- 1Department of Traditional Chinese Medicine Resource and Bee Products, College of Animal Sciences (College of Bee Science), Fujian Agriculture and Forestry University, Fuzhou, China
- 2State and Local Joint Engineering Laboratory of Natural Biotoxins, Fujian Agriculture and Forestry University, Fuzhou, China
- 3Department of Pharmaceutical Analysis, School of Pharmacy, Fujian Medical University, Fuzhou, China
Bee venom (BV), a type of defensive venom, has been confirmed to have favorable activities, such as anti-tumor, neuroprotective, anti-inflammatory, analgesic, anti-infectivity effects, etc. This study reviewed the recent progress on the pharmacological effects and mechanisms of BV and its main components against cancer, neurological disorders, inflammatory diseases, pain, microbial diseases, liver, kidney, lung and muscle injury, and other diseases in literature during the years 2018–2021. The related target proteins of BV and its main components against the diseases include Akt, mTOR, JNK, Wnt-5α, HIF-1α, NF-κB, JAK2, Nrf2, BDNF, Smad2/3, AMPK, and so on, which are referring to PI3K/Akt/mTOR, MAPK, Wnt/β-catenin, HIF-1α, NF-κB, JAK/STAT, Nrf2/HO-1, TrkB/CREB/BDNF, TGF-β/Smad2/3, and AMPK signaling pathways, etc. Further, with the reported targets, the potential effects and mechanisms on diseases were bioinformatically predicted via Kyoto Encyclopedia of Genes and Genomes (KEGG) pathway, disease ontology semantic and enrichment (DOSE) and protein-protein interaction (PPI) analyses. This review provides new insights into the therapeutic effects and mechanisms of BV and its main components on diseases.
Introduction
Under the dual selection of natural selection and genetic evolution, the animal kingdom has long evolved a unique defensive venom system (Casewell et al., 2013). Bee venom (BV), a type of defensive venom, is generated in the bee’s venom glands and stored in the abdominal poison sac. (e.g., Apis mellifera) (Aufschnaiter et al., 2020). BV is a clear liquid with bitter taste, strong fragrance, pH value at 4.5–5.5, and specific gravity of 1.13, which is prone to volatilize and crystallize in the air (Khalil et al., 2021). BV contains smaller proteins, peptides and enzymes such as melittin (MEL), apamin, phospholipase A2 (PLA2) and other components referring to amines, sugars and minerals (Aufschnaiter et al., 2020; Khalil et al., 2021).
Based on these active components, BV has multiple diverse pharmacological effects. Some reviews have retrieved the pharmacological progress on one or a few aspects of BV, mainly referring to anti-tumor (Dutta et al., 2019; Mirzaei et al., 2021), neuroprotective (El-Seedi H. R. et al., 2020), anti-inflammatory (Dutta et al., 2019), analgesic (Kim and Han, 2020), anti-infectivity effects (El-Seedi H. et al., 2020), improving wound healing (Kurek-Gorecka et al., 2021), and other effects. Recently, Khalil et al. (2021) also summarized the therapeutic effects of BV in treatment of cancers, multiple sclerosis, dementia, osteoarthritis, rheumatoid arthritis (RA), and wounds, etc. These demonstrates that BV has a wide range of clinical applications could be attributed to its multi-target and multi-pathway characteristics. However, so far, there is still a lack of comprehensive and systematic pharmacological analysis of BV with multiple targets and pathways, which is unbeneficial to understanding the integrative pharmacological effect and mechanism of BV and its main components on diseases.
In the past 10 years, bioinformatic analyses, e.g., Encyclopedia of Genes and Genomes (KEGG) pathway, disease ontology semantic and enrichment (DOSE) and protein-protein interaction (PPI) analyses, etc., have been widely used in the investigation fields of genomics and proteomics, due to that they can comprehensively discover the biological mysteries of large and complex biological data accounting for physiological and pathological alternations of organism, or changes of organism in response to external stimuli (Yu et al., 2015; Wen et al., 2022). For the bioinformatic analyses, differentially expressed miRNAs (differentially expressed genes (DEGs) or differentially expressed proteins (DEPs) from omics experiments are screened firstly, and KEGG and disease ontology (DO) databases can then be called online by R language platform with the screened DEGs or DEPs to identify enriched pathways and related diseases usually using a two-tailed Fisher’s exact test. Meanwhile, all DEGs or DEPs can be searched against the STRING database for protein-protein interactions and can be visualized in R package to predict the key hub targets (genes or proteins). At present, by means of these bioinformatic ideas and tools, the potential therapeutic effects and mechanisms of several natural active ingredients, such as ginsenoside Rb1, Re and Tanshinone IIA have been analyzed systematically and deeply through mining their reported targets and pathways from literature, which indeed provide a lot of inspiration and clues for the future study of these ingredients (Zhong et al., 2021; Cai et al., 2022; Lin et al., 2022). Reasonably, with the help of bioinformatic tools, it should also be able to comprehensively understand the therapeutic effects and potential targets and mechanisms of the main ingredients in BV by mining their reported targets and pathways from previous reports.
Hence, in this paper, articles published from 2018 to 2021 and archived in Web of Science and PubMed databases were searched mainly using the keywords “bee venom and pharmacology,” supplemented with the keywords “bee venom and cancer” and “melittin and cancer,” and the duplicate articles were excluded. Based on these articles, we reviewed the current progress mainly from year 2018–2021 on the investigation of pharmacological effects and mechanisms of BV and its main components, mainly MEL, bvPLA2 and apamin. The reported action targets and pathways of them against cancer, neurological disorders, inflammatory diseases, pain, microbial diseases, liver, kidney, lung and muscle injury, and other diseases were summarized. Further, the possible anti-ill mechanisms of BV and its main components were comprehensively and systematically studied through DOSE, KEGG pathway, and PPI analyses according to the reported targets. The present study has deeply understood the pharmacological effects and mechanisms of BV and its main components against ills, which will help to promote the development and clinical application for BV.
Main components of bee venom
MEL, bvPLA2 and apamin are three main components in BV, which are the important material basis for BV to exert its pharmacological effects, and their structures are shown in Figure 1.
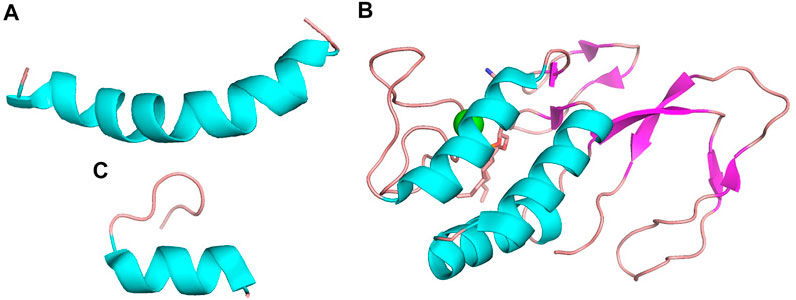
FIGURE 1. The structures of (A) MEL (PDB ID 6dst), (B) bvPLA2 (PDB ID 1poc) and (C) apamin (PDB ID 7oxf). Structure (A) and (C) appear to be dominated by α-helix while structure (B) is dominated by a combination of α-helix and β-pleated sheet.
Melittin
MEL is the dominant component, which is consisting of 26-residue peptide and representing about 40%–60% BV’s dry weight (Wehbe et al., 2019). The carboxyl terminal of MEL contains positively charged amino acids, while the amino terminal is hydrophobic. Therefore, it contains both hydrophilic and hydrophobic properties. Both the MEL molecules and the membrane-bound MEL are through α spirally connected (Raghuraman and Chattopadhyay, 2007). Apart from its non-specific biofilm dissolution characteristics (Carpena et al., 2020), it has significant antibacterial, anti-tumor, and other effects (Pashaei et al., 2019; Yu et al., 2020).
Bee venom phospholipase A2
PLA2 is a 128 amino acids single polypeptide chain containing four disulfide bridges. The bvPLA2 pertains to the group III secretory PLA2 (sPLA2) enzymes, accounting for 12%–15% of BV’s dry weight (Wehbe et al., 2019; Carpena et al., 2020). It hydrolyzes the sn-2 fatty acyl ester bond of membrane glycerol-3-phospholipids to liberate fatty acids and lysophospholipids, and this catalytic activity disrupts cell membranes, contributing to its anti-tumor, anti-infectivity, and other effects (Putz et al., 2007; Carpena et al., 2020). Besides, the abundant amino acids in bvPLA2, leucine and lysine, promotes the phenomenon of neurotoxicity (Pattabhiramaiah et al., 2020).
Apamin
Apamin, an 18 amino acid peptide, makes up 2%–3% of its total dry weight (Gu et al., 2020). It is formed by a disulfide bond between two cysteines, which shapes its highly stable and compact chemical structure (Nguyen et al., 2015). Apamin has demonstrated the potential benefits in anti-atherosclerosis, anti-heart failure, and improvement of neurological disorders (Gu et al., 2020).
Anticancer effects
The incidence rate of cancer, the most serious cause of death, is constantly testing the global medical system’s coping and resolving ability (Sung et al., 2021). The morbidity of many cancers, e.g., lung cancer, breast cancer, and colorectal cancer is still high, and the exploration of various forms, approaches and strategies of cancer treatment is still serious (Siegel et al., 2021). Animal-derived venoms are rich in a large number of active proteins and enzymes and have potential anticancer activities (Ejaz et al., 2018). As a promising natural product, BV and its main component MEL can regulate the cell cycle, change the permeability of cell membrane, inhibit the proliferation and migration, and promote endogenous/exogenous apoptosis and autophagy and other regulatory cell death modes to promote cell death (Mirzaei et al., 2021). Thus, it shows potential in strategies for inhibiting the occurrence and development of cancer and tumor (Wehbe et al., 2019; Carpena et al., 2020), as shown in Table 1, and the main affected targets and pathways in anticancer effects of BV is shown in Figure 2.
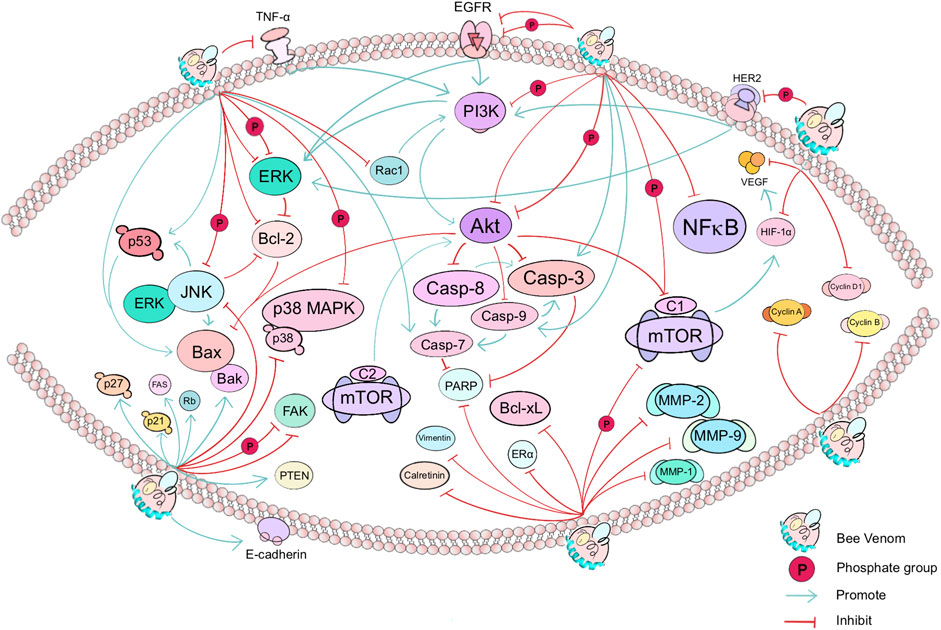
FIGURE 2. The main affected targets and pathways in anticancer effects of BV. In cancer, BV mainly affects the PI3K/Akt/mTOR pathway (e.g., PI3K, Akt, and mTOR), apoptosis signaling pathway (e.g., EGFR and TNF-α, including downstream effectors such as Casp-3, Casp-7, Casp-8, Casp-9, Bcl-2, Bax and Bcl-xL), p38 MAPK pathway, and thus affect the growth, differentiation, invasion, autophagy or migration of cancer cells in lung, breast, cervical and other cancers. Green arrows or red cut-off lines represent the “promote” or “inhibit” effect of the target (gene or protein) by the upstream target factor, respectively. Bee Venom, known as BV, is dispersed on the surface of a phospholipid bilayer. The text shows the direct or indirect targets of BV.
Anti-lung cancer research
In 2020, Lung cancer became the second most common malignancy worldwide with an incidence rate of 11.4% (Sung et al., 2021), mainly non-small cell lung cancer, occupying 80% of all new lung cancer cases (Sugarbaker and Dasilva, 2011). BV could inhibit epithelial-mesenchymal transition (EMT), increase the expression of vimentin, down-regulate the E-cadherin expression, and inhibit the recombination of F-actin related to the tumor metastasis in lung cancer A549, H1739 and H23 cells induced by epidermal growth factor (EGF). In A549 cells, BV decreased the phosphorylation of extracellular-regulated kinase (ERK), c-Jun N-terminal kinase (JNK), focal adhesion kinase (FAK) and mammalian target of rapamycin (mTOR), and eventually provoked the decrease of the transcription factors zinc finger E-box-binding homeobox 2 (ZEB2) and Slug in the EMT, suggesting its potential function in anti-cancer cell metastasis (Jeong et al., 2019). At the same time, as the main component of BV, MEL had a blockade effect on transforming growth factor-β (TGF-β), ERK and phosphorylated ERK in the ERK signaling pathway, resulting in the synthesis of Caspase-3 and Apaf-1 proteins that promoted apoptosis in A549 cells, and the cell growth, migration, invasion and other activities were blocked (Yu et al., 2020). Similarly, MEL also increased the apoptotic ratio in ChaGo-K1 of lung cancer, and the expression of mitogen activating protein-kinase activating death domain (MADD) decreased, which further brought about cell cycle arrest in G0/G1 phase (Tipgomut et al., 2018).
After cisplatin-resistant lung cancer cells A549/DDP were cultured in vitro and treated with MEL, the Warburg effect as well as phosphorylated protein kinase B (Akt) were suppressed; after vaccinating A549/DDP into Balb/c athymic nude mice and treating them via intraperitoneal (i.p.) injection of MEL, their tumor and cell sensitivity to cisplatin was enhanced and tumor size and mass were controlled (Zhang et al., 2021). In addition, the miR-183 played a role as a tumor marker of lung cancer, and was inhibited by MEL in NCI-H441 cells. Its inhibition further increased the expression of Caspase-2 and Bcl-2-associated X protein (Bax), and reduced the Bcl-2 expression. Not only that, after subcutaneous (s.c.) injection of MEL in Balb/c nu/nu mice, Caspase-2 was elevated and tumor growth was restricted similarly (Gao et al., 2018).
Besides, MEL-carried nanoparticles (NPs) systems have considerably enhanced the security of MEL in vivo and its efficacy against tumors, offering the possibility of tumor elimination (Zhou et al., 2021). Such drug nano-delivery platforms have been observed in lung cancer research. MEL-carried zeolitic imidazolate framework-8 (MLT@ZIF-8) NPs (Li et al., 2018) and lipid-coated polymer NP (MpG@LPN) (Ye et al., 2021) increased apoptosis in A549 cells and inhibited tumor growth. In the meanwhile, the cellular hemolysis caused by piggybacking on these two nanomaterials was reduced to a certain extent compared with MEL alone.
Anti-breast cancer research
Breast cancer, as the most commonplace tumor in female population, has a very strong time variant tumor metastasis and spatial heterogeneity associated with genotype and phenotypic differences, leading to a continuous change in the evaluation and treatment process of breast cancer (Fumagalli and Barberis, 2021).
BV and MEL induced strongly selective cell death in triple-negative breast carcinoma and human epidermal growth factor receptor 2 (HER2) enriched breast cancer with little effect in routine cells, through interfering with growth factor-dependent receptor tyrosine kinase interactions critical for receptor phosphorylation and activation of phosphoinositide 3-kinase (PI3K)/Akt and mitogen-activated protein kinase (MAPK) signaling. Besides, in an allograft model, the effect of docetaxel in suppressing breast tumor growth was potentiated by the administration of MEL, and the programmed death ligand-1 (PD-L1) protein expression, phosphorylated HER2 and epidermal growth factor receptor (p-EGFR), were significantly reduced (Duffy et al., 2020). Researchers also investigated the effect of BV on epigenetic changes in cancer cells: after confirming that BV decreased the viability and mitochondrial membrane permeability of MDA-MB-231 cells, they further inspected epigenetic and mitochondrial DNA (mtDNA) Copy Number Variation (CNV). The experimental results revealed that BV generated morphological changes in the nucleus of MDA-MB-231 cells, and the exploration of cytosine modification in cancer cells showed that 5′-methylcytosine (5meC), 5′-hydroxymethylcytosine (5hmC) cells decreased rapidly after treatment with BV in MDA-MB-231 cells. And 5′-formlylcytosine (5 fC) and 5′-carboxycytosine (5caC) exhibited a similar increasing and then decreasing process (Uzuner et al., 2021).
BV reduced the expression of nuclear translocation of nuclear factor-κB (NF-κB) and Cyclin D1, enhanced H2O2 production, blocked G1 cycle and inhibited breast cancer proliferation in MCF-7 cells (Yoon et al., 2018). MEL reduced the viability of 4T1 and MCF-7 cell lines, and the addition of irradiation resulted in a significant increase of Bax/Bcl-2 value. Besides, MEL enhanced tumor radiosensitivity and inhibited the tumor growth in 4T1 tumor-bearing mice (Chang et al., 2020). MEL also showed the potential to promote mitofusin-1 (Mfn1) and dynamin-related protein 1 (Drp1) expression and apoptosis in 4T1 cells (Moghaddam et al., 2020). After 5% O2 induction in MDA-MB-231 cells, the hypoxia-inducible factor-1α (HIF-1α) signaling pathway was inhibited by MEL and the expression of NF-κB, HIF-1α, vascular endothelial growth factor A (VEGFA) and lactate dehydrogenase A (LDHA) decreased, while the expression of Bax and tumor necrosis factor-α (TNF-α) was reversed, ultimately disrupting the tumor microenvironment (TME) of cancer cells and activating the phenomenon of apoptosis (Mir Hassani et al., 2021).
BV alone and in combination with other drugs or solutions had an anti-breast cancer impact (Khamis et al., 2018; Arani et al., 2019; El-Beltagy et al., 2021). In vitro, BV inhibited the growth of MCF7 and T47D cells, while blocking the cell cycle in the G2/M phase. BV alone or in combination with tamoxifen, hesperidin, and piperine resulted in reduced expression of Bcl-2, EGFR, and estrogen receptors α (ERα) receptors, and elevated expression of Bax, which eventually led to apoptosis. The anticancer and anti-drug resistance effects of tamoxifen were enhanced by the synergistic effect of BV (Khamis et al., 2018). In vivo, N-methylnitrosourea was able to induce breast cancer and ovarian complications in Wistar albino rats. After the combination of BV and Annona muricata fruit, serum levels of matrix metalloproteinase-1 (MMP-1), NF-κB, TNF-α, malondialdehyde (MDA), elevated Caspase-3, superoxide dismutase (SOD), catalase (CAT), and ovarian histopathological changes due to mammary carcinoma were improved in mothers and offspring rats. Additionally, calreticulin and p53 protein response in the ovarian stroma switched from positive to negative (El-Beltagy et al., 2021). In addition, the non-specific cytotoxicity of MEL in the clinical setting cannot be conveniently ignored. However, plasma-treated phosphate buffered saline solution can cause the death of MCF-7 and A375 melanoma cells on the one hand and circumvent the non-specific cytotoxicity of MEL to a certain extent on the other, revealing the value of the combination therapy (Shaw et al., 2019).
The application of nanomaterials in combination with MEL has also yielded beneficial results in the treatment of breast carcinoma. The disruption of cell membrane by MEL was unaffected by loading in the NPs after carrying the MEL, and its effect on causing necrosis or apoptosis of the tumor or cancer cells remained undisturbed, such as folic acid (FA)-polyelectrolyte nanocarriers (PENs) (Motiei et al., 2021), nanographene oxide (nGO) and graphene (GN) (Daniluk et al., 2020), the niosome (Moghaddam et al., 2021), citrate functionalized Fe3O4 magnetic NPs (CA-MNPs) (Hematyar et al., 2018), and activatable protein NPs (APNPs) (Yu et al., 2018). Besides, after carrying MEL in poly-ion complex (PIC) added with estrone, it prevented the degradation of MEL in cells and increased the uptake of MEL and cytotoxicity (Raveendran et al., 2020).
Anti-cervical cancer research
Cervical cancer is one of the most common cancers in the global female population (Volkova et al., 2021). BV demonstrated the ability to inhibit the growth and migration of HPV-positive cervical cancer Caski and HeLa cells, and cell cycle protein Cyclin A and Cyclin B, Akt, JNK and p38/44/42 and their phosphorylated proteins associated with mitogenic signaling pathways were inhibited, and pro-Caspase-3, pro-Caspase-9, cleaved polyadenosine-diphosphate-ribose polymerase (PARP), Bcl-2 and Bcl-xL expression was reduced. On the contrary, proteins such as p53, p21, and retinoblastoma (Rb) were upregulated in expression with the utility of BV, and the number of dead and apoptotic cells was significantly promoted (Kim D. H. et al., 2020). It is worth noting that the main mode of death of HeLa cells after BV treatment is apoptosis, which causes severe cell membrane damage and cell shrinkage (Borojeni et al., 2020), while MEL can show the effect of inhibiting HeLa cell proliferation and inducing apoptosis such as cell shrinkage and structural disorganization (Zarrinnahad et al., 2018).
Graphene oxide magnetic nanocomposites (PEG-GO-Fe3O4)/MEL complexes caused time-dependent toxic effects on HeLa cells with deformation lysis, membrane breakage and other abnormal cellular states. The experimental results demonstrated that this material achieved the long-lasting release and effect enhancement of MEL, while preventing the degradation or denaturation of MEL, ensuring the anti-cervical cancer effect of MEL (Qi et al., 2020). BV loaded on nano-fungal chitosan (NFC) also showed effective anticancer activity in promoting apoptosis in HeLa cells (Alalawy et al., 2020).
Anti-pancreatic cancer, gastric cancer, and colorectal cancer research
BV and MEL have also been used to treat cancers of the digestive system, such as pancreatic cancer, gastric cancer, and colorectal cancer.
In pancreatic ductal adenocarcinoma (PDAC), overexpression of NONHSAT105177 in long non-coding RNAs is associated with activities such as cell proliferation and migration (Wang et al., 2018). This RNA is able to increase its expression under the regulation of MEL, further promoting its inhibitory effect on PDAC, which is related to EMT pathway-related proteins, such as causing the repressive expression of Snail, Slug and vimentin and the up-regulated expression of E-cadherin.
MEL exhibited inhibitory effects on human gastric cancer AGS cell viability, adhesion, colony-forming ability, EMT, and a limiting effect on MMP-2, MMP-9 and MMP-13 proteins related to cell migration and invasion ability. In addition, MEL tended to act more in a variety of signaling pathways, containing bone morphogenetic protein (BMP)/Smad, Wnt/β-catenin and PI3K/Akt pathways (Huang et al., 2021).
When BV and MEL were applied to HCT-116 and SW-480 of colorectal cancer cells, respectively, the mitochondrial apoptotic pathway was activated, cancer cell viability was reduced, chromatin was contracted, and apoptosis was induced in early and late colorectal cancer cells. The expression of Caspase-9 and Fas death receptor increased, however, CYP1A1 and GSTP1, Bcl-2 decreased in the same trend in both cells; while the mRNA expression of Bax and multidrug resistance protein-2 (MRP-2) increased when BV treated HCT-116 cells and decreased when BV treated SW-480 cells. After MEL treatment, the expression of Bax decreased and MRP-2 increased in HCT-116 cells, while the expression of Bax and MRP-2 decreased in SW-480 cells (Nikodijevic et al., 2021). Besides, the high concentration of MEL could directly and quickly cause membrane damage, content outflow and cell death to gastric cancer and colorectal cancer cell membranes within 15 min. This rapid dissolution effect appeared in AGS cells, COLO205 and HCT-15 cells in different ways (Soliman et al., 2019). In addition, MEL and bvPLA2 inhibited HCT116 cell proliferation in a synergistic manner, demonstrating synergistic utility: MEL promoted the effect of bvPLA2 on cell membranes, and pretreatment of cells with bvPLA2 enhanced the inhibitory effect of MEL on cells (Yaacoub et al., 2021). Besides, the expression and activity of 15-lipoxygenase-1, a tumor suppressor in HT-29 cells, have elevated after being affected by BV, which in turn promoted apoptosis (Zare et al., 2019).
Meanwhile, the derivation of the side chain of oligopeptide-alginate NPs provided the basis for the specific binding of MEL, ultimately achieving potent killing ability on human cloned colon adenocarcinoma Caco-2 cells (Wattanakul et al., 2019).
Anti-liver cancer research
In the study of hepatocellular carcinoma, BV achieved the same breakthrough as MEL in anti-hepatocellular carcinoma growth with autophagy, which implied a possible anti-mutagenic effect on normal cells. The results showed that MEL down-regulated Bcl-2 and up-regulated cytochrome C (Cyt C), Caspase-3, and Caspase-9 expression, predicting that MEL may rely on the mitochondrial apoptotic pathway to induce tumor injury, and the ratio of apoptosis to necrosis in cancer cells was positively relative to the MEL concentration. On the other hand, MEL achieved its autophagy-inhibiting effect on HepG2 cells by downregulating p62 and upregulating Beclin 1 and LC3 expression. The anti-tumor effect of MEL was enhanced when the autophagy inhibitor chloroquine was applied; the enhanced autophagic effect of MEL on hepatocellular carcinoma cells was diminished after the application of the autophagy activator rapamycin (Lv et al., 2019). The shaping of hypoxic environment is strongly associated with tumor proliferation or angiogenesis, and the vasculogenic mimicry (VM) produced by SMMC-7721 cells induced by cobalt chloride (CoCl2) with EMT can also be inhibited by applying MEL. The hypoxia model caused upregulation of the expression of HIF-1α, VEGF, MMP-2 and MMP-9 in SMMC-7721, Huh7, and HepG2 cells, and MEL reversed this trend. In addition, in the presence of MEL, it decreased SMMC-7721 cell viability, inhibited EMT induced by CoCl2, upregulated E-cadherin, and downregulated p-Akt, vimentin and N-cadherin expression. An in vivo tumor treatment model of MEL was established by s.c. injecting SMMC-7721 cells into male BALB/c nude mice, which showed the significantly inhibited HIF-1α expression and tumor growth (Chen et al., 2019).
Sorafenib had unsatisfactory effects in the treatment of advanced hepatocellular carcinoma, while BV and MEL had certain efficacy in inhibiting hepatocellular carcinoma. Therefore, BV and MEL alone or in combination with sorafenib, respectively, showed synergistic effects in adjuvant inhibition of HepG2 cell proliferation. The expression of p53, Bax, Caspase-3, Caspase-7 and PTEN was elevated, meanwhile the expression of Bcl-2, Cyclin D1, HIF-1α, VEGF, Ras-related C3 botulinum toxin substrate 1 (Rac1), MMP-9 and NF-κB decreased in HepG2 cells. The promotion or suppression effects on the above genes were strengthened under the crosstalk conditions (Mansour et al., 2021).
Anti-bladder cancer and prostate cancer research
In an investigation of the Gene Expression Omnibus database of bladder cancer, MEL regulated and inhibited the expression of key module genes in the PI3K-Akt and TNF signaling pathways, referring to LPAR1, COL5A1, COL6A2, CXCL1, CXCL2 and CXCL3 in human bladder cancer cell lines T24 and 5637, and suppressed cell proliferation and migration activities, revealing the potential role of these genes as targets of MEL in bladder cancer (Jin et al., 2018). Similarly, bearing in mind the bioinformatics analysis of bladder cancer, the genes corresponding to two bladder cancer cells, UM-UC-3 and 5637, were selected for study. All these demonstrated that MEL could inhibit cell proliferation, migration and invasion by virtue of its effect on MAPK signaling pathway or V-ATPase (Yao et al., 2020).
Prostate cancer is divided into metastatic/non metastatic prostate cancer. As one of the familiar type diseases in masculinity, it faces several problems with drug resistance of cancer cells and inability to control the progress and spread of the disease. BV and MEL have certain effects on a variety of prostate cancer and xenotransplantation (Badawi, 2021). For example, BV produced selective antitumor effects on PC3 cells, reducing their cell viability (Viana et al., 2021).
Anti-skin cancer research
Melanoma stands out as one of the most lethal and invasive malignancies in skin cancer, yet it is highly resistant to drugs. BV and MEL can help to fight against the growth, migration and invasion of melanoma A375SM, B16F10 and SK-MEL-28 cells, causing apoptosis. Among them, MEL showed a more effective ability to inhibit migration and promote apoptosis. BV and MEL had similar inhibitory effects on PI3K/Akt/mTOR and MAPK signaling pathways in A375SM cells. At the same time, it elevated the cleaved Caspase-3 and Caspase-9 expression and reduced the microphthalmia-associated transcription factor (MITF) level. In addition, when MEL was combined with temozolomide, the growth and invasion inhibition of A375SM and SK-MEL-28 cells elevated (Lim et al., 2019). MEL from Apis florea (MEL-AF) similarly showed a proliferation inhibitory effect on A375 cells, where MEL-AF, upon binding to the cell membrane, caused an elevation of intracellular F-actin with a decrease in EGFR, ultimately resulting in apoptosis through the induced expression of Cyt C, Caspase-3 and Caspase-9 in the mitochondrial apoptotic pathway (Sangboonruang et al., 2020).
MEL alone or in combination with 5-fluorouracil was able to damage A431 cells of skin squamous cell carcinomas, causing morphological alternations, e.g., cell shedding, shrinkage, and plasma membrane damage. Besides, the combination of MEL and 5-fluorouracil caused a more significant decrease in terms of cell number and cell cycle arrest in both phases S and G2/M. More importantly, the drug combination re-sensitized A431 cells to 5-fluorouracil (Ombredane et al., 2021).
Head and neck squamous cell carcinoma (HNSCC) is also a type of skin cancer. Four types of HNSCC cells viability such as UMSCC12, UMSCC29, UMSCC38 and UMSCC47 were inhibited by BV alone or combined with cisplatin. Besides, mitosis was blocked in G2/M phase, during which the Bcl-2 and EGFR expression was significantly reduced, while the expression of Bax was significantly elevated. It is worth noting that different drugs and ratios of treatment were shown to significantly reduce the number of the S-phase cells (Grawish et al., 2020).
Anti-leukemia research
MEL induced apoptosis while inhibiting cell viability in CCRF-CEM and K562 cells, relying on activation of the hydrolytic activity of Caspase-3/7 in the mitochondrial pathway and the hemiphilic aspartate pathway (Ceremuga et al., 2020). In addition to inhibiting cell viability, MEL had high permeability to the plasma membrane of cells in human acute T cell leukemia Jurkat cells, which enhanced the permeability of MEL through the plasma membrane and further caused cell death (Gasanoff et al., 2021). The anti-infectivity peptide melectin from Melecta albifrons, by virtue of its α-helical structure, inhibited cell proliferation by interfering with the cell membrane of leukemic cells K562, decreasing the viability of various cells such as K562, K562/ADM and HL-60 while enhancing LDH output (Liang et al., 2021).
Anti-other cancer research
In a study of Hodgkin lymphoma, MEL produced toxicity in lymphoma cells L-428 and KM-H2, while increasing the sensitivity of drug-resistant L-428 cells to cisplatin. And MEL preferentially acted on tumor cells, demonstrating prospect of Hodgkin lymphoma therapy in the future (Kreinest et al., 2021). Besides, Cyclin D, MMP-2, MMP-9, lipoprotein receptor related protein 5 (LRP5), β-catenin and other proteins associated with the Wnt/β-catenin pathway were downregulated after moderate and high concentrations of MEL on human osteosarcoma 143B cells, a malignant bone tumor. The s.c. injection of 143B cells and treatment with MEL in female BALB/cnu/nu nude mice showed a reduction in tumor size, mass and number of lung metastatic nodules, and inhibition of tumor metastatic behavior (Zhu et al., 2021). Glioblastoma multiforme is also a malignant tumor. BV and MEL reduced the viability of Hs683, T98G and U373 cells, elevated Bak and Bax expression, inhibited Caspase-3 expression as well as promoted late apoptosis and necrosis in glioblastoma multiforme. In addition, the expression of long-chain non-coding RNARP11-838N2.4 and X inactive-specific transcript (XIST) was significantly elevated in glioblastoma multiforme cells (Lebel et al., 2021). BV or MEL alone inhibited the growth of Ehrlich ascites carcinoma cells. Injection of BV or MEL into female albino tumor-bearing mice resulted in destruction of tumor tissue and suppression of tumor size. In addition, after combined treatment with γ-radiation, the tumor size inhibition was enhanced by re-enforcing the elevated levels of TNF-α, VEGF-A, serum MMP-2 and MMP-9, and CAT in liver caused by BV or MEL alone (El Bakary et al., 2020).
Lipodisk-based paclitaxel and MEL co-delivery system functionalized with glycopeptide 9G-A7R (9G-A7R-Disk/PTX/MEL) were used as an anti-degradation delivery system for MEL on U87 glioma cells cultured in vitro contributing to the growth inhibitory effect. Besides, inoculation of U87 cells in female BALB/c nude mice and intravenous (i.v.) administration of 9G-A7R-Disk/PTX/MEL co-loaded liposomes resulted in increased apoptosis, tissue damage, and reduced angiogenesis at the glioma, demonstrating their targeted anti-tumor effects (Wang et al., 2019).
Effects on neurological disorders
Parkinson’s disease (PD) and Alzheimer’s disease (AD), belonging to neurodegenerative diseases, are caused by nervous system abnormalities, involving neurotransmitter abnormalities, the accumulation of false proteins, etc. (Guo and Ma, 2019). BV and its main component, bvPLA2, showed neuroprotective effects and could postpone the progression of degenerative diseases. The effects mainly included enhancing motor performance or alleviating memory impairments, inhibiting oxidative stress, decreasing neuroinflammation, protecting neurons, preventing apoptosis, etc. Besides, BV and its main components also had neuroprotective effects against other neurological disorders, seen in Table 2, and the main affected targets and mechanism of BV and its main components in treating neurological disorders is shown in Figure 3.
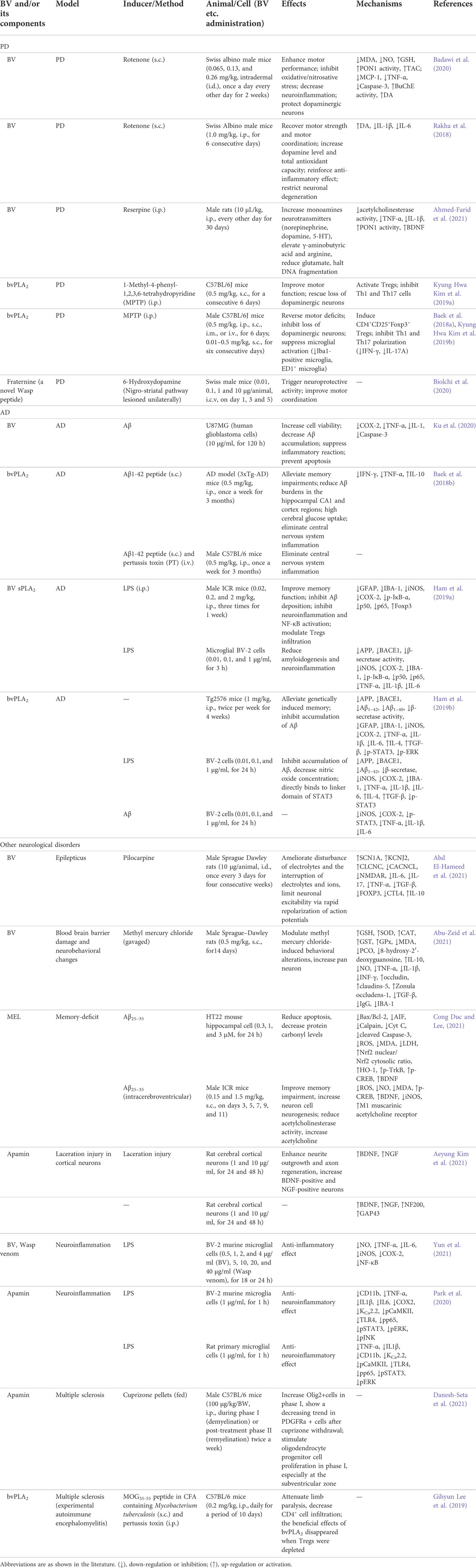
TABLE 2. Summary of the role and mechanism of BV and its main components in treating neurological disorders.
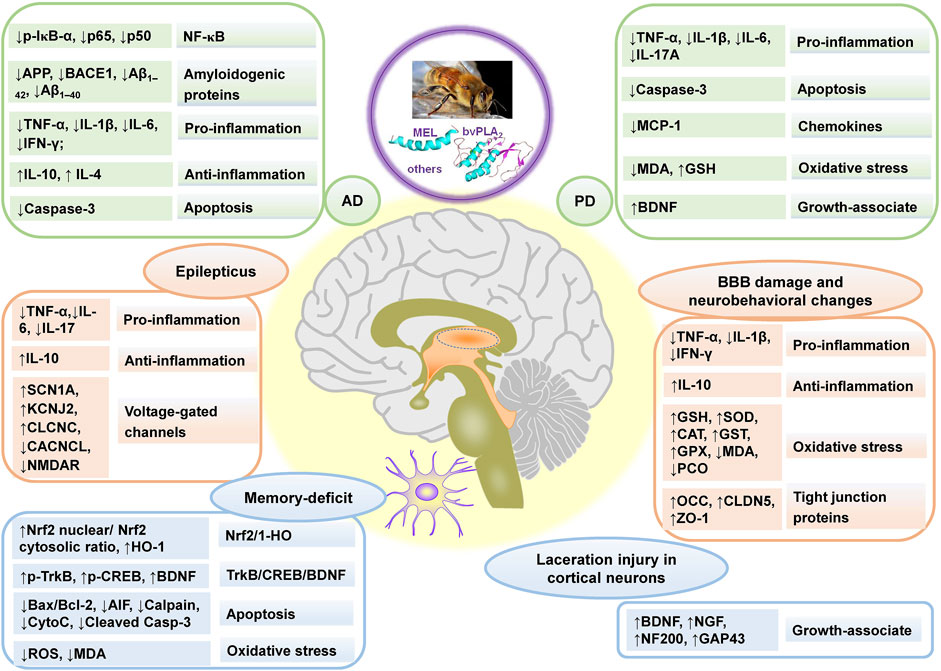
FIGURE 3. The main affected targets and mechanism of BV and its main components in treating neurological disorders, referring to Alzheimer’s disease (AD), Parkinson’s disease (PD), BBB damage and neurobehavioral changes, laceration injury in cortical neurons, memory-deficit, and epilepticus. “↑” and “↓” represent up-regulated and down-regulated targets (genes or proteins), respectively in the left column in each rounded rectangular box, and the texts demonstrate the effect and pathways in right column in each rounded rectangular box for BV and its main components.
Delaying the development of PD
BV was reported to have neuroprotective effect on dopaminergic neurons and alleviate PD symptoms. BV attenuated motor impairment, decreased oxidative/nitrosative stress, and TNF-α, Caspase-3, and monocyte chemoattractant protein-1 (MCP-1) expression, and increased dopamine (DA) content and butyrylcholinesterase (BuChE) activity in a rotenone-induced PD mice model (Badawi et al., 2020). Besides, BV restored the levels of DA, norepinephrine and serotonin (5-HT), balanced glutamate/γ-aminobutyric acid levels, prevented DNA fragmentation, reduced TNF-α and interleukin-1β (IL-1β), and increased the brain-derived neurotrophic factor (BDNF) and paraoxonase 1 (PON1) level in a reserpine-induced PD rat model (Ahmed-Farid et al., 2021). The above results implied that BV could be a potential adjuvant for PD treatment. The neuroprotective effects of bvPLA2 against PD have also been studied. Purified bvPLA2 showed dose-dependent neuroprotective effects on PD in mice, relating to the induction of CD4+CD25+Foxp3+ regulatory T cells (Tregs), which to some extent suppressed the polarization of T helper 1 (Th1) and Th17, and the microglia activation (Kim et al., 2019b). Fraternine, a novel wasp peptide, also showed neuroprotective effects and ameliorated motor coordination in a 6-hydroxydopamine-induced PD mice model (Biolchi et al., 2020).
Besides, current evidence was summarized and supported the therapeutic effects of acupuncture in treating PD patients and animal models of PD (Guo and Ma, 2019). Therefore, BV combined with acupuncture could have great advantages in the treatment of PD.
Delaying the development of AD
BV increased cell viability, decreased amyloid β-protein (Aβ) accumulation in U87MG AD mimic cells, as well as suppressed inflammatory reaction through inhibiting the mRNA expression of IL-1, TNF-α and cyclooxygenase-2 (COX-2), and prevented apoptosis by reducing the expression level of Caspase-3, indicating that BV could be a potential AD therapeutic drug (Ku et al., 2020). Besides, bvPLA2 also exerted neuroprotective effects against AD. It alleviated memory impairments, reduced Aβ burdens, showed high cerebral glucose uptake, and eliminate central nervous system inflammation through reducing TNF-α and interferon-γ (IFN-γ) level and elevating IL-10 level in a 3xTg-AD mouse model (Baek et al., 2018b). BV sPLA2 improved memory function, suppressed Aβ deposition, inhibited neuroinflammation and NF-κB activation through the downregulation of glial fibrillary acidic protein (GFAP), ionised calcium binding adaptor molecule 1 (IBA-1), inducible nitric oxide synthase (iNOS), COX-2, p-IκB-α, p50 and p65, and modulated Tregs infiltration through the upregulation of Foxp3 in a lipopolysaccharide (LPS)-induced AD mouse model brain. Besides, it reduced amyloidogenesis and neuroinflammation by reducing the level of amyloid precursor protein (APP), β-amyloid precursor protein-cleaving enzyme-1 (BACE1), iNOS, COX-2, IBA-1, p-IκB-α, p50, p65, TNF-α, IL-6, IL-1β, and the activity of β-secretase in LPS-treated microglial BV-2 cells. The in vivo and in vitro results indicated that BV sPLA2 inhibited inflammatory responses and amyloidogenesis via blockage of NF-κB signaling (Ham et al., 2019a). In addition, bvPLA2 also exerted anti-inflammatory and anti-amyloidogenic effects via inhibiting signal transducer and activator of transcription 3 (STAT3) activity (Ham et al., 2019b).
Effects on other neurological disorders
BV and its main components, such as MEL and apamin, also had neuroprotective effects against other neurological disorders, including epilepticus, blood brain barrier damage and neurobehavioral changes, memory-deficit, laceration injury in cortical neurons, neuroinflammation and multiple sclerosis.
BV rebalanced neurotransmitters and blood electrolytes, ameliorated alterations of voltage-gated channels expression, and regulated pro- and anti-inflammatory cytokines levels in a pilocarpine-induced epilepticus rat model, which demonstrated that BV could slow down the development of epilepticus as a combined treatment with other antiepileptic drugs (Abd El-Hameed et al., 2021). Besides, Egyptian BV ameliorated blood-brain barrier dysfunction and neurobehavioral toxicity in rats induced by methyl mercury chloride through regulation of the methyl mercury chloride altered behavioral responses, gene expression of tight junction proteins, and immune-expression markers for specific neural cell types (Abu-Zeid et al., 2021).
MEL exerted neuroprotective effects on HT22 cells treated with Aβ25–35 through activation of nuclear factor (erythroid-derived 2)-like 2 (Nrf2)/heme oxygenase-1 (HO-1), and tropomyosin-related kinase receptor B (TrkB)/cAMP response element-binding (CREB)/BDNF signaling pathways. Additionally, MEL restored exhausted learning and memory abilities in an Aβ25–35-induced cognitive deficits mouse model. The above results showed that MEL could be a candidate agent for neurodegenerative disorders (Cong Duc and Lee, 2021). Apamin enhanced neurite outgrowth and axon regeneration after laceration injury, and increased the expression of BDNF, nerve growth factor (NGF) and regeneration-related genes in mature cortical neurons (Kim H. et al., 2021). Apamin inhibited LPS-induced neuroinflammatory responses in BV2 and rat primary microglial cells. It significantly inhibited proinflammatory cytokine production and microglial cell activation by downregulating the expression of pCaMKII and toll-like receptor 4 (TLR4) (Park et al., 2020). Especially, apamin inhibited the translocation of p65/STAT3 and MAPK-ERK signaling, which was verified through inhibitors (Park et al., 2020). The above findings suggested that apamin could be a potential adjuvant for treating a variety of neurological diseases. Besides, Apamin and bvPLA2 contributed to the control of multiple sclerosis (Lee G. et al., 2019; Danesh-Seta et al., 2021).
Alleviating inflammatory diseases
Chronic inflammation could cause the development of many diseases, such as skin diseases and RA (Wehbe et al., 2019). In classical medicine, BV and its main components were used for treating chronic inflammatory disorders. Recent studies are shown in Table 3, and the main affected targets and mechanism of BV and its main components in alleviating inflammatory diseases is shown in Figure 4.
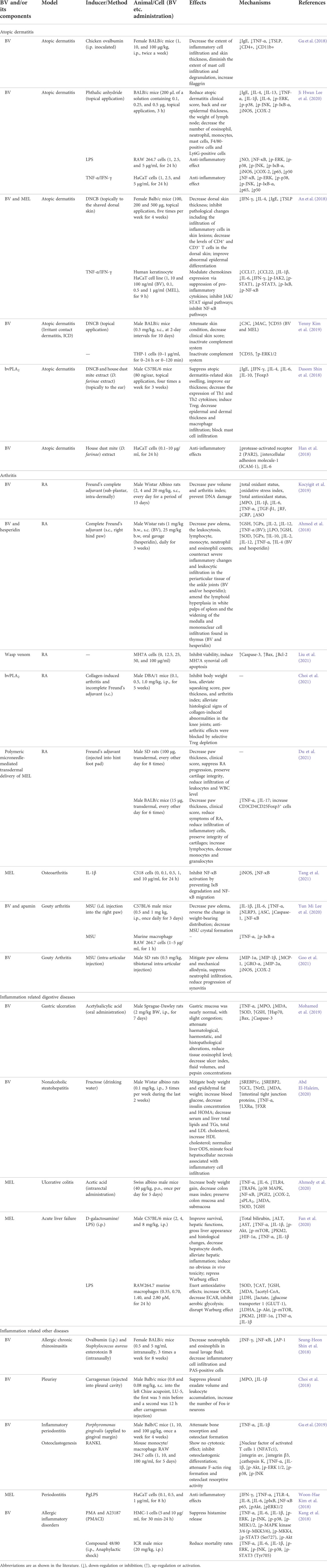
TABLE 3. Summary of the effects and mechanisms of BV and its main components on inflammatory diseases.
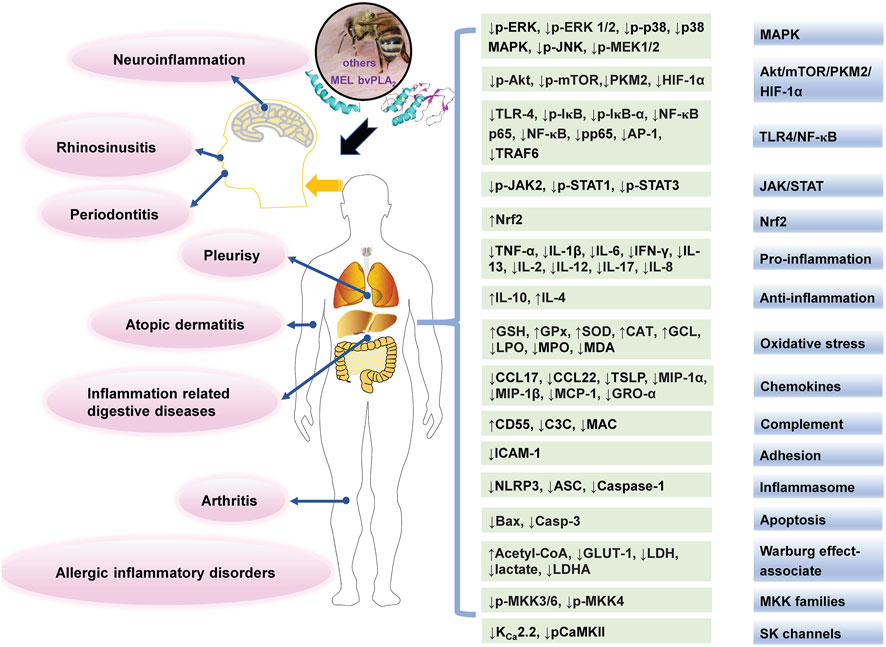
FIGURE 4. The main affected targets and mechanisms of BV and its main components in alleviating inflammatory diseases. The texts in pink oval boxes, light grey green boxes, and gradient grey blue boxes indicate related diseases, regulated targets and pathways (or mechanisms), respectively.
Effects on atopic dermatitis
As a chronic skin inflammatory disease, atopic dermatitis is caused by several genetic, inflammatory, and immunological abnormalities and characterized by recurrent eczema and itching (Gu et al., 2018; Lee Y. J. et al., 2020). BV and its main components, MEL and bvPLA2, have anti-inflammatory and atopic dermatitis effects in vivo and in vitro.
In vivo, BV treatment significantly reduced phthalic anhydride-induced atopic dermatitis clinical score, back and ear epidermal thickness, and the lymph node weight, decreased the level of IgE, IL-4, IL-13, TNF-α, IL-1β, and IL-6 and the number of eosinophil, neutrophil, monocytes, mast cells, F4/80-positive cells and Ly6G-positive cells, as well as inhibited the iNOS and COX-2 expression and activated MAPK and NF-κB in the skin tissues. In vitro, BV also suppressed MAPK and NF-κB pathway in RAW 264.7 murine macrophages treated with LPS and HaCaT human keratinocytes treated with TNF-α-/IFN-γ (Lee Y. J. et al., 2020). Besides MAPK and NF-κB pathways, Janus kinase (JAK)/STAT signal pathways were also blockaded by the treatment with BV or MEL in TNF-α/IFN-γ-treated HaCaT cells, and the chemokines CCL22 and CCL17 and pro-inflammatory cytokines IL-6, IL-1β, and IFN-γ decreased (An et al., 2018).
In addition, BV relieved atopic dermatitis by inactivating the complement system. In vitro, BV inhibited the complement system via the induction of CD55 by activating ERKs in THP-1 cells. In vivo, BV attenuated skin condition, and decreased clinical skin score in mice with atopic dermatitis-like lesions from 2.5% 2,4-dinitrochlorobenzene (DNCB) treatment; furthermore, BV and MEL inactivated the complement system, especially via the CD55 induction, which downregulated the membrane attack complex (MAC) and C3 convertase (C3C) levels (Kim Y. et al., 2019).
The bvPLA2 treatment suppressed atopic dermatitis-related skin swelling, improved ear thickness, reduced IgE and the expression of Th1 and Th2 cytokines (IFN-γ, IL-4, IL-6 and IL-10), induced Treg, and decreased epidermal and dermal thickness and macrophage and mast cell infiltration in Dermatophagoides farinae extract/DNCB-treated mice. However, the effects were eliminated in CD206−/− mice after D. farinae extract/DNCB treatment. The above data suggested that bvPLA2 could alleviate atopic skin inflammation through interaction with CD206 (Shin D. et al., 2018).
Effects on arthritis
Arthritis has a high incidence rate in the world and includes more than 100 forms, and the most common ones include RA, osteoarthritis, psoriatic arthritis and inflammatory arthritis (Tang, 2019). BV and its main components, MEL and apamin, and wasp venom, have anti-inflammatory, antioxidative, anti-apoptotic, and other effects on arthritis.
RA is a chronic inflammatory autoimmune disease, which is often affected by genetic, epigenetic, and environmental factors (Giannini et al., 2020). BV treatment decreased the paw volume and arthritis index in RA rats induced by Freund’s complete adjuvant, and showed anti-oxidant, anti-inflammatory, anti-genotoxic, and immunomodulatory effects through decreasing total oxidant status, oxidative stress index, myeloperoxidase (MPO), IL-1β, IL-6, TNF-α, TGF-β1 and mononuclear leukocyte DNA damage levels, and increasing total antioxidant status level (Kocyigit et al., 2019). In addition, wasp venom dramatically inhibited the viability of MH7A human RA synovial fibroblasts in vitro and induced MH7A cell apoptosis through up-regulating the expression of Caspase-3 and Bax and down-regulating that of Bcl-2 (Liu et al., 2021). Besides, bvPLA2 showed significant anti-inflammatory and arthritic effect on a RA mouse model though the induction of Tregs (Choi et al., 2021). Polymeric microneedle-mediated transdermal delivery of MEL inhibited the progression of RA in arthritis rat and mouse models, suppressed the levels of IL-17 and TNF-α, and raised the percentage of regulatory CD4 T cells, which suggested that polymeric microneedle-mediated MEL transdermal delivery could be used as a new treatment for RA and other autoimmune diseases (Du et al., 2021).
In addition, MEL showed anti-inflammatory effects on C518 rat knee joint cells induced by IL-1β via suppressing the expression of iNOS and preventing the degradation of IκB in cytoplasm and the migration of NF-κB from cytoplasm to nucleus, which suggested that MEL could be used as an anti-inflammatory candidate against osteoarthritis (Tang et al., 2021). BV and apamin treatment decreased the inflammatory paw edema, and reversed the alternation in weight-bearing distribution in monosodium urate (MSU)-administered mice by inhibiting proinflammatory cytokine production and inflammasome formation, as well as decreased MSU crystal formation, which suggested that BV and apamin could be potential novel agents for gouty arthritis (Lee Y. M. et al., 2020). Besides, BV also showed anti-inflammatory effect to alleviate pain and edema in gouty arthritis rats through reducing the expression of proinflammatory cytokines and chemokines (Goo et al., 2021).
Effects on inflammation related digestive diseases
BV and MEL exerted anti-inflammatory effects on inflammation related digestive diseases, such as gastric ulceration, nonalcoholic steatohepatitis, ulcerative colitis, acute liver failure, etc.
BV showed gastro-protective effects against rats gastric ulceration induced by acetylsalicylic acid. It attenuated the haematological, haemostatic, and histopathological alterations, reduced the tissue eosinophil level, as well as decreased the ulcer index, fluid volumes, and pepsin concentrations in acetylsalicylic acid-treated rats, which attributed to its anti-inflammatory and antioxidant effects by decreasing TNF-α, MPO levels and MDA concentration, and increasing SOD activity and glutathione (GSH) concentration, its anti-apoptotic property by downregulating Bax and Caspase-3 levels, as well as protection of gastric tissue by upregulating Hsp70 (Mohamed et al., 2019). Besides, BV possessed antioxidant, anti-inflammatory, and anti-hyperlipidemic effects against rats nonalcoholic steatohepatitis induced by fructose, which may be due to the enhanced expression of nuclear receptors, liver X receptor α (LXRα) and farnesoid X receptor (FXR), the reduced liver sterol regulatory element-binding protein 1/2 (SREBP1/2) levels, and the improved intestine tight junction protein expression (Abd El-Haleim, 2020).
MEL showed antiulcerogenic effect in acetic acid-induced ulcerative colitis in mice. It increased the body weight gain, decreased colon mass index and preserved colon mucosa and submucosa, which could be via mitigating TLR4/TNF receptor associated factor 6 (TRAF6) mediated NF-κB and p38 MAPK signaling (Ahmedy et al., 2020). Besides, MEL improved survival rate, reduced serious symptoms and signs, and relieved hepatic inflammation by reducing total bilirubin, alanine transaminase (ALT), aspartate aminotransferase (AST), TNF-α and IL-1β levels in acute liver failure mice. In LPS-stimulated RAW264.7 macrophages, MEL exerted antioxidant and anti-inflammatory effects. Furthermore, MEL disrupted the Warburg effect by inhibiting the Akt/mTOR/pyruvate kinase M2 (PKM2)/HIF-1α pathway to alleviate inflammation in vitro and in vivo. Molecular docking analysis confirmed that MEL targeted PKM2, and knockout of PKM2 produced anti-inflammatory effects similar to MEL in vitro. The above findings highlighted a new strategy of MEL in treating acute liver failure (Fan et al., 2020).
Effects on inflammation related other diseases
BV and its main components, including MEL, bvPLA2 and apamin, also exerted anti-inflammatory effects on other diseases, such as allergic chronic rhinosinusitis (Shin S. H. et al., 2018), pleurisy (Choi et al., 2018), inflammatory periodontitis (Kim W. H. et al., 2018; Gu et al., 2019), allergic inflammatory disorders (Kang et al., 2018), etc. For instance, BV showed anti-inflammatory effects in an allergic chronic rhinosinusitis mouse model. BV decreased neutrophil and eosinophil counts in nasal lavage fluid, reduced interferon-γ (INF-γ) level, inflammatory cell infiltration and PAS-positive cells, as well as suppressed NF-κB and activator protein-1 (AP-1) expressions in mouse nasal mucosa, which indicated that BV may possess potential value in treating allergic chronic rhinosinusitis (Shin S. H. et al., 2018).
Analgesic effect
BV is usually used to relieve pain, mainly by injecting it into acupoints, such as ST36 (Zusanli) (Li et al., 2020a). In recently 4 years, BV and its main components, including MEL and bvPLA2, have been reported to attenuate neuropathic pain, osteoarthritis pain, and burn injury pain, etc., as shown in Table 4.
Effects on neuropathic pain
Neuropathic pain is a chronic pain, often caused by damaged or abnormally discharged neurons in the peripheral or central nervous systems (Hamad et al., 2018). Some anti-cancer agents, such as oxaliplatin, vincristine and paclitaxel, could induce neuropathic pain. BV acupuncture alleviated cold and mechanical allodynia induced by oxaliplatin through regulating the action potential threshold of A-fiber dorsal root ganglia neurons (Lee J. H. et al., 2020). Besides, s.c. injection of MEL at acupoint ST36 relieved the mechanical and cold allodynia, and inhibited hyperexcitated spinal wide dynamic range (WDR) neuronal activity, via activating the spinal α1-and α2-adrenergic receptors (Choi et al., 2019). BV acupuncture inhibited abnormal hyperexcitation of the spinal WDR neurons caused by cutaneous cold and mechanical stimulation in rats treated with vincristine, which was mediated by the descending noradrenergic pathway (Li et al., 2020a). Co-treatment of BV and venlafaxine produced a lasting and additive analgesic effects on paclitaxel-induced cold and mechanical allodynia in mice, through spinal α2-adrenergic, 5-HT1/5-HT2, and 5-HT3 receptors, providing a promising clinical strategy (Li et al., 2020b).
Effects on other pain
BV acupuncture improved the pain-related behavior in the rat model of collagenase-induced osteoarthritis resulting from the partial partaking of the δ-opioid and α2-adrenergic receptors (Huh et al., 2018). Besides, BV biotherapy could sustainedly improve knee osteoarthritis pain and body function in a phase III clinical trial (Conrad et al., 2019).
Repeated BV treatment reduced the mechanical allodynia, recovered the ipsilateral paw print area and single stance, and reduced the tissue damage in a mice pain model induced by scalding burn. And BV stimulation inhibited the increase of Sub P expression in peripheral and central nervous systems. The above results suggested that BV treatment could effectively control the pain of burn patients (Kang et al., 2021).
Anti-infectivity effect
In recent 4 years, BV and main components, MEL, bvPLA2, melectin and MPX, have been reported to show anti-infectivity effects, as shown in Table 5. In particular, the effects on severe acute respiratory syndrome–coronavirus 2 (SARS-CoV-2) and drug resistant bacteria have attracted more and more attention.
Antiviral effect
Coronavirus disease (COVID-19), caused by SARS-CoV-2, has infected people in 210 countries and was declared a pandemic by WHO on 12 March 2020 (Al Naggar et al., 2021). BV has shown potent antiviral, anti-inflammatory and immunomodulatory effects, which suggested that BV treatment could be a promising complementary therapy to prevent SARS-CoV-2 (Kasozi et al., 2020; Al Naggar et al., 2021; Lima et al., 2021). In Hubei Province in China, the local beekeeper association investigated 5115 beekeepers (including 723 in Wuhan) from 23 February to 8 March in 2020. The results showed that no one had any symptoms related to COVID-19 (Yang et al., 2020). In addition, sitagliptin-MEL optimized nanoformula was repurposed, and showed antiviral potential against SARS-CoV-2 isolate with IC50 values of 8.439 µM and in vitro 3CL-protease inhibition with IC50 values of 7.216 µM. Sitagliptin-MEL could ensure improved delivery to target cells and cell uptaking, with anti-SARS-CoV-2 activity (Al-Rabia et al., 2021).
Human immunodeficiency virus (HIV) has been a major social and health problem for about 30 years. BV was safe and maybe therapeutic in a specified dose, owing to MEL uptake by HIV infected cells which resulted in reduced HIV gene expression and replication (Uzair et al., 2018). A recent study using bioinformatics tools indicated that in addition to envelope and long terminal repeat, HIV capsid and proteases could have great potential as MEL targets (Dehghani et al., 2020).
Hepatitis C virus (HCV) infection is a worldwide health concern, which can lead to serious liver diseases. BV inhibited the entry stages of HCV infection cycle by interacting with virus particles, through a non-common peptide(s), which demonstrated that the BV could be a potential candidate for characterizing and developing new natural anti-HCV agents (Sarhan et al., 2020).
Besides, chitosan/alginate nanoparticle encapsulated BV (CH/AL-BV) was developed, and nasal administration of CH/AL-BV induced non-specific immune stimulating effects, and protected against porcine reproductive and respiratory syndrome virus (PRRSV) infection, suggesting that nasal-delivered CH/AL-BV could produce effective protective immunity against PPRSV infection (Lee et al., 2018).
Antibacterial effect
BV and MEL showed antibacterial activity against MRSA (Kong et al., 2020; Pereira et al., 2020). In vitro, BV showed strong anti-infectivity effects against two MRSA strains, ATCC 33591 and USA300, and decreased the hla and agrA genes expression and the α-hemolysin production in ATCC 33591. BV alleviated the secretion of TNF-α and IL-6 in MRSA-stimulated 264.7 RAW macrophage. Besides, BV protected A549 cells from MRSA. In vivo, BV increased mouse survival rates, decreased the bacteria number in the lungs and relived symptoms of pneumonia induced by MRSA in mice. The above results indicated that BV could be a candidate for treating pneumonia caused by MRSA infection (Kong et al., 2020). BV and mainly MEL and bvPLA2, could inhibit E. coli F1F0-ATPase enzyme, a key molecular target for bacteria survival, which could be regarded as important candidate drugs against resistant bacteria (Nehme et al., 2020).
Besides, BV showed anti-infectivity activity against 16 Salmonella strains, and the minimum inhibitory concentrations (MIC) ranged from 256 to 1024 μg/ml. In addition, BV inhibited biofilm formation in 14 strains. The above findings indicated that BV was a potential anti-infectivity agent against food-borne pathogens (Arteaga et al., 2019).
Bacterial infection from antibiotic resistant bacteria has become a main cause of morbidity and mortality after burns. In vitro, MEL showed antibacterial activity against extensively drug-resistant (XDR) Acinetobacter spp., caused fluorescence release from XDR isolates which severed as a marker of membrane damage, and had membranolytic effect. In vivo, MEL eradicated the colonized XDR bacteria on infected mouse model with third-degree burn, and no toxicity at the therapeutic dose was observed (Pashaei et al., 2019). Furthermore, MEL-doripenem showed synergistic antibacterial activity against MDR A. baumannii isolates, while MEL-doripenem and MEL-ceftazidime showed synergistic antibacterial activity against multidrug-resistant (MDR) P. aeruginosa (Akbari et al., 2019). The above results showed that MEL, MEL-doripenem and MEL-ceftazidime could be regarded as topical drugs against burn infections from antibiotic resistant bacteria.
Melectin, from the venom of a bee, Melecta albifrons, showed broad-spectrum anti-infectivity activities against standard sensitive/clinical drug-resistant bacteria strains, and exhibited low or moderate cytotoxicity and no hemolytic activity. Besides, melectin reduced the release of TNF-α, IL-1β, IL-6, and IL-8 from S. aureus-stimulated human fibroblasts. These findings suggested that melectin could be considered as a new template against multidrug resistance (Ko et al., 2020; Liang et al., 2021).
MPX, from wasp venom, showed good antibacterial effect on Actinobacillus pleuropneumoniae, a causative agent of respiratory infections in pigs. MPX destroyed the bacterial cell membrane, blocked the bacterial biofilms formation, and modulated the expression of virulence factors of A. pleuropneumoniae in vitro, as well as protected mice from a lethal dose of A. pleuropneumoniae and relieved lung inflammation in vivo, which provided information for the clinical application of MPX (Wang et al., 2020).
Antifungal effect
The antifungal effects of BV components on Trichophyton rubrum have been evaluated, and the results showed that BV provided a significant level of inhibition in its overall form, and BV based products may play a potential antifungal therapeutic role (Park et al., 2018).
Protect effect on liver, kidney, lung and muscle injury
Protect effect on liver injury
BV and its main components, such as MEL and bvPLA2, showed protect effects on liver diseases, including non-alcoholic fatty liver, hepatoxicity and cholestatic liver disease, as shown in Table 6.
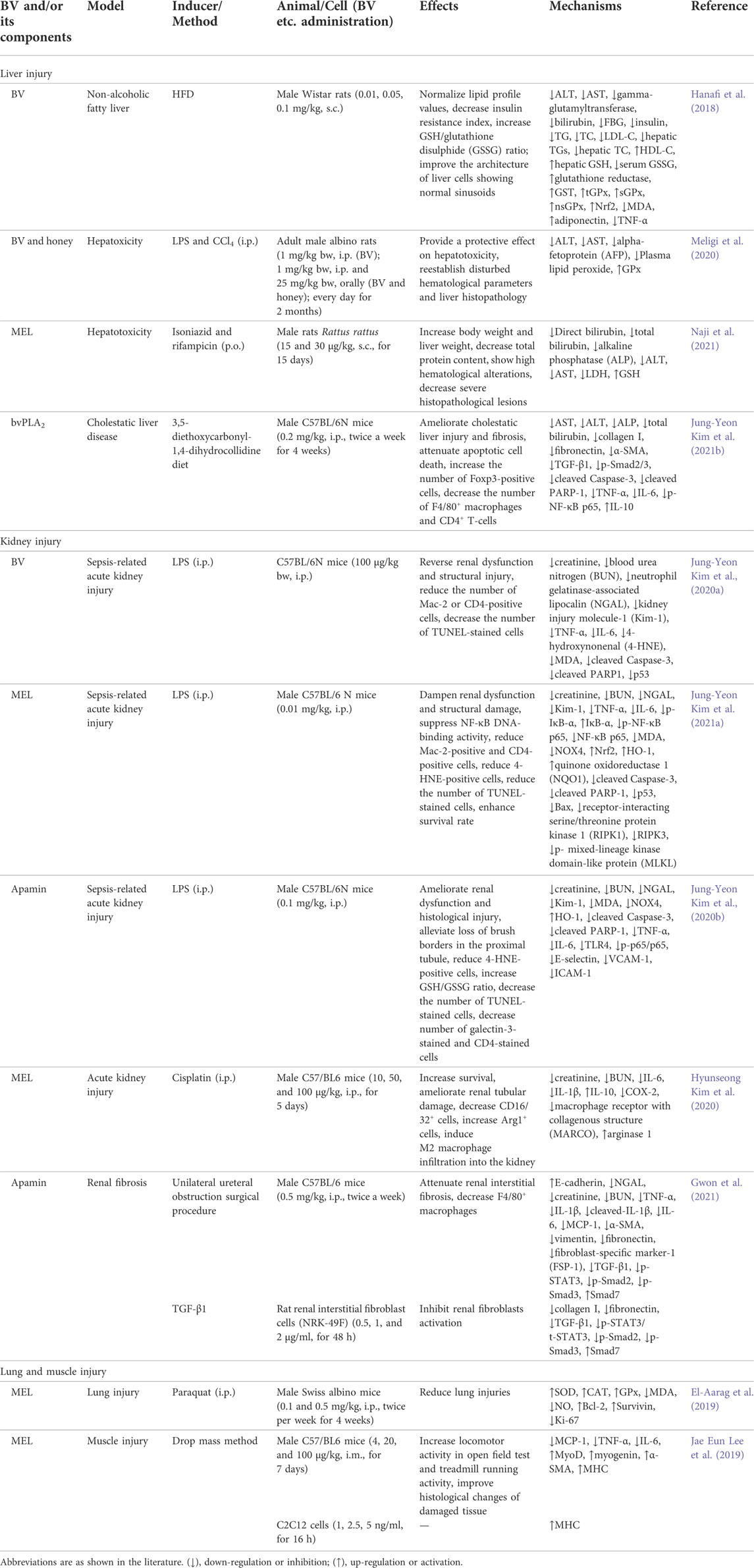
TABLE 6. Summary of the protect effects and mechanisms of BV and its main components on liver, kidney, lung and muscle injury.
The trend of elevated fasting glucose and insulin levels as well as lower levels of antioxidant parameters such as glutathione-S-transferase (GST), glutathione peroxidase (GPx), Nrf2 and GSH in rats induced by high-fat diet (HFD) was reversed by BV, and the levels of adiponectin and TNF-α were restored. The above results suggested that BV is a promising alternative treatment option for non-alcoholic fatty liver disease (Hanafi et al., 2018). BV and honey showed protective effects on hepatoxicity and lipid peroxidation in rats caused by LPS and carbon tetrachloride, while MEL possessed hepatoprotective activity on isoniazid- and rifampicin-induced liver hepatoxicity in rats, both of which suggested that a combination of BV and honey, or MEL could be beneficial for the prevention of hepatoxicity and could be used as potential therapeutic agents (Meligi et al., 2020; Naji et al., 2021). Besides, after treatment with bvPLA2, the levels of cholestasis markers in the serum were attenuated, the abnormal state of liver fibrosis was restored, and hepatocyte inflammation and apoptosis were inhibited in mice with diet feeding 3,5-diethoxycarbonyl-1,4-dihydrocollidine (Kim J.-Y. et al., 2021b).
Protect effect on kidney injury
BV and its main components, such as MEL and apamin, showed protect effects on acute kidney injury and renal fibrosis, as listed in Table 6.
Acute kidney injury associated with sepsis is a worldwide health dilemma. Sepsis-related acute kidney injury is a global health issue. During sepsis, the endotoxin LPS mediates systemic inflammatory responses. BV, MEL and apamin ameliorated LPS-induced acute kidney injury via the suppression of oxidative stress, inflammation and cell death in mice (Kim J. Y. et al., 2020a; Kim J. Y. et al., 2020b; Kim et al., 2021a). Take MEL as an example, it dampened renal dysfunction and structural damage, and at the same time, the levels of direct tubular injury markers were attenuated in LPS-treated mice. MEL decreased systemic and renal TNF-α and IL-6 levels, attenuated the accumulation of immune cells in the kidney, and suppressed NF-κB pathway. MEL reduced MDA level and inhibited the expression of nicotinamide adenine dinucleotide phosphate oxidase 4 (NOX4), and promoted Nrf2-mediated antioxidant defenses, as well as inhibited apoptosis and necrosis after LPS treatment. In addition, the survival duration of LPS-injected mice was significantly prolonged after MEL injection. The above results suggest that MEL could have a responsibility among the prospective options for the prevention and treatment of renal complications of sepsis (Kim J.Y. et al., 2021b). Besides, MEL alleviated mice acute kidney injury induced by cisplatin via regulating M2 macrophage expression (Kim H. et al., 2020).
With the chronic accumulation of progressive renal fibrosis, the kidneys continuously suffer one-way, irreversible damage, and end-stage diseases such as renal failure arrive as scheduled. In vivo, apamin inhibited renal fibrosis induced by unilateral ureteral obstruction; in vitro, it resisted renal fibroblasts activation induced by TGF-β1. This peptide suppressed TGF-β1/Smad2/3 and STAT3 pathways to inhibit inflammation response and tubular atrophy as well as reduce the activation of myofibroblast and the expression of fibrotic gene. These studies suggest that apamin may have a competitive advantage in the screening of therapeutic agent alternatives against renal fibrosis (Gwon et al., 2021).
Protect effect on lung and muscle injury
In addition, MEL reduced lung injuries induced by paraquat in mice. It increased SOD, CAT, and GPx activity, and decreased MDA and NO levels, as well as upregulated Bcl-2 and survivin expressions and downregulated Ki-67 expression, which demonstrated that MEL restored the pathological alternations of paraquat-induced lung injury in mice from the perspectives of enhancing antioxidant activity and reducing apoptosis (Table 6) (El-Aarag et al., 2019).
MEL ameliorated muscle function and histological changes of damaged tissue, and debilitated the production of pro-inflammatory factors, including IL-6, MCP-1, and TNF-α in muscle contusion mouse model. In addition, the expression of muscle recovery and regeneration-related biomarkers, such as myogenin, MyoD, α-smooth muscle actin (α-SMA) and myosin heavy chain (MHC), was elevated in mice. The results suggested that MEL could be a promising candidate for the muscle injury treatment (Table 6) (Lee J. E. et al., 2019).
Protect effect on other diseases
Anti-diabetic efficacy
Bee wax coated water-soluble fraction of BV (BWCBVA) and MEL-loaded NPs showed anti-diabetic efficacy in diabetic animal models, as shown in Table 7. BWCBVA decreased blood glucose level, normalized the serum biochemical parameters, and increased the body weight in streptozotocin-induced diabetic rats. Also, administration of BWCBVA reduced the expression of PI3K-p85 and enhanced the expression of glucokinase in liver, as well as increased the number of islet cells and decreased the damage of β-cell in pancreas. Furthermore, co-administering BWCBVA with nifedipine and nicorandil resulted in insulin secretion through enhancing the calcium ion influx and obstructing the potassium ion channel. The above results indicated that BWCBVA could be considered as an available mode to treat diabetes (Balamurugan et al., 2019). In addition, prolonged MEL release from polyelectrolyte-based nanocomplexes improved blood glycemic control in a type II diabetes mouse model (Gui et al., 2020).
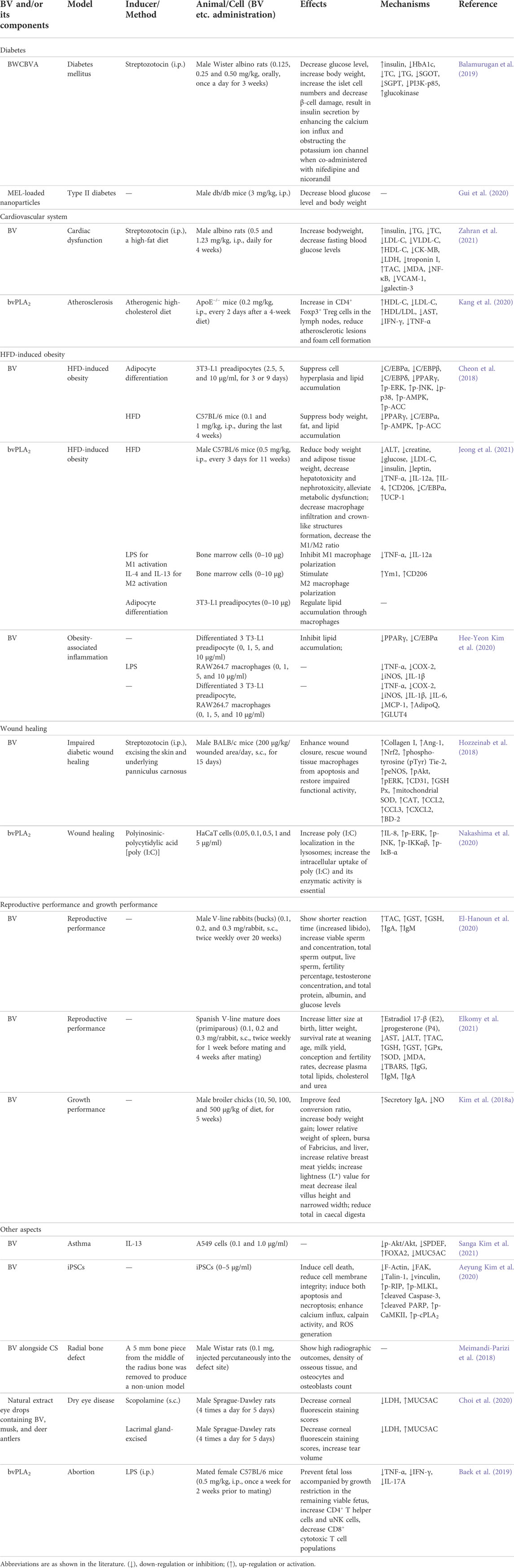
TABLE 7. Summary of the protect effects and mechanisms of BV and its main components on other diseases.
Protective effect on cardiovascular system
Administration of BV decreased fasting blood glucose, serum total cholesterol (TC), triglycerides (TG), LDL-C, VLDL-C, creatine kinase (CK)-MB, LDH, troponin I, and heart MDA, NF-κB levels, and heart vascular cell adhesion molecule-1 (VCAM-1) and galectin-3 expression, as well as increased bodyweight, plasma insulin, serum HDL-C and heart total antioxidant capacity (TAC) levels in diabetic hyperlipidemic rats, which indicated that BV could reduce oxidative stress and downregulate NF-κB pathway to improve cardiac dysfunction (Zahran et al., 2021). Besides, bvPLA2 could be a potential agent for atherosclerosis through inducing Treg cells (Kang et al., 2020). The above results are shown in Table 7.
Attenuating HFD-induced obesity
In vitro, BV suppressed lipid accumulation, and inhibited adipogenesis by reducing the expression of CCAAT/enhancer-binding proteins (C/EBPs), peroxisome proliferator-activated receptor γ (PPARγ), and p-p38, as well as by upregulating the expression of p-ERK, p-JNK, p-adenosine monophosphate–activated protein kinase (AMPK), and p-acetyl-coenzyme A carboxylase (ACC) during differentiation of 3T3-L1 preadipocytes. In vivo, BV inhibited fat and lipid accumulation and reduced body weight, through downregulating the expression of adipogenic markers, PPARγ and C/EBPα, and upregulating the expression of p-AMPK and p-ACC in HFD-induced obese mice, suggesting BV an effective preventive and therapeutic candidate for obesity (Cheon et al., 2018). Besides, bvPLA2 also attenuated HFD-induced obesity in mice (Jeong et al., 2021). In addition, BV could attenuate adipocyte hypertrophy, as well as improve insulin resistance and obesity-related inflammation in obese adipose tissue (Kim H. Y. et al., 2020). The above results are shown in Table 7.
Improving wound healing
Diabetic wound formation is one of the serious complications of diabetes. BV treatment improved wound closure in streptozotocin-induced mice diabetic model through enhancing β-defensin-2 (BD-2) and collagen expression and restoring angiopoietin-1 (Ang-1) and Nrf2 levels, and thereby increasing the Tie-2 downstream signaling, including the phosphorylation of endothelial nitric oxide synthase (eNOS), Akt and ERK. Furthermore, BV restored wounded tissue antioxidant enzymes GSH Px, mitochondrial SOD and CAT activities, and the chemokines CCL2, CCL3 and CXCL2 levels, and then rescued wound macrophages from apoptosis induced by mitochondrial membrane potential. The above results provided a new example for BV treatment to stimulate angiogenesis and improve the wound healing process of diabetes (Hozzeinab et al., 2018). Besides, bvPLA2 increased IL-8 production in HaCaT cells induced by poly (I:C), upregulated the expression of p-ERK, p-JNK, p-IKKαβ and p-IκB-α. It enhanced the intracellular poly (I:C) uptake, and the enzymatic activity was essential. These findings indicated that bvPLA2 could ameliorate wound healing via the enhancement of TLR3 responses (Nakashima et al., 2020). The above results are shown in Table 7.
Improving reproductive performance and growth performance
BV could improve reproductive performance and growth performance, as shown in Table 7. BV improved reproduction and immune response of male and female rabbits, which demonstrated that BV could be used as an effective and safe substitute for artificial chemical drugs (sex stimulants) in rabbit breeding to improve some reproductive characteristics, immune response and health (El-Hanoun et al., 2020; Elkomy et al., 2021). Besides, dietary BV improved growth performance, the yield and quality of breast meat, and the concentration of secretory IgA on ileal mucosa in broiler chickens, and lowered internal organs including spleen, bursa, and liver, caecal short-chain fatty acids concentrations and ileal villus height, which demonstrated that BV could be regarded as a natural value-added substitute for antibiotics in feed to enhance growth and animal health (Kim D. H. et al., 2018).
Other aspects
Moreover, BV and bvPLA2 had therapeutic and ameliorative effects on asthma, radial bone defect, dry eye disease and abortion, as well as eliminated teratogenic human induced pluripotent stem cells (iPSCs), as shown in Table 7.
BV prevented forkhead box A2 (FOXA2)-regulating mucin 5AC (MUC5AC) expression in IL-13-treated A549 cells not only through inhibiting Akt activation but also by suppressing enhanced SAM-pointed domain containing Ets-like factor (SPDEF), which demonstrated that BV could help prevent mucus metaplasia in asthma (Kim et al., 2021d). Although iPSCs is considerable practical in potential cell-based therapy, they remain confronted with problems such as teratoma formation. BV treatment resulted in impaired F-actin filaments within iPSCs, Ca2+ inward flow and accumulation to the cytoplasm, elevated calpain activity and reactive oxygen species (ROS) generation, selective induction and reduction of viability and adhesion capacity of undifferentiated stem cell iPSCs, and increased cell death. In addition, BV treatment before in ovo transplantation suppressed the formation of iPSC-derived teratoma. Moreover, BV was neither cytotoxic nor genotoxic to iPSC-derived differentiated cells. The above findings indicated that BV possessed anti-teratoma effect by getting rid of residual iPSCs, and could be a safe and effective cell preparation agent for stem cell therapy (Kim et al., 2020a).
BV alongside chitosan scaffold (CS) displayed high radiographic outcomes compared with the untreated and CS groups at day 56 postoperatively, and showed high osseous tissue density and high counts of osteocytes and osteoblasts compared with the CS and autograft groups, which highlighted the capability of CS-BV as a potential alternative and enhancer of bone graft substitute material (Meimandi-Parizi et al., 2018). The natural extract containing BV, deer antlers and musk as eye drops had therapeutic effects on scopolamine-injected dry eye disease rat models and lacrimal gland-excised dry eye disease rat models through repairing the damaged ocular surface, increasing the amount of tears, and restoring the tear mucin layer (Choi et al., 2020).
bvPLA2 prevented fetal loss with growth restriction in the remaining live fetuses in abortion mouse model induced by LPS. It increased Treg cells, and decreased TNF-α and IFN-γ expression. The prophylactic effect of bvPLA2 was not found in abortion prone mice lacking Treg. The above results indicated that bvPLA2 might have effects on LPS-induced abortion mice through regulating Treg populations (Baek et al., 2019).
Safety and venom immunotherapy
An important concern with BV has been its safety. BV can cause anaphylaxis (Cherniack and Govorushko, 2018). It is reported that up to 3.4% of children and up to 7.5% of adults have systemic allergic sting reactions. Allergic reactions can be mild in skin; however, it can also be moderate to severe with a risk of life-threatening allergic reactions (Sturm et al., 2018). In an individual patient education, it addressed to correctly and timely use epinephrine auto-injectors, and recommend to stop physical exercise and adopt supine position during acute allergic attack (Stoevesandt et al., 2020).
Venom immunotherapy (VIT) could prevent severe allergic reactions in 80–100% subjects allergic to Hymenoptera venom. An immunological study on the early phase of VIT performed on forty individuals indicated that cytokines (CCL5/RANTES and IL-17E/IL-25) and eosinophils were conducive to the immune response (Palgan et al., 2020).
MEL could be used in adjuvant immunotherapy. MEL combined with LPS produced an increase of IL-1β and IL-6 levels and a decrease of IL-10 level in phorbol 12-myristate 13-acetate (PMA)-differentiated THP-1 cells. Besides, the response to MEL and LPS was characterized by metabolic profiling, and the clearest effects were on glycolysis, tricarboxylic acid cycle, oxidative phosphorylation, and purine, pyrimidine, and fatty acid metabolism (Alqarni et al., 2018). Similarly, the same research group also studied the metabolomic profiling for the immune stimulatory effect of a major constituent of BV, (Z)-11-eicosenol, and its derivations methyl cis-11-eicosenoate and cis-11-eicosenoic acid on PMA-differentiated THP-1 cells. The results supported the proposed actions of the three components as immune system stimulators (Alqarni et al., 2019). Besides, bvPLA2 has attenuated several immune system-related diseases. In a recent study, bvPLA2 decreased apoptotic Treg cells, reduced early apoptosis in splenocytes and CD4+CD25+ Treg cells, and raised the expression of CTLA-4 and PD-1 on CD4+CD25+ Treg cells, which showed that bvPLA2 could induce Treg expansion by changing apoptotic signal (Baek et al., 2020).
As experts have commented, if the venom is selected correctly, and adequate venom preparation and maintenance dose are used, VIT may be a treatment method of significant value in preventing the systemic reaction of Hymenoptera stings. Besides, the duration of VIT is usually 5 years, which can provide long-term protection from stinging after treatment interruption, but it recommends indefinite (possibly life-long) treatment for patients with mastocytosis or other high-risk patients (Incorvaia et al., 2018).
Toxicity, administration dose and new drug delivery system
Although BV and its main components often show good results towards cancerous cells, bacteria, viruses as well as other disorders and diseases, there are always open questions regarding their potential toxicity on normal non-target or host cells and tissues making this kind of toxicity one of the biggest obstacles for the possibility of applying such natural products as medications. A single high dose of 100 μg/ml of BV damaged the DNA molecule in human lymphocytes in vitro, resulting in cellular instability (Garaj-Vrhovac and Gajski, 2009). A further study suggested that the DNA damage effects of BV could be at least partially related to oxidative stress (Gajski et al., 2012). In addition, BV decreased cell viability, altered cell morphology, as well as induced cytogenotoxicity and dominantly necrotic type of cell death, in human peripheral blood lymphocytes with a dose- and time-dependent manner (Gajski and Garaj-Vrhovac, 2011). Similarly, MEL was also found to induce cytogenetic damage and oxidative stress in human peripheral blood lymphocytes (Gajski et al., 2016).
Therefore, the administration dose is of great noteworthy. Wi-38, a species of human lung fibroblast, was less sensitive to MEL and has higher colony formation capacity than ChaGo-K1 cells (IC50 = 0.6 μM), with an IC50 value of 2.35 μM at 48 h (Tipgomut et al., 2018). After 72 h incubation, the cell viability of BESA-2B (normal lung epithelial cells) remained unaffected at MEL concentrations ≤4 μg/ml with some reduction in activity, while the viability of NCI-H441 and A549 cells (lung cancer cells) was significantly affected at 2 μg/ml (Gao et al., 2018). Besides, the Selective Index of NIH3T3 (healthy mouse fibroblast cells) was higher than 3 compared to HepG2 and MDA-MB-231 cells, implying that BV was more selective for both types of cancer cells and that NIH3T3 showed altered cell morphology only when BV was 100 μg/ml (Uzuner et al., 2021). Moreover, Melectin at around 15 μM only slightly inhibited NIH3T3; Concentration below 50 μM caused only 20% erythrocyte lysis, with no significant hemolytic effect (Liang et al., 2021). Comparable results existed for MEL-AF treatment, where MEL-AF remained non-significantly cytotoxic at doses in the range of ≤10 μg/ml after 24 h of MEL-AF treatment (Sangboonruang et al., 2020). Besides, NRK-49F (rat renal interstitial fibroblast cells) cell viability with 48 h apamin treatment was reduced at 10 μg/ml of apamin. However, 0.1, 0.5, 1, 2, and 5 μg/ml did not affect NRK-49F cell viability (Gwon et al., 2021).
The effect of MEL on the viability of MCF 10A (human normal mammary epithelial cells), HDFa (human dermal fibroblasts), and MCF-12A (human mammary epithelial cells) cells was more delayed and attenuated, with an IC50 value of 22.17 μg/ml for HDFa cells at 24 h (Duffy et al., 2020).
When BV and MEL treated THLE-2 (normal human liver cell line) for 24 h, the IC50 values were 95.73 μg/ml in the BV group and 78.38 μg/ml in the MEL group, both of which were significantly higher than the IC50 values of treated HepG2 (1.4 and 2.8 μg/ml, respectively), which showed negligible effects of BV and MEL on hepatocytes (Mansour et al., 2021).
After treating HaCaT (normal human keratinocytes) with 0.1–10 μg/ml of BV and MEL for 24 and 72 h, the toxic effects possessed by BV were more pronounced, with IC50 values reaching 6.72 and 6.38 μg/ml for the two time periods, respectively; the IC50 values of MEL-treated HaCaT were greater than 10 μg/ml exceeding the maximum MEL concentration used in the experiment (Nikodijevic et al., 2021).
BV and Wasp venom were non-toxic to BV-2 murine microglial cells at concentrations of ≤12 μg/ml and ≤160 μg/ml for 24 h, respectively (Yun et al., 2021). And 1 μg/ml of apamin showed a 10% decrease in BV2 microglial cell proliferation for 12 h (Park et al., 2020). Cell viability of 0.1–30 µM of MEL on HT22 mouse hippocampal cells for 24 h was assessed and 3 µM was found to be the maximum safe concentration for further study (Cong Duc and Lee, 2021).
BV at 0.1 and 1 mg/kg was an effective concentration and had no side effects on rats (Lee et al., 2020a). Similarly, 0.5 and 1.23 mg/kg of BV were also taken as therapeutic dosages to rats (Zahran et al., 2021). Besides, i.p. administration of 1, 2, and 4 mg/kg of MEL leaded to no toxicity in mice. The LD50 of mice with i.p. injection of MEL was 7.4 mg/kg (PubChem Database. MEL, CID = 16129627) (Fan et al., 2020).
In order to decrease the toxic and side effects such as hemolysis of BV and MEL, and improve the characteristics of drug targets and release, besides of NPs mentioned above (Zhou et al., 2021), other new drug delivery system has also been developed and applied in clinics (Lyu et al., 2019). After the amino acid modification of MEL or the addition of specific functional groups, it can improve the ability of MEL to target recognition and prevent degradation of target cells while reducing its toxicity to normal cells (Giribaldi et al., 2021). For example, after replacing valine at position 8 and proline at position 14 in MEL with lysine, MEL-pep had stronger ability to resist cell proliferation and inhibit tumor growth, reverse the drug resistance of cells to 5-fluorouracil, inhibit Akt pathway and P-glycoprotein (P-gp) of cells related to the exclusion of foreign bodies (Ke et al., 2018). Besides, a pH-sensitive amide bond was introduced between the 2,3-dimethyl maleimide and lysine of MEL to modify MEL, which could reduce the hemolytic status of MEL and maintain its low activity in the neutral or microalkaline environment, and show a stronger tumor targeting effect in the acidic microenvironment (Luo et al., 2018). The above investigations provided a feasible platform for improving the targeting and safety applications of BV and its main components, such as MEL.
Conclusion and perspectives
BV and its main constituents have multiple biological activities and applied to treat several diseases, such as cancer, neurological disorders, inflammatory diseases, pain, microbial diseases, liver, kidney, lung and muscle injury, etc. In this paper, we reviewed the recently published reports (2018–2021) on BV and its main constituents. These articles have indicated that BV and its main constituents exerted the above protections and were contained in a variety of signaling tranduction pathways (Figures 2–4).
BV played a vital role in cell proliferation, migration, invasion, inhibition of EMT and induction of apoptosis and autophagy by exerting multi-pathway and multi-target long-lasting effects on cells in lung cancer, breast cancer, cervical cancer, and many other cancers in vitro and in vivo. The mechanisms of action of BV were: upregulation of Caspase-3, Caspase-7, Caspase-9, Bax, p21, p27, p53, Rb, PTEN, and 15-lipoxygenase-1 expression, downregulation of MITF, PARP, Bcl-2, Bcl-xL, ERα, EGFR, VEGF, MMP-1, MMP-2, MMP-9, TNF-α, NF-κB, HIF-1α, Cyclin D1, Cyclin A, Cyclin B, and Rac1, etc. BV inhibited the mTOR pathway, PI3K/Akt and MAPK signaling pathway, and mitotic signaling pathway by reducing the expression of p-mTOR, Akt, p-Akt, p38, p-p38, JNK, p-JNK, ERK, p-ERK, p-PI3K, p-EGFR, and p-HER2, which in turn impaired cancer cell viability, reduced migration and invasion activities, and promoted cell death. BV activated the mitochondrial apoptotic pathway in cancer cells by increasing the expression of apoptosis signaling molecules such as Fas and Caspase-9. On the other hand, it inhibited EMT in cancer cells by decreasing the expression of vimentin, ZEB2, and Slug and elevating the expression of E-cadherin (Figure 2).
Except cancer, BV and its main components can treat a variety of other diseases, including neurological disorders, inflammatory diseases, liver, kidney, lung and muscle injury, etc., mainly via inhibiting the release of inflammatory cytokines and chemokines, regulating the redox balance in the body, modulating the expression of apoptosis related factors, and so on. The most common points were: first, BV and its main components reduced pro-inflammatory cytokines (TNF-α, IL-1β, IL-6, IFN-γ, IL-13, IL-2, IL-12, IL-17, etc.) and raiseed anti-inflammatory cytokines (IL-10 and IL-4); second, they improved antioxidant capacity by upregulating the levels of GSH, GPx, SOD, CAT, glutamate-cysteine ligase (GCL), GST, etc., and downregulating the levels of lipid peroxidation (LPO), MPO, MDA, protein carbonyl (PCO), ROS, etc.; third, they downregulated pro-apoptotic factors (Caspase-3, cleaved Caspase-3, cleaved PARP-1, Bax, p53, apoptosis-inducing factor (AIF), Calpain, Cyt C, etc.) and upregulated anti-apoptotic factors (Bcl-2 and Survivin); fourth, they reduced chemokines, such as CCL17, CCL22, thymic stromal lymphopoietin (TSLP), macrophage inflammatory protein-1α (MIP-1α), MIP-1β, MCP-1, growth related oncogene-α (GRO-α), and so on; finally, they often downregulated MAPK, TLR4/NF-κB, Akt/mTOR and JAK/STAT signaling pathways, and upregulated Nrf2/HO-1 signaling pathway thereby producing corresponding pharmacological effects on different pathophysiological environments. Besides, BV and its main components upregulated TrkB/CREB/BDNF signaling pathway and the expression of growth-associated factors (BDNF, NGF, neurofilament 200 kDa (NF200) and growth-associated protein 43 (GAP43)), as well as downregulated the expression of amyloidogenic proteins (APP, BACE1, Aβ1–42 and Aβ1–40) in treating neurological disorders. They inhibited hepatic and renal fibrosis via suppressing TGF-β/Smad2/3 signaling pathway. They also downregulated the expression of C/EBPs and PPARγ, and activated AMPK signaling pathway in the treatment of obesity.
The potential effects of BV and its main components on ills and their potential mechanisms were predicted through bioinformatic analysis, referring to DOSE analysis and KEGG pathway enrichment of the targets reported using bioinformatics tools in the light of our previous methods (Zhong et al., 2021; Lin et al., 2022). As shown in Figure 5A, beside the reported diseases by literature, BV and its main components maybe have effect on the connective tissue cancer and obstructive lung disease. Meanwhile, KEGG pathway enrichment analysis (Figure 5B) shows that BV and its main components can mainly regulate the proteins involved in the pathways of lipid and atherosclerosis, PI3K−Akt, Human cytomegalovirus infection, Human papillomavirus infection, etc. To further screen the pivotal targets, PPI analysis was performed with the reported proteins for BV and its main components using the STRING on-line sever (https://www.string-db.org). As shown in Figure 6, a central protein, TRAF6 is highlighted in the PPI “tree” (located in purplish red circle). Indeed, according to the KEGG database, this protein is truly involved in the regulation of up to 34 pathways, including MAPK, NF-κB, Pathways in cancer, etc. Moreover, seven subcentral proteins, X-linked inhibitor of apoptosis protein (XIAP), TNF, phosphoinositide-3-kinase regulatory subunit1 (PIK3R1), tumor protein p53 (TP53), SQSTM1 (p62), TLR4 and TGF-β receptor 1 (TGFBR1), which can be attributed to different functional pathways, are displayed the direct interaction with the central protein TRAF6.
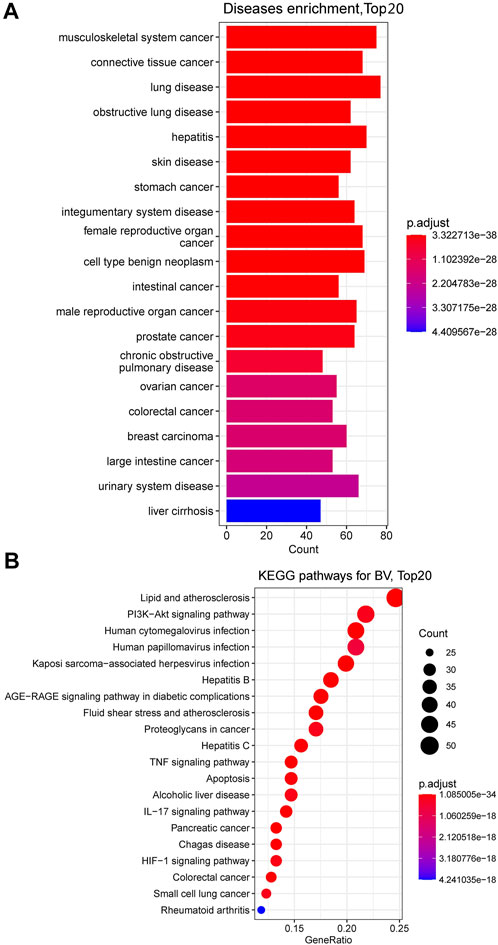
FIGURE 5. DOSE (A) and KEGG (B) analyses of proteins reported for BV and its main components in the literature. Top 20 diseases and KEGG pathways are enriched using R package “DOSE” and “topGO”, respectively with p-value Cutoff = 0.01, q-value Cutoff = 0.01).
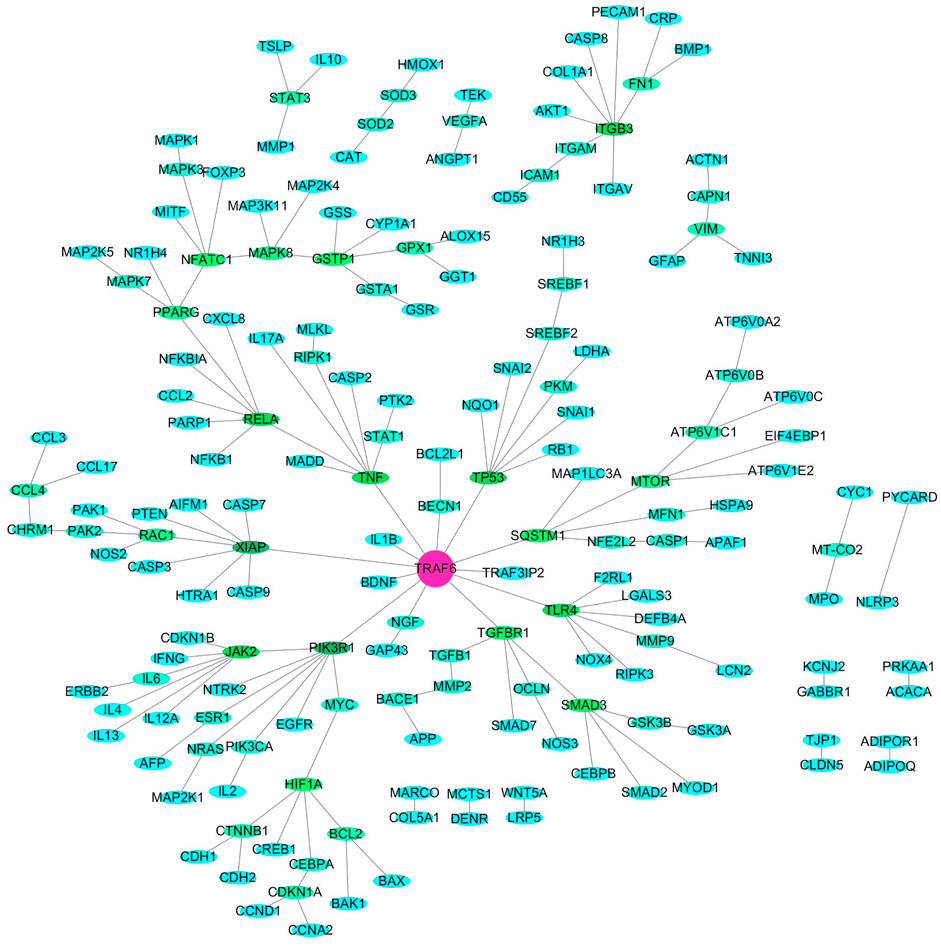
FIGURE 6. PPI analyses (confidence:0.4) of proteins and genes reported for BV and its main components in the literature visualized by Cytoscape 3.6.0 software. Texts in purple circle, green circle and light blue circle demonstrates the central hub target, secondary node target and the others node target.
Ultimately, based on the previous results and the present bioinformatics and PPI analysis, it gives the following perspectives.
(1) First, although all the reported targets were proved to be correlated with the interfere effects of BV and its main components mainly on cancers, the DOSE analysis strongly suggest that BV and its main components could have an effect on lung disease and obstructive pulmonary disease, which could be worth exploring in the current COVID-19 epidemic. Therefore, next, it is suggested to further validate the effect of BV and its main components on lung disease and obstructive pulmonary disease for seeking hope for the treatment of lung diseases, especially COVID-19.
(2) Second, the present KEGG enrichment analysis suggests that lipid and atherosclerosis, Human cytomegalovirus infection and Human papillomavirus infection pathways could be involved in the disease intervention, but these pathways are not mentioned in the previous reports for BV and its main components. For integratively understanding the intervention and mechanisms of BV and its main components on diseases, proteomics or genomics investigations should be performed using in vitro and in vivo disease models to confirm the unreported pathways in the future study.
(3) Last, TRAF6 is predicted as one core target and XIAP, TNF, PIK3R1, TP53, SQSTM1, TLR4 and TGFBR1 are predicted as subcentral proteins for the therapeutic effects of BV and its main components. Accordingly, it will be very meaningful to validate the target roles of these proteins for the further drug development of BV and its main components by gene silencing, knockout experiments, etc.
In conclusion, these ideas provide valuable clues or perspectives to further study on the pharmacological effects and mechanisms of BV and its main components, so as to the promoting the drug development and clinical applications for BV.
Author contributions
Conceptualization, PS, SS, and HY; writing—original draft preparation, PS and SX; figures and tables, PS, SX, and HY; writing—reviewing and editing, HY; data curation, PS, SX, JY, YZ, and SH. All authors have read and agreed to the published version of the manuscript.
Funding
The authors gratefully acknowledge the financial supports of the National Natural Science Foundation of China (81973558).
Conflict of interest
The authors declare that the research was conducted in the absence of any commercial or financial relationships that could be construed as a potential conflict of interest.
Publisher’s note
All claims expressed in this article are solely those of the authors and do not necessarily represent those of their affiliated organizations, or those of the publisher, the editors and the reviewers. Any product that may be evaluated in this article, or claim that may be made by its manufacturer, is not guaranteed or endorsed by the publisher.
References
Abd El-Haleim, E. A. (2020). Molecular study on the potential protective effects of bee venom against fructose-induced nonalcoholic steatohepatitis in rats. Pharmacology 105 (11-12), 692–704. doi:10.1159/000508511
Abd El-Hameed, A. M., Abuelsaad, A. S. A., and Khalil, A. (2021). Bee venom acupuncture therapy ameliorates neuroinflammatory alterations in a pilocarpine-induced epilepticus model. Metab. Brain Dis. 36 (7), 2047–2058. doi:10.1007/s11011-021-00766-9
Abu-Zeid, E. H., Khalifa, B. A., Elewa, Y. H. A., Arisha, A. H., Ismail, T. A., Hendam, B. M., et al. (2021). Bee venom Apis mellifera lamarckii rescues blood brain barrier damage and neurobehavioral changes induced by methyl mercury via regulating tight junction proteins expression in rat cerebellum. Food Chem. Toxicol. 154, 112309. doi:10.1016/j.fct.2021.112309
Ahmed, O., Fahim, H., Mahmoud, A., and Ahmed, E. (2018). Bee venom and hesperidin effectively mitigate complete Freund's adjuvant-induced arthritis via immunomodulation and enhancement of antioxidant defense system. Arch. Rheumatol. 33 (2), 198–212. doi:10.5606/ArchRheumatol.2018.6519
Ahmed-Farid, O. A., Taha, M., Bakeer, R. M., Radwan, O. K., Hendawy, H. A. M., Soliman, A. S., et al. (2021). Effects of bee venom and dopamine-loaded nanoparticles on reserpine-induced Parkinson's disease rat model. Sci. Rep. 11 (1), 21141. doi:10.1038/s41598-021-00764-y
Ahmedy, O. A., Ibrahim, S. M., Salem, H. H., and Kandil, E. A. (2020). Antiulcerogenic effect of melittin via mitigating TLR4/TRAF6 mediated NF-κB and p38MAPK pathways in acetic acid-induced ulcerative colitis in mice. Chem. Biol. Interact. 331, 109276. doi:10.1016/j.cbi.2020.109276
Akbari, R., Hakemi-Vala, M., Pashaie, F., Bevalian, P., Hashemi, A., and Bagheri, K. P. (2019). Highly synergistic effects of melittin with conventional antibiotics against multidrug-resistant isolates of Acinetobacter baumannii and Pseudomonas aeruginosa. Microb. Drug Resist. 25 (2), 193–202. doi:10.1089/mdr.2018.0016
Al Naggar, Y., Giesy, J. P., Abdel-Daim, M. M., Ansari, M. J., Al-Kahtani, S. N., and Yahya, G. (2021). Fighting against the second wave of COVID-19: Can honeybee products help protect against the pandemic? Saudi J. Biol. Sci. 28 (3), 1519–1527. doi:10.1016/j.sjbs.2020.12.031
Al-Rabia, M. W., Alhakamy, N. A., Ahmed, O. A. A., Eljaaly, K., Aloafi, A. L., Mostafa, A., et al. (2021). Repurposing of sitagliptin- melittin optimized nanoformula against SARS-CoV-2; antiviral screening and molecular docking studies. Pharmaceutics 13 (3), 307. doi:10.3390/pharmaceutics13030307
Alalawy, A. I., El Rabey, H. A., Almutairi, F. M., Tayel, A. A., Al Duais, M. A., Zidan, N. S., et al. (2020). Effectual anticancer potentiality of loaded bee venom onto fungal chitosan nanoparticles. Int. J. Polym. Sci. 2020, 1–9. doi:10.1155/2020/2785304
Alqarni, A. M., Dissanayake, T., Nelson, D. J., Parkinson, J. A., Dufton, M. J., Ferro, V. A., et al. (2019). Metabolomic profiling of the immune stimulatory effect of eicosenoids on PMA-differentiated THP-1 cells. Vaccines 7 (4), 142. doi:10.3390/vaccines7040142
Alqarni, A. M., Ferro, V. A., Parkinson, J. A., Dufton, M. J., and Watson, D. G. (2018). Effect of melittin on metabolomic profile and cytokine production in PMA-differentiated THP-1 cells. Vaccines 6 (4), 72. doi:10.3390/vaccines6040072
An, H. J., Kim, J. Y., Kim, W. H., Gwon, M. G., Gu, H. M., Jeon, M. J., et al. (2018). Therapeutic effects of bee venom and its major component, melittin, on atopic dermatitis in vivo and in vitro. Br. J. Pharmacol. 175 (23), 4310–4324. doi:10.1111/bph.14487
Arani, F. S., Karimzadeh, L., Ghafoori, S. M., and Nabiuni, M. (2019). Antimutagenic and synergistic cytotoxic effect of cisplatin and honey bee venom on 4T1 invasive mammary carcinoma cell line. Adv. Pharmacol. Sci. 2019, 7581318. doi:10.1155/2019/7581318
Arteaga, V., Lamas, A., Regal, P., Vázquez, B., Miranda, J. M., Cepeda, A., et al. (2019). Antimicrobial activity of apitoxin from Apis mellifera in Salmonella enterica strains isolated from poultry and its effects on motility, biofilm formation and gene expression. Microb. Pathog. 137, 103771. doi:10.1016/j.micpath.2019.103771
Aufschnaiter, A., Kohler, V., Khalifa, S., Abd El-Wahed, A., Du, M., El-Seedi, H., et al. (2020). Apitoxin and its components against cancer, neurodegeneration and rheumatoid arthritis: Limitations and possibilities. Toxins 12 (2), 66. doi:10.3390/toxins12020066
Badawi, H. M. M., Abdelsalam, R. M., Abdel-Salam, O. M. E., Youness, E. R., Shaffie, N. M., and Eldenshary, E. E. S. (2020). Bee venom attenuates neurodegeneration and motor impairment and modulates the response to L-dopa or rasagiline in a mice model of Parkinson's disease. Iran. J. Basic Med. Sci. 23 (12), 1628–1638. doi:10.22038/ijbms.2020.46469.10731
Badawi, J. K. (2021). Bee venom components as therapeutic tools against prostate cancer. Toxins 13 (5), 337. doi:10.3390/toxins13050337
Baek, H., Jang, H. L., Jeon, H. N., and Bae, H. (2018a). Comparison of administration routes on the protective effects of bee venom phospholipase A2 in a mouse model of Parkinson's disease. Front. Aging Neurosci. 10, 179. doi:10.3389/fnagi.2018.00179
Baek, H., Lee, C. J., Choi, D. D., Kim, N. S., Kim, Y. S., Ye, Y. J., et al. (2018b). Bee venom phospholipase A2 ameliorates Alzheimer's disease pathology in Aβ vaccination treatment without inducing neuro-inflammation in a 3xTg-AD mouse model. Sci. Rep. 8, 17369. doi:10.1038/s41598-018-35030-1
Baek, H., Park, S. Y., Ku, S. J., Ryu, K., Kim, Y., Bae, H., et al. (2020). Bee venom phospholipase A2 induces regulatory T cell populations by suppressing apoptotic signaling pathway. Toxins 12 (3), 198. doi:10.3390/toxins12030198
Baek, H., Yang, H., Lee, J. H., Kang, N. H., Lee, J., Bae, H., et al. (2019). Prophylactic effects of bee venom phospholipase A2 in lipopolysaccharide-induced pregnancy loss. Toxins 11 (7), 404. doi:10.3390/toxins11070404
Balamurugan, R., Kim, J. H., Jo, M. N., Xue, C., Park, J. K., and Lee, J. K. (2019). Bee wax coated water-soluble fraction of bee venom improved altered glucose homeostasis in streptozotocin-induced diabetic rats. J. Tradit. Chin. Med. 39 (6), 842–852.
Biolchi, A. M., de Oliveira, D. G. R., Amaral, H. D., Campos, G. A. A., Goncalves, J. C., de Souza, A. C. B., et al. (2020). Fraternine, a novel wasp peptide, protects against motor impairments in 6-OHDA model of parkinsonism. Toxins 12 (9), 550. doi:10.3390/toxins12090550
Borojeni, S. K., Zolfagharian, H., Babaie, M., and Javadi, I. (2020). Cytotoxic effect of bee (A. mellifera) venom on cancer cell lines. J. Pharmacopuncture 23 (4), 212–219. doi:10.3831/KPI.2020.23.4.212
Cai, J. S., Huang, K. L., Han, S. N., Chen, R. C., Li, Z. J., Chen, Y., et al. (2022). A comprehensive system review of pharmacological effects and relative mechanisms of ginsenoside Re: Recent advances and future perspectives. Phytomedicine. 102, 154119. doi:10.1016/j.phymed.2022.154119
Carpena, M., Nunez-Estevez, B., Soria-Lopez, A., and Simal-Gandara, J. (2020). Bee venom: An updating review of its bioactive molecules and its health applications. Nutrients 12 (11), 3360. doi:10.3390/nu12113360
Casewell, N. R., Wüster, W., Vonk, F. J., Harrison, R. A., and Fry, B. G. (2013). Complex cocktails: The evolutionary novelty of venoms. Trends Ecol. Evol. 28 (4), 219–229. doi:10.1016/j.tree.2012.10.020
Ceremuga, M., Stela, M., Janik, E., Gorniak, L., Synowiec, E., Sliwinski, T., et al. (2020). Melittin-A natural peptide from bee venom which induces apoptosis in human leukaemia cells. Biomolecules 10 (2), 247. doi:10.3390/biom10020247
Chang, S. N., Kim, H. J., and Lee, K. C. (2020). Melittin, A major polypeptide of bee venom, increases radiosensitivity of breast cancer in vitro and in vivo. Int. J. Radiat. Oncology*Biology*Physics 108 (3), E527. doi:10.1016/j.ijrobp.2020.07.1649
Chen, Q., Lin, W., Yin, Z., Zou, Y., Liang, S., Ruan, S., et al. (2019). Melittin inhibits hypoxia-induced vasculogenic mimicry formation and epithelial-mesenchymal transition through suppression of HIF-1α/Akt pathway in liver cancer. Evid. Based. Complement. Altern. Med. 2019, 9602935. doi:10.1155/2019/9602935
Cheon, S. Y., Chung, K. S., Roh, S. S., Cha, Y. Y., and An, H. J. (2018). Bee venom suppresses the differentiation of preadipocytes and high fat diet-induced obesity by inhibiting adipogenesis. Toxins 10 (1), 9. doi:10.3390/toxins10010009
Cherniack, E. P., and Govorushko, S. (2018). To bee or not to bee: The potential efficacy and safety of bee venom acupuncture in humans. Toxicon 154, 74–78. doi:10.1016/j.toxicon.2018.09.013
Choi, G.-M., Lee, B., Hong, R., Park, S.-Y., Cho, D.-E., Yeom, M., et al. (2021). Bee venom phospholipase A2 alleviates collagen-induced polyarthritis by inducing Foxp3(+) regulatory T cell polarization in mice. Sci. Rep. 11 (1), 3511. doi:10.1038/s41598-021-82298-x
Choi, H. S., Kang, S. Y., Roh, D. H., Choi, S. R., Ryu, Y., and Lee, J. H. (2018). Bee venom stimulation of a lung meridian acupoint reduces inflammation in carrageenan-induced pleurisy: An alternative therapeutic approach for respiratory inflammation. J. Vet. Sci. 19 (5), 708–715. doi:10.4142/jvs.2018.19.5.708
Choi, S., Chae, H. K., Heo, H., Hahm, D. H., Kim, W., and Kim, S. K. (2019). Analgesic effect of melittin on oxaliplatin-induced peripheral neuropathy in rats. Toxins 11 (7), 396. doi:10.3390/toxins11070396
Choi, S. Y., Eom, Y., Kim, J. Y., Jang, D. H., Song, J. S., and Kim, H. M. (2020). Effect of natural extract eye drops in dry eye disease rats. Int. J. Ophthalmol. 13 (7), 1023–1030. doi:10.18240/ijo.2020.07.02
Cong Duc, N., and Lee, G. (2021). Neuroprotective activity of melittin-the main component of bee venom-against oxidative stress induced by aβ25-35 in in vitro and in vivo models. Antioxidants 10 (11), 1654. doi:10.3390/antiox10111654
Conrad, V. J., Hazan, L. L., Latorre, A. J., Jakubowska, A., and Kim, C. M. H. (2019). Efficacy and safety of honey bee venom (Apis mellifera) dermal injections to treat osteoarthritis knee pain and physical disability: A randomized controlled trial. J. Altern. Complement. Med. 25 (8), 845–855. doi:10.1089/acm.2019.0121
Danesh-Seta, T., Emami, F., Nasr-Esfahani, M. H., Ghaedi, K., and Aliomrani, M. (2021). Bee venom-derived BBB shuttle and its correlation with oligodendrocyte proliferation markers in mice model of multiple sclerosis. Neurotox. Res. 39 (4), 1181–1188. doi:10.1007/s12640-021-00361-x
Daniluk, K., Kutwin, M., Grodzik, M., Wierzbicki, M., Strojny, B., Szczepaniak, J., et al. (2020). Use of selected carbon nanoparticles as melittin carriers for MCF-7 and MDA-MB-231 human breast cancer cells. Materials 13 (1), 90. doi:10.3390/ma13010090
Dehghani, B., Hasanshahi, Z., and Hashempour, T. (2020). HIV capsid and protease, new targets of melittin. Int. J. Pept. Res. Ther. 26 (4), 2057–2065. doi:10.1007/s10989-019-10002-9
Du, G., He, P., Zhao, J., He, C., Jiang, M., Zhang, Z., et al. (2021). Polymeric microneedle-mediated transdermal delivery of melittin for rheumatoid arthritis treatment. J. Control. Release 336, 537–548. doi:10.1016/j.jconrel.2021.07.005
Duffy, C., Sorolla, A., Wang, E., Golden, E., Woodward, E., Davern, K., et al. (2020). Honeybee venom and melittin suppress growth factor receptor activation in HER2-enriched and triple-negative breast cancer. NPJ Precis. Oncol. 4 (1), 24. doi:10.1038/s41698-020-00129-0
Dutta, P., Sahu, R. K., Dey, T., Lahkar, M. D., Manna, P., and Kalita, J. (2019). Beneficial role of insect-derived bioactive components against inflammation and its associated complications (colitis and arthritis) and cancer. Chem. Biol. Interact. 313, 108824. doi:10.1016/j.cbi.2019.108824
Ejaz, S., Hashmi, F. B., Malik, W. N., Ashraf, M., Nasim, F. U. H., and Iqbal, M. (2018). Applications of venom proteins as potential anticancer agents. Protein Pept. Lett. 25 (7), 688–701. doi:10.2174/0929866524666180614102104
El Bakary, N. M., Alsharkawy, A. Z., Shouaib, Z. A., and Barakat, E. M. S. (2020). Role of bee venom and melittin on restraining angiogenesis and metastasis in γ-irradiated solid Ehrlich carcinoma-bearing mice. Integr. Cancer Ther. 19, 1534735420944476. doi:10.1177/1534735420944476
El-Aarag, B., Magdy, M., AlAjmi, M. F., Khalifa, S. A. M., and El-Seedi, H. R. (2019). Melittin exerts beneficial effects on paraquat-induced lung injuries in mice by modifying oxidative stress and apoptosis. Molecules 24 (8), 1498. doi:10.3390/molecules24081498
El-Beltagy, A. F. B. M., Elsyyad, H. I. H., Abdelaziz, K. K., Madany, A. S., and Elghazaly, M. M. (2021). Therapeutic role of Annona muricata fruit and bee venom against MNU-induced breast cancer in pregnant rats and its complications on the ovaries. Breast Cancer 13, 431–445. doi:10.2147/BCTT.S306971
El-Hanoun, A., El-Komy, A., El-Sabrout, K., and Abdella, M. (2020). Effect of bee venom on reproductive performance and immune response of male rabbits. Physiol. Behav. 223, 112987. doi:10.1016/j.physbeh.2020.112987
El-Seedi, H., Abd El-Wahed, A., Yosri, N., Musharraf, S. G., Chen, L., Moustafa, M., et al. (2020). Antimicrobial properties of Apis mellifera's bee venom. Toxins 12 (7), 451. doi:10.3390/toxins12070451
El-Seedi, H. R., Khalifa, S. A. M., Abd El-Wahed, A., Gao, R. C., Guo, Z. M., Tahir, H. E., et al. (2020). Honeybee products: An updated review of neurological actions. Trends Food Sci. Technol. 101, 17–27. doi:10.1016/j.tifs.2020.04.026
Elkomy, A., El-Hanoun, A., Abdella, M., and El-Sabrout, K. (2021). Improving the reproductive, immunity and health status of rabbit does using honey bee venom. J. Anim. Physiol. Anim. Nutr. 105 (5), 975–983. doi:10.1111/jpn.13552
Fan, X. G., Pei, S. Y., Zhou, D., Zhou, P. C., Huang, Y., Hu, X. W., et al. (2020). Melittin ameliorates inflammation in mouse acute liver failure via inhibition of PKM2-mediated Warburg effect. Acta Pharmacol. Sin. 42 (8), 1256–1266. doi:10.1038/s41401-020-00516-0
Fumagalli, C., and Barberis, M. (2021). Breast cancer heterogeneity. Diagnostics 11 (9), 1555. doi:10.3390/diagnostics11091555
Gajski, G., Domijan, A. M., and Garaj-Vrhovac, V. (2012). Alterations of GSH and MDA levels and their association with bee venom-induced DNA damage in human peripheral blood leukocytes. Environ. Mol. Mutagen. 53 (6), 469–477. doi:10.1002/em.21708
Gajski, G., Domijan, A. M., Žegura, B., Štern, A., Gerić, M., Novak Jovanović, I., et al. (2016). Melittin induced cytogenetic damage, oxidative stress and changes in gene expression in human peripheral blood lymphocytes. Toxicon 110, 56–67. doi:10.1016/j.toxicon.2015.12.005
Gajski, G., and Garaj-Vrhovac, V. (2011). Bee venom induced cytogenetic damage and decreased cell viability in human white blood cells after treatment in vitro: A multi-biomarker approach. Environ. Toxicol. Pharmacol. 32 (2), 201–211. doi:10.1016/j.etap.2011.05.004
Gao, D. Q., Zhang, J. J., Bai, L., Li, F. B., Dong, Y., and Li, Q. S. (2018). Melittin induces NSCLC apoptosis via inhibition of miR-183. Onco. Targets. Ther. 11, 4511–4523. doi:10.2147/ott.s169806
Garaj-Vrhovac, V., and Gajski, G. (2009). Evaluation of the cytogenetic status of human lymphocytes after exposure to a high concentration of bee venom in vitro. Arh. Hig. Rada Toksikol. 60 (1), 27–34. doi:10.2478/10004-1254-60-2009-1896
Gasanoff, E., Liu, Y., Li, F., Hanlon, P., and Garab, G. (2021). Bee venom melittin disintegrates the respiration of mitochondria in healthy cells and lymphoblasts, and induces the formation of non-bilayer structures in model inner mitochondrial membranes. Int. J. Mol. Sci. 22 (20), 11122. doi:10.3390/ijms222011122
Giannini, D., Antonucci, M., Petrelli, F., Bilia, S., Alunno, A., and Puxeddu, I. (2020). One year in review 2020: Pathogenesis of rheumatoid arthritis. Clin. Exp. Rheumatol. 38 (3), 387–397.
Giribaldi, J., Smith, J. J., and Schroeder, C. I. (2021). Recent developments in animal venom peptide nanotherapeutics with improved selectivity for cancer cells. Biotechnol. Adv. 50, 107769. doi:10.1016/j.biotechadv.2021.107769
Goo, B., Lee, J., Park, C., Yune, T., and Park, Y. (2021). Bee venom alleviated edema and pain in monosodium urate crystals-induced gouty arthritis in rat by inhibiting inflammation. Toxins 13 (9), 661. doi:10.3390/toxins13090661
Grawish, M. E., Mourad, M. I., Esmaeil, D. A., Ahmed, R. A., Ateia, I. M., Hany, E., et al. (2020). Emerging therapeutic modality enhancing the efficiency of chemotherapeutic agents against head and neck squamous cell carcinoma cell lines. Cancer Treat. Res. Commun. 25, 100242. doi:10.1016/j.ctarc.2020.100242
Gu, H., An, H. J., Kim, J. Y., Kim, W. H., Gwon, M. G., Kim, H. J., et al. (2019). Bee venom attenuates Porphyromonas gingivalis and RANKL-induced bone resorption with osteoclastogenic differentiation. Food Chem. Toxicol. 129, 344–353. doi:10.1016/j.fct.2019.05.001
Gu, H., Han, S. M., and Park, K. K. (2020). Therapeutic effects of apamin as a bee venom component for non-neoplastic disease. Toxins 12 (3), 195. doi:10.3390/toxins12030195
Gu, H., Kim, W. H., An, H. J., Kim, J. Y., Gwon, M. G., Han, S. M., et al. (2018). Therapeutic effects of bee venom on experimental atopic dermatitis. Mol. Med. Rep. 18 (4), 3711–3718. doi:10.3892/mmr.2018.9398
Gui, Z. Z., Zhu, J. C., Ye, S., Ye, J., Chen, J., Ling, Y. Y., et al. (2020). Prolonged melittin release from polyelectrolyte-based nanocomplexes decreases acute toxicity and improves blood glycemic control in a mouse model of type II diabetes. Int. J. Pharm. 577, 119071. doi:10.1016/j.ijpharm.2020.119071
Guo, X. Y., and Ma, T. (2019). Effects of acupuncture on neurological disease in clinical- and animal-based research. Front. Integr. Neurosci. 13, 47. doi:10.3389/fnint.2019.00047
Gwon, M.-G., An, H.-J., Gu, H., Kim, Y.-A., Han, S. M., and Park, K.-K. (2021). Apamin inhibits renal fibrosis via suppressing TGF-β1 and STAT3 signaling in vivo and in vitro. J. Mol. Med. 99 (9), 1265–1277. doi:10.1007/s00109-021-02087-x
Ham, H. J., Han, J. H., Lee, Y. S., Kim, K. C., Yun, J., Kang, S. K., et al. (2019a). Bee venom soluble phospholipase A2 exerts neuroprotective effects in a lipopolysaccharide-induced mouse model of Alzheimer's disease via inhibition of nuclear factor-kappa B. Front. Aging Neurosci. 11, 287. doi:10.3389/fnagi.2019.00287
Ham, H. J., Han, S. B., Yun, J., Yeo, I. J., Ham, Y. W., Kim, S. H., et al. (2019b). Bee venom phospholipase A2 ameliorates amyloidogenesis and neuroinflammation through inhibition of signal transducer and activator of transcription-3 pathway in Tg2576 mice. Transl. Neurodegener. 8, 26. doi:10.1186/s40035-019-0167-7
Hamad, M. K., He, K., Abdulrazeq, H. F., Mustafa, A. M., Luceri, R., Kamal, N., et al. (2018). Potential uses of isolated toxin peptides in neuropathic pain relief: A literature review. World Neurosurg. 113, 333–347. e5. doi:10.1016/j.wneu.2018.01.116
Han, S. M., Kim, S. G., Jang, H. R., Woo, S. O., and Pak, S. C. (2018). Anti-atopic dermatitis of purified bee venom on keratinocytes via suppression of PAR2, ICAM-1, and IL-6 expression. J. Apic. Sci. 62 (2), 179–188. doi:10.2478/jas-2018-0016
Hanafi, M. Y., Zaher, E. L. M., El-Adely, S. E. M., Sakr, A., Ghobashi, A. H. M., Hemly, M. H., et al. (2018). The therapeutic effects of bee venom on some metabolic and antioxidant parameters associated with HFD-induced non-alcoholic fatty liver in rats. Exp. Ther. Med. 15 (6), 5091–5099. doi:10.3892/etm.2018.6028
Hematyar, M., Soleimani, M., Es-haghi, A., and Mokarram, A. R. (2018). Synergistic Co-delivery of doxorubicin and melittin using functionalized magnetic nanoparticles for cancer treatment: Loading and in vitro release study by LC-MS/MS. Artif. Cells Nanomed. Biotechnol. 46, S1226–S1235. doi:10.1080/21691401.2018.1536063
Hozzeinab, W. N., Badr, G., Badr, B. M., Allam, A., Al Ghamdi, A., Al-Wadaan, M. A., et al. (2018). Bee venom improves diabetic wound healing by protecting functional macrophages from apoptosis and enhancing Nrf2, ang-1 and tie-2 signaling. Mol. Immunol. 103, 322–335. doi:10.1016/j.molimm.2018.10.016
Huang, J.-Y., Peng, S.-F., Chueh, F.-S., Chen, P.-Y., Huang, Y.-P., Huang, W.-W., et al. (2021). Melittin suppresses epithelial-mesenchymal transition and metastasis in human gastric cancer AGS cells via regulating wnt/BMP associated pathway. Biosci. Biotechnol. Biochem. 85 (11), 2250–2262. doi:10.1093/bbb/zbab153
Huh, J. E., Seo, B. K., Lee, J. W., Kim, C., Park, Y. C., Lee, J. D., et al. (2018). Analgesic effects of diluted bee venom acupuncture mediated by δ-opioid and α2-adrenergic receptors in osteoarthritic rats. Altern. Ther. Health Med. 24 (2), 28–35.
Incorvaia, C., Mauro, M., Gritti, B. L., Makri, E., and Ridolo, E. (2018). Venom immunotherapy in patients with allergic reactions to insect stings. Expert Rev. Clin. Immunol. 14 (1), 53–59. doi:10.1080/1744666x.2018.1413350
Jeong, H., Lee, C., Cheng, C., Chou, H. C., Yang, H., and Bae, H. (2021). Targeting of adipose tissue macrophages by bee venom phospholipase A2 attenuates high-fat diet-induced obesity. Int. J. Obes. 45 (8), 1656–1667. doi:10.1038/s41366-021-00823-4
Jeong, Y. J., Park, Y. Y., Park, K. K., Choi, Y. H., Kim, C. H., and Chang, Y. C. (2019). Bee venom suppresses EGF-induced epithelial-mesenchymal transition and tumor invasion in lung cancer cells. Am. J. Chin. Med. 47 (8), 1869–1883. doi:10.1142/s0192415x19500952
Jin, Z., Yao, J., Xie, N., Cai, L., Qi, S., Zhang, Z., et al. (2018). Melittin constrains the expression of identified key genes associated with bladder cancer. J. Immunol. Res. 2018, 5038172. doi:10.1155/2018/5038172
Kang, D. W., Choi, J. G., Kim, J., Park, J. B., Lee, J. H., and Kim, H. W. (2021). Bee venom reduces burn-induced pain via the suppression of peripheral and central substance P expression in mice. J. Vet. Sci. 22 (1), e9. doi:10.4142/jvs.2021.22.e9
Kang, G. H., Lee, S. J., Choi, D. B., Shin, D., Kim, J., Yang, H., et al. (2020). Bee venom phospholipase A2 ameliorates atherosclerosis by modulating regulatory T cells. Toxins 12 (10), 609. doi:10.3390/toxins12100609
Kang, Y. M., Chung, K. S., Kook, I. H., Kook, Y. B., Bae, H., Lee, M., et al. (2018). Inhibitory effects of bee venom on mast cell-mediated allergic inflammatory responses. Int. J. Mol. Med. 41 (6), 3717–3726. doi:10.3892/ijmm.2018.3558
Kasozi, K. I., Niedbala, G., Alqarni, M., Zirintunda, G., Ssempijja, F., Musinguzi, S. P., et al. (2020). Bee venom-A potential complementary medicine candidate for SARS-CoV-2 infections. Front. Public Health 8, 594458. doi:10.3389/fpubh.2020.594458
Ke, M., Dong, J., Wang, Y., Zhang, J., Zhang, M., Wu, Z., et al. (2018). MEL-pep, an analog of melittin, disrupts cell membranes and reverses 5-fluorouracil resistance in human hepatocellular carcinoma cells. Int. J. Biochem. Cell. Biol. 101, 39–48. doi:10.1016/j.biocel.2018.05.013
Khalil, A., Elesawy, B. H., Ali, T. M., and Ahmed, O. M. (2021). Bee venom: From venom to drug. Molecules 26 (16), 4941. doi:10.3390/molecules26164941
Khamis, A. A. A., Ali, E. M. M., Abd El-Moneim, M. A., Abd-Alhaseeb, M. M., Abu El-Magd, M., and Salim, E. I. (2018). Hesperidin, piperine and bee venom synergistically potentiate the anticancer effect of tamoxifen against breast cancer cells. Biomed. Pharmacother. 105, 1335–1343. doi:10.1016/j.biopha.2018.06.105
Kim, A., Lee, S. Y., Kim, B. Y., and Chung, S. K. (2020). Elimination of teratogenic human induced pluripotent stem cells by bee venom via calcium-calpain pathway. Int. J. Mol. Sci. 21 (9), 3265. doi:10.3390/ijms21093265
Kim, D. H., Han, S. M., Keum, M. C., Lee, S., An, B. K., Lee, S. R., et al. (2018). Evaluation of bee venom as a novel feed additive in fast-growing broilers. Br. Poult. Sci. 59 (4), 435–442. doi:10.1080/00071668.2018.1476675
Kim, D. H., Lee, H. W., Park, H. W., and Chun, K. H. (2020). Bee venom inhibits the proliferation and migration of cervical-cancer cells in an HPV E6/E7-dependent manner. BMB Rep. 53 (8), 419–424. doi:10.5483/BMBRep.2020.53.8.031
Kim, H., Hong, J. Y., Jeon, W. J., Baek, S. H., and Ha, I. H. (2020). Bee venom melittin protects against cisplatin-induced acute kidney injury in mice via the regulation of M2 macrophage activation. Toxins 12 (9), 574. doi:10.3390/toxins12090574
Kim, H., Hong, J. Y., Lee, J., Jeon, W.-J., and Ha, I.-H. (2021). Apamin enhances neurite outgrowth and regeneration after laceration injury in cortical neurons. Toxins 13 (9), 603. doi:10.3390/toxins13090603
Kim, H. Y., Jo, M. J., Nam, S. Y., Kim, K. M., Choi, M. B., and Lee, Y. H. (2020). Evaluating the effects of honey bee (Apis mellifera L.) venom on the expression of insulin sensitivity and inflammation-related genes in Co-culture of adipocytes and macrophages. Entomol. Res. 50 (5), 236–244. doi:10.1111/1748-5967.12431
Kim, J. Y., Jang, H.-J., Leem, J., and Kim, G.-M. (2021b). Protective effects of bee venom-derived phospholipase A2 against cholestatic liver disease in mice. Biomedicines 9 (8), 992. doi:10.3390/biomedicines9080992
Kim, J. Y., Leem, J., and Hong, H. L. (2021a). Melittin ameliorates endotoxin-induced acute kidney injury by inhibiting inflammation, oxidative stress, and cell death in mice. Oxid. Med. Cell. Longev. 2021, 8843051. doi:10.1155/2021/8843051
Kim, J. Y., Lee, S. J., Maeng, Y. I., Leem, J., and Park, K. K. (2020a). Protective effects of bee venom against endotoxemia-related acute kidney injury in mice. Biology 9 (7), 154. doi:10.3390/biology9070154
Kim, J. Y., Leem, J., and Park, K. K. (2020b). Antioxidative, antiapoptotic, and anti-inflammatory effects of apamin in a murine model of lipopolysaccharide-induced acute kidney injury. Molecules 25 (23), 5717. doi:10.3390/molecules25235717
Kim, K. H., Kim, M., Lee, J., Jeon, H. N., Kim, S. H., and Bae, H. (2019a). Comparison of the protective effects of bee venom extracts with varying PLA2 compositions in a mouse model of Parkinson's disease. Toxins 11 (6), 358. doi:10.3390/toxins11060358
Kim, K. H., Lee, S. Y., Shin, J., Hwang, J. T., Jeon, H. N., and Bae, H. (2019b). Dose-dependent neuroprotective effect of standardized bee venom phospholipase A2 against MPTP-induced Parkinson's disease in mice. Front. Aging Neurosci. 11, 80. doi:10.3389/fnagi.2019.00080
Kim, M., and Han, C. H. (2020). Pharmacopuncture for stroke survivors: A systematic review of randomized controlled trials in South Korea. Complement. Ther. Clin. Pract. 40, 101179. doi:10.1016/j.ctcp.2020.101179
Kim, S., Kim, H.-W., Chang, S.-H., Leem, K.-H., and Park, H.-J. (2021). Bee venom prevents mucin 5AC production through inhibition of AKT and SPDEF activation in airway epithelia cells. Toxins 13 (11), 773. doi:10.3390/toxins13110773
Kim, W. H., An, H. J., Kim, J. Y., Gwon, M. G., Gu, H., Jeon, M., et al. (2018). Anti-inflammatory effect of melittin on Porphyromonas gingivalis LPS-stimulated human keratinocytes. Molecules 23 (2), 332. doi:10.3390/molecules23020332
Kim, Y., Lee, Y. W., Kim, H., and Chung, D. K. (2019). Bee venom alleviates atopic dermatitis symptoms through the upregulation of decay-accelerating factor (DAF/CD55). Toxins 11 (5), 239. doi:10.3390/toxins11050239
Ko, S. J., Park, E., Asandei, A., Choi, J. Y., Lee, S. C., Seo, C. H., et al. (2020). Bee venom-derived antimicrobial peptide melectin has broad-spectrum potency, cell selectivity, and salt-resistant properties. Sci. Rep. 10 (1), 10145. doi:10.1038/s41598-020-66995-7
Kocyigit, A., Guler, E. M., and Kaleli, S. (2019). Anti-inflammatory and antioxidative properties of honey bee venom on Freund's complete adjuvant-induced arthritis model in rats. Toxicon 161, 4–11. doi:10.1016/j.toxicon.2019.02.016
Kong, R., Lee, Y. S., Kang, D. H., Wang, S., Li, Q. Q., Kwon, D. Y., et al. (2020). The antibacterial activity and toxin production control of bee venom in mouse MRSA pneumonia model. BMC Complement. Med. Ther. 20 (1), 238. doi:10.1186/s12906-020-02991-8
Kreinest, T., Volkmer, I., and Staege, M. S. (2021). Melittin increases cisplatin sensitivity and kills KM-H2 and L-428 Hodgkin lymphoma cells. Int. J. Mol. Sci. 22 (1), 343. doi:10.3390/ijms22010343
Ku, Y. H., Kang, J. H., and Lee, H. (2020). Effect of bee venom on an experimental cellular model of Alzheimer's disease. Am. J. Chin. Med. 48 (8), 1803–1819. doi:10.1142/s0192415x20500901
Kurek-Gorecka, A., Komosinska-Vassev, K., Rzepecka-Stojko, A., and Olczyk, P. (2021). Bee venom in wound healing. Molecules 26 (1), 148. doi:10.3390/molecules26010148
Lebel, A. A., Kisembo, M. V., Soucy, M.-F. N., Hebert, M. P. A., Morin, P., and Boudreau, L. H. (2021). Molecular characterization of the anticancer properties associated with bee venom and its components in glioblastoma multiforme. Chem. Biol. Interact. 347, 109622. doi:10.1016/j.cbi.2021.109622
Lee, G., Kang, G. H., and Bae, H. (2019). Bee venom phospholipase A2 suppression of experimental autoimmune encephalomyelitis is dependent on its enzymatic activity. Mol. Cell. Toxicol. 15 (3), 307–313. doi:10.1007/s13273-019-0034-8
Lee, J. E., Shah, V. K., Lee, E. J., Oh, M. S., and Choi, J. J. (2019). Melittin - a bee venom component - enhances muscle regeneration factors expression in a mouse model of skeletal muscle contusion. J. Pharmacol. Sci. 140 (1), 26–32. doi:10.1016/j.jphs.2019.03.009
Lee, J. H., Gang, J., Yang, E., Kim, W., and Jin, Y. H. (2020). Bee venom acupuncture attenuates oxaliplatin-induced neuropathic pain by modulating action potential threshold in A-fiber dorsal root ganglia neurons. Toxins 12 (12), 737. doi:10.3390/toxins12120737
Lee, J., Kim, Y. M., Kim, J. H., Cho, C. W., Jeon, J. W., Park, J. K., et al. (2018). Nasal delivery of chitosan/alginate nanoparticle encapsulated bee (Apis mellifera) venom promotes antibody production and viral clearance during porcine reproductive and respiratory syndrome virus infection by modulating T cell related responses. Vet. Immunol. Immunopathol. 200, 40–51. doi:10.1016/j.vetimm.2018.04.006
Lee, Y. J., Oh, M. J., Lee, D. H., Lee, Y. S., Lee, J., Kim, D. H., et al. (2020). Anti-inflammatory effect of bee venom in phthalic anhydride-induced atopic dermatitis animal model. Inflammopharmacology 28 (1), 253–263. doi:10.1007/s10787-019-00646-w
Lee, Y. M., Cho, S. N., Son, E., Song, C. H., and Kim, D. S. (2020). Apamin from bee venom suppresses inflammation in a murine model of gouty arthritis. J. Ethnopharmacol. 257, 112860. doi:10.1016/j.jep.2020.112860
Li, D. X., Chung, G., and Kim, S. K. (2020a). The involvement of central noradrenergic pathway in the analgesic effect of bee venom acupuncture on vincristine-induced peripheral neuropathy in rats. Toxins 12 (12), 775. doi:10.3390/toxins12120775
Li, D. X., Yoo, J. H., and Kim, S. K. (2020b). Long-lasting and additive analgesic effects of combined treatment of bee venom acupuncture and venlafaxine on paclitaxel-induced allodynia in mice. Toxins 12 (10), 620. doi:10.3390/toxins12100620
Li, Y., Xu, N., Zhu, W., Wang, L., Liu, B., Zhang, J., et al. (2018). Nanoscale melittin@ zeolitic imidazolate frameworks for enhanced anticancer activity and mechanism analysis. ACS Appl. Mat. Interfaces 10 (27), 22974–22984. doi:10.1021/acsami.8b06125
Liang, X. L., Yan, J. X., Lu, Y. W., Liu, S., and Chai, X. J. (2021). The antimicrobial peptide melectin shows both antimicrobial and antitumor activity via membrane interference and DNA binding. Drug Des. devel. Ther. 15, 1261–1273. doi:10.2147/dddt.s288219
Lim, H. N., Baek, S. B., and Jung, H. J. (2019). Bee venom and its peptide component melittin suppress growth and migration of melanoma cells via inhibition of PI3K/AKT/mTOR and MAPK pathways. Molecules 24 (5), 929. doi:10.3390/molecules24050929
Lima, W. G., Brito, J. C. M., and Nizer, W. S. D. (2021). Bee products as a source of promising therapeutic and chemoprophylaxis strategies against COVID-19 (SARS-CoV-2). Phytother. Res. 35 (2), 743–750. doi:10.1002/ptr.6872
Lin, Z., Xie, R., Zhong, C., Huang, J., Shi, P., and Yao, H. (2022). Recent progress (2015-2020) in the investigation of the pharmacological effects and mechanisms of ginsenoside Rb1, a main active ingredient in Panax ginseng meyer. J. Ginseng Res. 46 (1), 39–53. doi:10.1016/j.jgr.2021.07.008
Lischer, K., Sitorus, S. R. A., Guslianto, B. W., Avila, F., Khayrani, A. C., and Sahlan, M. (2021). Anti-breast cancer activity on MCF-7 cells of melittin from Indonesia’s Apis cerana: An in vitro study. Asian pac. J. Cancer Prev. 22 (12), 3913–3919. doi:10.31557/APJCP.2021.22.12.3913
Liu, X. Q., Jin, J. R., Liu, G. M., Peng, F., Zhang, C. G., Guo, M. X., et al. (2021). Effect of wasp venom from vespa mandarinia smith on the proliferation and apoptosis of MH7A cells. Lat. Am. J. Pharm. 40 (2), 235–243.
Luo, L., Wu, W., Sun, D., Dai, H.-B., Wang, Y., Zhong, Y., et al. (2018). Acid-activated melittin for targeted and safe antitumor therapy. Bioconjug. Chem. 29 (9), 2936–2944. doi:10.1021/acs.bioconjchem.8b00352
Lv, C., Zhang, Z., Zhao, T., Han, M. F., Jia, D. P., Su, L. Z., et al. (2019). The anti-tumour effect of mel and its role in autophagy in human hepatocellular carcinoma cells. Am. J. Transl. Res. 11 (2), 931–941.
Lyu, C., Fang, F. F., and Li, B. (2019). Anti-tumor effects of melittin and its potential applications in clinic. Curr. Protein Pept. Sci. 20 (3), 240–250. doi:10.2174/1389203719666180612084615
Maitip, J., Mookhploy, W., Khorndork, S., and Chantawannakul, P. (2021). Comparative study of antimicrobial properties of bee venom extracts and melittins of honey bees. Antibiotics 10 (12), 1503. doi:10.3390/antibiotics10121503
Mansour, G. H., El-Magd, M. A., Mahfouz, D. H., Abdelhamid, I. A., Mohamed, M. F., Ibrahim, N. S., et al. (2021). Bee venom and its active component melittin synergistically potentiate the anticancer effect of sorafenib against HepG2 cells. Bioorg. Chem. 116, 105329. doi:10.1016/j.bioorg.2021.105329
Meimandi-Parizi, A., Oryan, A., Bigham-Sadegh, A., and Sayahi, E. (2018). Effects of chitosan scaffold along with royal jelly or bee venom in regeneration of critical sized radial bone defect in rat. Iran. J. Vet. Res. 19 (4), 246–254.
Meligi, N. M., Ismail, S. A., and Tawfik, N. S. (2020). Protective effects of honey and bee venom against lipopolysaccharide and carbon tetrachloride-induced hepatoxicity and lipid peroxidation in rats. Toxicol. Res. 9 (5), 693–705. doi:10.1093/toxres/tfaa077
Mir Hassani, Z., Nabiuni, M., Parivar, K., Abdirad, S., and Karimzadeh, L. (2021). Melittin inhibits the expression of key genes involved in tumor microenvironment formation by suppressing HIF-1α signaling in breast cancer cells. Med. Oncol. 38 (7), 77. doi:10.1007/s12032-021-01526-6
Mirzaei, S., Fekri, H. S., Hashemi, F., Hushmandi, K., Mohammadinejad, R., Ashrafizadeh, M., et al. (2021). Venom peptides in cancer therapy: An updated review on cellular and molecular aspects. Pharmacol. Res. 164, 105327. doi:10.1016/j.phrs.2020.105327
Moghaddam, F. D., Akbarzadeh, I., Marzbankia, E., Farid, M., Khaledi, L., Reihani, A. H., et al. (2021). Delivery of melittin-loaded niosomes for breast cancer treatment: An in vitro and in vivo evaluation of anti-cancer effect. Cancer Nanotechnol. 12 (1), 14. doi:10.1186/s12645-021-00085-9
Moghaddam, F. D., Mortazavi, P., Hamedi, S., Nabiuni, M., and Roodbari, N. H. (2020). Apoptotic effects of melittin on 4T1 breast cancer cell line is associated with up regulation of Mfn1 and Drp1 mRNA expression. Anticancer. Agents Med. Chem. 20 (7), 790–799. doi:10.2174/1871520620666200211091451
Mohamed, W. A., Abd-Elhakim, Y. M., and Ismail, S. A. A. (2019). Involvement of the anti-inflammatory, anti-apoptotic, and anti-secretory activity of bee venom in its therapeutic effects on acetylsalicylic acid-induced gastric ulceration in rats. Toxicology 419, 11–23. doi:10.1016/j.tox.2019.03.003
Motiei, M., Aboutalebi, F., Forouzanfar, M., Dormiani, K., Nasr-Esfahani, M. H., and Mirahmadi-Zare, S. Z. (2021). Smart Co-delivery of miR-34a and cytotoxic peptides (LTX-315 and melittin) by chitosan based polyelectrolyte nanocarriers for specific cancer cell death induction. Mat. Sci. Eng. C Mat. Biol. Appl. 128, 112258. doi:10.1016/j.msec.2021.112258
Naji, K. M., Al-Khatib, B. Y., Al-Haj, N. S., and D'Souza, M. R. (2021). Hepatoprotective activity of melittin on isoniazid- and rifampicin-induced liver injuries in male albino rats. BMC Pharmacol. Toxicol. 22 (1), 39. doi:10.1186/s40360-021-00507-9
Nakashima, A., Tomono, S., Yamazaki, T., Inui, M., Morita, N., Ichimonji, I., et al. (2020). Phospholipase A2 from bee venom increases poly(I:C)-Induced activation in human keratinocytes. Int. Immunol. 32 (6), 371–383. doi:10.1093/intimm/dxaa005
Nehme, H., Ayde, H., El Obeid, D., Sabatier, J. M., and Fajloun, Z. (2020). Potential inhibitory effect of Apis mellifera's venom and of its two main components-melittin and PLA2-on Escherichia coli F1F0-ATPase. Antibiotics 9 (11), 824. doi:10.3390/antibiotics9110824
Nguyen, H. V., Heger, Z., Kominkova, M., Michalek, P., Gumulec, J., Guran, R., et al. (2015). The electrochemical and statistical evaluation of isolation of mellitin and apamin from honey bee (Apis mellifera) venom. Int. J. Electrochem. Sc. 10, 1249–1260.
Nikodijevic, D. D., Milutinovic, M. G., Cvetkovic, D. M., Cupurdija, M. D., Jovanovic, M. M., Mrkic, I. V., et al. (2021). Impact of bee venom and melittin on apoptosis and biotransformation in colorectal carcinoma cell lines. Toxin Rev. 40 (4), 1272–1279. doi:10.1080/15569543.2019.1680564
Ombredane, A. S., de Andrade, L. R., Bonadio, R. S., Pinheiro, W. O., de Azevedo, R. B., and Joanitti, G. A. (2021). Melittin sensitizes skin squamous carcinoma cells to 5-fluorouracil by affecting cell proliferation and survival. Exp. Dermatol. 30 (5), 710–716. doi:10.1111/exd.14289
Palgan, K., Zbikowska-Gotz, M., and Bartuzi, Z. (2020). Expression of eosinophils, RANTES and IL-25 in the first phase of Hymenoptera venom immunotherapy. Postepy Dermatol. Alergol. 37 (4), 590–596. doi:10.5114/ada.2019.83655
Park, J., Jang, K. M., and Park, K. K. (2020). Apamin suppresses LPS-induced neuroinflammatory responses by regulating SK channels and TLR4-mediated signaling pathways. Int. J. Mol. Sci. 21 (12), 4319. doi:10.3390/ijms21124319
Park, J., Kwon, O., An, H. J., and Park, K. K. (2018). Antifungal effects of bee venom components on Trichophyton rubrum: A novel approach of bee venom study for possible emerging antifungal agent. Ann. Dermatol. 30 (2), 202–210. doi:10.5021/ad.2018.30.2.202
Pashaei, F., Bevalian, P., Akbari, R., and Bagheri, K. P. (2019). Single dose eradication of extensively drug resistant Acinetobacter spp. in a mouse model of burn infection by melittin antimicrobial peptide. Microb. Pathog. 127, 60–69. doi:10.1016/j.micpath.2018.11.055
Pattabhiramaiah, M., Ramesh, K., Vishwanath, K. V., and Reddy, S. M. (2020). Computational analysis of PhospholipaseA2 in the honey bee venom. J. Apic. Res. 59 (4), 706–721. doi:10.1080/00218839.2020.1754589
Pereira, A. F. M., Albano, M., Alves, F. C. B., Andrade, B. F. M. T., Furlanetto, A., Rall, V. L. M., et al. (2020). Influence of apitoxin and melittin from Apis mellifera bee on Staphylococcus aureus strains. Microb. Pathog. 141, 104011. doi:10.1016/j.micpath.2020.104011
Putz, T., Ramoner, R., Gander, H., Rahm, A., Bartsch, G., Bernardo, K., et al. (2007). Bee venom secretory phospholipase A2 and phosphatidylinositol-homologues cooperatively disrupt membrane integrity, abrogate signal transduction and inhibit proliferation of renal cancer cells. Cancer Immunol. Immunother. 56 (5), 627–640. doi:10.1007/s00262-006-0220-0
Qi, J. X., Chen, Y. H., Xue, T. T., Lin, Y., Huang, S. Y., Cao, S. Y., et al. (2020). Graphene oxide-based magnetic nanocomposites for the delivery of melittin to cervical cancer HeLa cells. Nanotechnology 31 (6), 065102. doi:10.1088/1361-6528/ab5084
Raghuraman, H., and Chattopadhyay, A. (2007). Melittin: A membrane-active peptide with diverse functions. Biosci. Rep. 27 (4-5), 189–223. doi:10.1007/s10540-006-9030-z
Rakha, M. K., Tawfiq, R. A., Sadek, M. M., Anwer, M. A., Salama, S. M., Mohamed, A. F., et al. (2018). Neurotherapeutic effects of bee venom in a rotenone-induced mouse model of Parkinson's disease. Neurophysiology 50 (6), 445–455. doi:10.1007/s11062-019-09777-w
Raveendran, R., Chen, F., Kent, B., and Stenzel, M. H. (2020). Estrone-decorated poly-ion complex micelles for targeted melittin delivery to hormone-responsive breast cancer cells. Biomacromolecules 21 (3), 1222–1233. doi:10.1021/acs.biomac.9b01681
Sangboonruang, S., Kitidee, K., Chantawannakul, P., Tragoolpua, K., and Tragoolpua, Y. (2020). Melittin from Apis florea venom as a promising therapeutic agent for skin cancer treatment. Antibiotics 9 (8), 517. doi:10.3390/antibiotics9080517
Sarhan, M., El-Bitar, A. M. H., and Hotta, H. (2020). Potent virucidal activity of honeybee "Apis mellifera" venom against Hepatitis C Virus. Toxicon 188, 55–64. doi:10.1016/j.toxicon.2020.10.014
Shaw, P., Kumar, N., Hammerschmid, D., Privat-Maldonado, A., Dewilde, S., and Bogaerts, A. (2019). Synergistic effects of melittin and plasma treatment: A promising approach for cancer therapy. Cancers 11 (8), 1109. doi:10.3390/cancers11081109
Shin, D., Choi, W., and Bae, H. (2018). Bee venom phospholipase A2 alleviate house dust mite-induced atopic dermatitis-like skin lesions by the CD206 mannose receptor. Toxins 10 (4), 146. doi:10.3390/toxins10040146
Shin, S. H., Ye, M. K., Choi, S. Y., and Park, K. K. (2018). Anti-inflammatory effect of bee venom in an allergic chronic rhinosinusitis mouse model. Mol. Med. Rep. 17 (5), 6632–6638. doi:10.3892/mmr.2018.8720
Siegel, R. L., Miller, K. D., Fuchs, H. E., and Jemal, A. (2021). Cancer statistics, 2021. Ca. Cancer J. Clin. 71 (1), 7–33. doi:10.3322/caac.21654
Soliman, C., Eastwood, S., Truong, V. K., Ramsland, P. A., and Elbourne, A. (2019). The membrane effects of melittin on gastric and colorectal cancer. PLoS One 14 (10), e0224028. doi:10.1371/journal.pone.0224028
Stoevesandt, J., Sturm, G. J., Bonadonna, P., Elberink, J. N. G. O., and Trautmann, A. (2020). Risk factors and indicators of severe systemic insect sting reactions. Allergy 75 (3), 535–545. doi:10.1111/all.13945
Sturm, G. J., Varga, E.-M., Roberts, G., Mosbech, H., Bilò, M. B., Akdis, C. A., et al. (2018). EAACI guidelines on allergen immunotherapy: Hymenoptera venom allergy. Allergy 73 (4), 744–764. doi:10.1111/all.13262
Sugarbaker, D. J., and Dasilva, M. C. (2011). Diagnostic workup of lung cancer. Surg. Oncol. Clin. N. Am. 20 (4), 667–679. doi:10.1016/j.soc.2011.08.003
Sung, H., Ferlay, J., Siegel, R. L., Laversanne, M., Soerjomataram, I., Jemal, A., et al. (2021). Global cancer statistics 2020: GLOBOCAN estimates of incidence and mortality worldwide for 36 cancers in 185 countries. Ca. Cancer J. Clin. 71 (3), 209–249. doi:10.3322/caac.21660
Tang, C. H. (2019). Research of pathogenesis and novel therapeutics in arthritis. Int. J. Mol. Sci. 20 (7), 1646. doi:10.3390/ijms20071646
Tang, F. Q., Wu, H., Zheng, J. Z., Guo, H. L., Yan, L. P., Lin, Y. S., et al. (2021). Effect of melittin on iNOS and NF-κB expression induced by IL-1β in C518 cells. Pak. J. Pharm. Sci. 34 (1), 95–101. doi:10.36721/pjps.2021.34.1.reg.095-101.1
Tanuwidjaja, I., Svecnjak, L., Gugic, D., Levanic, M., Juric, S., Vincekovic, M., et al. (2021). Chemical profiling and antimicrobial properties of honey bee (Apis mellifera L.) venom. Molecules 26 (10), 3049. doi:10.3390/molecules26103049
Tipgomut, C., Wongprommoon, A., Takeo, E., Ittiudomrak, T., Puthong, S., and Chanchao, C. (2018). Melittin induced G1 cell cycle arrest and apoptosis in chago-K1 human bronchogenic carcinoma cells and inhibited the differentiation of THP-1 cells into tumour- associated macrophages. Asian pac. J. Cancer Prev. 19 (12), 3427–3434. doi:10.31557/APJCP.2018.19.12.3427
Uzair, B., Bushra, R., Khan, B. A., Zareen, S., and Fasim, F. (2018). Potential uses of venom proteins in treatment of HIV. Protein Pept. Lett. 25 (7), 619–625. doi:10.2174/0929866525666180628161107
Uzuner, S. C., Birinci, E., Tetikoglu, S., Birinci, C., and Kolayli, S. (2021). Distinct epigenetic reprogramming, mitochondrial patterns, cellular morphology, and cytotoxicity after bee venom treatment. Recent Pat. anticancer. Drug Discov. 16 (3), 377–392. doi:10.2174/1574892816666210422125058
Viana, G. A., Freitas, C. I. A., de Almeida, J. G. L., de Medeiros, G. V. D., Teofilo, T. D., Rodrigues, V. H. V., et al. (2021). Antioxidant, genotoxic, antigenotoxic, and antineoplastic activities of apitoxin produced by Apis mellifera in northeast, Brazil. Cienc. Rural. 51 (4), e20200545. doi:10.1590/0103-8478cr20200545
Volkova, L. V., Pashov, A. I., and Omelchuk, N. N. (2021). Cervical carcinoma: Oncobiology and biomarkers. Int. J. Mol. Sci. 22 (22), 12571. doi:10.3390/ijms222212571
Wang, H., Wang, S., Wang, R., Wang, X., Jiang, K., Xie, C., et al. (2019). Co-Delivery of paclitaxel and melittin by glycopeptide-modified lipodisks for synergistic anti-glioma therapy. Nanoscale 11 (27), 13069–13077. doi:10.1039/c9nr01820d
Wang, L., Zhao, X. Q., Zhu, C. L., Zhao, Y. Y., Liu, S. S., Xia, X. J., et al. (2020). The antimicrobial peptide MPX kills Actinobacillus pleuropneumoniae and reduces its pathogenicity in mice. Vet. Microbiol. 243, 108634. doi:10.1016/j.vetmic.2020.108634
Wang, X., Li, H., Lu, X., Wen, C., Huo, Z., Shi, M., et al. (2018). Melittin-induced long non-coding RNA NONHSAT105177 inhibits proliferation and migration of pancreatic ductal adenocarcinoma. Cell. Death Dis. 9 (10), 940. doi:10.1038/s41419-018-0965-3
Wattanakul, K., Imae, T., Chang, W. W., Chu, C. C., Nakahata, R., and Yusa, S. (2019). Oligopeptide-side chained alginate nanocarrier for melittin-targeted chemotherapy. Polym. J. 51 (8), 771–780. doi:10.1038/s41428-019-0191-6
Wehbe, R., Frangieh, J., Rima, M., El Obeid, D., Sabatier, J.-M., and Fajloun, Z. (2019). Bee venom: Overview of main compounds and bioactivities for therapeutic interests. Molecules 24 (16), 2997. doi:10.3390/molecules24162997
Wen, Y. J., Zhu, M. L., Zhang, X. R., Xiao, H. D., Wei, Y., and Zhao, P. Q. (2022). Integrated analysis of multiple bioinformatics studies to identify microRNA-target gene-transcription factor regulatory networks in retinoblastoma. Transl. Cancer Res. 11 (7), 2225–2237. doi:10.21037/tcr-21-1748
Woo, S., Chung, G., Bae, H., and Kim, S. K. (2019). Suppressive effects of bee venom-derived phospholipase A2 on mechanical allodynia in a rat model of neuropathic pain. Toxins 11 (8), 477. doi:10.3390/toxins11080477
Yaacoub, C., Rifi, M., El-Obeid, D., Mawlawi, H., Sabatier, J.-M., Coutard, B., et al. (2021). The cytotoxic effect of Apis mellifera venom with a synergistic potential of its two main components-melittin and PLA2-on colon cancer HCT116 cell lines. Molecules 26 (8), 2264. doi:10.3390/molecules26082264
Yang, W., Hu, F. L., and Xu, X. F. (2020). Bee venom and SARS-CoV-2. Toxicon 181, 69–70. doi:10.1016/j.toxicon.2020.04.105
Yao, J., Zhang, Z., Li, S., Li, B., and Wang, X. H. (2020). Melittin inhibits proliferation, migration and invasion of bladder cancer cells by regulating key genes based on bioinformatics and experimental assays. J. Cell. Mol. Med. 24 (1), 655–670. doi:10.1111/jcmm.14775
Ye, R., Zheng, Y., Chen, Y., Wei, X., Shi, S., Chen, Y., et al. (2021). Stable loading and delivery of melittin with lipid-coated polymeric nanoparticles for effective tumor therapy with negligible systemic toxicity. ACS Appl. Mat. Interfaces 13 (47), 55902–55912. doi:10.1021/acsami.1c17618
Yoon, J. E., Hong, K., and Han, S. G. (2018). Antiproliferative effects of bee venom in human lung and breast cancer cells by decreasing Cyclin D1 and NF-kappa B. Curr. Top. Nutraceutical Res. 16 (4), 235–244.
Yu, G. H., Wang, L. -G., Yan, G. -R., and He, Q. -Y. (2015). DOSE: An R/bioconductor package for disease ontology semantic and enrichment analysis. Bioinformatics 31 (4), 608–609. doi:10.1093/bioinformatics/btu684
Yu, R., Wang, M., Wang, M., and Han, L. (2020). Melittin suppresses growth and induces apoptosis of non-small-cell lung cancer cells via down-regulation of TGF-β-mediated ERK signal pathway. Braz. J. Med. Biol. Res. 54 (2), e9017. doi:10.1590/1414-431X20209017
Yu, X., Gou, X., Wu, P., Han, L., Tian, D., Du, F., et al. (2018). Activatable protein nanoparticles for targeted delivery of therapeutic peptides. Adv. Mat. 30 (7), 1705383. doi:10.1002/adma.201705383
Yun, H. S., Oh, J., Lim, J. S., Kim, H. J., and Kim, J.-S. (2021). Anti-inflammatory effect of wasp venom in BV-2 microglial cells in comparison with bee venom. Insects 12 (4), 297. doi:10.3390/insects12040297
Zahran, F., Mohamad, A., and Zein, N. (2021). Bee venom ameliorates cardiac dysfunction in diabetic hyperlipidemic rats. Exp. Biol. Med. 246 (24), 2630–2644. doi:10.1177/15353702211045924
Zare, M., Khayatzadeh, J., Nia, H. S., Mojarad, M., Baghbani, F., Sargolzaei, J., et al. (2019). Effects of bee venom on activity and expression of 15-lipoxygenase-1 in human HT29 colon cancer. Acta Poloniae Pharm. - Drug Res. 76 (1), 75–82. doi:10.32383/appdr/92651
Zarrinnahad, H., Mahmoodzadeh, A., Hamidi, M. P., Mahdavi, M., Moradi, A., Bagheri, K. P., et al. (2018). Apoptotic effect of melittin purified from Iranian honey bee venom on human cervical cancer HeLa cell line. Int. J. Pept. Res. Ther. 24 (4), 563–570. doi:10.1007/s10989-017-9641-1
Zhang, S., Lv, X., Li, L., Luo, Y., Xiang, H., Wang, L., et al. (2021). Melittin inhibited glycolysis and induced cell apoptosis in cisplatin-resistant lung adenocarcinoma cells via TRIM8. Biocell 45 (1), 167–175. doi:10.32604/biocell.2021.013636
Zhong, C., Lin, Z., Ke, L., Shi, P., Li, S., Huang, L., et al. (2021). Recent research progress (2015-2021) and perspectives on the pharmacological effects and mechanisms of Tanshinone IIA. Front. Pharmacol. 12, 778847. doi:10.3389/fphar.2021.778847
Zhou, J., Wan, C., Cheng, J., Huang, H., Lovell, J. F., and Jin, H. (2021). Delivery strategies for melittin-based cancer therapy. Acs Appl. Mat. Interfaces 13 (15), 17158–17173. doi:10.1021/acsami.1c03640
Keywords: bee venom, melittin, phospholipase A2, pharmacological effect, effect mechanism, bioinformatic analysis
Citation: Shi P, Xie S, Yang J, Zhang Y, Han S, Su S and Yao H (2022) Pharmacological effects and mechanisms of bee venom and its main components: Recent progress and perspective. Front. Pharmacol. 13:1001553. doi: 10.3389/fphar.2022.1001553
Received: 23 July 2022; Accepted: 29 August 2022;
Published: 27 September 2022.
Edited by:
Michelle Yap, Monash University Malaysia, MalaysiaReviewed by:
Goran Gajski, Institute for Medical Research and Occupational Health, CroatiaWoojin Kim, Kyung Hee University, South Korea
Copyright © 2022 Shi, Xie, Yang, Zhang, Han, Su and Yao. This is an open-access article distributed under the terms of the Creative Commons Attribution License (CC BY). The use, distribution or reproduction in other forums is permitted, provided the original author(s) and the copyright owner(s) are credited and that the original publication in this journal is cited, in accordance with accepted academic practice. No use, distribution or reproduction is permitted which does not comply with these terms.
*Correspondence: Peiying Shi, cGVpeXNoaUAxMjYuY29t; Hong Yao, aG9uZ3lhb0BtYWlsLmZqbXUuZWR1LmNu
†These authors have contributed equally to this work