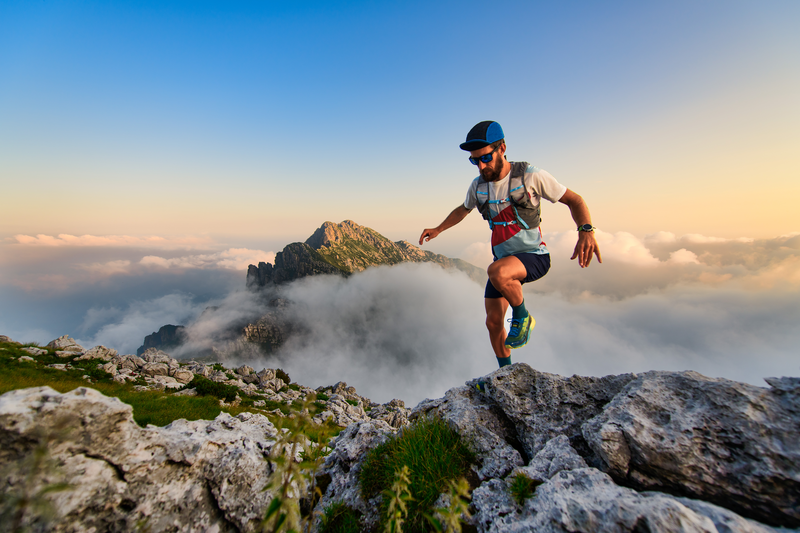
95% of researchers rate our articles as excellent or good
Learn more about the work of our research integrity team to safeguard the quality of each article we publish.
Find out more
REVIEW article
Front. Pharmacol. , 25 October 2022
Sec. Ethnopharmacology
Volume 13 - 2022 | https://doi.org/10.3389/fphar.2022.1000865
Rheumatoid arthritis (RA) is an autoimmune disease whose hallmarks are synovial inflammation and irreversible bone destruction. Bone resorption resulting from osteoclasts involves the whole immune and bone systems. Breakdown of bone remodeling is attributed to overactive immune cells that produce large quantities of cytokines, upregulated differentiation of osteoclasts with enhanced resorptive activities, suppressed differentiation of osteoblasts, invading fibroblasts and microbiota dysbiosis. Despite the mitigation of inflammation, the existing treatment in Western medicine fails to prevent bone loss during disease progression. Traditional Chinese medicine (TCM) has been used for thousands of years in RA treatment, showing great efficacy in bone preservation. The complex components from the decoctions and prescriptions exhibit various pharmacological activities. This review summarizes the research progress that has been made in terms of the bone-protective effect of some representative compounds from TCM drugs and proposes the substantial mechanisms involved in bone metabolism to provide some clues for future studies. These active components systemically suppress bone destruction via inhibiting joint inflammation, osteoclast differentiation, and fibroblast proliferation. Neutrophil, gut microenvironment and microRNA has been proposed as future focus.
Rheumatoid arthritis (RA) is a common autoimmune disease characterized by synovial inflammation and bone destruction. Early diagnosis and appropriate treatment are greatly meaningful for patients with risk factors for poor outcomes, such as high disease activity, the presence of autoantibodies, and early joint damage (Smolen et al., 2016). As one of the clinical indices, bone erosions are often attributed to irregular and ineffective treatment (Schett and Gravallese, 2012). The accompanying joint deformity of RA impairs their capacity for independent living, thus increasing the economic and psychological burden on their families (Ru et al., 2019).
The insidious onset of RA is connected with genetic, epigenetic and environmental factors. Activated immune system cells (macrophages, dendritic cells, neutrophils, B-cells and T-cells), inflammatory mediators (autoantibodies, cytokines, chemokines and proteases) and nonimmune factors (microbiota) are involved in the progression of synovial inflammation and bone destruction (Fang et al., 2020). The inflammatory state of the host stimulates osteoclasts to surpass osteoblasts, causing bone resorption instead of physiological bone remodeling maintained by balanced bone metabolism (Auréal et al., 2020).
The current treatment includes corticosteroids, traditional disease-modifying anti-rheumatic drugs (cDMARDs), nonsteroidal anti-inflammatory drugs (NSAIDs), biologics and alternative medicine, which indicates traditional Chinese medicine (TCM) in China. TCM has been employed to treat rheumatism diseases for thousands of years. The complex prescriptions that have shown significant efficacy in RA are composed of many effective ingredients, the explicit working mechanisms of which are still not understood. To date, many efforts have been made to reveal the potential targets regulated by the active components in RA. In this review, we outline the potential mechanisms whereby the active components from TCM botanical drugs inhibit bone resorption in RA and the latest findings of some representative compounds.
Bone resorption occurring in the joints of RA patients arises from overactivated immune cells, inflammatory effectors, imbalances in bone metabolism, microbiota dysbiosis and other nonimmune factors.
RA is often characterized by the destruction of juxta-articular bone and erosions due to bone resorption mediated by osteoclasts with elevated activities (Maruotti et al., 2011). Bone resorption attributes to the imbalance of bone metabolism mainly mediated by osteoclasts and osteoblasts. Osteoclasts are the principal cells responsible for bone resorption in RA. The number of osteoclasts increases when they are derived from bone marrow myeloid progenitors, peripheral monocytes and immature dendritic cells (Speziani et al., 2007). With the increased number of osteoclast precursors followed by enhanced activity and prolonged lifespan of osteoclasts, bone metabolism becomes imbalanced, leading to bone resorption and joint dysfunction. Macrophage colony-stimulating factor (M-CSF) and the ligand for the receptor activator of NF-κB (RANKL) are essential in osteoclast formation. M-CSF binds to receptors on osteoclast precursors. RANKL activates the receptor activator of NF-κB (RANK) on the surface of osteoclast precursors and subsequently activates downstream signaling pathways such as mitogen-activated protein kinases (MAPKs) and NF-κB. Finally, nuclear factor of activated T-cell cytoplasmic 1 (NFATc1), the master transcription factor of osteoclast differentiation, is upregulated and induces the expression of osteoclast function-associated genes, especially tartrate acid phosphatase (TRAP) (Takegahara et al., 2022). Costimulatory signals are also involved, such as immunoreceptor tyrosine-based activation motif (ITAM)-associated receptors and Toll-like receptors (TLRs). ITAM contributes to the activation of NFATc1, but the explicit mechanism by which TLRs assist in osteogenesis remains unknown (Auréal et al., 2020).
On the other hand, osteoblasts are devoted to building the host skeleton. They play an essential role in bone formation by synthesizing bone-associated proteins to form extracellular matrix and then mineralize (Wehmeyer et al., 2016). Osteoblasts are derived from mesenchymal stem cells (MSCs) through bone morphogenic protein (BMP) pathways and wingless-related integration site (Wnt) pathways (Ponzetti and Rucci, 2021). Transforming growth factor-β (TGF-β)-involved BMP pathways can be divided into Smad-dependent and non-Smad-dependent pathways, which both upregulate the transcription of runt-related transcription Factor 2 (RUNX2) (Chen et al., 2012). Wnt/β-catenin signaling regulates osteogenesis by repressing alternative differentiation pathways of MSCs, promoting osteoblast differentiation, proliferation, and mineralization activity while blocking osteoblast apoptosis (Ponzetti and Rucci, 2021). In addition, β-catenin represses osteoclastogenesis by inducing the expression of osteoprotegerin (OPG), which inhibits RANK-RANKL signal transduction. Dickkopf (DKK) families and Sclerostin are inhibitors of lipoprotein receptor–related protein 5 and 6 (LRP5/6) coreceptors that activate the Wnt pathway in osteoblast differentiation (Krishnan et al., 2006). Dickkopf-related protein 1 (DKK1) is inhibited by TGF-β in the mineralization stage of osteoblast differentiation in osteoprogenitors, which induces alkaline phosphatase (ALP) and collagen synthesis in the extracellular matrix maturation stage (Nam et al., 2020). After bone deposition, osteoblasts go on to apoptosis, or become bone lining cells or osteocytes (Ponzetti and Rucci, 2021). Given the mechanism of bone metabolism, TCM-mediated regulation of the differentiation and activity of osteoblasts and osteoclasts is an important therapeutic target in RA.
Fibroblast-like synoviocytes (FLS) also execute bone and cartilage degradation in RA progression (Harre and Schett, 2017). Under RA conditions, FLS undergo epigenetic changes toward an inflammatory phenotype (Neumann et al., 2010) that highly expresses tumor necrosis factor (TNF), interleukin-6 (IL-6), IL-1β and proteases, which fuel further inflammation and destruction of bone (Nygaard and Firestein, 2020). The production of OPG by FLS can be downregulated by TGF-β through Smad signaling (Hase et al., 2008). Active FLS primarily affect the activity of osteoblasts and osteoclasts. They induce osteoclast differentiation by producing RANKL in inflamed joints (Harre and Schett, 2017) in response to IL-17, IL-6, IL-1β (Hashizume et al., 2008) and autoantibodies (Kurowska et al., 2021). TNF-induced RANKL expression by FLS can be inhibited by sclerostin, which is mainly produced by osteocytes and is thought to suppress osteoblast formation (Wehmeyer et al., 2016; Weivoda et al., 2017). In addition, FLS regulate TNF-stimulated osteoblasts by expressing DKK-1 (Yeremenko et al., 2015). Additionally, migrating proliferated FLS invade and destroy the normal structure of bone. Therefore, it is beneficial for bone protection in RA treatment to consider FLS as promising therapeutic targets (Nygaard and Firestein, 2020).
Neutrophils, as an important part of innate immunity, play an important role in the initiation and development of RA. Neutrophils account for over 90% of cells in synovial fluid from RA patients (Weissmann and Korchak, 1984). They participate in the inflammation and bone erosion of RA by releasing TNF, IL-1β, IL-6 and other cytokines, as well as matrix metalloproteinase-9 (MMP-9), myeloperoxidase (MPO) (Strzepa et al., 2017) and neutrophil elastase (NE) (Krotova et al., 2020), which degrade the extracellular matrix to impair joints (Chen et al., 2018). In addition, the observation that neutrophils from RA cases exhibited increased spontaneous NET formation in vitro with enhanced NE and MPO expression and PAD-4-mediated citrullination of H3 (Sur Chowdhury et al., 2014) confirmed that NET formation is one of the mechanisms whereby neutrophils transmit immune responses in RA. Peptidyl-arginine-deiminases-4 (PAD-4) has been proven to be indispensable for NET formation and its antibacterial capability (Li et al., 2010). Autophagy is also closely associated with NET formation in that autophagy inducers significantly promote NET formation (Guo et al., 2021), while silencing Atg5 blocks NET formation (Xu et al., 2017). During the formation of NETs (Brinkmann et al., 2004), the extracellular components of NETs expose citrullinated and carbamylated autoantigens (O'Neil et al., 2020) to dendritic cells and macrophages for antigen presentation, thus activating B-cells for the subsequent synthesis of antibodies and the formation of ICs (O'Neil and Kaplan, 2019). Given that the production of antibodies is closely related to neutrophils, it is essential to inhibit the inflammatory responses and NET formation of neutrophils in the treatment of RA to reduce bone erosion (Wu et al., 2020).
B-cells are responsible for producing osteoclasogenic cytokines such as IL-6 (Mihara et al., 2011) and RANKL (Yeo et al., 2011) in RA progression, although they differentiate into regulatory B-cells that produce IL-10 and TGF-β to suppress osteoclasts (McInnes et al., 2016). Fc-receptor-like-4 (FcRL4) positive B-cells (Yeo et al., 2015), memory B-cells and the regulatory B10 cells were recognized as RANKL-producing subsets (Hu et al., 2017). The pathogenic RANKL-producing B10 cells were positively correlated with disease activity (Hu et al., 2017). More importantly, B-cells form plasma cells that produce autoantibodies in response to the emergence of autoantigens. The presence of autoantibodies such as rheumatoid factor (RF) are often recognized as hallmarks and clinical indices of RA (van Delft and Huizinga, 2020). The anti-citrullinated protein antibody (APCA) is another common and relatively characteristic autoantibody in RA. Citrullinated peptides that can serve as autoantigens are detectable in RA patients (Van Steendam et al., 2010; Sakkas et al., 2014). The neoepitopes may arise from NET formation (Karmakar and Vermeren, 2021). Autoantibodies in the RA can induce osteoclasts by inducing RANKL secretion in FLS (Kurowska et al., 2021). The formation of immune complexes (ICs) also activates the completement system, triggering downstream inflammation to induce local and systemic bone loss (Komatsu and Takayanagi, 2018).
The presentation of autoantigens by dendritic cells leads to the generation of various T helper (Th) cells, including Th1, Th2, Th17 and T follicular helper (Tfh) cells. In particular, the proportion of regulatory T (Treg)/Th17 cells plays a fundamental role in autoimmune diseases. An imbalance between Treg cells and Th17 cells often emerges in RA (Komatsu and Takayanagi, 2018). The transcription factor forkhead Box P3 (Foxp3) is critical for Treg differentiation and suppressive capability (Kondo et al., 2018) and is critically downregulated under RA conditions. Th17 differentiation can be induced by IL-1β and IL-6 through the expression of RAR-related orphan receptor gamma (RORγt) (Chung et al., 2009) and signal transducer and activator of transcription 3 (STAT3) (Nishihara et al., 2007), respectively. The ratio of Treg/Th17 often declines in RA, leading to the accumulation of inflammation with increased concentrations of IL-17, IL-23, IL-6, and TNF-α (Niu et al., 2012). Th1, Th2 and Treg subsets (Kelchtermans et al., 2009) inhibit osteoclastogenesis directly or indirectly through cytokines, including IFN-γ (Tang et al., 2018), IL-10 (Luo et al., 2011), IL-4 (Kim Y. G. et al., 2007), TGF-β(Luo et al., 2011) and cytotoxic T-lymphocyte antigen 4 (CTLA-4) (Axmann et al., 2008). Th17 cells are the primary T-cell subset leading to osteoclastogenesis by synthesizing IL-17 (Sato et al., 2006). Tregs secrete cytokines such as IL-10 and TGF-β to suppress the differentiation of osteoclasts and their resorptive activities (Luo et al., 2011). In view of the threat posed by the imbalance of Treg/Th17 to bone protection of RA, it is imperative to consider regulating the frequencies and activities of Th17 and Treg cells in RA treatment to limit bone destruction.
The presence of different cytokines in the synovial fluid of RA patients has been studied. TNF-α, IL-1β, IL-6 and IL-17 contribute to osteoclastogenesis. TNF-α is able to reduce osteoblast differentiation by inhibiting the expression of insulin-like growth Factor 1 (IGF-1) (Gilbert et al., 2000) and RUNX2 (Gilbert et al., 2002) in different stages. IL-1β induces MMPs and the differentiation of osteoclasts (Abramson and Amin, 2002) and Th17 cells (Chung et al., 2009). The proinflammatory effect of IL-6 is similar to that of TNF (Tanaka et al., 2014). For osteoclastogenesis, IL-6 directly induces RANKL expression in RA-FLSs and IL-6 is essential for RANKL induction by TNF and IL-17 (Hashizume et al., 2008). In combination with TGF-β, IL-6 is indispensable for Th17 differentiation (Korn et al., 2009). IL-17 activates FLS to produce RANKL (Komatsu and Takayanagi, 2018) and stromal cell-derived Factor 1 (SDF-1) (Kim K. W. et al., 2007). SDF-1 promotes the recruitment of osteoclast precursors, differentiation and bone resorptive activity of osteoclasts (Kim et al., 2014), as well as the expression of MMPs and IL-6 in RA (Villalvilla et al., 2014; Bragg et al., 2019).
Some essential cytokines counteract the osteoclastogenic effect of RANKL. IFN-γ strongly inhibits the fusion of osteoclasts originating from DCs, as well as TRAP and bone resorption activities (Speziani et al., 2007). IL-4 significantly increases OPG secretion by fibroblasts (Tunyogi-Csapo et al., 2008). CTLA-4 induces the apoptosis of osteoclast precursors (Takayanagi, 2015). TGF-β activates osteoblasts through the BMP pathway (Chen et al., 2012) while inhibiting OPG production from FLS in RA (Hase et al., 2008). The interplay between osteoclasts and osteoblasts can be regulated by targeting the network of cytokines and chemokines.
Organisms distributed in the oral and intestinal cavities contribute to the homeostasis of the host. With the brain-gut axis recognized as the primary effector in many diseases, research on the brain-gut-bone axis is starting to gain attention (Seely et al., 2021). As is seen in osteoporosis (Seely et al., 2021), gut organisms may affect osteoclastogenesis and bone remodeling progression through the regulation of RANKL. The functions that microbiota may perform in the progression of bone destruction in RA have several aspects. First, microbiota is closely related to bone metabolism, but the explicit mechanism is unknown. The implantation of gut bacteria from mice with conventional specific pathogen-free to germ-free mice increases bone mass with a significant increase in IGF-1 in serum (Yan et al., 2016). Supplementation with Lactobacillus rhamnosus GG increases trabecular bone volume in mice (Tyagi et al., 2018). In addition, microbiota dysbiosis affects the overall inflammation of the host. They are part of the physical and chemical barriers of the intestine (Mu et al., 2017). Changes in the microbiota constitution influences the expression level of TLRs of antigen-presenting cells and Th subsets (Chen et al., 2017). Lactobacillus casei was reported to alleviate bone loss in rats with adjuvant-induced arthritis (AA) by restoring the composition and function of microbiota and inhibiting proinflammatory cytokines (Pan et al., 2019). Furthermore, microbial metabolites, such as short-chain fatty acids (SCFAs), have been shown to play significant roles in the immune system. The protective effects of SCFAs on bone mass are associated with the inhibition of osteoclast differentiation and bone resorption in vitro and in vivo through the downregulation of NFATc1-involved pathways (Lucas et al., 2018). Metabolites also regulate the differentiation and activity of B-cells and T-cells (Huang et al., 2021). Specifically, butyrate suppresses the differentiation of osteoclasts in vitro and promotes Treg subsets in vivo (He et al., 2022). It regulates Treg cells to stimulate the differentiation of osteoblasts via the upregulation of Wnt10b (Tyagi et al., 2018). Butyrate administration alleviates joint swelling and increases the frequency of Treg cells and the concentration of IL-10. Butyrate also decreases the frequency of Th17 cells in vivo (Hui et al., 2019). Considering the function of the microbiome, modulating aberrant species and metabolites is one of the promising therapeutic targets of natural compounds in RA bone protection.
Apart from the immune cells discussed above, macrophages (Cutolo et al., 2022) and dendritic cells are responsible for antigen presentation, as is the synthesis of related cytokines in the inflammatory response. Some novel mechanisms whereby osteoclast differentiation is regulated have been proposed, such as noncoding RNA (ncRNA)-involved pathways (Ponzetti and Rucci, 2021). However, research on the effect of active components from TCM on bone protection in RA is somewhat limited to the classic mechanism.
Collectively, the interplay between bone metabolism and immunology in RA is delineated in Figure 1.
FIGURE 1. Mechanism of bone metabolism in RA. All targeted cells and cytokines are labeled. The bone resorptive activity is balanced by osteoblasts and osteoclasts. The invasion of fibroblasts also leads to bone destruction. The arrow end represents promotion effect while the blunt end represents the inhibitory effect.
The current treatment strategies for RA have some shortcomings despite satisfactory efficacy in the alleviation of symptoms and progression (Emery, 2006). Long-term administration of immunosuppressants and the excessive use of corticosteroids often result in serious adverse reactions (Bresalier et al., 2005; Sardar and Andersson, 2016; Smolen et al., 2020). The high expenses, unexpected side effects and low response of some patients to biologics make it difficult to promote biologics extensively (Keyser, 2011). In China, thousands of years have witnessed the efficacy of TCM in RA treatment. A systematic review overlooking the bone-protecting efficacy of TCM alone or in combination with Western medicine in the treatment of RA has proposed that Chinese decoctions have advantages over Western medicine in terms of bone protection (Shi et al., 2020). Since it is difficult to explore the enigmatic mechanism of the complex prescriptions, the functions of each single component of TCM botanical drugs are worth investigating. Notably, evaluating the molecular targets of some natural components showing great efficacy in RA can assist in improving the understanding of key underlying molecular mechanisms (Shen et al., 2020). Here, we provide an overview of recent research advances in TCM in the frontiers of RA osteoclastogenesis. The representative bioactive compounds that have been proven to be bone protective in animal models of RA will be primarily discussed. The main mechanism of the representative components and their chemical structure is depicted in Figure 2. The cells that are targeted by these compounds both in vivo and in vitro along with the adopted animal models are listed in Table 1.
FIGURE 2. The bone-protective mechanism and chemical structure of active components (A) Andrographolide. (B) Artesunate (C) Berberine. (D) Celastrol (E) Emodin. (F) Quercetin (G) Sinomenine. (H) Tetrandrine (I) Triptolide. (J) Total glucosides of paeony (TGP). The chemical structure of representative ingredients from TGP are shown.
Andrographolide (Figure 2A) is the major diterpenoid bioactive compound derived from Andrographis paniculata (Burm.f.) Nees [Acanthaceae]. The X-ray examination showed that the oral administration of andrographolide (25, 50 and 100 mg/kg) has a bone-protective effect in AA rats (Luo et al., 2020). It reduced the expression of TNF-α and IL-6 in the serum (Luo et al., 2020). In vivo, the upregulation of antioxidant enzymes such as superoxide dismutase and the downregulation of CXCL2, elastase and myeloperoxidases certainly contribute to the alleviation of oxidative stress measured by malondialdehyde, catalase, glutathione, superoxide dismutase and the ratio of nitrite/nitrate (Luo et al., 2020). The joint symptoms of the collagen-induced arthritis (CIA) mice treated with daily andrographolide (100 mg/kg) orally were alleviated. Also, andrographolide reduced serum anti-collagen II antibody, TNF-α, IL-1β and IL-6. Andrographolide (10 or 20 μM) showed a suppressive effect on TNF-α activated FLS by blocking the phosphorylation of P38 and ERK in vitro (Li et al., 2017). In AA rats, andrographolide (50 mg/kg) was given intraperitoneally (Gupta et al., 2018). Andrographolide (0.5 μg/ml) decreased LPS induced overexpression of COX-2, iNOS and NF-κB p65 in macrophages in vitro, although the reductions were insignificant (Gupta et al., 2018). Andrographolide (10–30 μM) further inhibited proliferation of FLS isolated from RA patients by arresting the cell cycle at the G0/G1 stage and triggering apoptosis in vitro (Yan et al., 2012). Reducing NET formation was also effective in ameliorating RA by andrographolide (Li X. et al., 2019). The oral administration of andrographolide (25–50 mg/kg) greatly ameliorated joint swelling of AA mice (Li X. et al., 2019). The local infiltration of neutrophil and formation of NETs was also inhibited in vivo (Li X. et al., 2019). In vitro, the treatment of andrographolide (25 μM) balanced autophagy and NET formation (Li X. et al., 2019). Additionally, although the changes in microbiota induced by andrographolide in animal models with RA remain undetermined, beneficial variation in the microbiota composition in blank mice treated with andrographolide (2–20 mg/kg) points to the hypothesis that regulation of gut microorganisms is one of the mechanisms whereby andrographolide ameliorates bone destruction (Wu et al., 2021).
Artesunate (Figure 2B), a derivative of artemisinin from artemisia annua L [Asteraceae], has been an effective antioxidant. As molybdenum target X-rays showed, oral administration of artesunate (5, 10 or 20 mg/kg) attenuated bone destruction in CIA rats (Liu et al., 2017). The expression of Foxp3 and IL-17 in the synovium and T-cells was also regulated by artesunate in vivo, which reflected the rebalance of Th 17 and Treg cells (Liu et al., 2017). In vitro assays indicated that artesunate (5, 10 or 20 ng/ml) also modulated Foxp3 expression of synovial cells isolated from CIA rats (Zhu et al., 2016). As for FLS, artesunate (10 μM) regulated the activation of FLS from RA patients by inhibiting the phosphorylation of protein kinase B (PKB) and decreased the secretion of IL-1β and IL-6 in vitro (Xu et al., 2007). Artesunate treatment (60 μM) also suppressed the horizontal and vertical migration of FLS from RA patients in vitro through 3-phosphoinositide-dependent protein kinase 1 (PDK-1) pathway, as well as the inhibition of MMP-2 and MMP-9 production (Ma et al., 2019). The above findings have revealed that artesunate may affect bone resorption in RA in an immunoregulatory way. Further research focusing on the effect of artesunate on the activity of osteoclast and osteoblast in vivo is worth performing.
Berberine (Figure 2C) is a bioactive isoquinoline alkaloid compound from Coptis deltoidea C.Y.Cheng and P.K.Hsiao [Ranunculaceae] that has been applied in the treatment of bone diseases for thousands of years known as Huanglian in China. Studies have defined its function as promoting bone regeneration and anti-inflammation (Zhang et al., 2021). The X-ray scanning confirmed that oral administration of berberine (200 mg/kg) protected bone from erosions in CIA rats with decreased transcription levels of TNF-α, IL-1β, IL-6 and RANKL in the synovium, as well as reduced Th17 differentiation by attenuating the phosphorylation of STAT3 in the spleen (Yue et al., 2017). Berberine also induced the production of cortistatin whose receptor antagonists reversed the anti-arthritic efficacy of berberine in vivo (Yue et al., 2017). In CIA rats, the treatment with berberine orally (200 mg/kg) reduced the expression levels of TNF-α, IL-1β, IL-6 and IL-17 in sera partly through the MAPK signaling pathway (Wang et al., 2014). In the in vitro assays, the FLS and T-cells were purified from AA rats. Berberine (15–45 μM) inhibited the IL-21-induced autophagy of FLS through phosphoinositide three kinase (PI3K)/AKT pathway in a dose dependent manner (Dinesh and Rasool, 2019). It also recovered the balance of Treg/Th17 by increasing the differentiation of Treg cells and reducing the IL-21-induced differentiation of Th17 cells via downregulation of RORγt (Dinesh and Rasool, 2019). Another in vivo study confirmed the upregulation of Treg cells and downregulation of Tfh cells, as well as the reduction of anti-bovine type II collagen in CIA mice after berberine treatment intraperitoneally (1 mg/kg) (Vita et al., 2021). Interestingly, oral administration of berberine (200 mg/kg) has been shown to regulate butyrate metabolism by elevating the abundance of butyrate-producing bacteria in CIA rats, thereby limiting the generation of nitrate and stabilizing physiological hypoxia in the intestine (Yue et al., 2019). As mentioned above, butyrate is able to suppress osteoclasts, promote Treg cells (He et al., 2022) and promote Th17 differentiation (Hui et al., 2019). The systematic regulation of berberine is somewhat linked to microbial metabolism.
Celastrol (Figure 2D), a main constituent from Tripterygium wilfordii Hook. f [Celastraceae], has shown anti-inflammatory and bone-protective activities both in vivo and in vitro. Celastrol exerts a bone-protective effect primarily by inhibiting osteoclast differentiation and activities. In CIA mice, celastrol treatment (3 mg/kg) inhibited the expression of osteoclast-specific genes and transcription factors in the synovium, such as TRAP and NFATc1, supporting the observation of microcomputed tomography (micro-CT) that celastrol protected ankle joints from severe bone erosion in vivo (Gan et al., 2015). The mechanism was further explored with RAW 264.7 cells, where celastrol treatment (0.03, 0.1 or 0.3 μM) inhibited RANKL-induced expression of TRAP, c-Fos, c-Jun and NFATc1 through the NF-κB and MAPK pathways in vitro (Gan et al., 2015). In heat-killed Mycobacterium tuberculosis H37Ra (Mtb)-induced arthritic (AA) rats, celastrol treatment (1 mg/kg) reduced bone resorption significantly. TRAP-positive staining, bone histomorphometry and radiographs of limbs indicated a decrease in osteoclasts and preservation of joint integrity by celastrol (Nanjundaiah et al., 2012). In the synovium-infiltrating cells (SICs) isolated from AA rats treated with celastrol, in vitro Mtb sonication stimulation failed to raise the ratio of RANKL/OPG and maintained the level of OPN, IGF-1 and MMP-9 at a lower level than those isolated from untreated AA rats (Nanjundaiah et al., 2012). In IL-17-activated MC3T3-E1 cells, there was also a decline in the ratio of RANKL/OPG, M-CSF and IL-6 regulated by celastrol (0.1 or 0.3 μM) in vitro (Nanjundaiah et al., 2012). Celastrol treatment (0.1 or 0.3 μM) also decreased the secretion of MMP-9 in RAW 264.7 in response to RANKL in vitro (Nanjundaiah et al., 2012). In addition, celastrol treatment (1, 2 or 5 μM) downregulated the proliferation of FLS by inducing DNA damage, cell cycle arrest, and apoptosis in vitro (Xu et al., 2013). One high-throughput analysis on the gene differences of FLS from RA patients with or without celastrol treatment (1 μM) revealed that the expression of several chemokine genes from FLS was downregulated by celastrol (Fang et al., 2017). In vitro experiments further indicated that NF-κB p65 pathway was also involved in the celastrol (1 μM)-suppressed IL-6 and MMP-9 production in FLS (Fang et al., 2017). In terms of the Th17/Treg balance, intraperitoneal injection of celastrol (1 mg/kg) reduced the ratio of Th17 to Treg cells in the synovial tissue (Astry et al., 2015). In the in vitro culture of mice T-cells, celastrol treatment (0.1–0.3 μM) inhibited Th17 differentiation through STAT3 (Astry et al., 2015). Additionally, celastrol treatment (5 or 10 μM) inhibited the neutrophil oxidative burst and NET formation induced by TNFα, ovalbumin:anti-ovalbumin immune complexes (Ova ICs) and immunoglobulin G (IgG) purified from the sera of RA patients (Yu et al., 2015). Intraperitoneal injection of celastrol (1 mg/kg) has shown anti-inflammatory effect on CIA rats in vivo, which was measured by the decreased TNF-α, IL-1β, IL-6 and oxidative stress. The effect was abolished by the injection of recombinant adenoviral vectors harboring NADPH oxidase in CIA rats (Gao et al., 2020). The decline of IL-1β and IL-18 in AA rats may be related to the inhibitory effect of celastrol (0.5 or 1 mg/kg) on ROS-NF-κB-NLRP3 (Jing et al., 2021). In LPS-induced human mononuclear macrophages (THP-1 cells), celastrol (12.5–50 nM) suppressed ROS-NF-κB-NLRP3 activation in vitro (Jing et al., 2021). Collectively, celastrol inhibits bone resorption in RA treatment in a multitarget way.
Emodin (Figure 2E) is an active ingredient in some Chinese botanical drugs, such as Rheum palmatum L [Polygonaceae]. It has shown antioxidant, anti-inflammatory and immunosuppressive effects in various diseases (Zheng et al., 2021). As illustrated by X-ray and radiological scores, the intraperitoneal injection of emodin (10 mg/kg) into CIA mice alleviated joint swelling with reduced bone destruction. It inhibited the activation of NF-κB pathways and differentiation of osteoclasts in vivo, as well as the expression levels of TNF-α, IL-1β, IL-17, RANKL and MMP-1/3 (Hwang et al., 2013). The osteoclast differentiation assay suggested that emodin treatment (10 or 20 μM) inhibited TRAP activity induced by RANKL and M-CSF in vitro (Hwang et al., 2013). Another in vitro report revealed that in MC3T3-E1 cells, emodin treatment (5 or 10 μM) increased the transcription level of BMP-2 and expression level of ALP through PI3K/AKT/MAPK pathways (Lee et al., 2008). In our former reports, AA mice intraperitoneally injected with emodin (30 μg/kg) showed decreased level of TNF-α, IL-6 and IFN-γ in the serum, which suggested the anti-inflammatory effect of emodin as a whole in vivo. In addition, emodin treatment (20 μM) significantly reduced phorbol 12-myristate 13-acetate (PMA)-triggered NET formation in vitro (Zhu et al., 2019). In summary, emodin has a regulatory effect on the differentiation of osteoblasts, osteoclasts and T-cells, in addition to its anti-inflammatory and anti-NET functions in RA treatment.
Quercetin (Figure 2F) is a representative flavonoid that is found in Morus alba L [Moraceae]. It is also present in fruits and vegetables. Apart from its anti-inflammatory and other diverse pharmacological activities (Guan et al., 2021), its bone-protective effect has been studied extensively in bone-related diseases such as osteoporosis (Wong et al., 2020). Quercetin performs dual-target regulation of bone metabolism. Quercetin treatment (50 μM) inhibited BMP-2- and TGF-β-induced Smad activation in MC3T3-E1 cells, which led to suppression of mineralization (Yamaguchi and Weitzmann, 2011). For osteoclastogenesis, quercetin suppressed osteoclast differentiation from RAW264.7 elicited by TNF and RANKL in a dose-dependent manner (0.1–25 μM) (Yamaguchi and Weitzmann, 2011). Similar conclusion could be drawn from the in vitro results that quercetin (25 μM) inhibited IL-17-stimulated RANKL production in RA-FLS and quercetin (1–25 μM) inhibited RANKL-stimulated TRAP expression in PBMCs (Kim et al., 2019). In the coculture of PBMCs and RA-FLS, quercetin (25 μM) also suppressed osteoclast formation induced by IL-17 (Kim et al., 2019). Interestingly, quercetin (25 μM) showed no effect on Treg differentiation, while it significantly reduced Th17 differentiation and IL-17 production in vitro (Kim et al., 2019). In CIA rats, the percentage of Th17 cells increased, and the percentage of Treg cells decreased after oral treatment with quercetin (150 mg/kg) (Yang et al., 2018). The further assays revealed that quercetin inhibited NLRP3 inflammation in the synovial tissues and production of anti-CII IgG2a in the serum (Yang et al., 2018). In AA mice, quercetin administration alleviated joint swelling and reduced NET formation in vivo. In vitro assays indicated that quercetin (25 μM) probably inhibited NET formation via autophagy suppression. It was also proposed that quercetin (25 μM) reversed the delay of neutrophil apoptosis induced by LPS in vitro (Yuan et al., 2020).
Sinomenine (Figure 2G), a major component of sinomenium acutum (Thunb.) Rehder and E.H.Wilson [Menispermaceae], has various pharmacological activities, especially a regulatory effect on bone protection in the treatment of RA. In sinomenine-treated AA rats, radiographs indicated that the oral administration of sinomenine (100 mg/kg) had significant protective effect on the joints (Mu et al., 2013). The declined expression levels of MyD88, TLR2, TLR4, TNF-α, IL-1β, and IL-6 in synovial tissues indicated that sinomenine greatly alleviated local inflammation in vivo (Mu et al., 2013). In addition, sinomenine treatment (0.25–1 mM) was proven to induce apoptosis of RAW 264.7-derived osteoclasts in vitro by activating caspase-3 and disrupting the actin ring structure (He et al., 2014). In terms of FLS, sinomenine (0.25–0.4 mM) further reduced TNF-α induced proliferation of FLS from the synovium of AA rats by inhibiting the expression of alpha seven nicotinic acetylcholine receptors in vitro (Yi et al., 2018). The frequency change of Th17 cells and Treg cells in the gut lymphoid tissues of CIA rats was attributed to the oral administration of sinomenine (120 mg/kg) (Tong et al., 2015). Noticeably, the frequency change of Th17 cells and Treg cells in the spleen was insignificant compared with the that of CIA rats (Tong et al., 2015). Sinomenine intervention also enhanced the migration of Treg cells from gut to joint marked by higher expression of Foxp3 in joint tissues (Tong et al., 2015). Decreased IL-17 and increased IL-10 in the serum was observed accordingly (Tong et al., 2015). In vitro, sinomenine treatment (1 mM) suppressed the proliferation and secretion of TNF-α and IL-17 in PBMCs activated by type II collagen (CII) (Tong et al., 2015). Interestingly, sinomenine (0.3–30 μM) has been defined as having a limited effect on Th cells and Treg cells from mitogen-activated PBMCs isolated from RA patients in vitro (Xu et al., 2021). As previously reported, intraperitoneal injection of sinomenine (20 mg/kg) failed to achieve expected efficacy in CIA rats (Tong et al., 2015). We proposed that the regulatory function of sinomenine may be further related to gut digestion and even the gut microbiota.
Tetrandrine (Figure 2H), known as a bioactive alkaloid derived from the dry root of stephania tetrandra S. Moore [Menispermaceae], has been studied in RA research. Yue Dai’s group revealed that tetrandrine attenuated osteoclastogenesis in CIA rats by delineating increased bone mineral density (BMD) and trabecular bone (Tb) of bone parameters in the micro-CT and decreased TRAP expression in vivo after oral administration of tetrandrine (30 mg/kg). In vitro, tetrandrine treatment (0.1–0.3 μM) greatly inhibited TRAP activity and other osteoclast related genes in both bone marrow-derived macrophages (BMDMs) and RAW264.7 cells. For RAW264.7, this may be related to blocking of the nuclear translocation of NF-κB-p65 and NFATc1 by reducing the activation of spleen tyrosine kinase (Syk) (Jia et al., 2018). Further in vitro experiments in RAW 264.7 cells and BMDMs suggested that tetrandrine (0.3 μM) enhanced the ubiquitination and degradation of Syk and downregulated the expression of NFATc1 in an AhR-dependent manner (Jia et al., 2019). The differentiation of T-cell subsets is also regulated by tetrandrine. It was proposed that the oral treatment of tetrandrine (20 or 40 mg/kg) can relieve cartilage destruction and joint swelling by restoring the balance of Th17 and Treg cells in mesenteric lymph nodes with a decrease in TNF-α, IL-1β, IL-6, IL-17A, total IgG and isotype-specific IgG2a in serum from CIA mice. After tetrandrine treatment, IL-10 also rose in CIA mice (Yuan et al., 2016). Then it was observed that tetrandrine (0.3–1 μM) modulated T-cell differentiation through AhR in a series of cell lines (Yuan et al., 2016). The former observation was further explored through elucidating the regulation of STAT-3 and STAT-5 by tetrandrine (1 μM) in inhibiting Th17 differentiation in vitro (Yuan et al., 2017). Tetrandrine affects the proliferation and migration of FLS to protect bone in RA. In both primary FLS isolated from the synovium of RA patients and the cell line MH7A, tetrandrine (0.3–1 μM) greatly impeded the migration and invasion of RA-FLS in vitro, as well as the expression of MMP-2/9 (Lv et al., 2015). In addition, NET formation was proven to be inhibited in AA mice after tetrandrine treatment with intraperitoneal injection (6 mg/kg) (Lu et al., 2022). In vitro, delineation of the reduced expression of PAD-4 and citrullinated histone H3 after tetrandrine treatment (10 μM) supported former observation in vivo (Lu et al., 2022).
Triptolide (Figure 2I) is another extract from the herb tripterygium wilfordii Hook. f [Celastraceae]. It alleviates bone destruction in RA in various ways (Fan et al., 2018). As discussed before, bone resorption mediated by osteoclasts mainly gives rise to bone destruction in RA. In CIA mice, micro-CT images suggested a protective function of triptolide in terms of preserved bone volume and quality after oral administration of triptolide (8–32 μg/kg) (Liu et al., 2013). In vivo triptolide treatment decreased TRAP-positive cells by downregulating RANKL and RANK along with the upregulation of OPG (Liu et al., 2013). A similar tendency was observed in the coculture of PBMCs and FLS, which supported that RANKL/RANK/OPG signaling was primarily modulated by triptolide in the bone protection of RA (Liu et al., 2013). The in vitro coculture of Treg cells and BMDMs with triptolide treatment (10 nM) revealed that triptolide upregulated IL-10 and TGF-β, inhibited the differentiation of osteoclasts, and reduced bone resorptive activities (Xu et al., 2016). The oral administration of triptolide (60 μg/kg) greatly reduced the percentage of Th17 cells in the spleen and the expression of pyruvate kinase M2 (PKM2) compared with that of CIA mice (Shen et al., 2022). Triptolide treatment (0.02–0.08 μg/ml) significantly suppressed Th17 differentiation, IL-17A production and PKM2-mediated glycolysis in vitro (Shen et al., 2022). Triptolide further acts on FLS to minimize their invading effect on bone. The migration of FLS and expression of MMP-9 was suppressed in severe combined immunodeficiency (SCID) mice with coimplantation RA model after treatment with triptolide (100 μg/kg) intraperitoneally (Yang et al., 2016). Intraperitoneal injection of triptolide (100 μg/kg) alleviated bone destruction of CIA mice through inhibiting phosphorylation of JNK in vivo (Yang et al., 2016). Triptolide (50 nM) inhibited FLS migration and cytoskeleton reorganization in vitro (Yang et al., 2016). Regarding ncRNA, triptolide-mediated downregulation of long noncoding RNA (lncRNA) RP11-83J16.1 decreased the proliferation and invasion of FLS in CIA rats after oral administration of triptolide (45 μg/kg) (Piao et al., 2021). So was in vitro after triptolide treatment (32 nM) in FLS isolated from RA patients (Piao et al., 2021). In addition to the decreased expression of MPO and NE in AA mice joint tissues after intraperitoneal injection of triptolide (45 μg/kg), in vitro treatment of triptolide (200 nM) also inhibited the migration and NET formation of neutrophils (Huang et al., 2018).
Total glucosides of paeony (TGP) from paeonia lactiflora Pall [Paeoniaceae] include a series of bioactive ingredients (Figure 2J). They have been proved effective in RA both clinically and experimentally. As micro-CT examinations showed, the bone resorption and joint destruction of the CIA mice treated with TGP intragastrically (0.36 and 0.72 g/kg) was significantly reduced compared with CIA model mice (Li H. et al., 2019). TGP treatment also alleviated inflammation measured by the decreased concentration of anti-CII IgG2a, TNF-α, IL-21 and IL-6 in the serum and reduced phosphorylation of p65 and STAT3 in the paws (Li H. et al., 2019). TGP also exerted immunosuppressive effect on CIA mice through decreasing the number of germinal center B cells and Tfh cells in the spleen (Li H. et al., 2019). The oral treatment of TGP (60 mg/kg) even prevented juxta-articular bone loss in AA rabbits with decreased expression level of RANKL. The FLS proliferation was greatly reduced in CIA rats after oral administration of TGP (25, 50 and 100 mg/kg). In vitro assays confirmed that TGP treatment (12.5 or 62.5 μg/ml) inhibited the proliferation of IL-1β-treated FLS from CIA rats, as well as the expression of G proteins (Jia et al., 2014). The oral administration of TGP (158, 474 and 948 mg/kg) lasting for 12 weeks significantly repaired the dysbiosis and dysfunction of the gut microbiota in CIA rats and regulated immune responses in various ways (Peng et al., 2019). TGP treatment also modulated the T-cell subsets in PBMCs and immune responses of the intestinal mucosa from CIA rats. In detail, TGP reversed the imbalance in both Th1/Th2 and Th17/Treg, and regulated the secretion of secretory immunoglobulin A (SIgA) and IFN-γ in vivo (Peng et al., 2019).
As shown in Table 1, several active components from TCM that have shown great protective effects on bone in RA modulated multiple cells both in vivo and in vitro to ameliorate the osteoclastogenic impact. Osteoclasts, osteoblasts, Th cells and FLS play fundamental roles in regulatory mechanisms. In addition, an extensive screen for the effective ingredients from decoctions or botanical drugs that have been clinically used has been performed by Chinese scientists. Hopefully, more components from TCM botanical herbs can be explored and transformed into commercial applications.
This review has reviewed the existing mechanisms of bone resorption in RA that can be regulated by TCM drugs. Neutrophils, macrophages, B-cells and T-cells participate in inflammatory responses. Along with cytokines, chemokines and proteases, an increase in RANKL stimulates the differentiation of osteoclasts. The weakened differentiation of osteoblasts fails to balance bone resorptive activities. Notably, the microbiota is specifically found to be a promising target for TCM intervention in RA because the gut-joint axis may explain the multitargeted regulation of compounds from TCM. Although numerous active ingredients of TCM prescriptions are effective in the experiments, no single component had achieved ideal effect clinically. Combination of different active components under the direction of TCM theories is a promising strategy to develop new drugs.
Manuscript writing: QL and JX. Figures: HJ and JX. Draft preparation: QW. Revision: GH and RH.
This research was supported by the National Natural Science Foundation of China (grant number: 82205068 and 81904142), Research Program from Beijing University of Chinese Medicine (grant number: 2021-JYB-XJSJJ-023), and the stem cell and regenerative medicine research project from Beijing Science and Technology Commission (grant number:Z191100001519006).
The authors declare that the research was conducted in the absence of any commercial or financial relationships that could be construed as a potential conflict of interest.
All claims expressed in this article are solely those of the authors and do not necessarily represent those of their affiliated organizations, or those of the publisher, the editors and the reviewers. Any product that may be evaluated in this article, or claim that may be made by its manufacturer, is not guaranteed or endorsed by the publisher.
Abramson, S. B., and Amin, A. (2002). Blocking the effects of IL-1 in rheumatoid arthritis protects bone and cartilage. Rheumatol. Oxf. 41, 972–980. doi:10.1093/rheumatology/41.9.972
Astry, B., Venkatesha, S. H., Laurence, A., Christensen-Quick, A., Garzino-Demo, A., Frieman, M. B., et al. (2015). Celastrol, a Chinese herbal compound, controls autoimmune inflammation by altering the balance of pathogenic and regulatory T cells in the target organ. Clin. Immunol. 157, 228–238. doi:10.1016/j.clim.2015.01.011
Auréal, M., Machuca-Gayet, I., and Coury, F. (2020). Rheumatoid arthritis in the view of osteoimmunology. Biomolecules 11, 48. doi:10.3390/biom11010048
Axmann, R., Herman, S., Zaiss, M., Franz, S., Polzer, K., Zwerina, J., et al. (2008). CTLA-4 directly inhibits osteoclast formation. Ann. Rheum. Dis. 67, 1603–1609. doi:10.1136/ard.2007.080713
Bragg, R., Gilbert, W., Elmansi, A. M., Isales, C. M., Hamrick, M. W., Hill, W. D., et al. (2019). Stromal cell-derived factor-1 as a potential therapeutic target for osteoarthritis and rheumatoid arthritis. Ther. Adv. Chronic Dis. 10, 2040622319882531. doi:10.1177/2040622319882531
Bresalier, R. S., Sandler, R. S., Quan, H., Bolognese, J. A., Oxenius, B., Horgan, K., et al. (2005). Adenomatous Polyp Prevention on Vioxx Trial, ICardiovascular events associated with rofecoxib in a colorectal adenoma chemoprevention trial. N. Engl. J. Med. 352, 1092–1102. doi:10.1056/NEJMoa050493
Brinkmann, V., Reichard, U., Goosmann, C., Fauler, B., Uhlemann, Y., Weiss, D. S., et al. (2004). Neutrophil extracellular traps kill bacteria. Science 303, 1532–1535. doi:10.1126/science.1092385
Chen, B., Sun, L., and Zhang, X. (2017). Integration of microbiome and epigenome to decipher the pathogenesis of autoimmune diseases. J. Autoimmun. 83, 31–42. doi:10.1016/j.jaut.2017.03.009
Chen, G., Deng, C., and Li, Y. P. (2012). TGF-β and BMP signaling in osteoblast differentiation and bone formation. Int. J. Biol. Sci. 8, 272–288. doi:10.7150/ijbs.2929
Chen, W., Wang, Q., Ke, Y., and Lin, J. (2018). Neutrophil function in an inflammatory milieu of rheumatoid arthritis. J. Immunol. Res. 2018, 8549329. doi:10.1155/2018/8549329
Chung, Y., Chang, S. H., Martinez, G. J., Yang, X. O., Nurieva, R., Kang, H. S., et al. (2009). Critical regulation of early Th17 cell differentiation by interleukin-1 signaling. Immunity 30, 576–587. doi:10.1016/j.immuni.2009.02.007
Cutolo, M., Campitiello, R., Gotelli, E., and Soldano, S. (2022). The role of M1/M2 macrophage polarization in rheumatoid arthritis synovitis. Front. Immunol. 13, 867260. doi:10.3389/fimmu.2022.867260
Dinesh, P., and Rasool, M. (2019). Berberine mitigates IL-21/IL-21R mediated autophagic influx in fibroblast-like synoviocytes and regulates Th17/Treg imbalance in rheumatoid arthritis. Apoptosis 24, 644–661. doi:10.1007/s10495-019-01548-6
Fan, D., Guo, Q., Shen, J., Zheng, K., Lu, C., Zhang, G., et al. (2018). The effect of triptolide in rheumatoid arthritis: From basic research towards clinical translation. Int. J. Mol. Sci. 19, 376. doi:10.3390/ijms19020376
Fang, Q., Zhou, C., and Nandakumar, K. S. (2020). Molecular and cellular pathways contributing to joint damage in rheumatoid arthritis. Mediat. Inflamm. 2020, 3830212. doi:10.1155/2020/3830212
Fang, Z., He, D., Yu, B., Liu, F., Zuo, J., Li, Y., et al. (2017). High-throughput study of the effects of celastrol on activated fibroblast-like synoviocytes from patients with rheumatoid arthritis. Genes (Basel) 8, E221. doi:10.3390/genes8090221
Gan, K., Xu, L., Feng, X., Zhang, Q., Wang, F., Zhang, M., et al. (2015). Celastrol attenuates bone erosion in collagen-Induced arthritis mice and inhibits osteoclast differentiation and function in RANKL-induced RAW264.7. Int. Immunopharmacol. 24, 239–246. doi:10.1016/j.intimp.2014.12.012
Gao, Q., Qin, H., Zhu, L., Li, D., and Hao, X. (2020). Celastrol attenuates collagen-induced arthritis via inhibiting oxidative stress in rats. Int. Immunopharmacol. 84, 106527. doi:10.1016/j.intimp.2020.106527
Gilbert, L., He, X., Farmer, P., Boden, S., Kozlowski, M., Rubin, J., et al. (2000). Inhibition of osteoblast differentiation by tumor necrosis factor-alpha. Endocrinology 141, 3956–3964. doi:10.1210/endo.141.11.7739
Gilbert, L., He, X., Farmer, P., Rubin, J., Drissi, H., Van Wijnen, A. J., et al. (2002). Expression of the osteoblast differentiation factor RUNX2 (Cbfa1/AML3/Pebp2alpha A) is inhibited by tumor necrosis factor-alpha. J. Biol. Chem. 277, 2695–2701. doi:10.1074/jbc.M106339200
Guan, F., Wang, Q., Bao, Y., and Chao, Y. (2021). Anti-rheumatic effect of quercetin and recent developments in nano formulation. RSC Adv. 11, 7280–7293. doi:10.1039/d0ra08817j
Guo, Y., Gao, F., Wang, X., Pan, Z., Wang, Q., Xu, S., et al. (2021). Spontaneous formation of neutrophil extracellular traps is associated with autophagy. Sci. Rep. 11, 24005. doi:10.1038/s41598-021-03520-4
Gupta, S., Mishra, K. P., Singh, S. B., and Ganju, L. (2018). Inhibitory effects of andrographolide on activated macrophages and adjuvant-induced arthritis. Inflammopharmacology 26, 447–456. doi:10.1007/s10787-017-0375-7
Harre, U., and Schett, G. (2017). Cellular and molecular pathways of structural damage in rheumatoid arthritis. Semin. Immunopathol. 39, 355–363. doi:10.1007/s00281-017-0634-0
Hase, H., Kanno, Y., Kojima, H., Sakurai, D., and Kobata, T. (2008). Coculture of osteoclast precursors with rheumatoid synovial fibroblasts induces osteoclastogenesis via transforming growth factor beta-mediated down-regulation of osteoprotegerin. Arthritis Rheum. 58, 3356–3365. doi:10.1002/art.23971
Hashizume, M., Hayakawa, N., and Mihara, M. (2008). IL-6 trans-signalling directly induces RANKL on fibroblast-like synovial cells and is involved in RANKL induction by TNF-alpha and IL-17. Rheumatol. Oxf. 47, 1635–1640. doi:10.1093/rheumatology/ken363
He, J., Chu, Y., Li, J., Meng, Q., Liu, Y., Jin, J., et al. (2022). Intestinal butyrate-metabolizing species contribute to autoantibody production and bone erosion in rheumatoid arthritis. Sci. Adv. 8, eabm1511. doi:10.1126/sciadv.abm1511
He, L. G., Li, X. L., Zeng, X. Z., Duan, H., Wang, S., Lei, L. S., et al. (2014). Sinomenine induces apoptosis in RAW 264.7 cell-derived osteoclasts in vitro via caspase-3 activation. Acta Pharmacol. Sin. 35, 203–210. doi:10.1038/aps.2013.139
Hu, F., Liu, H., Liu, X., Zhang, X., Xu, L., Zhu, H., et al. (2017). Pathogenic conversion of regulatory B10 cells into osteoclast-priming cells in rheumatoid arthritis. J. Autoimmun. 76, 53–62. doi:10.1016/j.jaut.2016.09.002
Huang, D. N., Wu, F. F., Zhang, A. H., Sun, H., and Wang, X. J. (2021). Efficacy of berberine in treatment of rheumatoid arthritis: From multiple targets to therapeutic potential. Pharmacol. Res. 169, 105667. doi:10.1016/j.phrs.2021.105667
Huang, G., Yuan, K., Zhu, Q., Zhang, S., Lu, Q., Zhu, M., et al. (2018). Triptolide inhibits the inflammatory activities of neutrophils to ameliorate chronic arthritis. Mol. Immunol. 101, 210–220. doi:10.1016/j.molimm.2018.06.012
Hui, W., Yu, D., Cao, Z., and Zhao, X. (2019). Butyrate inhibit collagen-induced arthritis via Treg/IL-10/Th17 axis. Int. Immunopharmacol. 68, 226–233. doi:10.1016/j.intimp.2019.01.018
Hwang, J. K., Noh, E. M., Moon, S. J., Kim, J. M., Kwon, K. B., Park, B. H., et al. (2013). Emodin suppresses inflammatory responses and joint destruction in collagen-induced arthritic mice. Rheumatol. Oxf. 52, 1583–1591. doi:10.1093/rheumatology/ket178
Jia, X. Y., Chang, Y., Sun, X. J., Wu, H. X., Wang, C., Xu, H. M., et al. (2014). Total glucosides of paeony inhibit the proliferation of fibroblast-like synoviocytes through the regulation of G proteins in rats with collagen-induced arthritis. Int. Immunopharmacol. 18, 1–6. doi:10.1016/j.intimp.2013.09.007
Jia, Y., Miao, Y., Yue, M., Shu, M., Wei, Z., and Dai, Y. (2018). Tetrandrine attenuates the bone erosion in collagen-induced arthritis rats by inhibiting osteoclastogenesis via spleen tyrosine kinase. FASEB J. 32, 3398–3410. doi:10.1096/fj.201701148RR
Jia, Y., Tao, Y., Lv, C., Xia, Y., Wei, Z., and Dai, Y. (2019). Tetrandrine enhances the ubiquitination and degradation of Syk through an AhR-c-src-c-Cbl pathway and consequently inhibits osteoclastogenesis and bone destruction in arthritis. Cell Death Dis. 10, 38. doi:10.1038/s41419-018-1286-2
Jing, M., Yang, J., Zhang, L., Liu, J., Xu, S., Wang, M., et al. (2021). Celastrol inhibits rheumatoid arthritis through the ROS-NF-κB-NLRP3 inflammasome axis. Int. Immunopharmacol. 98, 107879. doi:10.1016/j.intimp.2021.107879
Karmakar, U., and Vermeren, S. (2021). Crosstalk between B cells and neutrophils in rheumatoid arthritis. Immunology 164, 689–700. doi:10.1111/imm.13412
Kelchtermans, H., Geboes, L., Mitera, T., Huskens, D., Leclercq, G., and Matthys, P. (2009). Activated CD4+CD25+ regulatory T cells inhibit osteoclastogenesis and collagen-induced arthritis. Ann. Rheum. Dis. 68, 744–750. doi:10.1136/ard.2007.086066
Keyser, F. D. (2011). Choice of biologic therapy for patients with rheumatoid arthritis: The infection perspective. Curr. Rheumatol. Rev. 7, 77–87. doi:10.2174/157339711794474620
Kim, H. R., Kim, B. M., Won, J. Y., Lee, K. A., Ko, H. M., Kang, Y. S., et al. (2019). Quercetin, a plant polyphenol, has potential for the prevention of bone destruction in rheumatoid arthritis. J. Med. Food 22, 152–161. doi:10.1089/jmf.2018.4259
Kim, H. R., Kim, K. W., Kim, B. M., Jung, H. G., Cho, M. L., and Lee, S. H. (2014). Reciprocal activation of CD4+ T cells and synovial fibroblasts by stromal cell-derived factor 1 promotes RANKL expression and osteoclastogenesis in rheumatoid arthritis. Arthritis Rheumatol. 66, 538–548. doi:10.1002/art.38286
Kim, K. W., Cho, M. L., Kim, H. R., Ju, J. H., Park, M. K., Oh, H. J., et al. (2007a). Up-regulation of stromal cell-derived factor 1 (CXCL12) production in rheumatoid synovial fibroblasts through interactions with T lymphocytes: Role of interleukin-17 and cd40l-CD40 interaction. Arthritis Rheum. 56, 1076–1086. doi:10.1002/art.22439
Kim, Y. G., Lee, C. K., Nah, S. S., Mun, S. H., Yoo, B., and Moon, H. B. (2007b). Human CD4+CD25+ regulatory T cells inhibit the differentiation of osteoclasts from peripheral blood mononuclear cells. Biochem. Biophys. Res. Commun. 357, 1046–1052. doi:10.1016/j.bbrc.2007.04.042
Komatsu, N., and Takayanagi, H. (2018). Immune-bone interplay in the structural damage in rheumatoid arthritis. Clin. Exp. Immunol. 194, 1–8. doi:10.1111/cei.13188
Kondo, Y., Yokosawa, M., Kaneko, S., Furuyama, K., Segawa, S., Tsuboi, H., et al. (2018). Review: Transcriptional regulation of CD4+ T cell differentiation in experimentally induced arthritis and rheumatoid arthritis. Arthritis Rheumatol. 70, 653–661. doi:10.1002/art.40398
Korn, T., Bettelli, E., Oukka, M., and Kuchroo, V. K. (2009). IL-17 and Th17 cells. Annu. Rev. Immunol. 27, 485–517. doi:10.1146/annurev.immunol.021908.132710
Krishnan, V., Bryant, H. U., and Macdougald, O. A. (2006). Regulation of bone mass by Wnt signaling. J. Clin. Invest. 116, 1202–1209. doi:10.1172/JCI28551
Krotova, K., Khodayari, N., Oshins, R., Aslanidi, G., and Brantly, M. L. (2020). Neutrophil elastase promotes macrophage cell adhesion and cytokine production through the integrin-Src kinases pathway. Sci. Rep. 10, 15874. doi:10.1038/s41598-020-72667-3
Kurowska, W., Slowinska, I., Krogulec, Z., Syrowka, P., and Maslinski, W. (2021). Antibodies to citrullinated proteins (ACPA) associate with markers of osteoclast activation and bone destruction in the bone marrow of patients with rheumatoid arthritis. J. Clin. Med. 10, 1778. doi:10.3390/jcm10081778
Lee, S. U., Shin, H. K., Min, Y. K., and Kim, S. H. (2008). Emodin accelerates osteoblast differentiation through phosphatidylinositol 3-kinase activation and bone morphogenetic protein-2 gene expression. Int. Immunopharmacol. 8, 741–747. doi:10.1016/j.intimp.2008.01.027
Li, H., Cao, X. Y., Dang, W. Z., Jiang, B., Zou, J., and Shen, X. Y. (2019a). Total Glucosides of Paeony protects against collagen-induced mouse arthritis via inhibiting follicular helper T cell differentiation. Phytomedicine 65, 153091. doi:10.1016/j.phymed.2019.153091
Li, P., Li, M., Lindberg, M. R., Kennett, M. J., Xiong, N., and Wang, Y. (2010). PAD4 is essential for antibacterial innate immunity mediated by neutrophil extracellular traps. J. Exp. Med. 207, 1853–1862. doi:10.1084/jem.20100239
Li, X., Yuan, K., Zhu, Q., Lu, Q., Jiang, H., Zhu, M., et al. (2019b). Andrographolide ameliorates rheumatoid arthritis by regulating the apoptosis-NETosis balance of neutrophils. Int. J. Mol. Sci. 20, 5035. doi:10.3390/ijms20205035
Li, Z. Z., Tan, J. P., Wang, L. L., and Li, Q. H. (2017). Andrographolide benefits rheumatoid arthritis via inhibiting MAPK pathways. Inflammation 40, 1599–1605. doi:10.1007/s10753-017-0600-y
Liu, C., Zhang, Y., Kong, X., Zhu, L., Pang, J., Xu, Y., et al. (2013). Triptolide prevents bone destruction in the collagen-induced arthritis model of rheumatoid arthritis by targeting RANKL/RANK/OPG signal pathway. Evid. Based. Complement. Altern. Med. 2013, 626038. doi:10.1155/2013/626038
Liu, J., Hong, X., Lin, D., Luo, X., Zhu, M., and Mo, H. (2017). Artesunate influences Th17/Treg lymphocyte balance by modulating Treg apoptosis and Th17 proliferation in a murine model of rheumatoid arthritis. Exp. Ther. Med. 13, 2267–2273. doi:10.3892/etm.2017.4232
Lu, Q., Jiang, H., Zhu, Q., Xu, J., Cai, Y., Huo, G., et al. (2022). Tetrandrine ameliorates rheumatoid arthritis in mice by alleviating neutrophil activities. Evid. Based. Complement. Altern. Med. 2022, 8589121. doi:10.1155/2022/8589121
Lucas, S., Omata, Y., Hofmann, J., Böttcher, M., Iljazovic, A., Sarter, K., et al. (2018). Short-chain fatty acids regulate systemic bone mass and protect from pathological bone loss. Nat. Commun. 9, 55. doi:10.1038/s41467-017-02490-4
Luo, C. Y., Wang, L., Sun, C., and Li, D. J. (2011). Estrogen enhances the functions of CD4(+)CD25(+)Foxp3(+) regulatory T cells that suppress osteoclast differentiation and bone resorption in vitro. Cell. Mol. Immunol. 8, 50–58. doi:10.1038/cmi.2010.54
Luo, S., Li, H., Liu, J., Xie, X., Wan, Z., Wang, Y., et al. (2020). Andrographolide ameliorates oxidative stress, inflammation and histological outcome in complete Freund's adjuvant-induced arthritis. Chem. Biol. Interact. 319, 108984. doi:10.1016/j.cbi.2020.108984
Lv, Q., Zhu, X. Y., Xia, Y. F., Dai, Y., and Wei, Z. F. (2015). Tetrandrine inhibits migration and invasion of rheumatoid arthritis fibroblast-like synoviocytes through down-regulating the expressions of Rac1, Cdc42, and RhoA GTPases and activation of the PI3K/Akt and JNK signaling pathways. Chin. J. Nat. Med. 13, 831–841. doi:10.1016/S1875-5364(15)30087-X
Ma, J. D., Jing, J., Wang, J. W., Yan, T., Li, Q. H., Mo, Y. Q., et al. (2019). A novel function of artesunate on inhibiting migration and invasion of fibroblast-like synoviocytes from rheumatoid arthritis patients. Arthritis Res. Ther. 21, 153. doi:10.1186/s13075-019-1935-6
Maruotti, N., Grano, M., Colucci, S., D'onofrio, F., and Cantatore, F. P. (2011). Osteoclastogenesis and arthritis. Clin. Exp. Med. 11, 137–145. doi:10.1007/s10238-010-0117-2
Mcinnes, I. B., Buckley, C. D., and Isaacs, J. D. (2016). Cytokines in rheumatoid arthritis — Shaping the immunological landscape. Nat. Rev. Rheumatol. 12, 63–68. doi:10.1038/nrrheum.2015.171
Mihara, M., Hashizume, M., Yoshida, H., Suzuki, M., and Shiina, M. (2011). IL-6/IL-6 receptor system and its role in physiological and pathological conditions. Clin. Sci. 122, 143–159. doi:10.1042/CS20110340
Mu, H., Yao, R. B., Zhao, L. J., Shen, S. Y., Zhao, Z. M., and Cai, H. (2013). Sinomenine decreases MyD88 expression and improves inflammation-induced joint damage progression and symptoms in rat adjuvant-induced arthritis. Inflammation 36, 1136–1144. doi:10.1007/s10753-013-9648-5
Mu, Q., Kirby, J., Reilly, C. M., and Luo, X. M. (2017). Leaky gut as a danger signal for autoimmune diseases. Front. Immunol. 8, 598. doi:10.3389/fimmu.2017.00598
Nam, B., Park, H., Lee, Y. L., Oh, Y., Park, J., Kim, S. Y., et al. (2020). TGFβ1 suppressed matrix mineralization of osteoblasts differentiation by regulating SMURF1-C/EBPβ-DKK1 Axis. Int. J. Mol. Sci. 21, 9771. doi:10.3390/ijms21249771
Nanjundaiah, S. M., Venkatesha, S. H., Yu, H., Tong, L., Stains, J. P., and Moudgil, K. D. (2012). Celastrus and its bioactive celastrol protect against bone damage in autoimmune arthritis by modulating osteoimmune cross-talk. J. Biol. Chem. 287, 22216–22226. doi:10.1074/jbc.M112.356816
Neumann, E., Lefèvre, S., Zimmermann, B., Gay, S., and Müller-Ladner, U. (2010). Rheumatoid arthritis progression mediated by activated synovial fibroblasts. Trends Mol. Med. 16, 458–468. doi:10.1016/j.molmed.2010.07.004
Nishihara, M., Ogura, H., Ueda, N., Tsuruoka, M., Kitabayashi, C., Tsuji, F., et al. (2007). IL-6-gp130-STAT3 in T cells directs the development of IL-17+ Th with a minimum effect on that of Treg in the steady state. Int. Immunol. 19, 695–702. doi:10.1093/intimm/dxm045
Niu, Q., Cai, B., Huang, Z.-C., Shi, Y.-Y., and Wang, L.-L. (2012). Disturbed Th17/Treg balance in patients with rheumatoid arthritis. Rheumatol. Int. 32, 2731–2736. doi:10.1007/s00296-011-1984-x
Nygaard, G., and Firestein, G. S. (2020). Restoring synovial homeostasis in rheumatoid arthritis by targeting fibroblast-like synoviocytes. Nat. Rev. Rheumatol. 16, 316–333. doi:10.1038/s41584-020-0413-5
O'neil, L. J., Barrera-Vargas, A., Sandoval-Heglund, D., Merayo-Chalico, J., Aguirre-Aguilar, E., Aponte, A. M., et al. (2020). Neutrophil-mediated carbamylation promotes articular damage in rheumatoid arthritis. Sci. Adv. 6, eabd2688. doi:10.1126/sciadv.abd2688
O'neil, L. J., and Kaplan, M. J. (2019). Neutrophils in rheumatoid arthritis: Breaking immune tolerance and fueling disease. Trends Mol. Med. 25, 215–227. doi:10.1016/j.molmed.2018.12.008
Pan, H., Guo, R., Ju, Y., Wang, Q., Zhu, J., Xie, Y., et al. (2019). A single bacterium restores the microbiome dysbiosis to protect bones from destruction in a rat model of rheumatoid arthritis. Microbiome 7, 107. doi:10.1186/s40168-019-0719-1
Peng, J., Lu, X., Xie, K., Xu, Y., He, R., Guo, L., et al. (2019). Dynamic alterations in the gut microbiota of collagen-induced arthritis rats following the prolonged administration of total glucosides of paeony. Front. Cell. Infect. Microbiol. 9, 204. doi:10.3389/fcimb.2019.00204
Piao, X., Zhou, J., and Xue, L. (2021). Triptolide decreases rheumatoid arthritis fibroblast-like synoviocyte proliferation, invasion, inflammation and presents a therapeutic effect in collagen-induced arthritis rats via inactivating lncRNA RP11-83J16.1 mediated URI1 and β-catenin signaling. Int. Immunopharmacol. 99, 108010. doi:10.1016/j.intimp.2021.108010
Ponzetti, M., and Rucci, N. (2021). Osteoblast differentiation and signaling: Established concepts and emerging topics. Int. J. Mol. Sci. 22, 6651. doi:10.3390/ijms22136651
Ru, J., Ma, J., Niu, H., Chen, Y., Li, L., Liu, Y., et al. (2019). Burden and depression in caregivers of patients with rheumatoid arthritis in China. Int. J. Rheum. Dis. 22, 608–613. doi:10.1111/1756-185X.13397
Sakkas, L. I., Bogdanos, D. P., Katsiari, C., and Platsoucas, C. D. (2014). Anti-citrullinated peptides as autoantigens in rheumatoid arthritis-relevance to treatment. Autoimmun. Rev. 13, 1114–1120. doi:10.1016/j.autrev.2014.08.012
Sardar, S., and Andersson, A. (2016). Old and new therapeutics for rheumatoid arthritis: In vivo models and drug development. Immunopharmacol. Immunotoxicol. 38, 2–13. doi:10.3109/08923973.2015.1125917
Sato, K., Suematsu, A., Okamoto, K., Yamaguchi, A., Morishita, Y., Kadono, Y., et al. (2006). Th17 functions as an osteoclastogenic helper T cell subset that links T cell activation and bone destruction. J. Exp. Med. 203, 2673–2682. doi:10.1084/jem.20061775
Schett, G., and Gravallese, E. (2012). Bone erosion in rheumatoid arthritis: Mechanisms, diagnosis and treatment. Nat. Rev. Rheumatol. 8, 656–664. doi:10.1038/nrrheum.2012.153
Seely, K. D., Kotelko, C. A., Douglas, H., Bealer, B., and Brooks, A. E. (2021). The human gut microbiota: A key mediator of osteoporosis and osteogenesis. Int. J. Mol. Sci. 22, 9452. doi:10.3390/ijms22179452
Shen, M. Y., Wang, X., Di, Y. X., Zhang, M. F., Tian, F. X., Qian, F. Y., et al. (2022). Triptolide inhibits Th17 differentiation via controlling PKM2-mediated glycolysis in rheumatoid arthritis. Immunopharmacol. Immunotoxicol., 1–12. doi:10.1080/08923973.2022.2086139
Shen, P., Jiao, Y., Miao, L., Chen, J. H., and Momtazi-Borojeni, A. A. (2020). Immunomodulatory effects of berberine on the inflamed joint reveal new therapeutic targets for rheumatoid arthritis management. J. Cell. Mol. Med. 24, 12234–12245. doi:10.1111/jcmm.15803
Shi, Y., Shu, H., Wang, X., Zhao, H., Lu, C., Lu, A., et al. (2020). Potential advantages of bioactive compounds extracted from traditional Chinese medicine to inhibit bone destructions in rheumatoid arthritis. Front. Pharmacol. 11, 561962. doi:10.3389/fphar.2020.561962
Smolen, J. S., Aletaha, D., and Mcinnes, I. B. (2016). Rheumatoid arthritis. Lancet 388, 2023–2038. doi:10.1016/S0140-6736(16)30173-8
Smolen, J. S., Landewé, R. B. M., Bijlsma, J. W. J., Burmester, G. R., Dougados, M., Kerschbaumer, A., et al. (2020). EULAR recommendations for the management of rheumatoid arthritis with synthetic and biological disease-modifying antirheumatic drugs: 2019 update. Ann. Rheum. Dis. 79, 685–699. doi:10.1136/annrheumdis-2019-216655
Speziani, C., Rivollier, A., Gallois, A., Coury, F., Mazzorana, M., Azocar, O., et al. (2007). Murine dendritic cell transdifferentiation into osteoclasts is differentially regulated by innate and adaptive cytokines. Eur. J. Immunol. 37, 747–757. doi:10.1002/eji.200636534
Strzepa, A., Pritchard, K. A., and Dittel, B. N. (2017). Myeloperoxidase: A new player in autoimmunity. Cell. Immunol. 317, 1–8. doi:10.1016/j.cellimm.2017.05.002
Sur Chowdhury, C., Giaglis, S., Walker, U. A., Buser, A., Hahn, S., and Hasler, P. (2014). Enhanced neutrophil extracellular trap generation in rheumatoid arthritis: Analysis of underlying signal transduction pathways and potential diagnostic utility. Arthritis Res. Ther. 16, R122. doi:10.1186/ar4579
Takayanagi, H. (2015). Osteoimmunology in 2014: Two-faced immunology-from osteogenesis to bone resorption. Nat. Rev. Rheumatol. 11, 74–76. doi:10.1038/nrrheum.2014.219
Takegahara, N., Kim, H., and Choi, Y. (2022). RANKL biology. Bone 159, 116353. doi:10.1016/j.bone.2022.116353
Tanaka, T., Narazaki, M., and Kishimoto, T. (2014). IL-6 in inflammation, immunity, and disease. Cold Spring Harb. Perspect. Biol. 6, a016295. doi:10.1101/cshperspect.a016295
Tang, M., Tian, L., Luo, G., and Yu, X. (2018). Interferon-gamma-mediated osteoimmunology. Front. Immunol. 9, 1508. doi:10.3389/fimmu.2018.01508
Tong, B., Yu, J., Wang, T., Dou, Y., Wu, X., Kong, L., et al. (2015). Sinomenine suppresses collagen-induced arthritis by reciprocal modulation of regulatory T cells and Th17 cells in gut-associated lymphoid tissues. Mol. Immunol. 65, 94–103. doi:10.1016/j.molimm.2015.01.014
Tunyogi-Csapo, M., Kis-Toth, K., Radacs, M., Farkas, B., Jacobs, J. J., Finnegan, A., et al. (2008). Cytokine-controlled RANKL and osteoprotegerin expression by human and mouse synovial fibroblasts: Fibroblast-mediated pathologic bone resorption. Arthritis Rheum. 58, 2397–2408. doi:10.1002/art.23653
Tyagi, A. M., Yu, M., Darby, T. M., Vaccaro, C., Li, J. Y., Owens, J. A., et al. (2018). The microbial metabolite butyrate stimulates bone formation via T regulatory cell-mediated regulation of WNT10B expression. Immunity 49, 1116–1131. doi:10.1016/j.immuni.2018.10.013
Van Delft, M. a. M., and Huizinga, T. W. J. (2020). An overview of autoantibodies in rheumatoid arthritis. J. Autoimmun. 110, 102392. doi:10.1016/j.jaut.2019.102392
Van Steendam, K., Tilleman, K., De Ceuleneer, M., De Keyser, F., Elewaut, D., and Deforce, D. (2010). Citrullinated vimentin as an important antigen in immune complexes from synovial fluid of rheumatoid arthritis patients with antibodies against citrullinated proteins. Arthritis Res. Ther. 12, R132. doi:10.1186/ar3070
Villalvilla, A., Gomez, R., Roman-Blas, J. A., Largo, R., and Herrero-Beaumont, G. (2014). SDF-1 signaling: A promising target in rheumatic diseases. Expert Opin. Ther. Targets 18, 1077–1087. doi:10.1517/14728222.2014.930440
Vita, A. A., Aljobaily, H., Lyons, D. O., and Pullen, N. A. (2021). Berberine delays onset of collagen-induced arthritis through T cell suppression. Int. J. Mol. Sci. 22, 3522. doi:10.3390/ijms22073522
Wang, Z., Chen, Z., Yang, S., Wang, Y., Huang, Z., Gao, J., et al. (2014). Berberine ameliorates collagen-induced arthritis in rats associated with anti-inflammatory and anti-angiogenic effects. Inflammation 37, 1789–1798. doi:10.1007/s10753-014-9909-y
Wehmeyer, C., Frank, S., Beckmann, D., Böttcher, M., Cromme, C., König, U., et al. (2016). Sclerostin inhibition promotes TNF-dependent inflammatory joint destruction. Sci. Transl. Med. 8, 330ra35. doi:10.1126/scitranslmed.aac4351
Wei, C. C., You, F. T., Mei, L. Y., Jian, S., and Qiang, C. Y. (2013). Total glucosides of paeony prevents juxta-articular bone loss in experimental arthritis. BMC Complement. Altern. Med. 13, 186. doi:10.1186/1472-6882-13-186
Weissmann, G., and Korchak, H. (1984). Rheumatoid arthritis. The role of neutrophil activation. Inflammation 8, S3–S14. doi:10.1007/BF00915708
Weivoda, M. M., Youssef, S. J., and Oursler, M. J. (2017). Sclerostin expression and functions beyond the osteocyte. Bone 96, 45–50. doi:10.1016/j.bone.2016.11.024
Wong, S. K., Chin, K.-Y., and Ima-Nirwana, S. (2020). Quercetin as an agent for protecting the bone: A review of the current evidence. Int. J. Mol. Sci. 21, 6448. doi:10.3390/ijms21176448
Wu, C. Y., Yang, H. Y., and Lai, J. H. (2020). Anti-citrullinated protein antibodies in patients with rheumatoid arthritis: Biological effects and mechanisms of immunopathogenesis. Int. J. Mol. Sci. 21, E4015. doi:10.3390/ijms21114015
Wu, H., Wu, X., Huang, L., Ruan, C., Liu, J., Chen, X., et al. (2021). Effects of andrographolide on mouse intestinal microflora based on high-throughput sequence analysis. Front. Vet. Sci. 8, 702885. doi:10.3389/fvets.2021.702885
Xu, F., Zhang, C., Zou, Z., Fan, E. K. Y., Chen, L., Li, Y., et al. (2017). Aging-related Atg5 defect impairs neutrophil extracellular traps formation. Immunology 151, 417–432. doi:10.1111/imm.12740
Xu, H., He, Y., Yang, X., Liang, L., Zhan, Z., Ye, Y., et al. (2007). Anti-malarial agent artesunate inhibits TNF-alpha-induced production of proinflammatory cytokines via inhibition of NF-kappaB and PI3 kinase/Akt signal pathway in human rheumatoid arthritis fibroblast-like synoviocytes. Rheumatol. Oxf. 46, 920–926. doi:10.1093/rheumatology/kem014
Xu, H., Zhao, H., Lu, C., Qiu, Q., Wang, G., Huang, J., et al. (2016). Triptolide inhibits osteoclast differentiation and bone resorption in vitro via enhancing the production of IL-10 and TGF-β1 by regulatory T cells. Mediat. Inflamm. 2016, 8048170. doi:10.1155/2016/8048170
Xu, W., Chen, S., Wang, X., Wu, H., Tahara, K., Tanaka, S., et al. (2021). Effects of sinomenine on the proliferation, cytokine production, and regulatory T-cell frequency in peripheral blood mononuclear cells of rheumatoid arthritis patients. Drug Dev. Res. 82, 251–258. doi:10.1002/ddr.21748
Xu, Z., Wu, G., Wei, X., Chen, X., Wang, Y., and Chen, L. (2013). Celastrol induced DNA damage, cell cycle arrest, and apoptosis in human rheumatoid fibroblast-like synovial cells. Am. J. Chin. Med. 41, 615–628. doi:10.1142/S0192415X13500432
Yamaguchi, M., and Weitzmann, M. N. (2011). Quercetin, a potent suppressor of NF-κB and Smad activation in osteoblasts. Int. J. Mol. Med. 28, 521–525. doi:10.3892/ijmm.2011.749
Yan, J., Chen, Y., He, C., Yang, Z. Z., Lü, C., and Chen, X. S. (2012). Andrographolide induces cell cycle arrest and apoptosis in human rheumatoid arthritis fibroblast-like synoviocytes. Cell Biol. Toxicol. 28, 47–56. doi:10.1007/s10565-011-9204-8
Yan, J., Herzog, J. W., Tsang, K., Brennan, C. A., Bower, M. A., Garrett, W. S., et al. (2016). Gut microbiota induce IGF-1 and promote bone formation and growth. Proc. Natl. Acad. Sci. U. S. A. 113, E7554–E7563. doi:10.1073/pnas.1607235113
Yang, Y., Ye, Y., Qiu, Q., Xiao, Y., Huang, M., Shi, M., et al. (2016). Triptolide inhibits the migration and invasion of rheumatoid fibroblast-like synoviocytes by blocking the activation of the JNK MAPK pathway. Int. Immunopharmacol. 41, 8–16. doi:10.1016/j.intimp.2016.10.005
Yang, Y., Zhang, X., Xu, M., Wu, X., Zhao, F., and Zhao, C. (2018). Quercetin attenuates collagen-induced arthritis by restoration of Th17/Treg balance and activation of Heme Oxygenase 1-mediated anti-inflammatory effect. Int. Immunopharmacol. 54, 153–162. doi:10.1016/j.intimp.2017.11.013
Yeo, L., Lom, H., Juarez, M., Snow, M., Buckley, C. D., Filer, A., et al. (2015). Expression of FcRL4 defines a pro-inflammatory, RANKL-producing B cell subset in rheumatoid arthritis. Ann. Rheum. Dis. 74, 928–935. doi:10.1136/annrheumdis-2013-204116
Yeo, L., Toellner, K. M., Salmon, M., Filer, A., Buckley, C. D., Raza, K., et al. (2011). Cytokine mRNA profiling identifies B cells as a major source of RANKL in rheumatoid arthritis. Ann. Rheum. Dis. 70, 2022–2028. doi:10.1136/ard.2011.153312
Yeremenko, N., Zwerina, K., Rigter, G., Pots, D., Fonseca, J. E., Zwerina, J., et al. (2015). Tumor necrosis factor and interleukin-6 differentially regulate Dkk-1 in the inflamed arthritic joint. Arthritis Rheumatol. 67, 2071–2075. doi:10.1002/art.39183
Yi, L., Lyn, Y. J., Peng, C., Zhu, R. L., Bai, S. S., Liu, L., et al. (2018). Sinomenine inhibits fibroblast-like synoviocyte proliferation by regulating α7nAChR expression via ERK/Egr-1 pathway. Int. Immunopharmacol. 56, 65–70. doi:10.1016/j.intimp.2018.01.015
Yu, Y., Koehn, C. D., Yue, Y., Li, S., Thiele, G. M., Hearth-Holmes, M. P., et al. (2015). Celastrol inhibits inflammatory stimuli-induced neutrophil extracellular trap formation. Curr. Mol. Med. 15, 401–410. doi:10.2174/1566524015666150505160743
Yuan, K., Zhu, Q., Lu, Q., Jiang, H., Zhu, M., Li, X., et al. (2020). Quercetin alleviates rheumatoid arthritis by inhibiting neutrophil inflammatory activities. J. Nutr. Biochem. 84, 108454. doi:10.1016/j.jnutbio.2020.108454
Yuan, X., Dou, Y., Wu, X., Wei, Z., and Dai, Y. (2017). Tetrandrine, an agonist of aryl hydrocarbon receptor, reciprocally modulates the activities of STAT3 and STAT5 to suppress Th17 cell differentiation. J. Cell. Mol. Med. 21, 2172–2183. doi:10.1111/jcmm.13141
Yuan, X., Tong, B., Dou, Y., Wu, X., Wei, Z., and Dai, Y. (2016). Tetrandrine ameliorates collagen-induced arthritis in mice by restoring the balance between Th17 and Treg cells via the aryl hydrocarbon receptor. Biochem. Pharmacol. 101, 87–99. doi:10.1016/j.bcp.2015.11.025
Yue, M., Tao, Y., Fang, Y., Lian, X., Zhang, Q., Xia, Y., et al. (2019). The gut microbiota modulator berberine ameliorates collagen-induced arthritis in rats by facilitating the generation of butyrate and adjusting the intestinal hypoxia and nitrate supply. Faseb J. 33, 12311–12323. doi:10.1096/fj.201900425RR
Yue, M., Xia, Y., Shi, C., Guan, C., Li, Y., Liu, R., et al. (2017). Berberine ameliorates collagen-induced arthritis in rats by suppressing Th17 cell responses via inducing cortistatin in the gut. Febs J. 284, 2786–2801. doi:10.1111/febs.14147
Zhang, Y., Ma, J., and Zhang, W. (2021). Berberine for bone regeneration: Therapeutic potential and molecular mechanisms. J. Ethnopharmacol. 277, 114249. doi:10.1016/j.jep.2021.114249
Zheng, Q., Li, S., Li, X., and Liu, R. (2021). Advances in the study of emodin: An update on pharmacological properties and mechanistic basis. Chin. Med. 16, 102. doi:10.1186/s13020-021-00509-z
Zhu, M. Y., Lin, D., Liu, J., and Mo, H. Y. (2016). Artesunate interfere in modulation of Foxp3 expression in synovial cells in collagen-induced arthritis rats. Chin. J. Integr. Med. [Epub ahead of print]. doi:10.1007/s11655-016-2611-1
Keywords: rheumatoid arthritis, traditional Chinese medicine, osteoclast, active components, bone protection, bone destruction
Citation: Lu Q, Xu J, Jiang H, Wei Q, Huang R and Huang G (2022) The bone-protective mechanisms of active components from TCM drugs in rheumatoid arthritis treatment. Front. Pharmacol. 13:1000865. doi: 10.3389/fphar.2022.1000865
Received: 22 July 2022; Accepted: 10 October 2022;
Published: 25 October 2022.
Edited by:
Thomas Brzozowski, Jagiellonian University Medical College, PolandReviewed by:
Zhidong Qiu, Changchun University of Chinese Medicine, ChinaCopyright © 2022 Lu, Xu, Jiang, Wei, Huang and Huang. This is an open-access article distributed under the terms of the Creative Commons Attribution License (CC BY). The use, distribution or reproduction in other forums is permitted, provided the original author(s) and the copyright owner(s) are credited and that the original publication in this journal is cited, in accordance with accepted academic practice. No use, distribution or reproduction is permitted which does not comply with these terms.
*Correspondence: Guangrui Huang, aGdyQGJ1Y20uZWR1LmNu; Runyue Huang, cnlodWFuZ0BnenVjbS5lZHUuY24=
†These authors have contributed equally to this work
Disclaimer: All claims expressed in this article are solely those of the authors and do not necessarily represent those of their affiliated organizations, or those of the publisher, the editors and the reviewers. Any product that may be evaluated in this article or claim that may be made by its manufacturer is not guaranteed or endorsed by the publisher.
Research integrity at Frontiers
Learn more about the work of our research integrity team to safeguard the quality of each article we publish.