- 1Department of Cardiology, The Eighth Affiliated Hospital, Sun Yat-sen University, Shenzhen, China
- 2Department of Pathophysiology, Zhongshan School of Medicine, Sun Yat-sen University, Guangzhou, China
Introduction: Renal interstitial fibrosis is a common pathophysiological change in the chronic kidney disease (CKD). Nicotinamide adenine dinucleotide (NAD)-dependent deacetylase sirtuin 6 (SIRT6) is demonstrated to protect against kidney injury. Vitamin B3 is the mostly used form of NAD precursors. However, the role of SIRT6 overexpression in renal interstitial fibrosis of CKD and the association between dietary vitamin B3 intake and renal function remain to be elucidated.
Methods: Wild-type (WT) and SIRT6-transgene (SIRT6-Tg) mice were given with high-adenine diets to establish CKD model. HK2 cells were exposed to transforming growth factor β1 (TGF-β1) in vitro to explore related mechanism. Population data from Multi-Ethnic Study of Atherosclerosis (MESA) was used to examine the association between dietary vitamin B3 intake and renal function decline.
Results: Compared to WT mice, SIRT6-Tg mice exhibited alleviated renal interstitial fibrosis as evidenced by reduced collagen deposit, collagen I and α-smooth muscle actin expression. Renal function was also improved in SIRT6-Tg mice. Homeodomain interacting protein kinase 2 (HIPK2) was induced during the fibrogenesis in CKD, while HIPK2 was downregulated after SIRT6 overexpression. Further assay in vitro confirmed that SIRT6 depletion exacerbated epithelial-to-mesenchymal transition of HK2 cells, which might be linked with HIPK2 upregulation. HIPK2 was inhibited by SIRT6 in the post-transcriptional level. Population study indicated that higher dietary vitamin B3 intake was independently correlated with a lower risk of estimate glomerular filtration rate decline in those ≥65 years old during follow-up.
Conclusion: SIRT6/HIPK2 axis serves as a promising target of renal interstitial fibrosis in CKD. Dietary vitamin B3 intake is beneficial for renal function in the old people.
Introduction
Chronic kidney disease (CKD) is highly prevalent in the world, especially in those with diabetes, hypertension as well as the old population (Carney, 2020; Collaboration, 2020). Although the initial etiology varies in CKD patients, renal interstitial fibrosis is the common and final pathological change (Ruiz-Ortega et al., 2020). Renal interstitial fibrosis is characterized by abnormal accumulation of extracellular matrix, leading to progression to end-stage renal disease (ESRD) (Livingston et al., 2016; Luo et al., 2018). Notably, tubular epithelial cells are of large plasticity, which undergo epithelial-to-mesenchymal transition (EMT) in response to renal injury. Tubular epithelial cells increasingly express α-smooth muscle actin (αSMA) and produce collagen to potentiate fibrotic pathogenesis in CKD (Liu et al., 2019; Bozic et al., 2020).
Sirtuin 6 (SIRT6) belongs to the conserved nicotinamide adenine dinucleotide (NAD+)-dependent chromatin deacetylases family, which is implicated in genomic stability, inflammation and energy metabolism regulation (Chang et al., 2020). Overexpressed SIRT6 is demonstrated to protect against acute kidney injury (Li et al., 2018; Zhang et al., 2019). SIRT6 also impedes diabetic nephropathy by mitigating podocyte and proximal tubules injury (Huang et al., 2017; Muraoka et al., 2019; Yang et al., 2020). Hyperactivation of transforming growth factor β (TGF-β) signaling is the master inducer of fibrosis pathogenesis (Meng et al., 2016). SIRT6 deficiency significantly activates TGF-β signaling and exacerbates aging-related organ fibrosis (Maity et al., 2020). Meanwhile, knockdown of SIRT6 aggravates unilateral ureteral obstruction-induced renal fibrosis (Cai et al., 2020). As previous study shows that SIRT6 is upregulated during renal injury (Cai et al., 2020), whether further SIRT6 overexpression is capable of blocking CKD-associated renal interstitial fibrosis remains to be elucidated.
Homeodomain interacting protein kinase 2 (HIPK2) is a nuclear serine/threonine kinase and initially discovered as a corepressor of NK homeoproteins (Choi et al., 2013). Recently, HIPK2 is regarded as a central contributor to renal fibrosis and accounts for multiple pro-fibrosis signaling pathways including TGF-β, Wnt/β-catenin and Notch pathway (Jin et al., 2012; Xiao et al., 2020). Inhibiting of HIPK2 also exhibits a remarkable alleviation in renal fibrosis (Liu et al., 2017; Xu et al., 2019). However, its potential regulation by SIRT6 is still undetermined.
NAD is a critical co-enzyme involved in cellular physiological function, which determines the activity of diverse enzymes including sirtuins, PARPs, CD38 and so on (Chini et al., 2021). Although activator of SIRT6 is unavailable in clinical, intervention in NAD metabolism might be an alternative strategy. In fact, emerging evidence suggests that NAD level declines in kidney injury, while NAD supplements was demonstrated to protect against kidney diseases (Katsyuba et al., 2018; Manrique-Caballero et al., 2021; Bignon et al., 2022). Supplements with different forms of vitamin B3, the NAD precursors, are also demonstrated to improve SIRT6 activity and expression (Diani-Moore et al., 2017; Hou et al., 2018; Kim et al., 2022; Ru et al., 2022). Several NAD precursors have been tested in clinical, and vitamin B3 as the simple and mostly use form of NAD precursor shows a promisingly positive result (Bagcchi, 2015; Dollerup et al., 2018; Pirinen et al., 2020). Even though, the effect of dietary vitamin B3 intake on renal function progression remains unknown.
In this study, we generated SIRT6-transgenic (SIRT6-Tg) mice to see the protective effect of SIRT6 overexpression in CKD-associated renal interstitial fibrosis. Upregulation of HIPK2 in fibrotic kidney and tubular epithelial cells undergoing EMT was also blocked after SIRT6 overexpression. Using population data from MESA, we found that higher dietary vitamin B3 intake is related to lower risk of renal function decline in the old people. Our finding suggested an involvement of SIRT6/HIPK2 axis in renal interstitial fibrosis and provided a promising target in CKD prevention.
Materials and methods
Study population
The population data was obtained from the Multi-Ethnic Study of Atherosclerosis (MESA) dataset. The detailed design and examination of MESA have been described previously (Bild et al., 2002). Briefly, MESA is a prospective observational cohort study, which recruited 6,814 participants between July 2000 and September 2002 from different races. In this study, we included participants with baseline dietary vitamin B3 intake and estimate glomerular filtration rate (eGFR) measurements at Exam1 (2000–2002) and follow-up eGFR at Exam3 (2004–2005). The study flowchart was presented in Supplementary Figure S1. Of the 6,814 participants, those with missing covariates (n = 220), missing dietary vitamin B3 and total energy intake data (n = 546) and missing follow-up eGFR data from Exam 3 (n = 789) were excluded. Adjusted dietary vitamin B3 intake was calculated as vitamin B3 per 1,000 kcal total energy intake per day. Renal function decline was defined as ≥10% drop in eGFR according to the baseline (Huang et al., 2021).
Diet assessment
The usual diet of participants was characterized using a self-administered 120-item food frequency questionnaire (FFQ), which included the type and frequency of foods consumed over the past year. Participants were recorded with the serving size (small, medium or large) and frequency of consumption (average times per day, week or month), with corresponding weight estimated according to National Health and Nutrition Examination Survey data. Approximate amounts of dietary vitamin B3 intake was imputed with the DietSys Nutrient Analysis Program (Nettleton et al., 2006; Gao et al., 2021).
Animal experiments
Eight-week-old C57BL/6J mice were obtained from the Laboratory Animal Center of Sun Yat-sen University. SIRT6-Tg mice with C57BL/6J background were generated and bred as previously reported (Li et al., 2022). The mice were randomly given a chow diet as the control group, or a high adenine diet (0.25% adenine and 1.2% phosphorus) for 12 weeks as the CKD-related renal fibrosis group. All mice were housed in a constant temperature and a 12 h light-dark cycle with free access to water and food. The kidneys were harvested for further research. The animal experiment was conducted according to the animal ethical standards and approved by the Ethics Committee of Zhongshan School of Medicine, Sun Yat-sen University.
Cell culture
Human renal tubular epithelial cells line (HK2 cells) was purchased from the American Type Culture Collection and cultured in Dulbecco’s modified Eagle’s medium/F12 containing 10% fetal bovine serum (FBS) (Gibco). To induce cellular fibrotic model, TGF-β1 (R&D Systems) was added into the medium for 48 h. For SIRT6 depletion, HK2 cells were transfected with control small interfering RNA (siRNA) and SIRT6 siRNA (IGE Biotechnology, China) using Lipofectmaine RNAiMAX (Invitrogen) according to the manufacturer’s instructions. The sequence of SIRT6 siRNA was AGTTCGACACCACCTTTGA (5′-3′).
Western blot analysis
Cells or tissue homogenates were added with RIPA lysis buffer on ice for 15 min. The lysates were collected and centrifuged at 13000 rpm at 4°C for 20 min. Proteins were separated on sodium dodecyl sulfate polyacrylamide gel following with transferred to PVDF membranes (Millipore). The membranes were blocked with 5% skim milk and incubated with specified antibodies. Band intensity was analyzed with the ImageJ software.
Antibodies against α-SMA (ab21027), SIRT6 (ab191385) and HIPK2 (ab108543) were obtained from Abcam. Antibody against Tubulin (11224-1-AP) was obtained from Proteintech. Antibody against HIPK2 (sc-100383) was obtained from Santa Cruz Biotechnology. Antibodies against E-cadherin (3195S), collagen I (72026S), GAPDH (5174S), secondary anti-rabbit (4412S) and anti-mouse (4408S) were purchased from Cell Signaling Technology.
Reverse transcription and real-time qPCR
Cellular mRNA was isolated from HK2 cells using RNAiso Plus reagent (TaKaRa, Japan) and then reverse-transcribed to cDNA according to the manufacturer’s instructions (TaKaRa, Japan). Gene expression was quantified with the real-time PCR system (Roche LightCycler 480) and detected by SYBR Green Premix (TaKaRa, Japan). Relative expression of mRNA was determined using 2−ΔΔCT method with GAPDH as a reference gene.
Hematoxylin and eosin, masson trichrome staining
Paraffin-embedded kidney sections were deparaffinized and stained according to the manufacturer’s protocols by using the Hematoxylin and eosin staining kit (Solarbio, China), and Masson trichrome staining kit (Solarbio, China). Images were captured with light microscopy (Nikon NiU).
Immunohistochemical staining
Paraffin-embedded kidney sections were deparaffinized and rehydrated following by heat-mediated antigen retrieval with 10% citrate buffer. Endogenous peroxidase activity was eliminated with 3% H2O2. Then the sections were incubated with primary antibodies overnight at 4°C, covered with corresponding secondary antibodies in the room temperature and visualized with diaminobenzidine. Images were captured with light microscopy (Nikon NiU).
Immunofluorescence staining
HK2 cells were fixed with 4% paraformaldehyde for 15 min and permeabilized with 0.1% Triton X-100 for 10 min. After blocking, cells were incubated with primary antibiotics overnight and then detected with fluorescent dye-conjugated secondary antibody. Nuclei were stained with DAPI for 10 min. Images were captured with fluorescence microscope (Olympus IX83).
Statistical analysis
All data were analyzed with SPSS and GraphPad Prism 8.0 software. The results were presented as mean ± S.E.M. To compare two group, Student’s t test or nonparametric Mann-Whitney U test were performed, while ordinary one-way ANOVA was conducted to compare multiple groups. A logistic regression model was established to compute risk ratios (RRs) and 95% confidence intervals (CI) to assess the association between dietary niacin intake and risk of renal function decline. p value < 0.05 was considered statistically significant.
Results
SIRT6 overexpression impedes renal interstitial fibrosis and renal function deterioration in chronic kidney disease
To verify the effect of SIRT6 in renal interstitial fibrosis, high adenine diet-induced CKD model was established in WT and SIRT6-Tg mice. As expected, αSMA was produced in the kidney of CKD, accompanied by elevated SIRT6 expression (Figure 1A). In WT mice with CKD, hematoxylin and eosin staining confirmed an abroad mononuclear cells infiltration and kidney tubules damage, while Masson trichrome staining showed the collagen deposition, all of which were alleviated in SIRT6-Tg mice (Figure 1B). A significant upregulation of collagen I and αSMA in CKD was evidenced by immunohistochemistry staining. Such effect was also less apparent in SIRT6-Tg mice (Figure 1B). Additionally, the results of renal function assessments confirmed that serum creatinine, blood urea nitrogen (BUN) and proteinuria for 24 h were improved in SIRT6-Tg mice (Figures 1C–E). Taken together, these data reveal that SIRT6 overexpression protects against renal interstitial fibrosis in CKD.
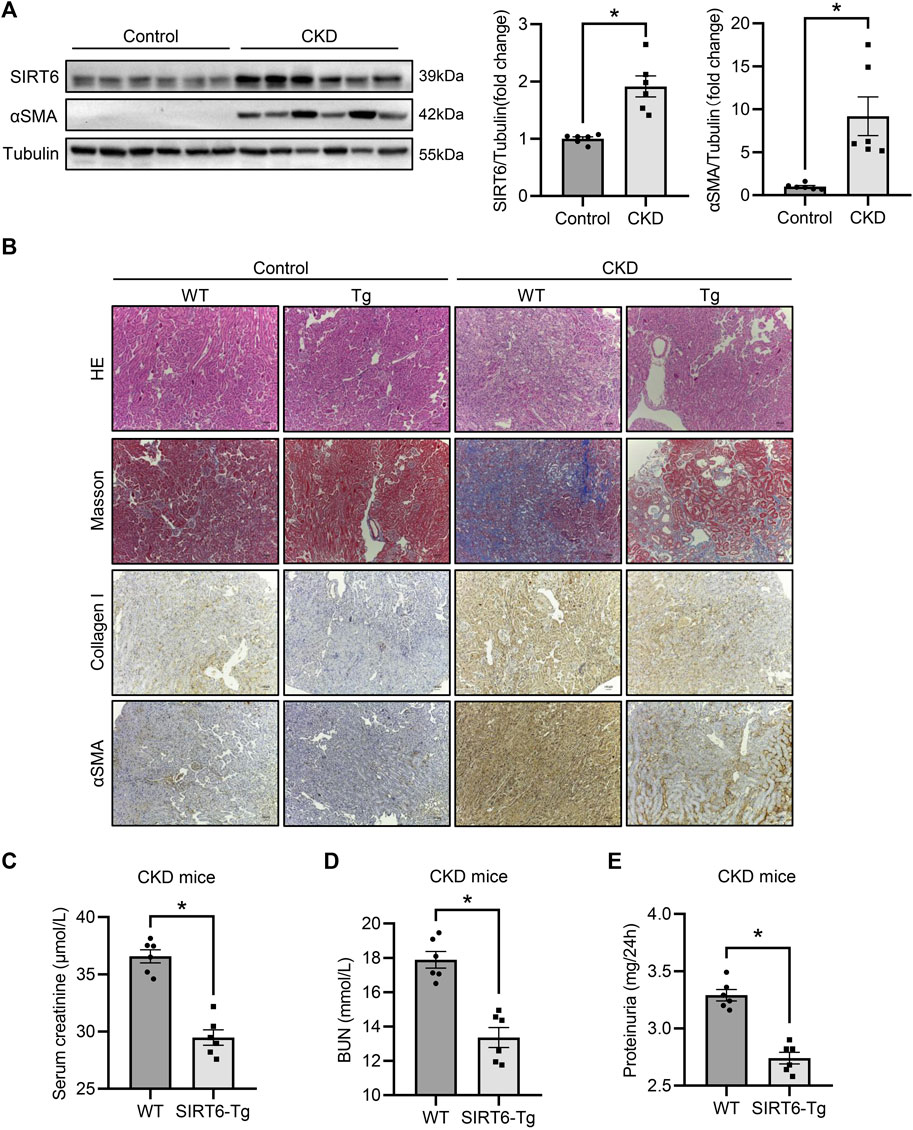
FIGURE 1. SIRT6 overexpression protected against renal interstitial fibrosis. (A) Western blot analysis of SIRT6 and αSMA expression in fibrotic kidneys of CKD mice. n = 6. (B) Representative hematoxylin and eosin staining, Masson trichrome staining and immunohistochemical images of collagen I and αSMA expression in kidneys. Scale bar, 100 μm. (C–E) Sera from WT and SIRT6-Tg of CKD mice were tested for serum creatinine, BUN and proteinuria for 24 h n = 6. All values are presented as mean ± SEM. *p < 0.05.
Upregulation of HIPK2 in chronic kidney disease is blocked by SIRT6 overexpression
Considering the key modulation of HIPK2 in pro-fibrosis signaling, we next detect the expression of HIPK2 in CKD. As expected, HIPK2 was largely induced in the fibrotic kidney of CKD (Figure 2A). Since SIRT6 overexpression exhibited a prominent mitigation in renal interstitial fibrosis, we speculated that such effect might be attributed to HIPK2 downregulation. Notably, compared to WT mice, SIRT6-Tg mice presented a reduced HIPK2 expression both in kidneys of control and CKD mice, as evidenced by immunohistochemical and western blot analysis (Figures 2B,C). These findings suggest that the beneficial role of SIRT6 in renal interstitial fibrosis prevention might be associated with HIPK2 signaling inhibition.
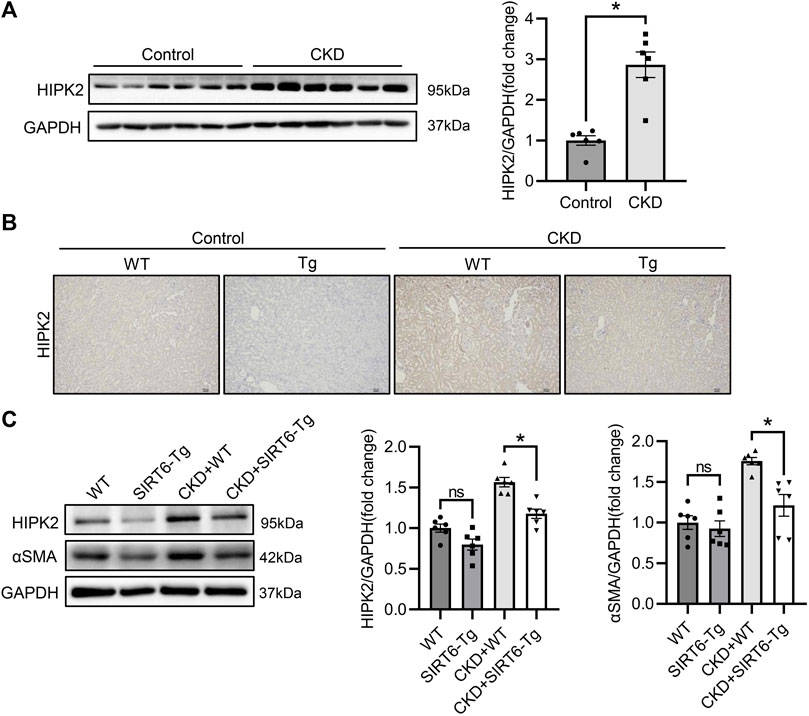
FIGURE 2. HIPK2 was downregulated in the kidneys of SIRT6-Tg mice. (A) Western blot analysis of HIPK2 expression in fibrotic kidneys of CKD mice. n = 6. (B) Representative immunohistochemical images of HIPK2 in kidneys of SIRT6-Tg mice. Scale bar, 100 μm. (C) Western blot analysis of HIPK2 and αSMA expression in fibrotic kidneys of WT and SIRT6-Tg mice. n = 6. All values are presented as mean ± SEM. *p < 0.05.
SIRT6 and HIPK2 are induced by TGF-β1 in renal tubular epithelial cells
To further elucidate the modulation of SIRT6 and HIPK2 in renal interstitial fibrosis, HK2 cells were treated with TGF-β1 to establish fibrotic model in vitro. As shown in Figure 3A, SIRT6 was upregulation in HK2 cells after TGF-β1 treatment for 48 h in a dose-dependent manner, together with increased collagen I expression. Consistently, HK2 cells switched from epithelial to mesenchymal phenotype, with the evidence of reduced epithelial marker E-cadherin and higher αSMA and collagen I expression. HIPK2 was also significantly elevated after TGF-β1 exposure (Figure 3B). Furthermore, quantitative real-time PCR analysis confirmed the phenotypic transition of HK2 cells, and both SIRT6 and HIPK2 were induced by TGF-β1 in transcriptional level (Figure 3C). Collectively, SIRT6 and HIPK2 are upregulated by TGF-β1 in renal tubular epithelial cells undergoing EMT.
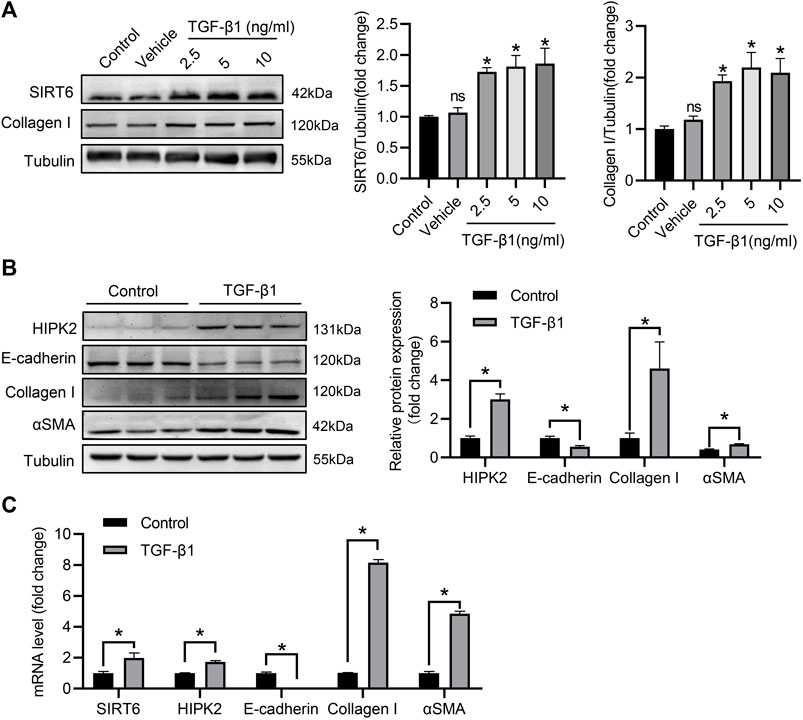
FIGURE 3. SIRT6 and HIPK2 are induced by TGF-β1 in HK2 cells. (A) Western blot analysis of SIRT6 and collagen I expression in HK2 cells exposed to TGF-β1 for 48 h with indicated dose. n = 3. (B) Western blot analysis of HIPK2 expression in HK2 cells exposed to 2.5 ng/ml TGF-β1 for 48 h n = 3. (C) Quantitative real-time PCR analysis of genes expression in HK2 cells treated with TGF-β1. n = 3. All values are presented as mean ± SEM. *p < 0.05.
SIRT6 inhibits HIPK2 signaling by post-transcriptional regulation
Accumulating study suggest that the protein level of HIPK2 is determined by the post-transcriptional modification (de la Vega et al., 2012; Wook Choi and Yong Choi, 2014). Deacetylation of HIPK2 by deacetylases subsequently leads to its proteasomal degradation (Hwang et al., 2013). To explore the potential regulation of SIRT6 in HIPK2, small intervening RNA was utilized to inhibit SIRT6 expression in HK2 cells. As expected, the expression of SIRT6 was largely blocked after intervention, while HIPK2 was upregulated oppositely in the same time (Figure 4A). However, SIRT6 deficiency showed no impact on the mRNA level of HIPK2 (Figure 4B), indicating that HIPK2 was regulated by SIRT6 in post-transcriptional manner. Immunofluorescence also confirmed that the induction of HIPK2 by TGF-β1 exposure was exacerbated after SIRT6 depletion (Figure 4C). SIRT6 depletion promoted the phenotypic transition of HK2 cells, accompanied by HIPK2 upregulation (Figure 4D). Together, these data suggest a post-transcriptional downregulation of HIPK2 by SIRT6.
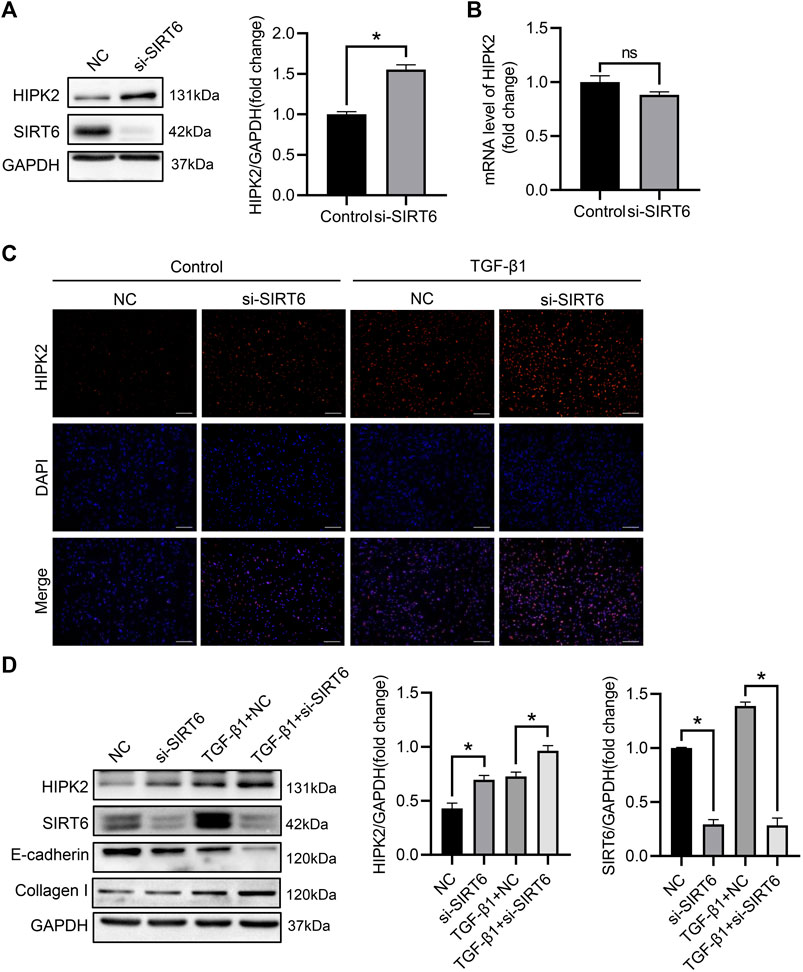
FIGURE 4. HIPK2 was downregulated by SIRT6 in the post-transcriptional level. (A) Western blot analysis of SIRT6 and HIPK2 expression after indicated transfection. n = 3. (B) Quantitative real-time PCR analysis of HIPK2 expression after SIRT6 depletion. n = 3. (C) Representative immunofluorescence images of HIPK2 in TGF-β1-induced HK2 cells after SIRT6 depletion. Scale bar, 200 μm. (D) Western blot analysis of SIRT6 and HIPK2 expression in TGF-β1-induced HK2 cells after SIRT6 depletion. n = 3. All values are presented as mean ± SEM. *p < 0.05.
Association between dietary vitamin B3 intake and renal function decline
Although the protective role of SIRT6 in CKD is prospective, drug target at SIRT6 is still unavailable in clinical. Indeed, the activity of SIRT6 is determined by the level of NAD. Since vitamin B3 is the mostly used form of NAD precursors, it propelled us to examine the association between dietary vitamin B3 intake and renal function progression. A total of 5,259 participants with a mean age of 61.8 ± 9.8 years old and 47.3% men from MESA was included in our study. As presented in Table 1, those with higher dietary vitamin B3 intake are prone to be Chinese American, diabetes, having higher fasting plasma glucose, less smoker and drinker, lower body mass index, total cholesterol, low-density lipoprotein cholesterol and homocysteine (p < 0.05). There was no significant difference in age, baseline eGFR, serum creatinine, hypertension prevalence, blood pressure, triglycerides, high-density lipoprotein cholesterol, use of angiotensin receptor blockers and angiotensin-converting enzyme inhibitors among the four groups.
After an average follow-up of 3.2 years, 1,261 participants exhibited renal function decline, 634 (20.9%) for those <65 years old and 627 (28.1%) for those ≥65 years old. As for participants ≥65 years old, highest dietary vitamin B3 intake group showed a reduced risk of renal function decline compared to the lowest group (Model 1: RR 0.744, 95%CI 0.574-0.964). The results did not appreciably change after adjustment of covariates (Model 2: RR 0.744, 95%CI 0.567-0.975; Model 3: RR 0.728, 95%CI 0.548–0.966). But this correlation could not be seen in the younger group with age <65 years old (Table 2). These finding suggests that high dietary vitamin B3 intake is associated with lower risk of renal function decline in the old population ≥65 years old.
Discussion
In this study, we demonstrated that SIRT6 overexpression mitigated the renal interstitial fibrosis and renal function deterioration in CKD. HIPK2 was induced during the fibrogenesis, which was blocked after SIRT6 overexpression. SIRT6 depletion exacerbated the EMT of renal tubular epithelial cells, together with HIPK2 upregulation. HIPK2 was downregulated by SIRT6 in the post-transcriptional level. Population study indicated that old people with high dietary vitamin B3 intake tended to have lower risk of renal function decline. Taken together, our findings suggest that SIRT6/HIPK2 axis is a potential target to intervene renal fibrosis and delay CKD progression.
Renal fibrosis, mainly referred to renal interstitial fibrosis, is the inevitably common outcome of CKD progression. However, effective intervention to retard fibrotic process is still unavailable (Humphreys, 2018). As a longevity gene, SIRT6 presents a favorable prospect in fibrosis prevention (Yang et al., 2021). Indeed, SIRT6 is demonstrated to protect against liver fibrosis by inactivating hepatic stellate cells (Zhong et al., 2020; Zhang et al., 2021). Diabetic myocardial fibrosis is alleviated through SIRT6/AMPK signaling pathway (Li et al., 2020). SIRT6 deficiency also accounts for the aging-related cardiac fibrosis (Pillai et al., 2021). Previous study suggests that loss of SIRT6 aggravates unilateral ureteral obstruction-induced tubulointerstitial inflammation and fibrosis (Cai et al., 2020; Jin et al., 2022). While our study verified that renal interstitial fibrosis in adenine-induced CKD was alleviated by SIRT6 overexpression. And renal function was improved a lot after SIRT6 overexpression in CKD. Interestingly, SIRT6 was upregulated during renal fibrogenesis. Since improvement in renal fibrosis can also be seen through further SIRT6 overexpression, we thought it might be a compensatory mechanism. Besides, the level of NAD was declined in the development of CKD, which might also contributed to the defective function of SIRT6 (Liu et al., 2021).
Tubular epithelial cell undergoing epithelial-to-mesenchymal transition is recognized as a key contributor to renal fibrosis (Lovisa et al., 2016). While blunting this transition is considered as a promising strategy to reduce fibrogenesis (Xavier et al., 2015). Here, we found SIRT6 deficiency exacerbated epithelial-to-mesenchymal transition of tubular epithelial cells induced by TGF-β1. Likewise, SIRT6 mitigates kidney ischemia/reperfusion injury through retarding hypoxia-induced phenotypic transition of tubular epithelial cells (Gao et al., 2020). Other study also supports the powerful effect of SIRT6 on suppression of phenotypic transition. SIRT6 prevents pulmonary fibrosis by inhibiting pulmonary epithelial to mesenchymal transition (Tian et al., 2017; Chen et al., 2019).
Another important finding in our research was the suppression of SIRT6 on HIPK2. HIPK2 is a common upstream of multiple pro-fibrosis signaling pathway during renal fibrogenesis (Fan et al., 2014). Following study also finds that knockdown of HIPK2 is capable of alleviating Angiotensin II-induced cardiac fibrosis (Xu et al., 2022). Liver fibrosis is also mediated by HIPK2 activation in hepatic stellate cells (He et al., 2017). In our study, upregulation of HIPK2 in CKD-related renal interstitial fibrosis was blocked by SIRT6 overexpression. SIRT6 depletion accelerated the epithelial-to-mesenchymal transition of tubular epithelial cells, which was also associated with elevated HIPK2. Further study suggested that HIPK2 was inhibited by SIRT6 in post-transcriptional way. Indeed, deacetylation of HIPK2 is linked with its protein instability and subsequent proteasomal degradation (Hwang et al., 2013; Choi et al., 2017). Even though, whether HIPK2 is directly deacetylated by SIRT6 remains to be elucidated.
The activity of SIRT6 was dependent on the level of NAD. NAD biosynthesis is interrupted in response to kidney injury, while NAD-replacement therapy is considered to protect against human diseases (Bignon et al., 2022). Indeed, the precursors of NAD such as vitamin B3 or the simpler forms of vitamin B3 like nicotinamide or nicotinic are demonstrated to impede renal diseases (Streja et al., 2015; Hyndman and Griffin, 2021). Nicotinamide reduces renal interstitial fibrosis in mice (Zheng et al., 2019). Long-time treatment with vitamin B3 in CKD patients is capable of lowing serum phosphorous concentrations (Müller et al., 2007; Maccubbin et al., 2010; Malhotra et al., 2018). Here, we found that higher dietary vitamin B3 intake was related to slower renal function decline in the old population, but not the younger group. This might be attributed to that the NAD levels decline with aging, especially in the old people (Katsyuba et al., 2020). Vitamin B3 is known to increase NAD level in tissue, inhibit inflammation and oxidative stress, as well as improve lipid metabolism, which may account for its benefits in renal function (Wu et al., 2012; Ganji et al., 2015; Montserrat-de la Paz et al., 2019; Horimatsu et al., 2020). Even though, the limitation should be noticed that other nutriment intake was not considered in this study, which might also contribute to confounding.
Conclusion
In summary, we uncovered the beneficial effect of SIRT6 overexpression on renal interstitial fibrosis of CKD, which might be associated with HIPK2 downregulation. We also highlighted the benefit of dietary vitamin B3 intake in the renal function of old population. SIRT6/HIPK2 axis may be a therapeutic target in the CKD treatment.
Data availability statement
The datasets presented in this study can be found in online repositories. The names of the repository/repositories and accession number(s) can be found in the article/Supplementary Material.
Ethics statement
The studies involving human participants were reviewed and approved by the Ethics Committee of Multi-Ethnic Study of Atherosclerosis (MESA). The patients/participants provided their written informed consent to participate in this study. The animal study was reviewed and approved by the Ethics Committee of Zhongshan School of Medicine, Sun Yat-sen University. Written informed consent was obtained from the individual(s) for the publication of any potentially identifiable images or data included in this article.
Author contributions
XL designed, performed, analyzed the research, and wrote the manuscript. WL performed the renal function test of mice. ZZ helped in statistical analysis. WW and HH assisted in designing and interpreting the study.
Funding
This work was supported by National Natural Science Foundation of China (NSFC) (82061160372 and 81870506), project of traditional Chinese medicine in Guangdong province (20201062), Basic Research Project of Shenzhen Science and Technology Innovation Committee (JCYJ20180306174648342, JCYJ20190808102005602 and KCXFZ20211020163801002), Futian District Public Health Scientific Research Project of Shenzhen (FTWS2019003) and Shenzhen Key Medical Discipline Construction Fund (SZXK002) and Guangdong Basic and Applied Basic Research Foundation (2021B1515120083) to HH.
Conflict of interest
The authors declare that the research was conducted in the absence of any commercial or financial relationships that could be construed as a potential conflict of interest.
Publisher’s note
All claims expressed in this article are solely those of the authors and do not necessarily represent those of their affiliated organizations, or those of the publisher, the editors and the reviewers. Any product that may be evaluated in this article, or claim that may be made by its manufacturer, is not guaranteed or endorsed by the publisher.
Supplementary material
The Supplementary Material for this article can be found online at: https://www.frontiersin.org/articles/10.3389/fphar.2022.1007168/full#supplementary-material
References
Bagcchi, S. (2015). Nicotinamide yields impressive results in skin cancer. Lancet. Oncol. 16, e591. doi:10.1016/s1470-2045(15)00460-x
Bignon, Y., Rinaldi, A., Nadour, Z., Poindessous, V., Nemazanyy, I., Lenoir, O., et al. (2022). Cell stress response impairs de novo NAD+ biosynthesis in the kidney. JCI insight 7, e153019. doi:10.1172/jci.insight.153019
Bild, D., Bluemke, D., Burke, G., Detrano, R., Diez Roux, A., Folsom, A., et al. (2002). Multi-ethnic study of Atherosclerosis: Objectives and design. Am. J. Epidemiol. 156, 871–881. doi:10.1093/aje/kwf113
Bozic, M., Caus, M., Rodrigues-Diez, R., Pedraza, N., Ruiz-Ortega, M., Garí, E., et al. (2020). Protective role of renal proximal tubular alpha-synuclein in the pathogenesis of kidney fibrosis. Nat. Commun. 11, 1943. doi:10.1038/s41467-020-15732-9
Cai, J., Liu, Z., Huang, X., Shu, S., Hu, X., Zheng, M., et al. (2020). The deacetylase sirtuin 6 protects against kidney fibrosis by epigenetically blocking β-catenin target gene expression. Kidney Int. 97, 106–118. doi:10.1016/j.kint.2019.08.028
Carney, E. (2020). The impact of chronic kidney disease on global health. Nat. Rev. Nephrol. 16, 251. doi:10.1038/s41581-020-0268-7
Chang, A., Ferrer, C., and Mostoslavsky, R. (2020). SIRT6, a mammalian deacylase with multitasking abilities. Physiol. Rev. 100, 145–169. doi:10.1152/physrev.00030.2018
Chen, P., Tian, K., Tu, W., Zhang, Q., Han, L., and Zhou, X. (2019). Sirtuin 6 inhibits MWCNTs-induced epithelial-mesenchymal transition in human bronchial epithelial cells via inactivating TGF-β1/Smad2 signaling pathway. Toxicol. Appl. Pharmacol. 374, 1–10. doi:10.1016/j.taap.2019.04.013
Chini, C., Zeidler, J., Kashyap, S., Warner, G., and Chini, E. (2021). Evolving concepts in NAD+ metabolism. Cell Metab. 33, 1076–1087. doi:10.1016/j.cmet.2021.04.003
Choi, D., Na, W., Kabir, M., Yi, E., Kwon, S., Yeom, J., et al. (2013). WIP1, a homeostatic regulator of the DNA damage response, is targeted by HIPK2 for phosphorylation and degradation. Mol. Cell 51, 374–385. doi:10.1016/j.molcel.2013.06.010
Choi, J., Lee, S., Shin, K., Choi, C., and Kang, S. (2017). p300-mediated acetylation increased the protein stability of HIPK2 and enhanced its tumor suppressor function. Sci. Rep. 7, 16136. doi:10.1038/s41598-017-16489-w
Collaboration, G. C. K. D. (2020). Global, regional, and national burden of chronic kidney disease, 1990-2017: A systematic analysis for the global burden of disease study 2017. Lancet 395, 709–733. doi:10.1016/s0140-6736(20)30045-3
de la Vega, L., Grishina, I., Moreno, R., Krüger, M., Braun, T., and Schmitz, M. (2012). A redox-regulated SUMO/acetylation switch of HIPK2 controls the survival threshold to oxidative stress. Mol. Cell 46, 472–483. doi:10.1016/j.molcel.2012.03.003
Diani-Moore, S., Shoots, J., Singh, R., Zuk, J., and Rifkind, A. (2017). NAD+ loss, a new player in AhR biology: Prevention of thymus atrophy and hepatosteatosis by NAD+ repletion. Sci. Rep. 7, 2268. doi:10.1038/s41598-017-02332-9
Dollerup, O., Christensen, B., Svart, M., Schmidt, M., Sulek, K., Ringgaard, S., et al. (2018). A randomized placebo-controlled clinical trial of nicotinamide riboside in obese men: Safety, insulin-sensitivity, and lipid-mobilizing effects. Am. J. Clin. Nutr. 108, 343–353. doi:10.1093/ajcn/nqy132
Fan, Y., Wang, N., Chuang, P., and He, J. (2014). Role of HIPK2 in kidney fibrosis. Kidney Int. Suppl. 4, 97–101. doi:10.1038/kisup.2014.18
Ganji, S., Kashyap, M., and Kamanna, V. (2015). Niacin inhibits fat accumulation, oxidative stress, and inflammatory cytokine IL-8 in cultured hepatocytes: Impact on non-alcoholic fatty liver disease. Metabolism. 64, 982–990. doi:10.1016/j.metabol.2015.05.002
Gao, J., Zhang, S., Hao, Q., Huang, F., Liu, Z., Zhang, H., et al. (2021). Association of dietary zinc intake with coronary artery calcium progression: The multi-ethnic study of Atherosclerosis (MESA). Eur. J. Nutr. 60, 2759–2767. doi:10.1007/s00394-020-02452-5
Gao, Z., Chen, X., Fan, Y., Zhu, K., Shi, M., and Ding, G. (2020). Sirt6 attenuates hypoxia-induced tubular epithelial cell injury via targeting G2/M phase arrest. J. Cell. Physiol. 235, 3463–3473. doi:10.1002/jcp.29235
He, P., Yu, Z., Sun, C., Jiao, S., and Jiang, H. (2017). Knockdown of HIPK2 attenuates the pro-fibrogenic response of hepatic stellate cells induced by TGF-β1. Biomed. Pharmacother. 85, 575–581. doi:10.1016/j.biopha.2016.11.066
Horimatsu, T., Blomkalns, A., Ogbi, M., Moses, M., Kim, D., Patel, S., et al. (2020). Niacin protects against abdominal aortic aneurysm formation via GPR109A independent mechanisms: Role of NAD+/nicotinamide. Cardiovasc. Res. 116, 2226–2238. doi:10.1093/cvr/cvz303
Hou, Y., Lautrup, S., Cordonnier, S., Wang, Y., Croteau, D., Zavala, E., et al. (2018). NAD+ supplementation normalizes key Alzheimer's features and DNA damage responses in a new AD mouse model with introduced DNA repair deficiency. Proc. Natl. Acad. Sci. U. S. A. 115, E1876–E1885. doi:10.1073/pnas.1718819115
Huang, A., Leipsic, J., Zekry, S., Sellers, S., Ahmadi, A., Blanke, P., et al. (2021). Effects of chronic kidney disease and declining renal function on coronary atherosclerotic plaque progression: A PARADIGM substudy. Eur. Heart J. Cardiovasc. Imaging 22, 1072–1082. doi:10.1093/ehjci/jeab029
Huang, W., Liu, H., Zhu, S., Woodson, M., Liu, R., Tilton, R., et al. (2017). Sirt6 deficiency results in progression of glomerular injury in the kidney. Aging 9, 1069–1083. doi:10.18632/aging.101214
Humphreys, B. (2018). Mechanisms of renal fibrosis. Annu. Rev. Physiol. 80, 309–326. doi:10.1146/annurev-physiol-022516-034227
Hwang, J., Lee, S., Choi, J., Shin, K., Choi, C., and Kang, S. (2013). SIRT1 negatively regulates the protein stability of HIPK2. Biochem. Biophys. Res. Commun. 441, 799–804. doi:10.1016/j.bbrc.2013.10.133
Hyndman, K., and Griffin, M. (2021). Could NAD precursor supplements induce a legacy of protection against diabetic nephropathy? J. Am. Soc. Nephrol. 32, 1270–1273. doi:10.1681/asn.2021020275
Jin, J., Li, W., Wang, T., Park, B., Park, S., and Kang, K. (2022). Loss of proximal tubular sirtuin 6 aggravates unilateral ureteral obstruction-induced tubulointerstitial inflammation and fibrosis by regulation of β-catenin acetylation. Cells 11, 1477. doi:10.3390/cells11091477
Jin, Y., Ratnam, K., Chuang, P., Fan, Y., Zhong, Y., Dai, Y., et al. (2012). A systems approach identifies HIPK2 as a key regulator of kidney fibrosis. Nat. Med. 18, 580–588. doi:10.1038/nm.2685
Katsyuba, E., Mottis, A., Zietak, M., De Franco, F., van der Velpen, V., Gariani, K., et al. (2018). De novo NAD+ synthesis enhances mitochondrial function and improves health. Nature 563, 354–359. doi:10.1038/s41586-018-0645-6
Katsyuba, E., Romani, M., Hofer, D., and Auwerx, J. (2020). NAD+ homeostasis in health and disease. Nat. Metab. 2, 9–31. doi:10.1038/s42255-019-0161-5
Kim, H., Ryoo, G., Jang, H., Rah, S., Lee, D., Kim, D., et al. (2022). NAD+-boosting molecules suppress mast cell degranulation and anaphylactic responses in mice. Theranostics 12, 3316–3328. doi:10.7150/thno.69684
Li, W., Feng, W., Su, X., Luo, D., Li, Z., Zhou, Y., et al. (2022). SIRT6 protects vascular smooth muscle cells from osteogenic transdifferentiation via Runx2 in chronic kidney disease. J. Clin. Invest. 132, e150051. doi:10.1172/jci150051
Li, Y., Liu, M., Song, X., Zheng, X., Yi, J., Liu, D., et al. (2020). Exogenous hydrogen sulfide ameliorates diabetic myocardial fibrosis by inhibiting cell aging through SIRT6/AMPK autophagy. Front. Pharmacol. 11, 1150. doi:10.3389/fphar.2020.01150
Li, Z., Xu, K., Zhang, N., Amador, G., Wang, Y., Zhao, S., et al. (2018). Overexpressed SIRT6 attenuates cisplatin-induced acute kidney injury by inhibiting ERK1/2 signaling. Kidney Int. 93, 881–892. doi:10.1016/j.kint.2017.10.021
Liu, R., Das, B., Xiao, W., Li, Z., Li, H., Lee, K., et al. (2017). βA novel inhibitor of homeodomain interacting protein kinase 2 mitigates kidney fibrosis through inhibition of the TGF-1/smad3 pathway. J. Am. Soc. Nephrol. 28, 2133–2143. doi:10.1681/asn.2016080841
Liu, X., Luo, D., Huang, S., Liu, S., Zhang, B., Wang, F., et al. (2021). Impaired nicotinamide adenine dinucleotide biosynthesis in the kidney of chronic kidney disease. Front. Physiol. 12, 723690. doi:10.3389/fphys.2021.723690
Liu, Y., Bi, X., Xiong, J., Han, W., Xiao, T., Xu, X., et al. (2019). MicroRNA-34a promotes renal fibrosis by downregulation of klotho in tubular epithelial cells. Mol. Ther. 27, 1051–1065. doi:10.1016/j.ymthe.2019.02.009
Livingston, M., Ding, H., Huang, S., Hill, J., Yin, X., and Dong, Z. (2016). Persistent activation of autophagy in kidney tubular cells promotes renal interstitial fibrosis during unilateral ureteral obstruction. Autophagy 12, 976–998. doi:10.1080/15548627.2016.1166317
Lovisa, S., Zeisberg, M., and Kalluri, R. (2016). Partial epithelial-to-mesenchymal transition and other new mechanisms of kidney fibrosis. Trends Endocrinol. Metab. 27, 681–695. doi:10.1016/j.tem.2016.06.004
Luo, C., Zhou, S., Zhou, Z., Liu, Y., Yang, L., Liu, J., et al. (2018). Wnt9a promotes renal fibrosis by accelerating cellular senescence in tubular epithelial cells. J. Am. Soc. Nephrol. 29, 1238–1256. doi:10.1681/asn.2017050574
Maccubbin, D., Tipping, D., Kuznetsova, O., Hanlon, W., and Bostom, A. (2010). Hypophosphatemic effect of niacin in patients without renal failure: A randomized trial. Clin. J. Am. Soc. Nephrol. 5, 582–589. doi:10.2215/cjn.07341009
Maity, S., Muhamed, J., Sarikhani, M., Kumar, S., Ahamed, F., Spurthi, K., et al. (2020). Sirtuin 6 deficiency transcriptionally up-regulates TGF-β signaling and induces fibrosis in mice. J. Biol. Chem. 295, 415–434. doi:10.1074/jbc.RA118.007212
Malhotra, R., Katz, R., Hoofnagle, A., Bostom, A., Rifkin, D., Mcbride, R., et al. (2018). The effect of extended release niacin on markers of mineral metabolism in CKD. Clin. J. Am. Soc. Nephrol. 13, 36–44. doi:10.2215/cjn.05440517
Manrique-Caballero, C., Kellum, J., Gómez, H., De Franco, F., Giacchè, N., and Pellicciari, R. (2021). Innovations and emerging therapies to combat renal cell damage: NAD+ as a drug target. Antioxid. Redox Signal. 35, 1449–1466. doi:10.1089/ars.2020.8066
Meng, X., Nikolic-Paterson, D., and Lan, H. (2016). TGF-Β: The master regulator of fibrosis. Nat. Rev. Nephrol. 12, 325–338. doi:10.1038/nrneph.2016.48
Montserrat-de la Paz, S., Naranjo, M., Millan-Linares, M., Lopez, S., Abia, R., Biessen, E., et al. (2019). Monounsaturated fatty acids in a high-fat diet and niacin protect from white fat dysfunction in the metabolic syndrome. Mol. Nutr. Food Res. 63, e1900425. doi:10.1002/mnfr.201900425
Müller, D., Mehling, H., Otto, B., Bergmann-Lips, R., Luft, F., Jordan, J., et al. (2007). Niacin lowers serum phosphate and increases HDL cholesterol in dialysis patients. Clin. J. Am. Soc. Nephrol. 2, 1249–1254. doi:10.2215/cjn.01470307
Muraoka, H., Hasegawa, K., Sakamaki, Y., Minakuchi, H., Kawaguchi, T., Yasuda, I., et al. (2019). Role of nampt-sirt6 Axis in renal proximal tubules in extracellular matrix deposition in diabetic nephropathy. Cell Rep. 27, 199–212.e5. doi:10.1016/j.celrep.2019.03.024
Nettleton, J., Steffen, L., Mayer-Davis, E., Jenny, N., Jiang, R., Herrington, D., et al. (2006). Dietary patterns are associated with biochemical markers of inflammation and endothelial activation in the Multi-Ethnic Study of Atherosclerosis (MESA). Am. J. Clin. Nutr. 83, 1369–1379. doi:10.1093/ajcn/83.6.1369
Pillai, V., Samant, S., Hund, S., Gupta, M., and Gupta, M. (2021). The nuclear sirtuin SIRT6 protects the heart from developing aging-associated myocyte senescence and cardiac hypertrophy. Aging 13, 12334–12358. doi:10.18632/aging.203027
Pirinen, E., Auranen, M., Khan, N., Brilhante, V., Urho, N., Pessia, A., et al. (2020). Niacin cures systemic NAD+ deficiency and improves muscle performance in adult-onset mitochondrial myopathy. Cell Metab. 31, 1078–1090.e5. doi:10.1016/j.cmet.2020.04.008
Ru, M., Wang, W., Zhai, Z., Wang, R., Li, Y., Liang, J., et al. (2022). Nicotinamide mononucleotide supplementation protects the intestinal function in aging mice and D-galactose induced senescent cells. Food Funct. 13, 7507–7519. doi:10.1039/d2fo00525e
Ruiz-Ortega, M., Rayego-Mateos, S., Lamas, S., Ortiz, A., and Rodrigues-Diez, R. (2020). Targeting the progression of chronic kidney disease. Nat. Rev. Nephrol. 16, 269–288. doi:10.1038/s41581-019-0248-y
Streja, E., Kovesdy, C., Streja, D., Moradi, H., Kalantar-Zadeh, K., and Kashyap, M. (2015). Niacin and progression of CKD. Am. J. Kidney Dis. 65, 785–798. doi:10.1053/j.ajkd.2014.11.033
Tian, K., Chen, P., Liu, Z., Si, S., Zhang, Q., Mou, Y., et al. (2017). Sirtuin 6 inhibits epithelial to mesenchymal transition during idiopathic pulmonary fibrosis via inactivating TGF-β1/Smad3 signaling. Oncotarget 8, 61011–61024. doi:10.18632/oncotarget.17723
Wook Choi, D., and Yong Choi, C. (2014). HIPK2 modification code for cell death and survival. Mol. Cell. Oncol. 1, e955999. doi:10.1080/23723548.2014.955999
Wu, B., Chen, K., Barter, P., and Rye, K. (2012). Niacin inhibits vascular inflammation via the induction of heme oxygenase-1. Circulation 125, 150–158. doi:10.1161/circulationaha.111.053108
Xavier, S., Vasko, R., Matsumoto, K., Zullo, J., Chen, R., Maizel, J., et al. (2015). Curtailing endothelial TGF-β signaling is sufficient to reduce endothelial-mesenchymal transition and fibrosis in CKD. J. Am. Soc. Nephrol. 26, 817–829. doi:10.1681/asn.2013101137
Xiao, W., E, J., Bao, L., Fan, Y., Jin, Y., Wang, A., et al. (2020). Tubular HIPK2 is a key contributor to renal fibrosis. JCI insight 5, e136004. doi:10.1172/jci.insight.136004
Xu, F., Mao, B., Li, Y., and Zhao, Y. (2022). Knockdown of HIPK2 attenuates angiotensin II-induced cardiac fibrosis in cardiac fibroblasts. J. Cardiovasc. Pharmacol. 80, 125–131. doi:10.1097/fjc.0000000000001292
Xu, L., Li, X., Zhang, F., Wu, L., Dong, Z., and Zhang, D. (2019). EGFR drives the progression of AKI to CKD through HIPK2 overexpression. Theranostics 9, 2712–2726. doi:10.7150/thno.31424
Yang, Q., Hu, J., Yang, Y., Chen, Z., Feng, J., Zhu, Z., et al. (2020). Sirt6 deficiency aggravates angiotensin II-induced cholesterol accumulation and injury in podocytes. Theranostics 10, 7465–7479. doi:10.7150/thno.45003
Yang, X., Feng, J., Liang, W., Zhu, Z., Chen, Z., Hu, J., et al. (2021). Roles of SIRT6 in kidney disease: A novel therapeutic target. Cell. Mol. Life Sci. 79, 53. doi:10.1007/s00018-021-04061-9
Zhang, J., Li, Y., Liu, Q., Huang, Y., Li, R., Wu, T., et al. (2021). Sirt6 alleviated liver fibrosis by deacetylating conserved lysine 54 on Smad2 in hepatic stellate cells. Hepatology 73, 1140–1157. doi:10.1002/hep.31418
Zhang, Y., Wang, L., Meng, L., Cao, G., and Wu, Y. (2019). Sirtuin 6 overexpression relieves sepsis-induced acute kidney injury by promoting autophagy. Cell cycle 18, 425–436. doi:10.1080/15384101.2019.1568746
Zheng, M., Cai, J., Liu, Z., Shu, S., Wang, Y., Tang, C., et al. (2019). Nicotinamide reduces renal interstitial fibrosis by suppressing tubular injury and inflammation. J. Cell. Mol. Med. 23, 3995–4004. doi:10.1111/jcmm.14285
Keywords: SIRT6, HIPK2, renal interstitial fibrosis, chronic kidney disease, vitamin B3, renal function
Citation: Li X, Li W, Zhang Z, Wang W and Huang H (2022) SIRT6 overexpression retards renal interstitial fibrosis through targeting HIPK2 in chronic kidney disease. Front. Pharmacol. 13:1007168. doi: 10.3389/fphar.2022.1007168
Received: 30 July 2022; Accepted: 22 August 2022;
Published: 12 September 2022.
Edited by:
Youhua Liu, University of Pittsburgh, United StatesReviewed by:
Zhanjun Jia, Nanjing Medical University, ChinaJohn Cijiang He, Icahn School of Medicine at Mount Sinai, United States
Copyright © 2022 Li, Li, Zhang, Wang and Huang. This is an open-access article distributed under the terms of the Creative Commons Attribution License (CC BY). The use, distribution or reproduction in other forums is permitted, provided the original author(s) and the copyright owner(s) are credited and that the original publication in this journal is cited, in accordance with accepted academic practice. No use, distribution or reproduction is permitted which does not comply with these terms.
*Correspondence: Hui Huang, aHVhbmdoOEBtYWlsLnN5c3UuZWR1LmNu,