- 1Department of General Surgery, Shandong Provincial Qianfoshan Hospital, Shandong University, Jinan, Shandong, China
- 2Department of General Surgery, The First Affiliated Hospital of Shandong First Medical University, Jinan, Shandong, China
- 3Department of Endocrinology, The First Affiliated Hospital of Shandong First Medical University, Jinan, Shandong, China
Continuously rising trends in diabetes render this disease spectrum an epidemic proportion worldwide. As the disease progresses, the pathological effects of diabetes may impair the normal function of several vital organs, eventually leading to increase the risk of other diabetic comorbidities with advanced fibrosis such as non-alcoholic fatty liver disease, diabetic cardiomyopathy, and diabetic kidney disease. Currently, lifestyle changes and drug therapies of hypoglycemic and lipid-lowering are effective in improving multi-organ function, but therapeutic efficacy is difficult to maintain due to poor compliance and drug reactions. Bariatric surgery, including sleeve gastrectomy and Roux-en-Y gastric bypass surgery, has shown better results in terms of prognosis for diabetes through long-term follow-up. Moreover, bariatric surgery has significant long-term benefits on the function of the heart, liver, kidneys, and other organs through mechanisms associated with reversal of tissue fibrosis. The aim of this review is to describe the impact of type 2 diabetes mellitus on hepatic, cardiac and renal fibrosis and to summarize the potential mechanisms by which bariatric surgery improves multiple organ function, particularly reversal of fibrosis.
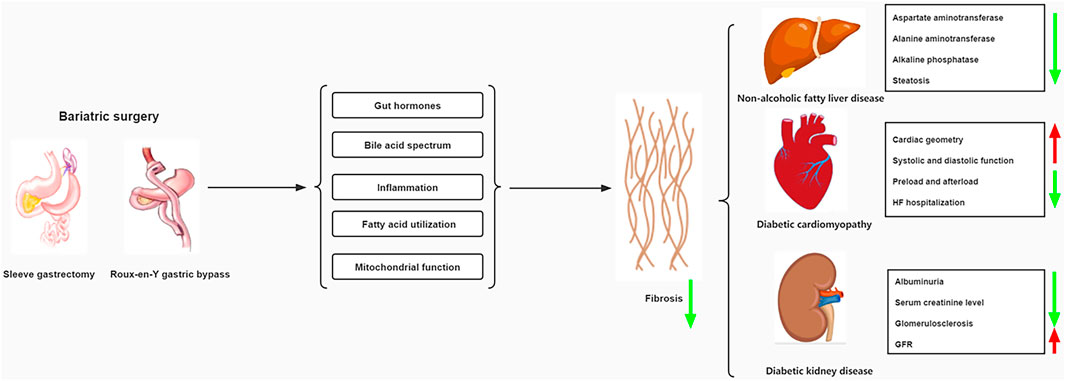
Graphical Abstract. Pharmacological treatment and mechanisms of bariatric surgery for diabetic comorbidities.
1 Introduction
As a global prevalent metabolic disease, diabetes mellitus (DM) is characterized by a lack of insulin production or cellular uptake of insulin (2018). Insulin is essential for controlling glucose levels in the body (Tokarz et al., 2018). In type 2 diabetes mellitus (T2DM), insulin resistance (IR) affects the metabolism of carbohydrates, proteins and lipids, thereby promoting the development of hyperglycemia and hyperlipidemia (Taylor, 2013).
The state of being chronically overweight combined with T2DM substantially increases the incidence of comorbidities such as non-alcoholic fatty liver disease (NAFLD), diabetic cardiomyopathy (DCM) and diabetic kidney disease (DKD) (Al-Sulaiti et al., 2019; La Sala and Pontiroli, 2020). As the degree of organ fibrosis increases, the functions of the liver, heart and kidney are gradually damaged and become more and more serious. The fibrotic process of these diseases has been slowed down to some extent by conservative medical management such as pharmacological interventions and lifestyle changes (Cox et al., 2013). However, low adherence makes it difficult to maintain positive treatment outcomes.
Over the past few decades, the role of bariatric surgery for long-term improvement in metabolic disease has received increasing attention (Hanipah and Schauer, 2020). There is substantial evidence that surgery is better at improving weight loss and T2DM outcomes than nonsurgical interventions, regardless of the type of surgery used. The 1991 National Institutes of Health guidelines recommend consideration of bariatric surgery in patients with a body mass index [BMI (calculated as weight in kilograms divided by height in meters squared)] of at least 40 kg or at least 35 kg with severe obesity-related comorbidities (1991). However, there is growing evidence that bariatric surgery should also be considered in people with T2DM with a BMI of 30–35 kg who are not adequately controlling hyperglycemia despite optimal T2DM medication Operation (Di Lorenzo et al., 2020). As the most commonly used bariatric surgery, Roux-en-Y gastric bypass (RYGB)and Sleeve gastrectomy (SG)not only have significant effect on weight loss and hypoglycemia, but also can improve or even reverse the complications of T2DM in the long term (Arterburn et al., 2020). Therefore, we summarize the effects of treating T2DM on fibrosis and the current status of bariatric surgery, with a focus on the underlying mechanisms by which bariatric surgery improves function and fibrosis of vital organs. This provides evidence that bariatric surgery may be a better treatment for these conditions.
2 Effects of type 2 diabetes mellitus on fibrosis of vital organs
Acute and chronic inflammation often triggers fibrosis in various vital organs (Mack, 2018; Henderson et al., 2020). Inflammation results in damage to resident epithelial cells and often endothelial cells, leading to increased release of inflammatory mediators including cytokines, chemokines, etc. This process contributes to the recruitment of numerous inflammatory cells, including lymphocytes, polymorphonuclear leukocytes, eosinophils, basophils, mast cells, and macrophages. These inflammatory cells trigger the activation of effector cells that drive the process of fibrosis (Wynn, 2004). Fibroblasts and myofibroblasts serve as key fibrotic effectors in several organs and are responsible for the synthesis of extracellular matrix (ECM) proteins (Hinz et al., 2007). The matrix proteins that make up fibrotic scars are mainly composed of interstitial collagens (types I and III), cellular fibronectin, and basement membrane proteins (Rockey et al., 1993). Molecular systems involved in fibrosis include platelet-derived growth factor (PDGF), connective tissue growth factor (CTGF), and the vasoactive peptide system, especially angiotensin II and endothelin-1 (Wynn, 2007).
Liver fibrosis is usually caused by an inflammatory process resulting in injuries to liver cells or bile duct cells. Inflammation leads to the activation of effector cells, which contributes to the deposition of ECM (Bessone et al., 2019). Although multiple effectors synthesize ECM in the liver, hepatic stellate cells (HSCs) appear to be the major source of ECM. There is substantial evidence that hepatic stellate cells transform into myofibroblasts upon injury (Rockey et al., 1993). IR worsens with NAFLD disease progression and is considered a component of the pathogenesis of liver fibrosis (Friedman et al., 2018; Schwabe et al., 2020). One study showed that IR-related hyperinsulinemia directly stimulates HSCs proliferation and type I collagen secretion by activating PI3K and ERK-dependent pathways (Svegliati-Baroni et al., 1999). Inflammation can be caused by IR itself. Simultaneously, hepatocyte stress and death also promote inflammation, leading to macrophage recruitment and secretion of fibrotic-derived mediators, such as transforming growth factor beta (TGF-β), which is central to the liver fibrotic response (Koyama and Brenner, 2017; Tsuchida and Friedman, 2017).
Cardiomyocyte hypertrophy and excessive deposition of ECM are pivotal to the cardiac remodeling after injury (Porter and Turner, 2009). Cardiac fibrosis is generally divided into two types including reactive fibrosis and replacement fibrosis (Liu et al., 2021). Reactive fibrosis occurs in perivascular spaces. Replacement fibrosis occurs at the site of muscle cell loss. Fibrosis in the heart is attributed to fibroblasts, which are the most abundant cell type in the heart muscle. These cells are derived from cardiomyocyte-native fibroblasts, circulating fibroblasts, and fibroblasts resulting from the epithelial-mesenchymal transition (Zeisberg et al., 2007; Moore-Morris et al., 2014). The process by which all these cell types proliferate and differentiate into myofibroblasts after injury is driven by factors such as TGF-β, endothelin-1, and angiotensin II (Ang II). Type I and III interstitial collagen deposition was significantly increased in DCM patients compared with myocardial biopsies in non-diabetic patients, corroborating reports of cardiac interstitial and perivascular fibrosis found at earlier autopsy (van Hoeven and Factor, 1990; Shimizu et al., 1993). It has also been suggested that an important component of the increased cardiac fibrosis caused by T2DM may be replacement fibrosis. The above indicates that the two types of fibrosis are likely to coexist in DCM (Regan et al., 1977).
T2DM and hypertension are two major causes of renal fibrosis (Kaissling et al., 2013). Renal fibrosis is mediated by cellular components (e.g., inflammatory cells) and molecular components (e.g., cytokines, TGF-β, and endothelin-1) (Liu, 2011). The renin-angiotensin-aldosterone axis is particularly important in hypertension-induced fibrosis (Mezzano et al., 2001). The kidney has a unique cellular structure consisting of glomeruli, tubules, interstitium and capillaries. Damage to any of these sites triggers the deposition of ECM. Abnormal and excessive deposition of ECM proteins in the glomerular and interstitial regions are typical features of renal fibrosis (Sun et al., 2016). In DKD, T2DM and systemic hypertension exacerbate glomerular capillary pressure, leading to proteinuria, activation of cytokines and complement, and infiltration of immune cells, ultimately resulting in epithelial and interstitial fibrosis (Liu, 2011).
3 Non-alcoholic fatty liver disease
A large number of diabetic patients develop NAFLD and non-alcoholic steatohepatitis (NASH). NAFLD is the most common cause of chronic liver disease and includes a series of liver diseases ranging from simple steatosis to NASH, fibrosis and cirrhosis (Powell et al., 2021). The incidence of NAFLD is rising due to the diabetes pandemic, with a global prevalence of 24% in the general population (Younossi et al., 2018). NASH is an inflammatory subtype of NAFLD with evidence of steatosis and hepatocellular damage (ballooning) and inflammation, with or without fibrosis (Chalasani et al., 2018). Regardless of the underlying mechanism, significant weight loss is known to have beneficial effects on NASH, with sustained weight loss of 10% being associated with reduced liver fibrosis (Vilar-Gomez et al., 2015). An ideal treatment would effectively reverse liver damage and fibrosis without negatively affecting other metabolic parameters or cardiovascular complications.
3.1 Effects and underlying mechanisms of bariatric surgery in improving non-alcoholic fatty liver disease
Bariatric surgery can improve liver metabolism and reduce biochemical indicators of nonalcoholic fatty liver disease, including aspartate aminotransferase, alanine aminotransferase, and alkaline phosphatase, as well as histological parameters such as steatosis, fibrosis, Lobular inflammation and hepatocyte ballooning (Bower et al., 2015). Reduction in hepatic fat content and improvement in hepatic insulin resistance is one of the earliest beneficial effects of bariatric surgery and thus constitutes an attractive treatment option for NAFLD (Fakhry et al., 2019). General retrospective or cohort studies suggest that improvement in NAFLD after bariatric surgery is more likely than with other interventions; however, current data are insufficient to demonstrate a reduction in liver-specific mortality (Wolfe et al., 2016). Among patients with biopsy-proven NAFLD/NASH, the majority of SG and RYGB patients had essentially normal liver function in the 1-year follow-up, and the two procedures were equally effective in improving liver function (Cherla et al., 2020; de Brito et al., 2021). In addition, NAFLD patients underwent RYGB were more likely to experience early transient deterioration of liver function than those underwent SG, possibly due to postoperative malabsorption or rapid weight loss (Kalinowski et al., 2017). However, RYGB seems to have more advantages in improving hepatic fibrosis (de Brito et al., 2021). Liver biopsies from 180 severely obese patients with biopsy-proven NASH after bariatric surgery showed that 84% of patients observed regression of NASH at 1 year after bariatric surgery, with no apparent recurrence within 1–5 years (p = 0.17). Fibrosis began to decrease 1 year after surgery and continued to decrease within 5 years (Lassailly et al., 2020).
3.1.1 Effects of altered gut hormone levels
GLP-1 is a peptide hormone and neurotransmitter with many metabolic and non-metabolic effects, secreted from the gut by L cells (Drucker, 2018). Increased GLP-1 levels improve beta cell function and insulin sensitivity (Lima et al., 2013). Elevated postprandial GLP-1 levels in patients after SG or RYGB (Jiménez et al., 2013; Jiménez et al., 2014). While most research on GLP-1 has focused on glucose metabolism, GLP-1 functions beyond glycemic control. GLP-1 promotes satiety, enhances insulin release, and inhibits glucagon release in response to nutrient intake (Jiménez et al., 2014). These findings point to possible mechanisms of relative glycemic control and weight loss found after surgery involving GLP-1, glucose homeostasis and T2DM remission. In NASH patients, GLP-1 reduces de novo lipogenesis, lipolysis-induced free fatty acid levels, and triglyceride-derived toxic metabolites (Armstrong et al., 2016b). In animal experiments comparing the therapeutic effects of liraglutide and bariatric surgery, bariatric surgery may enhance GLP-1 secretion by increasing serum bile acids and L cell proliferation in the ileum compared with liraglutide, thereby Ameliorates hepatic steatosis in obese rats (Kashihara et al., 2015). In addition, in a phase 2 trial on NAFLD, liraglutide resulted in some resolution of liver fibrosis (Armstrong et al., 2016a). On this basis, bariatric surgery appears to be more likely to alleviate the degree of liver fibrosis through a GLP-1-mediated mechanism.
In humans, hormone-like members of the FGF family include FGF-15/19 and FGF-21 (Itoh, 2010). The metabolism of FGF-19 and FGF-21 is involved in the regulation of bile acid biosynthesis, glucose metabolism and lipid metabolism in beta cells of pancreatic islets, liver and adipose tissue (Fisher et al., 2011). FGF-15 and FGF-21 have been shown to be protective against liver fibrosis in a mouse model (Zarei et al., 2018; Liu et al., 2020). Plasma FGF-19 and FGF-21 levels were analyzed in 28 patients who received RYGB (Harris et al., 2017). They found that RYGB surgery resulted in increased postprandial plasma FGF-19 concentrations. They also found that plasma FGF-21 exceeded baseline levels by approximately three times after RYGB surgery. Bariatric surgery induces FGF-15/19 expression in the distal small intestine via direct stimulation of FXR by bile acids (Huang et al., 2019; Eiken et al., 2020). Furthermore, FGF-15/19 acts in an endocrine-like manner to improve the metabolic function of insulin target tissues. DJB and SG surgery restores the FGF21 signaling pathway in the liver of high-fat diet/streptomycin-induced diabetic rats and increases FGF21 sensitivity (Liu et al., 2019).
PYY, also known as a short peptide of pancreatic polypeptide YY3-36, is released by L cells in the distal small intestine and colon (le Roux and Bloom, 2005). The main effects of PYY involve the central regulation of appetite as well as the regulation of blood glucose homeostasis (Batterham et al., 2003). In mouse models, PYY modulates weight loss after bypass surgery (Chandarana et al., 2011), while increased PYY has been observed in patients after bariatric surgery, both as a result of post-operative anatomical changes in the gastrointestinal tract (Magouliotis et al., 2017). Notably, several studies suggest that PYY3-36 levels are reduced in obesity and elevated after gastric bypass surgery, possibly contributing to increased satiety after this procedure (Price and Bloom, 2014; Guida et al., 2017). Overall, as PYY is strongly associated with weight loss and NAFLD after bariatric surgery, its role in metabolism warrants further investigation. Although more research is needed, elevated levels of PYY are associated with stabilization of blood glucose levels, metabolism, and body weight, which directly affect the incidence and complications of obesity and T2DM.
3.1.2 Changes in bile acid metabolism, transport and signal transduction
Both RYGB and SG have been shown to induce sustained increases in systemic bile acid (BA) concentrations and intestinal BA signaling, which are associated with improvements in insulin resistance and glycemic control (Belgaumkar et al., 2016; Risstad et al., 2017). Bile acids are signaling molecules and metabolic integrators, which involve in activating nuclear farnesoid X receptor (FXR) and membrane Takeda G-protein-coupled receptor 5 (TGR5) to regulate glucose, lipid and energy metabolism (Chiang and Ferrell, 2020). However, BA includes more than 20 components, each of which may have specific effects on metabolism (de Aguiar Vallim et al., 2013). Notably, 12α-hydroxylated bile acids promote liver fibrosis through TGR5-mediated p38MAPK and ERK1/2 signaling, but these bile acids were significantly reduced after SG (Wang et al., 2017; Xie et al., 2021). RYGB upregulates the expression of hepatic BA targets FXR and peroxisome proliferator-activated receptor-α (PPARα) in severely obese patients, and these changes are accompanied by regression of NASH and T2DM after 1 year (Francque et al., 2015; Mazzini et al., 2019). BA treatment of primary and immortalized human hepatocytes induces FXR and PPARα to downregulate glycolysis and restrict glucose entry into the Krebs cycle, thereby inhibiting fatty acid synthesis from excess glucose (Cai and Sewer, 2013). PPARα activation combined with PPARβ/δ agonists improves steatosis, inflammation and fibrosis in preclinical models of NAFLD, identifying new potential therapeutic areas (Pawlak et al., 2015). Activation of the gut FXR/FGF19 signaling pathway inhibits the key lipogenic enzyme, acetyl-CoA carboxylase, supporting the downregulation of de novo fatty acid production by hepatic FXR and indirectly promoting fatty acid oxidation by downregulating the expression of carnitine palmitoyl transferase 1α (CPT1α) serving as a key mitochondrial fatty acid transporter. Recent studies have shown that CPT1α is elevated in HSCs from fibrotic patients and mouse models of fibrosis, and that CPT1α induces activation of these cells leading to fibrosis (Fondevila et al., 2022).
Although studies of the effects of SG on FXR and its downstream targets are lacking in humans, the observed increase in post-operative systemic FGF19 concentrations is consistent with enhanced gut FXR signaling following SG. Likewise, findings in FXR-deficient mouse models receiving SG suggest that FXR is essential to achieve the full metabolic benefits of surgery, including postoperative recovery of glucose tolerance and reduced energy intake (Ryan et al., 2014; Cavin et al., 2016). It is worth mentioning that FXR agonists have emerged as a potential therapy against liver fibrosis, despite the issue of side effects (Li et al., 2021). In addition to the direct effects of FXR signaling on hepatic BA, glucose, and fat metabolism, inhibition of intestinal FXR after RYGB is also associated with upregulation of TGR5 (Zhai et al., 2018), which may contribute to postprandial insulin hormone pancreatic elevation in enteroendocrine L cells after surgery Increased levels of GLP1 and PYY suggest a multifaceted antidiabetic effect (Trabelsi et al., 2015; Li et al., 2019).
3.1.3 Reversing inflammation
In a group of patients undergoing bariatric surgery, the expression of inflammatory genes in subcutaneous and visceral adipose tissue was proportional to the severity of NAFLD histology (Rosso et al., 2019). One year post-surgery, SG improved liver histology, especially the degree of fibrosis, in all NASH patients (Cabré et al., 2019). At the same time, there were significant differences in C-C chemokine receptor type 2 (CCR2) and Tumor necrosis factor-α (TNF-α) in preoperative plasma and liver between NASH patients and non-NASH patients, so it is considered that the improvement of liver fibrosis involves the regulation of oxidative stress and inflammation levels. TNF-α, a key cytokine upregulated in obesity, is mainly produced by macrophages (Kunz et al., 2021). Serum levels of various inflammatory markers correlate with liver inflammation, CD11c+ CD206+ and CCR2+ macrophage expansion in visceral adipose tissue of patients with NASH and/or fibrosis (Wentworth et al., 2010; du Plessis et al., 2015). Transplantation of visceral adipose tissue in obese mice increased hepatic macrophage accumulation and exacerbated steatohepatitis compared with a high cholesterol diet model (Bijnen et al., 2018).
Toll-like receptor 4 (TLR4) is considered to be one of the cutting-edge cross-communicators between gut bacteria and the host regulating inflammatory signaling and energy homeostasis (Sala et al., 2020). A pathway specific to liver fibrosis involves TLR4 and is associated with enhanced TGF-β signaling (Seki et al., 2007). TLR4 is activated on the surface of HSCs by gut bacterial lipopolysaccharides from the gut, triggering cell activation and fibrosis formation, thereby linking fibrosis to the microbiome (Sorribas et al., 2019). TLR4 expression in patients with NAFLD is associated with fibrosis (Vespasiani-Gentilucci et al., 2015). It has been shown that RYGB specifically reduces TLR4 expression in the small and large intestines of C57Blc/6J mice, and that TLR4 and myeloid differentiation factor 8 (MyD88, a key downstream signaling regulators) are required for RYGB-induced metabolic responses (Abu El Haija et al., 2021).
4 Diabetic cardiomyopathy
There is a broad link between T2DM and cardiovascular disease (CVD). T2DM causes cardiomyopathy and increases the risk of heart failure (HF) even independent of traditional HF risks, including hypertension (HTN), coronary heart disease (CHD), and valvular heart disease (VHD) (Dillmann, 2019). DCM is characterized by abnormal diastolic relaxation at an early stage (Jia et al., 2018). Numerous pathophysiological abnormalities collectively promote interstitial fibrosis, diastolic dysfunction, and later systolic dysfunction in cardiac tissue, resulting in clinical HF syndrome. A retrospective cohort study of 8,231 people with T2DM showed a 2.5-fold increased risk of HF in people with T2DM (Nichols et al., 2004). In addition, an observational study involving 25,958 men and 22,900 women showed that a 1% increase in HbA1c was associated with an 8% increased risk of HF regardless of blood pressure, obesity, age and the presence of coronary heart disease (Iribarren et al., 2001). Therefore, glycemic control in patients with T2DM is a key mechanism to prevent cardiac dysfunction and HF.
4.1 Effects and underlying mechanisms of bariatric surgery in improving diabetic cardiomyopathy
Bariatric surgery significantly improves cardiac geometry, function, providing a unique and effective treatment for patients with DCM and HF (Roth et al., 2020). Studies have shown that the remission rate of high blood pressure is 60%–70% within a year after bariatric surgery (Benaiges et al., 2019). In addition to controlling blood pressure, RYGB and SG are effective in reducing cardiovascular risk and the incidence of sudden macrovascular disease (Fisher et al., 2018; Blanco et al., 2019). In severely obese patients with T2DM, weight loss after bariatric surgery improves left ventricular end diastolic dimension (LVDD) (Elagizi et al., 2018). Notably, many patients with pre-existing cardiovascular disease can reduce or completely discontinue cardiovascular medications following bariatric surgery (English and Williams, 2018). Furthermore, according to a large retrospective analysis, bariatric surgery reduces in-hospital mortality by nearly 50% and length of stay in hospitalized patients with HF (Aleassa et al., 2019).
4.1.1 Reducing heart load and cardiac fatty acid utilization
Preload and afterload, as well as systemic and local energy requirements, are decreased after bariatric surgery due to significant reductions in adipose tissue and mass (Lin et al., 2011). Concentrations of vasoconstrictors (e.g., enkephalinase, angiotensin II, renin, and endothelin-1) decreased significantly (p < 0.05) 6 months after RYGB by follow-up. These changes promote beneficial “reverse remodeling,” improve fibrosis and the incidence of ventricular tachycardia (Massare et al., 2010) and reduce the incidence of sudden death (Spinale, 2007). Due to lipid metabolism disorder, excessive fat deposition in the heart can create a lipotoxic environment, and at the same time increase the uptake and utilization rate of fatty acids by the myocardium, thereby impairing cardiac function over time (Labbé et al., 2012; Shulman, 2014). By studying positron emission tomography scans of the hearts of 12 obese subjects with T2DM before and after bariatric surgery, Carreau et al. (2020) found that bariatric surgery reduces cardiac fatty acid utilization and enhances left ventricular function.
4.1.2 Improving mitochondrial function and regulating calcium homeostasis
Both cellular and tissue mitochondrial respiration were increased in patients receiving RYGB or SG compared to preoperatively (Nijhawan et al., 2013). Evidence suggests that in a rat model of DCM, SG restores mitochondrial dysfunction and fragmentation by activating 5′ adenosine monophosphate-activated protein kinase (AMPK) signaling (Li et al., 2022). Myocardial Ca2+ transients exhibit significantly higher amplitudes and faster decay after SG and DJB, suggesting that bariatric surgery can promote myocardial Ca2+ homeostasis in DCM rats (Huang et al., 2018). The above-mentioned postoperative repair of mitochondrial function and Ca2+ homeostasis can improve cardiac structure and cardiac function by reducing myocardial collagen deposition and fibrosis (Huang et al., 2018; Li et al., 2022). Despite this, there are currently few clinical studies on specific improvements in postoperative cardiac mitochondrial function and calcium homeostasis in patients undergoing bariatric surgery (Kindel and Strande, 2018).
4.1.3 Adjusting the enterocardiac axis and inhibiting inflammation
Other beneficial effects of bariatric surgery include altered gastrointestinal microbiota, changed bile acid profile, and increased postprandial GLP-1 in the days following surgery (Chambers et al., 2011; Ryan et al., 2014). Bariatric surgery may beneficially alter the enterocardiac axis through one or more of these mechanisms to further improve cardiac function beyond the effects of weight loss. Gut-secreted GLP-1 in cardiomyocytes is now known to lead to decreased apoptosis and increased glucose uptake, and to reverse myocardial fibrosis, independent of canonical insulin signaling (Bose et al., 2005; Helmstädter et al., 2020). Increased level of GLP-1 after bariatric surgery can reverse endothelial dysfunction and restore the endothelial protective properties of high-density lipoprotein (HDL) (Osto et al., 2015). In addition, GLP-1R agonists have been used to treat ischemia-reperfusion injury and HF.
Adiponectin (APN), the most abundant product of white adipose tissue (WAT), has been proposed as a potential prognostic biomarker and therapeutic target in patients with cardiometabolic disease, which is inversely associated with obesity (Messina et al., 2018). Adiponectin levels are associated with a reduction in total fat mass and atherosclerosis risk (Umeda et al., 2013) and are significantly elevated after bariatric surgery (Valenzano et al., 2020). Current studies have shown that APN reduces the degree of cardiac fibrosis by activating autophagy in macrophages and inhibiting Ang II-induced inflammatory responses (Qi et al., 2014). Serum Hsp60 (a kind of adipokine) was elevated in patients with DCM but decreased after bariatric surgery. As a predictor of cardiovascular disease, Hsp60 correlates with inflammatory markers and may be a molecular link between adipose tissue inflammation and the development of cardiovascular disease (Sell et al., 2017). TLR4 mediates HSP60-induced apoptosis in cardiac myocytes from WT mice, which appears to be involved in replacement fibrosis (Heiserman et al., 2015).
5 Diabetic kidney disease
Rising global prevalence of T2DM and chronic kidney disease (CKD) has prompted research into DKD (Anders et al., 2018). The leading cause of kidney failure worldwide is DKD (Reidy et al., 2014). More serious, All-cause mortality in DKD patients was 31% and 10-year mortality was 20% (Afkarian et al., 2013). Hyperglycemia is the main cause of the development of DKD. The earliest signs of DKD are microalbuminuria (>30 mg/day), which progresses to macroalbuminuria (>300 mg/day) and decreased glomerular filtration rate (GFR), culminating in end-stage renal disease (ESRD) (Rocco and Berns, 2009). Metabolic changes associated with T2DM can lead to glomerular hypertrophy, glomerulosclerosis, and tubulointerstitial inflammation and fibrosis (Tervaert et al., 2010). More than 40% of people with obesity and T2DM develop renal impairment as microvascular complications, and 30% go on to develop end-stage renal disease (Adler et al., 2003). Tight glycemic control significantly reduces the incidence of diabetic nephropathy, suggesting that hyperglycemia-induced metabolic changes, including changes in energy utilization and mitochondrial dysfunction, play a key role in disease development (Reidy et al., 2014).
5.1 Effects and underlying mechanisms of bariatric surgery in improving diabetic kidney disease
A large retrospective observational cohort study of patients with T2DM showed that bariatric surgery significantly reduced the incidence of kidney disease relative to nonsurgical interventions (6.4% vs. 14%) (O’Brien et al., 2018). For patients with pre-existing kidney disease, almost 80% improvement in albuminuria in the short and long term after bariatric surgery including RYGB and SG (Young et al., 2019). Obvious renal histopathological lesions including glomerulosclerosis, mesangial matrix expansion, and podocyte hypertrophy are prevalent in T2DM and can be ameliorated by bariatric surgery (Navarro-Díaz et al., 2006). This is consistent with the anti-proteinuric effect of SG and RYGB in STZ-induced DKD rats (Canney et al., 2020; Xiong et al., 2020). Meanwhile, improvements in glomerular structure and ultrastructure were also observed in animal models. An observational retrospective cohort study demonstrated that bariatric surgery, particularly RYGB surgery, significantly improved Estimated glomerular filtration rate (eGFR) for up to 3 years. Bariatric surgery should be considered for patients with DKD who have failed lifestyle changes and medical therapy, which have potential benefits in slowing DKD progression (Friedman and Wolfe, 2016). As a means to improve outcomes in patients with renal disease, bariatric surgery has myriad potential roles that require more clinically relevant studies to explore (Martin et al., 2020).
Bariatric surgery reduces weight and improves hypertension and hyperglycemia, which may explain some of the positive effects on albuminuria (Navarro-Díaz et al., 2006; Getty et al., 2012). Important mechanisms of DKD pathogenesis are advanced glycation end products and oxidative stress (Giacco and Brownlee, 2010). However, the role of inflammation is increasingly recognized as key to the progression of kidney disease (Fenske et al., 2013).
Chronic low-grade inflammation is often associated with T2DM (Sabatino et al., 2017). C-reactive protein (CRP) is associated with the development of DKD (McDonald et al., 2004; Rao, 2012). CRP promotes renal fibrosis and inflammation in a TGF-β-dependent manner (You et al., 2021). Bariatric surgery may reduce CRP and repair the pro-inflammatory chemokine and cytokine milieu, while enhancing anti-inflammatory chemokine and cytokine production (Illán-Gómez et al., 2012). CC-chemokine ligand 2 (CCL2), a protein closely associated with obesity and diabetic nephropathy (Tesch, 2008). Bariatric surgery-induced reduction in CCL-2-related inflammation is associated with improved renal function and reduced renal fibrosis (Bueter et al., 2010; Monte et al., 2012; Fenske et al., 2013; Wu et al., 2014). Similar improvement in urinary CCL-2/creatinine ratio at 4 weeks after RYGB and SG, but additional effect of RYGB in reducing chemokine ligand 18 (CCL-18) (Bueter et al., 2010). The AMPK/TGF-β pathway has been identified as a key pathway regulating inflammation and profibrosis in diabetic nephropathy. In a rodent kidney model, RYGB partially reversed DKD fibrosis by reducing TGF-β signaling (Vangoitsenhoven et al., 2020). At the same time, renal fibrosis was reversed after surgery, which may be related to the blockade of the renin-angiotensin system (Lambers Heerspink et al., 2013; Berney et al., 2021). Furthermore, several recent observations suggest that elevated endogenous gut hormones, such as GLP-1, may be an intermediate mediator of the anti-inflammatory effects of RYGB (Navaneethan et al., 2010).
6 Summary and future perspective
In recent years, bariatric surgery has grown as an emerging treatment for T2DM and related comorbidities. Currently, there is a growing body of strong evidence for the efficacy and safety of bariatric surgery (Arterburn et al., 2020). Therefore, for severely obese patients with T2DM, it is necessary to add bariatric surgery to the treatment decision-making option.
High-quality evidence from randomized controlled trials suggests that bariatric surgery is superior to medical therapy for enhancing glycemic control and achieving T2DM remission. The continued global prevalence of NAFLD, DCM and DKD as comorbidities of T2DM means that this is an important priority for healthcare and research. Bariatric surgery to treat these major T2DM comorbidities has obvious advantages over medical treatment in terms of duration and improvement.
In T2DM, fibrosis in various organs is driven in part by inflammation caused by IR itself. TGF-β is a common mediator of organ fibrosis in T2DM (Rockey et al., 2015). Unfortunately, the role of TGF-β in bariatric surgery ameliorating myocardial fibrosis has not yet been elucidated. A liver-specific fibrotic pathway involving TLR4 has also been reported in studies of cardiomyocyte fibrosis. It can be seen from these that the molecular systems related to T2DM-induced fibrosis are very extensive and complex, and need to be further explored and clarified.
Future research should focus on identifying key transcriptional, proteomic, and metabolomic changes that occur in vital organs of the body such as the heart, liver, and kidneys after bariatric surgery, which will help differentiate between the benefits and harms of bariatric surgery in people with T2DM impact and can inform the design of novel medical treatments that replicate the beneficial effects of surgery, while providing ideas for addressing the adverse consequences of surgery, thereby maximizing its therapeutic benefits.
Author contributions
HD and YZ organize the article structure and write the full text. XM, ZZ, QX, and CL search literature. SD and BL make figure. JZ, LL, MZ, and GZ directed and revised the manuscript for this review.
Funding
This review was supported by the National Natural Science Foundation of China (Grant No. 81873647) and Major Basic Research Project of Natural Science Foundation of Shandong Province (Grant No. ZR2020ZD15).
Conflict of interest
The authors declare that the research was conducted in the absence of any commercial or financial relationships that could be construed as a potential conflict of interest.
Publisher’s note
All claims expressed in this article are solely those of the authors and do not necessarily represent those of their affiliated organizations, or those of the publisher, the editors and the reviewers. Any product that may be evaluated in this article, or claim that may be made by its manufacturer, is not guaranteed or endorsed by the publisher.
References
Abu El Haija, M., Ye, Y., Chu, Y., Herz, H., Linden, B., Shahi, S. K., et al. (2021). Toll-like receptor 4 and myeloid differentiation factor 88 are required for gastric bypass-induced metabolic effects. Surg. Obes. Relat. Dis. 17 (12), 1996–2006. doi:10.1016/j.soard.2021.07.019
Adler, A. I., Stevens, R. J., Manley, S. E., Bilous, R. W., Cull, C. A., Holman, R. R., et al. (2003). Development and progression of nephropathy in type 2 diabetes: The United Kingdom prospective diabetes study (UKPDS 64). Kidney Int. 63 (1), 225–232. doi:10.1046/j.1523-1755.2003.00712.x
Afkarian, M., Sachs, M. C., Kestenbaum, B., Hirsch, I. B., Tuttle, K. R., Himmelfarb, J., et al. (2013). Kidney disease and increased mortality risk in type 2 diabetes. J. Am. Soc. Nephrol. 24 (2), 302–308. doi:10.1681/asn.2012070718
Al-Sulaiti, H., Diboun, I., Agha, M. V., Mohamed, F. F. S., Atkin, S., Dömling, A. S., et al. (2019). Metabolic signature of obesity-associated insulin resistance and type 2 diabetes. J. Transl. Med. 17 (1), 348. doi:10.1186/s12967-019-2096-8
Aleassa, E. M., Khorgami, Z., Kindel, T. L., Tu, C., Tang, W. H. W., Schauer, P. R., et al. (2019). Impact of bariatric surgery on heart failure mortality. Surg. Obes. Relat. Dis. 15 (7), 1189–1196. doi:10.1016/j.soard.2019.03.021
Anders, H. J., Huber, T. B., Isermann, B., and Schiffer, M. (2018). CKD in diabetes: Diabetic kidney disease versus nondiabetic kidney disease. Nat. Rev. Nephrol. 14 (6), 361–377. doi:10.1038/s41581-018-0001-y
Armstrong, M. J., Gaunt, P., Aithal, G. P., Barton, D., Hull, D., Parker, R., et al. (2016a). Liraglutide safety and efficacy in patients with non-alcoholic steatohepatitis (LEAN): A multicentre, double-blind, randomised, placebo-controlled phase 2 study. Lancet 387 (10019), 679–690. doi:10.1016/s0140-6736(15)00803-x
Armstrong, M. J., Hull, D., Guo, K., Barton, D., Hazlehurst, J. M., Gathercole, L. L., et al. (2016b). Glucagon-like peptide 1 decreases lipotoxicity in non-alcoholic steatohepatitis. J. Hepatol. 64 (2), 399–408. doi:10.1016/j.jhep.2015.08.038
Arterburn, D. E., Telem, D. A., Kushner, R. F., and Courcoulas, A. P. (2020). Benefits and risks of bariatric surgery in adults: A review. Jama 324 (9), 879–887. doi:10.1001/jama.2020.12567
American Diabetes Association (2018). 2. Classification and diagnosis of diabetes: Standards of medical care in diabetes-2018. Diabetes Care 41 (1), S13-S27–s27. doi:10.2337/dc18-S002
Batterham, R. L., Cohen, M. A., Ellis, S. M., Le Roux, C. W., Withers, D. J., Frost, G. S., et al. (2003). Inhibition of food intake in obese subjects by peptide YY3-36. N. Engl. J. Med. 349 (10), 941–948. doi:10.1056/NEJMoa030204
Belgaumkar, A. P., Vincent, R. P., Carswell, K. A., Hughes, R. D., Alaghband-Zadeh, J., Mitry, R. R., et al. (2016). Changes in bile acid profile After laparoscopic sleeve gastrectomy are associated with improvements in metabolic profile and fatty liver disease. Obes. Surg. 26 (6), 1195–1202. doi:10.1007/s11695-015-1878-1
Benaiges, D., Climent, E., Goday, A., Flores-Le Roux, J. A., and Pedro-Botet, J. (2019). Bariatric surgery and hypertension: Implications and perspectives after the GATEWAY randomized trial. Cardiovasc. Diagn. Ther. 9 (1), 100–103. doi:10.21037/cdt.2018.10.04
Berney, M., Vakilzadeh, N., Maillard, M., Faouzi, M., Grouzmann, E., Bonny, O., et al. (2021). Bariatric surgery induces a differential effect on plasma aldosterone in comparison to dietary advice alone. Front. Endocrinol. 12, 745045. doi:10.3389/fendo.2021.745045
Bessone, F., Razori, M. V., and Roma, M. G. (2019). Molecular pathways of nonalcoholic fatty liver disease development and progression. Cell. Mol. Life Sci. 76 (1), 99–128. doi:10.1007/s00018-018-2947-0
Bijnen, M., Josefs, T., Cuijpers, I., Maalsen, C. J., van de Gaar, J., Vroomen, M., et al. (2018). Adipose tissue macrophages induce hepatic neutrophil recruitment and macrophage accumulation in mice. Gut 67 (7), 1317–1327. doi:10.1136/gutjnl-2016-313654
Blanco, D. G., Funes, D. R., Giambartolomei, G., Lo Menzo, E., Szomstein, S., and Rosenthal, R. J. (2019). Laparoscopic sleeve gastrectomy versus roux-en-Y gastric bypass in cardiovascular risk reduction: A match control study. Surg. Obes. Relat. Dis. 15 (1), 14–20. doi:10.1016/j.soard.2018.09.488
Bose, A. K., Mocanu, M. M., Carr, R. D., Brand, C. L., and Yellon, D. M. (2005). Glucagon-like peptide 1 can directly protect the heart against ischemia/reperfusion injury. Diabetes 54 (1), 146–151. doi:10.2337/diabetes.54.1.146
Bower, G., Toma, T., Harling, L., Jiao, L. R., Efthimiou, E., Darzi, A., et al. (2015). Bariatric surgery and non-alcoholic fatty liver disease: A systematic review of liver biochemistry and histology. Obes. Surg. 25 (12), 2280–2289. doi:10.1007/s11695-015-1691-x
Bueter, M., Dubb, S. S., Gill, A., Joannou, L., Ahmed, A., Frankel, A. H., et al. (2010). Renal cytokines improve early after bariatric surgery. Br. J. Surg. 97 (12), 1838–1844. doi:10.1002/bjs.7264
Cabré, N., Luciano-Mateo, F., Fernández-Arroyo, S., Baiges-Gayà, G., Hernández-Aguilera, A., Fibla, M., et al. (2019). Laparoscopic sleeve gastrectomy reverses non-alcoholic fatty liver disease modulating oxidative stress and inflammation. Metabolism. 99, 81–89. doi:10.1016/j.metabol.2019.07.002
Cai, K., and Sewer, M. B. (2013). Diacylglycerol kinase θ couples farnesoid X receptor-dependent bile acid signalling to Akt activation and glucose homoeostasis in hepatocytes. Biochem. J. 454 (2), 267–274. doi:10.1042/bj20130609
Canney, A. L., Cohen, R. V., Elliott, J. A., C, M. A., Martin, W. P., Docherty, N. G., et al. (2020). Improvements in diabetic albuminuria and podocyte differentiation following Roux-en-Y gastric bypass surgery. Diab. Vasc. Dis. Res. 17 (1), 1479164119879039. doi:10.1177/1479164119879039
Carreau, A. M., Noll, C., Blondin, D. P., Frisch, F., Nadeau, M., Pelletier, M., et al. (2020). Bariatric surgery rapidly decreases cardiac dietary fatty acid partitioning and hepatic insulin resistance through increased intra-abdominal adipose tissue storage and reduced spillover in type 2 diabetes. Diabetes 69 (4), 567–577. doi:10.2337/db19-0773
Cavin, J. B., Couvelard, A., Lebtahi, R., Ducroc, R., Arapis, K., Voitellier, E., et al. (2016). Differences in alimentary glucose absorption and intestinal disposal of blood glucose after roux-en-Y gastric bypass vs sleeve gastrectomy. Gastroenterology 150 (2), 454–464. e459. doi:10.1053/j.gastro.2015.10.009
Chalasani, N., Younossi, Z., Lavine, J. E., Charlton, M., Cusi, K., Rinella, M., et al. (2018). The diagnosis and management of nonalcoholic fatty liver disease: Practice guidance from the American Association for the Study of Liver Diseases. Hepatology 67 (1), 328–357. doi:10.1002/hep.29367
Chambers, A. P., Jessen, L., Ryan, K. K., Sisley, S., Wilson-Pérez, H. E., Stefater, M. A., et al. (2011). Weight-independent changes in blood glucose homeostasis after gastric bypass or vertical sleeve gastrectomy in rats. Gastroenterology 141 (3), 950–958. doi:10.1053/j.gastro.2011.05.050
Chandarana, K., Gelegen, C., Karra, E., Choudhury, A. I., Drew, M. E., Fauveau, V., et al. (2011). Diet and gastrointestinal bypass-induced weight loss: The roles of ghrelin and peptide YY. Diabetes 60 (3), 810–818. doi:10.2337/db10-0566
Cherla, D. V., Rodriguez, N. A., Vangoitsenhoven, R., Singh, T., Mehta, N., McCullough, A. J., et al. (2020). Impact of sleeve gastrectomy and Roux-en-Y gastric bypass on biopsy-proven non-alcoholic fatty liver disease. Surg. Endosc. 34 (5), 2266–2272. doi:10.1007/s00464-019-07017-0
Chiang, J. Y. L., and Ferrell, J. M. (2020). Bile acid receptors FXR and TGR5 signaling in fatty liver diseases and therapy. Am. J. Physiol. Gastrointest. Liver Physiol. 318 (3), G554-G573–g573. doi:10.1152/ajpgi.00223.2019
Cox, D. J., Gill Taylor, A., Dunning, E. S., Winston, M. C., Luk Van, I. L., McCall, A., et al. (2013). Impact of behavioral interventions in the management of adults with type 2 diabetes mellitus. Curr. Diab. Rep. 13 (6), 860–868. doi:10.1007/s11892-013-0423-7
de Aguiar Vallim, T. Q., Tarling, E. J., and Edwards, P. A. (2013). Pleiotropic roles of bile acids in metabolism. Cell. Metab. 17 (5), 657–669. doi:10.1016/j.cmet.2013.03.013
de Brito, E. S. M. B., Tustumi, F., de Miranda Neto, A. A., Dantas, A. C. B., Santo, M. A., and Cecconello, I. (2021). Gastric bypass compared with sleeve gastrectomy for nonalcoholic fatty liver disease: A systematic review and meta-analysis. Obes. Surg. 31 (6), 2762–2772. doi:10.1007/s11695-021-05412-y
Di Lorenzo, N., Antoniou, S. A., Batterham, R. L., Busetto, L., Godoroja, D., Iossa, A., et al. (2020). Clinical practice guidelines of the European association for endoscopic surgery (EAES) on bariatric surgery: Update 2020 endorsed by IFSO-ec, EASO and ESPCOP. Surg. Endosc. 34 (6), 2332–2358. doi:10.1007/s00464-020-07555-y
Dillmann, W. H. (2019). Diabetic cardiomyopathy. Circ. Res. 124 (8), 1160–1162. doi:10.1161/circresaha.118.314665
Drucker, D.J. (2018). Mechanisms of Action and Therapeutic Application of Glucagon-like Peptide-1. Cell Metab. 27 (4), 740–756. doi:10.1016/j.cmet.2018.03.001
du Plessis, J., van Pelt, J., Korf, H., Mathieu, C., van der Schueren, B., Lannoo, M., et al. (2015). Association of Adipose Tissue Inflammation With Histologic Severity of Nonalcoholic Fatty Liver Disease. Gastroenterology 149 (3), 635–648. doi:10.1053/j.gastro.2015.05.044
Eiken, A., Fuglsang, S., Eiken, M., Svane, M. S., Kuhre, R. E., Wewer Albrechtsen, N. J., et al. (2020). Bilio-enteric flow and plasma concentrations of bile acids after gastric bypass and sleeve gastrectomy. Int. J. Obes. 44 (9), 1872–1883. doi:10.1038/s41366-020-0578-7
Elagizi, A., Kachur, S., Lavie, C. J., Carbone, S., Pandey, A., Ortega, F. B., et al. (2018). An overview and update on obesity and the obesity paradox in cardiovascular diseases. Prog. Cardiovasc. Dis. 61 (2), 142–150. doi:10.1016/j.pcad.2018.07.003
English, W. J., and Williams, D. B. (2018). Metabolic and bariatric surgery: An effective treatment option for obesity and cardiovascular disease. Prog. Cardiovasc. Dis. 61 (2), 253–269. doi:10.1016/j.pcad.2018.06.003
Fakhry, T. K., Mhaskar, R., Schwitalla, T., Muradova, E., Gonzalvo, J. P., and Murr, M. M. (2019). Bariatric surgery improves nonalcoholic fatty liver disease: A contemporary systematic review and meta-analysis. Surg. Obes. Relat. Dis. 15 (3), 502–511. doi:10.1016/j.soard.2018.12.002
Fenske, W. K., Dubb, S., Bueter, M., Seyfried, F., Patel, K., Tam, F. W., et al. (2013). Effect of bariatric surgery-induced weight loss on renal and systemic inflammation and blood pressure: A 12-month prospective study. Surg. Obes. Relat. Dis. 9 (4), 559–568. doi:10.1016/j.soard.2012.03.009
Fisher, D. P., Johnson, E., Haneuse, S., Arterburn, D., Coleman, K. J., O'Connor, P. J., et al. (2018). Association between bariatric surgery and macrovascular disease outcomes in patients with type 2 diabetes and severe obesity. Jama 320 (15), 1570–1582. doi:10.1001/jama.2018.14619
Fisher, F. M., Estall, J. L., Adams, A. C., Antonellis, P. J., Bina, H. A., Flier, J. S., et al. (2011). Integrated regulation of hepatic metabolism by fibroblast growth factor 21 (FGF21) in vivo. Endocrinology 152 (8), 2996–3004. doi:10.1210/en.2011-0281
Fondevila, M. F., Fernandez, U., Heras, V., Parracho, T., Gonzalez-Rellan, M. J., Novoa, E., et al. (2022). Inhibition of carnitine palmitoyltransferase 1A in hepatic stellate cells protects against fibrosis. J. Hepatol. 77 (1), 15–28. doi:10.1016/j.jhep.2022.02.003
Francque, S., Verrijken, A., Caron, S., Prawitt, J., Paumelle, R., Derudas, B., et al. (2015). PPARα gene expression correlates with severity and histological treatment response in patients with non-alcoholic steatohepatitis. J. Hepatol. 63 (1), 164–173. doi:10.1016/j.jhep.2015.02.019
Friedman, A. N., and Wolfe, B. (2016). Is bariatric surgery an effective treatment for type II diabetic kidney disease? Clin. J. Am. Soc. Nephrol. 11 (3), 528–535. doi:10.2215/cjn.07670715
Friedman, S. L., Neuschwander-Tetri, B. A., Rinella, M., and Sanyal, A. J. (2018). Mechanisms of NAFLD development and therapeutic strategies. Nat. Med. 24 (7), 908–922. doi:10.1038/s41591-018-0104-9
Getty, J. L., Hamdallah, I. N., Shamseddeen, H. N., Wu, J., Low, R. K., Craig, J., et al. (2012). Changes in renal function following roux-en-Y gastric bypass: A prospective study. Obes. Surg. 22 (7), 1055–1059. doi:10.1007/s11695-012-0617-0
Giacco, F., and Brownlee, M. (2010). Oxidative stress and diabetic complications. Circ. Res. 107 (9), 1058–1070. doi:10.1161/circresaha.110.223545
Guida, C., Stephen, S., Guitton, R., and Ramracheya, R. D. (2017). The role of PYY in pancreatic islet physiology and surgical control of diabetes. Trends Endocrinol. Metab. 28 (8), 626–636. doi:10.1016/j.tem.2017.04.005
Hanipah, Z. N., and Schauer, P. R. (2020). Bariatric surgery as a long-term treatment for type 2 diabetes/metabolic syndrome. Annu. Rev. Med. 71, 1–15. doi:10.1146/annurev-med-053117-123246
Harris, L. L. S., Smith, G. I., Mittendorfer, B., Eagon, J. C., Okunade, A. L., Patterson, B. W., et al. (2017). Roux-en-Y gastric bypass surgery has unique effects on postprandial FGF21 but not FGF19 secretion. J. Clin. Endocrinol. Metab. 102 (10), 3858–3864. doi:10.1210/jc.2017-01295
Heiserman, J. P., Chen, L., Kim, B. S., Kim, S. C., Tran, A. L., Siebenborn, N., et al. (2015). TLR4 mutation and HSP60-induced cell death in adult mouse cardiac myocytes. Cell. Stress Chaperones 20 (3), 527–535. doi:10.1007/s12192-015-0577-0
Helmstädter, J., Frenis, K., Filippou, K., Grill, A., Dib, M., Kalinovic, S., et al. (2020). Endothelial GLP-1 (Glucagon-Like peptide-1) receptor mediates cardiovascular protection by liraglutide in mice with experimental arterial hypertension. Arterioscler. Thromb. Vasc. Biol. 40 (1), 145–158. doi:10.1161/atv.0000615456.97862.30
Henderson, N. C., Rieder, F., and Wynn, T. A. (2020). Fibrosis: From mechanisms to medicines. Nature 587 (7835), 555–566. doi:10.1038/s41586-020-2938-9
Hinz, B., Phan, S. H., Thannickal, V. J., Galli, A., Bochaton-Piallat, M. L., and Gabbiani, G. (2007). The myofibroblast: One function, multiple origins. Am. J. Pathol. 170 (6), 1807–1816. doi:10.2353/ajpath.2007.070112
Huang, H. H., Lee, W. J., Chen, S. C., Chen, T. F., Lee, S. D., and Chen, C. Y. (2019). Bile acid and fibroblast growth factor 19 regulation in obese diabetics, and non-alcoholic fatty liver disease after sleeve gastrectomy. J. Clin. Med. 8 (6), E815. doi:10.3390/jcm8060815
Huang, X., Liu, S., Wu, D., Cheng, Y., Han, H., Wang, K., et al. (2018). Facilitated Ca(2+) homeostasis and attenuated myocardial autophagy contribute to alleviation of diabetic cardiomyopathy after bariatric surgery. Am. J. Physiol. Heart Circ. Physiol. 315 (5), H1258-H1268–h1268. doi:10.1152/ajpheart.00274.2018
Hubbard, V. S., and Hall, W. H. (1991). Gastrointestinal surgery for severe obesity. Obes. Surg. 1 (3), 257–265. doi:10.1381/096089291765560962
Illán-Gómez, F., Gonzálvez-Ortega, M., Orea-Soler, I., Alcaraz-Tafalla, M. S., Aragón-Alonso, A., Pascual-Díaz, M., et al. (2012). Obesity and inflammation: Change in adiponectin, C-reactive protein, tumour necrosis factor-alpha and interleukin-6 after bariatric surgery. Obes. Surg. 22 (6), 950–955. doi:10.1007/s11695-012-0643-y
Iribarren, C., Karter, A. J., Go, A. S., Ferrara, A., Liu, J. Y., Sidney, S., et al. (2001). Glycemic control and heart failure among adult patients with diabetes. Circulation 103 (22), 2668–2673. doi:10.1161/01.cir.103.22.2668
Itoh, N. (2010). Hormone-like (endocrine) fgfs: Their evolutionary history and roles in development, metabolism, and disease. Cell. Tissue Res. 342 (1), 1–11. doi:10.1007/s00441-010-1024-2
Jia, G., Whaley-Connell, A., and Sowers, J. R. (2018). Diabetic cardiomyopathy: A hyperglycaemia- and insulin-resistance-induced heart disease. Diabetologia 61 (1), 21–28. doi:10.1007/s00125-017-4390-4
Jiménez, A., Casamitjana, R., Viaplana-Masclans, J., Lacy, A., and Vidal, J. (2013). GLP-1 action and glucose tolerance in subjects with remission of type 2 diabetes after gastric bypass surgery. Diabetes Care 36 (7), 2062–2069. doi:10.2337/dc12-1535
Jiménez, A., Mari, A., Casamitjana, R., Lacy, A., Ferrannini, E., and Vidal, J. (2014). GLP-1 and glucose tolerance after sleeve gastrectomy in morbidly obese subjects with type 2 diabetes. Diabetes 63 (10), 3372–3377. doi:10.2337/db14-0357
Kaissling, B., Lehir, M., and Kriz, W. (2013). Renal epithelial injury and fibrosis. Biochim. Biophys. Acta 1832 (7), 931–939. doi:10.1016/j.bbadis.2013.02.010
Kalinowski, P., Paluszkiewicz, R., Ziarkiewicz-Wróblewska, B., Wróblewski, T., Remiszewski, P., Grodzicki, M., et al. (2017). Liver function in patients with nonalcoholic fatty liver disease randomized to roux-en-Y gastric bypass versus sleeve gastrectomy: A secondary analysis of a randomized clinical trial. Ann. Surg. 266 (5), 738–745. doi:10.1097/sla.0000000000002397
Kashihara, H., Shimada, M., Kurita, N., Sato, H., Yoshikawa, K., Higashijima, J., et al. (2015). Duodenal-jejunal bypass improves diabetes and liver steatosis via enhanced glucagon-like peptide-1 elicited by bile acids. J. Gastroenterol. Hepatol. 30 (2), 308–315. doi:10.1111/jgh.12690
Kindel, T. L., and Strande, J. L. (2018). Bariatric surgery as a treatment for heart failure: Review of the literature and potential mechanisms. Surg. Obes. Relat. Dis. 14 (1), 117–122. doi:10.1016/j.soard.2017.09.534
Koyama, Y., and Brenner, D. A. (2017). Liver inflammation and fibrosis. J. Clin. Investig. 127 (1), 55–64. doi:10.1172/jci88881
Kunz, H. E., Hart, C. R., Gries, K. J., Parvizi, M., Laurenti, M., Dalla Man, C., et al. (2021). Adipose tissue macrophage populations and inflammation are associated with systemic inflammation and insulin resistance in obesity. Am. J. Physiol. Endocrinol. Metab. 321 (1), E105–e121. doi:10.1152/ajpendo.00070.2021
La Sala, L., and Pontiroli, A. E. (2020). Prevention of diabetes and cardiovascular disease in obesity. Int. J. Mol. Sci. 21 (21), E8178. doi:10.3390/ijms21218178
Labbé, S. M., Grenier-Larouche, T., Noll, C., Phoenix, S., Guérin, B., Turcotte, E. E., et al. (2012). Increased myocardial uptake of dietary fatty acids linked to cardiac dysfunction in glucose-intolerant humans. Diabetes 61 (11), 2701–2710. doi:10.2337/db11-1805
Lambers Heerspink, H. J., de Borst, M. H., Bakker, S. J., and Navis, G. J. (2013). Improving the efficacy of RAAS blockade in patients with chronic kidney disease. Nat. Rev. Nephrol. 9 (2), 112–121. doi:10.1038/nrneph.2012.281
Lassailly, G., Caiazzo, R., Ntandja-Wandji, L.C., Gnemmi, V., Baud, G., Verkindt, H., et al. (2020). Bariatric Surgery Provides Long-term Resolution of Nonalcoholic Steatohepatitis and Regression of Fibrosis. Gastroenterology 159 (4), 1290–1301. doi:10.1053/j.gastro.2020.06.006
le Roux, C.W., and Bloom, S.R. (2005). Peptide YY, appetite and food intake. Proc Nutr Soc 64(2), 213-216. doi:10.1079/pns2005427
Li, J., Liu, C., Zhou, Z., Dou, B., Huang, J., Huang, L., et al. (2021). Isotschimgine alleviates nonalcoholic steatohepatitis and fibrosis via FXR agonism in mice. Phytother. Res. 35 (6), 3351–3364. doi:10.1002/ptr.7055
Li, P., Zhu, L., Yang, X., Li, W., Sun, X., Yi, B., et al. (2019). Farnesoid X receptor interacts with cAMP response element binding protein to modulate glucagon-like peptide-1 (7-36) amide secretion by intestinal L cell. J. Cell. Physiol. 234 (8), 12839–12846. doi:10.1002/jcp.27940
Li, S., Dong, S., Xu, Q., Shi, B., Li, L., Zhang, W., et al. (2022). Sleeve gastrectomy-induced AMPK activation attenuates diabetic cardiomyopathy by maintaining mitochondrial homeostasis via NR4A1 suppression in rats. Front. Physiol. 13, 837798. doi:10.3389/fphys.2022.837798
Lima, M. M., Pareja, J. C., Alegre, S. M., Geloneze, S. R., Kahn, S. E., Astiarraga, B. D., et al. (2013). Visceral fat resection in humans: Effect on insulin sensitivity, beta-cell function, adipokines, and inflammatory markers. Obes. (Silver Spring) 21 (3), E182–E189. doi:10.1002/oby.20030
Lin, C. H., Kurup, S., Herrero, P., Schechtman, K. B., Eagon, J. C., Klein, S., et al. (2011). Myocardial oxygen consumption change predicts left ventricular relaxation improvement in obese humans after weight loss. Obes. (Silver Spring) 19 (9), 1804–1812. doi:10.1038/oby.2011.186
Liu, M., López de Juan Abad, B., and Cheng, K. (2021). Cardiac fibrosis: Myofibroblast-mediated pathological regulation and drug delivery strategies. Adv. Drug Deliv. Rev. 173, 504–519. doi:10.1016/j.addr.2021.03.021
Liu, Q., Wang, S., Wei, M., Huang, X., Cheng, Y., Shao, Y., et al. (2019). Improved FGF21 sensitivity and restored FGF21 signaling pathway in high-fat diet/streptozotocin-induced diabetic rats after duodenal-jejunal bypass and sleeve gastrectomy. Front. Endocrinol. 10, 566. doi:10.3389/fendo.2019.00566
Liu, Y. (2011). Cellular and molecular mechanisms of renal fibrosis. Nat. Rev. Nephrol. 7 (12), 684–696. doi:10.1038/nrneph.2011.149
Liu, Y., Chen, K., Li, F., Gu, Z., Liu, Q., He, L., et al. (2020). Probiotic lactobacillus rhamnosus GG prevents liver fibrosis through inhibiting hepatic bile acid synthesis and enhancing bile acid excretion in mice. Hepatology 71 (6), 2050–2066. doi:10.1002/hep.30975
Mack, M. (2018). Inflammation and fibrosis. Matrix Biol. 68-69, 106–121. doi:10.1016/j.matbio.2017.11.010
Magouliotis, D. E., Tasiopoulou, V. S., Sioka, E., Chatedaki, C., and Zacharoulis, D. (2017). Impact of bariatric surgery on metabolic and gut microbiota profile: A systematic review and meta-analysis. Obes. Surg. 27 (5), 1345–1357. doi:10.1007/s11695-017-2595-8
Martin, W. P., White, J., López-Hernández, F. J., Docherty, N. G., and le Roux, C. W. (2020). Metabolic surgery to treat obesity in diabetic kidney disease, chronic kidney disease, and end-stage kidney disease; what are the unanswered questions? Front. Endocrinol. 11, 289. doi:10.3389/fendo.2020.00289
Massare, J., Berry, J. M., Luo, X., Rob, F., Johnstone, J. L., Shelton, J. M., et al. (2010). Diminished cardiac fibrosis in heart failure is associated with altered ventricular arrhythmia phenotype. J. Cardiovasc. Electrophysiol. 21 (9), 1031–1037. doi:10.1111/j.1540-8167.2010.01736.x
Mazzini, G. S., Khoraki, J., Dozmorov, M., Browning, M. G., Wijesinghe, D., Wolfe, L., et al. (2019). Concomitant PPARα and FXR activation as a putative mechanism of NASH improvement after gastric bypass surgery: A GEO datasets analysis. J. Gastrointest. Surg. 23 (1), 51–57. doi:10.1007/s11605-018-3938-z
McDonald, S., Maguire, G., Duarte, N., Wang, X. L., and Hoy, W. (2004). C-reactive protein, cardiovascular risk, and renal disease in a remote Australian Aboriginal community. Clin. Sci. 106 (2), 121–128. doi:10.1042/cs20030186
Messina, A., Monda, M., Valenzano, A., Messina, G., Villano, I., Moscatelli, F., et al. (2018). Functional changes induced by orexin A and adiponectin on the sympathetic/parasympathetic balance. Front. Physiol. 9, 259. doi:10.3389/fphys.2018.00259
Mezzano, S. A., Ruiz-Ortega, M., and Egido, J. (2001). Angiotensin II and renal fibrosis. Hypertension 38 (3), 635–638. doi:10.1161/hy09t1.094234
Monte, S. V., Caruana, J. A., Ghanim, H., Sia, C. L., Korzeniewski, K., Schentag, J. J., et al. (2012). Reduction in endotoxemia, oxidative and inflammatory stress, and insulin resistance after Roux-en-Y gastric bypass surgery in patients with morbid obesity and type 2 diabetes mellitus. Surgery 151 (4), 587–593. doi:10.1016/j.surg.2011.09.038
Moore-Morris, T., Guimarães-Camboa, N., Banerjee, I., Zambon, A. C., Kisseleva, T., Velayoudon, A., et al. (2014). Resident fibroblast lineages mediate pressure overload-induced cardiac fibrosis. J. Clin. Investig. 124 (7), 2921–2934. doi:10.1172/jci74783
Navaneethan, S. D., Kelly, K. R., Sabbagh, F., Schauer, P. R., Kirwan, J. P., and Kashyap, S. R. (2010). Urinary albumin excretion, HMW adiponectin, and insulin sensitivity in type 2 diabetic patients undergoing bariatric surgery. Obes. Surg. 20 (3), 308–315. doi:10.1007/s11695-009-0026-1
Navarro-Díaz, M., Serra, A., Romero, R., Bonet, J., Bayés, B., Homs, M., et al. (2006). Effect of drastic weight loss after bariatric surgery on renal parameters in extremely obese patients: Long-term follow-up. J. Am. Soc. Nephrol. 17 (12), S213–S217. doi:10.1681/asn.2006080917
Nichols, G. A., Gullion, C. M., Koro, C. E., Ephross, S. A., and Brown, J. B. (2004). The incidence of congestive heart failure in type 2 diabetes: An update. Diabetes Care 27 (8), 1879–1884. doi:10.2337/diacare.27.8.1879
Nijhawan, S., Richards, W., O'Hea, M. F., Audia, J. P., and Alvarez, D. F. (2013). Bariatric surgery rapidly improves mitochondrial respiration in morbidly obese patients. Surg. Endosc. 27 (12), 4569–4573. doi:10.1007/s00464-013-3125-y
O'Brien, R., Johnson, E., Haneuse, S., Coleman, K. J., O'Connor, P. J., Fisher, D. P., et al. (2018). Microvascular outcomes in patients with diabetes after bariatric surgery versus usual care: A matched cohort study. Ann. Intern. Med. 169 (5), 300–310. doi:10.7326/m17-2383
Osto, E., Doytcheva, P., Corteville, C., Bueter, M., Dörig, C., Stivala, S., et al. (2015). Rapid and body weight-independent improvement of endothelial and high-density lipoprotein function after roux-en-Y gastric bypass: Role of glucagon-like peptide-1. Circulation 131 (10), 871–881. doi:10.1161/circulationaha.114.011791
Pawlak, M., Lefebvre, P., and Staels, B. (2015). Molecular mechanism of PPARα action and its impact on lipid metabolism, inflammation and fibrosis in non-alcoholic fatty liver disease. J. Hepatol. 62 (3), 720–733. doi:10.1016/j.jhep.2014.10.039
Porter, K. E., and Turner, N. A. (2009). Cardiac fibroblasts: At the heart of myocardial remodeling. Pharmacol. Ther. 123 (2), 255–278. doi:10.1016/j.pharmthera.2009.05.002
Powell, E. E., Wong, V. W., and Rinella, M. (2021). Non-alcoholic fatty liver disease. Lancet 397 (10290), 2212–2224. doi:10.1016/s0140-6736(20)32511-3
Price, S. L., and Bloom, S. R. (2014). Protein PYY and its role in metabolism. Front. Horm. Res. 42, 147–154. doi:10.1159/000358343
Qi, G. M., Jia, L. X., Li, Y. L., Li, H. H., and Du, J. (2014). Adiponectin suppresses angiotensin II-induced inflammation and cardiac fibrosis through activation of macrophage autophagy. Endocrinology 155 (6), 2254–2265. doi:10.1210/en.2013-2011
Rao, S. R. (2012). Inflammatory markers and bariatric surgery: A meta-analysis. Inflamm. Res. 61 (8), 789–807. doi:10.1007/s00011-012-0473-3
Regan, T. J., Lyons, M. M., Ahmed, S. S., Levinson, G. E., Oldewurtel, H. A., Ahmad, M. R., et al. (1977). Evidence for cardiomyopathy in familial diabetes mellitus. J. Clin. Investig. 60 (4), 884–899. doi:10.1172/jci108843
Reidy, K., Kang, H. M., Hostetter, T., and Susztak, K. (2014). Molecular mechanisms of diabetic kidney disease. J. Clin. Investig. 124 (6), 2333–2340. doi:10.1172/jci72271
Risstad, H., Kristinsson, J. A., Fagerland, M. W., le Roux, C. W., Birkeland, K. I., Gulseth, H. L., et al. (2017). Bile acid profiles over 5 years after gastric bypass and duodenal switch: Results from a randomized clinical trial. Surg. Obes. Relat. Dis. 13 (9), 1544–1553. doi:10.1016/j.soard.2017.05.024
Rocco, M. V., and Berns, J. S. (2009). KDOQI in the era of global guidelines. Am. J. Kidney Dis. 54 (5), 781–787. doi:10.1053/j.ajkd.2009.08.001
Rockey, D. C., Bell, P. D., and Hill, J. A. (2015). Fibrosis--a common pathway to organ injury and failure. N. Engl. J. Med. 372 (12), 1138–1149. doi:10.1056/NEJMra1300575
Rockey, D. C., Housset, C. N., and Friedman, S. L. (1993). Activation-dependent contractility of rat hepatic lipocytes in culture and in vivo. J. Clin. Investig. 92 (4), 1795–1804. doi:10.1172/jci116769
Rosso, C., Kazankov, K., Younes, R., Esmaili, S., Marietti, M., Sacco, M., et al. (2019). Crosstalk between adipose tissue insulin resistance and liver macrophages in non-alcoholic fatty liver disease. J. Hepatol. 71 (5), 1012–1021. doi:10.1016/j.jhep.2019.06.031
Roth, A. E., Thornley, C. J., and Blackstone, R. P. (2020). Outcomes in bariatric and metabolic surgery: An updated 5-year review. Curr. Obes. Rep. 9 (3), 380–389. doi:10.1007/s13679-020-00389-8
Ryan, K. K., Tremaroli, V., Clemmensen, C., Kovatcheva-Datchary, P., Myronovych, A., Karns, R., et al. (2014). FXR is a molecular target for the effects of vertical sleeve gastrectomy. Nature 509 (7499), 183–188. doi:10.1038/nature13135
Sabatino, A., Regolisti, G., Cosola, C., Gesualdo, L., and Fiaccadori, E. (2017). Intestinal microbiota in type 2 diabetes and chronic kidney disease. Curr. Diab. Rep. 17 (3), 16. doi:10.1007/s11892-017-0841-z
Sala, P., Torrinhas, R., Fonseca, D. C., Machado, N. M., Singer, J., Singer, P., et al. (2020). Intestinal expression of toll-like receptor gene changes early after gastric bypass surgery and association with type 2 diabetes remission. Nutrition 79-80, 110885. doi:10.1016/j.nut.2020.110885
Schwabe, R. F., Tabas, I., and Pajvani, U. B. (2020). Mechanisms of fibrosis development in nonalcoholic steatohepatitis. Gastroenterology 158 (7), 1913–1928. doi:10.1053/j.gastro.2019.11.311
Seki, E., De Minicis, S., Osterreicher, C. H., Kluwe, J., Osawa, Y., Brenner, D. A., et al. (2007). TLR4 enhances TGF-beta signaling and hepatic fibrosis. Nat. Med. 13 (11), 1324–1332. doi:10.1038/nm1663
Sell, H., Poitou, C., Habich, C., Bouillot, J. L., Eckel, J., and Clément, K. (2017). Heat shock protein 60 in obesity: Effect of bariatric surgery and its relation to inflammation and cardiovascular risk. Obes. (Silver Spring) 25 (12), 2108–2114. doi:10.1002/oby.22014
Shimizu, M., Umeda, K., Sugihara, N., Yoshio, H., Ino, H., Takeda, R., et al. (1993). Collagen remodelling in myocardia of patients with diabetes. J. Clin. Pathol. 46 (1), 32–36. doi:10.1136/jcp.46.1.32
Shulman, G. I. (2014). Ectopic fat in insulin resistance, dyslipidemia, and cardiometabolic disease. N. Engl. J. Med. 371 (12), 1131–1141. doi:10.1056/NEJMra1011035
Sorribas, M., Jakob, M. O., Yilmaz, B., Li, H., Stutz, D., Noser, Y., et al. (2019). FXR modulates the gut-vascular barrier by regulating the entry sites for bacterial translocation in experimental cirrhosis. J. Hepatol. 71 (6), 1126–1140. doi:10.1016/j.jhep.2019.06.017
Spinale, F. G. (2007). Myocardial matrix remodeling and the matrix metalloproteinases: Influence on cardiac form and function. Physiol. Rev. 87 (4), 1285–1342. doi:10.1152/physrev.00012.2007
Sun, Y. B., Qu, X., Caruana, G., and Li, J. (2016). The origin of renal fibroblasts/myofibroblasts and the signals that trigger fibrosis. Differentiation. 92 (3), 102–107. doi:10.1016/j.diff.2016.05.008
Svegliati-Baroni, G., Ridolfi, F., Di Sario, A., Casini, A., Marucci, L., Gaggiotti, G., et al. (1999). Insulin and insulin-like growth factor-1 stimulate proliferation and type I collagen accumulation by human hepatic stellate cells: Differential effects on signal transduction pathways. Hepatology 29 (6), 1743–1751. doi:10.1002/hep.510290632
Taylor, R. (2013). Type 2 diabetes: Etiology and reversibility. Diabetes Care 36 (4), 1047–1055. doi:10.2337/dc12-1805
Tervaert, T. W., Mooyaart, A. L., Amann, K., Cohen, A. H., Cook, H. T., Drachenberg, C. B., et al. (2010). Pathologic classification of diabetic nephropathy. J. Am. Soc. Nephrol. 21 (4), 556–563. doi:10.1681/asn.2010010010
Tesch, G. H. (2008). MCP-1/CCL2: A new diagnostic marker and therapeutic target for progressive renal injury in diabetic nephropathy. Am. J. Physiol. Ren. Physiol. 294 (4), F697–F701. doi:10.1152/ajprenal.00016.2008
Tokarz, V. L., MacDonald, P. E., and Klip, A. (2018). The cell biology of systemic insulin function. J. Cell. Biol. 217 (7), 2273–2289. doi:10.1083/jcb.201802095
Trabelsi, M. S., Daoudi, M., Prawitt, J., Ducastel, S., Touche, V., Sayin, S. I., et al. (2015). Farnesoid X receptor inhibits glucagon-like peptide-1 production by enteroendocrine L cells. Nat. Commun. 6, 7629. doi:10.1038/ncomms8629
Tsuchida, T., and Friedman, S. L. (2017). Mechanisms of hepatic stellate cell activation. Nat. Rev. Gastroenterol. Hepatol. 14 (7), 397–411. doi:10.1038/nrgastro.2017.38
Umeda, L. M., Pereira, A. Z., Carneiro, G., Arasaki, C. H., and Zanella, M. T. (2013). Postprandial adiponectin levels are associated with improvements in postprandial triglycerides after Roux-en-Y gastric bypass in type 2 diabetic patients. Metab. Syndr. Relat. Disord. 11 (5), 343–348. doi:10.1089/met.2012.0042
Valenzano, A., Tartaglia, N., Ambrosi, A., Tafuri, D., Monda, M., Messina, A., et al. (2020). The metabolic rearrangements of bariatric surgery: Focus on orexin-A and the adiponectin system. J. Clin. Med. 9 (10), E3327. doi:10.3390/jcm9103327
van Hoeven, K. H., and Factor, S. M. (1990). A comparison of the pathological spectrum of hypertensive, diabetic, and hypertensive-diabetic heart disease. Circulation 82 (3), 848–855. doi:10.1161/01.cir.82.3.848
Vangoitsenhoven, R., Mulya, A., Mosinski, J. D., Brethauer, S. A., Schauer, P. R., Kirwan, J. P., et al. (2020). Effects of gastric bypass surgery on expression of glucose transporters and fibrotic biomarkers in kidney of diabetic fatty rats. Surg. Obes. Relat. Dis. 16 (9), 1242–1248. doi:10.1016/j.soard.2020.04.017
Vespasiani-Gentilucci, U., Carotti, S., Perrone, G., Mazzarelli, C., Galati, G., Onetti-Muda, A., et al. (2015). Hepatic toll-like receptor 4 expression is associated with portal inflammation and fibrosis in patients with NAFLD. Liver Int. 35 (2), 569–581. doi:10.1111/liv.12531
Vilar-Gomez, E., Martinez-Perez, Y., Calzadilla-Bertot, L., Torres-Gonzalez, A., Gra-Oramas, B., Gonzalez-Fabian, L., et al. (2015). Weight loss through lifestyle modification significantly reduces features of nonalcoholic steatohepatitis. Gastroenterology 149 (2), 367–378. e365; quiz e314-365. doi:10.1053/j.gastro.2015.04.005
Wang, M., Wu, Q., Xie, H., Shao, Y., Zhong, M., Zhang, X., et al. (2017). Effects of sleeve gastrectomy on serum 12α-hydroxylated bile acids in a diabetic rat model. Obes. Surg. 27 (11), 2912–2918. doi:10.1007/s11695-017-2714-6
Wentworth, J. M., Naselli, G., Brown, W. A., Doyle, L., Phipson, B., Smyth, G. K., et al. (2010). Pro-inflammatory CD11c+CD206+ adipose tissue macrophages are associated with insulin resistance in human obesity. Diabetes 59 (7), 1648–1656. doi:10.2337/db09-0287
Wolfe, B. M., Kvach, E., and Eckel, R. H. (2016). Treatment of obesity: Weight loss and bariatric surgery. Circ. Res. 118 (11), 1844–1855. doi:10.1161/circresaha.116.307591
Wu, H. J., Yiu, W. H., Li, R. X., Wong, D. W., Leung, J. C., Chan, L. Y., et al. (2014). Mesenchymal stem cells modulate albumin-induced renal tubular inflammation and fibrosis. PLoS One 9 (3), e90883. doi:10.1371/journal.pone.0090883
Wynn, T. A. (2007). Common and unique mechanisms regulate fibrosis in various fibroproliferative diseases. J. Clin. Investig. 117 (3), 524–529. doi:10.1172/jci31487
Wynn, T. A. (2004). Fibrotic disease and the T(H)1/T(H)2 paradigm. Nat. Rev. Immunol. 4 (8), 583–594. doi:10.1038/nri1412
Xie, G., Jiang, R., Wang, X., Liu, P., Zhao, A., Wu, Y., et al. (2021). Dysregulated bile acid signaling contributes to the neurological impairment in murine models of acute and chronic liver failure. EBioMedicine 66, 294–306. doi:10.1016/j.ebiom.2018.10.030
Xiong, Y., Zhu, W., Xu, Q., Ruze, R., Yan, Z., Li, J., et al. (2020). Sleeve gastrectomy attenuates diabetic nephropathy by upregulating nephrin expressions in diabetic obese rats. Obes. Surg. 30 (8), 2893–2904. doi:10.1007/s11695-020-04611-3
You, Y. K., Wu, W. F., Huang, X. R., Li, H. D., Ren, Y. P., Zeng, J. C., et al. (2021). Deletion of Smad3 protects against C-reactive protein-induced renal fibrosis and inflammation in obstructive nephropathy. Int. J. Biol. Sci. 17 (14), 3911–3922. doi:10.7150/ijbs.62929
Young, L., Nor Hanipah, Z., Brethauer, S. A., Schauer, P. R., and Aminian, A. (2019). Long-term impact of bariatric surgery in diabetic nephropathy. Surg. Endosc. 33 (5), 1654–1660. doi:10.1007/s00464-018-6458-8
Younossi, Z., Anstee, Q. M., Marietti, M., Hardy, T., Henry, L., Eslam, M., et al. (2018). Global burden of NAFLD and NASH: Trends, predictions, risk factors and prevention. Nat. Rev. Gastroenterol. Hepatol. 15 (1), 11–20. doi:10.1038/nrgastro.2017.109
Zarei, M., Barroso, E., Palomer, X., Dai, J., Rada, P., Quesada-López, T., et al. (2018). Hepatic regulation of VLDL receptor by PPARβ/δ and FGF21 modulates non-alcoholic fatty liver disease. Mol. Metab. 8, 117–131. doi:10.1016/j.molmet.2017.12.008
Zeisberg, E. M., Tarnavski, O., Zeisberg, M., Dorfman, A. L., McMullen, J. R., Gustafsson, E., et al. (2007). Endothelial-to-mesenchymal transition contributes to cardiac fibrosis. Nat. Med. 13 (8), 952–961. doi:10.1038/nm1613
Zhai, H., Li, Z., Peng, M., Huang, Z., Qin, T., Chen, L., et al. (2018). Takeda G protein-coupled receptor 5-mechanistic target of rapamycin complex 1 signaling contributes to the increment of glucagon-like peptide-1 production after roux-en-Y gastric bypass. EBioMedicine 32, 201–214. doi:10.1016/j.ebiom.2018.05.026
Keywords: bariatric surgery, Roux-en-Y gastric bypass, sleeve gastrectomy, diabetic cardiomyopathy, non-alcoholic fatty liver disease, diabetic kidney disease, fibrosis
Citation: Ding H, Zhang Y, Ma X, Zhang Z, Xu Q, Liu C, Li B, Dong S, Li L, Zhu J, Zhong M and Zhang G (2022) Bariatric surgery for diabetic comorbidities: A focus on hepatic, cardiac and renal fibrosis. Front. Pharmacol. 13:1016635. doi: 10.3389/fphar.2022.1016635
Received: 11 August 2022; Accepted: 06 October 2022;
Published: 21 October 2022.
Edited by:
Yanping Liu, Qilu Hospital, Shandong University, ChinaReviewed by:
Reza Nemati, Canterbury Health Laboratories, New ZealandBingxian Xie, University of Pittsburgh, United States
Liyong Zhu, Third Xiangya Hospital, Central South University, China
Cunchuan Wang, First Affiliated Hospital of Jinan University, China
Copyright © 2022 Ding, Zhang, Ma, Zhang, Xu, Liu, Li, Dong, Li, Zhu, Zhong and Zhang. This is an open-access article distributed under the terms of the Creative Commons Attribution License (CC BY). The use, distribution or reproduction in other forums is permitted, provided the original author(s) and the copyright owner(s) are credited and that the original publication in this journal is cited, in accordance with accepted academic practice. No use, distribution or reproduction is permitted which does not comply with these terms.
*Correspondence: Guangyong Zhang, guangyongzhang@hotmail.com
†These authors have contributed equally to this work