably, it is plausible that the transcriptomic differences detected are directly linked with overall heightened cocaine-motivated behavior during both self-administration (cocaine-taking) and cue test (cocaine-seeking) sessions. A recent publication sought to identify the transcriptomic changes occurring in several mesocorticolimbic nodes in rats trained to self-administer saline or cocaine, animals were euthanized 24 h or 30 days following the final self-administration session (Walker et al., 2018). Although animals in the current study were euthanized at 10 days of abstinence from cocaine, the genes we highlight here still do not overlap with those identified as differentially expressed in the PFC in saline versus cocaine self-administration rats. This suggests variation in cocaine-seeking, but not cocaine exposure, is the primary driver of transcriptomic differences in mPFC of HC rats. Although, the discrepancy between these two findings may be related to species and/or timepoint differences. As we have demonstrated in the past, mechanisms associated with self-administration of cocaine are distinct relative to non-drug rewards such as sucrose (Swinford-Jackson et al., 2016). Inclusion of a sucrose self-administration control group in the future would be useful in parceling out neuromolecular drivers of drug and natural reinforcers. Lastly, female rodents frequently exhibit heightened psychostimulant-seeking following cessation of self-administration relative to male rats (for review) (Becker and Koob, 2016). However, the impact of chronic cocaine exposure and variation in cocaine-seeking on the mPFC transcriptome of female rats is underexplored; future studies are warranted to determine if our findings are generalizable across sex. In conclusion, we propose the 5-HT2CR, BBsome complex, and A2A-D2 receptor heteromer should be further investigated as prioritized candidates for the development of pharmacotherapeutics to reduce relapse vulnerability.
The datasets presented in this study can be found in online repositories. The names of the repository/repositories and accession number(s) can be found below: https://www.ncbi.nlm.nih.gov/geo/, GEO accession: GSE196374.
The animal study was reviewed and approved by University of Texas Medical Branch Institutional Animal Care and Use Committee.
CM, KD, NA, and KC conceived and designed the analysis. CM, KK, and GG contributed to data collection and analysis. All authors wrote and/or edited the manuscript.
This work was supported by the National Institute on Drug Abuse grants T32 DA07287 (CM), P50 DA033935 (KC and NA), and the Center for Addiction Research (including the Rodent In Vivo Assessment Core) at the University of Texas Medical Branch.
We would like to thank Mr. Robert Fox, Ms. Susan Stafford, and Ms. Sonja Stutz for their technical assistance and insightful comments.
The authors declare that the research was conducted in the absence of any commercial or financial relationships that could be construed as a potential conflict of interest.
All claims expressed in this article are solely those of the authors and do not necessarily represent those of their affiliated organizations, or those of the publisher, the editors and the reviewers. Any product that may be evaluated in this article, or claim that may be made by its manufacturer, is not guaranteed or endorsed by the publisher.
5-HT, serotonin; 5-HT2CR, serotonin 5-HT2C receptor; ANOVA, analysis of variance; BBS, Bardet-Biedl Syndrome; CUD, cocaine use disorder; FR, fixed ratio; GEO, Gene Expression Omnibus; GLM, Generalized Linear Model; GPCR, G protein-coupled receptor; HC, high cue; IPA, Ingenuity Pathway Analysis; KEGG, Kyoto Encyclopedia of Genes and Genomes; LC, low cue; mPFC, medial prefrontal cortex; NGS, next-generation sequencing; PPI, protein-protein interaction; RPKM, reads per kilobase per million; SA, self-administration; STRING, Search Tool for the Retrieval of Interacting Genes/Proteins.
Aci-SeChe, S., Sawma, P., Hubert, P., Sturgis, J. N., Bagnard, D., Jacob, L., et al. (2014). Transmembrane recognition of the semaphorin co-receptors neuropilin 1 and plexin A1: Coarse-grained simulations. PLOS One 9, e97779. doi:10.1371/journal.pone.0097779
Afgan, E., Baker, D., Batut, B., Van Den Beek, M., Bouvier, D., Cech, M., et al. (2018). The Galaxy platform for accessible, reproducible and collaborative biomedical analyses: 2018 update. Nucleic Acids Res. 46, W537–W544. doi:10.1093/nar/gky379
Ahmad, F. B., Rossen, L. M., and Sutton, P. (2022). Provisional drug overdose death counts. [Online]. Available: https://www.cdc.gov/nchs/nvss/vsrr/drug-overdose-data.htm#citation (Accessed May 22, 2022).
Anastasio, N. C., Gilbertson, S. R., Bubar, M. J., Agarkov, A., Stutz, S. J., Jeng, Y., et al. (2013). Peptide inhibitors disrupt the serotonin 5-HT2C receptor interaction with phosphatase and tensin homolog to allosterically modulate cellular signaling and behavior. J. Neurosci. 33, 1615–1630. doi:10.1523/JNEUROSCI.2656-12.2013
Anastasio, N. C., Liu, S., Maili, L., Swinford, S. E., Lane, S. D., Fox, R. G., et al. (2014a). Variation within the serotonin (5-HT) 5-HT₂C receptor system aligns with vulnerability to cocaine cue reactivity. Transl. Psychiatry 4, e369. doi:10.1038/tp.2013.131
Anastasio, N. C., Sholler, D. J., Fox, R. G., Stutz, S. J., Merritt, C. R., Bjork, J. M., et al. (2020). Suppression of cocaine relapse-like behaviors upon pimavanserin and lorcaserin co-administration. Neuropharmacology 168, 108009. doi:10.1016/j.neuropharm.2020.108009
Anastasio, N. C., Stutz, S. J., Fox, R. G., Sears, R. M., Emeson, R. B., Dileone, R. J., et al. (2014b). Functional status of the serotonin 5-HT2C receptor (5-HT2CR) drives interlocked phenotypes that precipitate relapse-like behaviors in cocaine dependence. Neuropsychopharmacology 39, 370–382. doi:10.1038/npp.2013.199
Ashburner, M., Ball, C. A., Blake, J. A., Botstein, D., Butler, H., Cherry, J. M., et al. (2000). Gene ontology: Tool for the unification of biology. The gene ontology consortium. Nat. Genet. 25, 25–29. doi:10.1038/75556
Azdad, K., Gall, D., Woods, A. S., Ledent, C., Ferre, S., and Schiffmann, S. N. (2009). Dopamine D2 and adenosine A2A receptors regulate NMDA-mediated excitation in accumbens neurons through A2A-D2 receptor heteromerization. Neuropsychopharmacology 34, 972–986. doi:10.1038/npp.2008.144
Becker, J. B., and Koob, G. F. (2016). Sex differences in animal models: Focus on addiction. Pharmacol. Rev. 68, 242–263. doi:10.1124/pr.115.011163
Bindea, G., Mlecnik, B., Hackl, H., Charoentong, P., Tosolini, M., Kirilovsky, A., et al. (2009). ClueGO: A Cytoscape plug-in to decipher functionally grouped gene ontology and pathway annotation networks. Bioinformatics 25, 1091–1093. doi:10.1093/bioinformatics/btp101
Brandon, T. H., Vidrine, J. I., and Litvin, E. B. (2007). Relapse and relapse prevention. Annu. Rev. Clin. Psychol. 3, 257–284. doi:10.1146/annurev.clinpsy.3.022806.091455
Ching, T., Huang, S., and Garmire, L. X. (2014). Power analysis and sample size estimation for RNA-Seq differential expression. RNA 20, 1684–1696. doi:10.1261/rna.046011.114
Cunningham, K. A., Anastasio, N. C., Fox, R. G., Stutz, S. J., Bubar, M. J., Swinford, S. E., et al. (2013). Synergism between a serotonin 5-HT2A receptor (5-HT2AR) antagonist and 5-HT2CR agonist suggests new pharmacotherapeutics for cocaine addiction. ACS Chem. Neurosci. 4, 110–121. doi:10.1021/cn300072u
Cunningham, K. A., Fox, R. G., Anastasio, N. C., Bubar, M. J., Stutz, S. J., Moeller, F. G., et al. (2011). Selective serotonin 5-HT2C receptor activation suppresses the reinforcing efficacy of cocaine and sucrose but differentially affects the incentive-salience value of cocaine- vs. sucrose-associated cues. Neuropharmacology 61, 513–523. doi:10.1016/j.neuropharm.2011.04.034
Cunningham, K. A., Howell, L. L., and Anastasio, N. C. (2020). “Serotonin neurobiology in cocaine use disorder,” in Handbook of the behavioral neurobiology of serotonin. Editors C. P. Muller, and K. A. Cunningham. 2nd Edition (United Kingdom: Academic Press), 745–802.
Deroche-Gamonet, V., Belin, D., and Piazza, P. V. (2004). Evidence for addiction-like behavior in the rat. Science 305, 1014–1017. doi:10.1126/science.1099020
Domire, J. S., Green, J. A., Lee, K. G., Johnson, A. D., Askwith, C. C., and Mykytyn, K. (2011). Dopamine receptor 1 localizes to neuronal cilia in a dynamic process that requires the Bardet-Biedl syndrome proteins. Cell. Mol. Life Sci. 68, 2951–2960. doi:10.1007/s00018-010-0603-4
Felsing, D. E., Anastasio, N. C., Miszkiel, J. M., Gilbertson, S. R., Allen, J. A., and Cunningham, K. A. (2018). Biophysical validation of serotonin 5-HT2A and 5-HT2C receptor interaction. PLoS One 13, e0203137. doi:10.1371/journal.pone.0203137
Fink, L. H., Anastasio, N. C., Fox, R. G., Rice, K. C., Moeller, F. G., and Cunningham, K. A. (2015). Individual differences in impulsive action reflect variation in the cortical serotonin 5-HT2A receptor system. Neuropsychopharmacology 40, 1957–1968. doi:10.1038/npp.2015.46
Fletcher, P. J., Chintoh, A. F., Sinyard, J., and Higgins, G. A. (2004). Injection of the 5-HT2C receptor agonist Ro60-0175 into the ventral tegmental area reduces cocaine-induced locomotor activity and cocaine self-administration. Neuropsychopharmacology 29, 308–318. doi:10.1038/sj.npp.1300319
Freeman, W. M., Patel, K. M., Brucklacher, R. M., Lull, M. E., Erwin, M., Morgan, D., et al. (2008). Persistent alterations in mesolimbic gene expression with abstinence from cocaine self-administration. Neuropsychopharmacology 33, 1807–1817. doi:10.1038/sj.npp.1301577
Gene Ontology, C. (2021). The gene ontology resource: Enriching a GOld mine. Nucleic Acids Res. 49, D325–D334. doi:10.1093/nar/gkaa1113
Geschwind, D. H., and Konopka, G. (2009). Neuroscience in the era of functional genomics and systems biology. Nature 461, 908–915. doi:10.1038/nature08537
Guo, D. F., Cui, H., Zhang, Q., Morgan, D. A., Thedens, D. R., Nishimura, D., et al. (2016). The BBSome controls energy homeostasis by mediating the transport of the leptin receptor to the plasma membrane. PLoS Genet. 12, e1005890. doi:10.1371/journal.pgen.1005890
Guo, D. F., Lin, Z., Wu, Y., Searby, C., Thedens, D. R., Richerson, G. B., et al. (2019). The BBSome in POMC and AgRP neurons is necessary for body weight regulation and sorting of metabolic receptors. Diabetes 68, 1591–1603. doi:10.2337/db18-1088
Hámor, P. U., Šírová, J., Páleníček, T., Zaniewska, M., Bubeníková-Valešová, V., and Schwendt, M. (2018). Chronic methamphetamine self-administration dysregulates 5-HT2A and mGlu2 receptor expression in the rat prefrontal and perirhinal cortex: Comparison to chronic phencyclidine and MK-801. Pharmacol. Biochem. Behav. 175, 89–100. doi:10.1016/j.pbb.2018.09.007
Harvey-Lewis, C., Li, Z., Higgins, G. A., and Fletcher, P. J. (2016). The 5-HT2C receptor agonist lorcaserin reduces cocaine self-administration, reinstatement of cocaine-seeking and cocaine induced locomotor activity. Neuropharmacology 101, 237–245. doi:10.1016/j.neuropharm.2015.09.028
Hasbi, A., Sivasubramanian, M., Milenkovic, M., Komarek, K., Madras, B. K., and George, S. R. (2020). Dopamine D1-D2 receptor heteromer expression in key brain regions of rat and higher species: Upregulation in rat striatum after cocaine administration. Neurobiol. Dis. 143, 105017. doi:10.1016/j.nbd.2020.105017
Hendershot, C. S., Witkiewitz, K., George, W. H., and Marlatt, G. A. (2011). Relapse prevention for addictive behaviors. Subst. Abuse Treat. Prev. Policy 6, 17. doi:10.1186/1747-597X-6-17
Howell, L. L., and Cunningham, K. A. (2015). Serotonin 5-HT2 receptor interactions with dopamine function: Implications for therapeutics in cocaine use disorder. Pharmacol. Rev. 67, 176–197. doi:10.1124/pr.114.009514
Jasinska, A. J., Stein, E. A., Kaiser, J., Naumer, M. J., and Yalachkov, Y. (2014). Factors modulating neural reactivity to drug cues in addiction: A survey of human neuroimaging studies. Neurosci. Biobehav. Rev. 38, 1–16. doi:10.1016/j.neubiorev.2013.10.013
John, W. S., and Wu, L. T. (2017). Trends and correlates of cocaine use and cocaine use disorder in the United States from 2011 to 2015. Drug Alcohol Depend. 180, 376–384. doi:10.1016/j.drugalcdep.2017.08.031
Johns, S. E., Keyser-Marcus, L., Abbate, A., Boone, E., Van Tassell, B., Cunningham, K. A., et al. (2021). Safety and preliminary efficacy of lorcaserin for cocaine use disorder: A phase I randomized clinical trial. Front. Psychiatry 12, 666945. doi:10.3389/fpsyt.2021.666945
Kalivas, P. W. (2005). How do we determine which drug-induced neuroplastic changes are important? Nat. Neurosci. 8, 1440–1441. doi:10.1038/nn1105-1440
Koob, G., and Kreek, M. J. (2007). Stress, dysregulation of drug reward pathways, and the transition to drug dependence. Am. J. Psychiatry 164, 1149–1159. doi:10.1176/appi.ajp.2007.05030503
Loktev, A. V., Zhang, Q., Beck, J. S., Searby, C. C., Scheetz, T. E., Bazan, J. F., et al. (2008). A BBSome subunit links ciliogenesis, microtubule stability, and acetylation. Dev. Cell 15, 854–865. doi:10.1016/j.devcel.2008.11.001
Mclaughlin, J., and See, R. E. (2003). Selective inactivation of the dorsomedial prefrontal cortex and the basolateral amygdala attenuates conditioned-cued reinstatement of extinguished cocaine-seeking behavior in rats. Psychopharmacol. Berl. 168, 57–65. doi:10.1007/s00213-002-1196-x
Moeller, F. G., Steinberg, J. L., Lane, S. D., Kjome, K. L., Ma, L., Ferre, S., et al. (2012). Increased orbitofrontal brain activation after administration of a selective adenosine A(2A) antagonist in cocaine-dependent subjects. Front. Psychiatry 3, 44. doi:10.3389/fpsyt.2012.00044
Nachury, M. V., Loktev, A. V., Zhang, Q., Westlake, C. J., Peränen, J., Merdes, A., et al. (2007). A core complex of BBS proteins cooperates with the GTPase Rab8 to promote ciliary membrane biogenesis. Cell 129, 1201–1213. doi:10.1016/j.cell.2007.03.053
Navandar, M., Martin-Garcia, E., Maldonado, R., Lutz, B., Gerber, S., and Ruiz De Azua, I. (2021). Transcriptional signatures in prefrontal cortex confer vulnerability versus resilience to food and cocaine addiction-like behavior. Sci. Rep. 11, 9076. doi:10.1038/s41598-021-88363-9
Parsons, L. H., Smith, A. D., and Justice, J. B. (1991). Basal extracellular dopamine is decreased in the rat nucleus accumbens during abstinence from chronic cocaine. Synapse 9, 60–65. doi:10.1002/syn.890090109
Paxinos, G., and Watson, C. (2005). The rat brain in stereotaxic coordinates. Burlington, MA: Elsevier Academic Press.
Pirtle, J. L., Hickman, M. D., Boinpelly, V. C., Surineni, K., Thakur, H. K., and Grasing, K. W. (2019). The serotonin-2C agonist lorcaserin delays intravenous choice and modifies the subjective and cardiovascular effects of cocaine: A randomized, controlled human laboratory study. Pharmacol. Biochem. Behav. 180, 52–59. doi:10.1016/j.pbb.2019.02.010
Prasad, K., De Vries, E. F. J., Elsinga, P. H., Dierckx, R., and Van Waarde, A. (2021). Allosteric interactions between adenosine A2A and dopamine D2 receptors in heteromeric complexes: Biochemical and pharmacological characteristics, and opportunities for PET imaging. Int. J. Mol. Sci. 22, 1719. doi:10.3390/ijms22041719
Price, A. E., Sholler, D. J., Stutz, S. J., Anastasio, N. C., and Cunningham, K. A. (2019). Endogenous serotonin 5-HT2A and 5-HT2C receptors associate in the medial prefrontal cortex. ACS Chem. Neurosci. 10, 3241–3248. doi:10.1021/acschemneuro.8b00669
Scheidecker, S., Etard, C., Pierce, N. W., Geoffroy, V., Schaefer, E., Muller, J., et al. (2014). Exome sequencing of Bardet-Biedl syndrome patient identifies a null mutation in the BBSome subunit BBIP1 (BBS18). J. Med. Genet. 51, 132–136. doi:10.1136/jmedgenet-2013-101785
Shannon, P., Markiel, A., Ozier, O., Baliga, N. S., Wang, J. T., Ramage, D., et al. (2003). Cytoscape: A software environment for integrated models of biomolecular interaction networks. Genome Res. 13, 2498–2504. doi:10.1101/gr.1239303
Shinde, S. R., Nager, A. R., and Nachury, M. V. (2020). Ubiquitin chains earmark GPCRs for BBSome-mediated removal from cilia. J. Cell Biol. 219, e202003020. doi:10.1083/jcb.202003020
Sholler, D. J., Merritt, C. R., Davis-Reyes, B. D., Golovko, G., Anastasio, N. C., and Cunningham, K. A. (2020). Inherent motor impulsivity associates with specific gene targets in the rat medial prefrontal cortex. Neuroscience 435, 161–173. doi:10.1016/j.neuroscience.2020.03.045
Sholler, D. J., Stutz, S. J., Fox, R. G., Boone, E. L., Wang, Q., Rice, K. C., et al. (2019). The 5-HT2A receptor (5-HT2AR) regulates impulsive action and cocaine cue reactivity in male Sprague-Dawley rats. J. Pharmacol. Exp. Ther. 368, 41–49. doi:10.1124/jpet.118.251199
Soto, C. A., Huang-Chi, D., Fox, R. G., Yang, T., Hooson, J., Anastasio, N. C., et al. (2019). In vivo and in vitro analyses of novel peptidomimetic disruptors for the serotonin 5-HT2C receptor interaction with phosphatase and tensin homolog (PTEN). Front. Pharmacol. 10, 907. doi:10.3389/fphar.2019.00907
Swinford-Jackson, S. E., Anastasio, N. C., Fox, R. G., Stutz, S. J., and Cunningham, K. A. (2016). Incubation of cocaine cue reactivity associates with neuroadaptations in the cortical serotonin (5-HT) 5-HT2C receptor (5-HT2CR) system. Neuroscience 324, 50–61. doi:10.1016/j.neuroscience.2016.02.052
Szklarczyk, D., Gable, A. L., Lyon, D., Junge, A., Wyder, S., Huerta-Cepas, J., et al. (2019). STRING v11: Protein-protein association networks with increased coverage, supporting functional discovery in genome-wide experimental datasets. Nucleic Acids Res. 47, D607–D613. doi:10.1093/nar/gky1131
Szklarczyk, D., Gable, A. L., Nastou, K. C., Lyon, D., Kirsch, R., Pyysalo, S., et al. (2021). The STRING database in 2021: Customizable protein-protein networks, and functional characterization of user-uploaded gene/measurement sets. Nucleic Acids Res. 49, D605–D612. doi:10.1093/nar/gkaa1074
Tao, Y. M., Yu, C., Wang, W. S., Hou, Y. Y., Xu, X. J., Chi, Z. Q., et al. (2017). Heteromers of mu opioid and dopamine D1 receptors modulate opioid-induced locomotor sensitization in a dopamine-independent manner. Br. J. Pharmacol. 174, 2842–2861. doi:10.1111/bph.13908
Thomsen, W. J., Grottick, A. J., Menzaghi, F., Reyes-Saldana, H., Espitia, S., Yuskin, D., et al. (2008). Lorcaserin, a novel selective human 5-hydroxytryptamine2C agonist: In vitro and in vivo pharmacological characterization. J. Pharmacol. Exp. Ther. 325, 577–587. doi:10.1124/jpet.107.133348
Valle-Leon, M., Callado, L. F., Aso, E., Cajiao-Manrique, M. M., Sahlholm, K., Lopez-Cano, M., et al. (2021). Decreased striatal adenosine A2A-dopamine D2 receptor heteromerization in schizophrenia. Neuropsychopharmacology 46, 665–672. doi:10.1038/s41386-020-00872-9
Van Den Oever, M. C., Spijker, S., Smit, A. B., and De Vries, T. J. (2010). Prefrontal cortex plasticity mechanisms in drug seeking and relapse. Neurosci. Biobehav. Rev. 35, 276–284. doi:10.1016/j.neubiorev.2009.11.016
Volkow, N. D., Koob, G., and Baler, R. (2015). Biomarkers in substance use disorders. ACS Chem. Neurosci. 6, 522–525. doi:10.1021/acschemneuro.5b00067
Walker, D. M., Cates, H. M., Loh, Y. E., Purushothaman, I., Ramakrishnan, A., Cahill, K. M., et al. (2018). Cocaine self-administration alters transcriptome-wide responses in the brain's reward circuitry. Biol. Psychiatry 84, 867–880. doi:10.1016/j.biopsych.2018.04.009
Wang, Z., Gerstein, M., and Snyder, M. (2009). RNA-seq: A revolutionary tool for transcriptomics. Nat. Rev. Genet. 10, 57–63. doi:10.1038/nrg2484
Wild, C. T., Miszkiel, J. M., Wold, E. A., Soto, C. A., Ding, C., Hartley, R. M., et al. (2019). Design, synthesis, and characterization of 4-undecylpiperidine-2-carboxamides as positive allosteric modulators of the serotonin (5-HT) 5-HT2C receptor. J. Med. Chem. 62, 288–305. doi:10.1021/acs.jmedchem.8b00401
Wold, E. A., Chen, J., Cunningham, K. A., and Zhou, J. (2019). Allosteric modulation of class A GPCRs: Targets, agents, and emerging concepts. J. Med. Chem. 62, 88–127. doi:10.1021/acs.jmedchem.8b00875
Yoon, Y. H., Chen, C. M., and Yi, H. Y. (2014). Unintentional alcohol and drug poisoning in association with substance use disorders and mood and anxiety disorders: Results from the 2010 nationwide inpatient sample. Inj. Prev. 20, 21–28. doi:10.1136/injuryprev-2012-040732
Keywords: cocaine use disorder, cocaine-seeking, medial prefrontal cortex, RNA-sequencing, transcriptomics, 5-HT2CR
Citation: Merritt CR, Smith AE, Khanipov K, Golovko G, Dineley KT, Anastasio NC and Cunningham KA (2022) Heightened cocaine-seeking in male rats associates with a distinct transcriptomic profile in the medial prefrontal cortex. Front. Pharmacol. 13:1022863. doi: 10.3389/fphar.2022.1022863
Received: 19 August 2022; Accepted: 25 November 2022;
Published: 14 December 2022.
Edited by:
Karen K. Szumlinski, University of California, Santa Barbara, United StatesReviewed by:
Nathan James Marchant, VU Medical Center, NetherlandsCopyright © 2022 Merritt, Smith, Khanipov, Golovko, Dineley, Anastasio and Cunningham. This is an open-access article distributed under the terms of the Creative Commons Attribution License (CC BY). The use, distribution or reproduction in other forums is permitted, provided the original author(s) and the copyright owner(s) are credited and that the original publication in this journal is cited, in accordance with accepted academic practice. No use, distribution or reproduction is permitted which does not comply with these terms.
*Correspondence: Kathryn A. Cunningham, a2N1bm5pbmdAdXRtYi5lZHU=
Disclaimer: All claims expressed in this article are solely those of the authors and do not necessarily represent those of their affiliated organizations, or those of the publisher, the editors and the reviewers. Any product that may be evaluated in this article or claim that may be made by its manufacturer is not guaranteed or endorsed by the publisher.
Research integrity at Frontiers
Learn more about the work of our research integrity team to safeguard the quality of each article we publish.