- 1School of Medicine, I-Shou University, Kaohsiung, Taiwan
- 2Department of Pathology, National Cheng Kung University Hospital, College of Medicine, National Cheng Kung University, Tainan, Taiwan
- 3Department of Medical Laboratory Science and Biotechnology, College of Medicine, National Cheng Kung University, Tainan, Taiwan
- 4Department of Microbiology and Immunology, College of Medicine, National Cheng Kung University, Tainan, Taiwan
- 5Center of Infectious Disease and Signaling Research, National Cheng Kung University, Tainan, Taiwan
- 6Department of Pediatrics, National Cheng Kung University Hospital, College of Medicine, National Cheng Kung University, Tainan, Taiwan
Neonatal infection with nonpolio enteroviruses (EVs) causes nonspecific febrile illnesses and even life-threatening multiorgan failure. Hepatitis, which often results in hepatic necrosis followed by disseminated intravascular coagulopathy, is one of the most severe and frequent fatal neonatal EV infection complications. Coxsackievirus B (CVB) 1–5 and many echoviruses have been most commonly identified. Neonatal EV infection treatment has usually involved initial supportive care. Studies for CVB and echovirus infection treatments were developed for more than thirty years. Intravenous immunoglobulin and pleconaril therapy was performed in some clinical trials. Additionally, other studies demonstrated antiviral and/or anti-inflammatory pathogenesis mechanisms of neonatal EV hepatitis in in vitro or in vivo models. These treatments represented promising options for the clinical practice of neonatal EV hepatitis. However, further investigation is needed to elucidate the whole therapeutic potential and safety problems.
Introduction
Hepatitis is characterized by jaundice, hepatomegaly, elevated transaminases, and hyperbilirubinemia and is frequently associated with thrombocytopenia and coagulopathy. Acute hepatic necrosis and endothelial injury, liver failure, bleeding complications, and renal failure may ensue (Wang et al., 2001). Acute hepatic necrosis is the most common mechanism of acute liver failure (ALF). ALF in children is a potentially devastating condition that occurs in previously healthy children of all ages. ALF is rare in early infancy, and its detection may be difficult in neonates. Often, a viral-like illness preludes the deterioration into jaundice, coagulopathy, encephalopathy, and death (Alonso et al., 2017). ALF has been invariably associated with high mortality, accounting for 80%–100% (Burdelski, 1994).
ALF is primarily caused by viruses, toxins, or idiopathies (Manns, 1991; Gotthardt et al., 2007). ALF has different etiologies in early infancy than in older children, with a greater contribution from metabolic disorders, neonatal hemochromatosis, hematological malignancies, and hypoxia (McClean et al., 2003; Rademacher et al., 2011). Viral infection accounts for 20%–30% of neonatal ALF (Taylor et al., 2016). Even though hepatitis A, B, and E viruses are the primary cause of ALF, especially in developing countries (Pandit et al., 2015; Manka et al., 2016), infections in neonates are usually asymptomatic and self-limited. Notably, neonates born to HBV carrier mothers with anti-HBe antibodies are prone to develop ALF (Beath et al., 1992). A national immunization program was implemented in 1984 to tackle hyperendemic hepatitis B in Taiwan (Chen et al., 1978). The hepatitis B carrier rate in children covered by the program decreased by 85%, from approximately 15% to <1%, 20 years after the program implementation (Wait and Chen 2012). The success of the hepatitis B vaccine, the most effective method for combating hepatitis B, continues worldwide. Partly, universal hepatitis B vaccination has been justified as ALF prevention in children.
Neonatal ALF may be caused by agents that typically produce mild illness in adults. Herpesviruses, especially herpes simplex viruses, are identified in 50%–70% of neonates with virus-induced ALF (Durand et al., 2001; Verma et al., 2006; Sundaram et al., 2011; Borovsky et al., 2021). Effective anti-HSV drugs are available. However, skin lesions are absent in 30% of neonates with HSV-induced hepatitis, which leads to difficult and often delayed diagnosis. Early diagnosis and prompt anti-HSV therapy are essential to reduce high mortality and morbidity (Verma et al., 2006).
Enterovirus (EV) infections are the most frequently recognized significant pathogens of neonate ALF without available antiviral drugs or vaccines. They account for 3%–50% of virus-induced ALF in neonates (Shanmugam et al., 2011; Sundaram et al., 2011; Bersani et al., 2020; Borovsky et al., 2021). Neonatal infection with EV is not rare. Asymptomatic, recent respiratory or gastrointestinal illness in the mother or family members should include EV infections. Neonates can be infected during the prenatal, intrapartum, or postnatal periods. A sentinel case is thought to occur due to a vertical transmission followed by horizontal transmission to other neonates. Most neonates (79%) who contract EV are typically asymptomatic or have mild illnesses (Jenista et al., 1984). However, 21% of infected neonates develop more severe illnesses, including hepatitis, meningoencephalitis, myocarditis, or fulminant sepsis-like illnesses, and require hospitalization (Jenista et al., 1984; Vergnano et al., 2015). Zhang et al. (2021) reviewed the studies focusing on neonatal EV infections and reported 237 severe cases previously. They demonstrated that 65% of those febrile cases developed hepatitis or coagulopathy. Additionally, 46% of the severe EV cases developed hepatitis or coagulopathy, with a death rate of 27%. Coxsackievirus B (CVB) and echoviruses are identified in more than 80% of EV-infected neonates with fever and hepatitis (Chuang et al., 2019; Zhang et al., 2021).
Echovirus
The echoviruses, which have been associated with neonatal ALF, lie within the EV taxonomy, especially the echovirus 11 outbreaks (Wang et al., 2001). Since May 2018, the Taiwan Centers for Disease Control was notified of increased reports related to severe EV infection in newborns. Through July 31, severe echovirus 11 infections were confirmed in eight neonatal patients (five males, age 0–12 days). Hepatitis with coagulopathy (n = 8), sepsis syndrome (n = 8), myocarditis (n = 2), and multiorgan dysfunction (n = 7) were included as clinical presentations. The mortality was 87.5% (n = 7) (Su et al., 2018).
Echovirus can cause disseminated infection with fulminant hepatic failure in neonates (Wang et al., 2001). Severe neonatal disseminated infections with fulminant liver disease have been linked to echovirus types 6, 7, 9, 10, 11, 12, 14, 19, and 30 (Hughes et al., 1972; Mostoufizadeh et al., 1983; Speer et al., 1984; Ho-Yen et al., 1989; Verboon-Maciolek et al., 1997; Pino-Ramírez et al., 2008). Associated virulence factors with certain echovirus serotypes appear as important neonatal infection determinants. Serotype 11 caused 70% of all cases (Modlin, 1986), while echovirus 11 is one of the most isolated serotypes obtained from patients in clinical laboratories. Khetsuriani et al. (2006) reported echovirus 11 (14%) as the leading serotype of commonly isolated EV from neonates from 1983 to 2003 in the United States of America. In Taiwan, the first reported fatal case of echovirus 11 infection was a newborn with fulminant hepatitis and disseminated intravascular coagulopathy (DIC) (Hsiao et al., 2003). Diffuse extensive centrilobular hemorrhagic necrosis and other findings suggestive of DIC are the dominant pathologic findings of echovirus 11 infection (Mostoufizadeh et al., 1983). Scattered foci of dystrophic myocardial calcification, hepatic architecture distortion with fibrous connective tissue surrounding regenerative nodules and large foci of dystrophic calcification, and adrenal hemorrhagic necrosis and calcification are other disseminated findings (Wells et al., 2021).
Wells et al. (2021) reported that human homolog of the neonatal Fc receptor (hFcRn) expression and type I interferon (IFN) signaling ablation are key host determinants that are involved in echovirus pathogenesis, similar to severe hepatitis observed in humans (Bose et al., 1983). Extensive zone 3 hemorrhagic necrosis, endothelial injury, and minimal inflammation were observed in the acute stage, followed by veno-occlusive changes as pathologically evidenced (Ventura et al., 2001).
Coxsackievirus B
Severe neonatal CVB diseases usually occurred in the first 2 weeks of life. A severe CVB neonatal infectious spectrum may be diagnosed as meningitis, encephalitis, meningoencephalitis, myocarditis, hepatitis, pancreatitis, coagulopathy, and pneumonitis (Khetsuriani et al., 2006; Adams et al., 2013). Infants with hepatitis clinically presented with jaundice, hepatomegaly, abdominal distension, and coagulopathy. Laboratory abnormalities included elevated transaminases, hyperbilirubinemia, thrombocytopenia, and evidence of DIC (Wang et al., 1998). Figure 1 for a start shows the serotype distribution of CVB isolates from 1998–2021 in the virological laboratory of the National Cheng Kung University Hospital.
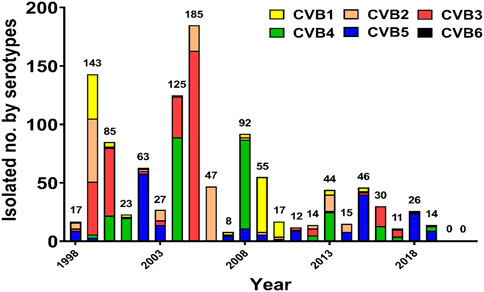
FIGURE 1. Annual distribution of coxsackievirus B isolates according to the serotype in National Cheng Kung University Hospital, 1998–2021.
Serum aspartate aminotransferase (AST) and alanine aminotransferase (ALT) are released from necrotic hepatocytes into circulation, causing elevated serum concentrations. Serum AST and ALT concentrations may dramatically increase with acute liver damage progression and subsequently decrease to the normal range by depleting their content in the liver. Soudée et al. (2014) revealed that the risk factors for severe neonatal EV infections included hepatitis (73%), thrombocytopenia (82%), and coagulopathy (64%). Lv et al. (2016) found 18 (13.7%) patients with low platelet counts. The risk of EV infections increases 3.647-fold once the platelet count is < 150 × 109/L. Thrombocytopenia is indicated as a major risk factor for development of severe EV infection in neonates. Bryant et al. (2004) reported two of 10 cases with hepatitis, both of whom had severe hepatitis, thrombocytopenia, and DIC. They had significantly increased liver enzymes and coagulopathy and the association between these features and mortality. Patients had DIC with elevated prothrombin time, activated partial thromboplastin time (APTT), D-dimer, and fibrinogen levels, similar to our previous findings (Bryant et al., 2004). CVB1-5 had the risk of the fatal outcome of EV neonatal hepatitis during the past 30 years in our hospital. However, only CVB4, with a very high (40%) reported case fatality to the National Enterovirus Surveillance System, had a significantly increased risk of death compared with other EV serotypes (Khetsuriani et al., 2006).
Wang et al. (1998) documented fatal neonatal cases of CVB infection and presented clinical and histologic evidence to demonstrate liver involvement and intravascular coagulation. Accordingly, ALF in early infancy has been invariably associated with high mortality. The emerging concept of “immune dysregulation” that leads to aberrant natural killer cell function and cytokine-related liver necrosis has assumed a prominent place in theories related to indeterminate pediatric ALF (Taylor et al., 2016). They further showed reduced blood AST, ALT, and lactate dehydrogenase (LDH) levels and mortality of CVB3-infected mice treated with the coxsackievirus-adenovirus receptor (CAR) antibody (Liu et al., 2013).
Treatment
Neonatal EV infection treatment focuses on managing the most severe physiological derangements. The mainstay of treatment for severe disease is supportive care. Intravenous immunoglobulin (IVIG) has been therapeutically used against EVs in the clinical settings of neonates (Rotbart, 2000). Previously, Abzug et al. (1995) performed a randomized trial using IVIG and supportive therapy in neonates with EV disease and revealed that IVIG products for the patient’s viral isolate provided a more rapid reduction in viremia than in patients who did not receive IVIG. High antibody concentrations of IVIG to neutralize the specific EV and reduce inflammations may alter the disease course in the infected neonates.
Antiviral therapy for neonatal EV infections is not commercially available. High-throughput screening of natural compounds or FDA-approved drugs provides mounting antiviral candidates. We selectively reviewed those compounds evaluated in vivo. Several compounds have been developed that selectively inhibit the interaction of CVB with CAR. The WIN compounds are antiviral drugs that interact with the hydrophobic pocket at the bottom of the canyon. Pleconaril, vapendavir, disoxaril, and pirodavir are the new generation of metabolically stable capsid function inhibitors (Kearns et al., 1999). Among them, pleconaril is the first to exert broad-spectrum anti-EV activity. Pleconaril is considered well-tolerated in treated patients (Abzug et al., 2003; Hayden et al., 2003; Desmond et al., 2006). The compassionate use of pleconaril in patients with life-threatening EV meningitis or meningoencephalitis shows favorable outcomes (Rotbart et al., 2001). However, the randomized, double-blinded, placebo-controlled studies show inconsistent efficacy in treating patients with EV meningitis or meningoencephalitis (Abzug et al., 2003; Desmond et al., 2006). Also, pleconaril might induce adverse effects due to its ability to increase cytochrome P-450 3A (CYP3A) activity (Ma et al., 2006) and reduce plasma levels of CYP3A substrates, including some contraceptive steroids (Hayden et al., 2003). These, taken together, lead to the halted development of pleconaril in clinical use. The NIH-sponsored collaborative antiviral study group performed a randomized, double-blind, placebo-controlled study of the virologic and clinical efficacy, pharmacokinetics, and safety of pleconaril for treating severe neonatal EV sepsis (hepatitis with coagulopathy and myocarditis) and revealed an increased survival among EV-infected persons who received pleconaril due to its potential efficacy for neonatal EV infections (Abzug et al., 2016). Further studies are needed to evaluate the efficacy of pleconaril in treating EV-induced ALF in neonates.
Drugs that interfere with viral and cellular proteins during replication were developed. EV 2C is the most conserved nonstructural protein among EVs and contains RNA helicase activity that is critical for the viral life cycle. Fang et al. (2021) demonstrated that targeting the helicase activity of 2C with a rationally designed peptide is an efficient antiviral strategy, stability, and bioavailability against CVB3 and E11 in the ICR mouse model. They suggested a promising clinical potential in these peptides to be further developed as broad-spectrum antiviral drugs. EV replicates within replication organelles composed of phosphatidylinositol-4-phosphate (PI4P)-enriched membranes from Golgi apparatuses and the endoplasmic reticulum (Hsu et al., 2010; Greninger et al., 2012; Sasaki et al., 2012). The phosphatidylinositol 4-kinase IIIβ (PI4KIIIβ), the kinase responsible for PI4P generation, is a potent broad-spectrum anti-EV target. Oxoglaucine, blocking PI4KIIIβ activity (Arita et al., 2015), and enviroxime, disrupting 3A interaction with PI4KIIIβ, are developed to suppress PI4KIIIβ function. Among them, enviroxime is the first one tested in trials, which, however, is halted at phase II due to the lack of therapeutic benefits in treating rhinovirus-infected patients (Phillpotts et al., 1983; Miller et al., 1985).
Repurposing existing medications would facilitate the development of antiviral therapy for EV. Licensed antiviral agents for treating highly pathogenic RNA virus infections included anti-influenza medications, M2 channel inhibitors (amantadine and rimantadine), and neuraminidase inhibitors (oseltamivir). Ribavirin is licensed for treating respiratory syncytial virus and hepatitis C virus infections (De Clercq, 2004). Park et al. (2011) revealed that amantadine and ribavirin exhibited antiviral activity against the Korean E18 strain, with amantadine showing stronger effects than ribavirin.
The high mutation rate (10–3 per nucleotide) of EV leads to the inevitable development of drug resistance and limits the general use of antiviral drugs in patients. For instance, in the trial using pleconaril to treat common colds, rhinoviruses with reduced susceptibility or complete resistance to pleconaril were isolated in 10.7% and 2.7% of drug-treated patients, respectively (Pevear et al., 2005). A single mutation in the viral 2C protein is sufficient to confer resistance against the antiviral treatment but was only investigated in in vitro experiments (De Palma et al., 2008). Combining two or more drugs with synergistic effects or distinct antiviral mechanisms is proposed to overcome the issue. Serial studies show that consecutive alternating administration of a WIN-like compound, pleconaril or disoxaril, followed by two replication inhibitors, guanidine hydrochloride or MDL-860 and oxoglaucine, protects mice from lethal CBV infections (Stoyanova et al., 2015a; Stoyanova et al., 2015b; Vassileva-Pencheva et al., 2016). Notably, pleconaril increases the cytochrome P-450 3A (CYP3A) enzyme activity (Ma et al., 2006) and might reduce other drug efficacy in combined use. Whether drug efficacy is diminished in combined use with pleconaril should be evaluated. Stoyanova et al. (2015a) showed that the triple combination (pleconaril, guanidine-HCl, and oxoglaucine) increases the barriers to drug resistance without altering drug sensibility. These studies demonstrate a promising strategy to treat fatal EV infection, which requires further investigation.
Another strategy to overcome drug resistance is targeting cellular proteins, especially those with pro-viral or immune-modulatory functions, since cellular targets are unlikely to mutate in response to therapies. Our previous studies of the liver in CVB-infected mice histopathologically demonstrated polymorphonuclear cell infiltration, massive hepatocyte necrosis, and apoptosis (Liu et al., 2013). Wang et al. (2019) showed that CVB infection decreased programmed cell death protein 1 (PD-1) ligand expression. Furthermore, systemic PD-L1 (B7H1; CD274) and PD-L2 (B7-DC; CD273) treatment markedly augmented the apoptosis of proliferating lymphocytes and alleviated injury in the cardiomyocytes caused by CVB infection. They suggested the PD-1 pathway as a potential immunotherapeutic target for CVB infection prevention and treatment.
Natural compounds, a large majority coming from plant origin, have enormously contributed as immunomodulatory therapeutics. Natural medicines have always fascinated humans with their quality of harmless treatments with minimal side effects. There are thousands of natural compounds, such as the compounds and their derivatives, which are known to influence the immune system either by affecting the immune cell functions, such as dendritic cells, macrophages, lymphocytes, and natural killer cells, or by affecting the antibody secretion to control the infection and maintain immune homeostasis (Bragg et al., 2018). Oroxylin A is an O-methylated flavone that is found in the medicinal plant Scutellaria baicalensis (Tebruegge et al., 2009). Several previous studies have shown broad-spectrum antiviral activities of extracts and compounds from S. baicalensis. Oroxylin A treatment mitigated the histological lesions and apoptotic cell death, reduced viral titers, and decreased the serum levels of interleukin-6 and tumor necrosis factor-α in CVB3-infected mice (Kwon et al., 2016). Saururus chinensis (Lour.) Baill is a folk medicine that had antiviral effects on HIV-1 (Lee et al., 2010), HSV (Tian et al., 2012), and EV71 (Wang et al., 2015). Schisandra chinensis Baill extract exerted significant antiviral activity against CVB3 infection in vero cells and demonstrated manassantin B (Man B) as one of the antiviral components. Man B protected from systemic infection of CVB3 and exerted antiviral activity by activation of the STING/TBK-1/IRF3 signaling pathway. Furthermore, Man B reduced CVB3-mediated inflammatory cytokine production in mice (Song et al., 2019). Ethyl 3-hydroxyhexanoate (EHX) is a fatty acid ethyl ester of 3-hydroxyhexanoic acid, which is a key volatile compound in several fruits and is a potent antiviral compound (Medina et al., 2020). Olasunkanmi et al. (2022) demonstrated that EHX treatment significantly inhibits CVB replication both in vivo and in vitro.
EV epidemics have highlighted the public health impact in Taiwan. The recurrences of known serotypes and the evolution of additional new variants may be anticipated. CVBs and echoviruses are one of the most important infectious agents associated with acute hepatitis in neonates. Both viral and immune mechanisms are involved based on further understanding of the hepatic injury pathogenesis caused by CVBs and echoviruses. Antiviral, anti-inflammatory therapy and the potential combination with immunomodulatory therapies may hold the greatest benefits for improving the outcome of CVBs and echovirus hepatitis. An extensive future investigation is required concerning their mechanisms of action at the systemic, cellular, and molecular levels to extend up to broad-spectrum clinical trials.
Author contributions
SM-W designed the research and revised the manuscript; SH-C and SM-W obtained funding; LC-W, HP-T, SH-C, and SM-W implemented research; LC-W and SM-W interpreted data, analyzed statistics, and drafted the manuscript.
Funding
This study was funded in part by the Ministry of Science and Technology Taiwan (MOST 109-2327-B-006-009, MOST 110-2327-B-006-004) and Higher Education Sprout Project, Ministry of Education to the Headquarters of University Advancement at National Cheng Kung University (NCKU).
Conflict of interest
The authors declare that the research was conducted in the absence of any commercial or financial relationships that could be construed as a potential conflict of interest.
Publisher’s note
All claims expressed in this article are solely those of the authors and do not necessarily represent those of their affiliated organizations, or those of the publisher, the editors, and the reviewers. Any product that may be evaluated in this article, or claim that may be made by its manufacturer, is not guaranteed or endorsed by the publisher.
References
Abzug, M. J., Cloud, G., Bradley, J., Sanchez, P. J., Romero, J., Powell, D., et al. (2003). Double blind placebo-controlled trial of pleconaril in infants with enterovirus meningitis. Pediatr. Infect. Dis. J. 22, 335–341. doi:10.1097/01.inf.0000059765.92623.70
Abzug, M. J., Keyserling, H. L., Lee, M. L., Levin, M. J., and Rotbart, H. A. (1995). Neonatal enterovirus infection: Virology, serology, and effects of intravenous immune globulin. Clin. Infect. Dis. 20, 1201–1206. doi:10.1093/clinids/20.5.1201
Abzug, M. J., Michaels, M. G., Wald, E., Jacobs, R. F., Romero, J. R., Sánchez, P. J., et al. (2016). A randomized, double-blind, placebo-controlled trial of pleconaril for the treatment of neonates with enterovirus sepsis. J. Pediatr. Infect. Dis. Soc. 5, 53–62. doi:10.1093/jpids/piv015
Adams, M. J., King, A. M. Q., and Carstens, E. B. (2013). Ratification vote on taxonomic proposals to the international committee on taxonomy of viruses (2013). Arch. Virol. 158, 2023–2030. doi:10.1007/s00705-013-1688-5
Alonso, E. M., Horslen, S. P., Behrens, E. M., and Doo, E. (2017). Pediatric acute liver failure of undetermined cause: A research workshop. Hepatology 65, 1026–1037. doi:10.1002/hep.28944
Arita, M., Philipov, S., and Galabov, A. S. (2015). Phosphatidylinositol 4-kinase III beta is the target of oxoglaucine and pachypodol (Ro 09-0179) for their anti-poliovirus activities, and is located at upstream of the target step of brefeldin A. Microbiol. Immunol. 59, 338–347. doi:10.1111/1348-0421.12261
Beath, S. V., Boxall, E. H., Watson, R. M., Tarlow, M. J., and Kelly, D. A. (1992). Fulminant Hepatitis B in infants born to anti-HBe Hepatitis B carrier mothers. BMJ 304, 1169–1170. doi:10.1136/bmj.304.6835.1169
Bersani, I., Auriti, C., Piersigilli, F., Dotta, A., Diomedi-Camassei, F., Di Pede, A., et al. (2020). Neonatal acute liver failure due to enteroviruses: A 14 years single NICU experience. J. Matern. Fetal. Neonatal Med. 33, 2576–2580. doi:10.1080/14767058.2018.1555806
Borovsky, K., Banc-Husu, A. M., Saul, S. A., Neighbors, K., Kelly, S., Alonso, E. M., et al. (2021). Applying an age-specific definition to better characterize etiologies and outcomes in neonatal acute liver failure. J. Pediatr. Gastroenterol. Nutr. 73, 80–85. doi:10.1097/MPG.0000000000003103
Bose, C. L., Gooch, W. M., Sanders, G. O., and Bucciarelli, R. L. (1983). Dissimilar manifestations of intrauterine infection with echovirus 11 in premature twins. Arch. Pathol. Lab. Med. 107, 361–363.
Bragg, R. R., Meyburgh, C. M., Lee, J. Y., and Coetzee, M. (2018). Potential treatment options in a post-antibiotic era. Adv. Exp. Med. Biol. 1052, 51–61. doi:10.1007/978-981-10-7572-8_5
Bryant, P. A., Tingay, D., Dargaville, P. A., Starr, M., and Curtis, N. (2004). Neonatal coxsackie B virus infection-a treatable disease? Eur. J. Pediatr. 163, 223–228. doi:10.1007/s00431-004-1408-y
Burdelski, M. (1994). Liver transplantation in children. Acta Paediatr. Suppl. 83, 27–30. doi:10.1111/j.1651-2227.1994.tb13225.x
Chen, D. S., and Sung, J. L. (1978). Hepatitis B virus infection and chronic liver disease in Taiwan. Acta hepatogastroenterol. (Stuttg) 25, 423–430.
Chuang, Y. Y., and Huang, Y. C. (2019). Enteroviral infection in neonates. J. Microbiol. Immunol. Infect. 52, 851–857. doi:10.1016/j.jmii.2019.08.018
De Clercq, E. (2004). Antiviral drugs in current clinical use. J. Clin. Virol. 30, 115–133. doi:10.1016/j.jcv.2004.02.009
De Palma, A. M., Vliegen, I., De Clercq, E., and Neyts, J. (2008). Selective inhibitors of picornavirus replication. Med. Res. Rev. 28, 823–884. doi:10.1002/med.20125
Desmond, R. A., Accortt, N. A., Talley, L., Villano, S. A., Soong, S. J., and Whitley, R. J. (2006). Enteroviral meningitis: Natural history and outcome of pleconaril therapy. Antimicrob. Agents Chemother. 50, 2409–2414. doi:10.1128/AAC.00227-06
Durand, P., Debray, D., Mandel, R., Baujard, C., Branchereau, S., Gauthier, F., et al. (2001). Acute liver failure in infancy: A 14-year experience of a pediatric liver transplantation center. J. Pediatr. 139, 871–876. doi:10.1067/mpd.2001.119989
Fang, Y., Wang, C., Wang, C., Yang, R., Bai, P., Zhang, X. Y., et al. (2021). Antiviral peptides targeting the helicase activity of enterovirus nonstructural protein 2C. J. Virol. 95, e02324–e02320. doi:10.1128/jvi.02324-20
Gotthardt, D., Riediger, C., Weiss, K. H., Encke, J., Schemmer, P., Schmidt, J., et al. (2007). Fulminant hepatic failure: Etiology and indications for liver transplantation. Nephrol. Dial. Transpl. 22 (Suppl. 8), viii5-viii8. doi:10.1093/ndt/gfm650
Greninger, A. L., Knudsen, G. M., Betegon, M., Burlingame, A. L., and Derisi, J. L. (2012). The 3A protein from multiple picornaviruses utilizes the golgi adaptor protein ACBD3 to recruit PI4KIIIβ. J. Virol. 86, 3605–3616. doi:10.1128/JVI.06778-11
Hayden, F. G., Herrington, D. T., Coats, T. L., Kim, K., Cooper, E. C., Villano, S. A., et al. (2003). Efficacy and safety of oral pleconaril for treatment of colds due to picornaviruses in adults: Results of 2 double-blind, randomized, placebo-controlled trials. Clin. Infect. Dis. 36, 1523–1532. doi:10.1086/375069
Ho-Yen, D. O., Hardie, R., Mcclure, J., Cunningham, N. E., and Bell, E. J. (1989). Fatal outcome of echovirus 7 infection. Scand. J. Infect. Dis. 21, 459–461. doi:10.3109/00365548909167452
Hsiao, C. C., Tsao, L. Y., and Chen, H. N. (2003). Echovirus 11 sepsis in a neonate: Report of one case. Acta Paediatr. Taiwan. 44, 104–105.
Hsu, N. Y., Ilnytska, O., Belov, G., Santiana, M., Chen, Y. H., Takvorian, P. M., et al. (2010). Viral reorganization of the secretory pathway generates distinct organelles for RNA replication. Cell 141, 799–811. doi:10.1016/j.cell.2010.03.050
Hughes, J. R., Wilfert, C. M., Moore, M., Benirschke, K., and De Hoyos-Guevara, E. (1972). Echovirus 14 infection associated with fatal neonatal hepatic necrosis. Am. J. Dis. Child. 123, 61–67. doi:10.1001/archpedi.1972.02110070111017
Jenista, J. A., Powell, K. R., and Menegus, M. A. (1984). Epidemiology of neonatal enterovirus infection. J. Pediatr. 104, 685–690. doi:10.1016/s0022-3476(84)80944-0
Kearns, G. L., Abdel-Rahman, S. M., James, L. P., Blowey, D. L., Marshall, J. D., Wells, T. G., et al. (1999). Single-dose pharmacokinetics of a pleconaril (VP63843) oral solution in children and adolescents. Pediatric Pharmacology Research Unit Network. Antimicrob. Agents Chemother. 43, 634–638. doi:10.1128/aac.43.3.634
Khetsuriani, N., Lamonte, A., Oberste, M. S., and Pallansch, M. (2006). Neonatal enterovirus infections reported to the national enterovirus surveillance system in the United States, 1983–2003. Pediatr. Infect. Dis. J. 25, 889–893. doi:10.1097/01.inf.0000237798.07462.32
Kwon, B. E., Song, J. H., Song, H. H., Kang, J. W., Hwang, S. N., Rhee, K. J., et al. (2016). Antiviral activity of oroxylin A against coxsackievirus B3 alleviates virus-induced acute pancreatic damage in mice. PLoS One 11, e0155784. doi:10.1371/journal.pone.0155784
Lee, J., Huh, M. S., Kim, Y. C., Hattori, M., and Otake, T. (2010). Lignan, sesquilignans and dilignans, novel HIV-1 protease and cytopathic effect inhibitors purified from the rhizomes of Saururus chinensis. Antivir. Res. 85, 425–428. doi:10.1016/j.antiviral.2009.11.002
Liu, J. Y., Wang, S. M., Chen, I. C., Yu, C. K., and Liu, C. C. (2013). Hepatic damage caused by coxsackievirus B3 is dependent on age-related tissue tropisms associated with the coxsackievirus-adenovirus receptor. Pathog. Dis. 68, 52–60. doi:10.1111/2049-632x.12044
Lv, X. Q., Qian, L. H., Wu, T., and Yuan, T. M. (2016). Enterovirus infection in febrile neonates: A hospital-based prospective cohort study. J. Paediatr. Child. Health 52, 837–841. doi:10.1111/jpc.13193
Ma, J. D., Nafziger, A. N., Rhodes, G., Liu, S., and Bertino, J. S. (2006). Duration of pleconaril effect on cytochrome P450 3A activity in healthy adults using the oral biomarker midazolam. Drug Metab. Dispos. 34, 783–785. doi:10.1124/dmd.105.007831
Manka, P., Verheyen, J., Gerken, G., and Canbay, A. (2016). Liver failure due to acute viral hepatitis (A-E). Visc. Med. 32, 80–85. doi:10.1159/000444915
Manns, M. P. (1991). New therapeutic aspects in fulminant hepatic failure. Chest 100, 193S–196s. doi:10.1378/chest.100.3_supplement.193s
Mcclean, P., and Davison, S. M. (2003). Neonatal liver failure. Semin. Neonatol. 8, 393–401. doi:10.1016/s1084-2756(03)00095-2
Medina, S., Perestrelo, R., Pereira, R., and Câmara, J. S. (2020). Evaluation of volatilomic fingerprint from apple fruits to ciders: A useful tool to find putative biomarkers for each apple variety. Foods 9, E1830. doi:10.3390/foods9121830
Miller, F. D., Monto, A. S., Delong, D. C., Exelby, A., Bryan, E. R., and Srivastava, S. (1985). Controlled trial of enviroxime against natural rhinovirus infections in a community. Antimicrob. Agents Chemother. 27, 102–106. doi:10.1128/AAC.27.1.102
Modlin, J. F. (1986). Perinatal echovirus infection: Insights from a literature review of 61 cases of serious infection and 16 outbreaks in nurseries. Rev. Infect. Dis. 8, 918–926. doi:10.1093/clinids/8.6.918
Mostoufizadeh, M., Lack, E. E., Gang, D. L., Perez-Atayde, A. R., and Driscoll, S. G. (1983). Postmortem manifestations of echovirus 11 sepsis in five newborn infants. Hum. Pathol. 14, 818–823. doi:10.1016/s0046-8177(83)80304-9
Olasunkanmi, O. I., Mageto, J., Avala Ntsigouaye, J., Yi, M., Fei, Y., Chen, Y., et al. (2022). Novel antiviral activity of ethyl 3-hydroxyhexanoate against coxsackievirus B infection. Front. Microbiol. 13, 875485. doi:10.3389/fmicb.2022.875485
Pandit, A., Mathew, L. G., Bavdekar, A., Mehta, S., Ramakrishnan, G., Datta, S., et al. (2015). Hepatotropic viruses as etiological agents of acute liver failure and related-outcomes among children in India: A retrospective hospital-based study. BMC Res. Notes 8, 381. doi:10.1186/s13104-015-1353-z
Park, K., Yeo, S., Baek, K., Cheon, D., Choi, Y., Park, J., et al. (2011). Molecular characterization and antiviral activity test of common drugs against echovirus 18 isolated in Korea. Virol. J. 8, 516. doi:10.1186/1743-422x-8-516
Pevear, D. C., Hayden, F. G., Demenczuk, T. M., Barone, L. R., Mckinlay, M. A., and Collett, M. S. (2005). Relationship of pleconaril susceptibility and clinical outcomes in treatment of common colds caused by rhinoviruses. Antimicrob. Agents Chemother. 49, 4492–4499. doi:10.1128/AAC.49.11.4492-4499.2005
Phillpotts, R. J., Wallace, J., Tyrrell, D. A., and Tagart, V. B. (1983). Therapeutic activity of enviroxime against rhinovirus infection in volunteers. Antimicrob. Agents Chemother. 23, 671–675. doi:10.1128/AAC.23.5.671
Pino-Ramírez, R. M., Pertierra-Cortada, A., Iriondo-Sanz, M., Krauel-Vidal, X., and Muñoz-Almagro, C. (2008). Neonatal echovirus 30 infection associated with severe hepatitis in twin neonates. Pediatr. Infect. Dis. J. 27, 88. doi:10.1097/INF.0b013e31815b395b
Rademacher, S., Oppert, M., and Jörres, A. (2011). Artificial extracorporeal liver support therapy in patients with severe liver failure. Expert Rev. Gastroenterol. Hepatol. 5, 591–599. doi:10.1586/egh.11.59
Rotbart, H. A. (2000). Antiviral therapy for enteroviruses and rhinoviruses. Antivir. Chem. Chemother. 11, 261–271. doi:10.1177/095632020001100402
Rotbart, H. A., Webster, A. D., and Pleconaril Treatment Registry, G. (2001). Treatment of potentially life-threatening enterovirus infections with pleconaril. Clin. Infect. Dis. 32, 228–235. doi:10.1086/318452
Sasaki, J., Ishikawa, K., Arita, M., and Taniguchi, K. (2012). ACBD3-mediated recruitment of PI4KB to picornavirus RNA replication sites. EMBO J. 31, 754–766. doi:10.1038/emboj.2011.429
Shanmugam, N. P., Bansal, S., Greenough, A., Verma, A., and Dhawan, A. (2011). Neonatal liver failure: Aetiologies and management--state of the art. Eur. J. Pediatr. 170, 573–581. doi:10.1007/s00431-010-1309-1
Song, J. H., Ahn, J. H., Kim, S. R., Cho, S., Hong, E. H., Kwon, B. E., et al. (2019). Manassantin B shows antiviral activity against coxsackievirus B3 infection by activation of the STING/TBK-1/IRF3 signalling pathway. Sci. Rep. 9, 9413. doi:10.1038/s41598-019-45868-8
Soudée, S., Schuffenecker, I., Aberchih, J., Josset, L., Lina, B., Baud, O., et al. (2014). [Neonatal enterovirus infections reported in France in 2012]. Arch. Pediatr. 21, 984–989. doi:10.1016/j.arcped.2014.06.022
Speer, M. E., and Yawn, D. H. (1984). Fatal hepatoadrenal necrosis in the neonate associated with echovirus types 11 and 12 presenting as a surgical emergency. J. Pediatr. Surg. 19, 591–593. doi:10.1016/s0022-3468(84)80111-6
Stoyanova, A., Nikolova, I., and Galabov, A. S. (2015a). Effect of consecutive alternating administration (CAA) of a triple anti-enteroviral combination on Coxsackievirus B1 neuroinfection in mice. Antivir. Res. 121, 138–144. doi:10.1016/j.antiviral.2015.07.004
Stoyanova, A., Nikolova, I., Purstinger, G., Dobrikov, G., Dimitrov, V., Philipov, S., et al. (2015b). Anti-enteroviral triple combination of viral replication inhibitors: Activity against coxsackievirus B1 neuroinfection in mice. Antivir. Chem. Chemother. 24, 136–147. doi:10.1177/2040206616671571
Su, W. J., Huang, W. T., Cheng, H. Y., and Wei, H. Y. (2018). Severe echovirus 11 infection in newborns, taiwan, 2018. Epidemiol. Bull. 34, 134. doi:10.6525/TEB.201811_34(21).0001
Sundaram, S. S., Alonso, E. M., Narkewicz, M. R., Zhang, S., and Squires, R. H.Pediatric Acute Liver Failure Study Group (2011). Pediatric Acute Liver Failure StudyCharacterization and outcomes of young infants with acute liver failure. J. Pediatr. 159, 813–818. doi:10.1016/j.jpeds.2011.04.016
Taylor, S. A., and Whitington, P. F. (2016). Neonatal acute liver failure. Liver Transpl. 22, 677–685. doi:10.1002/lt.24433
Tebruegge, M., and Curtis, N. (2009). Enterovirus infections in neonates. Semin. Fetal Neonatal Med. 14, 222–227. doi:10.1016/j.siny.2009.02.002
Tian, L., Li, X., Xu, Y., and Li, E. (2012). [Effect and mechanism of Saururus chinensis against herpes dimplex virus]. Zhongguo Zhong Yao Za Zhi 37, 1642–1645.
Vassileva-Pencheva, R., and Galabov, A. S. (2016). Effectiveness of the consecutive alternating administration course of a triple antiviral combination in coxsackievirus B3 infections in mice. Drug Res. 66, 639–643. doi:10.1055/s-0042-112970
Ventura, K. C., Hawkins, H., Smith, M. B., and Walker, D. H. (2001). Fatal neonatal echovirus 6 infection: Autopsy case report and review of the literature. Mod. Pathol. 14, 85–90. doi:10.1038/modpathol.3880260
Verboon-Maciolek, M. A., Swanink, C. M., Krediet, T. G., Van Loon, A. M., Bruning, H. J., Kaan, J. A., et al. (1997). Severe neonatal echovirus 20 infection characterized by hepatic failure. Pediatr. Infect. Dis. J. 16, 524–527. doi:10.1097/00006454-199705000-00016
Vergnano, S., Kadambari, S., Whalley, K., Menson, E. N., Martinez-Alier, N., Cooper, M., et al. (2015). Characteristics and outcomes of human parechovirus infection in infants (2008-2012). Eur. J. Pediatr. 174, 919–924. doi:10.1007/s00431-014-2483-3
Verma, A., Dhawan, A., Zuckerman, M., Hadzic, N., Baker, A. J., and Mieli-Vergani, G. (2006). Neonatal herpes simplex virus infection presenting as acute liver failure: Prevalent role of herpes simplex virus type I. J. Pediatr. Gastroenterol. Nutr. 42, 282–286. doi:10.1097/01.mpg.0000214156.58659.4c
Wait, S., and Chen, D. S. (2012). Towards the eradication of Hepatitis B in Taiwan. Kaohsiung J. Med. Sci. 28, 1–9. doi:10.1016/j.kjms.2011.10.027
Wang, C., Wang, P., Chen, X., Wang, W., and Jin, Y. (2015). Saururus chinensis (Lour.) Baill blocks enterovirus 71 infection by hijacking MEK1-ERK signaling pathway. Antivir. Res. 119, 47–56. doi:10.1016/j.antiviral.2015.04.009
Wang, J., Atchison, R. W., Walpusk, J., and Jaffe, R. (2001). Echovirus hepatic failure in infancy: Report of four cases with speculation on the pathogenesis. Pediatr. Dev. Pathol. 4, 454–460. doi:10.1007/s10024001-0043-0
Wang, S. M., Liu, C. C., Yang, Y. J., Yang, H. B., Lin, C. H., and Wang, J. R. (1998). Fatal coxsackievirus B infection in early infancy characterized by fulminant hepatitis. J. Infect. 37, 270–273. doi:10.1016/s0163-4453(98)92076-x
Wang, T., Chen, S., Wang, X., Huang, Y., Qiu, J., Fei, Y., et al. (2019). Aberrant PD-1 ligand expression contributes to the myocardial inflammatory injury caused by Coxsackievirus B infection. Antivir. Res. 166, 1–10. doi:10.1016/j.antiviral.2019.03.007
Wells, A. I., Grimes, K. A., Kim, K., Branche, E., Bakkenist, C. J., Depas, W. H., et al. (2021). Human FcRn expression and Type I Interferon signaling control Echovirus 11 pathogenesis in mice. PLoS Pathog. 17, e1009252 doi:10.1371/journal.ppat.1009252
Keywords: hepatitis, coxsackievirus B, echovirus, antiviral, anti-inflammatory, neonate
Citation: Wang L-C, Tsai H-P, Chen S-H and Wang S-M (2022) Therapeutics for fulminant hepatitis caused by enteroviruses in neonates. Front. Pharmacol. 13:1014823. doi: 10.3389/fphar.2022.1014823
Received: 09 August 2022; Accepted: 04 October 2022;
Published: 20 October 2022.
Edited by:
Paola Patrignani, University of Studies G. d'Annunzio Chieti and Pescara, ItalyReviewed by:
Angel Galabov, Bulgarian Academy of Sciences, BulgariaAnna Majer, Public Health Agency of Canada (PHAC), Canada
Copyright © 2022 Wang, Tsai, Chen and Wang. This is an open-access article distributed under the terms of the Creative Commons Attribution License (CC BY). The use, distribution or reproduction in other forums is permitted, provided the original author(s) and the copyright owner(s) are credited and that the original publication in this journal is cited, in accordance with accepted academic practice. No use, distribution or reproduction is permitted which does not comply with these terms.
*Correspondence: Shun-Hua Chen, c2h1bmh1YUBuY2t1LmVkdS50dw==; Shih-Min Wang, cGVkd2FuZ0BtYWlsLm5ja3UuZWR1LnR3
†These authors have contributed equally to this work and share first authorship