- Institute of Lipid Metabolism and Atherosclerosis, Innovative Drug Research Centre, School of Pharmacy, Weifang Medical University, Weifang, China
Dyslipidemias are disorders of plasma levels of lipids, such as elevated levels of total cholesterol and triglyceride, that are associated with various human diseases including cardiovascular disease (CVD) and non-alcoholic fatty liver disease (NAFLD). Statins are the first-line drugs for treatment of dyslipidemia. However, a substantial proportion of patients cannot reach the recommended LDL-c level even with the highest tolerated doses of statins, and there is no available drug specifically for NAFLD therapy. Sea cucumbers are one of the widely distributed invertebrates, and are an important resource of food and medicine. Sea cucumbers have many valuable nutrients including saponins, fatty acids, phospholipids, cerebrosides, sulfated polysaccharides, as well as proteins and peptides. In recent years, these natural products derived from sea cucumbers have attracted attentions for treatment of CVD and NAFLD because of their lipid-lowering effect and low toxicity. However, the hypolipidemic mechanisms of action and the structure-activity relationship of these bioactive components have not been well-documented in literature. This review article summarizes the signaling pathways and the potential structure-activity relationship of sea cucumber-derived bioactive compounds including saponins, lipids, carbohydrates as well as peptides and proteins. This article will provide information useful for the development of sea cucumber-derived lipid-lowering compounds as well as for investigation of hypolipidemic compounds that are derived from other natural resources.
Introduction
Lipid homeostasis is finely tuned by multiple systems and organs, which interact with each other through cross-talks via cellular signaling upon molecular stimulation. Peroxisome proliferator-activated receptors (PPARs) are lipid sensors and play key roles in lipid homeostasis. PPARα is primarily expressed in liver, brown adipose tissue, heart, and muscle tissue. It is the master regulator of lipid metabolism via modulating fatty acid (FA) transport and β-oxidation. PPARγ is expressed mainly in adipose tissue, where it regulates adipogenesis (Tian et al., 2020). The wingless-type MMTV integration site (WNT)/β-Catenin pathway plays a key role in regulating adipogenesis. Glycogen synthase kinase-3β can phosphorylate β-Catenin, thereby causing degradation of β-Catenin. Furthermore, the frizzled receptor (Fz) and lipoprotein receptor-related protein (LRP)5/6 coreceptors located at the cell membrane can bind to WNT10b, destructing the activated degradation of β-Catenin, thereby promoting the accumulation of β-Catenin in cytoplasm and the subsequent nuclear translocation. In the nucleus, β-Catenin binds to T cell factor lymphoid enhancer factor family to activate downstream genes, such as cyclin D1 and C-myc, leading to inhibition of the expression of PPARγ and CCAAT/enhancer binding protein-α (C/EBPα) (Christodoulides et al., 2009; Lee et al., 2010; Xu et al., 2014; Xu et al., 2015a). Additionally, accumulating evidence have demonstrated that gut microbiota consisted of trillions of bacteria affect host lipid homeostasis (Aron-Wisnewsky et al., 2021; de Vos et al., 2022).
Dyslipidemias are disorders of plasma levels of lipids, such as elevated levels of total cholesterol (TC) and triglyceride (TG), that are associated with various human diseases including cardiovascular disease (CVD) and fatty liver diseases (Pirillo et al., 2021). Accumulating evidence have demonstrated that hypercholesteremia, especially elevated level of low-density lipoprotein (LDL) cholesterol (LDL-c), is the major risk factor for CVD (Atar et al., 2021). Furthermore, hypertriglyceridemia is a key risk factor of the residual CVD and non-alcoholic fatty liver disease (NAFLD) (Heeren and Scheja, 2021; Zhang B. H. et al., 2022). According to the World Health Organization report in 2021, CVD remains the leading cause of human death and accounts for approximately 32% of the total deaths in 2019 (World Health Organization, 2021). NAFLD is characterized by the accumulation of TG and cholesterol in the liver and has a global prevalence of 25% (Powell et al., 2021). Lipid-lowering therapy is an effective strategy for prevention and/or treatment of CVD as well as NAFLD that are induced by dyslipidemia (Beshir et al., 2021; Ferraro et al., 2022). Statins are the first-line drugs for treatment of dyslipidemia. However, a substantial proportion of patients cannot reach the recommended LDL-c level even with the maximum tolerated doses of statins (De Backer et al., 2019). Furthermore, the overall efficacy of non-statin drugs on CVD outcomes is much less robust than that of statins (Visseren et al., 2021), and there is no available drug specifically for NAFLD therapy. In recent years, natural products have attracted attentions for treatment of CVD and NAFLD due to their powerful hypolipidemic effects and low toxicity (Singh and Sashidhara, 2017; Li et al., 2022).
Sea cucumbers are one of the widely distributed invertebrates, and are an important resource for food and medicine. The idea of “medicine and food are homologous (药食同源)” has been widely accepted in Asia, especially in China, and this idea is spreading all over the world. As reviewed previously, sea cucumbers have many valuable nutrients including vitamins, minerals, triterpene glycosides (saponins), sulfated polysaccharides, sterols, phenolics, cerebrosides, peptides, FA, and others. These components show various bioactivities such as anti-angiogenic, anti-tumor, anticoagulant, anti-hypertension, anti-inflammatory, anti-oxidant, antithrombotic, antimicrobial, immunomodulatory, and wound healing functions (Bordbar et al., 2011; Khotimchenko, 2018). Diets containing sea cucumber (Isostichopus badionotus) meals can reduce serum levels of TC and TG in young rats via modulating the expression of multiple genes including sterol regulatory element-binding transcription factor (SREBP), 3-hydroxy-3-methyl-glutaryl-CoA reductase (HMGCR), and liver X receptor (LXR) that are associated with lipogenesis (Olivera-Castillo et al., 2013). In the past decades, the lipid-modulatory mechanisms of action of the compounds derived from sea cucumbers have been understood. Additionally, novel technologies, such as liquid chromatography-tandem mass spectrometry (LC-MS/MS), are applied for the detection of metabolites of sea cucumbers (Wang et al., 2020; Zhao et al., 2020; Savarino et al., 2021). These advances make it possible to discuss the structure-activity relationship of these compounds obtained from sea cucumber. In this article, we summarize the lipid-lowering mechanisms of action and the potential structure-activity relationship of sea cucumber-derived bioactive components including saponin, lipid, long chain base, carbohydrate, peptide and protein. The related literature used in this article were mainly obtained as search results from PubMed using “sea cucumber and lipid” as keywords.
The lipid-modulatory mechanisms of sea cucumber-derived compounds
Saponin
Saponin is one of the most important secondary metabolites and bioactive constitutes of sea cucumbers (Meng et al., 2018). In a comparative study, saponins derived from Cucumaria frondosa show better lipid-lowering activity compared to other components of sea cucumber including polysaccharides, collagen peptides, dregs, or non-saponin residues in rats. The underlying mechanism of action is associated with inhibition of the activity of pancreatic lipase, which is responsible for hydrolysis of dietary fat in the small intestine (Hu X. et al., 2012; Hu X. Q. et al., 2012). The crude saponins of sea cucumbers are generally extracted with 60% ethanol, and the obtained mixture can be further extracted with water-saturated n-butanol to obtain the final crude extracts that are consisted approximately 66% of saponins. These extracts obtained from sea cucumbers, such as Thelenota ananas, Pearsonothuria graeffei Semper (Holothuriidae), and Holothuia fuscogliva, significantly downregulate the activity of pancreatic lipase in vitro. Of note, the major bioactive component of saponin, echinoside A (Figure 1) accounts for 35.6% of the above water-saturated n-butanol dissolved components and has a 50% inhibitory concentration of 0.76 μM for pancreatic lipase in vitro (Guo et al., 2016). The 60% ethanol extract of P. graeffei reduces the body weight, serum levels of TC, TG, and LDL-c, and hepatic TC and TG in C57BL/6 mice fed with a high-fat diet. Mechanistically, this extract upregulates the LXR-β signaling molecules including LXR-β, ATP-binding cassette transporter (ABC) G1, and cholesterol 7-α hydroxylase (CYP7A1). Importantly, the bioactive component echinoside A acts not only by upregulating the expression of LXR-β but also via enhancing the expression of LXR-α, ABCG1, apolipoprotein (apo) E, and CYP7A1 in HepG2 cells at the dose of 2.5 μM (Guo et al., 2016). These data suggest that saponins, such as echinoside A, may alleviate hyperlipidemia via promoting the conversion of cholesterol to bile acid as well as lipid excretion.
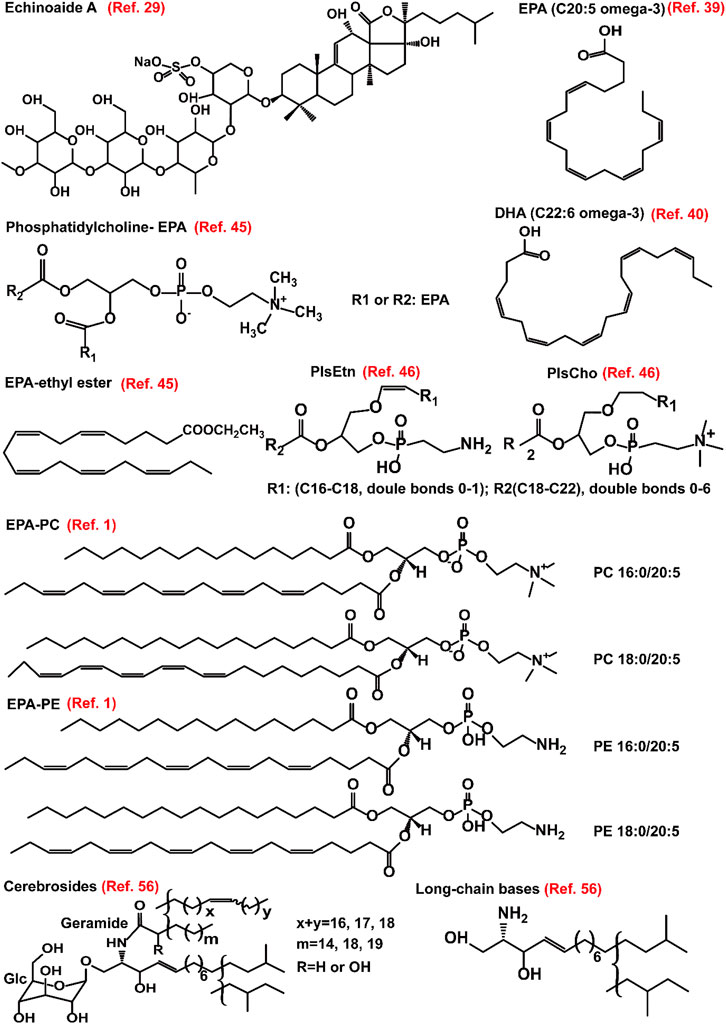
FIGURE 1. Structure of representative small molecules that are derived from sea cucumbers. These small molecules are found to modulate hyperlipidemia in different models including non-alcoholic fatty acid disease and atherosclerosis. EPA, eicosapentaenoic acid; DHA, docosahexaenoic acid; PC, phosphatidylcholine; PE, phosphatidylethanoamine; PlsCho, plasmanyl phosphatidylcholine; PlsEtn, plasmenyl phosphatidylethanoamine.
Furthermore, sea cucumber saponins reduce lipogenesis and promote FA β-oxidation via inhibiting SREBP-1c and enhancing the expression of PPARα and acyl-CoA oxidase 1 (ACOX1), respectively, thereby improving lipid deposition in Sprague-Dawley (SD) rats and C57BL/6 mice (Hu et al., 2010; Guo et al., 2018; Meng et al., 2018). Saponins inhibit the activity and mRNA expression of lipogenic enzymes including fatty acid synthase (FAS), malic enzyme, and glucose-6-phosphate dehydrogenase (G6PDH) in the liver of mice fed with the diet containing 1% OA and 0.05% saponins (Hu et al., 2010). In combination with eicosapentaenoic acid (EPA)-enriched phospholipids, sea cucumber saponins further reduce hepatic TG partially by enhancing the expression of PPARα. Furthermore, this combination shows better effect on improving glucose intolerance and systematic insulin sensitivity than monotherapy (Han et al., 2019). Interestingly, sea cucumber saponin treatment induces changes of lipid metabolism-related genes such as PPARα, SREBP-1c, carnitine palmitoyl transferase (CPT), and FAS in rhythm, suggesting saponin may modulate lipid metabolism by regulating the clock genes such as CLOCK and BMAL1 in the ICR male mice fed with 0.03% sea cucumber saponin in regular chow (Wen et al., 2014). The major bioactive component of saponin, echinoside A, also regulates the expression of some key genes involved in lipid metabolism in a diurnal manner (Wen et al., 2016). The mechanisms of action of saponins are summarized in Figure 2.
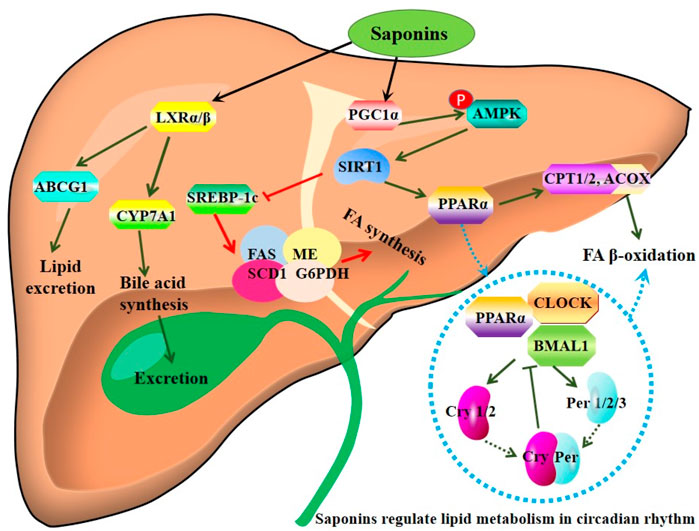
FIGURE 2. Mechanisms of action of saponins derived from sea cucumbers. Saponins stimulate LXR and AMPK/PPARα signaling pathways, thereby promoting lipid expression and fatty acid (FA) β-oxidation. Of note, these saponins regulate lipid metabolism-related genes in circadian rhythm. ABCG1: ATP-binding cassette transporter G1; ACOX1: acyl-CoA oxidase 1; AMPK: AMP-activated protein kinase; Cry: cryptochrome gene; CYP7A1: cholesterol 7-α-hydroxylase A1; FAS: fatty acid synthase; G6PDH: glucose-6-phosphate dehydrogenase; LXR: liver X receptor; ME: malic enzyme; CPT1: carnitine palmitoyl transferase 1; PGC-1α: peroxisome proliferator-activated receptor-γ co-activator 1α; Per: period gene; PPARα: peroxisome proliferator activated receptor α; SCD-1: Stearoyl-CoA desaturase-1; SIRT1: Sirtuin 1; SREBP-1c: sterol regulatory element-binding protein-1c. All the abbreviations are applicable for the rest Figures.
FA and phospholipid
Exogenous molecules can modulate lipid homeostasis by different signaling pathways. Exogenous lipids are recycled and/or degraded and participate in the formation of lipid raft, thereby affecting raft-related signaling pathways (Abumrad et al., 2012; Duan et al., 2012). Sea cucumber is a valuable food of FAs as well as other nutritional phospholipids (Roggatz et al., 2016; Roggatz et al., 2018). In the sea cucumber Athyonidium chilensis, saturated FAs are predominant in the tubule phospholipids (40.7%), while monounsaturated FAs account for approximately 42.0% and 38.0% of the phospholipids in the internal organs and body wall, respectively. The major polyunsaturated FAs are C20: 2ω-6 FA, arachidonic acid (C20: 4ω-6), and EPA (C20-5ω-3) (Figure 1) (Careaga et al., 2013). Furthermore, the presence of the odd carbon saturated FAs may be originated from the detritus, which is a part of the diet of sea cucumber (Careaga et al., 2013). Different solvents have distinct ability for extraction of FAs, and water is found to have a higher efficiency for extraction of docosahexaenoic acid (C22:6, Figure 1) compared to phosphate buffer saline, methanol, or ethanol (Fredalina et al., 1999). Accumulating evidence have demonstrated the health-beneficial effects of polyunsaturated FAs for treatment of lipid disorders (Djuricic and Calder, 2021; Mitrovic et al., 2022). Therefore, FAs, especially the n-3 polyunsaturated FAs that are enriched in sea cucumbers have potential application in pharmaceutical industries for intervention of dyslipidemias.
Some EPA-enriched phospholipids are shown in Figure 1. EPA-enriched phospholipids reduce hepatic TG and TC in orotic acid-induced SD rats with NAFLD via enhancing PPARα-mediated FA β-oxidation. Furthermore, these 1% EPA-enriched phospholipids promote the expression of ACOX1 but not CPT-1 and CPT-2 (Guo et al., 2018). In rats, EPA-enriched phosphatidylcholine (EPA-PC) (80 mg/kg) attenuates NAFLD induced by 1% orotic acid via suppressing the mRNA expression of HMGCR and increasing the expression of sterol carrying protein 2, thereby inhibiting cholesterol synthesis and improving fecal cholesterol excretion, respectively. The underlying mechanisms are associated with the upregulation of PPARα and adenosine monophosphate activated protein kinase (AMPK) as well as its upstream modulators including liver kinase B1 and Ca2+-dependent kinase (Liu et al., 2017). A recent study demonstrated that PC contained in EPA-PC and PE contained in EPA-enriched phosphatidylethanoamine (EPA-PE) directly bind to and activate PPARα and PPARγ. In mouse hepatocytes and liver, 0.3% EPA-PC and 0.3% EPA-PE reduce lipid accumulation via enhancing PPARα-mediated FA β-oxidation. Although EPA-PC and EPA-PE (200 μg/ml) promote the conversion of preadipocyte to mature adipocyte in a 3T3-L1 cell model, they reduce phosphorylation of PPARγ at Ser273 in vivo, which may partially explain the reductions in the weight of adipose and the size of adipocyte (Tian et al., 2020). Furthermore, EPA-enriched phospholipids isolated from sea cucumber C. frondosa suppress lipid accumulation in mouse liver and white adipose via inhibiting the expression of lipiddroplet associated protein FSP27 and enhancing the expression of lipolysis genes including hormone-sensitive lipase (HSL), adipose triglyceride lipase (ATGL) as well as the lipogenesis gene PPARγ in the white adipose of male C57BL/6J mice fed with high-sucrose diet (Zhang et al., 2020). However, EPA-PC has no effect on FA profiles in the brain (Wen et al., 2018). Sea cucumbers are rich in ether-linked phospholipids including plasmenyl phosphatidylethanoamine (PlsEtn) and plasmanyl phosphatidylcholine (PlsCho) (Figure 1). PlsEtn accounts for >83% of the total PE, and PlsCho accounts for >59% of the total PC in sea cucumber. Both 0.3% PlsEtn and 0.3% PlsCho, especially PlsEtn, significantly reduce hepatic TC and TG in C57BL/6N mice with alcoholic liver disease induced by ethanol gavage. Mechanistically, PlsEtn and PlsCho inhibit FA uptake and TG synthesis via downregulation of the mRNA expression of hepatic cluster of differentiation 36 (CD36) and diacylglycerol acyltransferase 1, respectively. Additionally, PlsCho enhances FA oxidation via increasing the mRNA expression of CPT-1α in the liver of mice (Wang et al., 2022). The mechanisms of action of FAs and phospholipids derived from sea cucumbers are summarized in Figure 3.
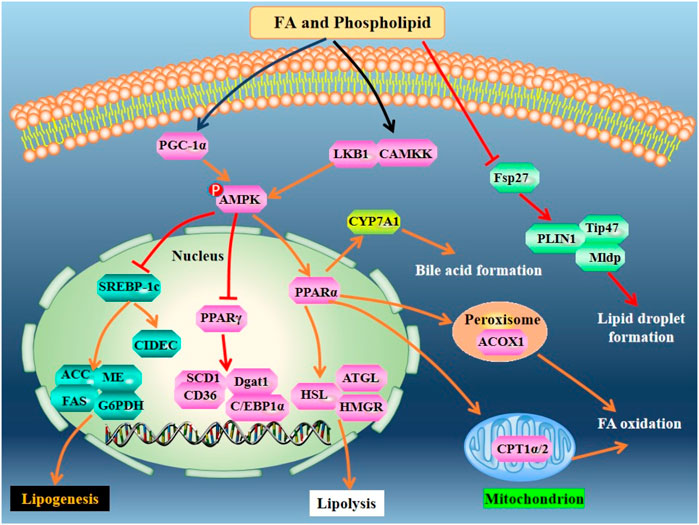
FIGURE 3. Mechanisms of action of FA and phospholipid derived from sea cucumbers. CAMKK, Ca2+-dependent kinase; C/EBPs: CCAAT/enhancer binding proteins; CIDEC: cell-death-inducing DFFA-like effector c; Dgat1, diacylglycerol acyltransferase 1; Fsp27, fat-specific protein 27; HMGR: hydroxymethyl glutaric acid acyl; LKB1, liver kinase B1; Mldp, perilipin5; PLIN1, perilipin1; Tip47, perilipin 3. All the abbreviations are applicable for the rest Figures.
Cerebroside
The structure of some glucocerebroside molecular species has been characterized by different groups (Figure 1) (Yamada et al., 2002; Kisa et al., 2005; Yamada et al., 2005). Except for the classical column separation in combination with high-performance liquid chromatography, cerebrosides can be isolated using high speed counter-current chromatography (Xu et al., 2013). Sea cucumber cerebrosides isolated from C. frondosa contain approximately 48.0% of EPA. These lipids in liposome forms have particle sizes ranging from 169 to 189 nm and can efficiently penetrate the cell membrane in an M cell monolayer model as well as in a Caco-2 cell monolayer model (Du et al., 2016). The cerebrosides isolated from Stichopus japonicus can be absorbed in vivo, and then they are incorporated into ceramides, thereby improving the skin barrier function and increasing the cecal content of short-chain FA (SCFA) (Duan et al., 2016). A recent study indicates that liposomes derived from sea cucumbers is safe even at the concentration of 0.1 mg/ml (Mecheta et al., 2020). These data suggest that sea cucumber-derived cerebrosides can be explored as drug-loading nanoparticles.
In an orotic acid-induced NALFD model, cerebrosides obtained from Acaudina molpadioides increase serum TG, but reduce liver index and hepatic TG. Mechanistically, these cerebrosides reduce the activities and expression of lipogenic enzymes including FAS, malic enzyme, and G6PDH, that are the target genes of SREBP-1c. Furthermore, the mRNA expression of SREBP-1c and the activity of microsomal triglyceride transfer protein are inhibited by cerebrosides at 0.006% in the liver of rats (Zhang et al., 2012). As a diet supplement, the cerebroside isolated from sea cucumber A. molpadioides, designated as AMC-2, can reduce hepatic TC and TG via down-regulating the activity and mRNA expression of stearoyl-CoA desaturase (SCD) in rats with NAFLD (Xu et al., 2011). In apolipoprotein E-deficient mouse, an atherosclerosis model, cerebrosides isolated from sea cucumber A. molpadioides reduce the formation of atherosclerotic plaques, serum levels of TC and LDL-c, and hepatic TC and TG when they are added to food at the dose of 0.06%. Mechanistically, cerebroside treatment promotes the expression of LDL receptor, CYP7A1 and ABCG5/G8, thereby promoting reverse cholesterol transport. Furthermore, these compounds improve FA oxidation via enhancing the expression of PPARα, CPT-1α, and ACOX1, and suppress lipogenesis by inhibiting the expression of SREBP-1c and its target genes including FAS and SCD1 (Zhang et al., 2018). The cerebrosides obtained from sea cucumber A. molpadioides also exhibit powerful effects on reduction of fat weight and serum and hepatic levels of TG via inhibiting the enzymatic activity of FAS and malic enzyme, the content of CPT, and the mRNA expression of SREBP-1c and FAS, in the liver of C57BL/6J mice fed with a diet containing 0.025% of cerebrosies. Furthermore, these cerebrosides significantly decrease the mRNA expression of very low-density lipoprotein receptor and lipoprotein lipase (LPL) and increase the expression of SREBP-1c, FAS, ATGL, and acetyl CoA carboxylase (ACC) in the white adipose tissue (Liu et al., 2015). In 3T3-L1 cells, 250 μg/ml cerebrosides isolated from sea cucumber C. frondosa promote the nuclear translocation of β-Catenin and the expression of its target genes such as cyclin D1 and C-myc, and the expression of Fz and LRPs, thereby suppressing the expression of PPARγ and C/EBPα (Xu et al., 2015a). Cerebrosides have different effects on modulation of adipocyte differentiation both in vitro and in vivo via regulation of related signaling pathways in a different manner. The mechanisms of action of cerebrosides derived from sea cucumber are summarized in Figure 4.
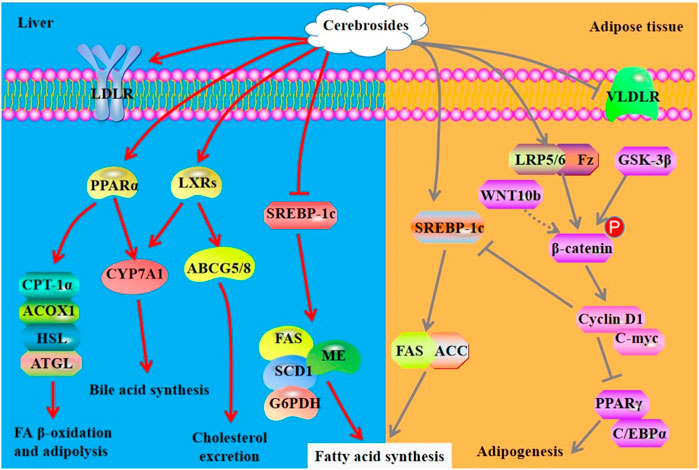
FIGURE 4. Mechanisms of action of cerebrosides derived from sea cucumbers. ACC, acetyl-CoA carboxylase; ATGL: adipose triglyceride lipase; GSK-3β, glycogen synthase kinase-3β; HSL, hormone sensitive lipase; LRP, lipoprotein receptor related protein; VLDLR, very low-density lipoprotein receptor; WNT10b, wingless-type MMTV integration site10b. All the abbreviations are applicable for the rest Figures.
Long-chain base
Long-chain bases can be obtained by acid hydrolysis of cerebrosides (using 10% HCl) (Figure 1), which are the main active structural units of cerebrosides for intervention of hyperlipidemia (Liu et al., 2015). Long-chain bases are found to ameliorate obesity by multiple pathways. The long-chain bases isolated from A. molpadioides significantly reduce the body weight, fat weight, plasma levels of TG, TC, LDL-c, glucose, leptin, and insulin, and increase the levels of plasma high density lipoprotien cholesterol (HDL-c), fecal SCFAs including acetate, propionate, and butyrate, as well as the expression of SCFAs-mediated G-protein-coupled receptors in mice. In the gut, long-chain bases induce reductions in Firmicultes and Actinobacteria phylum, and obesity-associated bacteria including Desulfovibro, Bifidobacterium, and Romboutsia at the genus level. They increase the abundance of Bacteroidetes, Proteobacteria, and Verrucomicrobia phylum, and the SCFAs-producing bacteria including Bacteroides, Lactobacillus, and Lachnospiraceae_NK4A136_group at the genus level (Hu et al., 2019). Phosphorylation of AMPK stimulates the phosphorylation of ACC, causing down-regulation of the activity of ACC and the expression of lipogenesis related enzymes including FAS. Furthermore, activation of AMPK through phosphorylation un-regulates lipidolysis via enhancing the expression of HSL and CPT-1 (Tian et al., 2016; Aslam and Ladilov, 2022). Of note, 50 μg/ml and 100 μg/ml of long-chain bases obtained from C. frondosa inhibit adipogenesis in 3T3-L1 pre-adipocytes via enhancing the phosphorylation of AMPK and ACC. Furthermore, they inhibit the transcriptional factors, such as C/EBPs and PPARγ, and activate WNT/β-catenin and its target genes including cyclin D1 and C-myc, thereby inhibiting adipocyte differentiation (Tian et al., 2016). The mechanisms of action of long chain bases derived from sea cucumber are summarized in Figure 5.
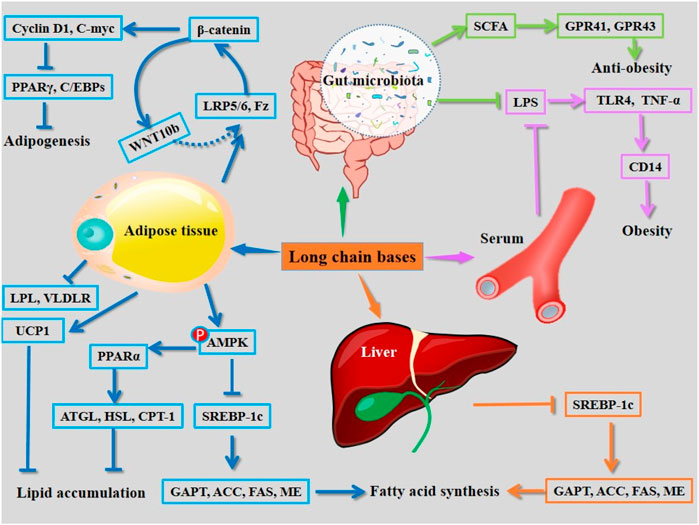
FIGURE 5. Mechanisms of action of long chain bases derived from sea cucumbers. CD14, cluster of differentiation 14; GPAT, glycerol-3-phosphate acyl-transferase; GPR, G protein-coupled receptor; LPL, lipoprotein lipase; LPS, lipopolysaccharide; SCFA, short chain fatty acid; TLR4, Toll-like receptor 4; TNF-α, tumor necrosis factor α; UCP1, uncoupling protein 1. All the abbreviations are applicable for the rest Figures.
Carbohydrate
Polysaccharides of sea cucumber reduce serum levels of TG, TC, and LDL-c, and increase the level of HDL-c in rats (Hu X.Q. et al., 2012; Liu et al., 2012). The health-beneficial activities including the hypolipidemic effect of fucosylated chondroitin sulfate (FCS) isolated from sea cucumbers have been reviewed recently by Xu et al. (2022). Here, we summarize the mechanisms of action and the structure-activity relationship of FCS as lipid-lowering agents (Figure 6). The structure of these FCSs extracted from different sea cucumbers are shown in Figure 7. FCS isolated from A. molpadioides elevates the expression of Wnt/β-Catenin signaling molecules, such as Wnt10b, β-Catenin, Fz, and LRP5, leading to down-regulation of the transcriptional factors including SREBP-1c, PPARγ, and C/EBPα, thereby exhibiting anti-adipogenic effects in 3T3-L1 cells and mice (Xu et al., 2015b). Of note, the FCS isolated from P. graeffei with 3,4-O-disulfated fucose branches exhibits a more powerful effect in lipid-lowering than the FCS obtained from I. badionotus with 2,4-O-disulfated fucose branches (Chen et al., 2012; Wu et al., 2016). These data suggest that the substitution position of the sulfate group at the branched fucosyls plays a key role in the hypolipidemic effect of FCS. Furthermore, the FCS (40 mg/kg) with a molecular weight (Mw) of 4.3 kDa obtained via degradation of FCS of I. badionotus shows hypolipidemic effect in C57BL/6 mice fed with a high-fat diet by down-regulating the mRNA expression of FAS and PPARγ in adipose tissues (Li et al., 2018a). These data suggest that depolymerized FCS with low Mw maintains the lipid-lowering effect of its native FCS. However, the influence of Mw on the hypolipidemic effect of FCS need to be further investigated by comparative studies.
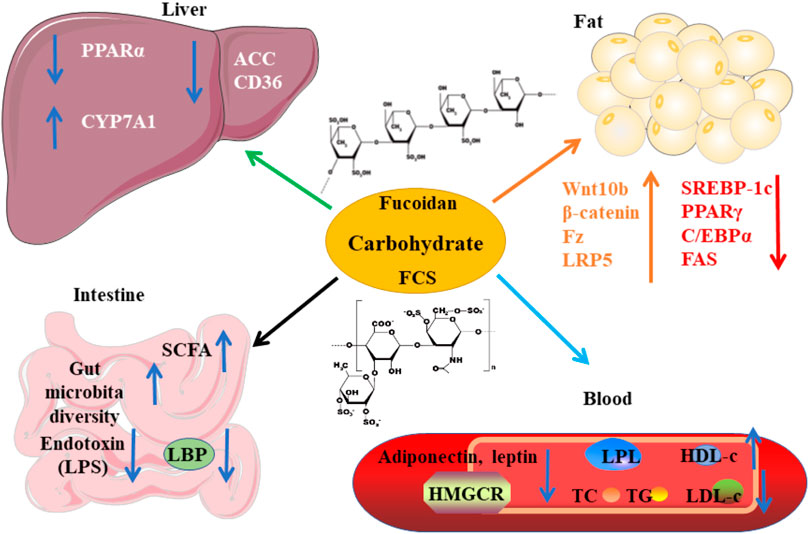
FIGURE 6. The mechanisms of action of carbohydrates derived from sea cucumbers. These polysaccharides include fucoidan and fucosylated chondroitin sulfate (FCS). HDL-c, high-density lipoprotein cholesterol; LBP, lipopolysaccharide binding protein; LDL-c, low-density lipoprotein cholesterol; LPS, lipopolysaccharide; TC, total cholesterol; TG, triglyceride. All the abbreviations are applicable for the rest Figures.
Most of the fucoidans extracted from sea cucumbers have →3)Fuc (1→ linked linear structure as shown in Figure 8. These fucoidans exhibit powerful lipid-modulatory effects. Fucoidan obtained from I. badionotus ameliorates serum levels of TC and TG in C57BL/6J mice fed with a high-fat high-sucrose diet at the dosage of 80 mg/kg/d (Wang et al., 2016). Of note, the fucoidan isolated from P. graeffei shows a better hypolipidemic effect than that from I. badionotus by increasing the expression of CYP7A1 in SD rats fed with a high-fat diet at the dosage of 40 mg/kg. The structure-activity relationship analysis suggests that 4-O-sulfation of the fucoidan obtained from P. graeffei benefits its lipid-lowering effect (Chen et al., 2012; Hu et al., 2015; Li et al., 2017). Furthermore, this fucoidan obtained from P. graeffei mainly act in the colon by increasing the abundance of Bacteroidetes and Actinobacteria and reducing the abundance of Firmicutes and Proteobacteria (Li et al., 2018b). Similar to FCS, fucoidan from the sea cucumber A. molpadioides inhibits adipocyte proliferation and differentiation as evaluated in 3T3-L1 cells and mice at the dose of 200 μg/ml and 80 mg/kg/d, respectively, via enhancing the expression of Wnt/β-Catenin signaling molecules, such as Wnt10b, β-Catenin, Fz, and LRP5, which suppress the expression of transcriptional factors including SREBP-1c, PPARγ, and C/EBPα, and their downstream genes such as FAS and glycerol-3-phosphate acyl-transferase (Yu et al., 2014a; Xu et al., 2014).
A recent study indicates that fucoidans extracted from T. ananas with low Mw and a random coil conformation have better effect than those with large Mw on attenuating hyperlipidemia and fat accumulation (Yu et al., 2014b; Xu et al., 2018; Zhu et al., 2021). Another study also demonstrates that the sulfated polysaccharides containing FCS and fucoidan that are isolated from sea cucumber Stichopus japonicus as well as its depolymerized derivatives decrease serum levels of TC and TG and fat accumulation in BALB/c mice fed with a high-fat diet by modulating gut microbiota at the dosage of 300 mg/kg/d. In addition to increasing the ratio of Bacteroidetes/Firmicutes, these polysaccharides enhance the abundance of the genus Akkermansia in the phylum Verrucomicrobia, which is associated with weight reduction. Furthermore, these polysaccharides increase the SCFAs-producing microbiota including Bacteroides and Alloprevotella as well as the content of SCFAs, which benefit glucose tolerance and insulin resistance. Of note, the depolymerized derivatives with similar structural characteristics as its native polysaccharides show better effect on preventing fat accumulation. These derivatives improve the enrichment of health-beneficial bacteria including Akkermansia muciniphila and Parabacteroides goldsteinii, suggesting that fucoidans with low Mw are superior to the growth of beneficial microbiota (Zhu et al., 2018a). These polysaccharides extracted from sea cucumber S. japonicus and its depolymerized derivatives also exhibit similar effects in mice fed with a normal chow diet (Zhu et al., 2018b). Presently, the hypolipidemic bioactivity of the →3)Fuc (1→ linked linear fucoidan isolated from Ludwigothurea grisea and Holothuria tubulosa as well as the →3,4)Fuc (1→ branched fucoidan extracted from sea cucumber Apostichopus japonicus has been reported (Figure 8) (Mulloy et al., 1994; Yu et al., 2015; Chang et al., 2016). The mechanisms of action of fucoidans are shown in Figure 6.
Glycosaminoglycans (GAGs) extracted from sea cucumber Metriatyla scabra also reduce serum levels of TC and LDL-c as well as atherosclerosis index and enhance HDL-c level via inhibiting HMGCR and improving the activity of LPL. These GAGs show dose-dependent effects when the dosages are lower than 20 mg/kg (Liu et al., 2002). The polysaccharides isolated from sea cucumber Holothuria leucospilota (HLP) are rich in sulfated GAGs. These polysaccharides with an oral dosage of 300 mg/kg/d reduce plasma levels of TC, TG, and LDL-c, and enhance the levels of HDL-c and SCFAs in male BALB/c mice. Mechanistically, HLP improves the expression of PPARα and CD36 as well as the abundance of gut microbiota. Accumulating studies have demonstrated that the reduced ratio of Bacteroidetes/Firmicutes promotes the development of obesity in different models (Zhu et al., 2018c). At the phylum level, HLP elevates the amount of Bacteroidetes, TM7, Cyanobacteria and Tenericutes and reduces the abundance of Firmicutes, Proteobacteria, Spirochaetes, and Actinobacteria. Of note, HLP intervention increases the abundance of SCFAs-producing bacterial genera including Clostridium, Turicibacter, Allobaculum, and Ruminococcus (Zhao et al., 2020). In line with these findings, gastrointestinal digestion reduces the Mw and changes the microstructure of polysaccharides that are extracted from H. leucospilota in vitro (Yuan et al., 2019a). Furthermore, these polysaccharides (200 mg/kg BW) are found to ameliorate hyperlipidemia in rats via reducing the expression of ACC and CD36 and potentially by enhancing the production of SCFAs (Yuan et al., 2019b). Although the structural characteristics of GAGs isolated from A. japonicus and H. tubulosa have been elucidated, their hypolipidemic effects have not been investigated (Yang et al., 2015; Ustyuzhanina et al., 2018; Chen et al., 2019). The mechanisms of action of carbohydrates derived from sea cucumbers are summarized in Figure 6.
Protein and peptide
A recent literature demonstrates that the sea cucumber ovum powder, which mainly composed of protein (62.08%) as well as other components such as polyunsaturated FAs (6.74%) can significantly reduce plasma TG and hepatic TG and TC, thereby improving NAFLD in rats fed with a high-fat diet at the dosage of 450 mg/kg (Han et al., 2020). Mechanistically, this powder modulates the relative expression of 767 proteins as revealed by LC-MS/MS. Some of these differentially expressed proteins are associated with FA oxidation and lipogenesis (Han et al., 2020). However, the contribution of other lipid-lowering components contained in this sea cucumber powder cannot be ruled out. Therefore, the actual hypolipidemic effects of sea cucumber proteins need to be further investigated in future.
Diet supplementation of collagen peptides (2.4 g/kg) isolated from C. frondosa reduces serum TG in rats (Hu X. Q. et al., 2012). The peptides obtained from sea cucumber S. japonicus enhance gluconeogenesis and maintain lipid homeostasis via increasing the mRNA expression of AMPK, PPARγ coactivator 1-α and its downstream genes PPARα and PPARβ in a dose-dependent manner in the liver, skeletal muscle, and heart. Furthermore, these peptides enhance the expression of LPL and CPT1, which are key rate-limiting enzyme genes for lipoprotein hydrolysis and FA β-oxidation, respectively (Yu et al., 2020). The peptides isolated from sea cucumber Stichopus japonicas can increase the content of unsaturated lipids in mouse and rat hippocampus (Lu et al., 2022a). As unsaturated lipids can modulate lipid metabolism (Djuricic and Calder, 2021; Mitrovic et al., 2022), the above results suggest that peptides alleviate hyperlipidemia by regulating the metabolism of unsaturated lipids. The potential mechanisms of action of peptides and proteins of sea cucumbers are summarized in Figure 9.
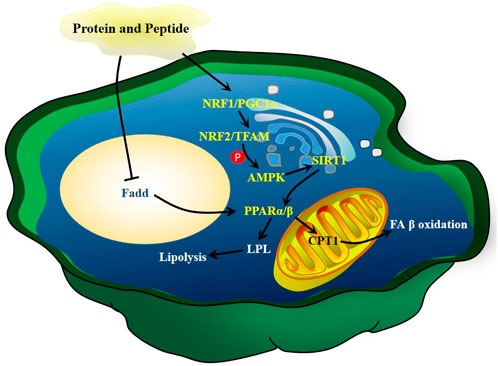
FIGURE 9. The mechanisms of action of peptides and proteins of sea cucumbers. Fadd, FAS associated death domain protein; NRF, nuclear respiratory factor; TFAM, mitochondrial transcription factor A.
The sea cucumber-devived coumpounds for treatment of cardiac complications
In addition to lipid-lowering, sea cucumber-derived compounds have other attractive bioactivities for treatment of cardiac complications. Studies on system pharmacology predict that sea cucumber-derived compounds have potential application for treatment of cardiac complications (Guo et al., 2015). Theses acitivites inculude anti-hypertensive, anti-angiogenic, anti-inflammatory, anti-diabetic, anti-coagulation, and antioxidation activities. As these activities have been reviewed by different groups, we make a brief review of these aspects in the following (Bordbar 2011; Khotimchenko, 2018; Hossain et al., 2020).
The cerebrosides and long-chain bases from A. molpadioides can reduce serum glucose level in addition to improving the lipid profiles in high fat diet-fed mice (Liu et al., 2015). A study demonstrates that sea cucumber-derived ceramides and glucosylceramides attenuate insulin resistance in high-fructose-diet-induced rats via upregulation of the insulin receptor substrate-phosphatidylinositol 3 kinase-serine/threonine kinase signalling pathway (Yang et al., 2021). As reviewed recently, the anti-diabetic effects of sea cucumber-derived peptides and carbohydrates are related to their modulation of angiogenesis (Khosravifar et al., 2022).
The sea cucumber-dereived carbohydrates and other components are able to inhibit clot and thrombus formation as reviewed previously (Pomin, 2014; Dashi and Naiwal, 2021). The mechanisms of action of these components have also been documented (Dashi and Naiwal, 2021). For instance, a clinical study has demonstrated that the Kang-Shuan Capsule, which consisted of Holothuria ldeucospilota acid mucopolysaccharide, improves lipid profiles, reduces blood viscosity, and exhibits good anticoagulant effect in patients with ischemic heart disease (Wang et al., 1997).
CVD is closely associated with nuclear factor kappa B (NF-κB)-mediate inflammatory response (Zhang Q. et al., 2022). Many sea cucumber-derived bioactive compounds including Ds-echinoside A, Frondoside A, Holothurin A1, and Psolusodie are found to inhibit NF-κB. As reviewed recently, sea cucumber compounds can target the NF-κB signalling pathway, which is involved in inflammation, immunity, cellular differentiation, cell adhesion, and survival (Wargasetia 2022). The extracts of Holothuria forskali and Parastichopus tremulus are reported to inhibit inflammation in endothelial cells (Mena-Bueno et al., 2016). In a triple-blinded randomized controlled trial, the Stichopus horrens extract toothpaste can alleviate plaque-induced gingivitis, suggesting the anti-inflammatory effect of the sea cucumber compounds in human (Bakar et al., 2020). The oligopeptides obtained from A. japonicus and A. leucoprocata may supress inflammatory response via inhibiting Toll-like receptor 4/myeloid differentiation factor 88/NF-κB signalling pathway (Wan et al., 2020). The fucoidan from A. japonicus reduces lipopolysaccharide-induced inflammation via inhibiting the phosphorylation of p38 mitogen-activated protein kinase/extracellular regulated protein kinase1/2 and the downstream NF-κB in mice (Yin et al., 2019). The anti-inflammatory effects of the carbohydrates obtained from sea cucumbers have been documented by various groups of researchers (Kapoor et al., 2019; Xu et al., 2022).
The anti-oxidant compounds, such as peptides, from sea cucumbers may also benefit CVD because the initiation and development of CVD are tightly associated with oxidative stress (Lu et al., 2022b; Qiao et al., 2022). The Reinhartdt and Sea Cucumber Capsule improves agitation in moderate to severe Alzheimer disease partly due to its effect on attenuating oxidative stress (Yu et al., 2017). The aqueous extract of sea cucumber Holothuria atra exhibits anti-oxidation activity in vitro and in doxorubicin-induced rats (Ibrahim et al., 2017). The saponins from Holothuria lessoni contribute to the anti-oxidant activity of this sea cucumber extracts (Bahrami et al., 2018). The health-beneficial effects including antioxidant, anti-diabetes, and immunomodulatory activities of sea cucumber peptides have been recently reviewed (Lu et al., 2022a).
Additionally, sea cucumber is an important constituent of food trerapy for treatment of hypertention in Traditional Chinese Medicine (Zou, 2016). The peptides obtained from Acaudina molpadioidea and Actinopyga lecanora show angiotensin I-converting enzyme inhibitor effect and exhibits antihypertensive activity in rats at 3 μM/kg and 800 mg/kg, respectively (Zhao et al., 2009; Vishkaei et al., 2016; Festa et al., 2020).
Conclusion and future perspetive
The components of sea cucumbers exhibit powerful lipid-lowering activity and have great potential applications for intervention of CVD and NALFD via modulating multiple signaling pathways involved in lipid homeostasis as well as gut microbiota. Saponins are one of the most important hypolipidemic components of sea cucumbers. Saponins of sea cucumbers enhance the LXR, SREBP-1c, and PPARα signaling pathways. Among saponins isolated from sea cucumbers, only the lipid-lowering effects and mechanisms of action of echinoside A have been well-documented. Other bioactive compounds derived from these saponins need to be evaluated in the future to understand their potential structure-activity relationship. FAs and phospholipids are well-documented lipid-lowering components of sea cucumbers. EPA, PC, and PE exhibit powerful hypolipidemic effects, and the combination of EPA-PC or EPA-PE shows attractive applications for treatment of NALFD. Long-chain bases are the main active structural units of cerebrosides for intervention of hyperlipidemia. These long-chain bases modulate multiple signaling pathways involved in lipid homeostasis as well as gut microbiota. However, the studies of the lipid-lowering activity of these lipids are mostly carried out in NAFLD models. The activity of these compounds needs to be investigated in CVD models in the future. Carbohydrates derived from sea cucumbers also have a potential application for treatment of hyperlipidemia. Structure-activity relationship analysis demonstrates that 4-O-sulfation of the glycosyls as well as low Mw polysaccharides benefit their hypolipidemic activities. However, comparative studies need to be performed to investigate the relationship between Mw and the lipid-lowering effect of these carbohydrates.
Author contributions
PL, NS, and FY performed reference collection, drew the figures, and wrote the manuscript; S-DG re-edited the manuscript.
Funding
This research was funded by the National Natural Science Foundation of China (82070469 and 81770463).
Conflict of interest
The authors declare that the research was conducted in the absence of any commercial or financial relationships that could be construed as a potential conflict of interest.
Publisher’s note
All claims expressed in this article are solely those of the authors and do not necessarily represent those of their affiliated organizations, or those of the publisher, the editors and the reviewers. Any product that may be evaluated in this article, or claim that may be made by its manufacturer, is not guaranteed or endorsed by the publisher.
References
Abumrad, N. A., Piomelli, D., Yurko-Mauro, K., Merrill, A., Clandinin, M. T., and Serhan, C. N. (2012). Moving beyond “good fat, bad fat”: The complex roles of dietary lipids in cellular function and health: Session abstracts. Adv. Nutr. 3, 60–68. doi:10.3945/an.111.000802
Aron-Wisnewsky, J., Warmbrunn, M. V., Nieuwdorp, M., and Clément, K. (2021). Metabolism and metabolic disorders and the microbiome: The intestinal microbiota associated with obesity, lipid metabolism, and metabolic health-pathophysiology and therapeutic strategies. Gastroenterology 160, 573–599. doi:10.1053/j.gastro.2020.10.057
Aslam, M., and Ladilov, Y. (2022). Emerging role of cAMP/AMPK signaling. Cells 11, 308. doi:10.3390/cells11020308
Atar, D., Jukema, J. W., Molemans, B., Taub, P. R., Goto, S., Mach, F., et al. (2021). New cardiovascular prevention guidelines: How to optimally manage dyslipidaemia and cardiovascular risk in 2021 in patients needing secondary prevention? Atherosclerosis 319, 51–61. doi:10.1016/j.atherosclerosis.2020.12.013
Bahrami, Y., Zhang, W., and M M Franco, C. (2018). Distribution of saponins in the sea cucumber Holothuria lessoni; the body wall versus the viscera, and their biological activities. Mar. Drugs 16, 423. doi:10.3390/md16110423
Bakar, N. A., Jayah, N. I., Mohamed, N. R., Ali, S. M., Nasir, S. H., Hashim, R., et al. (2020). The effectiveness of pure Stichopus horrens extract toothpaste in reducing plaque-induced gingivitis following orthodontic bonding: A triple-blinded randomized controlled trial. J. World Fed. Orthod. 9, 3–8. doi:10.1016/j.ejwf.2019.11.004
Beshir, S. A., Hussain, N., Elnor, A. A., and Said, A. S. A. (2021). Umbrella review on non-statin lipid-lowering therapy. J. Cardiovasc. Pharmacol. Ther. 26, 437–452. doi:10.1177/10742484211002943
Bordbar, S., Anwar, F., and Saari, N. (2011). High-value components and bioactives from sea cucumbers for functional foods--a review. Mar. Drugs 9, 1761–1805. doi:10.3390/md9101761
Careaga, V. P., Muniain, C., and Maier, M. S. (2013). Fatty acid composition of the edible sea cucumber Athyonidium chilensis. Nat. Prod. Res. 27, 638–646. doi:10.1080/14786419.2012.686909
Chang, Y., Hu, Y., Yu, L., McClements, D. J., Xu, X., Liu, G., et al. (2016). Primary structure and chain conformation of fucoidan extracted from sea cucumber Holothuria tubulosa. Carbohydr. Polym. 136, 1091–1097. doi:10.1016/j.carbpol.2015.10.016
Chen, S., Hu, Y., Ye, X., Li, G., Yu, G., Xue, C., et al. (2012). Sequence determination and anticoagulant and antithrombotic activities of a novel sulfated fucan isolated from the sea cucumber Isostichopus badionotus. Biochim. Biophys. Acta 1820, 989–1000. doi:10.1016/j.bbagen.2012.03.002
Chen, Y., Wang, Y., Yang, S., Yu, M., Jiang, T., and Lv, Z. (2019). Glycosaminoglycan from Apostichopus japonicus improves glucose metabolism in the liver of insulin resistant mice. Mar. Drugs 18, 1. doi:10.3390/md18010001
Christodoulides, C., Lagathu, C., Sethi, J. K., and Vidal-Puig, A. (2009). Adipogenesis and WNT signalling. Trends Endocrinol. Metab. 20, 16–24. doi:10.1016/j.tem.2008.09.002
Dashi, G., and Naiwal, N. (2021). A review on molecular mechanisms and patents of marine-derived anti-thrombotic agents. Curr. Drug Targets 22 (3), 318–335. doi:10.2174/1389450121666201020151927
De Backer, G., Jankowski, P., Kotseva, K., Mirrakhimov, E., Reiner, Ž., Rydén, L., et al. (2019). Management of dyslipidaemia in patients with coronary heart disease: Results from the ESC-EORP EUROASPIRE V survey in 27 countries. Atherosclerosis 285, 135–146. doi:10.1016/j.atherosclerosis.2019.03.014
De Vos, W. M., Tilg, H., Van Hul, M., and Cani, P. D. (2022). Gut microbiome and health: Mechanistic insights. Gut 71, 1020–1032. doi:10.1136/gutjnl-2021-326789
Djuricic, I., and Calder, P. C. (2021). Beneficial outcomes of omega-6 and omega-3 polyunsaturated fatty acids on human health: An update for 2021. Nutrients 13, 2421. doi:10.3390/nu13072421
Du, L., Yang, Y. H., Xu, J., Wang, Y. M., Xue, C. H., Kurihara, H., et al. (2016). Transport and uptake effects of marine complex lipid liposomes in small intestinal epithelial cell models. Food Funct. 7, 1904–1914. doi:10.1039/c6fo00066e
Duan, J., Ishida, M., Aida, K., Tsuduki, T., Zhang, J., Manabe, Y., et al. (2016). Dietary cerebroside from sea cucumber (Stichopus japonicus): Absorption and effects on skin barrier and cecal short-chain fatty acids. J. Agric. Food Chem. 64, 7014–7021. doi:10.1021/acs.jafc.6b02564
Ferraro, R. A., Leucker, T., Martin, S. S., Banach, M., Jones, S. R., and Toth, P. P. (2022). Contemporary management of dyslipidemia. Drugs 82, 559–576. doi:10.1007/s40265-022-01691-6
Festa, M., Sansone, C., Brunet, C., Crocetta, F., Di Paola, L., Lombardo, M., et al. (2020). Cardiovascular active peptides of marine origin with ACE inhibitory activities: Potential role as anti-hypertensive drugs and in prevention of SARS-CoV-2 infection. Int. J. Mol. Sci. 21, 8364. doi:10.3390/ijms21218364
Fredalina, B. D., Ridzwan, B. H., Abidin, A. A., Kaswandi, M. A., Zaiton, H., Zali, I., et al. (1999). Fatty acid compositions in local sea cucumber, Stichopus chloronotus, for wound healing. Gen. Pharmacol. 33, 337–340. doi:10.1016/s0306-3623(98)00253-5
Guo, L., Gao, Z., Zhang, L., Guo, F., Chen, Y., Li, Y., et al. (2016). Saponin-enriched sea cucumber extracts exhibit an anti-obesity effect through inhibition of pancreatic lipase activity and up-regulation of LXR-β signaling. Pharm. Biol. 54, 1312–1325. doi:10.3109/13880209.2015.1075047
Guo, Y., Ding, Y., Xu, F., Liu, B., Kou, Z., Xiao, W., et al. (2015). Systems pharmacology-based drug discovery for marine resources: An example using Sea cucumber (holothurians). J. Ethnopharmacol. 165, 61–72. doi:10.1016/j.jep.2015.02.029
Guo, Y., Han, X., Che, H., Li, Z., Dong, P., Xue, C., et al. (2018). Synergistic effect of eicosapentaenoic acid-enriched phospholipids and sea cucumber saponin on orotic acid-induced non-alcoholic fatty liver disease in rats. R. Soc. Open Sci. 5, 172182. doi:10.1098/rsos.172182
Han, B., Jian, Y., Xia, X., Hu, W., Zhang, L., and Zhou, P. (2020). Studying the effects of sea cucumber ovum powder on nonalcoholic fatty liver disease by proteomics techniques in a rat model. Food Funct. 11, 6139–6147. doi:10.1039/d0fo00741b
Han, X. Q., Zhang, L. Y., Ding, L., Shi, H. H., Xue, C. H., Zhang, T. T., et al. (2019). Synergistic effect of sea cucumber saponins and EPA-enriched phospholipids on insulin resistance in high-fat diet-induced obese mice. Food Funct. 10, 3955–3964. doi:10.1039/c9fo01147a
Heeren, J., and Scheja, L. (2021). Metabolic-associated fatty liver disease and lipoprotein metabolism. Mol. Metab. 50, 101238. doi:10.1016/j.molmet.2021.101238
Hossain, A., Dave, D., and Shahidi, F. (2020). Northern Sea cucumber (Cucumaria frondosa): A potential candidate for functional food, nutraceutical, and pharmaceutical sector. Mar. Drugs 18, 274. doi:10.3390/md18050274
Hu, S., Xu, Y., Gao, X., Li, S., Jiang, W., Liu, Y., et al. (2019). Long-chain bases from sea cucumber alleviate obesity by modulating gut microbiota. Mar. Drugs 17, 455. doi:10.3390/md17080455
Hu, X., Li, Z., Xue, Y., Xu, J., Xue, C., Wang, J., et al. (2012). Dietary saponins of sea cucumber ameliorate obesity, hepatic steatosis, and glucoseintolerance in high-fat diet-fed mice. J. Med. Food 15, 909–916. doi:10.1089/jmf.2011.2042
Hu, X. Q., Wang, Y. M., Wang, J. F., Xue, Y., Li, Z. J., Nagao, K., et al. (2010). Dietary saponins of sea cucumber alleviate orotic acid-induced fatty liver in rats via PPARalpha and SREBP-1c signaling. Lipids Health Dis. 9, 25. doi:10.1186/1476-511X-9-25
Hu, X. Q., Xu, J., Xue, Y., Li, Z. J., Wang, J. F., Wang, J. H., et al. (2012). Effects of bioactive components of sea cucumber on the serum, liver lipid profile and lipid absorption. Biosci. Biotechnol. Biochem. 76, 2214–2218. doi:10.1271/bbb.120495
Hu, Y., Li, S., Li, J., Ye, X., Ding, T., Liu, D., et al. (2015). Identification of a highly sulfated fucoidan from sea cucumber pearsonothuria graeffei with well-repeated tetrasaccharides units. Carbohydr. Polym. 134, 808–816. doi:10.1016/j.carbpol.2015.06.088
Ibrahim, D. M., Radwan, R. R., and Abdel Fattah, S. M. (2017). Antioxidant and antiapoptotic effects of sea cucumber and valsartan against doxorubicin-induced cardiotoxicity in rats: The role of low dose gamma irradiation. J. Photochem. Photobiol. B 170, 70–78. doi:10.1016/j.jphotobiol.2017.03.022
Kapoor, S., Nailwal, N., Kumar, M., and Barve, K. (2019). Recent patents and discovery of anti-inflammatory agents from marine source. Recent Pat. Inflamm. Allergy Drug Discov. 13 (2), 105–114. doi:10.2174/1872213X13666190426164717
Khosravifar, M., Sajadimajd, S., and Bahramic, G. (2022). Anti-diabetic effects of macronutrients via modulation of angiogenesis: A comprehensive review on carbohydrates and proteins. Curr. Mol. Med. 22. doi:10.2174/1566524022666220321125548
Khotimchenko, Y. (2018). Pharmacological potential of sea cucumbers. Int. J. Mol. Sci. 19, 1342. doi:10.3390/ijms19051342
Kisa, F., Yamada, K., Kaneko, M., Inagaki, M., and Higuchi, R. (2005). Constituents of Holothuroidea, 14. Isolation and structure of new glucocerebroside molecular species from the sea cucumber Stichopus japonicus. Chem. Pharm. Bull. (Tokyo) 53, 382–386. doi:10.1248/cpb.53.382
Lee, H., Bae, S., Kim, K., Kim, W., Chung, S. I., and Yoon, Y. (2010). Beta-Catenin mediates the anti-adipogenic effect of baicalin. Biochem. Biophys. Res. Commun. 398, 741–746. doi:10.1016/j.bbrc.2010.07.015
Li, S., Li, J., Mao, G., Hu, Y., Ye, X., Tian, D., et al. (2018a). Fucosylated chondroitin sulfate oligosaccharides from Isostichopus badionotus regulates lipid disorder in C57BL/6 mice fed a high-fat diet. Carbohydr. Polym. 201, 634–642. doi:10.1016/j.carbpol.2018.08.020
Li, S., Li, J., Mao, G., Wu, T., Hu, Y., Ye, X., et al. (2018b). A fucoidan from sea cucumber Pearsonothuria graeffei with well-repeated structure alleviates gut microbiota dysbiosis and metabolic syndromes in HFD-fed mice. Food Funct. 9, 5371–5380. doi:10.1039/c8fo01174e
Li, S., Li, J., Zhi, Z., Hu, Y., Ge, J., Ye, X., et al. (2017). 4-O-Sulfation in sea cucumber fucodians contribute to reversing dyslipidiaemia caused by HFD. Int. J. Biol. Macromol. 99, 96–104. doi:10.1016/j.ijbiomac.2017.01.145
Li, X., Zhang, Y., Wang, S., Shi, C., Wang, S., Wang, X., et al. (2022). A review on the potential use of natural products in overweight and obesity. Phytother. Res. 36, 1990–2015. doi:10.1002/ptr.7426
Liu, H. H., Ko, W. C., and Hu, M. L. (2002). Hypolipidemic effect of glycosaminoglycans from the sea cucumber Metriatyla scabra in rats fed a cholesterol-supplemented diet. J. Agric. Food Chem. 50, 3602–3606. doi:10.1021/jf020070k
Liu, X., Sun, Z., Zhang, M., Meng, X., Xia, X., Yuan, W., et al. (2012). Anti-oxidant and anti-hyperlipidemic activities of polysaccharides from sea cucumber Apostichopus japonicus. Carbohydr. Polym. 90, 1664–1670. doi:10.1016/j.carbpol.2012.07.047
Liu, X., Xu, J., Xue, Y., Gao, Z., Li, Z., Leng, K., et al. (2015). Sea cucumber cerebrosides and long-chain bases from Acaudina molpadioides protect against high fat diet-induced metabolic disorders in mice. Food Funct. 6, 3428–3436. doi:10.1039/c5fo00602c
Liu, Y., Shi, D., Tian, Y., Liu, Y., Zhan, Q., Xu, J., et al. (2017). Eicosapentaenoic acid-enriched phosphatidylcholine attenuated hepatic steatosis through regulation of cholesterol metabolism in rats with nonalcoholic fatty liver disease. Lipids 52, 119–127. doi:10.1007/s11745-016-4222-1
Lu, Z., Sun, N., Dong, L., Gao, Y., and Lin, S. (2022a). Production of bioactive peptides from sea cucumber and its potential health benefits: A comprehensive review. J. Agric. Food Chem. 70 (25), 7607–7625. doi:10.1021/acs.jafc.2c02696
Lu, Z., Xu, X., Li, D., Sun, N., and Lin, S. (2022b). Sea cucumber peptides attenuated the scopolamine-induced memory impairment in mice and rats and the underlying mechanism. J. Agric. Food Chem. 70, 157–170. doi:10.1021/acs.jafc.1c06475
Mecheta, A., Hanachi, A., Jeandel, C., Arab-Tehrany, E., Bianchi, A., Velot, E., et al. (2020). Physicochemical properties and liposomal formulations of hydrolysate fractions of four sea cucumbers (Holothuroidea: Echinodermata) from the northwestern Algerian coast. Molecules 25, 2972. doi:10.3390/molecules25132972
Mena-Bueno, S., Atanasova, M., Fernández-Trasancos, Á., Paradela-Dobarro, B., Bravo, S. B., Álvarez, E., et al. (2016). Sea cucumbers with an anti-inflammatory effect on endothelial cells and subcutaneous but not on epicardial adipose tissue. Food Funct. 7, 953–963. doi:10.1039/c5fo01246e
Meng, J., Hu, X., Zhang, T., Dong, P., Li, Z., Xue, C., et al. (2018). Saponin from sea cucumber exhibited more significant effects than ginsenoside on ameliorating high fat diet-induced obesity in C57BL/6 mice. Medchemcomm 9, 725–734. doi:10.1039/c7md00653e
Mitrovic, M., Sistilli, G., Horakova, O., and Rossmeisl, M. (2022). Omega-3 phospholipids and obesity-associated NAFLD: Potential mechanisms and therapeutic perspectives. Eur. J. Clin. Invest. 52, e13650. doi:10.1111/eci.13650
Mulloy, B., Ribeiro, A. C., Alves, A. P., Vieira, R. P., and Mourão, P. A. (1994). Sulfated fucans from echinoderms have a regular tetrasaccharide repeating unit defined by specific patterns of sulfation at the 0-2 and 0-4 positions. J. Biol. Chem. 269, 22113–22123. doi:10.1016/s0021-9258(17)31763-5
Olivera-Castillo, L., Davalos, A., Grant, G., Valadez-Gonzalez, N., Montero, J., Barrera-Perez, H. A. M., et al. (2013). Diets containing sea cucumber (Isostichopus badionotus) meals are hypocholesterolemic in young rats. PLoS One 8, e79446. doi:10.1371/journal.pone.0079446
Pirillo, A., Casula, M., Olmastroni, E., Norata, G. D., and Catapano, A. L. (2021). Global epidemiology of dyslipidaemias. Nat. Rev. Cardiol. 18, 689–700. doi:10.1038/s41569-021-00541-4
Pomin, V. H. (2014). Sea, carbohydrates and clotting: A triad on the road of drug discovery. Mini Rev. Med. Chem. 14 (9), 717–724. doi:10.2174/1389557514666140820121048
Powell, E. E., Wong, V. W., and Rinella, M. (2021). Non-alcoholic fatty liver disease. Lancet 397, 2212–2224. doi:10.1016/S0140-6736(20)32511-3
Qiao, Y. N., Zou, Y. L., and Guo, S. D. (2022). Low-density lipoprotein particles in atherosclerosis. Front. Physiol. 13, 931931. doi:10.3389/fphys.2022.931931
Roggatz, C. C., González-Wangüemert, M., Pereira, H., Rodrigues, M. J., da Silva, M. M., Barreira, L., et al. (2016). First report of the nutritional profile and antioxidant potential of Holothuria arguinensis, a new resource for aquaculture in Europe. Nat. Prod. Res. 30, 2034–2040. doi:10.1080/14786419.2015.1107555
Roggatz, C. C., González-Wangüemert, M., Pereira, H., Vizetto-Duarte, C., Rodrigues, M. J., Barreira, L., et al. (2018). A first glance into the nutritional properties of the sea cucumber Parastichopus regalis from the Mediterranean Sea (SE Spain). Nat. Prod. Res. 32, 116–120. doi:10.1080/14786419.2017.1331224
Savarino, P., Demeyer, M., Decroo, C., Colson, E., and Gerbaux, P. (2021). Mass spectrometry analysis of saponins. Mass Spectrom. Rev. doi:10.1002/mas.21728
Singh, S. P., and Sashidhara, K. V. (2017). Lipid lowering agents of natural origin: An account of some promising chemotypes. Eur. J. Med. Chem. 140, 331–348. doi:10.1016/j.ejmech.2017.09.020
Tian, Y., Hu, S., Xu, H., Wang, J., Xue, C., and Wang, Y. (2016). Long-chain bases from Cucumaria frondosa inhibit adipogenesis and regulate lipid metabolism in 3T3-L1 adipocytes. Food Sci. Biotechnol. 25, 1753–1760. doi:10.1007/s10068-016-0267-4
Tian, Y., Liu, Y., Xue, C., Wang, J., Wang, Y., Xu, J., et al. (2020). Exogenous natural EPA-enriched phosphatidylcholine and phosphatidylethanolamine ameliorate lipid accumulation and insulin resistance via activation of PPARα/γ in mice. Food Funct. 11, 8248–8258. doi:10.1039/d0fo01219j
Ustyuzhanina, N. E., Bilan, M. I., Dmitrenok, A. S., Nifantiev, N. E., and Usov, A. I. (2018). Fucosylated chondroitin sulfates from the sea cucumbers Holothuria tubulosa and Holothuria stellati. Carbohydr. Polym. 200, 1–5. doi:10.1016/j.carbpol.2018.07.035
Vishkaei, M. S., Ebrahimpour, A., Abdul-Hamid, A., Ismail, A., and Saari, N. (2016). Angiotensin-I converting enzyme (ACE) inhibitory and anti-hypertensive effect of protein hydrolysate from Actinopyga lecanora (sea cucumber) in rats. Mar. Drugs 14 (10), 176. doi:10.3390/md14100176
Visseren, F. L. J., Mach, F., Smulders, Y. M., Carballo, D., Koskinas, K. C., Bäck, M., et al. (2021). 2021 ESC guidelines on cardiovascular disease prevention in clinical practice. Eur. Heart J. 42, 3227–3337. doi:10.1093/eurheartj/ehab484
Wan, H., Han, J., Tang, S., Bao, W., Lu, C., Zhou, J., et al. (2020). Comparisons of protective effects between two sea cucumber hydrolysates against diet induced hyperuricemia and renal inflammation in mice. Food Funct. 11, 1074–1086. doi:10.1039/c9fo02425e
Wang, J., Hu, S., Jiang, W., Song, W., Cai, L., and Wang, J. (2016). Fucoidan from sea cucumber may improve hepatic inflammatory response and insulin resistance in mice. Int. Immunopharmacol. 31, 15–23. doi:10.1016/j.intimp.2015.12.009
Wang, X., Cong, P., Chen, Q., Li, Z., Xu, J., and Xue, C. (2020). Characterizing the phospholipid composition of six edible sea cucumbers by NPLC-Triple TOF-MS/MS. J. Food Compost. Anal. 94, 103626. doi:10.1016/j.jfca.2020.103626
Wang, X., Wang, H., Hu, D., Guo, X., Jin, C., Lu, N., et al. (1997). Clinical study on the antithrombotic effects of Holothuria Ldeucospilota acidic mucopolysaccharide. Chin. J. Hematol. 18, 457–459.
Wang, X., Wang, Y., Liu, Y., Cong, P., Xu, J., and Xue, C. (2022). Hepatoprotective effects of sea cucumber ether-phospholipids against alcohol-induced lipid metabolic dysregulation and oxidative stress in mice. Food Funct. 13, 2791–2804. doi:10.1039/d1fo03833h
Wargasetia, T. L., Ratnawati, H., and Widodo, N. (2022). sea cucumber compounds targeting NF-κB in cancer treatment. Bioinform. Biol. Insights 16, 11779322221091740. doi:10.1177/11779322221091740
Wen, M., Cui, J., Xu, J., Xue, Y., Wang, J., Xue, C., et al. (2014). Effects of dietary sea cucumber saponin on the gene expression rhythm involved in circadian clock and lipid metabolism in mice during nighttime-feeding. J. Physiol. Biochem. 70, 801–808. doi:10.1007/s13105-014-0349-9
Wen, M., Ding, L., Zhang, L., Cong, P., Zhang, T., Xu, J., et al. (2018). A comparative study of eicosapentaenoic acid enriched phosphatidylcholine and ethyl ester in improving cognitive deficiency in Alzheimer's disease model rats. Food Funct. 9, 2184–2192. doi:10.1039/c7fo01815k
Wen, M., Fu, X., Han, X., Hu, X., Dong, P., Xu, J., et al. (2016). Sea cucumber saponin Echinoside A (EA) stimulates hepatic fatty acid β-oxidation and suppresses fatty acid biosynthesis coupling in a diurnal pattern. J. Nutr. Sci. Vitaminol. (Tokyo) 62, 170–177. doi:10.3177/jnsv.62.170
World Health Organization, (2021). Cardiovascular diseases (CVDs). https://www.who.int/news-room/fact-sheets/detail/cardiovascular-diseases-(cvds (accessed sept 20, 2021).
Wu, N., Zhang, Y., Ye, X., Hu, Y., Ding, T., and Chen, S. (2016). Sulfation pattern of fucose branches affects the anti-hyperlipidemic activities of fucosylated chondroitin sulfate. Carbohydr. Polym. 147, 1–7. doi:10.1016/j.carbpol.2016.03.013
Xu, H., Wang, F., Wang, J., Xu, J., Wang, Y., and Xue, C. (2015b). The WNT/β-catenin pathway is involved in the anti-adipogenic activity of cerebrosides from the sea cucumber Cucumaria frondosa. Food Funct. 6, 2396–2404. doi:10.1039/c5fo00273g
Xu, H., Wang, J., Chang, Y., Xu, J., Wang, Y., Long, T., et al. (2014). Fucoidan from the sea cucumber Acaudina molpadioides exhibits anti-adipogenggggic activity by modulating the Wnt/β-catenin pathway and down-regulating the SREBP-1c expression. Food Funct. 5, 1547–1555. doi:10.1039/c3fo60716j
Xu, H., Wang, J., Zhang, X., Li, Z., Wang, Y., and Xue, C. (2015a). Inhibitory effect of fucosylated chondroitin sulfate from the sea cucumber Acaudina molpadioides on adipogenesis is dependent on Wnt/β-catenin pathway. J. Biosci. Bioeng. 119, 85–91. doi:10.1016/j.jbiosc.2014.05.026
Xu, H., Zhou, Q., Liu, B., Chen, F., and Wang, M. (2022). Holothurian fucosylated chondroitin sulfates and their potential benefits for human health: Structures and biological activities. Carbohydr. Polym. 275, 118691. doi:10.1016/j.carbpol.2021.118691
Xu, J., Guo, S., Du, L., Wang, Y. M., Sugawara, T., Hirata, T., et al. (2013). Isolation of cytotoxic glucoerebrosides and long-chain bases from sea cucumber Cucumaria frondosa using high speed counter-current chromatography. J. Oleo Sci. 62, 133–142. doi:10.5650/jos.62.133
Xu, J., Wang, Y. M., Feng, T. Y., Zhang, B., Sugawara, T., and Xue, C. H. (2011). Isolation and anti-fatty liver activity of a novel cerebroside from the sea cucumber Acaudina molpadioides. Biosci. Biotechnol. Biochem. 75, 1466–1471. doi:10.1271/bbb.110126
Xu, X., Chang, Y., Xue, C., Wang, J., and Shen, J. (2018). Gastric protective activities of sea cucumber fucoidans with different molecular weight and chain conformations: A structure-activity relationship investigation. J. Agric. Food Chem. 66, 8615–8622. doi:10.1021/acs.jafc.8b01497
Yamada, K., Sasaki, K., Harada, Y., Isobe, R., and Higuchi, R. (2002). Constituents of Holothuroidea, 12. Isolation and structure of glucocerebrosides from the sea cucumber Holothuria pervicax. Chem. Pharm. Bull. (Tokyo) 50, 1467–1470. doi:10.1248/cpb.50.1467
Yamada, K., Wada, N., Onaka, H., Matsubara, R., Isobe, R., Inagaki, M., et al. (2005). Constituents of Holothuroidea, 15. Isolation of ante-iso type regio-isomer on long chain base moiety of glucocerebroside from the sea cucumber Holothuria leucospilota. Chem. Pharm. Bull. (Tokyo) 53, 788–791. doi:10.1248/cpb.53.788
Yang, J., Wang, Y., Jiang, T., and Lv, Z. (2015). Novel branch patterns and anticoagulant activity of glycosaminoglycan from sea cucumber Apostichopus japonicus. Int. J. Biol. Macromol. 72, 911–918. doi:10.1016/j.ijbiomac.2014.10.010
Yang, J. Y., Zhang, T. T., Dong, Z., Shi, H. H., Xu, J., Mao, X. Z., et al. (2021). Dietary supplementation with exogenous sea-cucumber-derived ceramides and glucosylceramides alleviates insulin resistance in high-fructose-diet-fed rats by upregulating the IRS/PI3K/Akt signaling pathway. J. Agric. Food Chem. 69, 9178–9187. doi:10.1021/acs.jafc.0c06831
Yin, J., Yang, X., Xia, B., Yang, Z., Wang, Z., Wang, J., et al. (2019). The fucoidan from sea cucumber Apostichopus japonicus attenuates lipopolysaccharide-challenged liver injury in C57BL/6J mice. J. Funct. Foods 61, 103493. doi:10.1016/j.jff.2019.103493
Yu, E., Liao, Z., Tan, Y., Chou, Y., Zhu, J., Chen, Y., et al. (2017). [Efficacy and tolerance of Memantine monotherapy and combination therapy with Reinhartdt and Sea Cucumber Capsule on agitation in moderate to severe Alzheimer disease]. Zhonghua Yi Xue Za Zhi 97, 2091–2094. doi:10.3760/cma.j.issn.0376-2491.2017.27.003
Yu, L., Ge, L., Xue, C., Chang, Y., Zhang, C., Xu, X., et al. (2014a). Structural study of fucoidan from sea cucumber Acaudina molpadioides: A fucoidan containing novel tetrafucose repeating unit. Food Chem. 142, 197–200. doi:10.1016/j.foodchem.2013.06.079
Yu, L., Xue, C., Chang, Y., Hu, Y., Xu, X., Ge, L., et al. (2015). Structure and rheological characteristics of fucoidan from sea cucumber Apostichopus japonicus. Food Chem. 180, 71–76. doi:10.1016/j.foodchem.2015.02.034
Yu, L., Xue, C., Chang, Y., Xu, X., Ge, L., Liu, G., et al. (2014b). Structure elucidation of fucoidan composed of a novel tetrafucose repeating unit from sea cucumber Thelenota ananas. Food Chem. 146, 113–119. doi:10.1016/j.foodchem.2013.09.033
Yu, Y., Wu, G., Jiang, Y., Li, B., Feng, C., Ge, Y., et al. (2020). sea Cucumber peptides improved the mitochondrial capacity of mice: A potential mechanism to enhance gluconeogenesis and fat catabolism during exercise for improved antifatigue property. Oxid. Med. Cell. Longev. 2020, 4604387. doi:10.1155/2020/4604387
Yuan, Y., Li, C., Zheng, Q., Wu, J., Zhu, K., Shen, X., et al. (2019a). Effect of simulated gastrointestinal digestion in vitro on the antioxidant activity, molecular weight and microstructure of polysaccharides from a tropical sea cucumber (Holothuria leucospilota). Food Hydrocoll. 89, 735–741. doi:10.1016/j.foodhyd.2018.11.040
Yuan, Y., Liu, Q., Zhao, F., Cao, J., Shen, X., and Li, C. (2019b). Holothuria Leucospilota polysaccharides ameliorate hyperlipidemia in high-fat diet-induced rats via short-chain fatty acids production and lipid metabolism regulation. Int. J. Mol. Sci. 20, 4738. doi:10.3390/ijms20194738
Zhang, B. H., Yin, F., Qiao, Y. N., and Guo, S. D. (2022a). Triglyceride and triglyceride-rich lipoproteins in atherosclerosis. Front. Mol. Biosci. 9, 909151. doi:10.3389/fmolb.2022.909151
Zhang, B., Xue, C., Hu, X., Xu, J., Li, Z., Wang, J., et al. (2012). Dietary sea cucumber cerebroside alleviates orotic acid-induced excess hepatic adipopexis in rats. Lipids Health Dis. 11, 48. doi:10.1186/1476-511X-11-48
Zhang, L., Ding, L., Shi, H., Wang, C., Xue, C., Zhang, T., et al. (2020). Eicosapentaenoic acid-enriched phospholipids suppressed lipid accumulation by specific inhibition of lipid droplet-associated protein FSP27 in mice. J. Sci. Food Agric. 100, 2244–2251. doi:10.1002/jsfa.10250
Zhang, L., Zhang, T., Ding, L., Xu, J., Xue, C., Yanagita, T., et al. (2018). The protective activities of dietary sea cucumber cerebrosides against atherosclerosis through regulating inflammation and cholesterol metabolism in male mice. Mol. Nutr. Food Res. 62, e1800315. doi:10.1002/mnfr.201800315
Zhang, Q., Wang, L., Wang, S., Cheng, H., Xu, L., Pei, G., et al. (2022b). Signaling pathways and targeted therapy for myocardial infarction. Signal Transduct. Target. Ther. 7 (1), 78. doi:10.1038/s41392-022-00925-z
Zhao, G., Zhao, W., Han, L., Ding, J., and Chang, Y. (2020). Metabolomics analysis of sea cucumber (Apostichopus japonicus) in different geographical origins using UPLC-Q-TOF/MS. Food Chem. 333, 127453. doi:10.1016/j.foodchem.2020.127453
Zhao, Y., Li, B., Dong, S., Liu, Z., Zhao, X., Wang, J., et al. (2009). A novel ACE inhibitory peptide isolated from Acaudina molpadioidea hydrolysate. Peptides 30, 1028–1033. doi:10.1016/j.peptides.2009.03.002
Zhu, Y., Tian, Y., Wang, N., Chang, Y., Xue, C., and Wang, J. (2021). Structure-function relationship analysis of fucoidan from sea cucumber (Holothuria tubulosa) on ameliorating metabolic inflammation. J. Food Biochem. 45, e13500. doi:10.1111/jfbc.13500
Zhu, Z., Zhu, B., Ai, C., Lu, J., Wu, S., Liu, Y., et al. (2018b). Development and application of a HPLC-MS/MS method for quantitation of fucosylated chondroitin sulfate and fucoidan in sea cucumbers. Carbohydr. Res. 466, 11–17. doi:10.1016/j.carres.2018.07.001
Zhu, Z., Zhu, B., Sun, Y., Ai, C., Wang, L., Wen, C., et al. (2018c). Sulfated polysaccharide from sea cucumber and its depolymerized derivative prevent obesity in association with modification of gut microbiota in high-fat diet-fed mice. Mol. Nutr. Food Res. 62, e1800446. doi:10.1002/mnfr.201800446
Zhu, Z., Zhu, B., Sun, Y., Ai, C., Wu, S., Wang, L., et al. (2018a). Sulfated polysaccharide from sea cucumber modulates the gut microbiota and its metabolites in normal mice. Int. J. Biol. Macromol. 120, 502–512. doi:10.1016/j.ijbiomac.2018.08.098
Zou, P. (2016). Traditional Chinese medicine, food therapy, and hypertension control: A narrative review of Chinese literature. Am. J. Chin. Med. 44, 1579–1594. doi:10.1142/S0192415X16500889
Glossary
ABC ATP binding cassette
ACC acetyl CoA carboxylase
ACOX1 acyl-CoA oxidase 1
AMPK adenosine monophosphate activated protein kinase
Apo apolipoprotein
ATGL adipose triglyceride lipase
CD36 cluster of differentiation 36
C/EBPα CCAAT/enhancer binding protein-α
CPT carnitine palmitoyl transferase
CVD cardiovascular disease
CYP7A1 cholesterol 7-α-hydroxylase A1
EPA eicosapentaenoic acid
EPA-PC EPA-enriched phosphatidylcholine
EPA-PE EPA-enriched phosphatidylethanoamine
FA fatty acid
FAS fatty acid synthase
FCS fucosylated chondroitin sulfate
Fz frizzled receptor
GAGs glycosaminoglycans
G6PDH glucose-6-phosphate dehydrogenase
HDL-c high-density lipoprotein cholesterol
HLP polysaccharides isolated from sea cucumber Holothuria leucospilota
HMGCR 3-hydroxy-3-methyl-glutaryl-CoA reductase
HSL, hormone-sensitive lipase;
LC-MS/MS liquid chromatography-tandem mass spectrometry
LDL low-density lipoprotein
LDL-c low-density lipoprotein cholesterol
LPL lipoprotein lipase
LRP lipoprotein receptor-related protein
LXR liver X receptor
Mw molecular weight
NAFLD non-alcoholic fatty liver disease;
PlsCho plasmanyl phosphatidylcholine
PlsEtn plasmenyl phosphatidylethanoamine
PPAR peroxisome proliferator-activated receptor
SCD stearoyl-CoA desaturase
SCFA short-chain fatty acid
SD Sprague-Dawley
SREBP sterol regulatory element-binding protein
TC total cholesterol
TG triglyceride
Keywords: sea cucumber, bioactive component, lipid-lowering, mechanisms of action, NALFD, cardiovascular disease
Citation: Lin P, Shen N, Yin F and Guo S-D (2022) Sea cucumber-derived compounds for treatment of dyslipidemia: A review. Front. Pharmacol. 13:1000315. doi: 10.3389/fphar.2022.1000315
Received: 22 July 2022; Accepted: 29 August 2022;
Published: 14 September 2022.
Edited by:
Dong-Hua Yang, St. John’s University, United StatesReviewed by:
Hongyan Li, Qingdao University of Science and Technology, ChinaHanan Farouk Aly, National Research Centre, Egypt
Copyright © 2022 Lin, Shen, Yin and Guo. This is an open-access article distributed under the terms of the Creative Commons Attribution License (CC BY). The use, distribution or reproduction in other forums is permitted, provided the original author(s) and the copyright owner(s) are credited and that the original publication in this journal is cited, in accordance with accepted academic practice. No use, distribution or reproduction is permitted which does not comply with these terms.
*Correspondence: Shou-Dong Guo, U0QtR1VPQGhvdG1haWwuY29t
†These authors have contributed equally to this work