- 1Key Laboratory of Molecular Target & Clinical Pharmacology and the State & NMPA Key Laboratory of Respiratory Disease, School of Pharmaceutical Sciences, Fifth Affiliated Hospital, Guangzhou Medical University, Guangzhou, China
- 2Faculty of Science and Technology, The Technological and Higher Education Institute of Hong Kong, Hong Kong, Hong Kong SAR, China
- 3School of Nursing and Health Studies, Hong Kong Metropolitan University, Hong Kong, Hong Kong SAR, China
- 4School of Graduate Studies, Lingnan University, Tuen Mun, Hong Kong SAR, China
Obesity is a leading worldwide health threat with ever-growing prevalence, it promotes the incidence of various diseases, particularly cardiovascular disease, metabolic syndrome, diabetes, hypertension, and certain cancers. Traditional Chinese Medicine (TCM) has been used to control body weight and treat obesity for thousands of years, Chinese medicinal herbs provide a rich natural source of effective agents against obesity. However, some problems such as complex active ingredients, poor quality control, and unclear therapeutic mechanisms still need to be investigated and resolved. Prodrugs provide a path forward to overcome TCM deficiencies such as absorption, distribution, metabolism, excretion (ADME) properties, and toxicity. This article aimed to review the possible prodrugs from various medicinal plants that demonstrate beneficial effects on obesity and seek to offer insights on prodrug design as well as a solution to the global obesity issues.
Introduction
Obesity is epidemic disease with excess energy storage and fat accumulation. An abnormally accumulated fat is positively associated with diabetes, cardiovascular diseases, hyperlipidemia, hypertension, metabolic syndrome, fatty liver disease, a certain type of cancer, depression, and anxiety (Fulton et al., 2022). The high prevalence of obesity is imposing an enormous burden on people’s health. A number of studies have shown the impact of obesity on infectious diseases such as coronavirus disease 2019 (COVID-19), it might play a role in the progression of COVID-19 after infection (Pan et al., 2021), and increased risk of hospitalization and severe illness from COVID-19 (Loos and Yeo, 2022). Over the past decades, medical treatment of obesity mainly focuses on the following mechanisms: 1) promoting energy expenditure; 2) lowering calorie absorption and 3) reducing energy intake. Pharmacological management of obesity has an overlong history populated with multiple prominent disappointments (Tak and Lee, 2021). Lifestyle management, lipid reduction, and weight loss surgery, which measures to reduce or absorb food intake, as well as increase its utilization are the main tools used to treat obesity today. However, body weight increases and recovery is associated with adverse drug reactions, surgery, and other problems, without effective and safe therapies for obesity (Ghasemi and Jeddi, 2017). Meanwhile, some Western medicines for obesity have reported side effects such as gastrointestinal reactions, cardiovascular complications, and negative moods. TCM has developed over a long history, it accumulated extensive pharmacological information and clinical experience to treat obesity. The effectiveness and safety of TCM offer an alternative therapy for this unsolved medical problem, e.g., traditional Chinese herbs and acupuncture (Sui et al., 2012). According to the TCM theory of Health in “Huangdi Neijing,” obesity has been indicated as spleen and kidney dysfunction, deficiencies, damp heat, and blood stasis. TCM treats obesity based on the restoration of an internal balance by eliminating dampness and heat, invigorating the kidney and spleen, activating blood circulation, and dispersing stagnated liver. The underlying mechanisms involved in reducing oxidative stress, anti-inflammation, and inhibiting lipid activity such as production and accumulation, which enhance leptin sensitivity and adiponectin (Cho et al., 2009).
Traditional Chinese medicine consists of complex ingredients from plants, minerals and animals. The traditional Chinese herbs were generally prescribed for treating illnesses according to the overall symptoms and collective experience, but this lacks the strict experimental proof to illustrate the features of clear-cut molecular compositions, pharmacokinetic profile, and mechanism of action. Addressing the tremendous challenge of some chemical complexity of TCM and overcoming bottlenecks in the drug development from herbal medicines requires the combination of modern technology, and scientific thought, as well as the traditional theory (Chu et al., 2020). Recently, many innovative technologies and methods are being accomplished in the research of TCM such as computer-aided drug design (CADD), cell membrane electrophysiology, gene hybridization, gene chip, luminescence, and fluorescent probe, DNA gel electrophoresis, and differential mRNA display technology (Li et al., 2008). Thus, a combination of Chinese medicinal herbs with modern scientific technology provides us to have wide insights and guides the development of promising powerful novel drugs. Several Chinese medicinal herbs are possible candidates for obesity, but their compositions and mechanisms are vague. It is important to incorporate herbal medicines into standard Western drug development pipelines and ultimately expand them as promising drugs or promising alternatives in the management of obesity. This article focuses on the concept of “Prodrug,” and describes a series of Chinese medicinal herbs, e.g., “Curcumin,” “Ginsenoside,” “Celastrol,” “Berberine,” “Artemisinin,” and “Capsaicin” as potential prodrugs for anti-obesity, and the combination of prodrug as well as the nanotechnology effect on obesity.
Prodrug
Adrien Albert first announced the definition of “prodrug” in 1951 (Albert, 1958). The prodrug is inactive or less than the fully active form and converted into active compounds (drugs) through the metabolic pathways by enzymes such as hydrolases or other chemical actions within the human body. This undergoes a series of biochemical transformations to exert its pharmacological functions for targeting diseases (Cho and Yoon, 2018). In general, prodrugs are modified from parent molecule and selectively take effects in the target tissues, subsequently improving pharmaceutical (PH), pharmacokinetic (PK), and pharmacodynamics (PD) effects, as well as preventing undesirable side effects (Abet et al., 2017). The prodrug process more focuses on optimizing the ADMET (absorption, distribution, metabolism excretion, and toxicity) properties of potential herbal ingredients, and eventually increasing their efficiency and safety. These attach the poor water-soluble compound to a phosphate ester to increase its aqueous solubility (Raimund et al., 2011). In the presence of esterase, lipophilicity and membrane permeability of most prodrugs are increased, through esterase bioconversion, releasing rate of the prodrug is fostered (Perez et al., 2013).
Prodrugs exist in two types, carrier-bound and bioprecursors (Wermuth, 2008). Carrier-bound prodrugs consist of an active ingredient (drug) and a non-toxic carrier or promoiety via a covalent bond. The active ingredients are released from TCM through the action of enzymatic or non-enzymatic for covalent bond breaking (Shah et al., 2017). The carrier-linkers such as amides, esters, carbamates, phosphates, oximes, carbonates, N-Mannich, and imine are the main groups of prodrugs (Gandhi et al., 2019). Bioprecursors are the parent drug obtained by enzymatic transformation or biotransformation, via hydration, reduction, and oxidation (Shah et al., 2017).
One TCM prodrug example is “Resveratrol” for anti-obesity that can exist in the carrier-bound and bioprecursors, respectively. It is an active ingredient from TCM which is linked with the amino acid carbamate (Figure 1). The N-monosubstituted carbamate ester (-OC(O)NHR) as a carrier bound reacted to the OH group, preventing the binding of natural amino acids, e.g., Leu, Ile, Phe, Thr (Figure 2) and improving their molecular physicochemical properties, that significantly enhanced the absorption and stability of resveratrol in the bloodstream (Mattarei et al., 2015).
Trans-Resveratrol is the isotope of resveratrol, which is formed via the reduction by the human gut bacteria. That improved the bioavailability of resveratrol via biotransformation and confirmed dihydroresveratrol, lunularin, and 3,4-dihydroxy-stulbene as prodrugs of resveratrol metabolism (Figure 3) (Kokil and Rewatkar, 2010; Bode et al., 2013; Zawilska et al., 2013).
Most Chinese herbal medicines are poor aqueous solubility, low bioavailability and stability, and non-target specificity, limiting their clinical applications. The above resveratrol prodrug examples indicated that prodrug improved solubility, absorption as well as stability of active ingredients. On the other hand, it might enable tissue-selective delivery and in situ activation (Testa, 2009). Thus, Chinese medicinal herbs are a promising source and suitable for the research and development of prodrugs to treat obesity or other metabolic diseases.
Chinese medicinal herbs
Many traditional medicinal herbs and their ingredients have been tested and practiced over the years, such as Curcumin¸ Ginsenoside, Celastrol, Berberine, Artemisinin, and Capsaicin. These are natural Chinese herb-based therapeutics for obesity.
Curcumin, an essential active ingredient in traditional Chinese turmeric (Curcuma longa), possessed multiple pharmacological effects including antioxidant, anti-inflammatory, antiviral, antimicrobial, and antitumor functions (Koboziev et al., 2020; Law et al., 2022; Nurcahyanti et al., 2022). The physiological and pharmacological properties depend on the curcumin metabolites, e.g., Dihydrocurcumin (DHC), Tetrahydrocurcumin (THC), Hexahydrocurcumin (HDC), and Octahydrocurcumin (OHC). These curcumin metabolites act as prodrugs to interact with the human metabolic pathways, existing in Phase I and Phase II. “Reductase” is an enzyme in the phase I human metabolic pathway for the reduction of double bonds from curcumin, which converts it into di, tetra, hexa, and octahydrocurcumin. “Glucuronide” or “Sulfate” is a carrier bound in phase II human metabolic pathway conjugated to curcumin producing hydrogenated metabolites (Figure 4) (Ireson et al., 2002). The structure of curcumin derivatives contains two phenolic hydroxyl groups, it is easy to form hydrogen or ionic bonds and dissociate into negatively charged phenolic ions in the water, altering some protein functions in the human body (Wink, 2015). Curcumin metabolites may suppress the NF-κB in adipose tissue, and regulate the level of TNF-a, IL-6, monocyte chemotactic protein (MCP-1), plasminogen activator inhibitor type-1 (PAI-1), and increasing adiponectin expression to ameliorate the obesity risk factors (Bradford, 2013; Soleimani et al., 2018; Wink, 2022).
Obesity has corresponded to insulin resistance and diabetes (Bastard et al., 2006). NF-E2-related factor 2 (NRF2)-Kelch-like ECH-associated protein 1 (KEAP1) pathway and endoplasmic reticulum (ER) stress are the two important factors in the primary adipocytes causing the risk of obesity (Wang et al., 2016); while curcumin and its metabolites are the signaling modulators to improve the lipolysis and insulin resistance for preventing obesity (Ye et al., 2017).
Sterol regulatory element-binding protein 1C (SREBP-1C), peroxisome proliferator-activated receptor gamma (PPARγ), fatty acid synthase (FAS), and fatty acid-binding protein 4 (FABP4) are the lipogenic proteins that inhibited by the THC. It ameliorates free fatty acid-induced hepatic steatosis and regulates the secretion of insulin, as well as significantly decreased lipid accumulation that leads to non-alcoholic fatty liver disease (NAFLD). As the phosphorylation of an insulin receptor substrate 1 (IRS-1)/phosphoinositide 3-kinase (PI3K)/Akt and downstream signaling pathways, forkhead box protein O1 (FOXO1), and glycogen synthase kinase 3β (GSK3β) are modulated by THC to decrease the risk of obesity (Chen et al., 2018).
DHC manages glucose uptake, reduces the risk of obesity, and prevents diabetes because it regulates the levels of sterol regulatory element binding protein-1C (SREBP-1C), patatin-like phospholipase domain containing 3 (PNPLA3), and peroxisome proliferator-activated receptor-α (PPARα) in the mRNA and proteins It decreases the level of triglycerides (TG) when the phosphatidylinositol-3-kinase (PI3K) and phosphorylated serine-threonine protein kinase (pAKT) increases to improve lipid accumulation, oxidative stress, and insulin resistance (Yu et al., 2018).
Besides, DHC and THC can alleviate adiposity and suppress inflammatory responses as well as improve insulin sensitivity in white adipose tissue, which are regulated through the FNDC5/p38 mitogen-activated protein kinase (p38 MAPK) or extracellular signaling-related kinase (ERK) signaling pathways. These increase oxygen consumption and thermogenesis, also the respiratory exchange ratio within the body for reducing the risk of obesity (Zou et al., 2021).
The THC is also a potential agent to attenuate in a fatty food or streptozotocin causing adiposity, steatosis, and hyperglycemia. It is a novel therapeutic use for type 2 diabetes since it is improving insulin signaling, glucose utilization, and lipid metabolism through the AdipoR1/R2-APPL1-mediated pathway, which upregulated via the uncoupling protein 1 (UCP-1) in adipose tissue and elevated adiponectin levels in the circulation of liver and skeletal muscle (Tsai et al., 2021).
In 2019, Muangnoi et al. (2019) reported a prodrug for curcumin linked with diethyl succinate ester. “Curcumin diethyl succinate” (Figure 5) enhanced curcumin’s anti-proliferative effect on HepG2 cells via apoptotic induction. Recent study showed caspase induction and Bcl-2 inhibition for altering insulin signaling in human adipose tissue to prevent obesity.
Phumsuay et al. (2020) also identified another curcumin ester prodrug, which was linked with diglutaric acid. The “Curcumin diglutaric acid” (Figure 6) was an anti-inflammatory agent for the edema model. The levels of pro-inflammatory cytokine expression such as NO, IL-6, TNF-α, iNOS, and COX-2 expression were reduced. Curcumin diglutaric acid was a pro- and anti-inflammatory mediator secreted by adipose tissue subsequently lower systemic inflammation and the risk of metabolic diseases via the suppression of MAPK (ERK1/2, JNK, and p38) activity (Jayarathne et al., 2017; Phumsuay et al., 2020).
Khadka et al. (2021) also synthesized a prodrug of curcumin with monoglucuronide (CMG) (Figure 7), altering the gut microbiota and immune responses to treat obesity. As low fecal microbial diversity is associated with increased total fat and dyslipidemia, higher low-grade inflammation, and homeostasis, thus CMG administration could affect both the overall gut microbiome compositions and the abundance of individual bacteria in feces, ileal contents, and the ileal mucosa (Davis, 2016; Yoo et al., 2021).
Ginseng (Panax ginseng) has been widely used to improve human health. Asian countries are the most common but it is also used in the United States and Europe. Ginsenosides are the active ingredients, which involve a series of tetracyclic triterpenoid saponins. They are classified into oleanane type and dammarane type (17 carbons in a four-ring structure) according to their structural difference. Protopanaxadiol (PPD), and protopanaxatriol (PPT) groups are the prodrugs of ginsenosides from dammarane type. Similarly, an oleanane (Ro) type is sorted in the oleanolic acid group and ocotillol type pseudoginsenoside (Figure 8). Ra1, Ra2, Rb1, Rb2, Rc, Rd, Rg3, Rk1, Rg5, and Rh2, are the examples of PPD-type ginsenosides, etc., compound K (CK), and PPD, whereas PPT-type ginsenosides consist of Re, Rg1, Rg2, Rf, Rh1, and PPT, etc. (Shi et al., 2019). Among those chemical ingredients, the contents of Rb1, Rb2, Rc, Rd, Re, Rf, and Rg1 contribute more than 90% to ginsenosides (Fan et al., 2020).
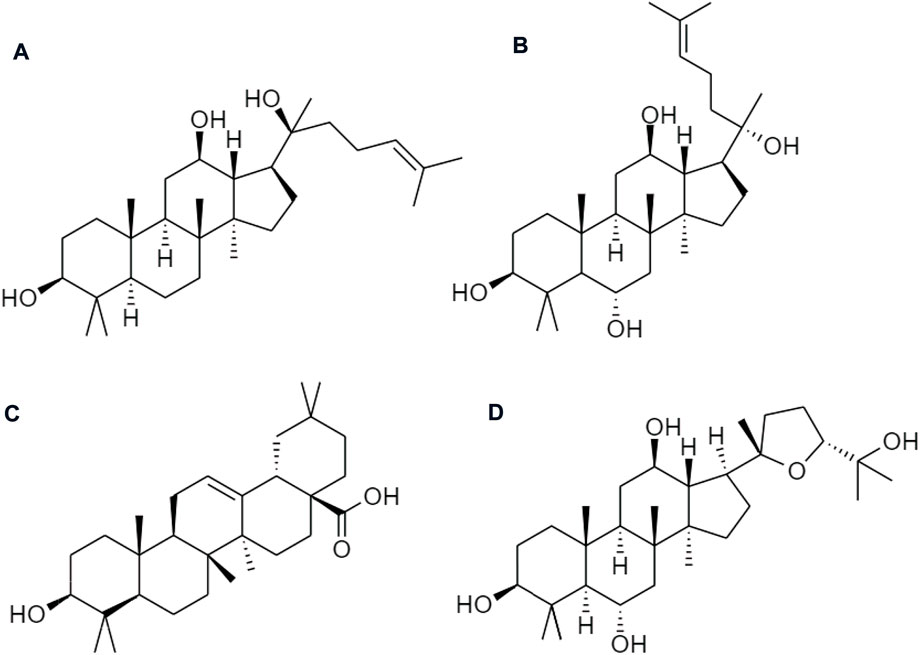
FIGURE 8. Chemical structures of (A) protopanaxadiol (PPD), (B) protopanaxatriol (PPT), (C) oleanane group (Ro), and (D) ocotillol type pseudoginsenoside.
Ginsenosides possess anti-inflammatory, anti-stress, anticancer, anti-oxidative, and anti-aging activities for preventing obesity, hyperlipidemia, hyperglycemia, and hepatic steatosis (Yan et al., 2018). Ginsenoside Rb2 is a novel AMP-activated protein kinase (AMPK) activator that reduces body weight, improves insulin sensitivity, and induces energy expenditure in diet-induced obese (DIO) rats. As ginsenosides target the central nervous system (CNS), which regulates leptin sensitivity in the cerebral cortex to prevent obesity and decreases the risk of central inflammation in the hypothalamus (Jeon et al., 2021).
Ginsenosides are distinctive triterpenoid dammarane saponins with very low solubility for oral administration of their slow absorption, extensive microbial deglycosylation, biliary excretion, and degradation of acidic ingredients such as Rg1 and Rb1 (Liu et al., 2009; Liu et al., 2010). Almost all ginsenoside dammarane is instability in the gastrointestinal tract. That is readily degraded or metabolized to secondary glycosides, aglycones, and other metabolites by gastric acid and/or the intestinal microflora. The bioavailability of ginsenosides are improved to improve the absorption by increasing water solubility, biofilm permeability, and stability in the gastrointestinal tract. The larger molecular size of ginsenosides with lower membrane permeability of the dammarane skeleton, and the sugar fractions have hydrophilic properties that promote the dissolution of ginsenosides. However, many ginsenosides have been reported as aglycones and lack sugars, thus showing low water solubility. On the other hand, ginsenoside is readily degraded by gastric acid hydrolysis via C-20 sugar radical epimerization and glycosyl elimination (Yang et al., 2011; Cheung and Law, 2022).
Celastrol (Figure 9) belongs to the “Celastraceae” family. It is extracted from the root of Tripterygium wilfordii (Venkatesha and Moudgil, 2016) containing natural triterpene with anti-inflammatory, antioxidant, and anti-cancer activities (Straub, 2017). Since obesity and diabetes are also associated with an excessive inflammatory response (Liu et al., 2015), celastrol acts as a leptin sensitizer in the pharmacological treatment of obesity. It could block food intake, reduce energy expenditure, and lead to a weight gain of about 45% in hyperleptinemia diet-induced (DIO) obese mice. It also regulates the leptin sensitivity in either leptin-deficient (ob/ob) or leptin receptor-deficient (db/db) mouse models (Li et al., 2018).
Other derivatives of celastrol such as (A) triptolide, (B) triptonide, and (C) wilforlide are also used as prodrugs for obesity (Figure 10) (Venkatesha and Moudgil, 2016). (A) Triptolide is an inflammatory mediator that inhibits the activation of the AMPK/mTOR signaling pathway to reduce the secretion of chemokine, monocyte chemotactic protein-1 (MCP-1) in Kupffer cells (KCs), Ma-CM, or 3T3-L1 to ameliorate obesity-induced inflammatory diseases (Li and Liu, 2021). (B) Triptonide without any toxic effect, but it may suppress the level of astrocyte-elevated gene-1 (AEG-1) (Fu et al., 2020). This regulates nuclear receptors to control lipid metabolism and reduces the risk of high-fat as well as high-cholesterol diets for obesity (Robertson et al., 2015). (C) Wilforlide improves rheumatoid arthritis progression, which inhibits M1 macrophage polarity and blocks Toll-like receptor 4 (TLR4) through activation of the NF-κB p65 pathway. It also deactivated the TLR4/NF-κB partially mediated signaling pathway (Cao et al., 2022) and modulates the obesity-induced inflammatory response (Rogero and Calder, 2018).
Several prodrugs of triptolide have been reported including omtriptolide, 5-hydroxytriptolide, and a disodium phosphonooxymethyl (Figure 11). These are involving carboxylic acid, amino acid esters, and a non-covalent interaction for targeting the protein to suppress kinases, and Hsp70 expression on the prevention of non-alcoholic fatty liver disease (NAFLD) progression and disodium phosphonooxymethyl reduce the activity of anti-inflammatory for obesity (Di Naso et al., 2015; Patil et al., 2015).
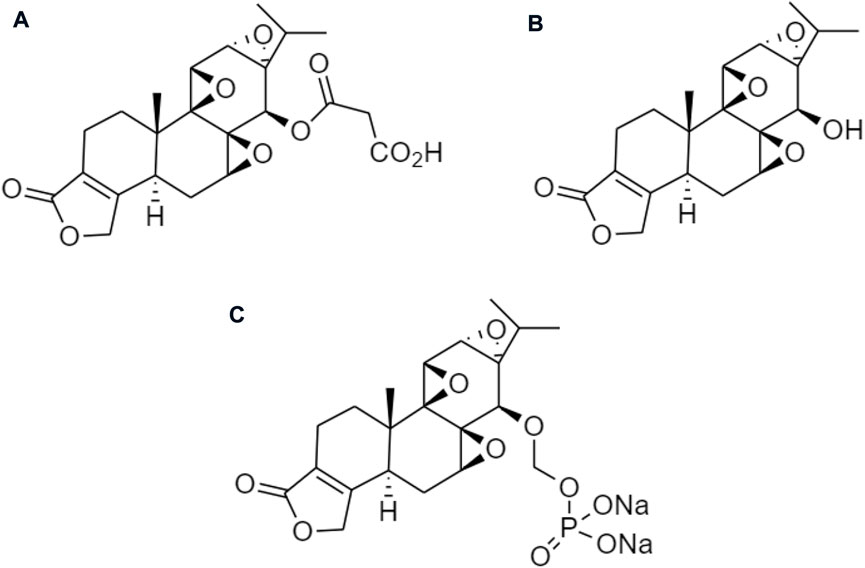
FIGURE 11. Chemical structures of (A) omtriptolide, (B) 5-hydroxytriptolide, and (C) disodium phosphonooxymethyl.
Berberine (Figure 12), is the major active ingredient from Coptis Chinensi. Berberine-containing medicinal plants have been applied in TCM to treat parasitic intestinal infection, bacterial diarrhea, and diabetes for a long time through the suppression of fatty acid synthase, acyl-CoA synthase, acetyl-CoA carboxylase, SREBP-1, C/EBPα, and PPARγ (Choi et al., 2006; Hu and Davies, 2009). Berberine blocks food absorption, reduces body gain, and visceral adipose weight by decreasing the level of PPARγ in high-fat diet-induced obesity mice when the level of GATA-binding proteins 3 increases and downregulates the expression of PPARγ and PPARα. GATA-binding proteins 2 and 3 (GATA-2 and GATA-3) are modulated differentiation of pre-adipocytes into mature adipocytes (Hu and Davies, 2010). Besides, gut bacteria act an important role to regulate the fat accumulation and its degradation metabolism, since berberine has antibacterial or anti-obesity activity. Han et al. (2011) reported that berberine lowered blood lipids and controls glycemic levels including free fatty acids and cholesterol, which activated thermogenesis in adipocytes and increase energy expenditure.
Despite the various therapeutic effects, berberine has not been yet developed as a marketed drug product. Poor absorption, fast metabolism, and wide tissue distribution lead to very low bioavailability and limit its translation to clinical settings. Most of the berberine is absorbed in the small intestine via oral administration. There are several reasons which are responsible for its low absorption and bioavailability. The chemical structure of berberine is a hydrophilic compound, which makes it difficult for it to cross the plasma membrane of intestinal cells. An intestinal first-pass elimination is extensive, around 50% of the berberine keep intact through the gastrointestinal tract. Berberrubine, thalifendine, demethyleneberberine, and jatrorrhizine are the four major metabolites (Figure 13) after being metabolized by the CYP450 isoenzyme (Li et al., 2011).
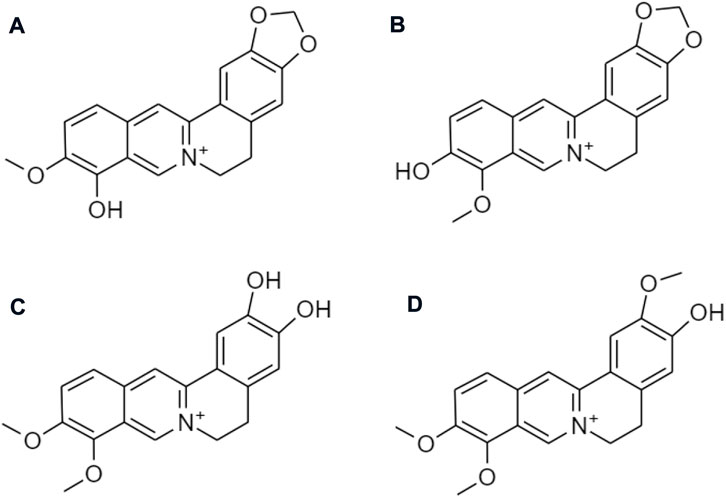
FIGURE 13. Chemical structures of (A) berberrubine, (B) thalifendine, (C) demethyleneberberine, and (D) jatrorrhizine.
Meanwhile, berberine is the substrate, which is regurgitated into the intestinal lumen by ATP-binding cassette (ABC) transporters. The ABC transporters include multidrug resistance-associated protein (MRP) and P-glycoprotein [P-gp, also named multidrug resistance protein1 (MDR1)] (Shitan et al., 2003).
In 2020, Habtemariam (2020) discovered a prodrug of berberine, 9O-Aryl berberine (Figure 14) for type-2 diabetes and associated diseases. It suppresses gluconeogenesis and lipid accumulation to prevent obesity through the inhibition of hepatocyte nuclear factor-4α (HNF-4α) and the microRNA miR122.
Artemisinin is derived from Artemisia annua (qinghao). Artemether and dihydroartemisinin (Figure 15) are the derivatives with anti-inflammatory properties, suitable for the inflammation of chronic metabolism (Law et al., 2020), which are implicated in their pathogenesis corresponding to obesity or other metabolic disorders (Shen et al., 2020). The artemisinin derivatives induce the accumulation of the browning of white adipose tissue (WAT) within the body and also enhance the brown adipose tissue (BAT) function to reduce the risk of obesity. PRDM16, PGC1a, and UCP1 are the brown genes upregulated by artemether and dihydroartemisinin, through the activation of the p38 MAPK/ATF2 axis and the deactivation of Akt/mTOR pathway for browning of C3H10T1/2 cells (Lu et al., 2016).
In 2007, Chung MC et al. reported a prodrug of dihydroartemisinin, “artesunate” through reduction and esterification using diisobutylaluminum hydride (DIBAL) and succinic anhydride (Figure 16). The prodrug with low toxicity and substantially increased solubility in water (Chung et al., 2007). The mechanism of artesunate for obesity included blocking the NF-κB pathway, inhibiting iNOS expression, and decreasing NO production (Jiang et al., 2020).
Zhang Y et al. also developed some prodrugs of artemisinin, such as SM934 and Anhydrodehydroartemisin (ADRT) (Figure 17). The SM934 ameliorated the experimental autoimmune encephalomyelitis (EAE) relating to obesity (Zhang et al., 2022a); whilst ADRT reduced the functions of CNS and decreased the immunity of a peripheral system (Lv et al., 2021). It inhibits adipogenesis and the implications for obesity, as well as inflammation due to the induction of a pro-inflammatory cytokine interleukin IL-17A, Th1/Th17, and IFN-γ (Ahmed and Gaffen, 2010).
Additionally, an artemisinin prodrug via modification of hydrocarbylene (Figure 18) with long-chain hydrocarbons was reported. It was a lipase inhibitor in the stomach and pancreas for reducing the absorption of dietary fats to manage obesity (Heck et al., 2000).
Recently, Sugiarto SR et al. investigated the pharmacokinetic properties of other prodrugs in artemisinin, which was the combination of artemether-lumefantrine (Figure 19) therapy for normal-weight, overweight, and obese male adults. Artemether and lumefantrine were metabolized through the hepatic CYP450 enzyme to produce protease inhibitors (PIs), and non-nucleoside reverse transcriptase inhibitors (NNRTIs), which suppressed the concentration of plasma to treat overweight (Sugiarto et al., 2022).
Chili pepper belongs to the genus Capsicum, which is an important spice widely consumed in various diets. It is used to treat pains, asthma, coughs, sore throat, common cold, and arthritis in TCM. Its unique components, including capsaicin and capsaicinoids (Figure 20), contribute to the pungent scent and heat sensation of hot chili pepper (Azlan et al., 2022).
Capsaicin (CAP) has many pharmacological benefits to humans, such as pain-alleviating, anti-inflammation, antioxidant, hypoglycemic, anticancer, antimicrobial, and anti-obesity effects. This is a potential agent for obesity by inhibiting fat accumulation and lipid oxidation, increasing the satiety of the hypothalamus, and preventing appetite and fat consumption. Glycerol-3-phosphate dehydrogenase (GDPH) and intracellular triglyceride are decreased in 3T3-L1 adipocytes because CAP inhibits the level of PPAR, C/EBP, and leptin. Besides, capsaicin significantly lowers the serum level of glucose and lipid including cholesterol and triglycerides in mice (Mahalak et al., 2022). Wang et al. proposed that capsaicin could change the structure and composition of gut microbiota to lower lipid absorption (Wang et al., 2020).
In 2021, Higgins et al. (2021) reported a prodrug of trans-form CAP, vocacapsaicin (trans-8-methyl-N-vanillyl-6-nonenamide) (Figure 21). This was a phenolic compound responsible for their characteristic taste and pungency. It was a water-soluble compound that rapidly converted to capsaicin. The transient receptor potential vanilloid 1 (TRPV1)-agonist was applied in the trigeminovascular system. CAP modulated the fasting blood glucose and insulin concentrations as well as decreased the levels of proinflammatory cytokines interleukin-1β and interleukin-6 to treat high-fat, or high-sucrose (HFHS) diet-induced obesity (Marics et al., 2017).
However, trans-CAP with high concentration inhibits gastric acid production, causing gastric inflammation and damaging our gastrointestinal mucosa. As a potent irritant, CAP with severe irritation, pain, and burning if exposed to the mucous membranes. Capsaicin with low bioavailability and a short half-life. It is rapidly metabolized in all tissues including the liver, kidney, intestine, serum, and blood after oral administration (Knotts et al., 2022).
Discussion
The prodrug is widely used in drug development because it is bio-reversible, inactive drug derivatives, and non-toxic, which can be converted into an active drug within the human body (Markovic et al., 2020). Prodrugs have many potential benefits with patient compliance, and more efficient absorption, distribution, metabolism, and excretion (ADME) properties that are suitable for lots of traditional Chinese medicines (TCMs). Some active compounds of TCM are insoluble and have lower absorption for the human body. Prodrugs of TCM improve the stability, increase solubility, and bioavailability of traditional Chinese medicines and reduce their toxicity, as well as foster the targetability.
Currently, prodrugs are further developed through the combination of “Nanotechnology.” Prodrugs with nanotechnology have many advantages, e.g., greater bioavailability, higher drug encapsulation, and loading efficiency, as well as the control of drug release (Luo et al., 2014; Lang et al., 2021; Zhang et al., 2021; Zhang et al., 2022b). Growing evidence has shown that the combination of nanotechnology and prodrug is more effective to treat obesity than the usage of prodrugs alone. In general, prodrugs processing with nanotechnology increase the drug solubility. It might fasten or increase the absorption rate of the original drug in an organ, which targeted the drug delivery to enhance the treatment effective rate and decrease the side effects, as well as the drug dose (Bahadori et al., 2019). These have been illustrated with the following examples:
(i) Ahmed et al. (2021) reported that curcumin-loaded chitosan/polyethylene glycol (PEG) blended poly lactic-co-glycolic acid (PLGA) nanoparticles, which reduced the body weight, body mass index (BMI), and Lee index. This diminished the weights of the liver, heart, and visceral adipose tissues. It also significantly reduced the weights of the gonadal and subcutaneous adipose tissues. (ii) Kim et al. (2018) discovered a diverse micro-/nano-sized delivery system using emulsions, polymers, and vesicles for improving bioavailability and maximizing the therapeutic potential of ginsenosides. The ginsenoside was easily absorbed by the human body as it was converted into secondary saponins and aglycones via this nano-system (Kim et al., 2018; Li et al., 2022), decreased body weight, regulated the concentration of insulin, and reduced energy expenditure to change the obese situations.
(iii) Liu et al. (2012) identified that celastrol linked with the chitosan, O-carboxymethyl chitosan, or N-[(2-hydroxy-3-N,N-dimethylhexadecyl ammonium)propyl] chitosan chloride was effective to treat overweight and insulin resistance in obesity, which decreased body mass, regulated the plasma glucose, insulin and leptin sensitivity, as well as an increased fecal lipid for preventing obesity.
(iv) Och et al. (2020) applied lysergol, D-α-tocopheryl polyethylene glycol 1000 succinate (TPGS), chitosan, and sodium caprate as polymers to form an anhydrous reverse micelle (ARM) delivery system of berberine nanoparticles for enhancing the oral bioavailability and absorption. The formulations of berberine increased the bioavailability of berberine compared to berberine alone. Berberine nanoparticle was an anti-obesity and anti-sclerotic agent by lowering the low-density lipoprotein (LDL) and testosterone levels. It stimulated glycolysis, improved insulin secretion, and inhibited gluconeogenesis and adipogenesis to treat obesity.
(v) Du et al. (2022) introduced a liposome-like nanosystem in the self-assembly of dimeric artesunate glycerophosphocholine conjugated to the antimalarial drug dihydroartemisinin (DHA) for killing parasites. Similarly, this liposome-like nanosystem of artemisinin might induce the differentiation and decrease of regulatory cells to minimize the number of adipose cells in obesity.
(vi) Lu et al. (2017) developed capsaicin-loaded nanoemulsions (C-NE) for enhancing the anti-obesity effect on gastric mucosa to degrade the high-fat diet. It also alleviated stomach inflammation to keep gastrointestinal smoothness prevent the adipose cells’ accumulation and reduce the risk of obesity.
Conclusion
Obesity is a global problem and promotes the incidence of various diseases, particularly cardiovascular disease, metabolic syndrome, diabetes, hypertension, osteoarthritis, and certain cancers. The Chinese herbal medicines’ derivatives, such as Curcumin¸ Ginsenoside, Celastrol, Berberine, Artemisinin, and Capsaicin are suitable Chinese medicinal herb-based therapeutics for Obesity. The above derivative as prodrugs are the possible approach to improve their poor solubility, absorption, and bioavailability. Up to the present, a combination of prodrugs from Chinese medicinal herbs and nanotechnology is the innovative strategy for treating obesity. However, much more works need to be done, especially in the clinical trial.
Author contributions
S‐KL and YW contributed to the draft and write; XL contributed to the information finding; D‐CT and WY‐L contributed to the read and make corrections; AW‐N and CX contributed to the supervise and final revise for the manuscript; and all authors approved the submitted version.
Funding
This work was supported by Funds of Talents for High-level University in the Construction of Guangzhou Medical University (02-412-2202-2104).
Conflict of interest
The authors declare that the research was conducted in the absence of any commercial or financial relationships that could be construed as a potential conflict of interest.
Publisher’s note
All claims expressed in this article are solely those of the authors and do not necessarily represent those of their affiliated organizations, or those of the publisher, the editors and the reviewers. Any product that may be evaluated in this article, or claim that may be made by its manufacturer, is not guaranteed or endorsed by the publisher.
References
Abet, V., Filace, F., Recio, J., Alvarez-Builla, J., and Burgos, C. (2017). Prodrug approach: An overview of recent cases. Eur. J. Med. Chem. 127, 810–827. doi:10.1016/j.ejmech.2016.10.061
Ahmed, H. H., Kotob, S. E., Abd-Rabou, A. A., Aglan, H. A., Elmegeed, G. A., and Mohawed, O. A. (2021). Pre-clinical evidence for the anti-obesity potential of quercetin and curcumin loaded chitosan/PEG blended PLGA nanoparticles. Biomed. Pharmacol. J. 14 (4), 1731–1759. doi:10.13005/bpj/2274
Ahmed, M., and Gaffen, S. L. (2010). IL-17 in obesity and adipogenesis. Cytokine Growth Factor Rev. 21 (6), 449–453. doi:10.1016/j.cytogfr.2010.10.005
Albert, A. (1958). Chemical aspects of selective toxicity. Nature 182, 421–422. doi:10.1038/182421a0
Azlan, A., Sultana, S., Huei, C. S., and Razman, M. R. (2022). Antioxidant, anti-obesity, nutritional and other beneficial effects of different chili pepper: A review. Molecules 27 (3), 898. doi:10.3390/molecules27030898
Bahadori, E., Farjami, Z., Rezayi, M., Lngari, H., Darroudi, M., Avan, A., et al. (2019). Recent advances in nanotechnology for the treatment of metabolic syndrome. Diabetes Metab. Syndr. 13 (2), 1561–1568. doi:10.1016/j.dsx.2019.03.002
Bastard, J-P., Maachi, M., Lagathu, C., Kim, M. J., Caron, M., Vidal, H., et al. (2006). Recent advances in the relationship among obesity, inflammation, and insulin resistance. Eur. Cytokine Netw. 17, 4–12. https://www.jle.com/fr/index.phtml
Bode, L. M., Bunzel, D., Huch, M., Cho, G. S., Ruhland, D., Bunzel, M., et al. (2013). In vivo and in vitro metabolism of trans-resveratrol by human gut microbiota. Am. J. Clin. Nutr. 2, 295–309. doi:10.3945/ajcn.112.049379
Cao, Y., Liu, J., Huang, C., Tao, Y., Wang, Y., Chen, X., et al. (2022). Wilforlide A ameliorates the progression of rheumatoid arthritis by inhibiting M1 macrophage polarization. J. Pharmacol. Sci. 148 (1), 116–124. doi:10.1016/j.jphs.2021.10.005
Chen, J. W., Kong, Z. L., Tsai, M. L., Lo, C. Y., Ho, C. T., and Lai, C. S. (2018). Tetrahydrocurcumin ameliorates free fatty acid-induced hepatic steatosis and improves insulin resistance in HepG2 cells. J. Food Drug Anal. 26 (3), 1075–1085. doi:10.1016/j.jfda.2018.01.005
Cheung, C. H. Y., and Law, S. K. (2022). Could "celastrol" Be suitable for the treatment of obesity? Regen. Med. Ther. 5 (1), 55–56. doi:10.36959/654/394
Cho, S., and Yoon, Y. R. (2018). Understanding the pharmacokinetics of prodrug and metabolite. Transl. Clin. Pharmacol. 26 (1), 1–5. doi:10.12793/tcp.2018.26.1.1
Cho, S. H., Lee, J. S., Thabane, L., and Lee, J. (2009). Acupuncture for obesity: Asystematic review and meta-analysis. Int. J. Obes. 33, 183–196. doi:10.1038/ijo.2008.269
Choi, B. H., Ahn, I. S., Kim, Y. H., Park, J. W., Lee, S. Y., Hyun, C. K., et al. (2006). Berberine reduces the expression of adipogenic enzymes and inflammatory molecules of 3T3-L1 adipocyte. Exp. Mol. Med. 3138 (6), 599–605. doi:10.1038/emm.2006.71
Chu, X., Sun, B., Huang, Q., Peng, S., Zhou, Y., and Zhang, Y. (2020). Quantitative knowledge presentation models of traditional Chinese medicine (TCM): A review. Artif. Intell. Med. 103, 101810. doi:10.1016/j.artmed.2020.101810
Chung, M. C., Ferreira, E. I., Santos, J. L., Giarolla, J., Rando, D. G., Almeida, A. E., et al. (2007). Prodrugs for the treatment of neglected diseases. Molecules 13 (3), 616–677. doi:10.3390/molecules13030616
Davis, C. D. (2016). The gut microbiome and its role in obesity. Nutr. Today 51 (4), 167–174. doi:10.1097/NT.0000000000000167
Di Naso, F. C., Porto, R. R., Fillmann, H. S., Maggioni, L., Padoin, A. V., Ramos, R. J., et al. (2015). Obesity depresses the anti-inflammatory HSP70 pathway, contributing to NAFLD progression. Obes. (Silver Spring) 23 (1), 120–129. doi:10.1002/oby.20919
Du, Y., Giannangelo, C., He, W., Shami, G. J., Zhou, W., Yang, T., et al. (2022). Dimeric artesunate glycerophosphocholine conjugate nano-assemblies as slow-release antimalarials to overcome kelch 13 mutant artemisinin resistance. Antimicrob. Agents Chemother. 66 (5), e0206521. doi:10.1128/aac.02065-21
Fan, W., Huang, Y., Zheng, H., Li, S., Li, Z., Yuan, L., et al. (2020). Ginsenosides for the treatment of metabolic syndrome and cardiovascular diseases: Pharmacology and mechanisms. Biomed. Pharmacother. 132, 110915. doi:10.1016/j.biopha.2020.110915
Fu, L., Niu, X., Jin, R., Xu, F., Ding, J., Zhang, L., et al. (2020). Triptonide inhibits metastasis potential of thyroid cancer cells via astrocyte elevated gene-1. Transl. Cancer Res. 9 (2), 1195–1204. doi:10.21037/tcr.2019.12.94
Fulton, S., Décarie-Spain, L., Fioramonti, X., Guiard, B., and Nakajima, S. (2022). The menace of obesity to depression and anxiety prevalence. Trends Endocrinol. Metab. 33 (1), 18–35. doi:10.1016/j.tem.2021.10.005
Gandhi, P. M., Chabukswar, A. R., and Jagdale, S. C. (2019). Carriers for prodrug synthesis: A review. Indian J. Pharm. Sci. 81 (3), 406–414. doi:10.36468/pharmaceutical-sciences.524
Ghasemi, A., and Jeddi, S. (2017). Anti-obesity and anti-diabetic effects of nitrate and nitrite. Nitric Oxide 70 (1), 9–24. doi:10.1016/j.niox.2017.08.003
Habtemariam, S. (2020). The quest to enhance the efficacy of berberine for type-2 diabetes and associated diseases: Physicochemical modification approaches. Biomedicines 8 (4), 90. doi:10.3390/biomedicines8040090
Han, J., Lin, H., and Huang, W. (2011). Modulating gut microbiota as an anti-diabetic mechanism of berberine. Med. Sci. Monit. 17 (7), RA164–7. doi:10.12659/msm.881842
Heck, A. M., Yanovski, J. A., and Calis, K. A. (2000). Orlistat, a new lipase inhibitor for the management of obesity. Pharmacotherapy 20 (3), 270–279. doi:10.1592/phco.20.4.270.34882
Higgins, O., Rule, S., and Cleary, J. (2021). Analgesics of the future: The potential of vocacapsaicin injections for knee pain. Pract. Pain Manag. 21 (2). https://www.practicalpainmanagement.com/
Hu, Y., and Davies, G. E. (2009). Berberine increases expression of GATA-2 and GATA-3 during inhibition of adipocyte differentiation. Phytomedicine 16 (9), 864–873. doi:10.1016/j.phymed.2009.03.002
Hu, Y., and Davies, G. E. (2010). Berberine inhibits adipogenesis in high-fat diet-induced obesity mice. Fitoterapia 81 (5), 358–366. doi:10.1016/j.fitote.2009.10.010
Ireson, C. R., Jones, D. J., Orr, S., Coughtrie, M. W., Boocock, D. J., Williams, M. L., et al. (2002). Metabolism of the cancer chemopreventive agent curcumin in human and rat intestine. Cancer Epidemiol. Biomarkers Prev. 11 (1), 105–111.
Jayarathne, S., Koboziev, I., Park, O. H., Oldewage-Theron, W., Shen, C. L., and Moustaid-Moussa, N. (2017). Anti-inflammatory and anti-obesity properties of food bioactive components: Effects on adipose tissue. Prev. Nutr. Food Sci. 22 (4), 251–262. doi:10.3746/pnf.2017.22.4.251
Jeon, H., Huynh, D. T. N., Baek, N., Nguyen, T. L. L., and Heo, K. S. (2021). Ginsenoside-Rg2 affects cell growth via regulating ROS-mediated AMPK activation and cell cycle in MCF-7 cells. Phytomedicine. 85, 153549. doi:10.1016/j.phymed.2021.153549
Jiang, Y. Y., Shui, J. C., Zhang, B. X., Chin, J. W., and Yue, R. S. (2020). The potential roles of artemisinin and its derivatives in the treatment of type 2 diabetes mellitus. Front. Pharmacol. 11, 585487. doi:10.3389/fphar.2020.585487
Khadka, S., Omura, S., Sato, F., Nishio, K., Kakeya, H., and Tsunoda, I. (2021). Curcumin β-D-glucuronide modulates an autoimmune model of multiple sclerosis with altered gut microbiota in the ileum and feces. Front. Cell. Infect. Microbiol. 11, 772962. doi:10.3389/fcimb.2021.772962
Kim, H., Lee, J. H., Kim, J. E., Kim, Y. S., Ryu, C. H., Lee, H. J., et al. (2018). Micro-/nano-sized delivery systems of ginsenosides for improved systemic bioavailability. J. Ginseng Res. 42 (3), 361–369. doi:10.1016/j.jgr.2017.12.003
Knotts, T., Mease, K., Sangameswaran, L., Felx, M., Kramer, S., and Donovan, J. (2022). Pharmacokinetics and local tissue response to local instillation of vocacapsaicin, a novel capsaicin prodrug, in rat and rabbit osteotomy models. J. Orthop. Res., 6. doi:10.1002/jor.25271
Koboziev, I., Scoggin, S., Gong, X., Mirzaei, P., Zabet-Moghaddam, M., Yosofvand, M., et al. (2020). Effects of curcumin in a mouse model of very high fat diet-induced obesity. Biomolecules 10 (10), 1368. doi:10.3390/biom10101368
Kokil, G. R., and Rewatkar, P. V. (2010). Bioprecursor prodrugs: Molecular modification of the active principle. Mini Rev. Med. Chem. 10, 1316–1330. doi:10.2174/138955710793564179
Lang, T., Li, N., Zhang, J., Li, Y., Rong, R., and Fu, Y. (2021). Prodrug-based nano-delivery strategy to improve the antitumor ability of carboplatin in vivo and in vitro. Drug Deliv. 28 (1), 1272–1280. doi:10.1080/10717544.2021.1938754
Law, S., Au, D. C., Leung, A. W., and Xu, C. (2022). Curcumin” for body’s immunity. Int. Jr. Infect Dis Epidemlgy. 3 (2), 50–51. doi:10.51626/ijide.2022.03.00020
Law, S., Leung, A. W., and Xu, C. (2020). Is the traditional Chinese herb "Artemisia annua" possible to fight against COVID-19? Integr. Med. Res. 9 (3), 100474. doi:10.1016/j.imr.2020.100474
Li, H., Jiang, H., Xu, L., Deng, Y., Xu, J., and Zhao, Y. (2022). Effects of different extraction methods in pharmacopoeia on the content and structure transformation of ginsenosides. Molecules 27 (14), 4347. doi:10.3390/molecules27144347
Li, W. F., Jiang, J. G., and Chen, J. (2008). Chinese medicine and its modernization demands. Arch. Med. Res. 39 (2), 246–251. doi:10.1016/j.arcmed.2007.09.011
Li, X., and Liu, Z. (2021). Triptolide exhibits anti-inflammatory effects on adipocytes and macrophages by inhibition of AMPK/mTOR pathway. North Carolina: Researchsquare. doi:10.21203/rs.3.rs-944266/v1
Li, Y., Cao, H., Liu, M., Zhang, B., Zhang, X., Shi, D., et al. (2018). Different modulation of Panax notoginseng on the absorption profiling of triptolide and tripterine from Tripterygium wilfordii in rat intestine. Chin. Med. 13, 1. doi:10.1186/s13020-017-0157-6
Li, Y., Ren, G., Wang, Y. X., Kong, W. J., Yang, P., Wang, Y. M., et al. (2011). Bioactivities of berberine metabolites after transformation through CYP450 isoenzymes. J. Transl. Med. 15 (9), 62. doi:10.1186/1479-5876-9-62
Liu, H., Yang, J., Du, F., Gao, X., Ma, X., Huang, Y., et al. (2009). Absorption and disposition of ginsenosides after oral administration of Panax notoginseng extract to rats. Drug Metab. Dispos. 37 (12), 2290–2298. doi:10.1124/dmd.109.029819
Liu, J., Lee, J., Salazar Hernandez, M. A., Mazitschek, R., and Ozcan, U. (2015). Treatment of obesity with celastrol. Cell 161 (5), 999–1011. doi:10.1016/j.cell.2015.05.011
Liu, R., Zhang, J., Liu, W., Kimura, Y., and Zheng, Y. (2010). Anti-Obesity effects of protopanaxdiol types of Ginsenosides isolated from the leaves of American ginseng (Panax quinquefolius L.) in mice fed with a high-fat diet. Fitoterapia 81 (8), 1079–1087. doi:10.1016/j.fitote.2010.07.002
Liu, X., Zhi, X., Liu, Y., Wu, B., Sun, Z., and Shen, J. (2012). Effect of chitosan, O-carboxymethyl chitosan, and N-[(2-hydroxy-3-N, N-dimethylhexadecyl ammonium)propyl] chitosan chloride on overweight and insulin resistance in a murine diet-induced obesity. J. Agric. Food Chem. 60 (13), 3471–3476. doi:10.1021/jf205226r
Loos, R. J. F., and Yeo, G. S. H. (2022). The genetics of obesity: From discovery to biology. Nat. Rev. Genet. 23, 120–133. doi:10.1038/s41576-021-00414-z
Lu, M., Cao, Y., Ho, C. T., and Huang, Q. (2017). The enhanced anti-obesity effect and reduced gastric mucosa irritation of capsaicin-loaded nanoemulsions. Food Funct. 8 (5), 1803–1809. doi:10.1039/c7fo00173h
Lu, P., Zhang, F. C., Qian, S. W., Li, X., Cui, Z. M., Dang, Y. J., et al. (2016). Artemisinin derivatives prevent obesity by inducing browning of WAT and enhancing BAT function. Cell Res. 26 (10), 1169–1172. doi:10.1038/cr.2016.108
Luo, C., Sun, J., Sun, B., and He, Z. (2014). Prodrug-based nanoparticulate drug delivery strategies for cancer therapy. Trends Pharmacol. Sci. 35 (11), 556–566. doi:10.1016/j.tips.2014.09.008
Lv, J., Zhuang, W., Zhang, Y., Xie, L., Xiang, Z., Zhao, Q., et al. (2021). 9, 10-anhydrodehydroartemisinin attenuates experimental autoimmune encephalomyelitis by inhibiting Th1 and Th17 cell differentiation. Inflammation 44 (5), 1793–1802. doi:10.1007/s10753-021-01456-5
Mahalak, K. K., Bobokalonov, J., Firrman, J., Williams, R., Evans, B., Fanelli, B., et al. (2022). Analysis of the ability of capsaicin to modulate the human gut microbiota in vitro. Nutrients 14 (6), 1283. doi:10.3390/nu14061283
Marics, B., Peitl, B., Pázmándi, K., Bácsi, A., Németh, J., Oszlács, O., et al. (2017). Diet-induced obesity enhances TRPV1-mediated neurovascular reactions in the dura mater. Headache 57 (3), 441–454. doi:10.1111/head.13033
Markovic, M., Ben-Shabat, S., and Dahan, A. (2020). Prodrugs for improved drug delivery: Lessons learned from recently developed and marketed products. Pharmaceutics 12 (11), 1031. doi:10.3390/pharmaceutics12111031
Mattarei, A., Azzolini, M., La Spina, M., Zoratti, M., Paradisi, C., and Biasutto, L. (2015). Amino acid carbamates as prodrugs of resveratrol. Sci. Rep. 5, 15216. doi:10.1038/srep15216
Muangnoi, C., Ratnatilaka, N. B. P., Jithavech, P., Supasena, W., Paraoan, L., Patumraj, S., et al. (2019). Curcumin diethyl disuccinate, a prodrug of curcumin, enhances anti-proliferative effect of curcumin against HepG2 cells via apoptosis induction. Sci. Rep. 9, 11718. doi:10.1038/s41598-019-48124-1
Nurcahyanti, A. D. R., Cokro, F., Wulanjati, M. P., Mahmoud, M. F., Wink, M., and Sobeh, M. (2022). Curcuminoids for metabolic syndrome: Meta-analysis evidences toward personalized prevention and treatment management. Front. Nutr. 9, 891339. doi:10.3389/fnut.2022.891339
Och, A., Podgórski, R., and Nowak, R. (2020). Biological activity of berberine-A summary update. Toxins (Basel) 12 (11), 713. doi:10.3390/toxins12110713
Pan, X. F., Wang, L., and Pan, A. (2021). Epidemiology and determinants of obesity in China. Lancet. Diabetes Endocrinol. 9 (6), 373–392. Erratum in: Lancet Diabetes Endocrinol. (2021) 9(7):e2. doi:10.1016/S2213-8587(21)00045-0
Patil, S., Lis, L. G., Schumacher, R. J., Norris, B. J., Morgan, M. L., Cuellar, R. A., et al. (2015). Phosphonooxymethyl prodrug of triptolide: Synthesis, physicochemical characterization, and efficacy in human colon adenocarcinoma and ovarian cancer xenografts. J. Med. Chem. 58 (23), 9334–9344. doi:10.1021/acs.jmedchem.5b01329
Perez, C., Daniel, K. B., and Cohen, S. M. (2013). Evaluating prodrug strategies for esterase-triggered release of alcohols. ChemMedChem 8 (10), 1662–1667. doi:10.1002/cmdc.201300255
Phumsuay, R., Muangnoi, C., Dasuni Wasana, P. W., Hasriadi Vajragupta, O., Rojsitthisak, P., et al. (2020). Molecular insight into the anti-inflammatory effects of the curcumin ester prodrug curcumin diglutaric acid in vitro and in vivo. Int. J. Mol. Sci. 21 (16), 5700. doi:10.3390/ijms21165700
Raimund, M., Kubinyi, H., and Folkers, G. (2011). Prodrugs and targeted delivery: Towards better ADME properties. John Wiley Sons 1 (47), 131–151. doi:10.1002/9783527633166.ch1
Robertson, C. L., Srivastava, J., Siddiq, A., Gredler, R., Emdad, L., Rajasekaran, D., et al. (2015). Astrocyte elevated gene-1 (AEG-1) regulates lipid homeostasis. J. Biol. Chem. 290 (29), 18227–18236. doi:10.1074/jbc.M115.661801
Rogero, M. M., and Calder, P. C. (2018). Obesity, inflammation, toll-like receptor 4 and fatty acids. Nutrients 10 (4), 432. doi:10.3390/nu10040432
Shah, K., Gupta, J. K., Chauhan, N. S., Upmanyu, N., Shrivastava, S. K., and Mishra, P. (2017). Prodrugs of NSAIDs: A review. Open Med. Chem. J. 11, 146–195. doi:10.2174/1874104501711010146
Shen, S., Liao, Q., Lyu, M., Wong, Y. K., Zhang, X., Zhou, J., et al. (2020). The potential of artemisinins as anti-obesity agents via modulating the immune system. Pharmacol. Ther. 216, 107696. doi:10.1016/j.pharmthera.2020.107696
Shi, Z. Y., Zeng, J. Z., and Wong, A. S. T. (2019). Chemical structures and pharmacological profiles of ginseng saponins. Molecules 24 (13), 2443. doi:10.3390/molecules24132443
Shitan, N., Bazin, I., Dan, K., Obata, K., Kigawa, K., Ueda, K., et al. (2003). Involvement of CjMDR1, a plant multidrug-resistance-type ATP-binding cassette protein, in alkaloid transport in Coptis japonica. Proc. Natl. Acad. Sci. U. S. A. 100 (2), 751–756. doi:10.1073/pnas.0134257100
Soleimani, V., Sahebkar, A., and Hosseinzadeh, H. (2018). Turmeric (curcuma longa) and its major constituent (curcumin) as nontoxic and safe substances: Review. Phytother. Res. 32, 985–995. doi:10.1002/PTR.6054
Straub, R. H. (2017). The brain and immune system prompt energy shortage in chronic inflammation and ageing. Nat. Rev. Rheumatol. 13, 743–751. doi:10.1038/nrrheum.2017.172
Sugiarto, S. R., Page-Sharp, M., Drinkwater, J. J., Davis, W. A., Salman, S., and Davis, T. M. E. (2022). Pharmacokinetic properties of the antimalarial combination therapy artemether-lumefantrine in normal-weight, overweight and obese healthy male adults. Int. J. Antimicrob. Agents 59 (1), 106482. doi:10.1016/j.ijantimicag.2021.106482
Sui, Y., Zhao, H. L., Wong, V. C., Brown, N., Li, X. L., Kwan, A. K., et al. (2012). A systematic review on use of Chinese medicine and acupuncture for treatment of obesity. Obes. Rev. 13 (5), 409–430. doi:10.1111/j.1467-789X.2011.00979.x
Tak, Y. J., and Lee, S. Y. (2021). Long-term efficacy and safety of anti-obesity treatment: Where do we stand? Curr. Obes. Rep. 10, 14–30. doi:10.1007/s13679-020-00422-w
Testa, B. (2009). Prodrugs: Bridging pharmacodynamic/pharmacokinetic gaps. Curr. Opin. Chem. Biol. 3, 338–344. doi:10.1016/j.cbpa.2009.04.620
Tsai, Y. Z., Tsai, M. L., Hsu, L. Y., Ho, C. T., and Lai, C. S. (2021). Tetrahydrocurcumin upregulates the adiponectin-AdipoR pathway and improves insulin signaling and pancreatic β-cell function in high-fat diet/streptozotocin-induced diabetic obese mice. Nutrients 13 (12), 4552. doi:10.3390/nu13124552
Venkatesha, S. H., and Moudgil, K. D. (2016). Celastrol and its role in controlling chronic diseases. Adv. Exp. Med. Biol. 928, 267–289. doi:10.1007/978-3-319-41334-1_12
Wang, L., Zhang, B., Huang, F., Liu, B., and Xie, Y. (2016). Curcumin inhibits lipolysis via suppression of ER stress in adipose tissue and prevents hepatic insulin resistance. J. Lipid Res. 57, 1243–1255. doi:10.1194/JLR.M067397
Wang, Y., Tang, C., Tang, Y., Yin, H., and Liu, X. (2020). Capsaicin has an anti-obesity effect through alterations in gut microbiota populations and short-chain fatty acid concentrations. Food Nutr. Res. 19, 64. doi:10.29219/fnr.v64.3525
Wink, M. (2022). Current understanding of modes of action of multicomponent bioactive phytochemicals: Potential for nutraceuticals and antimicrobials. Annu. Rev. Food Sci. Technol. 13, 337–359. doi:10.1146/annurev-food-052720-100326
Wink, M. (2015). Modes of action of herbal medicines and plant secondary metabolites. Medicines 2, 251–286. doi:10.3390/MEDICINES2030251
Yan, R., Yang, Y., and Chen, Y. (2018). Pharmacokinetics of Chinese medicines: Strategies and perspectives. Chin. Med. 13, 24. doi:10.1186/s13020-018-0183-z
Yang, Z., Gao, S., Wang, J., Yin, T., Teng, Y., Wu, B., et al. (2011). Enhancement of oral bioavailability of 20(S)-Ginsenoside Rh2 through improved understanding of its absorption and efflux mechanisms. Drug Metab. Dispos. 39 (10), 1866–1872. doi:10.1124/dmd.111.040006
Ye, M., Qiu, H., Cao, Y., Zhang, M., Mi, Y., Yu, J., et al. (2017). Curcumin improves palmitate-induced insulin resistance in human umbilical vein endothelial cells by maintaining proteostasis in endoplasmic reticulum. Front. Pharmacol. 8, 148. doi:10.3389/fphar.2017.00148
Yoo, S., Park, B. I., Kim, D. H., Lee, S., Lee, S. H., Shim, W. S., et al. (2021). Ginsenoside absorption rate and extent enhancement of black ginseng (CJ EnerG) over red ginseng in healthy adults. Pharmaceutics 13 (4), 487. doi:10.3390/pharmaceutics13040487
Yu, Q., Liu, Y., Wu, Y., and Chen, Y. (2018). Dihydrocurcumin ameliorates the lipid accumulation, oxidative stress and insulin resistance in oleic acid-induced L02 and HepG2 cells. Biomed. Pharmacother. 103, 1327–1336. doi:10.1016/j.biopha.2018.04.143
Zawilska, J. B., Wojcieszak, J., and Olejniczak, A. B. (2013). Prodrugs: A challenge for the drug development. Pharmacol. Rep. 65, 1–14. doi:10.1016/s1734-1140(13)70959-9
Zhang, Y., Cui, H., Zhang, R., Zhang, H., and Huang, W. (2021). Nanoparticulation of prodrug into medicines for cancer therapy. Adv. Sci. 8 (18), e2101454. doi:10.1002/advs.202101454
Zhang, Y., Lv, J., Zhang, S., Yang, H., Shen, J., Du, C., et al. (2022). Synthesis and biological evaluation of artemisinin derivatives as potential MS agents. Bioorg. Med. Chem. Lett. 64, 128682. doi:10.1016/j.bmcl.2022.128682
Zhang, Y., Pei, Q., Yue, Y., and Xie, Z. (2022). Binary dimeric prodrug nanoparticles for self-boosted drug release and synergistic chemo-photodynamic therapy. J. Mat. Chem. B 10 (6), 880–886. doi:10.1039/d1tb02638k
Zou, T., Li, S., Wang, B., Wang, Z., Liu, Y., and You, J. (2021). Curcumin improves insulin sensitivity and increases energy expenditure in high-fat-diet-induced obese mice associated with activation of FNDC5/irisin. Nutrition 90, 111263. doi:10.1016/j.nut.2021.111263
Glossary
ADME: Absorption, Distribution, Metabolism, Excretion
ADRT: Anhydrodehydroartemisin
AEG-1: Astrocyte Elevated Gene-1
AMPK: AMP-activated protein kinase
ARM: Anhydrous reverse micelle
BAT: Brown adipose tissue
CADD: Computer-aided drug design
CMG: Curcumin bound with monoglucuronide
C-NE: Capsaicin-loaded nanoemulsions
CNS: Central nervous system
DHC: Dihydrocurcumin
DIO: Diet-induced obese
EAE: Experimental autoimmune encephalomyelitis
Eotaxin: Eotaxin-1/Eotaxin-2/Eotaxin-3
ER: Endoplasmic reticulum
ERK: Extracellular signal-related kinase (ERK)
FABP4: Fatty acid-binding protein 4
FAS: Fatty acid synthase
FOXO1: Forkhead box protein O1
GATA-2 & GATA-3: GATA binding protein 2 and 3
GDPH: Glycerol-3-phosphate dehydrogenase
GSK3β: Glycogen synthase kinase 3β
HDC: Hexahydrocurcumin
HNF-4α: Hepatocyte nuclear factor-4α
HSP90: Heat shock protein 90
IRS-1: Insulin receptor substrate 1
KC: Kupffer cells
LDL: Low-density lipoprotein
LPS: Lipopolysaccharides
MAPK: Mitogen-activated protein kinase
MCP-1: Monocyte Chemoattractant Protein-1
MS: Multiple sclerosis
NAFLD: Non-alcoholic fatty liver disease
NF-κB: Nuclear factor kappa-light-chain-enhancer of activated B cells
NNRTIs: Non-nucleoside reverse transcriptase inhibitors
NRF2-KEAP1: NF-E2-related factor 2-Kelch-like ECH-associated protein 1
OHC: Octahydrocurcumin
PAI-1: Plasminogen activator inhibitor-1
pAKT: Phosphorylated serine-threonine protein kinase
PD: Pharmacodynamics
PEG: Polyethylene glycol
PH: Pharmaceutical
PI3K: Phosphatidylinositol 3-kinase
PIs: Protease inhibitors
PK: Pharmacokinetic
abrPLGA: Poly lactic-co-glycolic acid
PNPLA3: Patatin-like phospholipase domain containing 3
PPARα: Peroxisome proliferator-activated receptor-α
PPARγ: Peroxisome proliferator-activated receptor gamma
PPD: Protopanaxadiol
PPT: Protopanaxatriol
Rantes: Regulated on activation, normal T cell expressed and secreted
ROS: Reactive oxygen species
SREBP-1C: Sterol regulatory element-binding protein 1C
TCM: Traditional Chinese medicine
THC: Tetrahydrocurcumin
TLR4: Toll-like receptor 4 Beclin-1
TPGS: D-α-tocopheryl polyethylene glycol 1000 succinate
TRPV1: Transient receptor potential vanilloid 1
UCP-1: Uncoupling protein 1
WAT: White adipose tissue
Keywords: obesity, herbal medicine, prodrug, traditional Chinese medicine (TCM), active ingredient, medicinal plants, bioavailability, nanotechnology
Citation: Law SK, Wang Y, Lu X, Au DCT, Chow WYL, Leung AWN and Xu C (2022) Chinese medicinal herbs as potential prodrugs for obesity. Front. Pharmacol. 13:1016004. doi: 10.3389/fphar.2022.1016004
Received: 10 August 2022; Accepted: 14 September 2022;
Published: 03 October 2022.
Edited by:
Mingyue Wu, National University of Singapore, SingaporeReviewed by:
Jing Tang, University of Helsinki, FinlandMai Yan Yuen, The University of Hong Kong, Hong Kong, SAR China
Copyright © 2022 Law, Wang, Lu, Au, Chow, Leung and Xu. This is an open-access article distributed under the terms of the Creative Commons Attribution License (CC BY). The use, distribution or reproduction in other forums is permitted, provided the original author(s) and the copyright owner(s) are credited and that the original publication in this journal is cited, in accordance with accepted academic practice. No use, distribution or reproduction is permitted which does not comply with these terms.
*Correspondence: Chuanshan Xu, eGNzaGFuQDE2My5jb20=
†These authors have contributed equally to this work and share first authorship