- 1Department of Nuclear Medicine, Taihe Hospital, Hubei University of Medicine, Shiyan, China
- 2Medical Imaging Center, Taihe Hospital, Hubei University of Medicine, Shiyan, China
- 3Department of Infection Control, Taihe Hospital, Hubei University of Medicine, Shiyan, China
- 4Hubei Clinical Research Center for Precise Diagnosis and Treatment of Liver Cancer, Taihe Hospital, Hubei University of Medicine, Shiyan, China
Background: NPM1 is highly expressed in a variety of solid tumors and promotes tumor development. However, there are few comprehensive studies on NPM1 analysis in gastrointestinal cancer.
Methods: We used bioinformatics tools to study the expression difference of NPM1 between gastrointestinal cancer and control group, and analyzed the relationship between its expression level and the diagnosis, prognosis, functional signaling pathway, immune infiltration, m6A and cuproptosis related genes of gastrointestinal cancer. At the same time, the expression difference of NPM1 between esophageal carcinoma (ESCA) samples and control samples was verified by in vitro experiments.
Results: NPM1 was overexpressed in gastrointestinal cancer. In vitro experiments confirmed that the expression of NPM1 in ESCA samples was higher than that in normal samples. The expression of NPM1 has high accuracy in predicting the outcome of gastrointestinal cancer. The expression of NPM1 is closely related to the prognosis of multiple gastrointestinal cancers. Go and KEGG enrichment analysis showed that NPM1 co-expressed genes involved in a variety of biological functions. NPM1 expression is potentially associated with a variety of immune cell infiltration, m6A and cuproptosis related genes in gastrointestinal cancers.
Conclusion: NPM1 can be used as a diagnostic and prognostic marker of gastrointestinal cancer, which is related to the immune cell infiltration and the regulation of m6A and cuproptosis.
Introduction
About 10 million people die of malignant tumors every year in the world, among which gastrointestinal cancer is a common malignant tumor in the digestive system (Sung et al., 2021). Common gastrointestinal cancers include cholangiocarcinoma (CHOL), colon adenocarcinoma (COAD), esophageal carcinoma (ESCA), liver hepatocellular carcinoma (LIHC), pancreatic adenocarcinoma (PAAD), rectum adenocarcinoma (READ) and stomach adenocarcinoma (STAD). Although significant progress has been made in radical resection, radiotherapy and chemotherapy, the 5-years survival rate of patients with gastric cancer is still very low (Shen et al., 2020; Thrift, 2021). The occurrence and development of gastrointestinal cancer is the result of multiple genes and factors. Therefore, seeking diagnostic markers with high sensitivity and specificity is of great significance to improve the diagnostic level of gastrointestinal cancer, especially to improve the diagnostic rate of early cancer and prolong the life of patients.
In the nucleus, nucleophosmin 1 (NPM1) is a multifunctional protein that shuttles between the nucleus and the cytoplasm (Karimi Dermani et al., 2021). In previous studies, NPM1 has mainly been studied in relation to blood system diseases, and only a few reports have been published regarding solid tumors (Zarka et al., 2020; Dong et al., 2022). There are strong relationships between the expression of NPM1 and glycolysis related genes as well as 18F-FDG metabolism parameters in lung adenocarcinoma (LUAD) in our previous studies (Liu et al., 2021a; Zhou et al., 2021a). However, the study of NPM1 in gastrointestinal cancer, especially in esophageal cancer, has not been reported.
Many studies have shown that the tumor microenvironment (TME) with extensive immune infiltration and N6-methyladenosine (m6A) modification that regulates gene stability play an important role in the occurrence and development of tumors and the diagnosis and treatment of cancer (Chen et al., 2019; Wang et al., 2020a; Chamma et al., 2022). At the same time, a recently discovered copper dependent regulatory cell death (cuproptosis) has become a research hotspot (Cobine and Brady, 2022; Tang et al., 2022; Tsvetkov et al., 2022). NPM1 has been studied relatively little in gastrointestinal cancer despite its multifaceted nature, specifically on its relationship with immunotherapy, m6A modification, and cuproptosis.
We downloaded gastrointestinal cancer data from The Cancer Genome Atlas (TCGA) for this study. The R software package and other online databases were used to analyze the expression and prognostic value of NPM1 in gastrointestinal cancer. NPM1 expression in ESCA samples was compared to that in normal samples using cell experiments and immunohistochemistry (IHC). Furthermore, NPM1’s function and pathway enrichment in gastrointestinal cancer were examined as well as its co-expression network in gastrointestinal cancer. Finally, NPM1 and tumor immunity, m6A methylation modification, and cuproptosis related genes in ESCA were studied to establish a basis for developing new treatment strategies.
Materials and methods
Expression level and prognostic value of nucleophosmin 1 in public database
The expression difference of NPM1 in Pan-cancer was analyzed using UCSC XENA database (https://xenabrowser.net/datapages/) (Vivian et al., 2017). UCSC XENA database contains The Cancer Genome Atlas (TCGA; https://portal.gdc.cancer.gov/) (Tomczak et al., 2015) and Genotype-Tissue Expression (GTEx; http://commonfund.nih.gov/GTEx/) (Battle et al., 2017) data sets, which are mainly used to analyze and visualize gene expression in tumors. We downloaded the cholangiocarcinoma (CHOL), colorectal cancer (CRC), Esophageal carcinoma (ESCA), liver hepatocellular carcinoma (LIHC), pancreatic adenocarcinoma (PAAD) and stomach adenocarcinoma (STAD) data sets from the Gene Expression Omnibus database (GEO; GSE76297, GSE9348, GSE23400, GSE45267, GSE11838 and GSE66229; www.ncbi.nlm.nih.gov/geo) to verify whether NPM1 mRNA expression differs between tumors and controls. A human protein atlas database (HPA; https://www.proteinatlas.org/) immunohistochemistry image of NPM1 was obtained to demonstrate the protein’s expression. The clinical data of CHOL, COAD, ESCA, LIHC, PAAD, READ and STAD data sets were downloaded from TCGA database to study the relationship between the expression level of NPM1 and the prognostic value of patients with gastrointestinal tumors. A ROC curve was drawn to evaluate the diagnostic value of NPM1 in gastrointestinal tumors.
In vitro experiment
A qRT-PCR assay and immunohistochemistry (IHC) staining assay were used to determine whether NPM1 expression differed between tumors and normals. We referred to previous studies for qRT-PCR and IHC experimental steps (Liu et al., 2021b; Liu et al., 2021c). Technical details can be found in the Supplementary Materials.
Co-expression network analysis of nucleophosmin 1 in gastrointestinal cancer
We used the STAT package in R to analyze RNA sequencing data from TCGA patients with gastrointestinal cancer in order to study co-expressed genes associated with NPM1. For statistical analysis, Pearson correlation coefficient was used. In the case of |cor| > 0.3, p < 0.05, we consider the correlation to be significant. Correlation heat map and Veen map are drawn by using ggplot2 software package in R language. There are 30 genes that are positively correlated with NPM1 expression in gastrointestinal cancers, as shown in the correlation heat map. Veen map shows the intersection of genes related to NPM1 expression in gastrointestinal tumors, the threshold is cor >0.3, p < 0.05. In order to screen out the 30 genes most related to the expression of NPM1 in gastrointestinal cancer, we summed the correlation coefficients of each gene and sorted them according to the average correlation coefficient, and finally got the 30 most related genes.
Nucleophosmin 1 co-expression network enrichment analysis
The above intersection of genes related to NPM1 expression were enriched and analyzed, mainly including Gene ontology (GO, http://www.geneontology.org/) and Kyoto Encyclopedia of Genes and Genomes (KEGG, http://www.genome.jp/kegg/). ClusterProfiler package (Yu et al., 2012) of R language was used for enrichment analysis, and ggplot2 package was used for visualization.
The relationship between nucleophosmin 1 and tumor immune infiltrating cells in gastrointestinal cancers
Tumor Immune Estimation Resource (TIMER, https://cistrome.shinyapps.io/timer) is a comprehensive resource for systematical analysis of immune infiltrates across diverse cancer types (Li et al., 2016; Li et al., 2017). In this study, we used three algorithms in timer database, namely TIMER (22), QUANTISEQ (Finotello et al., 2019) and CIBERSORT(Newman et al., 2015), to determine whether NPM1 correlates with immune cells that contribute to gastrointestinal cancer. Next, we used TISIDB database (http://cis.hku.hk/TISIDB/index.php) to further study the expression level of NPM1 in different immune subtypes. There is a database that contains information about tumors and their interactions with the immune system (Ru et al., 2019).
Correlation between nucleophosmin 1 with m6A and cuproptosis related genes in gastrointestinal cancer
In order to further study the possibility that NPM1 may be involved in the regulation of m6A and cuproptosis in gastrointestinal cancer, an analysis of NPM1 expression along with m6A and cuproptosis related genes was conducted using the TCGA database. Based on previous studies (Li et al., 2019; Tsvetkov et al., 2022), we collected 20 m6A related genes and 10 cuproptosis related genes. The relationship between NPM1 with m6A and cuproptosis related gene expression in gastrointestinal cancer was analyzed by R software package. Based on the difference in NPM1 expression levels, we divided the TCGA ESCA cohort into two groups and analyzed the difference of m6A and cuproptosis related gene expression between high and low NPM1 expression groups. At the same time, we also analyzed the expression differences of m6A and cuproptosis related genes between the tumor and normal group in the TCGA ESCA cohort. Finally, according to the correlation of expression level and the difference of group expression, we screened the genes most likely to interact with NPM1. Use ggplot2 software package for data visualization.
Statistical methods
Most statistical analysis is done through the above bioinformatics tools. This includes Xiantao platform (www.xiantao.love). Xiantao platform is a database integrating TCGA tumor chip data, which contains R software and its appropriate R software package. It is mainly used for gene expression analysis, correlation analysis, enrichment analysis, interactive network analysis, clinical significance analysis, and related mapping. The value of p < 0.05 was considered statistically significant.
Results
Multiple databases verified that nucleophosmin 1 was highly expressed in gastrointestinal cancer
Based on analysis of the UCSC XENA database, NPM1 expression was higher in ACC (adrenocortical carcinoma), BRCA (breast invasive carcinoma), CHOL, COAD, DLBC (lymphoid neoplasm diffuse large b-cell lymphoma), ESCA, GBM (glioblastoma multiforme), HNSC (head and neck squamous cell carcinoma), KIRC (kidney renal clear cell carcinoma), KIRP (kidney renal papillary cell carcinoma), LGG (brain lower grade glioma), LIHC, LUAD (lung adenocarcinoma), LUSC (lung squamous cell carcinoma), PAAD, PRAD (prostate adenocarcinoma), READ, SKCM (skin cutaneous melanoma), STAD, TGCT (testicular germ cell tumors), THCA (thyroid carcinoma), THYM (thymoma) and UCS (uterine carcinosarcoma) than in controls, but lower in LAML (acute myeloid leukemia) and OV (ovarian serous cystadenocarcinoma) (Figure 1A). GEO database analysis further confirmed that the expression of NPM1 in gastrointestinal cancers were higher than that in normal control group (Figures 1B–G). A HPA database IHC staining revealed a significant increase in NPM1 expression in STAD, LIHC, PAAD, and CRC tissues (Figure 1H).
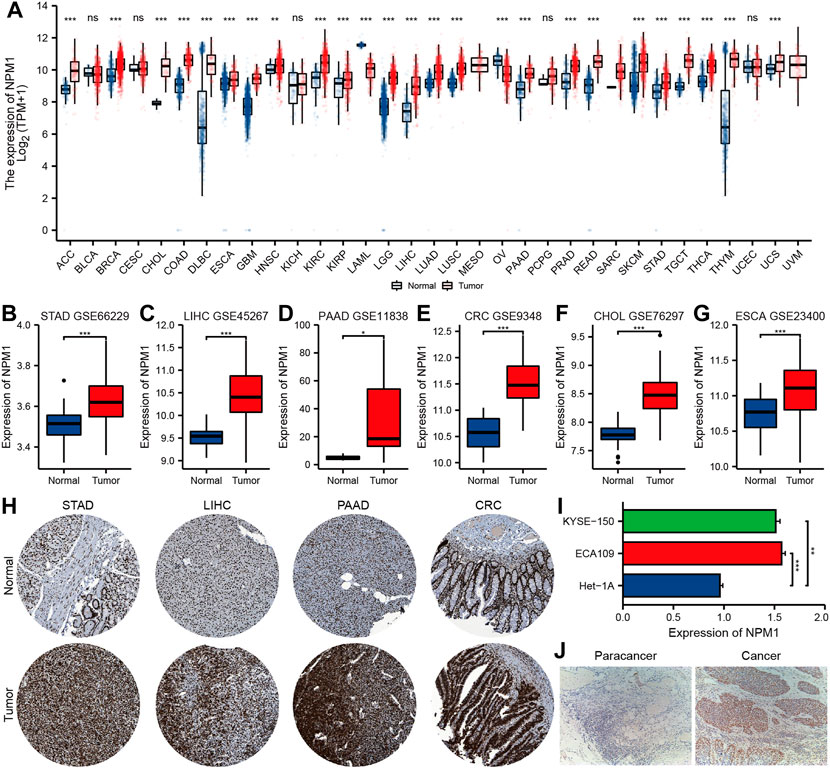
FIGURE 1. Multiple databases verified that NPM1 was highly expressed in gastrointestinal cancer. (A) The analysis of UCSC XENA database showed that NPM1 was highly expressed in a variety of tumor tissues. (B–G) According to the GEO database, compared to controls, gastrointestinal cancers expressed more NPM1. (H) There was a difference in NPM1 expression between tumor samples and normal samples in the HPA database. (I) Cell experiments showed that NPM1 expression was significantly higher in ESCA cells compared to normal esophageal cells. (J) According to IHC results, tumor tissues from ESCA patients expressed significantly more NPM1 than paracancer tissues. *p < 0.05; **p < 0.01; ***p < 0.001; ns, no significance.
In addition, qRT-PCR showed that the expression level of NPM1 mRNA in ESCA cell line was significantly higher than that in normal esophageal tissue cells (Figure 1I). A significant difference was observed between tumor tissues of ESCA patients and those of paracancer patients (Figure 1J).
Prognostic and diagnostic value of nucleophosmin 1 in gastrointestinal cancers
In order to further explore the relationship between NPM1 expression and gastrointestinal cancer, we analyzed the relationship between NPM1 expression and pathological characteristics of cancer patients. The results showed that patients with high expression of NPM1 were associated with worse OS in LIHC [HR: 1.977 (1.387–2.817), p < 0.001] and PAAD [HR: 1.746 (1.150–2.651), p = 0.009]. In ESCA [HR: 1.641 (1.044–2.581), p = 0.032], LIHC [HR: 1.539 (1.150–2.060), p = 0.004] and PAAD [1.494 (1.014–2.203), p = 0.043], PFI was lower in patients with high expression of NPM1. At the same time, we also found that NPM1 had high accuracy in diagnosing ESCA, STAD, LIHC, CHOL, PAAD, COAD, READ, and CRC patients and normal controls (Figure 2, AUC > 0.75).
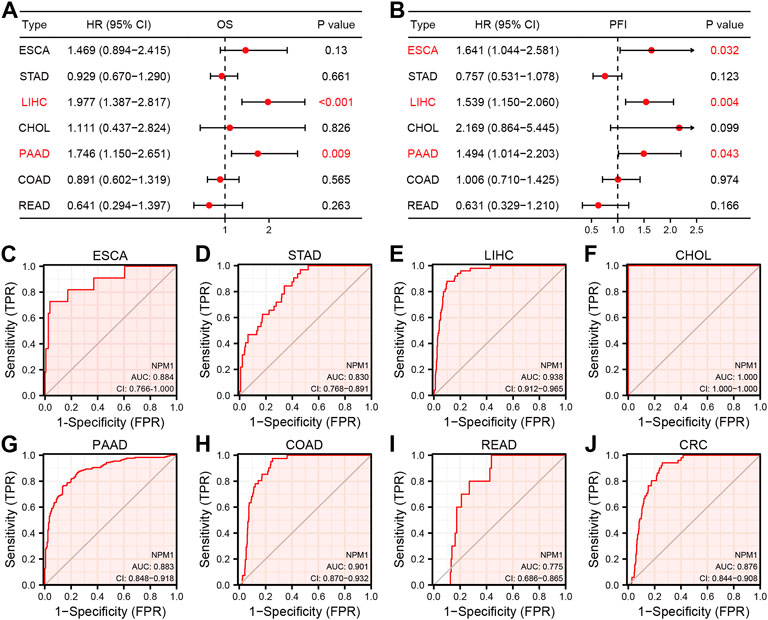
FIGURE 2. Prognostic and diagnostic value of NPM1 in gastrointestinal cancers. (A–B) Forest map shows the relationship between NPM1 expression level with OS and PFI, respectively. (C–J) ROC curve shows the value of NPM1 in the diagnosis of patients with gastrointestinal cancer, including ESCA, STAD, LIHC, CHOL, PAAD, COAD, READ and CRC.
Co-expression network analysis of nucleophosmin 1 in gastrointestinal cancer
We analyzed RNA sequencing data from the TCGA database of gastrointestinal cancer with R software, and only retained gene encoding proteins from the gene sequences. The analysis found that 1958 genes expression in ESCA were associated with NPM1, 3,302 genes expression in STAD were associated with NPM1, 6,438 genes expression in LIHC were associated with NPM1, 2,628 genes expression in CHOL were associated with NPM1, 7,721 genes expression in PAAD were associated with NPM1, 4,523 genes expression in COAD were associated with NPM1, and 5,602 genes expression in READ were associated with NPM1. |cor| > 0.3 and p < 0.05 were the thresholds. The top 30 genes in ESCA, STAD, LIHC, CHOL, PAAD, COAD, and READ that are positively linked with NPM1 expression are displayed in a heat map (Figures 3A–G). Veen map shows the intersection of these gene sets, and 385 genes are found to coincide (Figure 3H). Figure 3I shows the 30 co-expressed genes sorted according to the average correlation coefficient. The top three genes in the average correlation coefficient were RARS1 (Arginyl-TRNA Synthetase 1, average cor = 0.763), BTF3 (Basic Transcription Factor 3, average cor = 0.725) and HSPA4 (Heat Shock Protein Family A (Hsp70) Member 4, average cor = 0.716), respectively.
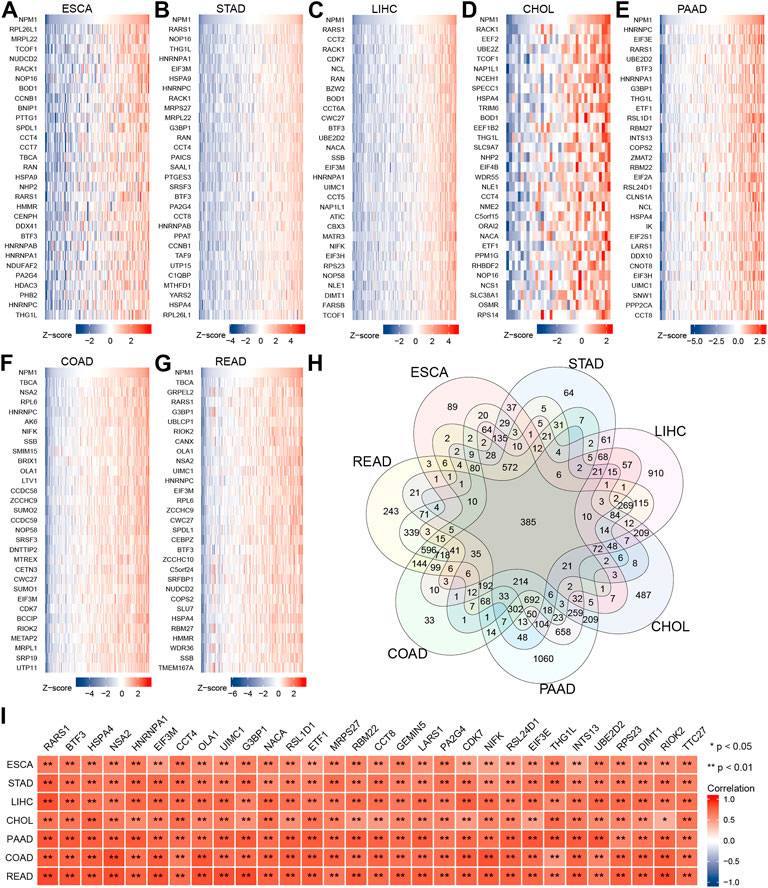
FIGURE 3. Co-expression network analysis of NPM1 in gastrointestinal cancer. The top 30 genes in ESCA (A), STAD (B), LIHC (C), CHOL (D), PAAD (E), COAD (F) and READ (G) that are positively linked with NPM1 expression are displayed in a heat map. (H) Veen map shows the intersection of these gene sets. (I) The related heat map shows the 30 co-expressed genes sorted according to the average correlation coefficient. *p < 0.05; **p < 0.01.
Nucleophosmin 1 co-expression network enrichment analysis
ClusterProfiler package in R was used to analyze the above intersection of genes related to NPM1 expression using GO terms and KEGG pathways. Based on p.adj <0.05 and q value <0.2, a total of 321 biological processes, 101 cellular components, 48 molecular functions, and 11 KEGG annotations were associated with NPM1 co-expressed genes. GO term notes indicate that these genes are mainly involved in ribosome biogenesis, cytosolic part and catalytic activity, acting on RNA. KEGG pathway studies showed that these genes were significantly associated with Ribosome, RNA transport and Spliceosome. The above results were shown by bubble plots (Figures 4A–D).
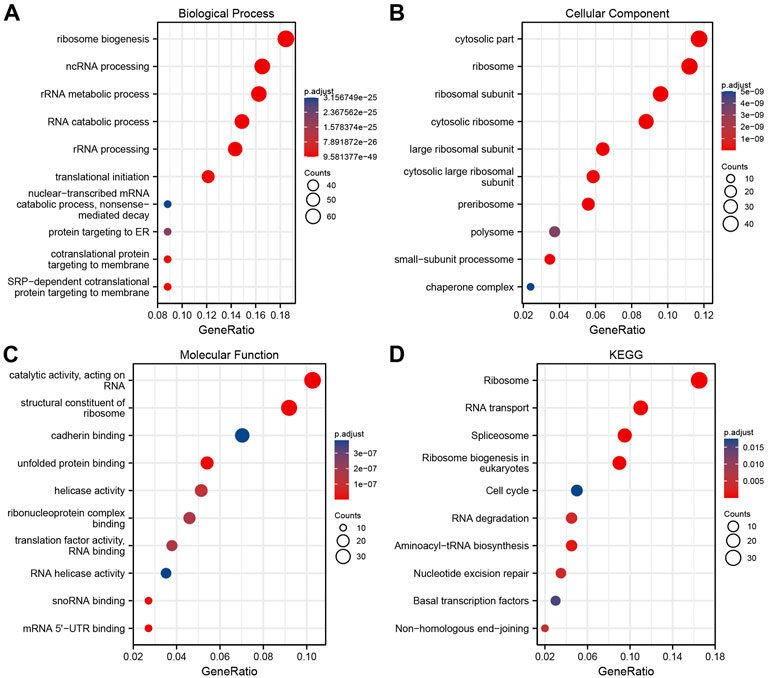
FIGURE 4. NPM1 co-expression network enrichment Analysis. (A–C) An enrichment analysis of GO terms for co-expression genes with NPM1. (D) An enrichment analysis of KEGG terms for NPM1 co-expression genes.
The relationship between nucleophosmin 1 and tumor immune infiltrating cells in gastrointestinal cancers
A TIMER analysis revealed a negative correlation between NPM1 and the expression of five immune cells in COAD (CD8+ T-cells, CD4+ T-cells, macrophages, neutrophils, and dendritic cells). LIHC results showed a positive correlation between NPM1 expression and the expression of five immune cells (CD4+ T-cells, B cells, macrophages, neutrophils, and dendritic cells). Positive correlations were observed between NPM1 and five immune cells (CD8+ T-cells, B cells, macrophages, neutrophils and dendritic cells), while negative correlations were observed between NPM1 and CD4+ T-cells in PAAD (Figure 5A). QUANTISEQ analysis showed that NPM1 was negatively correlated with the expression of six immune cells (B cells, Regulatory CD4+T-cells, Macrophages M2, Neutrophils, Monocytes and Dendritic cells) in COAD. In LIHC, NPM1 was positively correlated with the expression of 8 immune cells (B cells, CD8+T-cells, Non-regulatory CD4+T-cells, Regulatory CD4+T-cells, Macrophages M1, Macrophages M2, Monocytes and Dendritic cells), and negatively correlated with the expression of Neutrophils, which was similar to the result of TIMER analysis (Figure 5B). However, CIBERSORT analysis showed that NPM1 was associated with the expression of a variety of different immune cells in gastrointestinal cancer (Figure 5C). However, TISIDB analysis showed that NPM1 expression was significantly enriched in five immune subtypes, namely wound healing, IFN-gamma dominant, inflammatory, lymphocyte depleted, and TGF-β dominant, in COAD, LIHC, READ, and STAD, but not in CHOL, ESCA, and PAAD (Figure 5D).
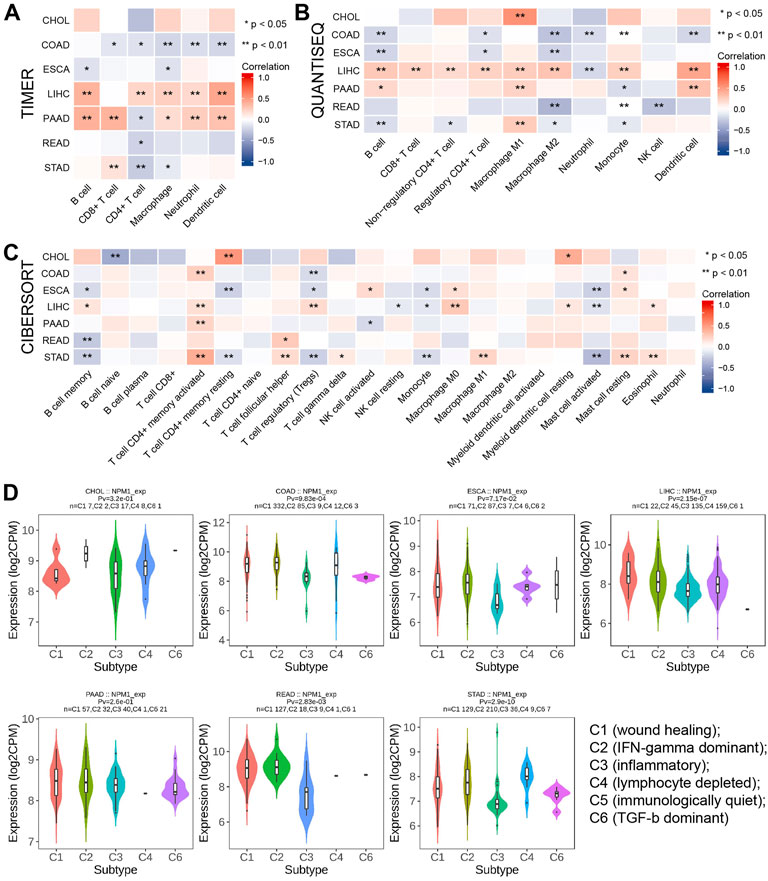
FIGURE 5. The relationship between NPM1 and tumor immune infiltrating cells in gastrointestinal cancers. (A–C) The correlation between NPM1 expression and immune cell infiltration was analyzed by TIMER, QUANTISEQ and CIBERSORT algorithm. (D) TISIDB analysis showed the association between NPM1 expression level and 5 immune subtypes.
Correlation between nucleophosmin 1 with m6A related genes in gastrointestinal cancer
A study conducted using the TCGA database explored the relationship between NPM1 and 20 m6A related genes expressed in gastrointestinal cancers in the CHOL, COAD, ESCA, LIHC, PAAD, READ, and STAD cohorts. In the CHOL cohort, NPM1 was not correlated with most m6A related genes as shown in Figure 6A. However, in the COAD, ESCA, LIHC, PAAD, READ, and STAD cohort, NPM1 was associated with the expression of most m6A related genes. ESCA cohorts were grouped based on expression levels of NPM1. A high NPM1 expression group had higher ALKBH5, HNRNPA2B1, HNRNPC, IGF2BP1, IGF2BP2, IGF2BP3, METTL3, RBM15, RBMX, VIRMA, WTAP, YTHDF1, and YTHDF2 expression than a low NPM1 expression group (Figure 6B). According to our analysis of differentially expressed m6A related genes among tumors and normals, ALKBH5, FTO, HNRNPA2B1, HNRNPC, IGF2BP1, IGF2BP2, IGF2BP3, METTL3, RBM15, RBMX, VIRMA, WTAP, YTHDC1, YTHDF1, YTHDF2, and YTHDF3 were expressed higher in tumors (Figure 6C). Finally, we screened 12 key genes (ALKBH5, HNRNPA2B1, HNRNPC, IGF2BP1, IGF2BP2, METTL3, RBM15, RBMX, VIRMA, WTAP, YTHDF1, and YTHDF2) according to the expression correlation and group expression differences (Figure 6D).
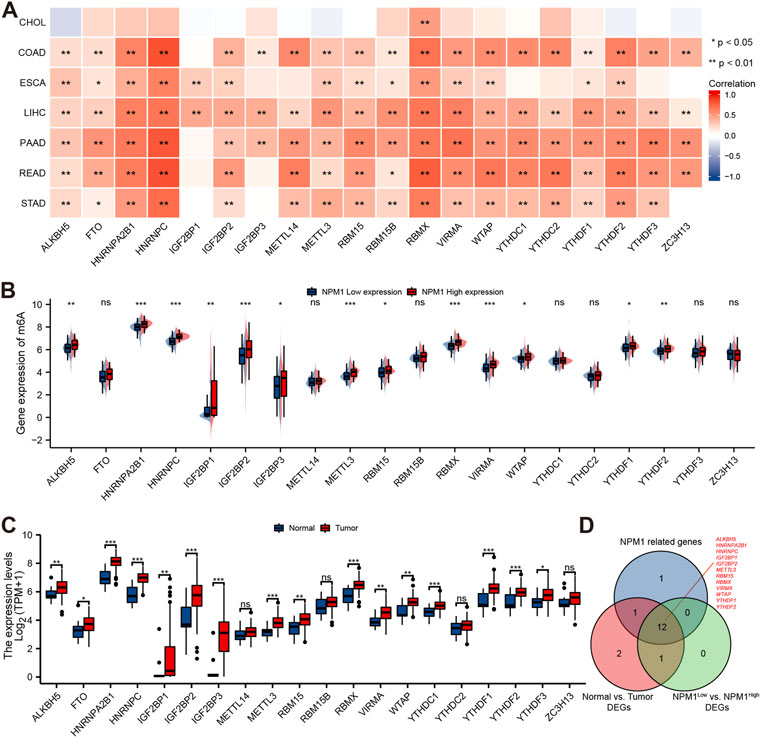
FIGURE 6. Correlation between NPM1 with m6A related genes in gastrointestinal cancer. (A) Correlation between the expression levels of NPM1 and m6A related genes in gastrointestinal cancer. (B) Differences in m6A related gene expression in ESCA cohorts based on low and high NPM1 expression. (C) ESCA cohort m6A related gene expression differs between tumor and normal groups. (D) Veen map shows overlapping genes.
We further verified the correlation between NPM1 and m6A related gene expression by analyzing the IHC score data of NPM1 and YTHDF1. Results as shown in Figure 7, the IHC score of NPM1 was positively correlated with YTHDF1 in ESCA samples (r = 0.403, p = 0.009).
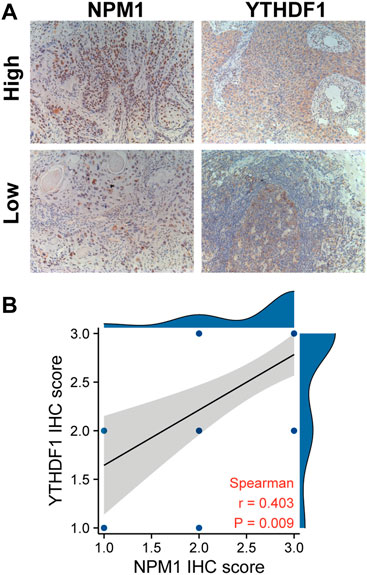
FIGURE 7. NPM1 was positively correlated with YTHDF1 IHC score in ESCA. (A) IHC picture of NPM1 and YTHDF1 in ESCA. (B) NPM1 was positively correlated with YTHDF1 IHC score.
Correlation between nucleophosmin 1 with cuproptosis related genes in gastrointestinal cancer
A study conducted using the TCGA database explored the relationship between NPM1 and 10 cuproptosis related genes expressed in gastrointestinal cancers in the CHOL, COAD, ESCA, LIHC, PAAD, READ, and STAD cohorts. In the CHOL cohort, NPM1 was not correlated with most cuproptosis related genes as shown in Figure 8A. However, in the COAD, ESCA, LIHC, PAAD, READ, and STAD cohort, NPM1 was associated with the expression of LIAS, DLAT, DLD, LIPT1, PDHA1, and PDHB. In the ESCA cohort, we grouped according to the expression of NPM1. LIAS, DLAT, DLD, LIPT1, and PDHB expression was higher in the high NPM1 expression group than in the low NPM1 expression group (Figure 8B). According to our analysis of differentially expressed cuproptosis related genes among tumors and normals, DLAT, GLS, and LIPT1 were expressed higher in tumors (Figure 8C). Finally, we screened 2 key genes (DLAT and LIPT1) according to the expression correlation and group expression differences (Figure 8D).
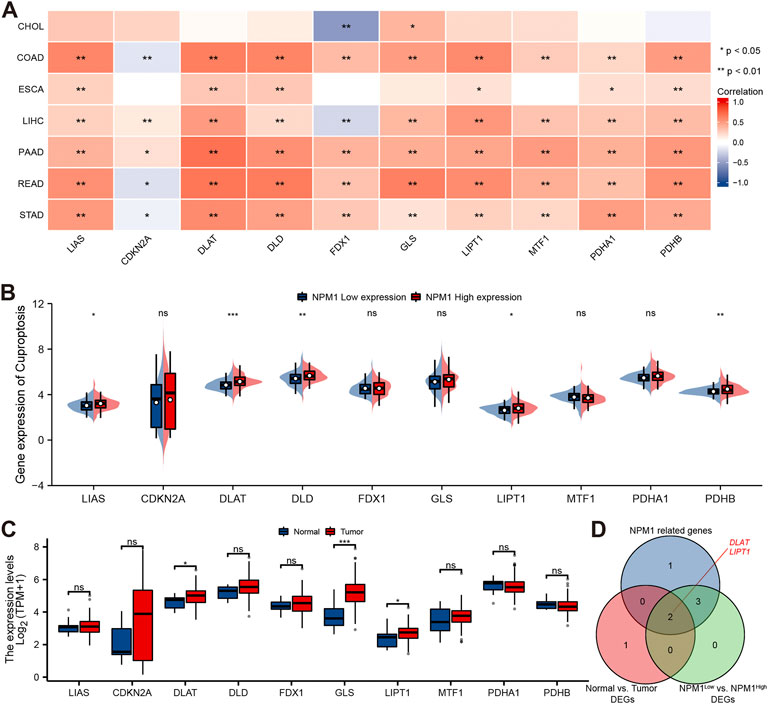
FIGURE 8. Correlation between NPM1 with cuproptosis related genes in gastrointestinal cancer. (A) Correlation between the expression levels of NPM1 and cuproptosis related genes in gastrointestinal cancer. (B) Differences in cuproptosis related gene expression in ESCA cohorts based on low and high NPM1 expression. (C) ESCA cohort cuproptosis related gene expression differs between tumor and normal groups. (D) Veen map shows overlapping genes.
Discussion
Currently, NPM1 research is focused more on cancer than blood diseases. Zhou et al. found that NPM1 was overexpressed in LIHC cells, and high NPM1 expression was significantly associated with decreased survival and increased recurrence in LIHC patients (Zhou et al., 2017). Liu et al. (2012) found that the overexpression of NPM1 in COAD cells enhanced cell migration and invasion, and improved cell proliferation. Although it has been confirmed that overexpression of NPM1 in a variety of gastrointestinal cancers can promote the proliferation and migration of tumor cells, no comprehensive analysis of NPM1 in gastrointestinal cancers has been reported.
In this study, we found that NPM1 was overexpressed in gastrointestinal cancer through the analysis of UCSC XENA database and GEO database, which was consistent with some previous research results (Liu et al., 2012; Zhu et al., 2015; Matsui et al., 2022). At the same time, At the same time, we verified the overexpression of NPM1 in ESCA by cell experiments and IHC. We also found that the expression level of NPM1 is highly accurate in the diagnosis of gastrointestinal cancer (AUC >0.75), and the overexpression of NPM1 is related to the low survival rate of some patients with gastrointestinal cancer, indicating that the clinical correlation between NPM1 and gastrointestinal cancer is significant.
In further functional exploration, we found that 385 genes were positively correlated with NPM1 expression in 7 gastrointestinal cancers. According to the sum of the correlations of these 385 genes in gastrointestinal cancer, we screened the 30 genes with the strongest correlations, which are RARS1, BTF3, HSPA4, NSA2, HNRNPA1, EIF3M, CCT4, OLA1, UIMC1, G3BP1, NACA, RSL1D1, ETF1, MRPS27, RBM22, CCT8, GEMIN5, LARS1, PA2G4, CDK7, NIFK, RSL24D1, EIF3E, THG1L, INTS13, UBE2D2, RPS23, DIMT1, RIOK2, and TTC27 respectively. Previous studies have reported that overexpression of BTF3, CCT8, CDK7, ELF3M, G3BP1, HSPA4, OLA1, and RSL1D1 can contribute to the occurrence and development of CRC (Goh et al., 2011; Zhou et al., 2019; Zhou et al., 2021b; Liao et al., 2021; Zhang et al., 2021; Liu et al., 2022a; Liu et al., 2022b; Li et al., 2022), overexpression of CCT4 and PA2G4 can contribute to the occurrence and development of LIHC(Li et al., 2021a; Sun et al., 2022), overexpression of DIMT1 and HNRNPA1 can contribute to the occurrence and development of STAD (Liu et al., 2017; Zhu et al., 2022), and overexpression of EIF3E and UIMC1 can contribute to the ESCA occurrence and development (Xu et al., 2018; Yang et al., 2018). Therefore, we believe that these genes have the potential to be called diagnostic and therapeutic targets of gastrointestinal cancer. However, studies on the interaction between NPM1 and these genes in gastrointestinal cancer have not been reported. We will continue to pay attention to this interesting phenomenon in the follow-up research. In further enrichment analysis, we found that the co expression of NPM1 was mainly related to Ribosome Biogenesis, Cytosolic Part and Catalytic Activity, Acting On RNA. According to KEGG pathway analysis, NPM1 co-expression is primarily associated with Ribosomes. It has been found that the above biological functions and pathways play an important role in the occurrence and development of tumors, according to some scholars (Ruggero and Pandolfi, 2003; Ding et al., 2022). These findings suggest that NPM1’s co-expression network plays a critical role in the formation and progression of tumors.
The components of TME are complex, in which immune regulation and immune escape are important components. More and more studies have shown that TME plays a key role in tumor progression (Wang et al., 2020b; Chamma et al., 2022; Malla et al., 2022). In this study, we found that the overexpression of NPM1 was negatively correlated with most immune infiltrating cells in COAD and ESCA, but positively correlated with immune infiltrating cells in LIHC and PAAD. It was also found that NPM1 expression was significantly enriched in five immune subtypes in COAD, LIHC, READ and STAD. Helmink et al., 2020 Found that B cells can secrete a series of cytokines, which may potentially promote the anti-tumor response by producing antibodies to tumors. The lack of B cell infiltration will affect the inhibitory effect of the immune system on tumor cells (49). Denardo and Ruffell (2019) Found that in the initial stage of tumor development, macrophages can directly promote anti-tumor response by killing tumor cells. Additionally, there was a negative correlation between NPM1 expression and macrophage and B cell infiltration in ESCA. We believe that patients with gastrointestinal cancer express high levels of NPM1 which may result in immune escape and anti-tumor immunity, suggesting that NPM1 may be important in regulating the immune response to gastrointestinal cancers.
M6A modification is a reversible dynamic RNA epigenetic process, which is regulated by m6A regulatory factors and is crucial in the development of cancer (Chen et al., 2019; Li et al., 2019; Wang et al., 2020a). In gastrointestinal cancers, however, there has been no research on NPM1 and m6A related genes. In this study, we found that most gastrointestinal cancers expressed NPM1 positively correlated with m6A related genes. Especially in the ESCA cohort, we screened 12 key genes, ALKBH5, HNRNPA2B1, HNRNPC, IGF2BP1, IGF2BP2, METTL3, RBM15, RBMX, VIRMA, WTAP, YTHDF1, and YTHDF2, according to the expression correlation and group expression differences. It has been reported that the overexpression of ALKBH5(Nagaki et al., 2020), HNRNPA2B1 (Li et al., 2021b), HNRNPC(Zhang et al., 2019), IGF2BP1(Fang et al., 2021), IGF2BP2(Huang et al., 2021), METTL3 (Liu et al., 2020), WTAP (Zhu et al., 2021) and YTHDF1 (Liu et al., 2022c) can contribute to the occurrence and development of ESCA through different regulatory methods. At the same time, the correlation analysis of IHC score also confirmed that the expression of NPM1 was positively correlated with YTHDF1. Therefore, it is believed that the cancer-promoting activity of NPM1 gene is related to the expression of genes related to m6A. It is possible that NPM1 may be able to influence the methylation level of ESCA by affecting m6A, and ultimately affect the development of cancer.
Copper is the basic element to maintain human life activities, and plays an essential role as a cofactor of essential enzymes. Compared with normal cells, cancer cells have a higher demand for copper (Cobine and Brady, 2022; Tang et al., 2022; Tsvetkov et al., 2022). However, the relationship between NPM1 and cuproptosis related genes in gastrointestinal tumors has not been studied. A significant correlation was found in this study between the expression level of NPM1 and the expression level of cuproptosis related genes in most gastrointestinal cancers. In the ESCA cohort, we screened two key genes, DLAT and LIPT1, based on expression correlation and group expression differences. Goh et al. Found that DLAT is highly expressed in STAD, and interfering with the expression of DLAT can inhibit the proliferation of STAD cells (Goh et al., 2015). Lv et al. Found that LIPT1 expression increased in skin cutaneous melanoma biopsy and was an independent prognostic indicator of skin cutaneous melanoma patients (Lv et al., 2022). Accordingly, we believe that the cancer-promoting effect of NPM1 gene is associated with cuproptosis related genes expression. The NPM1 may adversely affect ESCA cells’ cuproptosis process by altering copper ion levels, which ultimately affects cancer development.
In conclusion, this is the first comprehensive study to examine NPM1 expression in relation to m6A, cuproptosis and tumor cell immune infiltration in gastrointestinal cancer. This study shows that NPM1 is highly expressed in gastrointestinal cancers, and its expression level can accurately diagnose cancer, and is a prognostic indicator of ESCA, LIHC, and PAAD. The expression of NPM1 is negatively correlated with B cell and macrophages infiltration, which may affect the tumor immunity of ESCA by affecting B cell and macrophages infiltration. NPM1 is closely related to 12 m6A related genes, which may affect the tumor progression of ESCA by affecting the methylation level of m6A. However, NPM1 is also positively correlated with the expression of two cuproptosis related genes, which may affect the regulation of cuproptosis in tumor cells by affecting the expression of these genes. The potential biological function of NPM1 in ESCA is shown in Figure 9. Of course, further exploration is needed to confirm the function and potential mechanism of NPM1 in the occurrence and development of gastrointestinal cancer.
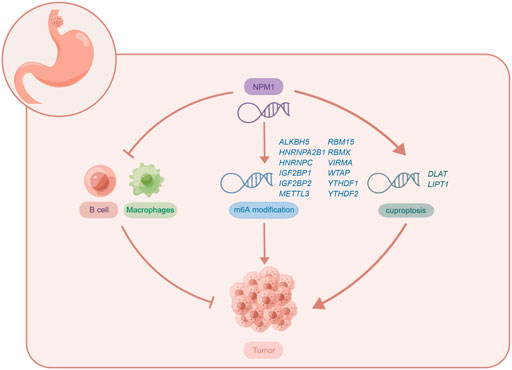
FIGURE 9. The potential biological function of NPM1 in ESCA. Figures were created by Figdraw (www.figdraw.com).
Data availability statement
The datasets presented in this study can be found in online repositories. The names of the repository/repositories and accession number(s) can be found in the article/Supplementary Material.
Ethics statement
The studies involving human participants were reviewed and approved by Ethics Committee of Taihe Hospital Affiliated with Hubei University of Medicine. Written informed consent for participation was not required for this study in accordance with the national legislation and the institutional requirements.
Author contributions
X-SL conceived the project and wrote the manuscript. X-SL, CL, and JZ participated in data analysis. X-SL, D-BZ, Y-JC, FT, YG, X-YL, YZ, and Y-HZ participated in discussion and language editing. Z-JP reviewed the manuscript. All authors contributed to the article and approved the submitted version.
Funding
This work was supported by the Hubei province’s Outstanding Medical Academic Leader program, the Foundation for Innovative Research Team of Hubei Provincial Department of Education T2020025, the general project of Hubei Provincial Department of Education (No. B2021160), Innovative Research Program for Graduates of Hubei University of Medicine (grant nos. YC2022042, YC2022037), Shiyan Taihe Hospital hospital-level project (2021JJXM001, 2021JJXM006) and the Key Discipline Project of Hubei University of Medicine.
Conflict of interest
The authors declare that the research was conducted in the absence of any commercial or financial relationships that could be construed as a potential conflict of interest.
Publisher’s note
All claims expressed in this article are solely those of the authors and do not necessarily represent those of their affiliated organizations, or those of the publisher, the editors and the reviewers. Any product that may be evaluated in this article, or claim that may be made by its manufacturer, is not guaranteed or endorsed by the publisher.
Supplementary material
The Supplementary Material for this article can be found online at: https://www.frontiersin.org/articles/10.3389/fphar.2022.1010879/full#supplementary-material
References
Battle, A., Brown, C. D., Engelhardt, B. E., and Montgomery, S. B. (2017). Genetic effects on gene expression across human tissues. Nature 550 (7675), 204–213. doi:10.1038/nature24277
Chamma, H., Vila, I. K., Taffoni, C., Turtoi, A., and Laguette, N. (2022). Activation of sting in the pancreatic tumor microenvironment: A novel therapeutic opportunity. Cancer Lett. 538, 215694. doi:10.1016/j.canlet.2022.215694
Chen, X., Zhang, J., and Zhu, J. (2019). The role of m(6)A RNA methylation in human cancer. Mol. Cancer. 18 (1), 103. doi:10.1186/s12943-019-1033-z
Cobine, P. A., and Brady, D. C. (2022). Cuproptosis: Cellular and molecular mechanisms underlying copper-induced cell death. Mol. Cell. 82 (10), 1786–1787. doi:10.1016/j.molcel.2022.05.001
Denardo, D. G., and Ruffell, B. (2019). Macrophages as regulators of tumour immunity and immunotherapy. Nat. Rev. Immunol. 19 (6), 369–382. doi:10.1038/s41577-019-0127-6
Ding, G., Zhu, C., Wang, Q., Cao, H., Li, B., Yang, P., et al. (2022). Molecularly engineered tumor acidity-responsive plant toxin gelonin for safe and efficient cancer therapy. Bioact. Mat. 18, 42–55. doi:10.1016/j.bioactmat.2022.02.001
Dong, H., Ham, J. D., Hu, G., Xie, G., Vergara, J., Liang, Y., et al. (2022). Memory-like NK cells armed with a neoepitope-specific CAR exhibit potent activity against NPM1 mutated acute myeloid leukemia. Proc. Natl. Acad. Sci. U. S. A. 119 (25), e2122379119. doi:10.1073/pnas.2122379119
Fang, X., Sun, J., Chen, S., Wu, K., Yu, Y., Zhang, C., et al. (2021). IGF2BP1/UHRF2 Axis mediated by miR-98-5p to promote the proliferation of and inhibit the apoptosis of esophageal squamous cell carcinoma. Ann. Clin. Lab. Sci. 51 (3), 329–338.
Finotello, F., Mayer, C., Plattner, C., Laschober, G., Rieder, D., Hackl, H., et al. (2019). Molecular and pharmacological modulators of the tumor immune contexture revealed by deconvolution of RNA-seq data. Genome Med. 11 (1), 34. doi:10.1186/s13073-019-0638-6
Goh, S., Hong, S., Hong, S., Lee, B., Ju, M., Jeong, J., et al. (2011). eIF3m expression influences the regulation of tumorigenesis-related genes in human colon cancer. Oncogene 30 (4), 398–409. doi:10.1038/onc.2010.422
Goh, W. Q. J., Ow, G. S., Kuznetsov, V. A., Chong, S., and Lim, Y. P. (2015). DLAT subunit of the pyruvate dehydrogenase complex is upregulated in gastric cancer-implications in cancer therapy. Am. J. Transl. Res. 7 (6), 1140–1151.
Helmink, B. A., Reddy, S. M., Gao, J., Zhang, S., Basar, R., Thakur, R., et al. (2020). B cells and tertiary lymphoid structures promote immunotherapy response. Nature 577 (7791), 549–555. doi:10.1038/s41586-019-1922-8
Huang, G., Chen, Q., Ma, C., Xie, L., and Gu, J. (2021). linc01305 promotes metastasis and proliferation of esophageal squamous cell carcinoma through interacting with IGF2BP2 and IGF2BP3 to stabilize HTR3A mRNA. Int. J. Biochem. Cell Biol. 136, 106015. doi:10.1016/j.biocel.2021.106015
Karimi Dermani, F., Gholamzadeh Khoei, S., Afshar, S., and Amini, R. (2021). The potential role of nucleophosmin (NPM1) in the development of cancer. J. Cell. Physiol. 236, 7832–7852. doi:10.1002/jcp.30406
Li, B., Severson, E., Pignon, J. C., Zhao, H., Li, T., Novak, J., et al. (2016). Comprehensive analyses of tumor immunity: Implications for cancer immunotherapy. Genome Biol. 17 (1), 174. doi:10.1186/s13059-016-1028-7
Li, C., Pan, B., Wang, X., Liu, X., Qin, J., Gao, T., et al. (2022). Upregulated LINC01088 facilitates malignant phenotypes and immune escape of colorectal cancer by regulating microRNAs/G3BP1/PD-L1 axis. J. Cancer Res. Clin. Oncol. 148, 1965–1982. doi:10.1007/s00432-022-03981-8
Li, F., Liu, C., Wu, P., Ling, A., Pan, Q., and Li, X. (2021a). CCT4 suppression inhibits tumor growth in hepatocellular carcinoma by interacting with Cdc20. Chin. Med. J.-Peking. 134 (22), 2721–2729. doi:10.1097/CM9.0000000000001851
Li, K., Chen, J., Lou, X., Li, Y., Qian, B., Xu, D., et al. (2021b). HNRNPA2B1 affects the prognosis of esophageal cancer by regulating the miR-17-92 cluster. Front. Cell Dev. Biol. 9, 658642. doi:10.3389/fcell.2021.658642
Li, T., Fan, J., Wang, B., Traugh, N., Chen, Q., Liu, J. S., et al. (2017). Timer: A web server for comprehensive analysis of tumor-infiltrating ImmuneCells. Cancer Res. 77 (21), e108–e110. doi:10.1158/0008-5472.CAN-17-0307
Li, Y., Xiao, J., Bai, J., Tian, Y., Qu, Y., Chen, X., et al. (2019). Molecular characterization and clinical relevance of m(6)A regulators across 33 cancer types. Mol. Cancer. 18 (1), 137. doi:10.1186/s12943-019-1066-3
Liao, Q., Ren, Y., Yang, Y., Zhu, X., Zhi, Y., Zhang, Y., et al. (2021). CCT8 recovers WTp53-suppressed cell cycle evolution and EMT to promote colorectal cancer progression. Oncogenesis 10 (12), 84. doi:10.1038/s41389-021-00374-3
Liu, G., Peng, X., Cai, Y., Cheng, A., Zha, L., and Wang, Z. (2017). DIMT1 overexpression correlates with progression and prognosis in gastric carcinoma. Hum. Pathol. 70, 35–42. doi:10.1016/j.humpath.2017.02.034
Liu, X., Chen, J., Long, X., Lan, J., Liu, X., Zhou, M., et al. (2022a). RSL1D1 promotes the progression of colorectal cancer through RAN-mediated autophagy suppression. Cell Death Dis. 13 (1), 43. doi:10.1038/s41419-021-04492-z
Liu, X., Gao, Y., Liu, C., Chen, X., Zhou, L., Yang, J., et al. (2021a). Comprehensive analysis of prognostic and immune infiltrates for E2F transcription factors in human pancreatic adenocarcinoma. Front. Oncol. 10, 606735. doi:10.3389/fonc.2020.606735
Liu, X., Gao, Y., Wu, L., Wan, H., Yan, P., Jin, Y., et al. (2021b). Comprehensive analysis of GLUT1 immune infiltrates and ceRNA network in human esophageal carcinoma. Front. Oncol. 11, 665388. doi:10.3389/fonc.2021.665388
Liu, X., Kui, X., Gao, Y., Chen, X., Zeng, J., Liu, X., et al. (2022b). Comprehensive analysis of YTHDF1 immune infiltrates and ceRNA in human esophageal carcinoma. Front. Genet. 13, 835265. doi:10.3389/fgene.2022.835265
Liu, X., Yuan, L., Gao, Y., Zhou, L., Yang, J., and Pei, Z. (2020). Overexpression of METTL3 associated with the metabolic status on 18F-FDG PET/CT in patients with Esophageal Carcinoma. J. Cancer. 11 (16), 4851–4860. doi:10.7150/jca.44754
Liu, X., Zhou, L., Yuan, L., Gao, Y., Kui, X., Liu, X., et al. (2021c). NPM1 is a prognostic biomarker involved in immune infiltration of lung adenocarcinoma and associated with m6A modification and glycolysis. Front. Immunol. 12, 724741. doi:10.3389/fimmu.2021.724741
Liu, Y., Kong, X., He, J., Xu, Y., Zhang, J., Zou, L., et al. (2022c). OLA1 promotes colorectal cancer tumorigenesis by activation of HIF1α/CA9 axis. BMC Cancer 22 (1), 424. doi:10.1186/s12885-022-09508-1
Liu, Y., Zhang, F., Zhang, X., Qi, L., Yang, L., Guo, H., et al. (2012). Expression of nucleophosmin/NPM1 correlates with migration and invasiveness of colon cancer cells. J. Biomed. Sci. 19 (1), 53. doi:10.1186/1423-0127-19-53
Lv, H., Liu, X., Zeng, X., Liu, Y., Zhang, C., Zhang, Q., et al. (2022). Comprehensive analysis of cuproptosis-related genes in immune infiltration and prognosis in melanoma. Front. Pharmacol. 13, 930041. doi:10.3389/fphar.2022.930041
Malla, R., Padmaraju, V., and Kundrapu, D. B. (2022). Tumor-associated macrophages: Potential target of natural compounds for management of breast cancer. Life Sci. 301, 120572. doi:10.1016/j.lfs.2022.120572
Matsui, T., Hamada-Tsutsumi, S., Naito, Y., Nojima, M., Iio, E., Tamori, A., et al. (2022). Identification of microRNA-96-5p as a postoperative, prognostic microRNA predictor in nonviral hepatocellular carcinoma. Hepatol. Res. 52 (1), 93–104. doi:10.1111/hepr.13674
Nagaki, Y., Motoyama, S., Yamaguchi, T., Hoshizaki, M., Sato, Y., Sato, T., et al. (2020). m(6) A demethylase ALKBH5 promotes proliferation of esophageal squamous cell carcinoma associated with poor prognosis. Genes cells. 25 (8), 547–561. doi:10.1111/gtc.12792
Newman, A. M., Liu, C. L., Green, M. R., Gentles, A. J., Feng, W., Xu, Y., et al. (2015). Robust enumeration of cell subsets from tissue expression profiles. Nat. Methods. 12 (5), 453–457. doi:10.1038/nmeth.3337
Ru, B., Wong, C. N., Tong, Y., Zhong, J. Y., Zhong, S. S. W., Wu, W. C., et al. (2019). Tisidb: An integrated repository portal for tumor-immune system interactions. Bioinforma. Oxf. Engl. 35 (20), 4200–4202. doi:10.1093/bioinformatics/btz210
Ruggero, D., and Pandolfi, P. P. (2003). Does the ribosome translate cancer? Nat. Rev. Cancer. 3 (3), 179–192. doi:10.1038/nrc1015
Shen, C., Xuan, B., Yan, T., Ma, Y., Xu, P., Tian, X., et al. (2020). m6A-dependent glycolysis enhances colorectal cancer progression. Mol. Cancer. 19 (1), 72. doi:10.1186/s12943-020-01190-w
Sun, S., Liu, Y., Zhou, M., Wen, J., Xue, L., Han, S., et al. (2022). PA2G4 promotes the metastasis of hepatocellular carcinoma by stabilizing FYN mRNA in a YTHDF2-dependent manner. Cell & Biosci. 12 (1), 55. doi:10.1186/s13578-022-00788-5
Sung, H., Ferlay, J., Siegel, R. L., Laversanne, M., Soerjomataram, I., Jemal, A., et al. (2021). Global cancer statistics 2020: GLOBOCAN estimates of incidence and mortality worldwide for 36 cancers in 185 countries. Ca. Cancer J. Clin. 71 (3), 209–249. doi:10.3322/caac.21660
Tang, D., Chen, X., and Kroemer, G. (2022). Cuproptosis: A copper-triggered modality of mitochondrial cell death. Cell Res. 32 (5), 417–418. doi:10.1038/s41422-022-00653-7
Thrift, A. P. (2021). Global burden and epidemiology of Barrett oesophagus and oesophageal cancer. Nat. Rev. Gastroenterol. Hepatol. 18 (6), 432–443. doi:10.1038/s41575-021-00419-3
Tomczak, K., Czerwińska, P., and Wiznerowicz, M. (2015). The cancer Genome atlas (TCGA): An immeasurable source of knowledge. Contemp. Oncol. 1A (1A), 68–77. doi:10.5114/wo.2014.47136
Tsvetkov, P., Coy, S., Petrova, B., Dreishpoon, M., Verma, A., Abdusamad, M., et al. (2022). Copper induces cell death by targeting lipoylated TCA cycle proteins. Science 375 (6586), 1254–1261. doi:10.1126/science.abf0529
Vivian, J., Rao, A. A., Nothaft, F. A., Ketchum, C., Armstrong, J., Novak, A., et al. (2017). Toil enables reproducible, open source, big biomedical data analyses. Nat. Biotechnol. 35 (4), 314–316. doi:10.1038/nbt.3772
Wang, F., Wang, S., and Zhou, Q. (2020a). The resistance mechanisms of lung cancer immunotherapy. Front. Oncol. 10, 568059. doi:10.3389/fonc.2020.568059
Wang, T., Kong, S., Tao, M., and Ju, S. (2020b). The potential role of RNA N6-methyladenosine in Cancer progression. Mol. Cancer. 19 (1), 88. doi:10.1186/s12943-020-01204-7
Xu, F., Gu, J., Wang, L., Liu, R., Yuan, Y., Wang, H., et al. (2018). Up-regulation Of EIF3e Is Associated with The Progression of Esophageal Squamous Cell Carcinoma and Poor Prognosis in Patients. J. Cancer. 9 (7), 1135–1144. doi:10.7150/jca.22546
Yang, Q., Lin, W., Liu, Z., Zhu, J., Huang, N., Cui, Z., et al. (2018). RAP80 is an independent prognosis biomarker for the outcome of patients with esophageal squamous cell carcinoma. Cell Death Dis. 9 (2), 146. doi:10.1038/s41419-017-0177-2
Yu, G., Wang, L., Han, Y., and He, Q. (2012). clusterProfiler: an R package for comparing biological themes among gene clusters. Omics a J. Integr. Biol. 16 (5), 284–287. doi:10.1089/omi.2011.0118
Zarka, J., Short, N. J., Kanagal-Shamanna, R., and Issa, G. C. (2020). Nucleophosmin 1 mutations in acute myeloid leukemia. Genes 11 (6), 649. doi:10.3390/genes11060649
Zhang, M., Dai, W., Li, Z., Tang, L., Chen, J., and Chen, C. (2021). HSPA4 knockdown retarded progression and development of colorectal cancer. Cancer Manag. Res. 13, 4679–4690. doi:10.2147/CMAR.S310729
Zhang, Y., Chen, W., Pan, T., Wang, H., Zhang, Y., and Li, C. (2019). LBX2-AS1 is activated by ZEB1 and promotes the development of esophageal squamous cell carcinoma by interacting with HNRNPC to enhance the stability of ZEB1 and ZEB2 mRNAs. Biochem. Biophys. Res. Commun. 511 (3), 566–572. doi:10.1016/j.bbrc.2019.02.079
Zhou, C., Zhang, W., Chen, W., Yin, Y., Atyah, M., Liu, S., et al. (2017). Integrated analysis of copy number variations and gene expression profiling in hepatocellular carcinoma. Sci. Rep. 7 (1), 10570. doi:10.1038/s41598-017-11029-y
Zhou, L., Yuan, L., Gao, Y., Liu, X., Dai, Q., Yang, J., et al. (2021a). Nucleophosmin 1 overexpression correlates with 18F-FDG PET/CT metabolic parameters and improves diagnostic accuracy in patients with lung adenocarcinoma. Eur. J. Nucl. Med. Mol. Imaging 48 (3), 904–912. doi:10.1007/s00259-020-05005-4
Zhou, W., Yun, Z., Wang, T., Li, C., and Zhang, J. (2021b). BTF3-mediated regulation of BMI1 promotes colorectal cancer through influencing epithelial-mesenchymal transition and stem cell-like traits. Int. J. Biol. Macromol. 187, 800–810. doi:10.1016/j.ijbiomac.2021.07.106
Zhou, Y., Lu, L., Jiang, G., Chen, Z., Li, J., An, P., et al. (2019). Targeting CDK7 increases the stability of Snail to promote the dissemination of colorectal cancer. Cell Death Differ. 26 (8), 1442–1452. doi:10.1038/s41418-018-0222-4
Zhu, W., Tan, L., Ma, T., Yin, Z., and Gao, J. (2022). Long noncoding RNA SNHG8 promotes chemoresistance in gastric cancer via binding with hnRNPA1 and stabilizing TROY expression. Dig. Liver Dis. S1590-8658 (22), 00202–X. doi:10.1016/j.dld.2022.02.011
Zhu, Y., Shi, M., Chen, H., Gu, J., Zhang, J., Shen, B., et al. (2015). NPM1 activates metabolic changes by inhibiting FBP1 while promoting the tumorigenicity of pancreatic cancer cells. Oncotarget 6 (25), 21443–21451. doi:10.18632/oncotarget.4167
Keywords: NPM1, gastrointestinal cancer, immune infiltrates, cuproptosis, m6A
Citation: Liu X-S, Liu C, Zeng J, Zeng D-B, Chen Y-J, Tan F, Gao Y, Liu X-Y, Zhang Y, Zhang Y-H and Pei Z-J (2022) Nucleophosmin 1 is a prognostic marker of gastrointestinal cancer and is associated with m6A and cuproptosis. Front. Pharmacol. 13:1010879. doi: 10.3389/fphar.2022.1010879
Received: 03 August 2022; Accepted: 29 August 2022;
Published: 14 September 2022.
Edited by:
Zhijie Xu, Central South University, ChinaReviewed by:
Ziheng Wang, University of Macau, ChinaHou Jian, The Chinese University of Hong Kong, China
Copyright © 2022 Liu, Liu, Zeng, Zeng, Chen, Tan, Gao, Liu, Zhang, Zhang and Pei. This is an open-access article distributed under the terms of the Creative Commons Attribution License (CC BY). The use, distribution or reproduction in other forums is permitted, provided the original author(s) and the copyright owner(s) are credited and that the original publication in this journal is cited, in accordance with accepted academic practice. No use, distribution or reproduction is permitted which does not comply with these terms.
*Correspondence: Xu-Sheng Liu, bHhza2luZ0B0YWloZWhvc3BpdGFsLmNvbQ==; Zhi-Jun Pei, cHpqem1sMTk4MEB0YWloZWhvc3BpdGFsLmNvbQ==
†These authors have contributed equally to this work